- Department of Biological Sciences, University of Montreal, Montreal, QC, Canada
Benthic filter-feeders form an essential role in marine food chains as they constitute the bridge between the microscopic primary producers and the consumers. Although filter-feeders mainly feed on solid particles, they also capture and ingest oil droplets. Usually, these microdroplets come from the decomposition of animals or algae or from petroleum oils that enter water via spills and leakages. Here, we used videography, TRITC fluorescence microscopy, and fluid mechanics to study the capture mechanisms of canola, fish, and four petroleum motor oil droplets by the filter feeding sabellid and a serpulid polychaetes. Schizobranchia insignis, Eudistylia vancouveri, Myxicola infundibulum and Serpula columbiana actively feed on waste motor oil droplets in seawater. A further experiment found that S. insignis fed on all types of oil droplets, demonstrating no selectivity based on type. The oil droplet capture mechanism of S. insignis were direct interception and sieving, like that of solid particles. The size range of droplets ingested was 10 to 300 µm in diameter, but these ranges differed depending on the density and viscosity of the oils. Higher density and viscous oils were captured at smaller droplet sizes. These results are the first to characterize the mechanics of oil droplet capture, transport and ingestion by benthic ciliary filter feeders, and contribute to understanding the behavior of animals in response to oil emulsions, and how oils enter marine food webs.
1 Introduction
Invertebrates have evolved a remarkable array of strategies for survival, and one such adaptation is particle capture. This process involves the acquisition of food particles suspended in the surrounding environment, typically in aquatic habitats. Various invertebrates employ specialized structures and behaviors to achieve this feat including bivalves that use gills, crustaceans that employ specialized appendages with setae, and sponges that use specialized choanocyte cells and a unique water flow system. These adaptations showcase the incredible diversity and ingenuity of invertebrates in their quest for sustenance, underscoring the vital role they play in ecological systems.
Key to understanding the mechanics of particle capture is the theoretical treatment of particle interactions developed by Rubenstein and Koehl (1977). These interactions between a particle in water and a filter include i) direct interception, ii) inertial interactions, iii) gravitational deposition, iv) diffusional deposition, and v) sieving. Extending this theory to oil droplet in water and filter fiber interactions, Mehrabian et al. (2018) introduce the role of different parameters including oil-to-water viscosity ratio, oil-water interfacial tension, oil-water density difference, and the wettability of the fiber on the success of oil droplet capture and detachment. Wettability is the affinity of one fluid (oil) to adhere to and spread on a solid surface in the presence of another immiscible fluid (water). It is governed by the texture and surface chemistry of the solid surface (Mehrabian et al., 2018; Letendre and Cameron, 2022). Experimental tests of this theory include investigating oil droplet capture by Daphnia and barnacles at different Reynolds numbers (Letendre and Cameron, 2022), the role of droplet size and viscosity on capture using copepods and barnacles (Letendre et al., 2020), and the role of surfactants in droplet capture and loss by Daphnia and copepods (Almeda et al., 2014; Letendre et al., 2023). The rate of oil droplets captured increases with increased droplet concentration by the pelagic doliolid Dolioletta gehenbauri (Lee et al., 2012). Doliolids capture particles with ciliated gills, but the capture mechanism of the oil droplets was not documented. Here we investigate oil droplet capture by benthic polychaetes that capture particles using ciliated radioles.
Polychaete worms are one of the most common groups of macro-invertebrates found in benthic environments. They come in many forms and have adapted to fill different niches such as predator, scavenger, surface deposit-feeder and filter-feeders (Fauchald and Jumars, 1979). Some of the most common of these are serpulids and sabellids that use a crown of radioles that capture and transport particles to the mouth (Riisgård and Larsen, 2000). Each radiole is lined by a pair of pinnules. On a pinnule, there are cilia, which are used to retain food particles in the water and carry them towards the frontal side of the radiole were a similarly ciliated groove guides the particles to the mouth (Fauchald and Jumars, 1979; Riisgård and Larsen, 2000). The cilia also create a water current to bring food towards the worm, the current flowing upwards through the crown and in between the pinnules. The animals can separate particles by their size: those that are too large are rejected, the medium-sized particles are used for tube building, and the smaller ones are eaten (Shimeta, 1996; Nash and Keegan, 2003). Filter-feeding polychaetes eat diatoms, dinoflagellates, and other unicellular algae, as well as small invertebrates including larvae (Fauchald and Jumars, 1979). It is unknown if polychaetes capture and ingest oil droplets that arise from the decomposition of animals or algae, or from petroleum oils enter the water from spills and leakages.
Here we determined whether three sabellids and a serpulid polychaetes (Schizobranchia insignis, Eudistylia vancouveri, Myxicola infundibulum and Serpula columbiana) capture and ingest droplets of waste motor oil. We then investigate oil droplet taste selection by Schizobranchia insignis on canola oil, fish oil and four types of motor oil. We then used S. insignis to quantify droplet size selectivity, depending on the density and viscosity of the oils. At low Re, density and viscosity are important variable for oil droplet capture by fibers. According to theory, neutrally buoyant and small droplets will be preferentially captured (Mehrabian et al., 2018). If the oil is less dense than water, then gravitational forces will move the oil to the top (creaming), and when the oil is heavier than water, the oil will move downwards (sedimentation). The smaller the emulsion size, the slower it will move, increasing the chance of capture. Similarly, neutrally buoyant droplets will have more opportunity to be captured by filter feeders because they have a longer duration in the environment. The effect of oil-to-water viscosity ratio (which we will henceforth refer to as ‘viscosity’) is important only in inertial interaction and gravitational deposition. Oil viscosity is generally greater than water viscosity. Therefore, all parameters being equal, a less viscous oil droplet has higher chance of being captured by a filter feeder compared to a higher viscous oil droplet through inertial interaction and gravitation deposition (Mehrabian et al., 2018). This study is significant to understand the physics of oil droplet capture, the behavior of animals in response to oil emulsions, the bioaccumulation and trophic transfer of oils and because it informs oil spill remediation strategies.
2 Materials and methods
2.1 Preparation of emulsions
To study and compare the ingestion of different types of oils, three types of oil emulsions were prepared. The first one, for larger animals, was mixed using either light crude, canola, fish, waste motor oil or motor oil (Table 1), and artificial saltwater or filtered seawater (1µm filtration). The salt water was made with dechlorinated tap water and Instant Ocean Sea salt at a specific gravity of 1.020 to 1.022 to simulate seawater. Seventy-five μL of oil was pipetted into 1 L of water. The second emulsion for smaller animals was prepared the same way, except that 32.5 µL of oil was mixed with 500 ml of water. The third one was exactly like the first one but using unfiltered sea water. All solutions were then mixed for 20 min using a magnetic stirrer at 900 RPM.
The density of each oil was calculated by using weight of 10 ml with a Mettler Toledo balance. The viscosity was determined using an Anton Paar Physica MCR 501. Both the density and viscosity were measured at room temperature (23°C).
2.2 Collection and animal care
Four species of polychaete worms from the eastern Pacific Ocean were used for this experiment: Schizobranchia insignis, Eudistylia vancouveri, Myxicola infundibulum and Serpula columbiana (Table 2). These species were chosen because they are common to marinas on the west coast of Canada, where individuals are habituated to boats, waves, and people, they can easily be removed from their tubes, they are commercially available through West Wind SeaLabs, and do well in aquariums. The dock and pilings or intertidal habitat of these species moreover likely exposes them to spilled motor oil droplets.
A survey of oil droplet capture by polychaetes was performed at the Bamfield Marine Sciences Centre, British Columbia, Canada. The animals were collected on the docks, or on the sea floor while diving. They fed on the algae and other particles in the sea water and were supplemented once a week with a plankton and algae blend. In Montreal, Schizobranchia insignis was bought and shipped from WestWind Sealabs, Vancouver Island, B.C., Canada, and kept in a saltwater aquarium. They were fed with the algae growing in the tank and supplemented with spirulina powder.
2.3 Feeding experiments
The four species of polychaete worms (Schizobranchia insignis, Eudistylia vancouveri, Myxicola infundibulum and Serpula columbiana) were exposed to oil-in-water emulsions to determine feeding on waste motor oil droplets. They were starved for 24 hours beforehand in artificial saltwater or filtered seawater to ensure a clear digestive tract and to facilitate oil observations, then put in an oil-in-water emulsion for 24 hours. Oil feeding was determined by observation of oil droplets in the fecal pellets using a light microscope mounted with a Samsung S21 camera.
Schizobranchia insignis was also used as a model species and tested with all types of oil. The individuals were starved in filtered seawater for 24 hours, then put in an oil-in-water emulsion for 24 hours. After the feeding period, the individuals were dissected and samples from the cilia, gut and fecal pellets were mounted on slides and photographed under fluorescence microscopy using a TRITC filter mounted on an Olympus BX51 equipped with a Teledyne Luminera Infinity 3 camera or a light microscope with a Samsung S21 camera.
Schizobranchia insignis was also tested with unfiltered seawater emulsion. Oil feeding was determined by observation of the fecal pellets using a light microscope.
2.4 Capture experiments
To understand the mechanics of particle capture, a single radiole was removed from the worm and placed in a small petri dish with the oil-in-water emulsion. The only flow was that generated by the cilia. The capture of oil was recorded with a Samsung S21 camera mounted on an SX16 Olympus microscope (Figure 1). Despite separation from the body, the radiole continued to filter food from the water, which facilitated observations. Pictures were taken of the oil droplets that were captured and transported along the radiole, including proximally where they normally would have been transported to the mouth. Different types of oils were used to determine changes in minimum, maximum and average droplet size range depending on the oil density (canola and waste engine oil) and viscosity (marine and semi-synthetic engine oil). To ensure that no difference in the results came from the size of the animals, the same individual was used with both emulsions. The oil droplet sizes captured by S. insignis were measured from light and fluorescence microscopy photographs of the oil droplets accumulated on the radioles. We used the program ImageJ and its ‘Analyze particles’ feature to calculate the area of round shapes on images using a set scale, when possible, and calculated the remainder by hand. The same technique was used to measure the droplets sizes in the emulsions. The minimum, maximum, and average size were determined. Using Rstudio, the normality of all distributions was confirmed or denied with a Shapiro-Wilk test, then the distributions were compared to determine if they were significantly different with multiple Mann–Whitney U tests.
3 Results
3.1 Feeding experiments
In the first experiment, the four species (Schizobranchia insignis, Eudistylia vancouveri, Myxicola infundibulum and Serpula columbiana) were exposed for 24 hours to an oil-in-water emulsion made with waste motor oil. All individuals fed on the oil droplets, as was seen from the presence of multiple oil drops in their fecal matter after the experiment (Figure 2). As a control, pictures of the normal fecal pellets of each species were taken. This highlights the translucent nature and round shape of the droplets in the pellets. This experiment was repeated with multiple individuals to verify the replicability of the results.
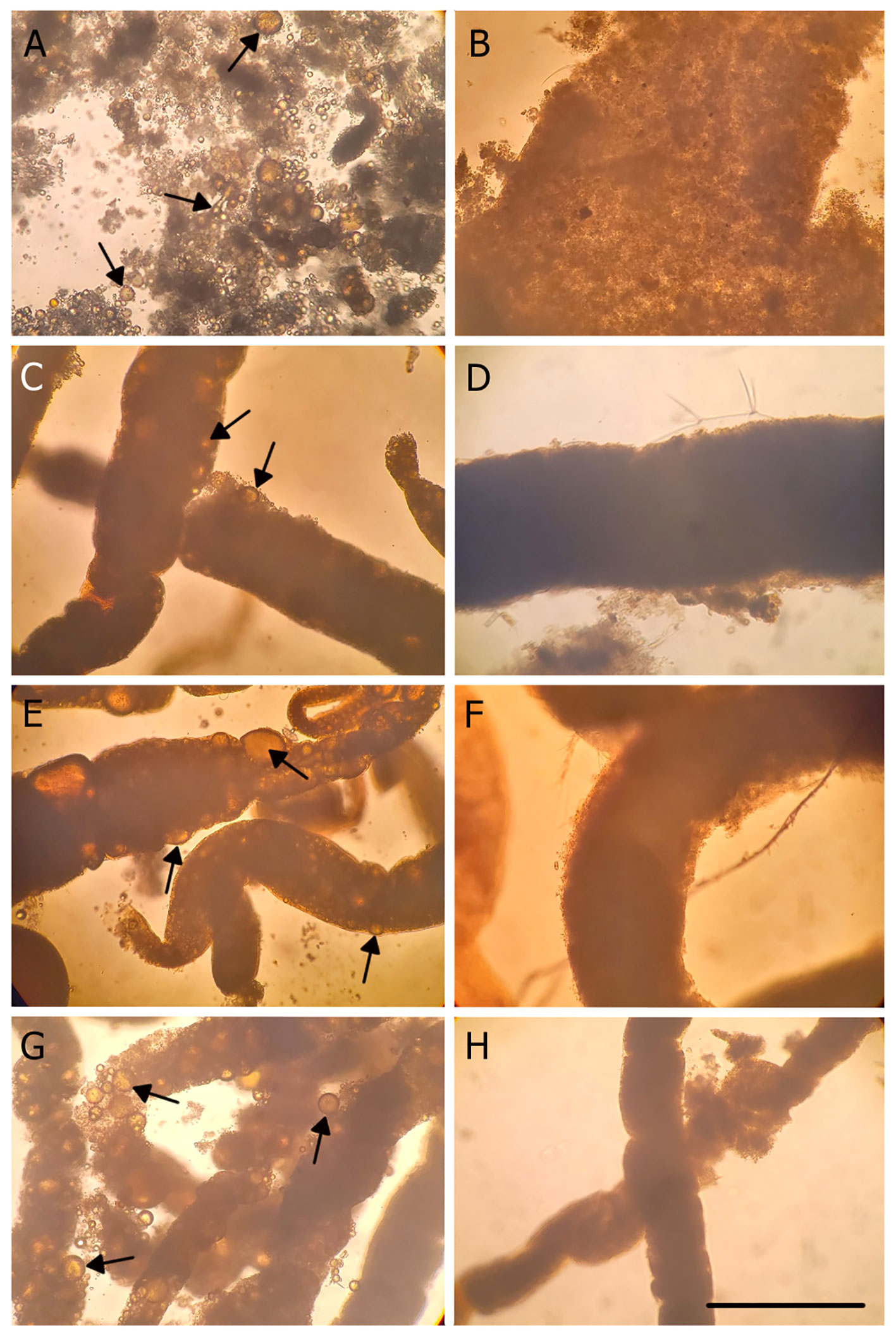
Figure 2 Fecal matter of multiple species of filter-feeding polychaete worms following feeding on a waste oil in seawater emulsion. Left column: fecal matter contaminated with oil; right column: control (without oil) fecal matter of the same species. (A, B) Eudistylia vancouveri. (C, D) Serpula columbiana. (E, F) Schizobranchia insignis. (G, H) Myxicola infundibulum. Arrows point to a sample of oil droplets. Scale bar: 500 μm.
The next experiment was performed using light crude oil, to determine the feeding capture mechanism. Crude oil was used because it is autofluorescent under a TRITC filter. After feeding, a dissection of an individual S. insignis was done. Oil droplets were present on the pinnules, in the gut, and in the fecal matter (Figure 3). Additional worms were put in filtered seawater and fed canola, fish, and motor oils (Figure 4). In all cases, oil droplets were present in the fecal matter of the worm. The semi-synthetic engine oil and marine engine oil droplets did not appreciably coalesce (Figures 4D, E). The fish oil was cleared from the seawater, and the feces were translucent, but with fewer distinct droplets, suggesting that it coalesced and was absorbed by the gut (Figure 4C). Canola oil was like fish oil in that few droplets were observed in translucent feces (Figures 4A, B). Then, individuals of S. insignis were put in unfiltered seawater with waste engine oil droplets. S. insignis was seen ingesting the oil droplets, and a high concentration of droplets was found in the feces (Figure 4F).
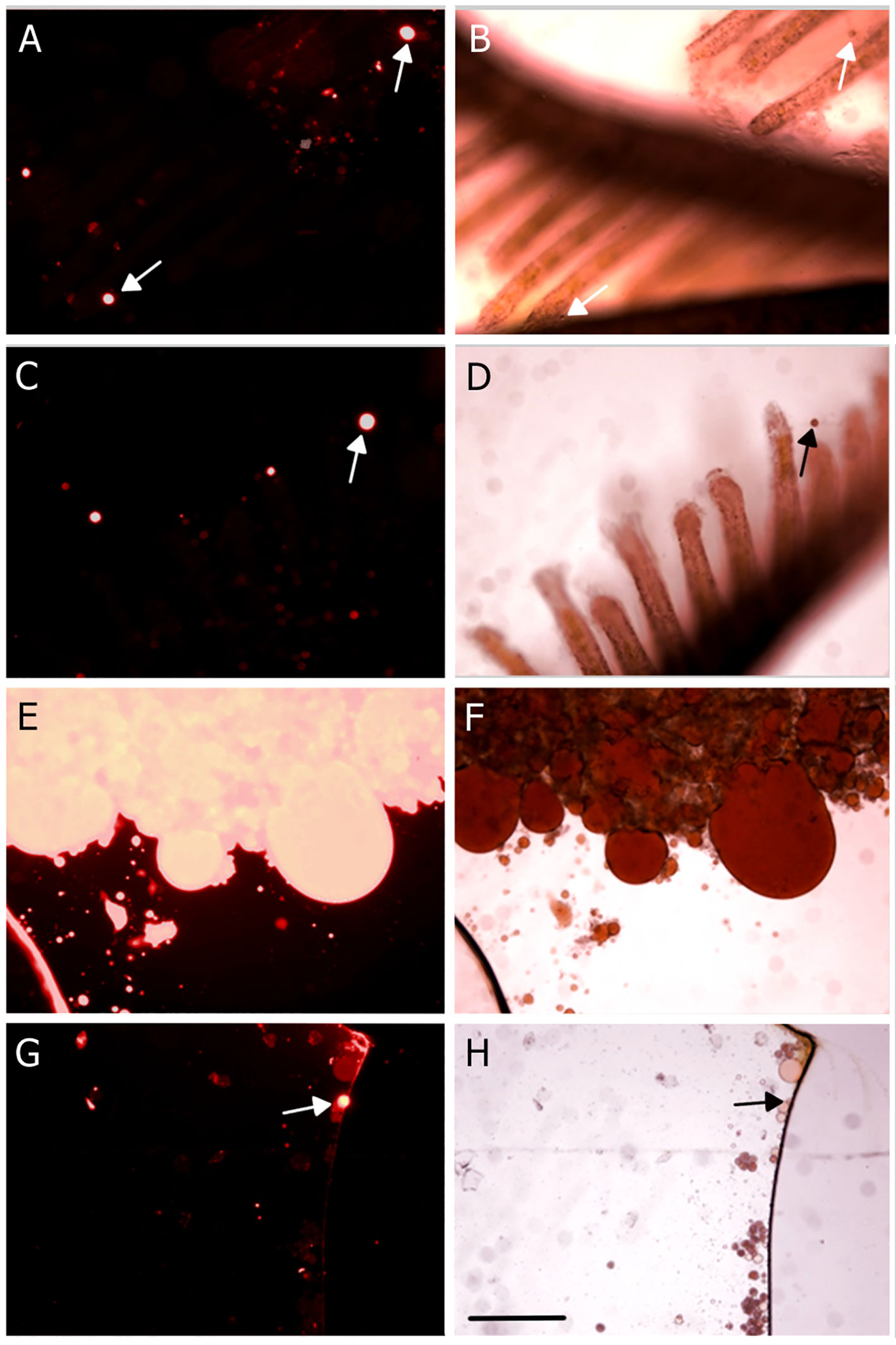
Figure 3 Captured and ingested crude oil droplets by Schizobranchia insignis following feeding on a waste oil in seawater emulsion and photographed under a TRITC filter (left column) and light (right column). (A, B) Radiole with a paired row of pinnules after feeding. (C, D) A different radiole of the same individual. (E, F) Fecal matter. (G, H) Gut contents. Arrows point to a sample of oil droplets. Scale bar: 200 μm.
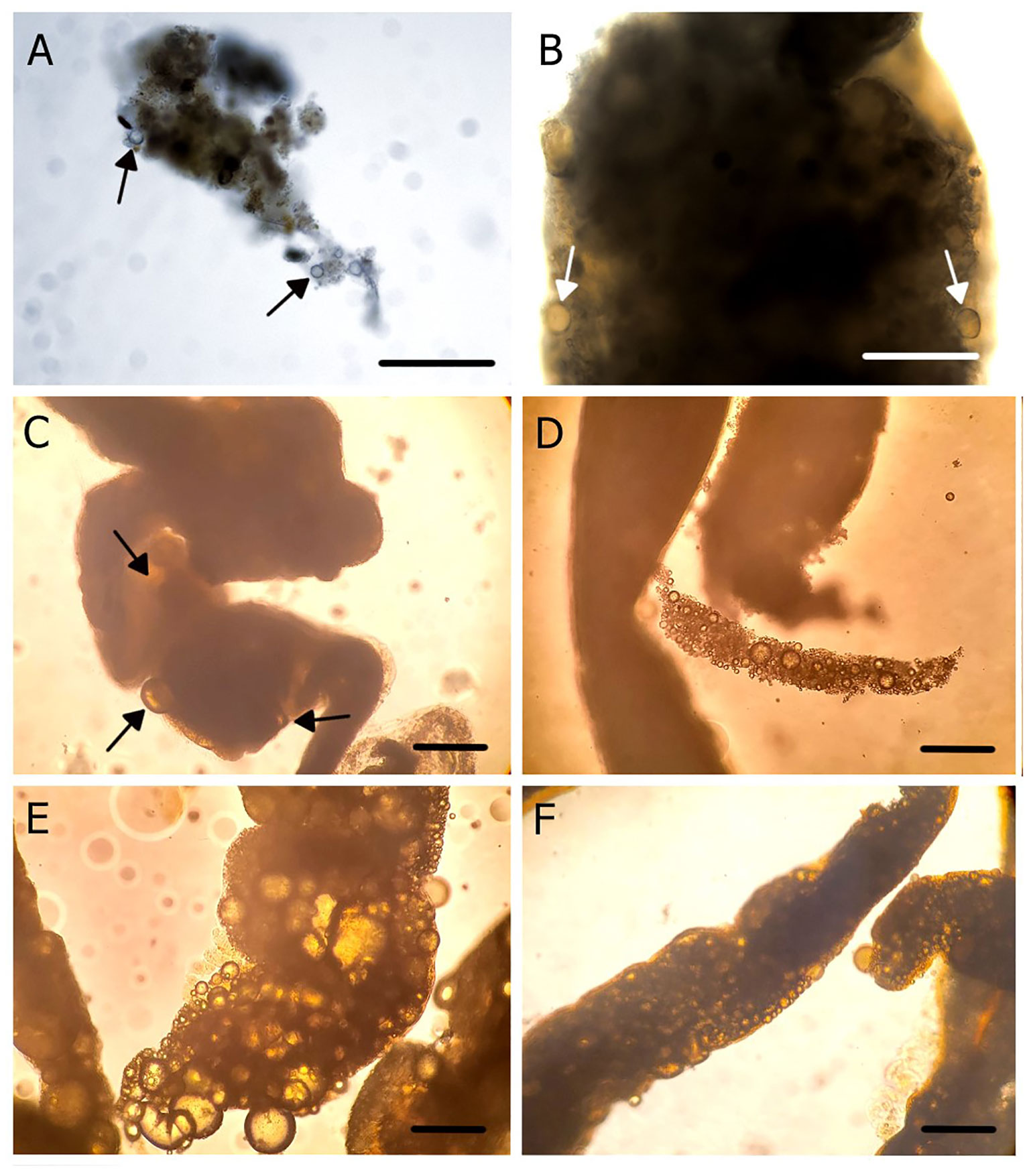
Figure 4 Captured and ingested oil droplets in the fecal matter of Schizobranchia insignis following feeding on a waste oil in seawater emulsion. (A, B) Canola oil. (C) Fish oil. (D) Marine engine oil. (E) Semi-synthetic engine oil. (F) Waste oil with unfiltered seawater. Arrows point to a sample of oil droplets. Scale bar: 200 μm.
These experiments were done at a Reynolds number of 0.000154, where the fluid velocity was 0.001 m/s, the characteristic cilia diameter was 0.2 μm, the seawater density was 1024 kg/m3, and the dynamic viscosity was 1.33 mPa•s. At Re of <1 flow is laminar.
3.2 Capture experiments
The mechanics of oil droplet capture was like that of particle capture (Figure 5; Supplementary Movie 1). The oil droplets in water (Figure 5A) were captured by the cilia and mucus on the pinnule (Figure 5B), transported down the pinnule to the radiole (Figure 5C), and then to the base of the radiole (Figure 5D). Supplementary Movie 2 also shows the transport of multiple droplets along the apical groove of a radiole.
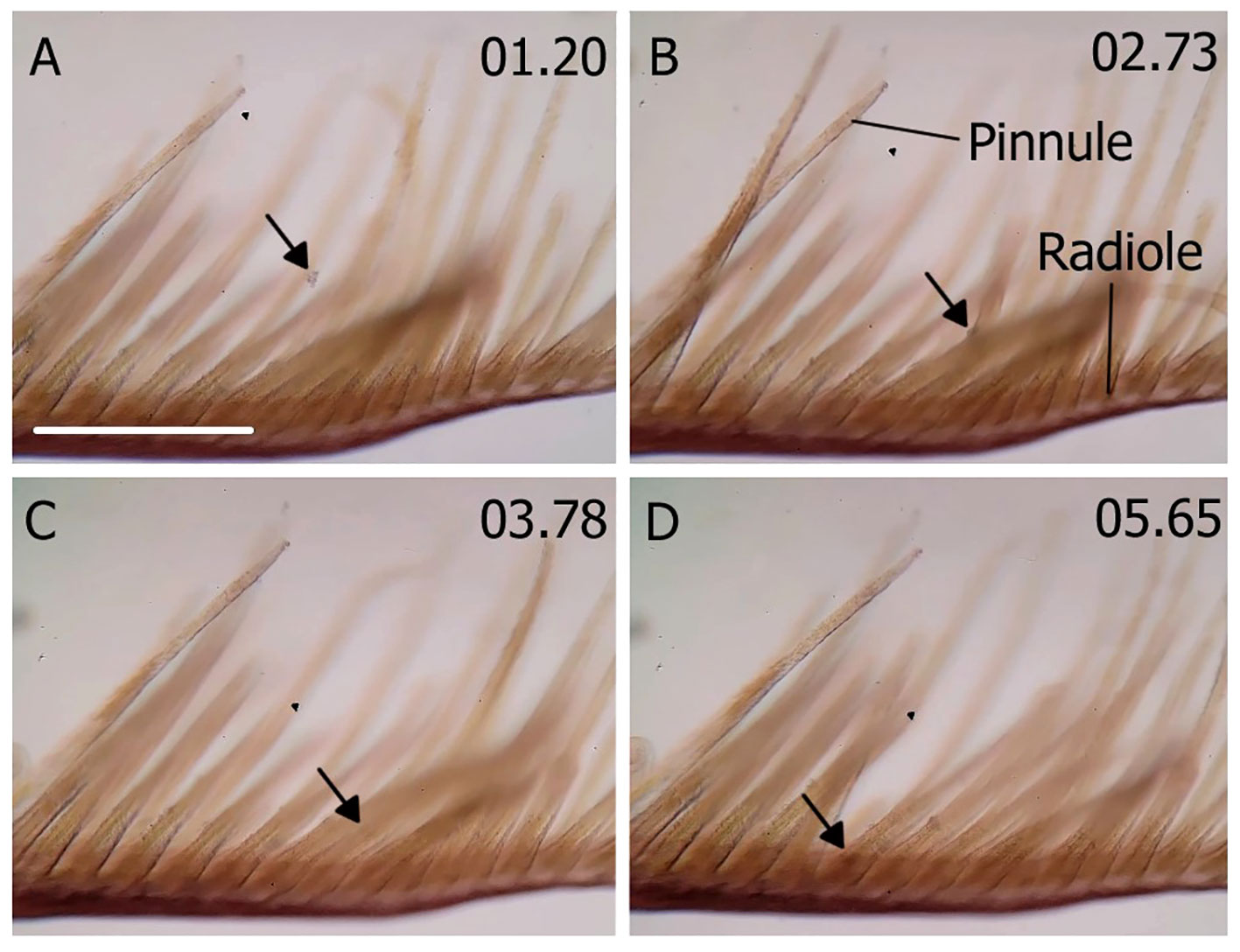
Figure 5 Transport of captured oil droplets on a pinnule and radiole of Schizobranchia insignis. The arrows point to a single droplet and follow its path. (A) An oil droplet in suspension. (B) The droplet captured by the cilia, (C) transported down the pinnule to the radiole and (D) to the base of the radiole. Time in seconds on top right corner. Scale bar: 200µm. See movie 1 and 2.
The next two experiments were done to understand the roles of density and viscosity on particle capture. Regarding density, the canola oil and waste motor oil were used as they have different densities and similar viscosities (Table 1). To ensure that the variation between the droplet sizes came from the oil density and not the size of the worm and its radioles, the same worm was used for the canola and the waste motor oil experiments. The waste motor oil emulsion droplet diameter varied from 2.77 to 281.35 μm (mean 40.12 μm) (Figure 6), whereas those captured varied from 27.67 to 225.25 μm (mean 95.88 μm). The mean emulsion droplet diameter for canola oil varied from 11.40 to 315.80 μm (mean 42.62 μm) (Figure 6), whereas those captured varied from 21.15 to 183.17 μm (mean 73.01 μm). To determine the normality of these distributions, Shapiro-Wilk tests were conducted, and all proved to be statistically significant at (P < 0.05), rejecting the null-hypothesis of normality. Thus, two Mann–Whitney U tests were performed to determine if the difference between the pre- and post-experimental canola oil and the pre- and post-experimental waste oil size distributions were statistically significant, and a p-value of P < 2.2e-16 was obtained for both in R. This shows that the droplet size distributions of the emulsion and the captured droplets are significantly different, be it canola oil or waste oil. Additionally, the capture size distribution between the canola and waste oil were compared with another Mann–Whitney U test, for which the p-value was of P = 2.84e-06. The sizes of the droplets captured were significatively different when the worm was exposed to two types of oils with different densities but similar viscosities, with the waste oil droplets captured being larger on average, with a higher minimum and maximum size droplets, despite the emulsion canola oil droplet having a larger maximum.
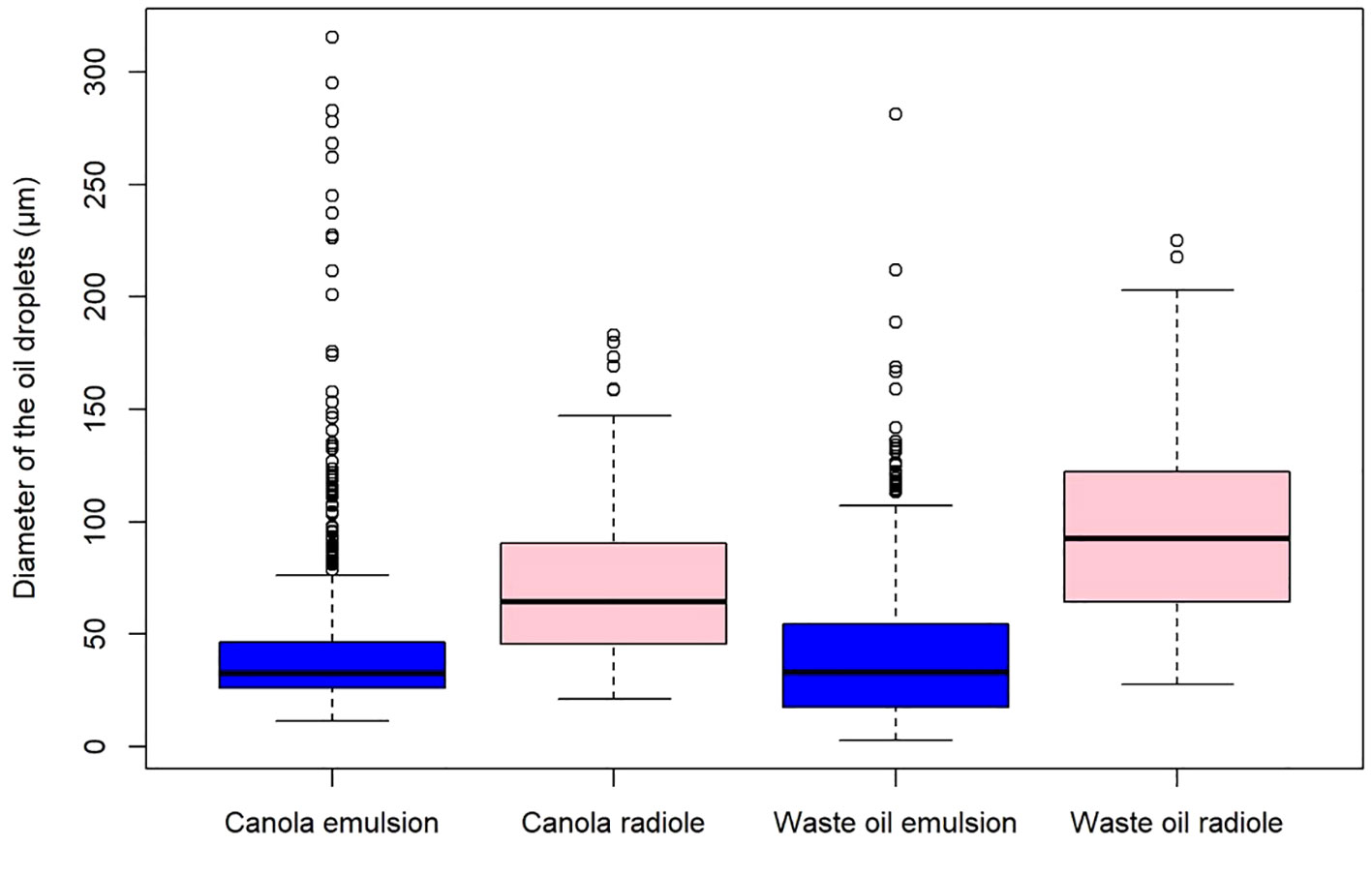
Figure 6 Boxplot of the distribution of canola oil (ρ = 950 kg/m3) and waste motor oil (ρ = 813 kg/m3) droplets present in the seawater emulsion versus those captured by Schizobranchia insignis. Canola emulsion n=812, canola radiole n=113, waste oil emulsion n=693, waste oil radiole n=132.
Finally, regarding viscosity, the same method was used as the density experiment, but the experimental oils were the marine engine oil and the semi-synthetic engine oil, because of similar densities and different viscosities (Table 1). The average marine engine oil emulsion droplet diameter varied from 25.50 to 365.36 μm (mean 95.27 μm), whereas those captured varied from 14.29 to 232.88 μm (mean 60.20 μm) (Figure 7). The semi-synthetic engine oil emulsion droplet diameter varied from 16.13 to 385.80 μm (mean 84.60 μm) (Figure 7), and the captured droplets varied from 14.43 to 310.15 μm (mean 93.63 μm). Since all distributions proved to be statistically significant at P < 0.05, rejecting the null-hypothesis of normality, two Mann–Whitney U tests were performed to determine if the difference between the two marine oil and the two semi-synthetic oil distributions were statistically significant. Whereas the marine oil distributions were significantly different with a p-value of < 2.2e-16, the semi-synthetic oil distributions were not (p-value = 0.1768). Additionally, the capture size distribution between the marine and semi-synthetic oils were compared with another Mann–Whitney U test, for which the p-value was of P = 4.768e-10, and so the distributions were significantly different. Although the size distributions for the marine and semi-synthetic oil emulsions were similar, it seems that the droplets captured by the worm were, on average, smaller with the more viscous marine engine oil.
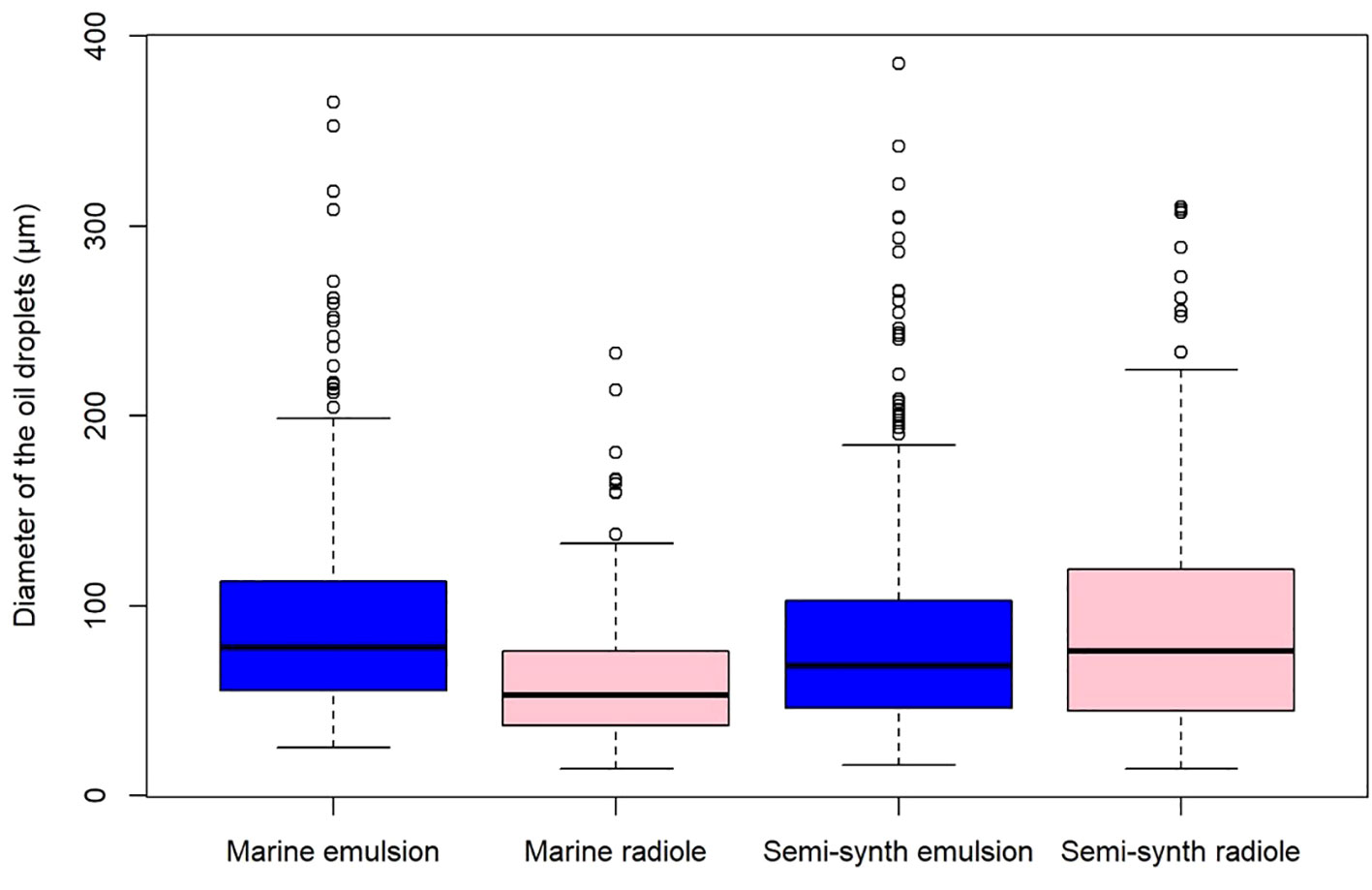
Figure 7 Boxplot of the distribution of the diameter of marine (μ = 156 mPa·s) and semi-synthetic (μ = 92 mPa·s) engine oil droplets present in the seawater emulsion versus those captured by Schizobranchia insignis. Marine emulsion n=456, marine radiole n=245, semi-synthetic emulsion n=482, semi-synthetic radiole n=253.
4 Discussion
Particle capture by polychaetes and other ciliated organisms is a crucial mechanism that plays a significant role in aquatic and marine systems ecology and pollution (Shimeta and Jumars, 1991; Ostroumov, 2005; Dean, 2008; Jang et al., 2018; Knutsen et al., 2020). These organisms have developed specialized mechanisms to capture and feed on suspended particles, such as phytoplankton, detritus, and small organic matter, helping to regulate nutrient cycling and energy transfer within aquatic food webs (Wallace et al, 1977; Humphrey et al., 1987; Gin et al., 2001). Concurrent with organic particles in aquatic systems are oil droplets, but the extent to which they are captured, and the mechanisms of the capture are unknown. Here we found that the sabellid polychaetes Schizobranchia insignis, Eudistylia vancouveri, Myxicola infundibulum and the serpulid Serpula columbiana readily capture waste motor oil droplets using their ciliated radioles. Closer observations with S. insignis found that, like particles, oil droplets were capture by the ciliated pinnules, then transported to the mouth along the apical groove of the radioles (Shimeta and Jumars, 1991; Riisgård and Larsen, 1995; Shimeta and Koehl, 1997). Further feeding trials found that S. insignis did not select oil droplets based on oil type. Following feeding trials, canola oil, fish oil and three kinds of motor oil were observed on the radioles, in the gut and fecal castings. Similarly, S. insignis fed on an oil emulsion in unfiltered seawater that contained food particles, seemingly not discriminating against oil in its diet. In fact, the fecal pellets mostly contained oil droplets and few if any algae cells. Amongst filter feeders, particle selectivity based on taste is common, such as with some copepods, Daphnia, cladocerans, and rotifers (Friedman and Strickler, 1975; Hartman and Hartman, 1977; DeMott and Watson, 1991; Kerfoot and Kirk, 1991), though non-selective particle capture is also common (DeMott, 1986). The capture of waste motor oil droplets in each of the species suggests that they do not differentiate oils based on type. Petroleum oil droplets are not a frequent food source for filter-feeders so they may generally lack adaptations to avoid them.
We did not find support for the hypothesis that small droplets are preferentially captured (Mehrabian et al., 2018). This hypothesis is based on theory that was developed for stiff fibers, whereas sabellid and serpulid worms capture droplets with flexible cilia. S. insignis preferentially captured droplets in its natural food particle range, no matter the type of oil used (canola and three different motor oils). Compared to the emulsion distributions, the particles captured with the canola and waste oil were significantly larger, whereas those captured with marine oil were significantly smaller, and for semi-synthetic oil insignificantly larger. In all cases, the droplets were around the same range of 10 to 300 µm, which corresponds with the normal particle size that sabellids capture. The filter-feeding polychaete Terebrasabella heterouncinata feeds in the range of 20 to 100 µm (Chalmers, 2003) and Serpula columbiana consumes particles that range at least from 40 to 250 µm (Pernet and Kohn, 1998). The emulsions created some larger droplets (>300 µm), but there were no droplets greater than 310 µm captured. At very small sizes, oil droplets interact with the cilia like particles due to the oil-to-water viscosity ratio and oil-water interfacial tension (Mehrabian et al., 2018). Our experiments were done at Reynolds numbers less than one where even miscible fluids are exceedingly difficult to mix (Vogel, 1994), and droplets are expected to maintain a spherical (un-deformed) shape (Taylor, 1934). Indeed, we found that the oil and seawater were not miscible, and the small oil droplets did not deform when contacted by cilia. Adding to droplet shape stability is an internal flow within the droplet that causes the oil to recirculate, and interfacial effects that resist droplet deformation (Mehrabian et al., 2018). All five of our oils were weakly buoyant, with a density ratio of 0.8 to 0.9 (Espinosa-Gayosso et al, 2015). The higher buoyancy of larger droplets meant that they had less time in the proximity of the animal and thus less time to be captured. Some of the larger droplets that were captured, may have then detached from the feeding appendage due to the positive buoyancy and deformation. For the same reasons, large droplets are difficult to manipulate comparted to small droplets (or particles).
Cilia and pinnule wettability were low for the oils. The oil and the water compete to wet the surface of cilia, and the water showed a higher affinity (i.e., the cilia are hydrophilic) compared to the oil (Mehrabian et al., 2018). We saw no oil droplets form a clam-shell shape along a cilium, further suggesting that the cilia are relatively oil-phobic. The droplets captured remained round, showing low affinity for these biological structures. In this way, the droplets were captured and behaved like solid particles. We can then assume that some droplets, like particles, might have been dislodged and rejected by a pinnule, and others might have been lost due to retention failure. In the latter case, the droplets slip off the pinnule because of an inadequate retention with the cilia or mucus (Shimeta and Koehl, 1997).
We were not able to clearly separate capture due to droplet size from capture due to viscosity or density. To do this, we would need to show that droplets of the same size (no variation) are preferentially captured based on a density or a viscosity closer to that of water. Unlike solid particles, oil droplets cannot be filtered to specific sizes because they coalescence and cream. Despite the inherent challenge with working with oils, we can draw some conclusions about capture based on viscosity and density.
We found that the captured oil droplets sizes varied depending on viscosity and density of oil, and the capture mechanism was sieving, and not inertial impaction or gravitational deposition. Our hypothesis stated that neutrally buoyant droplets would remain in the emulsion for a longer period, increasing the opportunity for capture. The denser canola oil is closer to the density of water, so the density difference is less, and the oil is less buoyant. As expected, this increased time in the capture field allowed for the capture of smaller droplets. A caveat here is that the denser canola oil droplets were significantly smaller than the lighter waste motor oil. The alternate interpretation, that capture was governed by inertial impaction and/or gravitational deposition is unlikely. In inertial impaction, the density difference between the particle and the fluid gives the particle momentum, thus its trajectory will deviate from the fluid streamlines around the fiber and cause the particle to be captured. Similarly, in gravitational deposition, the density difference between the particle and the fluid causes it to diverge and settle onto the fiber (Mehrabian et al., 2018). When using a lighter oil, the droplets are more likely to deviate from the streamlines due to buoyancy. In this scenario, smaller droplets would be more readily captured. Instead, we found that smaller droplets from more dense (less buoyant) oil were captured. For this reason, the length of time spent in the capture environment appears to be more important to capture than the divergence from streamlines. Sieving is not affected by the density of the droplets (or particles) (Mehrabian et al., 2018).
Viscous forces in the fluid might be expected to play a role in droplet capture. A less viscous oil droplet has a greater chance of capture through inertial interaction and gravitation deposition by a fiber. This may indicate that S. insignis would capture smaller droplets when using a less viscous oil, but this is not what we found. We found that smaller droplets of the more viscous marine engine oil were captured compared to the less viscous semi-synthetic oil. This may be due to the non-fiber like properties of cilia and pinnules, or because direct interception and sieving were the main capture mechanisms.
The fluid mechanics of oil droplet capture by filter feeding animals is a nascent field of study. Here we address the role of oil type, droplet size, oil density, oil viscosity and gravitational forces (buoyancy) on the capture, transport, and ingestion of droplets by sabellids and a serpulid polychaete. Some key factors and principals involved in the fluid mechanics of oil droplet capture that we did not test include the interfacial tension between the oil and the seawater that affects the stability of oil droplets. Lower interfacial tension makes it harder to coalesce droplets, potentially leading to smaller, more stable droplets that could affect capture. Our observations were done in calm water and so we did not investigate the role of Reynolds numbers at different flow regimes. In high Reynolds number flows, inertial forces dominate, which can affect oil droplet capture and detachment by fibrous appendages (Letendre and Cameron, 2022). Having said this, animals that use cilia for capture may especially be constricted to low Re numbers (Humphries, 2009). We did not investigate the Weber number that relates inertial forces to surface tension forces. It is important in understanding the breakup of oil droplets, which can influence capture (Mehrabian et al., 2018; Letendre et al., 2020). The addition of surfactants or demulsifying agents can alter the stability of emulsions and promote droplet coalescence (Letendre et al., 2023). There are no references to how these chemical agents may alter droplet capture by ciliated appendages. This new and nascent field of oil droplet capture by filter feeding animals parallels that of the more mature field of plastic particulate capture (Rogers et al., 2020). With respect to petroleum oils, it offers exciting new insights into animal form and function and needs to be accelerated with the same urgency to minimize environmental impacts and maximize biodiversity health.
Data availability statement
The raw data supporting the conclusions of this article will be made available by the authors, without undue reservation.
Author contributions
KB: Conceptualization, Data curation, Formal analysis, Investigation, Methodology, Writing – original draft, Writing – review & editing. CC: Conceptualization, Funding acquisition, Methodology, Project administration, Resources, Supervision, Writing – original draft, Writing – review & editing.
Funding
The author(s) declare financial support was received for the research, authorship, and/or publication of this article. KB is supported by a NSERC graduate student scholarship. This project was funded by NSERC Discovery grant RGPIN-2023-05647 to CC.
Acknowledgments
We are grateful to the director and staff of the Bamfield Marine Sciences Centre for accommodating our research.
Conflict of interest
The authors declare that the research was conducted in the absence of any commercial or financial relationships that could be construed as a potential conflict of interest.
Publisher’s note
All claims expressed in this article are solely those of the authors and do not necessarily represent those of their affiliated organizations, or those of the publisher, the editors and the reviewers. Any product that may be evaluated in this article, or claim that may be made by its manufacturer, is not guaranteed or endorsed by the publisher.
Supplementary material
The Supplementary Material for this article can be found online at: https://www.frontiersin.org/articles/10.3389/fmars.2024.1337358/full#supplementary-material
Supplementary Movie 1 | Transport of captured oil droplets on a pinnule and radiole of Schizobranchia insignis.
Supplementary Movie 2 | Transport of captured oil droplets along the apical groove on a radiole of Schizobranchia insignis.
References
Almeda R., Baca S., Hyatt C., Buskey E. J. (2014). Ingestion and sublethal effects of physically and chemically dispersed crude oil on marine planktonic copepods. Ecotoxicology 23, 988−1003. doi: 10.1007/s10646-014-1242-6
Chalmers R. (2003) An investigation into the feeding biology and factors influencing the population dynamics of Terebrasabella heterouncinata (Polychaeta: Sabellidae), a problematic tube-dwelling polychaete in farmed abalone in South Africa. Available online at: http://vital.seals.ac.za:8080/vital/access/manager/Repository/vital:5291?site_name=GlobalView.
Dean H. K. (2008). The use of polychaetes (Annelida) as indicator species of marine pollution: a review. Rev. Biología Trop. 56, 11–38.
DeMott W. R. (1986). The role of taste in food selection by freshwater zooplankton. Oecologia 69, 334–340. doi: 10.1007/BF00377053
DeMott W. R., Watson M. D. (1991). Remote detection of algae by copepods: responses to algal size, odors and motility. J. Plankton Res. 13, 1203–1222. doi: 10.1093/plankt/13.6.1203
Espinosa-Gayosso A., Ghisalberti M., Ivey G. N., Jones N. L. (2015). Density-ratio effects on the capture of suspended particles in aquatic systems. J. Fluid Mechanics 783, 191–210. doi: 10.1017/jfm.2015.557
Fauchald K., Jumars P. A. (1979). The diet of worms: A study of polychaete feeding guilds. Oceanography Mar. Biol. Annu. Rev. 17, 193–284.
Friedman M. M., Strickler J. R. (1975). Chemoreceptors and feeding in calanoid copepods (Arthropoda: Crustacea). Proc. Natl. Acad. Sci. 72, 4185–4188. doi: 10.1073/pnas.72.10.4185
Gin K. Y. H., Kamrul Huda Md., Kiat Lim W., Tkalich P. (2001). An oil spill–food chain interaction model for coastal waters. Mar. pollut. Bull. 42, 590−597. doi: 10.1016/S0025-326X(00)00205-8
Hartman H. B., Hartman M. S. (1977). The stimulation of filter feeding in the porcelain crab Petrolisthes cinctipes Randall by amino acids and sugars. Comp. Biochem. Physiol. Part A: Physiol. 56, 19–22. doi: 10.1016/0300-9629(77)90435-2
Humphrey B., Boehm P. D., Hamilton M. C., Norstrom R. J. (1987). The fate of chemically dispersed and untreated crude oil in arctic benthic biota. Arctic 40, 149−161. doi: 10.14430/arctic1810
Humphries S. (2009). Filter feeders and plankton increase particle encounter rates through flow regime control. Proc. Natl. Acad. Sci. 106, 7882–7887. doi: 10.1073/pnas.0809063106
Jang M., Shim W. J., Han G. M., Song Y. K., Hong S. H. (2018). Formation of microplastics by polychaetes (Marphysa sanGuinea) inhabiting expanded polystyrene marine debris. Mar. pollut. Bull. 131, 365–369. doi: 10.1016/j.marpolbul.2018.04.017
Kerfoot W. C., Kirk K. L. (1991). Degree of taste discrimination among suspension-feeding cladocerans and copepods: Implications for detritivory and herbivory. Limnology Oceanography 36, 1107–1123. doi: 10.4319/lo.1991.36.6.1107
Knutsen H., Cyvin J. B., Totland C., Lilleeng Ø., Wade E. J., Castro V., et al. (2020). Microplastic accumulation by tube-dwelling, suspension feeding polychaetes from the sediment surface: A case study from the Norwegian Continental Shelf. Mar. Environ. Res. 161, 105073. doi: 10.1016/j.marenvres.2020.105073
Lee R. F., Koster M., Paffenhofer G.-A. (2012). Ingestion and defecation of dispersed oil droplets by pelagic tunicates. J. Plankton Res. 34, 1058–1063. doi: 10.1093/plankt/fbs065
Letendre F., Cameron C. B. (2022). The capture of crude oil droplets by filter feeders at high and low Reynolds numbers. J. Exp. Biol. 225, jeb243819. doi: 10.1242/jeb.243819
Letendre F., Mehrabian S., Etienne S., Cameron C. B. (2020). The interactions of oil droplets with filter feeders: A fluid mechanics approach. Mar. Environ. Res. 161, 105059. doi: 10.1016/j.marenvres.2020.105059
Letendre F., Ramos P. A. S., Cameron C. B. (2023). The loss of crude oil droplets by filter feeders and the role of surfactants. Mar. pollut. Bull. 193, 115174. doi: 10.1016/j.marpolbul.2023.115174
Mehrabian S., Letendre F., Cameron C. B. (2018). The mechanisms of filter feeding on oil droplets : Theoretical considerations. Mar. Environ. Res. 135, 29−42. doi: 10.1016/j.marenvres.2018.01.006
Nash R., Keegan B. F. (2003). Aspects of the feeding biology of the fanworm Bispira volutacornis (Polychaeta: Sabellidae). J. Mar. Biol. Assoc. United Kingdom 83, 453−456. doi: 10.1017/S002531540300732Xh
Ostroumov S. A. (2005). Some aspects of water filtering activity of filter-feeders. Hydrobiologia 542, 275−286. doi: 10.1007/s10750-004-1875-1
Pernet B., Kohn A. J. (1998). Size-related obligate and facultative parasitism in the marine gastropod Trichotropis cancellata. Biol. Bull. 195, 349–356. doi: 10.2307/1543146
Riisgård H. U., Larsen P. (1995). Filter-feeding in marine macro-invertebrates: Pump characteristics, modelling and energy cost. Biol. Rev. Cambridge Philos. Soc. 70, 67−106. doi: 10.1111/j.1469-185X.1995.tb01440.x
Riisgård H. U., Larsen P. S. (2000). Comparative ecophysiology of active zoobenthic filter feeding, essence of current knowledge. J. Sea Res. 44, 169−193. doi: 10.1016/S1385-1101(00)00054-X
Rogers K. L., Carreres-Calabuig J. A., Gorokhova E., Posth N. R. (2020). Micro by-micro interactions: How microorganisms influence the fate of marine microplastics. Limnology Oceanography Lett. 5, 18–36. doi: 10.1002/lol2.10136
Rubenstein D. I., Koehl M. (1977). The Mechanisms of filter feeding: Some theoretical considerations. Am. Nat. 111, 981−994. doi: 10.1086/283227
Shimeta J. (1996). Particle-size selection by Pseudopolydora paucibranchiata (Polychaeta: Spionidae) in suspension feeding and in deposit feeding: influences of ontogeny and flow speed. Mar. Biol. 126, 479–488. doi: 10.1007/BF00354630
Shimeta J., Jumars P. A. (1991). Physical mechanisms and rates of particle capture by suspension feeders. Oceanogr. Mar. Biol. Annu. Rev. 29, 191–257.
Shimeta J., Koehl M. A. R. (1997). Mechanisms of particle selection by tentaculate suspension feeders during encounter, retention, and handling. J. Exp. Mar. Biol. Ecol. 209, 47–73. doi: 10.1016/S0022-0981(96)02684-6
Taylor G. I. (1934). The formation of emulsions in definable fields of flow. Proceedings of the Royal Society of London. Ser. A Containing Papers Math. Phys. Character 146, 501–523. doi: 10.1098/rspa.1934.0169
Vogel S. (1994). Life in Moving Fluids: The Physical Biology of Flow. 2nd edition (Princeton, USA: Princeton University Press).
Keywords: benthic ecology, petroleum pollution, emulsion, density, viscosity, carbon cycle, marine food web
Citation: Beaudry K and Cameron CB (2024) Oil Droplet Capture and Ingestion by Filter-Feeding Sabellid and Serpulid Polychaetes. Front. Mar. Sci. 11:1337358. doi: 10.3389/fmars.2024.1337358
Received: 12 November 2023; Accepted: 23 February 2024;
Published: 08 March 2024.
Edited by:
Stuart Humphries, University of Lincoln, United KingdomReviewed by:
Sriyutha Murthy, Bhabha Atomic Research Centre, IndiaVincent Hickl, Swiss Federal Laboratories for Materials Science and Technology, Switzerland
Copyright © 2024 Beaudry and Cameron. This is an open-access article distributed under the terms of the Creative Commons Attribution License (CC BY). The use, distribution or reproduction in other forums is permitted, provided the original author(s) and the copyright owner(s) are credited and that the original publication in this journal is cited, in accordance with accepted academic practice. No use, distribution or reproduction is permitted which does not comply with these terms.
*Correspondence: Katherine Beaudry, a2F0aGVyaW5lLmJlYXVkcnlAdW1vbnRyZWFsLmNh; Christopher B. Cameron, Yy5jYW1lcm9uQHVtb250cmVhbC5jYQ==