- 1Key Laboratory of Ocean and Marginal Sea Geology, South China Sea Institute of Oceanology, Chinese Academy of Sciences, Guangzhou, China
- 2South China Institute of Environmental Sciences, the Ministry of Ecology and Environment of the People's Republic of China, Guangzhou, China
- 3College of Mechanical and Power Engineering, Guangdong Ocean University, Zhanjiang, China
The coral reef ecosystem is one of the most productive ecosystems in the ocean, and is also an important calcium carbonate deposition region. Because excess production is very low in coral reefs, organic carbon reservoirs are very limited. During the calcification process, each mole of CaCO3 will produce 1 mol of CO2, approximately 60% of which will be released into the atmosphere through the sea-air interface. This causes coral reefs to be large inorganic carbon reservoirs, but at the same time, most coral reefs act as atmospheric CO2 sources (which also act as sinks in some coral reefs). Therefore, clarifying the sea-air CO2 exchange flux and carbon storage is critical for understanding the carbon cycle in coral reef ecosystems. In this paper, we summarize the carbon cycle processes in the coral reefs of the South China Sea (SCS) and estimate the total CO2 budget and carbon reserves. According to current research, the coral reefs in this area act as a source of atmospheric CO2, releasing 0.37−1.59 × 1011 g C a-1 into the atmosphere. Owing to their extremely high biological productivity and carbonate productivity, the carbon reserves of coral reefs in the SCS range from 1.66–3.78 × 1012 g C a-1, which is an order of magnitude greater than the CO2 emissions at the sea–air interface. Overall, coral reefs in the SCS are important carbon storage areas. As the current results are still approximate, a more comprehensive and in-depth investigation is needed to clarify the carbon source/sink processes, regulatory mechanisms, and carbon storage capacity of SCS coral reefs.
1 Introduction
Global CO2 emissions have consistently increased, with a 1% increase in 2022 compared with previous year, resulting in total emission of 10.0 Gt C a-1 (Friedlingstein et al., 2022). The global temperature is consistently increasing. The IPCC AR6 states that the global average surface temperature increased by 0.19 (0.16 to 0.22) °C between 2011 and 2020, compared with the period from 2003 to 2012 (Lee et al., 2023). The increase in temperature has led to the melting of the Arctic ice sheet, rising sea levels, and more frequent extreme weather events. These phenomena have significantly impacted the human living environment (Loucks, 2021). There has been increasing interest in the carbon cycle process, specifically regarding the dynamics of CO2 and CH4. This research area is one of the three core components of international global change, along with food and water resources (Liu, 2002). The accurate and comprehensive estimation of carbon fluxes is a key focus of carbon cycle research. The disclosure of the regulatory process and mechanism is beneficial for predicting the future evolution of the climate system and implementing effective countermeasures.
The ocean is widely acknowledged as the primary natural reservoir of atmospheric CO2. The IPCC AR6 (Lee et al., 2023) highlighted that CO2 emissions from fossil fuel combustion and industrial processes accounted for approximately 79% of the total increase in greenhouse gas (GHG) emissions in 2019. Importantly, approximately 35% of these emissions are absorbed by the ocean. The open ocean plays a crucial role in regulating the global climate by serving as a long-term carbon sink, that stores carbon out of contact with the atmosphere from decadal day to millennia (Boyd et al., 2019). The rising levels of atmospheric CO2 highlight the importance of ocean carbon sinks. Understanding the ocean’s capacity to absorb atmospheric CO2 and its long-term dynamic changes is essential for studying marine carbon sequestration technology and addressing global changes caused by the continuous increase in the atmospheric CO2 concentration.
In contrast to the extensive research conducted on the carbon cycle in the open ocean, relatively less attention has been given to investigating nearshore neritic ecosystems, such as mangroves, seagrasses, and coral reefs. These regions have the potential to act as carbon sinks because of their high productivity, impact on human activities, complex land-sea interaction processes, and transport of materials to the deep sea (Zhang et al., 2017). Coral reef ecosystems, which are located in tropical-subtropical neritic regions, are highly productive and biodiverse. They are commonly known as the “tropical rainforests of the ocean” (Zhao et al., 2006). Coral reefs are essential for providing valuable fishing resources, tourism opportunities, and coastal protection (Hoegh-Guldberg et al., 2019). Fishes from these reefs make up approximately 9–15% of global marine fisheries resources (Smith, 1978). Coral reefs consistently and significantly contribute to carbonate sedimentation. The CaCO3 productivity of these organisms accounts for 7–15% of the total productivity in the ocean, making them significant for the long-term removal of atmospheric CO2 (Suzuki and Kawahata, 2004). The famous “coral reef hypothesis” also attributed the sudden increase in atmospheric CO2 (~80 ppm) during the transition from ca. 14 ka preglacial to interglacial to the change in the coral reef CaCO3 deposition rate (Opdyke and Walker, 1992), indicating that there was a strong interaction between coral reefs and the change in atmospheric CO2 concentration. Therefore, it is crucial to study the carbon cycle process, regulatory mechanisms, and dynamic changes in the carbon budget of coral reefs to their impact and response to climate change.
The SCS is the largest marginal sea in tropical regions, located between latitudes 4° to 23° N and longitudes 105° to 121° E. It has a tropical or subtropical climate, a deep-sea basin at its center with a maximum depth of 4700 m, and is surrounded by extensive continental shelf systems to the north and south (Zhai et al., 2013). The SCS connected to the Western Pacific through the Luzon Strait in the northeast and to the Java Sea via the Karimata Strait in the southwest. Covering an area of 3.3 million km2, excluding the Gulf of Thailand and the Gulf of Tonkin (Morton and Blackmore, 2001), the SCS is part of the Indo-West Pacific marine biogeographic province, known for its diverse biodiversity. Positioned at the meeting point of the Pacific and Indian Ocean Basins (UNEP, 2004), the SCS serves as a key aggregation center for various species from both oceans, hosting 50 out of the world’s 70 coral generae (Tomascik et al., 1997).
Coral cover, diversity, and coral community structure determine the organic carbon production and CaCO3 production of a reef area, which in turn impacts the carbon budget of the reef area (Shi et al., 2021). Therefore, research on the carbon budget in coral reef areas in the SCS is highly important for studying the carbon cycle in the SCS.
There are more than 200 coral reef islands in the SCS, mainly distributed in the Nansha Islands, Xisha Islands, Zhongsha Islands, and Dongsha Islands. In addition, numerous fringing reefs are distributed along the coasts of the SCS (Figure 1). The area (excluding coral reefs along the Vietnamese, Malaysian, Brunei, and Philippine coasts and their offshore islands) of existing coral reefs was initially estimated to be approximately 8000 km2 (Yu, 2012). All major geomorphic types of coral reefs, such as atolls, patch or platform reefs, and fringing reefs, are distributed in the SCS (Vo et al., 2013). As the atmospheric CO2 concentration increases, seawater acidification increases concurrently, posing increasing risks to the coral reefs of the SCS (Liu et al., 2014). Understanding the carbon budget of the ocean and the impact of the carbon cycle process on ecosystems is crucial for assessing ecosystem resilience to global warming and ocean acidification. Additionally, it helps develop strategies and policies to mitigate these effects. This paper presents the initial organization of the carbon budget in coral reef areas of the SCS, provides an overview of carbon cycle research progress, and estimates the total carbon budget. We discuss the carbon budget from the two aspects of inorganic carbon and organic carbon processes (Figure 2).
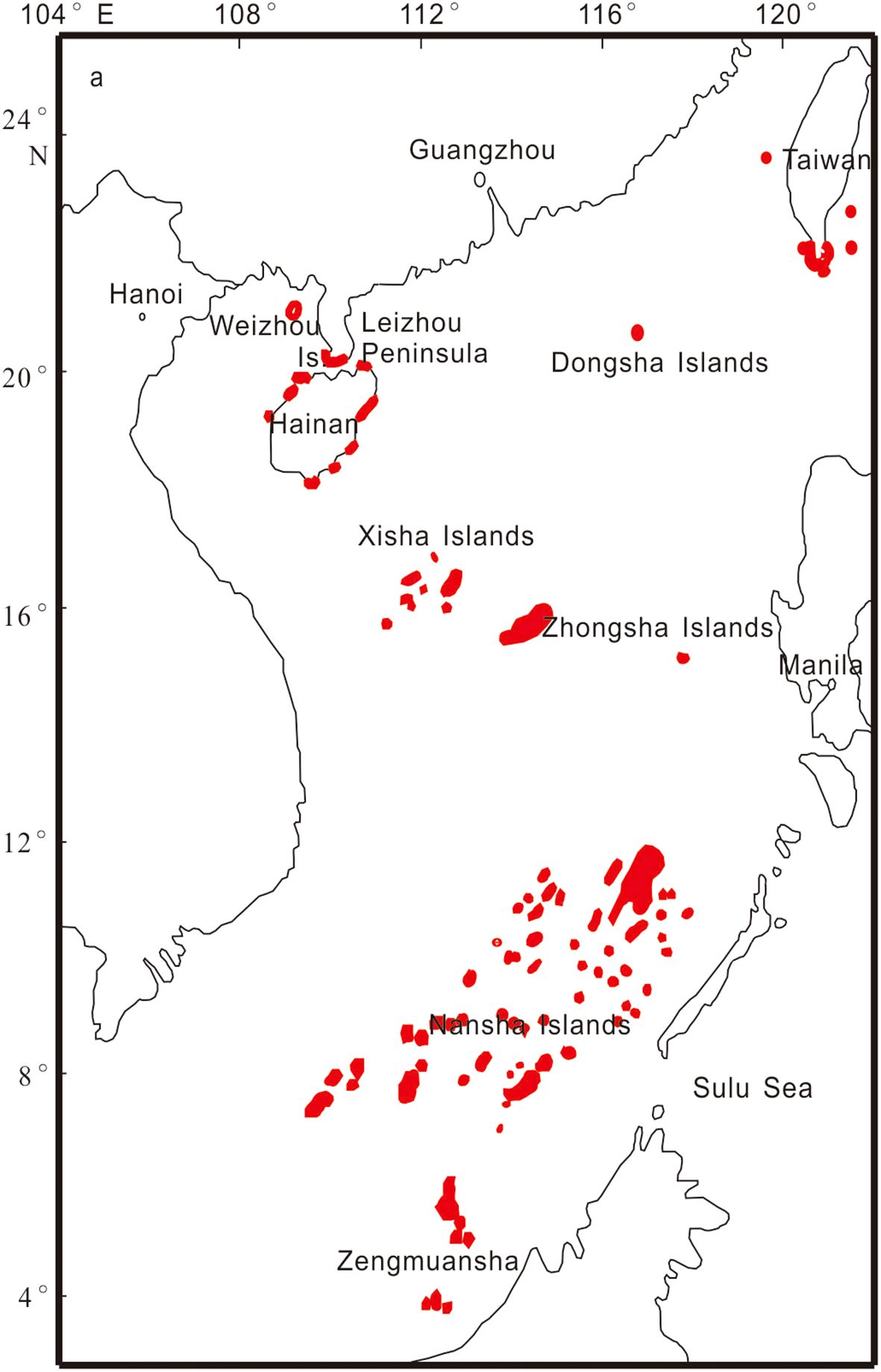
Figure 1. Distribution of coral reefs in the South China Sea (Yu and Zhao, 2009).
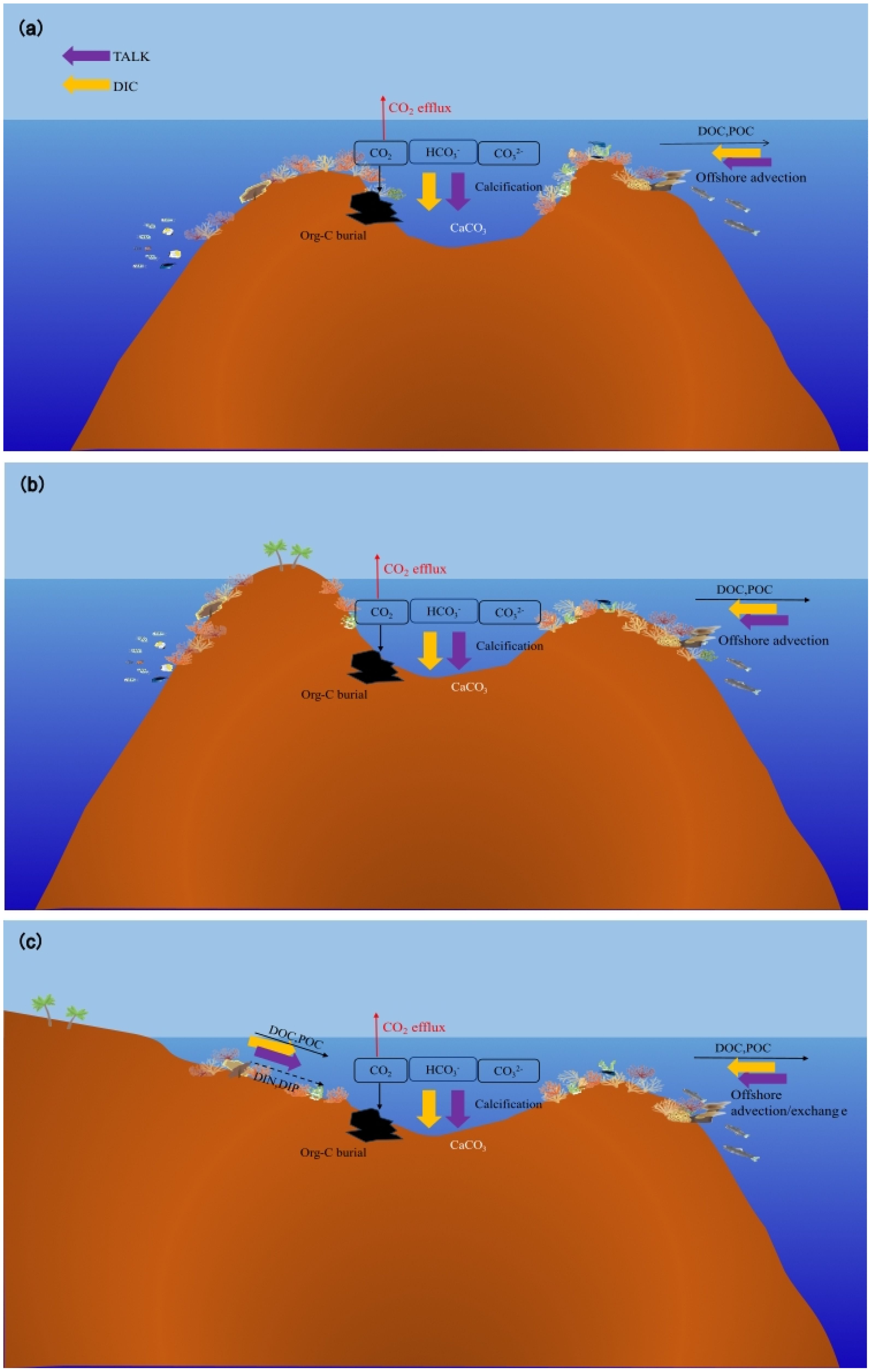
Figure 2. Schematics of the mode of carbon cycles of coral reefs modified from Suzuki et al (Suzuki and Kawahata, 2003). (A) a well flushed oceanic reef, (B) an enclosed reef, and (C) a coastal reef. TALk is the total alkalinity, and DIC is the dissolved inorganic carbon.
2 Sea-air CO2 exchange fluxes of coral reefs in the SCS
At present, research on sea-air CO2 exchange is based on the collection of seawater partial pressures of CO2 (pCO2) and atmospheric pCO2 data, and the use of these data to calculate sea-air CO2 flux using Equation 1.
where k is the gas transfer velocity of CO2 (calculated by the wind speed (u) and Schmidt number (Sc)) (Wanninkhof, 1992), and s is the solubility of CO2 in seawater (calculated by temperature and salinity) (Weiss, 1974). When F is greater than zero, CO2 is released to the atmosphere from seawater, making the sea a CO2 source; otherwise, it act as a CO2 sink. Field observations of coral reefs indicate that most coral reefs act as sources of atmospheric CO2 (Ware et al., 1992; Gattuso et al., 1999b; Fagan and Mackenzie, 2007; Yan et al., 2011; Massaro et al., 2012; Yan et al., 2016, 2018), whereas others act as sinks (Kayanne et al., 1995; Suzuki, 1998; Suzuki et al., 2001; Drupp et al., 2013; Shaw and McNeil, 2014). Therefore, whether a coral reef acts as a source or sink of atmospheric CO2 is variable.
Atmospheric pCO2 changes very little over short time scales and can be regarded as a constant value (e.g. during more than 10 cruises in the SCS, the standard deviation of atmospheric pCO2 was typically less than 3 ppmv, except for two coastal voyages influenced by terrestrial sources where atmospheric CO2 fluctuated significantly with standard deviations of 3.8 and 8.0 ppmv.) (Zhai et al., 2013). The seawater pCO2 determines whether a sea region acts as a source or sink. Seawater pCO2 is mainly determined by the dynamic equilibrium of the carbonate system in seawater, and is generally affected by temperature, water mixing and biological metabolism (Dai et al., 2009). Biological metabolism in coral reefs is the result of two major processes: organic carbon metabolism process (Equation 2) and an inorganic carbon metabolism process (Equation 3).
The sum of the net organic carbon production and net inorganic carbon production determines the CO2 budget. Crossland et al. (1991) reported that the net organic carbon production of Pacific reefs was almost zero (0 ± 0.7 g cm-2 d-1). Calcification primarily influences the sea-air CO2 flux in coral reefs. As indicated by Equation 3, one mole of CaCO3 will yield one mole of CO2. Due to the buffering effect of seawater, only approximately Ψ mol of CO2 is released (Ψ−in the marine environment, approximately Ψ mol of CO2 will be liberated per mol of CaCO3 deposited. When seawater pCO2=350 ppm, T=25°C, S=35, and Ψ=0.6, namely, the 0.6 rule) (Ware et al., 1992). According to Ware et al. (1992), global coral reefs release approximately 0.02–0.08 Gt C as CO2 annually through the production of CaCO3. This accounts for approximately 0.4–1.4% of the total CO2 emitted from fossil fuel use. Suzuki and Kawahata (2004) conducted a comparative study of various coral reefs and reported that they have various capacities as sources or sinks of atmospheric CO2. When coral reefs dominate an ecosystem, calcification becomes the primary factor influencing the seawater CO2 concentration. Coral reefs usually act as a net source of atmospheric CO2. However, when macroalgae or seagrass dominate, plant photosynthesis is greater, and the ecosystem has greater net productivity. The coral reef than acts as a net sink of atmospheric CO2. Coral reef ecosystems at different stages of ecological succession have varying dominant populations, resulting in variability in their role as sources or sinks of atmospheric CO2 (Suzuki and Kawahata, 2004).
Recent surveys of sea-air CO2 exchange in coral reefs in the SCS have shown that different coral reefs play different roles as sources/sinks of atmospheric CO2 (Table 1). The coral reefs of the Nansha Islands (Yongshu Atoll), Xisha Islands (Yongxing Islands and Yongle Atoll) and Hainan Island (Luhuitou fringing reef) have been identified as sources of atmospheric CO2. Further analysis indicated that the ratio of inorganic metabolism (calcification/dissolution) to organic carbon metabolism (photosynthesis/respiration) in these coral reefs is more than three times greater than that in the open ocean. The calcification process releases CO2, leading to an increase in the CO2 concentration in seawater, making coral reefs a source of atmospheric CO2. Dongsha Atoll, which is situated at relatively high latitudes, acts as a sink for atmospheric CO2. Tai et al. (2020) suggest that vertical mixing enhances nutrient supply, thereby improving biological productivity and CO2 absorption. The decrease in pCO2 due to CO2 absorption exceeds the increase in surface seawater pCO2 from mixing CO2-rich water mass and air-sea CO2 exchange. The various coral reef areas along the coast of South China exhibit varying roles as sources and sinks for atmospheric CO2, with marked seasonal variations. The coral reefs in this area are poorly developed, and the community structure is complex. Biological processes (photosynthesis and respiration) and mixing effects are the most important factors controlling the CO2 system (Yang et al., 2023). The air-sea CO2 fluxes in different coral reefs in the SCS are mainly affected by the coral community structure. In coral dominated reef areas, calcification leads to an increase in the seawater CO2 concentration, which makes coral reef a source of atmospheric CO2. When phytoplankton and benthic algae are dominant, coral reef areas act as sinks of atmospheric CO2 due to the consumption of CO2 by biological metabolism (Gattuso et al., 1999a, b). There is a nonlinear relationship between coral growth and sea surface temperature (SST) in the SCS. In the long term, as SST increases, coral skeleton growth transitions from increasing to decreasing linearly, with a critical SST threshold around 27.5°C (Yan et al., 2019). Coral reef areas at different latitudes in the SCS are distinguished according to the average annual SST. The zoning method mentioned above can also be applied to assess CO2 flux, as the deposition of CaCO3 in coral reef areas is directly proportional to the release of CO2 (Ware et al., 1992; Frankignoulle et al., 1994). To assess the overall CO2 budget in coral reefs of the SCS, we employed an upscaling approach by multiplying a reasonable flux value by the corresponding surface area if each coral area.
Combined with the isotherm (multiannual SST) (Yan et al., 2019) of the SCS and geomorphic characteristics of coral reefs in the SCS, the main coral reef areas in the SCS are divided into three regions, namely, the South China coast fringing reef zone (South China Coast and offshore islands), Hainan and Taiwan fringing reef zone (Hainan and offshore islands, Taiwan and offshore islands), and the atoll zone (Dongsha Islands, Zhongsha Islands, Xisha Islands, and Nansha Islands). The observation results of different geomorphic zones in the coral reef area of each region are considered reasonable flux values multiplied by the corresponding area, which is the annual flux of the geomorphic zone. The CO2 fluxes of the fringing reefs on the South China coast are -1.69-1.69 mmol cm-2d-1 (Table 1), with an area of 17 km2 (Yu and Zhao, 2009), and the calculated CO2 budget is -1.2 – 1.2 × 108 g C a-1. The same method is used to calculate the CO2 fluxes of the Hainan and Taiwan fringing reef zone, with a total area of 1120.0 km2 (Yu and Zhao, 2009), the calculated CO2 budget is approximately 1.3–4.7 × 1010 g C a-1. The CO2 fluxes of atoll reef flats and atoll lagoons are obviously different (Table 1), and are mainly influenced by differences in biological metabolism and seawater mixing (Yan et al., 2011). Research shows that coral coverage in lagoons such as Zhubi Atoll, Meiji Atoll in the Nansha Islands (Zhao et al., 2013), and Yongle Atoll in the Xisha Islands (Zhao et al., 2016) is lower compared to reef flats. This lower coverage reduces calcification and CO2 release capacity. Moreover, the depth of the atoll lagoon water in the SCS is significantly greater than that of the reef flat water (Zhao et al., 2013, 2016), resulting in a larger volume of water per unit area in the lagoon. Consequently, when biological processes absorb or release the same amount of CO2, the change in CO2 concentration in the lagoon water column is significantly lower than in the reef flat. Therefore, the CO2 fluxes of two different geomorphic types of atolls were measured separately, and the CO2 budget of the atolls was estimated using Equation 4.
The CO2 fluxes of the atoll lagoon and reef flat are 0.4-2.9 mmol C m-2 d-1 and 1.5-5.2 mmol C m-2 d-1 (Table 1), respectively, while the atoll lagoon area is 4380.6 km2, and the atoll reef flat (including fore-reef) area of the SCS is 2456.6 km2. The calculated atoll CO2 budget is 0.24 – 1.12 × 1011 g C a-1. The amount of CO2 released from coral reefs in the SCS is estimated to be 0.37–1.59 × 1011 g C a-1.
3 Calcium carbonate reservoir of coral reefs in the SCS
Coral reefs are distinct ecosystems that provide a habitat for a diverse range of organisms (Andersson and Gledhill, 2013; Eyre et al., 2014), including abundant calcifying organisms such as corals, nullipores, and foraminifera. These calcifying organisms build calcium carbonate frameworks and cement the reef together (Cornwall et al., 2021). The growth and persistence of coral reefs depend on net CaCO3 accretion, i.e. where calcification in coral areas exceeds the dissolution and erosion (Jones et al., 2015). The production of calcium carbonate by coral reefs is an important component of the global marine carbon cycle. Suzuki and Kawahata (2004) demonstrated that coral reefs, which cover less than 2.3% of global neritic carbonate regions worldwide, significantly impact CaCO3 production, contributing 32-43% of neritic and about 7-15% of global CaCO3 production. The importance of coral reefs worldwide is magnified when their role in the accumulation of CaCO3 is considered. The annual CaCO3 accumulation in coral reef areas is 0.084 Pg C, approximately 23-26% of the global CaCO3 accumulation (Shi et al., 2021). Coral reefs are significant carbonate reservoirs in the ocean.
Community metabolic (photosynthesis/respiration) impacts the alkalinity and acidity ratio of seawater, influencing the calcification rete (Mcconnaughey et al., 2000). Therefore, environmental factors such as sea water temperature, salinity, and solar radiation affect the calcification of coral skeletons by affecting coral metabolism, resulting in significant seasonal variation in skeletal density and annual growth boundaries (Shi et al., 2012). Coral skeletal extension and skeletal density estimated skeletal calcification using Equation 5. This data, along with coral cover, was then used to calculate net carbonate production (kg m−2 a−1) and gross carbonate production (t a−1) using Equations 6, 7, respectively (Shi et al., 2009).
There are many data on the skeletal extension of coral reefs in the SCS, and these data cover the main coral reef areas in the SCS. In the case that the corresponding coral skeletal density data are insufficient, since coral calcification is proportional to skeletal extension (Shi et al., 2012), it is calculated by the following formula:
SST is one of the main factors controlling coral growth. Yan et al. (2019) averaged the multi-annual SST in the SCS, and mapped the spatial distribution of multi-annual SST in the SCS. According to the spatial gradient of SST, the coral reefs in the SCS are divided into three regions: HN-TW (including Hainan Island, Dongsha Islands, and Taiwan Island), XS-ZS (including Xisha Islands and Zhongsha Islands), and NS-HY (including Nansha Islands and Huangyan Island). The annual average skeletal extension of the coral reefs in the three regions are 1.25, 1.10, and 0.91 cm a-1, respectively (Yan et al., 2019). According to Equation 8, the annual average calcification rates for the three regions are 1.77, 1.58, and 1.34 g·cm−2·a−1, respectively. Based on the existing coral coverage survey results, the CaCO3 production of coral reefs in the SCS was estimated (Table 2). According to the carbonate production in surviving coral reefs and the total area of coral reefs, coral reef CaCO3 production in the SCS is estimated to be 1.35-3.11 × 107 t a-1, which represents 0.9-4.3% of global carbonate production (Suzuki and Kawahata, 2004).
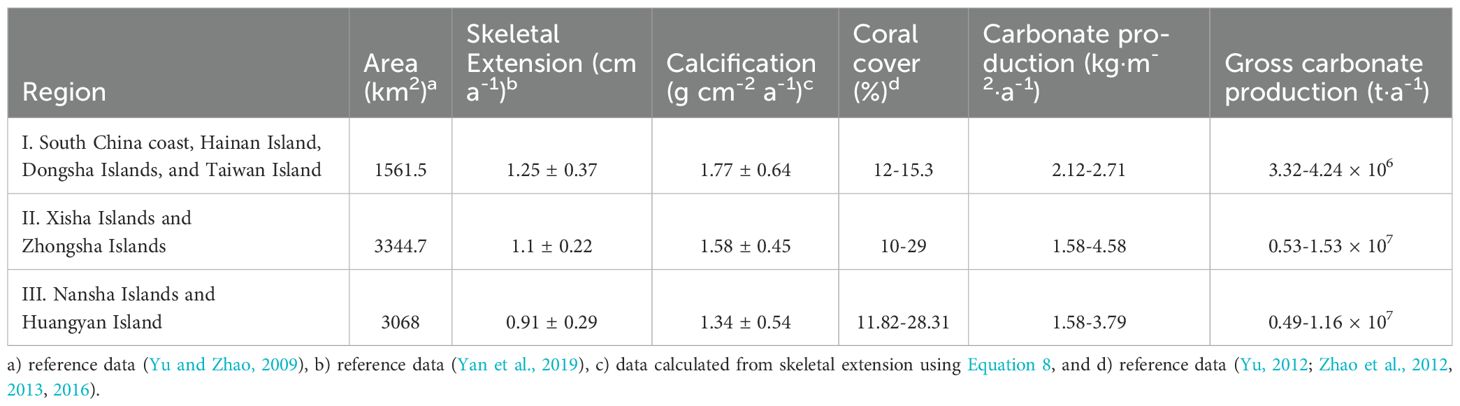
Table 2. Skeletal extension, calcification, coral cover, and carbonate production of coral reefs in the SCS.
4 Organic carbon pool and fluxes in coral reef waters in the SCS
In seawater, organic carbon (Org-C) exists as particulate organic carbon (POC) and dissolved organic carbon (DOC). POC migrates and transforms into water through a cascade of transfers within the food chain, excretion, death, and other biological processes (Hernández-León et al., 2019). DOC primarily consists of coral secretions, microbes, and other organisms smaller than 0.2 μm that are produced through biological metabolic processes (Ogawa and Tanoue, 2003). The main processes of Org-C migration and transformation include animal feeding, microbial recycling, physical sedimentation, and hydrodynamic forces (Wotton and Malmqvist, 2001). Coral reefs typically develop in shallow waters that are less than 100 m deep (Goreau et al., 1979). According to Reiswig (1981), there are no differences in the vertical distributions of POC in coral reef waters. Some Org-C is released through remineralization, animal feeding, and microbial decomposition during settlement, whereas some is exported to the ocean beyond the reef. Only a small amount of material enters the pores of coral skeletons or becomes buried by CaCO3 crystalloids (Figure 2). For example, the content of organic carbon in carbonate sand grains in a fringing reef in the northern Gulf of Aqaba is only 0.36% (Rasheed et al., 2006). It creates a stable and enduring structure. Bak and Laane (1987) analyzed coral core samples from Indonesian islands and reported that the total organic carbon content was less than 0.5 wt% (Ferrera et al., 2017). Ingalls et al. (2003) analyzed coral core samples from Florida and the Red Sea and found that the total organic carbon (TOC) contents were even lower, ranging from 0.04 to 0.11 wt% and 0.02 to 0.04 wt%, respectively, approximately 300 years ago. These findings show that although the productivity level of the coral reef area is high, the content of organic carbon that enters the sediment and remains very low is negligible compared with that associated with CaCO3 deposition. Similarly, a study conducted by Song et al. (2003) on the Zhubi Reef of the Nansha Islands showed that the POC content in settling particles was 0.03–0.05 wt%. In comparison, the POC content in the lagoon sand was even lower, ranging from only 0.01–0.02 wt%.
Due to the presence of swimming creatures and hydrodynamic forces (typically waves, tides, regional flow, wind, and buoyancy effects), there is a continuous exchange of seawater between the interior and exterior of coral reefs (Figure 2) (Rogers et al., 2017). Some reefs exhibit a net export of Org-C to the surrounding ocean (Hata et al., 1998). Delesalle et al. (1998) surveyed the Tiahura Reef, and the results showed that the mean export of organic carbon was estimated to be 0.7 g C m-2 d-1, accounting for 47% of the Org-C production of the reef. An investigation (Zheng et al., 2004) revealed that the distribution characteristics of DOC in Zhubi Reef were similar to those in Tiahura Reef, which were reef flat>lagoon>surrounding ocean. A survey (Hata et al., 1998) of the Palau Barrier Reef revealed that 7% of the gross community production(GCP) entered the lagoon, and that 4% of the GCP was exported to the open ocean. Observations (Hata et al., 2002) in the Shiraho Reef of Japan revealed that the DOC and POC fluxes from coral reefs to the open ocean were 30–36 and 5–7 mmol C m-2 d-1, respectively. Additionally, Miyajima et al. (2007) reported that the net output fluxes of DOC and POC were 15 and 2 mmol m-2 d-1, respectively, whereas Tanaka et al. (2011) reported a net DOC output flux of 12–24 mmol m-2 d-1. Hydrodynamic forces, such as wind, currents, and waves in different seasons primarily control the output of Org-C from coral reefs. The water volume in and out of lagoons (water flow between lagoons and the ocean) also changes seasonally, resulting in distinct variations in horizontal flux (Schrimm et al., 2002). Coral reefs with different geomorphologies have different reactions to hydrodynamic conditions. As shown in Figure 2, there are differences in a reef’s openness to the ocean. The width of channels for water flux in and off coral reefs varies, resulting in differences in Org-C outputs among different reefs (Charpy and Charpy-Roubaud, 1991).
Currently, quantitative studies on the biogeochemistry of Org-C in coral reefs in the SCS are lacking. The comprehensive scientific expedition of the Nansha Islands, which was conducted by the SCS Institute of Oceanology involved various research activities primarily from 1993 to 1994. Wu et al. (1997) deployed two sediment traps in the Zhubi Atoll lagoon. The results revealed that the average vertical flux of POC was estimated at 73.69(58.38 and 90.16) mg C m-2 d-1, with POC accounting for 1.78 and 1.96% of the sediment particles in the traps. Yang et al. (2011) measured the vertical flux of POC in Zhubi Reef to be 43 mg C m-2 d-1 using the 210Po/210Pb disequilibria, which is consistent with the results of Wu et al. (1997). The organic matter within coral reef sediments undergoes further degradation. Gao et al. (2023) collected multiple sediment samples at Yongle Atoll in the Xisha Islands and estimated the average burial flux of POC to be 15.24 (8.22-21.92) mg C m-2 d-1, which is significantly lower than the vertical flux of POC at Zhubi Atoll, as determined by combining sedimentation rates with dry density and TOC content. Cai et al. (1997) compared Yongshu, Chigua, Zhubi, Wufang, and Xinyi Reefs and reported that the DOC content ranged from 1.00 to 4.85 mg L-1, whereas the POC content ranged from 155.33 to 592.47 μg l-1. These values were higher than those typically observed in open oceans (ie: the DOC concentrations in the euphotic of the SCS are range from 0.66 to 1.02 mg L-1, and the POC concentrations in the euphotic of the SCS range from19.20 to 48.99 μg L-1 (Jiao et al., 2018)). Org-C is clearly exported from coral reefs to adjacent open seas. Studies estimate the POC output flux from Zhubi Reef to be 14.00 mgC m-2 d-1, accounting for 4% of the lagoon’s net primary productivity (Yang et al., 2011). This proportion is similar to other coral reef lagoons. For example, the POC exported from a barrier reef of the Palau Islands to the ocean accounts for about 4% of the total community production (Hata et al., 1998), while the POC exported from Tikehau atoll Lagoon constitutes approximately 6% of the total phytoplankton production (Charpy and Charpy-Roubaud, 1991). Current research faces challenges in accurately estimating the vertical and horizontal Org-C output fluxes from coral reefs in the SCS. Kinsey (1985) conducted a comparative analysis of organic and inorganic productivity in various coral reefs, establishing the average metabolic performance or “standard performance” of coral/algal reefs as P/R=1 ± 0.1. This suggests that net primary production is nearly equivalent to respiration in coral communities, with a minor difference, indicating a direct link between net primary production and primary production. Table 3 presents a comparison of the primary production levels in coral reefs across the SCS. Despite the unusually high primary production in Huangyan Atoll, the primary production levels in other coral reefs are relatively similar. Due to the limited availability of survey data, a crude estimate was derived using the average of existing available data to represent the SCS coral reefs. The average vertical flux of Org-C in the SCS coral reef lagoon is calculated to be 63.85 (ranging from 43.00 to 90.16) mgC m-2 d-1, with a POC burial flux of 15.24 (ranging from 8.22 to 21.92) mgC m-2 d-1, and a horizontal output flux of 14.00 mgC m-2 d-1. Considering the coral reef area of 7974.2 km2, the Org-C deposition flux in the SCS coral reef area is estimated to be approximately 1.86 × 1011 (ranging from 1.25 to 2.62 × 1011) gC a-1, with a burial flux of 4.44 × 1010 (ranging from 2.39 to 6.38 × 1010) gC a-1, and a horizontal output flux of approximately 4.07 × 1010 gC a-1.
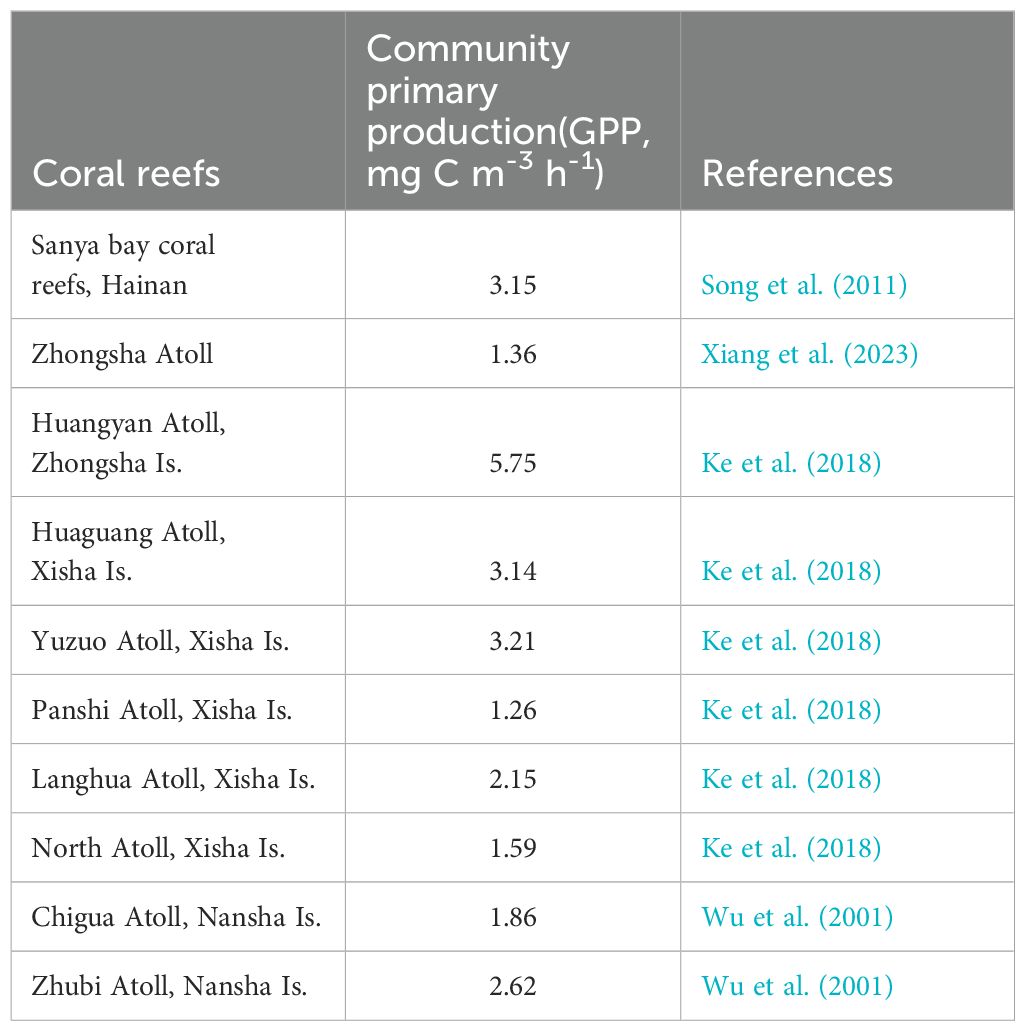
Table 3. Comparison of the planktonic community primary production (GPP, mg C m-3 h-1) of coral reefs in the SCS.
5 Carbon budget of coral reef ecosystems in the SCS
The main coral reefs in the SCS are significant sources of atmospheric CO2, releasing approximately 0.37–1.59 × 1011 g C a-1. These coral reefs also act as carbonate reservoirs, with carbonate production reaching 1.35–3.11 × 107 t a-1, equivalent to 1.62–3.74 × 1012 g C a-1. The amount of organic carbon entering the sediment and leaving the carbon cycle is approximately 4.44 × 1010 g C a-1. The amount of Org-C exported from reefs by tides or currents is approximately 4.07 × 1010 g C a-1. After POC enters the open ocean, during the sedimentation process, some Org-C is oxidized and decomposed, whereas others are ingested and enter the food chain. Only a small portion reaches deep water. For example, Hata et al. (1998) investigated the Org-C sedimentation process in the open ocean outside Palau Island and reported that only 0.6% of the POC was transferred below the thermocline (150 m depth). If we consider both carbonate and Org-C, the carbon storage in coral reefs in the SCS is estimated to be approximately 1.66–3.78 × 1012 g C a-1. However, these estimates are based on limited data, making them rough and of limited usefulness. In the future, it will be necessary to improve the monitoring of sea-air CO2 exchange in coral reefs of the SCS. This can be achieved by developing automatic observation systems and gathering long-term monitoring data. Continuous monitoring of environmental parameters allows accurate assessment of the annual contribution of coral reefs and the environmental impacts of global climate change on coral reef carbon flux. We introduce new methods and tools to study calcification and organic carbon processes. Our goal is to explore the potential for carbon storage and enhance the model of carbon sink enhancement for coral reefs in the SCS.
Author contributions
HY: Writing – original draft, Data curation. QS: Writing – review & editing, Data curation. LX: Investigation, Writing – review & editing. HZ: Investigation, Writing – review & editing. MZ: Investigation, Writing – review & editing. ST: Data curation, Writing – review & editing.
Funding
The author(s) declare financial support was received for the research, authorship, and/or publication of this article. Guangdong Basic and Applied Basic Research Foundation (No. 2021A1515011402), the National Natural Sciences Foundation of China (Grant Nos. 41776128, 42276070, 42376167, and 42376165), and the Key Technologies Research and Development Program of China (No. 2021YFC3100602).
Acknowledgments
The authors thank the Hainan Tropical Marine Biology Research Station of the Chinese Academy of Sciences for providing support with the fieldwork. We are grateful to associate editor Prof. Hajime Kayanne and reviewers for their constructive comments and suggestions that have helped improve this paper substantially.
Conflict of interest
The authors declare that the research was conducted in the absence of any commercial or financial relationships that could be construed as a potential conflict of interest.
Publisher’s note
All claims expressed in this article are solely those of the authors and do not necessarily represent those of their affiliated organizations, or those of the publisher, the editors and the reviewers. Any product that may be evaluated in this article, or claim that may be made by its manufacturer, is not guaranteed or endorsed by the publisher.
References
Andersson A. J., Gledhill D. (2013). Ocean acidification and coral reefs: effects on breakdown, dissolution, and net ecosystem calcification. Annu. Rev. Mar. Sci. 5, 321–348. doi: 10.1146/annurev-marine-121211-172241
Bak R., Laane R. (1987). Annual black bands in skeletons of reef corals (Scleractinia). Mar. Ecol. Prog. 38, 169–175. doi: 10.3354/meps038169
Boyd P. W., Claustre H., Levy M., Siegel D. A., Weber T. (2019). Multi-faceted particle pumps drive carbon sequestration in the ocean. Nature 568, 327–335. doi: 10.1038/s41586-019-1098-2
Cai Y., Han W., Wu L. (1997). “Research of organic carbon of in coral reef lagoon of Nansha islands(in Chinese),” in The Nansha Islands comprehensive scientific expedition of the Chinese Academy of Sciences (ed.), Researches on chemistry and biology in coral reef lagoons of Nansha islands. (Beijing: Science Press).
Charpy L., Charpy-Roubaud C. J. (1991). Particulate organic matter fluxes in a Tuamotu atoll lagoon (French Polynesia). Mar. Ecol. Prog. Ser. 71, 53–63. doi: 10.3354/meps071053
Cornwall C. E., Comeau S., Kornder N. A., Perry C. T., van Hooidonk R., DeCarlo T. M., et al. (2021). Global declines in coral reef calcium carbonate production under ocean acidification and warming. Proc. Natl. Acad. Sci. 118, e2015265118. doi: 10.1073/pnas.2015265118
Crossland C. J., Hatcher B. G., Smith S. V. (1991). Role of coral reefs in global ocean production. Coral Reefs 10, 55–64. doi: 10.1007/BF00571824
Dai M., Lu Z., Zhai W., Chen B., Cao Z., Zhou K., et al. (2009). Diurnal variations of surface seawater pCO2 in contrasting coastal environments. Anglais 54, 735–745. doi: 10.4319/lo.2009.54.3.0735
Delesalle B., Buscail R., Carbonne J., Courp T., Dufour V., Heussner S., et al. (1998). Direct measurements of carbon and carbonate export from a coral reef ecosystem (Moorea Island, French Polynesia). Coral Reefs 17, 121–132. doi: 10.1007/s003380050106
Drupp P., De Carlo E., Mackenzie F., Sabine C., Feely R., Shamberger K. (2013). Comparison of CO2 dynamics and air–sea gas exchange in differing tropical reef environments. Aquat Geochem. 19, 371–397. doi: 10.1007/s10498-013-9214-7
Eyre B. D., Andersson A. J., Cyronak T. (2014). Benthic coral reef calcium carbonate dissolution in an acidifying ocean. Nat. Climate Change 4, 969–976. doi: 10.1038/nclimate2380
Fagan K. E., Mackenzie F. T. (2007). Air-sea CO2 exchange in a subtropical estuarine-coral reef system, Kaneohe Bay, Oahu, Hawaii. Mar. Chem. 106, 174–191. doi: 10.1016/j.marchem.2007.01.016
Ferrera C. M., Jacinto G. S., Chen C.-T. A., San Diego-McGlone M. L., Datoc M. F. K. T., Lagumen M. C. T., et al. (2017). Carbonate parameters in high and low productivity areas of the Sulu Sea, Philippines. Mar. Chem. 195, 2–14. doi: 10.1016/j.marchem.2017.08.005
Frankignoulle M., Canon C., Gattuso J. P. (1994). Marine calcification as a source of carbon dioxide: Positive feedback of increasing atmospheric CO2. Limnol. Oceanogr. 39, 458–462. doi: 10.4319/lo.1994.39.2.0458
Friedlingstein P., O’sullivan M., Jones M. W., Andrew R. M., Gregor L., Hauck J., et al. (2022). Global carbon budget 2022. Earth Syst. Sci. Data Discuss. 2022, 1–159. doi: 10.5194/essd-14-4811-2022
Gao J., Yu K., Xu S., Huang X., Chen B., Wang Y. (2023). Content and source analysis of organic carbon in the outer slope sediments of the Yongle Atoll, Xisha Islands (In Chinese). J. Trop. Oceanogr. 43, 131–145. doi: 10.11978/2023112
Gattuso J. P., Allemand D., Frankignoulle M. (1999a). Photosynthesis and calcification at cellular, organismal and community levels in coral reefs: a review on interactions and control by carbonate chemistry. Amer. Zool. 39, 160–183. doi: 10.1093/icb/39.1.160
Gattuso J. P., Frankignoulle M., Smith S. V. (1999b). Measurement of community metabolism and significance in the coral reef CO2 source-sink debate. Proc. Natl. Acad. Sci. U.S.A. 96, 13017–13022. doi: 10.1073/pnas.96.23.13017
Goreau T. F., Goreau N. I., Goreau T. J. (1979). Corals and coral reefs. Sci. Am. 241, 124–137. doi: 10.1038/scientificamerican0879-124
Hata H., Kudo S., Yamano H., Kurano N., Kayanne H. (2002). Organic carbon flux in Shiraho coral reef (Ishigaki Island, Japan). Mar. Ecol.-progress Ser. 232, 129–140. doi: 10.3354/meps232129
Hata H., Suzuki A., Maruyama T., Kurano N., Miyachi S., Ikeda Y., et al. (1998). Carbon flux by suspended and sinking particles around the barrier reef of Palau, western Pacific. Limnol. Oceanogr. 43, 1883–1893. doi: 10.4319/lo.1998.43.8.1883
Hernández-León S., Olivar M. P., Fernández de Puelles M. L., Bode A., Castellón A., López-Pérez C., et al. (2019). Zooplankton and micronekton active flux across the tropical and subtropical Atlantic Ocean. Front. Mar. Sci. 6, 535. doi: 10.3389/fmars.2019.00535
Hoegh-Guldberg O., Pendleton L., Kaup A. (2019). People and the changing nature of coral reefs. Region. Stud. Mar. Sci. 30, 100699. doi: 10.1016/j.rsma.2019.100699
Ingalls A. E., Lee C., Druffel E. R. M. (2003). Preservation of organic matter in mound-forming coral skeletons. Geochim. cosmochim. Acta 67, 2827–2841. doi: 10.1016/S0016-7037(03)00079-6
Jiao N., Liang Y., Zhang Y., Liu J., Zhang Y., Zhang R., et al. (2018). Carbon pools and fluxes in the China Seas and adjacent oceans. Sci. China(Earth Sciences) 61, 5–33. doi: 10.1007/s11430-018-9190-x
Jones N. S., Ridgwell A., Hendy E. J. (2015). Evaluation of coral reef carbonate production models at a global scale. Biogeosciences 12, 1339–1356. doi: 10.5194/bg-12-1339-2015
Kayanne H., Suzuki A., Saito H. (1995). Diurnal changes in the partial pressure of carbon dioxide in coral reef water. Science 269, 214. doi: 10.1126/science.269.5221.214
Ke Z., Tan Y., Huang L., Liu H., Liu J., Jiang X., et al. (2018). Spatial distribution patterns of phytoplankton biomass and primary productivity in six coral atolls in the central South China Sea. Coral Reefs. 37, 919–927. doi: 10.1007/s00338-018-1717-7
Kinsey D. (1985). Metabolism, calcification and production: I systems level studies. Proc. Fifth Inter. Coral Reef Congr. 4, 503–542.
Lee H., Calvin K., Dasgupta D., Krinner G., Mukherji A., Thorne P., et al. (2023). Climate change 2023: Synthesis Report. (Geneva, Switzerland: IPCC).
Liu T. (2002). Global changes and sustainability science (in Chinese). Earth Sci. Front. 9, 1–9. doi: 10.3321/j.issn:1005-2321.2002.01.001
Liu Y., Peng Z., Zhou R., Song S., Liu W., You C.-F., et al. (2014). Acceleration of modern acidification in the South China Sea driven by anthropogenic CO2. Sci. Rep. 4, 5148. doi: 10.1038/srep05148
Loucks D. P. (2021). “Impacts of climate change on economies, ecosystems, energy, environments, and human equity: A systems perspective,” in The impacts of climate change. Ed. Letcher T. M. (Netherlands: Elsevier), 19–50. doi: 10.1016/B978-0-12-822373-4.00016-1
Massaro R. F. S., De Carlo E. H., Drupp P. S., Mackenzie F. T., Jones S. M., Shamberger K. E., et al. (2012). Multiple factors driving variability of CO2 exchange between the ocean and atmosphere in a tropical coral reef environment. Aquat Geochem. 18, 357–386. doi: 10.1007/s10498-012-9170-7
Mcconnaughey T. A., Adey W. H., Small A. M. (2000). Community and environmental influences on reef coral calcification. Limnol. Oceanogr. 45, 1667–1671. doi: 10.4319/lo.2000.45.7.1667
Miyajima T., Hata H., Yu U., Kayanne H., Koike I. (2007). Distribution and partitioning of nitrogen and phosphorus in a fringing reef lagoon of Ishigaki Island, northwestern Pacific. Mar. Ecol. Prog. 341, 45–57. doi: 10.3354/meps341045
Morton B., Blackmore G. (2001). South China sea. Mar. pollut. Bull. 42, 1236–1263. doi: 10.1016/S0025-326X(01)00240-5
Ogawa H., Tanoue E. (2003). Dissolved organic matter in oceanic waters. J. Oceanogr. 59, 129–147. doi: 10.1023/A:1025528919771
Opdyke B. N., Walker J. C. G. (1992). Return of the coral reef hypothesis: Basin to shelf partitioning of CaCO3 and its effect on atmospheric CO2. Geology 20, 733–736. doi: 10.1130/0091-7613(1992)020<0733:ROTCRH>2.3.CO;2
Rasheed M., Wild C., Jantzen C., Badran M. (2006). Mineralization of particulate organic matter derived from coral-reef organisms in reef sediments of the Gulf of Aqaba. Chem. Ecol. 22, 13–20. doi: 10.1080/02757540500456823
Reiswig H. M. (1981). Particulate organic carbon of bottom boundary and submarine cavern waters of tropical coral reefs. Mar. Ecol. Prog. Ser. 5, 129–133. doi: 10.3354/meps005129
Rogers J. S., Monismith S. G., Fringer O. B., Koweek D. A., Dunbar R. B. (2017). A coupled wave-hydrodynamic model of an atoll with high friction: Mechanisms for flow, connectivity, and ecological implications. Ocean Model. 110, 66–82. doi: 10.1016/j.ocemod.2016.12.012
Schrimm M., Heussner S., Buscail R. (2002). Seasonal variations of downward particle fluxes in front of a reef pass (Moorea Island, French Polynesia). Oceanol. Acta 25, 61–70. doi: 10.1016/S0399-1784(02)01182-9
Shaw E. C., McNeil B. I. (2014). Seasonal variability in carbonate chemistry and air–sea CO2 fluxes in the southern Great Barrier Reef. Mar. Chem. 158, 49–58. doi: 10.1016/j.marchem.2013.11.007
Shi Q., Yu K., Chen T., Zhang H., Zhao M., Yan H. (2012). Two centuries-long records of skeletal calcification in massive Porites colonies from Meiji Reef in the southern South China Sea and its responses to atmospheric CO2 and seawater temperature. Sci. China Earth Sci. 55, 1–12. doi: 10.1007/s11430-011-4320-0
Shi Q., Zhao M., Zhang Q., Yu K. F., Chen T., Li S., et al. (2009). Estimate of carbonate production by scleractinian corals at Luhuitou fringing reef, Sanya, China. Chin. Sci. Bull. 54, 696–705. doi: 10.1007/s11434-008-0533-9
Shi T., Zheng X., Zhang H., Wang Q., Zhong X. (2021). Coral reefs: potential blue carbon sinks for climate change mitigation. Bull. Chin. Acad. Sci. 36, 270–278. doi: 10.16418/j.issn.1000-3045.20210217102
Smith S. V. (1978). Coral-reef area and the contributions of reefs to processes and resources of the world’s oceans. Nature 273, 225–226. doi: 10.1038/273225a0
Song J., Zhao W., Li P., Lv X. (2003). Carbon cycling in Nansha coral reef ecosystem, South China Sea(in chinese). Oceanol. Limnol. Sin. 34, 586–592. doi: 10.3321/j.issn:0029-814X.2003.06.002
Song X., Tan Y., Huang L., Dong J., Zhang J., Wang H., et al. (2011). Primary production in Sanya Bay during spring and summer (in Chinese). Mar. Environ. Sci. 30, 19–23. doi: 10.3969/j.issn.1007-6336.2011.01.004
Suzuki A. (1998). Combined effects of photosynthesis and calcification on the partial pressure of carbon dioxide in seawater. J. oceanogr. 54, 1–7. doi: 10.1007/BF02744376
Suzuki A., Kawahata H. (2003). Carbon budget of coral reef systems: an overview of observations in fringing reefs, barrier reefs and atolls in the Indo-Pacific regions. Tellus B 55, 428–444. doi: 10.3402/tellusb.v55i2.16761
Suzuki A., Kawahata H. (2004). “Reef water CO2 system and carbon production of coral reefs: Topographic control of system-level performance,” in Global environmental change in the ocean and on Land. Ed. Shiyomi M. (Terrapub, Tokyo), 229–248.
Suzuki A., Kawahata H., Ayukai T., Goto K. (2001). The oceanic CO2 system and carbon budget in the Great Barrier Reef, Australia. Geophys. Res. Lett. 28, 1243–1246. doi: 10.1029/2000GL011875
Tai J.-H., Chou W.-C., Hung C.-C., Wu K.-C., Chen Y.-H., Chen T.-Y., et al. (2020). Short-term variability of biological production and CO2 system around Dongsha atoll of the northern South China sea: impact of topography-flow interaction. Front. Mar. Sci. 7, 511. doi: 10.3389/fmars.2020.00511
Tanaka Y., Miyajima T., Watanabe A., Nadaoka K., Yamamoto T., Ogawa H. (2011). Distribution of dissolved organic carbon and nitrogen in a coral reef. Coral Reefs 30, 533–541. doi: 10.1007/s00338-011-0735-5
Tomascik T., AJ Mah A. J., Nontji A., Moosa M. K. (1997). The ecology of the Indonesian seas (Oxford: Oxford University Press).
Vo S. T., Pernetta J. C., Paterson C. J. (2013). Status and trends in coastal habitats of the South China Sea. Ocean Coast. Manage. 85, 153–163. doi: 10.1016/j.ocecoaman.2013.02.018
Wanninkhof R. (1992). Relationship between wind speed and gas exchange over the ocean. J. Geophys. Res. 97, 7373–7382. doi: 10.1029/92JC00188
Ware J. R., Smith S. V., Reaka-Kudla M. L. (1992). Coral reefs: sources or sinks of atmospheric CO2? Coral Reefs 11, 127–130. doi: 10.1007/BF00255465
Weiss R. F. (1974). Carbon dioxide in water and seawater: the solubility of a non-ideal gas. Mar. Chem. 2, 203–215. doi: 10.1016/0304-4203(74)90015-2
Wotton R. S., Malmqvist B. (2001). Feces in aquatic ecosystems: feeding animals transform organic matter into fecal pellets, which sink or are transported horizontally by currents; these fluxes relocate organic matter in aquatic ecosystems. BioScience 51, 537–544. doi: 10.1641/0006-3568(2001)051[0537:FIAE]2.0.CO;2
Wu L., Wang H., Li P., Song J. (1997). “Vertical flux of the settling particulate matter in coral reef lagoon of the Nansha islands (in Chinese),” in The Nansha Islands comprehensive scientific expedition of the Chinese Academy of Sciences (ed.), Researches on chemistry and biology in coral reef lagoons of Nansha islands. Beijing: China Ocean Press.
Wu C., Zhang J., Huang L. (2001). Primary productivity in some coral reef lagoons and their adjacent sea areas of Nansha Islands in spring (in Chinese). J. Trop. Oceanogr. 20, 59–67.
Xiang C., Zhong Y., Li G., Song X., Huang Y., Wang Z., et al. (2023). Planktonic carbon metabolism of an underwater coral atoll in the oligotrophic sea: a case study of Zhongsha Atoll, Central South China Sea. Front Mar Sci. 10, 1296052. doi: 10.3389/fmars.2023.1296052
Yan H., Shi Q., Yu K., Tao S., Yang H., Liu Y., et al. (2019). Regional coral growth responses to seawater warming in the South China Sea. Sci. Total Environ. 670, 595–605. doi: 10.1016/j.scitotenv.2019.03.135
Yan H., Yu K., Shi Q., Lin Z., Zhao M., Tao S., et al. (2018). Air-sea CO2 fluxes and spatial distribution of seawater pCO2 in Yongle Atoll, northern-central South China Sea. Continent. Shelf Res. 165, 71–77. doi: 10.1016/j.csr.2018.06.008
Yan H., Yu K., Shi Q., Tan Y., Liu G., Zhao M., et al. (2016). Seasonal variations of seawater pCO2 and sea-air CO2 fluxes in a fringing coral reef, northern South China Sea. J. Geophys. Res.: Oceans 121, 998–1008. doi: 10.1002/2015JC011484
Yan H., Yu K., Shi Q., Tan Y., Zhang H., Zhao M., et al. (2011). Coral reef ecosystems in the South China Sea as a source of atmospheric CO2 in summer. Chinese Sci. Bull. 56, 676–684. doi: 10.1007/s11434-011-4372-8
Yang W., Huang Y., Chen M., Qiu Y., Li H., Zhang L. (2011). Carbon and nitrogen cycling in the Zhubi coral reef lagoon of the South China Sea as revealed by 210Po and 210Pb. Mar. pollut. Bull. 62, 905–911. doi: 10.1016/j.marpolbul.2011.02.058
Yang B., Zhang Z., Xie Z., Chen B., Zheng H., Liao B., et al. (2023). Seasonal controls of seawater CO2 systems in subtropical coral reefs: A case study from the eastern coast of Shenzhen, China. water 15, 4124. doi: 10.3390/w15234124
Yu K. (2012). Coral reefs in the South China Sea: Their response to and records on past environmental changes. Sci. China Earth Sci. 55, 1217–1229. doi: 10.1007/s11430-012-4449-5
Zhai W., Dai M., Chen B., Guo X., Li Q., Shang S., et al. (2013). Seasonal variations of sea-air CO2 fluxes in the largest tropical marginal sea (South China Sea) based on multiple-year underway measurements. Biogeosciences 10, 7775–7791. doi: 10.5194/bg-10-7775-2013
Zhang Y., Zhao M. X., Cui Q., Wei F., Jiaguo Q. I., Chen Y., et al. (2017). Processes of coastal ecosystem carbon sequestration and approaches for increasing carbon sink. Sci. China: Earth Sci. 60, 809–820. doi: 10.1007/s11430-016-9010-9
Zhao M., Yu K., Shi Q., Chen T., Zhang H., Chen T. (2013). Coral communities of the remote atoll reefs in the Nansha Islands, southern South China Sea. Environ. Monit. Assess. 185, 7381–7392. doi: 10.1007/s10661-013-3107-5
Zhao M., Yu K., Shi Q., Yang H., Riegl B., Zhang Q., et al. (2016). The coral communities of Yongle atoll: status, threats and conservation significance for coral reefs in South China Sea. Mar. Freshw. Res. 67, 1888–1896. doi: 10.1071/MF15110
Zhao M., Yu K., Zhang Q. (2006). Review on coral reefs biodiversity and ecological function (in Chinese). Acta ecol. Sin. 26, 186–194. doi: 10.3321/j.issn:1000-0933.2006.01.025
Zhao M., Yu K., Zhang Q., Shi Q., Price G. J. (2012). Long-term decline of a fringing coral reef in the Northern South China Sea. J. Coast. Res. 28, 1088–1099. doi: 10.2112/JCOASTRES-D-10-00172.1
Keywords: South China Sea, coral reefs, carbon budget, CO2 flux, CaCO3, organic carbon
Citation: Yan H, Shi Q, Xu L, Zhang H, Zhao M and Tao S (2024) Carbon budgets of coral reef ecosystems in the South China Sea. Front. Mar. Sci. 11:1335662. doi: 10.3389/fmars.2024.1335662
Received: 09 November 2023; Accepted: 20 August 2024;
Published: 06 September 2024.
Edited by:
Hajime Kayanne, The University of Tokyo, JapanReviewed by:
Shigeki Wada, University of Tsukuba, JapanRick Stafford, Bournemouth University, United Kingdom
Copyright © 2024 Yan, Shi, Xu, Zhang, Zhao and Tao. This is an open-access article distributed under the terms of the Creative Commons Attribution License (CC BY). The use, distribution or reproduction in other forums is permitted, provided the original author(s) and the copyright owner(s) are credited and that the original publication in this journal is cited, in accordance with accepted academic practice. No use, distribution or reproduction is permitted which does not comply with these terms.
*Correspondence: Qi Shi, shiqi@scsio.ac.cn; Shichen Tao, taoshichen@scsio.ac.cn