- 1Marine Science Program, Biological and Environmental Science and Engineering Division (BESE), King Abdullah University of Science and Technology (KAUST), Thuwal, Saudi Arabia
- 2Red Sea Research Center (RSRC), King Abdullah University of Science and Technology (KAUST), Thuwal, Saudi Arabia
- 3Computational Bioscience Research Center (CBRC), King Abdullah University of Science and Technology (KAUST), Thuwal, Saudi Arabia
- 4GEOMAR Helmholtz Centre for Ocean Research Kiel, Kiel, Germany
- 5OceanX, New York, NY, United States
- 6National Center for Wildlife (NCW), King Abdul Aziz, Riyadh, Saudi Arabia
Rhodaliids, a specific family of siphonophores inhabiting the benthic zone, have remained enigmatic due to their rarity and elusive nature. These unique organisms, primarily found in open ocean habitats, exhibit distinctive features and are characterized by complex structures. During the Red Sea Decade Expedition, two rhodaliid specimens were collected at the sea bed at water depths of 438 and 495 meters. Regardless of challenges in specimen preservation, detailed morphological analysis revealed unique characteristics. Genetic analysis, employing the 16S rRNA marker, revealed one specimen closely related to Thermopalia taraxaca, while the taxonomic traits of this specimen suggested the identification of Archangelopsis jagoa. While most of the morphological features of the second specimen were also very similar to A. jagoa, some differences in coloration suggest the discovery of either an unusual colour variant or a potential new species within this genus. Notably, this study reports the first sequencing of A. jagoa. This species demonstrated a capacity to thrive in low-oxygen environments, challenging conventional assumptions about their habitat requirements. Despite difficulties in specimen handling and genetic analysis limitations due to a lack of comprehensive data, this research sheds light on the elusive world of benthic rhodaliids.
Introduction
Siphonophores, a unique order within hydrozoan cnidarians, are renowned for their remarkable structural complexity. These delicate colonial organisms primarily inhabit the vast expanses of the open ocean. Among their various adaptations, the majority of siphonophore species rely on specialized structures known as nectophores for graceful and efficient propulsion through the water column. Additionally, some siphonophores feature buoyant floats called pneumatophores, which assist in maintaining their position in the water column. A defining characteristic of siphonophores is their elongated stem, to which numerous repeating units called cormidia are attached. Each cormidium comprises different types of medusoid and polypoid zooids that are fulfilling specific functions such as feeding, reproduction, protection, and buoyancy (Mapstone, 2014).
Rhodaliids, a distinctive family of siphonophores, are characterized by their benthic lifestyle, setting them apart from their pelagic counterparts (Pugh, 1983). Almost all species of this family are poorly known, and most of them were described from a few specimens, some of which collected more than 100 years ago and never recorded again (Sanamyan et al., 2018). Also the colonies of benthic siphonophores comprise a series of cormidial units, however, arranged in whorls along a shorter stem or corm. Positioned at the uppermost end of this corm is the pneumatophore, a gas-filled structure filled with carbon monoxide. Immediately following the pneumatophore are the nectophores, efficient medusoid zooids responsible for propelling the colony in various directions through coordinated contractions.
Historically assumed to occupy the deeper waters along continental shelves, rhodaliids have challenged conventional assumptions by being identified in various oceanic regions. They exhibit species-specific preferences for depths, ranging from 100 meters to 3,670 meters (Mapstone et al., 2016). Remarkably, there are currently only 15 documented species of rhodaliids, distributed across 10 distinct genera (Table 1). Unlike other siphonophores, they are classified as epibenthic due to their tethering to the seabed using some of their numerous tentacles, while their bodies extend upwards like buoyant balloons. The freely floating tentacles can extend to considerable lengths, in particular when outstretched for feeding (Mackie and Boag, 1963; Hissmann, 2005). Also the attaching tentacles can be extremely elongated, even in calm sea conditions (Hissmann, 2005). This elongation strategy likely helps prevent colonies from being completely carried away by bottom currents. Notably, the attaching tentacles can also detach distally to facilitate short-distance swimming. When the tentacles are extended, their numerous side branches (tentilla) become clearly visible, despite their relatively small size. Functioning as predators, benthic siphonophores employ their complex network of tentacles, like a giant underwater spider web, and probably use outstretching and retraction motion to lure prey.
As a consequence of their enigmatic nature, rhodaliids remain underexplored in terms of their ecological roles. Their scarcity and vulnerability hampers collection and laboratory study, inhibiting our understanding of their life cycles and larval stages. Like other siphonophores, rhodaliids may also undergo a relatively brief pelagic larval stage (Carré, 1969; Carré and Carré, 1991) followed by a potentially long-lived benthic stage (Pugh, 1983). The geographical isolation of rhodaliids, found both around continental shelves and on highly productive mid-ocean ridges, has probably played a crucial role in promoting speciation within this unique benthic siphonophore family (Mapstone et al., 2016).
In 1977, the very first underwater images of benthic siphonophores were captured during dives with the U.S. American manned submersible Alvin in the Galapagos Rift, but these creatures were not collected at that time. Previously, rhodaliids had only been documented through specimens gathered by early expeditions prior to 1908 (Pugh, 1983). However, recent developments in manned and unmanned underwater vehicles have enabled an increase in our understanding of benthic siphonophores. Out of the 15 known species, five have now been formally described based on pictures or material collected by submersibles (Hissmann et al., 1995; Hissmann, 2005; Mapstone et al., 2016), or citizen science (Manko et al., 2017). Furthermore, invaluable insights into rhodaliids have emerged from underwater photographs and videos recorded during deep-sea vehicle dives. While it remains challenging to precisely identify the species or genus of benthic siphonophores from such images, they offer substantial information about these elusive creatures. These images shed light on the external structure of rhodaliids in life, which is almost never obtainable in its entirety, as well as their behaviour, distribution and habitats.
In this study, we report the morphological and ecological attributes of three filmed and two collected rhodaliid specimens found in the Red Sea. We provide a tentative identification based on observable characteristics and DNA barcoding and a comparison with other known rhodaliid species, particularly Archangelopsis jagoa, the only species previously described from this region (Hissmann et al., 1995).
Materials and methods
During the Red Sea Decade Expedition (RSDE), which occurred between February 4th and June 18th, 2022, on board the R/V OceanXplorer, extensive underwater observations were carried out using a remote operated vehicle (ROV Chimera, model: Argus Mariner XL 6000m). This expedition encompassed the coastal regions of the Red Sea within Saudi Arabian waters, ranging from the shoreline to the boundaries of the Exclusive Economic Zone (EEZ), spanning from latitude 16.560°N to 29.303°N. In the course of this expedition, a total of 114 ROV dives were conducted. These dives explored the Saudi Arabian coastline of the Red Sea, investigating the marine environment down to a maximum depth of 2,414 meters. The specimens described in this study were encountered at three different sites in the Red Sea (Figure 1). They were first photographed and video filmed, and then two of them collected with the suction device attached to the manipulator of the ROV and placed individually in a transparent chamber. Once on board, one of the specimens was filmed alive with a smartphone camera, however, only from above. The second one was only photographed from the side. Both were then frozen at -80°C.
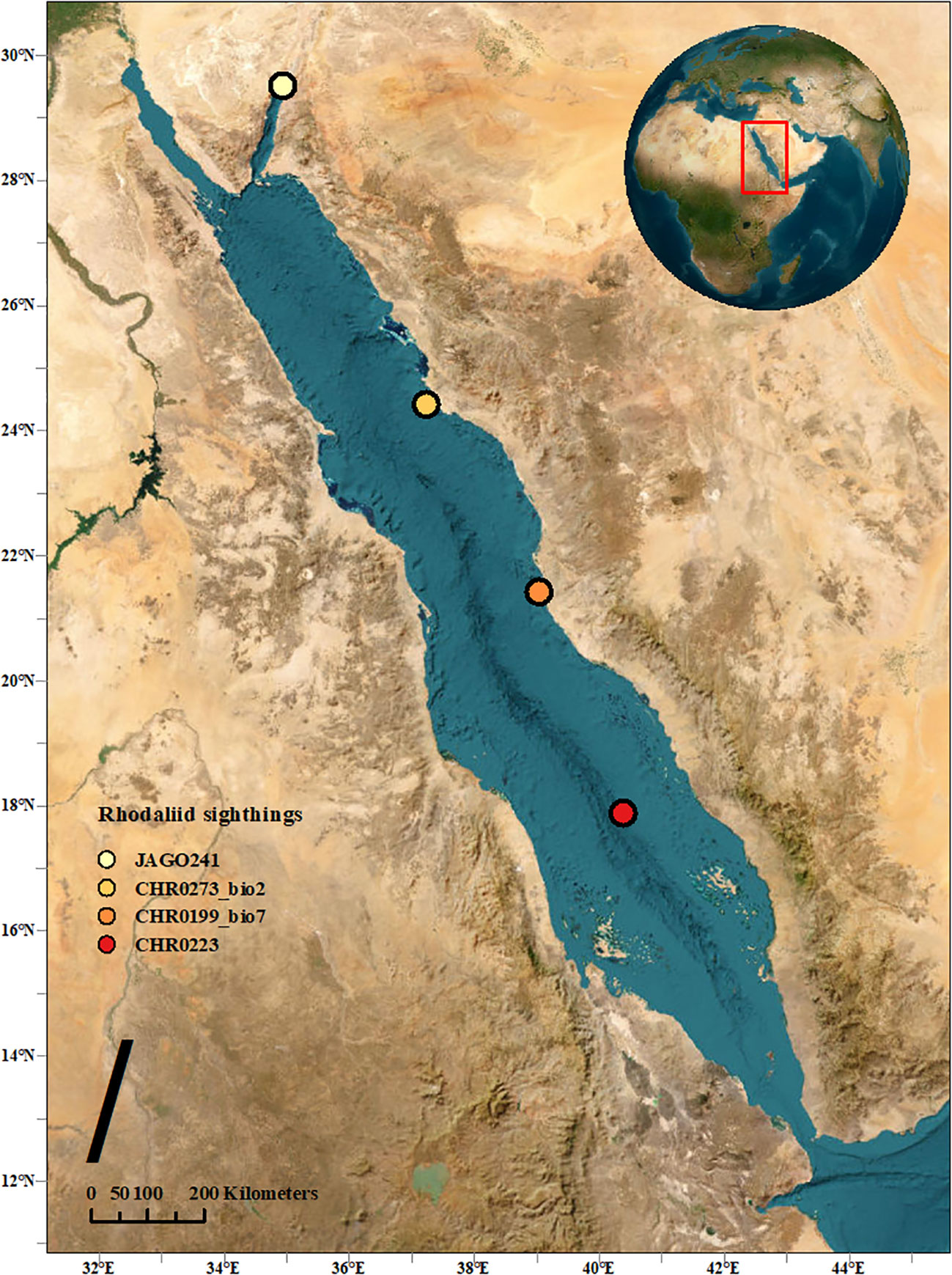
Figure 1 Map indicating the three observed locations in this study, along with the initial sighting location of A. jagoa in the Red Sea.
Morphological evaluation
To identify rhodaliids, certain key characteristics are crucial to consider. These include examining the texture of the pneumatophore and the adjacent aurophore or gas gland, evaluating the type of corm and the presence of cormidia, as well as determining the number of whorls and zooid types present on the corm (Pugh, 1983; Mapstone et al., 2016). Colour and patterning can also be diagnostic, with most rhodaliids displaying an overall bright or pale orange colouration (Dromalia, Stephalia, Thermopalia, Tridensa, Mapstone et al., 2016), or having a pale and almost white overall colour with bright pigments only on the pneumatophore and/or certain cormidial zooids. Examples of the latter include Arancialia (Hissmann, 2005), Archangelopsis (Hissmann et al., 1995; Hissmann, 2005), and Rhodalia (Araujo, 2012).
DNA extraction
Total genomic DNA was extracted from one specimen (~10mg) using DNeasy Blood & Tissue kit (Qiagen) following manufacturer’s protocol. The tissue for extraction was obtained from the tentacles, and the elution was performed in a volume of 100 μl. The initial digestion with proteinase K was performed for three hours. A fragment of 16S ribosomal RNA (16S rRNA; ~580 bp fragment) was amplified using modified primers from Cunningham and Buss (1993). 16S_F1Mod (TCGACTGTTTACCAAAAACATAGC), and 16S_R2 (ACGGAATGAACTCAAATCATGTAAG). The PCR was prepared using a QIAGEN Multiplex PCR kit consisting of 1x PCR Master Mix, 0.2 M of each primer, 2µl of gDNA and adjusted with RNase-free water to a final volume of 25µl. The thermal cycling condition on SimpliAmp Thermal Cycler was set to 95°C for 15 min for enzyme activation, followed by 35 cycles of denaturation (94°C for 30 s), annealing (50°C for 60 s) and extension (72°C for 60 s), then final extension at 72°C for 5 min. The amplified PCR products were cleaned using AMPure XP Beads (Agencourt) and then Sanger sequenced at KAUST BioScience Core Laboratory. Sequences were trimmed and edited in Geneious Prime 2023.1.2 (https://www.geneious.com). This step ensured the removal of any low-quality bases or regions and generated high-quality sequences for downstream analysis.
Phylogenetic analysis
Twelve 16S sequences belonging to the Rhodaliidae family were downloaded from NCBI and aligned with the sequence from this study. To construct the phylogenetic tree, relevant nucleotide sequences were sourced from GenBank using the search terms: “Rhodaliidae 16S”. Sequences shorter than 400 bp, lacking a 100 bp overlapping region with most sequences at either end during alignment, and complete mitochondrial genomes were excluded from the analysis. The sequence of Physalia physalis was selected as an outgroup. Alignment and trimming of all sequences were conducted using Geneious. The MUSCLE alignment was performed in MEGA (Tamura et al., 2021), to identify the best substitution model with the lowest Bayesian Information Criterion (BIC) value. The phylogenetic tree was constructed in Mr. Bayes (Huelsenbeck and Ronquist, 2001), using the default parameters in conjunction with the best substitution model. The Bayesian tree generated was visualized in Figtree (Rambaut, 2010) and further edited with Inkscape.
Results and discussion
Two specimens of benthic siphonophores were observed in the Central Red Sea and collected during ROV dives CHR0199 and CHR0273 on 24 February and 12 May 2022 at depths of 495 and 438 m, respectively (Supplementary Materials S1, S2). Another specimen was recorded further south on the benthic transect of the ROV dive CHR0223 on 18 March 2022 at 657.21 m depth. The location of the dives was 21.426’N, 39.032’E; 24.422’N, 37.228’E; and 17.887’N, 40.379’E off the Saudi coast respectively, with a considerable distance of almost 800 km among the most distant positions. Water temperature at the collection sites was 21.7°C, salinity was 40.5 psu, and oxygen was 2.16 ± 0.3 mg O2 L-1 on average for all the stations (Table 2). We encountered these organisms in only three out of 114 dives (~600 hours), suggesting that the benthic rhodaliids in the Red Sea are exceptionally rare. Despite their scarcity, these organisms seem to be distributed across the entire span of the Red Sea, ranging from close to the coast to the middle of the sea (Figure 1). Unfortunately, one of the collected organisms, CHR0199_bio7 was lost due to contingencies during the archiving process of the numerous biological samples, and we were unable to include it in our subsequent morphological and genetic analyses. Furthermore, when attempting to examine the physiological characteristics of the second specimen, CHR0273_bio2, we encountered another significant challenge, as the specimen underwent a rapid and severe degradation process. Consequently, conclusive morphological examinations of the two specimens were not possible. Our morphological description of the specimens is therefore exclusively based on thorough analysis of the still and video images (see Supplementary Materials S1-S6).
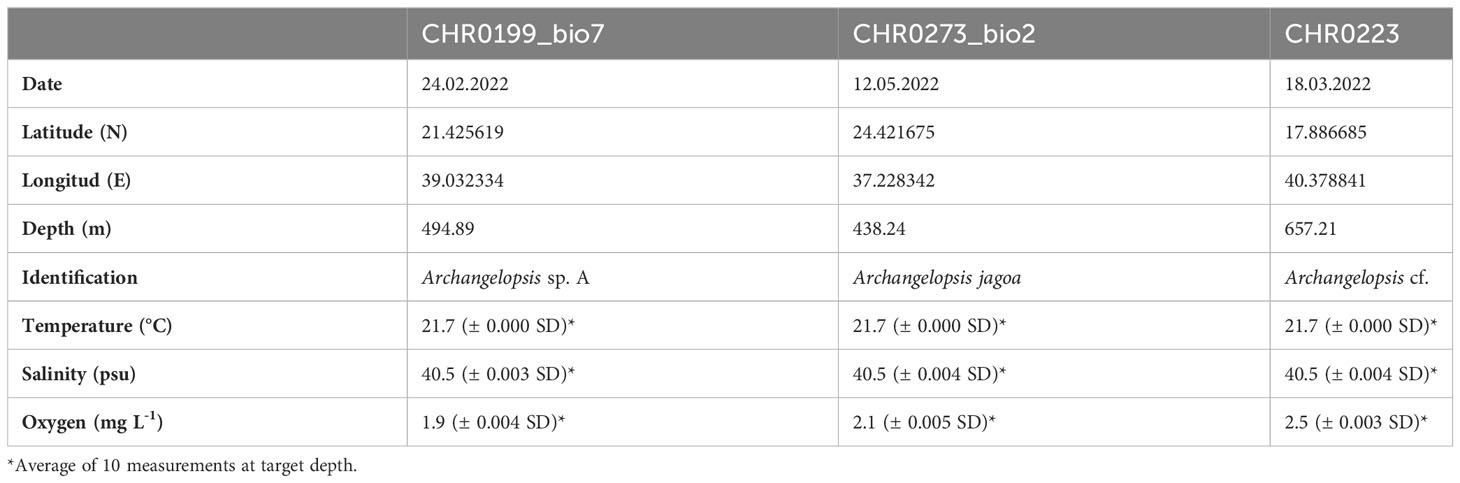
Table 2 Data for the three specimens of benthic siphonophores recorded during the Red Sea Decade Expedition in Saudi waters.
Genetic analysis
Sequence data of 16S has been deposited in Genbank (Accession number OR095776). Constructing a robust phylogenetic tree for the rhodaliid family has proven challenging due to the limited sequencing data available; only three species (Dendrogramma enigmatica, Stephalia dilata, and Thermopalia taraxaca) have been sequenced previously for this genetic marker (Table 1). Although genetic information on this family is very limited in public databases, a high posterior probability value of 98%, strongly supported that CHR0273_bio2 is more closely related to T. taraxaca compared to S. dilata or D. enigmatica, based on the phylogenetic inference (Figure 2, yellow box). However, it is crucial to note that a broader sequencing effort encompassing more individuals and species within this family is essential to draw conclusive inferences.
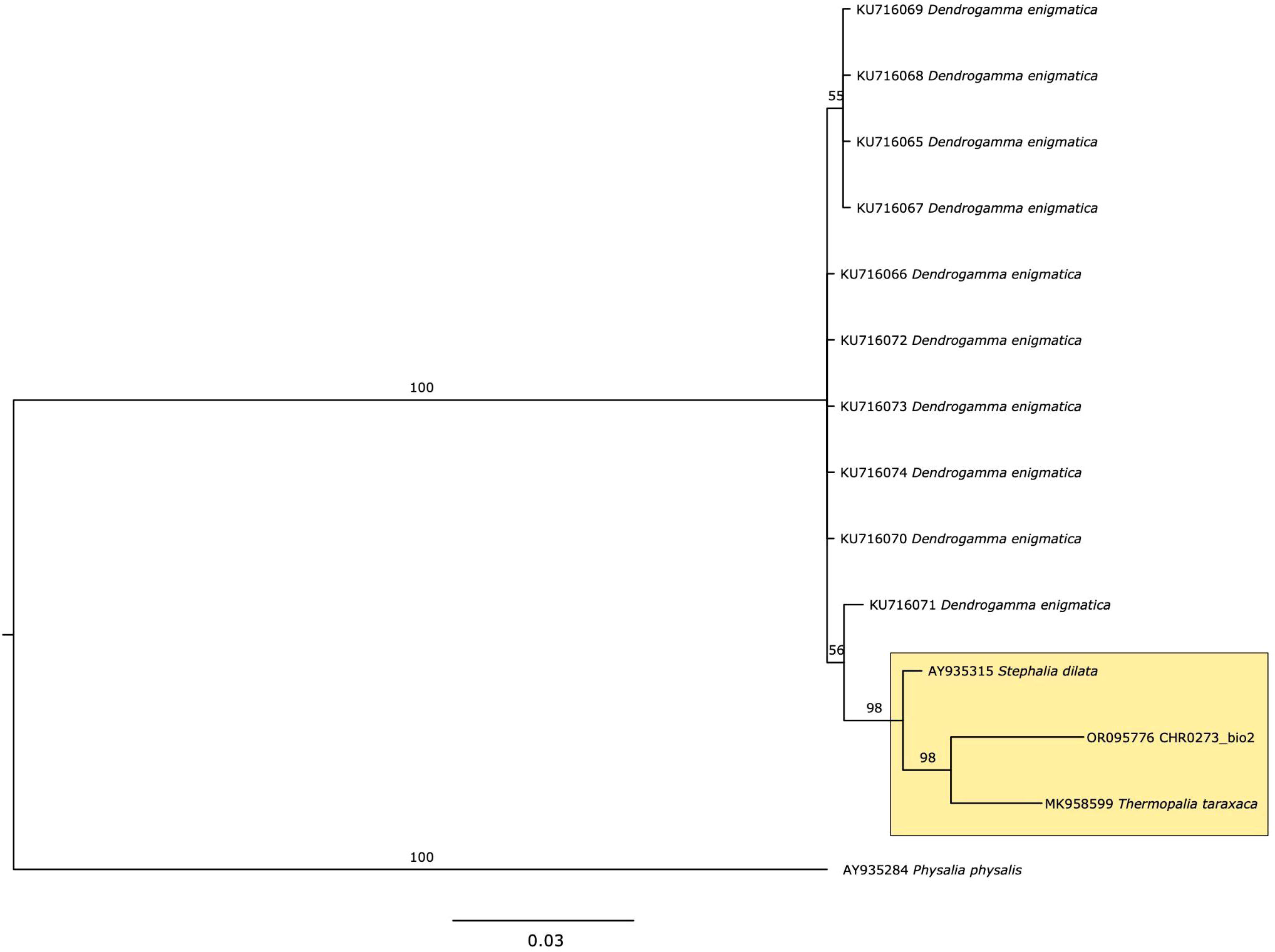
Figure 2 Phylogeny of 16S for Rhodaliidae family, using Bayesian analysis. Values on the branch labels indicate posterior probabilities.
Taxonomic identification
The combination of a smooth-walled pneumatophore and an aurophore with papilliform appendages (Figures 3, 4; Supplementary Materials S1-S5) was sufficient to establish Archangelopsis as the genus of the specimens. Up to now, Archangelopsis comprises two species, A. typica and A. jagoa. The genus was introduced by Lens and van Riemsdjik (1908) for three specimens of A. typica collected during the Dutch Siboga Expedition to Indonesia 1899–1900. A fourth specimen was found in the East China Sea off SW Japan during the 1906 Albatross Expedition (Bigelow, 1913). Almost a century later a second species A. jagoa was introduced for specimens from the Gulf of Aqaba in the Red Sea and the Comoro Islands in the western Indian Ocean (Hissmann et al., 1995; Hissmann, 2005). It is likely, therefore, that the populations of A. typica which inhabit the Indonesian Archipelago are completely isolated from those of A. jagoa in the Comoro Islands and the Gulf of Aqaba. A. jagoa is probably restricted to habitats along the continental shelves of the Indian Ocean including the Red Sea.
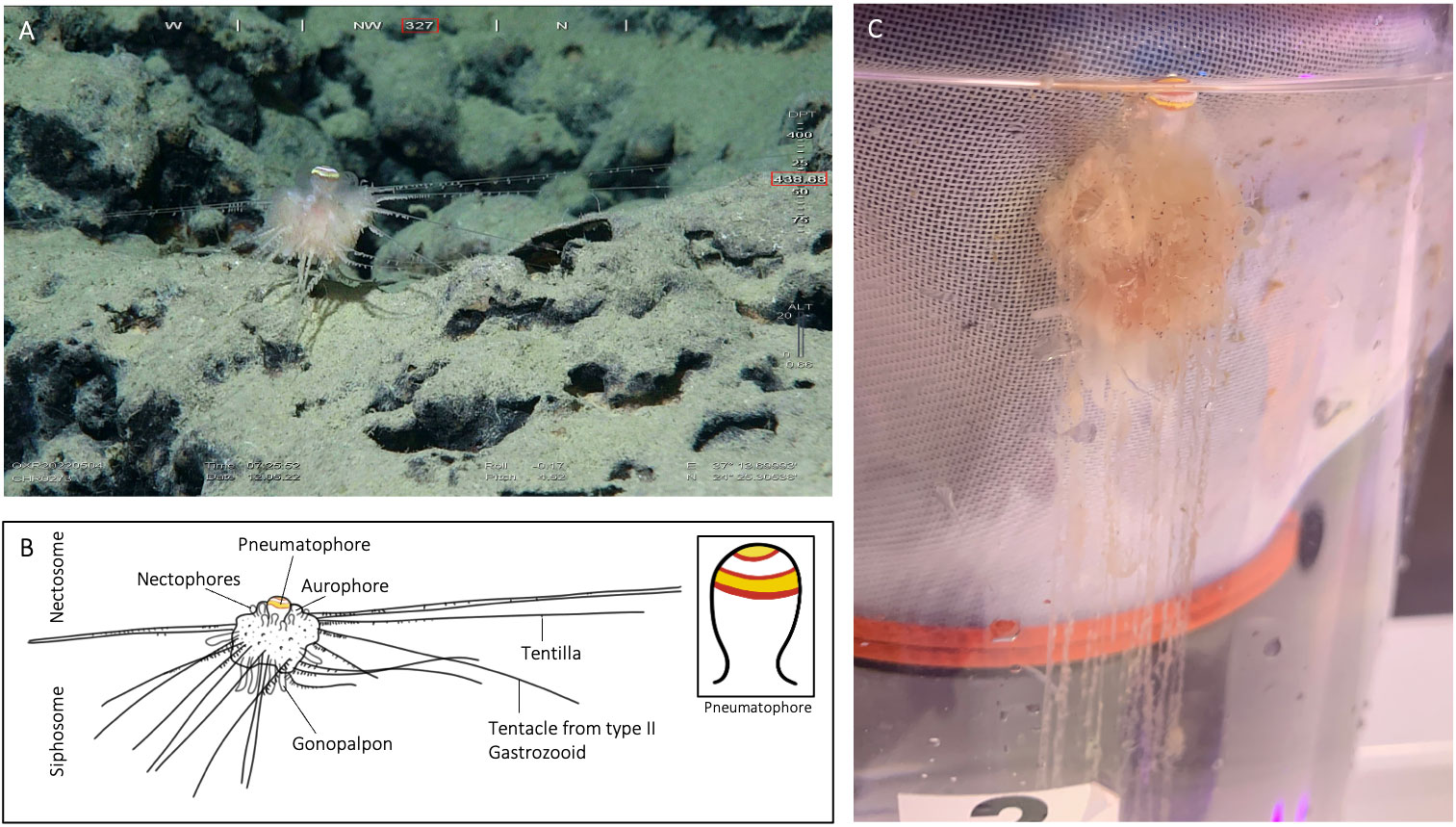
Figure 3 Illustration of specimen CHR0273_bio2 from the RSDE expedition: (A) ‘walking’ along the bottom by detaching and reattaching tentacles, probably disturbed by the presence of the ROV; (B) schematic drawing of the specimen, with inset of pneumatophore pattern rings; (C) same specimen after capture in container on board, lateral view.
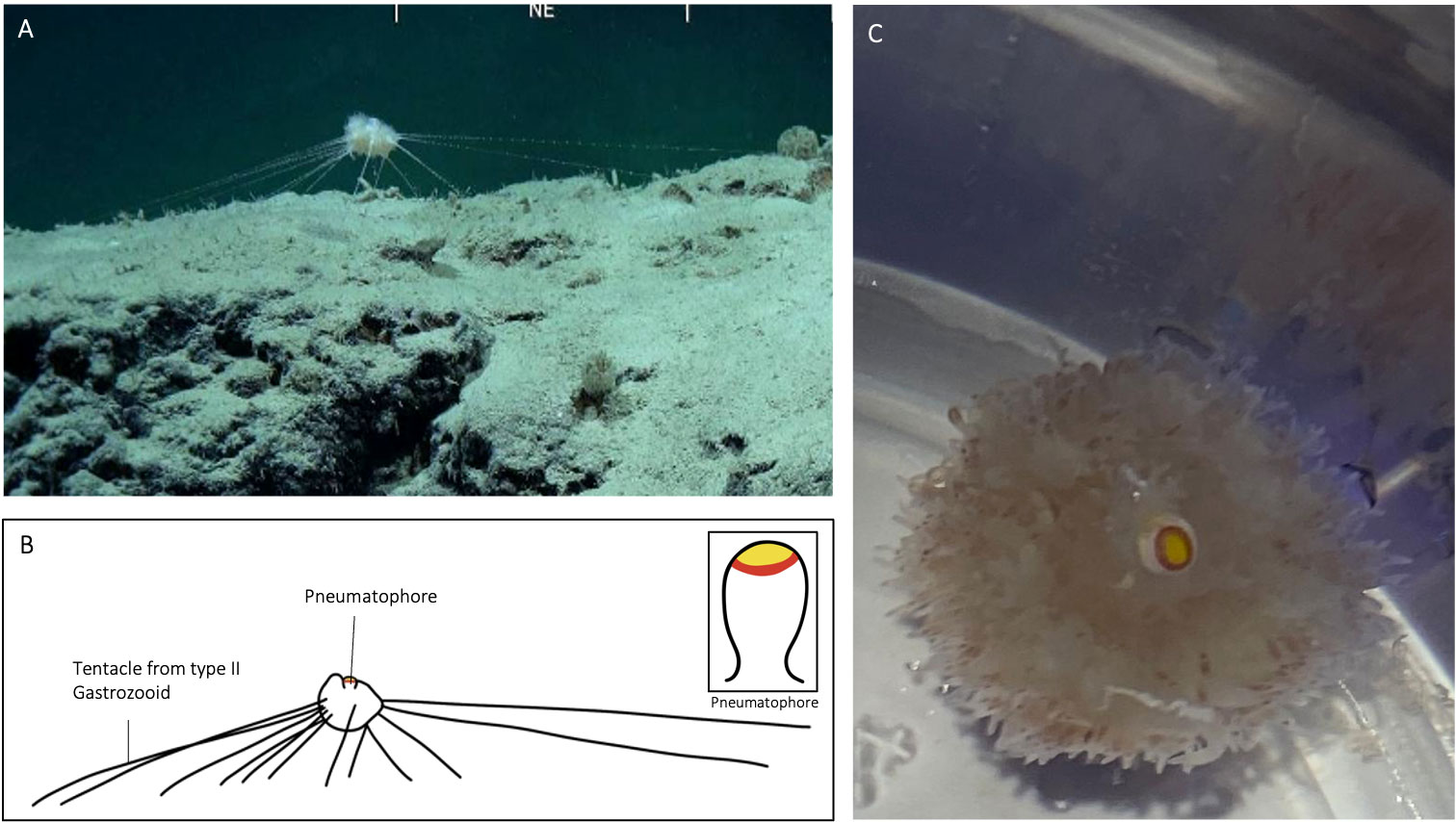
Figure 4 Illustration of specimen CHR0199_bio7 from the RSDE expedition: (A) suspended above the seafloor whilst ‘in situ’; (B) schematic drawing of specimen, with inset of pneumatophore coloration; (C) same specimen after capture in container on board, view from above.
In A. jagoa there are up to six circular pigment rings around the pneumatophore, including a particularly broad and distinctive white band just above the maximum circumference (Hissmann et al., 1995; Hissmann, 2005). The pneumatophore of CHR0273_bio2 had six rings that were coloured (Figure 3), in sequence from the apical pole, yellow, red, white, red, yellow, and red. Specimen CHR0273_bio2 had thus the same pneumatophore ring pattern as reported in A. jagoa (Hissmann et al., 1995). Specimen CHR0199_bio7 had a different ring coloration pattern. The pneumatophore of specimen CHR0199_bio7 had only three circular pigment rings, a distinctive wide yellow apex followed by a red ring, and white tissue (Figure 4; Supplementary Material S1).
In A. jagoa and CHR0273_bio2, the aurophore has appendages that are clearly white in colouration on their tips (Hissmann et al., 1995; Figure 3). In CHR0199_bio7, all appendages of the aurophore appeared to be pale and colourless (note the release of a gas bubble from the aurophore in the Supplementary Material Video S3). While the nectophores (swimming-bells) of A. jagoa have a bright red circular canal around the ostium (opening) and sometimes reddish radial canals (Hissmann et al., 1995), CHR0199_bio7 had nectophores without any coloration of the canals. The rhythmical contraction of the nectophores is clearly visible in the videos of CHR0199_bio7 recorded on board (see Supplementary Materials S3-S5). We counted about ten of them arranged around the nectosome. The gonopalpons are numerous on both specimens. The gonopalpons of specimen CHR0199_bio7 (Figure 4) had a red pigment spot at their distal end which is not so distinctively visible in CHR0273_bio2 (Figure 3), although its gonopalpons are slightly pigmented too. Gastrozooids of type II were difficult to identify on both specimens. On both specimens we observed several tubular elongated structures hanging out of the lower corm that were probably type II gastrozooids. In A. jagoa type II gastrozooids usually have a single, longitudinal bright orange stripe in the central stomach region (Hissmann et al., 1995). Such bright stripes were not visible on the images of both specimens. While the tentilla and tentacles of the CHR0273_bio2 specimen had a slightly orange colouration (Figure 3), they seemed to be colourless in CHR0199_bio7 (Figure 4; Supplementary Materials S3-S5). Both specimens exhibited sizes two to three times greater than the maximum diameter of the previously documented A. jagoa (Hissmann et al., 1995). Specifically, specimen CHR0199_bio7 was bigger than CHR0273_bio2, measuring five and three cm in length respectively.
All rhodaliids are dioecious, and in A. jagoa, the distinction between female and male specimens is evident, as females display a distinct bright orange/red bulge around the opening of the sub-umbrella cavity of the medusoid gonophore, whereas male gonophores appear as smaller, sac-like structures lacking any reddish coloration (Hissmann et al., 1995; Hissmann, 2005). The identification of gonophores in our recorded stills and videos proved challenging. The CHR0273_bio2 specimen is more likely to be a male, given the absence of the characteristic reddish bulge. Pale red colour-rings occasional observed in the videos of CHR0199_bio7 suggest their possible classification as female gonophores.
Rhodaliid bracts are usually triangular shaped, large zooids that are attached to the base of the cormidial stem and easily get detached. The mostly transparent bracts in A. jagoa are distinguished by the bright orange/red coloration of the bracteal canal which divides into two branches distally (Hissmann et al, 1995). No bracts were detected on the images of the CHR0273_bio2 and CHR0199_bio7 specimens. That, however, does not mean that they were not present. They might have gotten lost during handling of the specimens, or, at least on the CHR0199_bio7 specimen, they might be completely transparent and lack any pigmentation in their bracteal canals.
Both specimens were tethered to the substratum by tentacles of type II gastrozooids when encountered by the ROV. The number of tentacles that were used to attach the animals to the ground were 18 for CHR0199_bio2 and 14 for CHR0273_bio7 (Figures 3, 4). The number of attaching tentacles may depend on the type of substrate and the intensity of water current. The tentacles were greatly extended in all directions around the corm. Contrary to the previous existing observations of A. jagoa from the Red Sea, these two specimens were found attached to the top of rocky boulders covered with a thin layer of soft sediment, never on pure sand, as was noted for specimen CHR0223 (Supplementary Material S6).
Researchers of the Australian Institute of Marine Scientists (AIMS) have recently reported sightings of several rhodaliid specimens that are assumed to belong to the genus Archangelopis, maybe even to A. jagoa since the circular pigment rings around the pneumatophore are very similar in number and coloration (Karen Miller, 2020, https://www.aims.gov.au/information-centre/news-and-stories/rare-marine-creature-discovered-australian-waters). The specimens were recorded in a cluster attached to sandy ground between 100 to 150 meters depth in the Kimberley Marine Park off the Western Australian coast. This record would largely increase the geographical and depth distribution of the species within the Indian Ocean.
Based on the colony characteristics described above, both specimens should be assigned to the genus Archangelopsis. While both of them had many taxonomic characteristics that resemble those of A. jagoa, specimen CHR0199_bio7 showed distinct differences in coloration. It is possible that this specimen is an unusual colour variant of A. jagoa or belongs to another, perhaps even new species. More observations and detailed morphological analysis of well-preserved specimens are needed to substantiate this assumption. If, besides the mentioned difference, both specimens belong to the same species, then the ring pattern of the pneumatophore would no longer serve as a taxonomical key to identify this species.
Benthic siphonophores belonging to the Rhodaliidae family (Cnidaria, Hydrozoa) are highly delicate, challenging to gather, and consequently, they have received limited research attention. Since they are rarely ever seen, we consider our observations as scientifically valuable and worth sharing. As far as we know, the sequence provided here are the first reported for A. jagoa, which should allow confirming the presence of the species using eDNA or other indirect methods, which may help retrieve further information on the distribution of this elusive taxon. The sequence will also help to eventually build robust phylogenetic trees for the order siphonophore. The records presented here extend the depth range for A. jagoa, initially documented at depths of 250 to 370 meters in the Gulf of Aqaba (Hissmann et al., 1995), with CHR0223 specimen found at a depth of 657 meters. Hypoxic threshold for coastal water ecosystems is commonly used as dissolved oxygen (DO) levels under 2.8 mg L-1 (Diaz and Rosenberg, 1995). For deep sea ecosystems, where DO is naturally lower and no sunlight is available, organisms have evolved adaptations to cope with the environmental constrains. The environmental conditions in which the three specimens were discovered were nearly identical, indicating that these organisms can thrive even in extremely low oxygen levels, close to hypoxia.
Data availability statement
The datasets presented in this study can be found in online repositories. The names of the repository/repositories and accession number(s) can be found in the article/Supplementary Material.
Ethics statement
Ethical approval was not required for the study involving animals in accordance with the local legislation and institutional requirements because we only sample two organisms (invertebrates).
Author contributions
CA-P: Conceptualization, Formal analysis, Investigation, Methodology, Writing – original draft. AS: Conceptualization, Methodology, Writing – review & editing. KL: Methodology, Writing – review & editing. KH: Data curation, Methodology, Supervision, Writing – review & editing. VP: Resources, Writing – review & editing. MQ: Conceptualization, Funding acquisition, Writing – review & editing. CD: Conceptualization, Funding acquisition, Supervision, Writing – review & editing.
Funding
The author(s) declare financial support was received for the research, authorship, and/or publication of this article. This research was funded by the National Center of Wildlife (NCW). The project was also supported by King Abdullah University of Science and Technology (KAUST) baseline fundings to CD. All data collected onboard, including images collected by ROVs and submersibles are property of NCW. The videos, images obtained during the course of the RSDE are credited to the National Center of Wildlife, Kingdom of Saudi Arabia.
Acknowledgments
We thank the National Center of Wildlife (NCW) for leading the Red Sea Decade Expedition. We thank the ROV (Remote Operated Vehicle) pilots for their efforts, the crew, scientists, and cruise leaders involved in the RSDE (Red Sea Decade Expedition 2022) cruise on board of the OceanXplorer research vessel.
Conflict of interest
The authors declare that the research was conducted in the absence of any commercial or financial relationships that could be construed as a potential conflict of interest.
The author(s) declared that they were an editorial board member of Frontiers, at the time of submission. This had no impact on the peer review process and the final decision.
Publisher’s note
All claims expressed in this article are solely those of the authors and do not necessarily represent those of their affiliated organizations, or those of the publisher, the editors and the reviewers. Any product that may be evaluated in this article, or claim that may be made by its manufacturer, is not guaranteed or endorsed by the publisher.
Supplementary material
The Supplementary Material for this article can be found online at: https://www.frontiersin.org/articles/10.3389/fmars.2024.1338782/full#supplementary-material
Supplementary Material S1 | Specimen CHR0199_bio7, in situ record at 494 meters depth in the central Red Sea.
Supplementary Material S2 | Specimen CHR0273_bio2, in situ record at 438 meters depth in the north-central Red Sea.
Supplementary Material S3 | Specimen CHR0199_bio7, on board record.
Supplementary Material S4 | Specimen CHR0199_bio7, on board record.
Supplementary Material S5 | Specimen CHR0199_bio7, on board record.
Supplementary Material S6 | Specimen CHR0223, in situ record at 657 meters in the south-central Red Sea.
References
Araujo M. (2012). Sistemática y distribución de los Sifonóforos (Cnidaria/Hydrozoa) del océano Atlántico Sudoccidental (Argentina: Universidad Nacional de Mar del Plata).
Bigelow H. B. (1911). The Siphonophorae. Reports of the scientific research expedition to the tropical Pacific, Albatross XXIII. Mem. Mus. Com. Zool. Harv. 38, 173–401.
Bigelow H. B. (1913). Medusae and siphonophorae collected by the US fisheries streamer albatros in the N.W. Pacific 1906. Proc. US Natl. Museum 44, 1–119. doi: 10.5479/si.00963801.44-1946.1
Carré D. (1969). Etude du développement larvaire de Sphaeronectes gracilis (Claus 1873) et de Sphaeronectes irregularis (Claus 1873), Siphonophores Calycophores. Cah. Biol. Mar. 31-34 10, 325–341.
Carré C., Carré D. (1991). A complete life cycle of the calycophoran siphonophore Muggiaea kochi (Will) in the laboratory, under different temperature conditions: ecological implications. Philos. Trans. R. Soc. London. Ser. B: Biol. Sci. 334 (1269), 27–32. doi: 10.1098/rstb.1991.0095
Cunningham C. W., Buss L. W. (1993). Molecular evidence for multiple episodes of paedomorphosis in the family Hydractiniidae. Biochem. Syst. Ecol. 21, 57–69. doi: 10.1016/0305-1978(93)90009-G
Diaz R. J., Rosenberg R. (1995). Marine benthic hypoxia: a review of its ecological effects and the behavioural responses of benthic macrofauna. Oceanogr. Mar. Biol. Annu. Rev. 33, 245.
Fewkes J. W. (1886). Report on the Medusae collected by the U.S. F.C. Steamer Albatross, in the region of the Gulf Stream, in 1883-’84. Rep. U.S. Commnr. Fish. For 1884, 927–980.
Haeckel E. (1888). Report on the Siphonophorae collected by H.M.S. Challenger during the years 1873-1876. Rep. Sci. Res. H.M.S. Challenger (Zool.) 28, 1–380.
Hissmann K. (2005). In situ observations on benthic siphonophores (Physonectae: Rhodaliidae) and descriptions of three new species from Indonesia and South Africa. Syst. Biodivers. 2, 223–249. doi: 10.1017/S1477200004001513
Hissmann K., Schauer J., Pugh P. (1995). Archangelopsis jagoa, a new species of benthic siphonophore (Physonectae, Rhodaliidae) collected by submersible in the Red Sea. Oceanologica Acta 18 (6), 671–680. Available at: https://archimer.ifremer.fr/doc/00098/20877/.
Huelsenbeck J. P., Ronquist F. (2001). MRBAYES: Bayesian inference of phylogenetic trees. Bioinform 17, 754–755. doi: 10.1093/bioinformatics/17.8.754
Just J., Kristensen R. M., Olesen J. (2014). Dendrogramma, new genus, with two new non-bilaterian species from the marine bathyal of southeastern Australia (Animalia, Metazoa incertae sedis)–with similarities to some medusoids from the Precambrian Ediacara. PloS One 9 (9), e102976. doi: 10.1371/journal.pone.0102976
Lens D. A., van Riemsdjik T. A. A. (1908). The Siphonophora of the Siboga Expedition. (Vol. 6). Late EJ Brill.
Mackie G. O., Boag D. A. (1963). Fishing, feeding and digestion in siphonophores. Pubbl. Statz. Zool. Napoli 33, 178–196.
Manko M. K., Weydmann A., Mapstone G. M. (2017). A shallow-living Benthic Rhodaliid siphonophore: Citizen science discovery from Papua New Guinea. Zootaxa 4324, 189–194. doi: 10.11646/zootaxa.4324.1.11
Mapstone G. M. (2014). Global diversity and review of siphonophorae (Cnidaria: hydrozoa). PloS One 9, e87737. doi: 10.1371/JOURNAL.PONE.0087737
Mapstone G. M., Corbari L., Menot L. (2016). Two deep-living rhodaliids (Cnidaria, Siphonophora) from the Mid-Atlantic Ridge. Mar. Biol. Res. 13, 486–493. doi: 10.1080/17451000.2016.1232830
Moser F. (1924). “Ordnung: siphonophora,” in Handbuch der Zoologie, vol. 1 . Eds. Kuekenthal W., Krumbach T. (Berlin and Leipzig: Walter de Grypter & Co), 485–521.
Pugh P. R. (1983). Benthic Siphonophores: a review of the family Rhodaliidae (Siphonophora, Physonectae). Soc Lond. B 301, 165–300. doi: 10.1098/rstb.1983.0025
Rambaut A. (2010). FigTree v1. 3.1. Institute of Evolutionary Biology, University of Edinburgh, Edinburgh. doi: 10.1371/JOURNAL.PONE.0019543
Sanamyan K. E., Sanamyan N. P., Galkin S. V., Ivin V. V. (2018). A record of deep-water benthic siphonophore (Siphonophorae: Physonectae: Rhodaliidae) in vicinity of submarine Piyp Volcano (North-Western Pacific). Invertebr. Zool. 15, 323–332. doi: 10.15298/invertzool.15.4.01
Keywords: Physonectae, Rhodaliidae, deep-sea, exploration, ROVs
Citation: Angulo-Preckler C, Steckbauer A, Lim KK, Hissmann K, Pieribone V, Qurban M and Duarte CM (2024) In situ observation on two ‘elusive’ rhodaliid siphonophores (Cnidaria; Hydrozoa; Siphonophora) from the Red Sea, including a potential new species within the genus Archangelopsis. Front. Mar. Sci. 11:1338782. doi: 10.3389/fmars.2024.1338782
Received: 15 November 2023; Accepted: 17 January 2024;
Published: 01 February 2024.
Edited by:
Nathan Jack Robinson, Fundación Oceanográfica, SpainReviewed by:
Maciej Mańko, University of Gdansk, PolandGillian Mapstone, Natural History Museum, United Kingdom
Copyright © 2024 Angulo-Preckler, Steckbauer, Lim, Hissmann, Pieribone, Qurban and Duarte. This is an open-access article distributed under the terms of the Creative Commons Attribution License (CC BY). The use, distribution or reproduction in other forums is permitted, provided the original author(s) and the copyright owner(s) are credited and that the original publication in this journal is cited, in accordance with accepted academic practice. No use, distribution or reproduction is permitted which does not comply with these terms.
*Correspondence: Carlos Angulo-Preckler, carlos.preckler@kaust.edu.sa; carlospreckler@hotmail.com
†ORCID: Carlos Angulo-Preckler, orcid.org/0000-0001-9028-274X