- 1Institute of Ocean Engineering and Technology, Ocean College, Zhejiang University, Zhoushan, China
- 2Donghai Laboratory, Zhoushan, China
- 3Ocean Research Center of Zhoushan, Zhejiang University, Zhoushan, China
Marine water resources (including seawater and pore-water) provide important information for understanding the marine environment, studying marine organisms, and developing marine resources. Obtaining high-quality marine water samples is significant to marine scientific research and monitoring of marine resources. Since the 20th century, marine water resources sampling technology has become the key research direction of marine equipment. In order to have a comprehensive understanding of marine water resource sampling technology, promote the development of marine water resource sampling technology, and obtain high-quality marine water samples, this paper summarizes the current development status of the sampling technology of marine water resources from the aspects of research and application. This paper first provides an overview of seawater and pore water sampling techniques. The two sampling technologies are categorized and discussed according to different sampling means, and the advantages of different sampling means are compared. We also found similarities between seawater and pore water sampling means. Then, a comprehensive analysis of existing technologies and equipment reveals the development trend of marine water resources sampling technology, for example, the need for high temporal and spatial accuracy in sampling, etc. Finally, it explores the challenges facing deep-sea water sampling technology regarding future research, development and equipment industrialization. These reviews not only help researchers better understand the current development of marine water sampling technologies but also provide an important reference for the future development of marine water sampling technology, which provides guidance and support for in-depth marine scientific research and effective use of marine resources.
1 Introduction
The oceans are rich in natural resources and energy potential, and the sustainable utilization of marine resources and the strengthening of research in marine science and technology are essential to maintaining the ecological balance of the Earth and the sustainable development of human society (Karydis and Kitsiou, 2013; Jamieson, 2015; Zhao and others, 2022). Marine water resources (including seawater and sediment pore-water) contain many valuable substances (e.g., salts, minerals, active substances of marine organisms, etc.), which are of great significance in guiding conventional hydrological observation of the oceans, assessment of the marine environment, research on marine organisms, geological surveys, and resource monitoring (Naraoka et al., 2008; Connelly and others, 2012; Cunliffe and others, 2013; Tseng and Tang, 2014; Da Ros and others, 2019). Therefore, research on marine water resources sampling technology, development of marine water resources sampling equipment, and acquiring high-quality marine water resources are the basis for marine scientific research and management. The literature research shows that various marine water sampling techniques have been developed comprehensively, but the literature summarizing the marine water sampling techniques is relatively small, and all of them introduce the single principle of marine water sampling techniques (Jannasch et al., 2004; Mucciarone et al., 2021). Therefore, this paper wants to introduce the development history of marine water sampling technology so that marine researchers can understand the different types of marine water sampling technology.
Marine sampling technology is an important tool for scientific research, and similar to other sampling technologies, marine water resource sampling technology focuses on the following technical studies: (1) Integrity of samples, such as in-situ acquisition techniques, holding-pressure techniques, and low-contamination and low-disturbance techniques; (2) Flexibility in Sampling: for example, the device is adjusted to different needs and is suitable for sampling at different depths as well as in different sea locations; (3) Timeliness of Sampling: the device can continuously sample at a specific time or over a long period in order to provide samples with high temporal accuracy and to effectively monitor changes in the marine environment; (4) Diversity of samples: Sampling for different marine environments and sampling objectives, not limited to seawater above the sediment interface, pore-water below the sediment, and microorganisms in marine water (Wang and Wang, 2018; Stock et al., 2019; Judd and others, 2022).
With the development of marine resources worldwide, many new sampling technologies and types of equipment have emerged in marine water resource sampling (Yu et al., 2018). In order to better understand marine water resource sampling technology, this paper reviews it and its development. This paper summarizes the research progress of marine water resource sampling technology at this stage, analyzes the development trend of deep-sea marine water resource sampling technology, and finally discusses the challenges faced by marine water resource sampling technology regarding technological development, equipment research and development, and industrialization. Through this paper, researchers can understand the current research status of marine water resource sampling technology, which facilitates them in evaluating and comparing different types of seawater samplers and choosing the one suitable for their research purposes and conditions. Ultimately, through this paper, the problems existing in the existing research can be found to provide a theoretical basis and practical guidance for subsequent research and to improve the efficiency and quality of the research.
2 Advances in marine water resource sampling techniques
This chapter introduces the main research progress of current marine water resources regarding seawater and pore-water sampling technologies. Furthermore, we analyze the functional advantages and limitations of several major marine water resource sampling technologies at this stage. The classification of the main structure of marine water resources sampling technology is shown in Figure 1.
2.1 Main content of seawater sampling techniques
Seawater sampling technology can be categorized according to its different functions. It can be divided into the pressure-retaining and the non-pressure-retaining sampler according to pressure-retaining ability. Pressure-retaining sample technology refers to keeping the pressure of the seawater constant during sampling to avoid dissolved gas release, and it can accurately maintain the original physical and chemical properties of the sample. However, the pressure-retaining sampler has the disadvantages of cumbersome operation and high cost. Non-pressure-retaining sample technology collects seawater directly into the sampling container during sampling. This sampling method is easy to operate and low cost but will affect the authenticity of the samples. It can also be divided into active and passive sampler according to their power form. Active sample technology refers to the direct sampling by the operator during the sampling process, such as using water collection bottles. Active samplers can precisely control the time and location of sampling, but they may introduce external pollution and change the characteristics of the sample. Passive sample technology uses specially designed samplers for sampling without the operator intervening in the sampling process. The advantage is that the sampling can be monitored over a long period and can avoid the effects of operator intervention. However, it is not easy to control the sampling conditions accurately.
This section introduces the seawater sampling technology based on penetration and negative pressure forms.
2.1.1 Seawater sampling techniques based on penetration form
At the beginning of the 20th century, scientists put forward the form of seawater samplers, such as the Nansen sampler (Warren, 2008) and Niskin sampler (Niskin, 1962). The sampler consists of a through sampling chamber between the left and right end caps. By controlling the opening of the caps at both ends, seawater enters the sampling chamber; by controlling the closing of the caps at both ends, seawater is stored in the sampling chamber to complete sampling. In order to collect more quantities of seawater, the sampling chambers will be arranged as horizontal arrays, circular arrays or other forms to realize the discrete collection of seawater, as shown in Figure 2 (Crompton, 2006).
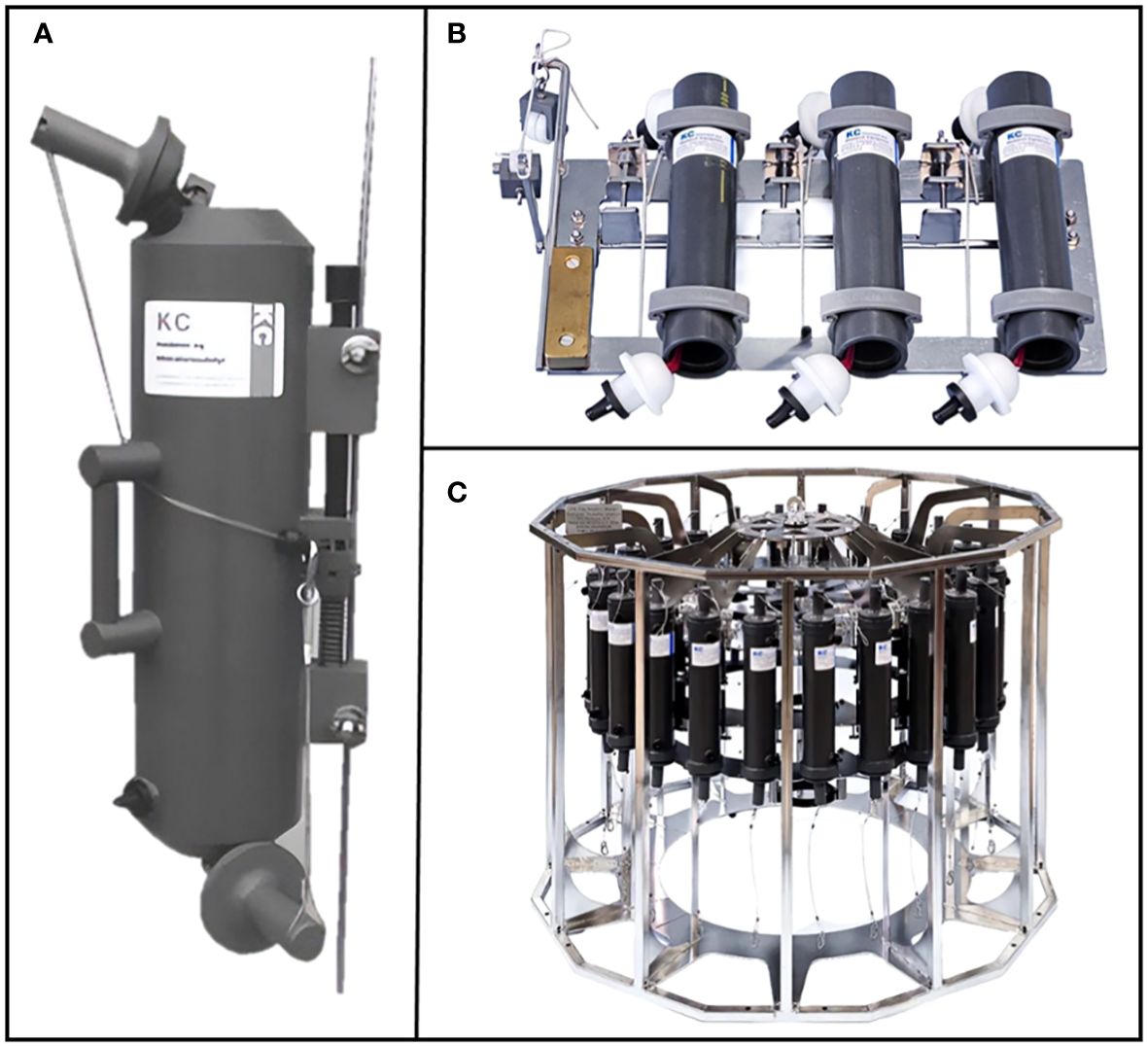
Figure 2 Structure of the Niskin sampler. (A) Single sampling chamber; (B) Sampling chambers in a horizontal array; (C) Sampling chambers in a circular array.
This sampling method is a simple and convenient operation. However, the sampling form of simultaneous closure through the caps will cause the target stratum seawater and other strata seawater to contaminate the samples due to inadequate replacement. Moreover, due to the limitation of the sampling chambers’ volume, when obtaining more samples is necessary, the sampler’s weight and volume will increase further, thus increasing the difficulty of sampling.
2.1.2 Seawater sampling techniques based on negative pressure form
With the development of marine technology, the sampling method of seawater samplers has made some progress, and the seawater samplers based on negative pressure form have also been gradually developed and applied. The negative pressure form is divided into the following three main sampling methods:
(1) Vacuum chamber-based sampling.
The vacuum chamber-based sampling is simple. Its sampling principle is that the sample chamber is pre-treated as a vacuum state. When the sampler reaches the sampling position, the manipulator or other triggering methods open the sampling valve. At this time, the seawater enters the sample chamber under pressure difference. The University of Washington developed the “Lupton” airtight sampler based on the vacuum chamber. However, using a vacuum chamber sampling form, the volume of the sampling chamber is usually fixed, and the seawater sample volume is uncontrollable (Edmond, 1992; Von Damm et al., 1997).
(2) Plunger-based sampling.
Plunger-based sampling is done through the piston movement in the sample chamber. The University of Delaware developed a plunger-based form of the seawater sampler - “Sipper”, which is used on the “Alvin” ROV and collected 12 samples in a dive (Di Meo et al., 1999). The University of Florida also designed an inexpensive, automatic, submersible water sampler consisting of sample chambers constructed of 10 spring-loaded 60 mL syringes connected to solenoid valves and electronics to control the solenoid valves (Martin et al., 2004). In addition, Monterey Bay Aquarium Research Institute researchers have developed a seawater sampling system based on a plunger sampling form - the “Gulper” sampler (Bird et al., 2007; Zhang et al., 2009). The “Gulper” sampler utilizes a spring-driven piston built into the chamber to pump seawater into the sample chamber. “Gulper” uses energy stored in the spring to move the piston, reducing energy consumption and allowing for rapid sample collection. As shown in Figure 3, seawater is drawn into the chamber from the marine environment through the inlet valve, and the seawater above the piston is discharged through the outlet at the end cap of the chamber. Researchers fixed “Gulper” sample chambers on the AUV “Dorado” and conducted many oceanographic surveys in Monterey Bay (Zhang et al., 2012; Pennington et al., 2016).
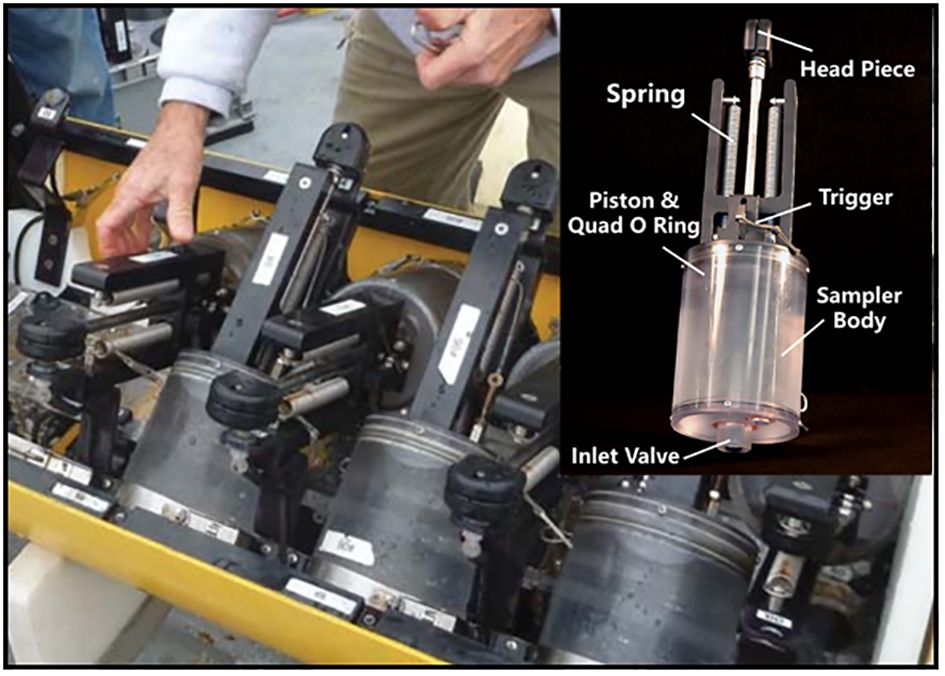
Figure 3 Structure and application of the “Gulper” sampler (Pennington et al., 2016).
Plunger-based samplers are simple in structure and can utilize different power systems to drive the piston movement. The volume of seawater samples can be adjusted to demand. Therefore, the plunger-based samplers are widely used. With the evolution of marine technologies, plunger-based sampling is gradually evolving from a non-pressure-retaining form to a pressure-retaining form for researchers to maintain the high quality of samples. In 1999, the University of the Mediterranean developed a high-pressure water sampler - the “HPSS”, to measure the activity of marine microorganisms. The sampler regulates the pressure of the sample using a self-contained accumulator, which allows it to sample and store seawater at different depths (Bianchi et al., 1999). As shown in Figure 4A, Woods Hole Oceanographic Institution designed a gas-tight isobaric seawater sampling system for hydrothermal fluid collection - “Jeff”, which maintains the pressure of the sample through compressed nitrogen and regulates the sampling rate through a damping hole between the two chambers (Seewald et al., 2002). When sampling, the sampling valve opens, and the pressure difference drives seawater into the sampling chamber. For secondary sampling, the pressure of the seawater sample in the sampling chamber is maintained by pumping high-pressure water into the reservoir chamber. The “Jeff” collects samples by differential pressure, which makes the sampling deployment faster. Zhejiang University has developed a novel mechanical gas-tight sampler for hydrothermal fluids (Chen et al., 2007). The sampler uses a manipulator to trigger the sampling valve, collects samples by compressing the piston inside the sampling chamber and maintains the sample pressure using an accumulator. However, the sampler is uncontrolled by the mechanical drive during sampling, which causes sample contamination. As shown in Figure 4B, Zhejiang University proposed a piston pressure adaptive gas-tight seawater sampler that can withstand 70 MPa pressure according to the pressure balance principle. The sampler can adjust the pressures according to the environmental pressure and ensure that the samples will not leak (Wu et al., 2010, 2011). For ease of application, Zhejiang University developed a gas-tight deep-sea water sampling system (GTWSS) by controlling the above multiple samplers through an integrated system, which utilizes electronically triggered sampling (Huang and others, 2012; Wu et al., 2015).
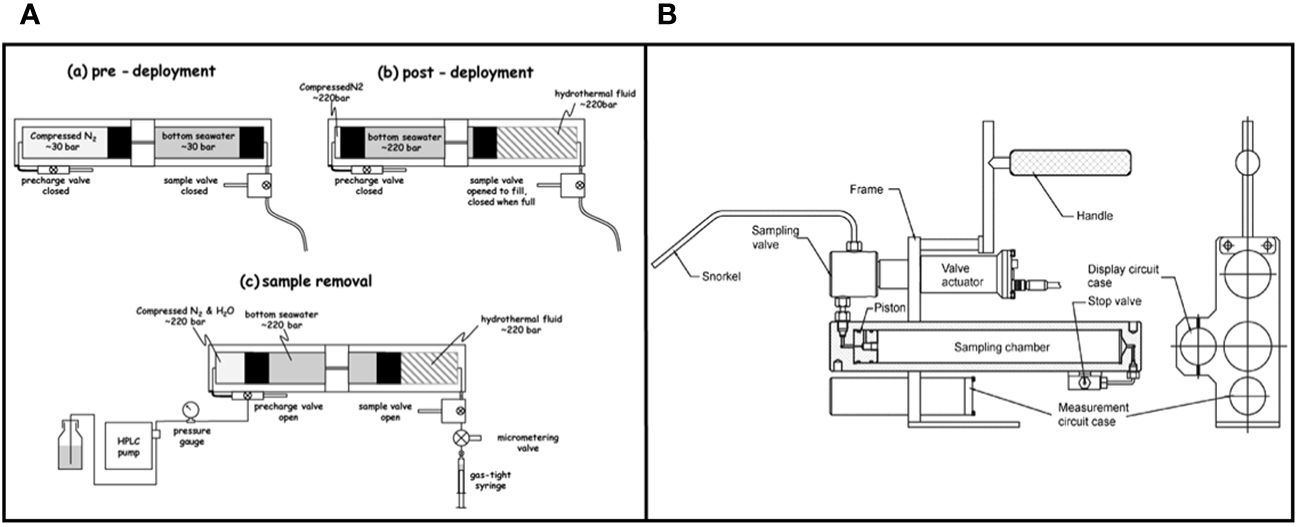
Figure 4 Structure of pressure-retaining plunger-based sampler. (A) “Jeff” sampling schematic (Seewald et al., 2002); (B) Schematic illustration of the electric control gas-tight sampler (Wu et al., 2014).
In order to obtain a larger number of samples, samplers based on the plunger form need to place sampling chambers in an array form, which increases the sampling complexity to a certain extent.
(3) Pump-based sampling.
With the gradual development of science and technology, in addition to the high demand for the quality of samples, scientists’ demand for the number of samples (high temporal and spatial accuracy) has also increased. Therefore, Pump-based sampling techniques have been developed. The pump can be connected directly to the sampling chamber, or the pump can be connected to a multi-channel valve to obtain multiple samples.
•The pump is directly connected to the sampling chamber.
Monterey Bay Aquarium Research Institute researchers first proposed osmotic form-based water sampling techniques. They developed an in-situ analyzer using a medical osmotic pump to continuously monitor nitrate concentrations in freshwater or marine water (Jannasch et al., 1994; Kastner et al., 2000). Subsequently, the team designed a long-term fluid sampling system (OsmoSampler) to collect water samples continuously and autonomously. The OsmoSampler consists of an osmotic pump connected to a sample storage tube, which relies only on osmotic pressure to drive water sampling and requires no external power or moving parts, as shown in Figure 5A. The principle of operation is that the reverse osmosis membrane divides the osmosis pump chamber into two compartments, one is filled with supersaturated NaCl solution and connected to the seawater environment; the other is connected to a long-storage sample tube and filled with pure water. Since the reverse osmosis membrane does not allow dissolved salts to pass through, the salinity difference between the two sides of the membrane causes the pure water on one side of the chamber to flow from the less concentrated side to the more concentrated side. Thus, seawater flows into the storage tube under this osmotic pressure. The Osmo sampler can be customized for different numbers of Alzet osmotic capsules and different sizes of RO membranes to determine the sampling rate and the volume of samples to be collected in a given period based on the flow rate of the osmotic pump. The osmotic pump does not require electrical power and relies only on the concentration difference to provide sampling power. Therefore, the OsmoSampler is simple, reliable, and suitable for long-term water sampling. The Osmosampler can be customized to the sampling rate according to the installation of a different number of Alzet pumps so that researchers can determine the sample position in different time scales according to the sampling rate, filling the gap in temporal accuracy of seawater samplers (Chapin et al., 2002; Wheat et al., 2003; Jannasch et al., 2004).
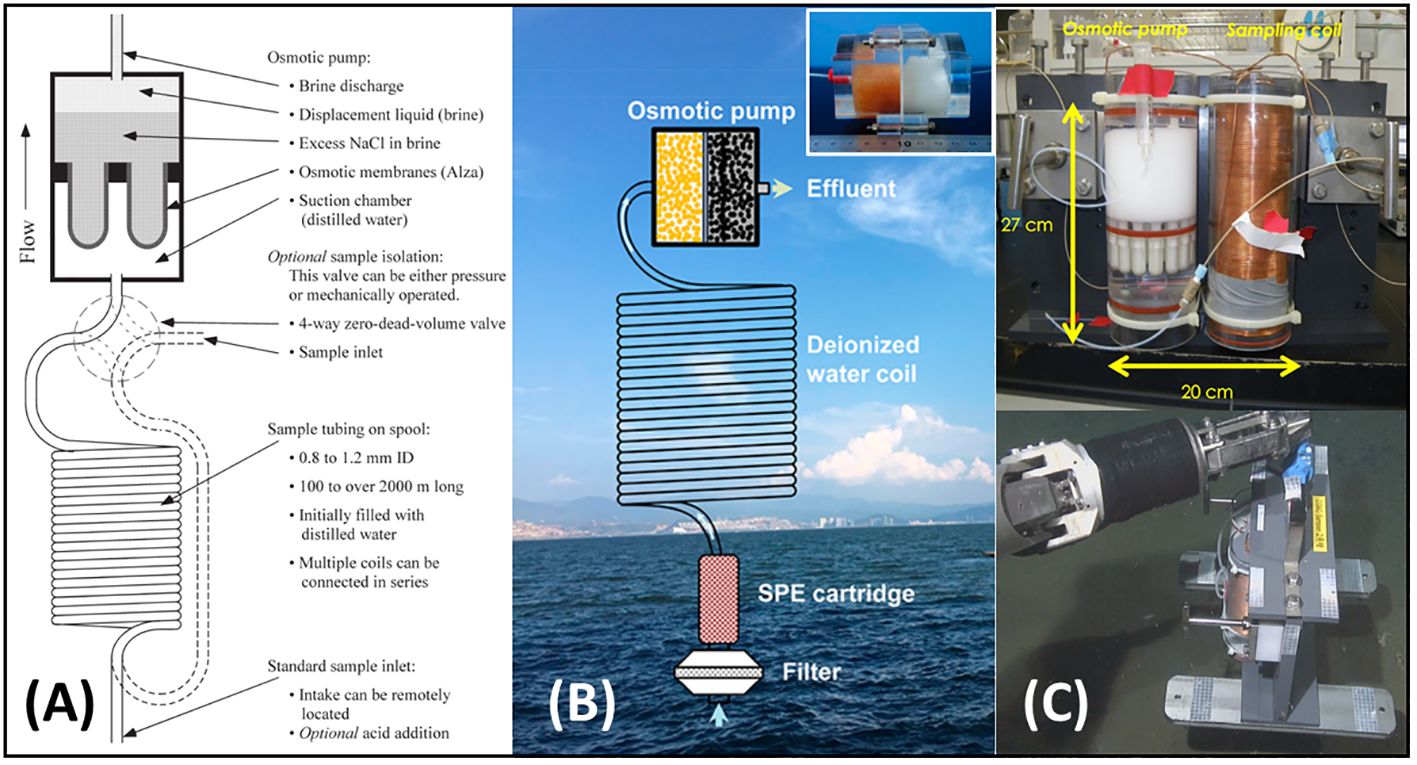
Figure 5 Structural and application of the OsmoSampler. (A) Schematic of an OsmoSampler (Jannasch et al., 2004); (B) Schematic of the OP-SPE (Lin and others, 2019); (C) Picture of the OsmoSampler for pore-water sampling (Owari et al., 2019).
Then, researchers at the University of Iceland time-stamped the OsmoSampler by adding rhodamine dye to record the sampling time series (Jones and others, 2015). The sampler was applied to the Lymington River for a month-long trial. It was eventually deployed in southern Iceland for up to 40 days of sampling and monitoring to identify activities during thawing by detecting rivers draining volcanic areas. OsmoSampler has completed several experiments and sea trials to verify its application value (Chapin et al., 2004; Gkritzalis-Papadopoulos et al., 2012). Based on the principle of osmosis sampling, researchers at Xiamen University developed an osmosis pump and solid phase extraction (OP-SPE) sampler by combining reverse osmosis (RO) membrane and solid phase extraction (SPE) technology (Lin and others, 2019), as shown in Figure 5B. At the end of sampling, the average concentration can be calculated based on the target analytes on the solid phase extraction column and the sample volume. The sampler was applied in the JiuLong River for environmental testing.
The Australian Cooperative Research Center developed an Autonomous Clean Environment Sampler (ACE Sampler) for trace metals in a time sequence (van der Merwe et al., 2019). The ACE Sampler consists of 12 sets of micro-peristaltic pumps connected with sample chambers and triggered to sample chronologically, as shown in Figure 6A. Furthermore, Stanford University described a compact and inexpensive autonomous submersible multiport water sampler –”AutoSampler” (Mucciarone et al., 2021). The sampler comprises a single peristaltic pump with pinch valves, silicone tubing, nylon fittings, and a manifold. The sampler can collect 12 discrete samples at user-controllable times that can be user-modified for different requirements. As shown in Figure 6B, the electronics mounting plates for the AutoSampler illustrate the locations of the Arduino Pro Mini, real-time clock, relay boards, terminal block, and USB cable.
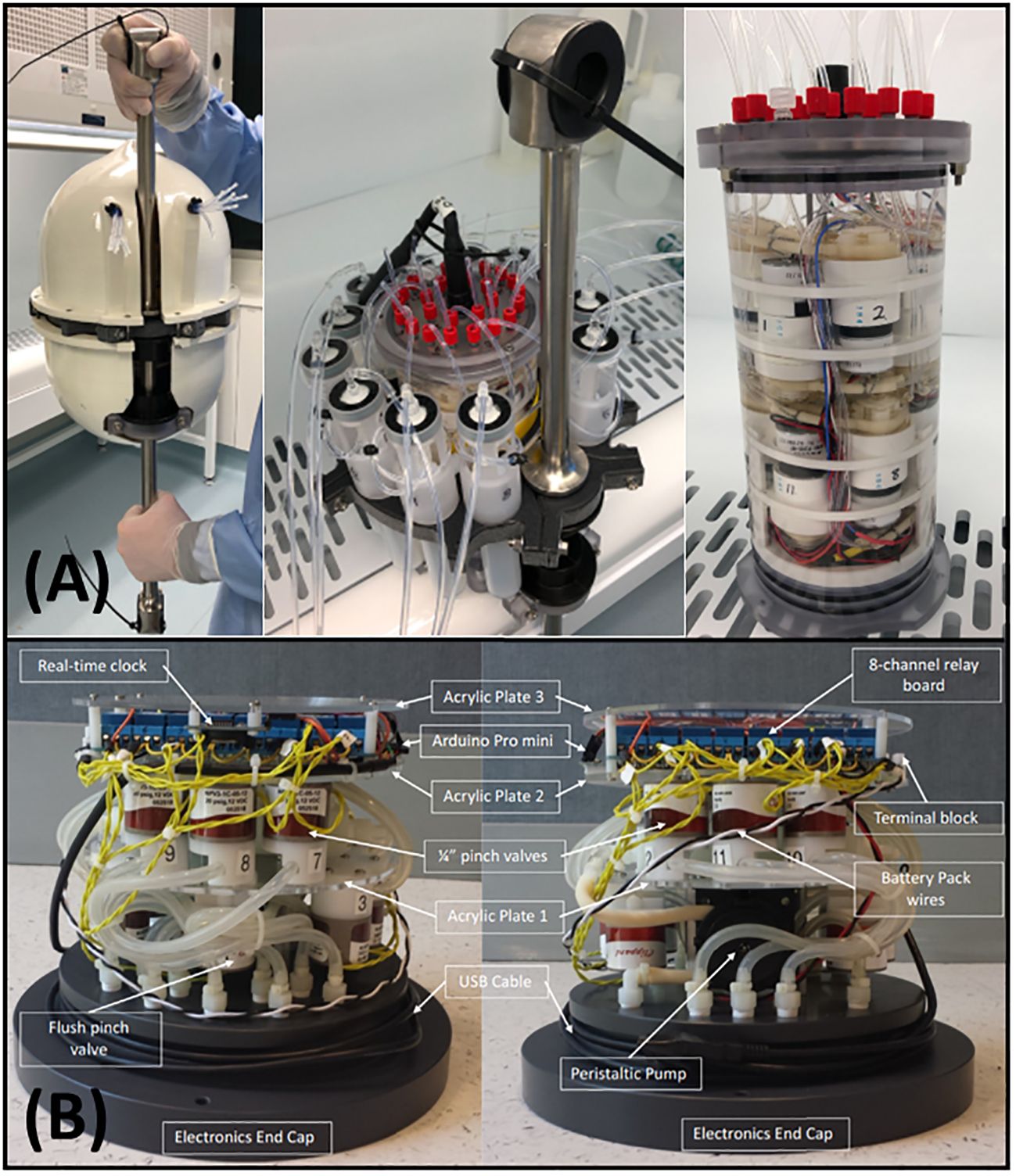
Figure 6 Seawater sampler based on peristaltic pumps. (A) Pictures of ACE Sampler (van der Merwe et al., 2019); (B) Schematic of the “AutoSampler” (Mucciarone et al., 2021).
•The pump is connected to a multi-channel valve.
The University of Tokyo developed a rotary pressure-preserving water sampler – WHATS, which uses a pump and a multi-channel rotary valve to control the sampling of six sampling chambers, and each chamber has a piston (Tsunogai et al., 2003). The initial state of the piston is located in the bottom of the sampling chamber, the upper part of the chamber is full of distilled water, the sampling control of the rotary valve to the pump and the chamber is connected to the pump when the pump to extract the distilled water, piston moving upward, and the hydrothermal samples flowing into the chamber. After that, the Water Hydrothermal-fluid Atsuryoku Tight Sampler II (WHATS-II) was developed by Hokkaido University based on the WHATS (Saegusa et al., 2006). WHATS-II consists of four 150 mL stainless steel sampling chambers, eight ball valves, a manipulator, a pneumatic peristaltic pump and a flexible inlet pipe. Subsequently, as shown in Figure 7, the researchers optimized and developed WHATS-3, including a T-shaped inlet, four double-chamber sampling chambers, a valve operating system and an impeller pump unit (Miyazaki and others, 2017).
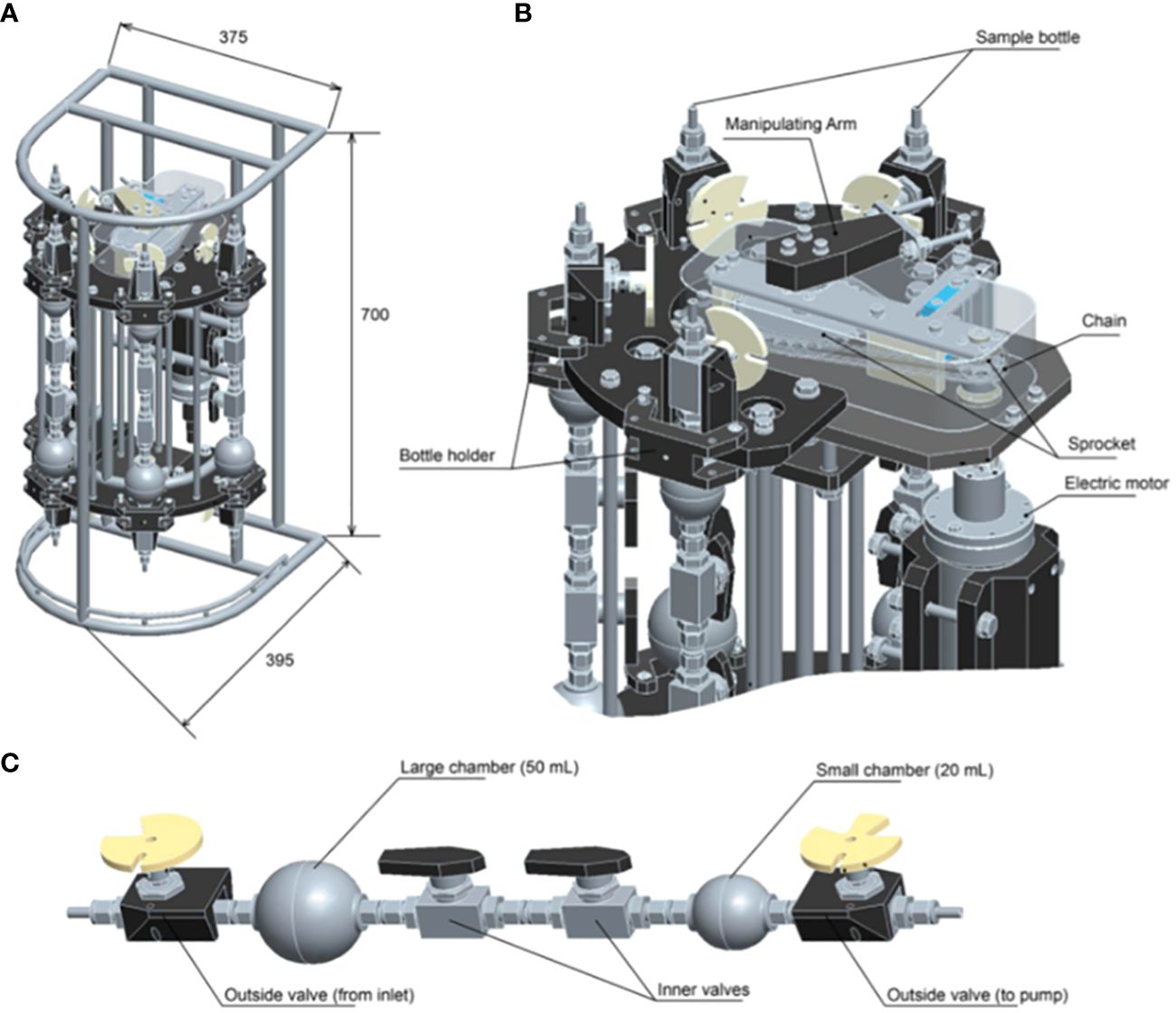
Figure 7 Diagram of the WHATS-3. (A) Structural of the WHATS-3 (mm); (B) Structural of valve manipulating mechanics; (C) Structural of sampling bottle (Miyazaki and others, 2017).
A 128-channel multi-seawater collection system - “ANEMONE-11”, was developed at Kochi University (Okamura and others, 2013). ANEMONE-11 consists of an in-situ pump unit, a valve unit, a sampling bottle set and a control unit, as shown in Figure 8A-1. The pump unit is arranged in four piezoelectric pumps, each with a 10 mL/min flow rate. The valve unit consists of four 32-channel solenoid valves, and all valves are opened and closed according to the preset time intervals. The valve unit is connected to 128 40mL sampling bottles, with the inlet of the bottles connected to the solenoid valve unit and storing seawater samples, and the outlet connected to the seawater environment. Before the deployment, all sampling bottles were filled with distilled water, and the sampler triggered sampling when the work depth exceeded the preset depth threshold. When sampling, the pump continuously pumps seawater to the valve unit and the sampling bottles. Sampling is stopped when the work depth is lower than the preset depth or when all samples have been sampled. In 2012, ANEMONE-11 was mounted on the “Shinkai 6500”, and a hydrothermal plume survey was conducted in the Okinawa Trough, as shown in Figure 8A-2. To spatially and temporally precise sampling in hydrothermal plumes, researchers at Woods Hole Oceanographic Institution have developed a novel Suspended Particulate Rosette (SUPR) multi-sampler that can be deployed either on a mooring for unattended time series sample collection or on a ROV to enable sampling tasks (Breier et al., 2009). After that, they designed a Suspended Particulate Rosette V2 Large-Volume Multi-sampling System (SUPR-V2) for collecting plume samples, as shown in Figures 8B-1, B-2 (Breier and others, 2014). SUPR-V2 was designed with a customized sample control valve. It consisted of two sets of 14-port valves, one for controlling the inlet switch of the sample container and the other for controlling the outlet switch of the sample container. Stepper motors synchronously actuate the two sets of valves to complete the sampling.
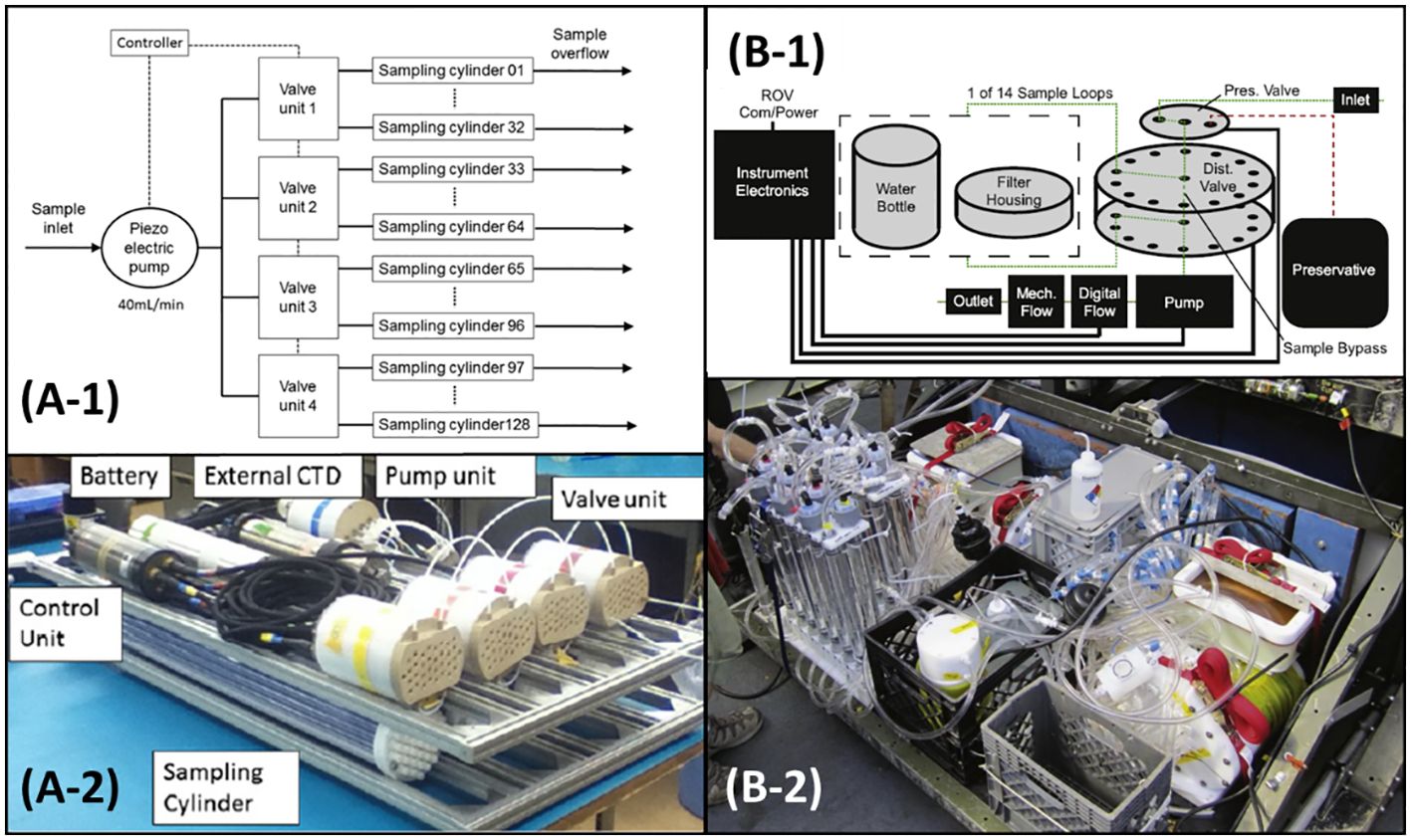
Figure 8 Seawater sampler with multi-channel valve. (A-1) Schematic of the Anemone-11; (A-2) Picture of the Anemone-11 (Okamura and others, 2013); (B-1) Schematic of the SUPR-V2; (B-2) Picture of the SUPR-V2 (Breier and others, 2014).
Table 1 shows the technical parameters of some multi-channel seawater samplers. According to Table 1, the existing seawater samplers are gradually developing in the direction of miniaturization and intelligence while ensuring the number and volume of samples. Therefore, portability and intelligence have also become the key technical difficulties to be overcome in designing and developing new seawater samplers.
2.2 Main content of pore-water sampling techniques
With the gradual increase in the demand for research on marine geological and energy development, marine water sampling is not limited to collecting seawater above the sediment interface. The sampling technology for pore-water below the sediment has also been developed. Sediment pore-water usually reflects the trend of seafloor geological changes, which is of great significance for marine geochemical changes and deep-sea resource acquisition (Matos et al., 2022). The main way to obtain pore-water in two ways: One is the non-in-situ pore-water sampling technology, as shown in Figure 9, through the first collection of sediments and then through pressure filtration (Bender et al., 1987), centrifugation (Carignan et al., 1985) or vacuum filtration (Dickens and others, 2007) to separate the pore-water. These methods are simple, but the sediment samples in the original environment after the departure of a large degree of destruction result in the physical and chemical properties changing, and there will be a certain error in the measurement. Besides, each sampling consumes human resources, and material resources are not conducive to a long period of multiple sampling.
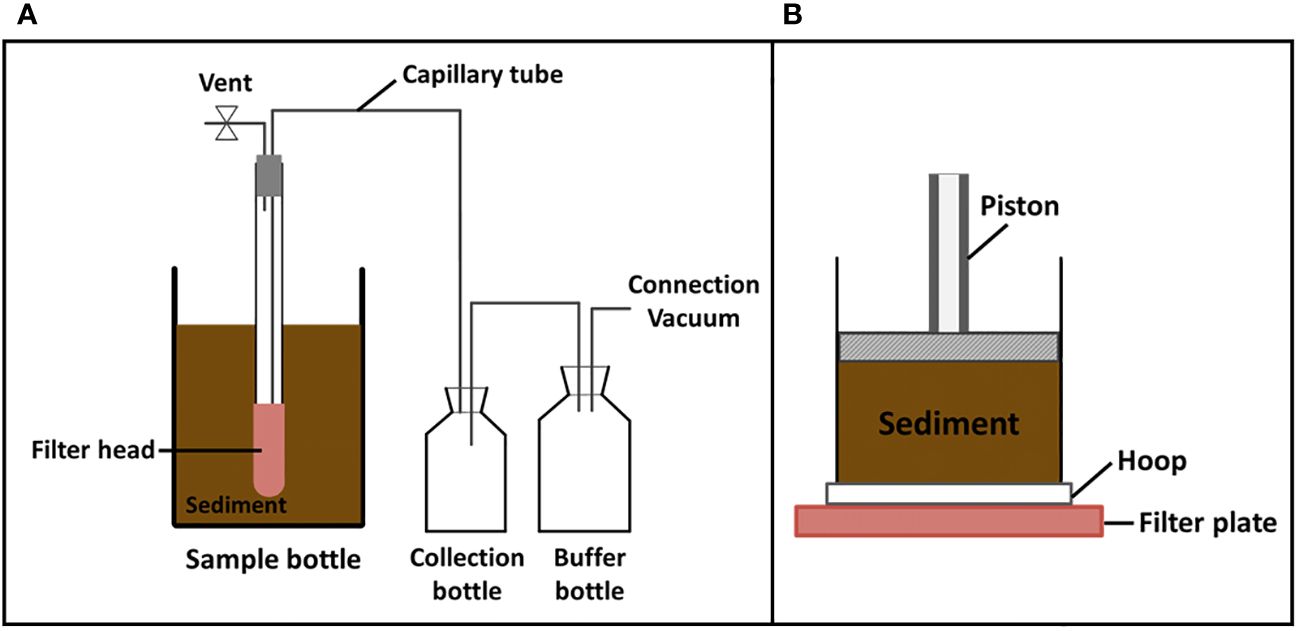
Figure 9 Non-in-situ pore-water sampling methods. (A) Structural of vacuum extraction method; (B) Structural of filtration method.
The other way is the in-situ pore-water sampling technology, which refers to the collection equipment being buried directly in the sediment sampling to obtain the pore-water samples. Scientists favor in-situ sampling because of its higher accuracy. However, in-situ sampling is limited by the sampling environment and time, plus the need to use large vessels or submersibles, the technical difficulty is high, and sampling is challenging (Barnes, 1973). Hence, researchers are more interested in developing in-situ pore-water sampling techniques. This section focuses on two in-situ pore-water sampling techniques.
2.2.1 In situ pore-water sampling techniques based on dialysis form
In 1976, Hesslein proposed an in-situ pore-water sampling technique using the principle of equilibrium dialysis - the Peeper sampler. The Peeper consists of many small cavities of the same volume, and both sides of the cavity are covered with dialysis membranes. The cavity is pre-encapsulated with a sampling medium (e.g., deionized water, electrolyte solution, etc.) (Teasdale et al., 1995). When sampling, with the characteristics of the membrane, some soluble ions and molecules in the pore-water exchange substances with the medium through the membrane. After a while, both sides of the membrane reach equilibrium. The ion concentration in the pore-water is calculated by measuring the concentration of the solution in the chamber. As shown in Figure 10, the Peeper can sample and monitor the sediment depth at the centimeter level. Due to the constraints on the time of equilibrium dialysis, the sampling time is usually between 2-20 days and has a low temporal resolution, which can lead to errors in the calculation of interfacial exchange fluxes in pore-water profiles (Wise, 2009; Xu et al., 2012).
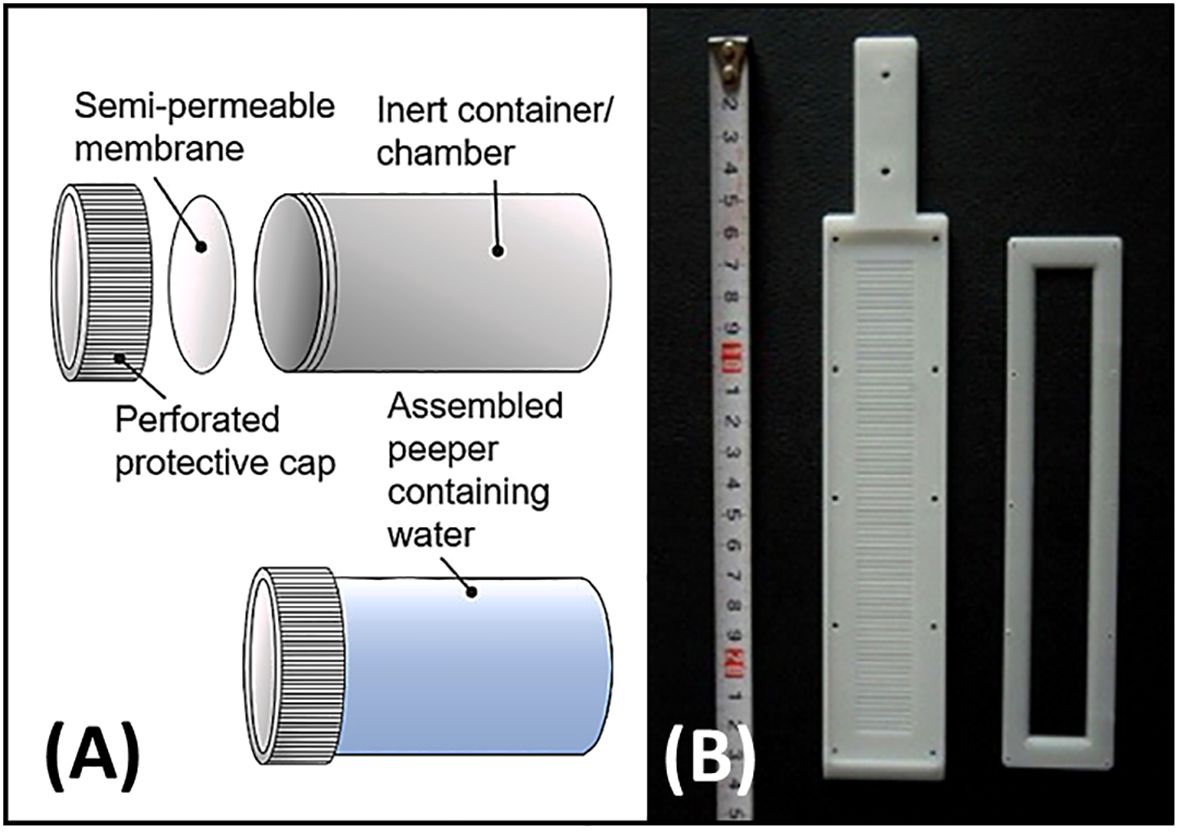
Figure 10 Peeper sampler sampling method. (A) Structural of the Peeper principle; (B) Picture of Peeper sampler.
2.2.2 In situ pore-water sampling techniques based on negative pressure form
Researchers at Woods Hole Oceanographic Institution designed an in-situ pore-water sampler using compressed springs to provide power to filter pore and bottom water from six depths in 2 m of the sediment (Sayles et al., 1976). After that, researchers at Harbor Branch Foundation proposed an in-situ sediment pore-water sampler, which collects pore-water simultaneously from four 1 cm sediment depths at 2 cm intervals. The filtration section of the sampler consists of four filter rings of different depths. The filtration section is connected to pumps, allowing the sample at four discrete locations without disturbing the sample. Furthermore, laboratory and field tests showed vertical profiles of ammonium, reactive phosphate and silicate (Montgomery et al., 1981).
Researchers at Zhejiang University designed an automatic core water sampler based on a microcontroller (Han et al., 2009). The sampler joins the stepper motor and microcontroller control system in the mechanical mechanism to realize the time-sharing automatic sampling. With differential pressure and check valves, pore-water can flow and be retained in sampling chambers, as shown in Figures 11A, B. Moreover, the Guangzhou Marine Geological Survey and other research institutes in China developed an in-situ gas-tight pore-water sampler for deep-sea sediments (Chen and others, 2009), as shown in Figure 11C. Subsequently, Liu et al. improved the in-situ gas-tight pore-water sampler for deep-sea sediments that can simultaneously sample pore-water and seawater (Liu et al., 2018). As shown in Figure 11D, the optimized sampler improves the sampling chamber to double chambers. Before sampling, the solenoid valve-1 and the solenoid valve-2 are closed. When sampling surface seawater, the solenoid valve-1 is opened and the solenoid valve-1 is closed after sampling is completed. When sampling pore-water, the solenoid valve-2 is opened, the pore-water enters sampling chamber-2, and the solenoid valve-2 is closed after completing sampling.
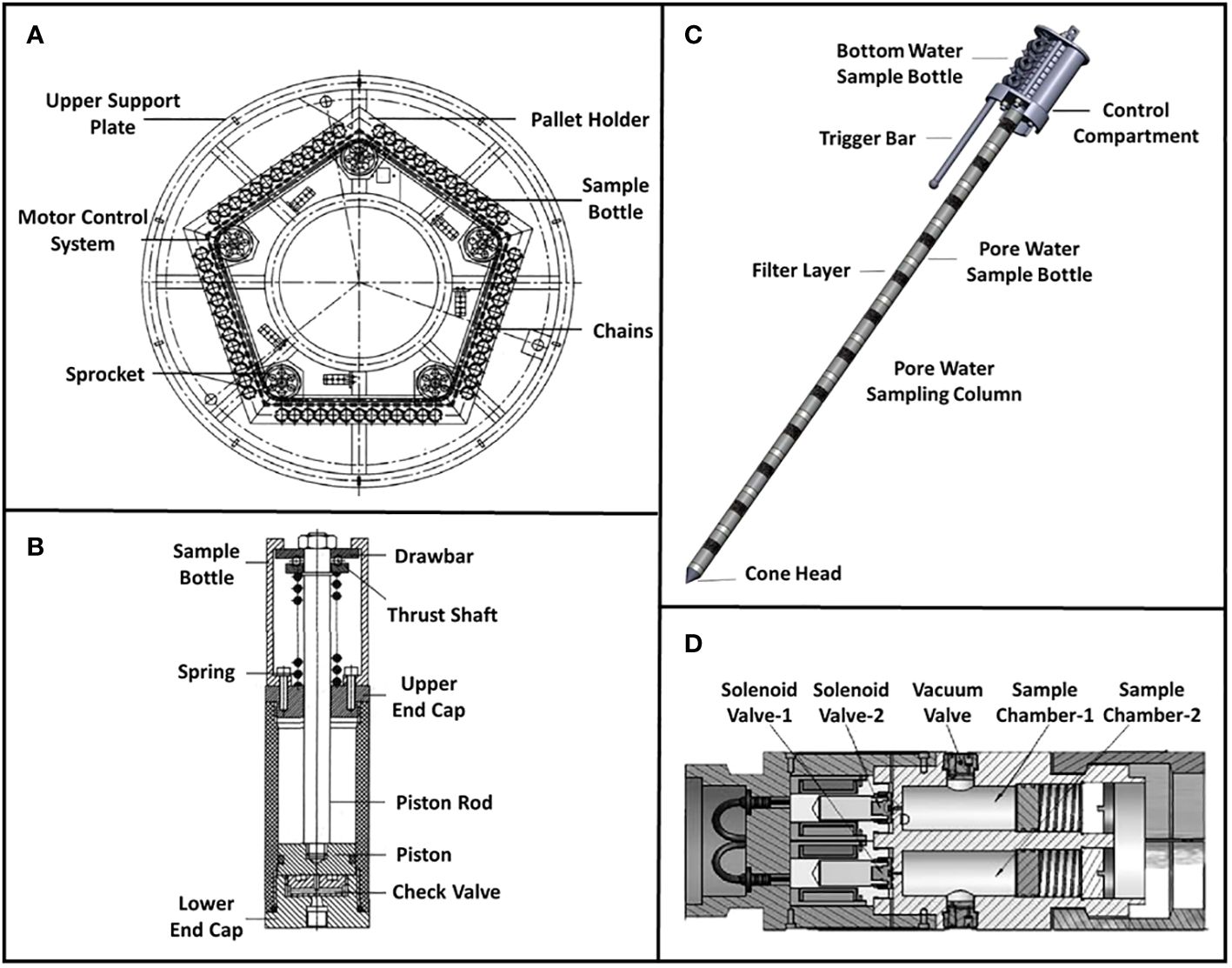
Figure 11 (A, B) Top view and schematic of sample bottle structure of the Automatic Core Water Sampler based on Microcontroller (Han et al., 2009); (C) Schematic of An In Situ Gas-tight Pore Water Sampler for Deep-sea Sediments (Chen and others, 2009); (D) Schematic of sample bottle structure of An In Situ Gas-tight Pore Water Sampler (Liu et al., 2018).
Although the sampler mentioned above is highly functional and capable of obtaining the pore-water of different sedimentary layers, the sampler is complex and disturbs the samples in application. In addition, the sampler is made of alloy materials, which can contaminate the samples.
The OsmoSampler is also suitable for pore-water sampling. Researchers at the University of California use OsmoSampler to monitor the chemistry and flow rates of fluids that diffuse from sediments into the ocean. Sampling at different sediment depths and ranges determines the flow field sediments. The samples are then analyzed chemically to determine the flux of chemical elements diffusing into the ocean. Similar to the principle of seawater sampling, the OsmoSampler only relies on the difference in osmotic pressure to sample the pore-water continuously and autonomously, which has high stability. In 2019, researchers from Chiba University deployed an OsmoSampler at a methane seep vent in the Japan Sea to sample in-situ pore water at 30 cm of sediment, as shown in Figure 5C. It is applied underwater for up to one year to analyze changes in geochemical elements in pore-water over time during gas hydrate formation and dissolution (Owari et al., 2019).
With the development of the front probe, the Rhizon sampler has been widely used. German scientists first developed the Rhizon in-situ sampler (RISS) and validated its application for sampling sediment pore-water (Seeberg-Elverfeldt et al., 2005). The Rhizon sampler was initially used as a micro-tensiometer to sample seepage water in the unsaturated zone, which allows the sampling of soil profiles with minimal disturbance to the sediment and the flow field. Scholars used a combination of field experiments, tracer studies, and numerical modeling to assess the applicability of Rhizon samplers for pore-water sampling. They demonstrated the utility of the RISS by sampling sediments in the Wadden Sea and validated that the Rhizon sampler is a promising form in in-situ pore-water sampling, as shown in Figures 12A-1–A-3. Zhejiang University designed a multi-depth in-situ pore-water pressure-retaining sampler (MIPPS) based on the Rhizon sampler, which can sample 24 different pore-water pressure-retaining samples with a depth resolution of 2 cm (Wang et al., 2022). Eventually, the sampler was applied in the South China Sea, as shown in Figures 12B-1, B-2. The Rhizon sampler can sample the pore-water in centimeter layers on the depth sequence. The in-situ pore-water sampler still relies on the submarine to carry and deploy, which is a high economic cost. Hence, the Rhizon sampler is seldom used for in-situ sampling. Most researchers still recover the deep-sea columnar sediment and then use the Rhizon sampler for secondary sampling (Miller et al., 2014; Steiner et al., 2018; Anschutz and Charbonnier, 2021). In 2021, scientists at Boston University designed a mass spectrometry-based pore-water sampling system for sandy sediments, in which sampling probes consist of PEEK tubes with POREX filters (Chua et al., 2021). The rotary valve can switch to seven individual probes, and a peristaltic pump extracts pore-water from the probes. However, this sampler mentioned above can only be used in offshore environments due to the limitation of the pump.
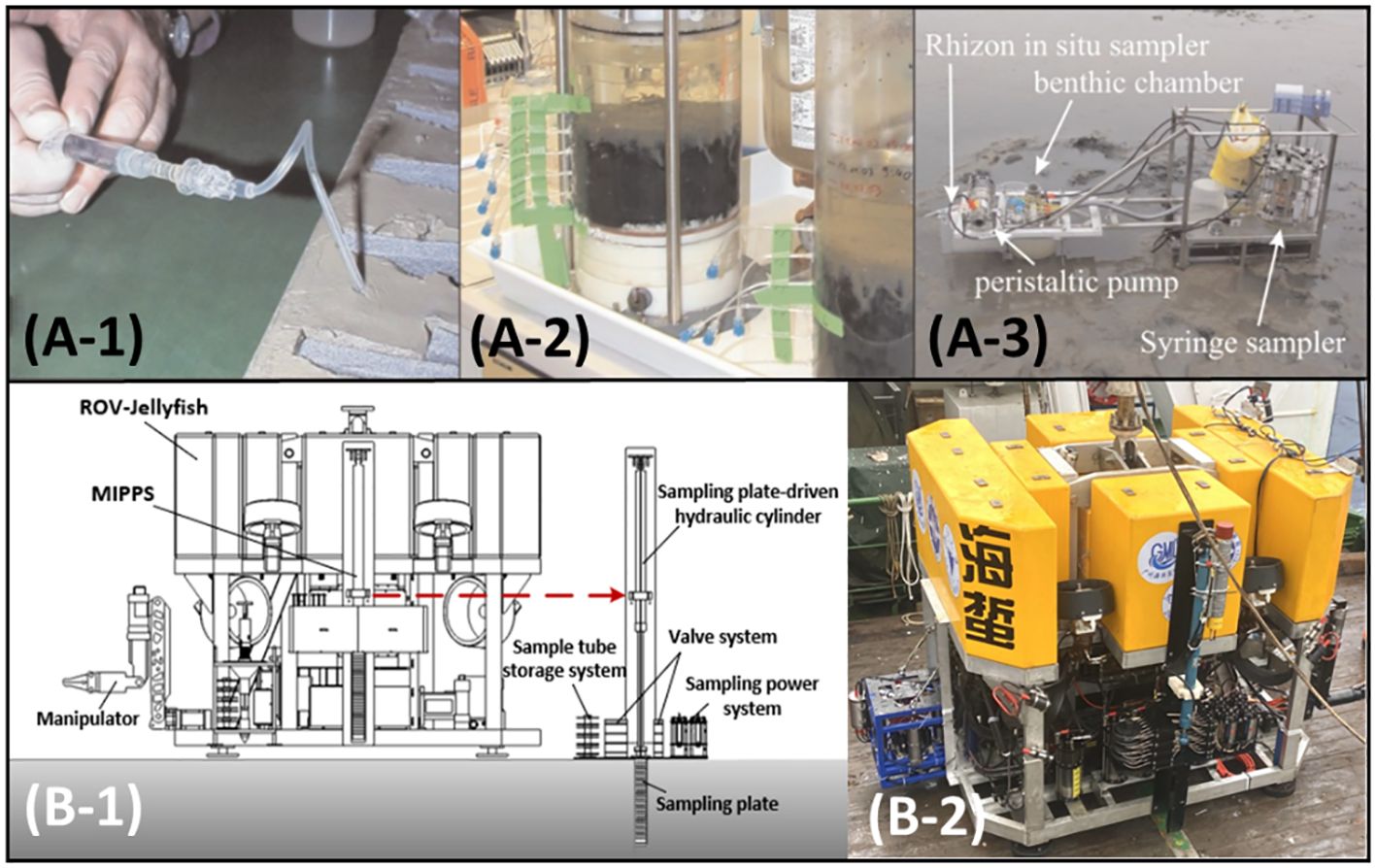
Figure 12 (A) Schematic of the Rhizon sampler to obtain pore water (Seeberg-Elverfeldt et al., 2005); (B) Schematic and application of the MIPPS (Wang et al., 2022).
2.3 Comparison of sampling systems for marine water resources
After much research and analysis, we summarize the technical parameters of main samplers in developing marine water resources sampling technology. Table 2 shows a comparison table of technical indexes of samplers for marine water resources (including seawater and pore-water). According to Table 2, the sampling object, sampling depth, sampling speed and quantity are the more important technical parameters in the sampling technology of marine water resources. When designing the sampler to meet the above indexes, we should consider how to simplify the structure of the sampler as much as possible. For example, in order to collect pressure-retaining samples with higher depth, we can reduce the number of samples appropriately and use Jeff, WHATS, etc, in monitoring the temporal and spatial changes of the marine environment and the need to obtain a larger number of samples, reduce the pressure-retaining capacity requirements appropriately and use OsmoSampler, ANEMONE-11, etc.
The following conclusions can be obtained through comparative analysis: (1) Seawater and pore-water sampling methods based on the negative pressure form are similar, and the sampling is mainly carried out by connecting the power element through the storage device. When samples must be prefiltered, adding a prefiltering element at the front of the power element is necessary. As shown in Figure 13, the pre-filtration element (including filter membrane, cartridge, Rhizon sampler, etc.) is used to filter impurities in marine water and large soil particles in sediment, and the size and pore size of the filter can be selected according to the need. Power elements (including vacuum tubes, syringes, peristaltic pumps, etc.) provide power for sampling. Although the principle of marine water resource sampling based on negative pressure form is the same, very few samplers can simultaneously be oriented to in-situ seawater and pore-water sampling. (2) In the deep-sea marine water resources sampling technology, pressure-balanced piston and piston suction sampling methods are the main sampling means of pressure-retaining sampling. (3) With the development of science and technology, the number of samples and the sampling time resolution have gradually become more important technical parameters of the marine water sampler. Ensuring the compactness, low power consumption, and economy of the sampler while sampling at high resolution is a problem that needs to be solved at this stage. (4) Researchers are increasingly concerned about the purity and cleanliness of the samples, such as the pollution of the sampler material to the environment, the disruption of the environment during sampling and so on.
3 Some reflections
3.1 Challenges in sampling techniques for marine water resources
3.1.1 Making in situ marine water resource samples more reliable
In the current research on marine water resource sampling, the most important concern is the authenticity and reliability of the samples obtained. Many factors make the samples distorted, such as changes in the temperature and pressure of the marine environment during sampling, changes in the properties of the samples due to poor sampling time, pollution and the disturbance of the sampler to the environment, etc., which leads to the distortion of the sample (in spatial, temporal or other aspects). In order to ensure the rigor of scientific research, we must use effective means to ensure the authenticity of the samples.
The sampler -”Jeff” was designed with heat and pressure preservation sampling methods to ensure the air tightness of the sample chamber during sample and storage to avoid the escape of soluble gases in the samples caused by environmental factors and the distortion of the samples. Researchers at Zhejiang University used CFD simulation to analyze the effects of different sample chamber structures on the seawater to replace the pure water to optimize the sample chambers. Through the numerical simulation, Seeberg-Elverfeldt et al. analyzed the sampling resolution in different depth layers during the Rhizon sampler sampling to improve spatial accuracy. Moreover, scientists at the Australian Cooperative Research Center designed and processed the sampler with clean materials to avoid contamination of samples by the materials.
In order to obtain and improve the quality of samples, the marine water resource sampling technology still needs to be upgraded further. For example, marine water resource sampling technology should be enhanced in pressure-retaining, temperature-retaining and material selection. Furthermore, the sampler should be simple in structure and light in weight while considering low pollution characteristics. It is also necessary to carry out in-depth theoretical and experimental research, such as the effect of environmental perturbations during sampling on the spatial and temporal resolution of samples, the diffusion and perturbation of the sample replacement process, etc. Thus, the design of the new samplers is optimized based on theoretical studies.
3.1.2 Enriched sampling functions for marine water resources
According to the literature analysis, with the development of the ocean, the application of samplers is also gradually complex, and the technical difficulty of sampling deep-sea marine water resources is increasing. For example, the osmotic pump sampler gradually moved from seawater to deep-sea pore-water collection, and deep-sea sediment pore-water in-situ gas-tight sampler evolved to sample both seawater and pore-water at the same time, and the number of samples taken by seawater sampler based on pump suction had grown from a dozen to hundreds.
With the application of samplers to the deep and distant sea, the new sampling technology will also face some bottlenecks. When an osmotic pump sampler samples pore-water, sediment particles gradually clog the sampler inlet in the complex sediment environment, unlike the simple seawater environment. It can lead to problems such as sampling failures or sampling rates becoming unstable due to environmental changes, leading to errors in the temporal resolution of the samples that are difficult to predict and resolve promptly. In addition, for seawater sampler based on pump suction, the stability of the dynamic sealing characteristics of the switching valve and the power consumption of the power supply system for obtaining more samples in the deep sea environment are also difficult problems that should be explored further.
3.2 Fresh ideas: how to promote technology development and industrialization
3.2.1 Integration with new ocean observation technologies
In order to obtain more comprehensive data on marine water resources and to compare the accuracy of samples taken by samplers, samplers can be used in integrated applications with sensors. Used in conjunction, they can provide more comprehensive and accurate ocean data, providing more information and insight for scientific research and environmental monitoring. For example, the ANEMONE-11 can carry a CTD to acquire real-time temperature, salinity and depth data. ANEMONE-11 can collect seawater samples for subsequent laboratory analysis and testing. Combining the two applications provides more comprehensive and accurate ocean information, providing insight into the ocean’s changing patterns and the ecosystem’s structure and function. Seawater samples can also be compared with sensor data to ensure the reliability and accuracy of the data. In addition, through the combination of sensor and seawater sample data, data on different time and spatial scales can be obtained. It is important for understanding marine ecosystems, biodiversity and ecological functions.
Therefore, marine water samplers can be equipped with deep-sea in situ observing systems for more complex studies to obtain comprehensive long-term, multi-parameter marine environmental data. Samplers can be deployed in specific marine areas for long-term marine environmental monitoring. Data obtained from physical, chemical and biological sensors in the in situ observing network can be compared and analyzed with in situ marine water samples for a more comprehensive understanding of the data. It has important implications for the study of seasonal and inter-annual variability.
3.2.2 Promoting diversification and commercialization of application scenarios
At the present stage, the development and application of marine water resource sampling technology is mostly limited to universities and research institutes, and few commercial marine water samplers have been put into marine environmental observation. We should emphasize the commercial development of marine water samplers, understand the different needs of people’s livelihood and environmental protection, and expand the application areas and scope of the application of water samplers, such as sewage discharge monitoring in the city, environmental pollution in the shallow sea, and monitoring different geological environments in the deep sea. A wide range of applications can enable researchers to understand users’ needs fully and clarify the difficulties that must be solved in developing the marine water resources sampling technology.
4 Conclusions and prospects
In summary, marine water resources (including seawater and pore-water) sampling technology has been developed to a great extent from the beginning to the present, and part of the sampler has been widely used in the fields of marine engineering, deep-sea ecology, deep-sea geoscience research and so on. The traditional sampler based on penetration form is still used in seawater sampling technology due to its simple operation, stable work, and other advantages. In order to obtain high-quality samples, researchers can make a targeted selection of samplers based on negative pressure forms. For example, it is possible to obtain pressure-retaining samples using power springs and vacuum chambers. However, the large disadvantage makes the sampler difficult to assemble and troublesome to deploy when more samples are needed. With the pump suction, tens to hundreds of samples can be collected using the switching valve, but the rotary valve requires a high switching accuracy. Pump suction can also be integrated through several mini-peristaltic pumps to collect samples. The pump suction sampler is small and simple but difficult to apply to the deep-sea environment to ensure the motor can be manipulated. Sampling using osmotic pumps is relatively energy-efficient and environmentally friendly, but its slow flow rate is only suitable for collecting seawater on a time scale. The osmotic pump is also used in the in-situ pore-water sampling. Like seawater sampling, it is more suitable for sampling on a time scale. In addition, due to the unique membrane structure of the Rhizon sampler, it is increasingly being used for in-situ sampling, and more accurate samples can be obtained at depth profiles.
At the present stage, the marine water resources sampler has been developed more completely, and researchers can choose the appropriate sampling technology according to their needs (time scale or space scale, etc.). The above analysis shows that seawater and pore-water sampling principles are similar. However, samplers are mostly limited to sampling a single type of marine water resource (seawater or pore-water). The subsequent development of marine water resources sampling technology can be considered to sample in-situ seawater and pore-water simultaneously and obtain high temporal and spatial resolution samples to broaden the application scene. In the deep sea environment, the samplers are carried by ROVs and other submersibles for power supply, deployment and recovery. The sampling time will be subject to certain limitations. Hence, developing samplers that can be independently deployed is also necessary. In addition, the marine water resources sampler design and development process also needs to consider the sampler weight and volume, sampling costs, material impact on the environment and other issues. To overcome the above problems, scientific research and technological innovation need to be strengthened, promoting the development and application of marine water resources. Sampling technology plays an important role in environmental protection and sustainable development.
Author contributions
YW: Conceptualization, Data curation, Writing – original draft. JC: Conceptualization, Funding acquisition, Writing – review & editing. JG: Data curation, Visualization, Writing – review & editing. ZY: Data curation, Writing – review & editing. YL: Resources, Supervision, Writing – review & editing. YHW: Funding acquisition, Supervision, Writing – review & editing.
Funding
The author(s) declare financial support was received for the research, authorship, and/or publication of this article. This study was supported by the National Key R&D Program of China (No. 2022YFC2803903) and the Finance Science and Technology Project of Hainan Province (Grant No. ZDKJ202019).
Conflict of interest
The authors declare that the research was conducted in the absence of any commercial or financial relationships that could be construed as a potential conflict of interest.
Publisher’s note
All claims expressed in this article are solely those of the authors and do not necessarily represent those of their affiliated organizations, or those of the publisher, the editors and the reviewers. Any product that may be evaluated in this article, or claim that may be made by its manufacturer, is not guaranteed or endorsed by the publisher.
References
Anschutz P., Charbonnier C. (2021). Sampling pore water at a centimeter resolution in sandy permeable sediments of lakes, streams, and coastal zones. Limnol. Oceanogr.: Methods 19, 96–114. doi: 10.1002/lom3.10408
Barnes R. O. (1973). An in situ interstitial water sampler for use in unconsolidated sediments. Deep-Sea. Res. Oceanogr. Abstr. 20, 1125–1128. doi: 10.1016/0011-7471(73)90026-0
Bender M., Martin W., Hess J., Sayles F., Ball L., Lambert C. (1987). A whole-core squeezer for interfacial pore-water sampling. Limnol. Oceanogr. 32, 1214–1225. doi: 10.4319/lo.1987.32.6.1214
Bianchi A., Garcin J., Tholosan O. (1999). A high-pressure serial sampler to measure microbial activity in the deep sea. Deep-Sea. Res. Part I. Oceanogr. Res. Pap. 46, 2129–2142. doi: 10.1016/s0967-0637(99)00039-4
Bird L. E., Sherman A., Ryan J. (2007). “Development of an active, large volume, discrete seawater sampler for autonomous underwater vehicles,” in OCEANS 2007 MTS/IEEE Vancouver (Vancouver, Canada: IEEE), 1–5. doi: 10.1109/oceans.2007.4449303
Breier J. A., et al (2014). A large volume particulate and water multi-sampler with in situ preservation for microbial and biogeochemical studies. Deep-Sea. Res. Part I. Oceanogr. Res. Pap. 94, 195–206. doi: 10.1016/j.dsr.2014.08.008
Breier J. A., Rauch C. G., McCartney K., Toner B. M., Fakra S. C., White S. N., et al. (2009). A suspended-particle rosette multi-sampler for discrete biogeochemical sampling in low-particle-density waters. Deep-Sea. Res. Part I. Oceanogr. Res. Pap. 56, 1579–1589. doi: 10.1016/j.dsr.2009.04.005
Carignan R., Rapin F., Tessier U. A. (1985). Sediment pore-water sampling for metal analysis: A comparison of techniques. Geochim. Cosmochim. Acta 49, 2493–2497. doi: 10.1016/0016-7037(85)90248-0
Chapin T. P., Caffrey J. M., Jannasch H. W., Coletti L. J., Haskins J. C., Johnson K. S. (2004). Nitrate sources and sinks in Elkhorn Slough, California: Results from long-term continuous in situ nitrate analyzers. Estuaries 27, 882–894. doi: 10.1007/bf02912049
Chapin T. P., Jannasch H. W., Johnson K. S. (2002). In situ osmotic analyzer for the year-long continuous determination of Fe in hydrothermal systems. Anal. Chim. Acta 463, 265–274. doi: 10.1016/s0003-2670(02)00423-3
Chen D., others (2009). An in situ gas-tight pore water sampler for deep-sea sediments. Mar. Geol. Quat. Geol. 29, 145–148. doi: 10.3724/SP.J.1140.2009.06145
Chen Y., Wu S., Xie Y., Yang C., Zhang J. (2007). A novel mechanical gas-tight sampler for hydrothermal fluids. IEEE J. Oceanic. Eng. 32, 603–608. doi: 10.1109/joe.2007.891887
Chua E. J., Short R. T., Cardenas-Valencia A. M., Savidge W., Fulweiler R. W. (2021). A mass spectrometer-based pore-water sampling system for sandy sediments. Limnol. Oceanogr.: Methods 19, 769–784. doi: 10.1002/lom3.10460
Connelly D. P., others (2012). Hydrothermal vent fields and chemosynthetic biota on the world’s deepest seafloor spreading centre. Nat. Commun. 3, 620. doi: 10.1038/ncomms1636
Crompton T. R. (2006). Analysis of seawater: A guide for the analytical and environmental chemist (Leipzig, Germany: Springer Science and Business Media). doi: 10.1007/b138036
Cunliffe M., others (2013). Sea surface microlayers: A unified physicochemical and biological perspective of the air-ocean interface. Prog. Oceanogr. 109, 104–116. doi: 10.1016/j.pocean.2012.08.004
Da Ros Z., others (2019). The deep sea: the new frontier for ecological restoration. Mar. Policy 108, 103642. doi: 10.1016/j.marpol.2019.103642
Dickens G. R., others (2007). Rhizon sampling of pore waters on scientific drilling expeditions: an example from the IODP Expedition 302, Arctic Coring Expedition (ACEX). Sci. Drill. 4, 22–25. doi: 10.5194/sd-4-22-2007
Di Meo C. A., Wakefield J. R., Cary S. C. (1999). A new device for sampling small volumes of water from marine micro-environments. Deep-Sea. Res. Part I. Oceanogr. Res. Pap. 46, 1279–1287. doi: 10.1016/s0967-0637(99)00002-3
Gkritzalis-Papadopoulos A., Palmer M. R., Mowlem M. C. (2012). Adaptation of an osmotically pumped continuous in situ water sampler for application in riverine environments. Environ. Sci. Technol. 46, 7293–7300. doi: 10.1021/es300226y
Han J., Tao J., Sheng Y., Luo G. (2009). Automatic core water sampler based on Microcontroller. Mech. Electr. Eng. Mag. 26, 37–40.
Huang H., others (2012). Novel gas-tight multi-sampler for discrete deep-sea water. Chin. J. Mech. Eng. 25, 722–728. doi: 10.3901/cjme.2012.04.722
Jamieson A. (2015). The hadal zone: life in the deepest oceans (Cambridge, United Kingdom: Cambridge University Press).
Jannasch H. W., Johnson K. S., Sakamoto C. M. (1994). Submersible, osmotically pumped analyzer for continuous determination of nitrate in situ. Anal. Chem. 66, 3352–3361. doi: 10.1021/ac00092a011
Jannasch H. W., Wheat C. G., Plant J. N., Kastner M., Stakes D. S. (2004). Continuous chemical monitoring with osmotically pumped water samplers: OsmoSampler design and applications. Limnol. Oceanogr.: Methods 2, 102–113. doi: 10.4319/lom.2004.2.102
Jones M. T. , others (2015). Monitoring of jökulhlaups and element fluxes in proglacial Icelandic rivers using osmotic samplers. J. Volcanol. Geotherm. Res. 291, 112–124. doi: 10.1016/j.jvolgeores.2014.12.018
Judd N. L., others (2022). Collection and use of pore-water data from sediment bioassay studies for understanding exposure to bioavailable metals. Integr. Environ. Assess. Manage. 18, 1321–1334. doi: 10.1002/ieam.4537
Karydis M., Kitsiou D. (2013). Marine water quality monitoring: A review. Mar. pollut. Bull. 77, 23–36. doi: 10.1016/j.marpolbul.2013.09.012
Kastner M., Jannasch H., Weinstein Y., Martin J. (2000). “A new sampler for monitoring fluid and chemical fluxes in hydrologically active submarine environments,” in OCEANS 2000 MTS/IEEE Providence (Providence, United States of America: IEEE), 109–112. doi: 10.1109/oceans.2000.881242
Lin K., others (2019). A novel active sampler coupling osmotic pump and solid phase extraction for in situ sampling of organic pollutants in surface water. Environ. Sci. Technol. 53, 2579–2585. doi: 10.1021/acs.est.8b03760.s001
Liu G. H., et al. (2018). Design improvement and sea test about in situ pore water sampler system for deep-sea sediments. Adv. Geosci. 8, 141–151. doi: 10.12677/ag.2018.81015
Martin J. B., Thomas R. G., Hartl K. M. (2004). An inexpensive, automatic, submersible water sampler. Limnol. Oceanogr.: Methods 2, 398–405. doi: 10.4319/lom.2004.2.398
Matos C. R., Berrêdo J. F., MaChado W., Metzger E., Sanders C. J., Faial K. C., et al. (2022). Seasonal changes in metal and nutrient fluxes across the sediment-water interface in tropical mangrove creeks in the Amazon region. Appl. Geochem. 138, 105217. doi: 10.1016/j.apgeochem.2022.105217
Miller M. D., Adkins J. F., Hodell D. A. (2014). Rhizon sampler alteration of deep ocean sediment interstitial water samples, as indicated by chloride concentration and oxygen and hydrogen isotopes. Geochem. Geophys. Geosyst. 15, 2401–2413. doi: 10.1002/2014gc005308
Miyazaki J., others (2017). WHATS-3: an improved flow-through multi-bottle fluid sampler for deep-sea geofluid research. Front. Earth Sci. 5. doi: 10.3389/feart.2017.00045
Montgomery J. R., Price M. T., Holt J., Zimmermann C. (1981). A close-interval sampler for collection of sediment pore waters for nutrient analyses. Estuaries 4, 75–77. doi: 10.2307/1351545
Mucciarone D. A., DeJong H. B., Dunbar R. B., Takeshita Y., Albright R., Mertz K. (2021). Autonomous submersible multiport water sampler. HardwareX 9, e00197. doi: 10.1016/j.ohx.2021.e00197
Naraoka H., Naito T., Yamanaka T., Tsunogai U., Fujikura K. (2008). A multi-isotope study of deep-sea mussels at three different hydrothermal vent sites in the northwestern Pacific. Chem. Geol. 255, 25–32. doi: 10.1016/j.chemgeo.2008.05.015
Niskin S. J. (1962). A water sampler for microbiological studies. Deep-Sea. Res. Oceanogr. Abstr. 9, 501–503. doi: 10.1016/0011-7471(64)90126-3
Okamura K., others (2013). Development of a 128-channel multi-water-sampling system for underwater platforms and its application to chemical and biological monitoring. Methods Oceanogr. 8, 75–90. doi: 10.1016/j.mio.2014.02.001
Owari S., Tomaru H., Matsumoto R. (2019). Long-term, continuous OsmoSampler results for interstitial waters from an active gas venting site at a shallow gas hydrate field, Umitaka Spur, eastern margin of the Japan Sea. Appl. Geochem. 104, 25–32. doi: 10.1016/j.apgeochem.2019.03.011
Pennington J. T., Blum M., Chavez F. P. (2016). Seawater sampling by an autonomous underwater vehicle: “Gulper” sample validation for nitrate, chlorophyll, phytoplankton, and primary production. Limnol. Oceanogr.: Methods 14, 14–23. doi: 10.1002/lom3.10065
Saegusa S., Tsunogai U., Nakagawa F., Kaneko S. (2006). Development of a multibottle gas-tight fluid sampler WHATS II for Japanese submersibles/ROVs. Geofluids 6, 234–240. doi: 10.1111/j.1468-8123.2006.00143.x
Sayles F. L., Mangelsdorf P. C. Jr., Wilson T. R. S., Hume D. N. (1976). A sampler for the in situ collection of marine sedimentary pore waters. Deep-Sea. Res. Oceanogr. Abstr. 23, 259–264. doi: 10.1016/0011-7471(76)91331-0
Seeberg-Elverfeldt J., Schlüter M., Feseker T., Kölling M. (2005). Rhizon sampling of pore-waters near the sediment-water interface of aquatic systems. Limnol. Oceanogr.: Methods 3, 361–371. doi: 10.4319/lom.2005.3.361
Seewald J. S., Doherty K. W., Hammar T. R., Liberatore S. P. (2002). A new gas-tight isobaric sampler for hydrothermal fluids. Deep-Sea. Res. Part I. Oceanogr. Res. Pap. 49, 189–196. doi: 10.1016/s0967-0637(01)00046-2
Steiner Z., Lazar B., Erez J., Turchyn A. V. (2018). Comparing Rhizon samplers and centrifugation for pore-water separation in studies of the marine carbonate system in sediments. Limnol. Oceanogr.: Methods 16, 828–839. doi: 10.1002/lom3.10286
Stock F., Kochleus C., Bänsch-Baltruschat B., Brennholt N., Reifferscheid G. (2019). Sampling techniques and preparation methods for microplastic analyses in the aquatic environment–A review. TrAC. Trends Anal. Chem. 113, 84–92. doi: 10.1016/j.trac.2019.01.014
Teasdale P. R., Batley G. E., Apte S. C., Webster I. T. (1995). Pore water sampling with sediment peepers. TrAC. Trends Anal. Chem. 14, 250–256. doi: 10.1016/0165-9936(95)91617-2
Tseng C. H., Tang S. L. (2014). Marine microbial metagenomics: from individual to the environment. Int. J. Mol. Sci. 15, 8878–8892. doi: 10.3390/ijms15058878
Tsunogai U., Toki T., Nakayama N., GAMO T. (2003). WHATS: a new multi-bottle gas-tight sampler for sea-floor vent fluids. Chikyukagaku (Geochem.) 37, 101. doi: 10.14934/chikyukagaku.37.101
van der Merwe P., Trull T. W., Goodwin T., Jansen P., Bowie A. (2019). The autonomous clean environmental (ACE) sampler: A trace-metal clean seawater sampler suitable for open-ocean time-series applications. Limnol. Oceanogr.: Methods 17, 490–504. doi: 10.1002/lom3.10327
Von Damm K. L., Buttermore L. G., Oosting S. E., Bray A. M., Fornari D. J., Lilley M. D., et al. (1997). Direct observation of the evolution of a seafloor’ black smoker’ from vapor to brine. Earth Planet. Sci. Lett. 149, 101–111. doi: 10.1016/s0012-821x(97)00059-9
Wang Y., Chen J., Guo J., Wang W., Fang Y., Ren X., et al. (2022). Design and study of a deep-sea multi-depth in-situ pore water pressure-retaining sampler. Mar. Georesour. Geotechnol. 41, 1370–1378. doi: 10.1080/1064119x.2022.2142175
Wang W., Wang J. (2018). Investigation of microplastics in aquatic environments: an overview of the methods used, from field sampling to laboratory analysis. TrAC. Trends Anal. Chem. 108, 195–202. doi: 10.1016/j.trac.2018.08.026
Warren B. A. (2008). Nansen-bottle stations at the Woods Hole Oceanographic Institution. Deep-Sea. Res. Part I. Oceanogr. Res. Pap. 55, 379–395. doi: 10.1016/j.dsr.2007.10.003
Wheat C. G., Jannasch H. W., Kastner M., Plant J. N., DeCarlo E. H. (2003). Seawater transport and reaction in upper oceanic basaltic basement: chemical data from continuous monitoring of sealed boreholes in a ridge flank environment. Earth Planet. Sci. Lett. 216, 549–564. doi: 10.1016/s0012-821x(03)00549-1
Wise D. E. (2009). Sampling techniques for sediment pore water in evaluation of reactive caping efficacy (Durham, United Kingdom: Univ. of New Hampshire).
Wu S. J., Yang C. J., Chen Y., Xie Y. Q. (2010). A study of the sealing performance of a new high-pressure cone valve for deep-sea gas-tight water samplers. J. Pressure Vessel. Technol. 132, 1–5. doi: 10.1115/1.4001204
Wu S. J., Yang C. J., Ding K., Tan C. Y. (2015). A remotely operated serial sampler for collecting gas-tight fluid samples. China Ocean. Eng. 29, 783–792. doi: 10.1007/s13344-015-0055-6
Wu S. J., Yang C. J., Huang H. C., Chen Y. (2014). Development of an electric control gas-tight sampler for seafloor hydrothermal fluids. J. Zhejiang. Univ. Sci. A. 15, 120–129. doi: 10.1631/jzus.a1300233
Wu S. J., Yang C. J., Pester N. J., Chen Y. (2011). A new hydraulically actuated titanium sampling valve for deep-sea hydrothermal fluid samplers. IEEE J. Oceanic. Eng. 36, 462–469. doi: 10.1109/joe.2011.2140410
Xu D., Wu W., Ding S., Sun Q., Zhang C. (2012). A high-resolution dialysis technique for rapid determination of dissolved reactive phosphate and ferrous iron in pore water of sediments. Sci. Total. Environ. 421, 245–252. doi: 10.1016/j.scitotenv.2012.01.062
Yu Y., Zhou D., Li Z., Zhu C. (2018). Advancement and challenges of microplastic pollution in the aquatic environment: a review. Water. Air. Soil pollut. 229, 1–18. doi: 10.1007/s11270-018-3788-z
Zhang Y., McEwen R. S., Ryan J. P., Bellingham J. G. (2009). “An adaptive triggering method for capturing peak samples in a thin phytoplankton layer by an autonomous underwater vehicle,” in OCEANS 2009 MTS/IEEE Biloxi (Biloxi, United States of America: IEEE), 1–5. doi: 10.23919/oceans.2009.5422436
Zhang Y., Ryan J. P., Bellingham J. G., Harvey J. B., McEwen R. S. (2012). Autonomous detection and sampling of water types and fronts in a coastal upwelling system by an autonomous underwater vehicle. Limnol. Oceanogr.: Methods 10, 934–951. doi: 10.4319/lom.2012.10.934
Keywords: marine water resources, seawater sampling techniques, pore-water sampling techniques, marine sampler development, deep-sea applications
Citation: Wang Y, Chen J, Guo J, Yu Z, Lin Y and Wang Y (2024) Advances and development in sampling techniques for marine water resources: a comprehensive review. Front. Mar. Sci. 11:1365019. doi: 10.3389/fmars.2024.1365019
Received: 03 January 2024; Accepted: 18 March 2024;
Published: 04 April 2024.
Edited by:
Toste Tanhua, Helmholtz Association of German Research Centres (HZ), GermanyReviewed by:
Jonathan McQuillan, University of Southampton, United KingdomNitin Agarwala, National Maritime Foundation, India
Copyright © 2024 Wang, Chen, Guo, Yu, Lin and Wang. This is an open-access article distributed under the terms of the Creative Commons Attribution License (CC BY). The use, distribution or reproduction in other forums is permitted, provided the original author(s) and the copyright owner(s) are credited and that the original publication in this journal is cited, in accordance with accepted academic practice. No use, distribution or reproduction is permitted which does not comply with these terms.
*Correspondence: Jiawang Chen, YXJ3YW5nQHpqdS5lZHUuY24=; Yuan Lin, c2FteWxpbkB6anUuZWR1LmNu