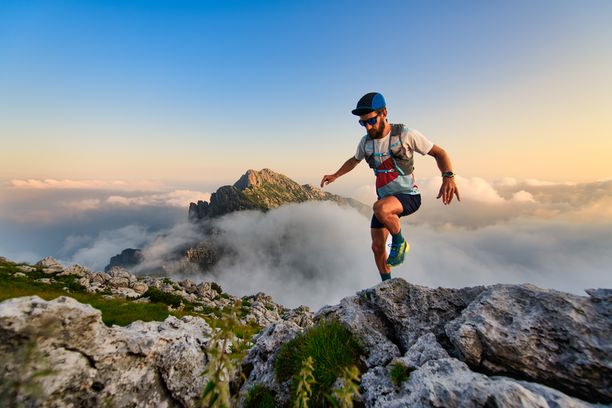
94% of researchers rate our articles as excellent or good
Learn more about the work of our research integrity team to safeguard the quality of each article we publish.
Find out more
ORIGINAL RESEARCH article
Front. Mar. Sci., 10 April 2024
Sec. Marine Ecosystem Ecology
Volume 11 - 2024 | https://doi.org/10.3389/fmars.2024.1354009
Foundation species that modify their habitat can facilitate other species, including other foundation species. Most studies focus solely on a single foundation species, overlooking such facilitation cascades. In this study, we investigated the interactions between the two coastal foundation species Mytilus edulis (blue mussel) and Lanice conchilega (sand mason worm). We investigated whether these species engage in facilitative interactions or if their association simply reflects a shared ecological niche on the soft-sediment intertidal flats of the Dutch Wadden Sea. To do so, we performed species distribution modeling, manipulative field experiments, and field surveys. We found a positive association between both foundation species, with a 2.45 times higher occurrence of both species compared to a random distribution. In addition, these species partially occupied the same ecological niche. We demonstrated that L. conchilega provided settlement substrate for M. edulis spat, increasing densities by 400 times compared to bare plots. Furthermore, M. edulis reefs facilitated L. conchilega occurrence in the wake of the reef. Biogenic reef development revealed that this interspecific facilitation resulted in spatial habitat heterogeneity. Therefore, we conclude that interspecific facilitation can significantly enhance the occurrence of these two important intertidal foundation species. Acknowledgment of such complex facilitation interactions has an untapped potential for improving the success of restoration and conservation programs.
Positive interactions between species are important drivers for species distribution and survival in many ecosystems worldwide (Bertness and Callaway, 1994). Mutualisms, for example, refer to a relationship where both species involved obtain a benefit, often through a tightly evolved and obligate connection. Commensalisms, on the other hand, describe a scenario where one species benefits without affecting the other. One-way facilitation refers to a situation where one species creates favorable conditions for another species. Last, reciprocal facilitation is a type of relationship where both species promote conditions that are favorable for each other (Bronstein, 2009, 2015). Some of the numerous examples of positive interactions in the marine realm are between coral polyps and their symbiotic algae (mutualism), epizoic barnacles and their sea turtle hosts (commensalism), or mangroves providing nursery habitats for a diverse assemblage of fishes (facilitation) (Nagelkerken et al., 2000; Stachowicz, 2001; Zardus, 2021).
Foundation species, species that dominate in abundance and biomass within a system and modify the physical environment, are known for their role in facilitating the survival or growth of other species by creating new habitats (Dayton, 1972; Bruno et al., 2003). The biogenic structures of foundation species can change the physical environment by e.g., reducing physical stress and/or providing complex structure. Other organisms can find refuge from predation and competition within this structure (Dayton, 1972; Jones et al., 1994). Foundation species can support many ecosystem functions and services, such as providing benefits for biodiversity, coastal defense, carbon storage, fisheries, nutrient cycling, and primary and secondary production (Costanza et al., 1998; Temmerman et al., 2013; Alongi, 2018). Kelp, corals, and mangroves are examples of marine foundation species that provide these important ecosystem services by adding structural complexity to the ecosystem (Kovalenko et al., 2012).
The role of single-foundation species facilitating entire communities and food webs through habitat creation has been extensively documented (Dayton, 1972; Bertness and Callaway, 1994). However, one foundation species can also facilitate another foundation species resulting in facilitation cascades (Altieri et al., 2007). Evidence is growing that ecosystems worldwide are structured by multiple foundation species that act within and among species and across different scales (Angelini et al., 2011; van de Koppel et al., 2015). Multiple foundation species can coexist in different assemblages: nested or adjacent (Angelini et al., 2011). In nested assemblages, the first foundation species inhabits a habitat and facilitates the colonization of a second foundation species by providing settlement substrate or shelter against biotic and abiotic stressors, such as cordgrass facilitating ribbed mussels (Altieri et al., 2007) or oysters attached to mangrove roots (Angelini and Silliman, 2014). In adjacent assemblages, foundation species change the environmental conditions to favorable conditions for others in an adjacent zone such as the interaction between corals, seagrasses, and mangroves (Gillis et al., 2017) or intertidal mussel beds facilitating nearby cockle beds (Donadi et al., 2013).
When facilitation is incorporated into the niche concept, it can expand the niche in a larger spatial range than predicted by the fundamental niche (Bruno et al., 2003; Chase and Leibold, 2011). For example, the realized niche of a species can be larger than the fundamental niche by increased resource availability, predation refuge, recruitment enhancement, and amelioration of physical stress (Bruno et al., 2003). These facilitative interactions can take place on both local and landscape scales (Bruno et al., 2003; Donadi et al., 2013; van de Koppel et al., 2015; Wang et al., 2022). Species rely more on positive interactions on the edges of their fundamental niche, where environmental factors determining species distribution are less optimal and more stressful for one or both species (Bruno et al., 2003). In these stressful environments, facilitation between species may be more important than competition because of stress amelioration, described as the stress-gradient hypothesis (Callaway, 2007).
Tidal flats, soft-sediment ecosystems bordering land and sea and regulated by the rhythm of the tides, are examples of ecosystems that are organized by high physical (wave action) and chemical (sulfides, anoxia, salinity) stresses (van Straaten, 1954; Gao, 2019). On tidal flats, emergent structures in the otherwise predominantly flat landscape contribute to the habitat complexity (Callaway, 2018; Nauta et al., 2023). Hence, organisms that form biogenic reefs, including oyster reefs, mussel beds, and reef-building polychaetes facilitate many other species by providing habitat complexity (De Smet et al., 2015; Christianen et al., 2017; van der Ouderaa et al., 2021). Under these challenging physical conditions, interspecific facilitation might be pivotal in community structure and ecosystem multifunctionality, such as regulating biological, geochemical, and physical processes of tidal flats (Angelini et al., 2011; Manning et al., 2018). For example, enhanced biodiversity and ecosystem multifunctionality, including decomposition, primary production, sediment accretion, and water infiltration rates, emerge exclusively where two or more foundation species overlap as seen with ribbed mussels aggregating around cordgrass stems (Angelini et al., 2015).
Two reef-building foundation species that can co-occur on tidal flats are the blue mussel, Mytilus edulis, and the sand mason worm, Lanice conchilega (Pallas 1766) (Hertweck, 1995). M. edulis is a foundation species that facilitates itself and other species in the surrounding tidal flat by decreasing near-bed hydrodynamic stress, increasing sediment stabilization, and increasing organic matter content through the production and deposition of pseudofeces (Graf and Rosenberg, 1997; Widdows et al., 1998; Gutiérrez et al., 2003; van der Zee et al., 2012). L. conchilega is a 10-20 cm long polychaete worm that builds tubes by cementing shell fragments and sand grains into a strong mucus layer. These tubes attenuate water flow velocity and change water flow direction, increasing sediment stabilization and sedimentation (Friedrichs et al., 2000; Borsje et al., 2014; Alves et al., 2017). Both foundation species facilitate a diverse assemblage of macroalgae, macrozoobenthos, fish, and birds (Albrecht and Reise, 1994; Van der Wal et al., 2014; De Smet et al., 2015; Christianen et al., 2017). Moreover, foundation species such as M. edulis and L. conchilega can contribute to the resilience of tidal flats as this process hinges on sediment budgets and accretion, especially in the face of rising sea levels (Huismans et al., 2022).
In this study, we investigated the interaction between M. edulis and L. conchilega and explored their general ecological niche on the tidal flats of the Dutch Wadden Sea. Specifically, we 1) examined species occurrence in a large-scale gridded sampling design (~4,500 samples per year) across the Dutch Wadden Sea, 2) explored the ecological niche of both species to examine their shared niche, 3) experimentally tested how L. conchilega tubes acted as settlement substrate for M. edulis, thereby facilitating nested assemblages, 4) observed whether M. edulis reefs enhanced the occurrence of L. conchilega in the adjacent hydrodynamic wake zone, 5) monitored the respective spatiotemporal biogenic reef development of both foundation species. In addition, we measured the effect of these two foundation species on the sediment properties to understand the mechanisms of facilitation. That is, we used sediment properties as proxies for hydrodynamic forces, where small grain sizes and high silt percentages are attributed to reduced hydrodynamic forces (Le Hir et al., 2000). We selected M. edulis and L. conchilega as study species because both species provide observable emergent structures to the soft-sediment tidal flats (Widdows et al., 1998; Alves et al., 2017). Furthermore, M. edulis historically served as the primary reef-building species before the establishment of the Pacific oyster, Magallana gigas (Markert et al., 2010; Markert, 2020). Studying these interactions in contemporary times holds significance as pressures on tidal flats, including the Wadden Sea, increase due to climate change factors such as rising temperatures, sea level fluctuations, erosion, sedimentation, and intensified extreme weather events.
The fieldwork was conducted in the Wadden Sea, the world’s largest system of tidal flats, gullies, and barrier islands. Its total tidal areas cover nearly 10,000 square kilometers along the coast of the Netherlands, Germany, and Denmark (Reise, 2005) with around 1,000 km2 of soft-sediment intertidal flats at mean low water and a total surface area of around 2,500 km2 at mean high water in the Dutch part of the Wadden Sea (Elias et al., 2012). The Wadden Sea is classified as a UNESCO World Heritage site (Unesco, 2014) and is protected as a Natura 2000 area under the European Bird- and Habitat directives, because of its high productivity and supports large numbers of invertebrates, fish, and birds with many long-distance migrants (van de Kam et al., 2004; van der Veer et al., 2015). Around 1990, the Dutch Wadden Sea experienced a drastic loss of nearly all (~4000 ha) intertidal mussel beds, primarily attributed to overfishing, storms, and recruitment failures (Dankers et al., 2001; Christianen et al., 2017). In 1991, the first Pacific oyster that attaches to mussel beds was observed outside cultivation plots (Reise, 1998). Consequently, mussels located within the interspace of oyster reefs exhibited reduced growth rates and body conditions due to interspecific competition (Dankers et al., 2001; Folmer et al., 2017). Moreover, climate change has impacted the Dutch Wadden Sea, leading to a temperature increase of 2.3°C over the last ~120 years, a decline in wind speed averaging -2% per decade, an increase in heavy rainfall events subsequently influencing sediment transport, and occurrence of extreme droughts in the years 2018, 2019 and 2020 (Hoekstra and Philippart, 2022).
We used the macrozoobenthos samples of the Synoptic Intertidal Benthic Survey (SIBES; (Bijleveld et al., 2012; Compton et al., 2013) to relate the abundance and biomass of M. edulis and L. conchilega to environmental predictors at the landscape scale. In the years 2008-2020, this large-scale gridded sampling design was sampled (~4,500 samples per year, in total 42,409 samples) with 500 m intervals and additional random points (Bijleveld et al., 2012) across all intertidal mudflats of the Dutch Wadden Sea (Figure 1). Sample sites were accessed by foot or boat and sampled with one (diameter 0.15 m, 0.0177 m2) or two cores (diameter 0.10 m, combined 0.0173 m2), respectively, to a depth of ~25 cm. At each sample site, densities and biomass of all macrozoobenthos and sediment characteristics were measured. All macrozoobenthos samples were sieved on a 1 mm round mesh and preserved in a 4% formaldehyde solution with rose Bengal dye (C.A.S. no. 632-68-8) for identification in the laboratory (Compton et al., 2013). All organisms were identified to the lowest taxonomic level possible. For this study, we only used the densities of M. edulis and L. conchilega. Sediment samples were collected and analyzed following the organic matter content, median grain size D50 (μm), and silt % <63 µm sampling procedures described in section 2.2.2.
Figure 1 Overview of sampling locations in the Dutch Wadden Sea: Synoptic Intertidal Benthic Survey (SIBES) grid (pink), field survey at Texel (light green; T), field survey at Griend (light green; G) and experimental set-up at Schiermonnikoog (light green; S).
We collected the top-1 and top-5 cm of the sediment surface with a small core (Ø 3 cm, 50 ml syringe) to determine the sediment properties: organic matter content, median grain size D50 (μm), and silt % <63 μm. Following sampling, the sediment samples were kept frozen. For calculation of organic matter content, the sediment samples were dried for 24 hours at 60°C and incinerated for 4 hours at 550°C to determine Ash Free Dry Weight (AFDW) and calculate weight loss on ignition (LOI wt%). Sediment samples were freeze-dried for 48 hours at -56°C, sieved over 1-mm mesh, and analyzed with the Malvern Mastersizer 2000 (Malvern Instruments, Worcestershire, United Kingdom, serial number 34403/139, model APA 2000 with Hydro G 2000 introduction unit and Autosampler 2000) to determine median grain size and silt%.
To construct the ecological niche of M. edulis and L. conchilega, we used the environmental parameters tidal emergence time in percentage, flow rate in m/s, shear stress in Pascal, salinity in parts per trillion, orbital velocity in m/s, median grain size (D50) in µm, and silt percentage in percentage <64 µm. Tidal emergence time, flow rate, and shear stress were predicted by Van Weerdenburg and Vroom (2021) according to the Dutch Wadden Sea Model (DWSM) in Delft3D-Flexible Mesh in the 2D version of the model with a horizontal resolution of 100x100 meter in the period between 23 June till 22 July 2017 (Van Weerdenburg and Vroom, 2021). This period represented the period from 2013 to 2017 because of similar average high-, and low-water levels (Van Weerdenburg and Vroom, 2021). Salinity was simulated by Van Weerdenburg and Vroom (2021) according to the 3D version of the DWSM with a horizontal resolution of 200x200 m for the year 2017. Orbital velocity was predicted by Van Weerdenburg and Vroom (2021) according to the SWAN-Kuststrook wave model with March 2020 as representative of the average annual wave conditions with a resolution of ~300 meters. Consequently, the root mean square of the amplitude of the predicted orbital movement within a certain time period was interpolated to a 100x100 meter grid (Van Weerdenburg and Vroom, 2021). In addition, sediment samples from the SIBES campaign were used and transformed into raster layers (grid cells 500x500m) for median grain size (D50), and silt percentage. If two samples were present within one grid cell (this was possible because of the random points), the mean of both samples was used. The DWSM may be too coarse to show the effects of biogenic reefs on the environment. On the other hand, sediment samples do reflect the effects of biogenic reefs on the surrounding tidal flat (lower grain size and higher silt percentage) (Widdows et al., 1998; Alves et al., 2017).
First, we studied the relation between M. edulis and L. conchilega occurrence in all sample locations of the SIBES data set by applying a Chi-square goodness of fit test (N=42409) to test for independence i.e., co-occurrence by chance. Second, the realized niche concerning the environmental predictors of M. edulis and L. conchilega was determined by fitting MaxEnt species distribution models (Research Resource Identifiers, RRID: SCR_021830). We used presence/background modeling; MaxEnt because it allows for complex, nonlinear responses between species presence and environmental parameters (Merow et al., 2013). To analyze the ecological niche of the species, we decided to use MaxEnt species distribution models (presence/background modeling) and not GLM (presence/absence modeling). The use of GLM is advised when absence data is available (Guillera-Arroita et al., 2014). However, the sampled surface of macrozoobenthos (0.0177 m2) was very small compared to the scale of the grid (500x500m). Foundation species might be absent in the sample but present in the nearby surroundings of the sampling point. Especially since our target species are both species that grow in patchy distributions with high variability from high densities to bare patches (Bertness and Grosholz, 1985; Callaway et al., 2010), sample points with absence data may be located in a suitable environment and not true absence samples (Degraer et al., 2008). Therefore, we choose to use presence/background modeling instead of presence/absence modeling. MaxEnt combined species presence data alongside randomly selected background locations, where species presence or absence could occur. The model then correlated this information with feature types: environmental parameters such as tidal emergence time, flow rate, shear stress, salinity, orbital velocity, median grain size (D50), and silt percentage to compute a relative occurrence rate (ROR). Consequently, the probability of species presence was calculated by applying a logistic output to the ROR (Merow et al., 2013). Presence data was obtained from the SIBES data and summarized in a grid (grid cells 500x500m). Species were labeled as present when observed in more than 2 out of the 13 years within one grid cell. Background locations were randomly created with a minimum distance of 2.27 km to correct for spatial autocorrelation and with a total number of >5 times the species’ presence sampling points within the intertidal area of the Dutch Wadden Sea (r-package: “sf”). The minimum distance between the background locations was calculated based on variograms (r-package: “gstats”) to prevent spatial autocorrelation, the likelihood that two locations are similar because of the distance between them. Variograms were calculated and the correlation between the sample locations was plotted as a function of their distance from each other. Consequently, we could determine the minimum distance in which the two locations were not spatial auto-correlated. Last, we derived the MaxEnt predicted presence probability maps of both species and averaged these two maps per grid cell, providing a probability of the overlapping or shared ecological niche between L. conchilega and M. edulis.
To test for the effect of L. conchilega tubes on mussel settlement, we performed a manipulative field experiment on an intertidal flat south of the island Schiermonnikoog (53°27’22.0”N, 6°9’30.2 “E) from August 2020 until March 2022 (Figure 1 and Appendix S1A). This tidal flat was characterized by bare sandy sediment and a tidal emergence time of approximately 30% per day. L. conchilega was present in medium-dense aggregations (~800 individual m-2), and at ~200-meter distance patches of mussels and oysters were observed. Within the L. conchilega aggregations, we created a randomized block design with two treatments in 3x3 m plots: ‘tubes present’ and ‘tubes removal’ (n=6). The plots were orientated in blocks of two, with four meters in between the plots and eight meters in between each block. No manipulation of the treatment ‘tubes present’ was necessary since the tubes were naturally present. A sled with a blade was pulled over the sediment surface (top 1 cm) to remove all tubes and associated fauna for the ‘tubes removal’ treatment. To reduce the modification of the sediment properties, we installed a sieve (Ø 1 mm) behind the blade that redeposited the sediment after cutting the tubes. After tube removal, we suffocated all fauna by digging in agricultural plastic sheets made from low-density polyethylene. After six days, we removed the plastic and removed all dead organic tissue on the sediment surface. Since it was impossible to selectively remove L. conchilega only, we had to remove all benthic fauna to prevent immediate new tube build-up. Subsequently, we performed measurements in August, October, and December 2020, June and September 2021 and March 2022. During the sampling rounds, we counted L. conchilega tubes with visible fringes and M. edulis abundance four times in the center of each plot with 20x20 cm frames. M. edulis was classified as a larva when its length measured less than one centimeter. We counted tubes with visible fringes because this indicates occupation by the worm itself (van Hoey et al., 2006). The fringes of the tubes are in general destroyed shortly after the worm abandons the tube or dies (van Hoey et al., 2006). In addition, we analyzed sediment properties according to the procedures described in section 2.2.2.
To measure the facilitation of L. conchilega abundance in the adjacent habitat zones of mixed mussel/oyster reefs (M. edulis mixed with Magallana gigas), we conducted surveys along six transects with two distinct characteristics: covering a mussel/oyster reef and a bare control at the north-east of the island Texel (53°8’41.5”N, 4°54’18.7”E), in August 2019, March 2020 and August 2020 (Figure 1 and Appendix S1A). The control site was chosen to be as near to the reef as possible and selected based on a similar habitat type: elevation measured with Real Time Kinematics dGPS (Trimble R8, GNSS system; –36 - –40 cm N.A.P. at 50 m distance from the reef), distance to the gully (~ 50 m during low tide), sediment type (sandy without dead shells). Hence, the distance between the reef and control sites was ~1500 m at Texel. The distance between the transects within one treatment and sample points was 50 meters, measured from the center of the reef (0 m distance from the reef, Appendix S1B). The sampling station –50 was positioned 50 meters from the hydrodynamically exposed edge of the reef. The sampling stations 50, 100, 150, and 200 meters were located in the wake of the reef. The sample points were positioned perpendicular to the incoming tide (Appendix S1B). At each sampling point, we counted L. conchilega tubes with visible fringes four times in 20x20 cm frames and estimated mussel/oyster reef cover in 50x50 cm frames (Van Hoey et al., 2008). In addition, we analyzed sediment properties according to procedures described earlier in section 2.2.2 Sediment properties sampling procedures and measured elevation with RTK dGPS. Furthermore, we compared the stable mixed mussel/oyster reef at Texel with a young mussel bed at the south of the island Griend (53°14’20.6”N, 5°15’31.6”E). This mussel bed disappeared within one year after the start of the monitoring. The survey set-up replicated that of Texel, with the exception that at Griend the distance between the reef and nearby control was ~300 m. This adjustment aimed to maintain constant environmental parameters including elevation and sediment type.
To monitor the establishment of a mussel bed on L. conchilega aggregations on a temporal scale, we surveyed reef development south-east of the island Griend (53°14’34.6”N, 5°18’6.5”E; Figure 1 and Appendix S1C). In August 2019, we measured the contours of dense L. conchilega aggregations with handheld GPS (Garmin GPSMap 66 Series). In April 2021, this reef was displaced by a young mussel bed. We measured the contours of this reef with drone imagery (DJI Mavic Pro 2) and handheld GPS and determined contours in qGIS (3.6.3-Noosa). In addition, we surveyed transects (n=9) that covered the young mussel bed and adjacent bare tidal flats in April and August 2021 (Appendix S1C). On these transects, we 1) counted L. conchilega tubes with intact fringes in 50x50 cm frames, and 2) measured elevation with RTK dGPS (Trimble R8, GNSS system) through sampling points (every 5 m).
We performed all statistical analyses in R (R Core Team, 2022). We validated model assumptions by 1) residuals versus fitted value plots to verify homogeneity of variances, 2) QQ-plots of the residuals to test for normality and 3) residuals versus each explanatory variable to check for independence. In addition, Shapiro-Wilks’s test (p > 0.05) and Bartlett’s test (p > 0.05) were used to test for normality and homogeneity of variance of the residuals, respectively.
For the removal experiment on Schiermonnikoog, M. edulis and L. conchilega tube counts were analyzed using generalized linear mixed models (GLMM) assuming a negative binomial, zero-inflated error distribution (absence was affected by the removal treatment; r-package: ‘glmmTMB’ (Brooks et al., 2017). The zero-inflation model (ZI) estimates the probability of an extra zero such that a positive estimate value indicates a higher chance of absence (e.g. ZI: tubes removal >0 means more absence). This is the opposite of the negative binomial model (NB) where a positive contrast indicates a higher abundance (e.g., NB: tubes removal >0 means higher abundances in this treatment) (Brooks et al., 2017). The sampling date and plot nested in blocks were included as random effects. For the transects on Texel, we summarized the counts of four pseudo-replicate L. conchilega tube counts per sampling station and analyzed these numbers by generalized linear models (GLM) with negative binomial distribution (glm.nb function from the R package MASS (Venables and Ripley, 2002). Tukey’s post-hoc comparisons were used to test for significant differences (p < 0.05) between treatments within distance from the reef (R package ‘emmeans’; (Lenth, 2019). Sampling dates (August 2019, March 2020, and August 2020) were used as replicates since there was no variation in means among the sampling time points. To test for differences between the treatments within distance from the reef, sediment D50 and silt percentage were analyzed with GLM with a negative binomial distribution, organic matter content was log-transformed and analyzed with linear models, and Tukey’s post-hoc comparisons were applied. To monitor biogenic reef development, we spatially visualized L. conchilega tube counts by ordinary kriging r-package: ‘automap’; Hiemstra, 2022). The function ‘autoKrige’ fits a variogram model to the given data set and returns the results of the interpolation: prediction, variance, and standard deviation. Consequently, the interpolated prediction was used to visualize L. conchilega tube counts.
To investigate the interlinkage between M. edulis and L. conchilega, we examined the occurrence frequency of both species at individual sample locations within the SIBES dataset. We found that both foundation species were positively associated with each other because the observed value of sample locations with the presence of both M. edulis and L. conchilega was 2.45 times higher than the expected value (X2(1, N=42409) = 831.45, p < 0.001, Figure 2 and Appendix S2). In addition, MaxEnt species distribution modeling revealed that 125 km2 and 81 km2 of the total intertidal area of the Dutch Wadden Sea was highly suitable (occurrence probability of > 0.6) for M. edulis and L. conchilega, respectively (Figure 3). Only 37 km2 of the ecological niches between both foundation species overlapped (Figure 3), which means that they share 30% and 46% of their niche with the other foundation species for M. edulis and L. conchilega respectively. The highly suitable areas for M. edulis were positioned closer to the mainland (training data AUC = 0.705), while these areas for L. conchilega are found closer to the barrier islands and tidal inlets (training data AUC = 0.734) (Figure 3).
Figure 2 Correlation matrix of the presence of both Lanice conchilega and Mytilus edulis in the sampling stations (n=42409) of the Synoptic Intertidal Benthic Survey (SIBES) in the Dutch Wadden Sea. Positive Pearson residuals indicate a positive associated between both foundation species.
Figure 3 Predicted species distribution with probability of occurrence showing logistic output predictions from MaxEnt for Lanice conchilega and Mytilus edulis individuals and their shared niche in soft-sediment intertidal flats in the Dutch Wadden Sea. Predictions are shown in intervals of probability of occurrence from 0 to 1, from orange (low probability; 0 – 0.5), yellow (mid probability; 0.5 – 0.6) to green (high probability; > 0.6).
To investigate the role of L. conchilega tubes as settlement substrate for M. edulis spat within nested assemblages, we experimentally removed L. conchilega tubes and measured the effect on M. edulis. High L. conchilega tube densities of 1542±160 m-2 (mean±SE) facilitated the settlement of M. edulis larvae (length: ~ 1 cm) (Figure 4A). M. edulis counts were 400 times higher in the ‘tubes present’ treatment compared to the ‘tubes removal’ treatment with 177±36 individual tube counts (mean±SE) (Figure 4B and Table 1). This facilitation within nested assemblage was visible until the end of the experiment in April 2022, almost one year after the first M. edulis settlement. Sediment properties did not differ between treatments, indicating that there was no significant attenuation of hydrodynamics by L. conchilega tubes (Appendix S3).
Figure 4 The development of (A) Lanice conchilega and (B) Mytilus edulis abundance after macrozoobenthos removal (i.e., aboveground tubes removal) in September 2020 showed enhanced establishment of M. edulis spat in the presence of L. conchilega tubes in nested assemblages. This implies that L. conchilega tubes facilitate the settlement of M. edulis larvae. Data are presented in mean±SE (n=24) and p-value are shown for negative binomial (NB), zero-inflated error distribution (ZI). ** P ≤ 0.01; *** P < 0.001.
Table 1 Statistical outcome of a) Lanice conchilega tube counts in removal experiment indicating a lower abundance (negative binomial part of the model < 0) and higher absence (zero-inflated part of the model > 0) of L. conchilega in the tubes removal treatment, b) the effect of tube removal on Mytilus edulis larvae settlement in nested assemblages indicating a lower abundance and higher absence of M. edulis in tubes removal, c) higher L. conchilega tube counts around mussel beds (50 and 150 m) in adjacent assemblages compared to nearby control.
To explore the facilitation of L. conchilega by mixed mussel/oyster reefs (M. edulis mixed with M. gigas), we surveyed L. conchilega tube densities and reef cover. No tubes 0±0 (mean±SE) were counted at sampling stations -50 m and 0 m mussel bed. The highest tube densities of 1606±122 m-2 (mean±SE) were found at 50 meters in the wake of the reef, and the lowest tube densities of 128±38 m-2 (mean±SE) were found at 200 m in the wake of the reef. The tube counts were on average 24 and 22 times higher at 50 (β = 3.17, SE ± 0.66, p < 0.001) and 150 m distances (β = 3.08, SE ± 0.67, p < 0.001), respectively, in the wake of the reef compared to the nearby control (Figure 5 and Table 1). The mussel/oyster reef area extent covered the sampling stations -50 and 0 m with an average reef cover of 45% at sampling station -50 and 75% at sampling station 0 (Figure 5A). At 50 meters in the wake of the reef, sediment silt percentage was higher (+17% silt), median grain size was lower (D50: –32 μm) and organic matter content was higher (+1% LOI) compared to the nearby control (Figure 5B and Appendix S4). The sediment surface around the reef center was elevated by ~ 0.35 meters (0 meters; Figure 5C). To compare these counts with a young mussel bed located south of the island Griend that disappeared within one year after the start of the monitoring, we observed the highest L. conchilega tube densities 3956±631 m-2 at 50 meters in the wake of the reef compared to the control with 171±106 tubes m-2. One year later, however, this mussel bed had disappeared, and L. conchilega tube densities were reduced to almost zero counts (Appendices S1, S5).
Figure 5 Field surveys indicate (A) interspecific facilitation in adjacent assemblages of Lanice conchilega densities at 50 and 150 meters in the wake of the mussel bed. The mussel bed affected environmental conditions by (B) higher sediment silt percentage at -50:50 meters distance from the reef and (C) elevation of the sediment surface around the reef center (0 meters). Significance levels show the difference between the mussel bed and nearby control. n.s. P > 0.05; *** P < 0.001.
To study the interlinkage between M. edulis and L. conchilega, we monitored biogenic reef development (Figure 6). In 2019, the monitored reef consisted of almost exclusively L. conchilega tubes with 2201±121 individual tubes m-2 (mean±SE, n=20) and covered a surface area of 383,507 m2 (Figure 6). In 2021, the reef was instead dominantly shaped by M. edulis, and L. conchilega tubes were mostly present in the adjacent habitat zone (50 – 150 m). The total surface of the biogenic reef dominated by M. edulis was 33,047 m2 and the highest L. conchilega tube densities (660 individual tubes m-2) were found within 50 m in the wake of the reef in 2021. Hence, biogenic reef development of M. edulis and L. conchilega indicated spatiotemporal dynamic habitat heterogeneity that consequently is important to promote biodiversity (van der Ouderaa et al., 2021).
Figure 6 Biogenic reef development from 2019 till 2021. In 2019 (A), the monitored reef consisted of almost exclusively Lanice conchilega aggregations 2201±121 individual tubes m-2 (mean±SE, n=20). In 2021 (B, C), the reef was dominantly shaped by Mytilus edulis and L. conchilega was present with the highest tube densities in the adjacent habitat zone ~ 50 m in the wake of the reef.
In this study, we defined the positive association of two coastal foundation species, Mytilus edulis and Lanice conchilega, from an ecological, experimental, and statistical perspective. These two reef-building species co-occurred in the same environment and partially occupied the same ecological niche (30-46%). In nested assemblages, L. conchilega tubes provided settlement substrate for M. edulis spat. On the other hand, mussel and mixed mussel/oyster reefs facilitated L. conchilega settlement in their wake (~50-150 m), sheltered from hydrodynamic forces. The interaction between these species may result in spatial and temporal habitat heterogeneity with fluctuating dominance of M. edulis and L. conchilega in which both foundation species facilitate their own survival indirectly: 1) L. conchilega tubes provide settlement substrate for M. edulis spat, 2) development of M. edulis beds drives to the exclusion of L. conchilega, 3) M. edulis beds facilitate L. conchilega in their wake, and this, as well as the eventual loss of the reef after a storm, followed by L. conchilega re-colonization, can initiate the cycle once again. Hence, we conclude that interspecific facilitation mechanisms may enhance the occurrence of both foundation species. While not being strictly necessary for the occurrence of either species, these species may and possibly expand each other’s niche on the tidal flats of the Wadden Sea. Therefore, these interactions among foundation species contribute to the species distribution of tidal flat’ communities and organize landscape-scale species patterns.
Our results showed a positive association between the occurrence of M. edulis and L. conchilega. In addition, M. edulis and L. conchilega share 30% and 46% of their ecological niche, respectively. These combined results suggest that interspecific facilitation may contribute to the enlargement of the ecological niche into the realized (and observed) ecological niche. Enlargement of the niche by facilitation has been described previously for mussel beds that stabilize the substratum and increase salt marsh production via the deposition of nutrients into the sediment (Bertness and Leonard, 1997; Stachowicz, 2001). Analyses of the large SIBES dataset (sampling stations: n=42409) establish a robust basis for the assumption that M. edulis and L. conchilega are positively correlated, potentially enhancing each other’s fundamental niche. For example, interspecific facilitation can ameliorate the environmental stressors that otherwise would limit species occurrences, such as providing settlement substrate or reducing hydrodynamic forces (Callaway, 2003; Walles et al., 2015).
Previous studies found that M. edulis settlement prefers structured habitat surfaces over bare substrata (Dean, 1981; Bourget et al., 1994) and that the structure of imitation tubes can provide settlement substrate for M. edulis larvae (Callaway, 2003). However, imitation tubes differed from natural tubes of L. conchilega because they were more rigid, more solid, not hollow, more persistent over time, and had no tentacle fringes (Callaway, 2003). This raised the question of whether natural L. conchilega tubes can also facilitate M. edulis larvae settlement. In this study, we showed that L. conchilega can indeed provide a suitable settlement substrate for M. edulis spat in natural conditions. The mechanisms behind this facilitation may be explained by two factors: the structure of the tubes reduces the hydrodynamic forces (Borsje et al., 2014) and/or the tubes provide a complex attachment substrate (Callaway, 2003). M. edulis larvae may use different factors to find a suitable substratum for settlement, e.g., physical [flow conditions, light, or gravity; (Bayne, 1964; Pernet et al., 2003)] or chemical [metabolites of algae or invertebrates; (Dobretsov, 1999)] cues. Our findings suggest that L. conchilega tubes provided settlement substrate for M. edulis spat, as we did not find indications of altered hydrodynamics based on sediment properties in the tubes removal plots compared to the tubes present plots (Le Hir et al., 2000). However, an additional effect of chemical metabolites produced by L. conchilega attracting M. edulis cannot be excluded since we have not been able to measure such cues.
By attenuating hydrodynamic forces from waves and currents, bivalve reefs typically have a much larger-scale impact on their surrounding physical landscape than the area they actually cover (Walles et al., 2015). This may facilitate other species such as seagrasses, salt marsh plants, and cockles in adjacent assemblages i.e., long-distance, cross-habitat facilitation (Wall et al., 2008; Donadi et al., 2013; van de Koppel et al., 2015; Walles et al., 2015). These reefs create habitat for other species by e.g., trapping water and increasing tidal emergence time (Nieuwhof et al., 2018; van der Ouderaa et al., 2021) or wave attenuation (Cheong et al., 2013). Walles et al. (2015) found that the spatial extent of habitat modification by bivalve reefs is of the same order of magnitude as the reef area (length, width, height). In our study area, the sediment surface of the bivalve reef was around 100 meters wide and on average 0.35 meters higher than the surrounding tidal flat. A similar elevation of the bare tidal flat to the height of the reef was reached at around 110 – 140 meters in the wake of the reef. Hence, the modified area leeward of the reef was expected to be around 100 – 140 meters. In addition, we found that the bivalve reefs increased silt percentage and reduced median grain size up to 50 meters in their wake. Both measurements are indicators of reduced hydrodynamic forces (Le Hir et al., 2000). However, mixed mussel/oyster reefs facilitated L. conchilega occurrence at 50 and 150 meters in their wake, but not at 100 meters. This might be because of the high patchy distribution of L. conchilega (Callaway et al., 2010). In general, our results imply that bivalve reefs create suitable habitats for L. conchilega in adjacent assemblage by mitigating hydrodynamic forces.
We observed that L. conchilega tubes facilitated M. edulis settlement and consequently reef establishment south-east of the island Griend. In addition, we observed that the M. edulis reef facilitated L. conchilega densities in the wake of the reef, which is hydrodynamically less exposed. This suggests that interspecific facilitation may promote the co-occurrence of both species by creating temporal shifts between both species. Our findings find support in the observations made by (Hertweck, 1995), who found M. edulis spatfall attached to L. conchilega tubes in dense aggregations on the tidal flats south of the island Spiekeroog, German Wadden Sea. In addition, L. conchilega is frequently found surrounding mussel beds (Hertweck, 1995; Bungenstock et al., 2021). Although the interplay between both species appears evident, these cause-effect relationships must be interpreted with caution since they rely on observational data (Hertweck, 1995; Bungenstock et al., 2021). Moreover, additional research approaches, such as experiments and species distribution modeling, further support the interdependency of the two species. This extensive effort was essential for testing the interdependency across various local contexts, long-term temporal scales, and large spatial scales, ensuring objectivity in statistical analyses. Yet, the question remains which environmental factors promote the dominance of a certain foundation species? For example, it is known that cold winters limit the growth of L. conchilega populations (Strasser and Pieloth, 2001). On the other hand, L. conchilega tubes are more resistant to storms or winter ice because they are anchored deeper (~30 cm) into the sediment than M. edulis beds (Alves et al., 2017). Furthermore, M. edulis beds are attached to the sediment surface and winter ice can dislodge the mussels from the substratum (Bertness and Grosholz, 1985; Bungenstock et al., 2021). Therefore, this study emphasizes the importance of supporting optimal growth strategies for biogenic reef development by biological interactions.
Long-term reef development of M. edulis and L. conchilega on tidal flats is a dynamic process shaped by complex interactions between environmental factors and biological interactions. M. edulis spat aggregate densely on substrates, such as rocks, and shells (Wehrmann, 2003). Over time, the accumulation of mussel beds alters sedimentation patterns, stabilizes substrates, and enhances the survival of the reef (Widdows et al., 1998). In this study, we described that L. conchilega tubes function as settlement substrates for M. edulis larvae. Eventually, the M. edulis spat may develop into the physical habitat structure of a M. edulis bed, as evidenced by Callaway (2003) findings from a six-year-long experiment. The settlement of L. conchilega larvae relies on the hydrodynamic conditions and the substrate to settle on (D’Hurlaborde et al., 2022). Whether L. conchilega aggregations will develop into a biogenic reef, depends on their resistance to disturbance, recovery potential, and their large-scale persistence (Rabaut et al., 2009; Callaway et al., 2010). We described that M. edulis beds create favorable conditions for L. conchilega reef development in their wake. Although we were unable to assess this long-distance facilitation over an extended duration, similar long-distance facilitation of the edible cockle Cerastoderma edule by M. edulis beds was reported in 2009 and 2011 (Donadi et al., 2013). Hence, the influence of interspecific facilitation seems to contribute to the long-term development of biogenic reefs.
The existence of biogenic reefs such as M. edulis and L. conchilega relies on the establishment and survival of the reefs. This existence can be enhanced by the optimization of the environmental variables as settlement substrate and/or reduced hydrodynamics. For instance, the protection of areas with relatively stable L. conchilega aggregations can improve reef settlement of M. edulis. Re-establishment of mussel beds after storm events may be encouraged when L. conchilega aggregations are present. For active restoration, it might be necessary to temporarily mimic the physical structure of biogenic reefs with artificial structures to improve the local environmental conditions for reef establishment (Temmink et al., 2022; Nauta et al., 2023). However, sufficient structure and size of the biogenic reef is needed to achieve the restoration of landscape-scale connectivity (Gillis et al., 2017). Biogenic reef structure and size of L. conchilega aggregations can be classified by using a ‘reefiness’ score with highest ‘reefiness’ scores for densities of >1,500 individual m-2, a total area extent of >100,000 m2, and a relative height of >9 cm (Hendrick and Foster-Smith, 2006; Rabaut et al., 2009). Based on this ‘reefiness’ classification, the L. conchilega aggregation in this study can be classified as high-scoring biogenic reefs. Restoring landscape-scale connectivity means that the one biogenic reef-building species needs to be restored to a sufficient physical scale to generate positive effects on the other (foundation) species. The methodology applied in this study, incorporating field surveys, experiments, and species distribution models, could serve as a recipe for further understanding and co-restoration of interspecific dependency in the Dutch Wadden Sea. Moreover, biogenic reefs including M. edulis and L. conchilega provide numerous ecosystem services such as enhanced biodiversity and fisheries, sediment stabilization, and reduced hydrodynamics (Rabaut et al., 2007, 2010; De Smet et al., 2015; Alves et al., 2017; zu Ermgassen et al., 2020). Therefore, we suggest that possibilities for co-restoring foundation species with other foundation species need to be further explored.
Our findings emphasize the significance of interspecific facilitation between foundation species. Hence, enhancing restoration and conservation initiatives centered around these foundation species can be achieved by integrating interspecific facilitation processes. We suggest that it is valuable to look beyond the target species itself and focus on co-restoring foundation species and landscape-scale ecosystem connectivity. Given the increasing pressures from climate change, especially on tidal flats where species are constantly subjected to environmental stressors, species strongly rely on each other for establishment and survival. Foundation species living in these dynamic and harsh circumstances can benefit from interspecific facilitation interactions, with associated advantages for higher biodiversity (De Smet et al., 2015; Christianen et al., 2017). Therefore, interspecific facilitation on landscape scales requires more attention in nature conservation and restoration programs.
The raw data supporting the conclusions of this article will be made available by the authors, without undue reservation.
JN: Conceptualization, Formal analysis, Investigation, Methodology, Validation, Visualization, Writing – original draft. KM: Investigation, Methodology, Validation, Writing – review & editing. LD: Investigation, Methodology, Writing – review & editing. VR: Conceptualization, Investigation, Methodology, Supervision, Writing – review & editing. TB: Conceptualization, Supervision, Writing – review & editing. DW: Conceptualization, Supervision, Writing – review & editing. HO: Conceptualization, Supervision, Writing – review & editing. SH: Investigation, Writing – review & editing. AB: Writing – review & editing. HV: Writing – review & editing. OF: Writing – review & editing. LG: Supervision, Writing – review & editing.
The author(s) declare financial support was received for the research, authorship, and/or publication of this article. VR was funded by NWO-Veni grant VI.Veni.212.059. AB was funded by NWO-Veni grant VI.Veni.192.051. LG was funded by NWO-VENI grant 016. Veni.181.087. KM and OF were funded by Waddenfonds grant “Waddenmosaic”. SIBES is currently financed by the Nederlandsche Aardolie Maatschappij NAM, Rijkswaterstaat RWS, and the Royal NIOZ.
We thank all volunteers, students, and professionals who helped in the field for their enthusiasm and hard work. In special, thanks to Henk Wiersma from the Fieldwork Company for his inventions; Jouke, Sien, and Saar; the crew of the Asterias from the ‘Wadden Unit’ for transportation; Franka Lotze, Nadia Hijner, Maarten Zwarts, Annieke Borst, Farah Kootstra, Dindi Hiddink Verberne, Vasiliki Mantzana Oikonomaki, Clazina Kwakernaak, Lies Bosma, Puck Heerdink, Chananja Riepma for their help in the field. We are grateful to the current SIBES core team for collecting and processing the thousands of samples. In alphabetical order they are Leo Boogert, Thomas de Brabander, Anne Dekinga, Job ten Horn, Loran Kleine Schaars, Jeroen Kooijman, Anita Koolhaas, Franka Lotze, Simone Miguel, Luc de Monte, Dennis Mosk, Amin Niamir, Dana Nolte, Dorien Oude Luttikhuis, Bianka Rasch, Reyhane Roohi, Juan Schiaffi, Marten Tacoma, Eveline van Weerlee and Bas de Wit. We also thank all former and current employees and the many volunteers and students who have ensured that the SIBES program has continued in recent years. The RV Navicula was essential for collecting the samples and we particularly thank the current crew Wim Jan Boon, Klaas Jan Daalder, Bram Fey, Hendrik Jan Lokhorst, and Hein de Vries. We thank the province of Fryslân for research permits (R. Deen) and Natuurmonumenten (Q. Smeele, E. Jansen, JW. Zwart) for site access. We thank Bram van Prooijen for his ideas and brainstorming about the effect of biogenic reefs on reduced hydrodynamics.
The authors declare that the research was conducted in the absence of any commercial or financial relationships that could be construed as a potential conflict of interest.
All claims expressed in this article are solely those of the authors and do not necessarily represent those of their affiliated organizations, or those of the publisher, the editors and the reviewers. Any product that may be evaluated in this article, or claim that may be made by its manufacturer, is not guaranteed or endorsed by the publisher.
The Supplementary Material for this article can be found online at: https://www.frontiersin.org/articles/10.3389/fmars.2024.1354009/full#supplementary-material
Albrecht A., Reise K. (1994). Effects of Fucus vesiculosus covering intertidal mussel beds in the Wadden Sea. Helgoländer. Meeresuntersuchungen. 48, 243–256. doi: 10.1007/BF02367039
Alongi D. M. (2018). Impact of global change on nutrient dynamics in mangrove forests. For. Trees Livelihoods. 9, 596. doi: 10.3390/f9100596
Altieri A. H., Silliman B. R., Bertness M. D. (2007). Hierarchical organization via a facilitation cascade in intertidal cordgrass bed communities. Am. Nat. 169, 195–206. doi: 10.1086/510603
Alves R. M. S., Van Colen C., Vincx M., Vanaverbeke J., De Smet B., Guarini J.-M., et al. (2017). A case study on the growth of Lanice conChilega (Pallas 1766) aggregations and their ecosystem engineering impact on sedimentary processes. J. Exp. Mar. Bio. Ecol. 489, 15–23. doi: 10.1016/j.jembe.2017.01.005
Angelini C., Altieri A. H., Silliman B. R., Bertness M. D. (2011). Interactions among foundation species and their consequences for community organization, biodiversity, and conservation. Bioscience 61, 782–789. doi: 10.1525/bio.2011.61.10.8
Angelini C., Silliman B. R. (2014). Secondary foundation species as drivers of trophic and functional diversity: evidence from a tree–epiphyte system. Ecology 95, 185–196. doi: 10.1890/13-0496.1
Angelini C., van der Heide T., Griffin J. N., Morton J. P., Derksen-Hooijberg M., Lamers L. P. M., et al. (2015). Foundation species’ overlap enhances biodiversity and multifunctionality from the patch to landscape scale in southeastern United States salt marshes. Proc. Biol. Sci. 282, 20150421. doi: 10.1098/rspb.2015.0421
Bayne B. L. (1964). The responses of the larvae of Mytilus edulis L. to light and to gravity. Oikos 15, 162. doi: 10.2307/3564753
Bertness M. D., Callaway R. (1994). Positive interactions in communities. Trends Ecol. Evol. 9, 191–193. doi: 10.1016/0169-5347(94)90088-4
Bertness M. D., Grosholz E. (1985). Population dynamics of the ribbed mussel, Geukensia demissa: The costs and benefits of an aggregated distribution. Oecologia 67, 192–204. doi: 10.1007/BF00384283
Bertness M. D., Leonard G. H. (1997). The role of positive interactions in communities: lessons from intertidal habitat. Ecology 78, 1976–1989. doi: 10.1890/0012-9658(1997)078[1976:TROPII]2.0.CO;2
Bijleveld A. I., van Gils J. A., van der Meer J., Dekinga A., Kraan C., van der Veer H. W., et al. (2012). Designing a benthic monitoring program with multiple conflicting objectives. Methods Ecol. Evol. 3, 526–536. doi: 10.1111/j.2041-210X.2012.00192.x
Borsje B. W., Bouma T. J., Rabaut M., Herman P. M. J., Hulscher S. J. M. H. (2014). Formation and erosion of biogeomorphological structures: A model study on the tube-building polychaete Lanice conChilega. Limnol. Oceanogr. 59, 1297–1309. doi: 10.4319/lo.2014.59.4.1297
Bourget E., DeGuise J., Daigle G. (1994). Scales of substratum heterogeneity, structural complexity, and the early establishment of a marine epibenthic community. J. Exp. Mar. Bio. Ecol. 181, 31–51. doi: 10.1016/0022-0981(94)90102-3
Bronstein J. L. (2009). The evolution of facilitation and mutualism. J. Ecol. 97, 1160–1170. doi: 10.1111/j.1365-2745.2009.01566.x
Bronstein J. L. (2015). Mutualism. Available at: https://books.google.com/books?hl=en&lr=&id=tlIdCgAAQBAJ&oi=fnd&pg=PP1&dq=mutualism+bronstein&ots=p3shYJscpL&sig=ZNvBS09FL6zQOFW1n2KYnb-1sfE.
Brooks M., Kristensen K., Benthem K., Magnusson A., Berg C., Nielsen A., et al. (2017). GlmmTMB balances speed and flexibility among packages for zero-inflated generalized linear mixed modeling. R. J. 9, 378. doi: 10.32614/RJ-2017-066
Bruno J. F., Stachowicz J. J., Bertness M. D. (2003). Inclusion of facilitation into ecological theory. Trends Ecol. Evol. 18, 119–125. doi: 10.1016/S0169-5347(02)00045-9
Bungenstock F., Hertweck G., Hochstein M. L., Wehrmann A. (2021). Distribution pattern and controls of biosedimentary facies in backbarrier tidal flats of the central Wadden Sea (North Sea). Z. Dtsch. Ges. Geowiss. 172, 409–428. doi: 10.1127/zdgg/2021/0248
Callaway R. (2003). Long-term effects of imitation polychaete tubes on benthic fauna: They anchor Mytilus edulis (L.) banks. J. Exp. Mar. Bio. Ecol. 283, 115–132. doi: 10.1016/S0022-0981(02)00474-4
Callaway R. M. (2007). Positive interactions and Interdependence in Plant Communities (Dordrecht, Netherlands: Springer). doi: 10.1007/978-1-4020-6224-7
Callaway R. (2018). Interstitial space and trapped sediment drive benthic communities in artificial shell and rock reefs. Front. Mar. Sci. 5, 288. doi: 10.3389/fmars.2018.00288
Callaway R., Desroy N., Dubois S. F., Fournier J., Frost M., Godet L., et al. (2010). Ephemeral bio-engineers or reef-building polychaetes: how stable are aggregations of the tube worm lanice conChilega (Pallas 1766)? Integr. Comp. Biol. 50, 237–250. doi: 10.1093/icb/icq060
Cheong S.-M., Silliman B., Wong P. P., van Wesenbeeck B., Kim C.-K., Guannel G. (2013). Coastal adaptation with ecological engineering. Nat. Clim. Change 3, 787–791. doi: 10.1038/nclimate1854
Christianen M. J. A., van der Heide T., Holthuijsen S. J., van der Reijden K. J., Borst A. C. W., Olff H. (2017). Biodiversity and food web indicators of community recovery in intertidal shellfish reefs. Biol. Conserv. 213, 317–324. doi: 10.1016/j.biocon.2016.09.028
Compton T. J., Holthuijsen S., Koolhaas A., Dekinga A., ten Horn J., Smith J., et al. (2013). Distinctly variable mudscapes: Distribution gradients of intertidal macrofauna across the Dutch Wadden Sea. J. Sea. Res. 82, 103–116. doi: 10.1016/j.seares.2013.02.002
Costanza R., d’Arge R., de Groot R., Farber S., Grasso M., Hannon B., et al. (1998). The value of ecosystem services: putting the issues in perspective. Ecol. Econ. 25, 67–72. doi: 10.1016/S0921-8009(98)00019-6
Dankers N., Brinkman A. G., Meijboom A., Dijkman E. (2001). Recovery of intertidal mussel beds in the Waddensea: Use of habitat maps in the management of the fishery. Hydrobiologia 465, 21–30. doi: 10.1023/A:1014592808410
Dayton P. K. (1972). “Toward an understanding of community resilience and the potential effects of enrichments to the benthos at McMurdo Sound, Antarctica,” in Proceedings of the colloquium on conservation problems in Antarctica (Blacksberg, VA), 81–96. Available at: http://daytonlab.ucsd.edu/.
Dean T. A. (1981). Structural aspects of sessile invertebrates as organizing forces in an Estuarine fouling community. J. Exp. Mar. Bio. Ecol. 53, 163–180. doi: 10.1016/0022-0981(81)90017-4
Degraer S., Moerkerke G., Rabaut M., Van Hoey G., Four I. D., Vincx M., et al. (2008). Very-high resolution side-scan sonar mapping of biogenic reefs of the tube-worm Lanice conChilega. Remote Sens. Environ. 112 (8), 3323–3328. doi: 10.1016/j.rse.2007.12.012
De Smet B., D’Hondt A. S., Verhelst P., Fournier J., Godet L., Desroy N., et al. (2015). Biogenic reefs affect multiple components of intertidal soft-bottom benthic assemblages: The Lanice conChilega case study. Estuar. Coast. Shelf. Sci. 152, 44–55. doi: 10.1016/j.ecss.2014.11.002
D’Hurlaborde A., Semeraro A., Sterckx T., Van Hoey G. (2022). Optimized screening methods for investigation of the larval settlement of lanice conChilega on artificial substrates. J. Mar. Sci. Eng. 10, 1443. doi: 10.3390/jmse10101443
Dobretsov S. V. (1999). Effects of macroalgae and biofilm on settlement of blue mussel (Mytilus edulis l.) larvae. Biofouling 14, 153–165. doi: 10.1080/08927019909378406
Donadi S., van der Heide T., van der Zee E., Eklöf J. S., Van de Koppel J., Ellen J., et al. (2013). Cross-habitat interactions among bivalves species control community structure on intertidal flats. Ecology 94, 489–498. doi: 10.1890/12-0048.1
Elias E. P. L., van der Spek A. J. F., Wang Z. B., de Ronde J. (2012). Morphodynamic development and sediment budget of the Dutch Wadden Sea over the last century. Neth. J. Geosci. 91, 293–310. doi: 10.1017/S0016774600000457
Folmer E., Büttger H., Herlyn M., Markert A., Millat G., Troost K., et al. (2017). Beds of blue mussels and Pacific oysters. Available at: https://www.narcis.nl/publication/RecordID/oai:imis.nioz.nl:292237 (Accessed April 4, 2023).
Friedrichs M., Graf G., Springer B. (2000). Skimming flow induced over a simulated polychaete tube lawn at low population densities. Mar. Ecol. Prog. Ser. 192, 219–228. doi: 10.3354/meps192219
Gao S. (2019). “Chapter 10 - geomorphology and Sedimentology of Tidal Flats,” in Coastal Wetlands, 2nd ed. Eds. Perillo G. M. E., Wolanski E., Cahoon D. R., Hopkinson C. S. (Elsevier), 359–381.
Gillis L. G., Jones C. G., Ziegler A. D., van der Wal D., Breckwoldt A., Bouma T. J. (2017). Opportunities for protecting and restoring tropical coastal ecosystems by utilizing a physical connectivity approach. Front. Mar. Sci. 4. doi: 10.3389/fmars.2017.00374
Graf G., Rosenberg R. (1997). Bioresuspension and biodeposition: a review. J. Mar. Syst. 11, 269–278. doi: 10.1016/S0924-7963(96)00126-1
Guillera-Arroita G., Lahoz-Monfort J. J., Elith J. (2014). Maxent is not a presence-absence method: a comment on Thibaudet al. Methods Ecol. Evol. 5, 1192–1197. doi: 10.1111/2041-210X.12252
Gutiérrez J. L., Jones C. G., Strayer D. L., Iribarne O. O. (2003). Mollusks as ecosystem engineers: the role of shell production in aquatic habitats. Oikos 101, 79–90. doi: 10.1034/j.1600-0706.2003.12322.x
Hendrick V. J., Foster-Smith R. L. (2006). Sabellariaspinulosa reef: a scoring system for evaluating ‘reefiness’ in the context of the Habitats Directive. J. Mar. Biol. Assoc. U. K. 86, 665–677. doi: 10.1017/S0025315406013555
Hertweck G. (1995). Verteilung charakteristischer Sedimentkörper und der Benthossiedlungen im Rückseitenwattder Insel Spiekeroog, südliche Nordsee: 1. Ergebnis der Wattkartierung 1988–92. Senkenb. Marit. 26, 81–94.
Hoekstra P., Philippart K. (2022). Klimaatverandering in Nederland: feiten trends en ontwikkeling die relevant zijn voor het Waddengebied (Waddenacademie).
Huismans Y., van der Spek A., Lodder Q., Zijlstra R., Elias E., Wang Z. B. (2022). Development of intertidal flats in the Dutch Wadden Sea in response to a rising sea level: Spatial differentiation and sensitivity to the rate of sea level rise. Ocean. Coast. Manage. 216, 105969. doi: 10.1016/j.ocecoaman.2021.105969
Jones C. G., Lawton J. H., Shachak M. (1994). Organisms as ecosystem Organisms engineers. Oikos 69, 373–386. doi: 10.2307/3545850
Kovalenko K. E., Thomaz S. M., Warfe D. M. (2012). Habitat complexity: approaches and future directions. Hydrobiologia. 685, 1–17. doi: 10.1007/s10750-011-0974-z
Le Hir P., Roberts W., Cazaillet O., Christie M., Bassoullet P., Bacher C. (2000). Characterization of intertidal flat hydrodynamics. Cont. Shelf. Res. 20, 1433–1459. doi: 10.1016/S0278-4343(00)00031-5
Lenth R. (2019). emmeans: Estimated Marginal Means, aka Least-Squares Means. R package version 461 1.4.3.01. Available at: https://CRAN.R-project.org/package=emmeans.
Manning P., van der Plas F., Soliveres S., Allan E., Maestre F. T., Mace G., et al. (2018). Redefining ecosystem multifunctionality. Nat. Ecol. Evol. 2, 427–436. doi: 10.1038/s41559-017-0461-7
Markert A. (2020). How dense is dense? Toward a harmonized approach to characterizing reefs of non-native Pacific oysters – with consideration of native mussels. Neobiota. 57, 7–52. doi: 10.3897/neobiota.57.49196
Markert A., Wehrmann A., Kröncke I. (2010). Recently established Crassostrea-reefs versus native Mytilus-beds: differences in ecosystem engineering affects the macrofaunal communities (Wadden Sea of Lower Saxony, southern German Bight). Biol. Invasions. 12, 15–32. doi: 10.1007/s10530-009-9425-4
Merow C., Smith M. J., Silander J. A. (2013). A practical guide to MaxEnt for modeling species’ distributions: What it does, and why inputs and settings matter. Ecography 36, 1058–1069. doi: 10.1111/j.1600-0587.2013.07872.x
Nagelkerken I., van der Velde G., Gorissen M. W., Meijer G. J., Van’t Hof T., den Hartog C. (2000). Importance of mangroves, seagrass beds and the shallow coral reef as a nursery for important coral reef fishes, using a visual census technique. Estuar. Coast. Shelf. Sci. 51, 31–44. doi: 10.1006/ecss.2000.0617
Nauta J., Christianen M. J. A., Temmink R. J. M., Fivash G. S., Marin-Diaz B., Reijers V. C., et al. (2023). Biodegradable artificial reefs enhance food web complexity and biodiversity in an intertidal soft-sediment ecosystem. J. Appl. Ecol. 60, 541–552. doi: 10.1111/1365-2664.14348
Nieuwhof S., Van Belzen J., Oteman B., Van De Koppel J., Herman P. M. J., van der Wal D. (2018). Shellfish reefs increase water storage capacity on intertidal flats over extensive spatial scales. Ecosystems 21, 360–372. doi: 10.1007/s10021-017-0153-9
Pernet F., Tremblay R., Bourget E. (2003). Settlement success, spatial pattern and behavior of mussel larvae Mytilus spp. in experimental “downwelling” systems of varying velocity and turbulence. Mar. Ecol. Prog. Ser. 260, 125–140. doi: 10.3354/meps260125
Rabaut M., Guilini K., Van Hoey G., Vincx M., Degraer S. (2007). A bio-engineered soft-bottom environment: The impact of Lanice conChilega on the benthic species-specific densities and community structure. Estuar. Coast. Shelf. Sci. 75, 525–536. doi: 10.1016/j.ecss.2007.05.041
Rabaut M., Van de Moortel L., Vincx M., Degraer S. (2010). Biogenic reefs as structuring factor in Pleuronectes platessa (Plaice) nursery. J. Sea. Res. 64, 102–106. doi: 10.1016/j.seares.2009.10.009
Rabaut M., Vincx M., Degraer S. (2009). Do Lanice conChilega (sandmason) aggregations classify as reefs? Quantifying habitat modifying eVects. Helgol. Mar. Res. 63, 37–46. doi: 10.1007/s10152-008-0137-4
R Core Team. (2022). R: A language and environment for statistical computing (Vienna, Australia: R Foundation for Statistical Computing).
Reise K. (1998). Pacific oysters invade mussel beds in the European Wadden Sea. Senckenb. Marit. 28, 167–175. doi: 10.1007/BF03043147
Reise K. (2005). Coast of change: Habitat loss and transformations in the Wadden Sea. Helgol. Mar. Res. 59, 9–21. doi: 10.1007/s10152-004-0202-6
Stachowicz J. J. (2001). Mutualism, Facilitation, and the Structure of Ecological Communities: Positive interactions play a critical, but underappreciated, role in ecological communities by reducing physical or biotic stresses in existing habitats and by creating new habitats on which many species depend. BioScience 51, 235–246. doi: 10.1641/0006-3568(2001)051[0235:MFATSO]2.0.CO;2
Strasser M., Pieloth U. (2001). Recolonization pattern of the polychaete Lanice conChilega on an intertidal sand flat following the severe winter of 1995/96. Helgol. Mar. Res. 55, 176–181. doi: 10.1007/s101520100081
Temmerman S., Meire P., Bouma T. J., Herman P. M. J., Ysebaert T., De Vriend H. J. (2013). Ecosystem-based coastal defense in the face of global change. Nature 504, 79–83. doi: 10.1038/nature12859
Temmink R. J. M., Fivash G. S., Govers L. L., Nauta J., Marin-Diaz B., Cruijsen P. M. J. M., et al. (2022). Initiating and upscaling mussel reef establishment with life cycle informed restoration: Successes and future challenges. Ecol. Eng. 175, 106496. doi: 10.1016/j.ecoleng.2021.106496
van de Kam J., Ens B., Piersma T., Zwarts L. (2004). Shorebirds: an illustrated behavioral ecology. KNNV. Publishers. doi: 10.1163/9789004277991
van de Koppel J., van der Heide T., Altieri A. H., Eriksson B. K., Bouma T. J., Olff H., et al. (2015). Long-distance interactions regulate the structure and resilience of coastal ecosystems. Ann. Rev. Mar. Sci. 7, 139–158. doi: 10.1146/annurev-marine-010814-015805
van der Ouderaa I. B. C., Claassen J. R., van de Koppel J., Bishop M. J., Eriksson B. K. (2021). Bioengineering promotes habitat heterogeneity and biodiversity on mussel reefs. J. Exp. Mar. Bio. Ecol. 540, 151561. doi: 10.1016/j.jembe.2021.151561
van der Veer H. W., Dapper R., Henderson P. A., Jung A. S., Philippart C. J. M., Witte J. I. J., et al. (2015). Changes over 50 years in fish fauna of a temperate coastal sea: Degradation of trophic structure and nursery function. Estuar. Coast. Shelf. Sci. 155, 156–166. doi: 10.1016/j.ecss.2014.12.041
Van der Wal D., Van Dalen J., Wielemaker-van den Dool A., Dijkstra J. T., Ysebaert T. (2014). Biophysical control of intertidal benthic macroalgae revealed by high-frequency multispectral camera images. J. Sea. Res. 90, 111–120. doi: 10.1016/j.seares.2014.03.009
van der Zee E. M., van der Heide T., Donadi S., Eklöf J. S., Eriksson B. K., Olff H., et al. (2012). Spatially extended habitat modification by intertidal reef-building bivalves has implications for consumer-resource interactions. Ecosystems 15, 664–673. doi: 10.1007/s10021-012-9538-y
Van Hoey G., Guilini K., Rabaut M., Vincx M., Degraer S. (2008). Ecological implications of the presence of the tube-building polychaete Lanice conChilega on soft-bottom benthic ecosystems. Mar. Biol. 154, 1009–1019. doi: 10.1007/s00227-008-0992-1
van Hoey G., Vincx M., Degraer S. (2006). Some recommendations for an accurate estimation of Lanice conChilega density based on tube counts. Helgol. Mar. Res. 60, 317–321. doi: 10.1007/s10152-006-0041-8
van Straaten L. M. J. U. (1954). Composition and structure of recent marine sediments in the Netherlands. Leidse. Geologische. Mededelingen. 19, 1–108.
Venables W. N., Ripley B. D. (2002). Modern Applied Statistics with S. Available at: https://www.stats.ox.ac.uk/pub/MASS4/.
Wall C. C., Peterson B. J., Gobler C. J. (2008). Facilitation of seagrass Zostera marina productivity by suspension-feeding bivalves. Mar. Ecol. Prog. Ser. 357, 165–174. doi: 10.3354/meps07289
Walles B., Salvador de Paiva J., van Prooijen B. C., Ysebaert T., Smaal A. C. (2015). The ecosystem engineer crassostrea gigas affects tidal flat morphology beyond the boundary of their reef structures. Estuaries. Coasts. 38, 941–950. doi: 10.1007/s12237-014-9860-z
Wang B., Zhang K., Liu Q.-X., He Q., van de Koppel J., Teng S. N., et al. (2022). Long-distance facilitation of coastal ecosystem structure and resilience. Proc. Natl. Acad. Sci. U.S.A. 119, e2123274119. doi: 10.1073/pnas.2123274119
Wehrmann A. (2003). Biogenic and taphonomic processes affecting the development of shell assemblages: an actuopaleontological case study from mussel banks on North Sea tidal flats. Facies 49, 19–30. doi: 10.1007/s10347-003-0022-1
Widdows J., Brinsley M. D., Salkeld P. N., Elliott M. (1998). Use of annular flumes to determine the influence of current velocity and bivalves on material flux at the sediment-water interface. Estuaries. 21, 552–559. doi: 10.2307/1353294
Zardus J. D. (2021). A global synthesis of the correspondence between epizoic barnacles and their sea turtle hosts. Integr. Org. Biol. 3, obab002. doi: 10.1093/iob/obab002
Keywords: facilitation, Lanice conchilega, Mytilus edulis, sand mason worm, mussel, foundation species, ecosystem engineers, tidal flats
Citation: Nauta J, Meijer KJ, de Groot LW, Reijers VC, Bouma TJ, van der Wal D, Olff H, Holthuijsen SJ, Bijleveld AI, van der Veer HW, Franken O and Govers LL (2024) Mutual facilitation between foundation species Mytilus edulis and Lanice conchilega promotes habitat heterogeneity on tidal flats. Front. Mar. Sci. 11:1354009. doi: 10.3389/fmars.2024.1354009
Received: 11 December 2023; Accepted: 22 March 2024;
Published: 10 April 2024.
Edited by:
Ibon Galparsoro, Technological Center Expert in Marine and Food Innovation (AZTI), SpainReviewed by:
Friederike Bungenstock, Lower Saxony Institute for Historical Coastal Research, GermanyCopyright © 2024 Nauta, Meijer, de Groot, Reijers, Bouma, van der Wal, Olff, Holthuijsen, Bijleveld, van der Veer, Franken and Govers. This is an open-access article distributed under the terms of the Creative Commons Attribution License (CC BY). The use, distribution or reproduction in other forums is permitted, provided the original author(s) and the copyright owner(s) are credited and that the original publication in this journal is cited, in accordance with accepted academic practice. No use, distribution or reproduction is permitted which does not comply with these terms.
*Correspondence: Janne Nauta, amFubmUubmF1dGFAZ21haWwuY29t
†ORCID: Janne Nauta, orcid.org/0000-0001-6368-775X
Kasper J. Meijer, orcid.org/0000-0002-3904-2510
Lissie W. de Groot, orcid.org/0009-0002-2449-3703
Valérie C. Reijers, orcid.org/0000-0002-7781-5019
Tjeerd J. Bouma, orcid.org/0000-0001-7824-7546
Daphne van der Wal, orcid.org/0000-0002-2319-056X
Han Olff, orcid.org/0000-0003-2154-3576
Sander J. Holthuijsen, orcid.org/0000-0002-7454-6933
Allert I. Bijleveld, orcid.org/0000-0002-3159-8944
Henk W. van der Veer, orcid.org/0000-0001-5035-661X
Oscar Franken, orcid.org/0000-0001-8361-3117
Laura L. Govers, orcid.org/0000-0003-4532-9419
Disclaimer: All claims expressed in this article are solely those of the authors and do not necessarily represent those of their affiliated organizations, or those of the publisher, the editors and the reviewers. Any product that may be evaluated in this article or claim that may be made by its manufacturer is not guaranteed or endorsed by the publisher.
Research integrity at Frontiers
Learn more about the work of our research integrity team to safeguard the quality of each article we publish.