- Department of Marine and Environmental Science, Halmos College of Arts and Sciences, Nova Southeastern University, Dania, FL, United States
The recent widespread mortality and tissue loss in Florida from stony coral tissue loss disease (SCTLD) has propelled the need for assisted reproduction to restore reefs, especially for the ESA listed species Orbicella faveolata. In situ gamete collection can be challenging due to the weather and resources (boats and divers) required during the expected spawning window. In the northern portion of the Florida coral reef tract, coral spawn collection has been even more difficult due to historical inconsistency in annual spawning times and the potential for “zombie” corals, i.e. large but reproductively senescent individuals. Therefore, we examined the current reproductive potential of seven large (>2 m diameter) O. faveolata colonies from this region, quantified their fecundity, and estimated the spawning timeframe using histology. Additionally, we explored whether previous SCTLD lesion amoxycillin treatments affected reproductive metrics. Understanding the reproductive capacity and spawning timing of these large corals, given their history of disease and disease treatment, is critical to evaluate potential impacts of SCTLD treatments and the success of assisted reproduction efforts. The histological analysis coupled with in-water observations indicated a probable split-spawn in these individuals in 2020, although the dates of spawning may not be consistent with predictions for the wider Caribbean or with other colonies in Miami and the Florida Keys. All seven large O. faveolata colonies were found to contain abundant oocytes, with no obvious impact of SCTLD treatments on gamete development or fecundity.
1 Introduction
Florida’s Coral Reef is currently experiencing a multi-year disease-related mortality event that has resulted in widespread mortality and tissue loss in multiple coral species (Precht et al., 2016). Approximately 21 species of coral, including both Endangered Species Act-listed and primary reef-building species, have exhibited lesions and tissue loss which often result in whole colony mortality (Precht et al., 2016; Walton et al., 2018; Rippe et al., 2019; Muller et al., 2020; Hayes et al., 2022). First observed in late 2014, stony coral tissue loss disease (SCTLD) has since spread to the northernmost extent of the Florida Reef Tract, southwest to the Dry Tortugas, and continues to spread throughout the Caribbean (Precht et al., 2016; Alvarez-Filip et al., 2019; Weil et al., 2019).
To save existing resources, part of the SCTLD response involves applying topical treatments of CoralCure mixed with antibiotics along active disease lesion progression (Neely et al., 2021; Shilling et al., 2021; Walker et al., 2021). The antibiotic paste is approximately 80-90% effective at stopping lesion progression and has kept thousands of corals alive. However, the potential effects of these treatments on the coral and/or the surrounding environment are unknown. Wide-spread overuse of antibiotics has previously led to unintended environmental consequences, including attribution of decreased reproductive capacity to antibiotics exposure in other vertebrates and invertebrates (Flaherty and Dodson, 2005; Yan et al., 2016; Kraemer et al., 2019). As continued application of antibiotic treatments is needed in the environment to prevent the complete loss of coral colonies to SCTLD, it is important to understand the possible unintended effects of these disease interventions.
As one of the main reef builders in the Caribbean, the endangered Orbicella faveolata is a species of particular importance for reef restoration. The spread of SCTLD has resulted in a substantially lower density of corals on the reef, contributing to a prezygotic barrier (reduced chance of sperm and eggs from different colonies to meet) which can ultimately reduce recruitment of corals back to the reefs (Quiroz et al., 2023). Natural recruitment of these hermaphrodite broadcast spawners is not keeping pace with losses on many reefs (van Woesik et al., 2014); therefore, they are a focal species for assisted reproduction where their gametes are collected, fertilized, reared in captivity, and outplanted to restore their populations. Large corals have a higher reproductive potential (Szmant, 1986), increasing their ability to naturally replenish reef communities and increasing the efficiency of gamete collection in the field to support assisted reproduction efforts. As a proxy for age, a boulder coral’s size is an indicator of resistance to previous perturbations, suggesting higher fitness, and their structure provides essential ecological functions such as habitat for many valuable species and shoreline protection. Prioritizing the largest, oldest colonies of Caribbean reef-building species for restoration efforts is imperative to preserve ecological function and genetic diversity of the most resilient corals.
Southeast Florida is home to several large colonies of O. faveolata which have been subject to multiple SCTLD intervention treatments over time and for which previous attempts at in-situ gamete collection from these colonies have been unsuccessful, with no spawning observed during predicted spawning windows during which other colonies of this species in Miami and the Florida Keys have been observed to spawn. This unpredictable spawning timing may be related to abiotic factors, such as ongoing temperature stress events (Fisch et al., 2019); it may be due to the fact that these colonies belong to a genetically distinct lineage from other O. faveolata found throughout the Kristin Jacobs Coral Reef Ecosystem Conservation Area (Coral ECA) and the Florida Keys (Klein et al., 2024); or it may be that they inhabit the far-Northern limit of their range which influences the otherwise predictable spawning window. Additionally, indirect impacts of disease on individual colonies currently or previously affected by SCTLD may include systemic effects resulting in reduced reproductive output, as has been described for O. faveolata affected by Yellow Band Disease (YBD) (Weil et al., 2009). The main purpose of this study was therefore to examine the reproductive status of these unique survivors, estimate their spawning timeframe, and evaluate any potential effect of the disease treatments on their reproductive potential.
2 Materials and methods
2.1 Coral samples
A 20 x 30 m site on the nearshore ridge complex in Fort Lauderdale, FL that contains 16 large, live Orbicella colonies up to 3 m in diameter at a depth of ~6 m was targeted for monitoring Orbicella spawning (Figure 1). The corals at this location were monitored monthly for 30 months and treated as needed for SCTLD (Figure 2). The proximity of these colonies to each other facilitated the complex logistics of collecting and fertilizing gametes from many presumed different genotypes in the field. In 2018 and 2019, this site was monitored for spawning activity during predicted spawning windows each September, without success.
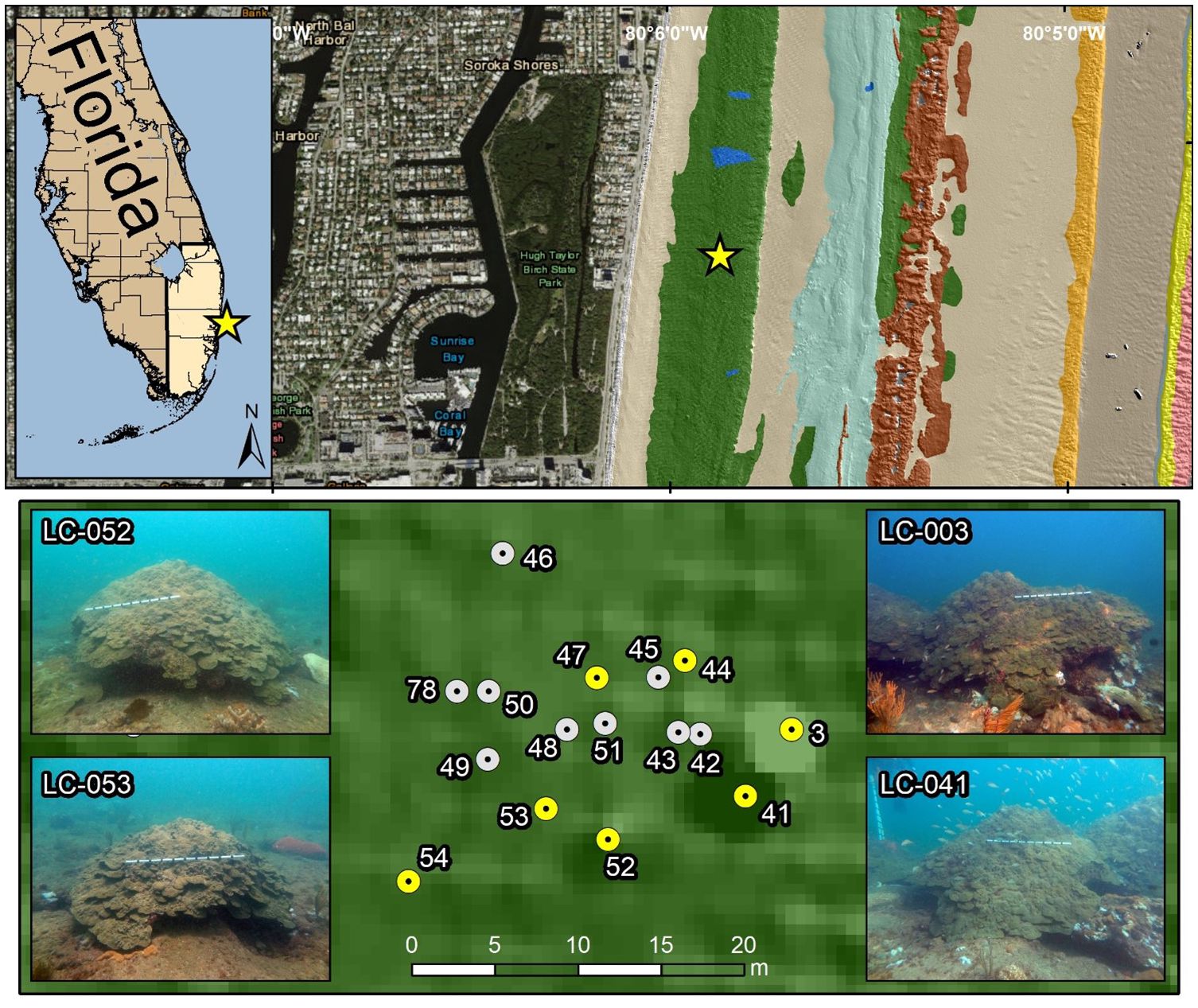
Figure 1 Site map showing the coral locations of all 16 monitored Orbicella faveolata colonies off Fort Lauderdale Beach, FL. Yellow dots indicate colonies sampled for histology. Four representative colony pictures are provided with a meter stick for scale.
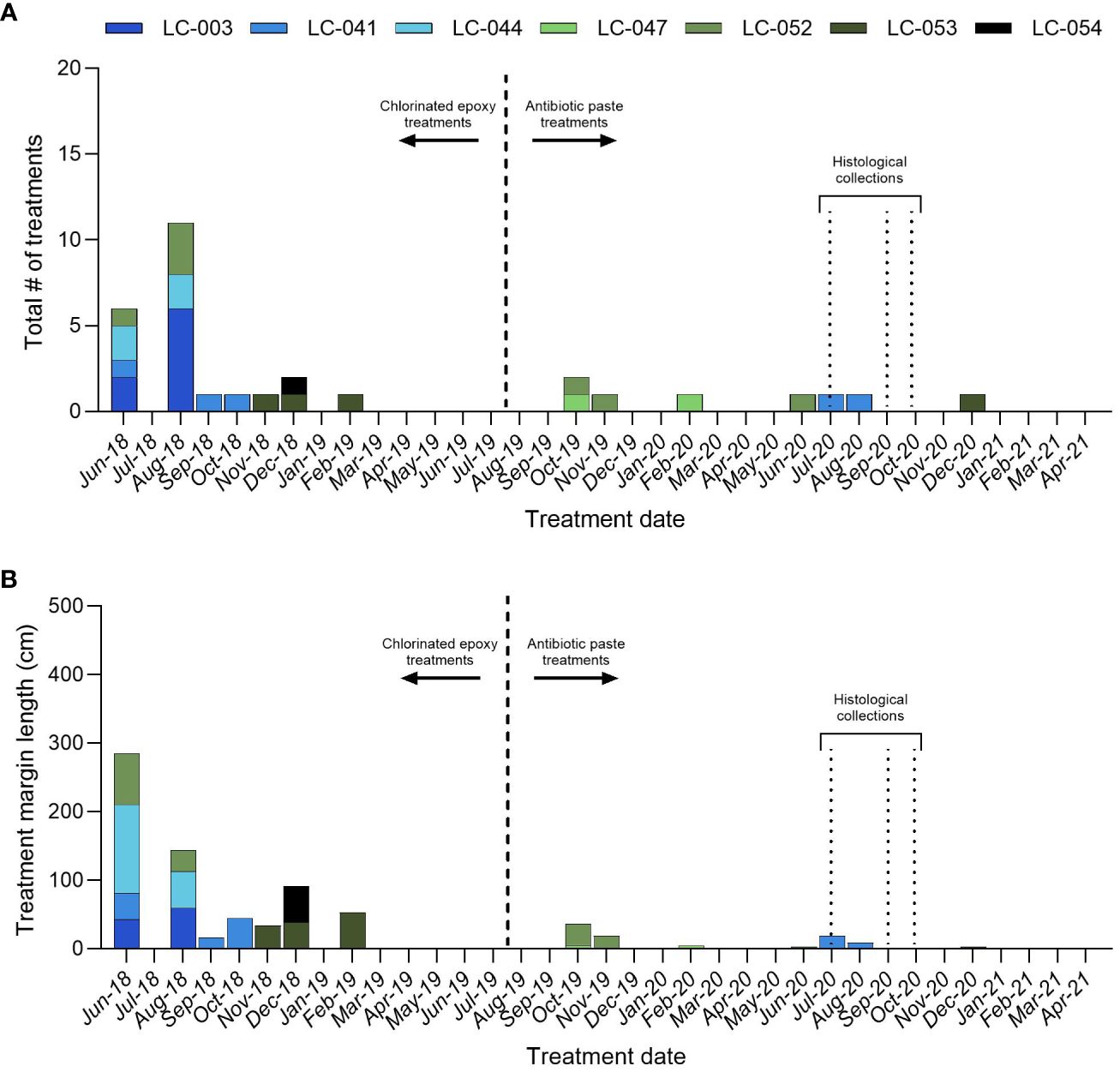
Figure 2 Orbicella faveolata. (A) The total number of disease interventions by monitoring period, and (B) the total length of diseased tissue margin treated by monitoring period for the large colonies. All treatments before August 2019 were chlorinated epoxy only, and all treatments after August 2019 were antibiotic paste only.
In 2020, seven O. faveolata colonies (LC-003, LC-041, LC-044, LC-047, LC-052, LC-053, and LC-054) were selected for histological sampling. These colonies had been monitored monthly since 2018, and all exhibited disease at some point in time. The majority of SCTLD interventions (25) were conducted prior to August 2019 using chlorinated epoxy. Beginning in August 2019, the more effective amoxycillin in CoralCure paste was used to treat all subsequent disease lesions (Walker et al., 2021). These interventions consisted of applying the antibiotic paste to the disease lesion margin and spreading so that the paste covered ~1cm of live tissue.
The colonies sampled for histology had a total of 34 disease interventions conducted between January 2018 and October 2020, totaling 840 cm in length. LC-003 had the greatest number of treatments (eight) followed by LC-052 (seven), LC-041 (five), LC-044 (four), LC-047 (four), LC-053 (three) and LC-054 (three) (Figure 2A). LC-044 had the longest total length of diseased tissue margin treated (182 cm) followed by LC-052 (159 cm), LC-041 (129 cm), LC-054 (129 cm), LC-053 (126 cm), LC-003 (103 cm) and LC-047 (17 cm) (Figure 2B). Between August 2019 and October 2020, six antibiotic treatments were conducted on three of the colonies: LC-041 (two), LC-047 (two), LC-052 (two). These totaled 73 cm in total length of diseased tissue margin treated: LC-052 (34 cm), LC-041 (28 cm), and LC-047 (10 cm) (Figure 2B). The remaining colonies were not treated with antibiotics.
In 2020, O. faveolata was predicted to have three spawning windows due to an early full moon in August. The spawning windows were: August 8-12, September 5-9, and October 5-9 (Vermeij et al., 2020) All seven colonies were sampled for histological analysis on July 9 (before predicted spawning) and September 3, 2020, and two colonies (LC-041 and LC 053) were sampled on September 18, 2020 to confirm that spawning had occurred. All colonies were cored at a central horizontal location near the apex of the colony using a 2.5 cm diamond core bit hole saw on a Nemo V2 Divers underwater drill. Cores were drilled to a depth of approximately 3 cm to ensure collection of the entire corallite. Upon removal from the colony, cores were placed in Whirl-Pak® sample bags and transported to the boat where they were placed in zinc-buffered formalin (Z-Fix). The core holes were filled with 2-part epoxy (Z Spar Splashzone) to deter herbivore predation and facilitate tissue regrowth, and monitored monthly.
2.2 Histology and estimation of fecundity
Coral samples for histological analysis were fixed in zinc-buffered formalin (Z-Fix) for at least 24 hours. Samples were then rinsed and decalcified in a 5% HCl/ethylenediamine tetraacetic acid (EDTA) seawater solution, dehydrated in a graded series of ethanols and xylene, and embedded in paraffin wax (Paraplast Plus®). Longitudinal and transverse sections (4 μm thick) were mounted on slides, cleared in xylene, and stained with modified Heidenhain’s aniline blue. Stained slides were viewed in an Olympus BX 43 light microscope at magnifications ranging from 4X to 60X and photographed with an Olympus DP21 digital camera.
Histological sections were assessed for the presence or absence of male and female gametes, the reproductive stage of gametes, the number of oocytes or ova per mesentery/number of mesenteries per polyp, and the diameter of oocytes. Fecundity per polyp was estimated as the product of the average number of mesenteries per polyp (in 5-10 polyps per sample) and the average number of oocytes or ova per mesentery in longitudinal section (in 3-5 polyps per sample). Oocyte/ova diameters were measured from 5 randomly selected polyps per coral.
The small number of large colonies at the site and lack of available control colonies (colonies with no history of disease or disease treatment) precluded a comprehensive sampling design and statistical analysis. Therefore, results are presented as a qualitative assessment of the effects of SCTLD occurrence and/or antibiotic treatments on reproductive potential.
3 Results
All colonies sampled on July 9, 2020 contained abundant oocytes/ova of Stages I-IV, with the majority at Stage III (Figures 3A, B). Early stage spermaries (mostly Stage II) were also observed (Figure 3B). Polyp fecundity (ova per polyp) was estimated for all coral samples collected in July 2020 based on the number of Stage III oocytes/ova, due to the limited samples with Stage IV oocytes collected in September 2020. This likely contributed to the high estimates of polyp fecundity for each coral, which were higher than most reported fecundity estimates for this species (Holstein et al., 2015). Numerous Stage V spermaries were also present (Figure 3D) on September 3, 2020.
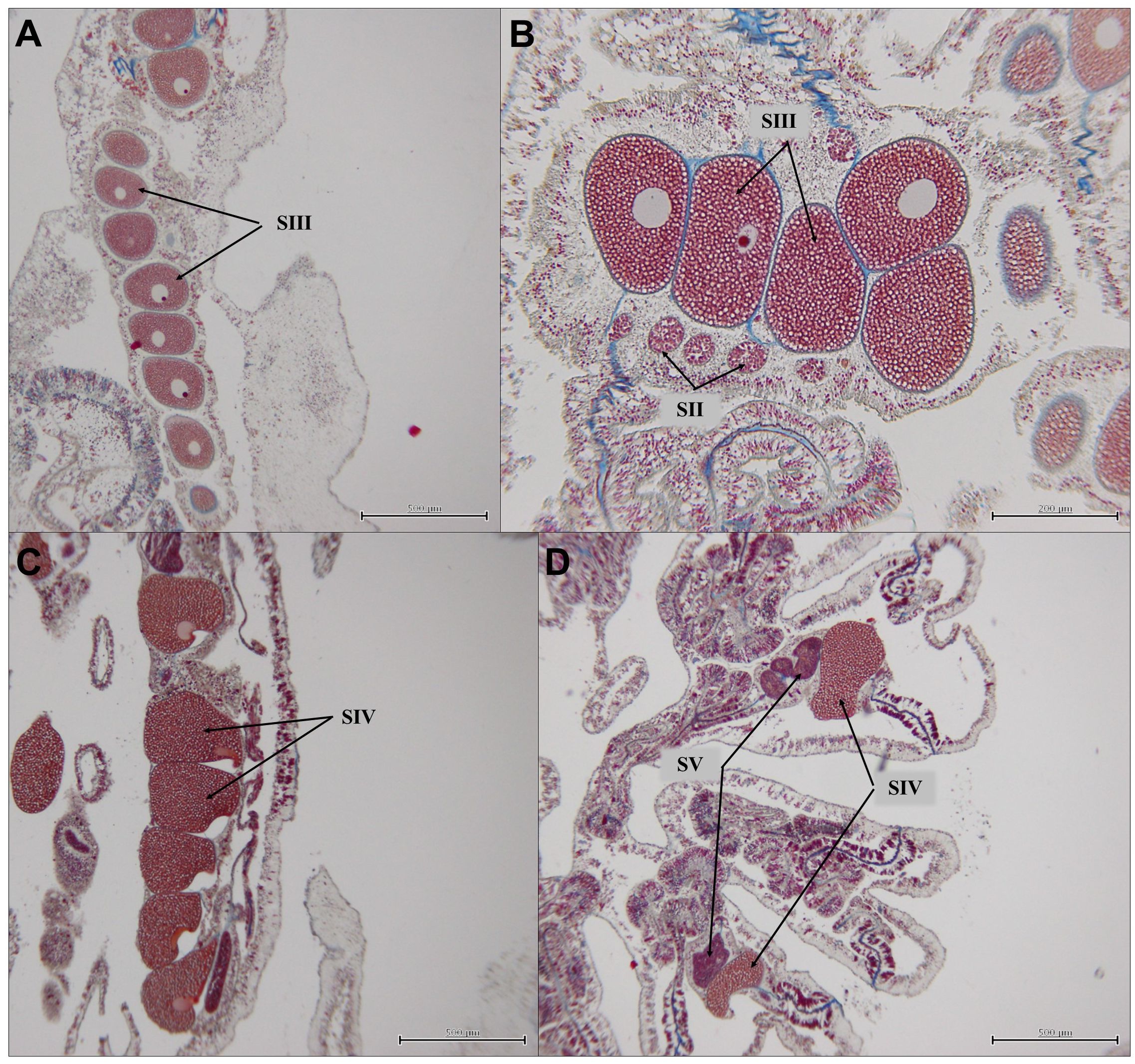
Figure 3 Orbicella faveolata. (A) Stage III oocytes/ova in mesentery, coral LC-052, sampled July 9, 2020; (B) Stage III oocytes/ova and Stage II spermaries, coral LC-044, sampled on July 9, 2020; (C) Stage IV oocytes/ova in coral LC-041, sampled on September 3, 2020; and (D) Stage IV oocytes/ova and Stage V spermaries in coral LC-041, sampled on September 3, 2020.
Overall, the mean oocyte/ova diameter increased between July 2020 and September 2020 (Figure 4A), while the mean polyp fecundity decreased over that same period (Figure 4B). Corals sampled in September 2020 had lower polyp fecundity (0.5–51.8 oocytes/polyp) compared to July 2020 (100.8–181.1 oocytes/polyp) (Figure 4B). Coral LC-003 contained the largest number of oocytes relative to the other individuals sampled in September 2020; fewer oocytes were present in September 2020 compared to July 2020, but oocytes present were larger and more fully developed, consisting of mainly Stage IV oocytes (Figure 3C). Although these oocytes were larger in size, a substantial decrease in fecundity from July to September sampling dates was found for all corals, indicating a likely split spawn in 2020. Two corals, LC-041 and LC-053 were sampled again on September 18, 2020 after the anticipated September spawning window. Very few gametes remained, with a limited number of oocytes present in either coral.
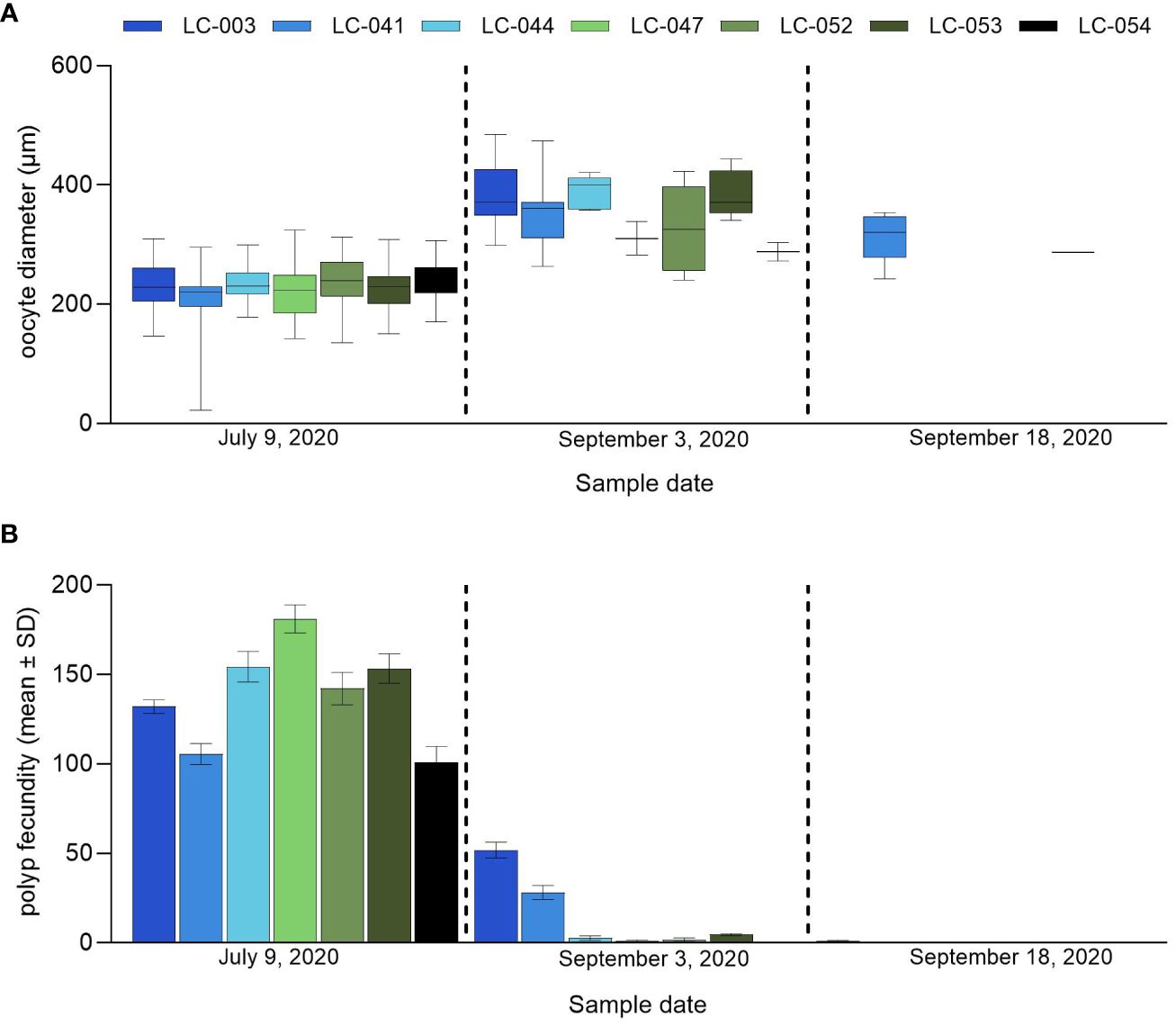
Figure 4 Orbicella faveolata. (A) oocyte/ova diameter (µm) and (B) mean (± SD) polyp fecundity for the individual corals sampled on July 9, 2020, September 3, 2020 and September 18, 2020.
A team of at least 5 divers monitored the large corals at the site for spawning each night from September 5 through September 9. Corals were checked for the presence of setting bundles regularly from approximately 7:00 pm until approximately 12:00 am; no setting bundles or spawning were observed by divers on the dates monitored.
4 Discussion
This study sought to examine the current reproductive potential of seven very large (>2 m diameter) O. faveolata colonies located on nearshore reefs of southeast Florida. It has been hypothesized that large, old corals such as these may be reproductively senescent or “zombie” corals (Woodley et al., 2013; Bythell et al., 2018; Grinblat et al., 2023), but our histological results clearly indicate that this is not the case, as all seven colonies were found to contain abundant oocytes. Similarly, the extensive use of antibiotics to treat SCTLD has raised concerns regarding potential impacts to coral reproductive capacity. No clear pattern of effects on oocyte size or polyp fecundity related to previous SCTLD treatment history was observed in these corals, with oocyte size very similar across all sampled corals. The coral with the highest overall polyp fecundity, LC-047, was treated for SCTLD twice during the 2019-2020 spawning year, and as recently as February 2020. The coral with the lowest overall polyp fecundity, LC-054, had no disease occurrence, and therefore no treatments, since December 2018. Polyp fecundity of coral LC-041 was similar to that of coral LC-054, however LC-041 had disease occurrence and treatment in both July and August 2020; this coral also had a number of previous treatments with chlorinated epoxy. Polyp fecundity in the colonies treated most frequently for SCTLD (e.g. LC-003, LC-041, LC-044, LC-052) did not appear to be different from colonies less frequently treated. This was also the case for colonies treated with antibiotic paste only (LC-047 and LC-052) compared to colonies treated with chlorinated epoxy only (LC-003, LC-044, LC-053 and LC-054) and the colony treated with both (LC-041). Overall, estimates of polyp fecundity, although based on Stage III oocytes/ova, were higher for these 7 large colonies compared to other reported fecundity estimates for this species (Weil et al., 2009; Borger and Colley, 2010; Holstein et al., 2015). Therefore previous SCTLD lesions and SCTLD treatments did not appear to result in the systemic reduction in reproductive potential that was observed for YBD-affected colonies of this species (Weil et al., 2009), or that is caused by abiotic stressors such as temperature stress and bleaching (Szmant and Gassman, 1990; Levitan et al., 2014; Fisch et al., 2019), at least in these very large corals where tissue area may offset energetic deficits or other constraints on reproduction (Johnston et al., 2020).
Spawning in these large O. faveolata was not directly observed at the site in September 2020, although the histological analysis demonstrated that all seven corals sampled on July 9 had a large number of later-stage developing oocytes, which were significantly larger by September 3. The observed polyp fecundity was however lower in early September, indicating that spawning or partial spawning had already occurred by that date. Additionally, polyp fecundity was higher in corals LC-003 and LC-041 compared to the other colonies sampled in early September, which could indicate that these corals had only partially spawned. By September 18, polyp fecundity in LC-003 and LC-041 had declined to a level comparable to the other previously sampled corals, indicating that these two corals may have spawned again in September, either just before or possibly after the predicted September spawning window for Caribbean O. faveolata (Alvarado-Chacon et al., 2020), as spawning was not directly observed during the spawning window dates.
Bright et al. (2021) monitored two nearby populations of O. faveolata in Miami and the upper Florida Keys in 2020. Spawning was observed in 14 out of 20 colonies of O. faveolata in Key Largo on August 9, with six of six monitored colonies in Miami spawning a day later, on August 10. A partial repeat spawn in two of six colonies in Miami was observed on September 8. It is therefore possible that peak spawning in the Broward colonies occurred in August 2020, with a partial repeat spawn in September, although this did not occur on the same date as other nearby populations or on predicted dates for spawning of this species in the Caribbean. It is unclear whether the deviation from the predicted spawning window is due to the early full moon in August which may have precipitated a split spawning, the presence of a subpopulation (Rosser, 2015; Klein et al., 2024) or a breakdown in regional spawning synchrony due to external stressors associated with inhabiting the northernmost boundaries of the species’ range (Harrison and Wallace, 1990; Shlesinger and Loya, 2019). It is possible that synchronicity at a finer scale (on the order of days) is compromised, potentially due to high turbidity and/or coastal night light pollution in this region of the reef tract. More frequent sampling and/or in-water monitoring would be required to determine this.
Although spawning was not directly observed in-situ, the histological analysis indicated that these seven unique individuals are still highly fecund, developing gametes and spawning synchronously with each other. No obvious temporal impact of SCTLD occurrence or antibiotic treatments on reproductive potential was found, and although more data is required given the small sample size described here, the results suggest that the use of antibiotics did not impact reproductive metrics. Given these corals’ large size, persistence in a SCTLD endemic zone and the fact that they are highly fecund, we suggest expanded monitoring around predicted spawning dates. This will allow us to obtain more definitive spawning data and collect gametes from these important corals.
Data availability statement
The raw data supporting the conclusions of this article will be made available by the authors, without undue reservation.
Ethics statement
The manuscript presents research on animals that do not require ethical approval for their study.
Author contributions
DR: Writing – original draft, Visualization, Methodology, Investigation. HN: Writing – original draft, Visualization, Investigation. NT: Visualization, Writing – original draft. JF: Writing – review & editing, Project administration, Funding acquisition, Conceptualization. BW: Conceptualization, Data curation, Project administration, Writing – original draft.
Funding
The author(s) declare financial support was received for the research, authorship, and/or publication of this article. Funding was provided by the Florida Department of Environmental Protection Award No. B77DF8. The views, statements, findings, conclusions, and recommendations expressed herein are those of the authors and do not necessarily reflect the views of the State of Florida or any of its sub-agencies.
Acknowledgments
This work was conducted under the State of Florida Special Activity License SAL-20-2238B-SCRP which authorized gamete collection of ESA-threatened Orbicella corals for the intent of assisting in sexual reproduction and the collection of histology cores to determine reproductive activity. Thank you to Samantha King, Landis Bullock, Alysha Brunelle, Sammi Buckley, Brooke Enright, Tommy Ingalls, Liz Fromuth, and Kristin Anderson for assisting with field operations.
Conflict of interest
The authors declare that the research was conducted in the absence of any commercial or financial relationships that could be construed as a potential conflict of interest.
The reviewer EW declared a past co-authorship with the author BW to the handling editor.
Publisher’s note
All claims expressed in this article are solely those of the authors and do not necessarily represent those of their affiliated organizations, or those of the publisher, the editors and the reviewers. Any product that may be evaluated in this article, or claim that may be made by its manufacturer, is not guaranteed or endorsed by the publisher.
References
Alvarado-Chacon E. M., Gómez-Lemos L. A., Sierra-Sabalza N. P., Hernández-Chamorro A. M., Lozano-Peña J. P., Valcárcel-Castellanos C. A., et al. (2020). Early life history of the Caribbean coral Orbicella faveolata (Scleractinia: Merulinidae). Rev. Biología Trop. 68, 1262–1274. doi: 10.15517/rbt.v68i4
Alvarez-Filip L., Estrada-Saldívar N., Pérez-Cervantes E., Molina-Hernández A., González-Barrios F. J. (2019). A rapid spread of the stony coral tissue loss disease outbreak in the Mexican Caribbean. PeerJ 7, e8069. doi: 10.7717/peerj.8069
Borger J. L., Colley S. (2010). The effects of a coral disease on the reproductive output of Montastraea faveolata (Scleractinia: Faviidae). Rev. Biol. Trop. 58, 99–110.
Bright A. J., Peterson A., Ladd M. C., Williams D. E. (2021). Quicklook Report: Coral spawning 2020: Activities and observations. (Southeast Fisheries Science Center). doi: 10.25923/y12y-7850
Bythell J. C., Brown B. E., Kirkwood T. B. (2018). Do reef corals age? Biol. Rev. 93, 1192–1202. doi: 10.1111/brv.12391
Fisch J., Drury C., Towle E. K., Winter R. N., Miller M. W. (2019). Physiological and reproductive repercussions of consecutive summer bleaching events of the threatened Caribbean coral Orbicella faveolata. Coral Reefs 38, 863–876. doi: 10.1007/s00338-019-01817-5
Flaherty C. M., Dodson S. I. (2005). Effects of pharmaceuticals on Daphnia survival, growth, and reproduction. Chemosphere 61, 200–207. doi: 10.1016/j.chemosphere.2005.02.016
Grinblat M., Eyal-Shaham L., Eyal G., Ben-Zvi O., Harii S., Morita M., et al. (2023). Energy allocation trade-offs as a function of age in fungiid corals. Front. Mar. Sci. 10, 1113987. doi: 10.3389/fmars.2023.1113987
Harrison P. L., Wallace C. C. (1990). Reproduction, dispersal and recruitment of scleractinian corals. Coral Reefs: Ecosyst. World 25, 133–207.
Hayes N. K., Walton C. J., Gilliam D. S. (2022). Tissue loss disease outbreak significantly alters the Southeast Florida stony coral assemblage. Front. Mar. Sci. 9. doi: 10.3389/fmars.2022.975894
Holstein D. M., Smith T. B., Gyory J., Paris C. B. (2015). Fertile fathoms: deep reproductive refugia for threatened shallow corals. Sci. Rep. 5 (1), 12407. doi: 10.1038/srep12407
Johnston E. C., Counsell C. W., Sale T. L., Burgess S. C., Toonen R. J. (2020). The legacy of stress: Coral bleaching impacts reproduction years later. Funct. Ecol. 34, 2315–2325. doi: 10.1111/1365-2435.13653
Klein A. M., Sturm A. B., Eckert R. J., Walker B. K., Neely K. L., Voss J. D. (2024). Algal symbiont genera but not oral host genotypes correlate to stony coral tissue loss disease susceptibility among Orbicella faveolata colonies in South Florida. Front. Mar. Sci. 11, 1287457. doi: 10.3389/fmars.2024.1287457
Kraemer S. A., Ramachandran A., Perron G. G. (2019). Antibiotic pollution in the environment: from microbial ecology to public policy. Microorganisms 7, 180. doi: 10.3390/microorganisms7060180
Levitan D. R., Boudreau W., Jara J., Knowlton N. (2014). Long-term reduced spawning in Orbicella coral species due to temperature stress. Mar. Ecol. Prog. Ser. 515, 1–10. doi: 10.3354/meps11063
Muller E. M., Sartor C., Alcaraz N. I., Van Woesik R. (2020). Spatial epidemiology of the stony-coral-tissue-loss disease in Florida. Front. Mar. Sci. 7, 163. doi: 10.3389/fmars.2020.00163
Neely K. L., Shea C. P., Macaulay K. A., Hower E. K., Dobler M. A. (2021). Short-and long-term effectiveness of coral disease treatments. Front. Mar. Sci. 8, 675349. doi: 10.3389/fmars.2021.675349
Precht W. F., Gintert B. E., Robbart M. L., Fura R., Van Woesik R. (2016). Unprecedented disease-related coral mortality in Southeastern Florida. Sci. Rep. 6, 31374. doi: 10.1038/srep31374
Quiroz S. M., Renteria R. T., Tapia G. G. R., Miller M. W., Grosso-Becerra M. V., Banaszak A. T. (2023). Coral affected by stony coral tissue loss disease can produce viable offspring. PeerJ 11, e15519. doi: 10.7717/peerj.15519
Rippe J. P., Kriefall N. G., Davies S. W., Castillo K. D. (2019). Differential disease incidence and mortality of inner and outer reef corals of the upper Florida Keys in association with a white syndrome outbreak. Bull. Mar. Sci. 95, 305–316. doi: 10.5343/bms.2018.0034
Rosser N. L. (2015). Asynchronous spawning in sympatric populations of a hard coral reveals cryptic species and ancient genetic lineages. Mol. Ecol. 24, 5006–5019. doi: 10.1111/mec.13372
Shilling E. N., Combs I. R., Voss J. D. (2021). Assessing the effectiveness of two intervention methods for stony coral tissue loss disease on Montastraea cavernosa. Sci. Rep. 11, 8566. doi: 10.1038/s41598-021-86926-4
Shlesinger T., Loya Y. (2019). Breakdown in spawning synchrony: A silent threat to coral persistence. Science 365, 1002–1007. doi: 10.1126/science.aax0110
Szmant A. M. (1986). Reproductive ecology of Caribbean reef corals. Coral Reefs 5, 43–53. doi: 10.1007/BF00302170
Szmant A., Gassman N. J. (1990). The effects of prolonged “bleaching“ on the tissue biomass and reproduction of the reef coral Montastrea annularis. Coral Reefs 8, 217–224. doi: 10.1007/BF00265014
van Woesik R., Scott W. J. IV, Aronson R. B. (2014). Lost opportunities: coral recruitment does not translate to reef recovery in the Florida Keys. Mar. pollut. Bull. 88, 110–117. doi: 10.1016/j.marpolbul.2014.09.017
Vermeij M. J. A., Chamberland V. F., Marhaver K. L. (2020). Coral spawning predictions, Southern Caribbean 2007-2020 (Curacao: CARMABI).
Walker B. K., Turner N. R., Noren H. K., Buckley S. F., Pitts K. A. (2021). Optimizing stony coral tissue loss disease (SCTLD) intervention treatments on Montastraea cavernosa in an endemic zone. Front. Mar. Sci. 8, 666224. doi: 10.3389/fmars.2021.666224
Walton C. J., Hayes N. K., Gilliam D. S. (2018). Impacts of a regional, multi-year, multi-species coral disease outbreak in Southeast Florida. Front. Mar. Sci. 5, 323. doi: 10.3389/fmars.2018.00323
Weil E., Cróquer A., Urreiztieta I. (2009). Yellow band disease compromises the reproductive output of the Caribbean reef-building coral Montastraea faveolata (Anthozoa, Scleractinia). Dis. Aquat. Organisms 87, 45–55. doi: 10.3354/dao02103
Weil E., Hernández-Delgado E. A., González M., Williams S., Suleimán-Ramos S. E., Figuerola M., et al. (2019). Spread of the new coral disease “SCTLD” into the Caribbean: implications for Puerto Rico. Reef Enc. 34, 38–43.
Woodley C. M., Burnett A., Downs C. (2013). Epidemiological assessment of reproductive condition of ESA priority coral. Available at: https://repository.library.noaa.gov/view/noaa/941.
Keywords: stony coral tissue loss disease, coral reproduction, Orbicella faveolata, antibiotics, fecundity
Citation: Renegar DA, Noren HKG, Turner NR, Figueiredo J and Walker BK (2024) Southeast Florida large Orbicella faveolata are highly fecund without evident disease intervention effects. Front. Mar. Sci. 11:1369222. doi: 10.3389/fmars.2024.1369222
Received: 11 January 2024; Accepted: 29 April 2024;
Published: 24 May 2024.
Edited by:
William F. Precht, Dial Cordy and Associates, Inc., United StatesReviewed by:
Ernesto F. Weil, University of Puerto Rico at Mayagüez, Puerto RicoJoshua Patterson, University of Florida, United States
Copyright © 2024 Renegar, Noren, Turner, Figueiredo and Walker. This is an open-access article distributed under the terms of the Creative Commons Attribution License (CC BY). The use, distribution or reproduction in other forums is permitted, provided the original author(s) and the copyright owner(s) are credited and that the original publication in this journal is cited, in accordance with accepted academic practice. No use, distribution or reproduction is permitted which does not comply with these terms.
*Correspondence: D. Abigail Renegar, drenegar@nova.edu