- 1Department of Science, Roma Tre University, Rome, Italy
- 2National Biodiversity Future Center, Palermo, Italy
The Arctic and Antarctica represent two of the most inhospitable and poorly investigated biomes in the world. Although polar regions are still perceived as some of the most pristine places still in existence, these remote places are no longer immune to anthropogenic pollution, in particular, micro- and nanoplastics. Seabirds, avian species feeding mainly at sea, are indicators of change in the environment and represent an early study group of ecological indicators for plastic pollution. The goal of this bibliometric overview is to evaluate international research trends on the impacts of microplastics (MPs) and nanoplastics (NPs) on seabirds inhabiting polar regions. A total of at least 13 seabird species were reported to have ingested MPs from 1983–2023. Overall, 1130 samples were investigated, including stomach content, pouch content, guano, and pellets. Pellets were the most investigated substrate (699), followed by stomach contents (309), guano (101), and pouch contents (21). A median of 31.5 MPs per sample was found in the Arctic, with an average of 7.2 MPs per sample. A median of 35 MPs per sample was found in Antarctica, with an average of 1.1 MPs per sample. Overall, MPs were most frequently found in fragment form. A total of 3526 MPs were retrieved from stomachs (3013), pellets (398), guano (75), and pouch contents (40). Polyethylene was the dominant plastic polymer found, followed by polypropylene and polystyrene. The monitoring of MP ingestion is crucial to mitigating the impacts on marine and terrestrial organisms. Standardized protocols could boost the safeguarding of seabirds and reduce the impacts of MPs on polar regions.
1 Introduction
Polar regions, alongside desert biomes, represent the most inhospitable and uninhabited places for humans (Anisimov et al., 2007). The Arctic, covering approximately 6% of the Earth’s surface, is considered a well-preserved ecosystem, distant from the industrial and agricultural pathways of lower latitudes (Donaldson et al., 2010). However, recently, the Arctic has been undergoing unprecedented change, being exposed to a wide range of human pressures of local, regional, and global origin (Townhill et al., 2022). The North Sea Route is gaining notoriety as an alternative maritime commercial route connecting the Atlantic and Pacific Oceans (Buixadé Farré et al., 2014). Furthermore, the Arctic is under heavy pressure from ongoing ocean warming and escalating human intervention, such as industrial fisheries (Christiansen et al., 2014) and oil and gas platforms (Young et al., 2020). Unfortunately, it is still one of the most poorly understood biomes in the world (Colella et al., 2020). Antarctica is the most isolated continent in the world (Verleyen et al., 2021), characterized by extreme climatic and ecological conditions (Koerich et al., 2023). This continent represents the largest freshwater reservoir in the world, playing an important role in the ecological balance of the globe and influencing the atmospheric and oceanic circulation (da Silva et al., 2023). Despite the increasing recent tourism fluxes (Tejedo et al., 2022) and human activities, the Artic and Antarctica are still perceived as two of the most pristine places still in existence (Gross, 2022); in particular, commercial activities in Antarctica have a lower impact on biota than those in the Arctic, resulting in a better conservation status regarding human pressures (Dibbern, 2010). The extreme cold temperatures, the dry lands, and the difficulty humans face in accessing these regions make the Arctic and Antarctica remote places, characterized by areas with high biodiversity values (Shaw et al., 2014).
Life on Earth is threatened daily by many anthropogenic pressures, including the emission of toxic chemicals and materials produced by human activities (Chown et al., 2022; Cordero et al., 2022; Akhtar et al., 2021; Folke et al., 2021). The main anthropogenic pollutants affecting the Earth’s ecosystems can be summarized as air pollutants, derived from the combustion of fossil fuels (coal, oil, and gas); water pollutants, Häder et al., 2020 i.e., chemicals or other foreign substances, such as fertilizers and pesticides/insecticides/herbicides from agricultural runoff, heavy metals, and chemical wastes from industrial discharges; and soil pollutants, like potential trace elements (PTEs) and organic pollutants (Arihilam and Arihilam, 2019). Among these, plastic represents a widely distributed anthropogenic pollutant (Windsor et al., 2019), which is a global threat affecting all continents and ecosystems, and polar regions are no exception (Mishra et al., 2021). Even though polar regions are remote (Chu et al., 2019), they are no longer immune to anthropogenic pollution (Cunningham et al., 2020). While the Southern Ocean is isolated from the input of lower latitude contaminants by oceanic circulation (Chu et al., 2019), the Arctic Ocean is impacted by water inflow from the Atlantic Ocean (Hänninen et al., 2021). Atlantic inflow is one of the biggest sources of microplastic in the Arctic (Berezina et al., 2023), and consequently, plastic debris is mainly transported from remote areas (Cózar et al., 2017) by oceanic circulation or derived from local fishing activities (Tošić et al., 2020).
The Arctic and Antarctica have been impacted since the 13th century (Silva-Sánchez et al., 2015; McConnell et al., 2021) by anthropogenic pollution, long before the first human commercial activities reached these lands. The first direct sources of pollution in Antarctica started in 1790 with commercial sealing and whaling in the Southern Ocean, followed by the construction of scientific research stations (75 in 2019) and oil tanks to refill ships, fisheries, tourism, and military presence (da Silva et al., 2023). Although the human presence in these continents is limited, research stations represent one of the largest forms of anthropogenic activity and are the main source of locally derived contamination in Antarctica (Lo Giudice et al., 2019; Stark et al., 2023). Annually, there are approximately 5000 national operator staff working in Antarctica (Hughes and Convey, 2020). Most of the Antarctic research stations are in ice-free areas within 5 km of the coast, which represent only a small part of the total land area of the continent (Poland et al., 2003) but are very rich in terms of biodiversity (Shaw et al., 2014). Moreover, more than 39,000 tourists visit this continent every year (Chu et al., 2019), looking for adventures in unexplored wild places (da Silva et al., 2023). In the last years, these continents have also lost important masses of ice, becoming ever more vulnerable to climate change (Stokes et al., 2022). Furthermore, available data suggest that many anthropogenic pollutants, in particular plastic, could reach the Arctic and Antarctica via Long-range Atmospheric Transport (LRAT) from other continents (Bargagli, 2008; Bard, 1999) and lower latitudes (Ikenoue et al., 2023b). Ikenoue et al. (2023a; 2023b) estimated the microplastic input in these remote regions at 5236 ± 6127 pieces km−2 for the Chukchi Sea and 7570 ± 7600 pieces km−2 for the Beaufort Sea (Arctic), highlighting an important plastic contamination issue originating both from local sources and ocean-water transport.
Plastic pollution in polar regions is an emerging threat, and the studies on this topic have increased over the last decades (Caruso et al., 2022). According to van Emmerik and Schwarz (2020), plastics are classified based on their size: macroplastics (> 5 cm) mesoplastics (5 mm – 5 cm), microplastics (0.1 μm – 5 mm), and nanoplastic (< 0.1 μm). Such remote regions represent potential model environments to understand the mechanisms and interactions between microplastics, nanoplastics, and biota (Hwengwere et al., 2022; Rota et al., 2022). The smallest fragments, derived from the degradation of larger plastics, can have several effects on biota, including blockage of the gastrointestinal tract of animals, translocation from the intestines into other tissues, particle toxicity, oxidative stress, and immune response (Besseling et al., 2019). Anthropogenic environmental pollution in polar regions has led to a recognition of the potential negative impacts on the organisms living there (Mishra et al., 2021). Seabirds, avian species that feed mainly at sea, are indicators of change in the environment and represent an early study group of ecological indicators for plastic pollution (Orlando-Bonaca et al., 2022). Indeed, plastic ingestion by seabirds is a large-scale problem affecting remote areas (Baak et al., 2020). Plastic ingestion is correlated with human activities: the more shipping activities increase, the more seabirds ingest plastics (Baak et al., 2020). Seabirds have been declining globally in recent years, particularly in polar regions (Descamps and Ramírez, 2021). To date, there are 64 seabird species inhabiting the Arctic (described as “seabirds” for their breeding distribution, due to the lack of a univocal definition) (Descamps and Ramírez, 2021). In Antarctica, there are 43 species of seabirds, 38 of them living both on land and at sea: 7 species of penguin; 2 of petrels; 2 of cormorants; and 5 species of gulls, skuas, and terns (Woods et al., 2009). In total, 43 Important Bird Areas were then identified in Antarctica, based on bird concentration across the continent (Harris et al., 2011). Threats to seabirds are represented by two types of biological interactions with plastic: entanglement and ingestion (Golubev et al., 2020). The effect of plastic ingestion on seabirds has been of concern. This concern is due to the high frequency of ingestion of plastic by seabirds and because of the emerging evidence of both impacts on bird general health and the biomagnification of toxic chemicals, which can influence mortality or reproduction (Wilcox et al., 2015).
In this review, we provide a synthesis of the peer-reviewed literature published from the late 1980s to 2023 reporting the ingestion of micro- and nanoplastics by seabirds from polar regions. This work documents prominent trends in research topics and methods, the kinds of plastic sources that have been studied, the substrates and organic material analyzed, and the gaps and perspectives in research coverage that merit attention in future research. Then, we highlight how this information is important for seabird conservation and the implementation of meaningful mitigation measures. The goal of this bibliometric overview is to evaluate international research trends on the impacts of microplastics (MPs) and nanoplastics (NPs) on seabirds inhabiting polar regions.
2 Materials and methods
The Preferred Reporting Items for Systematic Reviews and Meta-Analyses (PRISMA) guidelines and protocol were applied for this systematic review (Figure 1) (Moher et al., 2009). In this study, the bibliographic database was built from sources extracted from Scopus and Web of Science (WOS). Bibliometric data in a 40-year time frame (1983–2023) were collected using the following keyword combinations from the Scopus and WOS website:
a) Microplastic AND “seabird” AND “Arctic”
b) Microplastic AND “bird” AND “Arctic”
c) Nanoplastic AND “seabird” AND “Arctic”
d) Nanoplastic AND “bird” AND “Arctic”
e) Microplastic AND “seabird” AND “Antarctica”
f) Microplastic AND “bird” AND “Antarctica”
g) Nanoplastic AND “seabird” AND “Antarctica”
h) Nanoplastic AND “bird” AND “Antarctica”
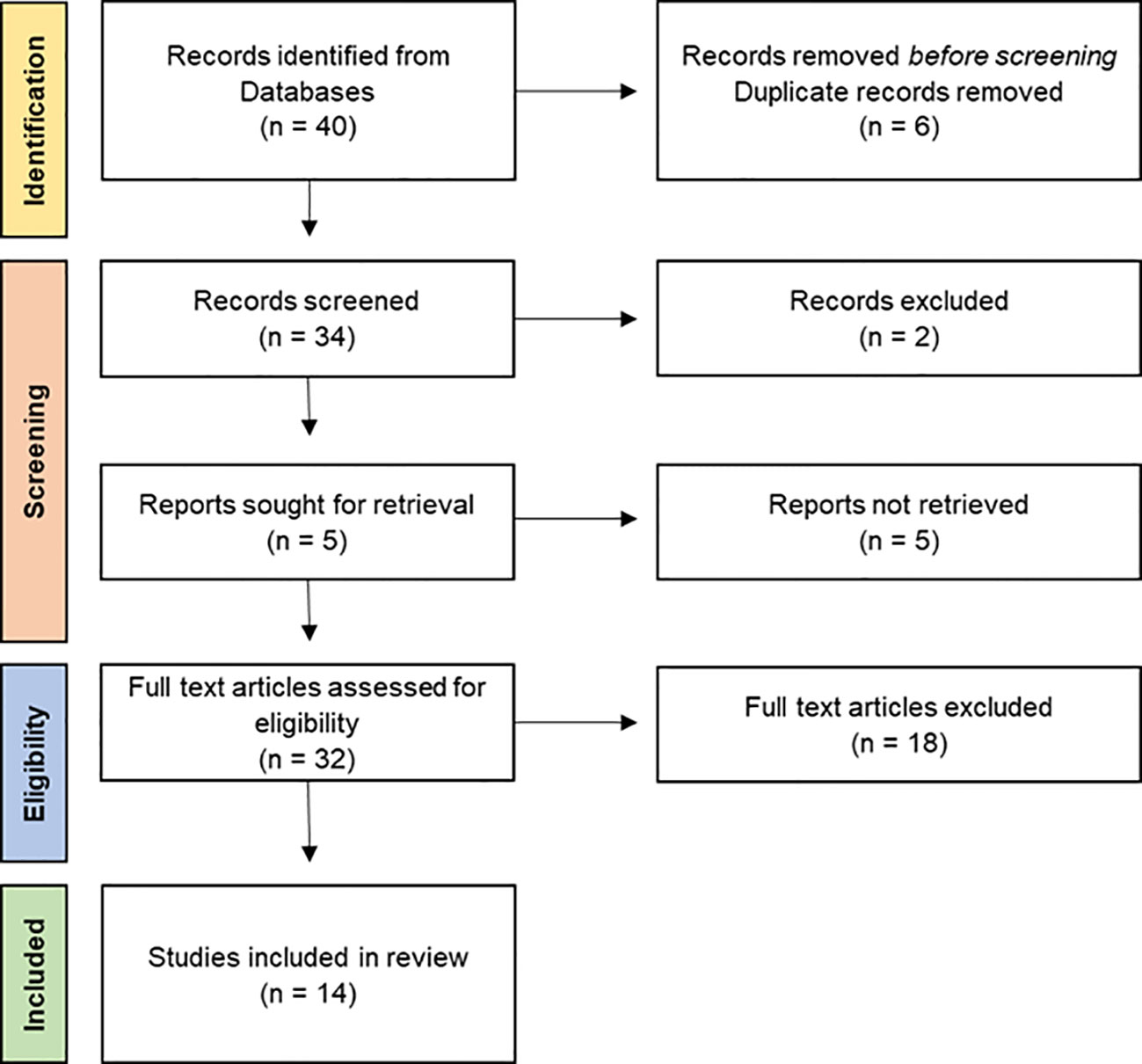
Figure 1 Flow diagram of the articles examined in the four stages of PRISMA. The numbers in brackets correspond to the number of articles included at each step. Source of PRISMA Flow Diagram: Moher et al. (2009).
The systematic review is structured in four stages: identification, screening, eligibility, and inclusion in the analysis. In the identification phase, all original publications were included. All the articles were screened, and information about title, year of publication, and DOI were collected. Firstly, duplicate articles were eliminated by a preliminary screening based on the title of the articles. From the analysis of the abstracts, articles about macro- and mesoplastic and seabirds not inhabiting the Arctic and Antarctica were excluded. Aiming to target the ingestion that occurred only under natural conditions in the marine and terrestrial environment, studies corresponding to MP and NP identification analyzing different biological comparts were included. Thus, studies based on the analysis of environmental matrices around bird nesting colonies and referring to terrestrial birds were also excluded. Papers on birds breeding in polar regions but inhabiting other continents were included. Throughout the eligibility stage, a full-text review was conducted, and the same exclusion criteria were applied. The collected information at this stage included (a) year of publication, (b) study area, (c) the organism (taxonomic groups, species, number of individuals, and MP and NP occurrences), (d) the research (research topic, methodological approach, and examined tissue), and (e) the MPs and NPs (number of total ingested MPs and NPs, MPs, and NPs per individual and description of the most frequently ingested type and polymer material). If the above-described information was grouped with findings of plastics greater than 5 mm in size or papers not showing plastic dimensions, it was excluded from the analysis. Information about the study sites and relative coordinates were taken from the paper body.
Online databases were used for the further collection of data related to the taxonomy (class and family) and the feeding behavior of the examined organism: Avibase (Lepage, 2021) and BirdLife Data Zone (BirdLife International Datazone, 2020). QGIS (QGIS Development Team, 2023) was used for mapping the distribution of sites.
3 Results
3.1 Description of the dataset
The primary search (identification stage) included 40 articles from 1983 to 2023, which mainly originated from the literature search on the Scopus database and WOS. Through the screening process and the application of the inclusion criteria, 14 articles were selected. Mainly, the articles were excluded because of a lack of data about plastic dimensions, because their focus was on plastics larger than 5 mm, or because they referred to birds not linked to the sea for reproductive strategies or foraging or birds not inhabiting the polar regions. Overall, 24 papers focused on meso- and macroplastics, highlighting an important discrepancy between the number of papers referring to these two-dimensional classes of plastics investigated. The first record of MP ingestion by seabird dates to 1983 (Furness, 1983), and the records have increased in the last 4 years, with 10 articles published since 2019 (5 for the Arctic, 6 for Antarctica). All the recorded articles refer to MP ingestion, excluding articles on macroplastic and mesoplastic ingestion, while there are no studies on NP ingestion by seabirds in polar areas.
3.2 Geographical distribution of study sites
Overall, 9 study sites were considered for the Arctic and 16 study sites were considered for Antarctica. The distribution of sites in the Arctic covers three countries: Canada (4), Denmark (1), and Norway (3). The distribution of sites in Antarctica covers one country: the United Kingdom (3). Other sampling sites in Antarctica are politically part of the continent (Table 1).
3.3 MP ingestion across seabirds
From 1983 to 2023, four seabird species from the Arctic and nine seabird species from Antarctica were analyzed under the scope of MP ingestion (Figure 2).
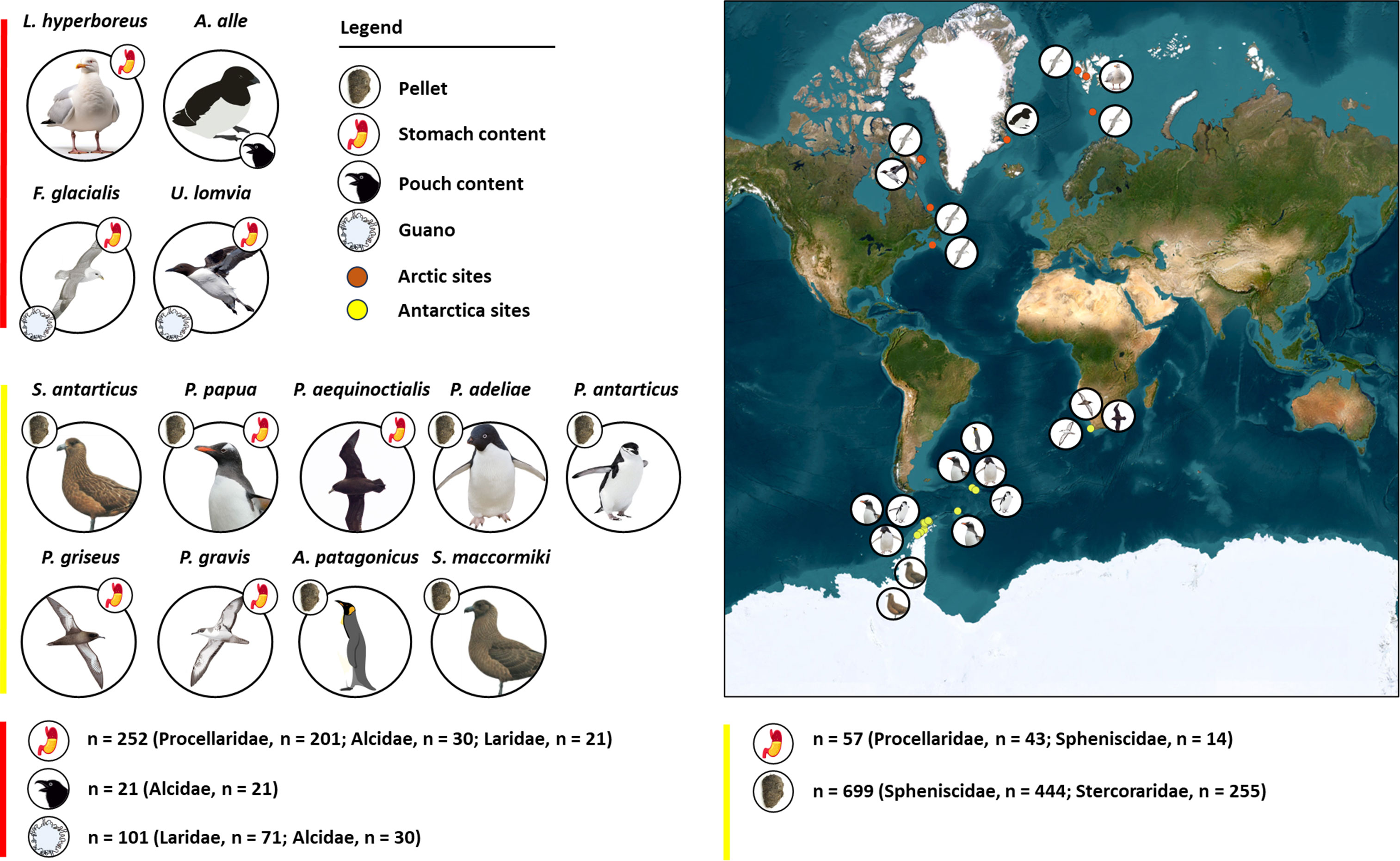
Figure 2 Global distribution of study sites and relative 13 species considered (red dots = Arctic sites; red line = Arctic species and samples; yellow dots = Antarctica sites; yellow line = Antarctica species and samples). For each species, the matrices analyzed are shown in a dot near the species’ picture. The articles considered analyzed pellets, stomach contents, pouch contents, and guano. The number of samples considered for each matrix is presented on the bottom, separated for the Arctic (red line) and Antarctica (yellow line).
In the Arctic, the species considered were Alle alle, Fulmarus glacialis, Larus hyperboreus, and Uria lomvia.
In Antarctica, the species considered were Aptenodytes patagonicus, Procellaria aequinoctialis, Puffinus gravis, Puffinus griseus, Pygoscelis adeliae, Pygoscelis antarcticus, Pygoscelis papua, Stercorarius antarcticus, and Stercorarius maccormicki.
All the species belong to five families: Alcidae, Laridae, Procellaridae, Spheniscidae, and Stercoraridae.
Overall, 1130 samples were investigated, including stomach content, pouch content, guano, and pellets. Stomach content represents the ingested compound of a bird present in its gastro-intestinal system at the moment of its death (Manko, 2016); pouch content is the food stored by little auks and few other birds on their foraging trips on a gular pouch located below the beak (Steen et al., 2007); guano is the complex excrement of seabirds, made of a mixture of food residues and metabolic waste products, with uric acid as the main component (De La Peña-Lastra, 2021); pellets are regurgitations of indigestible food (Jordan, 2005). Pellets were the most investigated substrate (699), followed by stomach contents (309), guano (101), and pouch contents (21). Studies on MPs in stomach contents date back to 1983, while investigations on MPs in pellets were first recorded in 2019.
Among the 374 samples investigated in the Arctic, 90% (all except 30) had at least one piece of MP in their content; 82% of stomach contents had MPs (Table 2). Pellets were not considered in studies in the Arctic. A median of 31.5 MPs per sample were found in the Arctic, with an average of 7.2 MPs per sample.

Table 2 Species considered, relative family, number of articles, number of samples, and relative number of MPs found (in brackets) for each biological matrix considered in the Arctic (N, number of studies considering the relative species).
For Antarctica, 756 birds (individuals) were considered, investigating 699 pellets and 57 stomach contents. Among the 756 samples investigated, 97% (all except 23) had at least one piece of MP in their content; 60% of stomach contents had MPs, while 100% of pellet samples contained MPs (Table 3). Guano and pouch content were not considered for studies in Antarctica. A median of 35 MPs per sample were found in Antarctica, with an average of 1.1 MPs per sample.
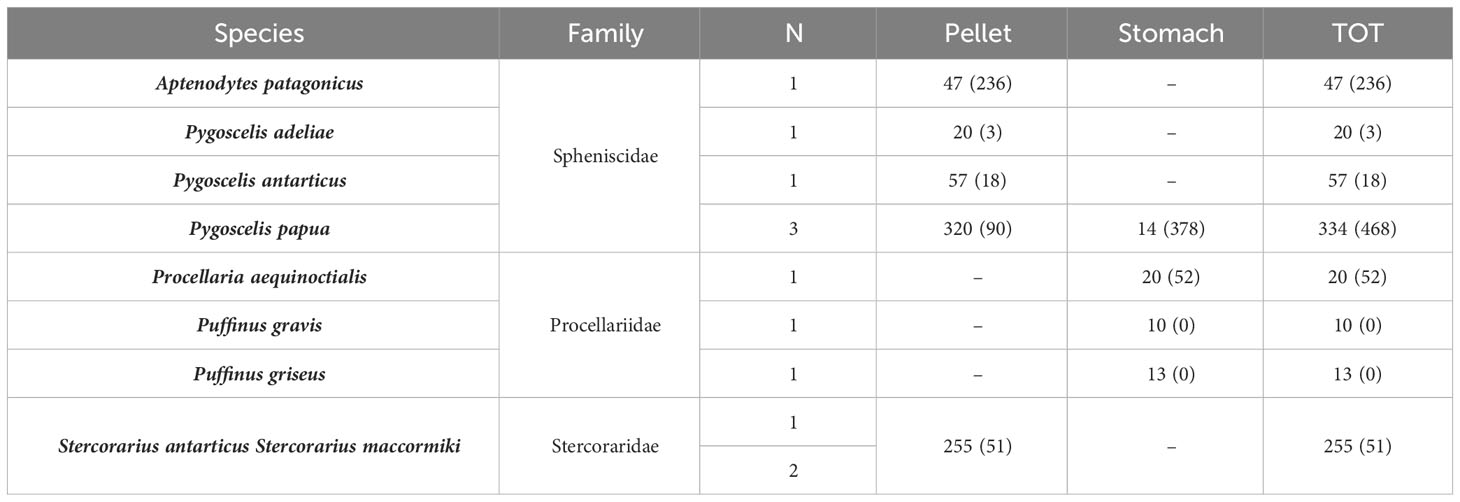
Table 3 Species considered, relative family, number of articles, number of samples, and relative number of MPs found (in brackets) for each biological matrix considered in Antarctica (N, number of studies considering the relative species).
3.4 Ingested MPs characterization
Overall, 3523 MPs were retrieved from stomachs, pellets, guano, and pouch contents. The number of MPs per sample was highly variable, with an average of 3.1 MPs per bird (min = 0, max = 36). Regarding MP shape, fragments were the dominant shape, making up 79%, while fibers accounted for 21% (Figure 3). Most of the fragments (Arctic, F.O. (Frequency of Occurrence) = 99%, n = 1687; Antarctic, F.O. = 79%, n = 308) were found in stomach contents, while the fibers were mainly found on pellets (Antarctica, F.O. = 81%, n = 315).
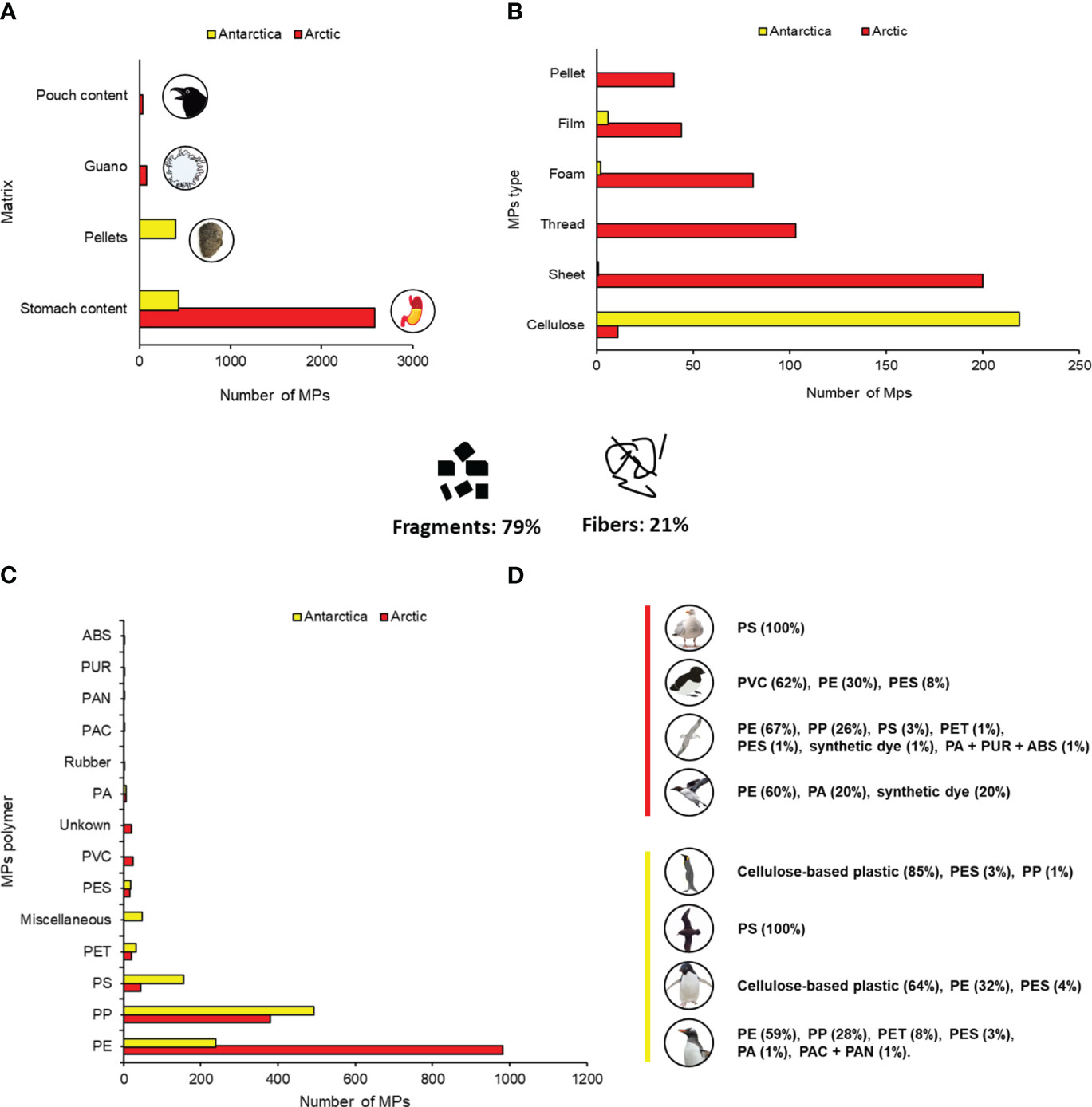
Figure 3 Number of MPs found in each matrix (A), number of MPs for each type of plastic (B), number of MP polymers (C), and percentage of MP polymers for each bird (D) (red line = Arctic species and polymers; yellow line = Antarctica species and polymers). PS, Polystyrene; PP, Polypropylene; PET, Polyethylene terephthalate; PA, Polyamide; PES, Polyester; PE, Polyethylene; PVC, Polyvinyl chloride; PUR, Polyurethane; ABS, Acrylonitrile butadiene styrene; PAC, Polyacrilate; PAN, Polyacrylonitrile.
Regarding MP type, cellulose-based plastic was the dominant type of plastic, making up 32%, followed by sheet (28%), plastic thread (15%), foam (12%), film (7%), and pellet (6%). Overall, 14 plastic polymers were identified and classified; in addition, two classes were created, called “miscellaneous” and “unknown”, to categorize, respectively, mixed polymers and unidentified polymers (some of the articles lacked information about the characterization of plastic polymers and/or did not perform an identification). Polystyrene (PS), polypropylene (PP), polyethylene terephthalate (PET), polyamide (PA), polyethylene (PE), and polyester (PES) were the polymers found in both the Arctic and Antarctica samples. Some MP polymers were not identified due to the author’s choice to analyze only a subsample or because of the impossibility of identifying them (small dimension of MPs, creation of a subsample, etc.); in this case, MP polymers were classified as “unknown” and were not reported in our results.
Summarizing the findings of the analysis, in the Arctic, PE was the only polymer to be found in all the sample types considered (stomach content, guano, pouch content), showing higher occurrence in Fulmarus glacialis (n = 987), Alle alle (n = 12) and Uria lomvia (n = 3); PES was also found in all the samples, showing lower frequencies (Fulmarus glacialis, n = 13; Alle alle, n = 3). PP was only found in Fulmarus glacialis (n = 380), while PVC was only found in Alle alle pouches (n = 24). PET was only found in Fulmarus glacialis stomach contents (Table 4).
In Antarctica, our results show that PE was the most occurring polymer in Spheniscidae (n = 238), while PES was only found in Spheniscidae (Genera Pygoscelis, n = 12; Gen. Aptenodytes, n = 6). PP, PE, and PES were the only polymers found both in stomach contents and pellets. PAC, PAN, PA, and PET were only found in Pygoscelis Papua (Table 5).
4 Discussions
4.1 Evaluation of the dataset
Under this review, the occurrence of MP ingestion by seabirds covered a period of 40 years. Although the number of publications on plastic ingestion by fauna has increased in recent years (Markic et al., 2020), the overall unseen growth of the research literature on our topic could be linked both with the still poorly studied emerging topic of seabird plastic contamination (Baak et al., 2020), the lacking identification of size and polymer type in plastic analysis by some publications, and the restricted knowledge about Arctic and Antarctic plastic pollution dynamics (Bergmann et al., 2022; Citterich et al., 2023). This study spans a period of 30 years (1983 – 2016): this data could be interpreted as an early, positive awareness of modern themes and actual conservation interests like the impact of plastic on seabirds. However, it could also be interpreted as a recent development of studies and research on MP ingestion by seabirds (Dehnhard et al., 2019; Roman et al., 2020), considering that only one study was published before 2016. The equal distribution of the number of studies in the polar regions (six for the Arctic, seven for Antarctica) reflects the comparable number of seabird species and that of scientific stations present in polar regions. There is no doubt that research on this topic is largely poor and needs to be boosted in the coming years to meet specific challenges from plastic pollution in remote regions (Eriksen et al., 2020). Thus, recent policies combined with the overall effort of the research community have highlighted the urgency of quantifying and monitoring the ingestion of MPs by marine fauna.
4.2 MP accumulation in seabirds compounds
To date, more than 100 seabird species inhabit polar regions (BirdLife International Datazone, 2020), and at least 13 species have been reported to have ingested MPs. MP ingestion was described for different species belonging to different families, some of them with similar evolutionary backgrounds: Alcidae, Laridae, and Stercoraridae belong to the Charadriiformes order, while Procellaridae belongs to the Procellariformes order and Spheniscidae belongs to the Sphenisciformes order (BirdLife International Datazone, 2020). Differences in biological compounds considered between the polar regions may be explained by the different species considered, differences in human population density, and the relative historical cultures. In the Arctic, stomach contents were considered three times more frequently than in Antarctica as a result of the presence of species highly adapted to marine life and swimming and with good flying abilities. Scientific activities considering this seabirds species can generate problems in birds catchment and to spot biological compounds like pellets. The analysis of the stomach content, sacrificing bird individuals, provide a complete overview of the MPs ingested. Moreover, many birds inhabiting the Arctic, like Fulmarus glacialis or Uria lomvia, use high cliffs as perches or as nesting places (Boertmann, 2023) and guano is easily recognizable. The absence of guano analysis on Laridae may be due to the low number of studies (n = 1) considering this species. It is also important to note that Arctic lands have a long history of indigenous peoples before and European colonizers after that were used to hunting seabirds (Winter et al., 2023); the destiny of the now-extinct Pinguinus impennis is emblematic of this (Hufthammer and Hufthammer, 2023). We hypothesize that this historical and cultural background alongside the relatively difficult-to-find seabird pellets (Barrett et al., 2007) has led to the use of hunting techniques to analyze MP ingestion in the Arctic rather than less invasive approaches. This hypothesis could also explain the differences observed in the data regarding the first records of MPs in stomach contents (1983) and pellets (2019), highlighting the recent development of MPs research techniques based on pellets. On the other hand, in Antarctica, a tendency has emerged to consider pellets as biological compounds to investigate MP accumulation rather than stomach contents. This result may be related to the high percentage of samples from Spheniscidae analyzed. Spheniscidae, represented by Aptenodytes patagonicus, Pygoscelis adeliae, Pygoscelis antarcticus, and Pigoscelis papua, are unable to fly (DeBlois and Motani, 2019) and are forced onto the mainland; their pellets are easy to recognize and can be used as a proxy for MP ingestion without sacrificing individual animals. Laridae and Stercorariidae are sister groups, sharing similar behavioral traits (Andersson, 1999); the different approaches to detecting MP accumulation (stomach contents vs. pellets) between the Arctic and Antarctica highlight how different scientific backgrounds regarding historical culture can influence approaches to investigating similar aims. At the same time, differences emerged between the occurrence of fragments, mainly found in stomach contents, and fibers, mainly found in pellets, highlighting a shift in these two approaches. Pellets are unlikely to represent the full plastic load of an individual and care must be taken in assessing plastic occurrence when using this technique (Provencher et al., 2017). Small pieces of plastics, like fragments, can potentially be lost to the environment (wind, sea waves) before collection, while fibers are likely to be incorporated into the intertwined structure of pellets. We also hypothesized that fibers, due to their thin and mouldable shape and structure, are easier to regurgitate by birds (Ghaffar et al., 2022) than fragments.
Hamilton (2021) demonstrated the similarity in MP accumulation between stomach contents and guano in Fulmarus glacialis. Bourdages (2021), on the contrary, found less MP accumulation in Fulmarus glacialis guano than in stomach contents (24 and 48 MP particles, respectively), while in Alle alle, only guano contained MPs. This difference in MP accumulation among similar biological matrices or different compounds in the same species may be due to different behavioral choices in foraging, different ecological preferences, and daily stochastic differences (Hoang and Mitten, 2022). In any case, given the relatively higher number of MPs found in pellets with respect to those in stomach contents and the non-invasive approach, we suggest, when possible, to analyze pellets instead of stomach contents (Duffy and Jackson, 1986). In other cases, when working with seabirds that are expert flyers, stomach content analysis is the easier or the only way to reach the aim of MP ingestion characterziation (Ryan and Jackson, 1986; Ryan, 1987). Fulmarus glacialis, in particular, tends not to regurgitate solid particles; as a consequence, its stomach contents indicate levels of pollution encountered by the bird over a precise period (Kühn and van Franeker, 2012).
In summary, we can suppose that the absence of non-flying seabirds in Arctic regions could have led to a focus on the analysis of the digestive tract of individual animals rather than pellets or guano. The pouch content analysis performed by Amélineau et al. (2016) on Alle alle individuals represents an interesting approach to investigating MP ingestion; despite the gentle handling and removal of pouch content, this must be considered an invasive method. However, this is the only technique used until now that has been able to characterize the exact number, dimension, and composition of plastic compounds, preventing fragmentation phenomena in the digestive tract (Karnovsky et al., 2012).
The total percentage of species that had ingested MPs was considered high, as almost 78% of the total examined species had ingested at least one MP item. It is also important to highlight that some studies included no significant findings, where MP ingestion was not detected, polymers were not identified, or only a subsample was considered, which may have affected the estimations (Marmara et al., 2023). In previous literature referring to plastic ingestion by seabirds of the genera Puffinus (Pierce et al., 2004; Acampora et al., 2014), data from Furness (1983) showing no MP ingestion in Puffinus griseus and Puffinus gravis are not explained by the author, also highlighting the similarity in feeding techniques between the representatives of that genus. The highest abundances of MPs came from ubiquitous (Fulmarus glacialis) and sedentary species (Pygoscelis papua) (Mallory, 2006; Bost and Jouventin, 1990). Fulmarus glacialis, considered a low concern for IUCN Red Lists (BirdLife International, 2018), is defined by the Oslo-Paris Convention (OSPAR) as a bioindicator for plastic pollution (Trevail et al., 2015), and the retrieved papers confirm the trend of this species to accumulate MPs mainly in their stomach contents. Pygoscelis papua is a top predator, considered a standard organism for monitoring plastic pollution in Antarctica (Bessa et al., 2019a). The data retrieved on MP accumulation confirm the effectiveness of this species as a bioindicator. Next to this species, our results evidence the effectiveness of Aptenodytes patagonicus as an indicator of MP accumulation, while Procellariidae efficacy was proven more frequently in the Arctic than in Antarctica. Fulmarus glacialis and Larus hyperboreus, two top predators in the Arctic food web (Bustnes et al., 2005) show similar ecology, breeding in close urban environments and foraging over coasts, bays, harbors, and inshore waters (Darby et al., 2021; Benjaminsen et al., 2022); however, MP abundances retrieved in this review don’t support this ecological overlapping, where a similar MP concentration was expected. Indeed, 13 MPs/sample for Fulmarus glacialis and 0.04 MPs/sample for Larus hyperboreus were detected, which is an important difference considering their similar foraging behavior.
4.3 MP polymer characterization
The sample size of the analyzed specimens could explain the high number of MPs found, although the ecological conditions and geographical isolation of polar regions could suggest a low level of MP accumulation (Corsi et al., 2020). Fragments were the most common type of MP in the Arctic, at 10 times higher than fibers, which is in line with previous studies (Hänninen et al., 2021). Fragments are often generated by the breakdown of larger plastic debris (Hallanger and Gabrielsen, 2018) and represent the most ingested type of MP by seabirds (Mallory, 2008; Baak et al., 2020). Fibers in the Arctic represent only a small percentage of the total types of MPs, which is in discordance with Marmara et al. (2023), where fibers were also the most common type among Aves. PE abundance in Arctic seabirds is in accordance with Bourdages et al. (2021) but not with Rodríguez-Torres et al. (2020), underling the affinity of birds with PE accumulation rather than other fauna. PET is a common thermoplastic widely used in commercial packages (Lionetto et al., 2021) and had a similar occurrence in the Arctic and Antarctica, impacting both Procellariidae and Spheniscidae. Kleptoparasitism in birds is well documented (Morand-Ferron et al., 2007), in particular in Laridae (Spencer et al., 2017). Many birds steal food from humans (Raghav and Boogert, 2022) or other birds (Morand-Ferron et al., 2007), with the associated risk of ingesting plastic from packaging. However, in such remote lands, it is difficult to identify the exact source of ingested plastic from seabirds; ingestion is higher in breeding birds, which can ingest high concentrations of plastics during migrations (Baak et al., 2020). In general, based on the data gained from the papers and the analysis conducted, it is clear that the use of standardized protocols for MP detection in seabirds could contribute to the exact quantification and comparison of MP ingestion. Analyzing the papers and data retrieved, a significant number of studies did not report some information considered necessary to categorize plastic polymers, and a complete overview is therefore difficult to show. Under this review, PE and PP were the most abundant polymers in Antarctica, found only in Pygoscelis Papua stomach contents. PE, mainly used in packaging, is the dominant pollutant polymer in the Mediterranean, followed by PP (Llorca et al., 2021). Zhang et al. (2017) found an inverse relationship between PP abundance and relative plastic dimensions in seas, suggesting an interesting trend in PP compounds to degrade in MPs. PE and PP are often present as marine aggregates, which deposit, after a certain period, at the bottom of the sea (Sharma et al., 2021). Pygoscelis papua takes shorter trips to forage for food than other species (e.g., Pygoscelis Adélie) (Williams and Rothery, 1990), suggesting that MP ingestion happened near the Antarctica coasts due to plastic pollution. Moreover, PE, PP, and PES were found in Antarctic krill, one of the main food resources of Pygoscelidae (Zhu et al., 2023). Krill represents an important limiting factor for the breeding success of Fulmarus glacialis, and data from the Arctic confirmed the high abundance of PE and PP in Fulmarus glacialis (Creuwels et al., 2007). The data retrieved suggest biomagnification of MPs through the food chain as the main driving force of MP ingestion. PS, found both in the Arctic and Antarctica, was ingested only by birds that can fly. Larus hyperboreus and Fulmarus glacialis are the two seabird species impacted by PS in the Arctic. The same ingestion trend was observed in Procellaria aequinoctialis in Antarctica. There is more evidence of PS ingestion by Laridae and Procellariidae in Europe (Nicastro et al., 2018; Ask et al., 2020); PS is a light plastic polymer, which tends to float on the water’s surface (Taurozzi et al., 2023). The observed occurrence of PS can be due to the trend of Laridae and Procellariidae to feed on small prey on the water’s surface, where plastics tend to float and accumulate, making them highly susceptible to plastic ingestion (Vanstreels et al., 2021).
Although the number of scientific papers related to MP ingestion is increasing and the gaps in knowledge about MP ingestion by seabirds have been partially bridged, the need for harmonized protocols, as well as increased focus on the impact of MPs on fauna from the polar regions remains. The use of a biological matrix instead of an alternative could create discrepancies in the correct evaluation of data: MPs found can be different in terms of abundance and the results can be different between sampling methods. Moreover, the use of established protocols for extraction and/or quantification of microplastics in biota, which can be categorized into two main classes, i.e. destructive and non-destructive methods, should be harmonized when studying comparable biological models (Bessa et al., 2019b). Stomach contents analysis is a highly sensitive method of detecting MP ingestion, more than pellets or guano, but it is a very invasive method. Here, we suggest evaluating MP concentration in seabirds using non-invasive methods or low-invasive methods, such as stomach flushing (Goldsworthy et al., 2016) or fecal analysis (Verkuil and Burg, 1996). The use of µFTIR or µRAMAN to identify MP polymers is a very informative and non-destructive approach (Bessa et al., 2019b). However, Independently of the selected protocol, monitoring programs need to be designed, allowing for comparisons across research teams and monitoring authorities and countries (Bessa et al., 2019b).
5 Conclusions
Under this review, MP ingestion in polar seabirds was examined in 13 species, and its impacts were confirmed for all the species, although with different levels of intensity. The most abundant MP shape was fragments, followed by fibers. The mean MPs ingested by individual animals was highly variable considering the different matrices analyzed: in any case, it was identified, on average, as 7.5 MPs/ind in the Arctic and 1.1 MPs/ind in Antarctica. Looking forward to the focus of the scientific community, the impact of MPs is expected to be further investigated. Moreover, temporal trends of papers on the impacts of MPs on polar fauna, in particular, seabirds, do not suggest a rapid increase of studies on this topic in recent future. The importance of seabirds as biological indicators and their crucial role as top predators in the polar food chains renders them in need of protection. The monitoring of MP ingestion is crucial to mitigating the impacts on marine and terrestrial organisms. Standardized protocols could boost the safeguarding of seabirds and polar regions. Furthermore, new analytical approaches should be developed considering all the stochastic and non-stochastic variables affecting seabird MP ingestion, as a valuable solution to mitigate the problem.
Data availability statement
The raw data supporting the conclusions of this article will be made available by the authors, without undue reservation.
Author contributions
DT: Conceptualization, Data curation, Writing – original draft, Writing – review & editing. MS: Conceptualization, Supervision, Validation, Writing – review & editing.
Funding
The author(s) declare that no financial support was received for the research, authorship, and/or publication of this article.
Acknowledgments
Dedicated to the memory of Paco, my best friend. Our souls will be together forever. We sincerely thank the editor and reviewers for taking the time to review our manuscript and providing constructive feedback to improve our manuscript. The authors acknowledge the support of NBFC to University of Roma Tre, funded by the Italian Ministry of University and Research, PNRR, Missione 4 Componente 2, “Dalla ricerca all' impresa”, Investimento 1.4, Project CN00000033.
Conflict of interest
The authors declare that the research was conducted in the absence of any commercial or financial relationships that could be construed as a potential conflict of interest.
The handling editor FC declared a past co-authorship with the author MS.
Publisher’s note
All claims expressed in this article are solely those of the authors and do not necessarily represent those of their affiliated organizations, or those of the publisher, the editors and the reviewers. Any product that may be evaluated in this article, or claim that may be made by its manufacturer, is not guaranteed or endorsed by the publisher.
References
Acampora H., Schuyler Q. A., Townsend K. A., Hardesty B. D. (2014). Comparing plastic ingestion in juvenile and adult stranded short-tailed shearwaters (Puffinus tenuirostris) in eastern Australia. Mar. pollut. Bull. 78, 63–68. doi: 10.1016/j.marpolbul.2013.11.009
Akhtar N., Syakir Ishak M. I., Bhawani S. A., Umar K. (2021). Various natural and anthropogenic factors responsible for water quality degradation: A review. Water 13, 2660. doi: 10.3390/w13192660
Amélineau F., Bonnet D., Heitz O., Mortreux V., Harding A. M., Karnovsky N., et al. (2016). Microplastic pollution in the Greenland Sea: Background levels and selective contamination of planktivorous diving seabirds. Environ. pollut. 219, 1131–1139. doi: 10.1016/j.envpol.2016.09.017
Andersson M. (1999). Phylogeny, behaviour, plumage evolution and neoteny in skuas Stercorariidae. J. Avian Biol. 30 (2), 205–215. doi: 10.2307/3677130
Anisimov O. A., Vaughan D. G., Callaghan T. V., Furgal C., Marchant H., Prowse T. D., et al. (2007). Polar regions (arctic and antarctic). Climate change. 15, 653–685.
Arihilam N. H., Arihilam E. C. (2019). Impact and control of anthropogenic pollution on the ecosystem–A review. J. Bioscience Biotechnol. Discovery 4, 54–59. doi: 10.31248/JBBD2019.098
Ask A., Cusa M., Danielsen J., Wing Gabrielsen G. (2020). Plastic characterization in northern fulmars (Fulmarus glacialis). Nordic Council Ministers. doi: 10.6027/temanord2020-537
Avery-Gomm S., Provencher J. F., Liboiron M., Poon F. E., Smith P. A. (2018). Plastic pollution in the Labrador Sea: an assessment using the seabird northern fulmar Fulmarus glacialis as a biological monitoring species. Mar. Pollut. Bull. 127, 817–822. doi: 10.1016/j.marpolbul.2017.10.001
Baak J. E., Linnebjerg J. F., Barry T., Gavrilo M. V., Mallory M. L., Price C., et al. (2020). Plastic ingestion by seabirds in the circumpolar Arctic: a review. Environ. Rev. 28, 506–516. doi: 10.1139/er-2020-0029
Bard S. M. (1999). Global transport of anthropogenic contaminants and the consequences for the Arctic marine ecosystem. Mar. pollut. Bull. 38, 356–379. doi: 10.1016/S0025-326X(99)00041-7
Bargagli R. (2008). Environmental contamination in Antarctic ecosystems. Sci. Total Environ. 400 (1-3), 212–226. doi: 10.1016/j.scitotenv.2008.06.062
Barrett R. T., Camphuysen K., Anker-Nilssen T., Chardine J. W., Furness R. W., Garthe S., et al. (2007). Diet studies of seabirds: a review and recommendations. ICES J. Mar. Sci. 64, 1675–1691. doi: 10.1093/icesjms/fsm152
Benjaminsen S. C., Bourgeon S., Herzke D., Ask A., Collard F., Gabrielsen G. W. (2022). First documentation of plastic ingestion in the arctic glaucous gull (Larus hyperboreus). Sci. Total Environ. 834, 155340. doi: 10.1016/j.scitotenv.2022.155340
Berezina A., Pakhomova S., Zhdanov I., Mekhova O., Polivanova T., Novikov M., et al. (2023). Surface microplastics in the kara sea: from the kara gate to the 83 N. doi: 10.3389/fmars.2023.1268879
Bergmann M., Collard F., Fabres J., Gabrielsen G. W., Provencher J. F., Rochman C. M., et al. (2022). Plastic pollution in the arctic. Nat. Rev. Earth Environ. 3, 323–337. doi: 10.1038/s43017-022-00279-8
Bessa F., Frias J., Kögel T., Lusher A., Andrade J. M., Antunes J., et al. (2019b). Harmonized protocol for monitoring microplastics in biota. JPI-Oceans BASEMAN project.
Bessa F., Ratcliffe N., Otero V., Sobral P., Marques J. C., Waluda C. M., et al. (2019a). Microplastics in gentoo penguins from the Antarctic region. Sci. Rep. 9, 14191. doi: 10.1038/s41598-019-50621-2
Besseling E., Redondo-Hasselerharm P., Foekema E. M., Koelmans A. A. (2019). Quantifying ecological risks of aquatic micro-and nanoplastic. Crit. Rev. Environ. Sci. Technol. 49, 32–80. doi: 10.1080/10643389.2018.1531688
BirdLife International (2018) Fulmarus glacialis (The IUCN Red List of Threatened Species 2018) (Accessed 15 November 2023).
BirdLife International (2020) BirdLife international Datazone. Available online at: http://datazone.birdlife.org/.
Boertmann D. (2023). Re-establishment of an extinct breeding colony of Brünnich’s Guillemot Uria lomvia in West Greenland. Seabird 35.
Bost C. A., Jouventin P. I. E. R. R. E. (1990). Evolutionary ecology of gentoo penguins (Pygoscelis papua). Penguin Biol., 85–112. doi: 10.1016/B978-0-08-057106-5.50011-5
Bourdages M. P., Provencher J. F., Baak J. E., Mallory M. L., Vermaire J. C. (2021). Breeding seabirds as vectors of microplastics from sea to land: evidence from colonies in Arctic Canada. Sci. Total Environ. 764, 142808. doi: 10.1016/j.scitotenv.2020.142808
Buixadé Farré A., Stephenson S. R., Chen L., Czub M., Dai Y., Demchev D., et al. (2014). Commercial Arctic shipping through the Northeast Passage: routes, resources, governance, technology, and infrastructure. Polar Geogr. 37, 298–324. doi: 10.1080/1088937X.2014.965769
Bustnes J. O., Miland Ø., Fjeld M., Erikstad K. E., Skaare J. U. (2005). Relationships between ecological variables and four organochlorine pollutants in an artic glaucous gull (Larus hyperboreus) population. Environ. pollut. 136, 175–185. doi: 10.1016/j.envpol.2004.09.026
Caruso G., Bergami E., Singh N., Corsi I. (2022). Plastic occurrence, sources, and impacts in Antarctic environment and biota. Water Biol. Secur. 1, 100034. doi: 10.1016/j.watbs.2022.100034
Chown S. L., Leihy R. I., Naish T. R., Brooks C. M., Convey P., Henley B. J., et al. (2022). Antarctic climate change and the environment: A decadal synopsis and recommendations for action. Faculty of science, medicine and health - papers: part B. Scientific Committee on Antarctic Research, Cambridge, United Kingdom.
Christiansen J. S., Mecklenburg C. W., Karamushko O. V. (2014). Arctic marine fishes and their fisheries in light of global change. Global Change Biol. 20, 352–359. doi: 10.1111/gcb.12395
Chu W. L., Dang N. L., Kok Y. Y., Yap K. S. I., Phang S. M., Convey P. (2019). Heavy metal pollution in Antarctica and its potential impacts on algae. Polar Sci. 20, 75–83. doi: 10.1016/j.polar.2018.10.004
Citterich F., Giudice A. L., Azzaro M. (2023). A plastic world: A review of microplastic pollution in the freshwaters of the Earth’s poles. Sci. Total Environ. 869, 161847. doi: 10.1016/j.scitotenv.2023.161847
Colella J. P., Talbot S. L., Brochmann C., Taylor E. B., Hoberg E. P., Cook J. A. (2020). Conservation genomics in a changing Arctic. Trends Ecol. Evol. 35, 149–162. doi: 10.1016/j.tree.2019.09.008
Collard F., Strøm H., Fayet M. O., Guðmundsson F. Þ., Herzke D., Hotvedt Å., et al. (2023). Evaluation of meso-and microplastic ingestion by the northern fulmar through a non-lethal sampling method. Mar. pollut. Bull. 196, 115646. doi: 10.1016/j.marpolbul.2023.115646
Cordero R. R., Sepúlveda E., Feron S., Damiani A., Fernandoy F., Neshyba S., et al. (2022). Black carbon footprint of human presence in Antarctica. Nat. Commun. 13, 984. doi: 10.1038/s41467-022-28560-w
Corsi I., Bergami E., Caruso G. (2020). Special issue plastics in polar regions. Environ. Int. Vol. No. 149. doi: 10.1016/j.envint.2020.106203
Cózar A., Martí E., Duarte C. M., García-de-Lomas J., Van Sebille E., Ballatore T. J., et al. (2017). The Arctic Ocean as a dead end for floating plastics in the North Atlantic branch of the Thermohaline Circulation. Sci. Adv. 3, e1600582. doi: 10.1126/sciadv.1600582
Creuwels J. C., Poncet S., Hodum P. J., van Franeker J. A. (2007). Distribution and abundance of the Southern Fulmar Fulmarus glacialoides. Polar Biol. 30, 1083–1097. doi: 10.1007/s00300-007-0276-0
Cunningham E. M., Ehlers S. M., Dick J. T., Sigwart J. D., Linse K., Dick J. J., et al. (2020). High abundances of microplastic pollution in deep-sea sediments: evidence from Antarctica and the Southern Ocean. Environ. Sci. Technol. 54, 13661–13671. doi: 10.1021/acs.est.0c03441
Darby J. H., De Grissac S., Arneill G. E., Pirotta E., Waggitt J. J., Börger L., et al. (2021). Foraging distribution of breeding northern fulmars is predicted by commercial fisheries. Mar. Ecol. Prog. Ser. 679, 181–194. doi: 10.3354/meps13887
da Silva J. R. M. C., Bergami E., Gomes V., Corsi I. (2023). Occurrence and distribution of legacy and emerging pollutants including plastic debris in Antarctica: Sources, distribution and impact on marine biodiversity. Mar. pollut. Bull. 186, 114353. doi: 10.1016/j.marpolbul.2022.114353
DeBlois M. C., Motani R. (2019). Flipper bone distribution reveals flexible trailing edge in underwater flying marine tetrapods. J. Morphology 280, 908–924. doi: 10.1002/jmor.20992
Dehnhard N., Herzke D., Gabrielsen G. W., Anker-Nilssen T., Ask A., Christensen-Dalsgaard S., et al. (2019). Seabirds as Indicators of Distribution, Trends and Population Level Effects of Plastics in the Arctic Marine Environment (Norsk institutt for naturforskning (NINA) Workshop Report).
De La Peña-Lastra S. (2021). Seabird droppings: Effects on a global and local level. Sci. Total Environ. 754, 142148. doi: 10.1016/j.scitotenv.2020.142148
Descamps S., Ramírez F. (2021). Species and spatial variation in the effects of sea ice on Arctic seabird populations. Diversity Distributions 27, 2204–2217. doi: 10.1111/ddi.13389
Dibbern J. S. (2010). Fur seals, whales and tourists: a commercial history of Deception Island, Antarctica. Polar Rec. 46, 210–221. doi: 10.1017/S0032247409008651
Donaldson S. G., Van Oostdam J., Tikhonov C., Feeley M., Armstrong B., Ayotte P., et al. (2010). Environmental contaminants and human health in the Canadian Arctic. Sci. Total Environ. 408, 5165–5234. doi: 10.1016/j.scitotenv.2010.04.059
Duffy D. C., Jackson S. (1986). Diet Studies of Seabirds: a Review of Methods (Waterbird Society) 9 (1), 1–17.
Eriksen M., Borgogno F., Villarrubia-Gómez P., Anderson E., Box C., Trenholm N. (2020). Mitigation strategies to reverse the rising trend of plastics in Polar Regions. Environ. Int. 139, 105704. doi: 10.1016/j.envint.2020.105704
Folke C., Polasky S., Rockström J., Galaz V., Westley F., Lamont M., et al. (2021). Our future in the Anthropocene biosphere. Ambio 50, 834–869. doi: 10.1007/s13280-021-01544-8
Fragão J., Bessa F., Otero V., Barbosa A., Sobral P., Waluda C. M., et al. (2021). Microplastics and other anthropogenic particles in Antarctica: Using penguins as biological samplers. Sci. Total Environ. 788, 147698. doi: 10.1016/j.scitotenv.2021.147698
Furness B. L. (1983). Plastic particles in three procellariiform seabirds from the Benguela Current, South Africa. Mar. pollut. Bull. 14, 307–308. doi: 10.1016/0025-326X(83)90541-6
Ghaffar I., Rashid M., Akmal M., Hussain A. (2022). Plastics in the environment as potential threat to life: an overview. Environ. Sci. pollut. Res. 29, 56928–56947. doi: 10.1007/s11356-022-21542-x
Goldsworthy B., Young M. J., Seddon P. J., van Heezik Y. (2016). Stomach flushing does not affect apparent adult survival, chick hatching, or fledging success in yellow-eyed penguins (Megadyptes antipodes). Biol. Conserv. 196, 115–123. doi: 10.1016/j.biocon.2016.02.009
Golubev S. (2020). Macroplastic in seabirds at Mirny, Antarctica. Birds (Lond.) 1, 13–18. doi: 10.3390/birds1010003
Gross M. (2022). Pristine Antarctica exposed to change. Curr. Biol. 32, R247–R249. doi: 10.1016/j.cub.2022.03.020
Häder D. P., Banaszak A. T., Villafañe V. E., Narvarte M. A., González R. A., Helbling E. W. (2020). Anthropogenic pollution of aquatic ecosystems: Emerging problems with global implications. Sci. Total Environ. 713, 136586. doi: 10.1016/j.scitotenv.2020.136586
Hamilton B. M., Bourdages M. P., Geoffroy C., Vermaire J. C., Mallory M. L., Rochman C. M., et al. (2021). Microplastics around an Arctic seabird colony: particle community composition varies across environmental matrices. Sci. Total Environ. 773, 145536. doi: 10.1016/j.scitotenv.2021.145536
Hänninen J., Weckström M., Pawłowska J., Szymańska N., Uurasjärvi E., Zajaczkowski M., et al. (2021). Plastic debris composition and concentration in the Arctic Ocean, the North Sea and the Baltic Sea. Mar. pollut. Bull. 165, 112150. doi: 10.1016/j.marpolbul.2021.112150
Harris C. M., Carr R., Lorenz K., Jones S. (2011). Important Bird Areas in Antarctica: Antarctic Peninsula, South Shetland Islands, South Orkney Islands–Final Report (Cambridge: Prepared for BirdLife International and the Polar Regions Unit of the UK Foreign & Commonwealth Office. Environmental Research & Assessment Ltd.), 4.
Hoang T. C., Mitten S. (2022). Microplastic accumulation in the gastrointestinal tracts of nestling and adult migratory birds. Sci. Total Environ. 838, 155827. doi: 10.1016/j.scitotenv.2022.155827
Hufthammer A. K., Hufthammer K. O. (2023). The great auk in Norway: From common to locally extinct. Int. J. Osteoarchaeology 33, 588–597. doi: 10.1002/oa.3161
Hughes K. A., Convey P. (2020). Implications of the COVID-19 pandemic for Antarctica. Antarctic Sci. 32, 426–439. doi: 10.1016/j.jenvman.2023.119200
Hwengwere K., Paramel Nair H., Hughes K. A., Peck L. S., Clark M. S., Walker C. A. (2022). Antimicrobial resistance in Antarctica: is it still a pristine environment? Microbiome 10 (1), 1–13. doi: 10.1186/s40168-022-01250-x
Ikenoue T., Nakajima R., Fujiwara A., Onodera J., Itoh M., Toyoshima J., et al. (2023a). Horizontal distribution of surface microplastic concentrations and water-column microplastic inventories in the Chukchi Sea, western Arctic Ocean. Sci. Total Environ. 855, 159564. doi: 10.1016/j.scitotenv.2022.159564
Ikenoue T., Nakajima R., Mishra P., Ramasamy E. V., Fujiwara A., Nishino S., et al. (2023b). Floating microplastic inventories in the southern Beaufort Sea, Arctic Ocean. Front. Mar. Sci. 10. doi: 10.3389/fmars.2023.1288301
Jordan M. J. R. (2005). Dietary analysis for mammals and birds: a review of field techniques and animal-management applications. Int. Zoo Yearbook 39, 108–116. doi: 10.1111/j.1748-1090.2005.tb00010.x
Karnovsky N. J., Hobson K. A., Iverson S. J. (2012). From lavage to lipids: estimating diets of seabirds. Mar. Ecol. Prog. Ser. 451, 263–284. doi: 10.3354/meps09713
Kerry K. R., Riddle M. J. (Eds.). (2009). Health of Antarctic wildlife: a challenge for science and policy. (New York: Springer) 2009, 1–10.
Kim Y., Kim H., Jeong M. S., Kim D., Kim J., Jung J., et al. (2023). Microplastics in gastrointestinal tracts of gentoo penguin (Pygoscelis papua) chicks on King George Island, Antarctica. Sci. Rep. 13, 13016. doi: 10.1038/s41598-023-39844-6
Koerich G., Fraser C. I., Lee C. K., Morgan F. J., Tonkin J. D. (2023). Forecasting the future of life in Antarctica. Trends Ecol. Evol. 38, 24–34. doi: 10.1016/j.tree.2022.07.009
Kühn S., van Franeker J. A. (2012). Plastic ingestion by the northern fulmar (Fulmarus glacialis) in Iceland. Mar. pollut. Bull. 64, 1252–1254. doi: 10.1016/j.marpolbul.2012.02.027
Le Guen C., Suaria G., Sherley R. B., Ryan P. G., Aliani S., Boehme L., et al. (2020). Microplastic study reveals the presence of natural and synthetic fibres in the diet of King Penguins (Aptenodytes patagonicus) foraging from South Georgia. Environ. Int. 134, 105303. doi: 10.1016/j.envint.2019.105303
Lenzi J., Bresesti F., Lozoya J. P., De Feo B., Krojmal E., Lacerot G., et al. (2022). Diet and debris ingestion of skuas on fildes peninsula, king george island, Antarctica. Mar. pollut. Bull. 185, 114211. doi: 10.1016/j.marpolbul.2022.114211
Lepage D. (2021) Avibase-The World Bird Database [WWW Document]. Available online at: https://avibase.bsc-eoc.org/checklist.jsp.
Lionetto F., Esposito Corcione C., Rizzo A., Maffezzoli A. (2021). Production and characterization of polyethylene terephthalate nanoparticles. Polymers 13, 3745. doi: 10.3390/polym13213745
Llorca M., Vega-Herrera A., Schirinzi G., Savva K., Abad E., Farré M. (2021). Screening of suspected micro (nano) plastics in the Ebro Delta (Mediterranean Sea). J. hazardous materials 404, 124022. doi: 10.1016/j.jhazmat.2020.124022
Lo Giudice A., Caruso G., Rizzo C., Papale M., Azzaro M. (2019). Bacterial communities versus anthropogenic disturbances in the Antarctic coastal marine environment. Environ. Sustainability 2, 297–310. doi: 10.1007/s42398-019-00064-2
Mallory M. L. (2006). The northern fulmar (Fulmarus glacialis) in Arctic Canada: ecology, threats, and what it tells us about marine environmental conditions. Environ. Rev. 14, 187–216. doi: 10.1139/a06-003
Mallory M. L. (2008). Marine plastic debris in northern fulmars from the Canadian high Arctic. Mar. pollut. Bull. 56, 1501–1504. doi: 10.1016/j.marpolbul.2008.04.017
Manko P. (2016). Stomach content analysis in freshwater fish feeding ecology (University of Prešov), 116.
Markic A., Gaertner J. C., Gaertner-Mazouni N., Koelmans ,. A. A. (2020). Plastic ingestion by marine fish in the wild. Crit. Rev. Environ. Sci. Technol. 50, 657–697. doi: 10.1080/10643389.2019.1631990
Marmara D., Katsanevakis S., Brundo M. V., Tiralongo F., Ignoto S., Krasakopoulou E. (2023). Microplastics ingestion by marine fauna with a particular focus on commercial species: a systematic review. Front. Mar. Sci. doi: 10.3389/fmars.2023.1240969
McConnell J. R., Chellman N. J., Mulvaney R., Eckhardt S., Stohl A., Plunkett G., et al. (2021). Hemispheric black carbon increase after the 13th-century Māori arrival in New Zealand. Nature 598, 82–85. doi: 10.1038/s41586-021-03858-9
Mishra A. K., Singh J., Mishra P. P. (2021). Microplastics in polar regions: an early warning to the world’s pristine ecosystem. Sci. Total Environ. 784, 147149. doi: 10.1016/j.scitotenv.2021.147149
Moher D., Liberati A., Tetzlaff J., Altman D. G. (2009). Research methodes and reporting. Bmj 8, 332–336. doi: 10.1136/bmj.b2535.
Morand-Ferron J., Sol D., Lefebvre L. (2007). Food stealing in birds: brain or brawn? Anim. Behav. 74, 1725–1734. doi: 10.1016/j.anbehav.2007.04.031
Nicastro K. R., Savio R. L., McQuaid C. D., Madeira P., Valbusa U., Azevedo F., et al. (2018). Plastic ingestion in aquatic-associated bird species in southern Portugal. Mar. pollut. Bull. 126, 413–418. doi: 10.1016/j.marpolbul.2017.11.050
Orlando-Bonaca M., Avio C. G., Bonanno G. (2022). “Marine organisms as bioindicators of plastic pollution,” in Plastic Pollution and Marine Conservation (Academic Press), 187–248). doi: 10.1016/B978-0-12-822471-7.00003-1
Pierce K. E., Harris R. J., Larned L. S., Pokras M. A. (2004). Obstruction and starvation associated with plastic ingestion in a Northern Gannet Morus bassanus and a Greater Shearwater Puffinus gravis. Mar. Ornithology 32, 187–189.
Poland J. S., Riddle M. J., Zeeb B. A. (2003). Contaminants in the Arctic and the Antarctic: a comparison of sources, impacts, and remediation options. Polar Records 39, 369–383. doi: 10.1017/S0032247403002985
Provencher J. F., Bond A. L., Avery-Gomm S., Borrelle S. B., Rebolledo E. L. B., Hammer S., et al. (2017). Quantifying ingested debris in marine megafauna: a review and recommendations for standardization. Analytical Methods 9, 1454–1469. doi: 10.1039/C6AY02419J
Provencher J. F., Vermaire J. C., Avery-Gomm S., Braune B. M., Mallory M. L. (2018). Garbage in guano? Microplastic debris found in faecal precursors of seabirds known to ingest plastics. Sci. Total Environ. 644, 1477–1484. doi: 10.1016/j.scitotenv.2018.07.101
QGIS Development Team (2023). QGIS Geographic Information System (Switzerland: Open Source Geospatial Foundation Project). Available at: http://qgis.osgeo.org.
Raghav S., Boogert N. J. (2022). Factors associated with Herring Gulls Larus argentatus stealing food from humans in coastal towns. Bird Study 69, 103–108. doi: 10.1080/00063657.2022.2162846
Rodríguez-Torres R., Almeda R., Kristiansen M., Rist S., Winding M. S., Nielsen T. G. (2020). Ingestion and impact of microplastics on arctic Calanus copepods. Aquat. Toxicol. 228, 105631. doi: 10.1016/j.aquatox.2020.105631
Roman L., Hardesty B. D., Hindell M. A., Wilcox C. (2020). Disentangling the influence of taxa, behaviour and debris ingestion on seabird mortality. Environ. Res. Lett. 15, 124071. doi: 10.1088/1748-9326/abcc8e
Rota E., Bergami E., Corsi I., Bargagli R. (2022). Macro-and microplastics in the Antarctic environment: ongoing assessment and perspectives. Environments 9, 93. doi: 10.3390/environments9070093
Ryan P. G. (1987). The incidence and characteristics of plastic particles ingested by seabirds. Mar. Environ. Res. 23, 175–206. doi: 10.1016/0141-1136(87)90028-6
Sharma S., Sharma V., Chatterjee S. (2021). Microplastics in the Mediterranean Sea: sources, pollution intensity, sea health, and regulatory policies. Front. Mar. Sci. 8. doi: 10.3389/fmars.2021.634934
Shaw J. D., Terauds A., Riddle M. J., Possingham H. P., Chown S. L. (2014). Antarctica’s protected areas are inadequate, unrepresentative, and at risk. PloS Biol. 12, e1001888. doi: 10.1371/journal.pbio.1001888
Silva-Sánchez N., Schofield J., Mighall T., Martínez Cortizas A., Edwards K., Foster I. (2015). Climate changes, lead pollution and soil erosion in south Greenland over the past 700 years. Quaternary Res. 84, 159–173. doi: 10.1016/j.yqres.2015.06.001
Spencer R., Russell Y. I., Dickins B. J., Dickins T. E. (2017). Kleptoparasitism in gulls Laridae at an urban and a coastal foraging environment: an assessment of ecological predictors. Bird Study 64, 12–19. doi: 10.1080/00063657.2016.1249821
Stark J. S., Johnstone G. J., King C., Raymond T., Rutter A., Stark S. C., et al. (2023). Contamination of the marine environment by Antarctic research stations: Monitoring marine pollution at Casey station from 1997 to 2015. PloS One 18, e0288485. doi: 10.1371/journal.pone.0288485
Steen H., Vogedes D., Broms F., Falk-Petersen S., Berge J. (2007). Little auks (Alle alle) breeding in a High Arctic fjord system: bimodal foraging strategies as a response to poor food quality? Polar Res. 26, 118–125. doi: 10.1111/j.1751-8369.2007.00022.x
Stokes C. R., Abram N. J., Bentley M. J., Edwards T. L., England M. H., Foppert A., et al. (2022). Response of the East Antarctic Ice Sheet to past and future climate change. Nature 608 (7922), 275–286. doi: 10.1038/s41586-022-04946-0
Taurozzi D., Cesarini G., Scalici M. (2023). Epiplastic microhabitats for epibenthic organisms: a new inland water frontier for diatoms. Environ. Sci. pollut. Res. 30, 17984–17993. doi: 10.1007/s11356-022-23335-8
Tejedo P., Benayas J., Cajiao D., Leung Y. F., De Filippo D., Liggett D. (2022). What are the real environmental impacts of Antarctic tourism? Unveiling their importance through a comprehensive meta-analysis. J. Environ. Manage. 308, 114634. doi: 10.1016/j.jenvman.2022.114634
Tošić T. N., Vruggink M., Vesman A. (2020). Microplastics quantification in surface waters of the Barents, Kara and White Seas. Mar. pollut. Bull. 161, 111745. doi: 10.1016/j.marpolbul.2020.111745
Townhill B. L., Reppas-Chrysovitsinos E., Sühring R., Halsall C. J., Mengo E., Sanders T., et al. (2022). Pollution in the Arctic Ocean: An overview of multiple pressures and implications for ecosystem services. Ambio 51, 471–483. doi: 10.1007/s13280-021-01657-0
Trevail A. M., Gabrielsen G. W., Kühn S., Van Franeker J. A. (2015). Elevated levels of ingested plastic in a high Arctic seabird, the northern fulmar (Fulmarus glacialis). Polar Biol. 38, 975–981. doi: 10.1007/s00300-015-1657-4
Tulatz F., Gabrielsen G. W., Bourgeon S., Herzke D., Krapp R., Langset M., et al. (2023). Implications of regurgitative feeding on plastic loads in northern fulmars (Fulmarus glacialis): A study from svalbard. Environ. Sci. Technol. 57, 3562–3570. doi: 10.1021/acs.est.2c05617
van Emmerik T., Schwarz A. (2020). Plastic debris in rivers. Wiley Interdiscip. Reviews: Water 7, e1398. doi: 10.1002/wat2.1398
Vanstreels R. E. T., Gallo L., Serafini P. P., Santos A. P., Egert L., Uhart M. M. (2021). Ingestion of plastics and other debris by coastal and pelagic birds along the coast of Espírito Santo, Eastern Brazil. Mar. pollut. Bull. 173, 113046. doi: 10.1016/j.marpolbul.2021.113046
Verkuil Y., Burg D. (1996). Stomach-pumping of waders does not necessarily provide more information on diet than faecal analysis. BULLETIN-WADER STUDY GROUP 79, 60–63.
Verleyen E., Van de Vijver B., Tytgat B., Pinseel E., Hodgson D. A., Kopalová K., et al. (2021). Diatoms define a novel freshwater biogeography of the Antarctic. Ecography 44, 548–560. doi: 10.1111/ecog.05374
Williams T. D., Rothery P. (1990). Factors affecting variation in foraging and activity patterns of gentoo penguins (Pygoscelis papua) during the breeding season at Bird Island, South Georgia. J. Appl. Ecol. 27 (3), 1042–1054. doi: 10.2307/2404394
Wilcox C., Van Sebille E., Hardesty B. D. (2015). Threat of plastic pollution to seabirds is global, pervasive, and increasing. Proceedings of the National Academy of Sciences. 112 (38), 11899–11904. doi: 10.1073/pnas.1502108112
Windsor F. M., Durance I., Horton A. A., Thompson R. C., Tyler C. R., Ormerod S. J. (2019). A catchment-scale perspective of plastic pollution. Global Change Biol. 25, 1207–1221. doi: 10.1111/gcb.14572
Winter K. B., Young R. C., Lyver P. O. B. (2023). Cultural aspects of seabird conservation. In Conservation of Marine Birds. Academic Press., 321–344. doi: 10.1016/B978-0-323-88539-3.00008-X
Young O. R., Berkman P. A., Vylegzhanin A. N. (2020). Governing Arctic Seas: Regional Lessons from the Bering Strait and Barents Sea Vol. 358 (Springer Nature Switzerland AG 202: Springer).
Zhang W., Zhang S., Wang J., Wang Y., Mu J., Wang P., et al. (2017). Microplastic pollution in the surface waters of the Bohai Sea, China. Environ. pollut. 231, 541–548. doi: 10.1016/j.envpol.2017.08.058
Keywords: Arctic, Antarctica, stomach contents, guano, pellet, pouch content, polyethylene, fragments
Citation: Taurozzi D and Scalici M (2024) Seabirds from the poles: microplastics pollution sentinels. Front. Mar. Sci. 11:1343617. doi: 10.3389/fmars.2024.1343617
Received: 23 November 2023; Accepted: 08 February 2024;
Published: 06 March 2024.
Edited by:
Fabiana Corami, National Research Council (CNR), ItalyReviewed by:
Takahito Ikenoue, Japan Agency for Marine-Earth Science and Technology (JAMSTEC), JapanAnna Vesman, Arctic and Antarctic Research Institute (AARI), Russia
Copyright © 2024 Taurozzi and Scalici. This is an open-access article distributed under the terms of the Creative Commons Attribution License (CC BY). The use, distribution or reproduction in other forums is permitted, provided the original author(s) and the copyright owner(s) are credited and that the original publication in this journal is cited, in accordance with accepted academic practice. No use, distribution or reproduction is permitted which does not comply with these terms.
*Correspondence: Davide Taurozzi, davide.taurozzi@uniroma3.it