- 1Veramaris V.O.F., Delft, Netherlands
- 2DSM-Firmenich AG, Kaiseraugst, Switzerland
- 3Manolin AS., Bergen, Norway
The shift from fish oil to vegetable oil (VO) sources has lowered eicosapentaenoic acid (EPA) and docosahexaenoic acid (DHA) levels in salmon aquafeeds. VOs are high in omega-6 and low in omega-3 essential fatty acids like EPA and DHA, crucial for fish metabolism and immunity. Algal oil (AO), with high EPA + DHA and lower omega-6 levels supports fish health and growth, but transferring lab-controlled conditions to real-world commercial farming remains a challenge. This study explored the benefits of high dietary EPA + DHA levels through a commercial farm (CF) study in Chile and a Big Data (BD) study in Norway. The CF study, involving 625,000 Atlantic salmon, compared standard feed with AO-supplemented feed. The latter resulted in improved health responses and better fillet quality. The BD study analyzed real data from 232.6 million fish, revealing that EPA + DHA levels > 8% reduce mortality variability by 21%, improve economic feed conversion ratio by 11%, and increase the likelihood of superior harvests by 27%, demonstrating productivity benefits. Both studies emphasize the advantages of feeds with EPA + DHA levels above typical industry practices, enhancing growth, health, and nutritional quality. Importantly, the BD study complements the CF study, bridging the gap between science and aquafarms, and providing evidence that diets with EPA + DHA > 8% offer biological performance benefits for farmers, regardless of farming environments.
1 Introduction
The production and market value of aquatic foods have been achieving all-time records driven mainly by the increased demand for such products, which are recognized as essential to global food security and nutrition (FAO, 2022). However, to sustain this growth, operators must encompass changes in farming practices, from feed ingredients’ source selection to management and investment policies, without compromising the farm productivity and business sustainability, which ultimately depend upon fish zootechnical and health performance. For instance, increasing the level of vegetable oils (VOs) in aquafeeds for the partial or total replacement of fish oil (FO) has helped to grow aquaculture production without increasing pressure on marine stocks but has negatively impacted the health and wellbeing of fish by leading to unbalanced essential fatty acid (EFA) profiles (Sprague et al., 2016; Roques et al., 2020). This is because VOs have particularly high content of omega-6 EFAs and relatively low content of omega-3 EFAs, such as eicosapentaenoic acid (EPA) and docosahexaenoic acid (DHA), both playing key metabolic and immune roles in fish (Tocher, 2015).
To tackle this issue, few studies have lately focused on better understanding the EPA and DHA requirements of farmed fish species at the different production stages, to which levels should EPA and DHA be increased, and how to supply nutrients using alternative sources such as genetically modified seed oil, algae biomasses, and algal oil (AO). The latter has received particular attention because it contains a combination of EPA, DHA, and arachidonic acid, low levels of omega-6 EFAs, improved food safety, is easy to incorporate in aquafeed formulations, and supports optimal fish health and growth (Santigosa et al., 2023). Inclusion of AO as a source of omega-3 EFAs also helps reducing the marine footprint of the feed, as indicated by a lower forage fish dependency ratio (FFDR). The added value of AO on providing high levels of EPA + DHA, as well as on maintaining the ratio of these omega-3 EFAs (the optimum omega nutrition concept), includes better health and welfare and better fillet quality, which has been extensively documented for Atlantic salmon in scientific lab-scale trials (Santigosa et al., 2018, 2020, 2023). Fillet quality has further repercussions in human health because Atlantic salmon is considered the most valuable source of EPA and DHA for humans (Horn et al., 2019; Carr et al., 2023; Santigosa et al., 2023) and low EPA+DHA levels lead to low fish fillet quality (Oliva-Teles et al., 2015). Indeed, recent studies have shown that the EPA + DHA levels of commercialized Atlantic salmon fillets have been reduced by more than half in recent decades (Sprague et al., 2016, 2020).
However, transferring the science-backed knowledge from the lab to commercial farms has been challenging primarily due to the difficulty in recreating all risks and variables fish are exposed to in the commercial farm environment, although the costs of farm trials and business priorities also play an important role. All these factors hinder farmers’ identification of a solid business case for changing EPA + DHA specifications in the feed they provide to their fish, as well as the business case for the feed industry to alter the sources and/or levels of EPA + DHA, particularly if this represents an extra cost. Moreover, commercial-scale studies are often performed on a single farm and using few cages, and significant differences between cages can arise due to many factors, including disease outbreaks. This was the case for a large commercial-scale study on the effects of reducing long-chain fatty acid levels in Atlantic salmon feed, where a pancreatic disease outbreak arose (Sissener et al., 2016) or the 15-month-long study evaluating the effect of increasing dietary levels of EPA + DHA up to 3.5% on the growth, welfare, and fillet quality of Atlantic salmon in sea cages, where a cardiomyopathy syndrome outbreak arose (Lutfi et al., 2022). Such challenges do not allow to attribute the differences found between group growth and health performances, if any, to the levels and ratios of EPA + DHA provided nor the generalization of the results to other farms and/or fish species. Furthermore, results are challenged by farmers as the trial conditions do not match that of their farms.
Big data (BD) is a relatively new approach that retrieves patterns and trend values from large datasets through advanced software programs (Sadiku et al., 2020). The use of BD in research papers on food science has grown by nearly 300% every five years since 2010 (Tao et al., 2021), and it may also help narrow the lab-to-commercial-scale gap in aquaculture. By aggregating real production data from many farms and extracting information related to feed composition and fish growth performance, health, and nutritional value, BD can find relationships between these variables that may then be used to confirm the effects observed at lab or single-farm scales.
In the present paper, we aim to highlight how providing below or above-average EPA + DHA levels can impact Atlantic salmon growth, health, and nutritional quality. The results presented here showcase two trials, one performed at a commercial scale and another using the BD approach, to ultimately demonstrate how the aquaculture industry can quantify the benefits of providing optimal levels and ratios of EPA + DHA to farmed Atlantic salmon.
2 Methods
To demonstrate the benefits of adequate EPA + DHA levels in farmed Atlantic salmon, the authors took two approaches. The methods used for each are described below.
2.1 Commercial farm study
For the CF study, a trial was conducted by Veramaris® (Delft, The Netherlands) in collaboration with Skretting (Stavanger, Norway) at a commercial salmon farm site in Chile’s Region XII. In this study, 208,000 Atlantic salmon (Control fish; initial body weight, IBW, ~3.0 kg)) were allocated to three cages and fed a Skretting Atlantic salmon feed (crude protein: 38.8 ± 0.14; crude lipid: 36.9 ± 0.28). In addition, 417,000 Atlantic salmon were allocated to six cages and fed a Skretting Atlantic salmon feed supplemented with Veramaris AO [OON fish; IBW, ~3.0 kg; (crude protein: 38.1 ± 0.14; crude lipid: 37.1 ± 0.78)]. Full fatty acid profile of the diets can be found in Supplementary Table 1. Commercial diet formulations cannot be disclosed. All efforts were made to minimize unintended variations between the diets.
Both Control and OON fish were fed for 114 days (June to October). Control and OON diets differed in their EPA+DHA content (6% and 10%, respectively) and EPA: DHA ratio (>1.5:1 and 1:1, respectively), achieved with the inclusion of the AO in the feed formulation.
At the end of the feeding period, biological and economic feed conversion ratios (bFCR and eFCR, respectively) were calculated to evaluate growth performance. Health and welfare responses, as well as the status of the mucosal barriers of gills, skin, and gut were sampled and determined for Control and OON fish sampled in June and October (n = 90 samples), and processed through an automated software developed by Quantidoc AS (https://www.quantidoc.no; n = 1452 relevant points at the time of analysis) for the stereological image analysis of the mucosa (Veribarr™). For the Quantidoc analysis, all samples of OON fish came from the same cage at the end of the trial. Fillets’ nutritional and physical quality were evaluated by calculating the total level of EPA + DHA per 100 grams of fillet (SGS SA, Chile) based on the Official Method 991.39 (AOAC, 2012) and the percentage of fish scoring 24 or above on the SalmoFan™ color measurement scale (dsm-firmenich AG, Kaiseraugst, Switzerland), respectively. The marine footprint was evaluated based on the forage fish dependency ratio for fish oil (FFDRoil), calculated following the ASC Salmon Standard (https://www.asc-aqua.org/).
The experimental unit for all endpoints was the cage. Differences across group means were investigated with a t-stest for pairwise comparisons (JMP 17.0 software; https://www.jmp.com). The accepted significance level was p <0.05. Means in tabulated data are given with standard deviations, SD.
2.2 Big data study
In the BD study, conducted by Veramaris and Manolin AS. (Bergen, Norway), the performance outcomes of utilizing feeds at three different EPA + DHA levels (< 7%, 7%–8%, and > 8%; Table 1) were evaluated based on real production data. The authors focused on mapping the variations in EPA+ DHA levels between categories. Other possible variations in dietary composition have not been taken into consideration and this is a potential limitation of the study. Feed products provided to 232.6 million smolts in 99 active Atlantic salmon farms along the Norwegian coastline between 2013 and 2022 were tagged with their batch-specific median EPA + DHA levels, which were then averaged per generation. This allowed distinguishing the three categories of EPA+DHA inclusion used in the study (Above Average, Average, and Below Average; Table 1) and the number of generations to which they were provided (Table 1). Atlantic salmon mortality (%), eFCR, and superior harvest quality (%) were then calculated for each category.
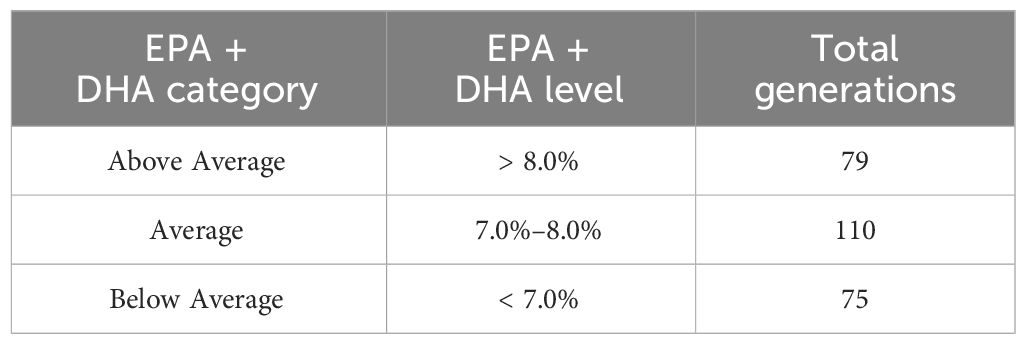
Table 1 The three categories considered in this study, their corresponding EPA + DHA levels, and the total number of generations to which they were provided.
A One-Way ANOVA test was utilized to see if the differences between the groups were significant. SciPy Statistical Library (https://scipy.org; BD study) was used. Values displayed are group means ± standard deviation (SD).
3 Results
3.1 Commercial farm study
Results indicated that fish gained 1.5 kg on average, corresponding to a 50% weight gain irrespective of treatment. This was below the expected 100% weight gain at this production stage, but the feeding period was 114 days (due to operational reasons) instead of the planned 184 days grow-out period. Although bFCR and eFCR were slightly higher for OON fish than for Control fish, the variability within the OON group was much higher than that within the Control group (Figure 1A). This was maybe due to predators on the OON cages, a factor that was not controlled for. Nevertheless, statistical testing revealed no significant differences between the two treatments.
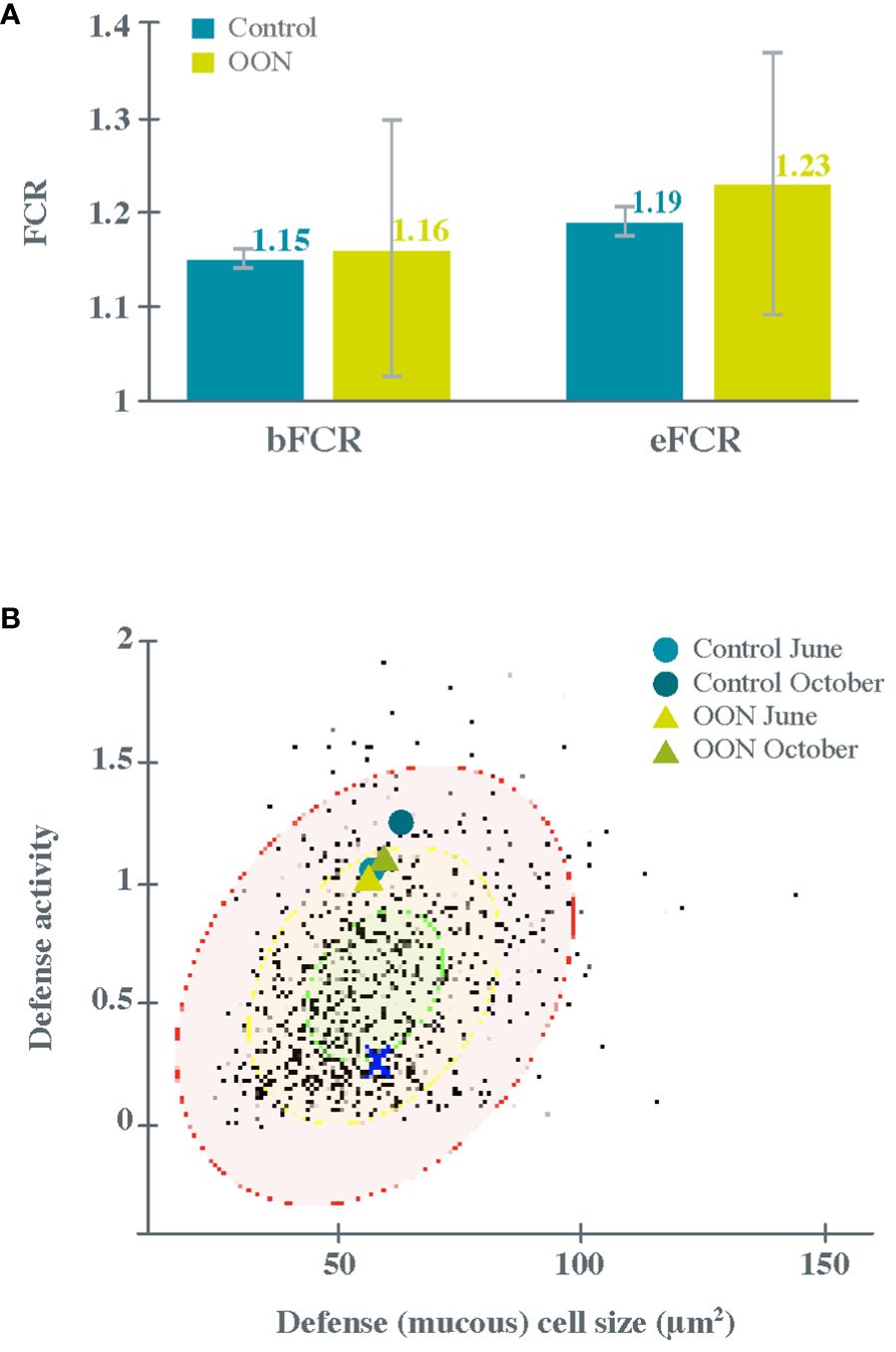
Figure 1 Growth (A) and health (B) performance of Atlantic salmon fed on the control and algal oil-supplemented diets (Control and OON groups, respectively). (A) The biological and economic feed conversion ratio (bFCR and eFCR) calculated for fish in both groups at the end of the trial is shown. Values indicated on each bar correspond to group means and the error bars to standard deviation. (B) Comparison of Atlantic salmon gill lamellar mucosal measures of mean mucous cell size and mean defense activity (including volumetric density) versus the Quantidoc database of the saltwater subset of salmon gill values in sea-based cages, recirculating water systems, and saltwater flow through systems (n =1452). Triangles = OON diet, circles = Control diet. X = mean for seven wild adult salmon. Green ellipse [30% confidence interval (CI)] is common for farmed salmon, yellow ellipse (70% CI) indicates transition to vulnerability or responding to threat, and red ellipse (95% CI) indicates active response to challenge or exhaustion; white area = 5% CI. Gills of healthy salmon have small and few mucous cells and increases in mucous cell size and abundance indicate a response to stress, water quality, particles, and pathogens.
At the beginning of the trial, the gill mucosa of fish in both groups (Figure 1B) were similar but much higher than that of wild adult Atlantic salmon gill cells (marked with X), indicating the activation of an immune response and therefore some degree of activated mucosal protection in the farmed fish. Throughout the trial, a slight improvement in OON fish compared to Control fish was observed, as both the activity and size of mucous cells clearly increased in Control fish throughout the trial but increased only slightly in OON fish. As a result, Control fish distanced more from the common, central zone (green circle) and moved into the red zone (vulnerable or responding), while OON fish remained in the amber zone of the database. This effect was particularly evident in the gills and foregut but not in the skin. In general, skin mucosa has a healthy shield from plentiful mucous cells, whereas gills in healthy circumstances have very few and small mucous cells thus granting easy passage of gasses through the lamellae.
At the beginning of the trial, the EPA + DHA level in 100 g of Atlantic salmon fillet was 0.80 g in Control fish and 0.75 g in OON fish; however, at end of the trial, the nutritional quality of Atlantic salmon fillets was significantly higher in OON fish than in Control fish (Figure 2A) as the EPA + DHA level almost doubled. This increase was even more meaningful as the feeding trial lasted only 114 days instead of the planned 184 days, i.e., the entire grow-out period. Regarding fillet physical quality, 27% more fish scored at least 24 in the SalmoFan color measurement scale in the OON group than in the control group (Figure 2B), indicating an improvement in pigmentation. Notably, these results were obtained using a feed with a higher total level of EPA + DHA (10% in OON vs. 6% in control) but with only a slightly higher FFDRoil (1.75 in OON and 1.68 in control), i.e., without any substantial increase in the marine footprint.
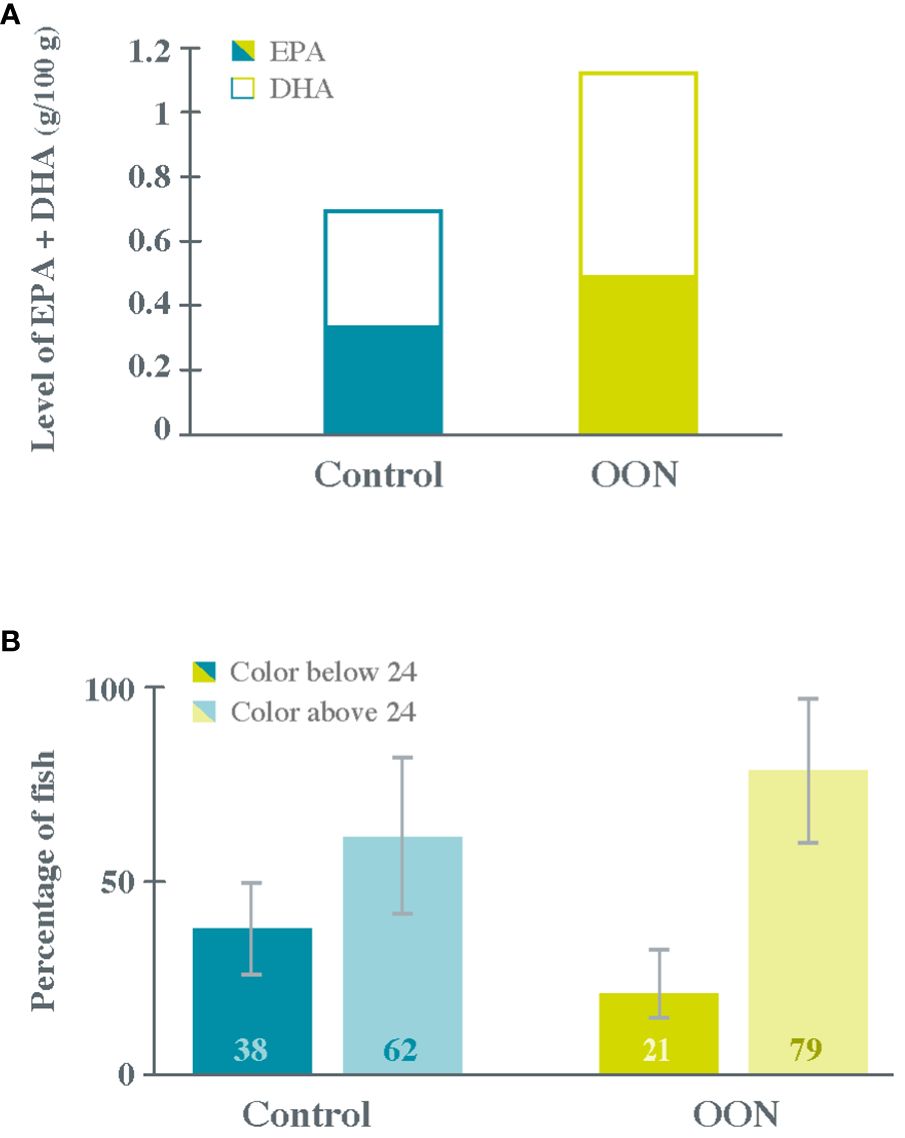
Figure 2 Nutritional (A) and physical (B) quality of Atlantic salmon fillets at the end of the commercial farm trial for fish fed on the control and algal oil-supplemented diets (Control and OON groups, respectively). Nutritional quality was measured as the level of EPA + DHA (g/100 g of fillet) and physical quality as the percentage of fish scoring at least 24 in the SalmoFan™ color measurement scale.
3.2 Big data study
In the BD study, the three EPA + DHA categories (i.e., groups) showed high variability (large SD) in mortality (Figure 3A), eFCR (Figure 3B), and superior harvest quality (Figure 3C). However, this variability was always smaller in the group with Above Average EPA + DHA level. The same trend was found for the interquartile range, indicating a higher predictability of the results obtained when providing EPA + DHA levels > 8%. Furthermore, this group showed the lowest mean mortality and eFCR and the highest mean superior harvest quality. Although the mortality mean was < 20% in all three groups, and no statistical differences were found among them, the interquartile range for the Above Average group was about half of that observed for the Below Average group (Figure 3A). Significant improvements were found in the eFCR and superior harvest quality of the Above Average group, with mean values decreasing to 1.29 and increasing to 90%, respectively (Figures 3B, C).
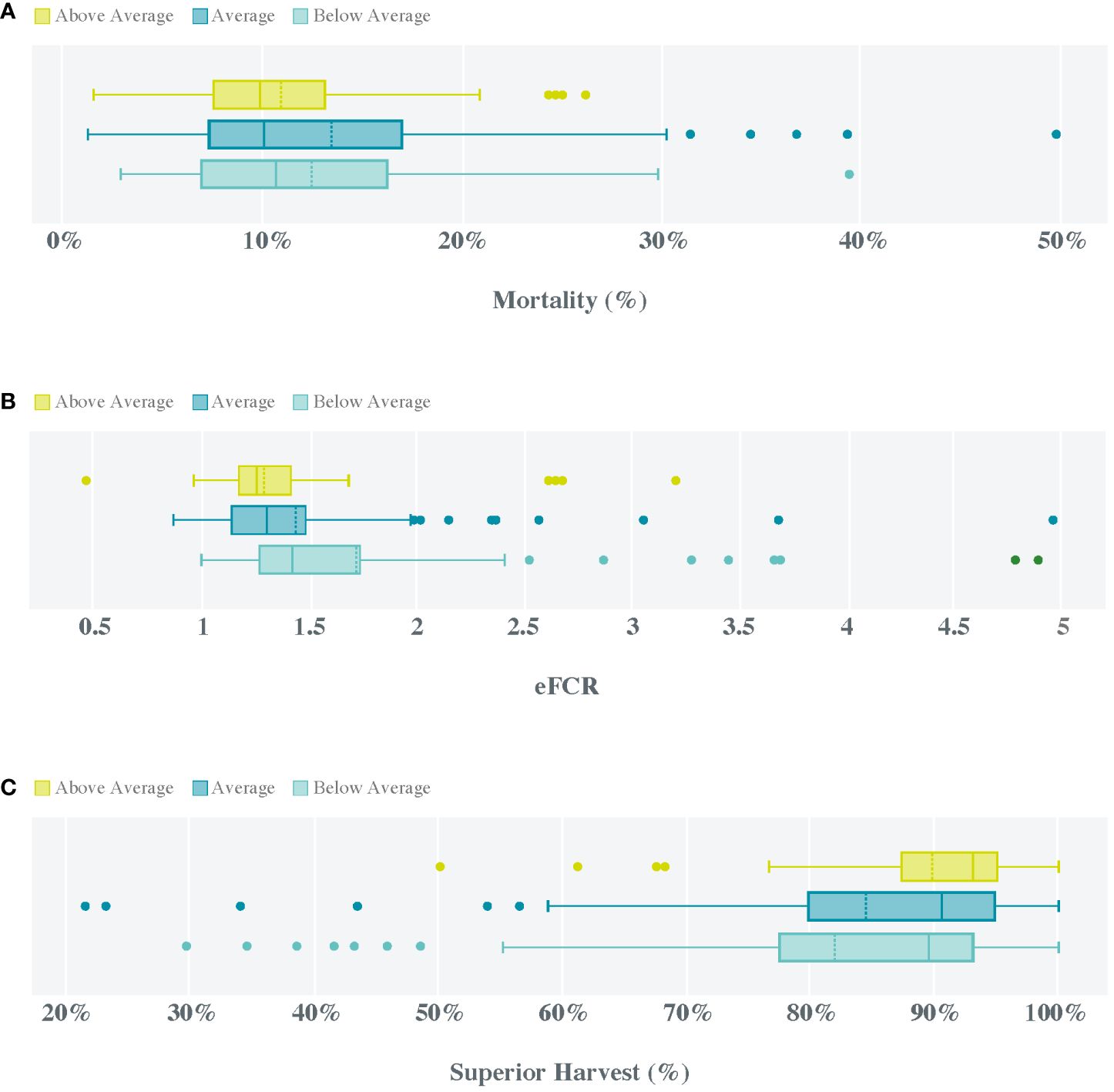
Figure 3 Mortality (%, A), economic feed conversion ratio (eFCR, B), and superior harvest percentage (%, C) in the three EPA + DHA categories (groups) examined in the present study, based on data obtained from 291 Atlantic salmon generations between 2013 and 2022 in 99 farms along the Norwegian coast.
In summary, the BD study revealed several important insights that can help feed managers to quantify the benefits of higher EPA + DHA levels in farmed Atlantic salmon diets. A key finding was that generations of fish supplied with EPA + DHA levels > 8% showed 21% less variability in the total mortality and an 11% improvement in the eFCR. For farmers, this translated into a 27% higher chance of obtaining a superior harvest quality higher than 90%. Overall, these results allow Atlantic salmon farmers to quantify the benefits of adding higher levels of EPA + DHA to their feed as an improved return on investment (ROI) without a major risk, as the effects observed in this study reflect real data from millions of fish.
4 Discussion
The aquaculture industry is currently facing the important challenge of increasing production, growth rates, and nutritional quality, without compromising fish health and welfare or increasing its environmental impact, thereby ultimately improving the industry’s productivity and sustainability. However, salmon feed formulations have changed considerably due to the limited availability of traditionally used marine ingredients, their high costs, and high environmental impact of exploiting such sources. Fish oil in Atlantic salmon feeds has been progressively replaced with vegetable alternatives, mostly rapeseed oil (20.1%) (Aas et al., 2022). This transition had a significant and immediate effect on EPA + DHA levels, which nearly halved in the flesh of Atlantic salmon farmed between 2006 and 2015 (~12% to ~5% of total lipids) (Sprague et al., 2016).
The deleterious effects of low dietary EPA + DHA levels on the growth, survival, health, wellbeing, and nutritional quality have been a concern in the Atlantic salmon industry, and therefore a plethora of studies using different amounts and ratios of EPA + DHA from different sources has been conducted (Katan et al., 2020; Mock et al., 2020; Huyben et al., 2021; Løvmo et al., 2021; Hatlen et al., 2022; Lutfi et al., 2022; Ruyter et al., 2022; Santigosa et al., 2023). Although a recent study found that Atlantic salmon in the freshwater phase can require an EPA + DHA level in feed as low as 3% of TFA because fish at this life stage can bioconvert α-linolenic acid to DHA at ~25% (Qian et al., 2020), EPA + DHA requirements depend on the growing phase and are likely to increase under challenging conditions (Sissener et al., 2016; Bou et al., 2017b; Lutfi et al., 2022). However, results have not always been consistent among studies and this has contributed to delays in the adoption of feeds with higher than required EPA + DHA levels by the farmers as there is no widely recognized model to calculate the ROI for optimum nutrition in aquaculture. Because of this uncertainty, feed producers and farmers currently have differing views on the optimum specifications for EPA + DHA in salmon feed; however, operators are transitioning towards higher specifications in feed as alternative ingredient sources, such as AO, have become commercially available.
Although some of these limitations could be at least partially overcome by collaborations between the feed and supplement industries and fish farms (Mock et al., 2020), to determine the optimal composition of feed for a particular species in a given environment that results in the best ROI and lowest waste, a large volume of diverse datasets need to be collected for the feed, the fish, and the farm (Neethirajan, 2020). However, this is not possible to achieve in commercial-scale trials, which are usually conducted at a single farm during a single production cycle. Furthermore, the few trials performed at commercial-scale and under the farm conditions generally revealed no significant differences in fish growth and bFCR among the groups fed different EPA + DHA levels at least during one production stage (e.g., Hatlen et al., 2022; Lutfi et al., 2022; Ytrestøyl et al., 2023), as was the case of the CF study presented here. However, eFCR results revealed two different and opposing trends in the CF and BD studies. While in the BD study, Atlantic salmon fed diets with EPA + DHA levels > 8% had a significantly lower and less variable eFCR than those fed EPA + DHA < 8%, in the CF study fish fed the 10% EPA + DHA diet showed a slightly higher eFCR, perhaps due to predator events. This contradiction between the two studies reflects the importance of using large sample sizes (several generations; 10-year dataset) to evaluate the consequences of providing different levels of EPA + DHA on the long term, as pointed out in the scientific literature (Mock et al., 2020; Lutfi et al., 2022). According to the latest data of The Marine Ingredients Organisation (IFFO), the eFCR of salmonids has decreased from 1.54 in 2000 to 1.27 in 2020 (https://www.iffo.com/efcr-data), a level that is in agreement with the 1.29 mean value found for Atlantic salmon fed on diets containing EPA + DHA > 8% in the BD study, suggesting that increased levels of these EFAs lead to better nutrient utilization and thus to improved sustainability. This is corroborated by the FFDRoil values obtained in the CF study, where increasing the level of EPA + DHA in feed by 60% resulted in only a 4% increase in the FFDRoil value.
The results of both studies presented here also support that estimating EPA + DHA requirements based primarily (or solely) on growth and survival is not feasible, in agreement with previous studies (Bou et al., 2017a; Qian et al., 2020; Lutfi et al., 2022), as several fish health and fillet quality impacts are connected with lower EPA + DHA levels. In the CF study, Atlantic salmon fed the diet with 10% EPA + DHA showed defense cells’ size and activity much closer to the norm than Atlantic salmon fed the control diet, particularly in the gills. The late stage of life at which this trial was conducted suggests that all the gills have likely been repeatedly exposed to a multitude of challenges. The small but positive effect of the OON diet on gills found in the CF study may be by promoting cell differentiation and cell viability (van Beelen et al., 2007). This improvement of defense mechanisms under challenging conditions, such as those in the farm environment, when consuming higher EPA + DHA levels is in agreement with previous findings (Arnemo et al., 2017; Bou et al., 2017b; Løvmo et al., 2021). Higher levels of EPA + DHA in the feed also resulted in significantly higher levels of these EFAs in the fillet throughout the CF study, indicating high retention of these EFAs in the muscle, as found in previous studies (e.g (Santigosa et al., 2021; Lutfi et al., 2022; Ruyter et al., 2022; Ytrestøyl et al., 2023). In the BD study, results indicate that feeds containing EPA + DHA levels > 8% result in Atlantic salmon fillets of better nutritional quality, which has a positive impact on the value of this species as a source of these omega-3 EFAs for humans. In the CF study, the better nutritional quality of Atlantic salmon fillets when the fish were fed a 10% EPA + DHA diet was accompanied by an improvement in fillet color, as shown by the significantly higher percentage of fillets with a score of at least 24 in the SalmoFan color scale. This positive relationship between EPA + DHA levels and increased SalmoFan scores is in agreement with the recent reported results for Atlantic salmon grown in sea cages in Norway (Lutfi et al., 2022; Ytrestøyl et al., 2023).
Overall, the results presented here indicate that BD can be used to describe, analyze, cluster, segment, score, and predict the effects of nutritional intervention (e.g., new feed ingredients) on improved feed efficiency, fish health, and survival to address the limitations of commercial farm tests, and ultimately help farmers on their decision-making (Roy, 2019). To the best of our knowledge, this paper presents the first approach to using BD to present proof that optimum nutrition, in this case EPA + DHA levels > 8%, leads to improved eFCR, mortality rate, and superior harvest quality. More fish of higher quality and with better feed conversion ability reaching the harvest size will clearly result in an improved biological performance and therefore a better ROI. Although this was demonstrated here for EPA + DHA levels, the BD approach could be utilized for other feed ingredients and functional additives, as well as other zootechnical and wellbeing indices. Moreover, as sensors and smart technologies expand to acquire more data related to the farm environment, feed quality, and productivity, more routes will open to model and forecast patterns related to growth, survival, and FCR, which are the most essential factors in aquaculture (Benjelloun et al., 2022), using BD technology and mathematical models. Such analytical methods based on expanded datasets also have the advantage of profiling and clustering farms according to their unique environmental (site, temperature, location) and operational (feed type and size, animal genetics) features to design the best ROI model. Hence, future BD analysis should integrate data from Atlantic salmon farms not only in Norway but from different world regions (e.g., Chile) that might help strengthen the findings reported here and reveal new patterns.
Both studies show that there are some unique limitations involved with transferring lab-controlled conditions to real-world commercial farming. These include limitations for the transparency of commercial diet formulations, difficulty in separating out potentially confounding factors that may also impact biological performance observations, and the reduced number of replicates to strengthen statistical analysis of data. However whilst recognizing these possible limitations, the results of the studies offer some important insights that can help the salmon farming industry to quantify the benefits of EPA+DHA in farmed Atlantic salmon diets.
In conclusion, the data provided here, are valuable insights to feed managers helping them to formulate a business case for transitioning from least cost formulations to optimum formulations for the fish and for farm productivity, which is based on including higher (> 8%) levels of EPA + DHA in the feed. This is conducive to optimum farm productivity, as demonstrated by reduced fish mortality, better FCR, and improved fish growth and harvest quality. Moreover, if the EPA and DHA originate from a sustainable alternative source and with high bioavailability for fish, as is the case of AO, the sustainability of the industry can be further improved, allowing it to keep on growing to feed the demands of the human population for nutritious and safe food, aligning with the United Nations Sustainable Development Goals 2 (zero hunger), 3 (good health and wellbeing), 12 (responsible consumption and production), 13 (climate action), and 14 (life below water). Although the current example shows the benefits of a sustainable alternative omega-3 oil source for salmon farming, using a BD approach on real farm data to complement lab-scale and farm trials may therefore be the most upfront pathway to demonstrate the benefits of nutritional interventions for the business, for the people, and for the planet.
Data availability statement
The datasets presented in this article are not readily available because raw data from farmers cannot be shared without prior farmer’s permission. Requests to access the datasets should be directed to aWFuLmNhcnJAdmVyYW1hcmlzLmNvbQ==.
Ethics statement
Ethical approval was not required for the studies involving animals in accordance with the local legislation and institutional requirements because Farm study (Chile) used commercial farm animals grown in a commercial set-up; Big data study (Norway) used only data obtained from salmon farms. Written informed consent was obtained from the owners for the participation of their animals in this study.
Author contributions
IC: Funding acquisition, Conceptualization, Investigation, Methodology, Validation, Visualization, Writing – original draft, Writing – review & editing. ES: Data curation, Formal Analysis, Methodology, Writing – original draft, Writing – review & editing. TC: Data curation, Formal Analysis, Methodology, Writing – review & editing. JC: Data curation, Formal Analysis, Methodology, Writing – review & editing.
Funding
The author(s) declare that financial support was received for the research, authorship, and/or publication of this article. Veramaris® partially funded the CF study and entirely funded the BD study.
Acknowledgments
The authors would like to thank: H. Wellmann, A. Castillo, P. Solis and J. Opitz at Blumar Seafoods for conducting the Commercial farm study; C. Gatica and M. Oyarzun at Skretting Chile for the provision of commercial diets for the Commercial farm study; K. Pittman at Quantidoc, M. Vera Gaedicke at VeHiCe, and F. Strachan at University of Stirling Institute of Aquaculture for their assistance with performing analysis for the Commercial farm study; Y. Le Gal, J. Torres, and F. Almendras at Veramaris for their contribution to the development of concepts explored in both the Commercial farm and Big Data studies and coordination of associated operations; J.F. Marques and M.C. Leal for their support with writing this manuscript.
Conflict of interest
IC is employed by Veramaris; ES is employed by dsm-firmenich; TC and JC are employed by Manolin. This study received funding from Veramaris. The funder had the following involvement with the study: the study design, collection, analysis, interpretation of data, the writing of this article and the decision to submit it for publication.
Publisher’s note
All claims expressed in this article are solely those of the authors and do not necessarily represent those of their affiliated organizations, or those of the publisher, the editors and the reviewers. Any product that may be evaluated in this article, or claim that may be made by its manufacturer, is not guaranteed or endorsed by the publisher.
Supplementary material
The Supplementary Material for this article can be found online at: https://www.frontiersin.org/articles/10.3389/fmars.2024.1379066/full#supplementary-material
References
Aas T. S., Åsgård T., Ytrestøyl T. (2022). Utilization of feed resources in the production of Atlantic salmon (Salmo salar) in Norway. Aquaculture Rep. 26, 101316. doi: 10.1016/j.aqrep.2022.101316
AOAC (2012). Official methods of analysis of AOAC international. 19th ed (Maryland: AOAC International Press), 27–29. Chapter 41.
Arnemo M., Kavaliauskis A., Andresen A. M. S., Bou M., Berge G. M., Ruyter B., et al. (2017). Effects of dietary n-3 fatty acids on Toll-like receptor activation in primary leucocytes from Atlantic salmon (Salmo salar). Fish Physiol. Biochem. 43, 1065–1080. doi: 10.1007/s10695-017-0353-4
Benjelloun S., El Aissi M. E. M., Lakhrissi Y., Ali S. E. H. B. (2022). Big Data technology architecture proposal for smart agriculture for Moroccan fish farming. WSEAS Trans. Inf. Sci. Appl. 19, 311–322. doi: 10.37394/23209.2022.19.33
Bou M., Berge G. M., Baeverfjord G., Sigholt T., Østbye T.-K., Romarheim O. H., et al. (2017a). Requirements of n-3 very long-chain PUFA in Atlantic salmon (Salmo salar L): effects of different dietary levels of EPA and DHA on fish performance and tissue composition and integrity. Br. J. Nutr. 117, 30–47. doi: 10.1017/S0007114516004396
Bou M., Berge G. M., Baeverfjord G., Sigholt T., Østbye T.-K., Ruyter B. (2017b). Low levels of very-long-chainn-3 PUFA in Atlantic salmon (Salmo salar) diet reduce fish robustness under challenging conditions in sea cages. J. Nutr. Sci. 6. doi: 10.1017/jns.2017.28
Carr I., Glencross B., Santigosa E. (2023). The importance of essential fatty acids and their ratios in aquafeeds to enhance salmonid production, welfare, and human health. Front. Anim. Sci. 4. doi: 10.3389/fanim.2023.1147081
Hatlen B., Larsson T., Østbye T.-K., Romarheim O. H., Rubio L. M., Ruyter B. (2022). Improved fillet quality in harvest-size Atlantic salmon fed high n-3 canola oil as a DHA-source. Aquaculture 560, 738555. doi: 10.1016/j.aquaculture.2022.738555
Horn S. S., Sonesson A. K., Krasnov A., Moghadam H., Hillestad B., Meuwissen T. H. E., et al. (2019). Individual differences in EPA and DHA content of Atlantic salmon are associated with gene expression of key metabolic processes. Sci. Rep. 9, 3889. doi: 10.1038/s41598–019-40391–2
Huyben D., Grobler T., Matthew C., Bou M., Ruyter B., Glencross B. (2021). Requirement for omega-3 long-chain polyunsaturated fatty acids by Atlantic salmon is relative to the dietary lipid level. Aquaculture 531, 735805. doi: 10.1016/j.aquaculture.2020.735805
Katan T., Xue X., Caballero-Solares A., Taylor R. G., Rise M. L., Parrish C. C. (2020). Influence of dietary long-chain polyunsaturated fatty acids and ω6 to ω3 ratios on head kidney lipid composition and expression of fatty acid and eicosanoid metabolism genes in atlantic salmon (Salmo salar). Front. Mol. Biosci. 7. doi: 10.3389/fmolb.2020.602587
Løvmo S. D., Whatmore P., Sundh H., Sigholt T., Madaro A., Bardal T., et al. (2021). Effects of Atlantic salmon (Salmo salar) fed low- and high HUFA diets on growth and midgut intestinal health. Aquaculture 539, 736653. doi: 10.1016/j.aquaculture.2021.736653
Lutfi E., Berge G., Baeverfjord G., Sigholt T., Bou M., Larsson T., et al. (2022). Increasing dietary levels of the omega-3 long-chain polyunsaturated fatty acids, EPA and DHA, improves the growth, welfare, robustness, and fillet quality of Atlantic salmon in sea cages. Br. J. Nutr. doi: 10.1017/S0007114522000642
Mock T. S., Francis D. S., Drumm D. W., Versace V. L., Glencross B. D., Smullen R. P., et al. (2020). A systematic review and analysis of long-term growth trials on the effect of diet on omega-3 fatty acid levels in the fillet tissue of post-smolt Atlantic salmon. Aquaculture 516, 734643. doi: 10.1016/j.aquaculture.2019.734643
Neethirajan S. (2020). The role of sensors, big data and machine learning in modern animal farming. Sens. BioSensing Res. 29, 100367. doi: 10.1016/j.sbsr.2020.100367
Oliva-Teles A., Enes P., Peres H. (2015). “Replacing fishmeal and fish oil in industrial aquafeeds for carnivorous fish,” in Feed and feeding practices in aquaculture (Elsevier), 203–233. doi: 10.1016/b978–0-08–100506–4.00008–8
Qian C., Hart B., Colombo S. M. (2020). Re-evaluating the dietary requirement of EPA and DHA for Atlantic salmon in freshwater. Aquaculture 518, 734870. doi: 10.1016/j.aquaculture.2019.734870
Roques S., Deborde C., Richard N., Skiba-Cassy S., Moing A., Fauconneau B. (2020). Metabolomics and fish nutrition: a review in the context of sustainable feed development. Rev. Aquac. 12, 261–282. doi: 10.1111/raq.12316
Roy A. K. (2019). Big data analytics to fight challenges of fisheries and aquaculture sector. J. Inland fisheries Soc. India 51, 30–36. doi: 10.13140/RG.2.2.36654.66884
Ruyter B., Bou M., Berge G. M., Mørkøre T., Sissener N. H., Sanden M., et al. (2022). A dose-response study with omega-3 rich canola oil as a novel source of docosahexaenoic acid (DHA) in feed for Atlantic salmon (Salmo salar) in seawater; effects on performance, tissue fatty acid composition, and fillet quality. Aquaculture 561, 738733. doi: 10.1016/j.aquaculture.2022.738733
Sadiku M. N. O., Ashaolu T. J., Ajayi-Majebi A., Musa S. M. (2020). Big data in food industry. Int. J. Sci. Adv. 1. doi: 10.51542/ijscia.v1i3.5
Santigosa E., Brambilla F., Milanese L. (2021). Microalgae oil as an effective alternative source of EPA and DHA for gilthead seabream (Sparus aurata) aquaculture. Anim. (Basel) 11. doi: 10.3390/ani11040971
Santigosa E., Constant D., Prudence D., Wahli T., Verlhac-Trichet V. (2020). A novel marine algal oil containing both EPA and DHA is an effective source of omega-3 fatty acids for rainbow trout (Oncorhynchus mykiss). J. World Aquac. Soc 51, 649–665. doi: 10.1111/jwas.12699
Santigosa E., Olsen R. E., Madaro A., Trichet V. V., Carr I. (2023). Algal oil gives control of long-chain omega-3 levels in full-cycle production of Atlantic salmon, without detriment to zootechnical performance and sensory characteristics. J. World Aquac. Soc 54, 861–881. doi: 10.1111/jwas.12947
Santigosa E., Verlhac-Trichet V., Olsen R. E., Figuereido-Silva C. (2018). “A microalgal oil containing EPA+DHA can be an effective source of omega 3 for Atlantic salmon post- smolts,” in Proceedings of the 18th International Symposium on Fish Nutrition & Feeding (ISFNF). 3–20.
Sissener N. H., Waagbø R., Rosenlund G., Tvenning L., Susort S., Lea T. B., et al. (2016). Reduced n-3 long chain fatty acid levels in feed for Atlantic salmon (Salmo salar L.) do not reduce growth, robustness or product quality through an entire full scale commercial production cycle in seawater. Aquaculture 464, 236–245. doi: 10.1016/j.aquaculture.2016.06.034
Sprague M., Dick J. R., Tocher D. R. (2016). Impact of sustainable feeds on omega-3 long-chain fatty acid levels in farmed Atlantic salmon 2006–2015. Sci. Rep. 6, 1–9. doi: 10.1038/srep21892
Sprague M., Fawcett S., Betancor M. B., Struthers W., Tocher D. R. (2020). Variation in the nutritional composition of farmed Atlantic salmon (Salmo salar L.) fillets with emphasis on EPA and DHA contents. J. Food Compost. Anal. 94, 103618. doi: 10.1016/j.jfca.2020.103618
Tao Q., Ding H., Wang H., Cui X. (2021). Application research: Big data in food industry. Foods 10, 2203. doi: 10.3390/foods10092203
Tocher D. R. (2015). Omega-3 long-chain polyunsaturated fatty acids and aquaculture in perspective. Aquaculture 449, 94–107. doi: 10.1016/j.aquaculture.2015.01.010
van Beelen V. A., Roeleveld J., Mooibroek H., Sijtsma L., Bino R. J., Bosch D., et al. (2007). A comparative study on the effect of algal and fish oil on viability and cell proliferation of Caco-2 cells. Food Chem. Toxicol. 45, 716–724. doi: 10.1016/j.fct.2006.10.017
Keywords: Atlantic salmon, EPA, DHA, omega-3, commercial trial, big data, quality, health
Citation: Carr I, Santigosa E, Chen T and Costantino J (2024) Optimum eicosapentaenoic acid + docosahexaenoic acid levels for farmed Atlantic salmon: closing the gap between science and commercial practice. Front. Mar. Sci. 11:1379066. doi: 10.3389/fmars.2024.1379066
Received: 30 January 2024; Accepted: 26 April 2024;
Published: 10 May 2024.
Edited by:
Yngvar Olsen, NTNU, NorwayReviewed by:
Ye Yuan, Shantou University, ChinaYang Jin, Johns Hopkins University, United States
Keshuai Li, BioMar, Norway
Copyright © 2024 Carr, Santigosa, Chen and Costantino. This is an open-access article distributed under the terms of the Creative Commons Attribution License (CC BY). The use, distribution or reproduction in other forums is permitted, provided the original author(s) and the copyright owner(s) are credited and that the original publication in this journal is cited, in accordance with accepted academic practice. No use, distribution or reproduction is permitted which does not comply with these terms.
*Correspondence: Ian Carr, aWFuLmNhcnJAdmVyYW1hcmlzLmNvbQ==