- Departamento de Botánica, Ecología y Fisiología Vegetal, Universidad de La Laguna, La Laguna, Spain
We studied the primary succession of benthic communities in the lava flows of the Tajogaite volcano at 2, 4.5, and 7 months after the eruption ended. The lava from the Tajogaite created several lava flows and sterile rocky reefs that were monitored in both intertidal and subtidal areas up to 20 m depth. Sampling included macroinvertebrates and algae in the intertidal, and fishes, macroinvertebrates, and algae in the subtidal. A control zone was selected to compare the early colonisation of lava flows with that of a mature ecosystem. Colonisation of the lava flow was swift, with numerous species arriving and proliferating soon after the eruption ended. After 7 months, the total number of species recorded in the lava flows was 70, representing 64% of those found in the control zone. thus, communities were gradually becoming increasingly complex owing to the continuous incorporation of species. The number of fishes, and macroinvertebrates in both the intertidal and subtidal, lava flows increased progressively, approaching the values of the control zone. However, algae, in terms of total cover, presented values similar to the control zone from the beginning of the monitoring. All the communities have followed the same trajectory to converge towards communities like those in the control zone, although the rate at which they have changed with time differs. After seven months, differences in fishes between lava flows and the control zone were small, but they were still large with respect to macroinvertebrates and algae. Thus, according to each community of organisms, the benthic ecosystem of the lava flows was found at different stages of succession.
Introduction
Volcanic eruptions are the most common natural disaster affecting marine ecosystems, with dozens of them occurring every year (Global Volcanism Program, 2023), and depending on their intensity and type of materials released, they totally or partially affect the ecosystems (Crisafulli et al., 2015). Thus, pyroclasts and ash can partially or even completely cover an area, significantly reducing or altering ecosystem biodiversity (Vroom and Zgliczynski, 2011; Schils, 2012; Hart et al., 2022), as incandescent lava flows obliterate all forms of life on the territory (Thorton, 2007). Despite their catastrophic effects, lava flows are suitable for studying key ecological processes because they are free habitats. Immediately after lava flows cool, species progressively settle on the new substrate. This process is known as ‘primary succession due to the ecosystem evolves from the sterile state (Prach and Walker, 2018). In contrast to a ‘secondary succession, where the recovery of an ecosystem
does not start from zero rather from those species that managed to survive the disturbance.
Primary succession often shaping the ecosystems trajectories over years, decades, or centuries (Walker and del Moral, 2011). The early stages of succession, especially primary succession, are stochastic and strongly influenced by the availability of propagules, their ability to migrate, the establishment and growth of colonisers, and the initial interactions among colonists. In marine habitats, colonisation occurs rapidly, and organisms can settle and grow after substrate formation. Benthic organisms, such as microalgae, can be observed in lava flows a few days after the rocks have cooled. Thereafter, the first communities to thrive are commonly formed by pioneer species characterised by low competitiveness and high demographic dynamics (Connell and Slatyer, 1977). Unlike terrestrial vegetation, marine plant species do not require the evolution of mineralised soil to prosper (Railkin, 2003). Additionally, the dispersion and ability to colonise a new substrate in marine environments encounter fewer limitations and barriers than they do in terrestrial environments. Consequently, the incorporation and turnover of species during marine succession occur continuously and swiftly (Valiela, 1995; Cowen and Sponaugle, 2009).
Studies of true primary succession are rare, likely because of the logistical impossibility of studying an ecosystem as soon as it is created. Both artificial and natural collectors have been used to examine the succession of sessile organisms (e.g., Underwood and Chapman, 2006; Antoniadou et al., 2010). However, these approaches have certain limitations that must be considered before deciding whether the case under study is actually one of authentic primary succession. For example, the experimental substrate is placed in close proximity to and is influenced by communities in a stable climax stage from the surroundings. Additionally, they tend to ignore communities of vagile species and their interactions with sessile species. Furthermore, the size limitations of the experimental substrate result in low spatial representativeness of successional communities. Indeed, only in a few natural environments, such as volcanic islands, has it been possible to study primary succession early during the first year after the volcanic eruption. Thus, for example, Doty (1967), Jonsson (1968), Jonsson and Gunnarsson (1982), and Jewett et al. (2010) found that macroalgal communities in the initial stages of succession were dominated by fast-growing species with low morphological complexity. Initially, these communities were highly dynamic, with the number of species increasing over time and perennial species tending to replace ephemeral ones. The capacity of these species for dispersal is one of the primary obstacles to substrate colonisation. Most studies on primary succession have focused on one or more years after the volcanic eruption by comparing the status of communities established in lava flows of different ages. Consequently, several years and even decades later, notable differences exist in the populations and community structures between lava flows, as has been observed particularly in sessile invertebrates (Parrish, 2015; Schleyer et al., 2016; Jouval et al., 2020).
On 19 September 2021 the Cumbre Vieja volcanic ridge on La Palma Island erupted, giving rise to the Tajogaite volcano. Over the course of eighty-five days, the lava flowed from a height of 1100 m to the sea, forming several lava flows (Civico et al., 2022). The western Canary Islands have experienced periodic coastal remodelling, often resulting in the devastation of mature habitats and ecosystems, which were then replaced by new and sterile reefs. Over the last five centuries, La Palma has experienced five eruptions that have significantly altered parts of its southwest coast (Carracedo et al., 2001, 2022). These events likely exerted a long-term influence on the spatiotemporal dynamics of benthic communities. Two months after the eruption ceased, we monitored the lava flows encompassing the intertidal and subtidal habitats. Here, we present an assessment of early colonization and primary succession involving fishes, macroinvertebrates, and algae within the first seven months after the eruption. To study this process, we focus on analysing (i) the changes in species density and the total abundance of each group, (ii) the changes in the structure of the communities, and (iii) the specific response of the most abundant taxa in each community. To evaluate the trajectories of the communities toward climax communities, we have used a nearby control zone unaffected by the lava flows as a reference point.
Methods
Description of the lava flows and control zones
The lava flows are located on the west coast of the island in the Tazacorte municipality (28.6075°N, 17.9252°W) (Figure 1). The lava reached the coast four times between September 29th and December 1st of 2021 (Carracedo et al., 2022). Before the eruption, the impacted zone consisted of a boulder field, gravel, and sand shoreline in the intertidal zone, whereas sandy bottoms predominated in the subtidal zone up to a depth of 50 m with some rocky enclaves (Martin-Garcia et al., 2013). The new coastal sections formed by the lavas included one in the old Playa de Los Guirres, which is approximately 1,900 m long, and the other in Playa de La Vina, which is approximately 650 m long. The new and heterogeneous coastline is primarily composed of cliffs that are a few meters high, along with boulders, gravel, and some platforms, although it has experienced significant erosion by waves. In the subtidal area, consolidated rocks are mixed with rocky fragments resulting from rapid cooling of the lava. The penetration of lava into the seabed was uneven. In the area of Playa de La Vina, the lava flows reaches a depth of 10 m, whereas in that of Playa de Los Guirres, the lava front reaches depths varying between 20 and 50 m, although at some points, the lava flowed down the insular slope to depths exceeding 125 m. The new rocky bottoms are highly abrupt, heterogeneous, and feature a notable slope. The predominant basaltic lavas appear stony and rough, including ‘type-aa, ‘type-pahoehoe, and ‘type-pillows. Consolidated flows of large rock blocks alternate with fragments of loose rock, varying in size and displaying irregular shapes (Sangil et al., 2023).
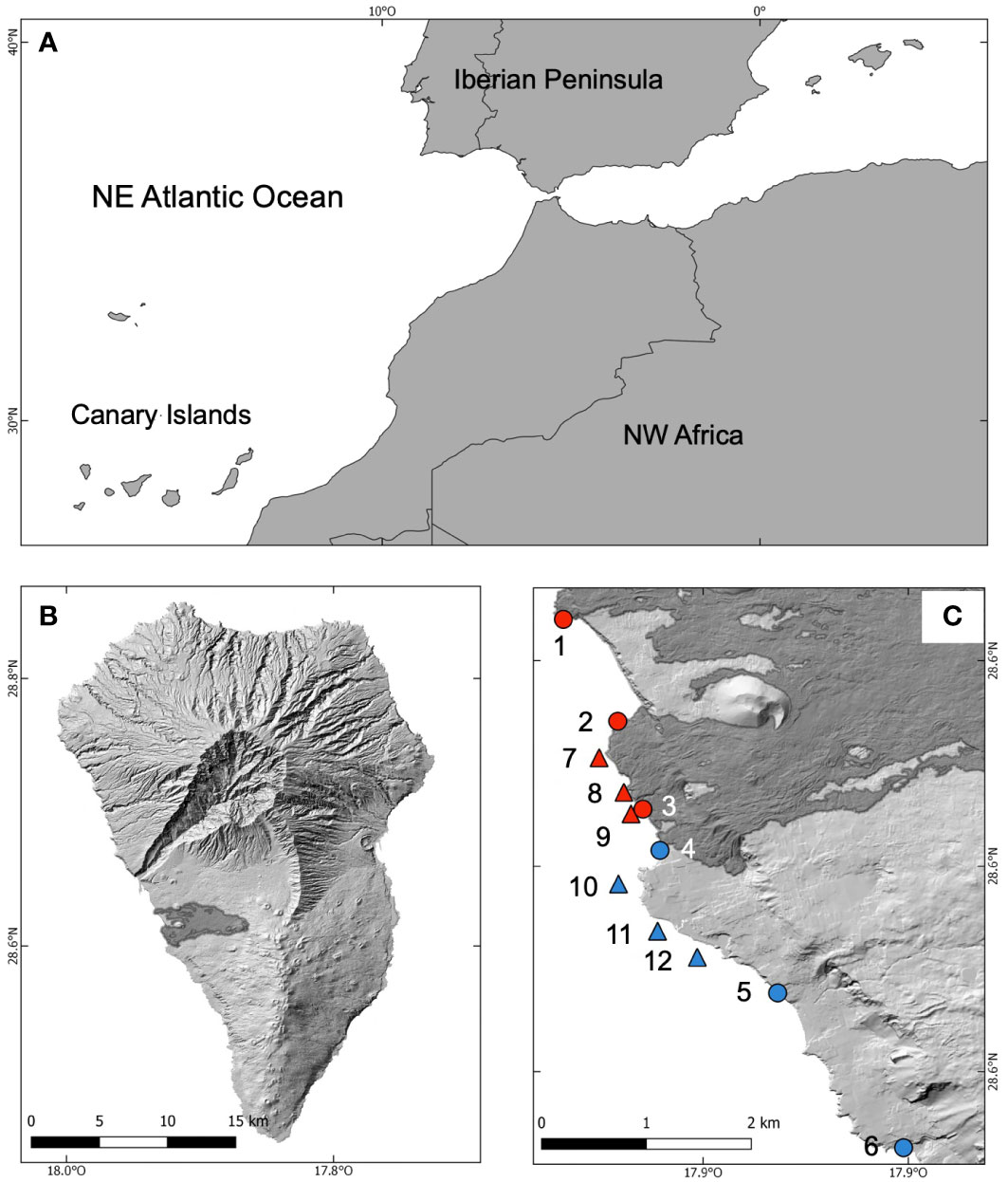
Figure 1 Location of the study area. The situation of the Canary Islands in the Northeastern Atlantic Ocean (A), a map of La Palma showing the lava flows of the Tajogaite volcano in dark grey (B), and the situation of the sampling sites (C). In red, sampling sites at the lava flows, in blue, sites at the control zone. Circles intertidal sites, triangles subtidal sites.
To compare the colonisation processes of the lava flows, we selected a nearby closed zone to the south (Figure 1). This choice was made to identify a zone that was as similar as possible to the new habitats in terms of geomorphology and oceanographic conditions. In so doing, we aimed to avoid or minimise site-specific effects. The sampling sites in the control zone are located on the lava flows formed after the San Juan and Tajuya eruptions in 1949 and 1585, respectively.
Benthic rocky communities in unaffected intertidal areas consist of cespitose macroalgae, such as non-crustose coralline (geniculate) and Rhodomelaceae, and crustose coralline algae (CCA) (Sangil et al., 2005). Mollusks are common, with some species such as Phorcus sauciatus and Patella spp. that are exploited for human consumption (Lopez et al., 2012). Meanwhile, non-crustose and crustose corallines thrive along with Dictyotaceae algae and some filamentous red algae in shallow subtidal areas. Wave action prevents the establishment of macroscopic organisms on the sandy bottoms of this part of the island. Populations of the garden eel Heteroconger longissimus and some macroinvertebrates develop only in deeper (3040 m) waters (Martin-Garcia et al., 2013).
Sampling
During the active eruption, anomalous data, such as pH, temperature, salinity, and CO2 inorganic system, were registered at the interface between the lava and seawater (Gonzalez-Santana et al., 2022). When we started monitoring the benthic communities, we also began collecting data on temperature, salinity, and pH at each sampling site using a portable multiparameter sonde (HANNA) and dataloggers (HOBO®). However, we did not observe anomalies at any of the monitored sites (Supplementary Table S1), although some persisted at other locations.
Sampling commenced immediately upon receiving authorization from the authorities responsible for the emergency, and it took place in February, April-May, and July 2022, corresponding to 2, 4.5, and 7 months after the eruption had concluded, respectively. In each zone, for both the lava flows and control zone, we selected three sites for sampling in the subtidal area and three sites in the intertidal area (Figure 1). In the subtidal area, sampling was performed on rocky reefs, while in the intertidal area, sampling was performed on rocky platforms. In the intertidal area, we studied all the initially formed coastal platforms, whereas in the subtidal area, sampling locations were chosen randomly, without following any specific criteria, except for ensuring a minimum distance between sites. The locations in the control zone were previously known. Fishes, macroinvertebrates, and algae were studied in the subtidal area, whereas macroinvertebrates and algae were studied in the intertidal area. Subtidal samples were obtained by scuba diving at depths ranging from 5 to 20 m. Within this depth range, we used a stratified sampling approach with three levels: 5-10 meters, 10-15 meters, and 15-20 meters, to ensure an equal distribution of samples. Intertidal sampling was conducted during the periods of maximum low tide each month. Samples were equally distributed across three intertidal levels defined for desiccation tolerance (i.e., low, medium, or high) of the different organisms collected (Lewis, 1964). The lower level ranged from 0-0.4 m above sea level during low tide, the medium level from 0.4-0.8 m, and the upper level from 0.8-1.2 m, approximately, coinciding with the regional mean tidal range. This design aimed to encompass all the spatial variability within these areas, establishing a robust method for long-term monitoring.
To estimate fish abundance, we used an in-situ point-count method (Bortone et al., 1989), in which the observer takes a position at the centre of a circle (100 m2) and records the number (abundance) and approximate size of individuals of each species for 5 min. At each depth range, three visual censuses (nine per site), minimum 25 m apart, were performed. To estimate visually in-situ the macroinvertebrate abundance (number of individuals of each species), we used the belt transect method (10 × 2 m). The minimal body size of individuals sampled was about 0.5 cm. During sampling, we checked crevices and holes but did not turn any rocks over to search for species underneath. At each level, we laid out a 50-m long tape to measure on the rocky bottom and counted the macroinvertebrates within the following intervals: 0-10 m, 20-30 m, and 40-50 m along this distance, leaving a separation of 10 m between samples. Sponges were excluded from the analysis due to the difficulty in identification. Algal cover was estimated from photographs of 25 × 25 cm quadrats divided into 5 × 5 cm. High-resolution photographs were obtained with a Nikon D700 camera housed in a Subal D700 housing and equipped with two Subtronic strobes. At each depth level, photographs were taken every 5 m along the tape measure used to estimate the abundance of macroinvertebrates, with 10 photoquadrats per level and 30 photoquadrants per site. To confirm the identity of small taxa sampled in the field, samples were collected for later confirmation in the laboratory at species or genus level. CCA, cyanophytes, were not identified at species level; instead, they were grouped for later analyses.
Intertidal sampling was conducted during the low-tide periods. Macroinvertebrate abundance was estimated also visually using 10 m × 1 m transects. We also checked crevices and holes but did not turn any rocks over, in these areas, we were able to count some gastropods that were only a few millimeters long. Three transects were established at each level. Similarly, we used a tape measure, but owing to the small area of the intertidal platforms, the transects were separated horizontally by 5 m only.
Algal cover was estimated using quadrats (25 × 25 cm). Sampling was conducted 5 m apart on the tape measure used for invertebrate estimation. At each site, seven quadrats were tested per tidal level, with 21 quadrats per site. We focused solely on the emerged surfaces; pools were not present on any of the platforms studied. The percentage cover of each taxa was estimated in-situ from its perpendicular projection onto the substrate. The cover percentage by epiphytes and other small species was also estimated in the field but species identification was conducted in the laboratory afterwards using samples taken in the field. Similarly to subtidal, some species were not identified at a specific level in the intertidal area. Many of these taxa require molecular studies for definitive species delimitation. In both the intertidal and subtidal, the algae belonging to the Order Ectocarpales and the Superclass Bacillariophyceae.s.l. grew forming tangled masses where it was impossible to separate one taxon from another, Consequently, in the analyses, both have been considered together as ectocarpaleans/diatoms.
All taxa data records for each study zone and period are shown in Supplementary Material (Supplementary Tables S2–S6).
Data interpretation and analysis
Species density and total abundance
The first analysis involved studying the diversity and abundance of each group of organisms. For each of these groups, species density (number of species per sample) and total abundance (total number of individuals or total cover, defined as the sum of the abundance or of the cover of all species) were statistically analysed using univariate permutational analysis of variance (PERMANOVA) based on Euclidean distance (Anderson et al., 2008). We design a multi-time control-impact model of three-ways which ‘zone (with two levels: lava flows vs control) and ‘time (with three levels: two, four and a half, and seven months) were treated as fixed factors to test the main hypothesis of the study: to assess the spatio-temporal changes between an affected and unaffected zone by a volcanic eruption. ‘Site was treated as a random factor nested within the interaction ‘zone x time to incorporate the variability between sites independent of the sampling timing and tested impact. The significant terms in the full model were also examined. When the number of possible permutations was small, Monte Carlo p-values (n = 4999) were obtained instead by random sampling from the asymptotic permutation distribution (Anderson et al., 2008).
Community structure
Permutational multivariate analysis of variance (Anderson et al., 2008) was performed to examine the community structure of each group of organisms. Resemblance matrices were obtained using Bray-Curtis dissimilarity for the number of fishes and invertebrate individuals, as well as the cover rate of the algae dataset. A ‘dummy species assigned a value of 1 was added to the matrix of intertidal invertebrates in the two-month sampling because no species were recorded at this time. The Bonferroni correction was applied to multivariate permutational analysis of variance (PERMANOVA) to avoid possible Type I errors that may occur when testing multiple hypotheses. The design of the model coincided with that of a previous univariate analysis and pairwise comparisons, with the corresponding Monte Carlo p-values, were conducted to test the differences between the levels of the studied terms. Non-metric multidimensional scaling (nMDS) was employed to visually illustrate the changes in community structure, with a dissimilarity matrix generated using the Bray-Curtis index.
Additionally, the contribution of the six most abundant taxa to the total abundance of each community was graphically represented to assist in interpreting the spatiotemporal trends and differences. An a posteriori pairwise-comparison was used to examine the significant terms. Taxa that contributed the most to the differences (up to 90%) were identified using similarity percentages (SIMPER) (Clarke and Gorley, 2006). To compare the temporal trajectory of communities across different organism groups and represent them in the same two dimensional-graph, we calculated the similarity between samples from both zones for each group of organisms and the sampling period using the BrayCurtis index, first graph dimension. This index discriminates groups of samples primarily based on taxa abundance. In addition, we calculated the distance between the centroids of the samples from each zone using the PERMDISP test, a technique sensitive to changes in taxa composition similar to beta-diversity, which is represented in a second dimension of the graph. The theoretically predictive trajectory of succession would tend toward a reduction in the differences in the abundance of different species within the communities and a decrease in beta-diversity (Anderson and Robinson, 2003).
Most abundant taxa
The abundance of the most abundant taxa in each group was studied separately using univariate permutational analysis of variance following the same three-way analysis with an equal number of factors and levels as in the preceding analysis. These analyses were based on Euclidean distances, and pairwise comparisons were performed to compare the contrast levels of significant factors with the corresponding Monte Carlo p-values if they were necessary.
The stratified sampling aimed to capture all the variability in the intertidal and shallow subtidal, although depth and intertidal level were not utilized as factors of analysis. These factors will be incorporated in future works to better explain long-term colonization at a smaller scale.
Sums of squares type III were employed in all designs (Anderson et al., 2008). Most data were left untransformed, with only some fishes and algae data undergoing square root transformation to avoid possible effects of outlier. The software PRIMER-E® v.6 + PERMANOVA+ (Clarke and Gorley, 2006) was used to perform the data analyses.
Results
Species density and total abundance
The mean species per sample of subtidal invertebrates and intertidal algae in the lava flows was lower and significantly different from that in the control zone, but there has not been a significant increase in the number of species in these groups over time (Table 1; Figures 2C, D). Indeed, little more than half of the species present in the control zone colonised the lava flows. Further, the mean species density of fishes, intertidal invertebrates and subtidal algae was also lower in lava flows in the first two sampling periods (Figures 2A, B, E). After seven months, species density was similar in both zones at least for fishes and subtidal algae. However, the ‘zone × time interaction effects detected by statistical analysis for these groups showed significant differences between zones at 2 and 4.5 months for the a posteriori pairwise comparisons. Further, the a posteriori analysis indicated significant differences in fishes and subtidal algae within each zone over time (Table 1; Figures 2A, E). For all groups, the species shared between both areas are common and dominant in the undisturbed benthic communities of the island (Supplementary Tables S2–S6). Particularly in algae, species observed in lava flows, some of them dominant, are considered rare in typical communities.
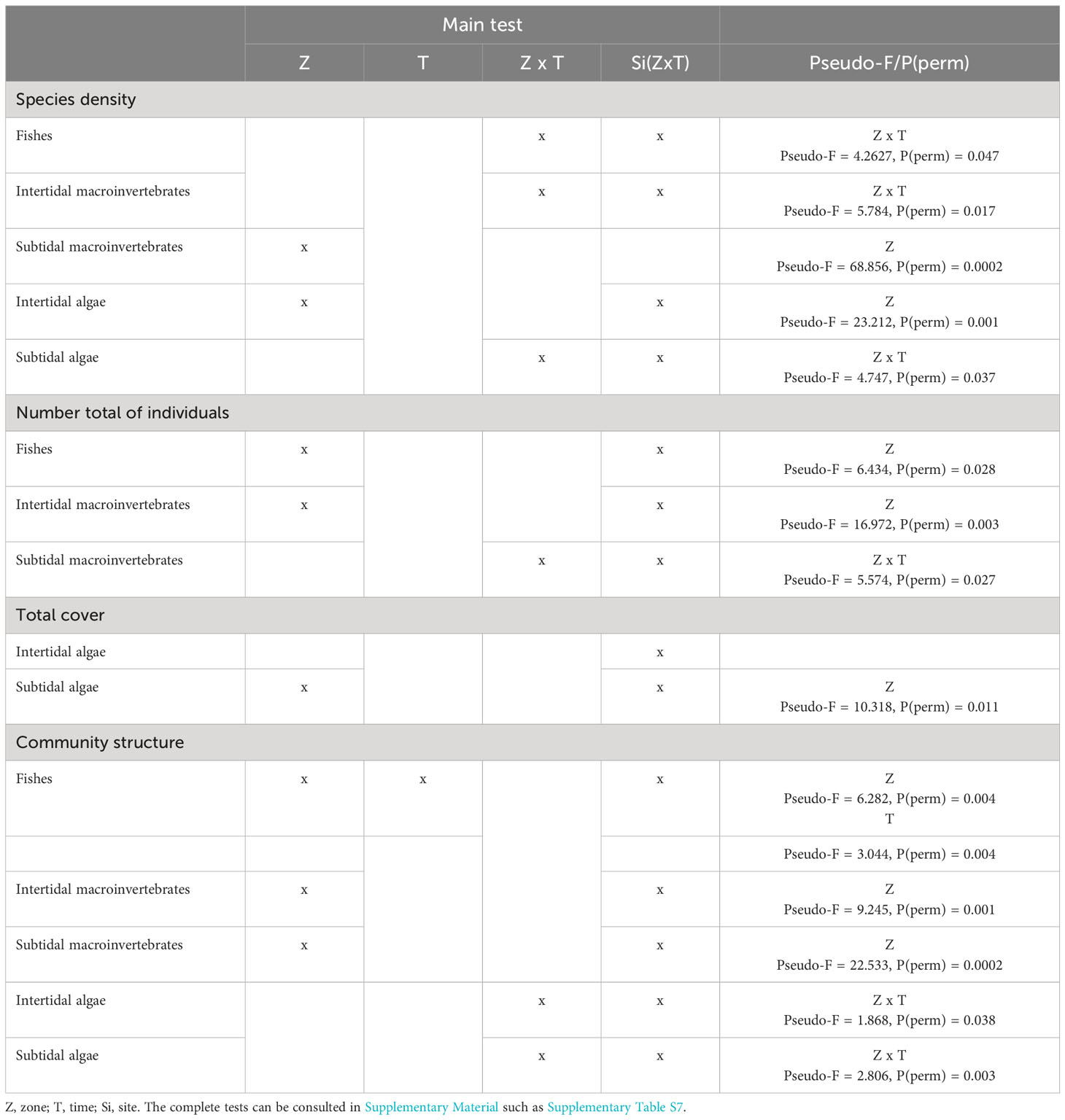
Table 1 Summary of results of distance-based permutational three-way PERMANOVA comparing spatio-temporal variation in species density, number total of individuals, total cover, and community structure using ‘zone (two levels: lava deltas, control zone), and ‘time (three levels: 2 months, 4.5 months, 7 months), as fixed factors and ‘sites according to the Figure 1 as a random factor nested in ‘zone × time interaction.
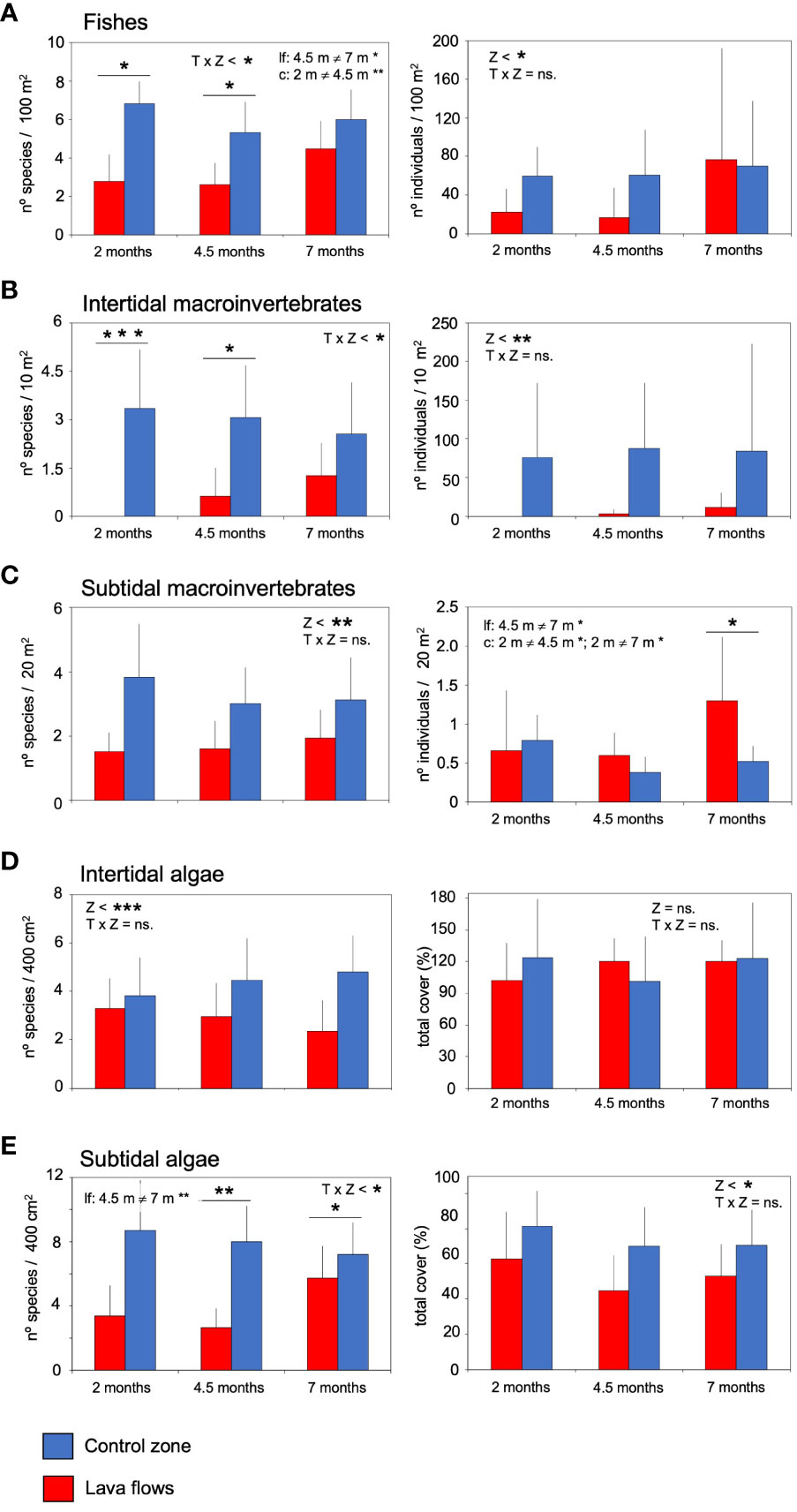
Figure 2 Species density (+sd) per group, and mean abundance number of individuals or total cover (+sd) per sample of fishes (A), intertidal macroinvertebrates (B), subtidal macroinvertebrates (C), intertidal algae (D) and subtidal algae (E) in the lava flows and control zone. The results of the univariate permutational analysis of variance (PERMANOVA), main test and post-hoc comparisons, have been included. The differences between zones for each sampled month are above the bars. Significant differences between months within each zone are compiled as text. Z, zone; T, Time; lf, lava flows; c, control zone, * = p< 0.05, ** = p< 0.01, *** = p< 0.001, ns, non-significant.
Total species abundance also showed different group-dependent temporal patterns. In the intertidal area of the lava flows, algae rapidly covered the rocky substrate, and no differences between zones were observed from the beginning of the monitoring (Table 1; Figure 2D). Fishes and subtidal algae abundance was significant different between zones. Subtidal algae showed lower total-abundance values in the lava flows in all periods, fishes showed lower values in the two first period and higher in the last sampling but the test did not find the interaction between time and zone factors (Figures 2A, E). The number of intertidal macroinvertebrates in the lava flow zone was much lower than that in the control zone. Similar to the trend observed in fish populations, there was an increasing in the number of individuals in the lava flows, but this was not supported by the analysis (Figure 2B). On the other hand, subtidal invertebrates constituted a different case altogether; i.e., the ‘zone × time interaction was significant, and, while the mean number of individuals was similar between zones at 2 and 4.5 months, it significantly differed at seven months, as shown by the a posteriori analysis, with the number of individuals in the lava flows more than doubling that in the control zone at the latter sampling time point (Table 1; Figure 2C).
Putting all the recognized species into context for each time and area, we observed that the number of species sampled in the first two samplings was similar but increased in the last sampling (Figure 3), and after 7 months, the total number of species recorded in the lava flows was 70, representing 64% of those found in the control zone. Overall, the number of species found in the lava flows for all groups was greater in the last sampling.
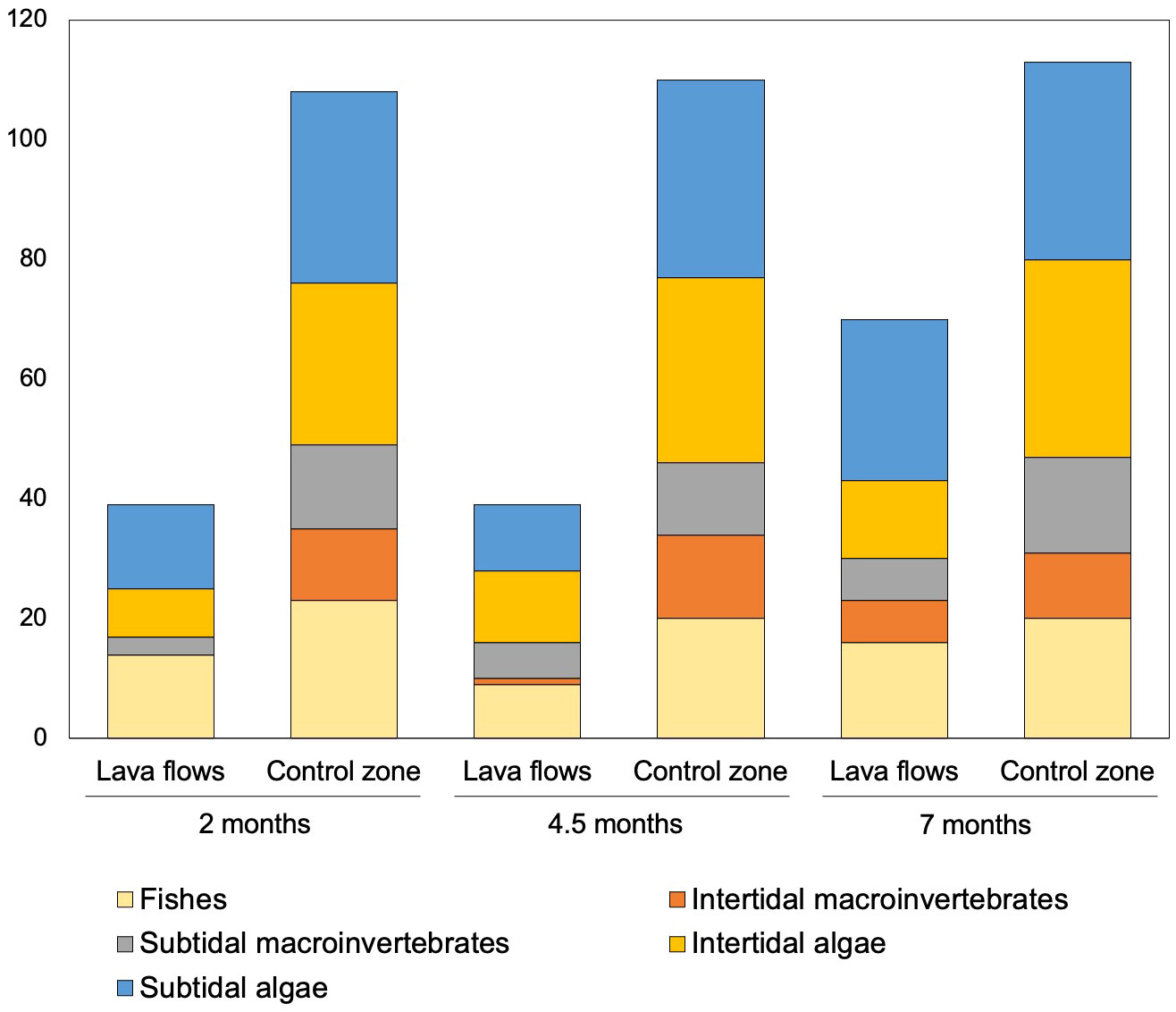
Figure 3 Cumulative graph of the total number of species for each group by area and sampling period.
Community structure
The analysis of the community structure found significant statistical differences between zones for all groups but following two different trend patterns. On one hand, fish and intertidal and subtidal invertebrate communities differed between lava flows and the control zone throughout the duration of the study, as shown by the statistical differences for the factor ‘zone (Table 1). Additionally, differences were found for fish in relation to the factor ‘time. On the other hand, intertidal and subtidal algae communities differed between zones but interacted with the factor ‘time. The a posteriori analysis for this interaction detected differences between lava flows and the control zone at all three sampling time points, as well as within lava flows, in the ‘time factor (Table 1). In most of the comparisons of the levels of the ‘T x Z terms, the level of significance obtained was lower than the Bonferroni-corrected significance level, supporting the robustness of the results (Supplementary Table S7). In other words, as supported by the results of the nMDS analyses (Figure 4), the intertidal and subtidal invertebrate samples within each zone (Figures 4B, C) were mixed and did not show significant differences over time. Alternatively, potential differences were not large enough to be considered significant. In the case of fish communities, the samples tended to be distinct across different times and zones whereas, intertidal and subtidal algal communities were distinct only in the lava flows (Figures 4A, D, E).
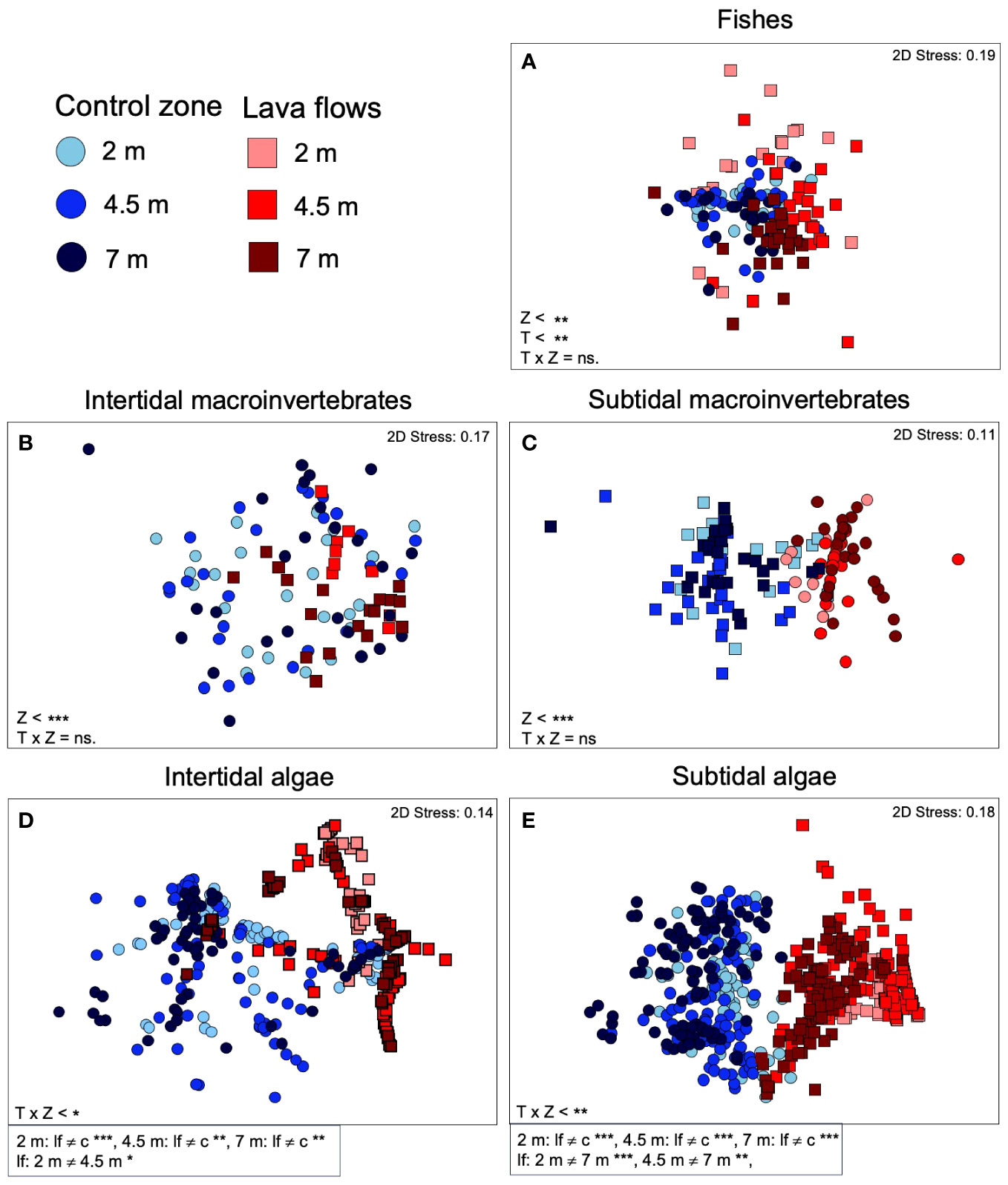
Figure 4 Nonmetric multidimensional scalings (nMDS) based on abundance (number of individuals of each species for fishes (A) and intertidal (B) and subtidal macroinvertebrate (C) communities, and cover for intertidal (D) and subtidal algal communities (E) data set at each studied zone. Squares, sampling sites at the lava flows, circles, sites at the control zone. The intensity of the color of the symbols corresponds to different sampled periods. The results of the multivariate permutational analysis of variance (PERMANOVA), the main test and post-hoc comparisons have been included. Z, zone; T, Time; lf, lava flows; c, control zone, * = p< 0.05, ** = p< 0.01, *** = p< 0.001, ns, non-significant.
Graphically, these distinctions between zones and temporal shifts were evident when the abundances of the six dominant taxa within each community were plotted (Figure 5). These six most abundant groups of taxa that contributed to total abundance as follows: fishes, 91%; intertidal invertebrates, 93%; subtidal invertebrates, 68%; intertidal algae, 81%; and subtidal algae, 65%. The SIMPER analyses (Table 2) enabled us to identify the main taxa contributing to these differences. In the case of fish communities, the predominant species remained consistent across both areas under study. However, all species exhibited a higher abundance towards the control area, except for Canthigaster capistrata. Some intertidal invertebrate species were not recorded in the lava flows even after seven months. Moreover, those sampled from lava flows were consistently more abundant and closely related to the control zone. With respect to subtidal invertebrates, we observed two distinct communities: one dominated by Percnon gibbesi and Antedon bifida in the lava flows, and the other dominated by Hermodice carunculata or Holoturia sanctorii in the control zone. As for algae, in both the intertidal and subtidal zones, differences between the zones were driven by distinct taxa compositions and abundances in the communities (Table 3). For instance, in the intertidal lava flows, ectocarpaleans/diatoms, Ulva spp., and Bangia atropurpurea were predominant, whereas Corallina berteroi, Jania virgata, and CCA were the most abundant taxa in the intertidal control zone. Similarly, in the subtidal lava flows, ectocarpaleans/diatoms dominated, and the CCA (notably after seven months) was significant. In contrast, Taonia atomaria, Lophocladia trichoclados, Canistrocarpus cervicornis, and CCA (the latter in the first two samples) were the most abundant taxa in the subtidal control zone. Temporal differences in the communities of lava flows were primarily attributed to changes in the abundance of taxa present throughout the study period.
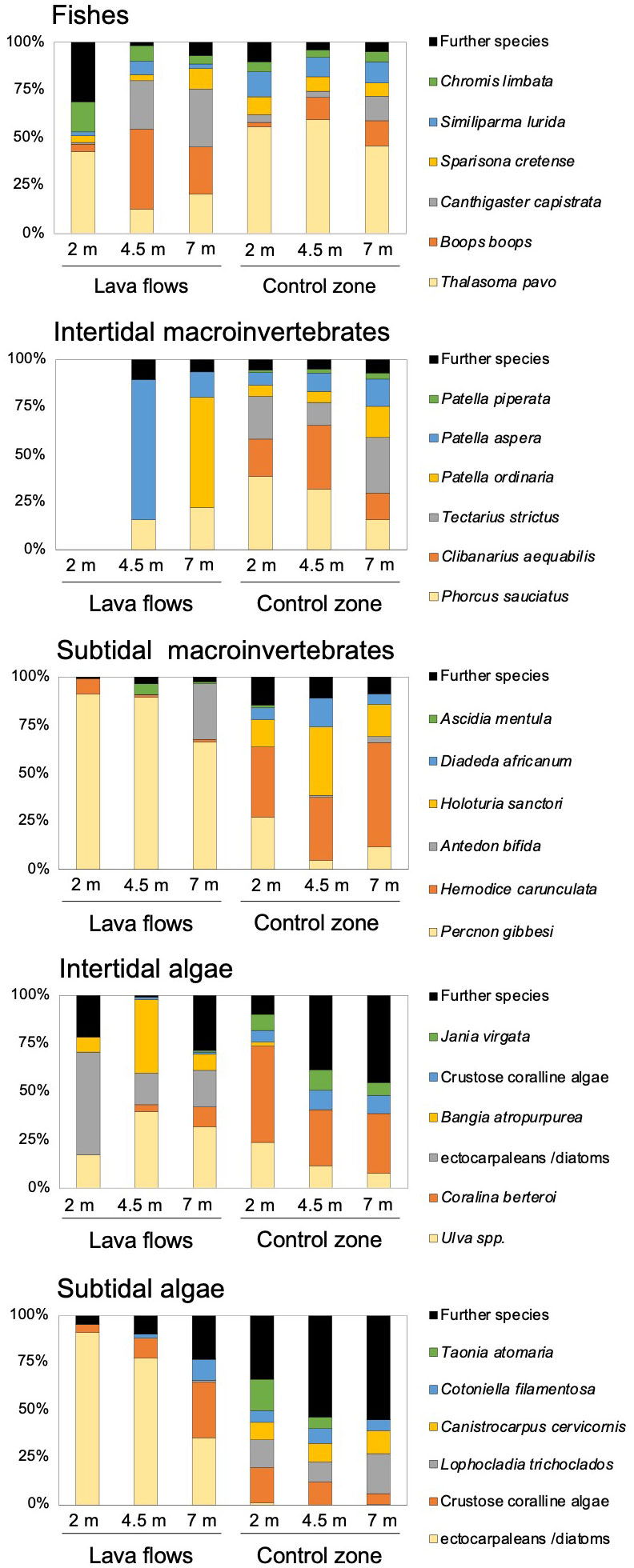
Figure 5 Relative contribution of the six most abundant taxa to total abundance in the studied communities (in number of individuals for fishes, intertidal invertebrates and subtidal invertebrates) and species cover (for intertidal algae and subtidal algae) at each studied zone and sampling period.
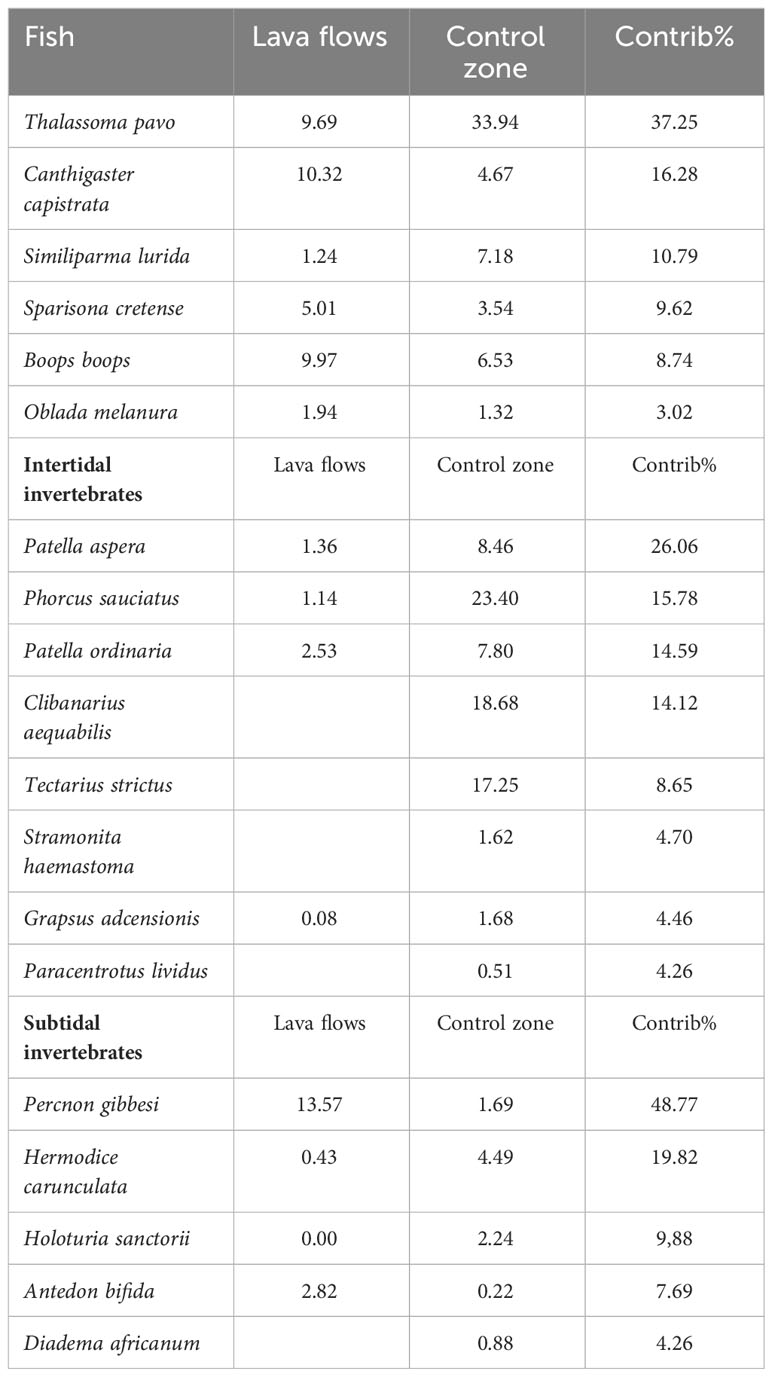
Table 2 Result of SIMPER analysis shows the species that contributed up 90% to the differences between levels of the ‘factor zone in the communities of fish, intertidal invertebrates, and subtidal invertebrates.
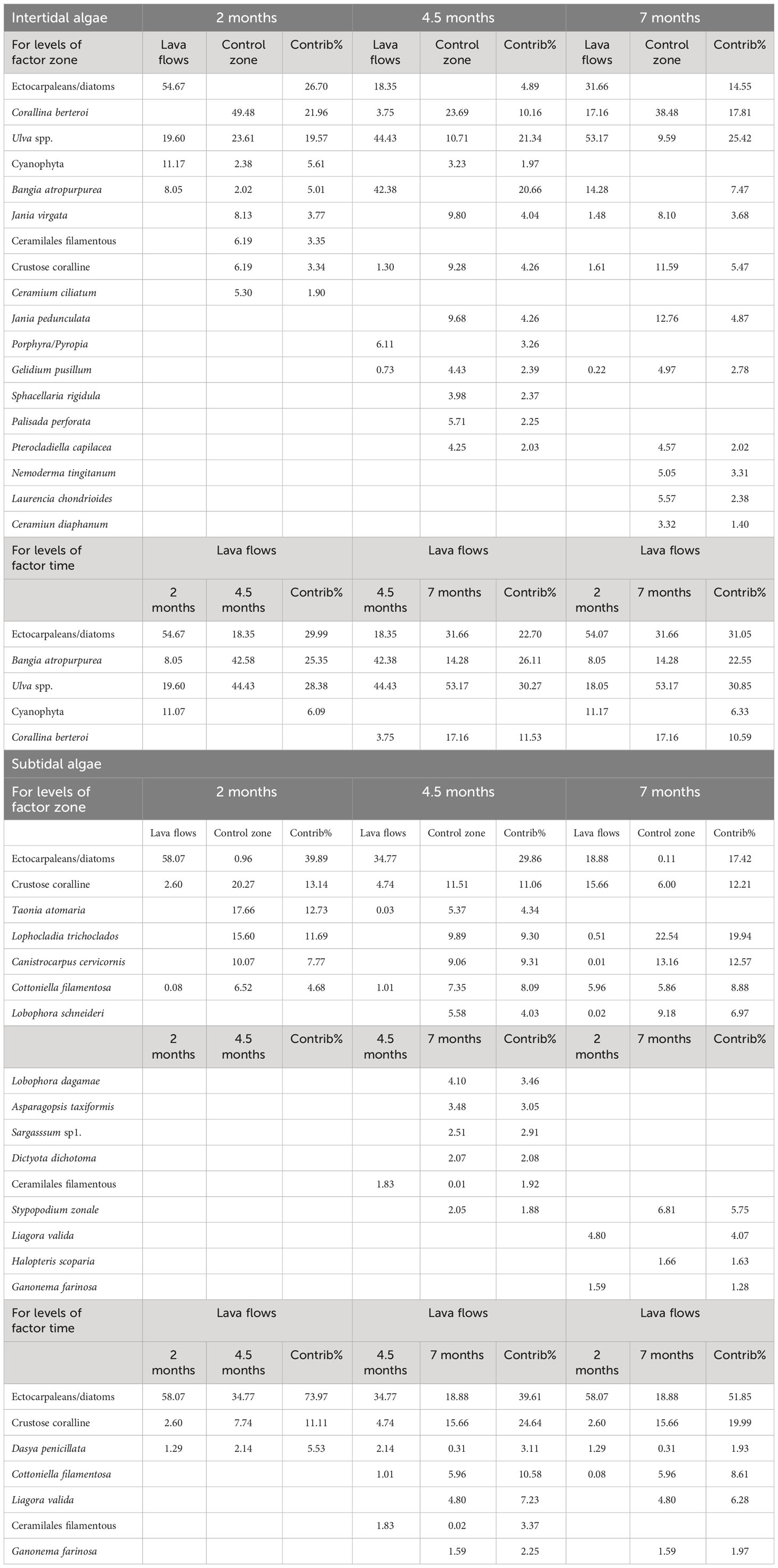
Table 3 Result of SIMPER analysis shows the species that contributed up 90% to the differences between significant levels of the interaction ‘zone x time in the communities of intertidal and subtidal algae.
All studied communities followed a similar temporal trajectory, and the differences between the lava flows and the control zone decreased over time. However, a regression was observed in invertebrate communities of the sublittoral area between months 2 and 4.5 (Figure 6). Further, the composition and structure of the communities in both zones became increasingly similar at each sampling time points, with the samples growing closer to each other over time. However, when comparing different communities of organisms, distinctions were observed from the establishment of the communities, which persisted for seven months. For example, the initial fish communities that colonised the lava flows closely resembled those in the control zone. This contrasted with the subtidal algae and invertebrate communities, in which case, the differences between zones after seven months were more pronounced than the initial differences between fish. Additionally, different rates of evolution were observed among the communities. Specifically, changes in algal communities were smaller than those in fish or intertidal invertebrates.
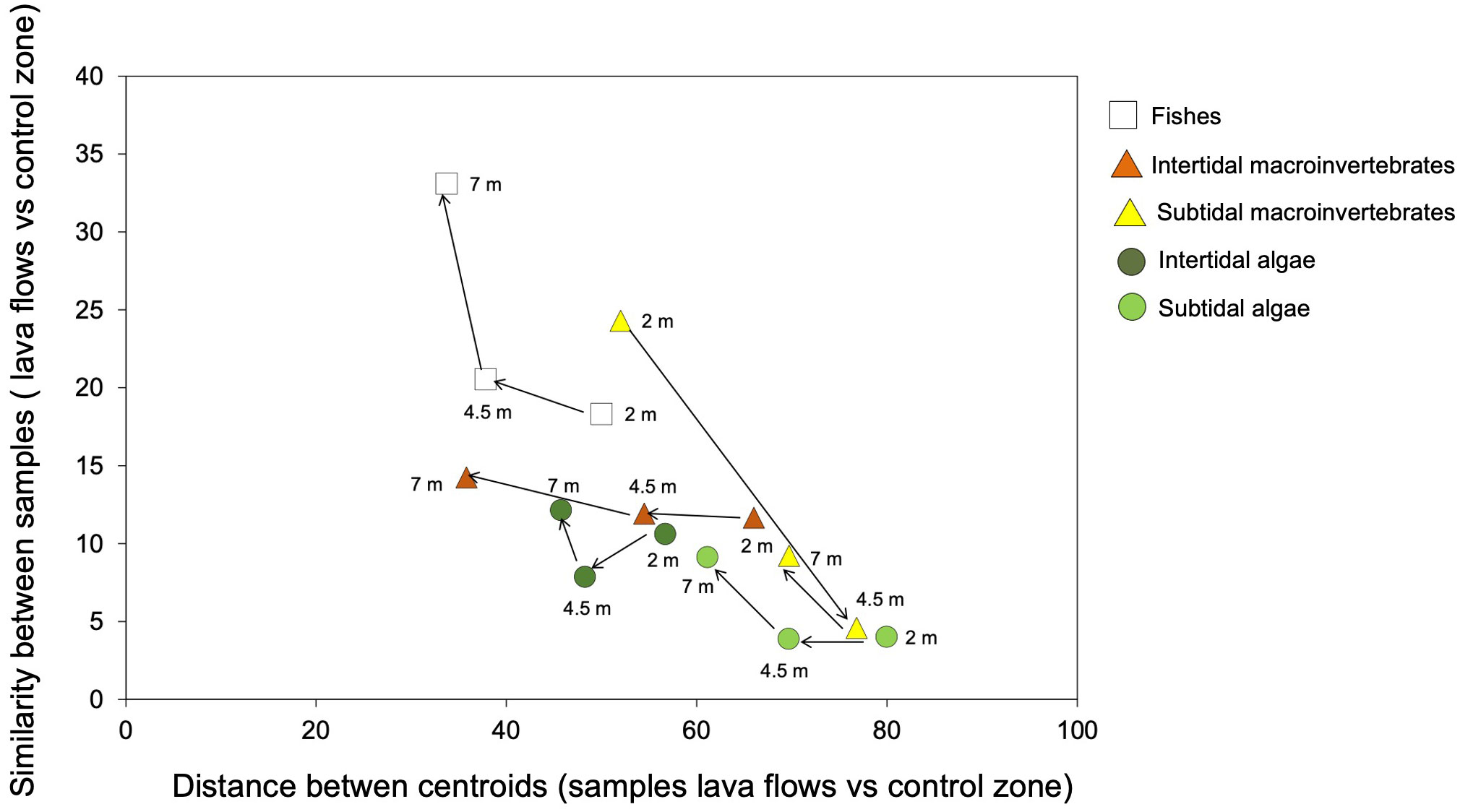
Figure 6 Bidimensional plot of the temporal trajectory of studied communities (fishes, intertidal invertebrate, subtidal invertebrate, intertidal algae, and subtidal algae). Y axis shows the similarity based on Bray-Curtis distances between samples of lava flows and control zone at each studied period. X axis shows the spatial distance based on centroid measures between samples of lava flows and control zone at each studied period.
Most abundant taxa
Species-specific responses of the main taxa revealed different colonisation patterns on lava flows, even within the same group of organisms (Table 4). Among fish species, Chromis limbata and Boops boops quickly colonised lava flows (Figures 7B, C), with no detectable differences, as early as within the first two months after the eruption. On the other hand, Thalassoma pavo and Similiparma lurida showed significant differences between zones, with populations of these species being higher in the control zone throughout the entire study (Figures 7A, E). As for Canthigaster capistrata and Sparisoma cretense populations, an interaction was observed between the ‘zone and ‘time factors. Thus, populations of C. capistrata were similar at two and four and a half months in both zones but significantly higher in lava flows after seven months. Meanwhile, populations of S. cretense were significantly higher at two and four and a half months in the control zone but were higher and statistically different in the lava flows at seven months (Figures 7C, D).
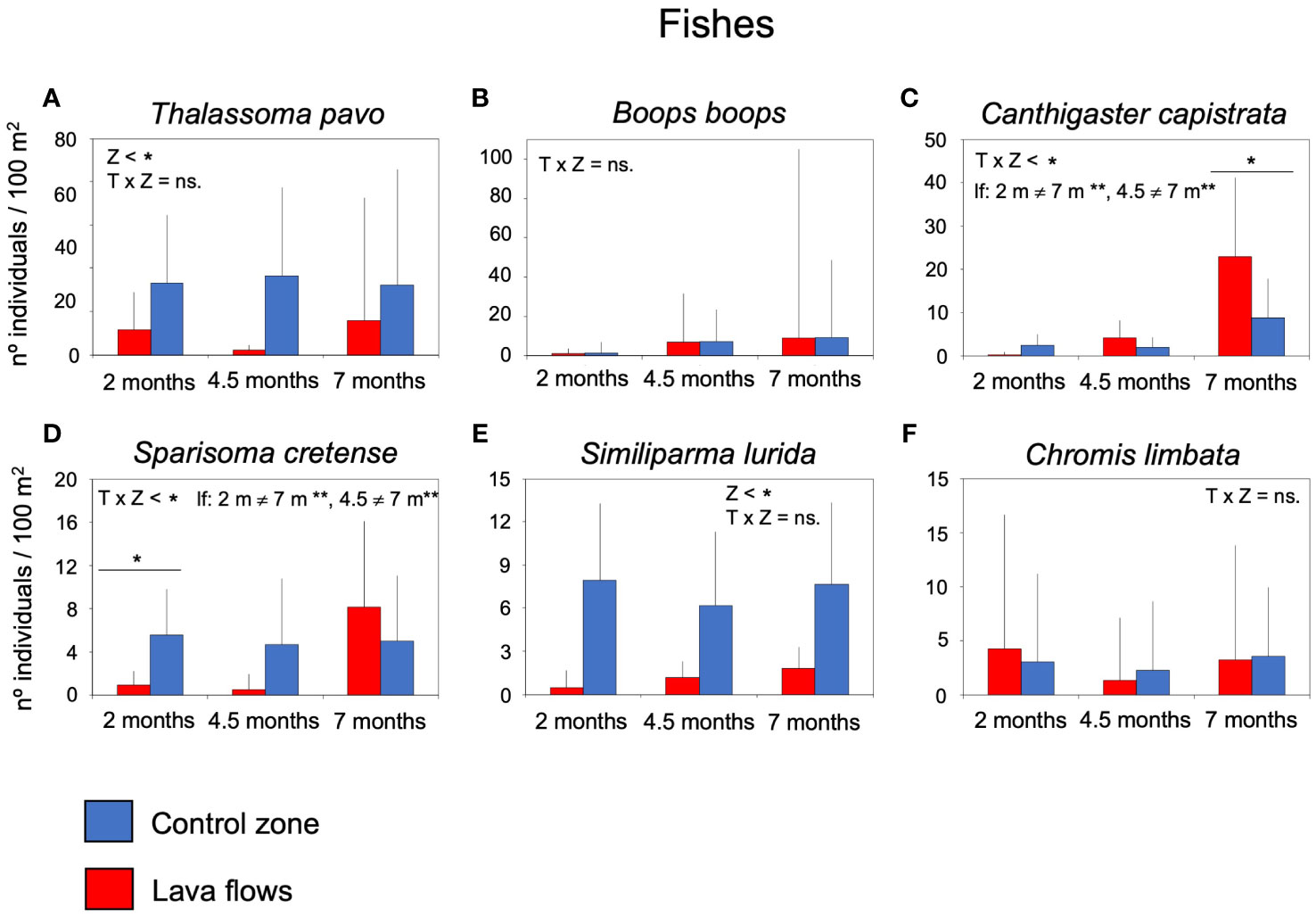
Figure 7 Mean number of individuals (+sd) of the most abundant species of fishes per sample in the lava flows and control zone (A) Thalassoma pavo, (B) Boops boops, (C) Canthigaster capistrata, (D) Sparisoma cretense, (E) Similiparma lurida, and (F) Chromis limbata. The results of the univariate permutational analysis of variance (PERMANOVA), the main test and post-hoc comparisons, have been included. The differences between zones for each sampled month are above the bars. Significant differences between months within each zone are compiled as text. Z, zone; T, Time; lf, lava flows; c, control zone, * = p< 0.05, ** = p< 0.01, n =, non-significant.
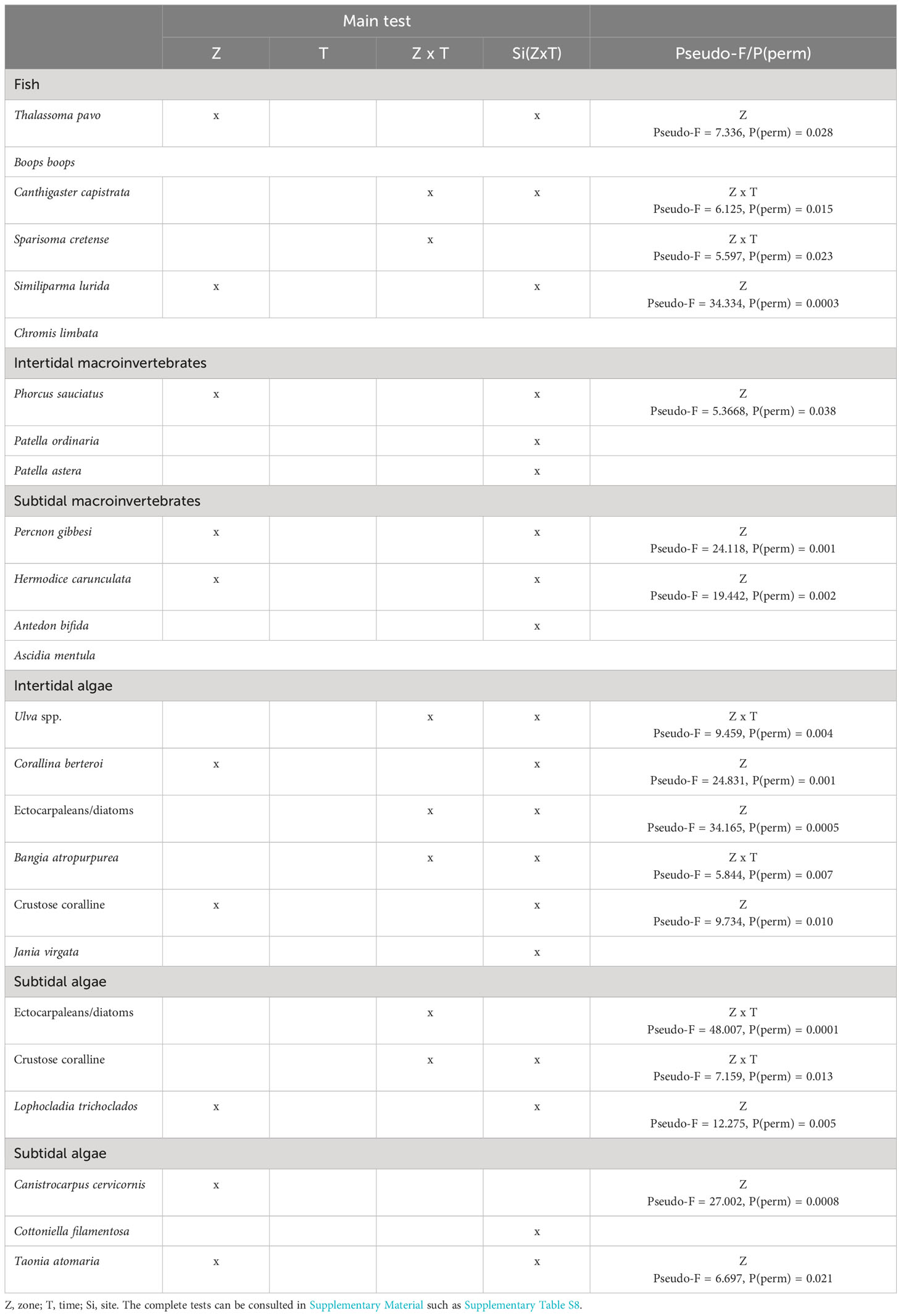
Table 4 Summary of results of distance-based permutational three-way PERMANOVA comparing spatio-temporal variation in the abundance of the main species of fish, intertidal macroinvertebrates, subtidal macroinvertebrates, intertidal algae, subtidal algae using ‘zone (two levels: lava deltas, control zone), and ‘time (three levels: 2 months, 4.5 months, 7 months), as fixed factors and ‘sites according to the Figure 1 as a random factor nested in ‘zone × time interaction.
In the intertidal area of the lava flows, some characteristic species of these habitats, such as the hermit crab Clibanarius aequabilis, and gastropods Tectarius strictus and Patella piperata, were not recorded during the first seven months (Figures 8B, C, F). However, other gastropods were present, such as Phorcus sauciatus, Patella ordinaria, and P. aspera. Populations of P. sauciatus were significantly lower in lava flows and incipient to observe any clear trends over time, and although P. ordinaria and P. aspera were less abundant in the lava flows, no significant differences were detected in these species (Table 4; Figures 8A, D, E). A similar pattern emerged for certain sublittoral macroinvertebrates and echinoderms, such as Holoturia sanctori and Diadema africanum, which were common in the control zone but were not observed in the lava flows (Figures 8J, K). However, unlike in the intertidal area, populations of some species, such as Percnon gibbesi, Antedon bifida and Ascidia mentula were more abundant in the lava flows, although for A. bifida and A. mentula no significant differences were found between areas (Table 4; Figures 8G, I, L). The proliferation of P. gibbesi was extraordinary, presenting a high density of individuals in the lava flows from the beginning of monitoring. Also notably, despite being one of the most abundant species in the lava flows, Hermodice carunculata showed significantly more numerous in the control zone through the time (Table 4; Figure 8H).
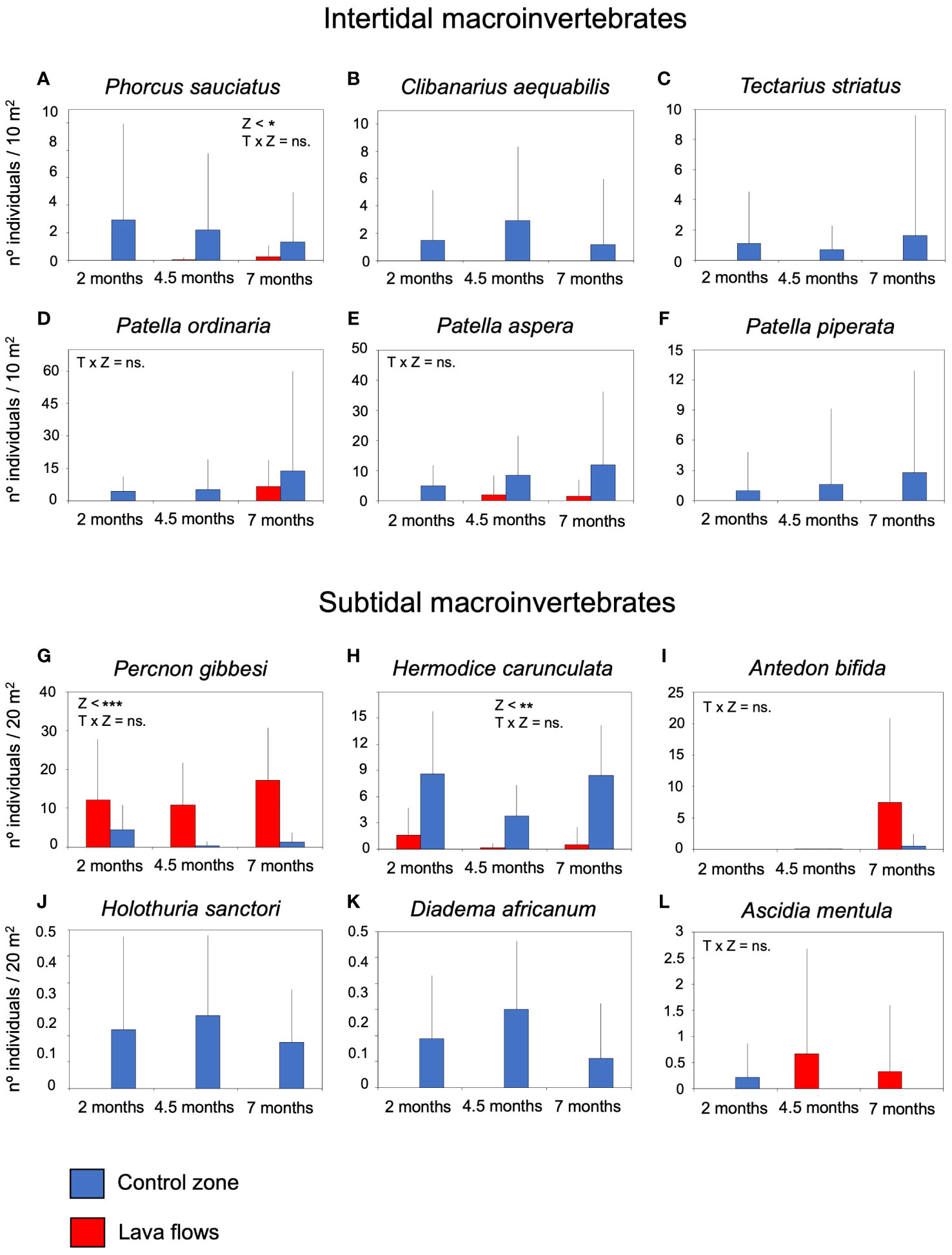
Figure 8 Mean number of individuals (+sd) of the most abundant species of intertidal and subtidal macroinvertebrates per sample in the lava flows and control zone, (A) Phorcus sauciatus, (B) Clibanarius equabilis, (C) Tectarius strictus, (D) Patella ordinaria, (E) Patella aspera, (F) Patella piperata, (G) Percnon gibbesi, (H) Hermodice carunculata, (I) Antedon bifida, (J) Holoturia sanctori, (K) Diadema africanum, (L) Ascidia mentula. The results of the univariate permutational analysis of variance (PERMANOVA), the main test and post-hoc comparisons, have been included. The differences between zones for each sampled month are above the bars. Significant differences between months within each zone are compiled as text. Z, zone; T, Time; lf, lava flows; c, control zone, * = p< 0.05, ** = p< 0.01, *** = p< 0.001, ns = non-significant.
Moreover, perennial algal species, such as Corallina berteroi and CCA were more abundant in the control zone. They colonised the flows slowly from two months, but their cover was significantly lower, and without very large changes between samplings to cause the interaction between the time and area factors. Jania virgata was also more abundant in the control zone. Although it was only detected in the third sampling timing, no significant differences were found (Table 4; Figures 9B, E, F). In contrast, fast-growing algae such as Ulva spp., ectocarpaleans/diatoms, and Bangia atropurpurea dominated lava flows. These taxa also showed significant temporal variations in the lava flows (Table 4; Figures 9A, C, D). Meanwhile, in the subtidal zone, algal species such as Lophocladia trichoclados, Canistrocarpus cervicornis, and Taonia atomaria established themselves in the lava flows from the beginning, but their cover was significantly lower than that of the control zone without proliferating excessively during the study (Table 4; Figures 9I, J, L). Conversely, Cotoniella filamentosa proliferated quickly and, although its cover in the flow zone was less than that in the control zone, the difference was not significant (Table 4; Figure 9K). Ectocarpaleans/diatoms were the most abundant taxa in the lava flows with limited cover in the control zone. However, these algae showed a significant decrease in cover over time under the lava flows. In contrast, CCA increased their cover in the lava flows reaching higher values by the seventh month, but were always less abundant than in the control zone (Table 4; Figures 9G, H).
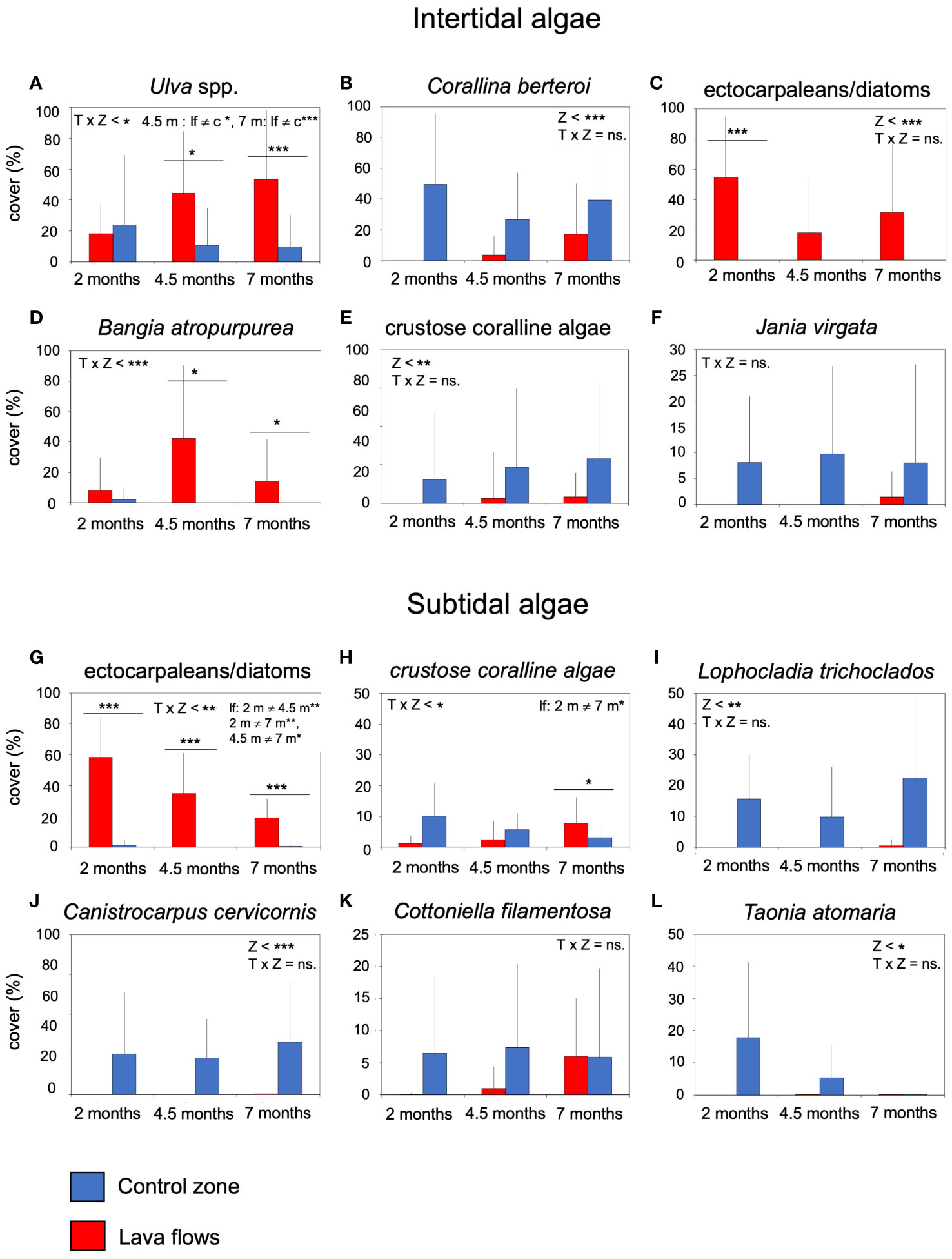
Figure 9 Mean cover (+sd) of the most abundant taxa of intertidal and subtidal algae per sample in the lava flows and control zone, (A) Ulva spp., (B) Corallina berteroi (C) ectocarpaleans/diatoms, (D) Bangia atropurpurea, (E) crustose coraline algae, (F) Jania virgata, (G) ectocarpaleans/diatoms, (H) crustose coralline algae, (I) Lophocladia trichoclados, (J) Canistrocarpus cervicornis, (K) Cottoniella filamentosa, (L) Taonia atomaria. The results of the univariate permutational analysis of variance (PERMANOVA), the main test and post-hoc comparisons, have been included. The differences between zones for each sampled month are above the bars. Significant differences between months within each zone are compiled as text. Z, zone; T, Time; lf, lava flows; c, control zone, * = p< 0.05, ** = p< 0.01, *** = p< 0.001, ns = non-significant.
Discussion
Colonisation of the lava flows of the Tajogaite volcano has been swift, and many species have arrived and proliferated in the zone soon after the eruption of the volcano. Biological assembly tended to become more complex over time owing to the progressive incorporation of new species. All communities followed a predictable succession (Connell and Slatyer, 1977), and tended to resemble the communities in the control zone over time. Nevertheless, communities differed in the speed at which they changed. After seven months, the differences between the lava flows and the control zone were small for some groups, but still significant for others. As a result, and according to each community of organisms, the benthic ecosystem of the lava flows was found at different stages of restoration.
Patterns in species density and total abundance
The capacity of a species for dispersal is a key factor governing the connectivity between zones and the initial stages of primary succession (Cowen and Sponaugle, 2009). There are various dispersal strategies in the marine environment (Cowen et al., 2007) and, depending on their effectiveness, certain species establish themselves before others. In lava flows, species arrived through different mechanisms, including active displacement of adults or juveniles or passive dispersion aided by currents and waves via larval, germinative, or vegetative propagules.
Fishes most rapidly reached and established new populations in the lava flows, as observed in similar lava flow environments (Godwin and Kosaki, 1989). With greater mobility, adult fishes have the capacity to quickly colonize new territories (Leitao et al., 2008). Fishes encountered minimal spatial or physical barriers such as sandbanks or great depths in the study area. They were able to migrate from nearby areas without losing contact with the rocky bottoms. Furthermore, the areas surrounding the lava flows served as the primary source of species because they did not experience significant impacts. Even populations of sessile organisms survived only a few meters from the advancing incandescent lava. After seven months, the species density of fish communities in the lava flows resembled that of the control zone, although the number of individuals in the lava flows was still lower. From a trophic perspective, it seems that there was no limitation for the fishes, despite the coexistence of different feeding strategies. The lava flows were not an inhospitable habitat; some species are planktivores, others are herbivores or omnivores that could benefit from the presence of highly palatable primocolonizing algae, and for the microcarnivorous species, there was also a high number of preys, such as Percnon gibbesi. The recovery of the fish community was also positively influenced by the establishment of a marine exclusion zone surrounding the lava flows where no activities, including fishing, were permitted. For the remaining organisms, species density was significantly lower after seven months. Only subtidal macroalgae showed a similar number in the lava flows to that in the control zone. With a few exceptions, such as the macroinvertebrates Octopus vulgaris, Sepia officinalis, and Cronius ruber (of which we observed some adults), all species colonised lava flows via larvae or propagules, which slowed the colonisation process. The incorporation of individuals through larvae or propagules depends on the characteristics of the life cycle of the species. The life cycle plays a fundamental role in determining new individuals integrate the populations (Levin, 1984; Coma et al., 2000), as well as their availability at specific times. Depending on the foundational events, the trajectories of community assemblages can take different directions (Underwood and Chapman, 2006). In this context, it is important to note that many dominant invertebrate species may not have been in their reproductive period immediately after the formation of lava flows. As it has been extensively documented, the abundance of larvae from benthic invertebrate can vary significantly through the year (Wong et al., 2022; Menendez, 2023). In this study, we observed distinct patterns in the number of species and population sizes in intertidal and subtidal habitats. Specifically, in the subtidal area, many species settled in lava flows from the beginning, soon after the eruption of the volcano. Conversely, in the intertidal area, both the number of species and their population sizes were significantly reduced and were notably lower than those in the control zone. Apparently, there were no larval limitations for species colonising subtidal habitats; for instance, Percnon gibbesi larvae are present in canary waters year-round (Landeira and Lozano-Soldevilla, 2018). However, it seems that this limitation existed for intertidal species. Intertidal invertebrate communities on La Palma Island are primarily dominated by limpets (Patella spp.) and marine snails (Phorcus sauciatus). Both species exhibited multiple breeding periods throughout the year, but gamete production occurred mainly in the summer. Furthermore, veliger larvae settle rapidly after a few days (Sousa et al., 2018). Consequently, their settlements are concentrated in summer and early autumn, which did not coincide with the formation of lava flows.
Although only a few species arrived at the lava flows during the first few months, algae proliferated exponentially within a short time. Many taxa colonizing the lava flows were ephemeral species capable of producing new generations throughout the year, both through sexual and asexual reproduction, such as the ectocarpaleans. In both the intertidal and subtidal habitats, they exhibited high cover values for the lava flows, similar to those in the control zone from the beginning. The early stages of primary succession are also characterised by low interactions between organisms, and the exclusion of herbivores can alter the trajectory of algal communities (Mayakun et al., 2020; Srednick et al., 2023). The limited presence of herbivore populations in lava flows, particularly in the intertidal zone, undoubtedly contributed to the abundance of algae (Williams et al., 2000). In subtidal habitats, the number of algal taxa increased at a faster rate. It is worth noting that habitat interactions also play a role in the primary succession of algae, as previously demonstrated (Gulliksen et al., 1980). The interaction with the habitat can be as important, or even more important, than the development and feeding modes of the species (Defeo and McLachlan, 2011). Although this aspect was not observed due to the limited time duration of the study, in subsequent samplings, we observed how the remodeling of lava flows by waves influences the longer-term trajectory of benthic communities in lava flows.
Community structure response
The disparities in colonisation became more pronounced when we examined the specific composition of each community. The fish species that dominated the lava flows during this period were essentially identical to those that dominated the control zone. The differences between the zones stemmed primarily from the varying abundances of the dominant species in each area. Species that were sparsely abundant in the control zone and not yet present in the lava flows had minimal influence on the statistical analysis. This contrasts with the previously described primary succession in similar studies, where either early in succession (a few months) (Godwin and Kosaki, 1989) or a few years later (Pinault et al., 2013), the fish community in lava flows differs from that in other areas in terms of specific composition. Although these examples pertain to coral reef communities, where fish species are strongly linked to other benthic organisms, such as corals, and their proliferation depends on prior colonization. Studies on the colonisation of new volcanic habitats by invertebrates have also noted that the early stages of succession are dominated by a few pioneer species that are later replaced (Gulliksen et al., 2004; Bollard et al., 2013). However, in our study of invertebrates, a similar pattern emerged as with fishes and lava flows, and the control zone shared many dominant species. Generally, these species were more abundant in the control zone, although some species such as Percnon gibbesi and Antedon bifida proliferated successfully in the lava flows. Again, the weak interactions between species in these early stages, particularly in terms of carnivory, may explain the proliferation of certain populations (McCook and Chapman, 1993).
While changes in the communities of fishes and invertebrates were driven solely by a progressive increase in the number of species and their population size, which resulted in primary succession without species turnover (Mori et al., 2008), this was not the case for algae. In both the intertidal and subtidal communities of the lava flows, the transformations were more profound and involved shifts in specific composition (Supplementary Figure S1), aligning with typical seaweed succession on new substrates (Serisawa et al., 1998; Jung and Choi, 2022). Further, as observed in previous studies on lava flows (Doty, 1967; Jonsson, 1968; Zubia et al., 2018), initially, a community partially dominated by primary colonising species proliferated, but many of these taxa were gradually replaced by the dominant species in the climax communities. While the structure of the algal communities was similar to that of the other animal communities, eventually converging towards the communities in the control zone, following a similar trajectory, the greatest changes in the algal communities resulted in a delay in their evolution compared to the other communities.
Specific response
By focusing on the abundance of the main species, which collectively represented over 90% of the total abundance in all groups, we successfully revealed additional events that occurred in this primary succession, as well as specific responses within each studied group. Specifically, with respect to fishes, species such as Boops boops and Chromis lumbata have few relationships with other benthic organisms, because they are bentho-pelagic and plankton-feeding species (El-Maremie and El-Mor, 2015; Anderson et al., 2017), and have established themselves in the lava flows with an abundance comparable to that in the control zone. On the other hand, Thalassoma pavo and Similiparma lurida, slowly and progressively colonised lava flows, primarily through adult migration from densely populated areas. Conversely, species such as Canthigaster capistrata and Sparisoma cretense proliferated in the lava flows through juvenile settlements, with most size records in month seven belonging to small individuals. This massive colonisation by juvenile fishes is a common phenomenon in lava flows (Godwin and Kosaki, 1989), and is likely attributable to low predation, as piscivorous fishes play a key role in primary succession processes by controlling recruitment populations (Herrera et al., 2002). Consistently, Aulostomus spp. are the primary piscivores in tropical and subtropical ecosystems (Aronson, 1983), including the Canary Islands. Indeed, we observed that the population density of Aulostomus strigosus was 20 times lower in lava flows than in the control zone.
Low predation pressure on certain subtidal invertebrate species may also have contributed to their initial success. Percnon gibbesi, a fast-growing invertebrate whose populations were observed early, serves as a common prey for many demersal fishes (Guerra-Marrero et al., 2023). Facilitative interactions, a mechanism of ecological succession (Connell and Slatyer, 1977; Bruno et al., 2003), suggests that the arrival of a species depends on the arrival of a preceding species. In this context, the absence of such interactions between organisms can slow down changes in communities. Although mollusk populations in the intertidal area were not very large, it is worth noting that they developed in the absence of their main predator, gastropod Stramonita haemastoma (Watanabe and Young, 2006). Without the prior mortality of Phorcus sauciatus, the hermit crab Clibanarius aequabilis, which is abundant in the control zone, has no opportunity for shell-selection behaviour, even with the large availability of this crab larvae in the surrounding waters.
As previously demonstrated, algae on lava flows followed different dynamics. Fast-growing species with a single-thallus morphology have competitive advantages in the early stages of succession (Sousa, 1979; Littler and Littler, 1980). These ephemeral species, including Ulva spp., Bangia atropurpurea, ectocarpaleans, and diatoms, not only grow rapidly, but invest significantly in reproduction as well. A substantial portion of their thalli, if not the entirety, can serve as propagative cells, enabling their populations to grow exponentially (De Wreede and Klinger, 1988; Santelices, 1990). In the intertidal area, Corallina berteroi and Jania virgata, as well as CCA in both intertidal and subtidal areas, have established themselves as the main perennial taxa in lava flows. Although they exhibit comparatively slower growth, they are highly competitive in primary succession (Mariath et al., 2013) and have begun to displace ephemeral species in specific environments, such as the lower intertidal and upper subtidal zones. The interplay between low competitiveness and high demographic dynamics is a common feature of all primary successions (Huston and Smith, 1987; McCook, 1994). This allowed for the proliferation of not only certain species, but also many other taxa that are uncommon in mature communities. In fact, 46 taxa (25% of those recorded in the study), primarily algae, displayed higher abundances or were exclusively detected in lava flows.
Conclusions
The eruption of the Tajogaite volcano gave rise to a new marine ecosystem, serving as an ideal natural laboratory for the study of ecological processes that are often challenging to replicate in controlled laboratory settings. Thus, we had the unique opportunity to study primary succession as early as two months after the conclusion of volcanic activity. Different organisms exhibited varying rates of colonisation of the resulting lava flows. The mobility of fishes confers an important advantage, enabling them to establish themselves quickly and progress towards climatic communities. In contrast, organisms with limited or no mobility in their adult state experience delays in colonisation. Notably, we observed a turnover of species within the algae during succession, whereas the fishes and macroinvertebrates that initially colonised the lava flows were largely the same as those living in the control zone. Our findings also describe the settlement and atypical proliferation of certain species, possibly due to scarce interaction and competition between organisms.
Data availability statement
The original contributions presented in the study are included in the article/Supplementary Material, further inquiries can be directed to the corresponding author.
Ethics statement
Ethical approval was not required for the studies on animals in accordance with the local legislation and institutional requirements because the study was observational only.
Author contributions
CS: Conceptualization, Data curation, Investigation, Writing – original draft. DA-C: Data curation, Investigation, Writing – original draft, Writing – review & editing. JRe: Investigation, Writing – original draft. JRo: Investigation, Writing – original draft. MS: Funding acquisition, Investigation, Writing – original draft, Writing – review & editing.
Funding
The author(s) declare financial support was received for the research, authorship, and/or publication of this article. This research was conducted as part of the project “Monitorization of the volcanic eruption in la Palma and its effects on the population and territory” of University of La Laguna. It was funded by the Ministerio de Ciencia e Innovacion del Gobierno de Espan˜a, and Naturgrad proyect (PID2021-124538NA-100) which also funded by the Ministerio de Ciencia e Innovacion (Agencia Estatal de Investigacion). The Cabildo de La Palma, through the program of collaboration with University of La Laguna, Campus Oceano, provide funding for our fieldwork on the island.
Acknowledgments
The PLOCAN (Plataforma Oceanica de Canarias) provided invaluable support during our fieldwork, providing both vessel and land facilities within the framework of MAMPALMA project financed by the Ministry of Science and Innovation. A special thanks goes to the PLOCAN staff Gabriel Juanes and Mariona Casamayor for their assistance.
Conflict of interest
The authors declare that the research was conducted in the absence of any commercial or financial relationships that could be construed as a potential conflict of interest.
Publisher’s note
All claims expressed in this article are solely those of the authors and do not necessarily represent those of their affiliated organizations, or those of the publisher, the editors and the reviewers. Any product that may be evaluated in this article, or claim that may be made by its manufacturer, is not guaranteed or endorsed by the publisher.
Supplementary material
The Supplementary Material for this article can be found online at: https://www.frontiersin.org/articles/10.3389/fmars.2024.1337894/full#supplementary-material
References
Anderson A. B., Salas E. M., Rocha L. A., Floeter S. R. (2017). The recent colonization of south Brazil by the Azores chromis Chromis limbata. J. Fish. Biol. 91, 558–573. doi: 10.1111/jfb.13363
Anderson M. J., Gorley R. N., Clark K. R. (2008). Permanova+ for Primer: Guide to Software and Statistical Methods (United Kingdom: Primer-E Ltd).
Anderson M. J., Robinson J. (2003). Generalized discriminative analysis based on distances. Aust. J. Stat. 45, 301–318. doi: 10.1111/1467-842X.00285
Antoniadou C., Voultsiadou E., Chintiroglou C. (2010). Benthic colonization and succession on temperate sublittoral rocky cliffs. J. Exp. Mar. Ecol. 382, 145–153. doi: 10.1016/j.jembe.2009.11.004
Aronson R. B. (1983). Foraging behavior of the west Atlantic trumpetfish, Aulostomus maculatus: use of large, herbivorous reef fishes as camouflage. Bull. Mar. Sci. 33, 166–171.
Bollard S., Pinault M., Quod J. P., Boissin E., Hemery L., Conand C. (2013). Biodiversity of echinoderms on underwater lava flows with different ages, from the Piton de la Fournaise (Reunion Island, Indian Ocean). Cah. Biol. Mar. 54, 491–497.
Bortone S. A., Kimmel J. J., Bundrick C. M. (1989). A comparison of three methods for visual assessing reefs fish communities: time and area compensated. Northeast Gulf Sci. 10, 85–96. doi: 10.18785/negs.1002.02
Bruno J. F., Stachowicz J. J., Bertness M. D. (2003). Inclusion of facilitation into ecological theory. Trends Ecol. Evol. 18, 119–125. doi: 10.1016/S0169-5347(02)00045-9
Carracedo J. C., Badiola E. R., Guillou H., de la Nuez J., Perez Torrado F. J. (2001). Geology and volcanology of la palma and el hierro, western canaries. Estud. Geol. 57, 175–273. doi: 10.3989/egeol.01575-6
Carracedo J. C., Troll V. R., Day J. M. D., Geiger H., Aulinas M., Albert H., et al. (2022). The 2021 eruption of the Cumbre Vieja volcanic ridge on La Palma, Canary Islands. Geol. Today 38, 94–107. doi: 10.1111/gto.12388
Civico R., Ricci T., Scarlato P., Taddeucci J. P., Andronico D., Del Bello E., et al. (2022). High-resolution Digital Surface Model of the 2021 eruption deposit of Cumbre Vieja volcano, La Palma, Spain. Sci. Data 9, 435. doi: 10.1038/s41597-022-01551-8
Coma R., Ribes M., Gili J. M., Zabala M. (2000). Seasonality in coastal benthic ecosystems. Trends Ecol. Evol. 15, 448–453. doi: 10.1016/S0169-5347(00)01970-4
Connell J. H., Slatyer R. O. (1977). Mechanisms of succession in natural communities and their role in community stability and organization. Am. Nat. 111, 1119–1144. doi: 10.1086/283241
Cowen R. K., Gawarkiewicz G., Pineda J., Thorrold S. R., Werner F. E. (2007). Population connectivity in marine systems an overview. Oceanography 20, 14–21. doi: 10.5670/oceanog
Cowen R. K., Sponaugle S. (2009). Larval dispersal and marine population connectivity. Ann. Rev. Mar. Sci. 1, 443–466. doi: 10.1146/annurev.marine.010908.163757
Crisafulli C. M., Swanson F. J., Halvorson J. J., Clarkson B. D. (2015). “Volcano Ecology: disturbance characteristics and assembly of biological communities,” in The encyclopedia of volcanoes, 2 edition. Ed. Sigurdsson H. (Oxford: Elsevier), 1265–1284. doi: 10.3354/meps09206
Defeo O., McLachlan A. (2011). Coupling between macrofauna community structure and beach type: a deconstructive meta-analysis. Mar. Ecol. Progr. Ser. 433, 29–41. doi: 10.3354/meps09206
De Wreede R. E., Klinger T. (1988). “Reproduction strategies algae,” In Plant Reprod. ecology: patterns strategies Eds. Lovett Doust J., Lovett Doust L. (Oxford Univ. Press), pp. 267–284.
Doty M. S. (1967). Pioneer intertidal population and the related general vertical distribution of marine algae in Hawaii. Blumea 15, 95–105.
El-Maremie H., El-Mor M. (2015). Feeding habits of the bogue, Boops boops (Linnaeu) (Teleostei: Sparidae) in Benghazi coast, eastern Libya. J. Life Sci. 9, 189–196. doi: 10.17265/1934-7391/2015.05.001
Godwin J. R., Kosaki R. K. (1989). Reef fish assemblages on submerged lava flows of three different ages. Pac. Sci. 43, 289–301.
Gonzalez-Santana D., Santana-Casiano M., Gonzalez A. G., Gonzalez-Davila M. (2022). Coastal carbonate system variability along an active lava-seawater interface. Front. Mar. Sci. 9, 952203. doi: 10.3389/fmars.2022.952203
Guerra-Marrero A., Bonino-Perez A., Espino-Ruano A., Couce-Montero L., Jimenez-Alvarado D., Castro J. J. (2023). Life history parameters and fishing aspects of the alien nimble spray srab Percnon gibbesi in a native area of the Central-East Atlantic. Animals 13, 1427. doi: 10.3390/ani13081427
Gulliksen B., Beuchel F., Brattegard T., Palerud R. (2004). “The Marine Sublittoral Fauna of Jan Mayen Island: Zoogeography and Succession on “New” Lava Grounds,” in Jan Mayen island in scientific focus (Springer Netherlands), 159–171.
Gulliksen B., Haug T., Sandnes O. K. (1980). Benthic macrofauna on new and old lava grounds at Jan Mayen. Sarsia 65, 137–148. doi: 10.1080/00364827.1980.10431480
Hart M. B., Fisher J. K., Smart C. W., Speers R., Wall-Palmer D. (2022). Re-colonization of hostile environments by benthic foraminifera: an example from Monserrat, Lesser Antilles Volcanic Arc. Micropaleontology 68, 1–27. doi: 10.47894/mpal
Herrera R., Espino F., Garrido M., Haroun R. J. (2002). Observations on fish colonization and predation on two artificial reefs in the Canary Islands. ICES Mar. Sci. 59, 69–73. doi: 10.1006/jmsc.2002.1291
Huston M., Smith T. (1987). Plant succession: life history and competition. Am. Nat. 130, 168–198. doi: 10.1086/284704
Jewett S. C., Bodkin J. L., Chenelot H., Esslinger G. G., Hoberg M. K. (2010). The nearshore benthic community of Kasatochi Island, one year after the 2008 volcanic eruption. Arct. Antarctic Alp. Res. 42, 315–324. doi: 10.1657/1938-4246-42.3.315
Jonsson S. (1968). Survey on the intertidal and subtidal algae on Surtsey in 1967. Surtsey Res. Progr. Rep. 4, 67–73.
Jonsson S., Gunnarsson K. (1982). Marine algal colonization at Surtsey. Surtsey Res. Progr. Rep. 9, 33–45. doi: 10.33112/surtsey
Jouval F., Bigot L., Bureau S., Quod J., Penin L., Adjeroud M. (2020). Diversity, structure, and demography of coral assemblages on underwater lava flows of different ages at Reunion Island and implications for ecological succession hypotheses. Sci. Rep. 10, 20821. doi: 10.1038/s41598-020-77665-z
Jung S. W., Choi C. G. (2022). Seaweed community and succession on a trapezoidal-shaped artificial reef. Ocean Sci. J. 57, 130–140. doi: 10.1007/s12601-021-00053-9
Landeira J. M., Lozano-Soldevilla F. (2018). Seasonal of planktonic crustacean decapod larvae in the subtropical waters of Gran Canaria Island, NE Atlantic. Sci. Mar. 82, 119–134. doi: 10.3989/scimar.2018.82n2
Leitao F., Santos M. N., Erzini K., Monteiro C. C. (2008). Fish assemblages and rapid colonization after enlargement of an artificial reef off the Algarve coast (Southern Portugal). Mar. Ecol. 29, 435–448. doi: 10.1111/j.1439-0485.2008.00253.x
Levin L. A. (1984). Life history and dispersal patterns in a dense infaunal polychaete assemblage: community structure and response to disturbance. Ecology 65, 1185–1200. doi: 10.2307/1938326
Littler M. M., Littler D. S. (1980). The evolution of thallus form and survival strategies in benthic marine macroalgae: field and laboratory tests of a functional form model. Am. Nat. 116, 25–44. doi: 10.1086/283610
Lopez C., Poladura A., Hernandez J. C., Martin L., Concepcion L., Sangil C., et al. (2012). Contrasting effects of protection from harvesting in populations of two limpet species in a recently established marine protected area. Sci. Mar. 76, 799–807. doi: 10.3989/scimar.03601.15C
Mariath R., Rodriguez R. R., Figueiredo M. A. (2013). Succession of crustose coralline red algae (Rhodophyta) on coralgal reefs exposed to physical disturbance in the southwest Atlantic. Helgol. Mar. Res. 67, 687–696. doi: 10.1007/s10152-013-0354-3
Martin-Garcia L., Sangil C., Concepcion-Francisco L., Barquin-Diez J. (2013). Cartografia de las comunidades bentonicas de los fondos litorales de la isla de la Palma. Consorcio Insular la Reserva Mundial la Biosfera, 62.
Mayakun J., Prathep A., Kim J. H. (2020). Timing of disturbance, top-down, and bottom-up driving on early algal succession patterns in a tropical intertidal community. Phycol. Res. 68, 135–143. doi: 10.1111/pre.12406
McCook L. J. (1994). Understanding ecological community succession: causal models and theories, a review. Vegetatio 110, 115–147. doi: 10.1007/BF00033394
McCook L. J., Chapman A. R. O. (1993). Community succession following massive ice-scour on a rocky intertidal shore: recruitment, competition, and predation during early, primary succession. Mar. Biol. 115, 565–575. doi: 10.1007/BF00349363
Menendez M. C., Baleani C. A., Acha E. M., Piccolo M. C. (2023). Holoplankton and meroplankton communities in surf zone waters of a temperate SW Atlantic sandy beach: Seasonal patterns. Mar. Ecol., e12750. doi: 10.1111/maec.12750
Mori A. S., Osono T., Uchida M., Kanda H. (2008). Changes in the structure and heterogeneity of vegetation and microsite environments with the chronosequence of primary succession on a glacier foreland in Ellesmere Island, high arctic Canada. Ecol. Res. 23, 363–370. doi: 10.1007/s11284-007-0388-6
Parrish F. A. (2015). Settlement, colonization, and succession patterns of gold coral Kulamanamana haumeaae in Hawaiian deep coral assemblages. Mar. Ecol. Prog. Ser. 533, 135–147. doi: 10.3354/meps11390
Pinault M., Loiseau N., Chabanet P., Durville P., Magalon H., Quod J. P., et al. (2013). Marine fish communities in shallow volcanic habitats. J. Fish Biol. 82, 1821–1847. doi: 10.1111/jfb.12110
Prach K., Walker L. R. (2018). Differences between primary and secondary plant succession among biomes of the world. J. Ecol. 107, 510–526. doi: 10.1111/1365-2745.13078
Railkin A. I. (2003). Marine Biofouling: Colonization Processeses and Defenses (Boca Raton: CRC Press). doi: 10.1201/9780203503232
Sangil C., Afonso-Carrillo J., Sanson C. (2005). Zonacion del fitobentos en el litoral noroeste de la Palma (Islas Canarias). Rev. Acad. Canar. Cienc. 4, 75–90.
Sangil C., Alvarez-Canali D., Reyes J., Rodriguez J., Afonso-Carrillo J., Sanson M., et al. (2023). “Primeros colonizadores en los deltas lavicos del volcan Tajogaite (La Palma, islas Canarias),” in Tajogaite. Ensenanzas de una erupcion volcanica (La Palma, otono 2021), XVIII Semana Cientifica Telesforo Bravo. Ed. Afonso-Carrillo J. (Puerto de La Cruz: Instituto de Estudios Hispanicos de Canarias), 63–116.
Santelices B. (1990). Patterns of reproduction, dispersal, and recruitment in seaweeds. Ocearrogr. Mar. Biol. Annu. Rev. 28, 177–276.
Schils T. (2012). Episodic eruptions of volcanic ash trigger a reversible cascade of nuisance species outbreaks in pristine coral habitats. PlosOne 7, e46639. doi: 10.1371/journal.pone.0046639
Schleyer M. H., Benayahu Y., Parker-Nance S., van Soest R. W. M., Quod J. P. (2016). Benthos on submerged lava beds of varying age off the coast of Reunion, western Indian Ocean: sponges, octocorals and ascidians. Biodiversity 17, 93–100. doi: 10.1080/14888386.2016.1190669
Serisawa Y., Taino S., Ohno M., Aruga Y. (1998). Succession of seaweeds on experimental plates immersed during different seasons in Tosa Bay, Japan. Bot. Mar. 41, 321–328. doi: 10.1515/botm.1998.41.1-6.321
Sousa W. P. (1979). Experimental investigations of disturbance and ecological succession in a rocky intertidal algal community. Ecol. Monogr. 49, 227–254. doi: 10.2307/1942484
Sousa R., Delgado J., Gonzalez J. A., Freitas M., Henriques P. (2018). “Marine snails of the genus Phorcus: biology and ecology of sentinel species for human impacts on the rocky shores,” in Biological resources of water. Ed. Ray S. (London: IntechOpen), 141–167.
Srednick G., Cohen A., Diehl O., Tyler K., Swearer S. E. (2023). Habitat attributes mediate herbivory and influence community development in algal metacommunities. Ecology 104, e3976. doi: 10.1002/ecy.3976
Thorton I. (2007). Island colonization: the origin and development of Island Communities (Cambridge: Cambridge University Press).
Underwood A. J., Chapman M. G. (2006). Early development of subtidal macrofaunal assemblages: relationships to period and timing of colonization. J. Exp. Mar. Ecol. 330, 221–233. doi: 10.1016/j.jembe.2005.12.029
Valiela I. (1995). “Development of Structure in Marine Communities: Colonization and Succession,” in Marine Ecological Processes (Springer, New York, NY). doi: 10.1007/978-1-4757-4125-4
Vroom P. S., Zgliczynski B. J. (2011). Effects of volcanic ash deposits on four functional groups of a coral reef. Coral Reefs 30, 1025–1032. doi: 10.1007/s00338-011-0793-8
Watanabe J. T., Young C. M. (2006). Feeding habits and phenotypic changes in proboscis length in the southern oyster drill, Stramonita haemastoma (Gastropoda: Muricidae), on Florida sabellariid worm reefs. Mar. Biol. 148, 1021–1029. doi: 10.1007/s00227-005-0152-9
Williams G. A., Davies M. S., Nagarkar S. (2000). Primary succession on a seasonal tropical rocky shore: the relative roles of spatial heterogeneity and herbivory. Mar. Ecol. Progr. Ser. 203, 81–94. doi: 10.3354/meps203081
Wong E., Yau C., Chan K. Y. K. (2022). Seasonal and spatial dynamics of mesozooplankton community in a subtropical embayment. Reg. Stud. Mar. Sci. 56, 102724. doi: 10.1016/j.rsma.2022.102724
Keywords: marine benthic ecosystems, ecosystem restoration, early successional stages, colonization, volcanic eruption
Citation: Sangil C, Álvarez-Canali D, Reyes J, Rodríguez J and Sansón M (2024) Primary ecological succession of marine communities on the Tajogaite lava flows (La Palma, Canary Islands), fishes colonize faster than macroinvertebrates and algae. Front. Mar. Sci. 11:1337894. doi: 10.3389/fmars.2024.1337894
Received: 13 November 2023; Accepted: 01 February 2024;
Published: 22 February 2024.
Edited by:
Elva G. Escobar-Briones, National Autonomous University of Mexico, MexicoReviewed by:
Antonietta Rosso, University of Catania, ItalyLucia Fanini, University of Salento, Italy
Maickel Armenteros, National Autonomous University of Mexico, Mexico
Copyright © 2024 Sangil, Álvarez-Canali, Reyes, Rodríguez and Sansón. This is an open-access article distributed under the terms of the Creative Commons Attribution License (CC BY). The use, distribution or reproduction in other forums is permitted, provided the original author(s) and the copyright owner(s) are credited and that the original publication in this journal is cited, in accordance with accepted academic practice. No use, distribution or reproduction is permitted which does not comply with these terms.
*Correspondence: Carlos Sangil, Y2FzYW5naWxAdWxsLmVkdS5lcw==