- 1Ministry of Education (MOE), Key Laboratory of Marine Genetics and Breeding, College of Marine Life Sciences, Ocean University of China, Qingdao, China
- 2Institute of Evolution and Marine Biodiversity, Ocean University of China, Qingdao, China
- 3South Australian Research and Development Institute, Aquatic Sciences Centre, Adelaide, SA, Australia
- 4Key Laboratory of Mariculture (Ministry of Education), Fisheries College, Ocean University of China, Qingdao, China
Sperm cryopreservation technique has been published in many farmed bivalve species. One of the key factors preventing its application in aquaculture and/or cryobanking is the knowledge gap on the performance of resultant progeny at late developmental stages and subsequent generations. An effective strategy to overcome these challenges is to use a model species with a short generation interval, such as the dwarf surfclam Mulinia lateralis (three months). This study evaluated the parameters key to the development of a non-programmable sperm cryopreservation technique in this species, with a D-stage larval rate similar to control being achieved when the sperm were cryopreserved under the conditions (cryoprotectant agent: 8% dimethyl sulfoxide; equilibration period: 10 min; rack height: 4 cm; thawing temperature: 60°C and sperm to egg ratio: 1100:1) optimized. This technique is the most common method applied in bivalve and the results from this study were all within the ranges published for other bivalve species, indicating this species would be an ideal bivalve model species for addressing cryopreservation evaluation issues that need a long extended time to collect data and/or challenging field experiments.
1 Introduction
Seafood supplied by fishery and aquaculture has been generally recognized as the key alternative protein source for the increasing global population (Zhang et al., 2022). Currently, the global fishery has reached the roof for sustainable production, whereas the aquaculture is in constant growth (Zhang et al., 2022). However, the acceleration of this growth will be needed to keep the pace of population growth in the world which is anticipated to be achieved through systemic approaches to address key issues identified in different species. So far, the application of many research findings has been compromised due to lack of information on late developmental stages or subsequent generations, especially in species with a long generation interval. In finfish, this issue has been alleviated by the introduction of model species. For example, zebrafish Danio rerio has been used as a model species to investigate issues with broader implications in aquaculture and fishery as this species has a short life cycle and could be easily maintained in laboratory conditions (Aleström and Winther-Larsen, 2016). In addition, zebrafish has also long been the model species for oocyte cryopreservation in fish and has recently been recommended as a model to study generation effects of sperm cryopreservation (Dong et al., 2008).
Molluscs represent the second largest farmed group worldwide, accounting for 20.2% of total world aquaculture production in 2020 (FAO, 2022). The main species currently farmed are oysters, clams, cockles, scallops and mussels, which all belong to bivalve (FAO, 2022). Although sperm cryopreservation techniques have been published in many of these species (Liu et al., 2015), their application in commercial productions and/or cryobanking has been restricted due to the lack of information on their potential long-term impacts (Fernández-Díez and Herráez, 2018; Bøe et al., 2021). These concerns have been further stressed recently as negative effects of sperm cryopreservation on progeny fitness have been reported in fish and mammalian species, such as brown trout Salmo trutta (Nusbaumer et al., 2019), Japanese eels Anguilla japonica (Müller et al., 2018), horses (Ortiz-Rodriguez et al., 2019) and mice (Jia et al., 2015), and a urgent study to address these knowledge gaps was recommended at the development of Atlantic salmon gene banking (Bøe et al., 2021). However, investigations on performance of economic important traits and generations are time-consuming and challenging in farmed marine bivalve species because of (1) their long generation interval, from one year in Pacific oysters Crassostrea gigas (Yang et al., 2021) to multiple years in scallop Patinopecten yessoensis (Silina, 2018); and/or (2) high costs and challenges to manage the experiments in the open marine environments (Heres et al., 2022). All these obstacles could be addressed if a model species could be applied.
The dwarf surfclam Mulinia lateralis is a dioecious marine bivalve species and has all the characters as a model species: a short generation interval (approximately three months), well established laboratory cultivation technique, and accessibility of genomic information (Yang and Guo, 2006; Li et al., 2022; Guo et al., 2023). In fact, this species has already been used as a model species in some studies, such as the optimization of tetraploid production methods (Yang and Guo, 2006) and the elucidation of shell formation mechanisms for bivalves (Guo et al., 2023). In order to use M. lateralis as a model species to investigate the effects of bivalve sperm cryopreservation on the performance of resultant progeny and/or subsequent generations, the first step is to develop a non-programmable sperm cryopreservation technique commonly used in this group. In this study, the parameters (type and concentration of cryoprotectant agent, equilibration period, rack height, thawing temperature and sperm to egg ratio) key to this technique have been optimized.
2 Materials and methods
2.1 Broodstock preparation and gamete collection
The mature M. lateralis were supplied by the Ministry of Education Key Laboratory of Marine Genetics and Breeding, Ocean University of China (Qingdao, China). They were about three months old and 0.25 ± 0.06 g in total body weight (n = 30). The genders of mature broodstock were identified according to their gonad colors observed directly through the shell, with males being white and females red or orange (Yang et al., 2021). The spawning was induced individually by the thermal shock method described by Yang et al. (2021). After the sperm debris were removed with a 24 µm sieve, a subsample was used to assess the sperm motility under a light microscope. Sperm with a motility rate of > 85% were pooled equally from at least 10 males in each experiment and kept on ice directly. The pooled sperm were then centrifuged (Centrifuge 5430R, Eppendorf, Germany) at 2000 rcf (4°C) for 5 min to increase the concentration by discarding the supernatant. The concentrated sperm were placed on ice again after their concentration was standardized to 1 × 108 cells mL-1. The eggs were gently poured into a 24 µm sieve with a 90 µm sieve on top to remove the debris. They were gently rinsed and then washed into a settlement beaker. After 20 min, a subsample of eggs on the bottom was assessed under a light microscope to ensure non-fertilized eggs (no polar body or dividing cells) being used in the subsequent experiments. The eggs collected from at least 5 individuals were mixed in each experiment and the egg density was standardized to 1 × 104 cells mL-1 in this study. Different batches of males and females were used in different experiments.
2.2 Chemicals
2.2.1 Cryoprotectant agent
Dimethyl sulfoxide (DMSO), ethylene glycol (EG) and propylene glycol (PG) used in this study were AR grade and purchased from Sigma-Aldrich Pty Ltd. The cryoprotective stock solution was prepared in 5 µm filtered seawater (FS) at a concentration twice that of the final concentration required. Therefore, when the same volume of stock solution and sperm were mixed, the required final chemical concentration was produced.
2.2.2 Fluorescent agent
The LIVE/DEAD sperm viability kit (L-7011) and LysoTracker green DND-26 (LYSO-G) kit (L-7526) were purchased from Invitrogen China to evaluate the integrities of plasma membrane (PMI) and acrosome (AI), respectively. Rhodamine 123 (Rh123) for mitochondrial membrane potential (MMP) evaluation and propidium iodide (PI) for AI and MMP evaluations were purchased from Sigma-Aldrich Pty Ltd. The working solutions of these fluorescent agents were modified according to Liu et al. (2014). For the PMI evaluation, both 4 µM SYBR14 and 200 µM PI were prepared with FS using the stock solutions provided in the kit. LysoTrack green DD-26 kit was used directly. FS was used to prepare the 10 µM Rh123 for MMP, and the 130 and 3000 µM PI for MMP and AI evaluations, respectively.
2.3 Equipment setup
The equipment used in this study was modified according to Liu et al. (2014), consisting of an esky (39.0 × 29.5 × 30.0 cm) and a foam rack with different heights (3 cm, 4 cm, 5 cm, 6 cm and 7 cm). At the start of the experiment, liquid nitrogen (LN) was added into the esky to a depth of about 5 cm and the rack was then floated on the LN surface. After 0.25 mL straws containing the sperm + cryoprotective solution mixture were placed at the required heights on the rack, the lid of esky was closed partially to maintain the constant flow of vaporized LN during cooling.
Two 10 L seawater baths were used to thaw and recover the cryopreserved sperm. The required temperatures (30 to 70°C) in the thawing bath were achieved by mixing ambient and boiled seawater. The 21°C temperature required in the recovery bath was achieved by mixing ambient and cold seawater.
2.4 Sperm quality evaluation methods
The quality of fresh and post-thaw sperm was assessed by measuring the motility, D-stage larval rate, activity of enzymes, lipid peroxidation level, and/or integrity or potential of sperm ultrastructure, components or organelles.
The motility was determined by diluting the sperm suspension to 1 × 107 cells mL-1 and expressed as the percentage of active sperm out of 100 under a microscope at 200 × magnification (Gwo et al., 2002; Dong et al., 2005). Sperm moving forward progressively were counted as active sperm while those vibrating or not moving at all were counted as dead sperm (Gwo et al., 2002; Dong et al., 2005). Each sample was assessed by two independent observers.
For the D-stage larval rate assessment, 1 mL concentrated eggs (10000 eggs) was taken into a 10 mL tube using a pipette and mixed gently with sperm to reach the predetermined sperm to egg ratio in the experiments. After mixing with sperm for 15 min, the eggs were washed gently on a 24 µm sieve by FS before being cultured in a 500 mL container at 21°C. The D-stage larval rate was determined 24 h post-fertilization and calculated as the percentage of eggs that developed into D-stage larvae. The controls were established with fresh sperm at a sperm to egg ratio of 5:1 (Yang et al., 2021).
Sperm PMI, MMP and AI were evaluated by SYBR14/PI, Rh123/PI and LYSO-G/PI methods, respectively. After the post-thaw sperm were diluted to 1 × 107 sperm/mL, the sperm were stained in 1 mL subsamples. For PMI and MMP evaluations, 100 µL SYBR14 or Rh123 were added for 20 min and then 100 µL PI for further 10 min. For AI evaluation, 5 µL LYSO-G was added for 30 min, and then 9 µL PI for further 10 min. All staining was carried out at the room temperature. The sperm quality was then assessed under a Leica DMi8 fluorescence microscope with viable sperm emitting green fluorescence, while dead sperm emitting red fluorescence. Their integrity or potential was expressed as the percentage of viable sperm in 200 sperm assessed randomly.
The activity of superoxide dismutase (SOD), catalase (CAT) or glutathione (GSH) was measured using a commercial kit (Thermo Fisher Scientific, USA) according to the manufacturer’s instructions. The sperm samples (2.5 × 107 cells/mL) were centrifuged at 250 × g for 10 min at 4°C to obtain sperm pellets first. (1) For the SOD activity assessment, the pellets were resuspended in 750 µL ice-cold PBS and centrifuged at the same condition before being sonicated for 1 min. The supernatant from each sample was transferred into another microcentrifuge tube after being centrifuged at 1500 × g for 10 min at 4°C. The level of SOD activity was then determined by the kit (EIASODC). The SOD concentration (U/mL) at 450 nm were measured using a microplate reader (Infinite 200 PRO, Thermo Fisher Scientific, USA). The final values were calculated from standard curves. (2) For catalase (CAT) activity analysis, the pellets were resuspended with 1 mL ice-cold 1 × assay buffer before being sonicated for 1 min. After being centrifuged at 10000 × g for 15 min at 4°C, the supernatant from each sample was collected. The level of CAT activity was then determined by the kit (EIACATC). The concentration (U/mL) of CAT was measured at 560 nm using a microplate reader. (3) For the glutathione (GSH) activity assessment, the pellets were homogenized with 5% aqueous 5-sulfosalicylic acid in 250 µL ice-cold PBS and incubated for 10 min at 4 °C. The homogenized samples were then centrifuged at 14000 rpm for 10 min at 4 °C to collect the supernatant for analysis. The GSH assay was performed using the kit (EIAGSHC). The GSH activity (μM) was measured at 405 nm using a microplate reader.
The lipid peroxidation (LPO) level in sperm (2.5×107 cells/mL) was detected using the LPO assay kit (ab118970, Abcam, UK) following the manufacturer’s protocol. Briefly, the samples were homogenized using the 300 µL LPO lysis buffer provided and centrifuged at 13000 × g for 10 min at 4 °C to remove the insoluble material. Then 200 μL supernatant was incubated with 600 μL thiobarbituric acid for 60 min at 95 °C. After being cooled to room temperature in an ice bath, 300μL n-butanol and 100μL 5 M sodium chloride were added to the sample and mixed with a vortex mixer. Subsequently, the samples were centrifuged at 16,000 × g for 3 min at room temperature. The supernatant was collected and heated to 55 °C to evaporate the n-butanol. The residue was resuspended in 200 µL ddH2O and then the sample was used to measure the absorbance of LPO at 532 nm with a microplate reader.
The sperm ultrastructure was observed under a scanning or transmission electron microscope. (1) Scanning electron microscopic observation. The sperm samples were fixed with 0.1 M PBS, and then post-fixed in 1% osmic acid for 2 h at room temperature. The samples were subsequently dehydrated with an increasing ethanol series of 30%, 50%, 70%, 80%, 90%, 95% and 100% with a 15 min duration at each concentration, followed by another 100% ethanol for 15 min and finally immersed in 100% isoamyl acetate for 15 min. The samples were dried using a K850 Critical Point Dryer (Quorum, UK) and coated with gold for 30 s in a metallizer. The samples were examined under the scanning electron microscope (Tescan, Czech Republic) at various magnifications. (2) Transmission electron microscopic observation. The sperm samples were fixed in 2.5% glutaraldehyde for 15 min, and then post-fixed in 0.1% osmic acid prepared in 0.1 M PBS for 2 h at room temperature. The samples were subsequently dehydrated with an increasing ethanol series of 30%, 50%, 70%, 80%, 95% and 100% for 20 min at each concentration, followed by another 100% ethanol for 20 min and finally immersed in 100% acetone twice at a 15 min interval. After being embedded into the resin, the ultrathin sections (80 nm) were obtained using an ultracut ultramicrotome (Leica UC7). The sections were collected on 150 mesh copper grids, stained with lead citrate and uranyl acetate before being examined and photographed under the HT7700 (Hitachi) transmission electron microscope.
2.5 Experiments
2.5.1 Effects of CPA types and concentrations on D-stage larval rate
In this experiment, DMSO, EG and PG were evaluated at six final concentrations (4%, 6%, 8%, 10%, 12% or 14%). Ice-cold fresh sperm were mixed with ice-cold DMSO, PG or EG stock solution at a 1:1 ratio, resulting in a final concentration required. After 10 min equilibration on ice, fertilization was conducted at a sperm to egg ratio of 300:1 and the D-stage larval rate was assessed 24 h post-fertilization. Each treatment was replicated three times using sperm from different pools.
2.5.2 Effects of equilibration periods on D-stage larval rate
DMSO and PG at a final concentration of 6%, 8% or 10% were selected for this experiment according to the results from the previous experiment. In this experiment, three equilibration periods (10, 20 or 30 min) were assessed on D-stage larval rate. Other procedures were the same as in section 2.5.1.
2.5.3 Effects of rack heights on post-thaw D-stage larval rate
Ten minutes equilibration was selected in this and subsequent experiments. In this experiment, rack height of 3 cm, 4 cm, 5 cm, 6 cm or 7 cm above the LN surface was evaluated. After equilibration on ice, the sperm and cryoprotective solution mixture was transferred into 0.25 mL straws and placed on a rack at required height. The straws were then exposed to LN vapour for 10 min before being stored in LN for at least 12 h. The sperm were thawed in a 60°C seawater bath for 5 s and then recovered in a 21°C seawater bath. Subsamples of post-thaw sperm were used for fertilization at a sperm to egg ratio of 900:1. Other procedures were the same as in section 2.5.2.
2.5.4 Effects of different thawing temperatures on post-thaw D-stage larval rate
In this experiment, sperm cryopreserved at 4 cm rack height with 8% or 10% DMSO were used to evaluate the effects of different thawing temperatures at 30 (8 s), 40 (7 s), 50 (6 s), 60 and 70 (4 s) °C on post-thaw D-stage larval rate. Other procedures were the same as in section 2.5.3.
2.5.5 Effects of different sperm to egg ratios on post-thaw D-stage larval rate
The highest post-thaw D-stage larval rate was achieved when the sperm were cryopreserved in 8% DMSO and thawed at 60°C. Thus, these parameters were used in this and subsequent experiments. In this experiment, the effects of different sperm to egg ratios (300:1, 500:1, 700:1, 900:1 or 1100:1) on post-thaw D-stage larval rate were compared. Other procedures were the same as in section 2.5.4.
2.5.6 Effects of cryopreservation on sperm quality
When the sperm were cryopreserved with the parameters (cryoprotective solution: 8% DMSO, equilibration period: 10 min, rack height: 4 cm and thawing temperature: 60°C) optimized in previous sections, a D-stage larval rate similar to the control group was achieved at a sperm to egg ratio of 1100:1. In this experiment, the sperm quality, including sperm motility, ultrastructure, activity of enzymes, lipid peroxidation level and integrity/potential of sperm components or organelles, was compared between the fresh and post-thaw sperm in order to improve the knowledge of cryodamage on sperm.
2.6 Statistical analysis
Results in this study were presented as mean ± standard deviation (SD). The original data in percentage were arcsine transformed before analysis with SPSS 22. Two-way analysis of variance (ANOVA) was applied to analyze the data on the effects of CPA type and concentration, equilibration period, rack height or thawing temperature on the D-stage larval rate. One-way ANOVA was used to analyze the data on the effects of different sperm to egg ratios on post-thaw D-stage larval rate. The Least Significant Difference (LSD) comparison was used when a significant difference was observed. A t-test was applied to compare the activity of enzymes, the level of lipid peroxidation, percentage of motility, PMI, MMP or AI between the fresh and post-thaw sperm. Differences were considered statistically significant at P < 0.05.
3 Results
3.1 Effects of CPA types and concentrations on D-stage larval rate
Results from this experiment showed that the M. lateralis sperm were more sensitive to EG than other two CPAs evaluated as < 60% D-stage larval rate was produced at the concentration of 6% (Figure 1). For DMSO and PG, there was no significant difference in D-stage larval rate among concentrations lower than 10% (P > 0.05; Figure 1). At the higher final concentration of 12% or 14%, both PG and DMSO produced a D-stage larval rate of < 70%, although the rate in PG was significantly higher than that in DMSO (P < 0.05; Figure 1).
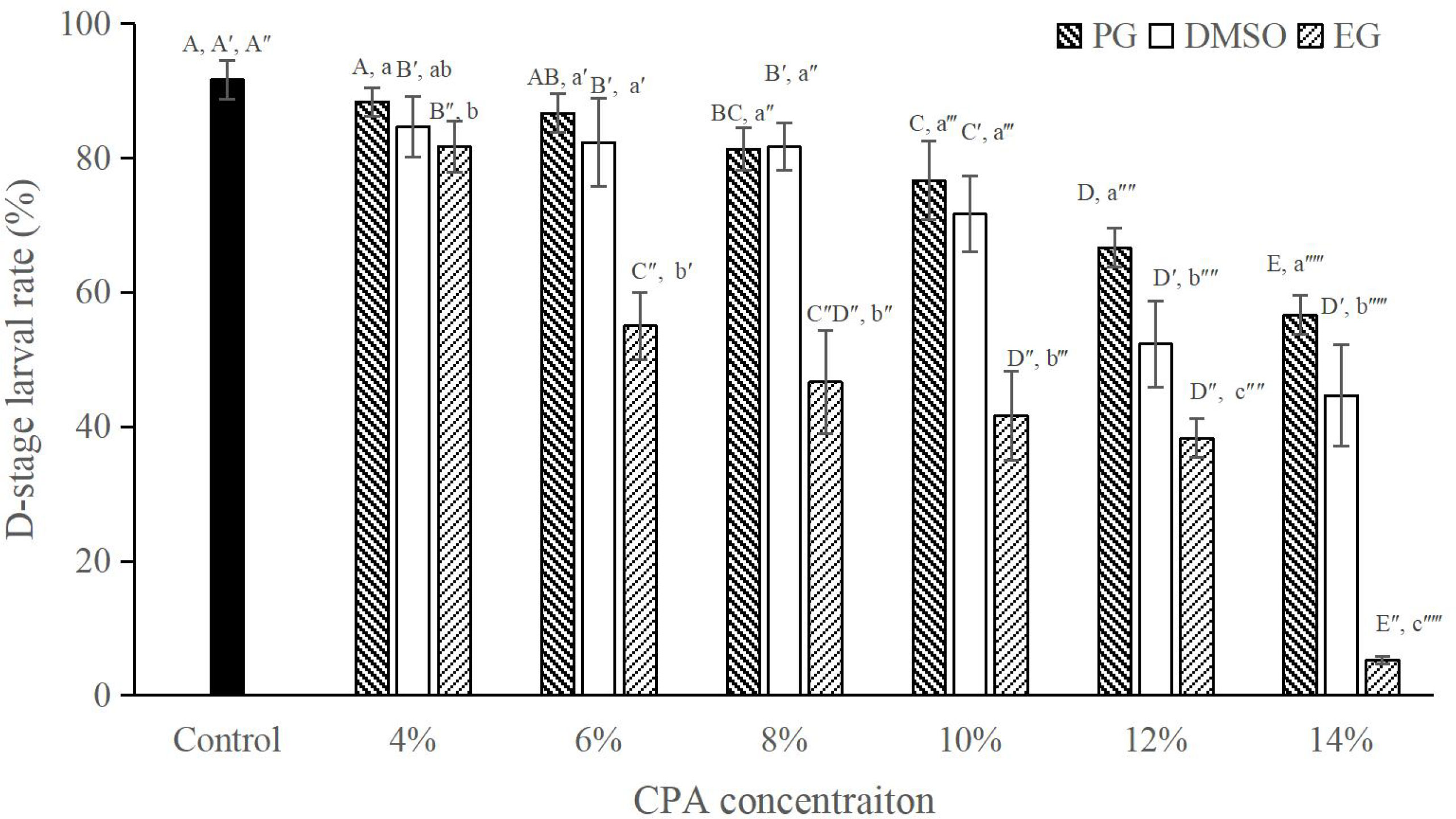
Figure 1 D-stage larval rates (%) after exposure to PG, DMSO or EG at different final concentrations for 10 min on ice, n = 3. Bars with different capital letters within each CPA type differ significantly between concentrations (P < 0.05). Bars with different lowercase letters within each concentration differ significantly between CPA types (P < 0.05).
3.2 Effects of equilibration periods on D-stage larval rate
The D-stage larval rate decreased with the increase in the equilibration period of all the CPAs evaluated. At 10 min equilibration period, CPA at 6% or 8% final concentration produced no significant difference in D-stage larval rate in comparison with control group (P > 0.05; Figure 2), whereas at 10% both CPAs produced a significantly lower D-stage larval rate than the control (P < 0.05; Figure 2). When the equilibration period extended to 20 min, only CPAs at the 6% final concentration produced a D-stage larval rate similar to the control (P > 0.05; Figure 2). At 30 equilibration period, although significant lower D-stage larval rate was produced in the treatment than that in the control (P < 0.05; Figure 2), there was no significant difference on D-stage larval rate between DMSO and PG at the same concentration (P > 0.05; Figure 2).
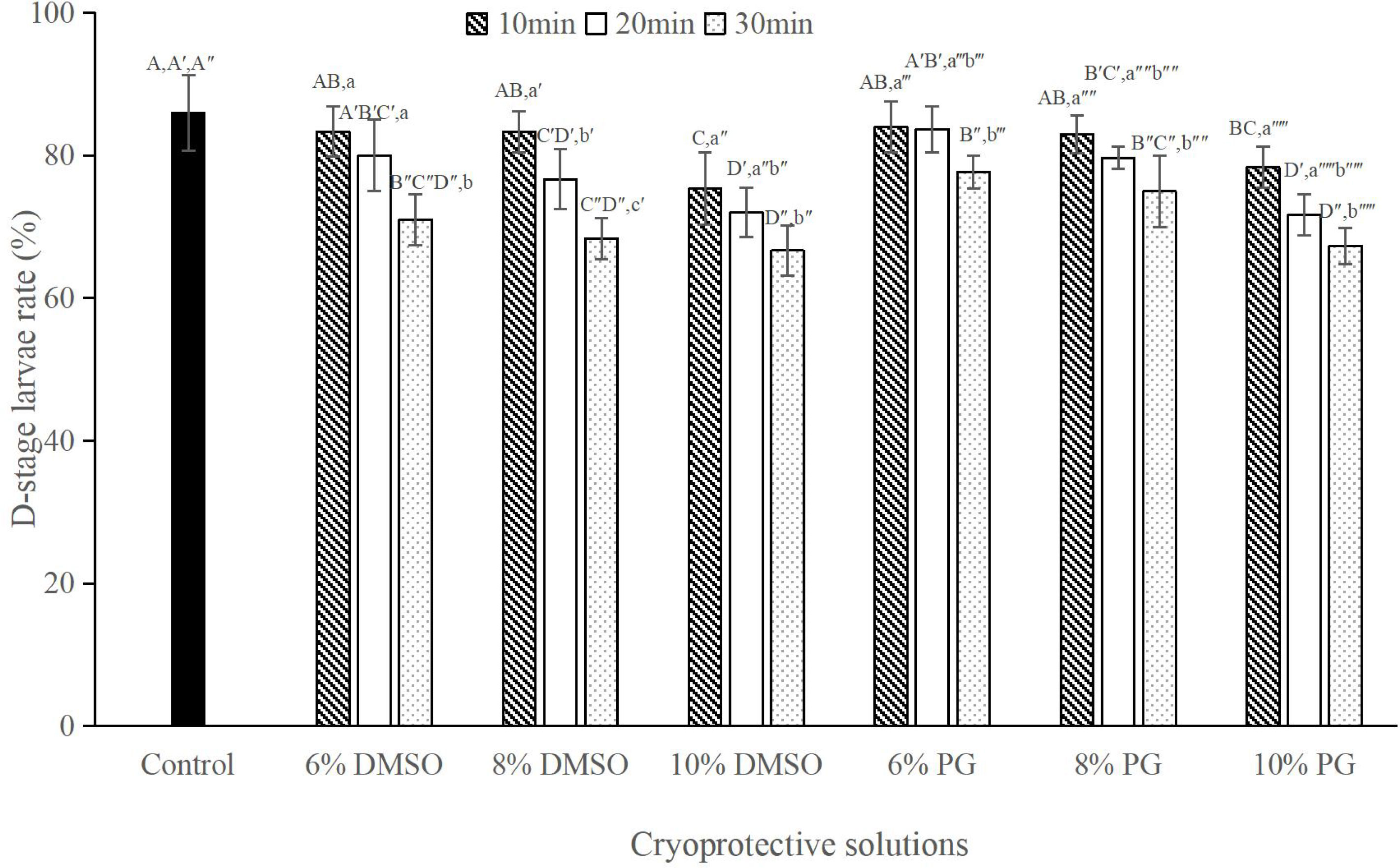
Figure 2 D-stage larval rates (%) after exposure to selected cryoprotective solutions at different equilibration periods, n = 3; Bars with different capital letters within each equilibration period differ significantly between cryoprotective solutions (P < 0.05). Bars with different lowercase letters within each cryoprotective solution differ significantly between equilibration periods (P < 0.05).
3.3 Effects of rack heights on post-thaw D-stage larval rate
Results showed that at the same concentration, DMSO produced significantly higher D-stage larval rate than that in PG (P < 0.05; Figure 3). The highest post-thaw D-stage larval rate of 80.00 ± 5.00% was achieved when the sperm were cryopreserved with 8% DMSO at 4 cm rack height (Figure 3). Although there was no significant difference in post-thaw D-stage larval rate between 8% and 10% DMSO at 4 cm rack height (P > 0.05; Figure 3), they were significantly lower than that in the control group (86.67 ± 2.89%, P < 0.05; Figure 3).
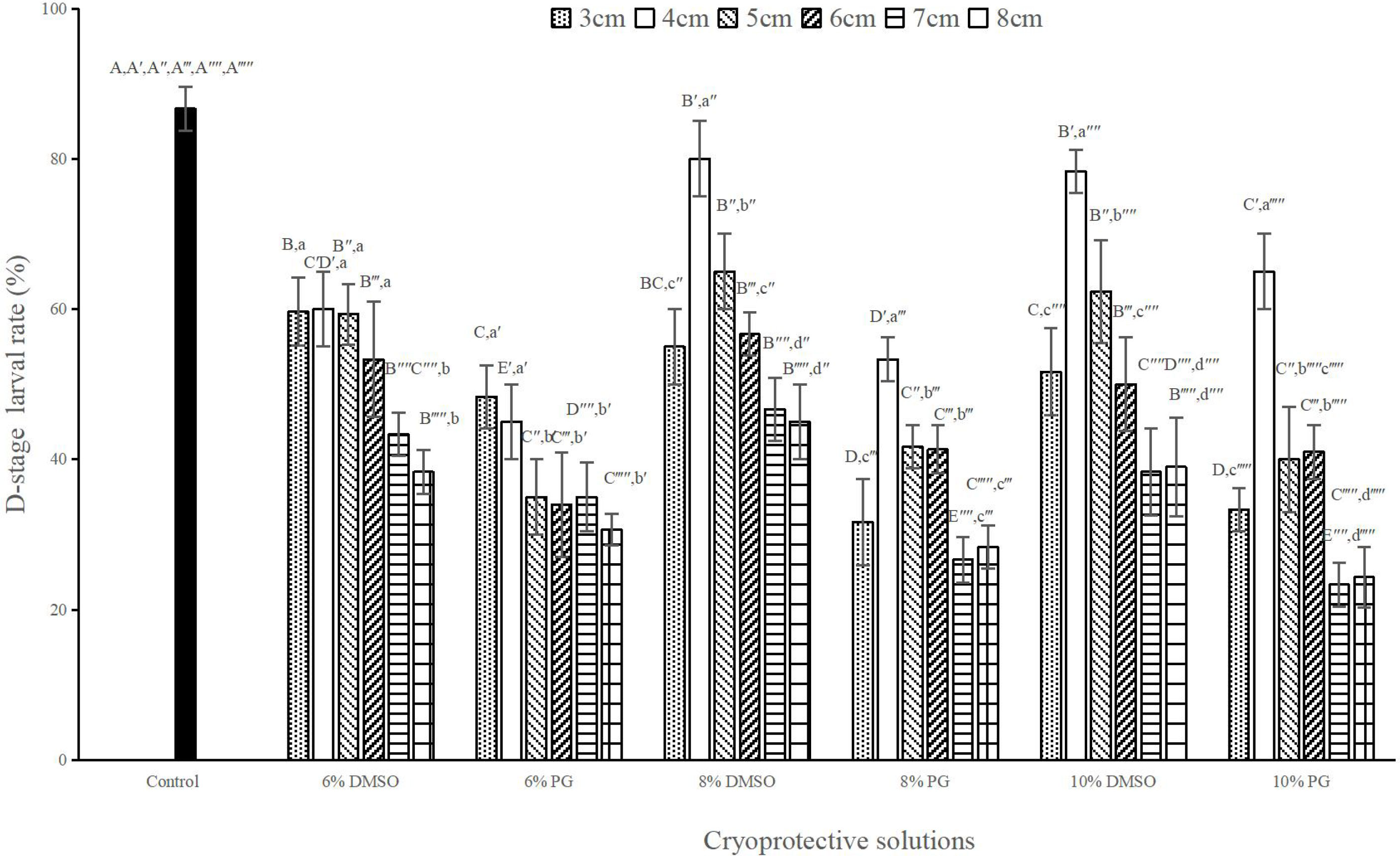
Figure 3 D-stage larval rates (%) after sperm cryopreservation at different rack heights with various cryoprotective solutions, n = 3; Bars with different capital letters within each rack height differ significantly between cryoprotective solutions (P < 0.05). Bars with different lowercase letters within each cryoprotective solution differ significantly between rack heights (P < 0.05).
3.4 Effects of different thawing temperatures on post-thaw D-stage larval rate
No significant difference in D-stage larval rate was observed between 8% and 10% DMSO at the same thawing temperature (P > 0.05; Figure 4). Although the highest D-stage larval rate of 80.00 ± 5.00% was achieved when the sperm were thawed at 60°C, it was significantly lower than that in the control group (88.33 ± 4.04%; P < 0.05; Figure 4).
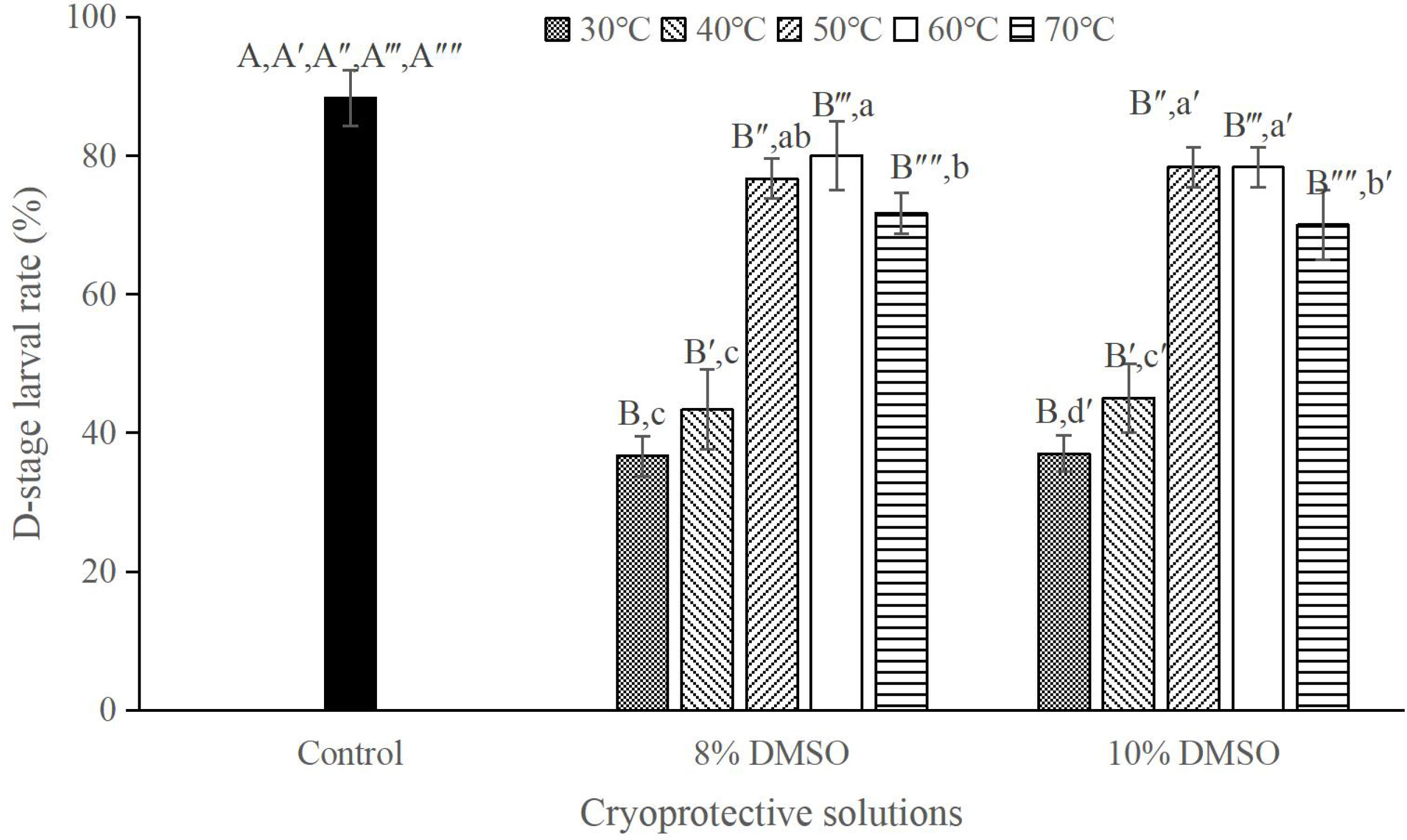
Figure 4 D-stage larval rates (%) after cryopreservation in 8% or 10% DMSO with different thawing temperatures, n = 3; Bars with different capital letters within each thawing temperature differ significantly between cryoprotective solutions (P < 0.05). Bars with different lowercase letters within each cryoprotective solution differ significantly between thawing temperatures (P < 0.05).
3.5 Effects of different sperm to egg ratios on post-thaw D-stage larval rate
The D-stage larval rate increased with the increase in sperm to egg ratios with the highest rate of 86.00 ± 5.29% being achieved at a sperm to egg ratio of 1100:1 (Figure 5). This rate did not significantly differ from those produced at a ratio of 900:1 and the control group (P > 0.05; Figure 5). However, the rate at the 900:1 ratio was significantly lower than that in the control (P < 0.05; Figure 5).
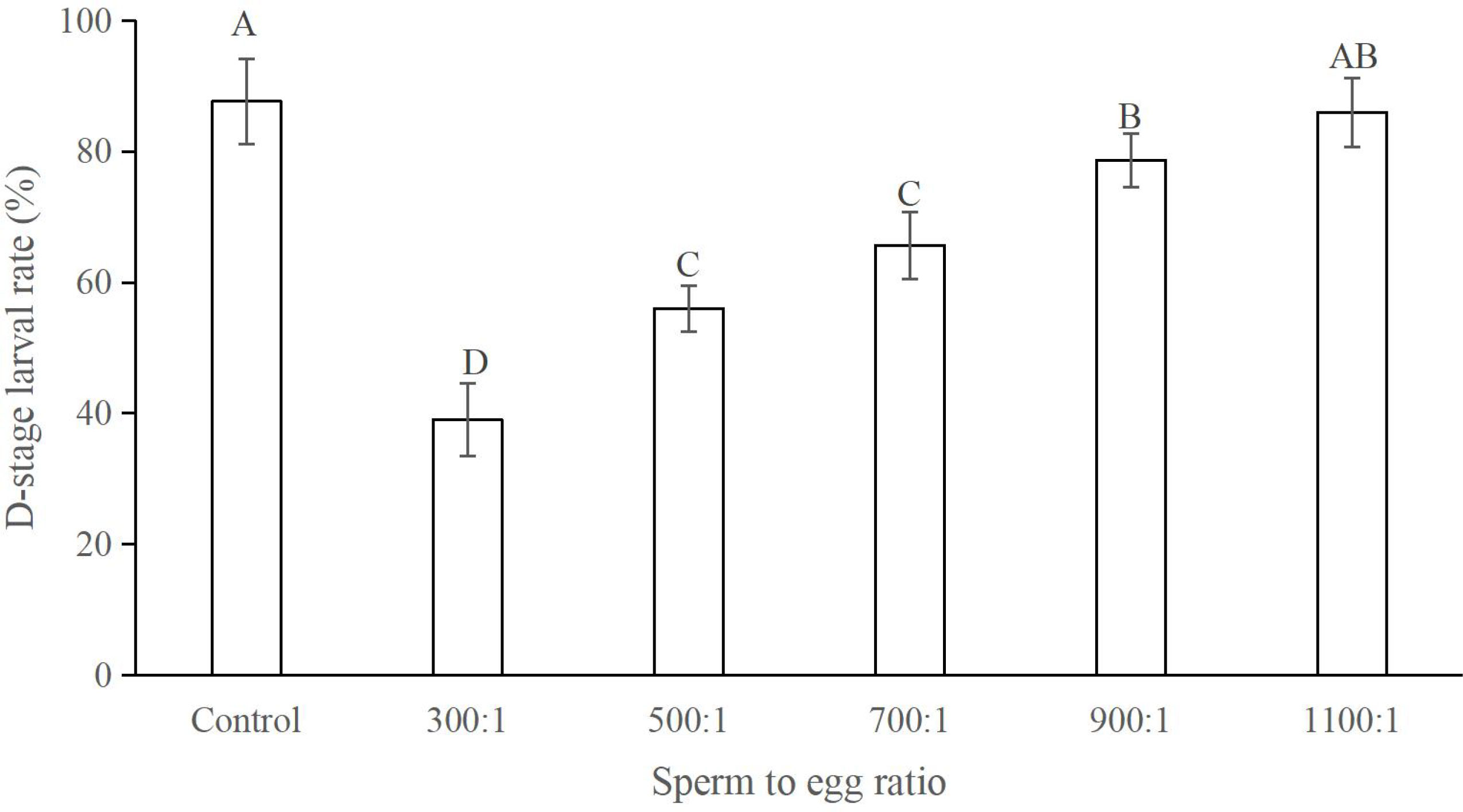
Figure 5 D-stage larval rates (%) at different sperm to egg ratios, n = 3. Different letters indicate significant difference.
3.6 Effects of cryopreservation on sperm quality
The images produced by the scanning electron microscope showed that the fresh sperm in M. lateralis had three distinct parts: head, midpiece and flagellum. The head was round with the acrosome being located at the anterior part, while the midpiece and a long flagellum being connected at the posterior end (Figures 6A, B). Scanning electron images of post-thaw sperm showed morphological changes in some sperm, including the flagellum damages, absence of acrosome, swollen head and decoupled midpiece (Figures 6C–F).
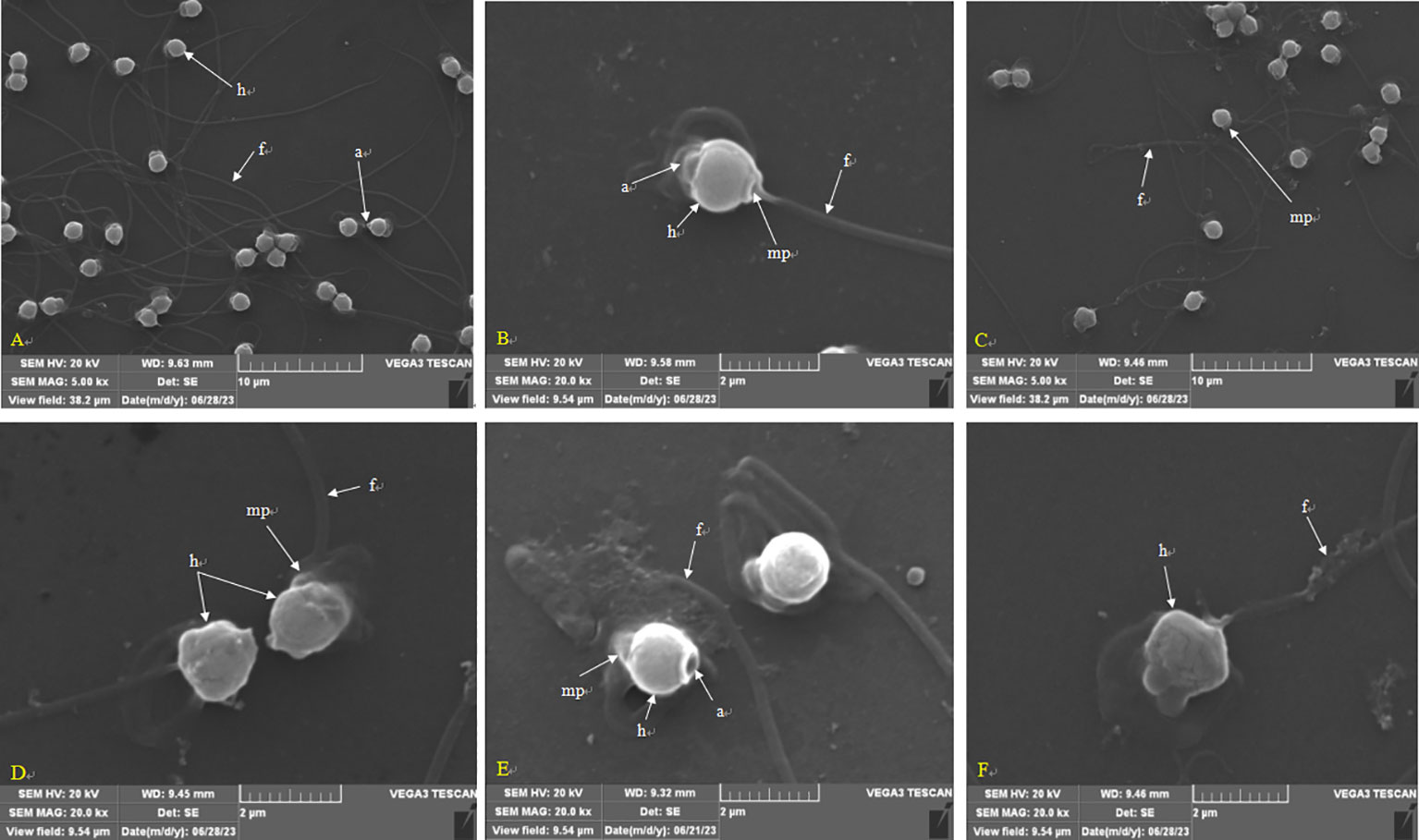
Figure 6 Scanning electron images of sperm in M. lateralis. (A) Fresh sperm showing different structures; (B) Magnified view of a single fresh sperm; (C) Post-thaw sperm at the low magnification; (D–F) Magnified view of post-thaw sperm showing damaged morphology and structure. a, acrosome; f, flagellum; h, head; mp, midpiece.
The images produced by the transmission electron microscope showed that the fresh sperm possessed a spherical head and a short midpiece. The dense nucleus covering by the nuclear envelope occupied most of the head and exhibited an anterior depression at the sub-acrosomal zone and a posterior depression at the basal pole. The proximal centriole was inserted centrally into a ring comprising four mitochondria which composed of normal cristae structures. The distal centriole located in the midpiece, near the base of flagellum. The flagellum was enveloped with the plasma membrane and axoneme was covered by flagellum plasma membrane and made up of microtubules that were arranged in a 9 + 2 microtubular pattern (Figures 7A–D). Transmission electron images of post-thaw sperm showed that the morphologies and ultrastructure have been altered in some sperm. The main changes included the swollen and/or disrupted plasma membrane and the enlargement in the gap between plasma membrane and nuclear. The membrane of mitochondrion was wrinkled and its cristae was impaired. In the flagellum, its membrane was wrinkled as well, and the peripheral and central microtubule architectures were damaged (Figures 7E–H).
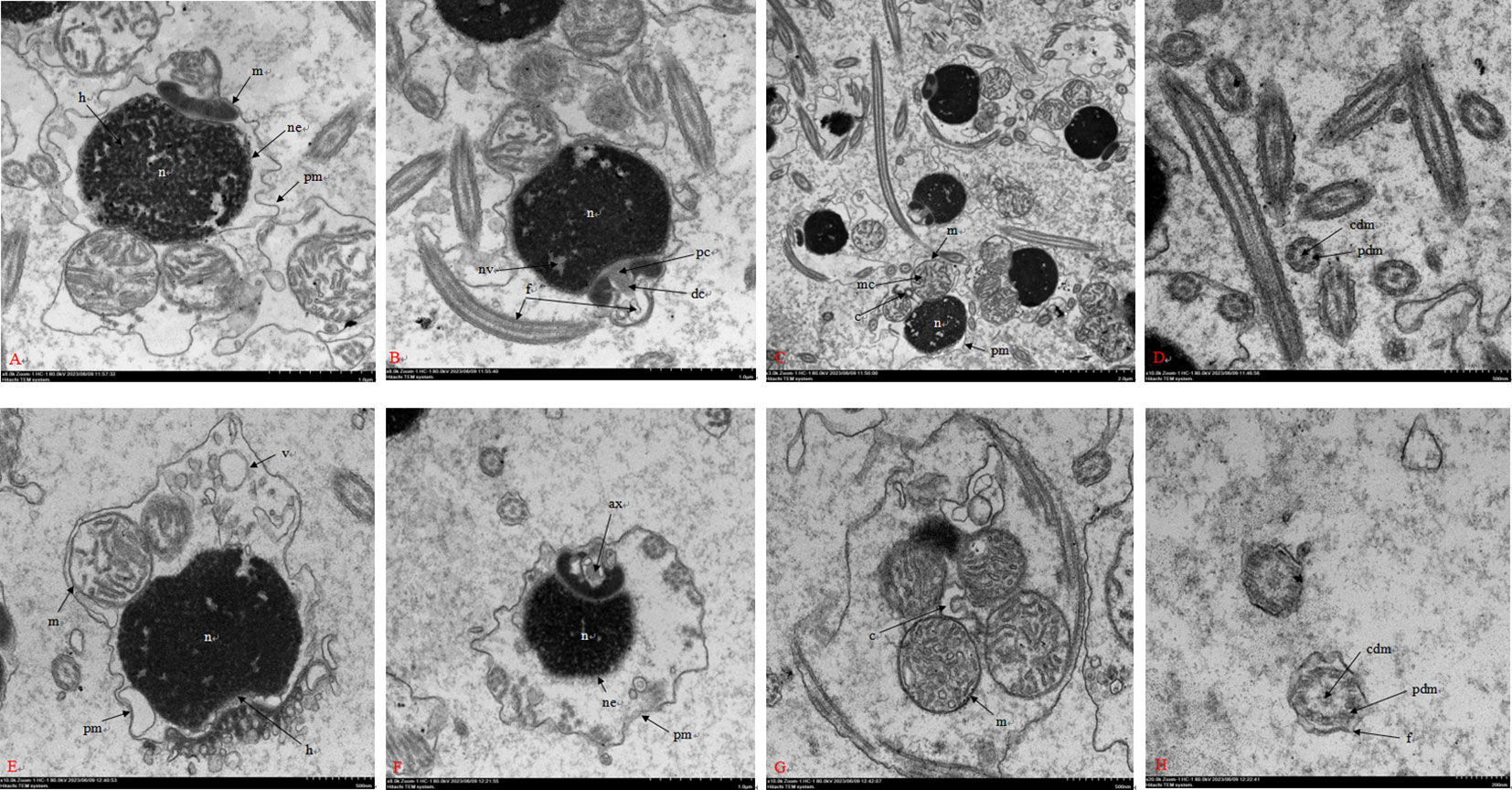
Figure 7 Transmission electron images of sperm in M. lateralis. (A–C) Longitudinal section of fresh sperm showing different structures; (D) Cross section of fresh sperm flagellum showing axoneme with proximal “9 + 2” microtubular structure; (E, F) Longitudinal section of post-thaw sperm with some damaged structures; (G) Cross section of post-thaw sperm midpiece; (H) Cross section of post-thaw sperm flagellum. c, centrosome; f, flagellum; h, head; m, mitochondria; n, nucleus; v, vesicle; ax, axoneme; dc, distal centriole; mc, mitochondrial crista; ne, nuclear envelope; nv, nuclear vacuole; pc, proximal centriole; pm, plasma membrane; cdm, central doublets of microtubules; pdm, peripheral doublets of microtubules.
The post-thaw sperm percentages of motility, PMI, MMP and AI (fluorescent images in Figure 8) were significantly lower than those in the fresh sperm (P < 0.05; Figure 9). Similarly, the activities of SOD, CAT and GSH in the post-thaw sperm were also significantly lower than those in the control (P < 0.05; Table 1). On the contrary, the level of lipid peroxidation in post-thaw sperm was significantly higher compared with the fresh counterpart (P < 0.05; Figure 10).
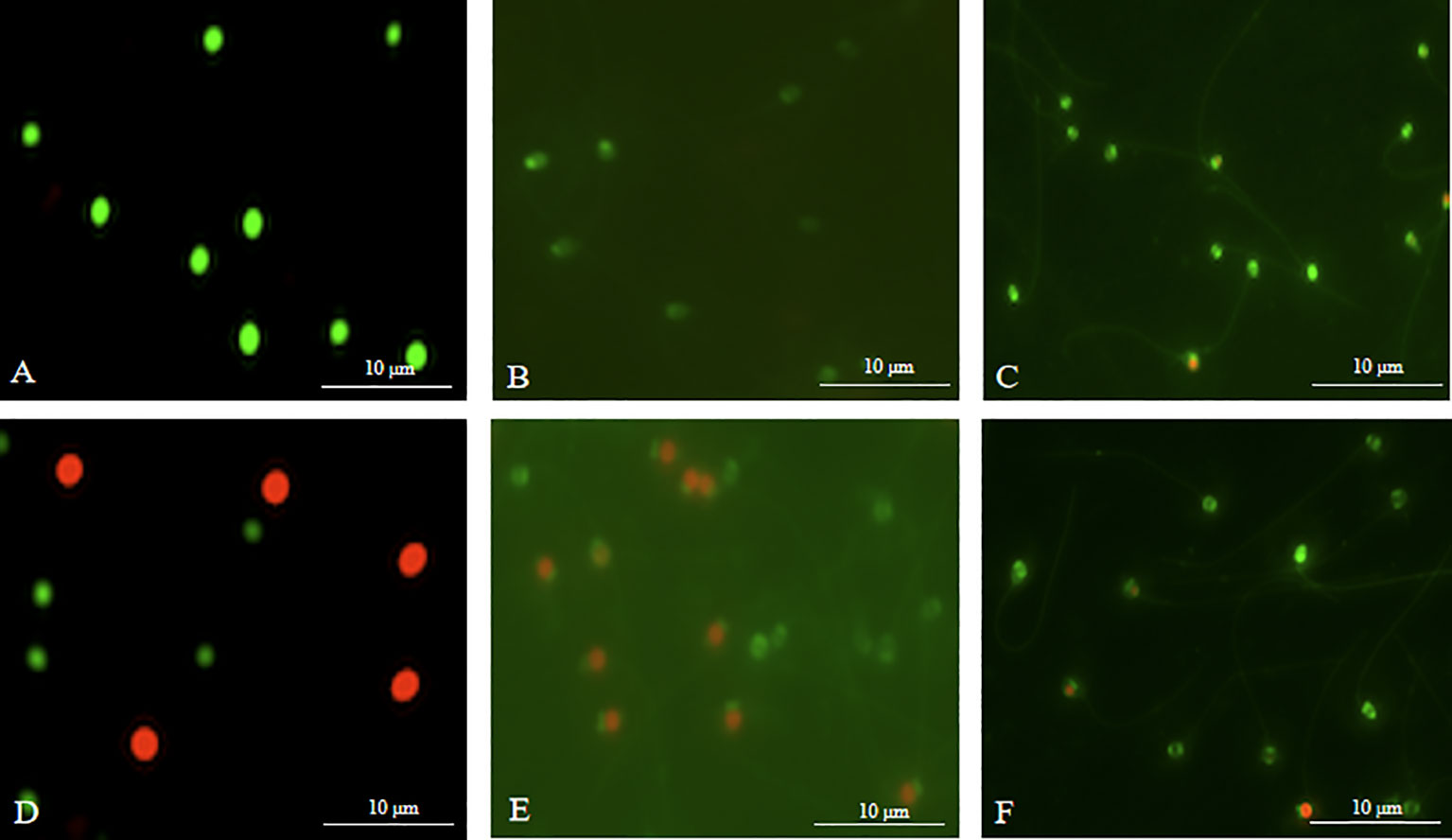
Figure 8 Photographs of sperm stained with fluorescents in M. lateralis (400 × magnification). (A-C) Fresh sperm - plasma membrane, mitochondria and acrosome, respectively; (D-F) Post-thaw sperm - plasma membrane, mitochondria and acrosome, respectively.
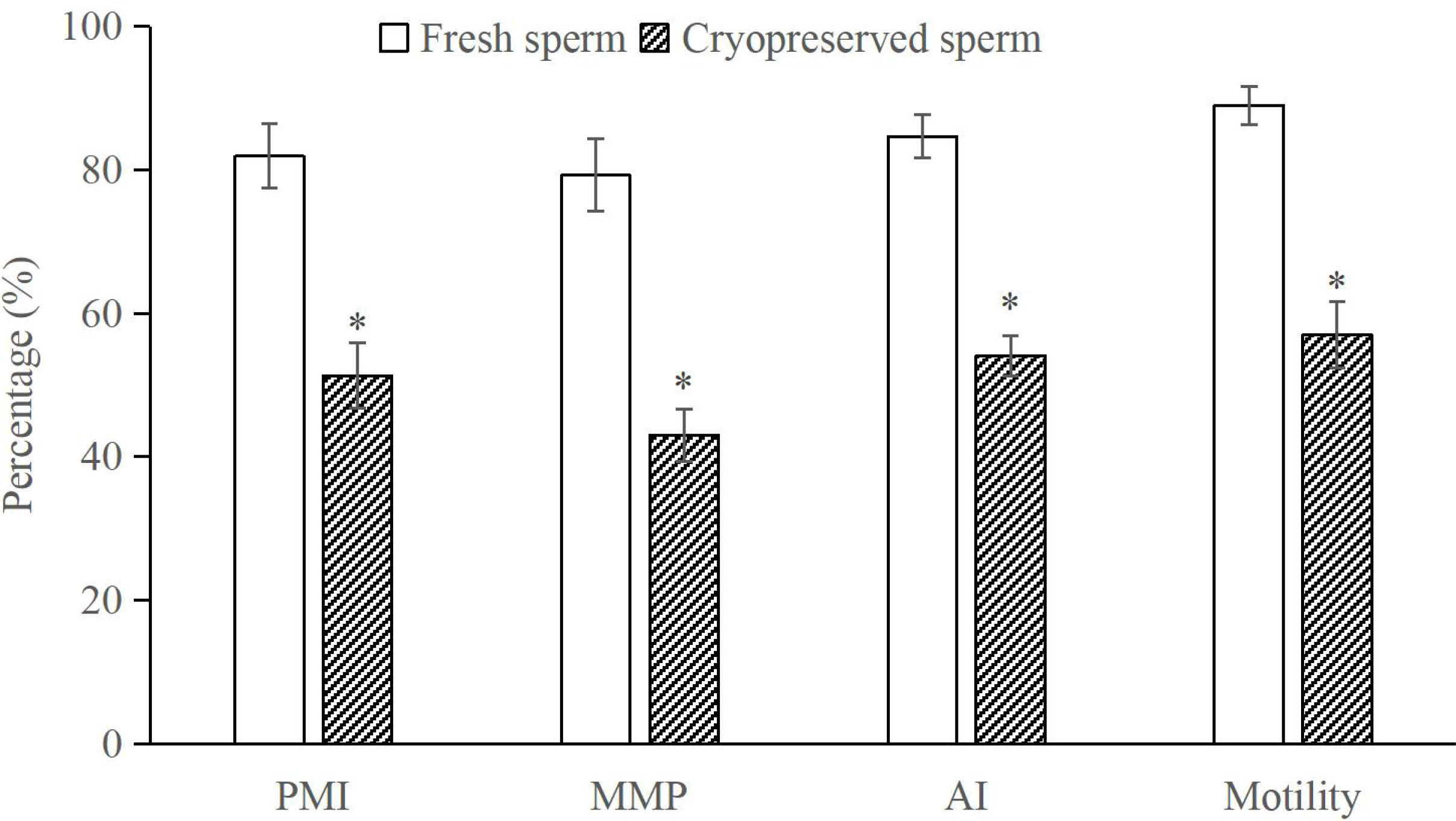
Figure 9 Comparison of motility, plasma membrane integrity (PMI), mitochondrial membrane potential (MMP) and acrosome integrity (AI) between fresh and cryopreserved sperm, n = 3. The asterisk at each parameter indicates significant difference.
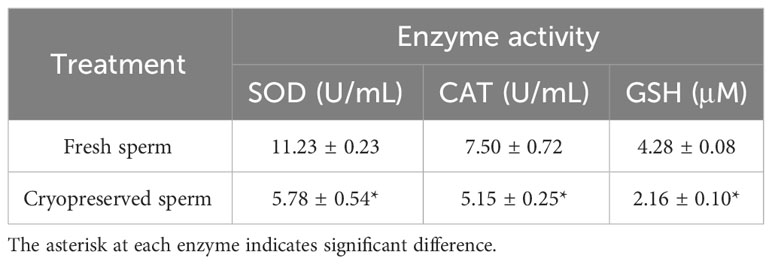
Table 1 Comparison of activities of superoxide dismutase (SOD), catalase (CAT) and glutathione (GSH) between fresh and cryopreserved sperm, n =3.
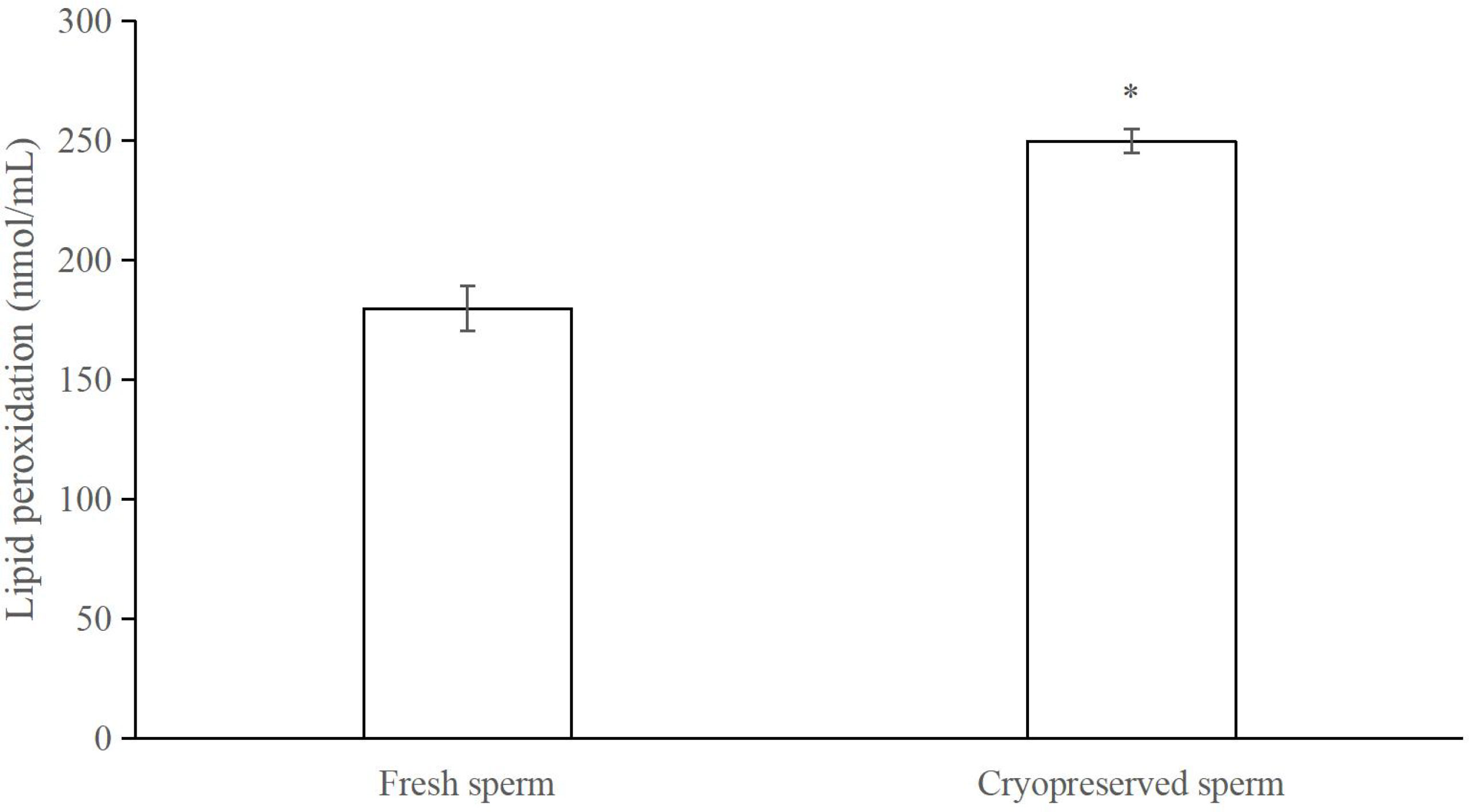
Figure 10 Comparison of lipid peroxidation levels between fresh and cryopreserved sperm, n = 3. The asterisk indicates significant difference.
4 Discussion
Maintaining sperm capability to fertilize eggs is the most important goal in the development of sperm cryopreservation techniques (Martínez-Páramo et al., 2017). In bivalves, although sperm motility is the common method to assess the post-thaw sperm quality, the published papers have suggested that the post-thaw D-stage larval rate would be more reliable to predict the further development of progeny produced by the cryopreserved sperm (Gwo et al., 2003; Ieropoli et al., 2004; Adams et al., 2008; Hassan et al., 2015). In addition, data on performance of D-stage larvae are easy to collect as the period required for fertilized eggs to develop into this stage is short (< one day) and they have not started feeding.
This study has developed a non-programmable sperm cryopreservation technique in the dwarf surfclam M. lateralis, resulting in a post-thaw D-stage larval rate similar to the fresh control with the optimized cryopreservation parameters. This result suggested that the technique commonly used in the bivalve sperm cryopreservation studies is suitable for M. lateralis. Therefore, this species could be used as a model to accelerate the investigation of sperm cryo-impact on the performance of resultant progeny at late developmental stages and/or subsequent generations, as these questions are the key concerns limiting the application of this technique in marine bivalve aquaculture and germplasm cryobanking.
Permeable CPAs could protect the cells intracellularly and extracellularly and are the essential ingredient for sperm cryopreservation in aquatic species (Martínez-Páramo et al., 2017). EG was found to be the most toxic to the sperm of M. lateralis in this study as a lower D-stage larval rate was produced in comparison with other CPAs at each concentration assessed. This result was in agreement with the findings in most marine molluscan, fish and crustacean sperm cryopreservation (Liu et al., 2015; Magnotti et al., 2018; Morales-Ueno and Paniagua-Chávez, 2020). However, it should note that this chemical has often been selected as a suitable CPA for oocyte and larvae cryopreservation in some aquatic and domestic animals (Tervit et al., 2005; Cirino et al., 2019; Tharasanit and Thuwanut, 2021; Zhu et al., 2023).
The equilibration time is the period for CPAs to enter into the sperm without or with limited toxicity prior to the initiation of cryopreservation process. In the current study, the D-stage larval rate was decreased with the increase in the equilibration period in all the CPAs evaluated. Except for 8% DMSO, there was no significant difference in the D-stage larval rate between 10 and 20 min equilibration periods in all other CPAs. This result agreed with the findings in oysters, abalone and mussels, where 10 min was normally identified as the optimal equilibration period (Liu et al., 2015).
Cooling is a key step for cryopreservation. Its rate should be fast enough to reduce the exposure time of sperm to the concentrated CPAs and also slow enough for dehydration to avoid the formation of intracellular ice crystals (Demoy-Schneider et al., 2020). In this study, the highest D-stage larval rate was achieved when the sperm were cryopreserved at 4 cm above the LN surface. This height was lower than those found in abalone H. laevigata and H. rubra (Liu et al., 2015), mussels M. galloprovincialis (Liu et al., 2016), pearl oysters Pinctada fucata martensii (Kawamoto et al., 2007) and P. margaritifera (Acosta-Salmόn et al., 2007), and great scallops Pecten maximus (Suquet et al., 2014), but higher than those in greenshell mussels Perna canaliculus (Smith et al., 2012) and pearl oysters P. margaritifera (Hui et al., 2011).
Thawing temperature is a critical factor to manage the risk of water recrystallization and its impact on sperm (Demoy-Schneider et al., 2020). Among the thawing temperatures evaluated in this study, 60°C produced the highest D-stage larval rate, although it did not differ significantly from 50°C. This temperature was the same as used in abalone H. laevigata and H. rubra (Liu et al., 2015). However, it was lower than those in oysters C. gigas (Gwo et al., 2003) and C. virginica (Paniagua-Chávez and Terisch, 2001), and abalone H. diversicolor (Gwo et al., 2002), but higher than those in oysters C. gigas (Adams et al., 2004; Dong et al., 2005) and O. edulis (Vitiello et al., 2011), abalone H. rufescens (Salinas-Flores et al., 2005), pearl oysters (Acosta-Salmόn et al., 2007; Kawamoto et al., 2007; Narita et al., 2008; Hui et al., 2011), mussels (Matteo et al., 2009; Smith et al., 2012) and scallops P. maximus (Suquet et al., 2014). These suggest that the thawing temperature might be species specific and also be related to the cooling methodology used in the same species.
It is generally accepted that cryodamge on sperm is inevitable, resulting in a higher sperm to egg ratio being required for the post-thaw sperm fertilization (Martínez-Páramo et al., 2017; Yánez-Ortiz et al., 2022). In this study, a ratio of 220 times higher than the fresh control was needed to produce a similar D-stage larval rate. This ratio was higher than that in oysters C. gigas (Ieropoli et al., 2004) and scallops P. maximus (Suquet et al., 2014), but lower than studies in most marine molluscan species, such as oysters (Paniagua-Chávez and Terisch, 2001; Gwo et al., 2003; Adams et al., 2004), abalone (Liu et al., 2015), pearl oysters (Hui et al., 2011) and mussels (Smith et al., 2012; Liu et al., 2016).
The assessment of post-thaw sperm quality could provide useful information for understanding cryodamage to further improve the sperm cryopreservation protocol (Yánez-Ortiz et al., 2022). The cryopreservation process could compromise the cellular structures and organelles, such as plasma membrane, mitochondria, acrosome and flagellum (Partyka et al., 2012; Peris-Frau et al., 2020; Xin et al., 2020), which have been clearly revealed by the electronic microscope images in this study. Sperm membranes are the primary sites of cryoinjury (Partyka et al., 2012). One of the key reasons responsible for altering the membrane integrity is the lipid peroxidation products generated from the oxidative stress, which has been shown in horse (Nelid et al., 2005), fish (Cabrita et al., 2010), fowl (Partyka et al., 2012) and boar (Guo et al., 2021) sperm cryopreservation. In this study, the increased lipid peroxidation level in post-thaw sperm would also contribute the reduced plasma membrane integrity in M. lateralis.
Acrosome reaction is the key event bringing together the fusogenic areas of sperm and egg during fertilization in marine bivalve species (Longo and Scarpa, 1991; Rosati, 1995). It involves in the fusion of plasma membrane of both sex gametes and the release of hydrolytic enzymes from sperm for penetrating the egg envelop (Rosati, 1995). Therefore, maintaining the acrosome integrity is vital during the cryopreservation. In the current study, the acrosome integrity of post-thaw sperm was significantly decreased, which might be due to the acrosomal membrane injury by the increased level of lipid peroxidation after cryopreservation as discussed previously or the formation of ice crystals. This phenomenon has also been reported in the fowl (Partyka et al., 2012) and bull (Jakop et al., 2023) sperm cryopreservation. Mitochondria are highly susceptible to the changes in intracellular reactive oxygen species which could be increased by the electron leakage from the uncoupled mitochondria oxidative phosphorylation during cryopreservation (Partyka et al., 2012). Consequently, as less number of post-thaw sperm could maintain the high MMP, their ATP production and motility rate would be compromised (Partyka et al., 2012). In this study, the post-thaw sperm motility was significantly compromised and this reduction has often been reported in other marine molluscan species (Liu et al., 2015). In this study, the significant decrease in the post-thaw sperm MMP might also be associated with the enhancement of lipid peroxidation level. Similar phenomena have been reported in the sperm cryopreservation in fowls (Partyka et al., 2012) and fishes (Figueroa et al., 2019).
Antioxidants are molecules capable of preventing, delaying or inhibiting the oxidation of other molecules, thus mitigating the oxidative damage [56]. In general, the antioxidant defense of biological systems could be mediated by either enzymatic or non-enzymatic antioxidants (Amidi et al., 2016; Sandoval-Vargas et al., 2021). GSH is a non-enzymatic and the most abundant antioxidant in almost all cell types (Len et al., 2019), participating directly in the neutralization of oxidative damage (Amidi et al., 2016). Both SOD and CAT belong to the enzymatic antioxidant and could quickly neutralize any reactive molecules by different reactions (Amidi et al., 2016; Sandoval-Vargas et al., 2021). In the current study, the activities of these enzymes were all significantly decreased in post-thaw sperm which agreed with the studies in fowls (Partyka et al., 2012), Pacific cods Gadus microcephalus (Wang et al., 2016). These results also indicate that the activities of intracellular antioxidant enzymes might not be enough to neutralize the damages produced by the oxidative stress during the sperm cryopreservation in M. lateralis.
5 Conclusions
The knowledge gap in cryo-impacts on resultant progeny at late developmental stages and/or next generations has constrained the application of sperm cryopreservation technique in bivalve aquaculture industry for different purposes, such as assistances in commercial production, selective breeding and cross breeding. The gap mainly resulted from the long generation interval, and challenges and high costs of maintaining experiments in the open marine environment where most bivalve species are farmed. These issues could be addressed if a model species, such as M. lateralis, could be used as this species has a very short generation interval (three months) and well established laboratory culture technique. The development of a non-programmable sperm cryopreservation technique in M. lateralis in this study is a cornerstone to bridge the knowledge gap as this technique is commonly used in bivalve species.
Data availability statement
The original contributions presented in the study are included in the article/supplementary material. Further inquiries can be directed to the corresponding authors.
Ethics statement
The requirement of ethical approval was waived by College of Marine Life Sciences, Ocean University of China for the studies involving animals because Mulinia lateralis belongs to the invertebrate and according to rules and regulations of ethics committee in College of Marine Life Sciences, Ocean University of China, no ethical approval needed. The studies were conducted in accordance with the local legislation and institutional requirements. Written informed consent was obtained from the owners for the participation of their animals in this study.
Author contributions
ZX: Data curation, Investigation, Methodology, Writing – original draft. ZY: Investigation, Methodology, Writing – original draft. LB: Data curation, Writing – review & editing. XL: Conceptualization, Methodology, Writing – review & editing. XH: Conceptualization, Methodology, Writing – review & editing. YL: Conceptualization, Investigation, Methodology, Writing – review & editing.
Funding
The author(s) declare financial support was received for the research, authorship, and/or publication of this article. This research was funded by the National Key Research and Development Program of China (2022YFD2400105; 2021YFD1200805), National Natural Science Foundation of China (32273153), Youth Innovation Team Plan of Higher Education Institutions in Shandong Province (2022KJ046), Taishan Scholars Youth Expert Program of Shandong Province (No.tsqnz20221110) and Earmarked Fund for Modern Agro-industry Technology Research System (CARS-49).
Conflict of interest
The authors declare that the research was conducted in the absence of any commercial or financial relationships that could be construed as a potential conflict of interest.
Publisher’s note
All claims expressed in this article are solely those of the authors and do not necessarily represent those of their affiliated organizations, or those of the publisher, the editors and the reviewers. Any product that may be evaluated in this article, or claim that may be made by its manufacturer, is not guaranteed or endorsed by the publisher.
References
Acosta-Salmόn H., Jerry D. R., Southgate P. C. (2007). Effects of cryoprotectant agents and freezing protocol on motility of black-lip pearl oyster (Pinctada margaritifera L.) spermatozoa. Cryobiology 54, 13–18. doi: 10.1016/j.cryobiol.2006.10.189
Adams S. L., Smith J. F., Roberts R. D., Janke A. R., Kaspar H. F., Tervit H. R., et al. (2004). Cryopreservation of sperm of the Pacific oyster (Crassostrea gigas): development of a practical method for commercial spat production. Aquaculture 242, 271–282. doi: 10.1016/j.aquaculture.2004.08.034
Adams S. L., Smith J. F., Roberts R. D., Janke A. R., King N. G., Tervit H. R., et al. (2008). Application of sperm cryopresrevation in selective breeding of the Pacific oyster, Crassostrea gigas (Thunberg). Aquac. Res. 39, 1434–1442. doi: 10.1111/j.1365-2109.2008.02013.x
Aleström P., Winther-Larsen H. C. (2016). “Zebrafish offer aquaculture research their services,” in Genomics in Aquaculture. Eds. MacKenzie S., Jentoft S. (Academic Press, UK), 165–194.
Amidi F., Pazhohan A., Nashtaei M. S., Khodarahmian M., Nekoonam S. (2016). The role of antioxidants in sperm freezing: a review. Cell. Tissue. Bank. 17, 745–756. doi: 10.1007/s10561-016-9566-5
Bøe K., Bjøru B., Bårdsen M. T., Wist A. N., Wolla S., Sivertsen A. (2021). Opportunities and challenges related to sperm cryopreservation in Atlantic salmon gene banks. Conserv. Sci. Pract. 3, e552. doi: 10.1111/csp2.552
Cabrita E., Sarasquete C., Martínez-Páramo S., Robles V., Beirão J., Pérez-Cerezales S., et al. (2010). Cryopreservation of fish sperm: applications and perspectives. J. Appl. Ichthyol. 26, 623–635. doi: 10.1111/j.1439-0426.2010.01556.x
Cirino L., Wen Z., Hsieh K., Huang C., Leong Q. L., Wang L., et al. (2019). First instance of settlement by cryopreserved coral larvae in symbiotic association with dinoflagellates. Sci. Rep. 9, 18851. doi: 10.1038/s41598-019-55374-6
Demoy-Schneider M., Schmitt N., Suquet M., Cosson J. (2020). “State of the art in cryopreservation of bivalve spermatozoa,” in Cryopreservation of fish gametes. Eds. Betsy J., Kumar S. (Springer, Singapore), 239–283.
Dong Q., Eudeline B., Huang C., Allen S. K. Jr., Tiersch T. R. (2005). Commercial-scale sperm cryopreservation of diploid and tetraploid Pacific oysters, Crassostrea gigas. Cryobiology 50, 1–16. doi: 10.1016/j.cryobiol.2004.09.003
Dong Q., Jing R., Miao M., Huang C. (2008). Cryopreservation of zebrafish sperm: advantages with aquatic model species. Cryobiology 57, 320. doi: 10.1016/j.cryobiol.2008.10.026
FAO (2022). The state of world fisheries and aquaculture 2022. Towards blue transformation (Rome: FAO). doi: 10.4060/cc0461en
Fernández-Díez C., Herráez M. P. (2018). Changes in transcriptomic profile of trout larvae obtained with frozen sperm. Aquaculture 492, 306–320. doi: 10.1016/j.aquaculture.2018.04.022
Figueroa E., Lee-Estevez M., Valdebenito I., Watanabe I., Oliveira R. P. S., Romero J., et al. (2019). Effects of cryopreservation on mitochondrial function and sperm quality in fish. Aquaculture 511, 634190. doi: 10.1111/raq.12105
Guo H., Wang J., Sun L., Jin X., Shi X., Lin J., et al. (2021). Effects of astaxanthin on plasma membrane function and fertility of boar sperm during cryopreservation. Theriogenology 164, 58–64. doi: 10.1016/j.theriogenology.2021.01.007
Guo X. L., Li X. X., Zhao F., Liu D. W., Yang Z. J., Li M. L., et al. (2023). Full-length transcriptome analysis provides insights into larval shell formation in Mulinia lateralis. Front. Mar. Sci. 9. doi: 10.3389/fmars.2022.1111241
Gwo J., Chen C., Cheng H. (2002). Semen cryopreservation of small abalone (Haliotis diversicolor supertexa). Theriogenology 58, 1563–1578. doi: 10.1016/S0093-691x(02)01055-5
Gwo J. C., Wu C. Y., Chang W. S. P., Cheng H. Y. (2003). Evaluation of damage in Pacific oyster (Crassostrea gigas) spermatozoa before and after cryopreservation using comet assay. CryoLetters 24, 171–180.
Hassan M. M., Qin J. G., Li X. X. (2015). Sperm cryopreservation in oysters: A review of its current status and potentials for future application in aquaculture. Aquaculture 438, 24–32. doi: 10.1016/j.aquaculture.2014.12.037
Heres P., Troncoso J., Paredes E. (2022). Long-term study on survival and development of successive generations of Mytilus galloprovincialis cryopreserved larvae. Sci. Rep. 12, 13632. doi: 10.1038/s41598-022-17935-0
Hui B., Vonau V., Moriceau J., Tetumu R., Vanaa V., Demoy-Schneider M., et al. (2011). Hatchery-scale trials using cryopreserved spermatozoa of black-lip pearl oyster, Pinctada margaritifera. Aquat. Living Resour. 24, 219–223. doi: 10.1051/alr/2011117
Ieropoli S., Masullo P., Santo M. D. E., Sansone G. (2004). Effects of extender composition, cooling rate and freezing on the fertilization viability of spermatozoa of the Pacific oyster (Crassostrea gigas). Cryobiology 49, 250–257. doi: 10.1016/j.cryobiol.2004.08.005
Jakop U., Engel K. M., Hürland M., Müller P., Osmers J., Jung M., et al. (2023). Lipid alterations by oxidative stress increase detached acrosomes after cryopreservation of semen in Holstein bulls. Theriogenology 197, 37–45. doi: 10.1016/j.theriogenology.2022.11.036
Jia G., Fu X., Cheng K., Yue M., Jia B., Hou Y., et al. (2015). Spermatozoa cryopreservation alters pronuclear formation and zygotic DNA demethylation in mice. Theriogenology 83, 1000–1006. doi: 10.1016/j.theriogenology.2014.11.036
Kawamoto T., Narita T., Isowa K., Aoki H., Hayashi M., Komaru A., et al. (2007). Effects of cryopreservation methods on post-thaw motility of spermatozoa from the Japanese pearl oyster, Pinctada fucata martensii. Cryobiology 54, 19–26. doi: 10.1016/j.cryobiol.2006.10.190
Len J. S., Koh W. S. D., Tan S. (2019). The roles of reactive oxygen species and antioxidants in cryopreservation. Biosci. Rep. 39, BSR20191601. doi: 10.1042/BSR20191601
Li Y. J., Liu L. J., Zhang L. J., Wei H. L., Wu S. X., Liu T., et al. (2022). Dynamic transcriptome analysis reveals the gene network of gonadal development from the early history life stages in dwarf surfclam Mulinia lateralis. Biol. Sex Differ. 13, 69. doi: 10.1186/s13293-022-00479-3
Liu B., Liu Y., Liu S., Xu T., Liu Q., Li X. (2016). Cryopreservation of strip spawned sperm using non-programmable freezing technique in the blue mussel Mytilus galloprovincialis. Aquacu. Res. 47, 3888–3898. doi: 10.1111/are.12839
Liu Y., Li X., Robinson N., Qin J. (2015). Sperm cryopreservation in marine mollusk: a review. Aquacu. Int. 23, 1505–1524. doi: 10.1007/s10499-015-9900-0
Liu Y., Xu T., Robinson N., Qin J., Li X. (2014). Cryopreservation of sperm in farmed Australian greenlip abalone Haliotis laevigata. Cryobiology 68, 185–193. doi: 10.1016/j.cryobiol.2014.01.002
Longo F. J., Scarpa J. (1991). Expansion of the sperm nucleus and association of the maternal and paternal genomes in fertilized Mulinia lateralis eggs. Biol. Bull. 180, 56–64. doi: 10.2307/1542428
Magnotti C., Cerqueira V., Lee-Estevez M., Farias J. G., Valdebenito I., Figueroa E. (2018). Cryopreservation and vitrification of fish semen: a review with special emphasis on marine species. Rev. Aquac. 10, 15–25. doi: 10.1111/raq.12145
Martínez-Páramo S., Horváth Á., Labbé C., Zhang T., Robles V., Herráez P., et al. (2017). Cryobanking of aquatic species. Aquaculture 472, 156–177. doi: 10.1016/j.aquaculture.2016.05.042
Matteo O., Langellotti A. L., Masullo P., Sansone G. (2009). Cryopreservation of the Mediterranean mussel (Mytilus galloprovincialis) spermatozoa. Cryobiology 58, 145–150. doi: 10.1016/j.cryobiol.2008.09.015
Morales-Ueno K., Paniagua-Chávez C. G. P. (2020). “Sperm cryopreservation in crustaceans,” in Cryopreservation of fish gametes. Eds. Betsy J., Kumar S. (Springer, Singapore), 211–238.
Müller T., Matsubara H., Kubara Y., Horváth Á., Kolics B., Taller J., et al. (2018). Testing cryopreserved European eel sperm for hybridization (A. japonica × A. Anguilla). Theriogenology 113, 153–158. doi: 10.1016/j.theriogenology.2018.02.021
Narita T., Kawamoto T., Isowa K., Aoki H., Hayashi M., Ohta H., et al. (2008). Effects of cryopreservation on sperm structure in Japanese pearl oyster Pinctada fucata martensii. Fish. Sci. 74, 1069–1074. doi: 10.1111/j.1444-2906.2008.01626.x
Nelid D. M., Brouwers J. F. H. M., Colenbrander B., Agüero A., Gadella B. M. (2005). Lipid peroxide formation in relation to membrane stability of fresh and frozen thawed stallion spermatozoa. Mol. Reprod. Dev. 72, 230–238. doi: 10.1002/mrd.20322
Nusbaumer D., de Cunha L. M., Wedekind C. (2019). Sperm cryopreservation reduces offspring growth. Proc. R. Soc B 286, 20191644. doi: 10.1098/rspb.2019.1644
Ortiz-Rodriguez J., Ortega-Ferrusola C., Gil M. C., Martín-Cano F. E., Gaitskell-Phillips G., Rodríguez-Martínez H., et al. (2019). Transcriptome analysis reveals that fertilization with cryopreserved sperm downregulates genes relevant for early embryo development in the horse. PLoS ONE 14, e0213420. doi: 10.1371/journal.pone.0213420
Paniagua-Chávez C. G., Terisch T. R. (2001). Laboratory studies of cryopreservation of sperm and trochophore larvae of the Eastern oyster. Crybiology 43, 211–223. doi: 10.1006/cryo.2001.2346
Partyka A., Łukaszewicz E., Niżański W. (2012). Effect of cryopreservation on sperm parameters, lipid peroxidation and antioxidant enzymes activity in fowl semen. Theriogenology 77, 1497–1504. doi: 10.1016/j.theriogenology.2011.11.006
Peris-Frau P., Soler A. J., Iniesta-Cuerda M., Martín-Maestro A., Sánchez-Ajofrín I., Medina-Chávez D. A., et al. (2020). Sperm cryodamage in ruminants: understanding the molecular changes induced by the cryopreservation process to optimize sperm quality. Int. J. Mol. Sci. 21, 2781. doi: 10.3390/ijms21082781
Rosati F. (1995). Sperm-egg interactions during fertilization in invertebrates. Boll. Zool. 62, 323–334. doi: 10.1080/11250009509356084
Salinas-Flores L., Paniagua-Chavez C. G., Jenkins J. A., Tiersch T. (2005). Cryopreservation of sperm of red abalone (Haliotis rufescens). J. Shellfish Res. 24, 415–420. doi: 10.1111/raq.12479
Sandoval-Vargas L., Jiménez M. S., González J. R., Villalobos E. F., Cabrita E., Isler I. V. (2021). Oxidative stress and use of antioxidants in fish semen cryopreservation. Rev. Aquac. 13, 365–387. doi: 10.1111/raq.12479
Silina A. V. (2018). Sex change in scallop Patinopecten yessoensis: response to population composition? PeerJ 6, 55240. doi: 10.7717/peerj.5240
Smith J. F., Adams S. L., Gale S. L., McGowan L. T., Tervit H. R., Roberts R. D. (2012). Cryopreservation of greenshellTM mussel (Perna canaliculus) sperm. I. establishment of freezing protocol. Aquaculture 334-337, 199–204. doi: 10.1016/j.aquaculture.2011.12.027
Suquet M., Arsenault-Pernet M. E. J., Ratiskol D., Mingant C. (2014). Cryopreservation of great scallop (Pecten maximus) sperm: effect of extender, cryoprotectant and cooling rate on sperm survival. Aquat. Living Resour. 27, 35–40. doi: 10.1051/alr/2014002
Tervit H. R., Adams S. L., Roberts R. D., McGowan L. T., Pugh P. A., Smith J. F., et al. (2005). Successful cryopreservation of Pacific oyster (Crassostrea gigas) oocytes. Cryobiology 51, 142–151. doi: 10.1016/j.cryobiol.2005.06.001
Tharasanit T., Thuwanut P. (2021). Oocyte cryopreservation in domestic animals and humans: principles, techniques and updated outcomes. Animals 11, 2949. doi: 10.3390/ani11102949
Vitiello V., Carlino P. A., Prete F. D., Langellotti A. L., Sansone G. (2011). Effects of cooling and freezing on the motility of Ostrea edulis (L. 1758) spermatozoa after thawing. Cryobiology 63, 118–124. doi: 10.1016/j.cryobiol.2011.07.004
Wang X., Shi X., Liu Y., Yu D., Guang S., Liu Q., et al. (2016). Effects of chilled storage and cryopreservation on sperm characteristics, antioxidant enzyme activities, and lipid peroxidation in Pacific cod Gadus microcephalus. Chin. J. Oceanol. Limnol. 34, 763–771. doi: 10.1007/s00343-016-5088-z
Xin M. M., Niksirat H., Shaliutina-Kolešová A., Siddique M. A. M., Sterba J., Boryshpolets S., et al. (2020). Molecular and subcellular cryoinjury of fish spermatozoa and approaches to improve cryopreservation. Rev. Aquac. 12, 909–924. doi: 10.1111/raq.12355
Yánez-Ortiz I., Catalán J., Rodríguez-Gil J. E., Mirό J., Yeste M. (2022). Advances in sperm cryopreservation in farm animals: cattle, horse, pig and sheep. Anim. Reprod. Sci. 246, 106904. doi: 10.1016/j.anireprosci.2021.106904
Yang H. P., Guo X. M. (2006). Polyploid induction by heat shock-induced meiosis and mitosis inhibition in the dwarf surfclam, Mulinia lateralis Say. Aquaculture 252, 171–182. doi: 10.1016/j.aquaculture.2005.07.017
Yang Z. J., Huang X. T., Wang H., Pan H. Y., Wang X. L., Teng M. X., et al. (2021). Effects of microalgae diets and stocking density on larval growth, survival and metamorphosis of dwarf surfclam, Mulinia lateralis. Aquaculture 536, 736440. doi: 10.1016/j.aquaculture.2021.736440
Zhang S. Q., Zhao X., Feng K. S., Hu Y. C., Tillotson M. R., Yang L. (2022). Do mariculture products offer better environment and nutritional choices compared to land-based protein products in China? J. Clean. Prod. 372, 133697. doi: 10.1016/j.jclepro.2022.133697
Keywords: Mulinia lateralis, sperm cryopreservation, dimethyl sulfoxide, cryobanking, cryo-impact investigation
Citation: Xu Z, Yang Z, Bao L, Li X, Huang X and Liu Y (2024) Development of a non-programmable sperm cryopreservation technique in dwarf surfclams Mulinia lateralis–a potential model species for bivalve research. Front. Mar. Sci. 11:1357454. doi: 10.3389/fmars.2024.1357454
Received: 18 December 2023; Accepted: 05 February 2024;
Published: 16 February 2024.
Edited by:
Bin Xia, Qingdao Agricultural University, ChinaReviewed by:
Shaharior Hossen, Chonnam National University, Republic of KoreaMuhammed Duman, Bursa Uludağ University, Türkiye
Copyright © 2024 Xu, Yang, Bao, Li, Huang and Liu. This is an open-access article distributed under the terms of the Creative Commons Attribution License (CC BY). The use, distribution or reproduction in other forums is permitted, provided the original author(s) and the copyright owner(s) are credited and that the original publication in this journal is cited, in accordance with accepted academic practice. No use, distribution or reproduction is permitted which does not comply with these terms.
*Correspondence: Yibing Liu, bGl1eWliaW5nQG91Yy5lZHUuY24=; Xiaoting Huang, eHRodWFuZ0BvdWMuZWR1LmNu