- 1School of Advanced Manufacturing/School Of Ocean, Fuzhou University, Fuzhou, China
- 2Technical Innovation Center for Utilization of Marine Biological Resources, Third Institute of Oceanography, Ministry of Natural Resources, Xiamen, China
Background: Fucoxanthin is a carotenoid found in seaweed. Its unique chemical structure gives it a variety of properties. Thus fucoxanthin have attracted the attention of companies and researchers.
Methods: Scientific papers were collected from the database. Duplicates and unavailable literature were excluded first. Then the remaining literature was categorized for referencing in the review.
Results: This article contains a summary of the microalgae species producing fucoxanthin and their progress in breeding and cultivation modes. Additionally, the review summarized the progress of research on physiological activities and organized the experimental models used in these studies.
Conclusions: These present findings may provide information for the upstream production of fucoxanthin from algal species selection to process optimization. The analysis of the physiological activity results will help advance subsequent physiological and biochemical experiments. Furthermore, it intends to pique researchers’ enthusiasm for fucoxanthin and enrich related research data to accelerate the development of this natural product.
1 Introduction
Fucoxanthin, a carotenoid, is a significant component of carotenoids found in nature, accounting for more than 10% (Matsuno, 2001). This natural product contains multiple functional groups, as depicted in Figure 1, enabling it to bind to a wider range of disease targets. It has shown biological activity and has good nutritional and health care value. Fucoxanthin nutraceuticals have gained approved from the Food Standards Agency of Australia and New Zealand (Abu-Ghosh et al., 2021), resulting in a high demand for this compound. However, it’s supply remains limited. A Flow chart illustrating the culture and product application of microalgae producing fucoxanthin is presented in Figure 2.
As of 2021, the price of high-purified fucoxanthin ranges from 40,000 to 80,000 USD/kg (Market Watch, 2020). This elevated price significantly hiders the commercialization, progress of fucoxanthin, necessitating the exploration of new approaches for industrialingze the production of fucoxanthin raw materials. Fucoxanthin products are primarily extracted from macroalgae such as kelp and sea mustard. However, macroalgae have several drawbacks, including a lengthy growth cycle, low fucoxanthin content (0.02~0.58 mg/g fresh weight) (Mori et al., 2004), and other shortcomings, such as thick algal cell walls, high content of polysaccharides, and difficulties in crushing, which contribute to high production costs (Kim et al., 2011; Joel, 2016). On the other hand, marine microalgae have been found to be rich in fucoxanthin and easy to obtain. These microalgae have a fucoxanthin content about 100 times higher than that of macroalgae. For example, the fucoxanthin content in Synurophyceae is as high as 26.6 mg/g dry weight (Petrushkina et al., 2017). Diatoms have a fucoxanthin content of up to 25.5 mg/g dry weight (Wang et al., 2018), and Prymnesiophyceae have a fucoxanthin content of 18.23 mg/g dry weight (Petrushkina et al., 2017).
This marine natural substance has gained increased attention in recent years for its potential in various diseases related to inflammation and oxidative stress reactions. These include skin inflammation (Rodríguez-Luna et al., 2018; Spagolla Napoleão Tavares et al., 2020), ulcerative colitis (Yang et al., 2020), and contact hypersensitivity (Sakai et al., 2011). It has also been a research hotspot for its anti-diabetic (Maeda et al., 2007; Sakai et al., 2011; Kong et al., 2019) and anti-obesity (Abidov et al., 2010; Hu et al., 2012; Hitoe and Shimoda, 2017; Gille et al., 2019; Koo et al., 2019) efficacy. Non-alcoholic steatohepatitis (Takatani et al., 2020; Shih et al., 2021; Ye et al., 2022), Alzheimer’s disease (Lin et al., 2017; Xiang et al., 2017; Alghazwi et al., 2019; Shih et al., 2021; Yang et al., 2021; Zhu et al., 2021), and other biological activities [e.g., antiviral (Tsushima et al., 1995; Tamama, 2021)] have also been proven.
Microalgae-produced fucoxanthin is green and environmentally friendly, which is in line with the development trend. However, the current production of fucoxanthin from microalgae is constrained by traditional technologies. The precise pharmacological targets and signaling mechanisms of fucoxanthin remain unclear. Therefore, there is a need to further develop research on the upstream and downstream of fucoxanthin.
This review focuses on the production of fucoxanthin by microalgae and its pharmacological activity. It provides a summary of fucoxanthin-rich microalgae and their culture modes. The review also discusses the recent developments in investigating the pharmaceutical properties of fucoxanthin.
2 Results
2.1 Microalgae producing fucoxanthin and its biological properties
2.1.1 Microalgae producing fucoxanthin
Fucoxanthin in microalgae often binds to chlorophyll to form a fucoxanthin chlorophyll a/c protein complex (FCP), which can respond quickly to changes in the light environment (Xia et al., 2013). While fucoxanthin can be chemically synthesized, it is more efficient to extract it from microalgae. Compared with plants, microalgae grow quickly, often doubling their number per hour. Their productivity is measured in days, whereas plants often take months or years to produce. Microalgae isolated from nature are small and easily viable, making them suitable for cultivation in closed systems in laboratories or factories. There do not require arable land and are unaffected by seasonal climate and the marine environment. Moreover, the high fucoxanthin content of microalgae, the relative ease of the extraction and preparation process compared to macroalgae, and the variery of strain sources make microalgae an ideal cellular factory for the sustainable, large-scale production of fucoxanthin.
2.1.2 Synthesis pathway of fucoxanthin in microalgae
The biosynthesis of fucoxanthin was studied using Phaeodactylum tricornutum as a common model species, with high photosynthetic efficiency (Figure 3). The process begins with the generation of isopentenyl pyrophosphate (IPP) through the mevalonate pathway, which is the rate-limiting step in the synthesis of β-carotene. Geranylgeranyl diphosphate (GGPP) is produced by IPP under the action of geranyl pyrophosphate synthase (GGPS) (Xia et al., 2013). Subsequently, octahedron lycopene synthase (PSY) enzyme converts GGPP to produce the first carotenoid, phytoene (Kadono et al., 2015). Phytoene undergoes continuous dehydrogenation by octahydron lycopene desaturase (PDS), ζ-carotene desaturase (ZDS), carotenoid isomerase (CRTISO), resulting in the formation of lycopene (Kadono et al., 2015). Lycopene can produce α-carotene or further forms β-carotene from γ-carotene. Under the action of β-carotene hydroxylase (BCH), β-carotene generates zeaxanthin. Zeaxanthin epoxidase (ZEP) cyclizes zeaxanthin to form violaxanthin through two-step catalysis (Coesel et al., 2008). Ultimately, fucoxanthin can be synthesized from Diadinoxanthin (Lohr and Wilhelm, 1999) or also from Neoxanthin (Kadono et al., 2015). There are two hypothetical pathways for fucoxanthin synthesis, Figure 3. These are the currently known pathway for fucoxanthin synthesis.
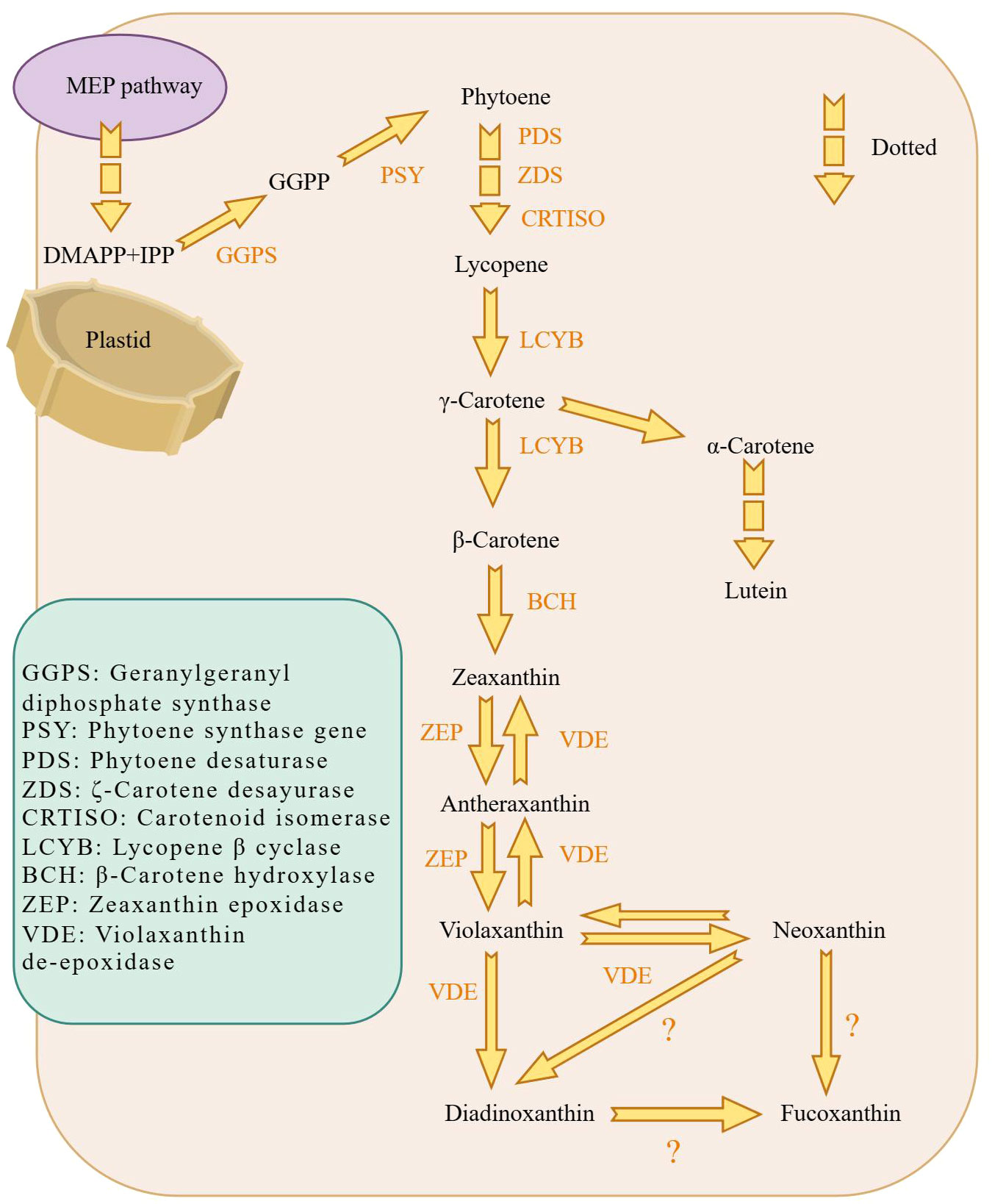
Figure 3 Schematic diagram of the biosynthetic pathway of fucoxanthin in diatoms. fucoxanthin is mainly generated in plastids. The MEP pathway provides the material prerequisites for fucoxanthin synthesis, which is then followed by GGPS to obtain GGPP. GGPP is processed by PSY to generate phytoene, the first carotenoid substance synthesized. And γ-Carotene continues to generate β-Carotene. Eventually, Violaxanthin synthesizes fucoxanthin via Neoxanthin and possibly fucoxanthin synthesized from Diadinoxanthin. Dashed arrows indicate that some synthesis steps were omitted.
Clarifying of the pathway of fucoxanthin synthes is also important for biomolecular experiments and molecular level studies. It is evidant that the enzymes involved in key synthetic pathways are not yet known and fuether research is needed to investigate potential branching pathways for synthesis.
2.1.3 Selection and breeding of fucoxanthin-producing microalgae
In the field of research and development of microalgae resources, one of the key problems that must be solved is the screening and breeding of excellent algal strains. Most of the microalgae cultured in current times are isolated directly from the natural environment. They are wild-type algae, which have single traits and poor adaptability to the environment. As a result, they are prone to trait degradation and are not suitable for industrial production and conditions (Benemann, 2013). Natural selection, is time-consuming and laborious. Hence, there is a need for effective breeding methods. Mutagenic breeding, an ideal method for microalgal breeding, is outstanding and easy to operate. Researchers screened a mutant of Phaeodactylum tricornutum using a fluorescence-based high-throughput method (Yi et al., 2018). The mutant showed a 69.3% increase in fucoxanthin compared to wild type, making it an industrially useful fucoxanthin-producing algal strain. UV mutagenesis is also an effective breeding strategy. Yi et al. used stress mutagenesis induced by UV light to enhance the accumulation of fucoxanthin in Phaeodactylum tricornutum and produced mutant strains with improved growth rates (Yi et al., 2015). Furthermore, mutagenesis has the advantage of not being classified as a method producing genetically modified organisms, which exempts it from regulatory issues in many places such as the EU (Tillich et al., 2012). However, there are curenthly challenges in microalgae mutation breeding. Such as obtaining ideal mutant strains, optimizing mutation conditions, and screening efficiency. It is necessary to adopt appropriate mutation methods for different algal strains. A combination of different mutation methods can also be used by applying higher mutation pressures in order to obtain the desired mutant strain.
2.1.4 Culture of fucoxanthin producing microalgae
Culture parameters for microalgae are typically species-specific, requiring individualized culture processes for each microalgae strain to achieve high-quality algal cultures. While most microalgae cultures adopt a one-stage culture mode. The culture conditions have no change during the whole process from microalgae growth to fucoxanthin accumulation (Heo et al., 2010; Petrushkina et al., 2017; Lu et al., 2018; Wang et al., 2018; Li et al., 2019; Marella and Tiwari, 2020; Mc et al., 2020; Kanamoto et al., 2021; Zhu et al., 2021; Jin et al., 2022), as shown in Supplementary Table 1.
However, the one-stage mode cannot solve the contradiction between biomass and metabolite production accumulation. It cannot fulfill the demands of industrial production. Microalgae metabolites are often produced under extreme conditions that may inhibit their growth. If the conditions for metabolite accumulation are met, it can lead to the death of the algal cells, while the conditions for microalgal growth are not satisfied for pigment accumulation.
In contrast, the two-stage culture mode separates periods of microalgal growth and product accumulation. In Stage I, provided sufficient cell factories are provided, while and Stage II optimizes growth conditions (e.g, autotrophic, heterotrophic, batch sequential, nutrient conditions) to enhance metabolite production (Wan et al., 2015), as shown in (Chang et al., 2018; Chiang et al., 2020; Dambek et al., 2012; Erdogan et al., 2021; Fierli et al., 2022; Gao et al., 2020; Kim et al., 2010b; Lakey-Beitia et al, 2019; Li et al, 2000; Li et al, 2021; Masse et al, 2004; Okcu et al., 2021; Qin et al., 2013; Tachihana et al., 2020; Wei et al., 2022; Wu et al., 2011; Yang and Wei, 2020; Zhu et al., 2021) Table 1. Compared to separate cultivation, the two-stage mode offers advantages such as high product yield, energy saving, environmental protection and a wide range of applications (Liyanaarachchi et al., 2021). Researchers have successfully improved the fucoxanthin contents and blue light amplification yield using two-stage photofermentation techniques. Yang et al. (Yang et al., 2023). raised the fucoxanthin content and yield by 45.98% and 48.3% reperceiving a two-stage culture technique. The highest recorded fucoxanthin yield among available results was obtained by Yang et al., measuring 8.22 mg/d·L (Yang et al., 2020). Li et al. achieved a fucoxanthin yield of 16.5 mg/d·L by employing a two-stage culture technique involves in high-density fermentation in the dark and blue-white light as a light source (Lu et al., 2018). The two stages of cultivation are detailed in Table 1. In conclusion, the two-stage culture method is considered to be a viable approach.
2.2 Physiological activity of fucoxanthin
2.2.1 Anti-inflammatory activity
Similar to other anti-inflammatory drugs, fucoxanthin has been found to play an anti-inflammatory role through the down-regulation of the release of relevant inflammatory mediators. It’s inhibition of the enzymatic activities, which are associated with inflammation induction in macrophages RAW264.7 (Heo et al., 2010; Kim et al., 2010a). Fucoxanthin has demonstrated beneficial effects in various conditions, such as ulcerative colitis (Yang et al., 2020), uveitis (Shiratori et al., 2005), and contact hypersensitivity (Sakai et al., 2011; Namkoong et al., 2012). In a colitis model using mice, fucoxanthin inhibited NF-κB and COX-2 expression, resulting in significant improvement of histological damage in the colon (Yang et al., 2020). Fucoxanthin has also been shown efficacy in treating uveitis in an LPS-induced inflammation rat model (Li et al., 2020). Moreover, fucoxanthin has been shown effectively alleviate dinitrofluorobenzene-induced contact hypersensitivity in BALB/c mice (Sakai et al., 2011). And prevent allergic diseases by inhibiting IgE-antigen complex-stimulated RBL-2H3 cells (Namkoong et al., 2012).
In previous studies, mechanistic studies of fucoxanthin have focused on the classical NF-κB and MAPK-related pathways. Fucoxanthin treatment has been shown to dose-dependently reduce the inflammatory factor expression levels of tumor necrosis factor-α (TNF-α), interleukin-1β (IL-1β), and interleukin-6 (IL-6) by inhibiting the MAPK and NF-κB pathways (Heo et al., 2010; Kim et al., 2010a). There are other related signaling pathways, such as the inflammasome, which is composed of NOD-like receptor thermal protein domain associated protein (NLRP), apoptosis-associated speck-like protein (ASC), and cysteinyl aspartate specific protein (caspase). Li et al (Li et al., 2020). found that fucoxanthin attenuated palmitate-induced transcriptional of NLRP3 inflammasomes for anti-inflammatory efficacy. Similarly, the union of fucoxanthin and rosmarinic acid has been shown to reduce the inflammatory response by downregulating inflammasome components including NLRP3, ASC, and caspase-1, as well as interleukin IL-1β production, according to Rodrguez-Luna et al., (Rodríguez-Luna et al., 2019). According to these studies, the product may have a protective impact by downregulating the inflammasome and inflammatory factors.
In this line, due to its anti-inflammatory activity, this carotenoid has been proposed as a protective compound. Given its promising anti-inflammatory effect, fucoxanthin holds potential as an independent anti-inflammatory drug or as a synergizedstic agent with other therapeutic drugs for other diseases to exert anti-inflammatory effect treatment of various diseases.
2.2.2 Antioxidant activity
Fucoxanthin is a very powerful antioxidant. Its six-oxygen-atom propylene structure makes fucoxanthin extremely reactive to free radicals. Oxidative stress is brought on by an increase in reactive oxygen species (ROS). Heo et al., (Heo et al., 2008). induced oxidative stress in Vero cells using H2O2, and the addition of fucoxanthin significantly reduced intracellular ROS production while also dose-dependently inhibiting oxidative-induced cellular damage. Fucoxanthin may scavenge ROS and free radicals by means of a three-step process that involves electron transfer, dehydrogenation, and addition (Rao and Rao, 2007). Additionally, fucoxanthin has demonstrated its efficacy in modifiedying skin inflammation and skin damage caused by UV-induced photo-oxidative stress (Ichihashi et al., 2003; D’Orazio et al., 2013). When fucoxanthin isolated from algae was incorporated into sunscreen at 0.5% (w/v), it inhibited the formation of ROS significantly in reconstructed skin cells and HaCaT cells (Tavares et al., 2020). Furthermore, intracellular ROS and oxidative stress were lower in cells exposed to UV-B radiation than in cells without different concentrations of fucoxanthin (Heo and Jeon, 2009). Its powerful anti-oxidant qualities protect the skin from oxidative stress-related skin damage and aging.
Other than directly interacting with free radicals, fucoxanthin enhances the synthesis of related proteins and antioxidant enzymes. Resulting in increased plasma antioxidant enzyme activity, total antioxidant capacity, and mRNA levels of Nrf2 (Liu et al., 2011; Ha et al., 2013). It also promotes the synthesis of antioxidant proteins such as heme oxygenase-1 (HO-1) and (NAD(P)H): quinone oxidoreductase 1 (NQO-1) through activation of the Nrf2/ARE system, thereby exerting its antioxidant activity in BNL CL2 cells from murine hepatic (Liu et al., 2011). Similarly, fucoxanthin activates the Nrf2 pathway and the gene NQO1, which reduced oxidative stress under high-fat diet feeding1 (Ha et al., 2013). Moreover, fucoxanthin has been found to alleviate oxidative stress by retinol deficiency in rats by modifying the Na(+)-K(+)-ATPase, catalase (CAT) and glutathione S-transferase (GST) activities (Ravi Kumar et al., 2008).
Collectively, these findings highlight the potent antioxidant activity of fucoxanthin. Specifically, fucoxanthin, a microalgal product, is a natural green substance. It is expected to become a valuable antioxidant in the food, pharmaceutical, and cosmetics industries in the future.
2.3 Pharmacological activity of fucoxanthin
2.3.1 Antitumor activity
Fucoxanthin can exhibit direct antitumor effects or work in conjunction with antitumor drugs to enhance their efficacy. Experimental studies have demonstrated that fucoxanthin impacts various types of cancer by promoting apoptosis (Jin et al., 2018; Yu et al., 2018). Yu et al., (Yu et al., 2018). suggested that fucoxanthin may decrease proliferation and induce apoptosis through the JAK/STAT signaling pathway, leading to cell arrest at the S phase in gastric cancer cells (SGC-7901) and at the G2/M phase in gastric cells (BGC-823). It also inhibits proliferation of cancer cells and blocking the cell cycle (Das et al., 2008) (Das et al., 2008; Hou et al., 2013; Liu et al., 2013; Long et al., 2020). Liu et al (Liu et al., 2013). found that fucoxanthin pretreatment increased the chemotherapeutic efficacy of cisplatin on human hepatoma HepG2 cells by potentially altering the DNA repair system associated with ERK, p38, and PI3K/AKT pathways, which subsequently increased cisplatin-induced apoptosis in cancer cells. Das conducted surveys showing that fucoxanthin-rich methanolic extract reduced the viability of HepG2 cells and induced G0/G1 cell cycle arrest through proteasomal degradation and inhibition of cell cycle protein D synthesis (Das et al., 2008). The effects of fucoxanthin on antitumor activity are illustrated in Figure 4.
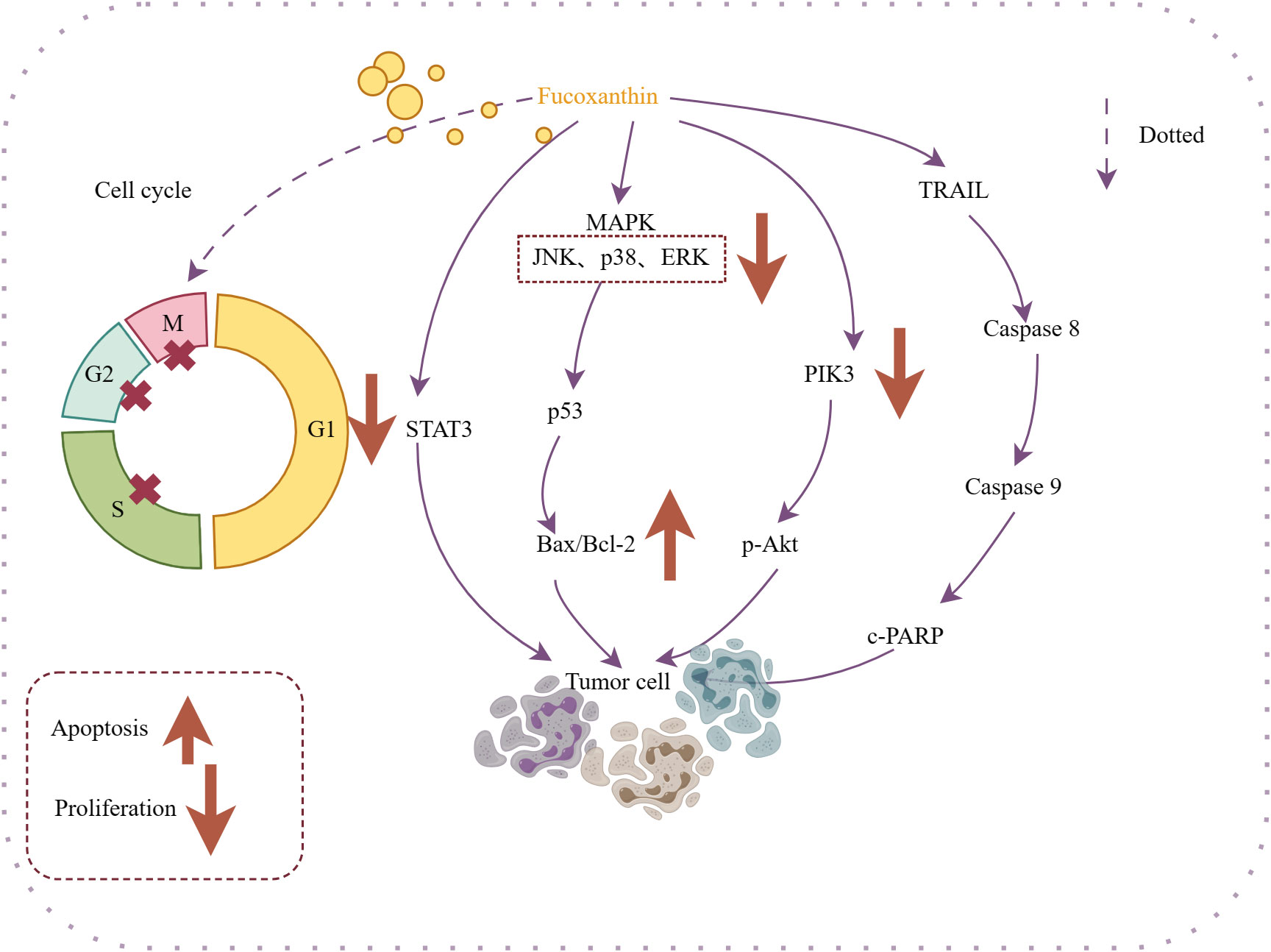
Figure 4 Mechanism of action of antitumor activity of fucoxanthin. Fucoxanthin causes cell cycle arrest in M, G2, and S cancer cells. Fucoxanthin promotes cancer cell apoptosis through different mechanisms: inhibiting STAT-3 expression, regulating the Bax/Bcl-2 ratio, targeting the PI3K/Akt/NF-κB signaling pathway to inhibit p-Akt expression, and energizing with TRAIL. Downward arrows indicate downregulation, and vice versa for upregulation. Dotted lines indicate omitted steps.
Therefore, it has a therapeutic effect on cancers. Fucoxanthin has shown potential in treating nasopharyngeal cancer (Long et al., 2020), cervical cancer, cervical cancer (Hou et al., 2013; Jin et al., 2018), lung cancer (Moreau et al., 2006), breast cancer (Wang et al., 2019) and other cancers to promote apoptosis and inhibit of tumor cells proliferation. In addition to its direct pharmacological effects, the anti-inflammatory and antioxidant properties of fucoxanthin contribute to its anti-tumor activity. Shin et al., (Shin et al., 2020). revealed that fucoxanthin may improve the efficacy of targeted anticancer drug therapy by reduce oxidative stress on tumor cells, while increasing oxidative stress on tumor cells.
Therefore, fucoxanthin can be used in anti-cancer treatment and in combination with anti-cancer drugs to assist anti-cancer treatment. At the same time, it can be used in combination with some anti-cancer drugs to improve the targeting of tumor cells and prevent damage to healthy cells.
2.3.2 Antimetabolic syndrome
2.3.2.1 Anti-obesitys
Studies on the anti-metabolic disease activity of fucoxanthin have focused on the anti-obesity direction. Fucoxanthin exerts its anti-obesitys effect by affecting white adipose mitochondrial uncoupling protein1 (UCP1) (Maeda et al., 2007; Kang et al., 2011; Gammone and D’Orazio, 2015), suppressed lipid accumulation (Marchesini et al., 2003; Woo et al., 2010; Takatani et al., 2020) and adipocyte differentiation (Kang et al., 2011).
In the clinical experimental group, overweight male and female Japanese adults were administered capsules containing fucoxanthin or placebo capsules for 4 weeks (Hitoe and Shimoda, 2017). The study showed a significant reduction in relative body weight and BMI with no observed abnormalities (Hitoe and Shimoda, 2017). Another clinical trial revealed an increase in resting energy expenditure (REE), in obese patients who were supplemented with 4 mg of fucoxanthin per day (Abidov et al., 2010). It was observed that 8 mg fucoxanthin demonstrated an even higher REE expenditure, suggesting that its efficacy may be dose-dependent (Abidov et al., 2010).
Being overweight has become a general problem for the world’s citizens and can lead to other diseases. The current results of clinical trials show that the administration of fucoxanthin can help mildly obese adults regain their normal weight. These facts highlight the potential of fucoxanthin as a valuable natural product with health-promoting and anti-obesity properties.
2.3.2.2 Anti-nonalcoholic steatohepatitis
Nonalcoholic fatty liver disease (NAFLD) is a signiciant cause of chronic liver disease, and fucoxanthin has been provern to effective in alleviate NAFLD and preventing its progression to hepatic fibrosis.
Firstly, NAFLD is closely associated with obesity (Marchesini et al., 2003). Thus, fucoxanthin may exert anti-NAFLD effects by preventing hepatic lipid accumulation. Fucoxanthin has dual roles in preventing hepatic lipid accumulation: maintaining mitochondrial homeostasis, reducing lipid synthesis, and accelerating lipid degradation. Ye et al (Ye et al., 2022). demonstrated that upregulation of Sirt1/AMPK expression by fucoxanthin accelerated fatty acid β-oxidation. Fucoxanthin supplementation decreases the activities of lipid synthesis-related enzymes [e.g., hydroxymethylglutaryl-coenzyme A reductase (HMG-CoA)] and modulates hepatic antioxidant activity and insulin sensitivity to achieve hepatic lipid metabolism in mice (Kang et al., 2010). Additionally, the anti-inflammatory and antioxidant activity of fucoxanthin can help alleviate free fatty acid-induced liver inflammation, stopping the progression of NAFLD. Activation of the Nrf2 antioxidant signaling pathway by fucoxanthin inhibited the TLR4-mediated inflammatory pathway and the production of inflammatory factors IL-6, IL-8, and TNF-α (Ye et al., 2022).
Furthermore, fucoxanthin prevents the progression of hepatitis to liver fibrosis. Takatani et al., (Takatani et al., 2020). revealed that dietary fucoxanthin also prevents the early phase of fibrosis in NAFLD model. Hepatic Stellate Cells (HSC) are considered as the primariy effector cells of liver fibrosis and are susceptible to activation by inflammation or oxidative stress. Fucoxanthin downregulates the activation markers of HSC, including α-smooth muscle actin (α-SMA). Transforming growth factor β1 (TGFβ1), fibrillar collagen 1 (Col1a1), matrix metalloproteinases-1 (Timp1) expression, and other fibrogenic factors, thus inhibiting hepatic fibrosis in mice (Takatani et al., 2020). Kim et al., (Kim et al., 2019). suggested that fucoxanthin exerts an anti-fibrogenic effect in HSCs. It primarily prevents pro-fibrogenic gene expression by inhibiting the activation of SMAD3 and quiescent HSCs (Kim et al., 2019).
In this context, fucoxanthin could serve as a natural product to protect liver health and shows great potential for the treatment of liver fibrosis and NAFLD treatments. It is important to know that there is no drug for NAFLD at the moment. This activity of fucoxanthin definitely brings a viable approach to the treatment of fatty liver and subsequent liver fibrosis. The effects of fucoxanthin on anti-nonalcoholic steatohepatitis are illustrated in Figure 5.
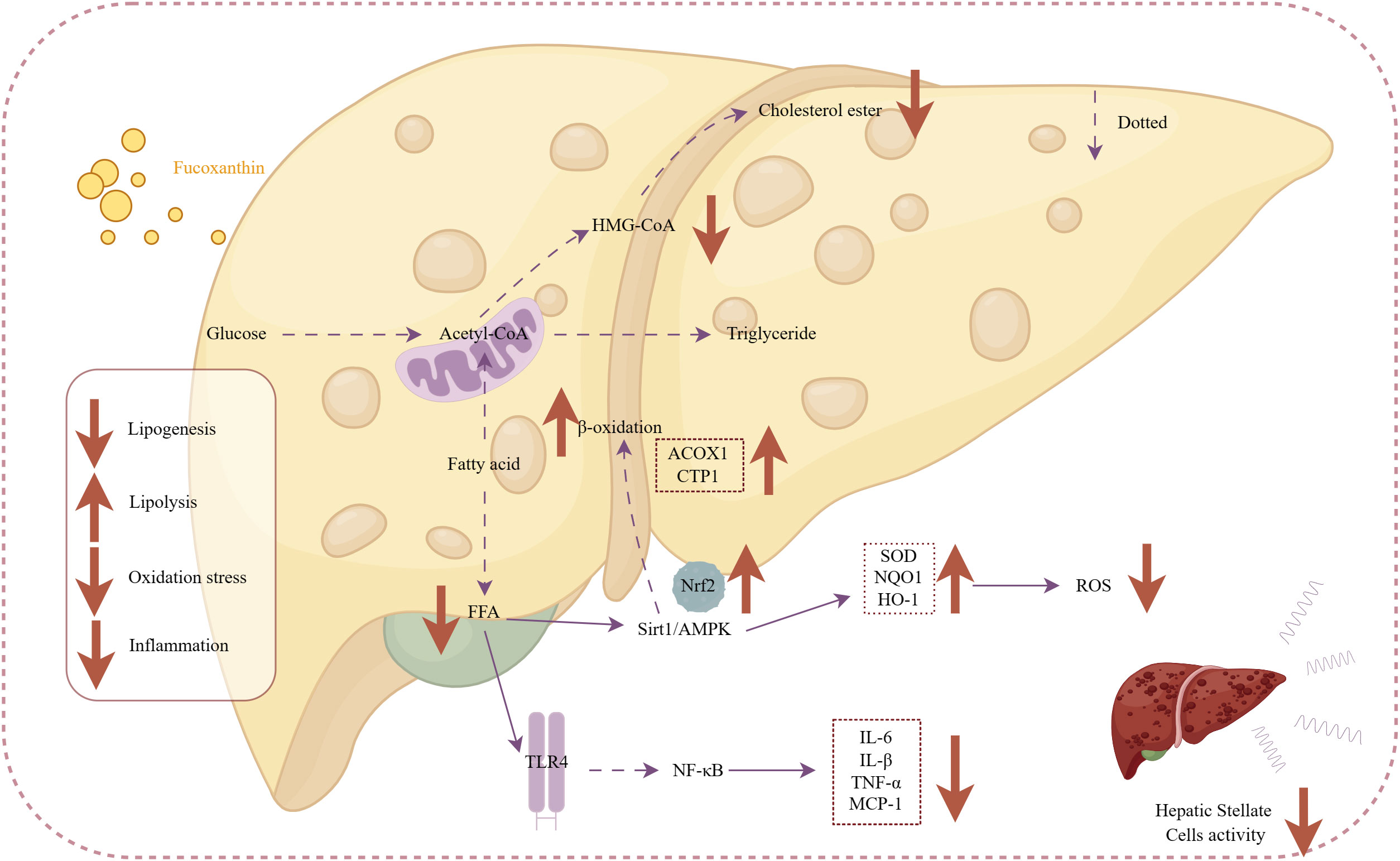
Figure 5 Mechanism of action of fucoxanthin in the treatment of nonalcoholic steatohepatitis. Fucoxanthin reduces HMG-CoA activity and inhibits cholesterol synthesis. Upregulation of Sirt1/AMPK expression promotes free fatty acid β-oxidation increasing expression of ACOX1-acyl-CoA oxidase 1 (ACOX1) gene, carnitine palmitoyltransferase 1 (CTP1). Activating the Nrf2 antioxidant signaling pathway increases antioxidant enzyme activity [superoxide dismutase (SOD), NAD(P)H: quinone acceptor oxidoreductase 1, and heme Heme oxygenase 1 (HO-1)], followed by a decrease in ROS pro-oxidative mediators. Inhibition of the TLR4-mediated inflammatory pathway and production of inflammatory mediators, such as IL-6 and IL-8, prevent the activation of hepatic stellate cells. Downward arrows indicate downregulation, and vice versa for upregulation. Dashed lines indicate omitted steps.
2.3.2.3 Antidiabetic
Studies have shown that fucoxanthin has excellent pharmacological effects on diabetes treatment, as it is able to lower blood glucose levels through multiple pathways.
Firstly, fucoxanthin has been found to have a strong effect on suppressor of cytokine signaling (SOCS-3) (Sakai et al., 2011), Monocyte Chemotactic Protein-1 (MCP-1) (Maeda et al., 2009) plasminogen activator inhibitor-1 (PAI-1) (Hosokawa et al., 2010) to alleviate insulin resistance in an in diabetes experiment models. While inflammation also affects the level of insulin release in adipocytes and hepatocytes (Ye, 2013). Due to the anti-inflammatory activity of fucoxanthin, it is possible to alleviate inflammation-induced insulin resistance and thus restore glucose consumption.
Other factors, leptin also affects insulin sensitivity. In an in vivo experiment, hyperglycamia and low plasma insulin concentrations were alleviated by fucoxanthin in KK-Ay mice, and TNF-α and leptin mRNA were also down-regulated (Maeda et al., 2007).
In addition, fucoxanthin also lowers blood glucose by affecting glucose uptake in normal tissues. Maeda et al., (Maeda et al., 2009). found that fucoxanthin promoted mRNA expression of β3-adrenergic receptor (Adrb3) and glucose transporter 4 (GLUT4) mRNA in skeletal muscle tissues to promote muscle glucose uptake in mice. Diets that contain fucoxanthin may help treat insulin resistance as well as alterations in lipid metabolism. Kang et al., (Kang et al., 2011). found that fucoxanthin differing effects on 3T3-L1 cells according to differentiation stage and inhibits glucose uptake in mature adipocytes by reducing the phosphorylation of insulin receptor substrate 1 (IRS-1). In diabetic/obese KK-Ay mice, fucoxanthin induced GLUT4 expression in flounder and toe extensor muscles and ameliorated symptoms of hyperglycemia by activating insulin expression for lowering blood sugar (Nishikawa et al., 2012).
Aside from antidiabetic, fucoxanthin also acts on the complications of diabetes. Chiang et al., (Kong et al., 2019). demonstrated that fucoxanthin was effective in improving diabetic retinopathy. While fucoxanthin has also shown promise in treating therapeutic effects on diabetes-induced male dysfunction (Sakai et al., 2011).
The most well-known pharmacological action of fucoxanthin is its antidiabetic efficacy, which has been demonstrated in numerous research. Fucoxanthin may provide therapeutic help to diabetics as well as treatment for the symptoms of diabetic complications.
2.3.3 Anti-Alzheimer’s
Alzheimer disease (AD) is a devastating neurodegenerative disorder (Shih et al., 2021). Fucoxanthin has shown potential neuroprotective effects against these diseases.
There is often associated with the binding of amyloidogenic fibers (e.g., Aβ, tau proteins) in Alzheimer’s disease. Drug design analysis and molecular docking simulations conducted by Lakey-Beitia et al., (Shih et al., 2021). revealed the action of carotenoids on Aβ amyloidogenic fibers. They found that carotenoids can bind to Aβ through hydrogen bonding and van der Waals interactions. These findings are consistent with the findings ofstudies by Xiang et al., (Xiang et al., 2017). and Yang et al., (Yang et al., 2021),which also showed that fucoxanthin inhibits Aβ assembly.
Additionally, Aβ deposition furthermore results in many issues that induce oxidative stress and neuronal cell death. In the mouse hippocampal regions, fucoxanthin has been shown to reduce oxidative stress in neuronal cells, increase brain-derived neurotrophic factor expression, and expand ChAT-positive areas (Xiang et al., 2017). This helps to maintain neuronal cell viability (Xiang et al., 2017). Fucoxanthin also prevented neuronal apoptosis and intracellular reactive oxygen stress by Aβ oligomer through activating the PI3K/Akt cascade and inhibiting the ERK pathway in SH-SY5Y cells (Lin et al., 2017). Zhu et al., (Zhu et al., 2021). in vitro research showed that 5-10 μM fucoxanthin reduced the activity of the senescence marker SA-β-galactosidase. Another in vitro study demonstrated that fucoxanthin modulated antioxidant enzymes such as superoxide dismutase (SOD) to exert antioxidant effects on neuronal cells, inhibited Aβ1-42 induced apoptosis (Zhu et al., 2021).
The available evidence supports the neuroprotective effects of fucoxanthin and highlights the potential marine-derived carotenoids as novel strategies for preventing or treating dementia. Figure 6 illustrates the mechanism of its anti-Alzheimer activity.
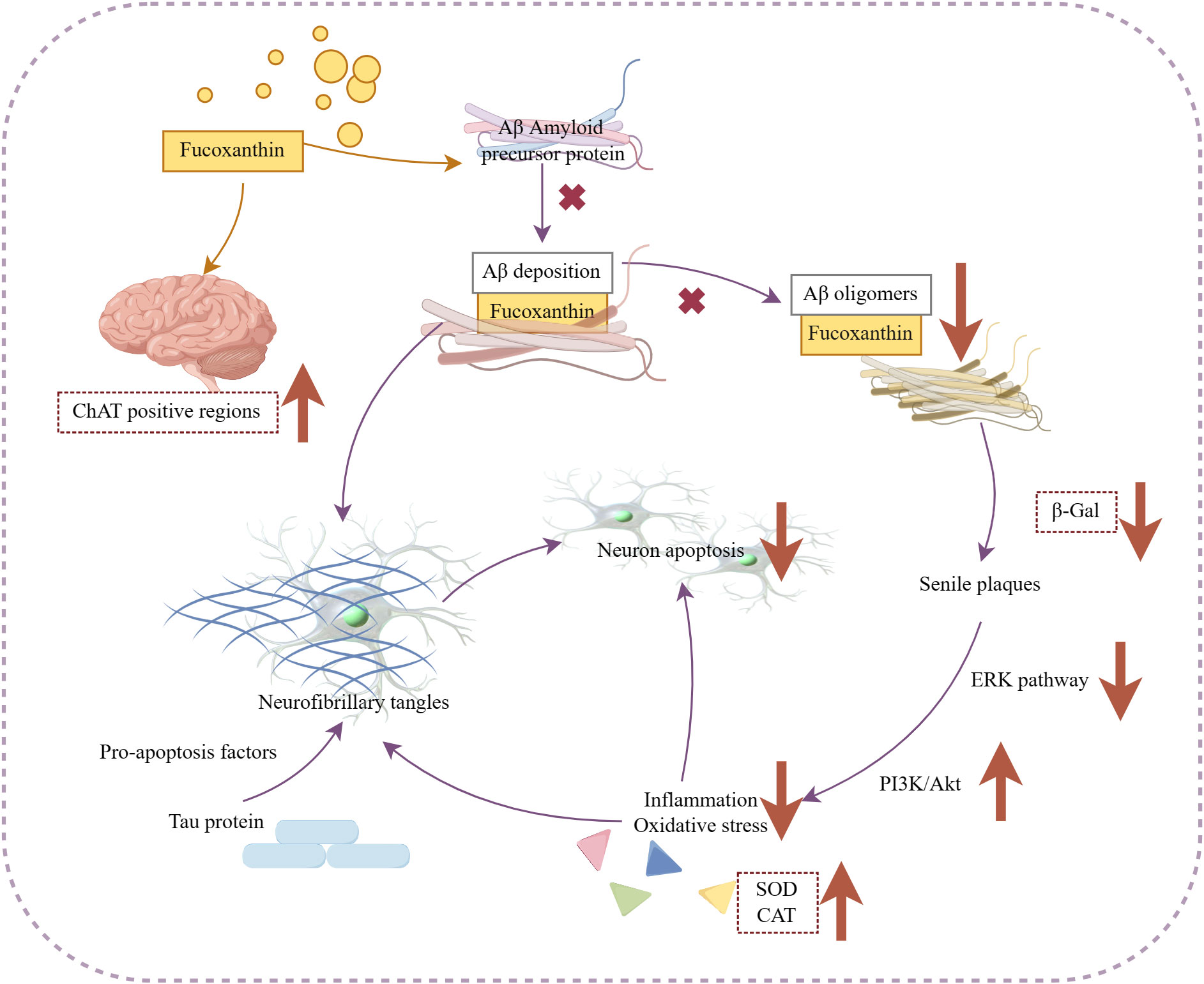
Figure 6 Mechanism of fucoxanthin in the treatment of Alzheimer’s disease. Fucoxanthin blocked Aβ assembly preventing Aβ deposition and inhibited the production of Aβ oligomers, which also caused the inhibition of β-Galactosidase (β-Gal) activity, a marker of cellular senescence. Fucoxanthin reduced Aβ deposition-induced neuronal cytotoxicity and inhibited neuronal apoptosis by activating the PI3K/Akt pathway, inhibiting the ERK pathway, and modulating antioxidant enzymes, such as SOD and catalase (CAT), to reduce inflammation oxidative stress. In addition, fucoxanthin enhanced the expression of brain-derived neurotrophic factors and increased the positive region of choline acetyltransferase (ChAT). Downward arrows indicate downregulation, and vice versa for upregulation.
2.4 Other active
2.4.1 Antimicrobial
Fucoxanthin can be a natural antimicrobial agent. Although it has antibacterial activity against both Gram-positive and negative bacteria, it does not show activity against strict anaerobic bacteria (Karpiński and Adamczak, 2019). Liu Z et al., (Liu et al., 2019). reported the antimicrobial properties of fucoxanthin against human pathogens (Streptococcus agalactiae, Staphylococcus epidermidis, and Staphylococcus aureus), indicating that fucoxanthin effectively inhibits their growth. Peraman M et al (Peraman and Nachimuthu, 2019). screened methanolic extracts of marine microalgae fucoxanthin for antimicrobial activity. Among them, both the extract of Dunaliella salina and Thalassiosira extract showed better antibacterial activity against bacteria and fungi (minimum inhibitory concentration MIC: 40 mg/mL), whereas the bacterial species acted were different. Transmission electron microscopy was applied for determining the morphological changes in bacterial after fucoxanthin treatment (El Shafay et al., 2016). Perforation of cell wall, leakage of cytoplasmic contents, severe distortion of outer cell shape, inner chromatin mild scattered cytoplasmic vacuolation, rupture of cell wall, and decreased cell size for bacteria treated with fucoxanthin-containing seaweed extracts (El Shafay et al., 2016). And carotenoids induce the accumulation of a lysozyme that can digest bacterial cell walls. The antimicrobial mechanism of fucoxanthin may be associated with it (Abu-Ghannam and Rajauria, 2013).
Based on these findings, fucoxanthin is expected to contribute to addressing the issue of bacterial drug resistance.
2.4.2 Antiviral
In terms of antiviral activity, fucoxanthin has been found to inhibit the activation of Epstein-Barr Virus at lower concentrations (Tsushima et al., 1995). Additionally, there is a hypothesis that the consumption of fucoxanthin-containing dietary seaweeds may provide resistance against COVID-19 damage through various mechanisms, but further investigation is needed to understand its pharmacological activity and mechanism (Tamama, 2021).
2.4.3 Anti-angiogenic
During the process of angiogenesis, the vascular endothelium releases proteases and migrates through the extracellular matrix in order to proliferate and differentiate into new blood vessels. Ponesakki G et al (Ganesan et al., 2013). conducted a study using human umbilical vein endothelial cells (HUVEC) to clarify the molecular mechanisms of fucoxanthin, an antivascular compound. Their findings revealed that fucoxanthin inhibits FGF-2-mediated intracellular signaling proteins, thereby suppressing the migration of endothelial cells. Their differentiation into tubular structures was also inhibited. In in vitro angiogenesis assays, fucoxanthin in doses more than 10 μM demonstrated significant suppression of HUVEC tube formation and proliferation (Sugawara et al., 2006). Moreover, in an ex vivo angiogenesis assay, fucoxanthin exhibited a dose-dependent suppression of microvessel outgrowth (Sugawara et al., 2006).
Angiogenesis is crucial for both the healing of wounds and the advancement of illnesses like rheumatoid arthritis and cancer. Many pathological conditions, such as tumors, atherosclerosis, and SARS-CoV-2, are known to be associated with angiogenesis (Viallard and Larrivée, 2017). Studies indicate that fucoxanthin is a potentially useful product that can be used to treat angiogenesis-related illnesses in a secure and efficient manner.
2.4.4 Osteoprotective
Osteoclasts and osteoblasts work in tandem to regulate the process of bone development and formation. Treatment with 2.5 μM fucoxanthin inhibited osteoclast RAW264.7 differentiation and induced osteoclast apoptosis to inhibit osteoclast formation. Notably, fucoxanthin induced apoptosis effect on osteoclasts was stronger compared to osteoblasts (Das et al., 2010). You-Jung et al (Ha et al., 2021). investigated the effects of fucoxanthin on osteoblast differentiation and its regulatory pathways. Their results demonstrated that fucoxanthin altered the expression of mitogen-activated protein kinase and Nrf2, leading to a significant inhibition of osteoclast differentiation and bone resorption.
Therefore, fucoxanthin potentially holds promise as a therapeutic agent for skeletal diseases, for example, rheumatoid arthritis and osteoporosis.
3 Methods
The scientific literature was primarily searched from two databases: Web of Science and PubMed. The search words are not limited to “fucoxanthin” AND “fucoxanthin anti-” OR “microalage”. If the article contains relevant citations of interest, the paper will also be included in the section to be searched. The obtained material was then screened. Articles that were inaccessible, irrelevant to the review’s direction, or duplicates.
To determine whether to include a review, the abstract and discussion sections of the article were read. Additionally, the full text was examined to extract information about microalgae species, culture process parameters, and models used in cellular experiments for subsequent tabulation.
4 Summary and outlook
Fucoxanthin has demonstrated its value in various applications due to its ability to bind to a wider range of targets, thanks to the presence of multiple functional groups. Its anti-inflammatory activity and antioxidant properties make it effective in alleviating diseases associated with inflammation and oxidative stress, such as skin inflammation, ulcerative colitis, and contact hypersensitivity. Additionally, fucoxanthin has been a subject of research interest for its potential anti-diabetic and anti-obesity effects, as well as its proven biological activities in non-alcoholic steatohepatitis and Alzheimer’s disease. The discovery of fucoxanthin-rich microalgae has opened up possibilities for industrial production. Furthermore, natural products are generally more preferable to chemically synthesized drugs.
However, the industrial production of fucoxanthin is still a distant goal, primarily due to the challenge of obtaining superior algal strains. Gene editing can be used for breeding, but the synthetic pathway needs to be clarified first. Currently, new physical and chemical mutagenesis technologies provide a convenient method for breeding without molecular alteration, although issues related to excessive unpredictability and poorly directed mutation must be considered. In terms of culture techniques, microalgae culture still relies on traditional cultivation methods. The optimization of cultivation processes can accelerate the commercial development of fucoxanthin, but researchers also need to address the challenges of transitioning from small trials to factory production.
Considerable advancements have been achieved in the study of the biological properties and activity of fucoxanthin. Notably, fucoxanthin extracts have shown no indications of drug toxicity in both in vivo and ex vivo experiments. However, the specific pharmacological targets and signaling mechanisms of fucoxanthin are still not fully understood and require further investigation. Additionally, it is crucial to ensure the safety and effectiveness of high-purity fucoxanthin obtained through biorefining methods for its incorporation in pharmaceuticals, food, and cosmetics.
Author contributions
BG: Writing – original draft, Writing – review & editing. SM: Project administration, Writing – review & editing. YY: Project administration, Writing – review & editing. ZW: Writing – review & editing.
Funding
The author(s) declare financial support was received for the research, authorship, and/or publication of this article. This work were supported by “Scientific Research Foundation of Third Institute of Oceanography, Ministry of Natural Resources, grant number 2019023”, “Key Technological Innovation and Industrialization Projects of Fujian Province, grant number 2023G025”.
Conflict of interest
The authors declare that the research was conducted in the absence of any commercial or financial relationships that could be construed as a potential conflict of interest.
Publisher’s note
All claims expressed in this article are solely those of the authors and do not necessarily represent those of their affiliated organizations, or those of the publisher, the editors and the reviewers. Any product that may be evaluated in this article, or claim that may be made by its manufacturer, is not guaranteed or endorsed by the publisher.
Supplementary material
The Supplementary Material for this article can be found online at: https://www.frontiersin.org/articles/10.3389/fmars.2024.1357425/full#supplementary-material
References
Abidov M., Ramazanov Z., Seifulla R., Grachev S. (2010). The effects of Xanthigen™ in the weight management of obese premenopausal women with non-alcoholic fatty liver disease and normal liver fat. Diabetes Obes. Metab. 12, 72–81. doi: 10.1111/j.1463-1326.2009.01132.x
Abu-Ghannam N., Rajauria G. (2013). “Antimicrobial activity of compounds isolated from algae,” in Functional ingredients from algae for foods and nutraceuticals (Cambridge, UK: Woodhead Publishing), 287–306. doi: 10.1533/9780857098689.2.287.
Abu-Ghosh S., Dubinsky Z., Verdelho V., Iluz D. (2021). Unconventional high-value products from microalgae: A review. Bioresource Technol. 329, 124895. doi: 10.1016/j.biortech.2021.124895
Alghazwi M., Smid S., Musgrave I., Zhang W. (2019). In vitro studies of the neuroprotective activities of astaxanthin and fucoxanthin against amyloid beta (Aβ1-42) toxicity and aggregation. Neurochem Int. 124, 215–224. doi: 10.1016/j.neuint.2019.01.010
Benemann J. (2013). Microalgae for biofuels and animal feeds. Energies 6, 5869–5886. doi: 10.3390/en6115869
Chang Y. H., Chen Y. L., Huang W. C., Liou C. J. (2018). Fucoxanthin attenuates fatty acid-induced lipid accumulation in FL83B hepatocytes through regulated Sirt1/AMPK signaling pathway. Biochem. Biophys. Res. Commun. 495, 197–203. doi: 10.1016/j.bbrc.2017.11.022
Chiang Y.-F., Chen H.-Y., Chang Y.-J., Shih Y.-H., Shieh T.-M., Wang K.-L., et al. (2020). Protective effects of fucoxanthin on high glucose- and 4-hydroxynonenal (4-HNE)-induced injury in human retinal pigment epithelial cells. Antioxidants 9, 1176. doi: 10.3390/antiox9121176
Coesel S., Oborník M., Varela J., Falciatore A., Bowler C. (2008). Evolutionary origins and functions of the carotenoid biosynthetic pathway in marine diatoms. PloS One 3, e2896. doi: 10.1371/journal.pone.0002896
Dambek M., Eilers U., Breitenbach J., Steiger S., Büchel C., Sandmann G. (2012). Biosynthesis of fucoxanthin and diadinoxanthin and function of initial pathway genes in Phaeodactylum tricornutum. J. Exp. Bot. 63, 5607–5612. doi: 10.1093/jxb/ers211
Das S. K., Hashimoto T., Kanazawa K. (2008). Growth inhibition of human hepatic carcinoma HepG2 cells by fucoxanthin is associated with down-regulation of cyclin D. Biochim. Biophys. Acta 1780, 743–749. doi: 10.1016/j.bbagen.2008.01.003
Das S. K., Ren R., Hashimoto T., Kanazawa K. (2010). Fucoxanthin induces apoptosis in osteoclast-like cells differentiated from RAW264.7 cells. J. Agric. Food Chem. 58, 6090–6095. doi: 10.1021/jf100303k
D’Orazio J., Jarrett S., Amaro-Ortiz A., Scott T. (2013). UV radiation and the skin. Int. J. Mol. Sci. 14, 12222–12248. doi: 10.3390/ijms140612222
El Shafay S. M., Ali S. S., El-Sheekh M. M. (2016). Antimicrobial activity of some seaweeds species from Red sea, against multidrug resistant bacteria. Egyptian J. Aquat. Res. 42, 65–74. doi: 10.1016/j.ejar.2015.11.006
Erdoğan A., Karataş A. B., Demirel Z., Dalay M. C. (2021). Purification of fucoxanthin from the diatom Amphora capitellata by preparative chromatography after its enhanced productivity via oxidative stress. J. Appl. Phycol 34, 301–309. doi: 10.1007/s10811-021-02625-7
Fierli D., Aranyos A., Barone M. E., Parkes R., Touzet N. (2022). Influence of exogenous phytohormone supplementation on the pigment and fatty acid content of three marine diatoms. Appl. Microbiol. Biotechnol. 106, 6195–6207. doi: 10.1007/s00253-022-12140-5
Gammone M. A., D’Orazio N. (2015). Anti-obesity activity of the marine carotenoid fucoxanthin. Mar. Drugs 13, 2196–2214. doi: 10.3390/md13042196
Ganesan P., Matsubara K., Sugawara T., Hirata T. (2013). Marine algal carotenoids inhibit angiogenesis by down-regulating FGF-2-mediated intracellular signals in vascular endothelial cells. Mol. Cell. Biochem. 380, 1–9. doi: 10.1007/s11010-013-1651-5
Gao F., Teles I., Wijffels R. H., Barbosa M. J. (2020). Process optimization of fucoxanthin production with Tisochrysis lutea. Bioresource Technol. 315, 123894. doi: 10.1016/j.biortech.2020.123894
Gille A., Stojnic B., Derwenskus F., Trautmann A., Schmid-Staiger U., Posten C., et al. (2019). A lipophilic fucoxanthin-rich phaeodactylum tricornutum extract ameliorates effects of diet-induced obesity in C57BL/6J mice. Nutrients 11, 796. doi: 10.3390/nu11040796
Ha Y. J., Choi Y. S., Oh Y. R., Kang E. H., Khang G., Park Y. B., et al. (2021). Fucoxanthin suppresses osteoclastogenesis via modulation of MAP kinase and nrf2 signaling. Mar. Drugs 19, 132. doi: 10.3390/md19030132
Ha A. W., Na S. J., Kim W. K. (2013). Antioxidant effects of fucoxanthin rich powder in rats fed with high fat diet. Nutr. Res. Pract. 7, 475–480. doi: 10.4162/nrp.2013.7.6.475
Heo S. J., Jeon Y. J. (2009). Protective effect of fucoxanthin isolated from Sargassum siliquastrum on UV-B induced cell damage. J. Photochem. Photobiol B Biol. 95, 101–107. doi: 10.1016/j.jphotobiol.2008.11.011
Heo S. J., Ko S. C., Kang S. M., Kang H. S., Kim J. P., Kim S. H., et al. (2008). Cytoprotective effect of fucoxanthin isolated from brown algae Sargassum siliquastrum against H 2 O 2-induced cell damage. Eur. Food Res. Technol. 228, 145–151. doi: 10.1007/s00217-008-0918-7
Heo S. J., Yoon W. J., Kim K. N., Ahn G. N., Kang S. M., Kang D. H., et al. (2010). Evaluation of anti-inflammatory effect of fucoxanthin isolated from brown algae in lipopolysaccharide-stimulated RAW 264.7 macrophages. Food Chem. Toxicol. 48, 2045–2051. doi: 10.1016/j.fct.2010.05.003
Hitoe S., Shimoda H. (2017). Seaweed fucoxanthin supplementation improves obesity parameters in mild obese Japanese subjects. Funct. Foods Health Dis. 7, 246–262. doi: 10.31989/2160-3855
Hosokawa M., Miyashita T., Nishikawa S., Emi S., Tsukui T., Beppu F., et al. (2010). Fucoxanthin regulates adipocytokine mRNA expression in white adipose tissue of diabetic/obese KK-Ay mice. Arch. Biochem. Biophysics 504, 17–25. doi: 10.1016/j.abb.2010.05.031
Hou L. L., Gao C., Chen L., Hu G. Q., Xie S. Q. (2013). Essential role of autophagy in fucoxanthin-induced cytotoxicity to human epithelial cervical cancer HeLa cells. Acta Pharmacol Sin. 34, 1403–1410. doi: 10.1038/aps.2013.90
Hu X., Li Y., Li C., Fu Y., Cai F., Chen Q., et al. (2012). Combination of fucoxanthin and conjugated linoleic acid attenuates body weight gain and improves lipid metabolism in high-fat diet-induced obese rats. Arch. Biochem. Biophysics 519, 59–65. doi: 10.1016/j.abb.2012.01.011
Ichihashi M., Ueda M., Budiyanto A., Bito T., Oka M., Fukunaga M., et al. (2003). UV-induced skin damage. Toxicology 189, 21–39. doi: 10.1016/S0300-483X(03)00150-1
Jin H., Guo Y., Li Y., Chen B., Ma H., Wang H., et al. (2022). Effective fucoxanthin production in the flagellate alga Poterioochromonas malhamensis by coupling heterotrophic high-cell-density fermentation with illumination. Front. Bioengineering Biotechnol. 10. doi: 10.3389/fbioe.2022.1074850
Jin Y., Qiu S., Shao N., Zheng J. (2018). Fucoxanthin and tumor necrosis factor-related apoptosis-inducing ligand (TRAIL) synergistically promotes apoptosis of human cervical cancer cells by targeting PI3K/Akt/NF-κB signaling pathway. Med. Sci. Monitor: Int. Med. J. Exp. Clin. Res. 24, 11–18. doi: 10.12659/MSM.905360
Kadono T., Kira N., Suzuki K., Iwata O., Ohama T., Okada S., et al. (2015). Effect of an introduced phytoene synthase gene expression on carotenoid biosynthesis in the marine diatom phaeodactylum tricornutum. Mar. Drugs 13, 5334–5357. doi: 10.3390/md13085334
Kanamoto A., Kato Y., Yoshida E., Hasunuma T., Kondo A. (2021). Development of a method for fucoxanthin production using the Haptophyte marine microalga Pavlova sp. OPMS 30543. Mar. Biotechnol. 23, 331–341. doi: 10.1007/s10126-021-10028-5
Kang S. I., Kim M. H., Shin H. S., Kim H. M., Hong Y. S., Park J. G., et al. (2010). A water-soluble extract of Petalonia binghamiae inhibits the expression of adipogenic regulators in 3T3-L1 preadipocytes and reduces adiposity and weight gain in rats fed a high-fat diet. J. Nutr. Biochem. 21, 1251–1257. doi: 10.1016/j.jnutbio.2009.11.008
Kang S. I., Ko H. C., Shin H. S., Kim H. M., Hong Y. S., Lee N. H., et al. (2011). Fucoxanthin exerts differing effects on 3T3-L1 cells according to differentiation stage and inhibits glucose uptake in mature adipocytes. Biochem. Biophys. Res. Commun. 409, 769–774. doi: 10.1016/j.bbrc.2011.05.086
Karpiński T. M., Adamczak A. (2019). Fucoxanthin-an antibacterial carotenoid. Antioxid (Basel Switzerland) 8, 239. doi: 10.3390/antiox8080239
Kim M. B., Bae M., Hu S., Kang H., Park Y. K., Lee J. Y. (2019). Fucoxanthin exerts anti-fibrogenic effects in hepatic stellate cells. Biochem. Biophys. Res. Commun. 513, 657–662. doi: 10.1016/j.bbrc.2019.04.052
Kim K. N., Heo S. J., Kang S. M., Ahn G., Jeon Y. J. (2010a). Fucoxanthin induces apoptosis in human leukemia HL-60 cells through a ROS-mediated Bcl-xL pathway. Toxicol. Vitro: Int. J. Assoc. BIBRA 24, 1648–1654. doi: 10.1016/j.tiv.2010.05.023
Kim K. N., Heo S. J., Yoon W. J., Kang S. M., Ahn G., Yi T. H., et al. (2010b). Fucoxanthin inhibits the inflammatory response by suppressing the activation of NF-κB and MAPKs in lipopolysaccharide-induced RAW 264.7 macrophages. Eur. J. Pharmacol. 649, 369–375. doi: 10.1016/j.ejphar.2010.09.032
Kim S. M., Shang Y. F., Um B. H. (2011). A preparative method for isolation of fucoxanthin from Eisenia bicyclis by centrifugal partition chromatography. Phytochem Anal. 22, 322–329. doi: 10.1002/pca.1283
Kong Z.-L., Sudirman S., Hsu Y.-C., Su C.-Y., Kuo H.-P. (2019). Fucoxanthin-rich brown algae extract improves male reproductive function on streptozotocin-nicotinamide-induced diabetic rat model. Int. J. Mol. Sci. 20, 4485. doi: 10.3390/ijms20184485
Koo S. Y., Hwang J.-H., Yang S.-H., Um J.-I., Hong K. W., Kang K. (2019). Anti-obesity effect of standardized extract of microalga phaeodactylum tricornutum containing fucoxanthin. Mar. Drugs 17, 311. doi: 10.3390/md17050311
Lakey-Beitia J., Kumar D. J., Hegde M., Rao K. S. (2019). Carotenoids as novel therapeutic molecules against neurodegenerative disorders: chemistry and molecular docking analysis. Int. J. Mol. Sci. 20, 5553. doi: 10.3390/ijms20225553
Li T. L., King J. M., Min D. B. (2000). Quenching mechanisms and kinetics of carotenoids in riboflavin photosensitized singlet oxygen oxidation of vitamin D2. J. Food Biochem. 24, 477–492. doi: 10.1111/j.1745-4514.2000.tb00717.x
Li S., Ren X., Wang Y., Hu J., Wu H., Song S., et al. (2020). Fucoxanthin alleviates palmitate-induced inflammation in RAW 264.7 cells through improving lipid metabolism and attenuating mitochondrial dysfunction. Food Funct. 11, 3361–3370. doi: 10.1039/D0FO00442A.
Li Y., Sun H., Wu T., Fu Y., He Y., Mao X., et al. (2019). Storage carbon metabolism of Isochrysis zhangjiangensis under different light intensities and its application for co-production of fucoxanthin and stearidonic acid. Bioresource Technol. 282, 94–102. doi: 10.1016/j.biortech.2019.02.127
Li S., Zheng X., Fang Q., Gong Y., Wang H. (2021). Exploring the potential of photosynthetic induction factor for the commercial production of fucoxanthin in Phaeodactylum tricornutum. Bioprocess Biosyst. Eng. 44, 1769–1779. doi: 10.1007/s00449-021-02559-x
Lin J., Yu J., Zhao J., Zhang K., Zheng J., Wang J., et al. (2017). Fucoxanthin, a marine carotenoid, attenuates β-amyloid oligomer-induced neurotoxicity possibly via regulating the PI3K/Akt and the ERK pathways in SH-SY5Y cells. Oxid. Med. Cell. Longevity 2017, 6792543. doi: 10.1155/2017/6792543
Liu C. L., Chiu Y. T., Hu M. L. (2011). Fucoxanthin enhances HO-1 and NQO1 expression in murine hepatic BNL CL.2 cells through activation of the Nrf2/ARE system partially by its pro-oxidant activity. J. Agric. Food Chem. 59, 11344–11351. doi: 10.1021/jf2029785
Liu C. L., Lim Y. P., Hu M. L. (2013). Fucoxanthin enhances cisplatin-induced cytotoxicity via NFκB-mediated pathway and downregulates DNA repair gene expression in human hepatoma HepG2 cells. Mar. Drugs 11, 50–66. doi: 10.3390/md11010050
Liu Z., Sun X., Sun X., Wang S., Xu Y. (2019). Fucoxanthin Isolated from Undaria pinnatifida Can Interact with Escherichia coli and lactobacilli in the Intestine and Inhibit the Growth of Pathogenic Bacteria. J. Ocean Univ. China 18, 926–932. doi: 10.1007/s11802-019-4019-y
Liyanaarachchi V. C., Premaratne M., Ariyadasa T. U., Nimarshana P. H. V., Malik A. (2021). Two-stage cultivation of microalgae for production of high-value compounds and biofuels: A review. Algal Res. 57, 102353. doi: 10.1016/j.algal.2021.102353
Lohr M., Wilhelm C. (1999). Algae displaying the diadinoxanthin cycle also possess the violaxanthin cycle. Proc. Natl. Acad. Sci. 96, 8784–8789. doi: 10.1073/pnas.96.15.8784
Long Y., Cao X., Zhao R., Gong S., Jin L., Feng C. (2020). Fucoxanthin treatment inhibits nasopharyngeal carcinoma cell proliferation through induction of autophagy mechanism. Environ. Toxicol. 35, 1082–1090. doi: 10.1002/tox.22944
Lu X., Sun H., Zhao W., Cheng K.-W., Chen F., Liu B. (2018). A hetero-photoautotrophic two-stage cultivation process for production of fucoxanthin by the marine diatom nitzschia laevis. Mar. Drugs 16, 219. doi: 10.3390/md16070219
Maeda H., Hosokawa M., Sashima T., Miyashita K. (2007). Dietary combination of fucoxanthin and fish oil attenuates the weight gain of white adipose tissue and decreases blood glucose in obese/diabetic KK-Ay mice. J. Agric. Food Chem. 55, 7701–7706. doi: 10.1021/jf071569n
Maeda H., Hosokawa M., Sashima T., Murakami-Funayama K., Miyashita K. (2009). Anti-obesity and anti-diabetic effects of fucoxanthin on diet-induced obesity conditions in a murine model. Mol. Med. Rep. 2, 897–902. doi: 10.3892/mmr_00000189
Marchesini G., Bugianesi E., Forlani G., Cerrelli F., Lenzi M., Manini R., et al. (2003). Nonalcoholic fatty liver, steatohepatitis, and the metabolic syndrome. Hepatol. (Baltimore Md.) 37, 917–923. doi: 10.1053/jhep.2003.50161
Marella T. K., Tiwari A. (2020). Marine diatom Thalassiosira weissflogii based biorefinery for co-production of eicosapentaenoic acid and fucoxanthin. Bioresource Technol. 307, 123245. doi: 10.1016/j.biortech.2020.123245
Market Watch. (2020). Available at: https://www.marketwatch.com/press-release/beta-carotene-market-industry-analysis-size-share-growth-trends-and-forecast-2018-2026-influence-of-covid-19-2020-04-29 [Accessed 22 April 2020].
Massé G., Belt S. T., Rowland S. J., Rohmer M. (2004). Isoprenoid biosynthesis in the diatoms Rhizosolenia setigera (Brightwell) and Haslea ostrearia (Simonsen). Proc. Natl. Acad. Sci. 101, 4413–4418. doi: 10.1073/pnas.0400902101
Matsuno T. (2001). Aquatic animal carotenoids. Fisheries Sci. 67, 771–783. doi: 10.1046/j.1444-2906.2001.00323.x
Mc G., Archer L., Fleming G. T., Gillespie E., Touzet N. (2020). The effect of nutrient and phytohormone supplementation on the growth, pigment yields and biochemical composition of newly isolated microalgae. Process Biochem. 92, 61–68. doi: 10.1016/j.procbio.2020.03.001
Moreau D., Tomasoni C., Jacquot C., Kaas R., Le Guedes R., Cadoret J. P., et al. (2006). Cultivated microalgae and the carotenoid fucoxanthin from Odontella aurita as potent anti-proliferative agents in bronchopulmonary and epithelial cell lines. Environ. Toxicol. Pharmacol. 22, 97–103. doi: 10.1016/j.etap.2006.01.004
Mori K., Ooi T., Hiraoka M., Oka N., Hamada H., Tamura M., et al. (2004). Fucoxanthin and its metabolites in edible brown algae cultivated in deep seawater. Mar. Drugs 2, 63–72. doi: 10.3390/md202063
Namkoong S., Joo H. M., Jang S. A., Kim Y. J., Kim T. S., Sohn E. H. (2012). Suppressive effects of fucoxanthin on degranulation in igE-antigen complex-stimulated RBL-2H3 cells. Korean J. Plant Resour. 25, 339–345. doi: 10.7732/kjpr.2012.25.3.339.
Nishikawa S., Hosokawa M., Miyashita K. (2012). Fucoxanthin promotes translocation and induction of glucose transporter 4 in skeletal muscles of diabetic/obese KK-A(y) mice. Phytomed: Int. J. Phytother Phytopharmacol 19, 389–394. doi: 10.1016/j.phymed.2011.11.001
Okcu G. D., Eustance E., Lai Y. S., Rittmann B. E. (2021). Evaluation of co-culturing a diatom and a coccolithophore using different silicate concentrations. Sci. Total Environ. 769, 145217. doi: 10.1016/j.scitotenv.2021.145217
Peraman M., Nachimuthu S. (2019). Identification and quantification of fucoxanthin in selected carotenoid-producing marine microalgae and evaluation for their chemotherapeutic potential. Pharmacognosy Magazine 15, S243–S249. doi: 10.4103/pm.pm_64_19.
Petrushkina M., Gusev E., Sorokin B., Zotko N., Mamaeva A., Filimonova A., et al. (2017). Fucoxanthin production by heterokont microalgae. Algal Res. 24, 387–393. doi: 10.1016/j.algal.2017.03.016
Qin Y., Meng L., Wang F. (2013). Complex enzymatic extraction of kelp fucoxanthin and its antioxidant activity analysis. Food Sci. 16), 279–283. doi: 10.7506/spkx1002-6630-20136057
Rao A. V., Rao L. G. (2007). Carotenoids and human health. Pharmacol. Res. 55, 207–216. doi: 10.1016/j.phrs.2007.01.012
Ravi Kumar S., Narayan B., Vallikannan B. (2008). Fucoxanthin restrains oxidative stress induced by retinol deficiency through modulation of Na(+)K(+)-ATPase [corrected] and antioxidant enzyme activities in rats. Eur. J. Nutr. 47, 432–441. doi: 10.1007/s00394-008-0745-4
Rodríguez-Luna A., Ávila-Román J., González-Rodríguez M., Cózar M., Rabasco A., Motilva V., et al. (2018). Fucoxanthin-containing cream prevents epidermal hyperplasia and UVB-induced skin erythema in mice. Mar. Drugs 16, 378. doi: 10.3390/md16100378
Rodríguez-Luna A., Ávila-Román J., Oliveira H., Motilva V., Talero E. (2019). Fucoxanthin and rosmarinic acid combination has anti-inflammatory effects through regulation of NLRP3 inflammasome in UVB-exposed haCaT keratinocytes. Mar. Drugs 17, 451. doi: 10.3390/md17080451
Sakai S., Sugawara T., Hirata T. (2011). Inhibitory effect of dietary carotenoids on dinitrofluorobenzene-induced contact hypersensitivity in mice. Biosci Biotechnol Biochem. 75, 1013–1015. doi: 10.1271/bbb.110104
Shih P.-H., Shiue S.-J., Chen C.-N., Cheng S.-W., Lin H.-Y., Wu L.-W., et al. (2021). Fucoidan and fucoxanthin attenuate hepatic steatosis and inflammation of NAFLD through modulation of leptin/adiponectin axis. Mar. Drugs 19, 148. doi: 10.3390/md19030148
Shin J., Song M. H., Oh J. W., Keum Y. S., Saini R. K. (2020). Pro-oxidant actions of carotenoids in triggering apoptosis of cancer cells: A review of emerging evidence. Antioxid (Basel Switzerland) 9, 532. doi: 10.3390/antiox9060532
Shiratori K., Ohgami K., Ilieva I., Jin X. H., Koyama Y., Miyashita K., et al. (2005). Effects of fucoxanthin on lipopolysaccharide-induced inflammation in vitro and in vivo. Exp. Eye Res. 81, 422–428. doi: 10.1016/j.exer.2005.03.002
Spagolla Napoleão Tavares R., Stuchi Maria-Engler S., Colepicolo P., Debonsi H. M., Schäfer-Korting M., Marx U., et al. (2020). Skin irritation testing beyond tissue viability: fucoxanthin effects on inflammation, homeostasis, and metabolism. Pharmaceutics 12, 136. doi: 10.3390/pharmaceutics12020136
Sugawara T., Matsubara K., Akagi R., Mori M., Hirata T. (2006). Antiangiogenic activity of brown algae fucoxanthin and its deacetylated product, fucoxanthinol. J. Agric. Food Chem. 54, 9805–9810. doi: 10.1021/jf062204q
Tachihana S., Nagao N., Katayama T., Hirahara M., Yusoff F. M., Banerjee S., et al. (2020). High productivity of eicosapentaenoic acid and fucoxanthin by a marine diatom Chaetoceros gracilis in a semi-continuous culture. Front. Bioengineering Biotechnol. 8. doi: 10.3389/fbioe.2020.602721
Takatani N., Kono Y., Beppu F., Okamatsu-Ogura Y., Yamano Y., Miyashita K., et al. (2020). Fucoxanthin inhibits hepatic oxidative stress, inflammation, and fibrosis in diet-induced nonalcoholic steatohepatitis model mice. Biochem. Biophys. Res. Commun. 528, 305–310. doi: 10.1016/j.bbrc.2020.05.050
Tamama K. (2021). Potential benefits of dietary seaweeds as protection against COVID-19. Nutr. Rev. 79, 814–823. doi: 10.1093/nutrit/nuaa126
Tavares R. S. N., Kawakami C. M., Pereira K. C., do Amaral G. T., Benevenuto C. G., Maria-Engler S. S., et al. (2020). Fucoxanthin for topical administration, a phototoxic vs. Photoprotective potential in a tiered strategy assessed by in vitro methods. Antioxid (Basel Switzerland) 9, 328. doi: 10.3390/antiox9040328
Tillich U. M., Lehmann S., Schulze K., Dühring U., Frohme M. (2012). The optimal mutagen dosage to induce point-mutations in Synechocystis sp. PCC6803 and its application to promote temperature tolerance. PloS One 7, e49467. doi: 10.1371/journal.pone.0049467
Tsushima M., Maoka T., Katsuyama M., Kozuka M., Matsuno T., Tokuda H., et al. (1995). Inhibitory effect of natural carotenoids on Epstein-Barr virus activation activity of a tumor promoter in Raji cells. A screening study for anti-tumor promoters. Biol. Pharm. Bull. 18, 227–233. doi: 10.1248/bpb.18.227
Viallard C., Larrivée B. (2017). Tumor angiogenesis and vascular normalization: alternative therapeutic targets. Angiogenesis 20, 409–426. doi: 10.1007/s10456-017-9562-9
Wan M., Zhang Z., Wang J., Huang J., Fan J., Yu A., et al. (2015). Sequential heterotrophy–dilution–photoinduction cultivation of Haematococcus pluvialis for efficient production of astaxanthin. Bioresource Technol. 198, 557–563. doi: 10.1016/j.biortech.2015.09.031
Wang J., Ma Y., Yang J., Jin L., Gao Z., Xue L., et al. (2019). Fucoxanthin inhibits tumour-related lymphangiogenesis and growth of breast cancer. J. Cell. Mol. Med. 23, 2219–2229. doi: 10.1111/jcmm.14151
Wang S., Verma S. K., Hakeem Said I., Thomsen L., Ullrich M. S., Kuhnert N. (2018). Changes in the fucoxanthin production and protein profiles in Cylindrotheca closterium in response to blue light-emitting diode light. Microbial Cell Factories 17, 1–13. doi: 10.1186/s12934-018-0957-0
Wei F., Gong Y., Zhang L., Chen R., Wang H., Yang B. (2022). Effects of rapamycin on the content of fucoxanthin and the expression of key enzyme genes in the fucoxanthin of Phaeodactylum tricornutum. J. Biol. 03), 72–77. doi: 10.3969/j.issn.2095-1736.2022.03.072
Woo M. N., Jeon S. M., Kim H. J., Lee M. K., Shin S. K., Shin Y. C., et al. (2010). Fucoxanthin supplementation improves plasma and hepatic lipid metabolism and blood glucose concentration in high-fat fed C57BL/6N mice. Chemico-biological Interact. 186, 316–322. doi: 10.1016/j.cbi.2010.05.006
Wu C., Ren D., Chen Q. (2011). Effects of kelp fucoxanthin on the inhibition of lipid peroxidation in mice. J. Dalian Ocean Univ. 5, 428–431. doi: 10.16535/j.cnki.dlhyxb.2011.05.006
Xia S., Wang K., Wan L., Li A., Hu Q., Zhang C. (2013). Production, characterization, and antioxidant activity of fucoxanthin from the marine diatom odontella aurita. Mar. Drugs 11, 2667–2681. doi: 10.3390/md11072667
Xiang S., Liu F., Lin J., Chen H., Huang C., Chen L., et al. (2017). Fucoxanthin inhibits β-amyloid assembly and attenuates β-amyloid oligomer-induced cognitive impairments. J. Agric. Food Chem. 65, 4092–4102. doi: 10.1021/acs.jafc.7b00805
Yang M., Jin L., Wu Z., Xie Y., Zhang P., Wang Q., et al. (2021). PLGA-PEG nanoparticles facilitate in vivo anti-Alzheimer’s effects of fucoxanthin, a marine carotenoid derived from edible brown algae. J. Agric. Food Chem. 69, 9764–9777. doi: 10.1021/acs.jafc.1c00569
Yang Y. P., Tong Q. Y., Zheng S. H., Zhou M. D., Zeng Y. M., Zhou T. T. (2020). Anti-inflammatory effect of fucoxanthin on dextran sulfate sodium-induced colitis in mice. Natural Product Res. 34, 1791–1795. doi: 10.1080/14786419.2018.1528593
Yang R., Wei D. (2020). Improving fucoxanthin production in mixotrophic culture of marine diatom Phaeodactylum tricornutum by LED light shift and nitrogen supplementation. Front. Bioengineering Biotechnol. 8. doi: 10.3389/fbioe.2020.00820
Yang Z., Yang R., Zhu D., Wei D. (2023). Multiple nitrogen supplementation and blue light enhancement promote the accumulation of fucoxanthin by Phaeodactylum tricornutum. J. Biol. Eng. 11, 4580–4592. doi: 10.13345/j.cjb.230192
Ye J. (2013). Mechanisms of insulin resistance in obesity. Front. Med. 7, 14–24. doi: 10.1007/s11684-013-0262-6
Ye J., Zheng J., Tian X., Xu B., Yuan F., Wang B., et al. (2022). Fucoxanthin attenuates free fatty acid-induced nonalcoholic fatty liver disease by regulating lipid metabolism/oxidative stress/inflammation via the AMPK/nrf2/TLR4 signaling pathway. Mar. Drugs 20, 225. doi: 10.3390/md20040225
Yi Z., Su Y., Xu M., Bergmann A., Ingthorsson S., Rolfsson O., et al. (2018). Chemical mutagenesis and fluorescence-based high-throughput screening for enhanced accumulation of carotenoids in a model marine diatom phaeodactylum tricornutum. Mar. Drugs 16, 272. doi: 10.3390/md16080272
Yi Z., Xu M., Magnusdottir M., Zhang Y., Brynjolfsson S., Fu W. (2015). Photo-oxidative stress-driven mutagenesis and adaptive evolution on the marine diatom phaeodactylum tricornutum for enhanced carotenoid accumulation. Mar. Drugs 13, 6138–6151. doi: 10.3390/md13106138
Yu R. X., Yu R. T., Liu Z. (2018). Inhibition of two gastric cancer cell lines induced by fucoxanthin involves downregulation of Mcl-1 and STAT3. Hum. Cell 31, 50–63. doi: 10.1007/s13577-017-0188-4
Zhu M., Li Y., Zhang M., Li X., Cheng M., Zhou Z., et al. (2021). Anti-senescence effect of fucoxanthin on D-gal-induced SH-SY5Y cells and its mechanism. Guangxi Sci. 3, 310–320. doi: 10.13656/j.cnki.gxkx.20210608.001
Keywords: microalage, carotenoid, fucoxanthin, biological properties, physiological activities
Citation: Gong B, Ma S, Yan Y and Wang Z (2024) Progress on the biological characteristics and physiological activities of fucoxanthin produced by marine microalgae. Front. Mar. Sci. 11:1357425. doi: 10.3389/fmars.2024.1357425
Received: 18 December 2023; Accepted: 06 February 2024;
Published: 26 February 2024.
Edited by:
Yang Liu, Shantou University, ChinaReviewed by:
Mahendran Sekar, Monash University Malaysia, MalaysiaJinlin Liu, Tongji University, China
Copyright © 2024 Gong, Ma, Yan and Wang. This is an open-access article distributed under the terms of the Creative Commons Attribution License (CC BY). The use, distribution or reproduction in other forums is permitted, provided the original author(s) and the copyright owner(s) are credited and that the original publication in this journal is cited, in accordance with accepted academic practice. No use, distribution or reproduction is permitted which does not comply with these terms.
*Correspondence: Zhaokai Wang, d2FuZ0B0aW8ub3JnLmNu
†These authors have contributed equally to this work