Erratum: Ecotoxicological consequences of polystyrene naturally leached in pure, fresh, and saltwater: lethal and nonlethal toxicological responses in Daphnia magna and Artemia salina
- 1Ecosystems and Environment Research Programme, Faculty of Biological and Environmental Sciences, University of Helsinki, Lahti, Finland
- 2Helsinki Institute of Sustainability Science (HELSUS), Helsinki, Finland
- 3Clayton H. Riddell Faculty of Environment, Earth, and Resources, University of Manitoba, Winnipeg, MB, Canada
- 4Korea Institute of Science and Technology Europe (KIST Europe) Forschungsgesellschaft GmbH, Joint Laboratory of Applied Ecotoxicology, Environmental Safety Group, Universität des Saarlandes, Saarbrücken, Germany
- 5Faculty of Biotechnology, College of Applied Life Sciences, Jeju National University, Jeju, Republic of Korea
- 6Division of Energy & Environment Technology, University of Science & Technology, Daejeon, Republic of Korea
Polystyrene is widely used in disposable products and is now a ubiquitous plastic pollutant in aquatic environments, where it degrades into smaller particles that leach potentially toxic chemicals. However, knowledge regarding the impacts of plastic leachates remains limited. This study investigates the lethal and nonlethal effects of polystyrene leachate on two ecologically significant aquatic organisms, Daphnia magna (water flea) and Artemia salina (brine shrimp). Polystyrene leachates were prepared in seawater, freshwater, and sterile, pure water by incubating the material in each of the media under natural conditions for six months. D. magna and A. salina were exposed to varying concentrations of the leachates under controlled laboratory conditions, monitoring their survival, as well as measuring reactive oxygen species and antioxidant responses as superoxide dismutase and catalase activity. The data show that A. salina was more significantly affected with higher mortality observed at lower leachate concentrations, potentially linked to seawater enhancing the leaching of toxic additives. Moreover, at non-lethal concentrations, the antioxidative responses maintained homeostasis in both organisms. Considering the current reported microplastic concentrations in the aquatics and the adequate antioxidative response, leachate from plastic potentially does not pose a severe threat to these organisms. Nevertheless, hydrological characteristics of waterbodies may cause microplastic hotspots, which could significantly concentrate plastics and thus their leachates, necessitating action to reduce the current microplastic pollution level and avoid future surges. This study highlights the ecological significance of polystyrene pollution, emphasizing the need for more comprehensive regulatory measures and the development of sustainable alternatives to polystyrene-based products. The distinct responses of D. magna and A. salina imply that the impact of plastic pollution varies among species, necessitating further research to elucidate broader ecological consequences. Understanding how polystyrene leachate affects keystone species provides crucial insights into the overall implications for aquatic ecosystems.
1 Introduction
Plastic pollution is a pressing environmental issue that is garnering merited attention. The growing global distribution of plastic waste in the environment stems from its widespread use and improper disposal (Dahlbo et al., 2018; van Velzen et al., 2019). While studies on the ecotoxicology of plastic and its breakdown products, such as micro- and nanoplastics, are increasing, and the impacts are being better understood (Esterhuizen and Kim, 2022; Samadi et al., 2022), another aspect of this problem, namely the toxicity of leaching chemicals, is being recognised (Lithner et al., 2009; Lithner et al., 2012; Pflugmacher et al., 2020).
Plastic items can leach harmful chemicals into their surroundings (Do et al., 2022). Additives which are not covalently bound to the polymer matrix and residual monomers could migrate into the environment from virgin plastics (Crompton, 1979; Araújo et al., 2002; Lithner et al., 2011; Kwan and Takada, 2016). Over time, when exposed to environmental factors like sunlight and water, plastics can break down and release a cocktail of toxic compounds, including phthalates, bisphenol A (BPA), and polychlorinated biphenyls (PCBs). These compounds are known as additives and are applied during the manufacture of plastics depending on the intended use and properties of the final product (Wiesinger et al., 2021; Do et al., 2022). They play a crucial role in tailoring plastics for specific applications by imparting desirable properties or allowing the retaining of the original plastic properties during moulding (OECD, 2009; Hahladakis et al., 2018). Regularly used additives include plasticisers, flame retardants, thermal stabilisers, photostabilisers, antioxidants, and pigments (Stevens, 1990; Andrady and Rajapakse, 2016).
Various media have been shown to facilitate leaching, including sea- and freshwater (Bridson et al., 2021). Release of additives from plastics was studied by Do et al. (2022). They found that polymer type, surface characteristics, the environment, and time are the main factors influencing leaching. Furthermore, biodegradation, thermal and photodegradation influenced the liberation of additives.
Some additives are known carcinogens, mutagens, and endocrine disruptors, and thus potentially hazardous to many organisms (EBC, 2008; Groh et al., 2019). As plastic and microplastic (MP) pollution has been found globally (Scopetani et al., 2019; Mammo et al., 2020; Patti et al., 2020; Prabhu et al., 2022), these leached chemicals can contaminate terrestrial and aquatic ecosystems and even enter the food chain, with potentially detrimental effects on the health of both wildlife and humans. Understanding and addressing this facet of plastic pollution is essential for mitigating its far-reaching consequences, especially concerning the toxicity of leachates to native biota.
In addition to intrinsic toxicants that may be liberated, MPs can adsorb and concentrate persistent organic pollutants (POPs, e.g., PCBs and organochlorine pesticides), heavy metals (lead, cadmium, and mercury), and non-plastic pollutants (e.g., polycyclic aromatic hydrocarbons (PAHs)) from the surrounding water (Caruso, 2019). Chemicals that adhere to MP surfaces have been shown to bioaccumulate in organisms that ingest the fragments (Caruso, 2019). Furthermore, microbial biofilms can develop on MPs in the environment, releasing metabolites, enzymes, and other chemicals that have been found to contribute to plastic degradation and, thus, facilitate leaching (Han et al., 2020; Sooriyakumar et al., 2022), which may contribute to toxicity.
Even though several studies have considered the ecotoxicological effects of leachates from plastics (reviewed by Gunaalan et al., 2020), many have used artificial leaching methods and have not considered the effect of factors such as the environment, climate fluctuations, and naturally occurring microbes. Therefore, the present study aimed to investigate the toxicological effects of natural leachate from polystyrene (PS), one of the most detected plastics in the environment (Scopetani et al., 2019; Tian et al., 2023). PS was leached in pure water (MilliQ), freshwater, and seawater for six months outside under environmental conditions and the ecotoxicological effects of the leachate, and dilutions thereof, were investigated in terms of immobility, oxidative stress, and enzymatic antioxidative defence response in Daphnia magna (pure and freshwater) and Artemia salina (seawater) with acute exposure. Many previous studies regarding MP and leachates were conducted with D. magna (Samadi et al., 2022), a recognised model bioindicator organism for evaluating ecotoxicology (Reilly et al., 2023). Likewise, A. salina has been suggested as a model species for ecotoxicity assessments (Kalčíková et al., 2012; Albarano et al., 2022). The three media were selected to assess how the properties of a natural media can influence the toxicity of the leachate, where both fresh- and seawater contained natural microbes and minerals compared to sterile MilliQ water which is both deionized and demineralized. This approach allows the direct comparison of the toxicities of the obtained PS leachates in the various media on keystone bioindicator organisms which have not previously been assessed. Thus, the selection of three distinct media aimed to investigate the impact of natural media properties on leachate toxicity. Both fresh- and seawater, rich in natural microbes and minerals, were compared to sterile MilliQ water, known for its deionized and demineralized composition. This method facilitates a direct comparison of the toxicities exhibited by the obtained PS leachates across different media. The chosen bioindicator organisms, considered keystone species, have not been previously evaluated under these conditions.
2 Materials and methods
2.1 Bioindicator organism cultivation
D. magna ephippia were supplied by MicroBiotests Inc. (Gent, Belgium). Hatching was facilitated under 7000 lux light with a 16-to-8-hour light-to-dark cycle at 20.0 ± 1.0°C in Elendt M4 medium (OECD, 2004). A population of 15 daphnid individuals was maintained in 1.5 L Elendt M4 medium in a controlled climate incubator. Once a day, the daphnids were with Chlorella vulgaris (~1.5 × 108 cells/mL), purchased from the Culture Collection of Algae (Cologne University, Essen, Germany), at a density of 0.1 - 0.2 mg C per individual. Additionally, twice a week, the culture was fed with yeast, cerophyl, and trout chow at a concentration of 0.5 μL/mL (v/v). Offspring were removed daily, and the cultural medium was renewed thrice weekly. The pH and dissolved oxygen content were checked and maintained according to OECD guidelines (OECD, 2004). Interlaboratory tests were performed using potassium dichromate (Sigma-Aldrich, St. Louis, MO, USA) as a reference toxicant. For the present study, neonates were ≤ 24 hours old.
Artemia cysts were purchased from Great Salt Lake Artemia (Utah, USA) and hatched in an artificial seawater medium. This medium was prepared to a salinity of 25 parts per thousand (ppt) by dissolving sodium chloride (Sigma Aldrich, St. Louis, MO, USA) in deionized water. To achieve a pH level above 8, sodium bicarbonate (Sigma Aldrich, St. Louis, MO, USA) was added to the medium. The incubation was carried out in a temperature-controlled environment at 25 ± 1°C, with continuous aeration and an illumination intensity of 3000 lux for 30 hours.
2.2 Consumables
Polystyrene (PS) granules (3-5 mm particle size; product Code: ST31-GL-000111; LS555533; batch C3586) were purchased from Goodfellow (Huntingdon, UK) and ground into smaller fragments employing low-temperature ball milling on a cryomill (Retsch GmbH, Haan, Germany) maintained between -196°C and -100°C using liquid nitrogen. A 50 mL cell and a 2 cm diameter steel ball were used for mechanical cryogenic grinding. A third of the cell was filled with the plastic material and ground for approximately 30 minutes, with 5 minutes of pre-cooling. The plastic fragments were separated using a Vibratory Sieve shaker AS 300 Control (Retsch GmbH, Haan, Germany) via four sieves with mesh sizes of 100, 63, 45, and 25 µm according to ISO 3310-01 (ISO, 2016) and the size fraction 45 to 63 µm was used for the environmental leaching.
All other consumables were purchased from Sigma Aldrich unless stated otherwise and were of analytical grade.
2.3 Leachate preparation
Seawater and freshwater were collected from the North Sea, Knokke-Heist, Belgium (coordinates: 51.359060, 3.306336) and the Handelsdok Canal in Bruges, Belgium (coordinates: 51.221321, 3.223855), respectively, on the September 9th, 2021, as 100 L grab samples. The pH of the seawater and freshwater measured as 8.1 and 8.08, respectively. In the seawater sample, the salinity and conductivity values were 29.52 ppt and 45.79 µs/cm and the media had a turbidity of 0.27. For the freshwater, the measurements were 2.12 ppt for salinity, 40.51 µs/cm for conductivity, and 0.67 for turbidity respectively. The collected water samples were filtered via vacuum filtration (0.22 μm pore 19.6 cm2 CA membrane) including a storage Bottle System (Cat. No. 430758, Corning Inc. Corning, US). PS fragments at a concentration of 100 g/L were added to each of the filtered waters (freshwater and seawater), respectively, as well as MilliQ water (pH 7.01) in capped glass vessels (n = 3) and placed outside for six months at the premises of KIST Europe in Saarbrücken from October 26, 2021, to April 30, 2022. The chosen PS concentration aligns with previous studies investigating the toxicity of microplastic (MP) leachates (Lithner et al., 2009; Lithner et al., 2012). MilliQ water was used to allow the evaluation of the toxicity of the PS leachate without the influence of ions, minerals, and microbes. The vessels were uncapped and aired daily. The leachate was filtered with a 0.45 µm GHP membrane prior to the exposure experiments to remove all MP. The seawater leachate had a pH of 7.88, salinity of 22.95 ppt, conductivity of 36.75 µs/cm and turbidity of 3.41. Meanwhile, the freshwater leachate showed a pH of 7.69, salinity of 2.01, conductivity of 38.61 µs/cm and turbidity of 1.54, respectively.
2.4 Exposures set up
D. magna neonates (5 organisms per replicate) were exposed to the PS leachate in MilliQ and freshwater, and A. salina (5 organisms per replicate) were exposed to the PS leachates in seawater, both at four concentrations (undiluted (100%), 50%, 25% and 10%) for 48 hours in triplicate. The dilutions were prepared in the organism cultivation media stipulated in section 2.2. These acute immobilisation tests were conducted according to the OECD guideline test no. 202 (OECD, 2004). After 12, 24, and 48 hours, immobilisation of each treatment replicate was observed and recorded. Organisms were considered immobile if they did not move after approximately 15 seconds of gentle stirring. The results were expressed as percentage mobility over time.
Based on the initial lethal response toxicity test, an exposure concentration of 50% dilution for D. magna and 10% for A. salina was selected. These leachate concentration percentages were selected based on the highest concentration that did not result in more than 80% mortality after 24 hours as live organisms were required to assess the physiological non-lethal effects.
For the analysis of the oxidative stress status (reactive oxygen species (ROS) level) and the antioxidative response analysis (catalase (CAT) and superoxide dismutase (SOD) activities), 70 neonate D. magna and 200 A. salina per replicate (n =3) were exposed to each of the specified dilutions of the leachates for 24 hours against a control consisting only of the exposure media. Thereafter, one-third of the organisms per replicate were collected for ROS analysis, and the other two-thirds were collected for enzyme extraction and antioxidant defence analysis.
2.5 Oxidative stress status and response assays
For the ROS determination, as a measure of the oxidative stress status, one-third of the mobile daphnids were snap-frozen in liquid nitrogen and thoroughly homogenised in 1.0 mL phosphate-buffered saline (PBS), followed by centrifugation at 3,000 × g at 4°C for 10 minutes. Then, 10 μL of supernatant was used to determine the protein content using a bicinchoninic acid kit (Thermo Fisher Scientific, Waltham, MA, USA). ROS levels were assessed by measuring the oxidation product of 2′,7′-dichlorofluorescein (DCFDA) with excitation and emission spectra at 495 and 529 nm using the DCFDA/H2DCFDA Cellular ROS Assay Kit (catalogue no. ab113851; Abcam, Cambridge MA, USA) as per the manufacturer’s instructions on a high-performance multi-mode microplate reader (SPARK, TECAN, Switzerland) using 20 μL of supernatant from each sample. The fluorescence intensities were normalised by the protein content of each specimen.
For extracting the S9 enzyme fraction, the other two-thirds of the mobile daphnids were snap-frozen in liquid nitrogen and homogenised with a micro-pestle in 20 mM potassium phosphate buffer (pH 7) on ice. Cell debris was removed by centrifugation at 13,000 × g at 4°C for 10 minutes, and the supernatant was used to assess the enzyme activities. The SOD activity was evaluated using the SOD Assay Kit (19160-1KT-F; Sigma-Aldrich, St. Louis, Missouri, USA). CAT (E.C. 1.11.1.6) activity was assayed using hydrogen peroxide as substrate as per Claiborne (1985) on a Tecan Infinite 200 Pro Infinite M Nano+ (Tecan GmbH, Grödig, Austria). Catalase activity was normalised against the protein content, determined as per Bradford (1976), i.e., enzyme activity was calculated as nanokatal per milligram protein.
2.6 Statistical analysis
Statistical analysis was performed using IBM® SPSS® Statistics 28.0.0.0 (190) (2021). Descriptive analysis was performed on all data sets, followed by normality and homogeneity analysis. For the analysis of percentage survival over time, a repeated measures analysis of variance (ANOVA) was performed, followed by Bonferroni posthoc. One-way ANOVA was performed for the antioxidant response data followed by Tukey posthoc tests observing an alpha value of 0.05, indicating statistical significance.
3 Results
D. magna neonates were exposed for 48 h to leachate prepared by incubating PS fragments in freshwater and pure (MilliQ) water, respectively, for 6 months in nature. At the same time, A. salina were exposed to leachate prepared by incubating PS-MP in seawater for six months. For neonate D. magna, exposed to the PS leachate in freshwater (Figure 1A), 53.3% fewer neonates were mobile after 48 h of exposure to the undiluted leachate compared to the control (p = 1.000). At the same time, exposure to the 10%, 25%, and 50% concentrations of the leachate did not reduce daphnid mobility (p = 0.001). However, for neonates exposed to PS-leached in pure water (Figure 1B), mobility was not significantly affected with any of the concentrations of leachate over the 48 h exposure period (p = 0.596).
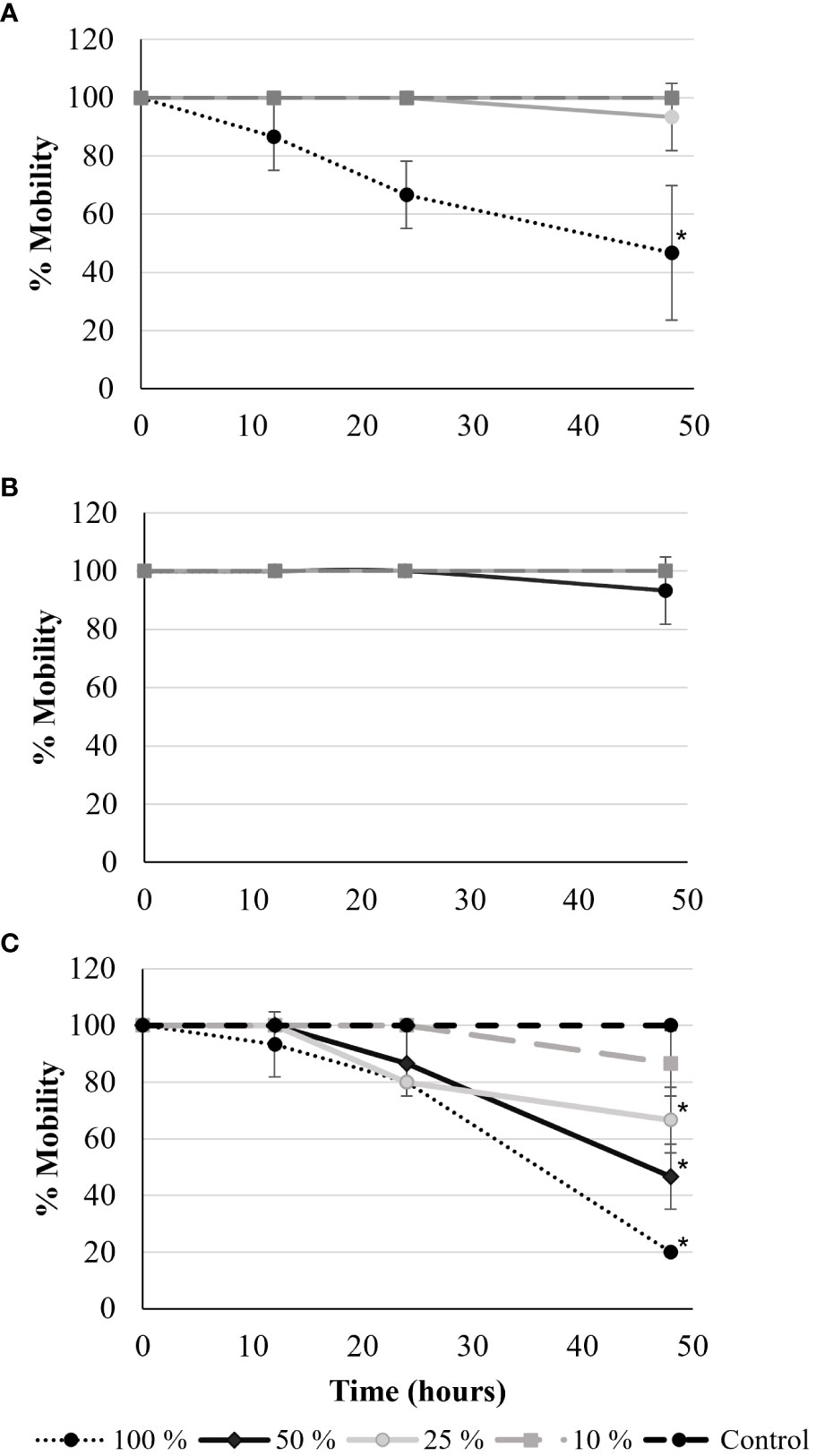
Figure 1 Percentage mobile neonate Daphnia magna over a period of 48 hours exposed to four concentrations of PS leachate prepared in (A) lake water and (B) pure (MilliQ) water. (C) The percentage of mobile Artemia salina exposed to seawater PS leachate over 48 hours. PS fragments were leached in the media for six months, and all microplastic particles were removed prior to exposure. Data points denote the average mobility of live daphnids and artemia ± standard deviation (n = 3). Asterisks (*) denote statistical significance compared to the control (p < 0.05) determined by repeated measures ANOVA.
A. salina (Figure 1C) mobility was the most severely affected by the undiluted seawater leachate, with 80.0% of the organisms remaining mobile after 48 h compared to the control (p < 0.001). With the 50% and 25% concentrations, the mobility was 53.3% (p < 0.001) and 33.3% (p = 0.001), respectively. However, with 10% leachate, mobility was not affected (p = 1.000).
To evaluate physiological adverse effects in live, healthy organisms, the lowest leachate concentration that exhibited sublethal adverse effects was chosen for further assessment. Specifically, a concentration of 50% leachate was selected for D. magna, while for A. salina, a concentration of 10% leachate was deemed appropriate. The selection was based on the data obtained in Figure 1.
The cellular ROS concentration relative to the protein content was not elevated after 48 h of exposure to the 50% dilution of the freshwater PS-leachate (p = 0.116; Figure 2A). The ROS concentration in the neonates exposed to the 50% diluted pure water PS-leachate for 48 h was increased by 24.2% (p = 0.022; Figure 2A). As for A. salina after 48 h of exposure to the 10% seawater PS-leached (Figure 2B), the cellular ROS level was statistically equivalent compared to the control (p = 0.064).
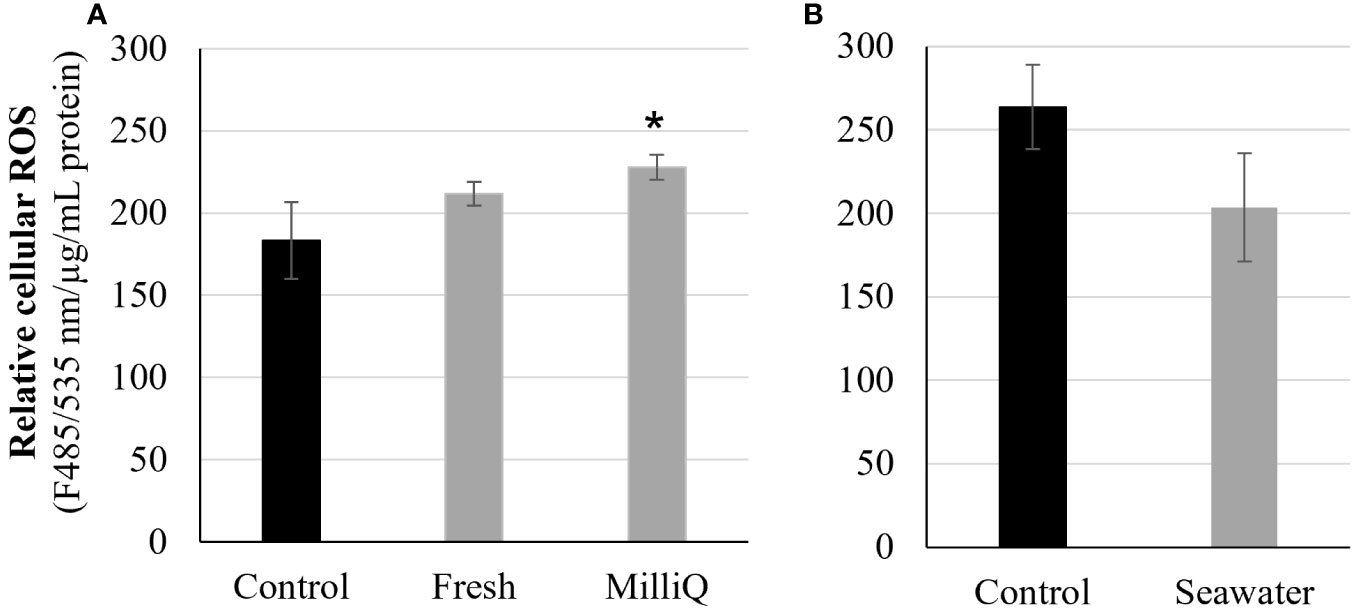
Figure 2 Relative cellular reactive oxygen species (ROS) (fluorescence/mg/mL protein) levels in (A) neonate Daphnia magna exposed to 50% PS-leachate in freshwater and pure water as well as (B) Artemia salina exposed to 10% PS-leachate in seawater after 48 (h) Bars represent the average ROS (fluorescence/mg/mL protein) concentration ± standard deviation (n = 3). Asterisks (*) denote statistical significance compared to the control (p < 0.05) determined by one-way ANOVA and Tukey posthoc.
The SOD activity was significantly elevated in neonate daphnids after 48 h of exposure to both freshwater PS-leachate as well as pure water PS-leachate compared to the control (Figure 3A). With exposure to the freshwater PS-leachate, the SOD activity was increased 104.6% (p = 0.011) and 100.3% with exposure to the pure water PS leachate (p = 0.0140). The SOD activity in A. salina (Figure 3B) was statistically equal to that of the control after 48 h of exposure to the seawater PS leachate (p = 0.479).
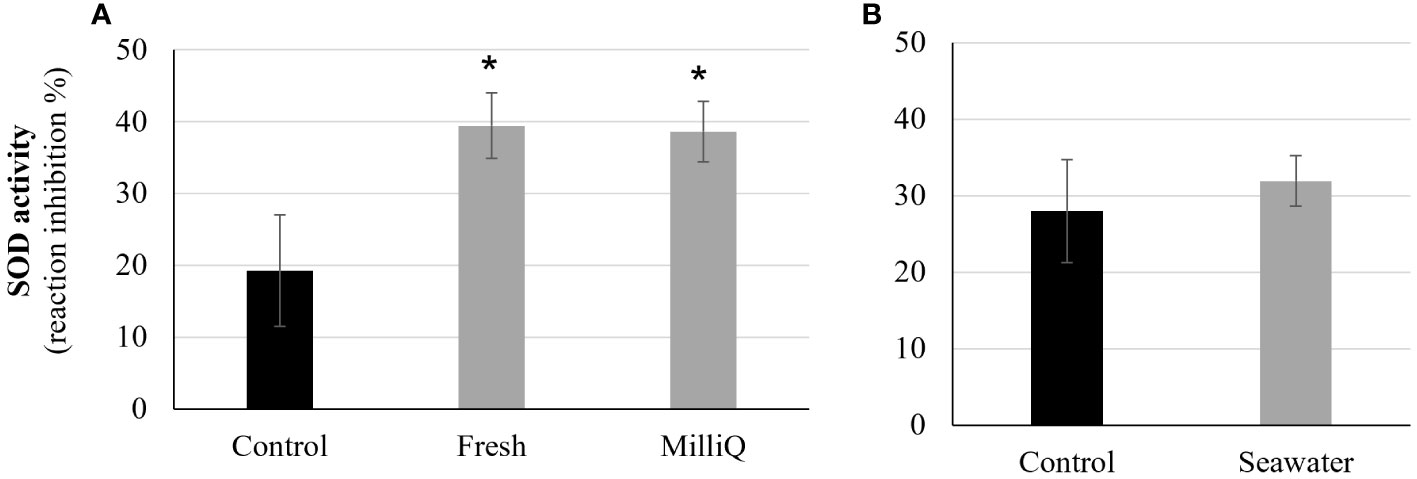
Figure 3 Superoxide dismutase (SOD) activity (expressed as percentage inhibition of the coupled reaction) in (A) neonate Daphnia magna exposed to 50% PS-leachate in freshwater and pure water as well as (B) Artemia salina exposed to 10% PS-leachate in seawater for 48 (h) Bars represent the average SOD activity ± standard deviation (n = 3). Asterisks (*) denote statistical significance compared to the control (p < 0.05) determined by one-way ANOVA and Tukey posthoc.
The CAT activities in neonate daphnids were unchanged relative to the control CAT activity after 48 h of exposure to freshwater as well as pure water PS-leachate (p = 0.897; Figure 4A). However, in A. salina (Figure 4B), CAT activity was significantly increased by 127.9% (p = 0.002).
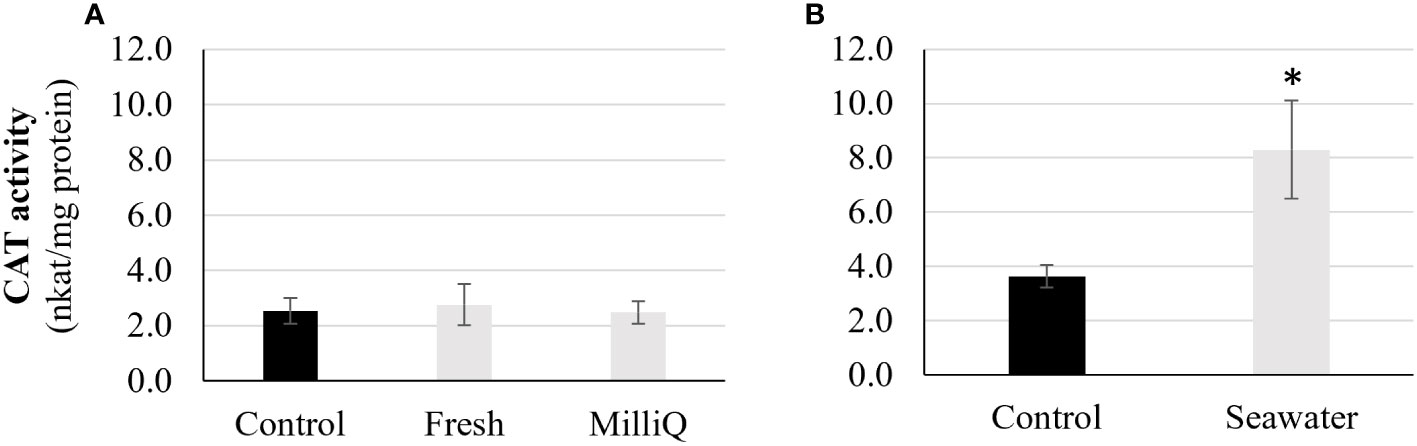
Figure 4 Catalase (CAT) activity (nkat/mg protein) in (A) neonate Daphnia magna exposed to 50% PS-leachate in freshwater and pure water as well as (B) Artemia salina exposed to 10% PS-leachate in seawater for 48 (h) Bars represent the average CAT activity ± standard deviation (n = 3). Asterisks (*) denote statistical significance compared to the control (p < 0.05) determined by one-way ANOVA and Tukey posthoc.
4 Discussion
The goal of this study was to compare the toxicities of PS leachates prepared in three distinct media, seawater, freshwater, and sterile, pure water (MilliQ). These media were selected to represent the properties of natural water bodies in terms of pH, salinity, conductivity, and turbidity, compared to sterile, deionized, and demineralized water. The leachates produced using the various media resulted in significantly different toxic responses in the bioindicator organisms tested. Notably, PS leachate in MilliQ water was significantly less toxic to D. magna compared to PS leachate in freshwater as is evidenced by the immobilization over time. The immobile organisms were not swimming after 15 seconds of agitation. They had all sunken to the bottom of the exposure vessel and their antennae or feeding apparatus showed no movement, which could indicate physiological toxicity leading to death rather than a physical impairment. Additives, which are mostly bound to the MPs by weak dispersion forces (Zhang and Chen, 2014), can leach from plastic materials with fragmentation and degradation accelerating the release of chemicals (Engler, 2012). Since all microplastic fragments were removed before exposure, only leached chemicals could have contributed to the observed immobilization, an established indicator of acute toxicity (OECD, 2004). The additives used in the manufacture of the PS could not be disclosed by the supplier. Nevertheless, several common plastic additives (Smith and Taylor, 2002; Samadi et al., 2022) have been identified as toxic to D. magna and other aquatic organisms (Thaysen et al., 2018; Schiavo et al., 2021; Song et al., 2021; Blinova et al., 2023).
In general, D. magna was less susceptible to the PS-leachate. Only the undiluted PS-leachate in freshwater caused significant changes in mobility. Lithner et al. (2012) leached plastics from old electronic products in water for three days at the same concentration as used in the present study (100 g/L). They found that after acute exposure (24 h and 48 h) the leachate was non-toxic to D. magna. Furthermore, in 2009, Lithner (2011) reported that leachate prepared from PS (100 g/L) did not cause immobilisation of D. magna. However, as deionized water was used, this result concurs with our immobilization findings of daphnids exposed to PS leachate prepared in MilliQ water.
A previous study reported an LD50 of 67 mg/L for 1 μm PS beads in D. magna (Miloloža et al., 2021). Nevertheless, the PS concentration used to prepare the leachate in the present study (100 g/L) far exceeds the highest environmental concentrations reported for PS (Schirinzi et al., 2019; Scopetani et al., 2019; Badylak et al., 2021) and MP in general. Up to 4650 particles/m3 have been detected in freshwater and 102,000 particles/m3 in coastal waters (Wong et al., 2020; Zhang et al., 2020). A conversion from particles per volume to weight per volume was proposed by Leusch and Ziajahromi (2021). However, this accounts for the specific uniform size of reference particles and the density of a specific polymer. Assuming an average size of 1 µm and the density of PS (1.05 g/cm3), the environmental concentrations reported may be equivalent to several orders of magnitude less than the PS concentration used in the present study to prepare the leachate. Thus, leachates from the current level of MP pollution in the environment should not pose a significant threat to D. magna. Nevertheless, MP hotspots may form due to water currents, with localized concentrations exceeding millions of particles per litre (Kane et al., 2020), which should be considered in the comprehensive risk assessment of MPs and their leachates in the environment.
A. salina was significantly more affected by the leachate compared to the D. magna. This may indicate an overall higher susceptibility, or it could be related to the seawater and associated microbiota facilitating a larger concentration of toxicants to be released from the MP. However, in studies comparing the sensitivities of common bioassay organisms to toxicants, A. salina was significantly less susceptible compared to D. magna (Minguez et al., 2014). Furthermore, the study by Wang et al. (2019) showed that Artemia parthenogenetica was not significantly affected by exposure to various concentrations of PS-MP in terms of survival, growth, or development, irrespective of acute or chronic exposure. In support of this, the study by Sait et al. (2021) demonstrated that salinity facilitates additive leaching.
Due to the mobility and distribution of MP, it has been distributed ubiquitously throughout the global marine environment (Cai et al., 2023; Li et al., 2023; Tian et al., 2023). Furthermore, in a review by Wagner et al. (2014), they stated that due to specific oceanic hydrology, plastic pollution accumulates in large oceanic gyres (garbage patches), resulting in more MP than zooplankton. Considering this concentration of plastic pollution in oceanic hotspots, together with the significant impact of the leachate observed on A. salina, the risk of leachate from pollution in marine environments to zooplankton is of concern and poses a threat to marine ecosystems.
Microbes, including bacteria, algae, and fungi, are recognized for their role in facilitating the degradation of plastics through both enzymatic and nonenzymatic hydrolysis of polymers (Devi et al., 2016; Amobonye et al., 2021). Even though the collected waters were filtered through a 0.22 µm filter, removing the majority of microbes, some may have passed into the leaching media (Hahn, 2004). Consequently, the increased toxicity leading to immobilization observed with the leachates in fresh- and seawater, compared to the pure water, could, to some degree, be attributed to microbial action facilitating degradation and allowing more toxicants to be liberated from the plastic material. Furthermore, the potential impact of microbial metabolites, such as exopolysaccharides, on oxidative stress and, consequently, overall toxicity should not be underestimated (Kavitake et al., 2022).
Furthermore, POPs, antibiotics, and heavy metals sorb to plastic debris from the surrounding environment (Liu et al., 2023). Therefore, as the fresh and seawater were collected from natural sources, POPs may have been present and could have contributed to increased toxicity in addition to leached chemicals. Thus, when assessing the risk of MP and leachate, it is essential to these chemical toxicants and their potential additive or synergistic effects on the overall toxicity.
One of the most common non-lethal endpoints observed as a measure of toxicity is oxidative stress and the corresponding antioxidant responses (consisting of superoxide dismutase (SOD), glutathione peroxidase (GPx), catalase (CAT), glutathione reductase(GR), glutathione-S-transferase (GST) and other low molecular weight scavengers such as glutathione, ascorbate, vitamin E, β-carotene and proteins) which combat cellular damage by maintaining the homeostasis of ROS (Yu, 1994). Elevated ROS, an indication of oxidative stress, was only observed in daphnids when exposed to the leachate prepared in pure water. However, this was accompanied by a corresponding increase in the SOD activity, which catalysis the conversion of ROS to hydrogen peroxide. Despite CAT not being activated, this daphnid treatment had the lowest corresponding immortality. Thus, hydrogen peroxide generated from SOD may have been made innocuous by other antioxidative enzymes such as peroxidase, glutathione peroxidase or ascorbate peroxidase (Yu, 1994). In neonates exposed to the leachate prepared in freshwater, ROS homeostasis was maintained as is evident by the still lingering elevated SOD activity. A previous study investigating the effects of PS leachate (50 mg/L) on neonate and adult daphnia found that in neonates ROS was not elevated and SOD activity was not affected but CAT activity was suppressed, with no associated mortality. The authors speculated that the non-enzymatic oxidative stress system may have been involved in maintaining homeostasis. In contrast to D. magna’s antioxidant response, the A. salina’s catalase activity was elevated after 48 h of exposure indicating its involvement in maintaining homeostasis and ensuring mobility and survival. This illustrates the vast differences in species’ response to toxicants.
In conclusion, the physiological data shows that both indicator organisms’ antioxidant response systems could maintain homeostasis at low concentrations of the leachate and considering the currently reported average MP concentrations, leachates from plastic do not pose a severe threat to these organisms. Nevertheless, the potential formation of plastic accumulation points, commonly known as “garbage patches,” raises concerns, as these circumstances may lead to elevated concentrations of leachates, potentially resulting in severe adverse effects on native biota, particularly in saline environments.
Data availability statement
The raw data supporting the conclusions of this article will be made available by the authors, without undue reservation.
Ethics statement
The manuscript presents research on animals that do not require ethical approval for their study.
Author contributions
ME: Conceptualization, Data curation, Funding acquisition, Methodology, Project administration, Resources, Supervision, Validation, Visualization, Writing – original draft, Writing – review & editing. S-AL: Conceptualization, Formal analysis, Methodology, Resources, Supervision, Writing – review & editing. YK: Conceptualization, Investigation, Methodology, Supervision, Validation, Writing – review & editing. RJ: Formal analysis, Investigation, Methodology, Writing – review & editing. YK: Conceptualization, Funding acquisition, Project administration, Supervision, Writing – review & editing.
Funding
The author(s) declare financial support was received for the research, authorship, and/or publication of this article. This work was partly supported by the Nanomaterial Technology Development Program (NRF-2017M3A7B6052455), funded by the South Korean Ministry of Science and the National Research Council of Science & Technology (NST, Global-23-004) grant by the Strategies for Establishing Global Research Networks. The University of Helsinki Library provided open-access funding.
Conflict of interest
ME and RJ were not employed by KIST EU. This is a research institute and we are affiliated via research agreements.
Publisher’s note
All claims expressed in this article are solely those of the authors and do not necessarily represent those of their affiliated organizations, or those of the publisher, the editors and the reviewers. Any product that may be evaluated in this article, or claim that may be made by its manufacturer, is not guaranteed or endorsed by the publisher.
References
Albarano L., Ruocco N., Lofrano G., Guida M., Libralato G. (2022). Genotoxicity in Artemia spp.: An old model with new sensitive endpoints. Aquat. Toxicol. 252, 106320. doi: 10.1016/j.aquatox.2022.106320
Amobonye A., Bhagwat P., Singh S., Pillai S. (2021). Plastic biodegradation: Frontline microbes and their enzymes. Sci. Total Environ. 759, 143536. doi: 10.1016/j.scitotenv.2020.143536
Andrady A. L., Rajapakse N. (2016). “Additives and chemicals in plastics,” in Hazardous chemicals associated with plastics in the marine environment, the Handbook of Environmental Chemistry, vol. 78 . Eds. Takada H., Karapanagioti H. (Cham: Springer), 1–17. doi: 10.1007/698_2016_124
Araújo P. H. H., Sayer C., Giudici R., Poço J. G. R. (2002). Techniques for reducing residual monomer content in polymers: A review. Polym. Eng. Sci. 184, 1442–1468. doi: 10.1002/pen.11043
Badylak S., Phlips E., Batich C., Jackson M., Wachnicka A. (2021). Polystyrene microplastic contamination versus microplankton abundances in two lagoons of the Florida Keys. Sci. Rep. 11, 6029. doi: 10.1038/s41598-021-85388-y
Blinova I., Lukjanova A., Vija H., Mortimer M., Heinlaan M. (2023). Toxicity of plastic additive 1-hydroxycyclohexyl phenyl ketone (1-HCHPK) to freshwater microcrustaceans in natural water. Water 15 (18), 3213. doi: 10.3390/w15183213
Bradford M. M. (1976). A rapid and sensitive method for the quantitation of microgram quantities of protein utilizing the principle of protein-dye binding. Anal. Biochem. 72, 248–254. doi: 10.1006/abio.1976.9999
Bridson J. H., Gaugler E. C., Smith D. A., Northcott G. L., Gaw S. (2021). Leaching and extraction of additives from plastic pollution to inform environmental risk: a multidisciplinary review of analytical approaches. J. Hazard. Mater. 414, 125571. doi: 10.1016/j.jhazmat.2021.125571
Cai C., Zhu L., Hong B. (2023). A review of methods for modeling microplastic transport in the marine environments. Mar. pollut. Bull. 193, 115136. doi: 10.1016/j.marpolbul.2023.115136
Caruso G. (2019). Microplastics as vectors of contaminants. Mar. pollut. Bull. 146, 921–924. doi: 10.1016/j.marpolbul.2019.07.052
Claiborne A. (1985). “Catalase activity,” in CRC Handbook of Methods for Oxygen Radical Research. Ed. Greenwald R. A. (Boca Raton: CRC Press), 283–284.
Crompton T. R. (1979). Additive migration from plastics into food. 1st ed (New York: Pergamon Press).
Dahlbo H., Poliakova V., Mylläri V., Sahimaa O., Anderson R. (2018). Recycling potential of post-consumer plastic packaging waste in Finland. Waste Manage. 71, 52–61. doi: 10.1016/j.wasman.2017.10.033
Devi R. S., Kannan V. R., Natarajan K., Nivas D., Kannan K., Chandru S., et al. (2016). “The role of microbes in plastic degradation”,” in Environmental Waste Management. Ed. Chandra R. (Boca Raton: CRC Press), 341–370.
Do A. T. N., Ha Y., Kwon J. H. (2022). Leaching of microplastic-associated additives in aquatic environments: a critical review. Environ. pollut. 305, 119258. doi: 10.1016/j.envpol.2022.119258
Engler R. E. (2012). The complex interaction between marine debris and toxic chemicals in the ocean. Environ. Sci. Technol. 46 (22), 12302–12315. doi: 10.1021/es3027105
Esterhuizen M., Kim Y. J. (2022). Effects of polypropylene, polyvinyl chloride, polyethylene terephthalate, polyurethane, high-density polyethylene, and polystyrene microplastic on Nelumbo nucifera (Lotus) in water and sediment. Environ. Sci. pollut. Res. 29, 17580–17590. doi: 10.1007/s11356-021-17033-0
European Chemicals Bureau (ECB) (2008) ESIS – European Chemical Substances Information System. Available at: http://ecb.jrc.it/esis/ (Accessed April 12, 2023).
Groh K. J., Backhaus T., Carney-Almroth B., Geueke B., Inostroza P. A., Lennquist A., et al. (2019). Overview of known plastic packaging-associated chemicals and their hazards. Sci. Total Environ. 651, 3253–3268. doi: 10.1016/j.scitotenv.2018.10.015
Gunaalan K., Fabbri E., Capolupo M. (2020). The hidden threat of plastic leachates: A critical review on their impacts on aquatic organisms. Water Res. 184, 116170. doi: 10.1016/j.watres.2020.116170
Hahladakis J. N., Velis C. A., Weber R., Iacovidou E., Purnell P. (2018). An overview of chemical additives present in plastics: Migration, release, fate and environmental impact during their use, disposal and recycling. J. Hazard. Mater. 344, 179–199. doi: 10.1016/j.jhazmat.2017.10.014
Hahn M. W. (2004). Broad diversity of viable bacteria in ‘sterile’ (0.2 μm) filtered water. Res. Microbiol. 155 (8), 688–691. doi: 10.1016/j.resmic.2004.05.003
Han Y. N., Wei M., Han F., Fang C., Wang D., Zhong Y. J., et al. (2020). Greater biofilm formation and increased biodegradation of polyethylene film by a microbial consortium of Arthrobacter sp. and Streptomyces sp. Microorganisms 8, 1979. doi: 10.3390/microorganisms8121979
International standards organisation (ISO). (2016). 3310-1: Test Sieves Technical Requirements and Testing—Part 1: Test Sieves of Metal wire Cloth. Geneva, Switzerland: ISO.
Kalčíková G., Zagorc-Končan J., Žgajnar Gotvajn A. (2012). Artemia salina acute immobilization test: a possible tool for aquatic ecotoxicity assessment. Water Sci. Technol. 66 (4), 903–908. doi: 10.2166/wst.2012.271
Kane I. A., Clare M. A., Miramontes E., Wogelius R., Rothwell J. J., Garreau P., et al. (2020). Seafloor microplastic hotspots controlled by deep-sea circulation. Science 368 (6495), 1140–1145. doi: 10.1126/science.aba589
Kavitake D., Veerabhadrappa B., Sudharshan S. J., Kandasamy S., Devi P. B., Dyavaiah M., et al. (2022). Oxidative stress alleviating potential of galactan exopolysaccharide from Weissella confusa KR780676 in yeast model system. Sci. Rep. 12, 1089. doi: 10.1038/s41598-022-05190-2
Kwan C. S., Takada H. (2016). “Release of additives and monomers from plastic wastes,” in Hazardous chemicals associated with plastics in the marine environment. The Handbook of Environmental Chemistry, vol. 78 . Eds. Takada H., Karapanagioti H. (Cham: Springer), 51–70. doi: 10.1007/698_2016_122
Leusch F. D., Ziajahromi S. (2021). Converting mg/L to particles/L: Reconciling the occurrence and toxicity literature on microplastics. Environ. Sci. Technol. 55 (17), 11470–11472. doi: 10.1021/acs.est.1c04093
Li J., Shan E., Zhao J., Teng J., Wang Q. (2023). The factors influencing the vertical transport of microplastics in marine environment: A review. Sci. Total Environ. 870, 161893. doi: 10.1016/j.scitotenv.2023.161893
Lithner D. (2011). Environmental and health hazards of chemicals in plastic polymers and products. PhD thesis (Sweden: University of Gothenburg).
Lithner D., Damberg J., Dave G., Larsson Å. (2009). Leachates from plastic consumer products-screening for toxicity with Daphnia magna. Chemosphere 74, 1195–1200. doi: 10.1016/j.chemosphere.2008.11.022
Lithner D., Halling M., Dave G. (2012). Toxicity of electronic waste leachates to Daphnia magna: screening and toxicity identification evaluation of different products, components, and materials. Arch. Environ. Contam. Toxicol. 62, 579–588. doi: 10.1007/s00244-011-9729-0
Lithner D., Larsson Å., Dave G. (2011). Environmental and health hazard ranking and assessment of plastic polymers based on chemical composition. Sci. Total Environ. 409, 3309–3324. doi: 10.1016/j.scitotenv.2011.04.038
Liu P., Dai J., Huang K., Yang Z., Zhang Z., Guo X. (2023). Sources of micro (nano) plastics and interaction with co-existing pollutants in wastewater treatment plants. Crit. Rev. Environ. Sci. Technol. 53 (7), 865–885. doi: 10.1080/10643389.2022.2095844
Mammo F. K., Amoah I. D., Gani K. M., Pillay L., Ratha S. K., Bux F., et al. (2020). Microplastics in the environment: Interactions with microbes and chemical contaminants. Sci. Total Environ. 743, 140518. doi: 10.1016/j.scitotenv.2020.140518
Miloloža M., Kučić Grgić D., Bolanča T., Ukić Š, Cvetnić M., Ocelić Bulatović V., et al. (2021). Ecotoxicological assessment of microplastics in freshwater sources—A review. Water 13 (1), 56. doi: 10.3390/w13010056
Minguez L., Di Poi C., Farcy E., Ballandonne C., Benchouala A., Bojic C., et al. (2014). Comparison of the sensitivity of seven marine and freshwater bioassays as regards antidepressant toxicity assessment. Ecotoxicol. 23, 1744–1754. doi: 10.1007/s10646-014-1339-y
OECD. (2004). Daphnia sp. Acute Immobilisation Test, OECD Guidelines for the Testing of Chemicals, Section 2, OECD Publishing Paris. doi: 10.1787/9789264069947-en
Organisation for Economic Co-operation and Development (OECD). (2009). Emission Scenario Document on Plastic Additives: Series on Emission Scenario Documents (Paris: OECD Environmental Health and Safety Publications No. 3. OECD Environment Directorate). doi: 10.1787/9789264221291-en
Patti T. B., Fobert E. K., Reeves S. E., da Silva K. B. (2020). Spatial distribution of microplastics around an inhabited coral island in the Maldives, Indian Ocean. Sci. Total Environ. 748, 141263. doi: 10.1016/j.scitotenv.2020.141263
Pflugmacher S., Sulek A., Mader H., Heo J., Noh J. H., Penttinen O. P., et al. (2020). The influence of new and artificial aged microplastic and leachates on the germination of Lepidium sativum L. Plants 9, 339. doi: 10.3390/plants9030339
Prabhu P. P., Pan K., Krishnan J. N. (2022). Microplastics: Global occurrence, impact, characteristics and sorting. Front. Mar. Sci. 9. doi: 10.3389/fmars.2022.893641
Reilly K., Ellis L. A., Davoudi H. H., Supian S., Maia M. T., Silva G. H., et al. (2023). Daphnia as a model organism to probe biological responses to nanomaterials-from individual to population effects via adverse outcome pathways. Front. Toxicol. 5. doi: 10.3389/ftox.2023.1178482
Sait S. T., Sørensen L., Kubowicz S., Vike-Jonas K., Gonzalez S. V., Asimakopoulos A. G., et al. (2021). Microplastic fibres from synthetic textiles: Environmental degradation and additive chemical content. Environ. pollut. 268, 115745. doi: 10.1016/j.envpol.2020.115745
Samadi A., Kim Y., Lee S. A., Kim Y. J., Esterhuizen M. (2022). Review on the ecotoxicological impacts of plastic pollution on the freshwater invertebrate Daphnia. Environ. Toxicol. 37, 2615–2638. doi: 10.1002/tox.23623
Schiavo S., Oliviero M., Chiavarini S., Dumontet S., Manzo S. (2021). Polyethylene, Polystyrene, and Polypropylene leachate impact upon marine microalgae Dunaliella tertiolecta. J. Toxicol. Environ. Health A 84 (6), 249–260. doi: 10.1080/15287394.2020.1860173
Schirinzi G. F., Llorca M., Seró R., Moyano E., Barceló D., Abad E., et al. (2019). Trace analysis of polystyrene microplastics in natural waters. Chemosphere 236, 124321. doi: 10.1016/j.chemosphere.2019.07.052
Scopetani C., Chelazzi D., Cincinelli A., Esterhuizen-Londt M. (2019). Assessment of microplastic pollution: occurrence and characterisation in Vesijärvi lake and Pikku Vesijärvi pond, Finland. Environ. Monit. Assess. 191, 652. doi: 10.1007/s10661-019-7843-z
Smith S. H., Taylor L. T. (2002). Extraction of various additives from polystyrene and their subsequent analysis. Chromatographia 56, 65–169. doi: 10.1007/BF02493206
Song J., Na J., An D., Jung J. (2021). Role of benzophenone-3 additive in chronic toxicity of polyethylene microplastic fragments to Daphnia magna. Sci. Total Environ. 800, 149638. doi: 10.1016/j.scitotenv.2021.149638
Sooriyakumar P., Bolan N., Kumar M., Singh L., Yu Y., Li Y., et al. (2022). Biofilm formation and its implications on the properties and fate of microplastics in aquatic environments: a review. J. Hazard. Mater. Adv. 6, 100077. doi: 10.1016/j.hazadv.2022.100077
Thaysen C., Stevack K., Ruffolo R., Poirier D., De Frond H., DeVera J., et al. (2018). Leachate from expanded polystyrene cups is toxic to aquatic invertebrates (Ceriodaphnia dubia). Front. Mar. Sci. 5. doi: 10.3389/fmars.2018.00071
Tian W., Song P., Zhang H., Duan X., Wei Y., Wang H., et al. (2023). Microplastic materials in the environment: Problem and strategical solutions. Prog. Mater. Sci. 132, 101035. doi: 10.1016/j.pmatsci.2022.101035
van Velzen E. U. T., Brouwer M. T., Feil A. (2019). Collection behaviour of lightweight packaging waste by individual households and implications for the analysis of collection schemes. Waste Manage. 89, 284–293. doi: 10.1016/j.wasman.2019.04.021
Wagner M., Scherer C., Alvarez-Muñoz D., Brennholt N., Bourrain X., Buchinger S., et al. (2014). Microplastics in freshwater ecosystems: what we know and what we need to know. Environ. Sci. Eur. 26 (1), 1–9. doi: 10.1186/s12302-014-0012-7
Wang Y., Zhang D., Zhang M., Mu J., Ding G., Mao Z., et al. (2019). Effects of ingested polystyrene microplastics on brine shrimp, Artemia parthenogenetica. Environ. pollut. 244, 715–722. doi: 10.1016/j.envpol.2018.10.024
Wiesinger H., Wang Z., Hellweg S. (2021). Deep dive into plastic monomers, additives, and processing aids. Environ. Sci. Technol. 55, 9339–9351. doi: 10.1021/acs.est.1c00976
Wong J. K. H., Lee K. K., Tang K. H. D., Yap P.-S. (2020). Microplastics in the freshwater and terrestrial environments: Prevalence, fates, impacts and sustainable solutions. Sci. Total Environ. 719, 137512. doi: 10.1016/j.scitotenv.2020.137512
Yu B. P. (1994). Cellular defenses against damage from reactive oxygen species. Physiol. Rev. 74 (1), 139–162. doi: 10.1152/physrev.1994.74.1.139
Zhang X., Chen Z. (2014). Observing phthalate leaching from plasticized polymer films at the molecular level. Langmuir 30, 17, 4933–4944. doi: 10.1021/la500476u
Keywords: aquatic organisms, planktonic organisms, plastic leachate, microplastic, oxidative stress, ecotoxicology
Citation: Esterhuizen M, Lee S-A, Kim Y, Järvinen R and Kim YJ (2024) Ecotoxicological consequences of polystyrene naturally leached in pure, fresh, and saltwater: lethal and nonlethal toxicological responses in Daphnia magna and Artemia salina. Front. Mar. Sci. 11:1338872. doi: 10.3389/fmars.2024.1338872
Received: 15 November 2023; Accepted: 19 January 2024;
Published: 05 February 2024.
Edited by:
Xuchun Qiu, Jiangsu University, ChinaReviewed by:
Mohamed Mohsen, Jimei University, ChinaCarola Murano, Anton Dohrn Zoological Station Naples, Italy
Copyright © 2024 Esterhuizen, Lee, Kim, Järvinen and Kim. This is an open-access article distributed under the terms of the Creative Commons Attribution License (CC BY). The use, distribution or reproduction in other forums is permitted, provided the original author(s) and the copyright owner(s) are credited and that the original publication in this journal is cited, in accordance with accepted academic practice. No use, distribution or reproduction is permitted which does not comply with these terms.
*Correspondence: Maranda Esterhuizen, bWFyYW5kYS5lc3Rlcmh1aXplbkBoZWxzaW5raS5maQ==