- 1Faculty of Biological and Environmental Sciences. University of Helsinki, Helsinki, Finland
- 2Faculty of Bioeconomy, Novia University of Applied Sciences, Ekenäs, Finland
- 3Helsinki University Lipidomics Unit (HiLIPID), Helsinki Institute of Life Science (HiLIFE) and Biocenter Finland, Helsinki, Finland
- 4Molecular and Integrative Biosciences Research Programme, Faculty of Biological and Environmental Sciences, University of Helsinki, Helsinki, Finland
Eutrophication, climate-induced warming, and salinity fluctuations are altering the fatty acid profiles and the availability of essential polyunsaturated fatty acids (PUFAs) in marine zooplankton communities. Limnocalanus macrurus Sars G.O., 1863 is a large calanoid copepod inhabiting the low-salinity areas in the Baltic Sea, where it is a major source of docosahexaenoic acid (DHA) and eicosapentaenoic acid (EPA) to commercially important fish. L. macrurus is sensitive to warming, eutrophication and hypoxia. As an opportunistic feeder, it is capable of dietary shifts, which affects its fatty acid profiles. Although much studied in boreal lakes, there are only a few studies on the fatty acid profiles of the Baltic Sea populations. This study aimed to compare the fatty acid profiles of L. macrurus in three basins of the Baltic Sea, in relation to the community fatty acids and environmental variables. We collected samples of L. macrurus and filtered plankton community for gas chromatographic fatty acid analyses in August 2021 on R/V Aranda. The nutritional quality of L. macrurus to consumers was lower in the Gulf of Finland (GoF) compared to the Gulf of Bothnia, indicated by the low levels of DHA and EPA, as well as the low n-3/n-6 ratio of PUFAs. The lower ratio of 18:1n-7 to 18:1n-9 implied higher degree of omnivory in GoF. In contrast, a diatom marker 16:1n-7 had high proportion in the Bothnian Bay. High temperatures in GoF may have restricted feeding in the upper water column, possibly forcing a shift towards cyanobacteria or seston-based diet, as interpreted from a high proportion of 18:2n-6 and 18:3n-3. We conclude that the ability of L. macrurus to utilize multiple food sources increases its resilience to environmental change, while the consequences on the nutritional quality may have further cascading effects on the food webs.
1 Introduction
Climate change-induced warming is challenging marine ecosystems globally by decreasing the availability of polyunsaturated fatty acids (PUFAs) from dietary lipids (Tan et al., 2022). The two physiologically most important PUFAs to all marine consumers are docosahexaenoic acid (22:6n-3, DHA) and eicosapentaenoic acid (20:5n-3, EPA). Both DHA and EPA are essential for lipid-protein interactions of cell membranes and therefore, all cellular functions (Lee et al., 2006; Parrish, 2013). Fatty acids synthesized by autotrophs often remain unchanged in food webs and thus, serve as dietary markers: 16:1n-7 and EPA are considered as markers for diatoms, and 18:4n-3 and DHA as markers for dinoflagellates (Dalsgaard et al., 2003). Fatty acids can also be used for indicating trophic level in the marine ecosystem. For instance, the ratio of 18:1n-7 to 18:1n-9 is considered as a marker for omnivory, while ratio of EPA to DHA typically decreases in higher trophic levels due to accumulation of DHA (Dalsgaard et al., 2003). Finally, nutritional quality of fatty acids profiles are interpreted from high ratios of n-3 to n-6 PUFAs and unsaturated (UFA) to saturated (SFA) fatty acids (Tan et al., 2022).
Temperature has direct effects on metabolic rates of aquatic organisms and fatty acid modifications in their membrane lipids to maintain fluidity and function (Parrish, 2013). Moreover, warming is shifting the plankton communities towards smaller size and thus, reduced individual lipid content (Daufresne et al., 2009; Mäkinen et al., 2017b; Renaud et al., 2018; Gorokhova, 2019), and towards increased heterotrophy, which decreases the availability of DHA and EPA (Dahlgren et al., 2011). The Baltic Sea is a large pool of brackish water, where salinity determines the species distribution and community structure (Diekmann et al., 2012). According to recent climate predictions, the increasing precipitation, river runoffs, and the salt water inflows through the Danish Straits induce salinity fluctuations (Meier et al., 2022). Moreover, shallow depth, low turnover rate and large catchment area relative to water volume make the Baltic Sea vulnerable to eutrophication, which decreases the availability of high-quality PUFAs to grazers through community shifts (Strandberg et al., 2022; Taipale et al., 2022). Along with eutrophication, some basins in the Baltic Sea suffer from hypoxia (Conley et al., 2011). The area of hypoxic sea floor has increased along with eutrophication (Carstensen et al., 2014). Low oxygen in deeper water layers forms a vertical migration barrier to species with high demand for oxygen (Webster et al., 2015).
Large, lipid-rich copepods are an important food source for fish in terms of food quality and quantity; they are rich in PUFAs, and they store energy densely in oil sacs or droplets in the form of wax esters and triacylglycerols (Lee et al., 2006). Planktivorous fish are size selective and thus, a large calanoid copepod Limnocalanus macrurus is a major food source for Baltic herring (Flinkman et al., 1992; Rajasilta et al., 2014; Rajasilta et al., 2019). L. macrurus is a cold-stenothermic copepod that dwells in deep water layers and is widely distributed in lake ecosystems, but also in the Baltic Sea, where it is considered as a glacial relict (Kane et al., 2004; Hiltunen et al., 2014; Einberg et al., 2019). It is an opportunistic omnivorous feeder: it preys on other copepods (Warren, 1985; Dahlgren et al., 2012) and utilizes phytoplankton blooms (Hirche et al., 2003; Mäkinen et al., 2017a). Weak tolerance to high temperatures makes L. macrurus vulnerable to climate change; high water temperatures in the upper water column may restrict its access to food (Mäkinen et al., 2017a), while hypoxia and eutrophication may further reduce its abundance (Kane et al., 2004). Although the low-oxygen layers in the water column may serve as refuges against predation (Webster et al., 2015), L. macrurus benefits physiologically from high oxygen and low temperature via increased antioxidant activity (Vuori et al., 2015). Previous studies have described the seasonality of the fatty acid profiles of L. macrurus in lakes (Hiltunen et al., 2014) and in the Gulf of Bothnia (Dahlgren et al., 2012; Mäkinen et al., 2017a), but large-scale spatial comparisons in the Baltic Sea are not conducted.
The main objectives of this work were to 1) investigate the nutritional quality of L. macrurus for consumers as assessed by fatty acids based on the essential PUFAs and their n-3/n-6 ratio, and 2) study the trophic status of the species by comparing the dietary fatty acid markers to the fatty acid profiles available in the plankton community. Hydrographical features of the sampling regions were considered as possible explanatory variables to the variation in the fatty acid profiles of L. macrurus between three basins in the Baltic Sea. We hypothesized that L. macrurus from areas with lower temperature and chl a (i.e., lower eutrophication) have higher nutritional quality to consumers, and that the dietary fatty acid markers of L. macrurus reflect those abundant in the fatty acid profiles of the plankton community. The vulnerability to environmental changes, importance to the community, and highly variable feeding behaviour make studies on L. macrurus relevant for understanding how lipids are affected by climate change in large scale.
2 Materials and methods
2.1 Field sampling
Zooplankton sampling took place in 7–13 August 2021 as part of the annual HELCOM COMBINE III expedition performed by the Finnish Environment Institute (SYKE) aboard of R/V Aranda (HELCOM, 2013). Zooplankton samples were obtained from five HELCOM monitoring stations: stations F2 and BO3 were located in Bothnian Bay (BB), US5B in the Bothnian Sea (BS), and LL3A and XV1 in the eastern Gulf of Finland (GoF) (Figure 1). WP2 plankton net (mesh size: 100 µm, diameter 57 cm) with a cod-end was hauled from 60 m; the net was closed 20 m below the surface during the light hours to avoid algae bloom in the sample. During dark hours, sampling continued to the surface, except for station XV1, where the sampling terminated at 10 m due to abundant cyanobacteria bloom at surface. We expected the target species L. macrurus to dwell in deeper waters during the day (Webster et al., 2015) and possibly ascend closer to surface at night, as copepods are known to perform diel vertical migration behavior (Lampert, 1989). The samples were immediately transferred to a cooling box with 10-µm-filtered sea water. A community sample was concentrated on an acidified and 450°C-combusted GF/F filter (Whatman). The community samples included all plankon in a size fraction of >100 µm; low amounts of phytoplankton were possibly included. The rest of the sample was stored in <10°C with continuous oxygenation using an aquarium pump. The storage time never exceeded 6 hours. For each replicate of a fatty acid sample (n=3–5), 15 mixed-gender individuals of L. macrurus were carefully picked with forceps into a 1.5-mL Eppendorf tube under a stereo microscope (Nikon SMZ800). The samples were kept on ice during the collection, which took approximately 10–15 minutes for each replicate; the ready samples were immediately snap-frozen in liquid nitrogen and stored in -80°C.
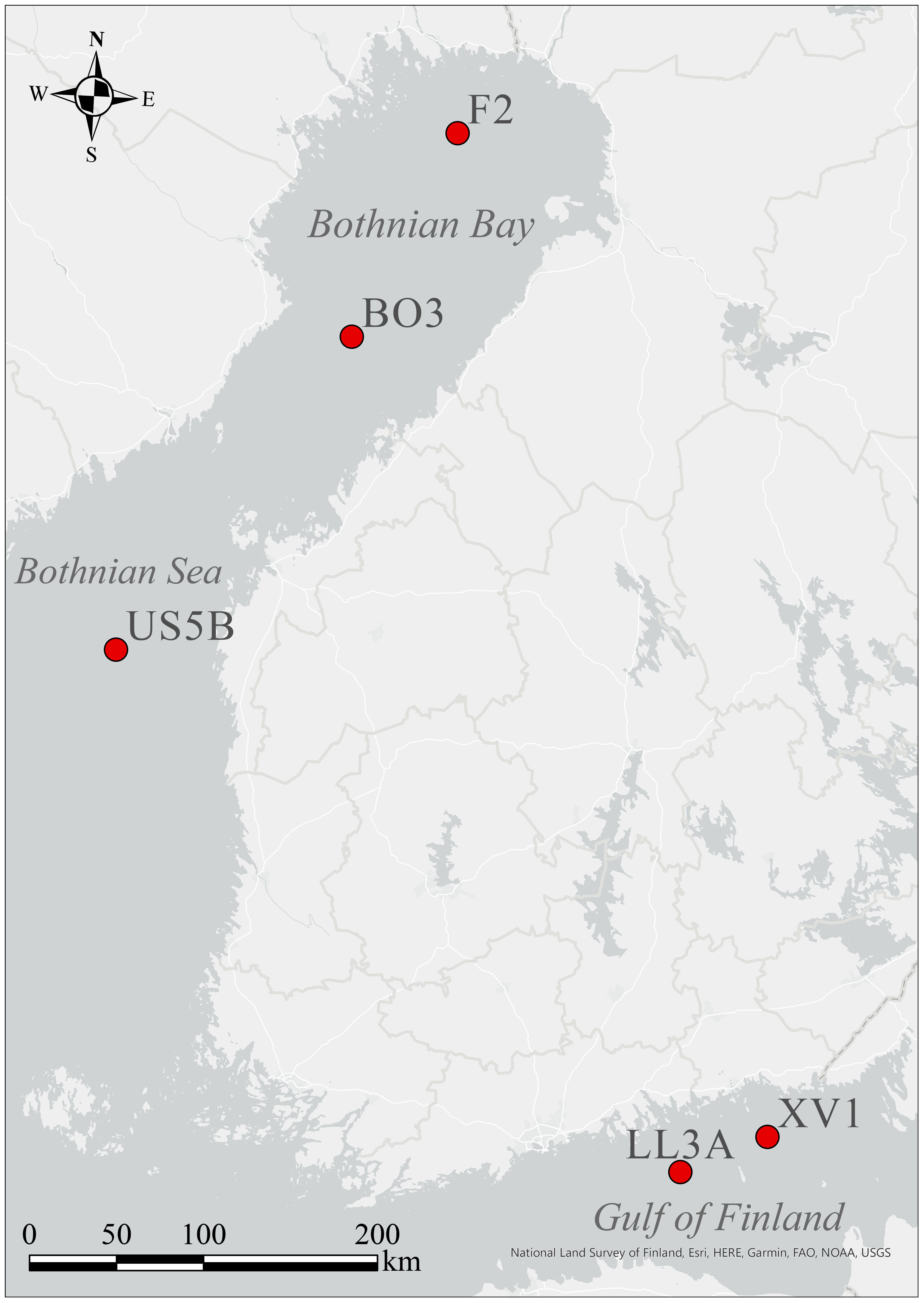
Figure 1 HELCOM monitoring stations used for sampling in 7–13 August 2021 during COMBINE III expedition aboard R/V Aranda in Bothnian Bay, Bothnian Sea and Gulf of Finland.
A zooplankton subsample was preserved in acid Lugol’s solution and later determined to species or genus level under the stereo microscope, using the identification key by Rajasilta and Vuorinen (2008). The relative abundance of zooplankton taxa were estimated as (+++) = highly abundant, (++) = abundant and (+) = present in low numbers, and used only for descriptive purposes. Environmental data were collected by SYKE and the Finnish Meteorological Institute from the entire expedition period as part of the annual HELCOM monitoring. Practical salinity (unitless), temperature and oxygen were measured by a SeaBird CTD sonde, and chlorophyll a (chl a) concentration and dissolved oxygen were measured from water collected from different depths using a Rosette serial bottle sampler.
2.2 Fatty acid analyses
The total lipids of L. macrurus and the community samples were converted to fatty acid methyl esters (FAME) using a direct transmethylation reaction according to the recommendations of Christie (1993): the samples were transferred into a solution of 1 mL hexane and 2 mL 1% H2SO4 in methanol and incubated in 95°C in a nitrogen atmosphere for 120 minutes. The produced FAMEs were then extracted into hexane, and the residual water was removed on sodium sulphate. Prior to gas chromatography, the FAMEs were concentrated to the volume of 200 µL hexane by evaporation under N2 flow. The FAMEs were identified based on their mass spectra recorded by GCMS-QP2010 Ultra (Shimadzu Scientific Instruments, Kyoto, Japan) which were compared with the spectra we have recorded for several authentic standard mixtures of FAME (including Supelco CRM47885, 47033, 47085-U, 47015-U and 47080-U that contain 37 components, marine source, menhaden oil, animal and bacterial FAMEs, respectively), and published reference mass spectra (Christie, 2023). FAMEs where then quantified with GC-FID (Shimadzu GC-2010 Plus with flame-ionization detector) using Zebron ZB-wax capillary columns (30 m, 0.25 mm ID and film thickness 0.25 µm; Phenomenex, Torrence CA, USA), and otherwise as detailed in Käkelä et al. (2005). Fatty acid compositions are expressed as molar percentages per total fatty acids (mol%) to give all fatty acids the same importance, irrespective of their structure and weight. Fatty acids were abbreviated: (carbon number):(number of double bonds) n-(position of the first double bond calculated from the methyl end) (e.g., 22:6n-3 for docosahexaenoic acid).
2.3 Statistical analyses
All statistical analyses were carried out using R version 4.2.1 (R Core Team, 2022), and package ggplot2 was used for graphics (Wickham, 2016). A Kruskal-Wallis test was used for analyzing differences of mol% of fatty acid trophic markers between sea basins, and further analysed with a Dunn’s test by using package dunn.test (Dunn, 1961; Dinno, 2017).
Principal component analysis (PCA) was run using the prcomp function in R, where the variables were scaled to unit variance and centered to zero. These variables included environmental variables (temperature, salinity and dissolved oxygen at 40 m, and chl a at 5 m) and selected fatty acid variables: ΣPUFA, DHA, EPA, 18:3n-3, 18:4n-3, 16:1n-7,18:1n-9, 18:2n-6, 20:4n-6, and Σbranched. L. macrurus was expected to dwell in deeper water columns; in order to compare stations, 40 m depth was chosen for comparing temperature, salinity, and dissolved oxygen between stations. However, the chl a concentration was selected from the depth with maximum chl a (5 m) to represent the eutrophication level of the sampling site; it is worth noting that L. macrurus usually dwell below the productive layer. The plot was created using ggbiplot package (Vu, 2011).
3 Results
3.1 Hydrographical conditions
All sampling sites had a strong thermocline, reached in approximately 10–15 m at station F2, but in 15–20 m in all other locations (Table 1). The average temperature of the upper 10 m was >18°C in GoF, which was higher than in BB (>16°C) and BS (17°C). Temperatures in GoF were also higher below the thermocline, 4.6°C in 40 m, while the temperature at the same depth was 2.5°C in BB and 3.9°C in BS. All sampling areas were characterized by low salinity: the surface salinity in GoF was 3.5 in XV1 and 5.0 in LL3A, while salinity ranged from 4.5 at the surface to 6.3 at the lowest measuring point in deeper water layers at station US5B. The lowest salinity was in BB, where the highest measured salinity was 3.6. The average concentration of chl a in 1–7.5 m was 1.9 µg L−1 in BB and BS, whereas it was 6.7 µg L−1 in the GoF. Hypoxia (dissolved O2 < 2 mL L−1) occurred at both stations in GoF (LL3A, and XV1).
3.2 Zooplankton community composition
The zooplankton communities in the Gulf of Bothnia were characterized by the dominance of Eurytemora affinis and L. macrurus, accompanied by equally high abundances of cladocerans, especially Bosmina coregoni (Table 2). Station BO3 had high abundance of rotifers Keratella spp. Calanoid copepod Acartia sp. was highly abundant at US5B, but absent in the BB. The GoF zooplankton was dominated by E. affinis, and also other copepods, such as L. macrurus, Pseudocalanus spp. and Acartia sp. were abundant. Rotifers Synchaeta spp. were abundant in GoF, while cladocerans were only present in low numbers.
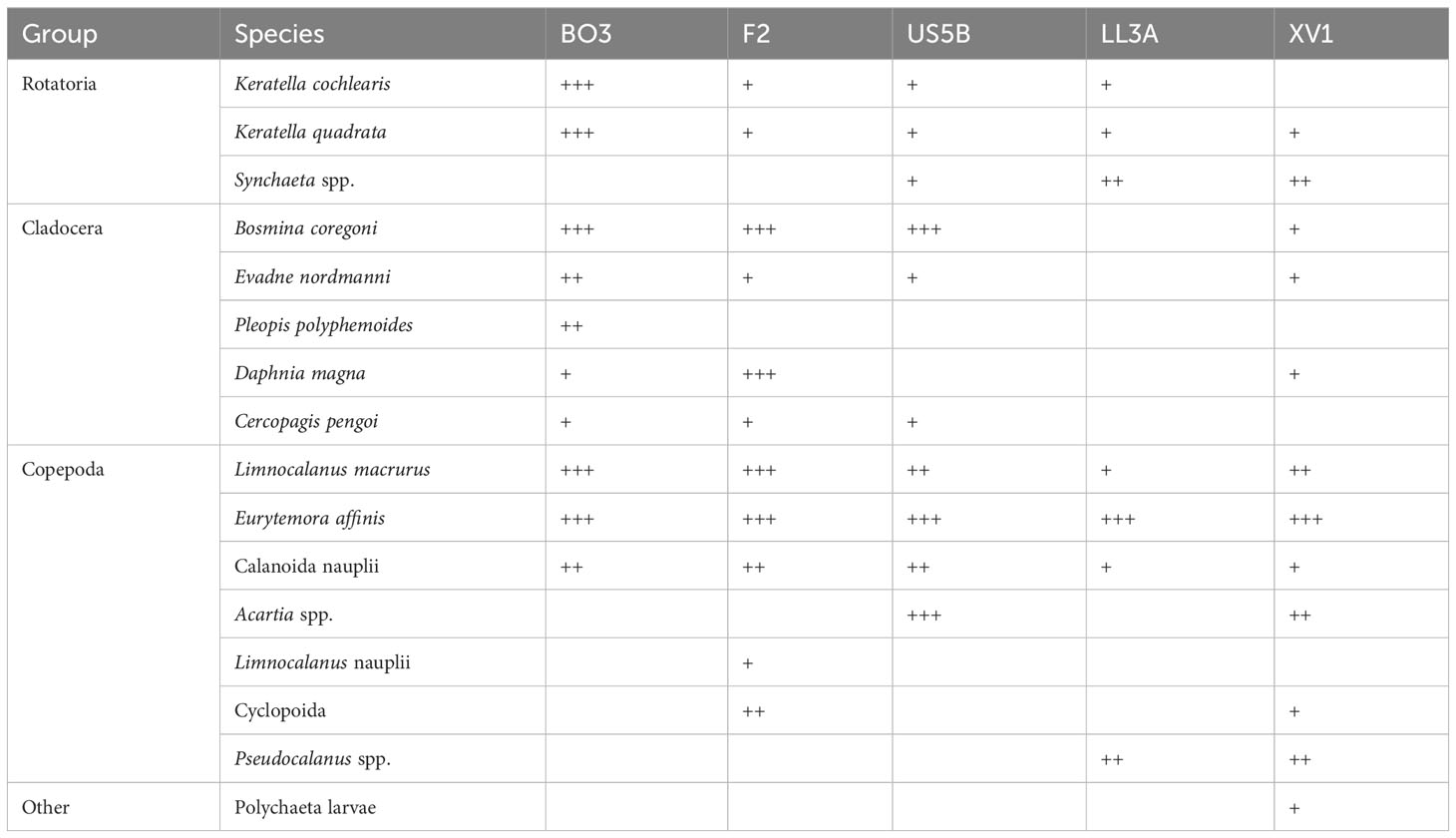
Table 2 Zooplankton community presented as relative abundances: (+++) = highly abundant, (++) = abundant and (+) = present in low numbers.
3.3 Fatty acid profiles of Limnocalanus macrurus
Fatty acid profiles of L. macrurus were dominated by DHA, EPA, 18:2n-6, 18:1n-9, and 16:0 (Figure 2A). The total PUFA-content of L. macrurus varied between 59 and 76 mol% in the whole study area, including 45–61 mol% n-3 family members. C18 PUFAs comprised 20 mol% of the total fatty acids. The proportion of C24 PUFAs varied between 0.5 and 15 mol% and comprised mostly of 24:4n-3 and 24:5n-3. Long-chain monounsaturated fatty acids were abundant: the highest proportions were found in 18:1n-9, 18:1n-7, and 24:1n-9. Additionally, 20:1n-9 and 22:1n-11 were found in low proportions, less than 1 mol%. The major saturated fatty acids were 16:0, 14:0, and 18:0. Branched fatty acids were rare, but their sum varied between 0.6 and 1.6 mol%. The main branched fatty acids were 4,8,12-trimethyl-tridecanoate, 15:0, 15:0iso, phytanate, and 14:0iso.
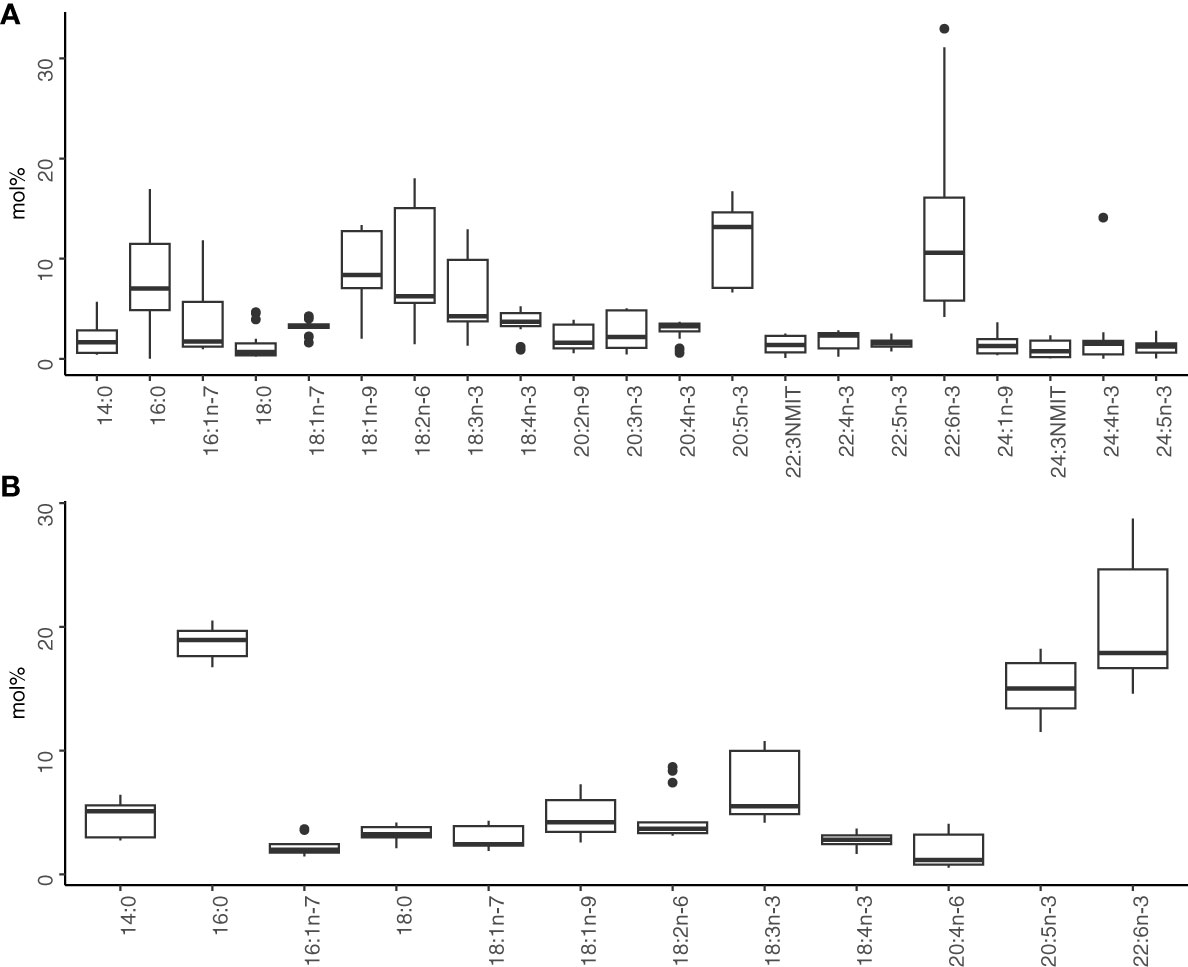
Figure 2 (A) Mean mol% of the major fatty acids (> 1 mol%) in (A) Limnocalanus macrurus and (B) plankton community. All samples of the sample type were combined. Community fatty acids not present in Limnocalanus samples are not shown. NMIT, non-methylene interrupted trienoic fatty acid.
The fatty acid profiles of L. macrurus population of BB and BS differed from the profile of the population in GoF. The proportions of EPA and DHA were significantly lower, but the ratio of unsaturated to saturated fatty acids was significantly higher in GoF compared to the other sea basins (Figures 3A–C). The high UFA/SFA ratio in GoF was mainly due to high proportion of α-linolenic acid 18:3n-3 (Figure 4). The ratio of n-3 to n-6 fatty acids was significantly higher in both BB and BS compared to GoF (Figure 3D). L. macrurus was significantly richer in C24 PUFAs in GoF compared to BB (Figure 3E). The ratio of 18:1n-7 to 18:1n-9 was significantly lower in GoF compared to BB and BS (Figure 3F).
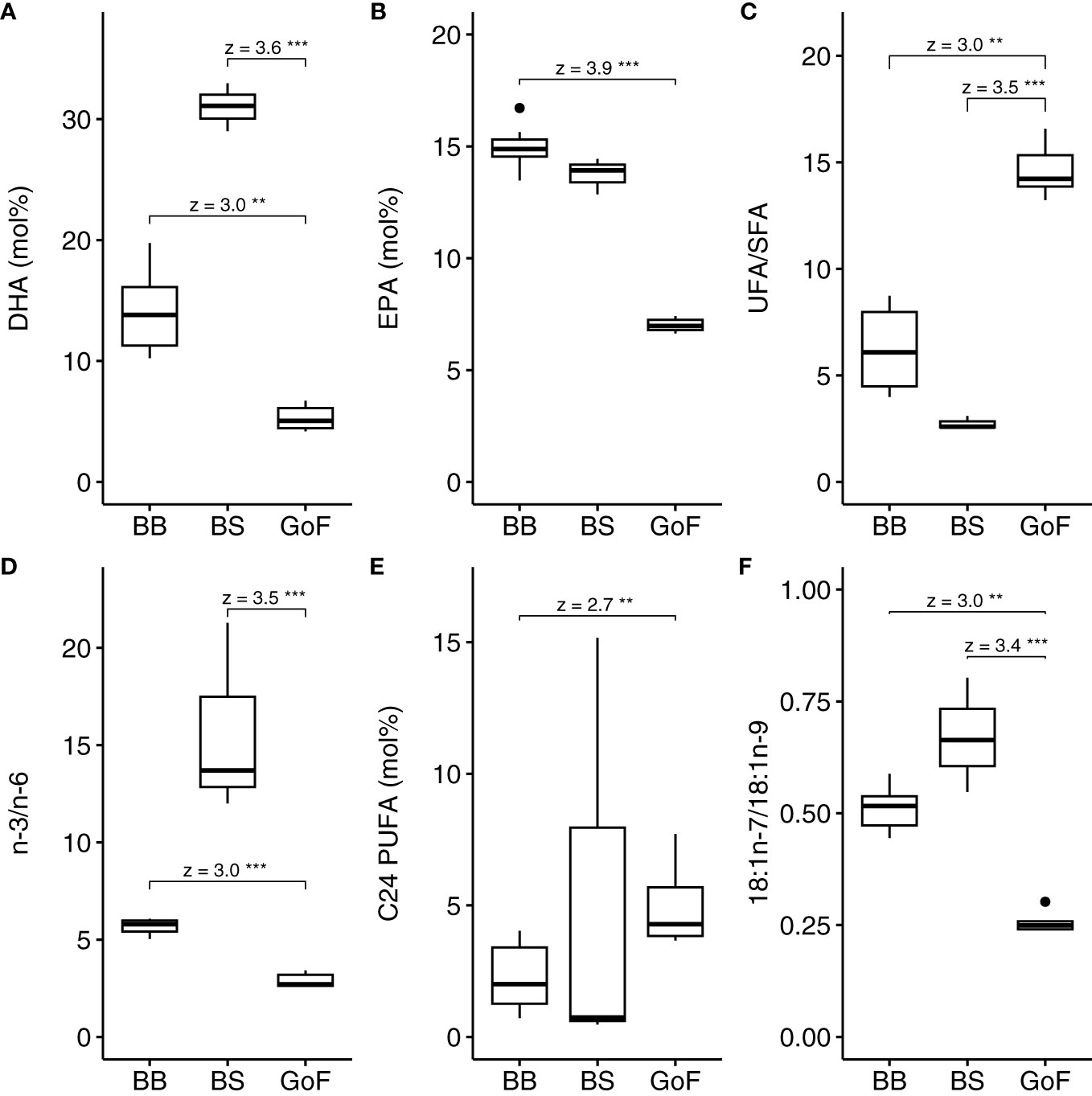
Figure 3 Fatty acid trophic markers (A) 22:6n-3 (DHA), (B) 20:5n-3 (EPA), (C) ratio of unsaturated to saturated fatty acids (UFA/SFA), (D) ratio of n-3 to n-6 polyunsaturated fatty acids (PUFAs), (E) C24 PUFAs and (F) ratio of 18:1n-7 to 18:1n-9 in Limnocalanus samples. Post-hoc test for Kruskal-Wallis, Dunn test, was used. The z-value shows the difference between the mean rank of a group and the mean rank of all observations. UFA/SFA, ratio of unsaturated to saturated fatty acids. P-values: 0.05 = *, <0.01 = **, <0.001 = ***.
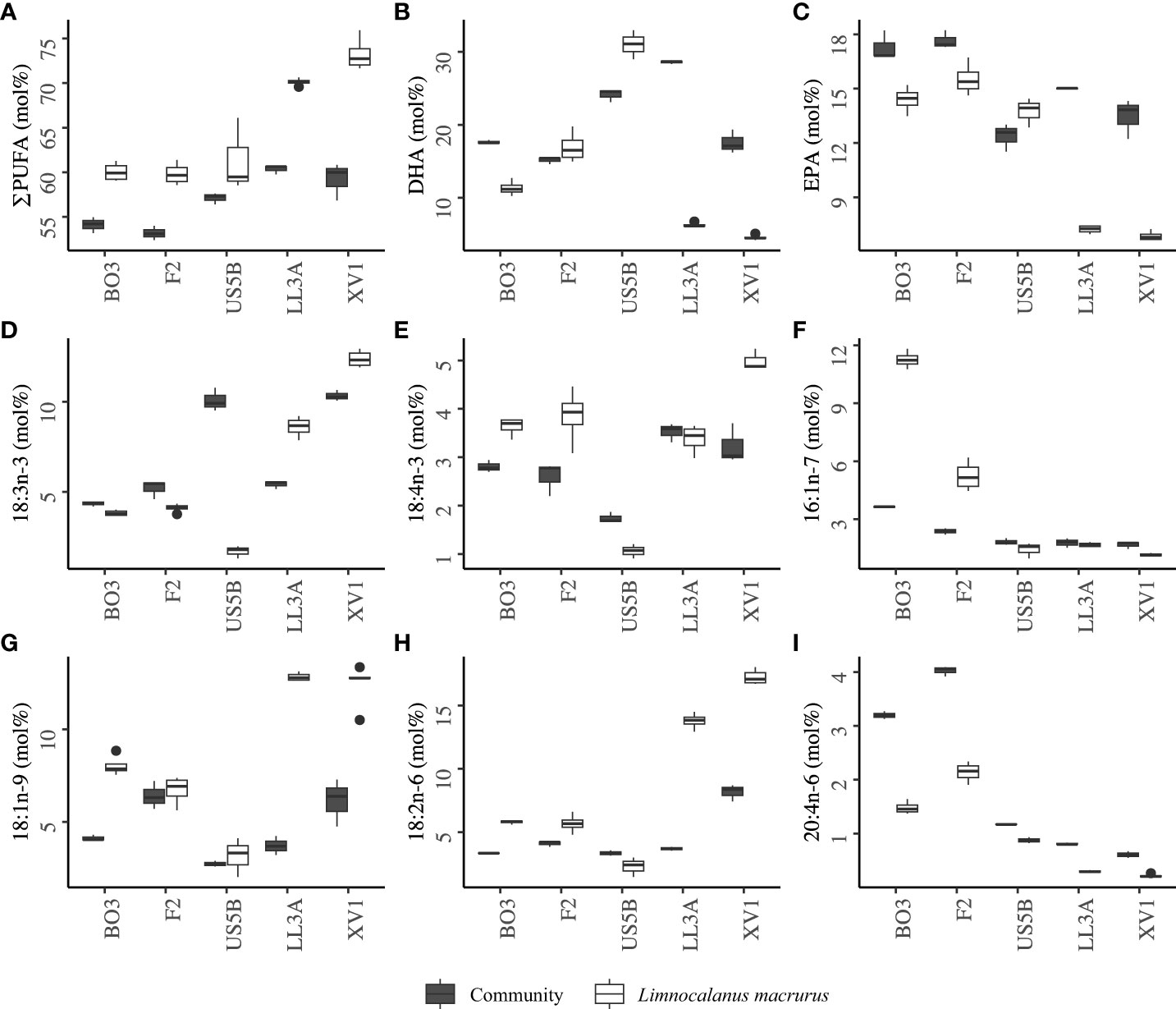
Figure 4 Mean mol% of fatty acid trophic markers in the plankton community and Limnocalanus macrurus: (A) sum of polyunsaturated fatty acids (PUFA), (B) 22:6n-3 (DHA), (C) 20:5n-3 (EPA), (D) 18:3n-3, (E) 18:4n-3, (F) 16:1n-7, (G) 18:1n-9, (H) 18:2n-6, and (I) 20:4n-6.
3.4 Comparing the fatty acid profiles of the community and Limnocalanus macrurus
The main fatty acids in the plankton community were DHA, 16:0, and EPA (Figure 2B). Altogether, they comprised 44.5% of the total lipids. The sum of PUFAs was 57% in average, which included 48% n-3 PUFAs. Although the mean of total PUFA was higher in L. macrurus compared to the plankton community at all stations, the comparisons of the mol% values of individual PUFAs between the community and L. macrurus showed varying relationships across the study area (Figure 4A). In GoF, plankton community was on mol% basis three times richer in DHA and two times richer in EPA compared to L. macrurus, whereas L. macrurus had higher proportion of 18:3n-3 than the community (Figures 4B–D). The proportion of dinoflagellate marker 18:4n-3 is in the same magnitude in both sample types, although higher mean values were recorded at stations BO3, F2 and XVI (Figure 4E). The smallest mean of 18:4n-3 in L. macrurus and plankton community coincided at station US5B. L. macrurus was three times richer in the diatom marker 16:1n-7 than the plankton community at station BO3, and two times at F2 (Figure 4F). In both sample types, the mean mol% of 16:1n-7 was relatively low in GoF and BS. L. macrurus had 2–4 times higher proportion of the omnivory marker 18:1n-9 than the plankton community in GoF and at station BO3 (Figure 4G). At other stations, the mol% of 18:1n-9 was at the same level between the sample types. The PUFAs in n-6 family, 18:2n-6 and arachidonic acid 20:4n-6 showed varying relative values between the samples from different sea basins: the 18:2n-6 had the highest proportions in GoF, where the L. macrurus samples contained 2–3-fold levels compared to the plankton community (Figure 4H). The 20:4n-6 was particularly rich in the community samples from BB (Figure 4I).
3.5 Environmental effects
The biplot of the principal component analysis (showing the scores and loadings) clustered the L. macrurus samples according to the stations and sea basins. The environmental variables were used as loadings together with the main fatty acid markers (Figure 5). Principal components 1 and 2 (later PC1 and PC2) had eigenvalues >1 and together they explained 90% of the total data variance. The PC1 separated the BB and BS samples from the GoF samples, and the PC2 separated the BB samples from BS samples (Figure 5). The characteristic fatty acid markers enriched in BB samples were 16:1n-7, 20:4n-6, branched and EPA. The BS samples were separated from all other samples by their higher DHA content and n-3/n-6 PUFA ratio, and low proportion of 18:4n-3. The characteristic markers of GoF samples were 18:1n-9, 18:2n-6, 18:3n-3 and ΣPUFA. The biplot also indicated that these fatty acid markers high in GoF correlated positively with the environmental factors chl a, temperature and salinity, and negatively with dissolved O2, which, in turn, correlated positively with the fatty acid markers high in BB. On PC1 the drivers with the largest separation power were 18:2n-6, EPA and dissolved O2, and on PC2 the 18:4n-3 and n-3/n-6 ratio (Table 3).
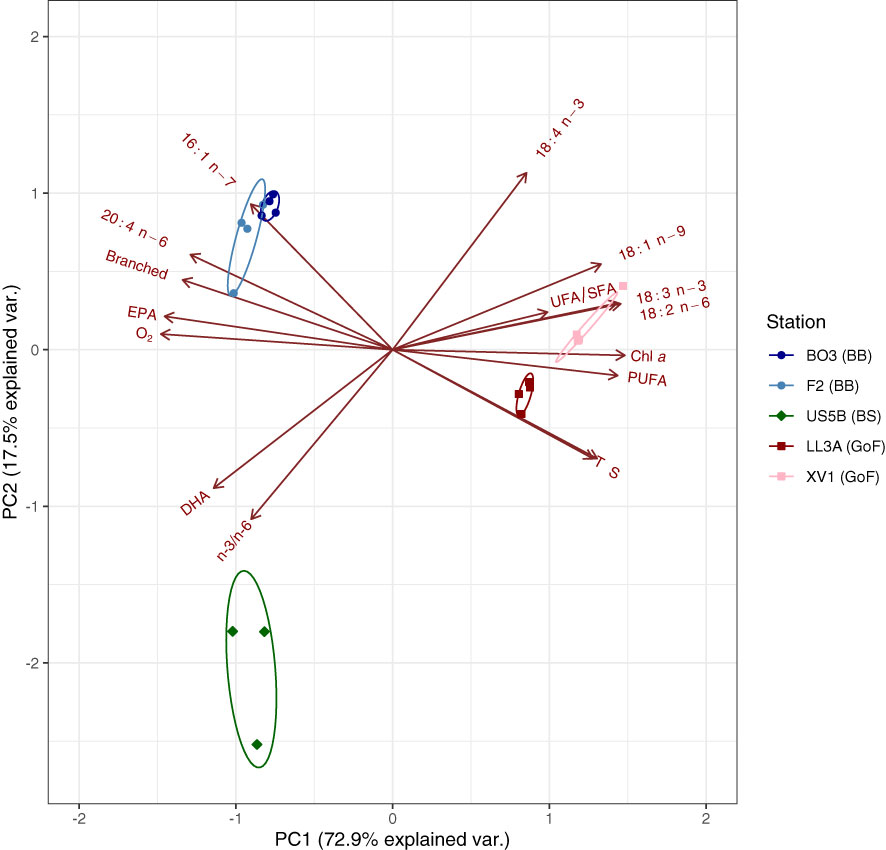
Figure 5 Biplot of principal component analysis (PCA) showing both the scores (samples) and loadings (variables) on the two most important principal components PC1 and PC2, which explained 72.9 and 17.5% of the total data variance, respectively. The variables used include both environmental factors and several fatty acids measured from Limnocalanus macrurus in Bothnian Bay (BB), Bothnian Sea (BS), and in the Gulf of Finland (GoF). T, temperature at 40 m; S, practical salinity at 40 m; chl a, chlorophyll a concentration at 5 m; O2, dissolved oxygen (mg/L) at 40 m; DHA, docosahexaenoic acid (22:6n-3); EPA, eicosapentaenoic acid (20:5n-3); UFA, unsaturated fatty acids; SFA, saturated fatty acids; PUFA, polyunsaturated fatty acids.
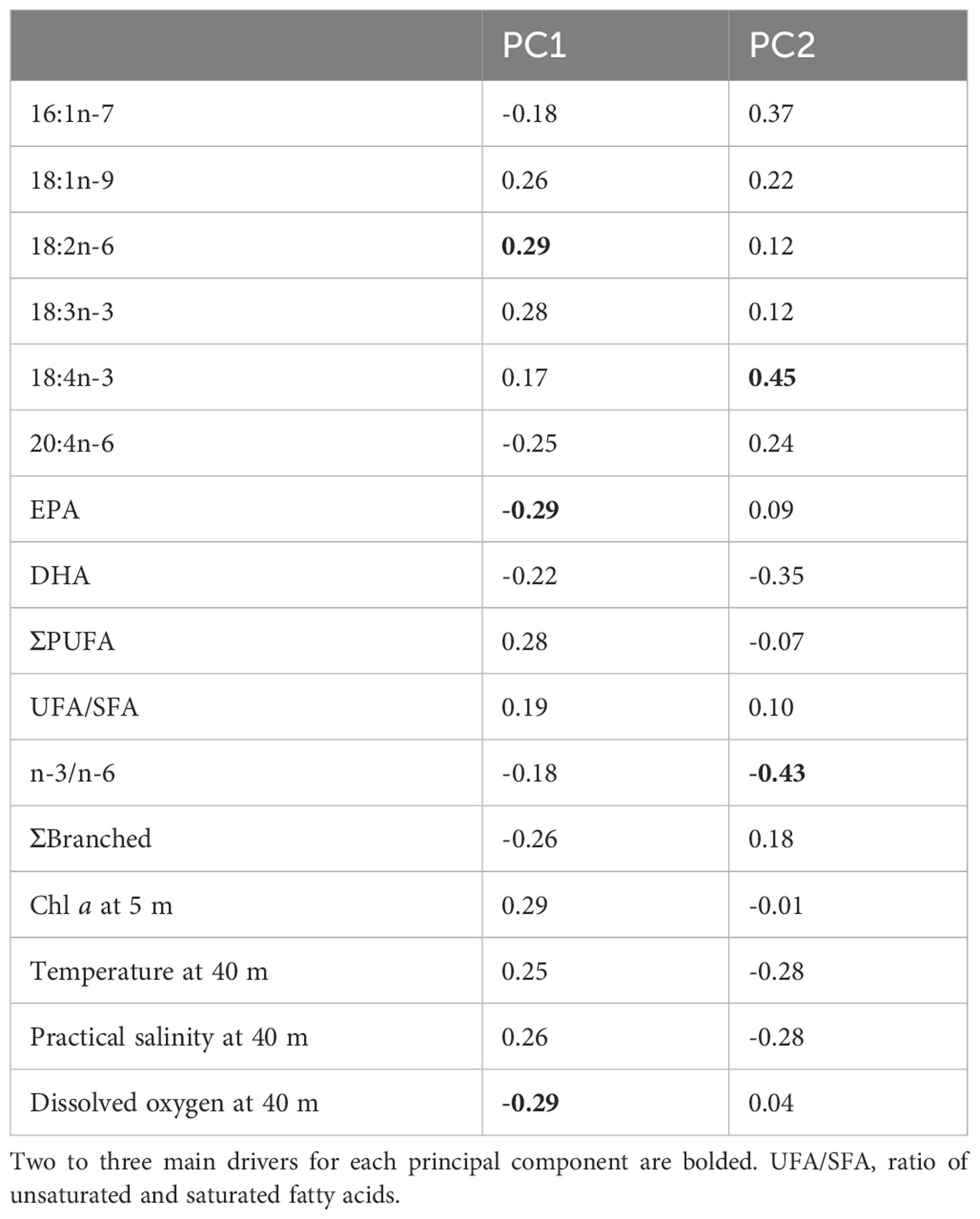
Table 3 Component matrix of the first two principal components (PC1 and PC2) of a principal component analysis (Figure 5).
4 Discussion
Limnocalanus macrurus of the northern Baltic Sea was rich in PUFAs, but the PUFA composition differed between the Gulf of Finland and the Bothnian Bay and the Bothnian Sea, suggesting differences in the plankton diet of this filter feeder. We found wide variability in the diet of L. macrurus across the study area: varying degree of herbivory, detritus-feeding and carnivory on other copepods was interpreted from the fatty acid markers for diatoms (16:1n-7), dinoflagellates (18:4n-3), cyanobacteria or seston (18:2n-6 and 18:3n-3), herbivory–omnivory (18:1n-7/18:n-9), and carnivory of herbivorous copepods (20:1n-9 and 22:1n-11)(Dalsgaard et al., 2003; Falk-Petersen et al., 2009; Peters et al., 2013; Strandberg et al., 2015; Helenius et al., 2020). Nutritional quality of L. macrurus to consumers differed significantly between sea basins, interpreted from the n-3 to n-6 ratio of PUFAs, and differences in the proportion of essential fatty acids EPA and DHA (Tan et al., 2022).
4.1 Fatty acid profiles of Limnocalanus macrurus
L. macrurus is described as a PUFA-rich copepod, which was confirmed in the present study. The average proportions of the major fatty acids (DHA, EPA, 18:2n-6, 18:1n-9 and 16:0) were similar to the values reported by Mäkinen et al. (2017a). However, variation occurred between sea basins: the proportion of total PUFA was higher in GoF than in BB and BS. The levels of essential fatty acids DHA and EPA were significantly lower in GoF, approximately one half of the average of all samples. Hiltunen et al. (2014) have previously reported that Baltic L. macrurus (BB, BS, and GoF sampled in August) had lower proportions of DHA and EPA compared to lakes, but the mean DHA proportion in GoF was even lower in the present study. The result is alarming, since DHA and EPA are essential fatty acids needed for the cell membrane functioning, growth and development of all consumers from zooplankton to fish, and humans (Lee et al., 2006). The health effects of DHA and EPA are widely reported for vertebrates, but they are also important for copepod reproduction (Parrish, 2009). L. macrurus in GoF was particularly rich in 18:3n-3. There are differences in the ability of copepod species to biosynthesize EPA and DHA through elongation and desaturation from 18:3n-3 and 18:4n-3. In starving conditions, the Sprecher pathway via C24 PUFAs to DHA proceeds in some species inefficiently and may not meet the physiological requirements, but in others, endogenous synthesis maintains high DHA contents even for two generations (Bell et al., 2007; Nielsen et al., 2019). L. macrurus is regarded as a PUFA-rich species but its different lake populations have still shown varying DHA levels (Hiltunen et al., 2014; Strandberg et al., 2023), and therefore its DHA levels may reflect environmental and dietary conditions. Our data suggest that this is true also for the Baltic Sea populations: despite abundant 18:3n-3, the low proportion of physiologically essential DHA and EPA indicate poor dietary lipid quality in GoF.
Poor quality in GoF is also indicated from lower n-3/n-6 ratio compared to other basins. Further, the major n-6 PUFA was 18:2n-6 in GoF, whereas the long-chain 20:4n-6 had higher proportion in BB and BS. Like DHA and EPA, 20:4n-6 is an essential fatty acid in aquatic ecosystems, and its availability is important for the development of fish larvae (Bell et al., 2003; Parrish, 2009; Parrish, 2013). Freshwater fish have higher proportion of 18:2n-6 and 20:4n-6 than marine fish, and relatively high proportion of n-6 fatty acids is typical for freshwater algae and terrestrial matter (Parzanini et al., 2020). Relatively high proportions of 20:4n-6 (2.3–2.9%) have also been reported for freshwater L. macrurus (Strandberg et al., 2023), and comparisons between Baltic and lake L. macrurus have shown significantly higher proportions of this fatty acid in lakes (Hiltunen et al., 2014). However, higher proportions of 18:2n-6 have been reported in Baltic populations compared to lakes (Hiltunen et al., 2014). The water salinity in BB is close to freshwater level and thus, it is possible that the influence of freshwater phytoplankton and terrestrial organic matter are responsible for the high proportion of 20:4n-6 in both L. macrurus and the plankton community from BB.
Interestingly, very long-chain C24 PUFAs had high proportions in GoF and BS, but low at station F2 in BB. Hiltunen et al. (2014) have proposed that L. macrurus synthesizes 24:5n-3 and 24:6n-3 from 18:3n-3 and 18:4n-3, possibly for energy storages or cell signaling purposes. In the present study, 18:3n-3 and 18:4n-3 had high proportion in L. macrurus, especially in GoF, and may have served as building material for the observed C24 PUFAs. Hiltunen et al. (2014) hypothesized that accumulation of C24 PUFAs are related to maximizing lipid reserves for winter because they were stored in wax esters. In the present study, sampling occurred in August, which is an ideal time for L. macrurus to accumulate its lipid reserves – Hiltunen et al. (2014) reported that the percentage of C24 PUFAs in L. macrurus peaked in the late summer, when winter lipid reserves were being accumulated.
4.2 Fatty acids reveal dietary variability
L. macrurus was feeding omnivorously in the whole study area, interpreted from the high PUFA content, and the high share of a fatty acid 18:1n-9 characteristic to omnivores (Dalsgaard et al., 2003; Lee et al., 2006). High proportions of 18:1n-9 in Baltic L. macrurus were also reported by Mäkinen et al. (2017a), and a comparison between Baltic and lake populations of L. macrurus have shown three times higher 18:1n-9 proportions in the Baltic populations (Hiltunen et al., 2014). In the present study, fatty acids profiles indicate that the L. macrurus populations in GoF, BB and BS were using different food sources. Interestingly, L. macrurus are located at significantly higher trophic level in the GoF compared to BS or BB, interpreted from their low ratios of EPA/DHA, 18:1n-7/18:1n-9, and high UFA/SFA ratio, which are markers of higher trophic level (Dalsgaard et al., 2003). The relatively high mol% of a diatom marker 16:1n-7 in BB, together with a dinoflagellate marker 18:4n-3, indicate partly herbivorous feeding in BB and confirms that diatoms were consumed (Dalsgaard et al., 2003), as reported also by Hirche et al. (2003).
DHA was three times and EPA two times more abundant in the plankton community compared to L. macrurus in GoF. This is surprising because copepods assimilate DHA efficiently from their diet (Müller-Navarra, 2006; Burns et al., 2011). The result implies that L. macrurus had a restricted access to DHA and EPA, possibly due to high temperature in the upper water column, which have previously been suggested to have caused starvation in BS Mäkinen et al. (2017a). At the same time, the ability of L. macrurus to elongate and desaturate their large stores of the precursors 18:3n-3 and 18:4n-3 to EPA and DHA seems poor, as reported for other copepod species (Bell et al., 2007). Open-water season is important to the energy accumulation of L. macrurus to provide energy reserves for reproduction that occurs during the ice cover (Dahlgren et al., 2012). This further highlights the importance of the availability of essential fatty acids.
Despite the low levels of C20 and C22 PUFAs, the very-long chain C24 PUFAs were accumulated in L. macrurus in GoF. The biological functions of C24 PUFAs are poorly known. They are the immediate precursors of DHA, and high supply of 18:3n-3, as in GoF L. macrurus, may compete with 24:5n-3 for delta-6 desaturation, which would reduce the synthesis of DHA and keep the precursor level high (Portolesi et al., 2007). The C24 PUFAs influence on membrane physical properties and numerous biological functions (Deák et al., 2019), and may balance membrane properties if the shorter C18 PUFAs are incorporated in excess into membranes. The C24 PUFAs were not detected from the community samples in this study. In previous studies, C24 PUFAs have been found in L. macrurus, but not in other zooplankton taxa or seston (Hiltunen et al., 2014; Strandberg et al., 2023). Thus, it is unlikely that C24 PUFAs were acquired from diet. In the future, the role of C24 PUFAs could be clarified by studying their distribution between membrane and storage lipids by using mass spectrometry-based lipidomics. In addition, the ability for endogenous synthesis of C24 PUFAs can be studied in experiments where the copepods are given labeled PUFA precursors and their conversion to the longer and more unsaturated labeled PUFAs is monitored.
Temperature and the influence of terrestrial organic matter may increase heterotrophy in the plankton community, which decreases the availability of DHA and EPA (Dahlgren et al., 2011; Andersson et al., 2023). For the past decades, GoF has been influenced by high amounts of dissolved organic matter, which has driven strong microbial loops in the ecosystem, despite the improved water quality (Golubkov et al., 2010). Furthermore, GoF is characterized by very high cyanobacteria biomass, whereas BB is typically low in cyanobacteria (Kownacka et al., 2022). The high proportion of fatty acids 18:3n-3 and 18:2n-6 in GoF indicates feeding on cyanobacteria or seston (Dalsgaard et al., 2003; Peters et al., 2013; Strandberg et al., 2015). Interestingly, the high mol% of 18:3n-3 in L. macrurus and the plankton community rarely coincided. The plankton community in BS was rich in 18:3n-3, while L. macrurus was not; in contrast, the L. macrurus of GoF had higher mean mol% of 18:3n-3 than the GoF plankton community had.
Long-chain monounsaturated fatty acids 20:1n-9 and 22:1n-11 were present in L. macrurus samples, although in low percentages. Both are important components of wax esters, and synthesized de novo by herbivorous copepods (Falk-Petersen et al., 2009). It is possible that they originate from herbivorous copepod prey, such as Acartia sp. or Eurytemora sp. (Lee et al., 2006; Helenius et al., 2020). Carnivorous feeding on other copepods in L. macrurus occurs in the Northern Baltic Sea, as reported by Dahlgren et al. (2012).
4.3 Future implications
The differences in fatty acid profiles between sea areas were explained by environmental factors, most importantly dissolved O2, chl a, salinity, and temperature. GoF was characterized by high temperature, salinity and chl a, but low levels of dissolved O2; the opposite was true to BB, which was low in temperature, salinity and chl a, but had relatively high dissolved O2. It is difficult to point direct effects of any of these environmental variables alone. Salinity may affect the fatty acid profiles of the plankton community by shaping the species composition (Schwenk et al., 2013; Lichti et al., 2017). Although temperature has direct effects on the metabolism and may at worst accelerate lipid peroxidation that is especially harmful to PUFAs (Hulbert et al., 2007; von Weissenberg et al., 2022), it is more likely that low EPA and DHA resulted from poor nutrition, instead of direct metabolic effects.
The spatial variability in the fatty acid composition of L. macrurus confirms that it is an opportunistic omnivore capable of utilizing multiple food sources. The diet shaped the fatty acid profiles and revealed that the supply of essential PUFAs was reduced in GoF possibly due to high temperature restricting the access to high-quality food in the upper water column. Mäkinen et al. (2017a) reported starvation of L. macrurus in BS due to high temperatures in the surface water layer and increased competition in the vertically narrowed habitat, which also decreased the survival of the adult population. The consequences of low-quality food or even starvation of L. macrurus can cascade further in the food web. L. macrurus is an important food source for a commercially important Baltic herring (Flinkman et al., 1992; Rajasilta et al., 2014). Rajasilta et al. (2019) reported a large decline in the lipid content of Baltic herring during 1987–2014, and concluded that L. macrurus was the most important source of DHA and EPA to herring. Therefore, poor levels of these essential fatty acids in L. macrurus may affect fisheries in the Gulf of Finland.
The present study shows wide dietary variability of L. macrurus in the northern Baltic Sea. While the ability to utilize multiple food sources may increase the resilience of L. macrurus to environmental change, the further consequences of decreasing fatty acid quality may have cascading effects in planktivorous fish populations. Eutrophication and warming are suggested to decrease the availability of EPA and DHA, also in global scale (Strandberg et al., 2022; Taipale et al., 2022; Tan et al., 2022). Moreover, terrestrial organic matter together with increasing temperatures may lead to an increase of heterotrophic bacteria in the ecosystem (Andersson et al., 2023). The results in the present study show poor reserves of DHA and EPA in L. macrurus from the eutrophicated, hypoxic and warm GoF, which raises concerns about future trends in the pelagic communities of the Baltic Sea when temperature rises due to climate change.
Data availability statement
The original contributions presented in the study are included in the article/Supplementary Material. Further inquiries can be directed to the corresponding author.
Ethics statement
The manuscript presents research on animals that do not require ethical approval for their study.
Author contributions
EW: Visualization, Writing – original draft, Conceptualization, Formal analysis, Investigation, Writing – review & editing, Funding acquisition. HR: Writing – review & editing, Formal analysis, Methodology. MH: Writing – review & editing, Methodology. RK: Writing – review & editing, Formal analysis, Methodology. JE-Ö: Writing – review & editing, Supervision, Conceptualization, Funding acquisition, Methodology.
Funding
The author(s) declare financial support was received for the research, authorship, and/or publication of this article. Funding for this research has been received from Onni Talaan säätiö and Svenska Kulturfonden, and the publication of the research article is covered by the University of Helsinki.
Acknowledgments
We want to thank the Finnish Environment Institute and the Finnish Meteorological Institute for hydrographical data and sampling operation, and R/V Aranda as part of the Finnish Marine Infrastructure (FINMARI) for excellent research facilities. Thanks to the cruise leaders Pekka Kotilainen and Maiju Lehtiniemi, and the crew of COMBINE III for your hospitality and guidance on board of R/V Aranda. Special thanks to Lisa Naeve for the invaluable assistance in sampling during the cruise. We are grateful for the laboratory facilities provided by the Helsinki University Lipidomics Unit. Thanks to Sanna Sihvo for gas chromatography and guidance in the laboratory.
Conflict of interest
The authors declare that the research was conducted in the absence of any commercial or financial relationships that could be construed as a potential conflict of interest.
Publisher’s note
All claims expressed in this article are solely those of the authors and do not necessarily represent those of their affiliated organizations, or those of the publisher, the editors and the reviewers. Any product that may be evaluated in this article, or claim that may be made by its manufacturer, is not guaranteed or endorsed by the publisher.
Supplementary material
The Supplementary Material for this article can be found online at: https://www.frontiersin.org/articles/10.3389/fmars.2024.1340349/full#supplementary-material
References
Andersson A., Grinienė E., Berglund M. M., Brugel S., Gorokhova E., Figueroa D., et al. (2023). Microbial food web changes induced by terrestrial organic matter and elevated temperature in the coastal northern Baltic Sea. Front. Mar. Sci. 10. doi: 10.3389/fmars.2023.1170054
Bell J. G., McEvoy L. A., Estevez A., Shields R. J., Sargent J. R. (2003). Optimising lipid nutrition in first-feeding flatfish larvae. Aquaculture 227, 211–220. doi: 10.1016/S0044-8486(03)00504-0
Bell M. V., Dick J. R., Anderson T. R., Pond D. W. (2007). Application of liposome and stable isotope tracer techniques to study polyunsaturated fatty acid biosynthesis in marine zooplankton. J. Plankton Res. 29, 417–422. doi: 10.1093/plankt/fbm025
Burns C. W., Brett M. T., Schallenberg M. (2011). A comparison of the trophic transfer of fatty acids in freshwater plankton by cladocerans and calanoid copepods. Freshw. Biol. 56, 889–903. doi: 10.1111/j.1365-2427.2010.02534.x
Carstensen J., Conley D. J., Bonsdorff E., Gustafsson B. G., Hietanen S., Janas U., et al. (2014). Hypoxia in the baltic sea: biogeochemical cycles, benthic fauna, and management. AMBIO 43, 26–36. doi: 10.1007/s13280-013-0474-7
Christie W. W. (1993). Preparation of ester derivatives of fatty acids for chromatographic analysis. Adv. Lipid Method. – Two, 69–111.
Christie W. (2023). LIPID MAPS lipidomics gateway, the lipid web, methyl esters of fatty acids, archive of mass spectra. Available at: https://lipidmaps.org/resources/lipidweb/index.php?page=ms/methesters/me-arch/index.htm (Accessed Accessed: 2022–2023).
Conley D. J., Carstensen J., Aigars J., Axe P., Bonsdorff E., Eremina T., et al. (2011). Hypoxia is increasing in the coastal zone of the Baltic sea. Environ. Sci. Technol. 45, 6777–6783. doi: 10.1021/es201212r
Dahlgren K., Olsen B. R., Troedsson C., Båmstedt U. (2012). Seasonal variation in wax ester concentration and gut content in a Baltic Sea copepod [Limnocalanus macrurus (Sars 1863)]. J. Plankton Res. 34, 286–297. doi: 10.1093/plankt/fbs005
Dahlgren K., Wiklund A. K. E., Andersson A. (2011). The influence of autotrophy, heterotrophy and temperature on pelagic food web efficiency in a brackish water system. Aquat. Ecol. 45, 307–323. doi: 10.1007/s10452-011-9355-y
Dalsgaard J., John M. S., Kattner G., Müller-Navarra D., Hagen W. (2003). Fatty acid trophic markers in the pelagic marine environment. Adv. Mar. Biol. 46, 225–340. doi: 10.1016/S0065-2881(03)46005-7
Daufresne M., Lengfellner K., Sommer U. (2009). Global warming benefits the small in aquatic ecosystems. Proc. Natl. Acad. Sci. United States America 106, 12788–12793. doi: 10.1073/pnas.0902080106
Deák F., Anderson R. E., Fessler J. L., Sherry D. M. (2019). Novel cellular functions of very long chain-fatty acids: insight from ELOVL4 mutations. Front. Cell. Neurosci. 13. doi: 10.3389/fncel.2019.00428
Diekmann A. B. S., Clemmesen C., St. John M. A., Paulsen M., Peck M. A. (2012). Environmental cues and constraints affecting the seasonality of dominant calanoid copepods in brackish, coastal waters: A case study of Acartia, Temora and Eurytemora species in the south-west Baltic. Mar. Biol. 159, 2399–2414. doi: 10.1007/s00227-012-1955-0
Dinno A. (2017). dunn.test: Dunn’s test of multiple comparisons using rank sums. (Vienna, Austria: CRAN repository).
Dunn O. J. (1961). Multiple comparisons among means. J. Am. Stat. Assoc. 56, 52–64. doi: 10.1080/01621459.1961.10482090
Einberg H., Klais R., Rubene G., Kornilovs G., Putnis I., Ojaveer H. (2019). Multidecadal dynamics of the Arctic copepod Limnocalanus macrurus in relation to environmental variability in the Baltic Sea. ICES J. Mar. Sci. 76, 2427–2436. doi: 10.1093/icesjms/fsz101
Falk-Petersen S., Mayzaud P., Kattner G., Sargent J. R. (2009). Lipids and life strategy of Arctic Calanus. Mar. Biol. Res. 5, 18–39. doi: 10.1080/17451000802512267
Flinkman J., Vuorinen I., Aro E. (1992). Planktivorous baltic herring (Clupea harengus) prey selectively on reproducing copepods and cladocerans. Can. J. Fisheries Aquat. Sci. 49, 73–77. doi: 10.1139/f92-008
Golubkov S. M., Maximov A. A., Golubkov M. S., Litvinchuk L. F. (2010). “A functional shift in the ecosystem of the eastern Gulf of Finland caused by natural and anthropogenic factors,” in Doklady biological sciences, vol. 432. (Moscow, Russia: Doklady Akademii Nau), 198.
Gorokhova E. (2019). Individual body size as a predictor of lipid storage in Baltic Sea zooplankton. J. Plankton Res. 41, 273–280. doi: 10.1093/plankt/fbz010
HELCOM. (2013). Manual for marine monitoring in the COMBINE 2017. (Helsinki, Finland: HELCOM), 309–332.
Helenius L., Budge S. M., Johnson C. L. (2020). Stable isotope labeling reveals patterns in essential fatty acid growth efficiency in a lipid-poor coastal calanoid copepod. Mar. Biol. 167, 1–13. doi: 10.1007/s00227-020-03794-8
Hiltunen M., Strandberg U., Keinänen M., Taipale S., Kankaala P. (2014). Distinctive lipid composition of the copepod Limnocalanus macrurus with a high abundance of polyunsaturated fatty acids. Lipids 49, 919–932. doi: 10.1007/s11745-014-3933-4
Hirche H.-J., Fetzer I., Graeve M., Kattner G. (2003). Limnocalanus macrurus in the Kara Sea (Arctic Ocean): an opportunistic copepod as evident from distribution and lipid patterns. Polar Biol. 26, 720–726. doi: 10.1007/s00300-003-0541-9
Hulbert A. J., Pamplona R., Buffenstein R., Buttemer W. A. (2007). Life and death: Metabolic rate, membrane composition, and life span of animals. Physiol. Rev. 87, 1175–1213. doi: 10.1152/physrev.00047.2006
Käkelä R., Käkelä A., Kahle S., Becker P. H., Kelly A., Furness R. W. (2005). Fatty acid signatures in plasma of captive herring gulls as indicators of demersal or pelagic fish diet. Mar. Ecol. Prog. Ser. 293, 191–200. doi: 10.3354/meps293191
Kane D. D., Gannon J. E., Culver D. A. (2004). The status of Limnocalanus macrurus (Copepoda: Calanoida: Centropagidae) in Lake Erie. J. Great Lakes Res. 30, 22–30. doi: 10.1016/S0380-1330(04)70326-3
Kownacka J., Busch S., Göbel J., Gromisz S., Hällfors H., Höglander H., et al. (2022). Cyanobacteria biomass, 1990-2020 (Helsinki, Finland: HELCOM).
Lampert W. (1989). The adaptive significance of diel vertical migration of zooplankton. Funct. Ecol. 3 (1), 21–27. doi: 10.2307/2389671
Lee R. F., Hagen W., Kattner G. (2006). Lipid storage in marine zooplankton. Mar. Ecol. Prog. Ser. 307, 273–306. doi: 10.3354/meps307273
Lichti D. A., Rinchard J., Kimmel D. G. (2017). Changes in zooplankton community, and seston and zooplankton fatty acid profiles at the freshwater/saltwater interface of the Chowan River, North Carolina. PeerJ 5, e3667. doi: 10.7717/peerj.3667
Mäkinen K., Elfving M., Hänninen J., Laaksonen L., Rajasilta M., Vuorinen I., et al. (2017a). Fatty acid composition and lipid content in the copepod Limnocalanus macrurus during summer in the southern Bothnian Sea. Helgoland Mar. Res. 71, 1–12. doi: 10.1186/s10152-017-0491-1
Mäkinen K., Vuorinen I., Hänninen J. (2017b). Climate-induced hydrography change favours smallbodied zooplankton in a coastal ecosystem. Hydrobiologia 792, 83–96. doi: 10.1007/s10750-016-3046-6
Meier H. E. M., Dieterich C., Gröger M., Dutheil C., Börgel F., Safonova K., et al. (2022). Oceanographic regional climate projections for the Baltic Sea until 2100. Earth Syst. Dynam. 13, 159–199. doi: 10.5194/esd-13-159-2022
Müller-Navarra D. C. (2006). The nutritional importance of polyunsaturated fatty acids and their use as trophic markers for herbivorous zooplankton: Does it contradict? Archiv Fur Hydrobiologie 167, 501–514. doi: 10.1127/0003-9136/2006/0167-0501
Nielsen B. L. H., Gøtterup L., Jørgensen T. S., Hansen B. W., Hansen L. H., Mortensen J., et al. (2019). n-3 pufa biosynthesis by the copepod Apocyclops royi documented using fatty acid profile analysis and gene expression analysis. Biol. Open 8, bio038331. doi: 10.1242/bio.038331
Parrish C. C. (2009). Essential fatty acids in aquatic food webs. Lipids Aquat. Ecosyst., 309–326. doi: 10.1007/978-0-387-89366-2_13
Parzanini C., Colombo S. M., Kainz M. J., Wacker A., Parrish C. C., Arts M. T. (2020). Discrimination between freshwater and marine fish using fatty acids: ecological implications and future perspectives. Environ. Rev. 28, 546–559. doi: 10.1139/er-2020-0031
Peters J., Dutz J., Hagen W. (2013). Trophodynamics and life-cycle strategies of the copepods Temora longicornis and Acartia longiremis in the Central Baltic Sea. J. Plankton Res. 35, 595–609. doi: 10.1093/plankt/fbt004
Portolesi R., Powell B. C., Gibson R. A. (2007). Competition between 24:5n-3 and ALA for Δ6 desaturase may limit the accumulation of DHA in HepG2 cell membranes. J. Lipid Res. 48, 1592–1598. doi: 10.1194/jlr.M700081-JLR200
Rajasilta M., Hänninen J., Laaksonen L., Laine P., Suomela J.-P., Vuorinen I., et al. (2019). Influence of environmental conditions, population density, and prey type on the lipid content in Baltic herring (Clupea harengus membras) from the northern Baltic Sea. Can. J. Fisheries Aquat. Sci. 76, 576–585. doi: 10.1139/cjfas-2017-0504
Rajasilta M., Hänninen J., Vuorinen I. (2014). Decreasing salinity improves the feeding conditions of the Baltic herring (Clupea harengus membras) during spring in the Bothnian Sea, northern Baltic. ICES J. Mar. Sci. 71, 1148–1152. doi: 10.1093/icesjms/fsu047
Rajasilta M., Vuorinen I. (2008). Suomen murtovesialueen eläinplankton-määritysopas Vol. 5 (Turku, Finland: SEILI Archipelago Research Institute Publications), 1–62.
Renaud P. E., Daase M., Banas N. S., Gabrielsen T. M., Søreide J. E., Varpe O., et al. (2018). Pelagic food-webs in a changing Arctic: A trait-based perspective suggests a mode of resilience. ICES J. Mar. Sci. 75, 1871–1881. doi: 10.1093/icesjms/fsy063
Schwenk D., Seppälä J., Spilling K., Virkki A., Tamminen T., Oksman-Caldentey K.-M., et al. (2013). Lipid content in 19 brackish and marine microalgae: influence of growth phase, salinity and temperature. Aquat. Ecol. 47, 415–424. doi: 10.1007/s10452-013-9454-z
Strandberg U., Hiltunen M., Creed I. F., Arts M. T., Kankaala P. (2023). Browning-induced changes in trophic functioning of planktonic food webs in temperate and boreal lakes: insights from fatty acids. Oecologia 201, 183–197. doi: 10.1007/s00442-022-05301-w
Strandberg U., Hiltunen M., Jelkänen E., Taipale S. J., Kainz M. J., Brett M. T., et al. (2015). Selective transfer of polyunsaturated fatty acids from phytoplankton to planktivorous fish in large boreal lakes. Sci. Total Environ. 536, 858–865. doi: 10.1016/j.scitotenv.2015.07.010
Strandberg U., Hiltunen M., Syväranta J., Levi E. E., Davidson T. A., Jeppesen E., et al. (2022). Combined effects of eutrophication and warming on polyunsaturated fatty acids in complex phytoplankton communities: A mesocosm experiment. Sci. Total Environ. 843, 157001. doi: 10.1016/j.scitotenv.2022.157001
Taipale S. J., Ventelä A., Litmanen J., Anttila L. (2022). Poor nutritional quality of primary producers and zooplankton driven by eutrophication is mitigated at upper trophic levels. Ecol. Evol. 12, e8687. doi: 10.1002/ece3.8687
Tan K., Zhang H., Zheng H. (2022). Climate change and n-3 LC-PUFA availability. Prog. Lipid Res. 86, 101161. doi: 10.1016/j.plipres.2022.101161
von Weissenberg E., Jansson A., Vuori K. A., Engström-Öst J. (2022). Copepod reproductive effort and oxidative status as responses to warming in the marine environment. Ecol. Evol. 12, e8594. doi: 10.1002/ece3.8594
Vuori K. A., Lehtonen K. K., Kanerva M., Peltonen H., Nikinmaa M., Berezina N. A., et al. (2015). Oxidative stress biomarkers in the copepod Limnocalanus macrurus from the northern Baltic Sea: Effects of hydrographic factors and chemical contamination. Mar. Ecol. Prog. Ser. 538, 131–144. doi: 10.3354/meps11471
Warren G. J. (1985). Predaceous feeding habits of Limnocalanus macrurus. J. plankton Res. 7, 537–552. doi: 10.1093/plankt/7.4.537
Webster C. N., Hansson S., Didrikas T., Gorokhova E., Peltonen H., Brierley A. S., et al. (2015). Stuck between a rock and a hard place: zooplankton vertical distribution and hypoxia in the Gulf of Finland, Baltic Sea. Mar. Biol. 162, 1429–1440. doi: 10.1007/s00227-015-2679-8
Keywords: fatty acid composition, PUFA, eutrophication, zooplankton, climate change, brackish water, fatty acid trophic marker, Limnocalanus macrurus
Citation: von Weissenberg E, Ruhanen H, Holopainen M, Käkelä R and Engström-Öst J (2024) Fatty acid profiles reveal dietary variability of a large calanoid copepod Limnocalanus macrurus in the northern Baltic Sea. Front. Mar. Sci. 11:1340349. doi: 10.3389/fmars.2024.1340349
Received: 17 November 2023; Accepted: 16 January 2024;
Published: 07 February 2024.
Edited by:
Pedro Morais, Florida International University, United StatesReviewed by:
Per Meyer Jepsen, Roskilde University, DenmarkYen-Ju Pan, National Taiwan Ocean University, Taiwan
Copyright © 2024 von Weissenberg, Ruhanen, Holopainen, Käkelä and Engström-Öst. This is an open-access article distributed under the terms of the Creative Commons Attribution License (CC BY). The use, distribution or reproduction in other forums is permitted, provided the original author(s) and the copyright owner(s) are credited and that the original publication in this journal is cited, in accordance with accepted academic practice. No use, distribution or reproduction is permitted which does not comply with these terms.
*Correspondence: Ella von Weissenberg, ella.vonweissenberg@helsinki.fi