- 1Department of Animal Sciences, College of Agricultural and Life Sciences, University of Florida, Gainesville, FL, United States
- 2Aquatic Animal Health Program, College of Veterinary Medicine, University of Florida, Gainesville, FL, United States
The effects of anthropogenic sources of light on the circadian biology of marine animals are largely unexplored at the molecular and cellular level. Given that light is a major driver of circadian rhythms at the behavioral, physiological, cellular, and even molecular levels, it is important to consider the effects that anthropogenic light, especially at night, has on aquatic species. With the expanding data generated from circadian clock research, it is surprising that these techniques have not been applied more frequently to better understand how artificial light affects animal circadian rhythms. Circadian research has been limited to behavioral and physiological observations in wild marine animals rather than a cellular and molecular understanding due to the logistical constraints. While there are some benefits to using artificial light at night (ALAN), there have also been many studies reporting physiological and behavioral consequences in response to exposure to ALAN. Here, the benefits and consequences of using ALAN in the marine environment are reviewed. Furthermore, perspectives on research limitations and future research directions are discussed. Taken together, this is an important area in which more information is required to translate our understanding of circadian biology into better practices to promote the health and welfare of marine animals.
Introduction
Environmental stimuli such as photoperiod (light/dark cycles), temperature, and tidal changes govern many behavioral and physiological processes in animals. Because light is a major driver of circadian rhythms, the use of artificial light, especially at night, should be considered in efforts to conserve species in both natural populations and those under managed human care. Disruption of natural lighting through the exposure of artificial light at night (ALAN) or the incorrect use of ALAN in managed care settings can disrupt these pathways. In most animals, the circadian clock is the internal molecular timekeeping mechanism. The hallmark of the circadian clock mechanism is a transcriptional/translational feedback loop where one revolution of this feedback loop takes approximately 24 hours to complete under normal environmental conditions. (Dubruille and Emery, 2008; Takahashi, 2017; Stanton et al., 2022). This mechanism is integrated with numerous other physiological pathways including metabolism, sleep regulation, reproduction, development, cell turnover, and immune regulation (Morris et al., 2020; Stanton et al., 2022). Outputs of these pathways can also fine-tune the circadian clock mechanism through post-translational modifications, which are well characterized in vertebrate model organisms (Morris et al., 2020).
The circadian clock is entrained by internal and external environmental stimuli referred to as ‘zeitgebers’ (Figure 1). Many specific behaviors and biological/physiological processes are evolutionarily adapted to the rhythms of natural photoperiods (Tidau et al., 2021). Tidal cycles, as well as photoperiod, function as zeitgebers that entrain biological clocks of marine species and synchronize large scale responses (Tidau et al., 2021). While peripheral circadian clocks can be entrained by localized zeitgebers, such as feeding, the master circadian regulator is entrained by light (Morris et al., 2020; Stanton et al., 2022). Animals perceive light information through their eyes or through photoreceptors in the absence of eyes (Stanton et al., 2022). Cryptochromes (CRYs) function as primitive photoreceptors activated in response to blue light (Schlacher et al., 2007; Mendes et al., 2017; Cheng et al., 2022; Stanton et al., 2022). Sessile organisms such as corals respond to different wavelengths of light, whereas, mobile aquatic species use phototaxis (movement toward or away from a light stimulus). The phototactic nature of fish results in light-directed swimming and aggregation with varying preferences for different wavelengths of light (Marchesan et al., 2005). Furthermore, different wavelengths of light result in different behavioral and physiological effects. For example, red light has been shown to stimulate feeding behavior and metabolism in Nile tilapia (Oreochromis niloticus) (Volpato et al., 2013).
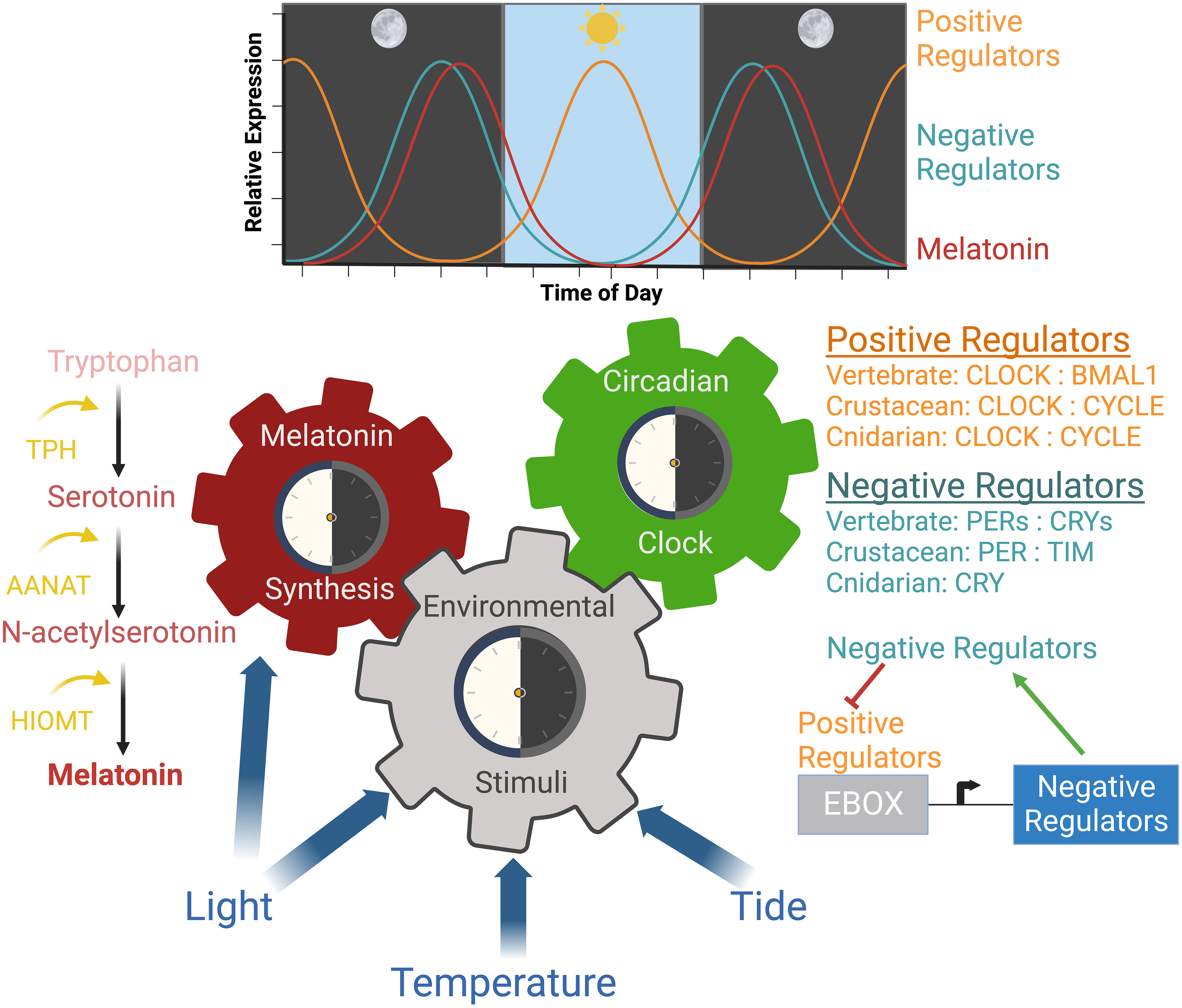
Figure 1 Mechanisms and rhythms of circadian regulation. Molecular and hormonal circadian mechanisms occur in response to environmental stimuli. The molecular clock is a transcription/translational feedback loop where the expression of positive regulators are highest during the day and expression of negative regulators are highest at night. Light is the master regulator of this mechanism. Light also regulates melatonin synthesis, which occurs during the night leading to high melatonin accumulation before dawn. Melatonin decreases as it is circulated and utilized. Both mechanisms regulate physiological and cellular processes in a circadian manner in marine animals. Created with BioRender.com.
Melatonin is another regulator of an animal’s circadian biology that is superseded by the molecular circadian clock (Falcón et al., 2009; Tan et al., 2010). Melatonin production has been identified in aquatic invertebrates including cnidarians, dinoflagellates, mollusks, annelids, flatworms, nematodes, and crustaceans (Hardeland and Poeggeler, 2003; Muñoz et al., 2011; Roopin and Levy, 2012; Sainath et al., 2013; Peres et al., 2014; Tosches et al., 2014; Tran et al., 2020; Zhu et al., 2022). ALAN suppresses melatonin synthesis and has been linked to a reduction in fitness (Jones et al., 2015; Grubisic et al., 2019). The ability to monitor melatonin synthesis is another method that may be used to assess the impact of ALAN on marine animals.
Here, the effects of using ALAN are discussed. Considerations such as the timing of light/dark periods and wavelength of light are both important factors when adapting these practices to minimize impacts on an animal’s circadian clock mechanism. Accordingly, a perspective on current gaps in knowledge and research opportunities to inform best care and conservation practices for managed marine animals is provided.
The effects of ALAN on marine animals in coastal environments
The use of artificial light at night (ALAN) near coastal environments brings few documented benefits, which are generally restricted to enhancing fish yield through aquaculture. This is exemplified by the continuous ALAN illumination in ocean pens that is common practice for species of salmonids (McConnell et al., 2010). This continuous illumination attracts a multitude of invertebrate species that aggregate in the illuminated area, increasing the available food resources for fish in the pens (Delupis and Rotondo, 1988; Jékely et al., 2008; McConnell et al., 2010; McLeod and Costello, 2017; Siddik and Satheesh, 2021). However, the resounding effects that ALAN has on the circadian biology of these farmed fish is largely understudied and it is reasonable to hypothesize that there will be long-term effects on behavior and physiology due to chronic circadian clock disruption.
In coastal communities, specifically marine intertidal areas, the use of ALAN is noted to have substantial negative effects on the aquatic species inhabiting these nearshore locations. These species receive relatively constant exposure to varying levels of ALAN due to significant coastal development and urbanization. Constant exposure to ALAN may disrupt normal circadian clock function by interfering with an individual’s ability to function appropriately during natural cycles of light and dark (Kopperud and Grace, 2017). This has downstream effects on different behavioral and physiological processes, many of which are innately tied to circadian or circatidal rhythms (Brüning et al., 2015). Such alterations can be observed in variations in activity patterns or disruptions in the expression of circadian clock genes. Examples include loss of circadian rhythm of activity in the isopod (Tylos spinulosus), arrhythmic expression of circadian clock genes in the Pacific oyster (Crassostrea gigas), higher activity levels in rockfish (Girella laevifrons) that may be attributed to a loss of internal circadian and circatidal rhythms over time, and direct effects on the rhythmic production of melatonin in European perch (Perca fluviatilis) with many fish lacking circadian melatonin rhythms as compared to control fish (Brüning et al., 2015; Duarte et al., 2019; Pulgar et al., 2019; Botté et al., 2023).
The use of ALAN in the oceanic environment
Pelagic species may be exposed to ALAN through different venues including lighting from bridges, oil platforms, or vessels such as commercial fishing vessels or cruise ships (Hölker et al., 2010; Davies et al., 2014; Szekeres et al., 2017). Interruptions to the natural biological clocks may have significant detrimental effects on individual marine organisms and biodiversity as a whole (Figure 2). Data is severely lacking in regards to the effects of ALAN on the circadian rhythms of pelagic species, which is likely due to the logistical constraints of research in the pelagic environment. Regardless, some information does exist demonstrating the advantages and disadvantages of ALAN exposure, particularly in commercial fisheries and coral research, respectively.
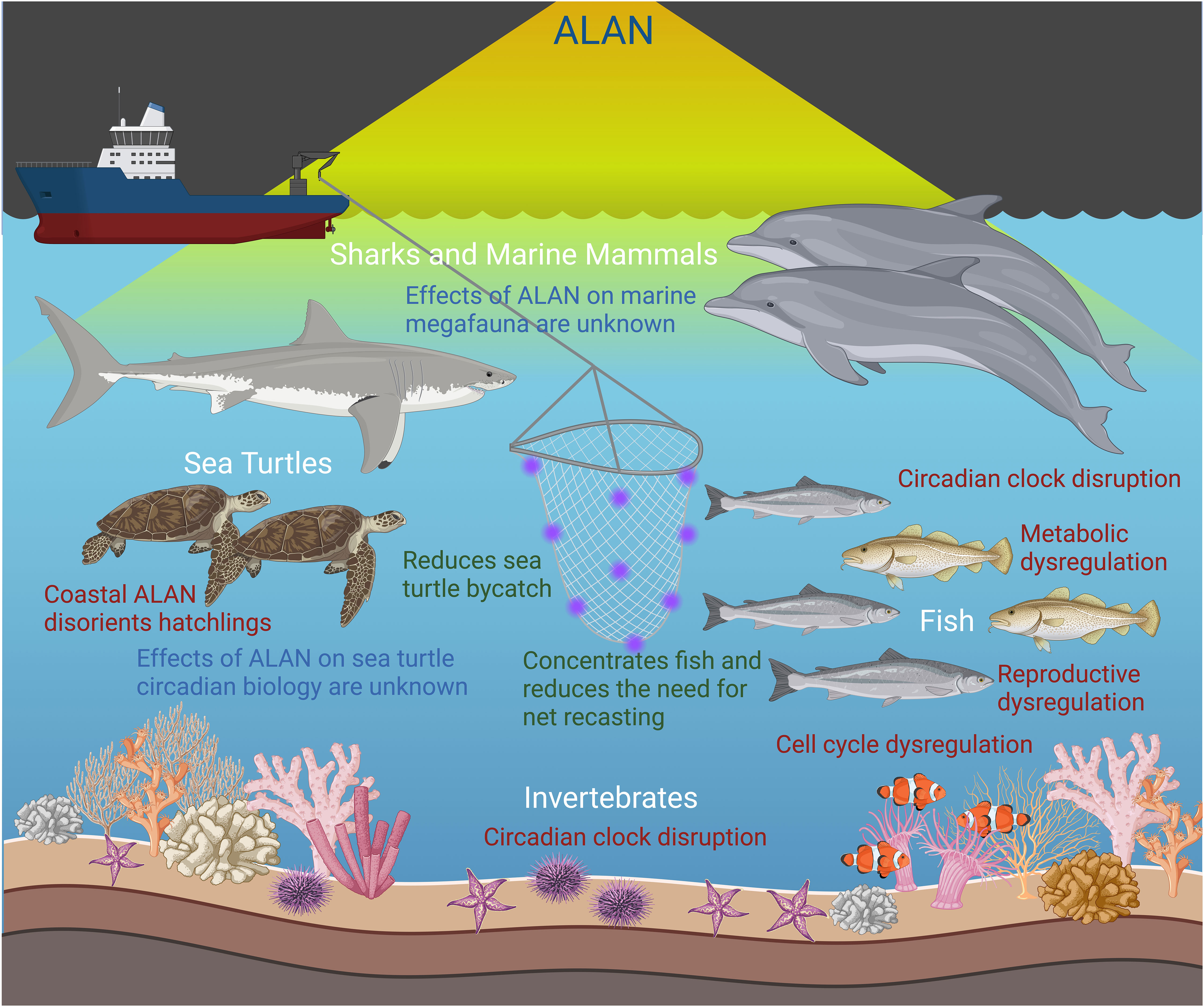
Figure 2 Effects of artificial light at night (ALAN) exposure in the aquatic environment. ALAN is beneficial in enhancing fishing practices and reducing bycatch. However, ALAN exposure disrupts the circadian clock in fish and some invertebrates leading to alterations in important processes such as metabolism, cell division, and reproduction. The effects of ALAN on the circadian biology of marine megafauna are currently unknown. Created with BioRender.com.
ALAN has been utilized on commercial fishing vessels to enhance fish yields as well as reduce bycatch of unwanted species (Arakawa et al., 1998; Marchesan et al., 2005; Nguyen and Winger, 2019). This reduces the need for continuous net redeployment to meet fish quotas and reduces fuel consumption (Nguyen and Winger, 2019). Ultraviolet, blue, and green light-emitting diode (LED) lights on nets and lines have been shown to reduce the amount of bycatch of fish and sea turtles; however, the mechanism by which this occurs is unknown (Wang et al., 2013; Hannah et al., 2015; Virgili et al., 2018). Therefore, use of ALAN in commercial fisheries has proven to be a beneficial conservation tool. The potential effects from commercial fishery use of ALAN on the circadian clock of marine organisms may be short-lived as the clock can resynchronize with the subjective day and animals may not encounter a fishing vessel every night.
Outside of commercial fisheries, simulated ALAN exposure experiments have demonstrated the negative effects of ALAN on different offshore species. The majority of this research has focused on corals, which are highly photosensitive and have well-developed circadian behaviors (Rosenberg et al., 2019), and are thus intensely vulnerable to the effects of ALAN (Fobert et al., 2023). In coral species collected from oceanic environments, ALAN exposure reduced reproductive fitness by delaying gametogenesis and inhibiting synchronization of gamete release (Ayalon et al., 2021). Furthermore, it has also been demonstrated that spawning occurs closer to a full moon in corals exposed to ALAN, suggesting that speeding up the spawning time can lead to decreased fertilization and survival of gametes (Davies et al., 2023). Additionally, metabolic fitness was reduced as a result of a lower rate of photosynthesis, a lower electron transport rate, and the disruption of coral-dinoflagellate symbiosis (Ayalon et al., 2019; Levy et al., 2020; Tamir et al., 2020). In Acropora eurystoma, constant exposure to ALAN resulted in arrhythmic expression of known clock genes and alteration of cellular pathways (Rosenberg et al., 2019).
ALAN exposure has also been shown to negatively impact the physiology and overall fitness of reef fishes with significant effects on metabolism, embryo quality, growth, and survival (Fobert et al., 2023). In certain reef fishes, such as clownfish (Amphiprion ocellaris) and sergeant majors (Abudefduf saxatilis), the timing of embryo hatching is linked to specific intensities of light within the natural photoperiod. Constant illumination by ALAN eliminates photoperiod cues necessary for hatching, thus inhibiting successful hatching in these species (Robertson et al., 1990; Fobert et al., 2019; Fobert et al., 2023). These negative effects demonstrate the potential disruption of the circadian rhythms of various oceanic organisms in response to ALAN exposure, representing yet another conservation concern for overall biodiversity in illuminated areas.
Lessons learned from the laboratory
The circadian clock mechanism and the effects of ALAN on circadian biology have been well-studied in laboratory settings. Exposure to ALAN can result in widespread disruption of the circadian clock mechanism, changes in behavior, irregular sleep and activity patterns, reproductive dysfunction, immune regulation issues, and metabolic dysfunction (Morris et al., 2020; Fobert et al., 2021; Hillyer et al., 2021). Although limited in number, these studies have been instrumental in beginning to elucidate the effects of ALAN on circadian biology, physiology, and behavior of aquatic species.
Circadian clock genes have been identified in several marine invertebrate species including the marine annelid Platynereis dumerilii, the Pacific oyster (Crassostrea gigas), and a few Xenacoelomorphan and platyhelminth marine worms (Zantke et al., 2013; Stanton, 2015; Stanton et al., 2022; Botté et al., 2023). Laboratory experiments have demonstrated the negative effects of ALAN exposure on these species. For example, changes in activity patterns have been observed after switching P. dumerilii from a diurnal photoperiod to continuous light (Zantke et al., 2013). Similarly, the Pacific oyster demonstrates a comparable pattern of disruption in circadian clock gene expression and increase in valve activity when cultured under ALAN conditions (Botté et al., 2023).
Laboratory studies examining the circadian biology of fish have also provided useful insights on the effects of ALAN in ex situ populations. This has allowed extensive studies of fish light reception and circadian clock entrainment including: studies of eyes, photoreceptors, pineal gland, brain receptivity to light and their downstream effects on behavior, physiological, and cellular processes (Whitmore et al., 2000; Doyle and Menaker, 2007; Tamai et al., 2012; Foulkes et al., 2016). In the zebrafish (Danio rerio), ALAN exposure resulted in arrhythmic melatonin synthesis and an increase in inflammatory cytokines (Khan et al., 2018). In Japanese medaka (Oryzias latipes), zebrafish, and clownfish, ALAN exposure inhibited displays of dominance resulting in fish becoming subordinate and leading to a decline in reproductive success where males under constant light produced half the number of offspring that males maintained under diel lighting conditions produced (Tamai et al., 2004; Fobert et al., 2019; Frøland Steindal and Whitmore, 2019; Fobert et al., 2021; Closs et al., 2023). ALAN exposure also increased the interval between spawning events, resulting in smaller eggs and a reduction in clutch size (Tamai et al., 2004; Fobert et al., 2019; Frøland Steindal and Whitmore, 2019; Fobert et al., 2021).
These examples demonstrate that behavioral and physiological responses are regulated in a circadian manner by the circadian clock mechanism and disruptions to these natural rhythms have significant downstream effects. This emphasizes the need for additional research to understand the effects of ALAN and the development of mitigation strategies to minimize these effects on the aquatic ecosystem. Furthermore, these findings can be used for the improvement of artificial conditions to recapitulate the natural environment for animals under managed care.
Perspectives on the use of ALAN
While there are some benefits to using ALAN, the stark reality is that there is insufficient data to understand how detrimental the use of ALAN can be to species in the aquatic environment. There has been limited research on the effects of ALAN on the potential disruption of the circadian biology of marine animals. The duration of using intermittent ALAN, in the case of commercial fisheries, likely will not have as pronounced an effect on the circadian clock mechanism. With this, daylight will likely re-entrain circadian rhythms and the animal may not encounter a commercial fishing vessel again for some time. Therefore, this represents a situation in which the potential negative effects of ALAN would be minimal. However, the disruption of the circadian clock is likely in animals maintained in aquaculture pens as they would not be able to escape constant ALAN exposure. This is one area of opportunity for additional research to better understand the effects of circadian disruption and increase overall welfare in these animals.
Data from aquatic invertebrates, fish, and marine reptiles have begun to illuminate the consequences of ALAN in managed settings. While laboratory studies have been important in understanding circadian biology and how perturbations alter aspects of their circadian clock, physiology, and behavior, there are additional challenges. Given that the laboratory setting is highly controlled, it is possible that the results of a laboratory experiment are an artifact of the controlled conditions and are not able to be recapitulated in a natural setting. Comparative studies are needed to better understand the effects of ALAN-associated circadian disruption on behavior and physiology.
Apart from sea turtles, there is a lack of studies examining the effects of ALAN on marine megafauna, including marine mammals and elasmobranchs. Effects of ALAN exposure on the circadian clock mechanism and melatonin biosynthesis in marine megafauna represents another area where research efforts should be directed. It is likely that there is variation in circadian regulation among marine megafauna. For instance, vertebrate melatonin produced by the pineal gland appears to be a synapomorphy amongst most vertebrates (Falcón et al., 2009; Tan et al., 2010). The pineal gland has been identified in several aquatic species including fish, sharks, and pinnipeds (Little and Bryden, 1990; Boyd, 1991; Frøland Steindal and Whitmore, 2019; Carroll and Harvey-Carroll, 2023). However, it appears to be vestigial or lost along with the associated melatonin synthesis and receptor genes that have been either inactivated through a mutation or lost in the cetacean and sirenian lineages (Ralph et al., 1985; Huelsmann et al., 2019; Lopes-Marques et al., 2019). Taken together, variation in melatonin regulation should be expected and is likely lineage specific.
Understanding circadian disruption in response to adverse exposure to ALAN is also important for animals under managed care. Facilities often cater to the needs of guests by extending hours beyond the subjective day and leaving work lights on as part of emergency lighting systems (Rose et al., 2017). It is currently unknown if these lights are detrimental and if altering the wavelength could improve aquatic animal welfare. The adjustment of lighting regimens based on research for a given species or groups of species should be considered. The development of LED-based aquarium lighting systems has been beneficial in aquarium settings and has solved problems with light penetrating the water column in deep aquarium environments (Soni and Devendra, 2005; Cheng et al., 2019). Studies are needed to understand the basic circadian biology and the effects of circadian perturbations on behavior and physiology in managed animals. It is possible that disruption of the circadian clock mechanism can impact marine animal health and delay healing in sick and injured megafauna in managed care.
Future directions
While our knowledge of circadian biology and its integration with different physiological systems has expanded over the last twenty years, there remains a significant knowledge gap in regards to aquatic species. This reflects a critical need to gain a foundational understanding of the natural circadian biology of different aquatic species, in particular, marine megafauna, in order to sufficiently understand the potential negative effects of ALAN exposure on different aquatic species. Given that many cell types have an endogenous circadian clock, utilizing samples that are minimally invasive, such as skin/oral mucosa, hair follicles, and blood, may be viable options for assessing changes to an animal’s circadian rhythm. Due to the logistical constraints of sampling and/or re-sampling free-ranging animals, adaptations for the laboratory setting, such as cell cultures and CRISPR technologies, can prove to be powerful tools (Gabriel et al., 2021).
Animal activity and sleep patterns have also been measured to understand how patterns of activity change with different perturbations in light exposure. (Alves-Simoes et al., 2016; Wang et al., 2020). This type of data is not easily obtainable in many aquatic species; however, data logger technology implanted into animals has significantly advanced research efforts to record data such as body temperature, heart rate, activity, telemetry, and even depth patterns. This technology has been successfully used in some aquatic species including fish, sharks, pinnipeds, and cetaceans (Lin et al., 2013; Krause et al., 2016; Chaise et al., 2017; Elisio et al., 2019; Piot et al., 2023; Morgenroth et al., 2024). This technology could be translated for use in animals both in wild and managed settings to observe changes in circadian biology in response to ALAN exposure, feeding, water temperature, and/or water quality. These techniques can also be utilized to test the efficacy of different mitigation strategies for improving animal health and welfare.
While these approaches will have multiple applications, a central focus on understanding how light regulates the circadian biology of aquatic species is needed. Knowledge of the effects abnormal lighting conditions, including the use of ALAN, have on an animal’s circadian biology is paramount to developing improved mitigation practices. Implementation of novel techniques will allow researchers to gather a vast amount of data that can be directly applied to the development of mitigation strategies to minimize the adverse effects on an animal’s circadian biology. This provides an avenue for applying research that will not only benefit species in managed care, but also those in natural settings.
Data availability statement
The original contributions presented in the study are included in the article/supplementary material. Further inquiries can be directed to the corresponding author.
Author contributions
DS: Conceptualization, Writing – original draft, Writing – review & editing. JC: Funding acquisition, Writing – review & editing.
Funding
The author(s) declare that no financial support was received for the research, authorship, and/or publication of this article.
Conflict of interest
The authors declare that the research was conducted in the absence of any commercial or financial relationships that could be construed as a potential conflict of interest.
Publisher’s note
All claims expressed in this article are solely those of the authors and do not necessarily represent those of their affiliated organizations, or those of the publisher, the editors and the reviewers. Any product that may be evaluated in this article, or claim that may be made by its manufacturer, is not guaranteed or endorsed by the publisher.
References
Alves-Simoes M., Coleman G., Canal M. M. (2016). Effects of type of light on mouse circadian behaviour and stress levels. Lab. Anim. 50, 21–29. doi: 10.1177/0023677215588052
Arakawa H., Choi S., Arimoto T., Nakamura Y. (1998). Relationship between underwater irradiance and distribution of Japanese common squid under fishing lights of a squid jigging boat. Fish. Sci. 64, 553–557. doi: 10.2331/fishsci.64.553
Ayalon I., De Barros Marangoni L. F., Benichou J. I. C., Avisar D., Levy O. (2019). Red Sea corals under Artificial Light Pollution at Night (ALAN) undergo oxidative stress and photosynthetic impairment. Glob. Change Biol. 25, 4194–4207. doi: 10.1111/gcb.14795
Ayalon I., Rosenberg Y., Benichou J. I. C., Campos C. L. D., Sayco S. L. G., Nada M. A. L., et al. (2021). Coral gametogenesis collapse under artificial light pollution. Curr. Biol. 31, 413–419.e3. doi: 10.1016/j.cub.2020.10.039
Botté A., Payton L., Tran D. (2023). Artificial light at night at environmental intensities disrupts daily rhythm of the oyster Crassostrea gigas. Mar. pollut. Bull. 191, 114850. doi: 10.1016/j.marpolbul.2023.114850
Boyd I. L. (1991). Environmental and physiological factors controlling the reproductive cycles of pinnipeds. Can. J. Zool. 69, 1135–1148. doi: 10.1139/z91-162
Brüning A., Hölker F., Franke S., Preuer T., Kloas W. (2015). Spotlight on fish: Light pollution affects circadian rhythms of European perch but does not cause stress. Sci. Total Environ. 511, 516–522. doi: 10.1016/j.scitotenv.2014.12.094
Carroll D., Harvey-Carroll J. (2023). The influence of light on elasmobranch behavior and physiology: a review. Front. Mar. Sci. 10. doi: 10.3389/fmars.2023.1225067
Chaise L. L., Paterson W., Laske T. G., Gallon S. L., McCafferty D. J., Théry M., et al. (2017). Implantation of subcutaneous heart rate data loggers in southern elephant seals (Mirounga leonina). Polar Biol. 40, 2307–2312. doi: 10.1007/s00300-017-2144-x
Cheng C.-M., Cheng Y.-R., Lin H.-Y., Sun W.-T., Pan C.-H., Ding D.-S. (2022). Effects of LED light illumination on the growth, digestive enzymes, and photoacclimation of goniopora columna in captivity. Animals 12, 306. doi: 10.3390/ani12030306
Cheng J.-H., Lin H.-H., Yen C.-C. (2019). “The spotlight effects of LED lighting design for deep aquarium tanks,” in 2019 IEEE 2nd International Conference on Knowledge Innovation and Invention (ICKII), Seoul, Korea (South. 358–361 (IEEE). doi: 10.1109/ICKII46306.2019.9042603
Closs L. E., Royan M. R., Sayyari A., Mayer I., Weltzien F.-A., Baker D. M., et al. (2023). Artificial light at night disrupts male dominance relationships and reproductive success in a model fish species. Sci. Total Environ. 900, 166406. doi: 10.1016/j.scitotenv.2023.166406
Davies T. W., Duffy J. P., Bennie J., Gaston K. J. (2014). The nature, extent, and ecological implications of marine light pollution. Front. Ecol. Environ. 12, 347–355. doi: 10.1890/130281
Davies T. W., Levy O., Tidau S., De Barros Marangoni L. F., Wiedenmann J., D’Angelo C., et al. (2023). Global disruption of coral broadcast spawning associated with artificial light at night. Nat. Commun. 14, 2511. doi: 10.1038/s41467-023-38070-y
Delupis G. D. D., Rotondo V. (1988). Phototaxis in aquatic invertebrates: possible use in ecotoxicity tests’. Ecotoxicol. Environ. Saf. 16, 189–193. doi: 10.1016/0147-6513(88)90049-8
Doyle S., Menaker M. (2007). Circadian photoreception in vertebrates. Cold Spring Harb. Symp. Quant. Biol. 72, 499–508. doi: 10.1101/sqb.2007.72.003
Duarte C., Quintanilla-Ahumada D., Anguita C., Manríquez P. H., Widdicombe S., Pulgar J., et al. (2019). Artificial light pollution at night (ALAN) disrupts the distribution and circadian rhythm of a sandy beach isopod. Environ. pollut. 248, 565–573. doi: 10.1016/j.envpol.2019.02.037
Dubruille R., Emery P. (2008). A plastic clock: how circadian rhythms respond to environmental cues in drosophila. Mol. Neurobiol. 38, 129–145. doi: 10.1007/s12035-008-8035-y
Elisio M., Awruch C. A., Massa A. M., Macchi G. J., Somoza G. M. (2019). Effects of temperature on the reproductive physiology of female elasmobranchs: The case of the narrownose smooth-hound shark (Mustelus schmitti). Gen. Comp. Endocrinol. 284, 113242. doi: 10.1016/j.ygcen.2019.113242
Falcón J., Besseau L., Fuentès M., Sauzet S., Magnanou E., Boeuf G. (2009). Structural and functional evolution of the pineal melatonin system in vertebrates. Ann. N. Y. Acad. Sci. 1163, 101–111. doi: 10.1111/j.1749-6632.2009.04435.x
Fobert E. K., Burke Da Silva K., Swearer S. E. (2019). Artificial light at night causes reproductive failure in clownfish. Biol. Lett. 15, 20190272. doi: 10.1098/rsbl.2019.0272
Fobert E. K., Miller C. R., Swearer S. E., Mayer-Pinto M. (2023). The impacts of artificial light at night on the ecology of temperate and tropical reefs. Philos. Trans. R. Soc B Biol. Sci. 378, 20220362. doi: 10.1098/rstb.2022.0362
Fobert E. K., Schubert K. P., Burke Da Silva K. (2021). The influence of spectral composition of artificial light at night on clownfish reproductive success. J. Exp. Mar. Biol. Ecol. 540, 151559. doi: 10.1016/j.jembe.2021.151559
Foulkes N. S., Whitmore D., Vallone D., Bertolucci C. (2016). “Studying the evolution of the vertebrate circadian clock,” in Adv. Genet. (Elsevier), 1–30. doi: 10.1016/bs.adgen.2016.05.002
Frøland Steindal I., Whitmore D. (2019). Circadian Clocks in Fish—What Have We Learned so far? Biology 8, 17. doi: 10.3390/biology8010017
Gabriel C. H., Del Olmo M., Zehtabian A., Jäger M., Reischl S., Van Dijk H., et al. (2021). Live-cell imaging of circadian clock protein dynamics in CRISPR-generated knock-in cells. Nat. Commun. 12, 3796. doi: 10.1038/s41467-021-24086-9
Grubisic M., Haim A., Bhusal P., Dominoni D. M., Gabriel K. M. A., Jechow A., et al. (2019). Light pollution, circadian photoreception, and melatonin in vertebrates. Sustainability 11, 6400. doi: 10.3390/su11226400
Hannah R. W., Lomeli M. J. M., Jones S. A. (2015). Tests of artificial light for bycatch reduction in an ocean shrimp (Pandalus Jordani) trawl: Strong but opposite effects at the footrope and near the bycatch reduction device. Fish. Res. 170, 60–67. doi: 10.1016/j.fishres.2015.05.010
Hardeland R., Poeggeler B. (2003). Non-vertebrate melatonin. J. Pineal Res. 34, 233–241. doi: 10.1034/j.1600-079X.2003.00040.x
Hillyer K. E., Beale D. J., Shima J. S. (2021). Artificial light at night interacts with predatory threat to alter reef fish metabolite profiles. Sci. Total Environ. 769, 144482. doi: 10.1016/j.scitotenv.2020.144482
Hölker F., Moss T., Griefahn B., Kloas W., Voigt C. C., Henckel D., et al. (2010). The dark side of light: A transdisciplinary research agenda for light pollution policy. Ecol. Soc. 15, art13. doi: 10.5751/ES-03685-150413
Huelsmann M., Hecker N., Springer M. S., Gatesy J., Sharma V., Hiller M. (2019). Genes lost during the transition from land to water in cetaceans highlight genomic changes associated with aquatic adaptations. Sci. Adv. 5, eaaw6671. doi: 10.1126/sciadv.aaw6671
Jékely G., Colombelli J., Hausen H., Guy K., Stelzer E., Nédélec F., et al. (2008). Mechanism of phototaxis in marine zooplankton. Nature 456, 395–399. doi: 10.1038/nature07590
Jones T. M., Durrant J., Michaelides E. B., Green M. P. (2015). Melatonin: a possible link between the presence of artificial light at night and reductions in biological fitness. Philos. Trans. R. Soc B Biol. Sci. 370, 20140122. doi: 10.1098/rstb.2014.0122
Kopperud K., Grace M. (2017). Circadian rhythms of retinomotor movement in a marine megapredator, the atlantic tarpon, megalops atlanticus. Int. J. Mol. Sci. 18, 2068. doi: 10.3390/ijms18102068
Krause D. J., Goebel M. E., Marshall G. J., Abernathy K. (2016). Summer diving and haul-out behavior of leopard seals (Hydrurga leptonyx) near mesopredator breeding colonies at Livingston Island, Antarctic Peninsula. Mar. Mammal Sci. 32, 839–867. doi: 10.1111/mms.12309
Levy O., Fernandes De Barros Marangoni L., Benichou J. I. C., Rottier C., Béraud E., Grover R., et al. (2020). Artificial light at night (ALAN) alters the physiology and biochemistry of symbiotic reef building corals. Environ. pollut. 266, 114987. doi: 10.1016/j.envpol.2020.114987
Lin T.-H., Akamatsu T., Chou L.-S. (2013). Tidal influences on the habitat use of Indo-Pacific humpback dolphins in an estuary. Mar. Biol. 160, 1353–1363. doi: 10.1007/s00227-013-2187-7
Little G. J., Bryden M. M. (1990). The pineal gland in newborn southern elephant seals, Mirounga leonina. J. Pineal Res. 9, 139–148. doi: 10.1111/j.1600-079X.1990.tb00702.x
Lopes-Marques M., Ruivo R., Alves L. Q., Sousa N., MaChado A. M., Castro L. F. C. (2019). The singularity of cetacea behavior parallels the complete inactivation of melatonin gene modules. Genes 10, 121. doi: 10.3390/genes10020121
Marchesan M., Spoto M., Verginella L., Ferrero E. A. (2005). Behavioural effects of artificial light on fish species of commercial interest. Fish. Res. 73, 171–185. doi: 10.1016/j.fishres.2004.12.009
McConnell A., Routledge R., Connors B. (2010). Effect of artificial light on marine invertebrate and fish abundance in an area of salmon farming. Mar. Ecol. Prog. Ser. 419, 147–156. doi: 10.3354/meps08822
McLeod L. E., Costello M. J. (2017). Light traps for sampling marine biodiversity. Helgol. Mar. Res. 71, 2. doi: 10.1186/s10152-017-0483-1
Mendes C., Fernandes C., Moreira A., Chambel J., Maranhao P., Leandro S. (2017). Effect of LEDs light spectrum on success of fragmentation and growth of leather coral Sarcophyton spp. Int. J. Aquac. 7 (8). doi: 10.5376/ija.2017.07.0008
Morgenroth D., Kvaestad B., Økland F., Finstad B., Olsen R.-E., Svendsen E., et al. (2024). Under the sea: How can we use heart rate and accelerometers to remotely assess fish welfare in salmon aquaculture? Aquaculture 579, 740144. doi: 10.1016/j.aquaculture.2023.740144
Morris A. R., Stanton D. L., Roman D., Liu A. C. (2020). Systems level understanding of circadian integration with cell physiology. J. Mol. Biol. 432, 3547–3564. doi: 10.1016/j.jmb.2020.02.002
Muñoz J. L. P., López Patiño M. A., Hermosilla C., Conde-Sieira M., Soengas J. L., Rocha F., et al. (2011). Melatonin in octopus (Octopus vulgaris): tissue distribution, daily changes and relation with serotonin and its acid metabolite. J. Comp. Physiol. A 197, 789–797. doi: 10.1007/s00359-011-0641-x
Nguyen K. Q., Winger P. D. (2019). Artificial light in commercial industrialized fishing applications: A review. Rev. Fish. Sci. Aquac. 27, 106–126. doi: 10.1080/23308249.2018.1496065
Peres R., Reitzel A. M., Passamaneck Y., Afeche S. C., Cipolla-Neto J., Marques A. C., et al. (2014). Developmental and light-entrained expression of melatonin and its relationship to the circadian clock in the sea anemone Nematostella vectensis. EvoDevo 5, 26. doi: 10.1186/2041-9139-5-26
Piot E., Picard B., Badaut J., Gilbert C., Guinet C. (2023). Diving behaviour of southern elephant seals: new models of behavioural and ecophysiological adjustments of oxygen store management. J. Exp. Biol. 226, jeb245157. doi: 10.1242/jeb.245157
Pulgar J., Zeballos D., Vargas J., Aldana M., Manriquez P. H., Manriquez K., et al. (2019). Endogenous cycles, activity patterns and energy expenditure of an intertidal fish is modified by artificial light pollution at night (ALAN). Environ. Pollut. 244, 361–366. doi: 10.1016/j.envpol.2018.10.063
Ralph C. L., Young S., Gettinger R., O’Shea T. J. (1985). Does the manatee have a pineal body? Acta Zool. 66, 55–60. doi: 10.1111/j.1463-6395.1985.tb00647.x
Robertson D. R., Petersen C. W., Brawn J. D. (1990). Lunar reproductive cycles of benthic-brooding reef fishes: reflections of larval biology or adult biology? Ecol. Monogr. 60, 311–329. doi: 10.2307/1943060
Roopin M., Levy O. (2012). Melatonin distribution reveals clues to its biological significance in basal metazoans. PloS One 7, e52266. doi: 10.1371/journal.pone.0052266
Rose N. A., Hancock Snusz G., Brown D. M., Parsons E. C. M. (2017). Improving captive marine mammal welfare in the United States: science-based recommendations for improved regulatory requirements for captive marine mammal care. J. Int. Wildl. Law Policy 20, 38–72. doi: 10.1080/13880292.2017.1309858
Rosenberg Y., Doniger T., Levy O. (2019). Sustainability of coral reefs are affected by ecological light pollution in the Gulf of Aqaba/Eilat. Commun. Biol. 2, 289. doi: 10.1038/s42003-019-0548-6
Sainath S. B., Swetha C., Reddy P. S. (2013). What do we (Need to) know about the melatonin in crustaceans? J. Exp. Zool. Part Ecol. Genet. Physiol. 319, 365–377. doi: 10.1002/jez.1800
Schlacher T. A., Stark J., Fischer A. B. P. (2007). Evaluation of artificial light regimes and substrate types for aquaria propagation of the staghorn coral Acropora solitaryensis. Aquaculture 269, 278–289. doi: 10.1016/j.aquaculture.2007.04.085
Siddik A. A., Satheesh S. (2021). Interactive effects of light and substrate colour on the recruitment of marine invertebrates on artificial materials. Community Ecol. 22, 69–78. doi: 10.1007/s42974-020-00037-0
Soni N. B., Devendra P. (2005). The Transition to Led Illumination: A Case Study on Energy Conservation J Theor. Appl. Inf. Technol. 4 (11).
Stanton D. (2015). Time Enough At Last: Identification and Analysis of Core-Clock Proteins and the Evolution of ARNT and PERIOD in the Lower Bilateria. Available at: https://digitalcommons.winthrop.edu/graduatetheses/12/.
Stanton D., Justin H. S., Reitzel A. M. (2022). Step in time: conservation of circadian clock genes in animal evolution. Integr. Comp. Biol. 62 (6), pp.1503–1518. doi: 10.1093/icb/icac140
Szekeres P., Wilson A., Haak C., Danylchuk A., Brownscombe J., Elvidge C., et al. (2017). Does coastal light pollution alter the nocturnal behavior and blood physiology of juvenile bonefish (Albula vulpes)? Bull. Mar. Sci. 93, 491–505. doi: 10.5343/bms.2016.1061
Takahashi J. S. (2017). Transcriptional architecture of the mammalian circadian clock. Nat. Rev. Genet. 18, 164–179. doi: 10.1038/nrg.2016.150
Tamai T. K., Vardhanabhuti V., Foulkes N. S., Whitmore D. (2004). Early embryonic light detection improves survival. Curr. Biol. 14, 446. doi: 10.1016/j.cub.2004.02.040
Tamai T. K., Young L. C., Cox C. A., Whitmore D. (2012). Light acts on the zebrafish circadian clock to suppress rhythmic mitosis and cell proliferation. J. Biol. Rhythms 27, 226–236. doi: 10.1177/0748730412440861
Tamir R., Eyal G., Cohen I., Loya Y. (2020). Effects of light pollution on the early life stages of the most abundant Northern Red Sea coral. Microorganisms 8, 193. doi: 10.3390/microorganisms8020193
Tan D., Hardeland R., Manchester L. C., Paredes S. D., Korkmaz A., Sainz R. M., et al. (2010). The changing biological roles of melatonin during evolution: from an antioxidant to signals of darkness, sexual selection and fitness. Biol. Rev. 85, 607–623. doi: 10.1111/j.1469-185X.2009.00118.x
Tidau S., Smyth T., McKee D., Wiedenmann J., D’Angelo C., Wilcockson D., et al. (2021). Marine artificial light at night: An empirical and technical guide. Methods Ecol. Evol. 12, 1588–1601. doi: 10.1111/2041-210X.13653
Tosches M. A., Bucher D., Vopalensky P., Arendt D. (2014). Melatonin signaling controls circadian swimming behavior in marine Zooplankton. Cell 159, 46–57. doi: 10.1016/j.cell.2014.07.042
Tran D., Perrigault M., Ciret P., Payton L. (2020). Bivalve mollusc circadian clock genes can run at tidal frequency. Proc. R. Soc B Biol. Sci. 287, 20192440. doi: 10.1098/rspb.2019.2440
Virgili M., Vasapollo C., Lucchetti A. (2018). Can ultraviolet illumination reduce sea turtle bycatch in Mediterranean set net fisheries? Fish. Res. 199, 1–7. doi: 10.1016/j.fishres.2017.11.012
Volpato G. L., Bovi T. S., De Freitas R. H. A., Da Silva D. F., Delicio H. C., Giaquinto P. C., et al. (2013). Red light stimulates feeding motivation in fish but does not improve growth. PloS One 8, e59134. doi: 10.1371/journal.pone.0059134
Wang J., Barkan J., Fisler S., Godinez-Reyes C., Swimmer Y. (2013). Developing ultraviolet illumination of gillnets as a method to reduce sea turtle bycatch. Biol. Lett. 9, 20130383. doi: 10.1098/rsbl.2013.0383
Wang C., Guerriero L. E., Huffman D. M., Ajwad A. A., Brooks T. C., Sunderam S., et al. (2020). A comparative study of sleep and diurnal patterns in house mouse (Mus musculus) and Spiny mouse (Acomys cahirinus). Sci. Rep. 10, 10944. doi: 10.1038/s41598-020-67859-w
Whitmore D., Foulkes N. S., Sassone-Corsi P. (2000). Light acts directly on organs and cells in culture to set the vertebrate circadian clock. Nature 404, 87–91. doi: 10.1038/35003589
Zantke J., Ishikawa-Fujiwara T., Arboleda E., Lohs C., Schipany K., Hallay N., et al. (2013). Circadian and circalunar clock interactions in a marine annelid. Cell Rep. 5, 99–113. doi: 10.1016/j.celrep.2013.08.031
Keywords: circadian biology, marine animals, artificial light, light pollution, animal welfare
Citation: Stanton DL and Cowart JR (2024) The effects of artificial light at night (ALAN) on the circadian biology of marine animals. Front. Mar. Sci. 11:1372889. doi: 10.3389/fmars.2024.1372889
Received: 18 January 2024; Accepted: 24 January 2024;
Published: 07 February 2024.
Edited by:
Stelios Katsanevakis, University of the Aegean, GreeceReviewed by:
Timothy James Smyth, Plymouth Marine Laboratory, United KingdomCopyright © 2024 Stanton and Cowart. This is an open-access article distributed under the terms of the Creative Commons Attribution License (CC BY). The use, distribution or reproduction in other forums is permitted, provided the original author(s) and the copyright owner(s) are credited and that the original publication in this journal is cited, in accordance with accepted academic practice. No use, distribution or reproduction is permitted which does not comply with these terms.
*Correspondence: Daniel L. Stanton, stantond2@ufl.edu