- 1Laboratory of Marine Invertebrate Morphology and Ecology, A.N. Severtsov Institute of Ecology and Evolution, Russian Academy of Sciences (RAS), Moscow, Russia
- 2Coastal Branch of the Joint Vietnam-Russia Tropical Science and Technology Research Center, Nha Trang, Vietnam
Introduction: Coral reefs are one of the most productive yet vulnerable ecosystems on Earth. An important step in understanding the functioning of coral communities is studying the environmental impact on the state of reefs. The present study aimed to assess the impacts of the water temperature, precipitation, wave action, and population density on the seasonal bleaching, partial and complete mortality of shallow-water branching corals of Pocillopora verrucosa species complex.
Materials and methods: The present study was conducted at the Dam Bay research station (Nha Trang Bay) from 26 April 2020 to 2 April 2021. The environmental data was collected from the Dam Bay weather station and using a temperature logger planted on the nursery, coral bleaching and mortality were assessed visually using photos taken underwater.
Results: Throughout the study, the percentage of bleached coral colonies varied from 1 to 41.5% with two peaks, in autumn (October) and spring (April). Rates of the development of partial mortality varied from 0 to 10%, with a pronounced peak in December. At the end of the experiment, the percentage of coral colonies suffering from partial mortality reached 47%. Only 4,5% of experimental colonies died to the end of the study. Analysis revealed that water temperature and partial mortality are the drivers of bleaching, while wave action, temperature and bleaching are the factors with a significant impact on partial mortality.
Discussion: Thus, both bleaching and patrial mortality are interlinked and the effect of partial mortality on bleaching is stronger than the opposite. Both phenomena have pronounced seasonal variation, but their maximum manifestation does not coincide in time allowing corals to avoid their synergetic effect. Moreover, it was found that the impact of individual stress on mortality prevailed over the impact of seasonal bleaching and partial mortality events. The main cause of coral death was the development of algal fouling at the sites of colony lesions, which gradually spread over the entire surface of the colony.
1 Introduction
Coral reefs are one of the most productive yet vulnerable ecosystems on the planet (Naumov et al., 1985; Reaka-Kudla et al., 1997). Millions of people in tropical countries depend on them for essential ecological and economic services such as food, tourism income, and coastal protection from wave impact (Wilkinson, 2004; Ferrario et al., 2014). However, due to various stress factors in the Anthropocene, coral reefs continue to degrade worldwide (Bellwood et al., 2003; Pandolfi et al., 2003; Hoegh-Guldberg, 2011; Hoegh-Guldberg, 2014; Hughes et al., 2017). From 1957 to 2007, there was approximately a 50% reduction in live coral cover worldwide, and reef degradation continues to this day (Eddy et al., 2021; Rivas et al., 2023; Tkachenko, 2023; Tkachenko et al., 2023).
Coral bleaching is probably the most iconic manifestation of reef degradation. During bleaching events, intracellular symbionts, dinoflagellates of the family Symbiodiniaceae (zooxanthellae), are released from coral tissues into the external environment, reducing the energy supply to coral colonies which, in case of severe bleaching, can lead to their death (Brown, 1997; Douglas, 2003). Branching Acroporidae and Pocilloporidae corals are especially susceptible to bleaching, as their zooxanthellae density is lower than in massive coral species (Shu et al., 2008; Shu et al., 2011). Coral bleaching can be caused by a variety of global and local stressors. Among the global factors, ocean acidification and rising sea temperatures have the most prominent impact on reefs (Hoegh-Guldberg, 1999; Fine and Tchernov, 2007; Fabricius, 2011; Hoegh-Guldberg, 2014; Weiss and Martindale, 2017; Lough et al., 2018; Cornwall et al., 2021; regarding the species of interest see also Table 1). Local stress factors can be both natural, such as storms, sediment accumulation, and anthropogenic origin, including eutrophication, pesticide, heavy metals, industrial and pharmaceutical pollution, and over- and destructive fishing (Wilkinson, 2004; Banaszak and Lesser, 2009; Wenger et al., 2015).
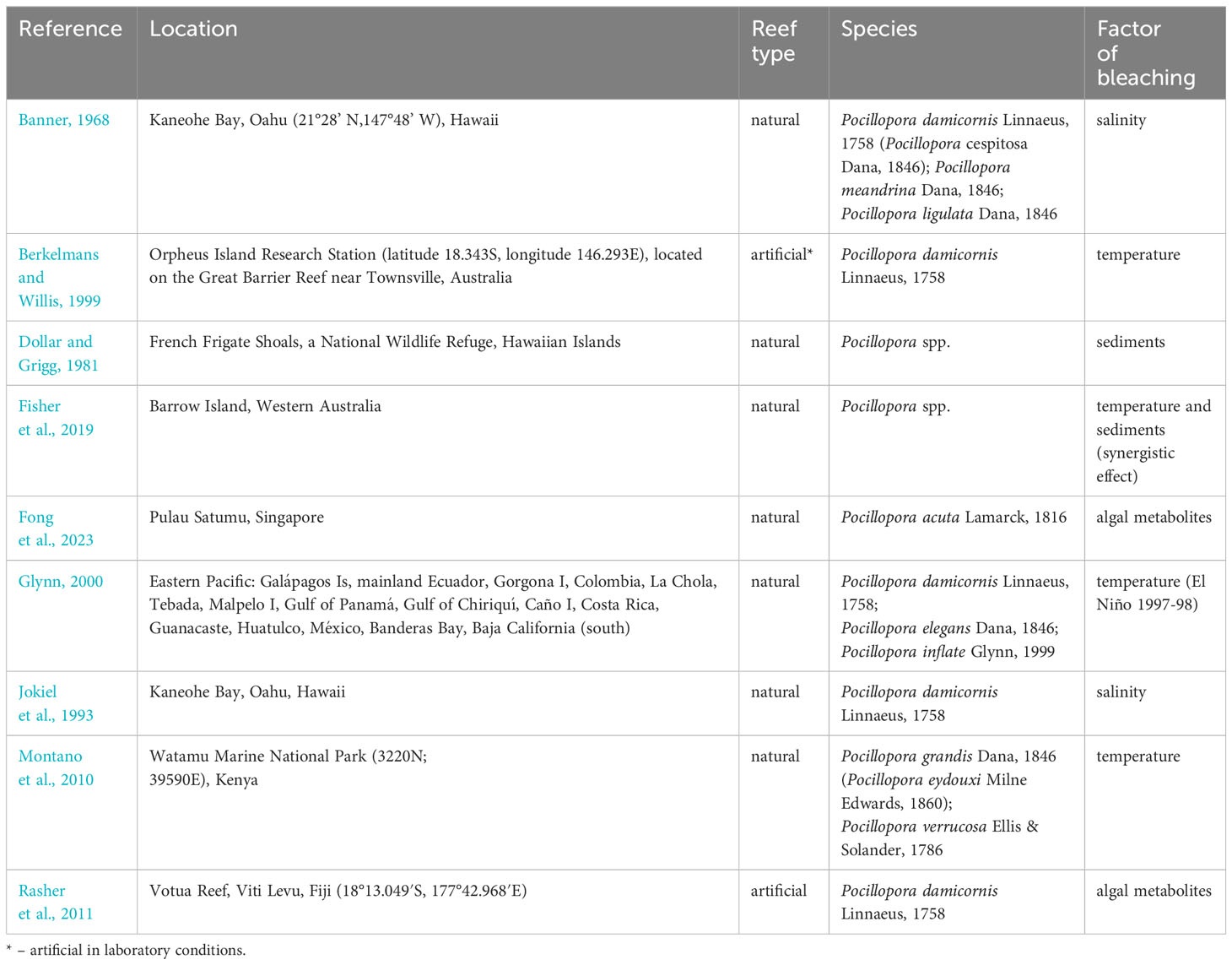
Table 1 Selection of studies on environmental parameters responsible for the bleaching in Pocilloporid corals.
For the most part, the literature on coral bleaching events contains studies of severe bleaching events leading to coral death (Eakin et al., 2010; Rodgers et al., 2017). In the past decade, research has focused on the simultaneous effects of multiple factors on severe coral bleaching, which can amplify each other or act as antagonists (Brown et al., 2013; Fisher et al., 2019).
Much less studied is seasonal bleaching, a normal phenomenon likely occurring across all reefs in the world (Fitt et al., 2001; Lesser, 2011), except mesophotic corals (Nir et al., 2014). The process of seasonal bleaching is largely determined by seasonal fluctuations in environmental parameters that underlie the cyclical nature of seasonal bleaching. It is associated with the response of zooxanthellae to seasonal fluctuations in environmental parameters such as sea surface temperature (SST) or light intensity (Brown et al., 1999; Fitt et al., 2000; Dellisanti et al., 2023). It is believed that seasonal bleaching does not lead to coral death, but prolonged exposure to a stress factor, such as sustained high temperatures during a season, can contribute to their mortality (Warner et al., 2002; Nir et al., 2014). Global climate change can interact with local stress factors determining the overall impact on coral ecosystems based on the type of these interactions (additive, synergistic, or antagonistic) (Coles and Jokiel, 1978; Crain et al., 2008; Brown et al., 2013; Fisher et al., 2019). The combination of local natural-climatic and anthropogenic factors creates a complex pattern of seasonal bleaching formation, the regularities of which are yet to be determined. Therefore, to understand the general patterns of seasonal bleaching dynamics and its consequences for coral communities as a whole, specific data are needed for different regions of the tropical zone of the World Ocean.
Another significant threat to corals is the formation of dead areas on the coral surface, which can spread to the entire surface of the coral colony and lead to its mortality (Smith et al., 2006; Rodríguez-Villalobos et al., 2015; Muller et al., 2020). Its appearance can be caused by physical impacts (feeding activity of corallivores or destructive wave action), bacterial outbreaks, or hypoxia due to intense sedimentation (Table 2, also see Brown, 1997; Anthony and Larcombe, 2000; McCook, 2001; Nugues and Roberts, 2003). Macroalgae quickly colonize the dead surfaces and, by releasing metabolites, can contribute to the expansion of dead areas or even be the cause of their formation (Smith et al., 2006; Wangpraseurt et al., 2012). Therefore, studying the formation of such areas, a process called partial mortality (PM) under conditions of an ecosystem shift towards the dominance of algae is of particular interest. This shift occurs in the context of increasing eutrophication, sedimentation, and overfishing of herbivorous fish (Birrell et al., 2005; Diaz-Pulido et al., 2010; Wenger et al., 2015; Silbiger et al., 2018). This situation is characteristic of coastal areas in Southeast Asia, including the Nha Trang Bay (Tkachenko et al., 2016), where the present research was conducted.
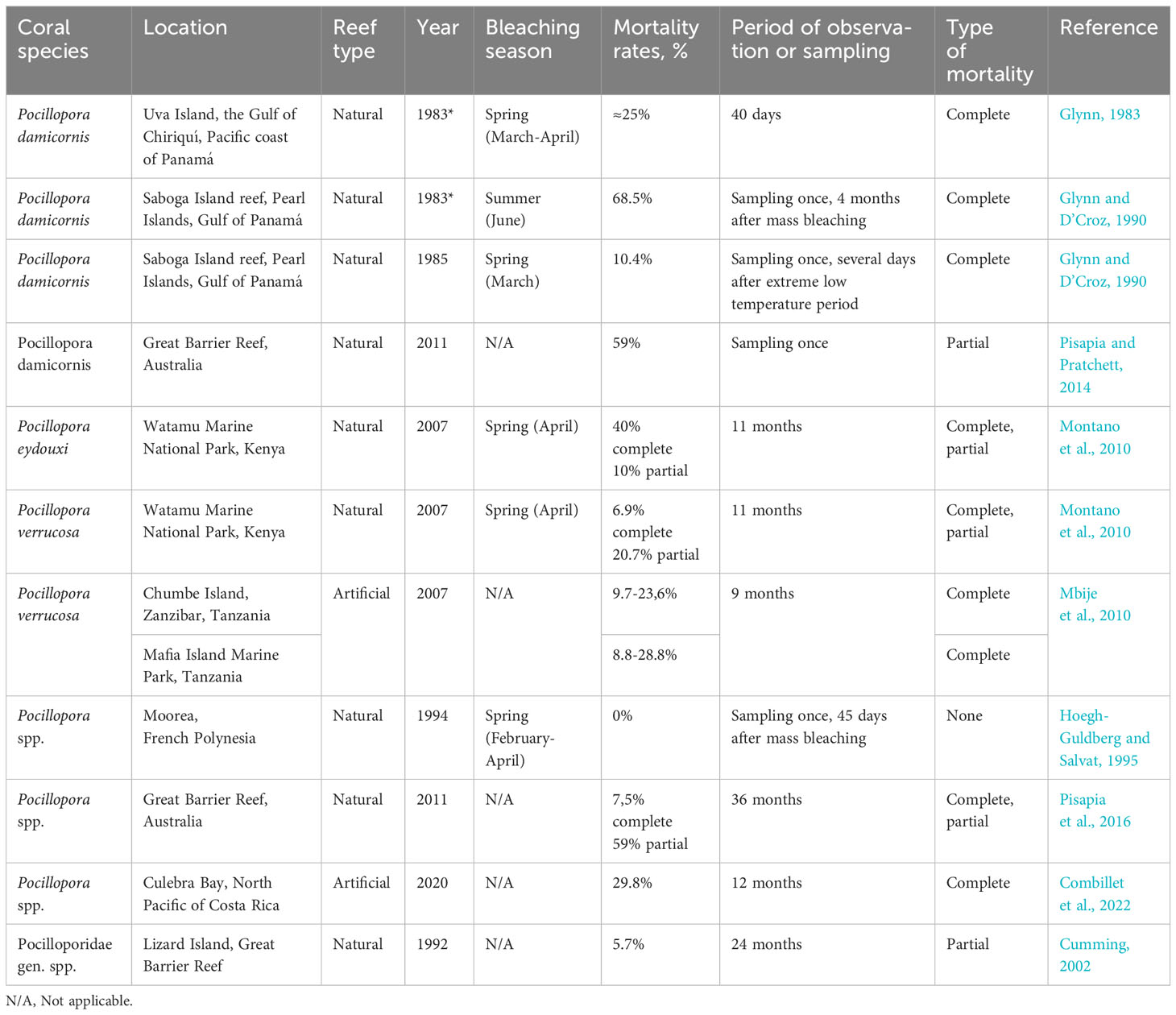
Table 2 Data on partial and complete mortality in branching corals referred to or relevant to the present research.
This study aimed to describe the seasonal dynamic of bleaching and PM of the shallow-water branching coral Pocillopora verrucosa, and to analyze the influence of temperature, precipitation, wind speed and coral population density on these processes. According to our hypothesis, both bleaching and partial mortality processes are mutually interlinked and driven by the impact of examined environmental factors. The study was conducted on transplanted fragments of corals grown in nurseries, which made it possible to reliably assess the influence of these factors on the coral population based on a large sample size of coral heads, as well as to trace their influence on the fate of individual colonies.
2 Materials and methods
2.1 Research location and studied coral
The present study was conducted at the Dam Bay research station of the Coastal Branch of the Joint Russian-Vietnamese Tropical Research and Technological Center (Tropical Center), from 26 April 2020 to 2 April 2021 (Figure 1, also see Figure 1 in Britayev et al., 2023). The scientific station is located on the shore of the Dam Bay on Tre Island, Central Vietnam. The bay is relatively shallow even in comparison with adjacent Nha Trang Bay, the depth of Dam Bay never exceeds 15 m. The area is protected to a significant extent from the wave impact by Mun Island and the eastern part of Tre Island. On another hand, any potential impact of precipitation is amplified in the area since two creeks flow into the bay in its north-western part. During the dry season creek inflow varies on a minimal level, having no impact on the ecosystem in the area, but in the rainy season it may increase greatly, leading to a significant increase in sedimentation. The bottom of the bay is predominantly muddy, scattered with sandy and rocky areas. One such sandy area was selected for the placement of coral nurseries. Depth at the nursery varies by about 3 m, which reduces the potential impact of desalination on experimental coral colonies.
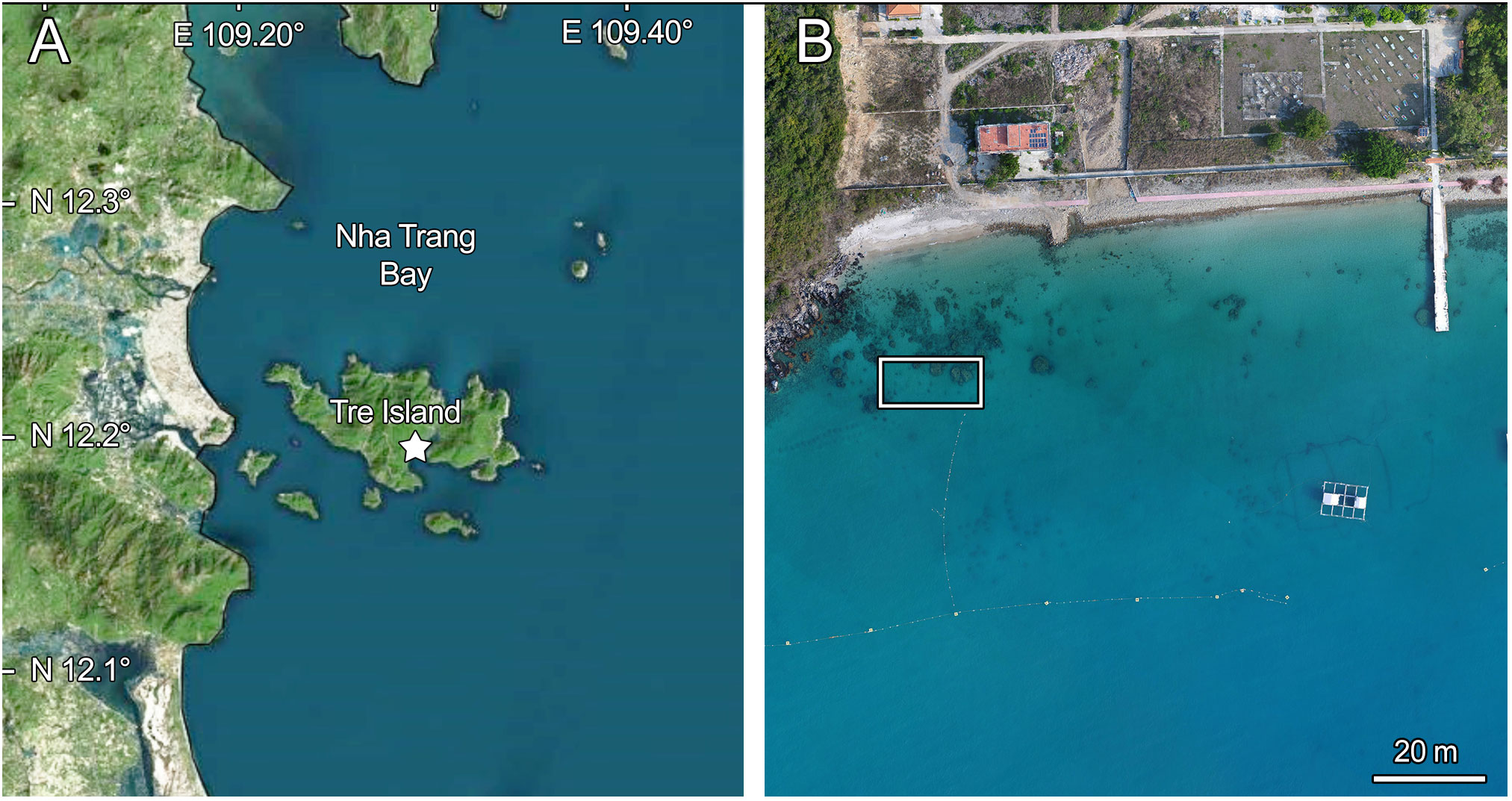
Figure 1 Nha Trang Bay (A), the star marks the location of the Dam Bay research station. Area of research (B), rectangle indicates the location of coral nurseries.
Branching scleractinian corals of the genus Pocillopora are among the most common coral species in the Indo-Pacific (Loya et al., 2001; Qin et al., 2019). They are widely distributed in the region and commonly used in aquaculture (Combillet et al., 2022), including the waters of Central Vietnam (Latypov, 2016) where the present study was carried out. In the area of our research, the most common and morphologically variable species of the genus was previously identified by Dautova et al. (2007) as P. verrucosa. However, it has recently become clear that P. verrucosa is a group of cryptic species that cannot be identified by morphological characteristics alone (Johnston et al., 2017; Oury et al., 2023). Since molecular studies of the taxonomic affiliation of the species we used were not the scope of our work, in the text we will use the name of the species complex P. verrucosa.
2.2 Environmental parameters
The environmental factors studied can be divided into two groups: those influencing the fitness and mortality of corals directly (1) and indirectly (2). The first group includes only one factor, the water temperature in the study area. The ambient temperature was measured to the nearest 0.01°C twice a day (at 11:00 AM and 11:00 PM) during the whole period of the study via HOBO U22-001 temperature logger which was deployed at a depth of 3 m on the coral nursery. For the further analysis the obtained data was averaged on a daily basis.
Studied environmental factors with indirect impact on corals include the precipitation, the wind speed and the density of coral populations. None of these represent factors affecting coral condition on their own, but all appear to be the proxy of other environmental parameters which may affect physiological condition and mortality of corals.
Specifically, seasonal increase of precipitation is the main cause of increase of sedimentation, and among the key factors determining the water transparency and salinity in the area. Thereby, in the framework of this study, precipitation applied as a proxy of all these environmental factors.
Similarly, the wind is the main factor which determines the wave surge power and wave action in general. Consequently, even though variation of the wind speed does not affect corals directly, it affects them via modification of the wave impact. It should be noted that, since the location of Dam Bay research station is protected from the winds blowing from the directions other than the east and south-east (80-190°, “eastern wind” below) by the Hon Tre and Hon Mun islands, speed of the winds blowing only from these directions was considered.
Both environmental parameters, precipitation in mm and the eastern wind speed in m/s were collected once a day, using Campbell CR3000 datalogger mounted at Dam Bay research station. It should be noted that since only the daily average wind speed was registered, we are unable to indicate the peak wind speed, or the speed of gusts.
Finally, the density of coral population determines water dynamics at the nursery, which in turn may amplify or mitigate the impact of other environmental factors. In particular, it was demonstrated that high-density coral colonies are more susceptible to bleaching due to the disruption of water circulation around the colonies (Nakamura and van Woesik, 2001; Nakamura et al., 2003). Authors found that moderate to high water flow prevents colonies from bleaching under high temperature and light conditions and promotes their rapid recovery after bleaching. On another hand, the impact of coral population density on the development of PM is unpredictable. Disruption of water circulation may lead to the death of coral colonies (Hennige et al., 2021), bacterial and fungal infections spread more easily in dense populations. But at the same time, in dense populations, individual colonies are better protected from predators and less exposed to mechanical impact.
To estimate the influence of density, coral fragments were transplanted to five rectangular iron frames forming the settlements with different densities, namely, the number of colonies on each frame varied from 20 to 60 with a step of 10 colonies (Figure 2). In total 200 colonies were transplanted to the nursery.
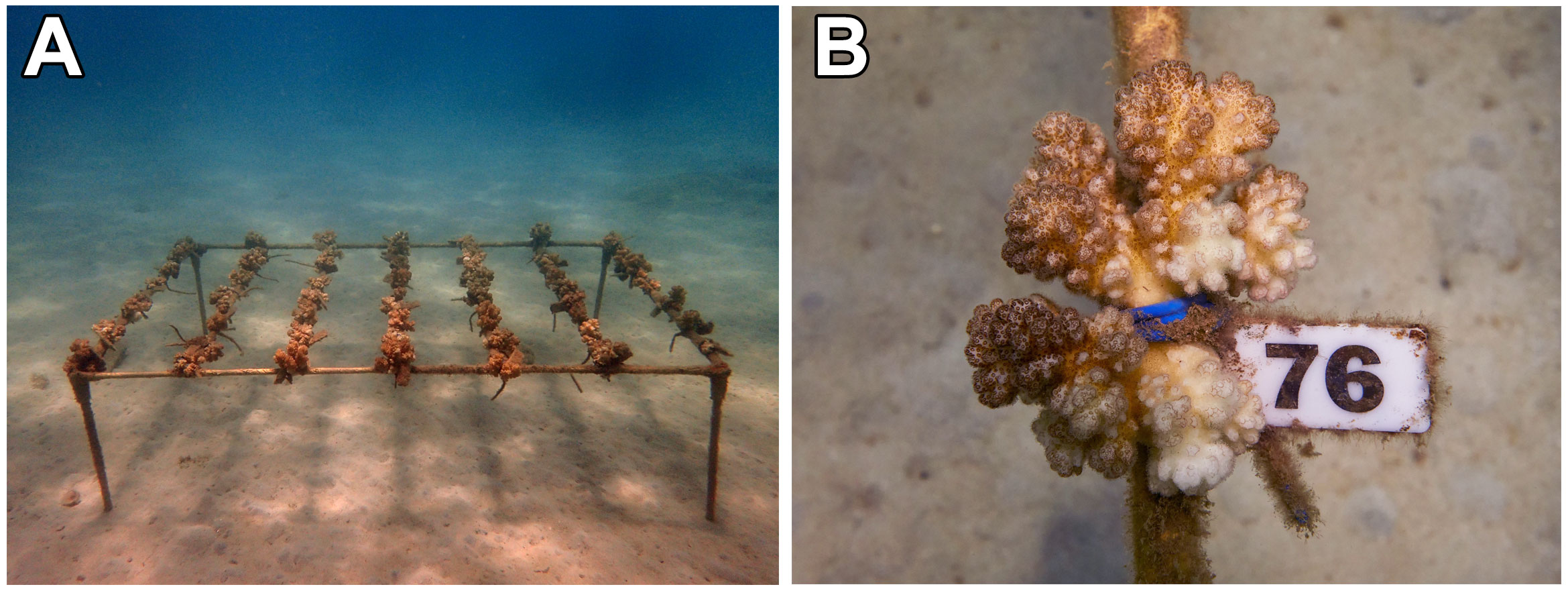
Figure 2 Rectangular iron nursery used in the present experiment (A) and individual coral head planted on the nursery (B).
2.3 Assessment of bleaching and mortality
The first two months after the planting of fragments (MayJ-June) colonies were adapting to the new location and during this period the bleaching was not assessed. Thus, even though some of colonies were bleached during this period, we treated it as consequence of adaptation to nurseries.
Exercise on bleaching and mortality assessment had begun on 03.07.2020 and lasted up to 02.04.2021. Colonies were photographed using Panasonic Lumix G80 digital camera in underwater housing twice a month since June to October, in November corals’ condition was recorded only once (on 21.11.2020) due to poor weather conditions. Additionally, since January to April 2021 condition of corals also was recorded monthly due to technical limitations.
Acquired photos were used for assessment of coral health using Coral Health Monitoring Chart (Siebeck et al., 2006). Often corals experienced uneven bleaching, in this case the central parts of the colonies were more pigmented than the branches, or individual branches were more bleached than others. Therefore, to avoid assessment bias, the bleaching score was assessed as a mean between the most and the least bleached parts of the colony (Siebeck et al., 2006). The coloration of the tips of the branches was not taken into account, because newly developed tips normally have paler coloration than the internal zone. Colonies with a score of less than 3 were considered bleached, following the CoralWatch monitoring program instructions (2021_CW-DIY-instructions-4-pages-www.pdf (coralwatch.org). Assessment of colony bleaching was performed by a single beholder twice to avoid bias caused by differences in color perception between different individuals or different screens.
Partial mortality (PM) was assessed visually as well. In all but one (the survey on 21 November 2020) cases it wasn’t possible to find the exact moment of establishment the PM, so the algal fouling was used as a proxy of PM. We recorded only the presence of PM, since assessment of coral condition was performed using non-life image but photos, it was not possible to accurately estimate the affected area of the colony. The colony was considered dead when its surface was completely covered by lesions or algal fouling.
In order to find out the causes of mortality the backtracking was performed. Individual histories of 9 colonies which died to the end of the study were studied, the time and the duration of suffering from PM and bleaching were considered.
2.4 Statistical treatment
To improve the readability of the graphs illustrating a variation of the environmental factors, a moving average with a smoothing interval of 15 days was applied. Such an interval was selected to avoid mixing survey periods since the average time between the surveys was two weeks. Except for this case, the analysis of the impacts of environmental factors on corals was performed using raw data.
The Kolmogorov–Smirnov test was used to test the distributions of dependent variables’ values (the percentage of bleached colonies and the percentage of colonies with PM). The test showed that both variables are distributed normally, so general linear models were applied to assess the impacts of the environmental factors, including water temperature, precipitation, eastern wind speed, coral population density on the percentage of bleached colonies and colonies with developed PM. Additionally, the model developed to estimate impact of environmental factors on bleaching included accumulated share of colonies with PM recorded in each previous survey. Similarly, the model for assessment of environmental impact on PM included the percentage of bleached colonies recorded in a previous survey. Since no statistically significant effect of coral population density was confirmed by the models, additional tests for the same predictors, but only in the months with the greatest levels of bleaching and PM was performed. The impact of each factor was considered significant if p was below 0.05 (p < 0.05), the power of effect was measured following partial eta squared (ηp2) value: if ηp2 was less than 0.06, the effect size was considered small, if it was from 0.06 to 0.14, the effect size was considered moderate and if ηp2 was more than 0.12, the effect size was considered large.
To describe the effect of each individual environmental factor on bleaching and development of PM, linear trends were plotted based on the mean values for each survey and planting density.
All tests were performed in Statistica v. 12.0.
3 Results
3.1 Variation of the environmental factors
Most of the studied environmental factors experienced significant seasonal variation. In particular, the water temperature in the area of research varied from 22.9 to 31.3˚C during the studied period, precipitation level varied from 0 to 165 mm/day. Daily average speed of the eastern wind was the least variable environmental parameter; it ranged from 0 to 3.27 m/s. The detailed overview of monthly variation of these parameters is presented in the Supplementary Table S1. The trends of water temperature, precipitation and eastern wind speed variation are presented in the Figure 3 (Figures 3A–G) respectively.
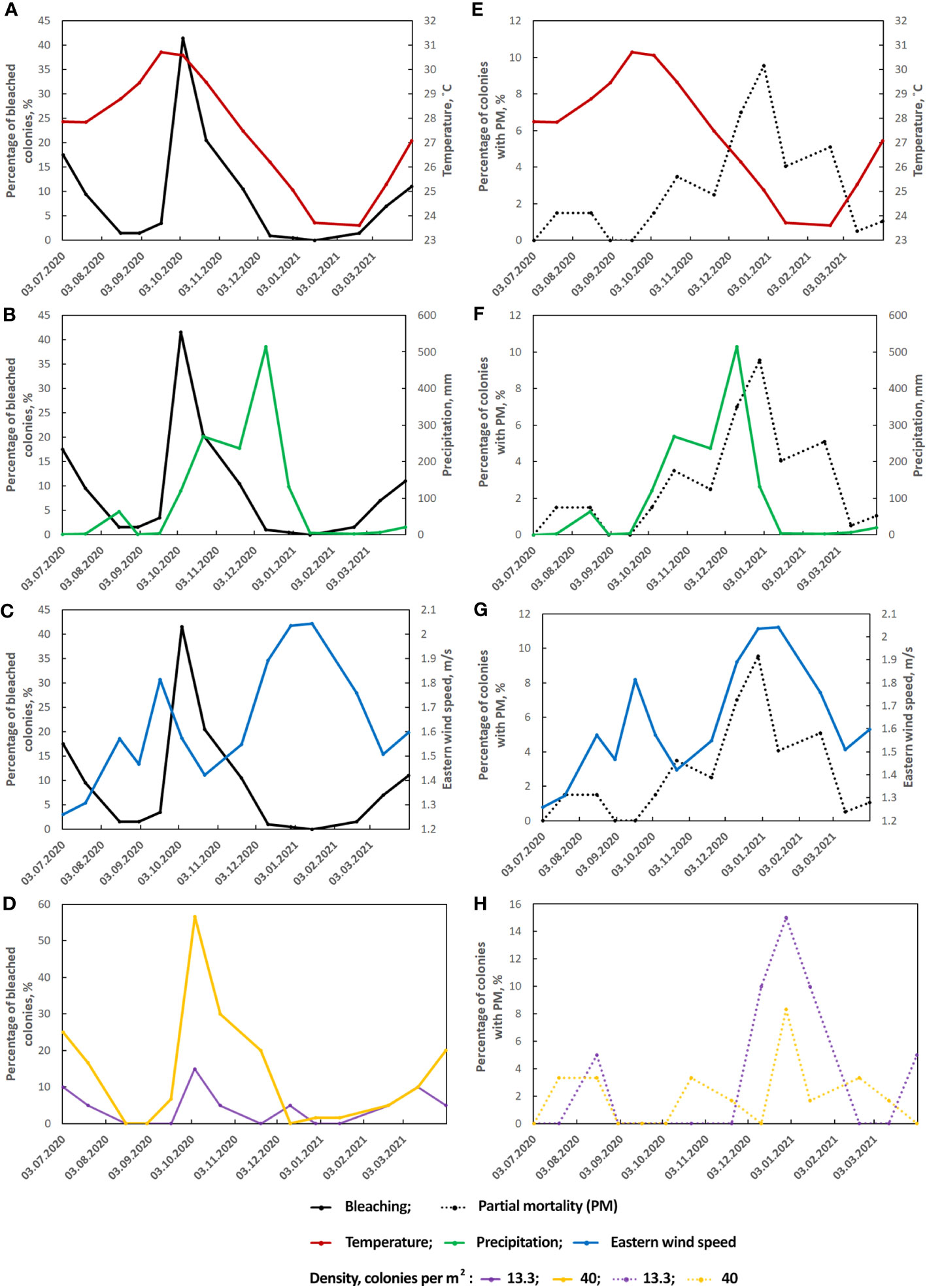
Figure 3 Variation of the water temperature (A, E), precipitation (B, F), eastern wind speed (C, G) coupled with variation of bleaching (A–D) and partial mortality (E–H). Graphs (D, H) illustrate the differences in variation of bleaching and PM on the nurseries with different coral population density (13.3 and 40 coral heads per m2).
3.2 Bleaching variability
During the observation period the intensity of colonies coloration varied from pale to dark (Figure 4). When the observation part of the experiment has begun after two months of colony restoration after transplantation, 17.5% of the colonies were bleached. Either way, summer surveys were characterized by the steady trend on improving of corals condition: in the end of July the percentage of bleached colonies reduced to 9.5%, then, in August to 1.5% (Figure 5). Short after the temperature rise in the middle of September, the percentage of bleached colonies began increasing again, reaching 41.5% in the beginning of October, which was the greatest share of bleaching colonies over the whole study period. Since the second half of October corals began recovering, reducing the percentage of bleached colonies to 20.5% to the end of the month, to 10.5% in the end of November, and to lowest observed values (1%) in December. January and February were characterized by a rather stable condition of corals with a very weak trend in increasing of the bleached colonies share. From March bleaching intensified, in the beginning of the month the percentage of bleached colonies increased to 9%. Finally, in the end of the experiment this trend continued, leading to increase of the share of bleached colonies to 15.5%.
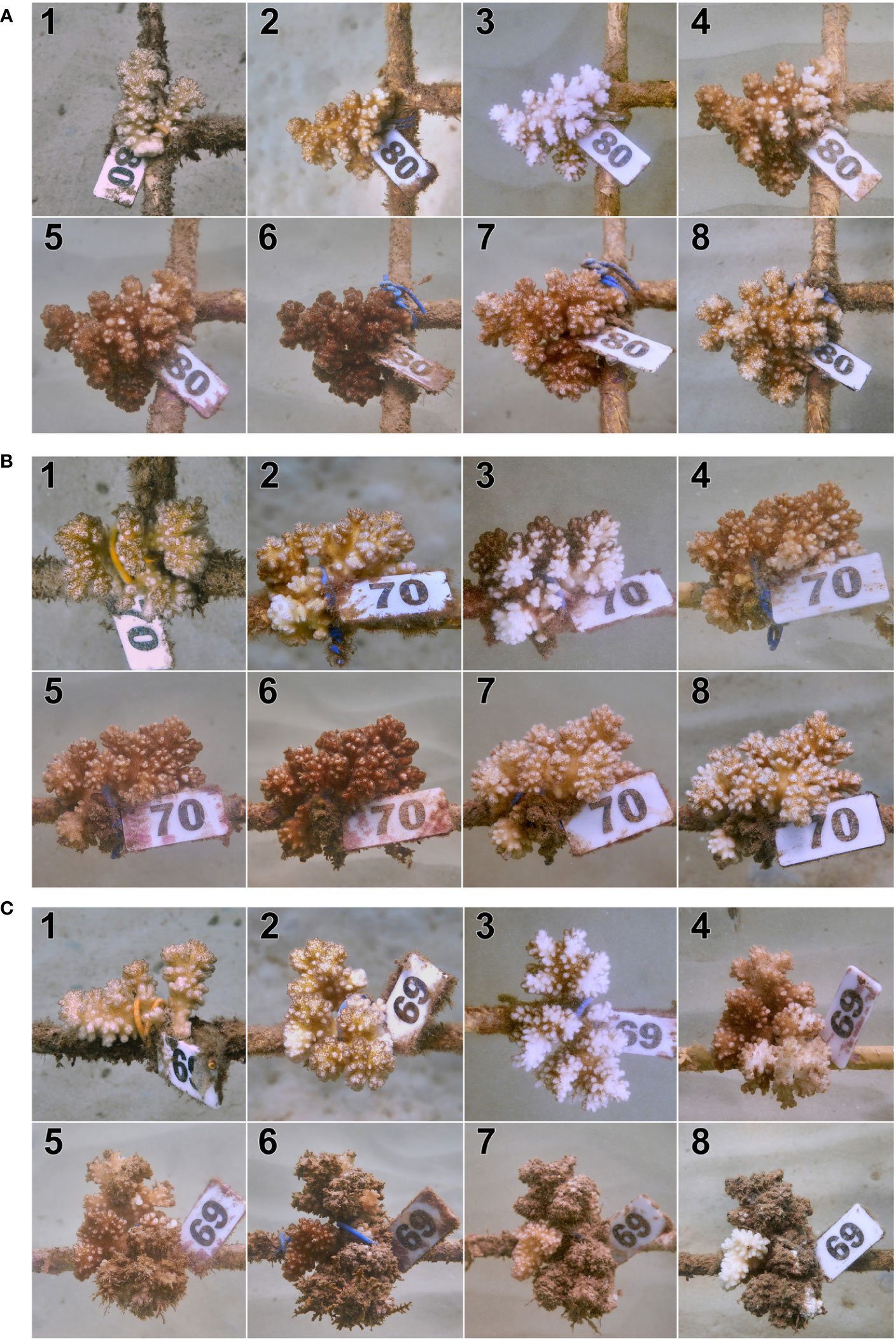
Figure 4 Visual representation of recovery (A), partial mortality (B) and mortality (C) processes in Pocillopora verrucosa species complex corals in the framework of the present research.
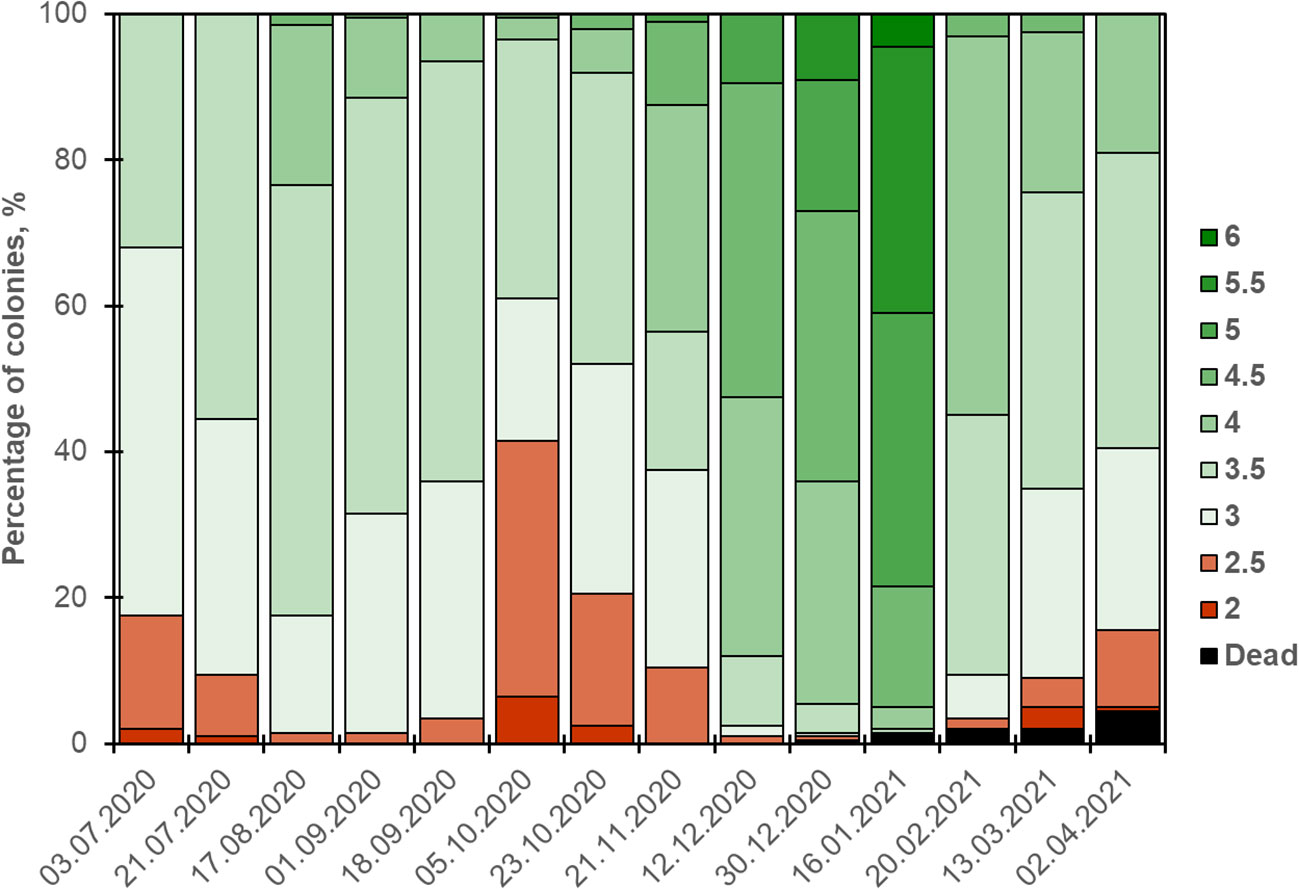
Figure 5 Variation of the proportion of coral colonies in the specific health condition over the course of the present study.
Thus, during the period of the study, two periods of the seasonal bleaching were observed, namely, the autumn bleaching event with the peak in the beginning of October, and the spring bleaching event characterized by the peak in the end of March - beginning of April (Figure 6A). Potentially, the third peak was observed in the very beginning of the experiment (May-June), but, since it’s not possible to clearly distinguish its causes, it won’t be considered in the further analysis.
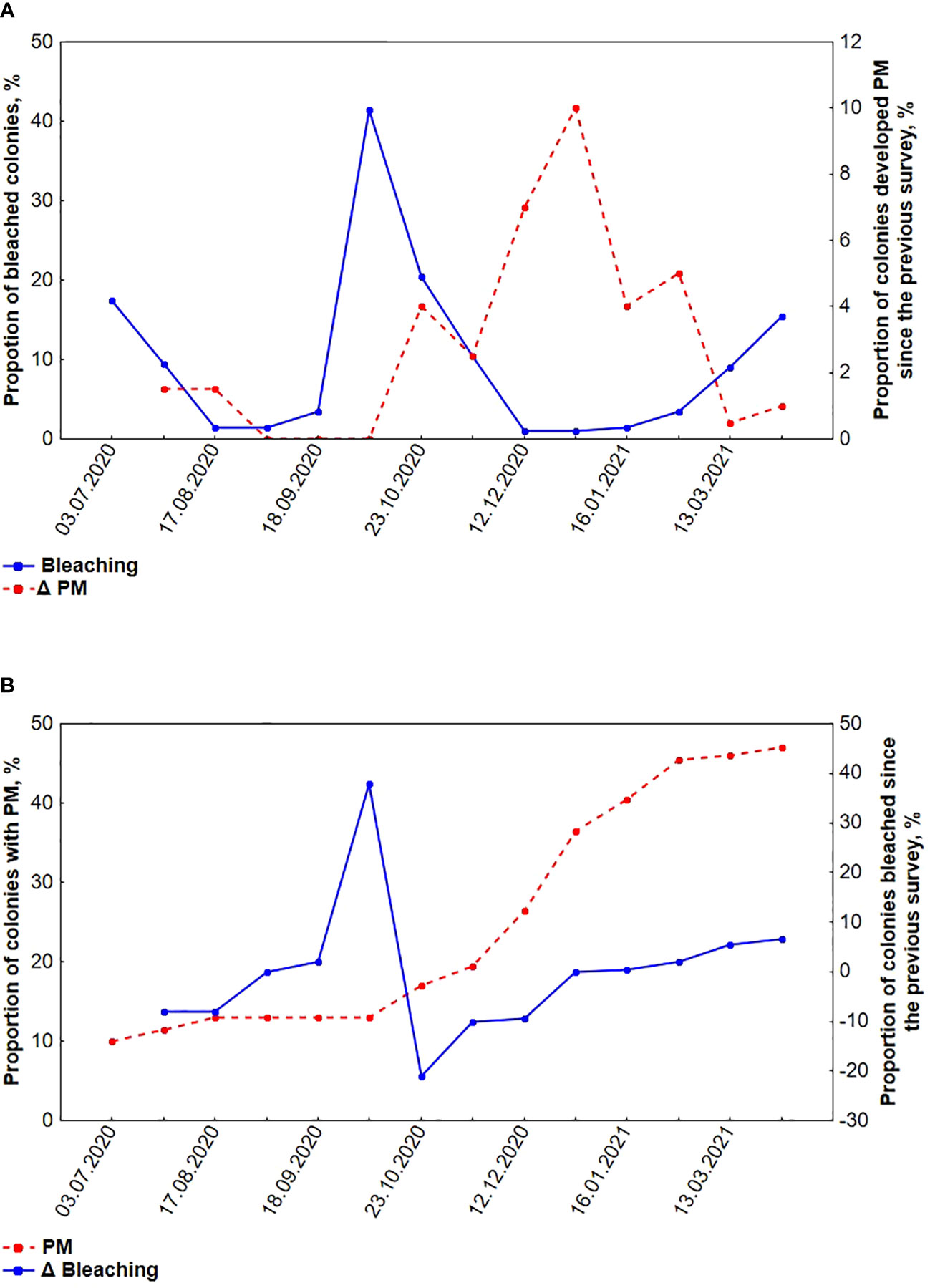
Figure 6 Variation of the proportion of bleached coral colonies and colonies with PM over the course of the present study. Proportion of bleached colonies vs the proportion of colonies that suffered from PM since the previous survey (A), proportion of colonies with PM vs the proportion of colonies bleached since the previous survey (B).
3.3 Partial mortality
Since the very beginning of the study algal fouling was observed on the experimental platforms. First, it appeared on the metal bars on which coral fragments were mounted, in the areas not contacting with life coral branches. After that, fouling spread to wires used for mounting coral fragments. Finally, as a part of partial mortality process it appeared on the fragments themselves. In a single case (on November 21) the colonies with newly died surfaces were observed, which allowed direct identification of colonies suffering from PM.
In July only a little portion of coral colonies suffered from PM, 10% of them (Figures 3E–H) were fouled by algae to some extent. During the following months the number of fouled colonies gradually increased, but even to the end of September it did not exceed 13% of the sample. From the middle of October PM began spreading again, at the end of the month the percentage of colonies with PM reached 18%. In November, the spread of colonies with PM continued at a slightly reduced rate (reaching 20% at the end of the month). December was characterized with the greatest recorded PM rates. Just 20 days after the survey in November the percentage of colonies suffering from it increased to 26.5%, and 18 days later their number increased to 36.5%. After such a pronounced mortality event, In January, the percentage of colonies with PM increased only to 40.5%, and in February this value increased by another 5%. In spring mortality rates kept decreasing, reaching just 0.5% in March and 1% in April.
To the end of the period of research more than 47% of living coral colonies suffered PM (Figure 6B). In all the cases, the dead surface was at least partially fouled by algae.
3.4 Mortality
In total, during the whole experiment only 9 coral colonies (4.5%) have died, which is the extremely low number in comparison with natural mortality in the area (Figure 5).
The first dead colony was observed in December, right at the moment, when the vast majority of colonies were recovering after autumn bleaching and suffering of PM. In January two more colonies have died, increasing the total percentage of dead colonies to 1.5%. One more colony has died in February. The number of dead colonies haven’t change up to the middle of March, but in the end of the month the peak level of mortality was recorded, 5 colonies have died in less than 20 days (Figures 4C, 5).
3.5 Impact of environmental factors on bleaching and partial mortality
A general linear model was performed on the percentage of bleached colonies. A significant effect of the temperature (Figures 3A, E, 7A, E), the accumulated PM and intercept was observed (Supplementary Table S2). Power of the specific effect (effect size) ranged from moderate (intercept and the accumulated PM) to large (temperature). The most pronounced effect of the temperature was observed with a two-week delay. Modelling also revealed moderate effects of precipitation, eastern wind speed and colonies density, but none of them was statistically significant (Figures 3B–H, 7B–H). Worth mentioning that impact of colonies density appeared significant (F (1) = 7.35; p = 0.02; ηp2 = 0.42), but only during the periods of mass bleaching and only between nurseries with the lowest and greatest density of coral colonies (Figures 3D, H, 7D, H). It should be noted that overall fit of the model was relatively low yet significant (R2 = 0.36, p = 0.001).
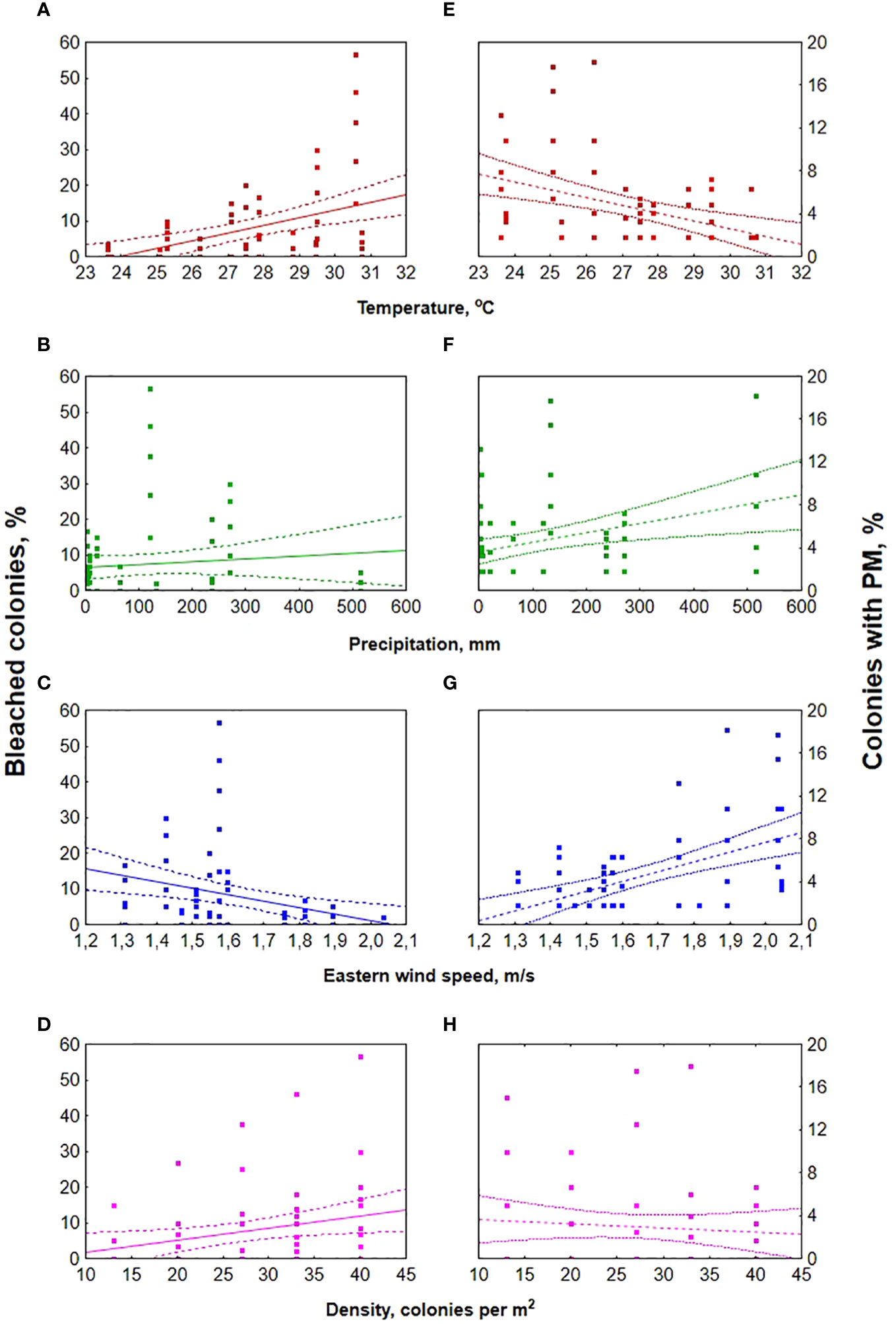
Figure 7 Trends in environmental factors’ impacts on coral bleaching and partial mortality. Impact of the temperature on bleaching (A) and on PM (E), impact of precipitation on bleaching (B) and on PM (F), impact of the eastern wind speed on bleaching (C) and on PM (G), impact of the coral population density on bleaching (D) and on PM (H).
The general linear model performed on the percentage of the colonies with PM fit the data better, even though the percentage of variance explained was still less than 50% (adjusted R2 = 0.47, p < 0.001). According to the model, several environmental variables significantly affected PM, including eastern wind speed, bleaching, experienced in the previous survey and water temperature (Figures 6B, 7E–H; Supplementary Table S3). The most powerful was the effect of the wind speed (large effect size), followed by the bleaching and ambient temperature, both having moderate effect. Unlike the case of bleaching, the temperature was negatively correlated with the percentage of colonies with PM, while the eastern wind speed was correlated positively. Precipitation and colony density had weak and insignificant effect in the model (Figures 3E–H). A separate test revealed that colonies density may have impact (F (1) = 4.70; p = 0.02; ηp2 = 0.63) on PM during the periods of its mass development (typhoon and post typhoon surveys), but this impact is opposite to the impact of colonies density on bleaching, i.e. nurseries with higher colonies density were less subjected to development of PM than ones with lower colonies density (Figure 3H).
3.6 Impact of bleaching and partial mortality on mortality
Backtracking of dead colonies’ condition revealed that bleaching alone couldn’t lead the whole colony to death. Three colonies (33%) were not bleached before death, while the remaining two-thirds of the sample were bleached for two weeks to two months before death. Among the colonies that suffered from bleaching before dying, the bleaching did not coincide with the peak of bleaching (October) but occurred later, in the period when the most of colonies were recovering (December and February). In all these cases bleaching was preceded by the development of PM.
At the same time, all the dead colonies suffered from PM before dying, but the duration of this condition varied greatly. In the shortest case recorded it was less than two months, the first lesions were observed at the beginning of December while in the middle of January, the colony was already dead. In other, less radical cases, colonies suffered from PM from 2.5 to more than 9 months before death. Only 33% of colonies got PM at the main PM event at the end of November, while the majority were suffering from PM even before that.
4 Discussion
4.1 Impact of environmental factors on bleaching
Among the factors we analyzed (temperature, wind speed, precipitation, colony density and PM) only the temperature and PM significantly affected the development of bleaching. It is known that the dynamics of bleaching usually corresponds to the dynamics of SST (sea surface temperature) (Glynn, 2000; Eakin et al., 2009; Table 1; Supplementary Table S4). However, in the study area, the high DHW index (reaching 10°C per week, indicating a Level 2 bleaching risk) calculated based on sea surface temperature during the July-August of 2020 (Supplementary Figure S1) did not affect the condition of the experimental colonies. This situation is related to the development of coastal upwelling (Tkachenko et al., 2023), which mitigated the impact of anomalously high temperature in the subsurface layer where the experimental colonies were located. High and prolonged water heating (30-31.7˚C) at the experimental site was observed only in September, which corresponds to the decline of upwelling by the beginning of that month (Figure 3A). The observed dynamics of bleaching overall corresponded to the temperature dynamics directly around the experimental colonies (Figure 7A).
It should be noted that the peak of temperature occurred mid-September, while the peak of bleaching was registered in early October, about two weeks later (Figure 3A). Similar lag between heating and bleaching has been observed by other authors. For example, Berkelmans and Willis (1999) found a one-month delay in bleaching for thermally sensitive corals Acropora formosa and Pocillopora damicornis on the Great Barrier Reef, Australia. A comparable situation was observed on the reefs of the Bahamas, where the decline in zooxanthellae density (start of bleaching) occurred after the period of maximum temperatures (Fitt et al., 2000). The lag in bleaching could be attributed to cumulative stress persists on the reef after the period of water heating (Afrin, 2021).
It is known that typhoons have a destructive impact on coral communities, causing mechanical damage to colonies (Harmelin-Vivien, 1994; Jokiel, 2006; Guillemot et al., 2010). Typhoons bring abundant rainfall and, as a result of increased river runoff, increase turbidity and decrease salinity of coastal waters. In turn, decreased water transparency leads to a reduction in the photosynthetic activity of zooxanthellae. Therefore, during typhoons, deterioration of the overall health of the colonies and bleaching can be expected (Banner, 1968; Jokiel et al., 1993; Philipp and Fabricius, 2003; Piniak and Storlazzi, 2008).
In the area of our study, typhoons occur in the autumn months, from late October to December. In 2020, rainy season began in the late September and continued until December, with the highest amount of precipitation in the late November, which corresponds to long-term observations of precipitation in Nha Trang (Le et al., 2022). However, contrary to expectations, we did not observe an increase in bleaching during this period. Moreover, a positive correlation between wind speed (a proxy for wave impact) and the percentage of bleached colonies (Figure 7C), many of which recovered during this period was registered. So, what explains this contradiction?
We believe that the negative wave impact on the condition of corals may exist, but it is not prominent since it is largely compensated by the positive effect of decreased water temperature during typhoons. This assumption is supported by data from other studies on the mitigating effect of hurricanes on accumulated thermal stress due to temperature decrease (Skirving et al., 2006; Manzello et al., 2007; Heron et al., 2008). In particular, it was found that increased mixing between the surface and deeper waters characterized by lower temperatures affects the rise of the temperature, providing the corals with the time necessary for thermal acclimation (Skirving et al., 2006). In addition, colonies in the present experiment likely managed to avoid the negative impact of increased water turbidity due to rapid rates of photo-acclimation, as a likely mechanism by which corals maintain a positive energy balance on coral reefs in turbid season (Anthony and Larcombe, 2000; Zweifler et al., 2021). Finally, it is known that a critical salinity decrease (to 20-26 psu) may lead to coral bleaching and death, negatively impact the reproductive success of corals (Banner, 1968; Jokiel et al., 1993; Chui et al., 2016; Xie et al., 2017). However, such a decrease in salinity is usually recorded at the surface level (Jokiel et al., 1993; Xie et al., 2017), whereas colonies in the present study were located below this freshened layer of water at the depth (3 m) where such a decrease of salinity was never recorded before (Britayev, personal observation). Thus, experimental colonies avoided exposure to low salinity.
Colony density is another factor which positively, yet statistically insignificant influences bleaching. We found that the percentage of bleached colonies was higher on frames with higher colony density. The maximum differences in shares of bleached colonies were observed between frames with the minimum and maximum densities (13 and 40 colonies per m², respectively), but even in this case they were statistically significant only during periods of maximum bleaching development (from October to December, Figure 3D). Possible reasons for increased bleaching in higher density coral settlements could be the deterioration of water circulation around the colonies. This assumption is supported by data of Nakamura et al. (2003) and Nakamura and van Woesik (2001) showed that moderate to high water flow prevents colonies from bleaching under high temperature and light conditions and promotes their rapid recovery after bleaching.
Partial mortality is a factor of bleaching, that differs in nature from others reviewed here. First of all, it is clearly a factor that influences coral fitness directly. At the same time, this is a factor, which development is significantly determined by the impact of other environmental parameters (Figures 7E, G). Summing up, we treat PM as a secondary factor that directly affects bleaching. According to the analysis, PM has moderate and significant negative impact on the condition of the whole coral colony. We tend to believe that the initial lesions (mechanical damage) unlikely could affect the condition of the whole colony, but algal fouling which always follows the lesions, may significantly aggravate colony health. Metabolites of algae, which cause hypoxia in coral tissues, can be the inductor of weakening of the whole colony (Smith et al., 2006). Moreover, crude extracts of macroalgae affect coral microbiome and caused significant bleaching and reduction in coral photosynthetic efficiency (Rasher et al., 2011; Fong et al., 2023). The reality of this scenario is confirmed by other authors as well. For example, Wangpraseurt et al. (2012) demonstrated that the fouling of Porites spp. by turf- and coralline algae, was toxic to corals. Thus, metabolites of fouling algae are poisoning the colony, which leads to bleaching, and the more severe is fouling, the more severe is its impact on bleaching.
4.2 Impact of environmental factors on partial mortality
By the end of the observation period (11 months of exposure), the percentage of colonies with PM reached 47%. Since we recorded PM only after the development of algal overgrowth, this value should actually be slightly higher. PM varies depending on the local characteristics of habitats and coral species (Table 2; Supplementary Table S5). Specifically, it was higher for Pocillopora eydouxi and P. verrucosa on the coast of Kenya (10% and 20.7% respectively, Montano et al., 2010), but lower for P. damicornis on the coast of Australia - 59% (Pisapia and Pratchett, 2014). With age and, consequently, the size of colonies, the prevalence of PM increases (Figure 6B), indicating a predominance of damage formation over regeneration and/or irreversible transformation of lesions into PM as it has been recorded by Meesters et al. (1996) on two species of Atlantic corals Diploria strigosa and Porites astreoides.
Among the factors we analyzed (temperature, wind speed, precipitation, colony density and bleaching), only the first and the third were found to affect significantly the development of PM (Figures 7E, G). It is known that temperature stress indirectly impacts the occurrence of PM by causing severe bleaching, which leads to the emergence of areas with PM (Carilli et al., 2012). However, Montano et al. (2010), who studied the influence of temperature stress on Pocillopora eydouxi and P. verrucosa corals (MNPs Watamu, Kenya), observed total and partial mortality after bleaching, but did not associate PM with bleaching. In our case, temperature also has a significant effect on the occurrence of PM, but this effect is negative. At high temperatures (September - early October), increase of the percentage of colonies with PM was close to zero, but decrease of the temperature in November-January, lead to rapid increase of the share of colonies with PM (Figure 6A). However, in this case, we observe the influence not of temperature, but of the impact of storms, coinciding in time with a decrease in temperature (Figure 7G).
Eastern wind speed was found to have strong positive impact on the development of PM (Figure 7G). The highest values of this measure correspond to the period of typhoons in Nha Trang (Le et al., 2022). The appearance of PM is largely related to mechanical damage to coral tissues by suspended particles of sand and debris, which we observed directly during colony surveys in November (Britayev, personal observation).
Intense sedimentation (the consequence of increased precipitation) can lead to local hypoxia on the colony surface and contribute to the formation of PM (Nugues and Roberts, 2003), however in the present case impact of precipitation was not statistically significant.
Although high colony density can disrupt local hydrodynamics and cause partial or complete colony mortality (Hennige et al., 2021), in or study, the impact of this factor was evident only at the peak of the PM development. Moreover, higher-density nurseries tended to be less susceptible to PM development than low-density nurseries, likely because in dense populations, colonies protect each other from exposure to mechanical impact of solid particles carried by wave currents.
We believe also that even seasonal bleaching can impact the development of PM (Figure 6A). This was proven by the analysis of impacts on experimental colonies, which revealed that prior to the development of PM affected colonies experienced more severe bleaching than those that did not suffer from PM.
Another direct cause of the appearance of PM on colonies could be the spread of algal fouling from the inorganic substrate (metallic frames or fastening wire) onto the coral tissue. According to our observations, this can occur when colonies are weakened by bleaching or mechanical damage (Figure 4C). As it was mentioned before, metabolites of fouling algae are toxic to corals and may induce weakening of the whole coral colony (Smith et al., 2006; Wangpraseurt et al., 2012). Moreover, there are algae capable to colonize directly live corals, causing the death of their tissues (Jompa and McCook, 2003), and subsequently, the dead surface areas are colonized by various species of filamentous algae (mixed-species turfs). The active development of macroalgae on the surface of corals in our experiment is expected in conditions of chronic eutrophication and long-term total overfishing, which are characteristic of the Nha Trang Bay and lead to a shift in coral-algae interactions towards algae (Tkachenko et al., 2016). However, further experimental studies are needed to understand whether fouling development causes mortality of adjacent coral tissues.
4.3 Colony mortality and its causes
By the end of the 11-month experiment, the number of dead fragments accounted for 4.5%, which is a low figure compared to data from other authors. For example, off the coast of Sumatra (Indonesia), the mortality rate of P. verrucosa fragments after 11 months of transplantation was approximately 35-40% (Ferse, 2010). In nurseries off the coast of Tanzania (Zanzibar and Mafia islands), the mortality of P. verrucosa over 9 months of exposure was slightly lower and ranged from 10 to 40% depending on the specific nursery and its location (Mbije et al., 2010).
Interestingly, the dynamics of mortality in this case are fundamentally different from what was observed in our experiment. Within our framework, all P. verrucosa colonies were alive during the first eight months, and the first dead colonies appeared in the ninth month of the experiment, while the mortality off the coast of Tanzania occurred mainly in the early stages of colony formation - during the first 2-4 months, and then ceased (Mbije et al., 2010). Such a difference in the dynamics of mortality clearly indicates differences in its causes. The mortality of colonies off the coast of Tanzania was caused by fish bites, predation by coralivorous animals, and competition with fouling organisms (Mbije et al., 2010) whereas in Nha Trang Bay, the main cause for the death of colonies is the development of PM. Really, in Nha Trang Bay, the impact of coral-eating fish is minimal due to a drastic decrease in fish diversity and abundance, regardless of their trophic affiliation and commercial value that has occurred over the past 10-15 years (Tkachenko et al., 2016; Tkachenko, 2023). Additionally, the design of the nurseries we used (frames raised above the bottom) prevents attacks on coral colonies by the main predators, coral-eating starfish Acanthaster planci and limits the attacks by the mollusk Drupella cornus. Thus, coralivorous animals were nearly excluded in our experiment. Seasonal bleaching itself also does not lead to the death of colonies: there was not a single colony whose death was preceded only by bleaching without PM. At the same time, the death of all colonies was preceded by the development of PM. We did not register the area of the PM, but the photos show that all the dead colonies were covered by fouling (Figure 4С). Moreover, backtracking of dead colonies demonstrated that a third of lost colonies suffered from PM without bleaching before death. Based on these arguments we tend to consider PM as the main cause of mortality in experimental colonies.
It is interesting that two thirds of the dead colonies suffered from bleaching before death, and their bleaching did not coincide with the peak of bleaching (October), but occurred after development of PM on colonies. Considering that the dying colonies either were not suffering from bleaching or had recovered from bleaching, as well as data on impact of macroalgae on the coral microbiome (Fong et al., 2023) we believe that pre-mortality bleaching was induced by severely developed PM. This conclusion corresponds well to our data on the impact of PM on bleaching. Another important finding of our study is that the role of mass bleaching or mass PM events in mortality tends to be overestimated. Only one colony suffered from bleaching during autumn bleaching event died to the end of the experiment, and only one third of dead colonies got PM during the typhoon season.
Our observation shows that in case of most colonies which died to the end of experiment, impact of individual stress (development of PM or bleaching) prevailed over the impact of mass or seasonal events. Otherwise, monitoring of seasonal bleaching and PM development on the population scale provides insufficient information for reliable predicting of individual colonies’ mortality. Thus we believe that even though both processes are associated with a potential threat to corals, they represent the variation of normal condition.
5 Summary
The present study demonstrated that both bleaching and PM have pronounced seasonal variation, however, the maximum manifestation of these phenomena does not coincide in time. Bleaching peaks occurred in spring (April) and autumn (October), while the maximum of PM was observed in winter (December). This is due to the difference and, even, the divergence of factors affecting bleaching and PM (Figures 7, 8). In particular, the main predictor of bleaching is the temperature stress, but the increase in the percentage of colonies with PM is minimal during the temperature maximum. At the same time, the vast majority of colonies recovered from bleaching during the period of increased wave action, which is the main factor of the PM development. Even though, in most cases the density of coral population does not affect bleaching and PM, during the harshest periods it affects both measures in the opposite ways. Colonies in high-density settlements are more susceptible to bleaching than in sparse settlements, at the same time, nurseries with a higher colony density are less susceptible to PM development than low-density ones. Worth mentioning that both bleaching, and PM significantly yet not very strongly affect each other (Figure 8). Bleaching contributes to development of partial mortality. Even more is pronounced the effect of PM on corals, colonies suffering from PM become much more susceptible to bleaching.
The present experiment was designed in the way that excluded or minimized such potential causes of colony mortality as exposure to corallophagous fish, starfish Acanthaster planci and the mollusk Drupella cornus. This was due to both the design of nurseries, which excludes or reduces the impact of predatory starfish and mollusks, and to the consequences of chronic overfishing in Nha Trang Bay. The main cause of colony mortality in the present study was the development of algal fouling at the sites of colony lesions, which gradually spread over the entire surface of the colony. In turn, the intensive development of algal fouling is probably driven by the eutrophication of the bay (Tkachenko et al., 2016).
Considering that in the present experiment the young corals were studied, in the future ageing of corals will lead to increase of mortality rates. Moreover, other causes of mortality, such as bacterial or fungal infestation or destruction by perforating organisms, may manifest themselves. However, testing of this hypothesis requires further research. Additionally, future studies would require analysis of the factors directly affecting corals (such as wave action, sedimentation, salinity, etc.) and not their proxies, since the latter have an overly complex structure, including multiple, potentially multidirectional Impacts.
The findings of the present study have valuable practical implications, providing insights for coral restoration plan for Central Vietnam. Specifically, it confirmed that late spring is the best season for planting of corals, it showed that in the late autumn corals require additional protection to negate the destructive wave impact. Based on the data obtained, we suggest planting corals on elevated nurseries at the densities of 25-30 colonies per m2.
Data availability statement
The raw data supporting the conclusions of this article will be made available by the authors, without undue reservation.
Ethics statement
The manuscript presents research on animals that do not require ethical approval for their study.
Author contributions
FL: Data curation, Formal analysis, Investigation, Methodology, Visualization, Writing – original draft, Writing – review & editing. YB: Data curation, Investigation, Methodology, Visualization, Writing – original draft. RP: Data curation, Investigation, Writing – review & editing. TN: Data curation, Investigation, Resources, Validation, Writing – review & editing. TB: Conceptualization, Data curation, Formal analysis, Funding acquisition, Investigation, Methodology, Project administration, Resources, Supervision, Validation, Visualization, Writing – original draft, Writing – review & editing.
Funding
The author(s) declare financial support was received for the research, authorship, and/or publication of this article. This work was supported by a grant from the Russian Science Foundation, RSF 22-24-00836. Open-access publication fees were covered by the state of Bremen.
Acknowledgments
We are sincerely grateful to K.S. Tkachenko for providing us the temperature data. This work was performed in the framework of the research program Ecolan E3.1 “Conservation, restoration and sustainable development of marine coastal ecosystems based on the study of their structural and functional organization” of the Joint Vietnam-Russia Tropical Science and Technology Research Center.
Conflict of interest
The authors declare that the research was conducted in the absence of any commercial or financial relationships that could be construed as a potential conflict of interest.
Publisher’s note
All claims expressed in this article are solely those of the authors and do not necessarily represent those of their affiliated organizations, or those of the publisher, the editors and the reviewers. Any product that may be evaluated in this article, or claim that may be made by its manufacturer, is not guaranteed or endorsed by the publisher.
Supplementary material
The Supplementary Material for this article can be found online at: https://www.frontiersin.org/articles/10.3389/fmars.2024.1338464/full#supplementary-material
References
Afrin Z. (2021). Determining Thermal stress using indices: sea surface temperature anomalies, degree heating days and heating rate to allow for-casting of coral bleaching risk (Suva, Fiji: School of Marine Science, University of the South Pacific).
Anthony K. R. N., Larcombe P. (2000). “Coral reefs in turbid waters: sediment-induced stresses in corals and likely mechanisms of adaptation,” in Proceedings of the international 9th coral reef symposium, Vol. 1. Ed. Hidup K. M. N. L. (Bali, Indonesia: Ministry of Environment), 239–244.
Banaszak A. T., Lesser M. P. (2009). Effects of solar ultraviolet radiation on coral reef organisms. Photochem. Photobiol. Sci. 8 (9), 1276–1294. doi: 10.1039/b902763g
Banner A. (1968). A fresh-water “kill” on the coral reefs of Hawaii, Vol. 15 (Honolulu, Hawaii: Hawaii Institute of Marine Biology, University of Hawaii).
Bellwood D. R., Hoey A. S., Choat J. H. (2003). Limited functional redundancy in high diversity systems: resilience and ecosystem function on coral reefs. Ecol. Lett. 6 (4), 281–285. doi: 10.1046/j.1461-0248.2003.00432.x
Berkelmans R., Willis B. L. (1999). Seasonal and local spatial patterns in the upper thermal limits of corals on the inshore Central Great Barrier Reef. Coral Reefs. 18 (3), 219–228. doi: 10.1007/s003380050186
Birrell C. L., McCook L. J., Willis B. L. (2005). Effects of algal turfs and sediment on coral settlement. Mar. pollut. Bull. 51, 408–414. doi: 10.1016/j.marpolbul.2004.10.022
Britayev T. A., Petrochenko R. A., Burmistrova Y. A., Nguyen T. H., Lishchenko F. V. (2023). Density and bleaching of corals and their relationship to the coral symbiotic community. Diversity. 15 (3), 456. doi: 10.3390/d15030456
Brown B. E. (1997). Coral bleaching: causes and consequences. Coral Reefs. 16, S129–S138. doi: 10.1007/s003380050249
Brown B. E., Dunne R. P., Ambarsari I., Le Tissier M., Satapoomin U. (1999). Seasonal fluctuations in environmental factors and variations in symbiotic algae and chlorophyll pigments in four Indo-Pacific coral species. MEPS. 191, 53–69. doi: 10.3354/meps191053
Brown C. J., Saunders M. I., Possingham H. P., Richardson A. J. (2013). Managing for Interactions between local and global stressors of ecosystems. PloS One 8 (6), e65765. doi: 10.1371/journal.pone.0065765
Carilli J., Donner S. D., Hartmann A. C. (2012). Historical temperature variability affects coral response to heat stress. PloS One 7 (3), e34418. doi: 10.1371/journal.pone.0034418
Chui A. P. Y., Yeung C. W., Leung Tsang R. H., Leung Y. H., Ng T. Y., Chai K. H., et al. (2016). Lowered temperature and reduced salinity retarded development of early life history stages of Acropora valida from the marginal environment. Regional Stud. Mar. Science. 8 (3), 430–438. doi: 10.1016/j.rsma.2016.04.004
Coles S. L., Jokiel P. L. (1978). Synergistic effects of temperature, salinity and light on the hermatypic coral Montipora verrucosa. Mar. Biol. 49 (3), 187–195. doi: 10.1007/BF00391130
Combillet L., Fabregat-Malé S., Mena S., Marín-Moraga J. A., Gutierrez M., Alvarado J. J. (2022). Pocillopora spp. growth analysis on restoration structures in an Eastern Tropical Pacific upwelling area. PeerJ. 10, e13248. doi: 10.7717/peerj.13248
Cornwall C. E., Comeau S., Kornder N. A., Perry C. T., Hooidonk R., DeCarlo T. M., et al. (2021). Global declines in coral reef calcium carbonate production under ocean acidification and warming. PNAS. 118 (21), e2015265118. doi: 10.1073/pnas.2015265118
Crain C. M., Kroeker K., Halpern B. S. (2008). Interactive and cumulative effects of multiple human stressors in marine systems. Ecol. Lett. 11, 1304–1315. doi: 10.1111/j.1461-0248.2008.01253.x
Cumming R. L. (2002). Tissue injury predicts colony decline in reef-building corals. Mar. Ecol. Prog. Ser. 242, 131–141. doi: 10.3354/meps242131
Dautova T. N., Latypov Y. Y., Savinkin O. V. (2007). “Annotated checklist of scleractinian corals, Millepores and Heliopora of Nhatrang Bay,” in Bentic fauna of the Bay of Nhatrang Southern Vietnam. Eds. Britayev T. A., Pavlov D. S. (Moscow: KMK Scientific Press), 13–61.
Dellisanti W., Chung J. T. H., Yiu S. K. F., Tsang R. H. L., Ang P., Yeung Y. H., et al. (2023). Seasonal drivers of productivity and calcification in the coral Platygyra carnosa in a subtropical reef. Front. Mar. Sci. 10. doi: 10.3389/fmars.2023.994591
Diaz-Pulido G., Harii S., McCook L. J., Hoegh-Guldberg O. (2010). The impact of benthic algae on the settlement of a reef-building coral. Coral Reefs. 29 (1), 203–208. doi: 10.1007/s00338-009-0573-x
Dollar S. J., Grigg R. W. (1981). Impact of a kaolin clay spill on a coral reef in Hawaii. Mar. Biol. 65, 269–276. doi: 10.1007/BF00397121
Douglas A. E. (2003). Coral bleaching – how and why? Mar. pollut. Bulletin. 46 (4), 385–392. doi: 10.1016/S0025-326X(03)00037-7
Eakin C. M., Lough J. M., Heron S. F. (2009). “Climate Variability and Change: Monitoring Data and Evidence for Increased Coral Bleaching Stress,” in Coral Bleaching: Patterns, Processes, Causes and Consequences. Eds. Oppen M. J. H., Lough J. M. (Berlin, Heidelberg: Springer), 41–67.
Eakin C. M., Morgan J. A., Heron S. F., Smith T. B., Liu G., Alvarez-Filip L., et al. (2010). Caribbean corals in crisis: record thermal stress, bleaching, and mortality in 2005. PloS One 5 (11), e13969. doi: 10.1371/journal.pone.0013969
Eddy T. D., Lam V. W. Y., Reygondeau G., Cisneros-Montemayor A. M., Greer K., Palomares M., et al. (2021). Global decline in capacity of coral reefs to provide ecosystem services. One Earth. 4, 1278–1285. doi: 10.1016/j.oneear.2021.08.016
Fabricius K. (2011). “Factors determining the resilience of coral reefs to eutrophication: a review and conceptual model,” in Coral Reefs: An Ecosystem in Transition. Eds. Dubinsky Z., Stambler N. (Netherlands: Springer), 493–505.
Ferrario F., Beck M. W., Storlazzi C. D., Micheli F., Shepard C. C., Airoldi L. (2014). The effectiveness of coral reefs for coastal hazard risk reduction and adaptation. Nat. Commun. 5, 3794. doi: 10.1038/ncomms4794
Ferse S. C. A. (2010). Poor performance of corals transplanted onto substrates of short durability. Restor. Ecology. 18 (4), 399–407. doi: 10.1111/j.1526-100X.2010.00682.x
Fine M., Tchernov D. (2007). Scleractinian coral species survive and recover from decalcification. Science. 315 (5820), 1811. doi: 10.1126/science.1137094
Fisher R., Bessell-Browne P., Jones R. (2019). Synergistic and antagonistic impacts of suspended sediments and thermal stress on corals. Nat. Commun. 10, 2346. doi: 10.1038/s41467-019-10288-9
Fitt W. K., Brown B. E., Warner M. E., Dunne R. P. (2001). Coral bleaching: Interpretation of thermal tolerance limits and thermal thresholds in tropical corals. Coral Reefs. 20 (1), 51–65. doi: 10.1007/s003380100146
Fitt W. K., McFarland F. K., Warner M. E., Chilcoat G. C. (2000). Seasonal patterns of tissue biomass and densities of symbiotic dinoflagellates in reef corals and relation to coral bleaching. Limnol. Oceanogr. 45 (3), 677–685. doi: 10.4319/lo.2000.45.3.0677
Fong J., Tang P. P. Y., Deignan L. K., Seah J. C. L., McDougald D., Rice S. A., et al. (2023). Chemically mediated interactions with macroalgae negatively affect coral health but induce limited changes in coral microbiomes. Microorganisms. 11 (9), 2261. doi: 10.3390/microorganisms11092261
Glynn P. W. (1983). Extensive ‘Bleaching’ and death of reef corals on the pacific coast of panamá. Environ. Conserv. 10, 149–154. doi: 10.1017/S0376892900012248
Glynn P. W. (2000). “Effects of the 1997-98 ENSO on Eastern Pacific corals and coral reefs: an overview,” in Proceedings of the international 9th coral reef symposium, Vol. 2. Eds. Moosa M. K., Hidup K. M. N. L. (Bali, Indonesia: Ministry of Environment), 1169–1174.
Glynn P. W., D’Croz L. (1990). Experimental evidence for high temperature stress as the cause of El Niño-coincident coral mortality. Coral Reefs 8, 181–191. doi: 10.1007/BF00265009
Guillemot N., Chabanet P., Le Pape O. (2010). Cyclone effects on coral reef habitats in New Caledonia (South Pacific). Coral Reefs. 29 (2), 445–453. doi: 10.1007/s00338-010-0587-4
Harmelin-Vivien L. M. (1994). The effects of storms and cyclones on coral reefs: A review. J. Coast. Res. 12, 211–231. doi: 10.2307/25735600
Hennige S., Larsson A., Orejas C., Gori A., De Clippele L., Lee Y., et al. (2021). Using the Goldilocks Principle to model coral ecosystem engineering. Proc. Biol. Sci. 288 (1956), 20211260. doi: 10.1098/rspb.2021.1260
Heron S., Morgan J., Eakin M., Skirving W. (2008). “Hurricanes and their effects on coral reefs,” in Status of Caribbean Coral Reefs after Bleaching and Hurricanes in 2005. Eds. Wilkinson C., Souter D. (Townsville, Australia: Global Coral Reef Monitoring Network and Reef and Rainforest Research Centre), 31–36.
Hoegh-Guldberg O. (1999). Climate change, coral bleaching and the future of the world’s coral reefs. Mar. Freshw. Res. 50 (8), 839–866. doi: 10.1071/MF99078
Hoegh-Guldberg O. (2011). Coral reef ecosystems and anthropogenic climate change. Regional Environ. Change. 11, 215–227. doi: 10.1007/s10113-010-0189-2
Hoegh-Guldberg O. (2014). Coral reefs in the Anthropocene: persistence or the end of the line? Geol. Soc Spec. 395 (1), 167–183. doi: 10.1144/SP395.17
Hoegh-Guldberg O., Salvat B. (1995). Periodic mass-bleaching and elevated sea temperatures: Bleaching of outer reef slope communities in Moorea, French Polynesia. Mar. Ecol. Prog. Ser. 121, 181–190. doi: 10.3354/meps121181
Hughes T. P., Barnes M. L., Bellwood D. R., Cinner J. E., Cumming G. S., Jeremy B., et al. (2017). Coral reefs in the anthropocene. Nature. 546 (7656), 82–90. doi: 10.1038/nature22901
Johnston E. C., Forsman Z. H., Flot J. F., Schmidt-Roach S., Pinzón J. H. C., Knapp I., et al. (2017). A genomic glance through the fog of plasticity and diversification in Pocillopora. Sci. Rep. 7 (1), 5991. doi: 10.1038/s41598-017-06085-3
Jokiel P. (2006). “Impact of storm waves and storm floods on Hawaiian reefs,” in Proceedings of the 10th International Coral Reef Symposium. Ed. Y. i Suzuki (Okinawa, Japan: Japanese Coral Reef Society), 282–284.
Jokiel P., Hunter C., Taguchi S., Watarai L. (1993). Ecological impact of a fresh-water “reef kill” in Kaneohe Bay, Oahu, Hawaii. Coral Reef. 12, 177–184. doi: 10.1007/BF0033447
Jompa J., McCook L. (2003). Contrasting effects of turf algae on corals: massive Porites spp. are unaffected by mixed-species turfs, but killed by the red alga Anotrichium tenue. MEPS. 258, 79–86. doi: 10.3354/meps258079
Latypov Y. Y. (2016). Results of thirty years of research on corals and reefs of Vietnam. Open J. Mar. Science. 06 (02), 283–292. doi: 10.4236/ojms.2016.62023
Le D. M., Vlasova G. A., Nguyen D. T. T., Pham H. S., Nguyen T. V. (2022). Distribution features of the weather conditions in Nha Trang Bay (The South China Sea). Russian J. Earth Sci. 22, Es3001. doi: 10.2205/2022ES000791
Lesser M. P. (2011) “Coral bleaching: causes and mechanisms,” in: Coral reefs: An ecosystem in transition. Eds. Dubinsky Z., Stambler N. (Dordrecht: Springer), 405–419. doi: 10.1007/978-94-007-0114-4_23
Lough J. M., Anderson K. D., Hughes T. P. (2018). Increasing thermal stress for tropical coral reefs: 1871–2017. Sci. Rep. 8, 6079. doi: 10.1038/s41598-018-24530-9
Loya Y., Sakai K., Yamazato K., Nakano Y., Sambali H., van Woesik R. (2001). Coral bleaching: the winners and the losers. Ecol. Lett. 4 (2), 122–131. doi: 10.1046/j.1461-0248.2001.00203.x
Manzello D., Brandt M., Smith T., Lirman D., Hendee J., Nemeth R. (2007). Hurricanes benefit bleached corals. PNAS. 104 (29), 12035–12039. doi: 10.1073/pnas.0701194104
Mbije N. E. J., Spanier E., Rinkevich B. (2010). Testing the first phase of the ‘gardening concept’ as an applicable tool in restoring denuded reefs in Tanzania. Ecol. Engineering. 36 (5), 713–721. doi: 10.1016/j.ecoleng.2009.12.018
McCook L. (2001). Competition between corals and algal turfs along a gradient of terrestrial influence in the nearshore central Great Barrier Reef. Coral Reefs. 19 (4), 419–425. doi: 10.1007/s003380000119
Meesters E. H., Wesseling I., Bak R. P. M. (1996). Partial mortality in three species of reef-Building corals and the relation with colony morphology. Bull. Mar. Science. 58 (3), 838–852.
Montano S., Seveso D., Galli P., Obura D. (2010). Assessing coral bleaching and recovery with a colour reference card in Watamu Marine Park, Kenya. Hydrobiologia. 655 (1), 99–108. doi: 10.1007/s10750-010-0407-4
Muller E. M., Sartor C., Alcaraz N., van Woesik R. (2020). Spatial epidemiology of the stony-coral-tissue-loss disease in Florida. Front. Mar. Sci. 7. doi: 10.3389/fmars.2020.00163
Nakamura T., van Woesik R. (2001). Water-flow rates and passive diffusion partially explain differential survival of corals during the 1998 bleaching event. MEPS. 212, 301–304. doi: 10.3354/meps212301
Nakamura T., Yamasaki H., van Woesik R. (2003). Water flow facilitates recovery from bleaching in the coral Stylophora pistillata. MEPS. 256, 287–291. doi: 10.3354/meps256287
Nir O., Gruber D. F., Shemesh E., Glasser E., Tchernov D. (2014). Seasonal mesophotic coral bleaching of stylophora pistillata in the northern red sea. PloS One 9 (1), e84968. doi: 10.1371/journal.pone.0084968
Nugues M. M., Roberts C. M. (2003). Partial mortality in massive reef corals as an indicator of sediment stress on coral reefs. Mar. pollut. Bulletin. 46 (3), 314–323. doi: 10.1016/S0025-326X(02)00402-2
Oury N., Noël C., Mona S., Aurelle D., Magalon H. (2023). From genomics to integrative species delimitation? The case study of the Indo-Pacific Pocillopora corals. Mol. Phylogenet. Evolution. 184, 107803. doi: 10.1016/j.ympev.2023.107803
Pandolfi J. M., Bradbury R. H., Sala E., Hughes T. P., Bjorndal K. A., Cooke R. G., et al. (2003). Global trajectories of the long-term decline of coral reef ecosystems. Science. 301 (5635), 955–958. doi: 10.1126/science.1085706
Philipp E., Fabricius K. (2003). Photophysiological stress in scleractinian corals in response to short-term sedimentation. J. Exp. Mar. Biol. Ecol. 287 (1), 57–78. doi: 10.1016/S0022-0981(02)00495-1
Piniak G., Storlazzi C. (2008). Diurnal variability in turbidity and coral fluorescence on a fringing reef flat: Southern Molokai, Hawaii. Estuarine Coast. Shelf Science. 77 (1), 56–64. doi: 10.1016/j.ecss.2007.08.023
Pisapia C., Anderson K. D., Pratchett M. S. (2016). Temporal consistency in background mortality of four dominant coral taxa along Australia’s Great Barrier Reef. Coral Reefs 35, 839–849. doi: 10.1007/s00338-016-1421-4
Pisapia C., Pratchett M. S. (2014). Spatial variation in background mortality among dominant coral taxa on Australia’s great barrier reef. PloS One 9 (6), e100969. doi: 10.1371/journal.pone.0100969
Qin Z., Yu K., Wang Y., Xu L., Huang X., Chen B., et al. (2019). Spatial and intergeneric variation in physiological indicators of corals in the south China sea: insights into their current state and their adaptability to environmental stress. JGR Oceans. 124, 3317–3332. doi: 10.1029/2018JC014648
Rasher D. B., Stout E. P., Engel S., Kubanek J., Hay M. E. (2011). Macroalgal terpenes function as allelopathic agents against reef corals. Proc. Natl. Acad. Sci. U.S.A. 108 (43), 17725–17731. doi: 10.1073/pnas.1108628108
Reaka-Kudla M., Wilson D., Wilson E. (1997). “The Global Biodiversity of Coral Reefs: A Comparison with Rain Forests,” in Biodiversity II: Understanding and protecting our biological resources. Eds. Reaka-Kudla M. L., Wilson D. E., Wilson E. O. (Washington, D.C.: Joseph Henry Press), 83–108.
Rivas N., Gómez C. E., Millán S., Mejía-Quintero K., Chasqui L. (2023). Coral reef degradation at an atoll of the Western Colombian Caribbean. PeerJ. 11, e15057. doi: 10.7717/peerj.15057
Rodgers K. S., Bahr K. D., Jokiel P. L., Richards Donà A. (2017). Patterns of bleaching and mortality following widespread warming events in 2014 and 2015 at the Hanauma Bay Nature Preserve, Hawai’i. PeerJ 5, e3355. doi: 10.7717/peerj.3355
Rodríguez-Villalobos J. C., Work T. M., Calderon-Aguilera L. E., Reyes-Bonilla H., Hernández L. (2015). Explained and unexplained tissue loss in corals from the Tropical Eastern Pacific. DAO. 116 (2), 121–131. doi: 10.3354/dao02914
Shu L., Yu. K.-f., Chen T., Shi Qi. (2011). Assessment of coral bleaching using symbiotic zooxanthellae density and satellite remote sensing data in the Nansha Islands, South China Sea. Chin. Sci. Bulletin. 56 (10), 1031–1037. doi: 10.1007/s11434-011-4390-6
Shu L., Yu. K.-f., Shi Qi., Chen T. (2008). Interspecies and spatial diversity in the symbiotic Zooxanthellae density in corals from northern South China Sea and its relationship to coral reef bleaching. Chin. Sci. Bulletin. 53 (2), 295–303. doi: 10.1007/s11434-007-0514-4
Siebeck U. E., Marshall N. J., Klüter A., Hoegh-Guldberg O. (2006). Monitoring coral bleaching using a colour reference card. Coral Reefs. 25 (3), 453–460. doi: 10.1007/s00338-006-0123-8
Silbiger N. J., Nelson C. E., Remple K., Sevilla J. K., Quinlan Z. A., Putnam H. M., et al. (2018). Nutrient pollution disrupts key ecosystem functions on coral reefs. Proc. Biol. Sci. 285 (1880), 20172718. doi: 10.1098/rspb.2017.2718
Skirving W., Heron M., Heron S. (2006). “The hydrodynamics of a bleaching event: implications for management and monitoring,” in Coral Reefs and Climate Changed: Science and Management. Eds. Phinney J. T., HoeghGuldberg O., Kleypas J., Skirving W., Strong A. (Washington DC, USA: AGU), 145–161.
Smith J. E., Shaw M., Edwards R., Obura D., Pantos O., Sala E., et al. (2006). Indirect effects of algae on coral: algae-mediated, microbe-induced coral mortality. Ecol. Lett. 9 (7), 835–845. doi: 10.1111/j.1461-0248.2006.00937.x
Tkachenko K. S. (2023). Degradation of coral reefs under complex impact of natural and anthropogenic factors with nha trang bay (Vietnam) as an example. Biol. Bull. Rev. 13 (5), 442–459. doi: 10.1134/S2079086423050079
Tkachenko K. S., Britayev T. A., Huan N. H., Pereladov M. V., Latypov Y. Y. (2016). Influence of anthropogenic pressure and seasonal upwelling on coral reefs in Nha Trang Bay (Central Vietnam). Mar. Ecol. 37 (5), 1131–1146. doi: 10.1111/maec.12382
Tkachenko K. S., Dung V., Ha V., Huan N. H. (2023). Coral reef collapse in South-Central Vietnam: a consequence of multiple negative effects. Aquat. Ecol. 57 (3), 65–83. doi: 10.1007/s10452-022-09994-2
Wangpraseurt D., Weber M., Røy H., Polerecky L., de Beer D., Suharsono, et al. (2012). In situ oxygen dynamics in coral-algal interactions. PloS One 7 (2), e31192. doi: 10.1371/journal.pone.0031192
Warner M. E., Chilcoat G. C., McFarland F. K., Fitt W. K. (2002). Seasonal fluctuations in the photosynthetic capacity of photosystem II in symbiotic dinoflagellates in the Caribbean reef-building coral Montastraea. Mar. Biol. 141 (1), 31–38. doi: 10.1007/s00227-002-0807-8
Weiss A., Martindale R. (2017). Crustose coralline algae increased framework and diversity on ancient coral reefs. PloS One 12 (8), e0181637. doi: 10.1371/journal.pone.0181637
Wenger A. S., Fabricius K., Jones G. P., Brodie J. E. (2015). “Effects of sedimentation, eutrophication, and chemical pollution on coral reef fishes,” in Ecology of Fishes on Coral Reefs. Ed. Mora C. (Cambridge: Cambridge University Press), 145–153.
Wilkinson C. (2004). Status of coral reefs of the world (Australian Institute of Marine Science (Townsville, Queensland: AIMS).
Xie J. Y., Lau D. C. C., Kei K., Yu V. P. F., Chow W.-K., Qiu J.-W. (2017). The 2014 summer coral bleaching event in subtropical Hong Kong. Mar. pollut. Bull. 124 (2), 653–659. doi: 10.1016/j.marpolbul.2017.03.061
Keywords: branching corals, bleaching, mortality, temperature stress, turbidity, density, wave action
Citation: Lishchenko FV, Burmistrova YA, Petrochenko RA, Nguyen TH and Britayev TA (2024) Seasonal bleaching and partial mortality of Pocillopora verrucosa corals of the coast of central Vietnam. Front. Mar. Sci. 11:1338464. doi: 10.3389/fmars.2024.1338464
Received: 15 November 2023; Accepted: 29 January 2024;
Published: 13 February 2024.
Edited by:
Lena Rölfer, Leuphana University Lüneburg, GermanyReviewed by:
Ramesh Chatragadda, Council of Scientific and Industrial Research (CSIR), IndiaWalter Dellisanti, University of Copenhagen, Denmark
Copyright © 2024 Lishchenko, Burmistrova, Petrochenko, Nguyen and Britayev. This is an open-access article distributed under the terms of the Creative Commons Attribution License (CC BY). The use, distribution or reproduction in other forums is permitted, provided the original author(s) and the copyright owner(s) are credited and that the original publication in this journal is cited, in accordance with accepted academic practice. No use, distribution or reproduction is permitted which does not comply with these terms.
*Correspondence: Fedor V. Lishchenko, Fedor-LN@ya.ru