- 1Research Center for Oceanography, The Indonesian National Research and Innovation Agency, Jakarta, Indonesia
- 2Centre for Collaborative Research on Aquatic Ecosystem in Eastern Indonesia (Pusat Kolaboratif Riset Ekosistem Perairan Indonesia Timur), the Indonesian National Research and Innovation Agency, Ambon, Indonesia
- 3Department of Aquatic Resources Management, Study Program of Aquatic Resources Management, Faculty of Fisheries and Marine Sciences, Institut Pertanian Bogor (IPB) University, Bogor, Indonesia
- 4Chinese Academy of Sciences (CAS) Key Laboratory of Ocean Circulation and Waves, Institute of Oceanology, Chinese Academy of Sciences, Qingdao, China
- 5Jiaozhou Bay National Marine Ecosystem Research Station, Institute of Oceanology, Chinese Academy of Sciences, Qingdao, China
- 6University of Chinese Academy of Sciences, Beijing, China
The issue of plastic pollution in the marine environment is a matter of great concern. Our research presents pioneering findings on sub-surface microplastics in the Indonesian archipelagic waters. Data on microplastic presence in sub-surface water in the Indonesian Archipelagic Water is crucial for expanding our understanding of microplastic distribution from the surface to the bottom layers of the ocean, a research area that has been relatively overlooked. We discovered microplastic particles at 5 m depth below the surface through simultaneous pump method. The highest concentration of microplastics was discovered in Ambon, followed by North Java and North Sulawesi. In contrast, the lowest abundance of microplastics was detected in the Maluku Sea. These findings are consistent with prior studies establishing a relationship between human activity–as indicated by population density–and microplastic pollution. However, our results indicate that levels of microplastics in Ambon were significantly higher than those found in other sampled areas, particularly compared to North Java, which boasts the highest population density in Indonesia. This disparity is likely due to a faster seawater flushing rate and shorter water residence time in North Java relative to Ambon. The origins and pathways through which these microplastics are introduced into Indonesian archipelagic waters remain uncertain. Based on particle composition (52.73% fibers and 51.38% size <500 µm), it appears that they may result from either larger plastic items being broken down during transportation over long distances by prevailing currents from input from coastal areas or as a byproduct of local activities. Additional research is necessary to gain a comprehensive understanding of microplastic in water columns. This includes investigating the fate of microplastics and examining their impact on marine organisms within this treasured ecosystem. Moreover, it is important to develop methods for mapping the worldwide distribution of microplastics.
Introduction
Over the past twenty years, there has been an increasing interest and focus on studying and understanding the issue of litter, particularly in coastal and marine ecosystems. Among different types of waste materials, it is evident that plastic generates significant concerns due to its widespread use, with negative impacts on the health of ecosystems and the survival of wildlife (GESAMP, 2015). The presence of plastic pollution is a global concern, with its prevalence growing in various ecosystems worldwide (Law, 2017). With continuous mass production since the 1950s, plastics have become a commonly consumed material (Matsuguma et al., 2017). The production of plastic has significantly escalated, reaching approximately 8.3 billion metric tons and surpassing the growth rates of all other synthetic materials (Geyer et al., 2017). Recent data provided by PlasticsEurope and EPRO (2021) indicate that in 2021, approximately 367 million tonnes of plastic were produced, reflecting a considerable increase of 32 million tonnes since 2016. However, the increase in consumption has resulted in extensive environmental pollution, especially within marine environments (Knoblauch and Mederake, 2021; Meng et al., 2021; Cordova et al., 2021a). This is attributed mainly to inadequate waste management practices and overreliance on open-dumping systems for landfill disposal practices (Meidiana and Gamse, 2010; Demirbilek et al., 2013; Klemeš et al., 2020; Nurhasanah et al., 2021).
Extensive research has illustrated that the presence of plastic litter exerts a detrimental influence on the natural environment (Sheavly and Register, 2007; Lechner and Ramler, 2015; Watkins et al., 2015; Provencher et al., 2017; Williams and Rangel-Buitrago, 2019; Iskandar et al., 2021). In addition to the immediate risks of ingestion, plastic litter contributes to an increased potential for harmful substances to be released into the environment and enter the food chain (Rios et al., 2007; Moriwaki et al., 2009; Andrady, 2011; Bakir et al., 2014; Adyasari et al., 2021; Meng et al., 2021; Herrera et al., 2022). Due to the persistent nature of plastics, they have the ability to disperse over long distances and accumulate on remote shorelines and ocean floors (Barnes et al., 2009; Monteiro et al., 2018; Lavers et al., 2019; Courtene-Jones et al., 2020; Cunningham et al., 2020). When plastic enters the marine environment, it undergoes mechanical, chemical, and biological degradation processes, resulting in smaller particles known as microplastics (range size 1-5000 µm) (GESAMP, 2015; Wright and Kelly, 2017; Alimi et al., 2018). Microplastics can enter the ecosystem through various means, such as pellets, fibers, and powders used in plastic manufacturing, abrasive ingredients in personal care products, medications containing plastic components, and shedding synthetic clothing during washing (Browne et al., 2007; Driedger et al., 2015; Boucher and Friot, 2017; Napper et al., 2022).
Introducing microplastics into natural aquatic environments (Thompson et al., 2004; Rocha-Santos and Duarte, 2015; Vriend et al., 2021; Sulistyowati et al., 2022; Cordova et al., 2023) leads to the presence of these particles in various organisms, including microorganisms (Cole et al., 2014; Long et al., 2015; Kvale et al., 2020), plants (Yin et al., 2021; Yu et al., 2021; Huang et al., 2022a), fish (Collard et al., 2017; Steer et al., 2017; Lubis et al., 2019), mega-fauna (Germanov et al., 2018; Yong et al., 2021), mammals (Provencher et al., 2017; Omeyer et al., 2023), seabirds (Hardesty and Wilcox, 2011; Luna-Jorquera et al., 2019), and even food and drinks that humans consume (Kniggendorf et al., 2019; Toussaint et al., 2019; Diaz-Basantes et al., 2020). However, there is limited consideration given to the interconnectedness between research conducted on different environmental components (Monteiro et al., 2018). Moreover, the problem of microplastic pollution is likely to be especially severe in urban areas of archipelagic nations, where the coastal marine ecosystems are crucial for sustaining local livelihoods (FAO, 2018; Clifton et al., 2021). In these regions, waste management practices are often lacking or inadequately implemented (Mohee et al., 2015; Fuldauer et al., 2019; Weekes et al., 2021). Furthermore, recent migration trends in archipelagic countries driven by economic incentives may further exacerbate the issue by contributing to increased plastic and primary microplastic release into coastal waters (Lee, 2015; ten Brink et al., 2018).
Archipelagic states consist of islands that form a unified entity, with the islands and the surrounding waters considered internal territory (Baumert and Melchior, 2015). The country of Indonesia, as one of the largest archipelagic states with more than 1.9 million km2 and over 270 million people (Butcher et al., 2017; Rochwulaningsih et al., 2019), is faced with the issue of microplastic pollution in its surface water (Adyasari et al., 2021). The likelihood of microplastic presence in the marine waters of Indonesia is significant, particularly due to Indonesia’s ranking as one of the top five contributors to plastic waste entering the world’s oceans (Lebreton and Andrady, 2019; Meijer et al., 2021). Limited research on microplastics in sub-surface water areas has been conducted, with most studies focusing on surface water or sediments near the bottom (Van Sebille et al., 2015), leaving a significant knowledge gap regarding the abundance, dispersal and fate of microplastics throughout most of the ocean volume. Research on sub-surface microplastics remains uncommon, largely due to the vertical nature of this type of investigation, which spans from the surface to the bottom sediment. Despite this, there is a clear dearth of vertical research on sea microplastics. Such sub-surface research could provide valuable insights into the movement of microplastics from the surface to sediments in open sea areas. This is especially true in the case of Indonesia, which may serve as both a sink and a source of microplastics in global oceans. The one comprehensive survey conducted in the Arctic Central Basin (Kanhai et al., 2018) revealed that movement of microplastics from surface to deep ocean areas does occur, highlighting this as an surface to deep sea ocean area for further exploration. The limited research on microplastics in the sub-surface is also due to various methodologies. While most studies utilize simultaneous pumps, only a small minority use net sampling and water bottle sampling methods (see discussion section for details). The use of simultaneous pumps for sub-surface water research generates fewer samples compared to net sampling, but it can cover a larger area and be conducted while the ship is in motion. A vertical study of microplastics in China indicated that the abundance of microplastics in sub-surface water exceeds that found in surface and bottom waters (Wu et al., 2022). This suggests that sub-surface water serves as a temporary location when microplastics undergo resuspension processes in surface water and deposition processes in bottom water and sediment layers. Research investigating microplastics in sub-surface water offers additional information on the microplastics’ journey from the water’s surface to its depths. Furthermore, the Indonesian archipelagic water, which acts as a conduit for the significant flow of water between the Pacific and Indian Oceans, known as the Indonesian Throughflow (Sprintall et al., 2009), transports large volumes of water, along with its associated materials (Sprintall et al., 2019), including microplastic particles. Given these gaps in knowledge, it is crucial to conduct research studies globally focusing on microplastics below the surface (sub-surface) of oceans. Further research is needed to comprehensively understand the distribution and fate of microplastics in sub-surface waters. While research on sub-surface microplastics has been conducted in other archipelagic states like Fiji (Dehm et al., 2020) and regions such as the Arctic (Lusher et al., 2015), Atlantic (Lusher et al., 2014), and Pacific (Desforges et al., 2014) areas, no studies have focused on this topic specifically within Indonesian waters. Therefore, it is crucial to establish a baseline for understanding the distribution and concentration of microplastics in order to implement effective monitoring strategies for addressing this form of pollution within the Indonesian archipelago.
This study aimed to assess the occurrence and distribution of microplastics found in the sub-surface water surrounding the Indonesian archipelagic region. We also aimed to identify the most common types of shapes, sizes, and polymers present. Based on human impact, we hypothesized that areas with higher anthropogenic activities would exhibit a greater abundance of microplastics compared to those with lower levels of human activity. The findings from this investigation will contribute to a comprehensive report on microplastic contamination in Indonesia’s archipelagic water area, potentially enhancing efforts for managing and preventing such contamination within marine ecosystems.
Method
Sampling methods
Water samples were obtained during the Western Pacific Ocean System (WPOS) joint cruise between IOCAS-RCO Research Expedition (https://sims.qdio.ac.cn/joint3.aspx) on board RV Baruna Jaya VIII, which took place in September-October 2018. (Figure 1). The sampling process commenced upon departure from outer Jakarta Bay and extended towards the Sulawesi Sea (Sampling zone area please refer to Supplementary Material Table SM1).
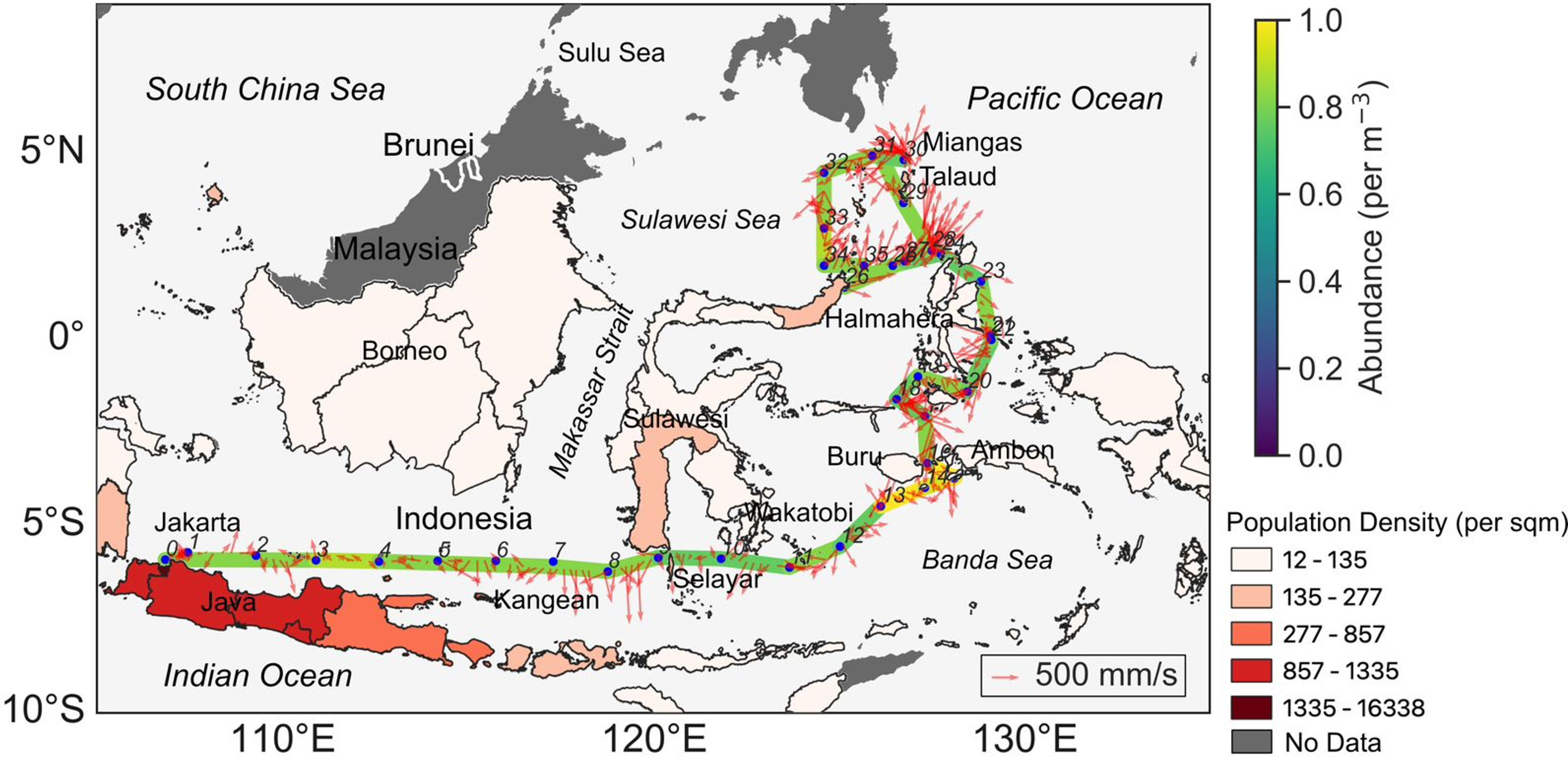
Figure 1 Microplastics abundance and ocean currents in sampling area in the Indonesian archipelagic water overlayed with population density (per square meter) in the island. Blue dots and its numbers represent the sampling locations and their stations. The red arrow indicates the speed of the sub-surface water current.
Seawater samples were collected from below the surface (sub-surface) in accordance with established protocols described in previous publications (Lusher et al., 2014; 2015). In a careful procedure, seawater from a depth of 5 meters was circulated using a seawater pump (DAB Multi 4 SW) through stainless steel pipes under low pressure conditions (2 Bar) to the vessel’s deck. The low-pressure conditions were used to prevent sample water overflow and to avoid damage to the microplastic. Briefly, the seawater pump was directly linked to a hose that transports the inflow from a stainless-steel pipe located 5 meters below the surface. The sampling depth was approximated using the ship’s draft and sea waves, resulting in an estimated depth of 5.01 ± 1.12 meters. The hose is then attached to a stainless-steel filtering compartment with a diameter of 5 inches and a height of 20 cm. Within this filtering compartment, there are dual-level sieves with mesh sizes of 5000 microns and 200 microns. Adapters with lids and handles are positioned at the top of these sieves for easier retrieval of filtered material (please see Supplementary Material Figure SM1 for sampling devices). The seawater pump and filtering procedure were operated during the vessel’s voyage from one sampling point to another. The seawater intake point was positioned in the middle, positioned slightly forward on the port side and opposite to the wastewater intake. Furthermore, it has been placed a considerable distance away from the bilge and float pump outlet to prevent any potential cross-contamination from the outlet. The measured volume of sub-surface water was determined using a flowmeter installed before the filtering compartment, along with manual calculations and calibration at each sampling point before any adjustments were made. While using the flowmeter for all sampling and filtration processes is preferred, damage occurred during the final sampling stage at the second sampling point. As a result, only manual calculation methods were used to determine the filtered water output from the filtering compartment for the following 33 sampling points. After filtering between 17-33 m3 over approximately 11-14 hours (please see Supplementary Materials Table SM1), each material collected within the sieve was carefully transferred into sterile jars with a capacity of 350 ml using appropriate tools such as tweezers, droppers, and glass spatulas. The material remaining in the sieve is rinsed with double distilled deionized water (DDDW). Throughout this transfer process, it was ensured that the sterile jars were not overfilled with water. For laboratory examination, the jars were sealed with ParaFilm® sealing film and stored at 4 ± 2°C for further analysis.
Sample treatment and microplastics identification
The microplastic extraction process from seawater samples was employed using a previously documented method involving high-density solvents for density separation and biological digestion (2017b; Lusher et al., 2014; GESAMP, 2019; Michida et al., 2019; Nurhasanah et al., 2021; Cordova et al., 2022b). Initially, filtered water samples were dried at 50°C in an oven (B-One OV-30) for 72-96 hours before being treated with a highly saturated NaCl solution (1.2 g cm-3). To mitigate potential extraction variation associated with the usage of NaCl (Li et al., 2019; Cutroneo et al., 2021), the separation process was repeated six times (please refer to QA/QC section for microplastic recovery test). Subsequently, the dried samples were placed in a sterile 50 ml Pyrex test tube and subjected to heating at 50°C in an oven for 48 hours. A Fenton reagent prepared from 30% H2O2 (20 ml, Merck Millipore, Emprove® Essential, Ph Eur, BP, USP) and Fe(II)SO4 (10 ml, 10 mg/ml, Merck Millipore, EMSURE® ACS, ISO, Reag. Ph Eur) was then added to the test tube, followed by further heating in a water bath (B-One DWBC-30L-6H) at 50°C throughout 48 to 72 hours. Finally, sterile gridded filter paper (Merck Whatman™ cellulose nitrate, sterile, diameter 47 mm and pore size 0.45 µm) was used to facilitate the identification and characterization examination of the obtained sample materials. The filter paper membrane was observed under a Leica M205C stereo microscope with a Leica IC90 E camera. Suspected microplastics were identified using established identification methods (Cole et al., 2013; Cordova et al., 2019), and their shape, size, and images were recorded promptly upon detection. The particle was classified based on criteria including consistent coloration without organic or cellular characteristics, as well as absence of segmentation (Crawford and Quinn, 2017; Shim et al., 2017; GESAMP, 2019). In our research, we categorized the microplastic particles we found into two shapes: fibers and fragments. We determined the size of the fiber shape based on the length of the fiber, while the size of the fragment shape was determined by its maximum dimension. The microplastics that were proportionally selected representative of each sampling station (30.09%, n = 99 out of the total of 329 recovered particles, please refer to the proportional selection of particle data in Supplementary Material Table SM1), underwent manual identification and were then analyzed using micro-Raman spectroscopy (Renishaw inVia™ Qontor® confocal μRaman microscope) to determine their functional groups. Sample composition proportions for analyzing the chemical makeup of microplastic particles can be found in Supplementary Material Table SM1. To identify the synthetic polymer using μRaman spectroscopy, we analyzed the most prominent bands in the vibrational spectrum based on established findings from prior research combined with a standard and advanced library provided by μRaman. The library data used has matching rates above 70%, which are considered alongside peak identification, based on the findings of Jin et al. (2022).
Quality assurance and quality control
Recovery experiments were conducted on a variety of commonly used polymers (PlasticsEurope and EPRO, 2021) to assess microplastic retrieval. Seven different types of polymers (size 400 µm to 1000 µm), including low-density and high-density polyethylene, polypropylene, polystyrene, polyvinyl chloride, polyamide, and polyurethane, were introduced into pure water (Milli-Q®) along with 5 mg/l Now Solution® Red Clay Powder. This concentration was chosen to closely mimic the average total suspended solid content in the Java and North Flores Seas (Tarigan and Edward, 2010; Suryantini et al., 2011; Rustiah et al., 2018). A method utilizing density separation using a highly saturated NaCl solution and a biological digestion procedure involving Fenton’s reagent was employed to achieve complete recovery. The overall recovery rate was determined to be 90.04% through three repetitions of the density separation process along with one iteration of the biological digestion treatment. In contrast, when six repetitions of the density separation process were conducted followed by one iteration of the biological digestion treatment, a 100% recovery rate was achieved.
Furthermore, several precautions were taken to ensure the sampling process’s integrity and minimize contamination. The hose in the seawater pump and sieve underwent thorough cleaning with seawater followed by DDDW before each subsequent sampling. Glassware was also rinsed with DDDW and then wrapped in aluminum foil. A blank sample approach was implemented to estimate potential contamination levels during the analysis and sampling processes. During sampling in each area (n=7), a procedural blank was conducted by placing 100 ml of distilled water in a sterile 300 ml glass beaker. The same procedure was also carried out during the laboratory’s microplastic extraction from sub-surface water (n=5). All 12 blank samples underwent the previously described sample treatment procedure. No microplastic contamination was detected in the blank samples. Additionally, to reduce any possible errors during sampling and analysis, we adhered to strict protocols such as wearing 100% cotton clothing and using glass laboratory supplies. All materials were promptly wrapped after treatments, while instruments were thoroughly sanitized prior to conducting laboratory analyses. Chemical solutions underwent filtration through sterile filter paper to eliminate any residual microparticles present.
Statistical analyses
The assessment of microplastic abundance and properties and the generation of graphical representations were performed using PAST software version 4.03. To streamline the statistical analysis, we divided the 35 sampling locations into seven areas: [1] Outer Jakarta - Kangean Island (7 sampling points), [2] Kangean Island - Selayar Island - Wakatobi Archipelago (4 sampling points), [3] Wakatobi Archipelago - South Buru Island - Outside Ambon Island (5 sampling points), [4] Lifamatora Passage (3 sampling points), [5] Halmahera Island - Talaud Island (6 sampling points), [6] Maluku Sea (4 sampling points), and [7] Talaud - Miangas Island - North Sulawesi (5 sampling points) (please see to Supplementary Material Table SM1 for more detail). By categorizing these areas accordingly, we aim to facilitate our understanding of their respective microplastic abundances in Indonesian Regional Fisheries Management (please see Supplementary Materials Table SM1). In descriptive statistics, we present both the mean ± standard error and the median ± standard deviation as results. In order to examine the correlation between the number of microplastics identified in different sampling regions, a non-parametric analysis (Kruskal-Wallis test followed by Dunn’s post hoc analysis) was conducted, following a non-normality indication from The Shapiro-Wilks test and The Anderson-Darling A test conducted on the data’s results. A significant level of 0.05 was applied to all statistical examinations undertaken.
Results
This study presents the initial comprehensive documentation of microplastics found in sub-surface layers up to a depth of 5 m within the Indonesian archipelagic water. To our understanding, no previous research has reported such findings. A comprehensive survey was conducted, sampling nearly 950,000 liters of sub-surface seawater. The survey encompassed a track length of nearly 5200 km across various regions in the Indonesian archipelagic waters, including the sea boundaries of the Java Sea, Flores Sea, Banda Sea, Seram Sea, Maluku Sea, and Sulawesi Sea.
Microplastic abundance
The presence of microplastics was observed consistently across all sampling intervals and throughout the entire study area. A total of 329 microplastic particles were found in the samples taken from 35 different sampled regions, ranging between 2 and 44 particles per sample. The abundance of microplastics in the sub-surface varied from 0.07 to 2.89 particles per m3, with a mean and standard error of 0.38 ± 0.08 particles per m3 and median and standard deviation of 0.27 ± 0.49 particles per m3.
The levels of microplastic abundance varied across sub-surface layers collected from Indonesian archipelagic water (Figures 2) spanning [1] Outer Jakarta - Kangean Island (mean and standard error of 0.48 ± 0.07 particles per m3 with median and standard deviation of 0.40 ± 0.18 particles per m3), [2] Kangean Island - Selayar Island - Wakatobi Archipelago (mean of 0.20 ± 0.06 particles per m3 and median of 0.12 ± 0.19 particles per m3), [3] Wakatobi Island - South Buru Island - Outside Ambon Island (mean of 1.03 ± 0.50 particles per m3 and median of 0.64 ± 1.12 particles per m3), [4] Lifamatora Passage (mean of 0.19 ± 0.04 particles per m3 and median of 0.16 ± 0.07 particles per m3), [5] Halmahera Island - Talaud Island (mean of 0.21 ± 0.03 particles per m3 and median of 0.20 ± 0.08 particles per m3), [6] Maluku Sea (mean of 0.16 ± 0.04 particles per m3 and median of 0.14 ± 0.08 particles per m3), and [7] Talaud - Miangas Island - North Sulawesi (mean of 0.30 ± 0.05 particles per m3 and median of 0.27 ± 0.12 particles per m3), respectively (Figures 2). Based on the statistical analysis using mean values and conducting a Kruskal-Wallis test followed by Dunn’s post hoc analysis (p < 0.05), it is evident that there is variation in microplastic abundance within sample across. The ranking from highest to lowest abundance is as follows: [3] > [1] > [7] > [5] ≈ [2] ≈ [4] > [6]. Sub-surface seawater in regions with high levels of human activity, e.g., Ambon (area [3]), North Java (area [1]), and North Sulawesi (area [7]), exhibits a substantially greater concentration (p < 0.05) of microplastics. The concentrations of microplastics in these regions are 4-10 times higher compared to areas with less human impact, e.g., area [5], [2], [4], and [6].
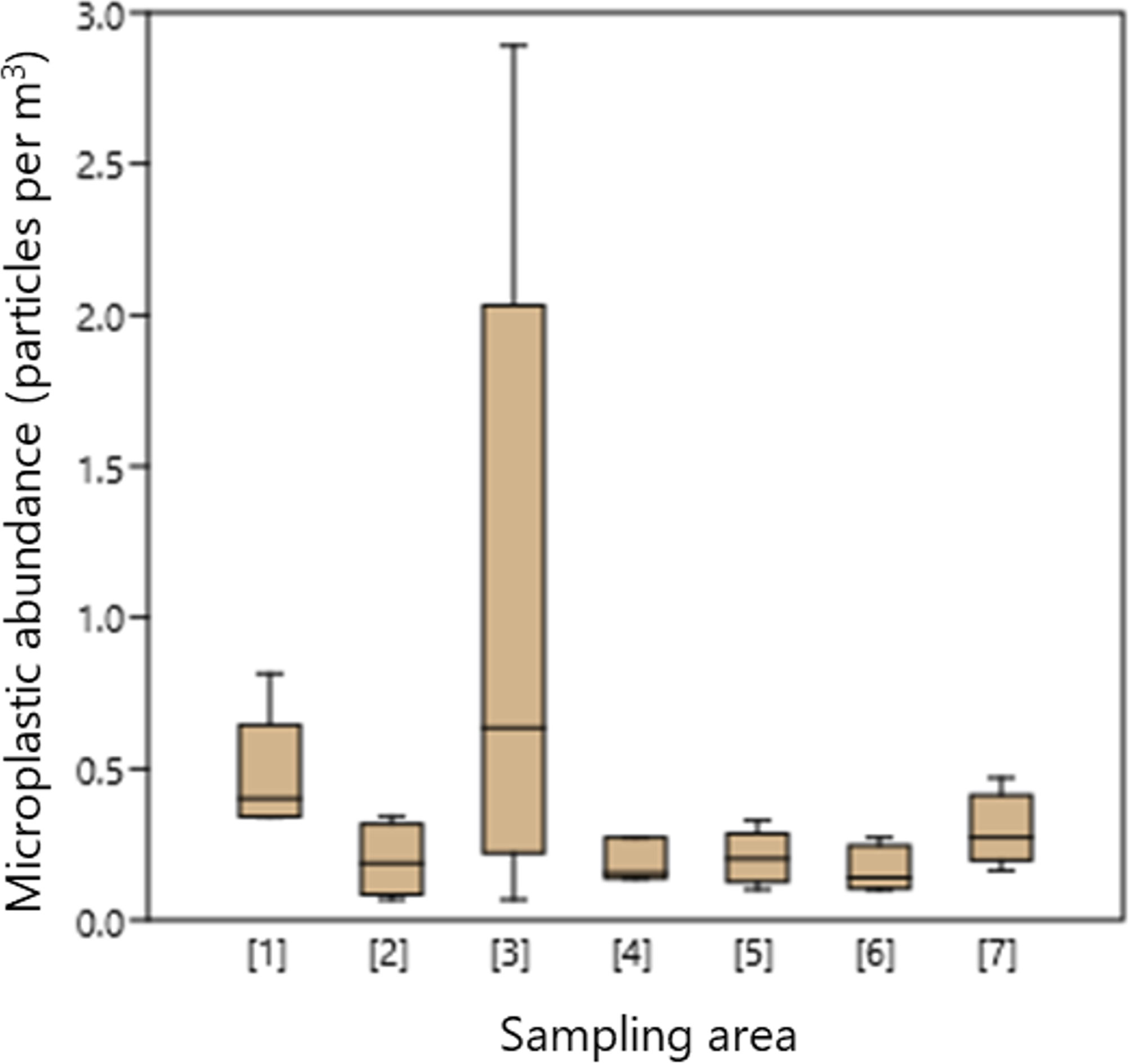
Figure 2 Microplastics abundance in each sampling area. [1] Outer Jakarta - Kangean Island (n=7), [2] Kangean Island - Selayar Island - Wakatobi Archipelago (n=4), [3] Wakatobi Archipelago - South Buru Island - Outside Ambon Island (n=5), [4] Lifamatora Passage (n=3), [5] Halmahera Island - Talaud Island (n=6), [6] Maluku Sea (n=4), and [7] Talaud - Miangas Island - North Sulawesi (n=5).
Microplastic characteristics
In each sampling area, microplastics were categorized into two shapes based on their morphological properties: fiber and fragment (Figures 3A–D). The percentage of fibers (52.73% with a mean and standard error of 0.17 ± 0.03 particles per m3 with median and standard deviation of 0.14 ± 0.16 particles per m3) was higher than that of fragments (47.27%, 0.21 ± 0.07 particles per m3 and median of 0.10 ± 0.40 particles per m3). Additionally, no significant differences were observed between fiber shapes and fragment shape of microplastics during this investigation (Kruskal-Wallis’s test, p=0.492). The distribution of microplastics in the sub-surface waters of the Indonesian Archipelagic Water shows interesting patterns (Figures 3D–F), mean abundance of fragment was larger than fibers due to outlier data in the Wakatobi - South Buru - outside Ambon (sampling area [3], Figure 3E). Specifically, two outliers were observed for fragment shape at sampling points 14 (0.96 particles per m3) and 16 (2.30 particles per m3). This particular sampling area ([3]) exhibited a dominant presence of fragment-shaped microplastics (67.01%) compared to other locations. Based on the sampling area analysis, it was found that sampling areas [3] and [1] had higher concentrations of fibers (mean of 0.31 ± 0.10 and 0.26 ± 0.09 particles per m3 and median of 0.34 ± 0.22 and 0.21 ± 0.24 particles per m3) and fragments (mean of 0.72 ± 0.43 and 0.22 ± 0.06 particles per m3 and median of 0.24 ± 0.96 and 0.20 ± 0.16), compared to other sampling areas examined in the study. Furthermore, statistical tests also did not show any significant differences in microplastic shapes (fiber and fragment) in each sampled area (Kruskal-Wallis’s test, p>0.05).
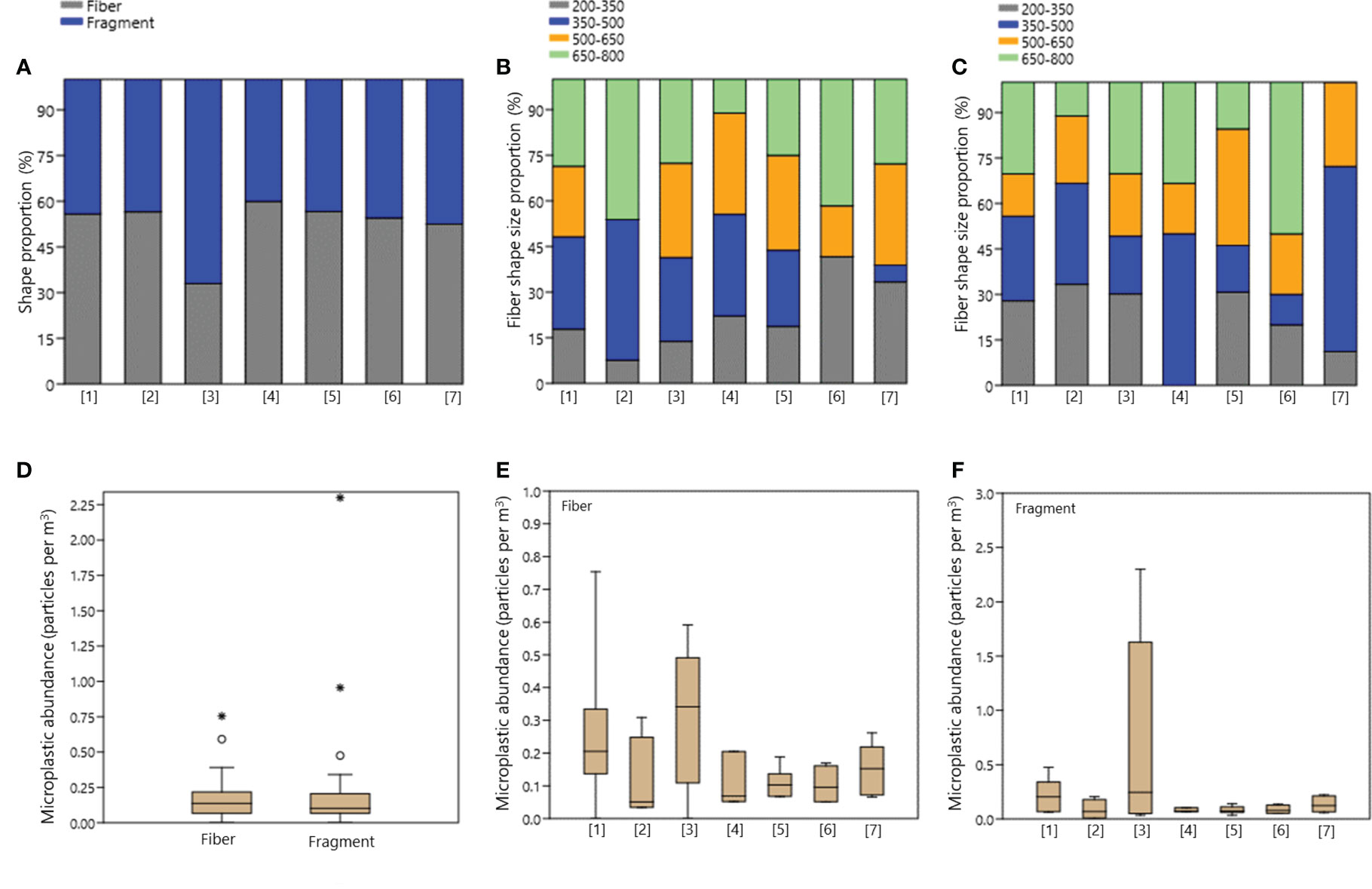
Figure 3 Sub-surface microplastics characteristics by shape. (A) Proportion for all shapes; (B) Fiber shape size proportion (size in µm); (C) Fragment shape size proportion (size in µm); (D) Microplastic abundance by shapes; (E) Fiber shape abundance in each sampling area; (F) Fragment shape abundance in each sampling area. Sampling area: [1] Outer Jakarta - Kangean Island, [2] Kangean Island - Selayar Island - Wakatobi Archipelago, [3] Wakatobi Archipelago - South Buru Island - Outside Ambon Island, [4] Lifamatora Passage, [5] Halmahera Island - Talaud Island, [6] Maluku Sea, and [7] Talaud - Miangas Island - North Sulawesi.
In this study, microplastics were detected in the size range of 201.4-796.9 µm (mean of 494.3 ± 9.97 µm with median of 493.20 ± 180.90 µm). With a fiber length range of 203.7-796.9 µm (mean of 500.64 ± 14.05 µm with median of 516.45 ± 176.03 µm) and a maximum fragment dimension range of 201.4-792.1 µm (mean of 488.31 ± 14.33 µm with median of 471.40 ± 185.72 µm). To analyze the distribution of these microplastic particles by size, we categorized them into four groups: 200-350 µm, 350-500 µm, 500-650 µm, and 650-800 µm (Figures 4A–F). Among these categories, the most commonly found microplastic sizes were between 650-800 µm (26.38%, mean of 0.10 ± 0.02 particles per m3 with median of 0.17 ± 0.14 particles per m3) and 350-500 µm (26.10%, mean of 0.10 ± 0.02 particles per m3 with median of 0.17 ± 0.14 particles per m3), followed by particles sized between ranges of 500–650 µm (22.24%, mean of 0.08 ± 0.02 particles per m3 with median of 0.05 ± 0.11 particles per m3) and 200–350 µm (21.59%, mean of 0.08 ± 0.02 particles per m3 with median of 0.07 ± 0.11 particles per m3), respectively (Figures 4C–F). Additionally, there were statistically significant differences in the size distribution of microplastics at different sampling locations. Specifically, a difference was found in the range of 350-500 µm between sampling areas [1] with [5] and [6]; sampling areas [3] and [4] with sampling area [6]; and sampling area [6] with [7] (Kruskal-Wallis’s test, p <0.05; Dunn’s Post Hoc, p<0.05, Figure 4E).
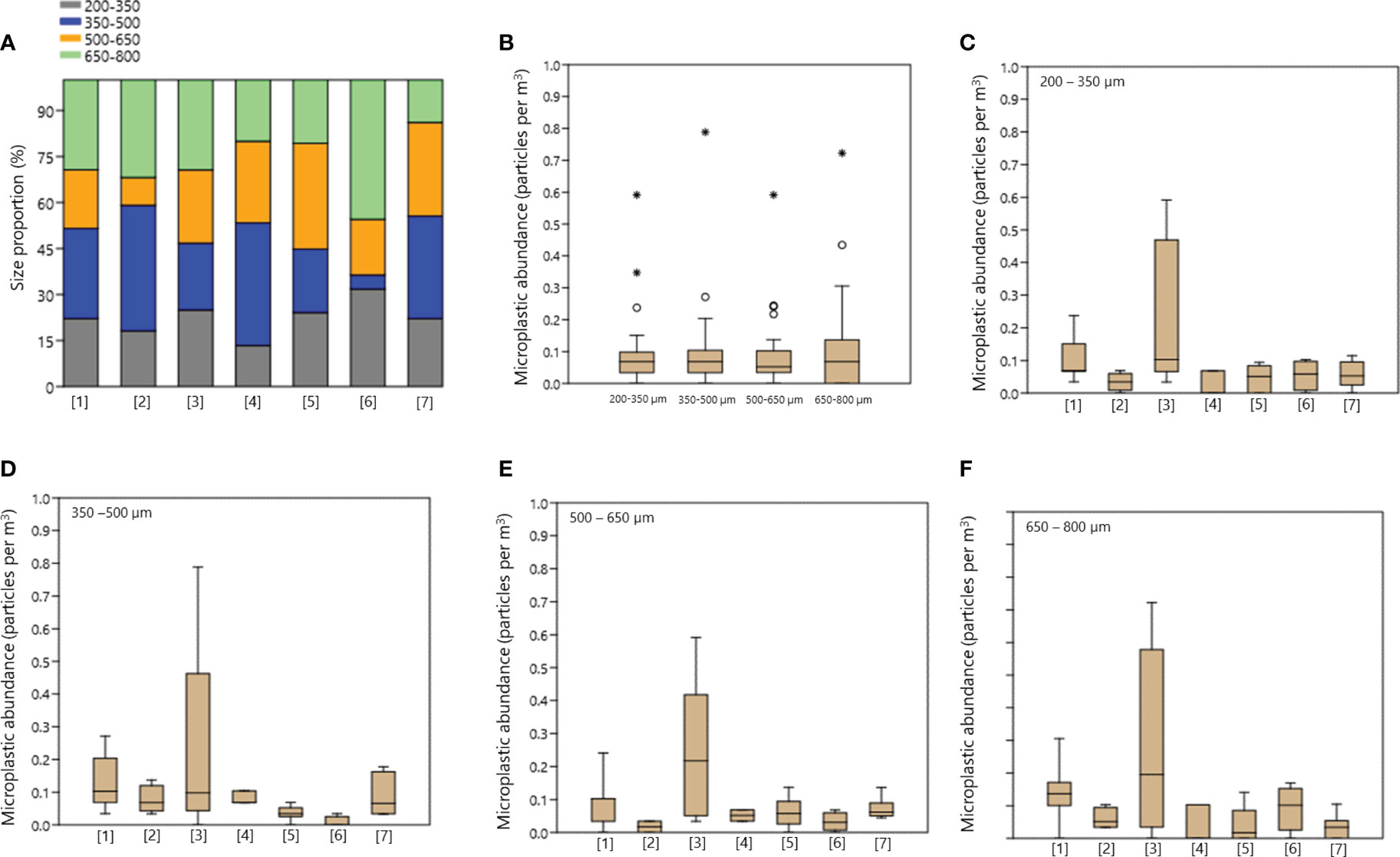
Figure 4 Sub-surface microplastics characteristics size. (A) Proportion for all size range (size in µm); (B) Microplastic abundance by size range; (C) Abundance of microplastics size 200-350 µm in each sampling area; (D) Abundance of microplastics size 350-500 µm in each sampling area; (E) Abundance of microplastics size 500-650 µm in each sampling area; (F) Abundance of microplastics size 650-800 µm in each sampling area. Sampling area: [1] Outer Jakarta - Kangean Island, [2] Kangean Island - Selayar Island - Wakatobi Archipelago, [3] Wakatobi Archipelago - South Buru Island - Outside Ambon Island, [4] Lifamatora Passage, [5] Halmahera Island - Talaud Island, [6] Maluku Sea, and [7] Talaud - Miangas Island - North Sulawesi.
Raman spectroscopy determined the chemical composition for 99 of the detected microplastic particles (30.09% of the total recovered particles). Chemical composition testing involves randomly and proportionally selecting particles in fiber and fragment shapes, with a 25-50% distribution at each station. More detailed data can be found in Supplementary Material Table SM1. All 99 particles were identified as being made of a synthetic polymer. We identified eight different forms of microplastic polymers (Figure 5, Table 1). polypropylene (20.20%), polyethylene (19.19%), nylon 6 and 9 (15.15%), and polyethylene terephthalate (15.15%) dominated the chemical composition analyses, accounting for 69.70% of total microplastics. The remaining polymers (30.30%) included cellophane (10.10%), polyurethanes (8.08%), polybutadiene (8.08%) and polyvinyl chloride (4.04%).
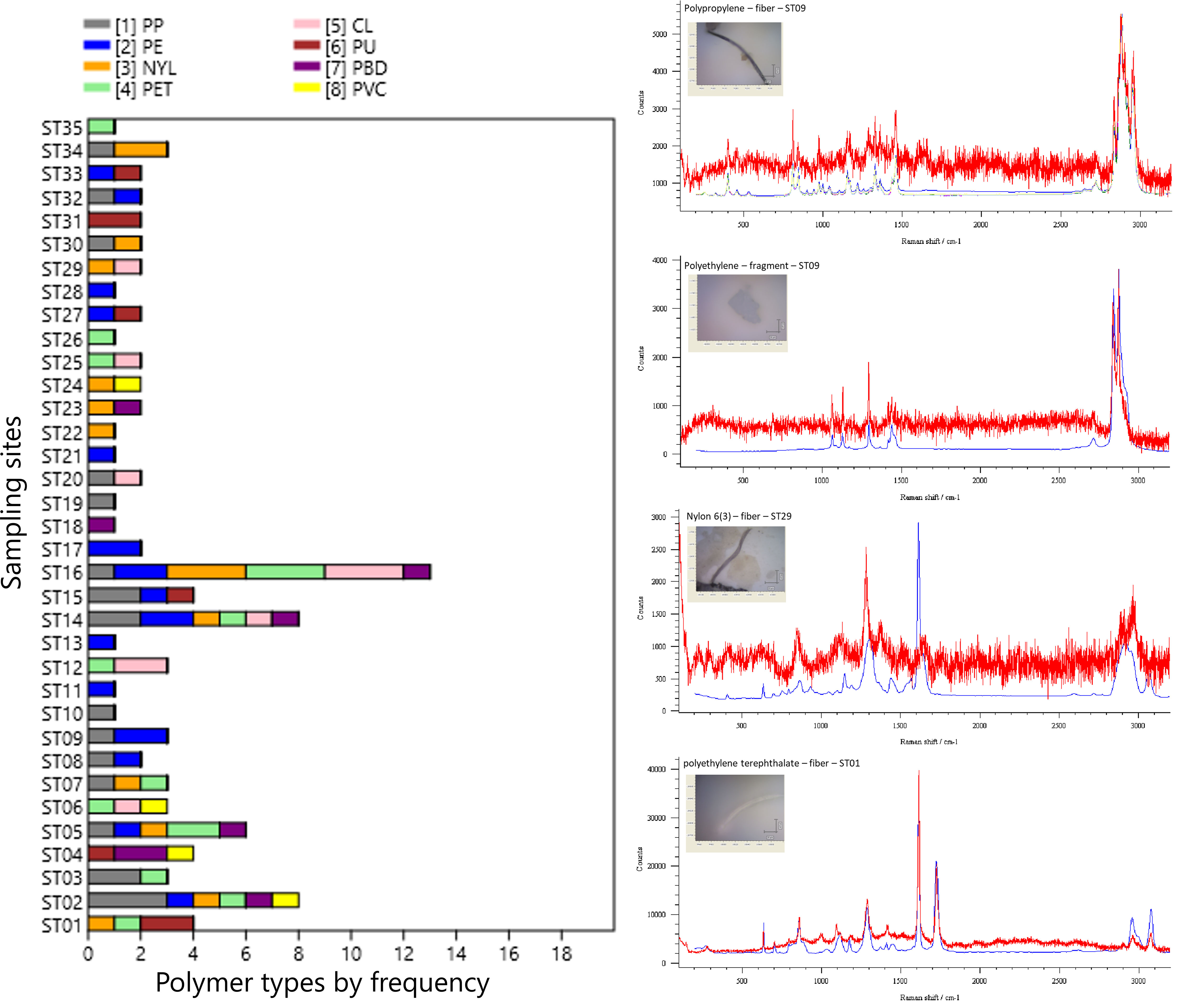
Figure 5 The Microplastic polymer profiles in each sampling station (left) and Raman spectra of the microplastic sample with corresponding photos (right). The red curves represent the sample spectra, while the blue curves depict data from the library.
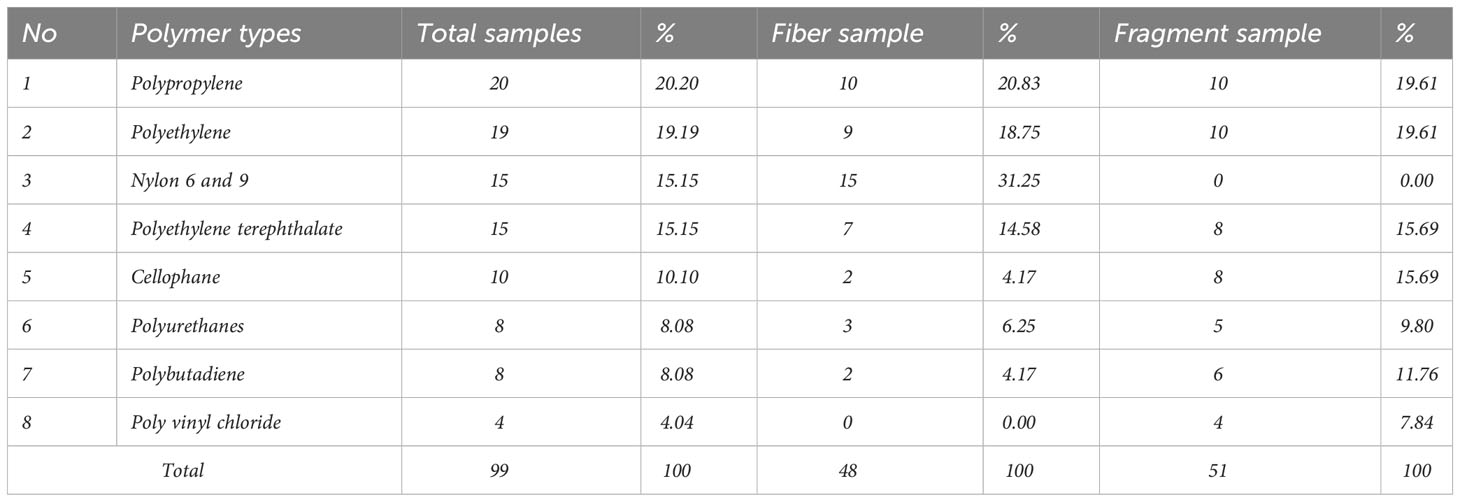
Table 1 Chemical composition from recovered microplastics in the sub-surface of the Indonesian archipelagic water.
Discussion
In this comprehensive research, we documented and analyzed the first occurrence, abundance, and characteristics of microplastics in Indonesian archipelagic sub-surface seawater. This study of sub-surface water can serve as an additional reference for understanding the fate of microplastics from the surface to the bottom of ocean waters, a topic that has been insufficiently explored. The presence of microplastics in sub-surface water within the Indonesian Archipelagic Water suggests that these particles are part of the vertical movement pathway for zooplankton in this area. Furthermore, given that microplastics fall within the size range of zooplankton food, particularly copepods, which make up a significant portion, it is crucial to monitor them regularly using suitable methodologies. This sampling technique proves to be efficient as it allows for the simultaneous collection of continuous seawater samples during vessel operations. The use of a continuous intake system on most research vessels makes this method easily adaptable for other studies. Similar monitoring approaches have been utilized in the Northeast Pacific (Desforges et al., 2014) and Northeast Atlantic regions (Lusher et al., 2014) since almost a decade ago.
The presence of microplastic particles was detected in the samples studied from Indonesian archipelagic water, indicating a problem with microplastic pollution in the catchment areas within our research area (Lusher et al., 2015; Constant et al., 2020; Dehm et al., 2020). This finding aligns with previous studies and suggests that both non-point and point sources contribute to this widespread issue (Siegfried et al., 2017). The statistical analysis findings indicate that the concentration of microplastic particles in Indonesian archipelagic water differs based on location, with the highest levels observed in the Ambon (sampling area [3]), North Java (sampling area [1]), and North Sulawesi (sampling area [7]). This study’s results support previous research that has established a correlation between human activity, as reflected by population density, and the abundance of microplastics (Wang et al., 2017). Human activities have been identified as one of the significant contributors to microplastics in marine habitats (Cordova et al., 2020). The study also presents a visualization of the population density in each province of Indonesia, as shown in Figure 1. The data reveals that Java Island has the highest population density, with over 150 million people (Statistics Indonesia, 2021). Surprisingly, despite having a lower population than Java Island, Ambon exhibits higher microplastic abundance in its waters compared to the North Java area. This disparity is attributed to the swifter seawater flushing rate and shorter water residence time in North Java as opposed to Ambon (Nugrahadi et al., 2013; Mayer et al., 2015; Atmadipoera et al., 2022; Iskandar et al., 2022). Conversely, Inner Ambon Bay’s unique characteristics impede material from leaving this area compared to other study locations (Salamena et al., 2023). The third most sub-surface water-abundant microplastics were discovered in the Talaud - Miangas Island - North Sulawesi sampling area [7]. It is believed that these originate from human activities in North Sulawesi, where there is a relatively higher population density in the eastern region of Indonesia. Additionally, this area directly borders the southern Philippines and the Pacific Ocean, potentially serving as a convergence point for inflows carrying diverse materials, including microplastics, into Indonesia from this vicinity (Purba et al., 2021; Yuan et al., 2023). Moreover, the presence of a high quantity of microplastics in the environment could be connected to degraded water quality resulting from specific economic activities (Mintenig et al., 2020; Kutralam-Muniasamy et al., 2021).
Our findings demonstrate that microplastic particles are prevalent in the sub-surface layer of Indonesian archipelagic water, which is to be expected considering their widespread presence. The reported abundance is comparable to previous studies conducted in various regions (Table 2), such as the Arctic Central Basin (Kanhai et al., 2018), North to South Atlantic Ocean waters (Kanhai et al., 2017), and Antarctica (Cincinelli et al., 2017). However, it is worth noting that the methodologies used in these studies differ (Table 2). Studies conducted in Arctic polar waters, the Pacific Ocean (including small islands, inland areas, and high seas), and the Atlantic Ocean (inland areas and high seas) reported significantly higher mean abundances compared to our study, ranging from 5-10,000 times greater (2015; Lattin et al., 2004; Desforges et al., 2014; Lusher et al., 2014; Enders et al., 2015; Dehm et al., 2020; Montoto-Martínez et al., 2020; Pabortsava and Lampitt, 2020; Jones-Williams et al., 2021). These variations could be attributed to differences between geographical locations and periods of sampling collection (Lusher et al., 2014). Our study was conducted during the onset of the wet season, when sea currents were predominantly westward, coinciding with the direction of research vessel travel from west to east Indonesia. The relatively low presence of microplastics in sub-surface samples obtained during this period can be attributed to a reduced input of microplastics from land sources due to decreased runoff associated with the rainy season peak (Purwiyanto et al., 2022). Previous research has indicated a positive link between precipitation levels and the presence of microplastic particles in aquatic environments (Moore et al., 2011; Dris et al., 2015; Faure et al., 2015; Lima et al., 2015; Gündoğdu et al., 2018; Wong et al., 2020; Xia et al., 2020). The hydrological processes within these ecosystems facilitate runoff from rainfall, resulting in the deposition of microplastics in sediments and riverbanks during dry periods. While UV exposure may be more prevalent during this time (which will enhance the probability of plastic fragmentation), an increase in rainfall during the wet season leads to the reactivation of previously deposited microplastics, causing a higher concentration of these particles in rivers that eventually flow into the ocean (Hurley et al., 2018; Wang et al., 2021). Moreover, prior studies have examined different sampling methods for analyzing microplastics, leading to significant variations in the observed levels of microplastic abundance (Zheng et al., 2021). A harmonized approach is crucial for accurate comparison between data sets, while simultaneous testing may aid in standardizing methods used for sample analysis.
In regard to the overall distribution pattern in the region, we did not observe a significant increase in the abundance of microplastics below the surface of waters surrounding the Indonesian archipelago. These sub-surface microplastics are likely transported from land by prevailing winds, currents, and local circulation (Iwasaki et al., 2017; Pabortsava and Lampitt, 2020). Figure 1 illustrates the significant variability of the ocean ship’s track current. This variability can be attributed to multiple factors, including wind patterns, tides, and topography encountered along the ship’s route. Furthermore, Figure 1 highlights that the high concentration of microplastics is located to the east of Buru Island and west of Ambon with similar concentrations observed in the southernmost region of the island. The ocean currents depicted in Figure 1, around Ambon, exhibit a southward flow towards southern Sulawesi. These currents have the potential to transport microplastics from one area to another, potentially leading to their dispersion over considerable distances within the sea (Iskandar et al., 2021). Furthermore, the circular currents in the western part of the Sulawesi Sea are believed to be associated with the activity of eddies in the region (Masumoto et al., 2001; Hao et al., 2022). Eddies may potentially contribute to the transport of materials, including microplastics, within the water column and thus could redistribute the microplastics around Miangas and Talaud Islands.
Furthermore, the positioning of microplastics at particular depths can be attributed to gravity, advection, and ingestion by plankton or other organisms (2016; Maximenko et al., 2012; Cole et al., 2013; Steer et al., 2017; Iskandar et al., 2022; Omeyer et al., 2023). Subsequently, these microplastics may be adsorbed with the fecal material of marine organisms, leading to their descent to the bottom of the water column (Yong et al., 2021). The specific mechanisms responsible for the redistribution of microplastics in different marine regions worldwide currently need to be understood completely but are expected to differ across various areas. However, in this study, we were unable to describe the movement of microplastics and areas of accumulation in certain regions of the Indonesian archipelago water. There needs to be more measurement of plastic debris sizes, particularly in sub-surface, throughout the entire extent of waters surrounding Indonesia, which results in the underrepresentation of both ocean surface and subsurface areas. These limitations introduce uncertainties when trying to determine distribution patterns for sub-surface plastic pollution on regional scales such as within Indonesian waters or globally. It is crucial to comprehensively assess and predict the consequences of plastic waste on marine ecosystems.
The prevalence of microplastic particles found in this study, specifically fibers, aligns with the global pattern observed in inland or coastal and open waters globally (Suaria et al., 2020; Xue et al., 2020; Zhou et al., 2020; Forero-López et al., 2021), including in the sub-surface water study in Table 2. However, it is likely that there are variations in the sources of these microplastics. The primary focus of previous research has been on the impact of laundry washing as a significant contributor to microplastic pollution (Boucher and Friot, 2017; Carr, 2017; Siegfried et al., 2017; Dalla Fontana et al., 2020; Mohamed et al., 2023). Studies have also found a connection between the extent of fishery activities, such as mariculture and capture fisheries, and the levels of microplastic contamination (Lusher et al., 2017a; Xue et al., 2020; Huang et al., 2022b). The research revealed the presence of small-sized fibers, with the majority measuring less than 500 microns. According to Suaria et al. (2020), these fibers will decompose upon entering coastal and marine environments, making them susceptible to long-distance transportation by air and water currents (Allen et al., 2019; Pradit et al., 2022). Moreover, this transportation is minimally influenced by their proximity to potential source areas (Suaria et al., 2020). These human-induced activities are likely contributing to the presence of microplastics in the sub-surface waters around the Indonesian archipelago. Fragmented microplastics contribute to the presence of microplastics on the coastlines of Indonesia, particularly in the western region (Vriend et al., 2021). It is believed that these fragments originate from degraded large plastic items. Throughout coastal areas of Indonesia, there is a significant amount of single-use plastic litter, including shopping bags, sachets, food containers, wrappers, bottles, and cups (Cordova et al., 2022a). Over time, due to natural weathering and physical stressors like biological deterioration or environmental factors, larger plastic waste breaks down into smaller pieces (Fok et al., 2017; He et al., 2019; Nurhasanah et al., 2021; Sulistyowati et al., 2022). One can speculate that increasing levels of anthropogenic activity may be linked to an increase in fragment-shaped microplastics present in marine waters; however, further research is needed to validate this hypothesis and gain deeper insights into the matter. Furthermore, the distribution of plastics in varying sizes shows a higher prevalence of relatively smaller particles.
The microplastics discovered in our study are predominantly categorized as small-microplastic (<1000 µm), with approximately 51.38% being below a size of 500 µm. Our findings may initially appear lower compared to the reported values (Table 2). Based on the data we compiled, 15 studies focus on microplastics in sub-surface water. Limited research has been conducted in this area, as most studies have focused on surface water, vertical areas, or sediments near the bottom. However, the volume of water filtered vertically or near the sediment is generally lower than surface water filtration. Typically, samples from vertically and near-sediment filtered waters are obtained from a sample bottle (<50 liters). These studies, including the 15 mentioned above, primarily used a simultaneous pump for broader coverage but with relatively less filtered water volume than surface water sampling. The 15 studies listed in Table 2 have varying cutoff sizes, with most falling either below or above 200 μm. This study established a detection limit of 200 μm, which was slightly smaller than the size range mentioned in Lao and Wong’s (Lao and Wong, 2023) examination across various environmental matrices. In instances where the cutoff sizes exceeded 200 microns, the levels of microplastic abundance were consistently similar to or lower than those observed in studies with cutoff sizes below 200 μm. It is worth noting that some of these studies indicate particles smaller than 100 µm are predominantly found at the research sites (Pabortsava and Lampitt, 2020; Jones-Williams et al., 2021). The abundance of floating microplastics decreases significantly as water depth increases (Reisser et al., 2015; Song et al., 2018; Ryan et al., 2020), making it advisable to collect samples from the water surface. Exploring microplastics across a range of depths is important rather than focusing on just one level of depth. Research should span from the surface down to the bottom of the water. Smaller microplastics tend to have an even distribution across surface waters and can be found down to a depth of 50 meters (Song et al., 2018). Previous studies have shown that microplastic levels at the water’s surface can be 2-5 times higher than those in subsurface areas (Reisser et al., 2015; Song et al., 2018; Ryan et al., 2020). Fiber-type microplastics, in particular, tend to mix vertically within these waters due to their lower buoyancy compared to microplastic fragments (Kooi et al., 2016; Bagaev et al., 2017). Further investigation is required to understand how fiber density decreases with increasing water depth and how this process varies under different sea conditions, such as increased vertical mixing during or after rough weather. Moreover, it should be emphasized that the true abundance of these smaller particles (<200 µm) may exceed what was established in our study due to limitations in analytical capabilities. Irrespective of their origin, the small dimensions of microplastics seem to play a significant role in facilitating downward transportation (Pabortsava and Lampitt, 2020). Microplastics that are smaller in size are more susceptible to vertical dispersal through processes such as mixing and diffusion, particularly in the mixed layer (Enders et al., 2015; Kooi et al., 2016). Therefore, further research utilizing more advanced tools is necessary to accurately assess the presence of small-sized microplastics, particularly within sub-surface waters and near the seabed.
Based on an analysis of the chemical composition, it has been found that four specific types of polymers (i.e., polypropylene, polyethylene, nylon 6 and 9, and polyethylene terephthalate) are prevalent in marine ecosystems. The findings align with earlier research in surface and sub-surface water, including in Table 2, indicating that these polymers were the predominant ones found in marine environments globally and are associated with single-use plastics waste and fishery activities (Au et al., 2017; Hahladakis et al., 2018; Erni-Cassola et al., 2019; Walkinshaw et al., 2020; Xue et al., 2020). It is conjectured that the identified microplastics in this study were probably linked to plastic waste accumulation on beaches in Indonesia (Cordova et al., 2022a) and it connects to studies concerning microplastics present specifically in Indonesian seas (Vriend et al., 2021). Moreover, the presence of microplastics in sub-surface water believed to be linked to fishing activities is indicated by the microplastic fiber samples, as shown in Table 1. The polymer types of Nylon 6 and 9 account for 15 particles, constituting 31.25% of all fiber samples, while polypropylene contributes 10 particles (20.83%), and polyethylene accounts for 9 particles (18.75%). These three polymers collectively comprise more than 70% of the analyzed fiber samples based on their polymer type, aligning with previous research, highlighting synthetic polymers such as nylon, polyethylene, and polypropylene in fishing gear (Galgani et al., 2000; Pawar et al., 2016; Ryan, 2018; Nelms et al., 2021; Richardson et al., 2021). However, given the limited research on the relationship between microplastics and fishing in Indonesian waters (Vriend et al., 2021; Cordova et al., 2021b), coupled with Indonesia’s significant potential as a major global fish producer (Statista, 2023), it is crucial to undertake thorough studies to enhance the management of this concern. The inclusion of cellophane in this investigation, as an addition to the four main polymer types identified, is significant given its prevalence (>10% of the particle samples). While there is an ongoing debate on whether cellophane should be classified as a synthetic polymer due to its cellulose-derived nature, its presence in sub-surface water could explain the common occurrence of cellophane particles found in sea salt measurements conducted in China (Yang et al., 2015). In this research, we also observed natural cellulose fibers were arranged in a stacked and tied manner. Due to their size exceeding 5 mm and chemical composition analysis using Raman spectroscopy, these fibers are not classified as microplastics. We acknowledge the resemblance of cellophane and cotton spectra; therefore, apart from matching rates >70%, we compare peaks based on findings documented by (Jin et al., 2022). Moreover, Remy et al. (2015) research indicated minor variations in Raman spectra between artificial cellulose and pure cotton fiber. Further comprehensive investigation is necessary to resolve the ongoing dispute regarding the classification of cellophane as a synthetic polymer.
Further investigation is needed to understand the impact of environmental factors such as UV radiation, heat, hydrodynamic activity, and waves on fishing gear. A previous study suggests that microplastics can be released from worn-out polypropylene ropes due to aging and abrasion (Napper et al., 2022). Although larger plastic debris eventually breaks down into smaller particles, we currently lack information on the direct input of these particles to the area. Studies should concentrate on local and regional sources to identify potential nearby contributors to microplastic pollution. This discovery highlights the importance of preventing larger plastic pieces from breaking down once they enter the environment. In order to address this issue, it is crucial to implement stringent regulations, raise public awareness, and launch campaigns that promote proper disposal methods and systemic improvements in managing plastic waste. Furthermore, further research is needed to understand the pathways through which microplastic particles enter the aquatic ecosystem and their origins, such as residential areas, industrial activities, agricultural runoff, and other potential sources of microplastics.
Conclusion
Our study revealed the presence of microplastic particles in all samples collected from the waters surrounding the Indonesian archipelago. This finding suggests that these waters are contaminated with microplastics. The presence of microplastics in the sub-surface water of the Indonesian archipelago is notably high near areas influenced by human activities. The order of abundance, from highest to lowest is as follows: Wakatobi Archipelago - South Buru Island - Outside Ambon [3] > Outer Jakarta - Kangean Island [1] > Talaud - Miangas Island - North Sulawesi [7] > Halmahera Island - Talaud Island [5] ≈ Kangean Island - Selayar Island - Wakatobi Archipelago [2] ≈ Lifamatora Passage [4] > Maluku Sea [6]. The concentration of microplastics found in the sub-surface water is relatively lower compared to global studies. However, it should be noted that this research focuses on microplastics measuring 200 µm and larger. Further investigation may reveal higher levels of microplastic abundance if conducted with more comprehensive methods. The presence of microplastic particles, particularly fibers, found in this study is consistent with the global trend observed in inland and coastal waters worldwide. Among all the sampling areas examined, only one sampling area (sampling area 3) demonstrated a dominance of fragment-shaped microplastics. These fragments are believed to originate from degraded large plastic items commonly found along the Indonesian coast. The type of polymer detected in our analysis further supports the prevalence of fiber-shaped microplastics. Additionally, due to legal and illegal fishing practices, there is an increased likelihood of accumulated ALDFG-derived microplastics in these archipelagic waters. Further investigation is necessary to address the limited data on this issue. To mitigate the influx of microplastics into marine environments, it is essential to conduct research aimed at implementing strict regulations, enhancing public awareness, and initiating campaigns that encourage proper disposal practices and systematic enhancements in plastic waste management. This should specifically focus on reducing single-use plastics and addressing ALDFG.
Data availability statement
The original contributions presented in the study are included in the article/Supplementary Material. Further inquiries can be directed to the corresponding authors.
Author contributions
MC: Conceptualization, Data curation, Formal analysis, Funding acquisition, Investigation, Methodology, Supervision, Validation, Visualization, Writing – original draft, Writing – review & editing. MI: Conceptualization, Resources, Validation, Visualization, Writing – original draft. DS: Conceptualization, Funding acquisition, Project administration, Visualization, Writing – review & editing. MK: Data curation, Formal analysis, Investigation, Validation, Writing – review & editing. SW: Data curation, Formal analysis, Investigation, Validation, Writing – review & editing. RS: Data curation, Formal analysis, Investigation, Validation, Writing – review & editing. YU: Data curation, Formal analysis, Investigation, Validation, Writing – review & editing. TP: Data curation, Formal analysis, Investigation, Validation, Writing – review & editing. DY: Data curation, Formal analysis, Investigation, Validation, Writing – review & editing. SS: Formal analysis, Investigation, Validation, Writing – review & editing, Data curation. RP: Data curation, Formal analysis, Investigation, Validation, Writing – review & editing. RR: Data curation, Formal analysis, Investigation, Validation, Writing – review & editing. ER: Data curation, Formal analysis, Investigation, Validation, Writing – review & editing. SZ: Data curation, Funding acquisition, Investigation, Project administration, Validation, Writing – review & editing. XS: Data curation, Funding acquisition, Investigation, Project administration, Validation, Writing – review & editing. ZW: Data curation, Funding acquisition, Investigation, Project administration, Validation, Writing – original draft, Writing – review & editing.
Funding
The author(s) declare financial support was received for the research, authorship, and/or publication of this article. This research is supported by the Strategic Priority Research Program of the Chinese Academy of Sciences (No. XDA19060204, No. XDB42000000) and the Key Collaborative Research Program of the Alliance of International Science Organization (Grant No. ANSO-CR-KP-2022-08).
Acknowledgments
We express our gratitude to the captain and crew on RV Baruna Jaya VIII for their outstanding performance and invaluable support throughout our journey. We also want to thank the WPOS joint cruise between IOCAS and RCO. This collaboration work is facilitated by the platform of Sino-Indonesia Joint Laboratory for Marine Sciences (SIMS).
Conflict of interest
The authors declare that the research was conducted in the absence of any commercial or financial relationships that could be construed as a potential conflict of interest.
Publisher’s note
All claims expressed in this article are solely those of the authors and do not necessarily represent those of their affiliated organizations, or those of the publisher, the editors and the reviewers. Any product that may be evaluated in this article, or claim that may be made by its manufacturer, is not guaranteed or endorsed by the publisher.
Supplementary material
The Supplementary Material for this article can be found online at: https://www.frontiersin.org/articles/10.3389/fmars.2024.1362414/full#supplementary-material
References
Adyasari D., Pratama M. A., Teguh N. A., Sabdaningsih A., Kusumaningtyas M. A., Dimova N. (2021). Anthropogenic impact on Indonesian coastal water and ecosystems: Current status and future opportunities. Mar. pollut. Bull. 171. doi: 10.1016/j.marpolbul.2021.112689
Alimi O. S., Farner Budarz J., Hernandez L. M., Tufenkji N. (2018). Microplastics and nanoplastics in aquatic environments: aggregation, deposition, and enhanced contaminant transport. Environ. Sci. Technol. 52, 1704–1724. doi: 10.1021/acs.est.7b05559
Allen S., Allen D., Phoenix V. R., Le Roux G., Durántez Jiménez P., Simonneau A., et al. (2019). Atmospheric transport and deposition of microplastics in a remote mountain catchment. Nat. Geosci. 12, 339–344. doi: 10.1038/s41561-019-0335-5
Andrady A. L. (2011). Microplastics in the marine environment. Mar. pollut. Bull. 62, 1596–1605. doi: 10.1016/j.marpolbul.2011.05.030
Atmadipoera A. S., Koch-Larrouy A., Madec G., Grelet J., Baurand F., Jaya I., et al. (2022). Part I: Hydrological properties within the eastern Indonesian throughflow region during the INDOMIX experiment. Deep. Res. Part I Oceanogr. Res. Pap 182. doi: 10.1016/j.dsr.2022.103735
Au S. Y., Lee C. M., Weinstein J. E., van den Hurk P., Klaine S. J. (2017). Trophic transfer of microplastics in aquatic ecosystems: Identifying critical research needs. Integr. Environ. Assess. Manage. 13, 505–509. doi: 10.1002/ieam.1907
Bagaev A., Mizyuk A., Khatmullina L., Isachenko I., Chubarenko I. (2017). Anthropogenic fibres in the Baltic Sea water column: Field data, laboratory and numerical testing of their motion. Sci. Total Environ. 599–600, 560–571. doi: 10.1016/j.scitotenv.2017.04.185
Bakir A., Rowland S. J., Thompson R. C. (2014). Transport of persistent organic pollutants by microplastics in estuarine conditions. Estuar. Coast. Shelf Sci. 140, 14–21. doi: 10.1016/j.ecss.2014.01.004
Barnes D. K. A., Galgani F., Thompson R. C., Barlaz M. (2009). Accumulation and fragmentation of plastic debris in global environments. Philos. Trans. R. Soc B Biol. Sci. 364, 1985–1998. doi: 10.1098/rstb.2008.0205
Baumert K., Melchior B. (2015). The practice of archipelagic states: A study of studies. Ocean Dev. Int. Law 46, 60–80. doi: 10.1080/00908320.2015.995970
Boucher J., Friot D. (2017). “Primary microplastics in the oceans,” in A global evaluation of sources ([IUCN] International Union for Conservation of Nature and Natural Resources, Gland, Switzerland). doi: 10.2305/IUCN.CH.2017.01.en
Browne M. A., Galloway T., Thompson R. (2007). Microplastic-an emerging contaminant of potential concern? Integr. Environ. Assess. Manage. 3, 559–561. doi: 10.1897/1551-3793(2007)3[559:LD]2.0.CO;2
Butcher J. G., Elson R. E., Bernard L. (2017). Sovereignty and the sea: how Indonesia became an archipelagic state. Contemp. Southeast Asia 39, 594–596. doi: 10.1355/cs39-3o
Carr S. A. (2017). Sources and dispersive modes of micro-fibers in the environment. Integr. Environ. Assess. Manage. 13, 466–469. doi: 10.1002/ieam.1916
Cincinelli A., Scopetani C., Chelazzi D., Lombardini E., Martellini T., Katsoyiannis A., et al. (2017). Microplastic in the surface waters of the Ross Sea (Antarctica): Occurrence, distribution and characterization by FTIR. Chemosphere 175, 391–400. doi: 10.1016/j.chemosphere.2017.02.024
Clifton J., Osman E. O., Suggett D. J., Smith D. J. (2021). Resolving conservation and development tensions in a small island state: A governance analysis of Curieuse Marine National Park, Seychelles. Mar. Policy 127. doi: 10.1016/j.marpol.2019.103617
Cole M., Lindeque P. K., Fileman E., Clark J., Lewis C., Halsband C., et al. (2016). Microplastics alter the properties and sinking rates of zooplankton faecal pellets. Environ. Sci. Technol. 50, 3239–3246. doi: 10.1021/acs.est.5b05905
Cole M., Lindeque P., Fileman E., Halsband C., Goodhead R., Moger J., et al. (2013). Microplastic ingestion by zooplankton. Environ. Sci. Technol. 47, 6646–6655. doi: 10.1021/es400663f
Cole M., Webb H., Lindeque P. K., Fileman E. S., Halsband C., Galloway T. S. (2014). Isolation of microplastics in biota-rich seawater samples and marine organisms. Sci. Rep. 4. doi: 10.1038/srep04528
Collard F., Gilbert B., Compère P., Eppe G., Das K., Jauniaux T., et al. (2017). Microplastics in livers of European anchovies (Engraulis encrasicolus, L.). Environ. pollut. 229, 1000–1005. doi: 10.1016/j.envpol.2017.07.089
Constant M., Ludwig W., Kerhervé P., Sola J., Charrière B., Sanchez-Vidal A., et al. (2020). Microplastic fluxes in a large and a small Mediterranean river catchments: The Têt and the Rhône, Northwestern Mediterranean Sea. Sci. Total Environ. 716, 136984. doi: 10.1016/j.scitotenv.2020.136984
Cordova M. R., Iskandar M. R., Muhtadi A., Nurhasanah, Saville R., Riani E. (2022a). Spatio-temporal variation and seasonal dynamics of stranded beach anthropogenic debris on Indonesian beach from the results of nationwide monitoring. Mar. pollut. Bull. 182, 114035. doi: 10.1016/j.marpolbul.2022.114035
Cordova M. R., Purbonegoro T., Puspitasari R., Subandi R., Kaisupy M. T., Wibowo S. P. A., et al. (2021a). Preliminary study of the effect of tourism activities on litter pollution:a case study on padar island, komodo national park, Indonesia. J. Ecol. Eng. 22, 131–139. doi: 10.12911/22998993/140265
Cordova M. R., Purwiyanto A. I. S., Suteja Y. (2019). Abundance and characteristics of microplastics in the northern coastal waters of Surabaya, Indonesia. Mar. pollut. Bull. 142, 183–188. doi: 10.1016/j.marpolbul.2019.03.040
Cordova M. R., Riani E., Shiomoto A. (2020). Microplastics ingestion by blue panchax fish (Aplocheilus sp.) from Ciliwung Estuary, Jakarta, Indonesia. Mar. pollut. Bull. 161, 111763. doi: 10.1016/j.marpolbul.2020.111763
Cordova M. R., Ulumuddin Y. I., Lubis A. A., Kaisupy M. T., Wibowo S. P. A., Subandi R., et al. (2023). Microplastics leaving a trace in mangrove sediments ever since they were first manufactured: A study from Indonesia mangroves. Mar. pollut. Bull. 195, 115517. doi: 10.1016/j.marpolbul.2023.115517
Cordova M. R., Ulumuddin Y. I., Purbonegoro T., Puspitasari R., Afianti N. F., Rositasari R., et al. (2022b). Seasonal heterogeneity and a link to precipitation in the release of microplastic during COVID-19 outbreak from the Greater Jakarta area to Jakarta Bay, Indonesia. Mar. pollut. Bull. 181, 113926. doi: 10.1016/j.marpolbul.2022.113926
Cordova M. R., Ulumuddin Y. I., Purbonegoro T., Shiomoto A. (2021b). Characterization of microplastics in mangrove sediment of Muara Angke Wildlife Reserve, Indonesia. Mar. pollut. Bull. 163, 112012. doi: 10.1016/j.marpolbul.2021.112012
Courtene-Jones W., Quinn B., Ewins C., Gary S. F., Narayanaswamy B. E. (2020). Microplastic accumulation in deep-sea sediments from the Rockall Trough. Mar. pollut. Bull. 154. doi: 10.1016/j.marpolbul.2020.111092
Crawford C. B., Quinn B. (2017). “Microplastic identification techniques,” in Microplastic Pollutants, 219–267. doi: 10.1016/B978-0-12-809406-8.00010-4
Cunningham E. M., Ehlers S. M., Dick J. T. A., Sigwart J. D., Linse K., Dick J. J., et al. (2020). High abundances of microplastic pollution in deep-sea sediments: evidence from Antarctica and the southern ocean. Environ. Sci. Technol. 54, 13661–13671. doi: 10.1021/acs.est.0c03441
Cutroneo L., Reboa A., Geneselli I., Capello M. (2021). Considerations on salts used for density separation in the extraction of microplastics from sediments. Mar. pollut. Bull. 166. doi: 10.1016/j.marpolbul.2021.112216
Dalla Fontana G., Mossotti R., Montarsolo A. (2020). Assessment of microplastics release from polyester fabrics: The impact of different washing conditions. Environ. pollut. 264, 113960. doi: 10.1016/j.envpol.2020.113960
Dehm J., Singh S., Ferreira M., Piovano S. (2020). Microplastics in subsurface coastal waters along the southern coast of Viti Levu in Fiji, South Pacific. Mar. pollut. Bull. 156. doi: 10.1016/j.marpolbul.2020.111239
Demirbilek D., Öztüfekçi Önal A., Demir V., Uslu G., Arslanoglu-Isık H. (2013). Characterization and pollution potential assessment of Tunceli, Turkey municipal solid waste open dumping site leachates. Environ. Monit. Assess. 185, 9435–9449. doi: 10.1007/s10661-013-3263-7
Desforges J. P. W., Galbraith M., Dangerfield N., Ross P. S. (2014). Widespread distribution of microplastics in subsurface seawater in the NE Pacific Ocean. Mar. pollut. Bull. 79, 94–99. doi: 10.1016/j.marpolbul.2013.12.035
Diaz-Basantes M. F., Conesa J. A., Fullana A. (2020). Microplastics in honey, beer, milk and refreshments in Ecuador as emerging contaminants. Sustain 12. doi: 10.3390/su12145514
Driedger A. G. J., Dürr H. H., Mitchell K., Van Cappellen P. (2015). Plastic debris in the Laurentian Great Lakes: A review. J. Great Lakes Res. 41, 9–19. doi: 10.1016/j.jglr.2014.12.020
Dris R., Gasperi J., Rocher V., Saad M., Renault N., Tassin B. (2015). Microplastic contamination in an urban area: A case study in Greater Paris. Environ. Chem. 12, 592–599. doi: 10.1071/EN14167
Enders K., Lenz R., Stedmon C. A., Nielsen T. G. (2015). Abundance, size and polymer composition of marine microplastics ≥10μm in the Atlantic Ocean and their modelled vertical distribution. Mar. pollut. Bull. 100, 70–81. doi: 10.1016/j.marpolbul.2015.09.027
Erni-Cassola G., Zadjelovic V., Gibson M. I., Christie-Oleza J. A. (2019). Distribution of plastic polymer types in the marine environment; A meta-analysis. J. Hazard. Mater. 369, 691–698. doi: 10.1016/j.jhazmat.2019.02.067
Faure F., Demars C., Wieser O., Kunz M., De Alencastro L. F. (2015). Plastic pollution in Swiss surface waters: Nature and concentrations, interaction with pollutants. Environ. Chem. 12, 582–591. doi: 10.1071/EN14218
Fok L., Cheung P. K., Tang G., Li W. C. (2017). Size distribution of stranded small plastic debris on the coast of Guangdong, South China. Environ. pollut. 220, 407–412. doi: 10.1016/j.envpol.2016.09.079
Forero-López A. D., Rimondino G. N., Truchet D. M., Colombo C. V., Buzzi N. S., Malanca F. E., et al. (2021). Occurrence, distribution, and characterization of suspended microplastics in a highly impacted estuarine wetland in Argentina. Sci. Total Environ. 785, 147141. doi: 10.1016/j.scitotenv.2021.147141
Fuldauer L. I., Ives M. C., Adshead D., Thacker S., Hall J. W. (2019). Participatory planning of the future of waste management in small island developing states to deliver on the Sustainable Development Goals. J. Clean. Prod. 223, 147–162. doi: 10.1016/j.jclepro.2019.02.269
Galgani F., Leaute J. P., Moguedet P., Souplet A., Verin Y., Carpentier A., et al. (2000). Litter on the sea floor along European coasts. Mar. pollut. Bull. 40, 516–527. doi: 10.1016/S0025-326X(99)00234-9
Germanov E. S., Marshall A. D., Bejder L., Fossi M. C., Loneragan N. R. (2018). Microplastics: no small problem for filter-feeding megafauna. Trends Ecol. Evol. 33, 227–232. doi: 10.1016/j.tree.2018.01.005
GESAMP (2015). Sources, fate and effects of microplastics in the marine environment: a global assessment. (London, UK: IMO). doi: 10.13140/RG.2.1.3803.7925
GESAMP (2019) Guidelines for the monitoring and assessment of plastic litter in the ocean. Available online at: http://www.gesamp.org/publications/guidelines-for-the-monitoring-and-assessment-of-plastic-litter-in-the-ocean.
Geyer R., Jambeck J. R., Law K. L. (2017). Production, use, and fate of all plastics ever made. Sci. Adv. 3. doi: 10.1126/sciadv.1700782
Gündoğdu S., Çevik C., Ayat B., Aydoğan B., Karaca S. (2018). How microplastics quantities increase with flood events? An example from Mersin Bay NE Levantine coast of Turkey. Environ. pollut. 239, 342–350. doi: 10.1016/j.envpol.2018.04.042
Hahladakis J. N., Velis C. A., Weber R., Iacovidou E., Purnell P. (2018). An overview of chemical additives present in plastics: Migration, release, fate and environmental impact during their use, disposal and recycling. J. Hazard. Mater. 344, 179–199. doi: 10.1016/j.jhazmat.2017.10.014
Hao Z., Xu Z., Feng M., Zhang P., Yin B. (2022). Dynamics of interannual eddy kinetic energy variability in the sulawesi sea revealed by OFAM3. J. Geophys. Res. Ocean. 127, e2022JC018815. doi: 10.1029/2022JC018815
Hardesty B. D., Wilcox C. (2011). Understanding the types, sources and at-sea distribution of marine debris in Australian waters (Australia: CSIRO). Available at: https://www.environment.gov.au/system/files/pages/8ff786ed-42cf-4a50-866e-13a4d231422b/files/marine-debris-sources.pdf.
He P., Chen L., Shao L., Zhang H., Lü F. (2019). Municipal solid waste (MSW) landfill: A source of microplastics? -Evidence of microplastics in landfill leachate. Water Res. 159, 38–45. doi: 10.1016/j.watres.2019.04.060
Herrera A., Acosta-Dacal A., Pérez Luzardo O., Martínez I., Rapp J., Reinold S., et al. (2022). Bioaccumulation of additives and chemical contaminants from environmental microplastics in European seabass (Dicentrarchus labrax). Sci. Total Environ. 822, 153396. doi: 10.1016/j.scitotenv.2022.153396
Huang Y., Fan J., Liu H., Lu X. (2022b). Vertical distribution of microplastics in the sediment profiles of the Lake Taihu, eastern China. Sustain. Environ. Res. 32. doi: 10.1186/s42834-022-00154-7
Huang D., Wang X., Yin L., Chen S., Tao J., Zhou W., et al. (2022a). Research progress of microplastics in soil-plant system: Ecological effects and potential risks. Sci. Total Environ. 812, 151487. doi: 10.1016/j.scitotenv.2021.151487
Hurley R., Woodward J., Rothwell J. J. (2018). Microplastic contamination of river beds significantly reduced by catchment-wide flooding. Nat. Geosci. 11, 251–257. doi: 10.1038/s41561-018-0080-1
Iskandar M. R., Cordova M. R., Park Y.-G. (2022). Pathways and destinations of floating marine plastic debris from 10 major rivers in Java and Bali, Indonesia: A Lagrangian particle tracking perspective. Mar. pollut. Bull. 185, 114331. doi: 10.1016/j.marpolbul.2022.114331
Iskandar M. R., Surinati D., Cordova M. R., Siong K. (2021). Pathways of floating marine debris in Jakarta Bay, Indonesia. Mar. pollut. Bull. 169. doi: 10.1016/j.marpolbul.2021.112511
Iwasaki S., Isobe A., Kako S., Uchida K., Tokai T. (2017). Fate of microplastics and mesoplastics carried by surface currents and wind waves: A numerical model approach in the Sea of Japan. Mar. pollut. Bull. 121, 85–96. doi: 10.1016/j.marpolbul.2017.05.057
Jin N., Song Y., Ma R., Li J., Li G., Zhang D. (2022). Characterization and identification of microplastics using Raman spectroscopy coupled with multivariate analysis. Anal. Chim. Acta 1197. doi: 10.1016/j.aca.2022.339519
Jones-Williams K., Galloway T. S., Peck V. L., Manno C. (2021). Remote, but not isolated—Microplastics in the sub-surface waters of the canadian arctic archipelago. Front. Mar. Sci. 8. doi: 10.3389/fmars.2021.666482
Kanhai L. D. K., Gårdfeldt K., Lyashevska O., Hassellöv M., Thompson R. C., O’Connor I. (2018). Microplastics in sub-surface waters of the Arctic Central Basin. Mar. pollut. Bull. 130, 8–18. doi: 10.1016/j.marpolbul.2018.03.011
Kanhai L. D. K., Officer R., Lyashevska O., Thompson R. C., O’Connor I. (2017). Microplastic abundance, distribution and composition along a latitudinal gradient in the Atlantic Ocean. Mar. pollut. Bull. 115, 307–314. doi: 10.1016/j.marpolbul.2016.12.025
Klemeš J. J., Van Fan Y., Tan R. R., Jiang P. (2020). Minimising the present and future plastic waste, energy and environmental footprints related to COVID-19. Renew. Sustain. Energy Rev. 127. doi: 10.1016/j.rser.2020.109883
Kniggendorf A. K., Wetzel C., Roth B. (2019). Microplastics detection in streaming tap water with raman spectroscopy. Sensors (Switzerland) 19. doi: 10.3390/s19081839
Knoblauch D., Mederake L. (2021). Government policies combatting plastic pollution. Curr. Opin. Toxicol. 28, 87–96. doi: 10.1016/j.cotox.2021.10.003
Kooi M., Reisser J., Slat B., Ferrari F. F., Schmid M. S., Cunsolo S., et al. (2016). The effect of particle properties on the depth profile of buoyant plastics in the ocean. Sci. Rep. 6. doi: 10.1038/srep33882
Kutralam-Muniasamy G., Pérez-Guevara F., Elizalde-Martínez I., Shruti V. C. (2021). How well-protected are protected areas from anthropogenic microplastic contamination? Review of analytical methods, current trends, and prospects. Trends Environ. Anal. Chem. 32. doi: 10.1016/j.teac.2021.e00147
Kvale K. F., Friederike Prowe A. E., Oschlies A. (2020). A critical examination of the role of marine snow and zooplankton fecal pellets in removing ocean surface microplastic. Front. Mar. Sci. 6. doi: 10.3389/fmars.2019.00808
Lao W., Wong C. S. (2023). How to establish detection limits for environmental microplastics analysis. Chemosphere 327, 138456. doi: 10.1016/j.chemosphere.2023.138456
Lattin G. L., Moore C. J., Zellers A. F., Moore S. L., Weisberg S. B. (2004). A comparison of neustonic plastic and zooplankton at different depths near the southern California shore. Mar. pollut. Bull. 49, 291–294. doi: 10.1016/j.marpolbul.2004.01.020
Lavers J. L., Dicks L., Dicks M. R., Finger A. (2019). Significant plastic accumulation on the Cocos (Keeling) Islands, Australia. Sci. Rep. 97102. doi: 10.1038/s41598-019-43375-4
Law K. L. (2017). Plastics in the marine environment. Ann. Rev. Mar. Sci. 9, 205–229. doi: 10.1146/annurev-marine-010816-060409
Lebreton L. C. M., Andrady A. (2019). Future scenarios of global plastic waste generation and disposal. Palgrave Commun. 5. doi: 10.1057/s41599-018-0212-7
Lechner A., Ramler D. (2015). The discharge of certain amounts of industrial microplastic from a production plant into the River Danube is permitted by the Austrian legislation. Environ. pollut. 200, 159–160. doi: 10.1016/j.envpol.2015.02.019
Lee J. (2015). Economic valuation of marine litter and microplastic pollution in the marine environment: An initial assessment of the case of the United Kingdom. London, UK: SOAS-CeFiMS 126, 1–16.
Li Q., Wu J., Zhao X., Gu X., Ji R. (2019). Separation and identification of microplastics from soil and sewage sludge. Environ. pollut. 254. doi: 10.1016/j.envpol.2019.113076
Lima A. R. A., Barletta M., Costa M. F. (2015). Seasonal distribution and interactions between plankton and microplastics in a tropical estuary. Estuar. Coast. Shelf Sci. 165, 213–225. doi: 10.1016/j.ecss.2015.05.018
Long M., Moriceau B., Gallinari M., Lambert C., Huvet A., Raffray J., et al. (2015). Interactions between microplastics and phytoplankton aggregates: Impact on their respective fates. Mar. Chem. 175, 39–46. doi: 10.1016/j.marchem.2015.04.003
Lubis I. E. N., Melani W. R., Syakti A. D. (2019). “Plastic debris contamination in Grey-eel catfish (Plotosus canius) in Tanjungpinang water, Riau Islands-Indonesia,” in AIP Conference Proceedings, (USA: American Institute of Physics) Vol. 020035. doi: 10.1063/1.5097504
Luna-Jorquera G., Thiel M., Portflitt-Toro M., Dewitte B. (2019). Marine protected areas invaded by floating anthropogenic litter: An example from the South Pacific. Aquat. Conserv. Mar. Freshw. Ecosyst. 29, 245–259. doi: 10.1002/aqc.3095
Lusher A. L., Burke A., O’Connor I., Officer R. (2014). Microplastic pollution in the Northeast Atlantic Ocean: Validated and opportunistic sampling. Mar. pollut. Bull. 88, 325–333. doi: 10.1016/j.marpolbul.2014.08.023
Lusher A. L., Hollman P., Mendozal J. (2017a). Microplastics in fisheries and aquaculture: status of knowledge on their occurrence and implications for aquatic organisms and food safety. (Rome, Italy: FAO).
Lusher A. L., Tirelli V., O’Connor I., Officer R. (2015). Microplastics in Arctic polar waters: The first reported values of particles in surface and sub-surface samples. Sci. Rep. 5, 14947. doi: 10.1038/srep14947
Lusher A. L., Welden N. A., Sobral P., Cole M. (2017b). Sampling, isolating and identifying microplastics ingested by fish and invertebrates. Anal. Methods 9, 1346–1360. doi: 10.1039/C6AY02415G
Masumoto Y., Kagimoto T., Yoshida M., Fukuda M., Hirose N., Yamagata T. (2001). Intraseasonal eddies in the Sulawesi Sea simulated in an ocean general circulation model. Geophys. Res. Lett. 28, 1631–1634. doi: 10.1029/2000GL011835
Matsuguma Y., Takada H., Kumata H., Kanke H., Sakurai S., Suzuki T., et al. (2017). Microplastics in sediment cores from asia and africa as indicators of temporal trends in plastic pollution. Arch. Environ. Contam. Toxicol. 73, 230–239. doi: 10.1007/s00244-017-0414-9
Maximenko N., Hafner J., Niiler P. (2012). Pathways of marine debris derived from trajectories of Lagrangian drifters. Mar. pollut. Bull. 65, 51–62. doi: 10.1016/j.marpolbul.2011.04.016
Mayer B., Stacke T., Stottmeister I., Pohlmann T. (2015). Sunda Shelf Seas: flushing rates and residence times. Ocean Sci. Discuss. 12, 863–895. doi: 10.5194/osd-12-863-2015
Meidiana C., Gamse T. (2010). The new Waste Law: Challenging opportunity for future landfill operation in Indonesia. Waste Manage. Res. 29, 20–29. doi: 10.1177/0734242X10384013
Meijer L. J. J., van Emmerik T., van der Ent R., Schmidt C., Lebreton L. (2021). More than 1000 rivers account for 80% of global riverine plastic emissions into the ocean. Sci. Adv. 7, eaaz5803. doi: 10.1126/sciadv.aaz5803
Meng M., Yang L., Wei B., Cao Z., Yu J., Liao X. (2021). Plastic shed production systems: The migration of heavy metals from soil to vegetables and human health risk assessment. Ecotoxicol. Environ. Saf. 215. doi: 10.1016/j.ecoenv.2021.112106
Michida Y., Chavanich S., Chiba S., Cordova M. R., Cozsar Cabanas A., Glagani F., et al. (2019). Guidelines for harmonizing ocean surface microplastic monitoring methods. Version 1.1 (Chiyoda-ku, Tokyo, Japan: Ministry of the Environment Japan). doi: 10.25607/OBP-867
Mintenig S. M., Kooi M., Erich M. W., Primpke S., Redondo- Hasselerharm P. E., Dekker S. C., et al. (2020). A systems approach to understand microplastic occurrence and variability in Dutch riverine surface waters. Water Res. 176. doi: 10.1016/j.watres.2020.115723
Mohamed C. A., Shahruddin A. N., Pradit S., Loh P. S., Nitiratsuwan T., Kobkeatthawin T., et al. (2023). Depth profiles of microplastic in sediment cores in the mangrove area of kuala gula mangrove, Malaysia. J. Mar. Sci. Eng. 11. doi: 10.3390/jmse11061223
Mohee R., Mauthoor S., Bundhoo Z. M. A., Somaroo G., Soobhany N., Gunasee S. (2015). Current status of solid waste management in small island developing states: A review. Waste Manage. 43, 539–549. doi: 10.1016/j.wasman.2015.06.012
Monteiro R. C. P., Ivar do Sul J. A., Costa M. F. (2018). Plastic pollution in islands of the Atlantic Ocean. Environ. pollut. 238, 103–110. doi: 10.1016/j.envpol.2018.01.096
Montoto-Martínez T., Hernández-Brito J. J., Gelado-Caballero M. D. (2020). Pump-underway ship intake: An unexploited opportunity for Marine Strategy Framework Directive (MSFD) microplastic monitoring needs on coastal and oceanic waters. PloS One 15, e0232744. doi: 10.1371/journal.pone.0232744
Moore C. J., Lattin G. L., Zellers A. F. (2011). Quantity and type of plastic debris flowing from two urban rivers to coastal waters and beaches of Southern California. Rev. Gestão Costeira Integr. 11, 65–73. doi: 10.5894/rgci194
Moriwaki H., Kitajima S., Katahira K. (2009). Waste on the roadside, “poi-sute” waste: Its distribution and elution potential of pollutants into environment. Waste Manage. 29, 1192–1197. doi: 10.1016/j.wasman.2008.08.017
Napper I. E., Wright L. S., Barrett A. C., Parker-Jurd F. N. F., Thompson R. C. (2022). Potential microplastic release from the maritime industry: Abrasion of rope. Sci. Total Environ. 804. doi: 10.1016/j.scitotenv.2021.150155
Nelms S. E., Duncan E. M., Patel S., Badola R., Bhola S., Chakma S., et al. (2021). Riverine plastic pollution from fisheries: Insights from the Ganges River system. Sci. Total Environ. 756. doi: 10.1016/j.scitotenv.2020.143305
Nugrahadi M. S., Duwe K., Schroeder F., Goldmann D. (2013). Seasonal variability of the water residence time in the madura Strait, East Java, Indonesia. Asian J. Water Environ. pollut. 10, 117–128.
Nurhasanah, Cordova M. R., Riani E. (2021). Micro- and mesoplastics release from the Indonesian municipal solid waste landfill leachate to the aquatic environment: Case study in Galuga Landfill Area, Indonesia. Mar. pollut. Bull. 163, 111986. doi: 10.1016/j.marpolbul.2021.111986
Omeyer L. C. M., Duncan E. M., Abreo N. A. S., Acebes J. M. V., AngSinco-Jimenez L. A., Anuar S. T., et al. (2023). Interactions between marine megafauna and plastic pollution in Southeast Asia. Sci. Total Environ. 874, 162502. doi: 10.1016/j.scitotenv.2023.162502
Pabortsava K., Lampitt R. S. (2020). High concentrations of plastic hidden beneath the surface of the Atlantic Ocean. Nat. Commun. 11. doi: 10.1038/s41467-020-17932-9
Pawar P. R., Shirgaonkar S. S., Patil R. B. (2016). Plastic marine debris: Sources, distribution and impacts on coastal and ocean biodiversity. PENCIL Publ. Biol. Sci. 3, 40–54.
PlasticsEurope, EPRO (2021). Plastics - the Facts 2021 an analysis of European plastics production, demand and waste data (Brussels, Belgium: Plastics Europe (the Association of Plastics Manufacturers in Europe) and EPRO (the European Association of Plastics Recycling and Recovery Organisations). Available at: https://plasticseurope.org/wp-content/uploads/2021/12/Plastics-the-Facts-2021-web-final.pdf.
Pradit S., Noppradit P., Loh P. S., Nitiratsuwan T., Le T. P. Q., Oeurng C., et al. (2022). The occurrence of microplastics in sediment cores from two mangrove areas in southern Thailand. J. Mar. Sci. Eng. 10. doi: 10.3390/jmse10030418
Provencher J. F., Bond A. L., Avery-Gomm S., Borrelle S. B., Bravo Rebolledo E. L., Hammer S., et al. (2017). Quantifying ingested debris in marine megafauna: A review and recommendations for standardization. Anal. Methods 9, 1454–1469. doi: 10.1039/C6AY02419J
Purba N. P., Faizal I., Cordova M. R., Abimanyu A., Afandi N. K. A., Indriawan D., et al. (2021). Marine debris pathway across Indonesian boundary seas. J. Ecol. Eng. 22, 82–98. doi: 10.12911/22998993/132428
Purwiyanto A. I. S., Prartono T., Riani E., Naulita Y., Cordova M. R., Koropitan A. F. (2022). The deposition of atmospheric microplastics in Jakarta-Indonesia: The coastal urban area. Mar. pollut. Bull. 174, 113195. doi: 10.1016/j.marpolbul.2021.113195
Reisser J., Slat B., Noble K., Du Plessis K., Epp M., Proietti M., et al. (2015). The vertical distribution of buoyant plastics at sea: an observational study in the North Atlantic Gyre. Biogeosciences 12, 1249–1256. doi: 10.5194/bg-12-1249-2015
Remy F., Collard F., Gilbert B., Compère P., Eppe G., Lepoint G. (2015). When microplastic is not plastic: the ingestion of artificial cellulose fibers by macrofauna living in seagrass macrophytodetritus. Environ. Sci. Technol. 49, 11158–11166. doi: 10.1021/acs.est.5b02005
Richardson K., Hardesty B. D., Vince J. Z., Wilcox C. (2021). Global causes, drivers, and prevention measures for lost fishing gear. Front. Mar. Sci. 8. doi: 10.3389/fmars.2021.690447
Rios L. M., Moore C., Jones P. R. (2007). Persistent organic pollutants carried by synthetic polymers in the ocean environment. Mar. pollut. Bull. 54, 1230–1237. doi: 10.1016/j.marpolbul.2007.03.022
Rocha-Santos T., Duarte A. C. (2015). A critical overview of the analytical approaches to the occurrence, the fate and the behavior of microplastics in the environment. TrAC - Trends Anal. Chem. 65, 47–53. doi: 10.1016/j.trac.2014.10.011
Rochwulaningsih Y., Sulistiyono S. T., Masruroh N. N., Maulany N. N. (2019). Marine policy basis of Indonesia as a maritime state: The importance of integrated economy. Mar. Policy 108. doi: 10.1016/j.marpol.2019.103602
Rustiah W., Noor A., Gaffar M., Lukman M. (2018). Spatial and temporal variation of the total suspended solid in the South Sulawesi coastal waters. Mar. Chim. Acta 19, 59–65. doi: 10.20956/mca.v19i2.5391
Ryan P. G. (2018). Entanglement of birds in plastics and other synthetic materials. Mar. pollut. Bull. 135, 159–164. doi: 10.1016/j.marpolbul.2018.06.057
Ryan P. G., Suaria G., Perold V., Pierucci A., Bornman T. G., Aliani S. (2020). Sampling microfibres at the sea surface: The effects of mesh size, sample volume and water depth. Environ. pollut. 258. doi: 10.1016/j.envpol.2019.113413
Salamena G. G., Heron S. F., Ridd P. V., Whinney J. C. (2023). A risk assessment of marine plastics in coastal waters of a small island: Lessons from Ambon Island, eastern Indonesia. Reg. Stud. Mar. Sci. 65. doi: 10.1016/j.rsma.2023.103086
Sheavly S. B., Register K. M. (2007). Marine debris & plastics: Environmental concerns, sources, impacts and solutions. J. Polym. Environ. 15, 301–305. doi: 10.1007/s10924-007-0074-3
Shim W. J., Hong S. H., Eo S. E. (2017). Identification methods in microplastic analysis: A review. Anal. Methods 9, 1384–1391. doi: 10.1039/C6AY02558G
Siegfried M., Koelmans A. A., Besseling E., Kroeze C. (2017). Export of microplastics from land to sea. A modelling approach. Water Res. 127, 249–257. doi: 10.1016/j.watres.2017.10.011
Song Y. K., Hong S. H., Eo S., Jang M., Han G. M., Isobe A., et al. (2018). Horizontal and vertical distribution of microplastics in korean coastal waters. Environ. Sci. Technol. 52, 12188–12197. doi: 10.1021/acs.est.8b04032
Sprintall J., Gordon A. L., Wijffels S. E., Feng M., Hu S., Koch-Larrouy A., et al. (2019). Detecting change in the Indonesian seas. Front. Mar. Sci. 6. doi: 10.3389/fmars.2019.00257
Sprintall J., Wijffels S. E., Molcard R., Jaya I. (2009). Direct estimates of the Indonesian throughflow entering the Indian ocean: 2004-2006. J. Geophys. Res. Ocean. 114. doi: 10.1029/2008JC005257
Statista (2023) Contribution of fisheries to the gross domestic product (GDP) in Indonesia from 2014 to 2021. Available online at: https://www.statista.com/statistics/1083946/Indonesia-fisheries-contribution-to-gdp/ (Accessed May 10, 2023).
Statistics Indonesia (2021) Hasil sensus penduduk 2020 (Jakarta, Indonesia). Available online at: https://www.bps.go.id/pressrelease/2021/01/21/1854/hasil-sensus-penduduk-2020.html (Accessed January 1, 2022).
Steer M., Cole M., Thompson R. C., Lindeque P. K. (2017). Microplastic ingestion in fish larvae in the western English Channel. Environ. pollut. 226, 250–259. doi: 10.1016/j.envpol.2017.03.062
Suaria G., Achtypi A., Perold V., Lee J. R., Pierucci A., Bornman T. G., et al. (2020). Microfibers in oceanic surface waters: A global characterization. Sci. Adv. 6. doi: 10.1126/sciadv.aay8493
Sulistyowati L., Nurhasanah, Riani E., Cordova M. R. (2022). The occurrence and abundance of microplastics in surface water of the midstream and downstream of the Cisadane River, Indonesia. Chemosphere 291, 133071. doi: 10.1016/j.chemosphere.2021.133071
Suryantini S., Ismanto A., Aji I. K., Saputri D. F., Helfinalis H. (2011). Marine sediment characteristics at karimun java sea based on stratigraphic profile analysis, total suspended solid (Tss) and grain-size analysis (Granulometry). J. Ilmu dan Teknol. Kelaut. Trop. 3. doi: 10.28930/jitkt.v3i1.7832
Tarigan M. S., Edward E. (2010). Kandungan total zat padat tersuspensi (Total suspended solid) di perairan raha, sulawesi tenggara. MAKARA Sci. Ser. 7. doi: 10.7454/mss.v7i3.362
ten Brink P., Schweitzer J.-P., Watkins E., Janssens C., De Smet M., Leslie H., et al. (2018). “Circular economy measures to keep plastics and their value in the economy, avoid waste and reduce marine litter,” in G20 Germany 2017 think 20 dialogue, vol. 12. (Berlin, Germany).
Thompson R. C., Olson Y., Mitchell R. P., Davis A., Rowland S. J., John A. W. G., et al. (2004). Lost at sea: where is all the plastic? Sci. (80-.). 304, 838. doi: 10.1126/science.1094559
Toussaint B., Raffael B., Angers-Loustau A., Gilliland D., Kestens V., Petrillo M., et al. (2019). Review of micro- and nanoplastic contamination in the food chain. Food Addit. Contam. - Part A Chem. Anal. Control. Expo. Risk Assess. 36, 639–673. doi: 10.1080/19440049.2019.1583381
Van Sebille E., Wilcox C., Lebreton L., Maximenko N., Hardesty B. D., Van Franeker J. A., et al. (2015). A global inventory of small floating plastic debris. Environ. Res. Lett. 10. doi: 10.1088/1748-9326/10/12/124006
Vriend P., Hidayat H., van Leeuwen J., Cordova M. R., Purba N. P., Löhr A. J., et al. (2021). Plastic pollution research in Indonesia: state of science and future research directions to reduce impacts. Front. Environ. Sci. 9. doi: 10.3389/fenvs.2021.692907
Walkinshaw C., Lindeque P. K., Thompson R., Tolhurst T., Cole M. (2020). Microplastics and seafood: lower trophic organisms at highest risk of contamination. Ecotoxicol. Environ. Saf. 190, 1–14. doi: 10.1016/j.ecoenv.2019.110066
Wang G., Lu J., Li W., Ning J., Zhou L., Tong Y., et al. (2021). Seasonal variation and risk assessment of microplastics in surface water of the Manas River Basin, China. Ecotoxicol. Environ. Saf. 208. doi: 10.1016/j.ecoenv.2020.111477
Wang W., Ndungu A. W., Li Z., Wang J. (2017). Microplastics pollution in inland freshwaters of China: A case study in urban surface waters of Wuhan, China. Sci. Total Environ. 575, 1369–1374. doi: 10.1016/j.scitotenv.2016.09.213
Watkins E., ten Brink P., Withana S., Mutafoglu K., Schweitzer J.-P., Russi D., et al. (2015). Marine litter: socio-economic study. (Nairobi, Kenya: UNEP).
Weekes J. G., Wasil J. C. M., Llamas K. M., Agrinzoni C. M. (2021). Solid waste management system for small island developing states. Glob. J. Environ. Sci. Manage. 7, 259–272. doi: 10.22034/gjesm.2021.02.08
Williams A. T., Rangel-Buitrago N. (2019). Marine litter: Solutions for a major environmental problem. J. Coast. Res. 35, 648–663. doi: 10.2112/JCOASTRES-D-18-00096.1
Wong G., Löwemark L., Kunz A. (2020). Microplastic pollution of the Tamsui River and its tributaries in northern Taiwan: Spatial heterogeneity and correlation with precipitation. Environ. pollut. 260. doi: 10.1016/j.envpol.2020.113935
Wright S. L., Kelly F. J. (2017). Plastic and human health: A micro issue? Environ. Sci. Technol. 51, 6634–6647. doi: 10.1021/acs.est.7b00423
Wu Y., Wang S., Wu L., Yang Y., Yu X., Liu Q., et al. (2022). Vertical distribution and river-sea transport of microplastics with tidal fluctuation in a subtropical estuary, China. Sci. Total Environ. 822. doi: 10.1016/j.scitotenv.2022.153603
Xia W., Rao Q., Deng X., Chen J., Xie P. (2020). Rainfall is a significant environmental factor of microplastic pollution in inland waters. Sci. Total Environ. 732. doi: 10.1016/j.scitotenv.2020.139065
Xue B., Zhang L., Li R., Wang Y., Guo J., Yu K., et al. (2020). Underestimated microplastic pollution derived from fishery activities and “Hidden” in deep sediment. Environ. Sci. Technol. 54, 2210–2217. doi: 10.1021/acs.est.9b04850
Yang D., Shi H., Li L., Li J., Jabeen K., Kolandhasamy P. (2015). Microplastic pollution in table salts from China. Environ. Sci. Technol. 49, 13622–13627. doi: 10.1021/acs.est.5b03163
Yin L., Wen X., Huang D., Du C., Deng R., Zhou Z., et al. (2021). Interactions between microplastics/nanoplastics and vascular plants. Environ. pollut. 290, 117999. doi: 10.1016/j.envpol.2021.117999
Yong M. M. H., Leistenschneider C., Miranda J. A., Paler M. K., Legaspi C., Germanov E., et al. (2021). Microplastics in fecal samples of whale sharks (Rhincodon typus) and from surface water in the Philippines. Microplastics Nanoplastics 1. doi: 10.1186/s43591-021-00017-9
Yu Z., Song S., Xu X., Ma Q., Lu Y. (2021). Sources, migration, accumulation and influence of microplastics in terrestrial plant communities. Environ. Exp. Bot. 192, 104635. doi: 10.1016/j.envexpbot.2021.104635
Yuan D., Corvianawatie C., Cordova M. R., Surinati D., Li Y., Wang Z., et al. (2023). Microplastics in the tropical Northwestern Pacific Ocean and the Indonesian seas. J. Sea Res. 194, 102406. doi: 10.1016/j.seares.2023.102406
Zheng Y., Li J., Sun C., Cao W., Wang M., Jiang F., et al. (2021). Comparative study of three sampling methods for microplastics analysis in seawater. Sci. Total Environ. 765. doi: 10.1016/j.scitotenv.2020.144495
Keywords: microplastics, sub-surface, pump filtration, archipelagic, Indonesia, distribution, abundance, characteristics
Citation: Cordova MR, Iskandar MR, Surinati D, Kaisupy MT, Wibowo SPA, Subandi R, Ulumuddin YI, Purbonegoro T, Yogaswara D, Sani SY, Puspitasari R, Rositasari R, Riani E, Zheng S, Sun X and Wang Z (2024) Microplastic occurrence in sub-surface waters of the Indonesian archipelago. Front. Mar. Sci. 11:1362414. doi: 10.3389/fmars.2024.1362414
Received: 28 December 2023; Accepted: 21 February 2024;
Published: 20 March 2024.
Edited by:
Teresa Bottari, National Research Council (CNR), ItalyReviewed by:
Jheng-Jie Jiang, Chung Yuan Christian University, TaiwanGiuseppe Suaria, National Research Council, Italy
Copyright © 2024 Cordova, Iskandar, Surinati, Kaisupy, Wibowo, Subandi, Ulumuddin, Purbonegoro, Yogaswara, Sani, Puspitasari, Rositasari, Riani, Zheng, Sun and Wang. This is an open-access article distributed under the terms of the Creative Commons Attribution License (CC BY). The use, distribution or reproduction in other forums is permitted, provided the original author(s) and the copyright owner(s) are credited and that the original publication in this journal is cited, in accordance with accepted academic practice. No use, distribution or reproduction is permitted which does not comply with these terms.
*Correspondence: Muhammad Reza Cordova, muhammad.reza.cordova@brin.go.id; cordova@marpol.id; Zheng Wang, wangzheng@qdio.ac.cn