- 1University of the Virgin Islands, Center for Marine and Environmental Studies, St. Thomas, VI, United States
- 2University of Miami, Rosenstiel School of Marine, Atmospheric, and Earth Science, Miami, FL, United States
- 3Water Research Group, Unit of Environmental Sciences and Management, North-West University, Potchefstroom, South Africa
Because they serve as the main architects of coral reefs, the distribution and abundance of stony coral species have major impacts on other associated community members. Thus, coral diseases can have significant cascading effects throughout the ecosystem. Stony coral tissue loss disease (SCTLD) is the most recent of many diseases documented to impact Caribbean stony corals. SCTLD is known to impact over 20 species of reef-building corals and can cause complete colony mortality of large corals in only one month. Among the coral species impacted are those occupied as cleaning stations by Caribbean cleaner gobies. This study examined the persistence of these gobies on living coral cleaning stations where SCTLD was most recently or not yet affected (emergent), recently established (epidemic), and well-established (endemic),. Timed surveys were conducted at nine reefs in the U.S. Virgin Islands between October 2019 and March 2021. Study sites were surveyed both before and after the outbreak/establishment of SCTLD where possible. Monitoring sites were established at six reefs by tagging 25 live coral cleaning stations at each of two endemic and two epidemic sites and 50 cleaning stations at each of two emergent sites. Goby abundance at each site was monitored at least five times from March 2020 to April 2021. Timed surveys found cleaner goby abundance was 50% lower in the endemic zone compared to epidemic and emergent zones. Overall, goby abundance declined on tagged cleaning stations throughout the course of this study at all sites. However, overall goby density within monitored areas remained stable across most sites from the beginning to the end of the study. One emergent site experienced a two-fold increase in goby density and one epidemic site experienced a decline in goby density. This suggests that gobies are remaining at the site but may be abandoning live coral cleaning stations as the individual colonies are affected by SCTLD. Given the benefit cleaner gobies have on local coral reef fishes, changes in cleaning activity associated with coral disease have the potential to negatively impact Caribbean reef fish communities.
1 Introduction
Coral reefs are complex and productive ecosystems that support a multitude of species (Marsh, 1977; Muscatine and Porter, 1977; D’Elia et al., 1981; Dizon and Yap, 2006; Paytan et al., 2006). Because they are the chief architects of these ecosystems, changes in the distribution and abundance of stony corals can have major cumulative effects throughout the ecosystem (Jones et al., 2004; Graham et al., 2007; Pratchett et al., 2008). Anthropogenic stressors combined with the emergence and spread of novel diseases have significantly impacted stony corals in recent decades, leading to losses of coral cover and localized extinctions (Dizon and Yap, 2006; Carpenter et al., 2008; Cerrano et al., 2013; Neely et al., 2021). There has been considerable effort to understand the broader community impacts of the resulting loss of coral cover (Jones et al., 2004; Graham et al., 2007; Pratchett et al., 2008).
Coral reef communities support and depend on myriad symbiotic interactions. This includes mutualisms involving the corals themselves (Knowlton and Rohwer, 2003), such as that between the coral polyp and their algal symbionts of the family Symbiodiniaceae, as well as those involving other invertebrates and fishes (Hata and Kato, 2006; Caves, 2021). Arguably one of the most iconic and recognizable among these symbioses involves cleaner fishes and shrimps (Losey, 1974; Côté, 2000; Vaughan et al., 2017; Caves, 2021). Cleaners remove ectoparasites and other material from other reef organisms, referred to as “clients”. Unlike terrestrial mutualisms, cleaning assemblages typically involve a few cleaner species that serve a diverse client group (Guimarães et al., 2007; Sazima et al., 2010; Caves, 2021). Mainly because of their role as consumers of parasites (Grutter, 1999; Grutter et al., 2008; Côté and Soares, 2011; Grutter et al., 2018; Triki et al., 2018; Caves, 2021) the presence of these cleaners has extensive and multiple community-level impacts. These include impacts on fish movement and habitat utilization (Sikkel et al., 2004, Sikkel et al., 2005), decreased client stress (Bshary et al., 2007; Côté and Soares, 2011; Soares et al., 2011; Triki et al., 2016; Grutter et al., 2018; Caves, 2021), overall health of clients (Côté and Soares, 2011), and enhanced local fish diversity, abundance, and biomass (Bshary, 2003; Grutter et al., 2003; Côté and Soares, 2011; Waldie et al., 2011). Thus, these cleaners act as “keystone species” (Sazima et al., 2010; Di Santo and Lobel, 2017; Whittey et al., 2021), having community effects that are disproportionate to their biomass (Paine, 1969).
Cleaner fishes include facultative cleaners that clean opportunistically or only for part of their lifecycle, and obligate or dedicated cleaners, which are specialists that preferentially obtain their food from cleaning throughout their lifecycle (Côté, 2000; Whiteman and Côté, 2002; Grutter and Feeney, 2016; Vaughan et al., 2017; Huie et al., 2020; Nicholson et al., 2024). This group is represented by cleaner wrasses (Labroides spp.) in the tropical Indo-Pacific, and cleaner gobies (Elacatinus spp.) in the tropical western Atlantic and eastern Pacific (Côté, 2000; Floeter et al., 2007; Côté and Soares, 2011; Grutter and Feeney, 2016; Vaughan et al., 2017). Cleaning behavior in these two genera evolved independently, but their coloration is convergent and readily recognized by client fish species (Stummer et al., 2004; Cheney et al., 2009). While these two species share many similarities, cleaner wrasses have been more thoroughly studied and thus are better understood than cleaner gobies (Côté and Soares, 2011).
Caribbean cleaner gobies are benthic, spending most of their time on the substrate, and rarely travel more than a meter from the substrate to interact with a client (Côté and Soares, 2011). Thus, characteristics of the benthic substrate can impact their distribution (Whiteman and Côté, 2002; White et al., 2007; Whittey et al., 2021). Previous studies demonstrate that, while they can occupy sponge and dead coral, cleaner gobies appear to show a preference for living “boulder” corals such as the stony coral species Siderastrea siderea, Montastraea cavernosa, Orbicella annularis, Pseudodiploria strigosa, but they can also be found on smaller-sized stony corals such as Porites astreoides and Agaricia agaricites, as well as on the hydrozoan Millepora squarrosa (Whiteman and Côté, 2002, Whiteman and Côté, 2004; White et al., 2007; Whittey et al., 2021). Some other fish in the region clean facultatively (Johnson and Ruben, 1988; Côté and Soares, 2011; Quimbayo et al., 2017; Dunkley et al., 2018), and other dedicated cleaner organisms, such as shrimps, exist in the region (McCammon et al., 2010; Soares et al., 2011; Huebner and Chadwick, 2012; Titus et al., 2015). However, cleaner gobies are the most consistent consumers of parasites and serve a larger, more diverse client group (Sazima et al., 2010; Dunkley et al., 2019). cleaner gobies are known to clean piscivorous fishes more often than other cleaners and are the only Caribbean cleaners known to interact with elasmobranchs, including several species of shark (Côté and Soares, 2011).
The novel stony coral tissue loss disease (SCTLD) was first documented off the coast of Florida near Miami in 2014 and has now spread throughout the Caribbean region (Miller et al., 2016; Precht et al., 2016; Walton et al., 2018; Gintert et al., 2019). It was first observed in the U.S. Virgin Islands (USVI) in January 2019 at Flat Cay, a reef site off the southwest coast of St. Thomas (Brandt et al., 2021). It spread rapidly to surround the island, leaving few unaffected areas by March of 2020 and had reached all islands within the territory by September of 2020 (Brandt et al., 2021; VI-CDAC, n.d.).
SCTLD is unlike many previously described coral diseases because of its ability to affect many species of stony corals, its high mortality rate, and its unrelenting spread (Brandt et al., 2021). Most other Caribbean coral diseases (e.g., white-band, yellow band, dark spot, black band) mainly affect only a few Caribbean coral species, rarely kill entire colonies, and are often limited temporally or spatially in spread (Ritchie and Smith, 1998; Cervino et al., 2001; Miller et al., 2009; Weil et al., 2009; Brandt et al., 2021). Due to its wide host range, high mortality rates, and persistent spread, significant declines in coral diversity and density as a result of SCTLD (Walton et al., 2018; Brandt et al., 2021; Costa et al., 2021).
SCTLD is currently known to impact more than 20 species of reef-building coral (Florida Coral Disease Response Research & Epidemiology Team, 2018; Landsberg et al., 2020) and can cause complete colony death of large corals in as little as one month (Precht et al., 2016; Walton et al., 2018). On St. Thomas, more diverse sites experienced greater loss of species diversity but less decline in coral cover (Costa et al., 2021). This indicated that smaller, already rare species were lost in these areas while the larger and more common species, such as S. siderea and Orbicella spp. which exhibit slower lesion rates, remained (Brandt et al., 2021; Costa et al., 2021). As Caribbean coral reefs decline further due to the recent outbreak of SCTLD, the implications of this disease on cleaning goby populations, and thus ectoparasite and reef fish assemblages, are unknown. Among the coral species commonly occupied by cleaner gobies, one is highly susceptible to SCTLD (P. strigosa), and three are moderately susceptible (S. siderea, M. cavernosa, and O. annularis) in this region (Costa et al., 2021). Juvenile gobies have been documented on two additional species of stony corals, Diploria labyrinthiformis and Colpophyllia natans, which are highly susceptible to SCTLD (Whiteman and Côté, 2002, 2004; Florida Coral Disease Response Research & Epidemiology Team, 2018; Landsberg et al., 2020; Muller et al., 2020; Costa et al., 2021).
Through this study, we utilized the natural experiment presented by the emergence and expansion of SCTLD through the northern USVI to examine the persistence of cleaning gobies at coral reef sites in various stages of SCTLD outbreak. We hypothesized that SCTLD would have a noticeable impact on the abundance of Elacatinus spp. and the distribution patterns of their living coral cleaning stations.
2 Methods
2.1 Site selection and study species
Study sites were selected based on the availability of historical data from the U.S. Virgin Islands Territorial Coral Reef Monitoring Program (TCRMP) (Ennis et al., 2020) and data on SCTLD distribution that was tracked weekly by the Virgin Islands Coral Disease Advisory Committee (Costa et al., 2021; VI-CDAC, n.d.). TCRMP has monitored sites in the USVI annually since 2002. TCRMP methodology captures long-term baseline data on the benthic cover, coral and fish species abundance, and diversity. Additionally, coral health metrics are recorded following modified Atlantic and Gulf Rapid Reef Assessment (AGRRA) methodology (Ennis et al., 2020). TCRMP sites have six permanent transects at each site where a diver on SCUBA records video of the benthos, maintaining a distance of 0.4 m above the substrate through use of a guide. Twenty-five images per transect are then captured from these videos, representing an area of approximately 0.312 m. On each image 20 random points are generated using either Coral Point Count with Excel Extension (CPCe, used prior to 2019) (Kohler and Gill, 2006) or R statistical analysis “imager” (Barthelme et al., 2020) and “spatstat” (Baddeley et al., 2015). The substrate under each point are identified and coral cover calculated for each transect (Ennis et al., 2020; Brandt et al., 2021). Total living coral cover and coral cover by coral species calculated for each transect were used in this study.
Three distinct phases of SCTLD on an affected reefs were recognized by VI-CDAC and defined in Costa et al. (2021). These included emergent, epidemic, and endemic phases characterized by the timing of SCTLD affecting a site, and sites were categorized into zones based on these phases. The “Emergent Zone”, or disease front, included sites with no disease present but were near the disease front or where only highly susceptible species showed signs of disease, indicating a recent emergence of SCTLD estimated to be within one month of disease arriving at the site. The “Epidemic Zone” included sites experiencing peak prevalence. In this zone, highly susceptible species were still prevalent and were highly affected, but the moderately and low susceptible species including Orbicella spp. and Porites astreoides were also showing signs of disease. In the epidemic zone, it was estimated that the disease had been present between one and nine months. Lastly, at sites in the “Endemic Zone”, Orbicella spp. were well beyond initial onset and most highly susceptible species had experienced significant mortality from disease, or may have been lost from the site entirely. This phase is estimated to represent the time period of greater than nine months from the first signs of disease (Costa et al., 2021). Survey sites were chosen from each of these disease zones (emergent, epidemic, and endemic) at the time of the surveys or long-term study site establishment. The timeline of SCTLD affecting sites and characterization of sites into the zones was based on weekly, territorial-wide monitoring and reporting of the disease (Brandt et al., 2021; Costa et al., 2021; VI-CDAC, n.d.). All sites were represented by diverse fringing reefs in 5-20 m depth (Figure 1; Tables 1 and 2).
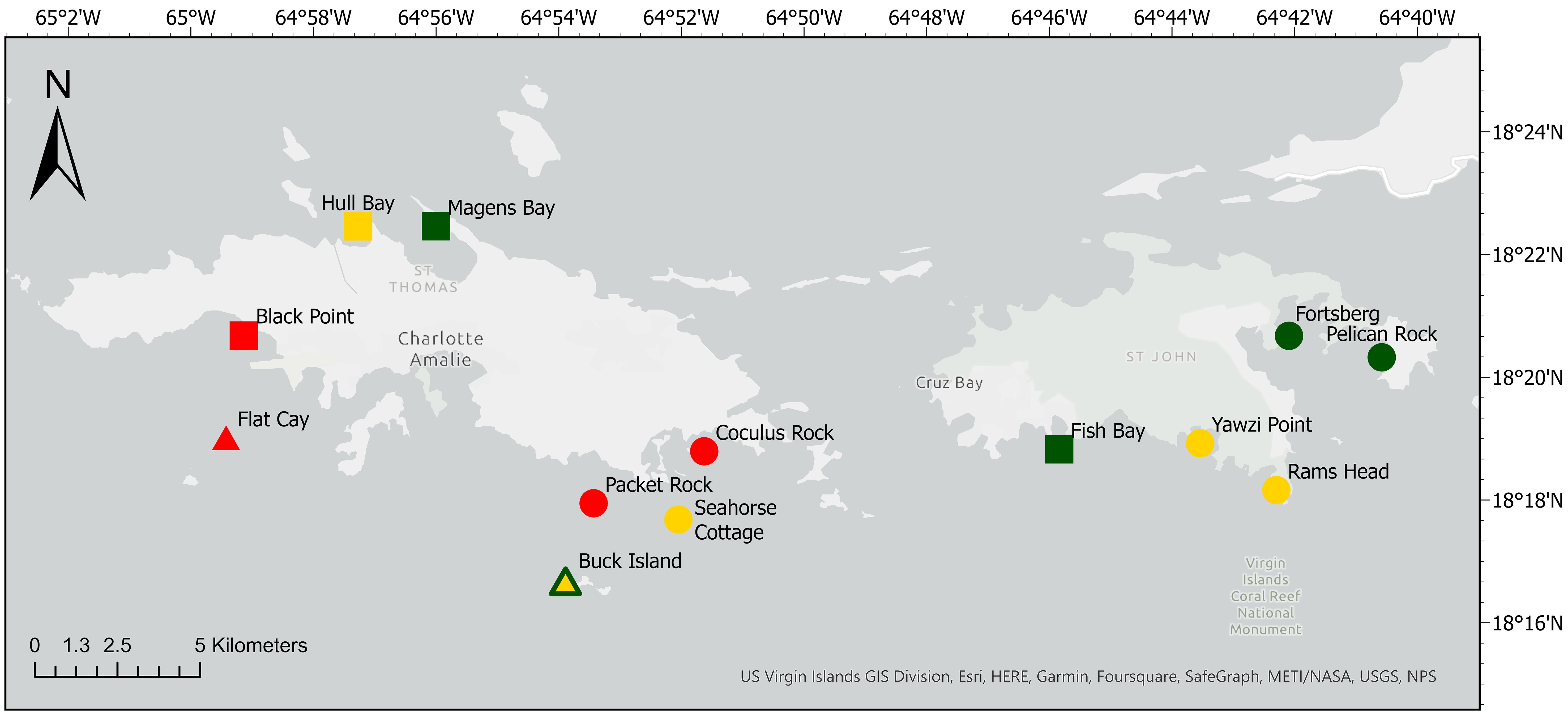
Figure 1 Cleaning goby and coral health assessment sites off the coast of St. Thomas and St. John, USVI. SCTLD disease zones were endemic (red), epidemic (yellow), and emergent (green). Survey types included rapid assessment (circle), long-term monitoring (square), or both (triangle). When a site was included in both survey types, and disease zone changed between surveys, the border color represents disease zone for the rapid assessment, and the fill color represents disease zone for the long-term monitoring survey.
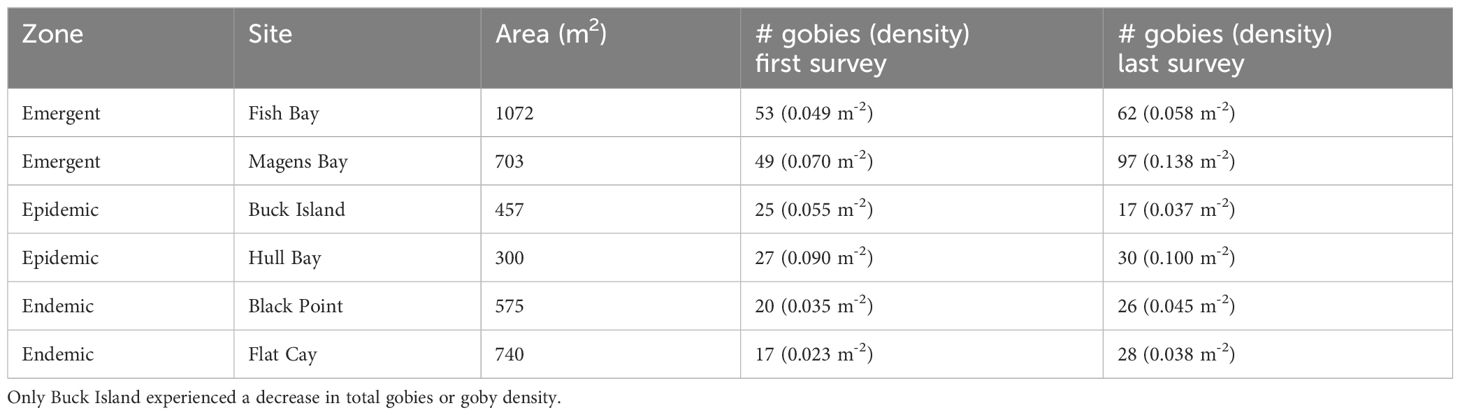
Table 2 Number of gobies and goby density (# m-2) during first and last area surveys at six long-term monitoring sites within three disease zones.
2.2 Goby and coral health assessment
Rapid goby population assessments were conducted by two divers who swam three meters apart in a haphazard pattern across the reef for 50 - 60 min and searched for the presence of Elacatinus spp. on corals, sponges, and other substrates. When a goby was sighted, the coral head or other substrate was searched and the number and size ( ± 1 mm) of gobies, depth, and substrate type were recorded, and a photo was taken of the cleaning station. If the substrate type was live coral, then coral species, presence/absence of SCTLD, percent (%) recent mortality associated with SCTLD lesions, and coral width, length, and height ( ± 1 cm) were recorded.
At each long-term monitoring site, divers established one or two 25-m reference transects and marked the beginning and ending points with sub-surface buoys tied to dead coral. Live coral cleaning stations occupied by Elacatinus spp. cleaner gobies were located by divers who swam in a systematic search pattern along reference transects at each site. All corals, sponges, and other substrate types were carefully searched, and when a cleaning station was located, the distance along the transect and distance to left or right of the transect were recorded to relocate goby cleaning stations in future surveys. Each coral head with a cleaning station was tagged with a unique number plate hammered into adjacent dead substrate with a masonry nail and flagging tape, data recorded as described above. For endemic and epidemic sites, 25 cleaning stations on healthy corals were tagged. At emergent sites, 50 healthy cleaning stations were tagged to increase the probability of capturing disease stages from initial signs through colony death during the study. After corals were tagged, health data were taken following the National Oceanographic and Atmospheric Administration (NOAA) National Coral Reef Monitoring Program (NCRMP) coral demographic methods (Coral Demographics Survey Protocol for the Atlantic Region: U.S. Caribbean, Florida and Gulf of Mexico: 2018, 2020). The presence, number, and size of gobies as well as condition of corals were reassessed at least five times over the following seven months (Table 1). During each subsequent survey, transect tapes were reattached to buoy lines and datasheets with goby cleaning station location were used to relocate marked coral heads. Coral health was reassessed, corals were photographed, and the number of gobies and their sizes were recorded. If no gobies were seen, a two-meter radius around the original coral head was searched for gobies that may have relocated to another coral or sponge. If a goby of similar size was found, the alternate location was recorded.
To evaluate the persistence of cleaning gobies around the marked cleaning stations, area surveys were conducted during the last survey of tagged corals at each site. These surveys consisted of delineating the study area with transect tapes so that the most distant tagged corals to the left and right side of the original transect defined the rectangular population-survey area. All live and dead corals, sponges and other substrate types were systematically searched for the presence of cleaner gobies. When a goby was located, data were recorded as described above (Coral Demographics Survey Protocol for the Atlantic Region: U.S. Caribbean, Florida and Gulf of Mexico: 2018, 2020).
2.3 Data analysis
Due to SCUBA diving limitations, timed surveys at deeper sites (i.e., Buck Island STT, Seahorse) were a few minutes short of the full 60 minutes (Table 1). Thus, disease zones were compared using gobies encountered per minute. These data were analyzed with a 1-way Analysis of Variance (ANOVA) to test for an effect of disease zone. Area surveys, unrestricted by time, at long-term monitoring sites were used to calculate the density of gobies among sites and disease zones during the initial and final surveys, a 9-to-12-month period (Table 1). Since timed surveys and area surveys used a similar methodology and collected comparable data while searching for goby-occupied corals, we compared the average size of Elacatinus spp. and the number of gobies per cleaning station using a 2-way ANOVA with disease zone and survey type (timed vs. area) as independent variables. These results were not significant for survey type. Therefore, data were combined and the number of gobies per cleaning station and goby lengths were each tested among disease zones with 1-way ANOVAs. For long-term monitoring surveys of tagged goby cleaning stations, recruitment of juvenile gobies after the initial population survey may have affected persistence estimates over time. Therefore, to standardize persistence data we excluded likely recruits (TL < 13 mm) arriving after the first survey from analysis (Supplementary Table 1).
Use of habitat by cleaner gobies was calculated using percent cover values from TCRMP data and goby occupation from this study. We applied Ivlev’s Electivity Index because certain habitat options utilized by gobies were so scarce that they were not detectible by TCRMP methods, resulting in a recorded zero value for those habitat types. Ivlev’s Electivity Index assigns the maximum selection value (1) to those habitats that are occupied but have a cover value of zero (Manly et al., 1993). Electivity indices were only calculated for Fish Bay, Magens Bay, Buck Island STT, and Black Point where both TCRMP and long-term monitoring data were available.
3 Results
3.1 Goby abundance among zones
Goby encounter rates were significantly different among SCTLD disease zones (F2.6 = 12.97, p<0.007). Endemic reef sites had 50% fewer Elacatinus spp. (0.31 ± 0.015 SE gobies min-1 search time) compared to epidemic (0.61 ± 0.076 SE gobies min-1) and emergent (0.67 ± 0.055 SE gobies min-1) sites (Figure 2).
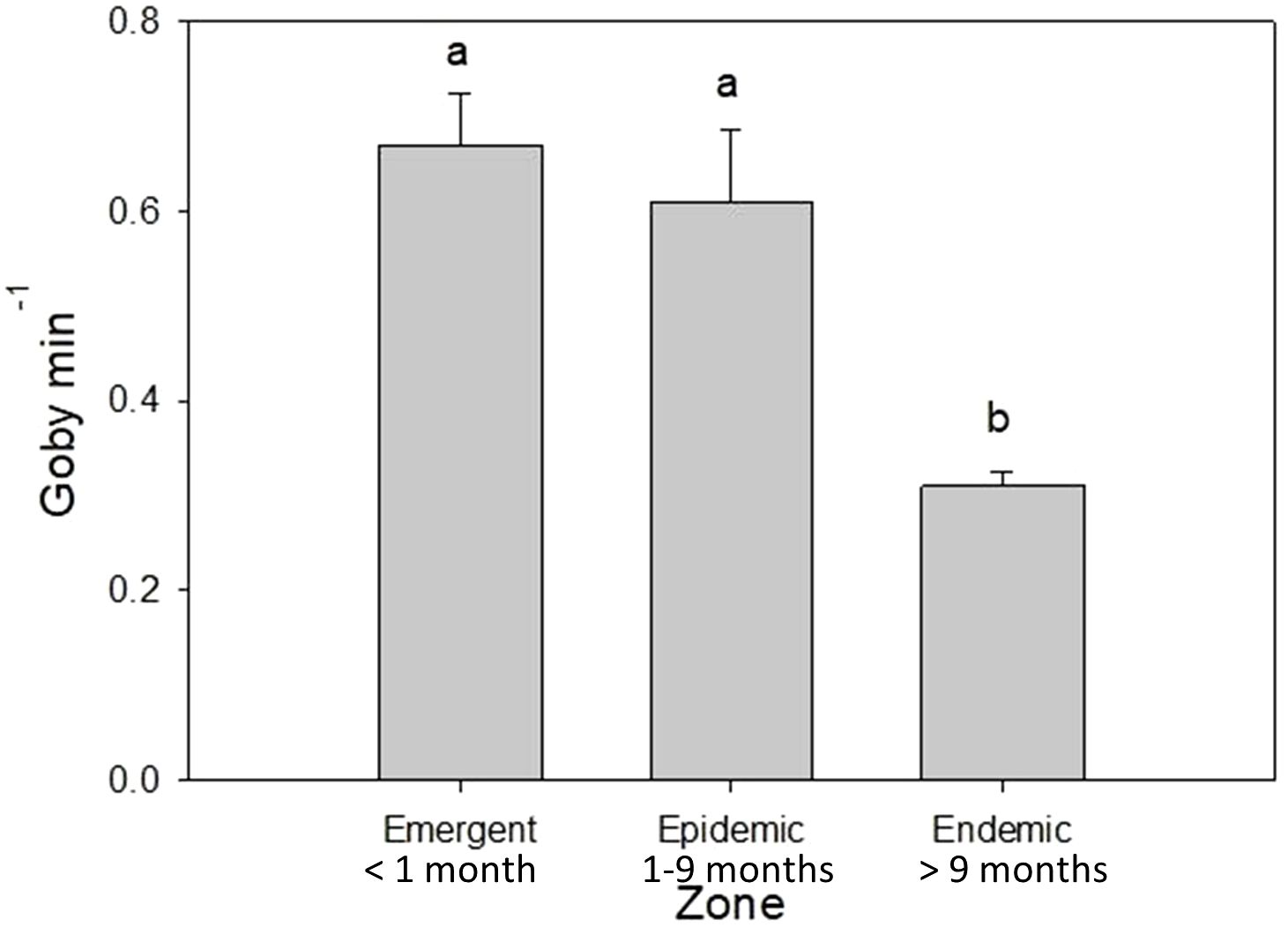
Figure 2 Rapid assessment surveys of cleaning goby abundance at emergent, epidemic, and endemic SCTLD disease zones conducted throughout the study. Zones were designated based on the disease status at each surveyed site at the time of the survey: Endemic – SCTLD present > 9 months, Epidemic – SCTLD present 1-9 months, Emergent – SCTLD not present but expected or present < 1 month. Bars with different letters indicate statistically significant differences at p < 0.01.
Areas surveyed at monitoring sites for total goby population varied from 300 m2 to 1072 m2 (Table 1). Goby density ranged from 0.023 m-2 at Flat Cay and 0.09 m-2 at Hull Bay during initial surveys to 0.037 m-2 at Buck Island and 0.138 m-2 at Magens Bay during final surveys (Table 1). Changes in density from first to last survey varied across sites but were relatively similar between sites on the same side of the islands (North or South). All observed density losses occurred at sites on the south side of St. Thomas or St. John and the greatest increases in density occurred at the northern sites (Magens Bay and Hull Bay, Figures 1 and 3). The most notable decrease in goby density occurred at Buck Island, St. Thomas where decreases were observed across all life stages and persistence of gobies >13 mm was lowest (Figure 3). Low settlement, immigration and persistence of juveniles and adults resulted in negative changes in density at this epidemic site which experienced high coral mortality from SCTLD (Table 1; Figure 3). This site is the furthest away from the shoreline of St. Thomas or St. John (Figure 1). Changes in density were influenced by goby life history stages where larval settlement, persistence of all life stages, and immigration of gobies from degrading habitat resulted in positive or negative changes in density from first to last surveys at most sites (Figure 3).
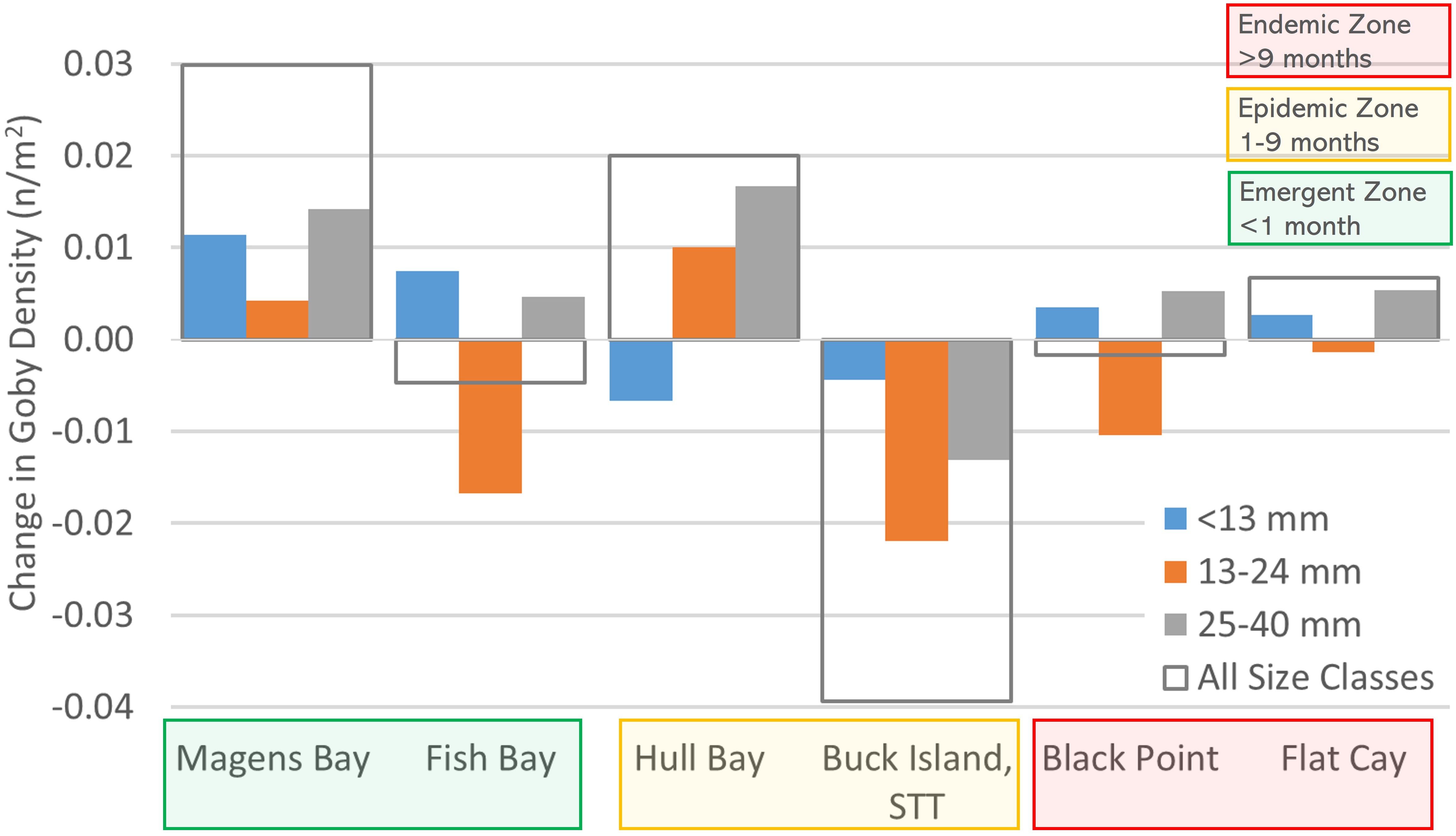
Figure 3 Change in goby density (n/m-2) from first survey to last survey at each site by size class and overall. Reductions observed in smaller size categories may be attributed to factors such as predation or the transition of smaller gobies into larger size classes.
Similar to other studies, this study confirmed goby occupation of live coral more often than expected by chance when accounting for available habitat area (Supplementary Figure 6). Combined data for rapid assessment surveys and area surveys at monitoring sites showed that the number of Elacatinus spp. per live coral cleaning station was not significantly different among disease zones (F = 0.15, P = 0.86) and averaged 1.4 gobies/coral ± 0.06. The maximum number of gobies per coral head ranged from 1 – 5 at emergent sites, 1 – 7 at epidemic sites and 1-8 at endemic sites (Supplementary Figure 1). The percent frequency of gobies per coral head was nearly identical among disease zones (Supplementary Figure 1).
Long term monitoring data revealed no relationship between the number of gobies per coral head and coral head maximum diameter (r2 = 0.05) or surface area (r2 = 0.04). Coral species that hosted greater than three Elacatinus spp. (>13mm) per cleaning station included M. cavernosa (n = 6, max = 6 gobies), Siderastrea siderea (n = 4, max = 5 gobies), O. faveolata (n = 1, max = 4), O. franksi (n = 1, max = 4) and C. natans (n = 5 max = 7). Coral species that hosted three or less Elacatinus spp. per cleaning station included D. labyrinthiformis (n = 12, max = 3), O. annularis (n =10, max = 3), P. astreoides (n = 4, max = 1), and P. strigosa (n = 12, max = 3).
3.2 Distribution of Elacatinus spp. among coral species
Results of both long-term monitoring sites and rapid assessments showed similar Elacatinus spp. distribution patterns among live coral cleaning stations (Figures 4, 5). Live stony coral cleaning stations mostly consisted of M. cavernosa, S. siderea and O. faveolata across disease zones (Figures 4, 5). M. cavernosa was the most frequently used coral in endemic sites, more than double the percent frequency of any other live coral species (Figures 4, 5). Use of sponges and gorgonians was greater in epidemic and endemic zones when compared to emergent zones while use of some major reef building corals, such as Orbicella spp. and C. natans, was less in the epidemic and endemic zones (Figures 4, 5). In this study, adult cleaner gobies were also observed to maintain cleaning stations on previously unreported or underreported coral species, D. labyrinthiformes and C. natans, which are highly susceptible to SCTLD, and two additional moderately susceptible species, O. faveolata and O. franksi (Whiteman and Côté, 2002, Whiteman and Côté, 2004; Florida Coral Disease Response Research & Epidemiology Team, 2018; Landsberg et al., 2020; Muller et al., 2020; Costa et al., 2021).
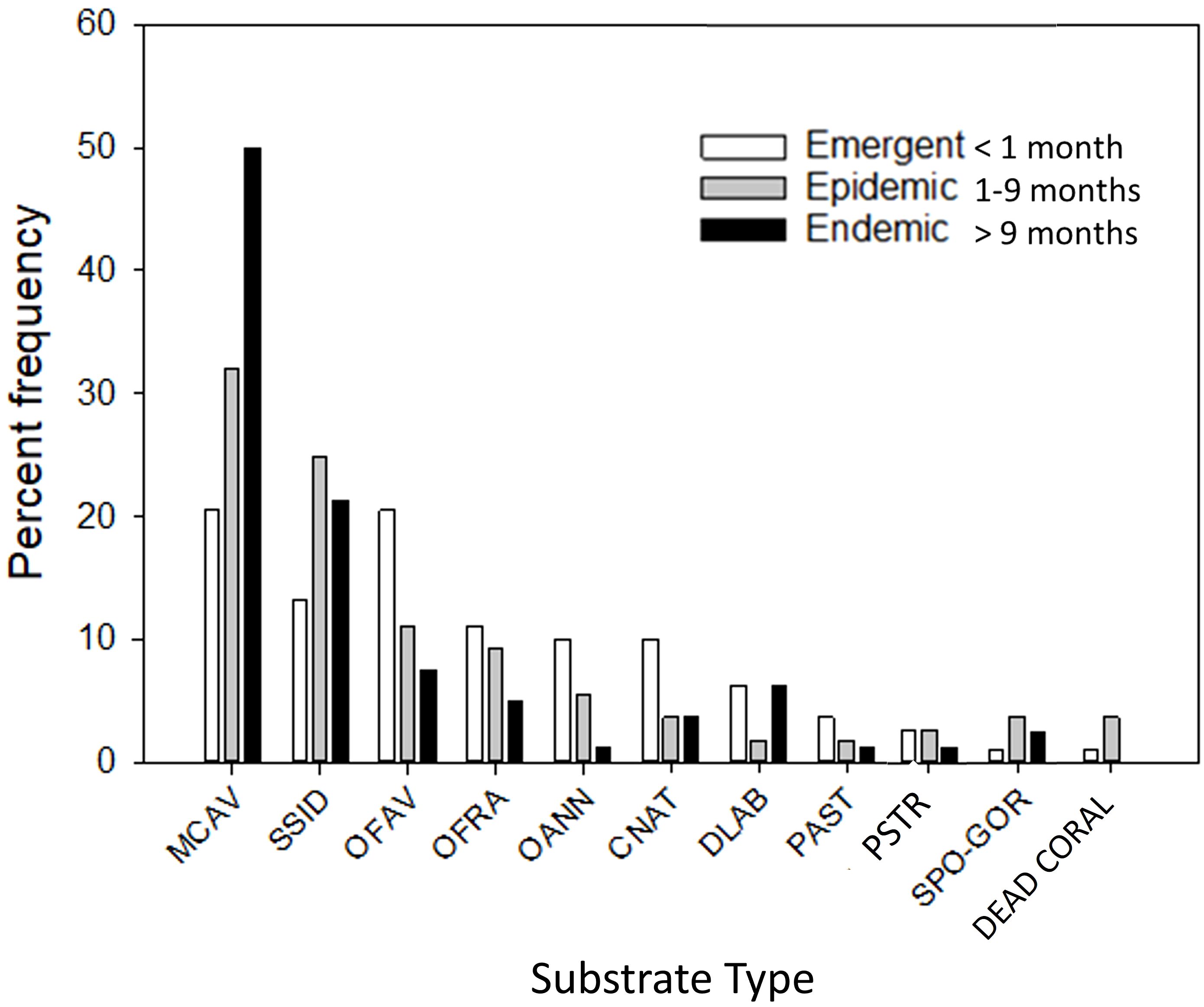
Figure 4 Percent frequency distribution of goby cleaning stations among various substrate types during timed surveys for emergent, epidemic, and endemic disease zones. Cleaning stations include Montastraea cavernosa (MCAV), Siderastrea siderea (SSID), Orbicella faveolota (OFAV), Orbicella franksi (OFRA), Orbicella annularis (OANN), Colpophyllia natans (CNAT), Diploria labyrinthiformis (DLAB), Porites astreoides (PAST), Pseudodiploria strigosa (PSTR), sponges and gorgonians (SPO-GOR), and dead coral.
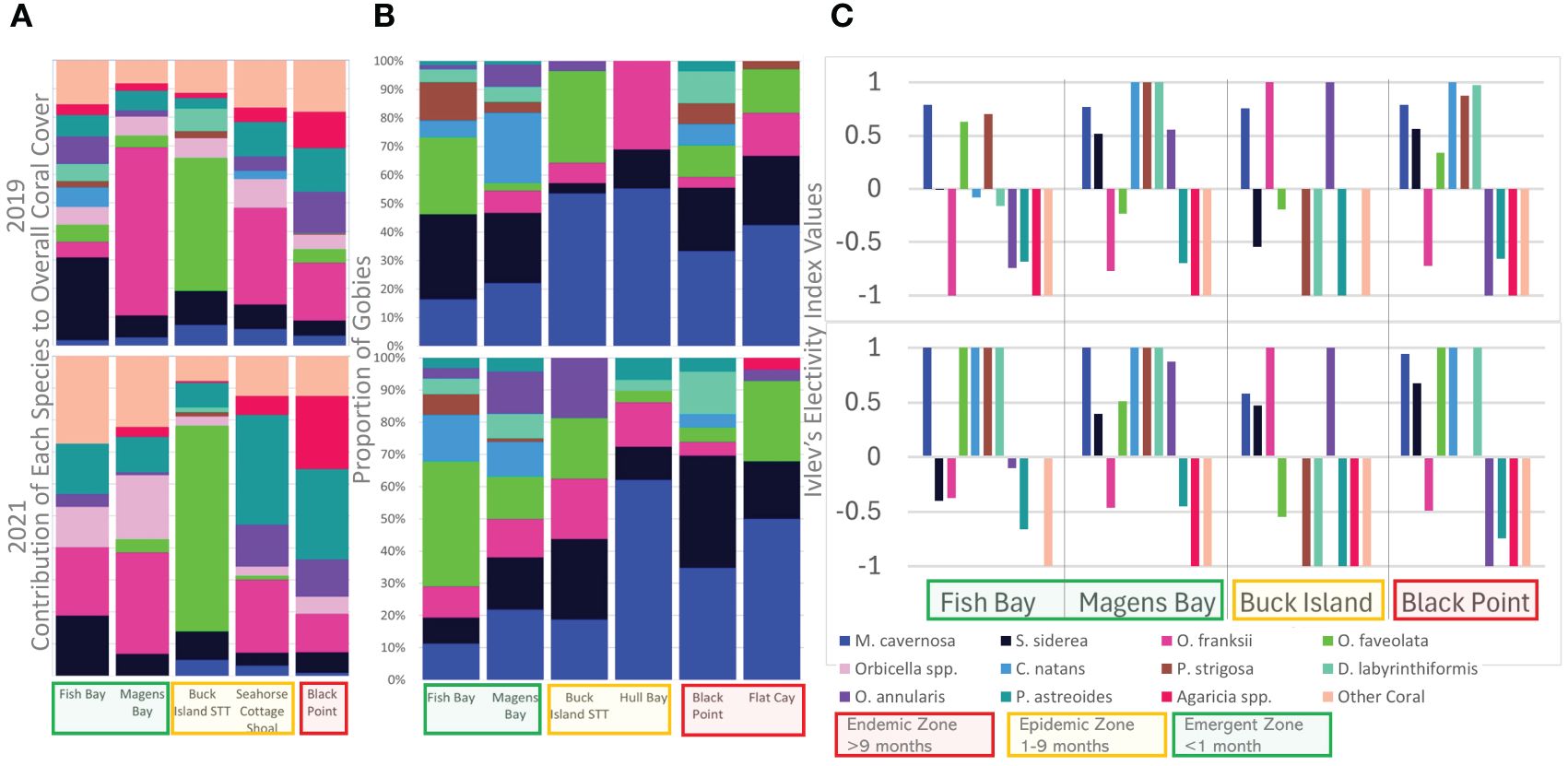
Figure 5 (A) Average contribution of each coral species to total coral cover at sites with TCRMP data available (B) Proportion of goby occupation of live coral species at long term monitoring sites (C) Ivlev’s Electivity Index values for long-term monitoring sites with TCRMP data available. Upper and lower figures represent data from 2019 and 2021, respectively and colors represent coral species. Other Species includes all coral species recorded by TCRMP (Ennis et al., 2020) that were not observed to be occupied by gobies during this study. Disease zonation at establishment of a site/time of rapid survey are indicated by colored boxes Electivity values range from a minimum of -1, meaning that coral species was not occupied when present at a site, to a maximum of 1, meaning that this coral is occupied by gobies if it is available. A value of 0 represents goby occupation is no different from random.
At the beginning of the study in 2019, O. franski contributed most to coral cover at most sites, followed by S. siderea,O. faveolata and P. astreoides. By the end of the study in 2021, O. franksi and P. astreoides contributed most to coral cover at most sites, followed by S. siderea, and O. faveolata. Note the reduction in contribution made to coral cover by M. cavernosa and C. natans at most sites and relative extirpation at all sites for C. natans and at sites where M. cavernosa made up a small proportion of the coral cover in 2019 (Seahorse Cottage Shoal and Magens Bay, Figure 5) (Ennis et al., 2020). Even though M. cavernosa contributed little to coral cover at most sites, it was one of the most chosen live coral cleaning stations by Caribbean cleaner gobies, followed by S. siderea. When taking available live coral habitat into account, S. siderea was less preferred than C. natans and M. cavernosa (Figure 5).
M. cavernosa was the only coral species habitat that gobies preferred across all sites and both years. Goby occupation of different coral species varied across sites and changed through time (Figure 5). The most prominent changes occurred in the emergent zone where several species that were occupied less than randomly in 2019 were found to be occupied more than random in 2021(Figure 5). Most of these changes represented an increase in preference with the exception of S. siderea at Fish Bay (emergent) and O. faveolata at Buck Island (epidemic), which decreased in preference (Figure 5).
M. cavernosa was also the only species to experience ≧ 75% tissue loss on individual colonies at all sites and near total tissue loss (≧ 90%) at all but one site (Figure 6, Supplementary Figure 3). During the study, near total tissue loss (≧ 90%) of tagged coral cleaning stations following SCTLD incidence was lowest at endemic sites [Black Point (5%) and Flat Cay (8%)], but otherwise did not follow any discernable pattern (Supplementary Figure 3). Of the nine M. cavernosa colonies to experience 90% tissue loss, only two were observed to be occupied by gobies following this loss and each of those had only one cleaner goby occupant after reaching 90% tissue loss. Tissue loss rates ≧ 75% on tagged coral heads following SCTLD incidence was greatest at emergent sites [Magens Bay (21%) and Fish Bay (23%)], and one epidemic site [Hull Bay (26%)] and lowest at endemic sites [Black Point (15%) and Flat Cay (8%)] and one epidemic site [Buck Island (16%)] (Figures 6, 7).
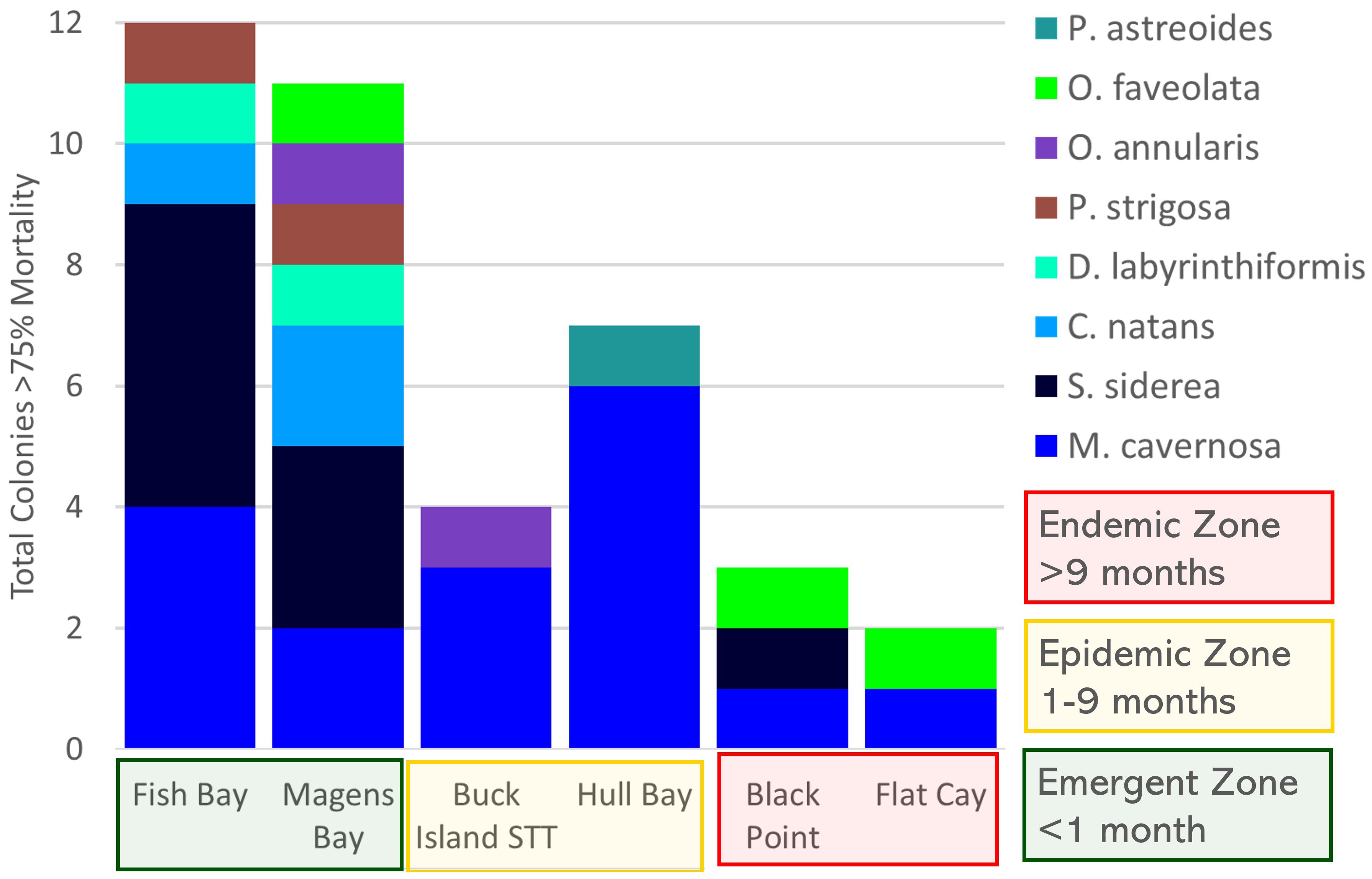
Figure 6 Tagged live coral cleaning stations that experienced ≧ 75% colony mortality by coral species per site. Colors represent coral species. Montastrea cavernosa was the only coral species to experience ≧ 75% mortality across sites regardless of disease zone and made up most of these colonies in the epidemic zone.
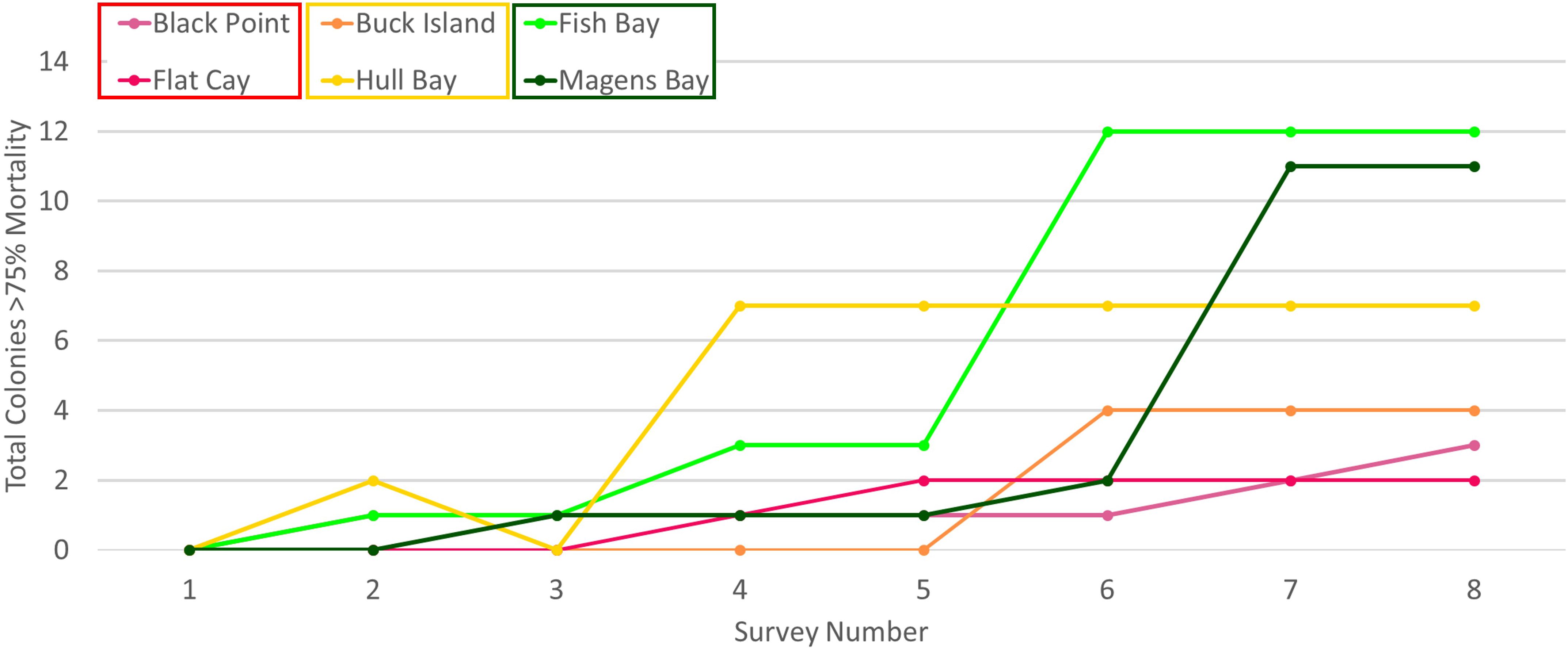
Figure 7 ≧ 75% mortality of tagged coral cleaning stations per site through time. Colors represent site and disease zone [endemic zone = Black Point (red) and Flat Cay (pink), epidemic zone = Buck Island (yellow) and Hull Bay (orange), emergent zone = Fish Bay (light green) and Magens Bay (dark green)]. The greatest coral mortality was seen at both sites in the emergent zone (greens), followed by epidemic (yellow and orange), and endemic (pink and red).
Over the course of the study SCTLD-related mortality resulted in a 3.6% decline in coral cover across study sites ranging from a loss of 10.8% at Seahorse Cottage Shoal to a gain of 2.6% at Fish Bay (Table 1). Higher mortality rates of more susceptible species (i.e., C. natans, D. labyrinthiformis) changed the relative abundance of species across most sites and reduced coral species richness most notably at Black Point and Magens Bay (6 species each, 29% and 40% decline respectively) (Figure 5).
3.3 Goby size structure
Elacatinus spp. size ranged from 6 mm to 45 mm across all sites with highest abundance in the 11 mm - 15 mm TL size class (Supplementary Figure 4). Combined data for rapid assessments and area surveys at monitoring sites found Elacatinus spp. sizes were not significantly different (F = 0.5, p = 0.62) among emergent (20.1 mm ± 0.85 SE), epidemic (18.4 mm ± 2.32 SE) and endemic (19.44 mm ± 1.17 SE) sites.
Goby size frequencies at study sites indicated recruitment of juvenile gobies occurred throughout the study (Figure 3; Supplementary Figure 2; Supplementary Table 1) but peaked between the months of September and December at endemic and epidemic sites and in June at emergent sites and decreased again throughout the spring. (Supplementary Figures 2, 4, 5; Supplementary Table 1).
4 Discussion
Dedicated cleaner fishes have been shown to have multiple, positive, impacts on their reef fish clients and thus coral reef-associated communities at large, possibly extending even to microbial communities (Pereira et al., 2022). The services provided appear to be unique and thus not completely replaceable (Nicholson et al., 2024). Thus, environmental impacts on their populations or behavior are likely to have knock-on community-level effects. To the extent that Caribbean Elacatinus cleaner gobies reside on the substrate, changes in substrate composition stand to have a significant impact on their abundance and functional role.
This study supported findings from studies at other sites indicating that Elacatinus cleaner gobies have a strong association with live stony corals. It further supported two key predictions of the hypothesis that stony coral tissue loss disease (SCTLD) impacts the distribution and abundance of Elacatinus spp. in the eastern Caribbean. The rapid population assessments initially indicated that goby abundance was 50% lower at sites where SCTLD had become well established (i.e., endemic) and thus where coral cover was lowest. Long-term monitoring of over 200 marked live coral cleaning stations at all sites further suggested that when a coral head cleaning station was affected by SCTLD and died, the cleaning goby relocated to a nearby coral or other substrate. A change in abundance on tagged corals but stability in site density suggested that gobies were remaining at the site but may be abandoning individual cleaning stations that were located on live or once-live corals. Further, as these gobies die off, they may not be replaced by new recruits which may be attracted to live coral habitat (Supplementary Figure 6). Combined, these results are consistent with other studies that show that mass mortality of corals has widespread impacts on other coral reef community members (Pratchett et al., 2008; Stuart-Smith et al., 2018; Sikkel et al., 2019; Russ et al., 2021). However, the impacts of such mass mortality likely depend on which coral species are most heavily impacted and thus what other community members are most closely associated with them.
In a recent study on the Great Barrier Reef, Triki et al. (2018) found that the consecutive disturbances of a tropical cyclone, mass bleaching event, and subsequent coral loss combined to contribute to an 80% decrease in populations of Labroides dimidiatus cleaner wrasses. However, the exact cause of coral loss was unclear. Moreover, the resulting decrease in availability of cleaner fish resulted in fundamental changes in the interaction between cleaners and clients. Other studies have found that populations of coral-dwelling gobies in the Indo-Pacific rapidly decline following coral losses (Munday, 2004; Pratchett et al., 2008; Herler et al., 2011). Unlike some previous studies on coral-associated goby populations, our long-term monitoring population surveys included searching both the coral colony where each goby was originally found and substrate in the surrounding area. Other study methodologies only included searching the colonies originally occupied by gobies and thus would not have found gobies that moved to a previously unoccupied colony or substrate type in the same area (Munday, 2004). These studies focused on highly specialized goby species that are found on only a few coral species which may be unable to utilize alternative substrates and suffer worse declines in abundances when their preferred habitat declines (Munday et al., 1997; Herler et al., 2011). In contrast, Elacatinus spp. have been observed to occupy many substrates. These goby species can shift to alternative coral hosts or even other substrate and move further when preferred habitats become less abundant (Caley and Munday, 2003; Feary, 2007; Herler et al., 2011).
The ability of reefs to recover the services provided by cleaning gobies depends upon successful recruitment of juvenile gobies. Epidemic sites had the lowest recruitment and emergent the highest. However, some recruitment was observed at endemic sites, suggesting recovery is possible following initial disease impacts. These recruitment pulses occurred at different times throughout the year for each site, ranging from June through November. This recruitment affected population estimates and average sizes. Most sites experienced a decrease in the juvenile size class from beginning to end of study. This may be partially due to fish growing and aging out of this class. If this was the only driver of juvenile loss in density, an equal increase in the next size class should be seen, but this was not the case. Juveniles appeared to exhibit more bold and less selective cleaning behavior where they readily approach and attempt to clean fish, divers, tools, or other things that came near their cleaning station (K. Budd, personal observation). This less cautious behavior may expose them to greater predation risk and higher energy costs compared with other life stages. Smaller individuals may also be forced out of high-quality habitat by larger fish (Whiteman and Côté, 2004). As coral cleaning stations die, larger gobies may also take up the remaining live coral substrate, forcing smaller individuals to seek out less desirable habitat options.
Comparisons of emergent sites with sites that were experiencing more disease stress suggests that long-term cleaner goby populations are likely to decline over time as coral cover decreases. However, rapid survey comparisons suggest that gobies may be relocating within sites as coral disease spreads. Long-term comparisons, especially those including coral restoration areas, could give valuable insight into the potential for natural goby repopulation and cleaning station recovery. When live coral heads were made available through goby removal, E. prochilos was observed to re-colonize the available coral habitat before available sponge habitat in Barbados (Whiteman and Côté, 2004). With more information on coral species preference by cleaner gobies, reef restoration projects could utilize cleaner goby population management to boost the health of other fish populations by including preferred cleaning station habitat in coral restoration plans. This represents an opportunity to leverage the disproportionally large effect that cleaner gobies have on the fish community to bolster the ecological impacts of reef restoration projects. Results from this study would suggest that adding M. cavernosa into in situ restoration projects may attract cleaner gobies to a site and lead to increased benefits for the local fish assemblages.
4.1 Potential causal mechanisms of SCTLD-associated changes in distribution and abundance of Elacatinus cleaner gobies
All study sites experienced loss of tagged cleaning stations (≧ 75% tissue loss on an individual colony). M. cavernosa cleaning stations experienced the greatest loss in the epidemic zone and was the only species to experience ≧ 75% tissue loss across all zones. Similar patterns can be seen in colonies that experienced ≧90% tissue loss. The initial die-off, captured in the monitoring of this zone, in this study and others, of a variety of coral species caused the loss of cleaning station species diversity in the early stages of the disease on a reef (Brandt et al., 2021; Costa et al., 2021). Interestingly, the disease zone with the least live coral cleaning station richness was the epidemic zone. Corals in this area were actively experiencing disease but had already experienced whole colony losses. This occupation to a few coral species may be due to a concentration of gobies onto the most preferred, readily available, least compromised live coral habitat. These losses potentially restrict cleaning station options to less optimal coral species that may not be as easily recognized by clients.
The coral most occupied relative to its availability, and thus preferred by Elacatinus spp. cleaner gobies overall was M. cavernosa. In the epidemic zone, M. cavernosa made up more than half of the tagged cleaning stations and made up the greatest proportion of live coral cleaning stations in the endemic zone compared to all other species and was consistently preferred when taking available coral cover into account regardless of disease zone. S. siderea and C. natans were the most common cleaning stations tagged in the emergent zone. Both M. cavernosa and S. siderea are categorized as moderately susceptible to SCTLD while C. natans is categorized as highly susceptible (Florida Coral Disease Response Research & Epidemiology Team, 2018; Muller et al., 2020; Costa et al., 2021).
In zones where the disease was established, C. natans was occupied less than half the frequency (n = 1 coral at Black Point) seen in the emergent zone (n = 10 corals) This tagged colony was observed with a maximum of three gobies and did not experience tissue loss throughout this study. However, it did experience partial bleaching in October 2020 during which none of its resident gobies were found. A single adult goby (28 mm) was located on this coral in April 2021 during the last survey of this site,166 days after the coral had regained color. This species was consistently preferred with the maximum electivity index value at all site-year combinations except Fish Bay in 2019 (emergent) where it was occupied slightly less than expected by chance and at Buck Island STT where it was occupied as expected by chance in both 2019 and 2021 (Figure 5C). The abundance of this species at Black Point, Fish Bay, and Seahorse Cottage Shoal is so low as to be undetectable by TCRMP surveys in 2019 and at all study sites with available data in 2021. In 2019 C. natans made up 3.16% of the coral cover at Buck Island, St. Thomas, 7.04% at Magens Bay and 0.21% of the coral cover at Flat Cay (Ennis et al., 2020). This represents a substantial loss of some of the most chosen cleaning station habitat by cleaner gobies.
M. cavernosa may represent a more reliable, preferred option for cleaner gobies in comparison to highly susceptible species. This is supported by its presence and high levels of occupation at all sites as well as both a high preference value and an increase in electivity index value across sites. As other corals die, S. siderea may represent the next best option for Elacatinus spp. gobies to maintain a cleaning station. It also increased in preference value at two of four sites. S. siderea is also moderately impacted but rarely experiences acute tissue loss and affected colonies do not experience the same rapid mortality as other species (Florida Coral Disease Response Research & Epidemiology Team, 2018; Muller et al., 2020; Brandt et al., 2021; Costa et al., 2021). The presence of these two species on a reef may increase the stability of an actively cleaning goby population by providing alternative, more resilient alternatives to large brain coral species such as C. natans.
4.2 Implications for effects of SCTLD- associated coral loss on cleaner goby populations
Isopods of the family Gnathiidae are the most common marine fish ectoparasites consumed by cleaner fishes and represent the primary food source for cleaner gobies in the eastern Caribbean region (Losey, 1974; Cheney and Côté, 2005; White et al., 2007; Côté and Soares, 2011; Artim et al., 2017; Nicholson et al., 2024). Although other microcarnivorous fish species will occasionally consume gnathiids, and even herbivores may ingest them accidentally, in our study area, cleaner gobies consume far more gnathiids per unit biomass or abundance than any other fish functional group (Artim et al., 2017; Nicholson et al., 2024) and thus appear to fill a unique functional role. The reasons cleaner gobies appear to associate strongly with certain types of live corals is unclear. One hypothesis is that they may be more conspicuous to potential clients compared to those on other substrates (Stummer et al., 2004; Cheney et al., 2009). When corals die or otherwise become unavailable, cleaner gobies can occupy other habitat and feed on other small invertebrates. However, changes in diet associated with such habitat-switches impacts their functional role. Indeed, several studies have shown that cleaner gobies associated with live coral spend more time cleaning and comsume more fish parasites than cleaner gobies occupying other substrate (Whiteman and Côté, 2002; White et al., 2007; Artim et al., 2017).
While cleaner gobies are well-known predators of gnathiids, whose cleaning behavior appears linked to the presence of live coral, recent studies have also shown that corals themselves can be major predators of gnathiids (Artim and Sikkel, 2013; Santos and Sikkel, 2019; Paula et al., 2021). A recent study conducted in the same region (and including some of the same sites) as the present study found that among 16 reef sites, live coral cover was the single best predictor of gnathiid density, with gnathiid densities reaching highest levels below 15% coral cover (Artim et al., 2020). Thus, diseases such as SCTLD could impact gnathiid isopod abundance through impacts of two of their major predators: corals directly and cleaner gobies indirectly.
4.3 Future directions
While cleaner gobies have received increased attention in recent years, they are still much less understood than other, more habitat-specialized, goby species or the Indo-Pacific cleaner wrasse which fill a similar functional role. One key difference from the latter is the strong association to the benthos.
This study detected Elacatinus spp. cleaning stations on four previously unreported coral species, highlighting the need for a comprehensive study of habitat association and live coral preference, and the multitude of factors that influence cleaner goby recruitment and retention throughout their range. Moreover, to the extent that cleaner goby diets in association with substrate have been examined at only a few sites and in relation to a small range of habitat types, more studies on this association are needed.
Among the potential ecological impacts of cleaner gobies, the impact on microbial communities and dynamics is just beginning to be explored (Xavier et al., 2019; Narvaez et al., 2022; Sazima, 2023). Because of their association with live corals and their contact with many fish and fish species per day, cleaner gobies stand to serve as distributors of microbes between fishes and corals and thus could contribute to the spread of or prevention of and recovery from disease for both corals and fishes. Thus, more studies on the role of cleaner gobies in microbial dynamics are needed.
This study offers a glimpse into the potential indirect impacts of SCTLD on the wider ecosystem through an important species that impacts many members of the nearshore fish community. The loss or reduction of ecological services provided by these cleaners may lead to consequences for the health of other reef fish that rely on them which includes many commercially important species and may have consequences for the local fishing economy. The impact of this disease on the wider ecosystem and local fisheries are not well understood. More comprehensive assessments of such diseases on the wider ecosystem are needed to begin understanding the full impacts on the ecosystem beyond coral colonies and reef structure. Our findings have important implications for the science and practice of coral reef restoration that has thus far focused on a narrow range of fast-growing coral species that do not appear to be suitable habitat for cleaner gobies.
Data availability statement
The raw data supporting the conclusions of this article will be made available by the authors, without undue reservation.
Ethics statement
Ethical approval was not required for the study involving animals in accordance with the local legislation and institutional requirements because animals were observed from a distance in their natural habitat without manipulation.
Author contributions
KB: Conceptualization, Data curation, Formal analysis, Funding acquisition, Investigation, Methodology, Project administration, Resources, Validation, Visualization, Writing – original draft, Writing – review & editing. PS: Conceptualization, Funding acquisition, Methodology, Resources, Writing – review & editing. MB: Conceptualization, Funding acquisition, Methodology, Resources, Writing – review & editing. SC: Investigation, Methodology, Resources, Writing – review & editing. TS: Data curation, Writing – review & editing. RN: Conceptualization, Data curation, Formal analysis, Funding acquisition, Investigation, Methodology, Project administration, Resources, Supervision, Validation, Visualization, Writing – review & editing.
Funding
The author(s) declare that financial support was received for the research, authorship, and/or publication of this article. This research was supported by grants from VI-EPSCoR (NSF # 1355437 and 1946412), Lana Vento Charitable Trust, and Virgin Islands Water Resources Research Institute (#205236).
Acknowledgments
We thank Joseph Townsend and Stephanie Hibberts for assistance in field data collection and data analysis; Jason Quetel, Elizabeth “Shaun” Kadison, Kelsey Vaughn, Madyson Miller, Amanda Byus, Kaya Carrion-Banuchi, and Alexys Long for assistance in field data collection; and Moriah Sevier for assistance with supplies acquisition and general troubleshooting. We also thank the following for help and support throughout the study: Janice and Robert Budd Sr., Darrell Budd, Sheila Skwiat, Bruce Budd, and Minky. This is contribution number 288 to the University of the Virgin Islands’ Center for Marine and Environmental Studies and was completed in partial fulfilment of a Master of Science in the Marine and Environmental Science Program.
Conflict of interest
The authors declare that the research was conducted in the absence of any commercial or financial relationships that could be construed as a potential conflict of interest.
The author(s) declared that they were an editorial board member of Frontiers, at the time of submission. This had no impact on the peer review process and the final decision.
Publisher’s note
All claims expressed in this article are solely those of the authors and do not necessarily represent those of their affiliated organizations, or those of the publisher, the editors and the reviewers. Any product that may be evaluated in this article, or claim that may be made by its manufacturer, is not guaranteed or endorsed by the publisher.
Supplementary material
The Supplementary Material for this article can be found online at: https://www.frontiersin.org/articles/10.3389/fmars.2024.1359168/full#supplementary-material
References
(2020) Coral demographics survey protocol for the Atlantic Region: U.S. Caribbean, Florida and Gulf of Mexico: 2018. Available online at: https://www.nodc.noaa.gov/archive/arc0151/0208322/1.1/data/0-data/NCRMP_Florida_2018_Benthics/NCRMP_Florida_2018_Benthics/Data_Documentation/Protocols/NCRMP_CoralDemographic_Protocol_2018.pdf.
Artim J. M., Sikkel P. C. (2013). Live coral repels a common reef fish ectoparasite. Coral Reefs 32, 487–494. doi: 10.1007/s00338-012-0995-8
Artim J. M., Hook A., Grippo R. S., Sikkel P. C. (2017). Predation on parasitic gnathiid isopods on coral reefs: a comparison of Caribbean cleaning gobies with non-cleaning microcarnivores. Coral Reefs 36, 1213–1223. doi: 10.1007/s00338-017-1613-6
Artim J. M., Nicholson M. D., Hendrick G. C., Brandt M., Smith T. B., Sikkel P. C. (2020). Abundance of a cryptic generalist parasite reflects degradation of an ecosystem. Ecosphere 11, 1–17. doi: 10.1002/ecs2.3268
Baddeley A., Rubak E., Turner R. (2015). Spatial point patterns: methodology and applications with R (Boca Raton, FL: CRC Press).
Barthelme S., Tschumperle D., Wijffels J., Assemlal H. E., Ochi S., Robotham A., et al. (2020) imager: image processing library based on “CImg”. Available online at: https://cran.r-project.org/web/packages/imager/index.html.
Brandt M. E., Ennis R. S., Meiling S. S., Townsend J., Cobleigh K., Glahn A., et al. (2021). The emergence and initial impact of stony coral tissue loss disease (SCTLD) in the United States Virgin Islands. Front. Mar. Sci. 8. doi: 10.3389/fmars.2021.715329
Bshary R. (2003). The cleaner wrasse, Labroides dimidiatus, is a key organism for reef fish diversity at Ras Mohammed National Park, Egypt. J. Anim. Ecol. 72, 169–176. doi: 10.1046/j.1365-2656.2003.00683.x
Bshary R., Oliveira R. F., Oliveira T. S., Canário A. V. (2007). Do cleaning organisms reduce the stress response of client reef fish? Front. Zool 4, 21. doi: 10.1186/1742-9994-4-21
Caley M. J., Munday P. L. (2003). Growth trades off with habitat specialization. Proc. R. Soc Lond. B 270, S175–S177. doi: 10.1098/rsbl.2003.0040
Carpenter K. E., Abrar M., Aeby G., Aronson R. B., Banks S., Bruckner A., et al. (2008). One-third of reef-building corals face elevated extinction risk from climate change and local impacts. Science 321, 560–563. doi: 10.1126/science.1159196
Caves E. M. (2021). The behavioural ecology of marine cleaning mutualisms. Biol. Rev. 96, 2584–2601. doi: 10.1111/brv.12770
Cerrano C., Cardini U., Bianchelli S., Corinaldesi C., Pusceddu A., Danovaro R. (2013). Red coral extinction risk enhanced by ocean acidification. Sci. Rep. 3, 1457. doi: 10.1038/srep01457
Cervino J., Goreau T. J., Nagelkerken I., Smith G. W., Hayes R. (2001). “Yellow band and dark spot syndromes in Caribbean corals: distribution, rate of spread, cytology, and effects on abundance and division rate of zooxanthellae,” in The ecology and etiology of newly emerging marine diseases. Ed. Porter J. W. (Springer Netherlands, Dordrecht), 53–63. doi: 10.1007/978-94-017-3284-0_4
Cheney K. L., Côté I. M. (2005). Mutualism or parasitism? The variable outcome of cleaning symbioses. Biol. Lett. 1, 162–165. doi: 10.1098/rsbl.2004.0288
Cheney K. L., Grutter A. S., Blomberg S. P., Marshall N. J. (2009). Blue and yellow signal cleaning behavior in coral reef fishes. Curr. Biol. 19, 1283–1287. doi: 10.1016/j.cub.2009.06.028
Costa S. V., Hibberts S. J., Olive D. A., Budd K. A., Long A. E., Meiling S. S., et al. (2021). Diversity and disease: the effects of coral diversity on prevalence and impacts of stony coral tissue loss disease in Saint Thomas, U.S. Virgin Islands. Front. Mar. Sci. 8. doi: 10.3389/fmars.2021.682688
Côté I. M. (2000). “Evolution and ecology of cleaning symbioses in the sea,” in Oceanography and marine biology (United Kingdom: Aberdeen University Press), 311–355.
Côté I. M., Soares M. C. (2011). “Gobies as cleaners,” in The biology of gobies. Eds. Patzner R., Tassell J.L.V., Kovacic M., Kapoor B. G. (Boca Raton, FL: CRC Press), 563–592. doi: 10.1201/b11397-53
D’Elia C. F., Webb K. L., Porter J. W. (1981). Nitrate-rich groundwater inputs to discovery bay, Jamaica: A significant source of N to local coral reefs? Bull. Mar. Sci. 31, 903–910.
Di Santo V., Lobel P. S. (2017). Body size and thermal tolerance in tropical gobies. J. Exp. Mar. Biol. Ecol. 487, 11–17. doi: 10.1016/j.jembe.2016.11.007
Dizon R. T., Yap H. T. (2006). Understanding coral reefs as complex systems: degradation and prospects for recovery. Sci. Mar. 70, 219–226. doi: 10.3989/scimar.2006.70n2219
Dunkley K., Cable J., Perkins S. E. (2018). The selective cleaning behaviour of juvenile blue-headed wrasse (Thalassoma bifasciatum) in the Caribbean. Behav. Processes 147, 5–12. doi: 10.1016/j.beproc.2017.12.005
Dunkley K., Ellison A. R., Mohammed R. S., Van Oosterhout C., Whittey K. E., Perkins S. E., et al. (2019). Long-term cleaning patterns of the sharknose goby (Elacatinus evelynae). Coral Reefs 38, 321–330. doi: 10.1007/s00338-019-01778-9
Ennis R. S., Kadison E., Heidmann S., Brandt M. E., Henderson L. M., Smith T. B. (2020). TCRMP-coral reefs report 2020 (United States Virgin Islands: University of the Virgin Islands). Available at: https://drive.google.com/file/d/1V7ZnHnKitHaLDIIbt4ru4tv8J0R7mWeM/view.
Feary D. A. (2007). The influence of resource specialization on the response of reef fish to coral disturbance. Mar. Biol. 153, 153–161. doi: 10.1007/s00227-007-0791-0
Floeter S. R., Vázquez D. P., Grutter A. S. (2007). The macroecology of marine cleaning mutualisms. J. Anim. Ecol. 76, 105–111. doi: 10.1111/j.1365-2656.2006.01178.x
Florida Coral Disease Response Research & Epidemiology Team (2018) Case definition: stony coral tissue loss disease (SCTLD). Available online at: https://floridadep.gov/sites/default/files/Copy%20of%20StonyCoralTissueLossDisease_CaseDefinition%20final%2010022018.pdf.
Gintert B. E., Precht W. F., Fura R., Rogers K., Rice M., Precht L. L., et al. (2019). Regional coral disease outbreak overwhelms impacts from a local dredge project. Environ. Monit Assess. 191, 630. doi: 10.1007/s10661-019-7767-7
Graham N. A. J., Wilson S. K., Jennings S., Polunin N. V. C., Robinson J., Bijoux J. P., et al. (2007). Lag effects in the impacts of mass coral bleaching on coral reef fish, fisheries, and ecosystems. Conserv. Biol. 21, 1291–1300. doi: 10.1111/j.1523-1739.2007.00754.x
Grutter A. S., De Brauwer M., Bshary R., Cheney K. L., Cribb T. H., Madin E. M. P., et al. (2018). Parasite infestation increases on coral reefs without cleaner fish. Coral Reefs 37, 15–24. doi: 10.1007/s00338-017-1628-z
Grutter A. S., Feeney W. E. (2016). Equivalent cleaning in a juvenile facultative and obligate cleaning wrasse: an insight into the evolution of cleaning in labrids? Coral Reefs 35, 991–997. doi: 10.1007/s00338-016-1460-x
Grutter A. S., Murphy J. M., Choat J. H. (2003). Cleaner fish drives local fish diversity on coral reefs. Curr. Biol. 13, 64–67. doi: 10.1016/S0960-9822(02)01393-3
Grutter A. S., Pickering J. L., McCallum H., McCormick M. I. (2008). Impact of micropredatory gnathiid isopods on young coral reef fishes. Coral Reefs 27, 655–661. doi: 10.1007/s00338-008-0377-4
Guimarães P. R., Sazima C., dos Reis S. F., Sazima I. (2007). The nested structure of marine cleaning symbiosis: is it like flowers and bees? Biol. Lett. 3, 51–54. doi: 10.1098/rsbl.2006.0562
Hata H., Kato M. (2006). A novel obligate cultivation mutualism between damselfish and Polysiphonia algae. Biol. Lett. 2, 593–596. doi: 10.1098/rsbl.2006.0528
Herler J., Munday P., Hernaman V. (2011). “Gobies on coral reefs,” in The biology of gobies. Eds. Patzner R., Tassell J.L.V., Kovacic M., Kapoor B. G. (Boca Raton, FL: CRC Press), 493–529. doi: 10.1201/b11397-27
Huebner L. K., Chadwick N. E. (2012). Patterns of cleaning behaviour on coral reef fish by the anemoneshrimp Ancylomenes pedersoni. J. Mar. Biol. Ass. 92, 1557–1562. doi: 10.1017/S0025315411001822
Huie J. M., Thacker C. E., Tornabene L. (2020). Co-evolution of cleaning and feeding morphology in western Atlantic and eastern Pacific gobies. Evolution 74, 419–433. doi: 10.1111/evo.13904
Johnson W. S., Ruben P. (1988). Cleaning behavior ofBodianus rufus, Thalassoma bifasciatum, Gobiosoma evelynae, andPericlimenes pedersoni along a depth gradient at Salt River Submarine Canyon, St. Croix. Environ. Biol. Fish 23, 225–232. doi: 10.1007/BF00004913
Jones G. P., McCormick M. I., Srinivasan M., Eagle J. V. (2004). Coral decline threatens fish biodiversity in marine reserves. Proc. Natl. Acad. Sci. U.S.A. 101, 8251–8253. doi: 10.1073/pnas.0401277101
Knowlton N., Rohwer F. (2003). Multispecies microbial mutualisms on coral reefs: the host as a habitat. Am. Nat. 162, S51–S62. doi: 10.1086/378684
Kohler K. E., Gill S. M. (2006). Coral Point Count with Excel extensions (CPCe): A Visual Basic program for the determination of coral and substrate coverage using random point count methodology. Comput. Geosciences 32, 1259–1269. doi: 10.1016/j.cageo.2005.11.009
Landsberg J. H., Kiryu Y., Peters E. C., Wilson P. W., Perry N., Waters Y., et al. (2020). Stony coral tissue loss disease in florida is associated with disruption of host–zooxanthellae physiology. Front. Mar. Sci. 7. doi: 10.3389/fmars.2020.576013
Losey G. S. (1974). Cleaning symbiosis in Puerto Rico with comparison to the tropical pacific. Copeia 1974, 960. doi: 10.2307/1442596
Manly B. F. J., McDonald L. L., Thomas D. L., McDonald T. L., Erickson W. P. (1993). Resource selection by animals, 2nd Edn.
Marsh J. A. (1977). “Terrestrial inputs of nitrogen and phosphorus on fringing reefs of Guam,” in Proceedings of the 3rd International Coral Reef Symposium, University of Miami, Miami FL, Vol. 1. 331–336.
McCammon A., Sikkel P. C., Nemeth D. (2010). Effects of three Caribbean cleaner shrimps on ectoparasitic monogeneans in a semi-natural environment. Coral Reefs 29, 419–426. doi: 10.1007/s00338-009-0583-8
Miller M. W., Karazsia J., Groves C. E., Griffin S., Moore T., Wilber P., et al. (2016). Detecting sedimentation impacts to coral reefs resulting from dredging the Port of Miami, Florida USA. PeerJ 4, e2711. doi: 10.7717/peerj.2711
Miller J., Muller E., Rogers C., Waara R., Atkinson A., Whelan K. R. T., et al. (2009). Coral disease following massive bleaching in 2005 causes 60% decline in coral cover on reefs in the US Virgin Islands. Coral Reefs 28, 925–937. doi: 10.1007/s00338-009-0531-7
Muller E. M., Sartor C., Alcaraz N. I., van Woesik R. (2020). Spatial epidemiology of the stony-coral-tissue-loss disease in Florida. Front. Mar. Sci. 7. doi: 10.3389/fmars.2020.00163
Munday P. L. (2004). Habitat loss, resource specialization, and extinction on coral reefs. Global Change Biol. 10, 1642–1647. doi: 10.1111/j.1365-2486.2004.00839.x
Munday P., Jones G., Caley M. (1997). Habitat specialisation and the distribution and abundance of coral-dwelling gobies. Mar. Ecol. Prog. Ser. 152, 227–239. doi: 10.3354/meps152227
Muscatine L., Porter J. W. (1977). Reef corals: mutualistic symbioses adapted to nutrient-poor environments. BioScience 27, 454–460. doi: 10.2307/1297526
Narvaez P., Morais R. A., Vaughan D. B., Grutter A. S., Hutson K. S. (2022). Cleaner fish are potential super-spreaders. J. Exp. Biol. 225, jeb244469. doi: 10.1242/jeb.244469
Neely K. L., Lewis C. L., Lunz K. S., Kabay L. (2021). Rapid population decline of the pillar coral dendrogyra cylindrus along the Florida reef tract. Front. Mar. Sci. 8. doi: 10.3389/fmars.2021.656515
Nicholson M. D., Pagán J. A., Hendrick G. C., Sikkel P. C. (2024). Functional diversity among coral reef fishes as consumers of ectoparasites. Coral Reefs. doi: 10.1007/s00338-023-02459-4
Paine R. T. (1969). The pisaster-tegula interaction: prey patches, predator food preference, and intertidal community structure. Ecology 50, 950–961. doi: 10.2307/1936888
Paula J. R., Sun D., Pissarra V., Narvaez P., Rosa R., Grutter A. S., et al. (2021). The role of corals on the abundance of a fish ectoparasite in the Great Barrier Reef. Coral Reefs 40, 535–542. doi: 10.1007/s00338-021-02051-8
Paytan A., Shellenbarger G. G., Street J. H., Gonneea M. E., Davis K., Young M. B., et al. (2006). Submarine groundwater discharge: An important source of new inorganic nitrogen to coral reef ecosystems. Limnol. Oceanogr. 51, 343–348. doi: 10.4319/lo.2006.51.1.0343
Pereira A., Soares M. C., Santos T., Pocas A., Perez-Losada M., Apprill A., et al. (2022). Reef location and client diversity influence the skin microbiome of the caribbean cleaner goby elacatinus evelynae. Microb. Ecol. 85, 372–382. doi: 10.1007/s00248-022-01984-z
Pratchett M., Munday P., Wilson S., Graham N., Cinner J., Bellwood D., et al. (2008). “Effects of climate-induced coral bleaching on coral-reef fishes,Äî Ecological and economic consequences,” in Oceanography and marine biology. Eds. Gibson R., Atkinson R., Gordon J. (Boca Raton: CRC Press), 251–296. doi: 10.1201/9781420065756.ch6
Precht W. F., Gintert B. E., Robbart M. L., Fura R., van Woesik R. (2016). Unprecedented disease-related coral mortality in Southeastern Florida. Sci. Rep. 6, 31374. doi: 10.1038/srep31374
Quimbayo J. P., Nunes L. T., Ozekoski R., Floeter S. R., Morais R. A., Fontoura L., et al. (2017). Cleaning interactions at the only atoll in the South Atlantic. Environ. Biol. Fish 100, 865–875. doi: 10.1007/s10641-017-0612-3
Russ G. R., Rizzari J. R., Abesamis R. A., Alcala A. C. (2021). Coral cover a stronger driver of reef fish trophic biomass than fishing. Ecol. Appl. 31, 1–22. doi: 10.1002/eap.2224
Santos T. R. N., Sikkel P. C. (2019). Habitat associations of fish-parasitic gnathiid isopods in a shallow reef system in the central Philippines. Mar. Biodiv 49, 83–96. doi: 10.1007/s12526-017-0756-6
Sazima I. (2023). Escorting and chafing behaviours as potential pathways to spread pathogens among cleaner fishes and their clients. Ichthyological Res. 70, 398–401. doi: 10.1007/s10228-022-00890-1
Sazima C., Guimarães P. R., Dos Reis S. F., Sazima I. (2010). What makes a species central in a cleaning mutualism network? Oikos 119, 1319–1325. doi: 10.1111/j.1600-0706.2009.18222.x
Sikkel P. C., Cheney K. L., Côté I. M. (2004). In situ evidence for ectoparasites as a proximate cause of cleaning interactions in reef fish. Anim. Behav. 68, 241–247. doi: 10.1016/j.anbehav.2003.10.023
Sikkel P., Herzlieb S., Kramer D. (2005). Compensatory cleaner-seeking behavior following spawning in female yellowtail damselfish. Mar. Ecol. Prog. Ser. 296, 1–11. doi: 10.3354/meps296001
Sikkel P. C., Richardson M. A., Sun D., Narvaez P., Feeney W. E., Grutter A. S. (2019). Changes in abundance of fish-parasitic gnathiid isopods associated with warm-water bleaching events on the northern Great Barrier Reef. Coral Reefs 38, 721–730. doi: 10.1007/s00338-019-01835-3
Soares M. C., Oliveira R. F., Ros A. F. H., Grutter A. S., Bshary R. (2011). Tactile stimulation lowers stress in fish. Nat. Commun. 2, 534. doi: 10.1038/ncomms1547
Stuart-Smith R. D., Brown C. J., Ceccarelli D. M., Edgar G. J. (2018). Ecosystem restructuring along the Great Barrier Reef following mass coral bleaching. Nature 560, 92–96. doi: 10.1038/s41586-018-0359-9
Stummer L. E., Weller J. A., Johnson M. L., Côté I. M. (2004). Size and stripes: how fish clients recognize cleaners. Anim. Behav. 68, 145–150. doi: 10.1016/j.anbehav.2003.10.018
Titus B. M., Daly M., Exton D. A. (2015). Temporal patterns of Pederson shrimp (Ancylomenes pedersoni Chace 1958) cleaning interactions on Caribbean coral reefs. Mar. Biol. 162, 1651–1664. doi: 10.1007/s00227-015-2703-z
Triki Z., Grutter A. S., Bshary R., Ros A. F. H. (2016). Effects of short-term exposure to ectoparasites on fish cortisol and hematocrit levels. Mar. Biol. 163, 187. doi: 10.1007/s00227-016-2959-y
Triki Z., Wismer S., Levorato E., Bshary R. (2018). A decrease in the abundance and strategic sophistication of cleaner fish after environmental perturbations. Glob Change Biol. 24, 481–489. doi: 10.1111/gcb.13943
Vaughan D. B., Grutter A. S., Costello M. J., Hutson K. S. (2017). Cleaner fishes and shrimp diversity and a re-evaluation of cleaning symbioses. Fish Fish 18, 698–716. doi: 10.1111/faf.12198
VI-CDAC. VI-CDAC data. Available at: vicoraldisease.org.
Waldie P. A., Blomberg S. P., Cheney K. L., Goldizen A. W., Grutter A. S. (2011). Long-term effects of the cleaner fish labroides dimidiatus on coral reef fish communities. PloS One 6, e21201. doi: 10.1371/journal.pone.0021201
Walton C. J., Hayes N. K., Gilliam D. S. (2018). Impacts of a regional, multi-year, multi-species coral disease outbreak in Southeast Florida. Front. Mar. Sci. 5. doi: 10.3389/fmars.2018.00323
Weil E., Cróquer A., Urreiztieta I. (2009). Yellow band disease compromises the reproductive output of the Caribbean reef-building coral Montastraea faveolata (Anthozoa, Scleractinia). Dis. Aquat. Org. 87, 45–55. doi: 10.3354/dao02103
White J. W., Grigsby C. J., Warner R. R. (2007). Cleaning behavior is riskier and less profitable than an alternative strategy for a facultative cleaner fish. Coral Reefs 26, 87–94. doi: 10.1007/s00338-006-0161-2
Whiteman E. A., Côté I. M. (2002). Cleaning activity of two Caribbean cleaning gobies: intra- and interspecific comparisons. J. Fish Biol. 60, 1443–1458. doi: 10.1111/j.1095-8649.2002.tb02439.x
Whiteman E. A., Côté I. M. (2004). Individual differences in microhabitat use in a caribbean cleaning goby: A buffer effect in a marine species? J. Anim. Ecol. 73, 831–840. doi: 10.1111/j.1095-8649.2003.216ap.x
Whittey K. E., Dunkley K., Young G. C., Cable J., Perkins S. E. (2021). Microhabitats of sharknose goby (Elacatinus evelynae) cleaning stations and their links with cleaning behaviour. Coral Reefs 40, 1069–1080. doi: 10.1007/s00338-021-02105-x
Keywords: stony coral tissue loss disease, ecosystem services, coral reef, disease, keystone species, parasite, cleaner, Elacatinus
Citation: Budd KA, Sikkel PC, Brandt ME, Costa SV, Smith TB and Nemeth RS (2024) Impacts of stony coral tissue loss disease on the persistence of Caribbean cleaner gobies. Front. Mar. Sci. 11:1359168. doi: 10.3389/fmars.2024.1359168
Received: 20 December 2023; Accepted: 21 March 2024;
Published: 03 April 2024.
Edited by:
Lorenzo Alvarez-Filip, National Autonomous University of Mexico, MexicoReviewed by:
Kimberly B. Ritchie, University of South Carolina Beaufort, United StatesMichael J. Childress, Clemson University, United States
Copyright © 2024 Budd, Sikkel, Brandt, Costa, Smith and Nemeth. This is an open-access article distributed under the terms of the Creative Commons Attribution License (CC BY). The use, distribution or reproduction in other forums is permitted, provided the original author(s) and the copyright owner(s) are credited and that the original publication in this journal is cited, in accordance with accepted academic practice. No use, distribution or reproduction is permitted which does not comply with these terms.
*Correspondence: Kayla A. Budd, Kayla.Budd@uvi.edu
†Present address: Sophia V. Costa, Florida International University, Institute of Environment, Miami, FL, United States