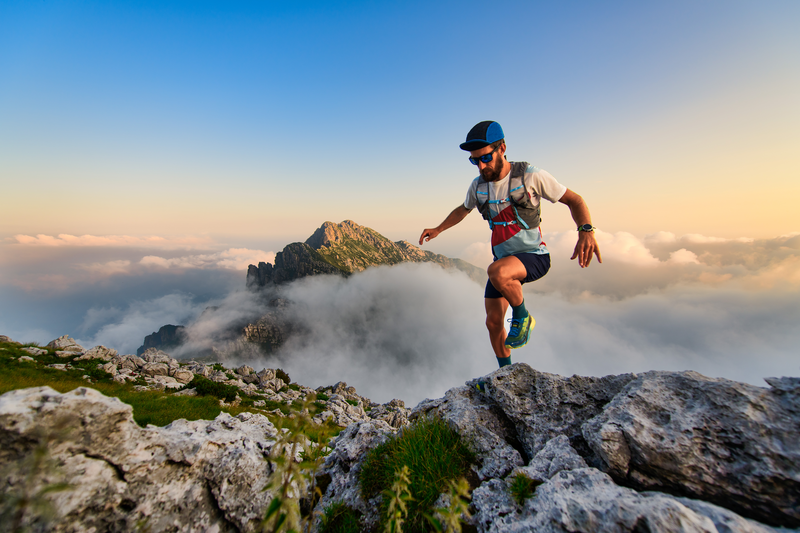
95% of researchers rate our articles as excellent or good
Learn more about the work of our research integrity team to safeguard the quality of each article we publish.
Find out more
ORIGINAL RESEARCH article
Front. Mar. Sci. , 12 March 2024
Sec. Marine Pollution
Volume 11 - 2024 | https://doi.org/10.3389/fmars.2024.1334237
Plastic additives include a wide range of pollutants, added throughout the production process of plastics aiming to improve their properties. Given that they are not chemically bound to the plastic items, they can easily migrate in the marine environment allowing their uptake by marine organisms and accumulation in their tissues. Representatives of Phthalic acid esters (PAEs) and bisphenols have been characterized for their ability to impact not only marine organisms but also humans via fish and seafood consumption. In this study, a liquid-liquid method was applied to determine the concentrations of selected PAEs and Bisphenol A (BPA) in the tissues of E.encrasicolus, S.pilchardus, B.boops, and M.barbatus from two important divisions of fisheries (North Aegean and the Western Ionian Seas, thereof NAS and IOS respectively) of the Mediterranean Sea. The level of contamination varied among the different species and geographical locations. DEHP (bis(2-ethylhexyl) phthalate) had the highest mean concentrations of the examined PAEs for all four species examined. Statistically significant differences in DEHP mean concentrations were observed between the tissues of B.boops (collected from NAS) and S.pilchardus (from IOS) (p < 0.05, Wilcoxon rank test). DIDP (di-isodecyl phthalate) was the following most common PAEs, although no statistically significant differences were presented between sites, species, and tissues. DINP (di-isononyl phthalate) was recorded only in the GIT of E.encrasicolus (from NAS), while the rest of the examined PAEs were presented in a smaller subset of the samples. Noticeably, the parent diester DBP and the metabolic monoester MNBP were concurrently detected in a part of the analyzed samples. The level of risk via fish consumption, based on the Estimated Daily Intake and the Target Hazard Quotient (THQ), showed that the PAEs and BPA had a low likelihood of a negative effect occurring for every scenario that was examined, although THQ for BPA indicated there was a higher likelihood than PAEs of an adverse effect to be presented. The results of this study highlighted the need for future efforts focusing on the factors affecting plastic additives occurrences in the marine environment, especially for marine organisms intended for consumption.
The last decades’ rapidly growing production and demand for plastics, combined with the lack of sufficient waste management procedures led to the recognition of the hazard, that plastic pollution can pose to marine wildlife (e.g., UNEP, 2016; Alimi et al., 2018). Among the different regions of the world’s ocean, the Mediterranean Sea is considered one of the most impacted areas by plastics, as due to its semi-enclosed structure tends to hold on the plastics ending up in the basin (Eriksen et al., 2014; Cózar et al., 2015). Efforts made to estimate the plastics’ quantity that enters the Mediterranean Sea and to simulate their pathways and fate have led to considerably varying results (e.g., Jambeck et al., 2015; Liubartseva et al., 2018; Kaandorp et al., 2020). More recently, Tsiaras et al. (2021), taking into account only major land-based sources of plastics, have estimated the total annual plastics load at ~17,600 tons and the total amount of plastics floating on the surface layer and in the water column of the Mediterranean at 3,842 tons. Accumulation of floating debris has been found to form areas with high concentrations of plastics (Tsiaras et al., 2021; Baudena et al., 2022), which are favored by anticyclonic circulation features (Tsiaras et al., 2021). The total plastic load in the Mediterranean is considered comparable to that in the oceanic subtropical gyres (Cózar et al., 2015; Suaria et al., 2016).
From the early stages of plastics usage, their properties, mainly the durability, and persistence to external conditions, led to their adoption in a wide range of applications but also raised concerns about their impact on marine organisms (e.g., Andrady and Neal, 2009; Andrady, 2015; Lusher et al., 2015). Plastics are commonly formulated with different chemical additives aiming to upgrade their performance and appearance, categorized as plasticizers, fire retardants, and colorants (Andrady and Neal, 2009; Andrady, 2011). More than 3.200 chemical substances used as plastic additives and processing aids have been considered as substances of potential concerns (Aurisano et al., 2021; Wiesinger et al., 2021; UNEP and BRS Secretariat et al., 2023). Phthalic acid esters (PAEs), commonly known as phthalates, are the most frequently used group among plasticizers and can consist of up to 60% of the product’s quantity (Net et al., 2015). PAEs, are high-molecular-weight substances usually added to plastics to improve their longevity and flexibility (Andrady and Neal, 2009). Depending on the length of the side chains, PAEs dissolve in water to varying degrees, and the partition coefficient octanol/water (Kow) provides an estimate of the hydrophobicity of a given molecule (Savoca et al., 2023). Through plastics breakdown, the plastic additives can easily migrate out of plastics and disperse in the marine environment, as they are not chemically bound to the polymer matrix (Andrady, 2011; Net et al., 2015). Once absorbed by biota, degradation and metabolization of PAEs can occur, while in case of mammals the diesters of phthalic acid undergo a two-phase biotransformation. The first phase that comprises hydrolysis e.g. monoesters formation is followed by conjugation and consequent excretion (Calafat et al., 2006; Savoca et al., 2023), however the metabolism of PAEs for fish species is not yet well described (Hu et al., 2016).
Although PAEs are not considered persistent compounds, their immense production has led to an excessive and constant supply of PAEs in the marine environment, making them ubiquitous contaminants (Net et al., 2015). High concentrations of PAEs have been found in the upper layer of the water column, suggesting that the process of plastic degradation may control the presence of PAEs. However, there was not always a strong statistical correlation between plastic abundances and PAE concentrations, implying that they may not be a good indicator of each other (Paluselli et al., 2018a; Schmidt et al., 2021). High concentrations of PAEs have also been detected in bottom water layers and were correlated with chemical diffusion from PAEs-rich sediments, or sediment resuspension/bioturbation processes (Paluselli et al., 2018b).
Among plastic additives, bisphenols are another commonly applied group, generally used in the production of polycarbonate plastics, epoxy resins, food and beverages packaging with bisphenol A (BPA) being the most prevalently used compound (Hermabessiere et al., 2017; Kim et al., 2019). Similar to phthalates, BPA is used in PVC production acting as an antioxidant and heat stabilizer substance (Wang et al., 2021). It is estimated that BPA annual manufactured and imported quantities largely exceed the one million tons for the European countries (ECHA, 2023). BPA has become pervasive in the marine environment, as the increasing demand of the substance has led to the rise of BPA production and therefore its continuous leaching in the natural environment, mainly through wastewater discharges, effluents of the manufacture processing (Klečka et al., 2009; Corrales et al., 2015), and release from BPA-containing products (Flint et al., 2012).
Several PAEs are characterized as substances of concern and BPA as one of highly concern based on their ability to prompt negative effect on human health (ECHA, 2018; Aurisano et al., 2021). The concentration of both PAEs and BPA has been reported in the tissue of several marine organisms, as zooplankton, fish, bivalves, holothurians, and sea turtles in the Mediterranean Sea (e.g. Guerranti et al., 2016; Cerkvenik-Flajs et al., 2018; Salvaggio et al., 2019; Schmidt et al., 2021; Blasi et al., 2022; Rios-Fuster et al., 2022). PAEs and BPA, both have been characterized as endocrine-disrupting chemicals (EDCs) (Hermabessiere et al., 2017). EDCs are able to interfere with the endocrine system of the organism and cause abnormalities in hormones regulated functions such as growth, reproduction, metabolism, etc (WHO, 2012). The risk that EDCs pose is not limited to individual marine organisms but is also expanding to the level of organisms’ population as they have been reported to affect the reproduction (such as fertility rates, ratio between sexes, imposex responses etc.) with the risk of declining or collapsing (Fernadez, 2019; Langston, 2020). PAEs and BPA can accumulate in the human body through the ingestion of contaminated food and the uptake from airborne exposure (inhalation and dermal absorption), thus the level of exposure to risk via the different pathways is under regulatory monitoring and tolerable limits of their intake by humans have been set (EFSA, 2014; 2020; 2012).
Marine fishes are an essential nutritional source for human diet by providing high-quality proteins, amino acids and vitamins, but also constitute a fundamental resource for the majority of fisheries and aquaculture activities (FAO, 2022). The current research focused on four marine fish, Engraulis encrasicolus (Linnaeus, 1758), Sardina pilchardus (Walbaum, 1792), Mullus barbatus (Linnaeus, 1758), and Boops boops (Linnaeus, 1758) of the Mediterranean Sea to better understand the extend of plastic additives pollution. The selection of the species was based on (i) their commerciality; and (ii) the habitat that they occur. The species occupy the continental shelves of Mediterranean Sea (Froese and Pauly, 2023) and are commercially important fisheries products for the basin (Eurostat, 2023). S.pilchardus and E.encrasicolus are co-occurring pelagic species presenting differences in their feeding preferences (van der Lingen et al., 2009). These small pelagic fish are the most fished in the region, accounting for 40% of total Mediterranean catches (Eurostat, 2023). B.boops is benthopelagic species that can be found in various habitats and in a wider depth range (up to 350 m) (Froese and Pauly, 2023); more than half of the species EU catches originates from the Mediterranean region (Eurostat, 2023). M.barbatus is among the most commonly found and fished species of the demersal habitats within the basin, usually occupying muddy bottoms up to 400 m depth (Eurostat, 2023; Froese and Pauly, 2023). The objectives of the study were: (i) to investigate the occurrence of PAEs and BPA in the four fishes; (ii) to examine the spatial variations of the concentration levels of the plastic additives between two fisheries important divisions of Mediterranean Sea, Aegean (Division 37.3.1) and Ionian Seas (Division 37.2.2) (FAO, 2023); (iii) to investigate the relationship between plastic additives occurrence between the selected species of different habitats; and (iv) to estimate the human daily intake of the selected plastic additives based on different scenarios of fish consumption.
The fish samples of E.encrasicolus, S.pilchardus, B.boops, and M. barbatus were purchased during 2021-2022 from the local markets of the North Aegean Sea (NAS; Greece) and the western Ionian Sea (IOS; Catania, Italy) and were transported to the laboratory facilities for further processing. In total, approximately 90 samples were collected corresponding to 15 individuals per species. The gastrointestinal tracts (GITs) and the muscles were carefully extracted from each specimen and placed in borosilicate glass vials of 6 mL with a coated metal foil cup (®Wheaton) to prevent plastic additives contamination by the leaching. The samples were stored in -20°C until further analysis.
For the extraction of PAEs and BPA, a liquid-liquid method was applied as described before by Salvaggio et al. (2019). The targeted phthalate diesters were Bis(2-ethylhexyl) phthalate (DEHP), di-isodecylphthalate (DIDP), di-isononylphthalate (DINP), dibutyl phthalate (DBP), dibenzyl phthalate (DBzP), as well as the mono-alkyl phthalates monoNOctyl phthalate (MNOP), monoNButyl phthalate (MNBP), and monomethyl phthalate (MMP) metabolic products of di-n-octyl phthalate (DNOP), dimethyl phthalate (DMP) and dibutyl phthalate (DBP), respectively. For each sample 1 g of tissue was analyzed for the determination of the plastic additives. The separation of the organic phase succeeded with centrifugation, followed by evaporation through nitrogen insufflation to dry the sample (Salvaggio et al., 2019). Ultra-High-Pressure Liquid Chromatography with Electro-Spray ionization and Mass Quadrupole Mass Spectrometry were used to perform the analysis. A Waters Acquity system (UHPLC-ESI-TQD) was used with the Acquity UPLC® HSS C18 and the samples proceeded to Mass Spectrometry, while the quantification of plastic additives was performed using multiple reaction monitoring. The limit of quantification (LOQ) was estimated on 10 ng/g ww.
To evaluate the risk of exposure to PAEs and BPA via fish consumption, three scenarios’ concentrations were considered (EFSA, 2012). The first scenario was based on the exposure via ingestion of fish muscle to low concentration of contaminants (P5: the estimated concentration of 5th percentile), the second based on the mean concentration of contaminants (P50: the mean concentration), and the last on the exposure to high concentration (P95: the estimated concentration of 95th percentile). The risk exposure assessment was applied to two different population groups, adults, and children (from 36 months up to 9 years old) of NAS and IOS (EFSA, 2012). The Estimated Daily Intake (Equation 1) and the Target Hazard Quotient (THQ) for non-carcinogenic adverse effects were estimated for each species (Equation 2).
where, Cp: the concentration of each plastic additive in the fish muscle (ng/g); DI: the daily intake of fish consumption for a person (mean intake of children was 16.5 g/day of fish meat and for adults was 48.9 g/day (EFSA, 2014) converted to Kg/person/day); BW: body weight of children was 23.1 kg and of adults was 70 kg (EFSA, 2012).
The level of the risk exposure was characterized based on the Target Hazard Quotient (THQ), where the estimated daily intake is compared with the tolerable benchmark value assessed for each plastic additive (Equation 2):
where, EDI is calculated from Equation (1); TDI is the tolerable daily intake for each contaminant in μg/kg/day where available (DEHP: 50; DIDP, DINP: 150; DBP: 50; BPA: 4) (EFSA, 2019).
The concentrations of each plastic additive were organized into a dataset and values lower than the LOQ were included (left-censored data). A primary analysis was conducted to select the appropriate statistical approach based on the characteristics of the data. Firstly, the percentage of the censored data to the total values was estimated. Phthalates had a range of 8.3 to 100% of censored values among the different species of NAS (Table 1), while IOS samples had a range from 16.7 to 100% of censored values to the total (Table 2). BPA’s censored values ranged from 33.3 to 66.7% of the total of NAS samples (Table 1) and 33.3 to 83.3% of the samples originating from IOS (Table 2). Further, the probability plots were produced, and both phthalates and BPA followed a lognormal distribution. Taking into account the high percentage of censored values and the sample size of PAEs and BPA, the robust Regression on Order Statistics (ROS) was applied to calculate the median and mean (± standard deviation) for the plastic additives with censored values up to 70% (Helsel, 2012). When censored values were greater than 70% no further descriptive statistics were calculated, and thus the total of the found concentrations of the analytes were described as<LOQ. The nonparametric Peto and Peto modification of the Gehan-Wilcoxon test was selected to detect significant differences between tissues and areas (Helsel, 2012). The Principal Components Analysis (PCA) of the loadings of each plastic additives was applied to examine similarities and dissimilarities between the two fisheries divisions (N= 45 samples for each area). Based on the percentage of the censored values the presence (above LOQ) and absence (below LOQ) were considered the most appropriate approachs. The statistical analysis and visualization were produced with the usage of the NADA (Lee, 2017), the NADA2 (Julian and Helsel, 2021), and the ggplot2 (Wickham, 2016) package in R (R Core Team, 2022).
Table 1 Summary table of descriptive statistics of PAEs and BPA detected in the tissues (GIT and muscle) of the fishes collected from the North Aegean Sea (NAS).
Table 2 Summary table of descriptive statistics of PAEs and BPA detected in the tissues (GIT and muscle) of the fishes collected from the Western Ionian Sea (IOS).
The mean concentrations of plastic additives detected in GIT and muscle were estimated for the four fish species of both areas (Tables 1, 2). Seven out of the eight plastic additives were detected above LOQ for NAS samples, namely DEHP, DIDP, DINP, DBP, DBzP, MNOP and MNBP (Table 1). For IOS samples six out of eight compounds were detected, namely DEHP, DIDP, DBP, DBzP, MNOP and MNBP (Table 2). MMP was below LOQ for the total of the samples analyzed.
DEHP and DIDP were present in most of the species examined for both areas, while the presence of the rest of the examined PAEs varied between the different species. DEHP was the predominant plasticizer in both areas (Figure 1). Overall, E.encrasicolus, S.pilchardus from NAS, and B.boops and M.barbatus from IOS had the highest mean DEHP concentrations. Among the different species, S.pilchardus from NAS exhibited the highest mean DEHP concentration (Table 1). Examining the differences between the two areas and species, statistically significant differences were revealed for DEHP concentrations of S.pilchardus (p< 0.05, Wilcoxon rank test). To further investigate the differences between the tissues (GIT and muscle; Supplementary Table-S1, Supplementary Material) for each species a comparison analysis was conducted. Significant differences in DEHP concentrations between GIT and muscle for B.boops collected from NAS (p< 0.05, Wilcoxon rank test) and S. pilchardus collected from IOS (p< 0.05, Wilcoxon rank test) were proved.
Figure 1 Boxplot of the concentrations of DEHP, DIDP and BPA (ng/g ww) in fish tissue from (A) the North Aegean Sea (NAS) and (B) Western Ionian Sea (IOS). The black line within each boxplot is the median value, while LOQ (equal to 10 ng/g ww) is presented with the dashed black line. The shaded part of each boxplot corresponds to the values below LOQ (censored value). For the fish species that the censored values of the plastic additive were above 70%, the total of the samples were treated as below LOQ.
DIDP was detected in most samples that were examined (Figure 1) with mean concentration ranging from< LOQ up to 12.6 ng/g for B.boops from NAS (Table 2). In contrary, DIDP was below LOQ (Figure 1) in most of the B.boops collected from IOS (77.8% of the total analyzed samples, Table 2) and it was considered that the total of the samples was below LOQ, while it has more frequent occurrence in the pelagic species of the area S.pilchardus and E.encrasicolus (Figure 1). DIDP mean concentration in muscle was smaller than the mean concentration found in GIT for each species, however no significant statistical differences were observed between the two tissues (p > 0.05, Wilcoxon rank test). On the contrary, DINP was detected only in E.encrasicolus collected from NAS and only in the GIT tissue, with no presence on the muscle of the fish (Table 1).
The rest of PAEs examined, DBP, DBzP, MNOP, and MNBP had high percentages of censored values ranging from 55.6 up to 100% of the total samples analyzed. The determination of the monoalkyl phthalate esters showed the presence of MNBP in B.boops from NAS, in a small number of analyzed samples of S.pilchardus and E.encrasicolus from the same area and in a small percentage of analyzed samples of M.barbatus from IOS (Table 1, 2). Both the parent diester DBP and the metabolic monoester MNBP were concurrently detected in all above species, and B.boops from NAS had the highest mean concentrations (Table 1). Considering the rest of the monoesters, MNOP was detected above LOQ in approximately only 1/5 of S.pilchardus from NAS and M. barbatus from IOS, while MMP was below LOQ for the total of the analyzed samples.
BPA was detected above LOQ in all species from NAS, and in E.encrasicolus, B.boops, and M.barbatus from IOS. The highest mean BPA concentration at IOS was spotted in M.barbatus, while for NAS the highest mean concentration was detected in B.boops (Table 1). Comparison between the two areas highlighted that there were no significant differences between the two fisheries divisions (p > 0.05, Wilcoxon rank test). Similarly, no differences between the muscle and GIT of the fish species were observed. A further analysis within each fisheries division was performed, to identify potential differences between the species and the tissues, but no statistical differences were detected (p > 0.05, Wilcoxon rank test).
Summarizing, for the total of the selected plastic additives examined within both NAS and IOS, the most frequently found plastic additives were DEHP, DIDP and BPA, while within each fish species great variation in their occurrence was presented (Figure 1). The levels of plastic additives between the four species for each area for the mean concentrations above the LOQ are as follows: a. E. encrasicolus (NAS): DEHP > BPA = DIDP > DBzP > DINP; b. E. encrasicolus (IOS): DEHP > DIDP > DBzP > BPA, c. S. pilchardus (NAS): DEHP > DIDP > BPA > DBP; d. S. pilchardus (IOS): DEHP > DIDP > DBzP; e. B.boops (NAS): DEHP > DBP > BPA > DIDP > MNBP > DBzP; f. B. boops (IOS): DEHP > BPA; g. M. barbatus (NAS): DEHP > DIDP > BPA > DBzP, and h. M. barbatus (IOS): DEHP > BPA > DIDP > DBP.
By examining the sequence of plastic additives values, different patterns arise among the areas and the species. To further investigate potential similarities of the variability between the two areas, a first indicative Principal Component Analysis (PCA) was performed for the group findings of the plastic additives, considering all four species within each area. Concentrations above LOQ were treated as presence and below LOQ as absence of the plastic additive. Two principal components (PC1, PC2) were considered appropriate to describe the variability for each area as the cumulative percentages of the first two PCs for NAS was 62% and for IOS was 65%. The loadings of the plastic additives in the tissues of NAS (Figure 2A) and IOS (Figure 2B) samples revealed that BPA, DIDP and DEHP were positively related to each other within both fisheries divisions. Similarly, the diester parent DBP and its metabolic product MNBP were also positively related to each other in both areas, verifying their co-occurrence in the tissues of the fish species (Figure 2). Lastly, in the case of NAS samples where DINP were presented, it was positively related to BPA, DIDP and DEHP, although it had a low influence on the overall variability (Figure 2).
Figure 2 Summary loadings PCA plots of plastic additives presence and absence in fish tissues from (A) the North Aegean Sea (NAS) and (B) Western Ionian Sea (IOS).
Considering that the fish was intended for human consumption the level of exposure to risk was estimated based on the different species and areas. The EDI and THQ estimations were based on the detected muscle concentrations in the analyzed samples (Supplementary Table-S1, Supplementary Material).
DEHP, DIDP, and BPA were present in the muscle of the fish collected from both areas, thus the EDI of those contaminants was grouped in a graph (Figure 3). The examined contaminants were below the TDI benchmark values (as mentioned in Materials and Methods) even in all scenarios of exposure, low (P5), moderate (P50) and high (P95) for both population groups. Between the two areas, IOS had greater EDIs for BPA than NAS (Figure 3A), while the opposite presented for DEHP (Figure 3B). Additionally, EDIDBP was detected only in the NAS area with similar values for adults and children (P5: 5.4, 5.6; P50: 8.1, 8.3; P95: 10.8, 11 10-3 respectively for adults and children), while the Target Hazard Quotient (THQ) indicated low likelihood of exposure to DBP (THQDBP< 0.22 10-3) via consumption in all scenarios examined.
Figure 3 Estimated Daily Intake (EDI) (μg/day-kgbw) (10-3) of (A) BPA, (B) DEHP, and (C) DIDP for adults and children in North Aegean Sea (NAS) and Western Ionian Sea (IOS). EDI based on three scenarios of plastic additives concentration (P5, P50, P95).
THQ indicated a low likelihood of toxicological response due to fish consumption for the examined contaminants for both NAS and IOS, as it was below 1, thus the adverse effect on humans via fish consumption is not likely to occur. To further examine the effect of the consuming habits on the level of exposure via fish consumption, THQ was estimated based on the mean concentrations of contaminants detected in both muscle and GIT for NAS (Table 1) and IOS (Table 2), only for the small pelagic fish (E.encrasicolus and S.pilchardus) that could be consumed as a whole (Karami et al., 2017). THQDEHP THQDIDP and THQBPA were below 1 thus, were not likely to cause an adverse effect, although BPA had a greater likelihood compared to PAEs. Similarly, DINP and DBP which were presented only for the NAS area, were unlikely to cause a toxicological effect in both age groups examined under any scenario.
In this study, E. encrasicolus, S. pilchardus, B. boops and M. barbatus collected from the northern Aegean Sea (NAS) were found to contain seven of the eight examined PAEs (Table 1), while the same species collected from the western Ionian Sea (IOS) contained six PAEs (Table 2). The accumulation of PAEs was tested in GIT and muscle tissues for the samples collected from both fisheries divisions of the Mediterranean Sea. The most common contaminant having also the highest mean concentration was DEHP. Similar findings were presented in several previous studies examining PAEs occurrence and analyzing different fish species and tissues, where DEHP was reported as the most frequently found PAE and with the highest concentrations (e.g. Salvaggio et al., 2019; Vered et al., 2019; Panio et al., 2020; Rios-Fuster et al., 2022; Castro et al., 2023; Squadrone et al., 2023). DEHP is the most common representative of the PAEs group and is extensively used as a plasticizer in many products (e.g., medical devices, furniture materials, etc.) and is utilized in manufacturing a wide variety of consumer products (e.g., wall coverings, cosmetics, and personal care products (Rowdhwal and Chen, 2018). Its impact as an EDC has been recognized and DEHP was characterized as a very highly concern compound and mitigation restrictions have been enforced, which are indeed reflected on the current lower levels of exposure compared to those a few decades ago (EEA, 2023). On the other hand, several studies within the Mediterranean Sea, have also been reported the widespread and predominant distribution of DEHP within the different compartments of the marine environment (seawater -Grigoriadou et al., 2008; Sánchez-Avila et al., 2012; Paluselli et al., 2018a, b; Jebara et al., 2021; sediment -Alkan et al., 2021; Jebara et al., 2021).
Examining the occurrences of DEHP between the different fisheries divisions and among the different fish species of this study, the highest mean concentration occurred in B.boops and M. barbatus from IOS, while the lowest ones presented in the small pelagic species (E.encrasicolus and S.pilchardus) (Table 2). M.barbatus is a demersal fish depending on the seafloor fauna to be fed, while B.boops is a benthopelagic species occurring both in benthic and pelagic habitats (Froese and Pauly, 2023). Notable differences in the concentration of PAEs between the different fish species have recently been reported in fish collected from Tyrrhenian and Ligurian Sea (Squadrone et al., 2023; Squillante et al., 2023). The habitat and the feeding habits and preferences of the species are considered important factors affecting the uptake of phthalates from the marine environment and their accumulation in the different tissues (Savoca et al., 2023). It has been highlighted that fish fed on benthic organisms or demersal species presented higher levels of hydrophobic phthalates (such as DEHP: logKow= 7.33) highlighting their relationship with the sediment of their habitats (Hu et al., 2016), as usually hydrophobic phthalates have higher concentrations in the sediment (Savoca et al., 2023). Species habitat and subsequently the PAEs load within this matrix, as well as the biological factors of the species could explain the variations of concentrations presented between the different species of IOS within this study. Future research efforts focusing on samples collections from biota, sediment, and seawater may contribute to the knowledge of those relationships.
The opposite pattern of PAEs concentrations was presented for the fish species collected from NAS, thus the small pelagic fish had the highest mean concentrations of DEHP, followed by B.boops and M.barbatus (Table 1). Overall, samples from NAS area had on average higher mean concentration of PAEs that could reflect the local level of PAEs pollution in the environment. These results could be possibly related to the increased plastic loads that the NAS receives from different sources, which could be mediated to the overall mean higher concentrations of plastic additives in the NAS. Greek and Turkish rivers are discharging in NAS releasing several hundred tons of plastic per year (Kaandorp et al., 2020). In addition, NAS receives less saline surface waters of Black Sea origin outflowing through the Dardanelles that are heavily loaded with plastic debris (Tunçer et al., 2018), while it is considered an area of intense fisheries activity (Kaandorp et al., 2020). In addition to the potentially elevated PAEs levels in the surface layer of the NAS caused by the influx of plastic-enriched waters, another factor that may partially explain the higher DEHP concentration in pelagic species is the relatively lower aerobic degradation rates of DEHP compared to low molecular weight PAEs by marine microorganisms (Chang et al., 2004; Yuan et al., 2010), which may contribute to its widespread local presence at the surface.
A recent study also reported DEHP as the predominant PAEs in the fish that were analyzed, while the rest of the examined compounds were in lower concentrations or below LOQ (Squadrone et al., 2023). The long-term DEHP presence in the marine environment -even in low concentrations- has been reported to cause abnormalities in fish development, especially when they are in the early life stages, as it can cause a reduction in body growth (Yang et al., 2018), negatively affect the functions of the reproduction system and increase the mortality rates (Uren-Webster et al., 2010; Yuen et al., 2020). In the last years, DEHP was substituted in various applications by DIDP and DINP in an effort to reduce the risk associated with DEHP (Net et al., 2015; Hermabessiere et al., 2017). In this study, DIDP was found in all species collected from NAS (Table 1) and in lower mean concentrations in three out of four species collected from IOS (Table 2). Although previous findings of DIDP for the selected species are scarce, it has been reported for the fish Lepidopus caudatus in IOS area ranging from 9 to 41 ng/g ww (Salvaggio et al., 2019). Under laboratory controlled experiments, DIDP has been found to produce abnormalities in fish functions, as biomarkers responses were decreased within several tissues when DIDP was introduced (Poopal et al., 2020). Further, our findings indicated statistical differences of DIDP mean concentrations between the two tissues, as they were significantly lower in muscle than in GIT (p > 0.05, Wilcoxon rank test), in accordance with findings previously reported for IOS (Salvaggio et al., 2019). DIDP (similar to DEHP) is a high molecular weight phthalate with very low solubility (logKow > 8), that could accumulate in the sediments in high concentrations and indicates an increased likelihood to accumulate in marine organisms feed from benthos (Net et al., 2015; Hu et al., 2016; Hermabessiere et al., 2017), although this was not verified under this study for DIDP as not significant differences were found between the species. The results highlighted the presence of DINP only in GIT tissue of E.encrasicolus species collected from NAS, while it was not detected in the muscle of the fish (Table 1). Higher concentrations of DINP in the GIT, in comparison to the other fish tissues examined, have also been cited for fish samples from the Tyrrhenian Sea (Squillante et al., 2023) and the Catania market (Salvaggio et al., 2019). Both DIDP and DINP presence has been found to impair the reproduction system and alter the behavioral patterns of the fish (e.g., Forner-Piquer et al., 2019; Poopal et al., 2020; Godoi et al., 2021), while unlike to DEHP that is monitored in the seawater due to legislative enforcement (EU, 2000), DIDP and DINP presence is only regulated for food contact materials (Hidalgo-Serrano et al., 2022). DBP was detected only in a part of the samples, specifically in M.barbatus from IOS and S.pilchardus, and B.boops from NAS, with the latter having the highest mean concentration. In addition, DBP and its metabolic monoester MNBP were both detected in the B.boops (NAS) and M.barbatus (IOS). DBP in M.barbatus has previously been reported for samples collected in the Tyrrhenian Sea (Squillante et al., 2023), with mean concentrations within its muscle tissues much higher than those reported under this study, that could occur due to variations in concentration levels between the two geographical sites (Savoca et al., 2023). The accumulation of DBP by marine organisms within Mediterranean Sea, has been previous detected (Vered et al., 2019; Panio et al., 2020). In contrary to the above described PAEs, DBP is a lower molecular weight PAEs, with greater solubility than the higher molecular weight PAEs (e.g. DEHP, DIDP, DINP), thus it is expected to degrade in a higher rate (Chang et al., 2004). The latter could explain DBP partially detection within this study. Furthermore, previous effort highlighted a significant linear correlation between monoalkyl phthalate ester of short-branched PAEs with the sum of PAEs detected in marine organisms, indicating that they could act as an indicator of exposure to PAEs (Hu et al., 2016). Although, the findings presented a primary indication of this relationship, this study was not designed with a purpose to explore parent’s and metabolic products co-occurrence, thus they were treated as no conclusive evidence. Out of the rest of monoesters examined (MNOP and MMP), only MNOP was detected above LOQ, within specific species and in a small part of the total samples analyzed. The high percentage of censored values does not allow for confident conclusions; however, it is suggested that further investigation of the relationship between the metabolite compound and the corresponding parent PAE is needed. MNOP and MMP have previously been reported for marine fish in low concentration, and especially MNOP was detected in higher frequencies than the corresponding PAE (DNOP) (Hu et al., 2016).
In the context of this study, BPA was detected in most fishes (all four species of NAS and three out of four from IOS), while species from NAS had on average the highest mean concentration, the maximum average concentration was detected in IOS M.barbatus (Table 2). Further, no significant statistical differences of BPA occurrence were found between the two fisheries divisions (p > 0.05, Wilcoxon rank test). Similarly, no differences were observed between species (when applicable) and tissues (p > 0.05, Wilcoxon rank test). BPA concentrations of S.pilchardus (IOS) were below LOQ and were in accordance with previous findings examining sardines collected from supermarkets of Spain (Míguez et al., 2012). Below detection limit values have also been reported for M.barbatus from Aegean Sea, although the findings referred to liver samples that was not included in this study, thus can not be directly compared (see Supplementary Table S1, Supplementary Material) (Yilmaz et al., 2016). Previous examination of bisphenols on the Tyrrhenian coast, detected BPA in the muscle and liver of M.barbatus (see Supplementary Table S1, Supplementary Material), while variations occurred between the tissues and the sites (Errico et al., 2017). Higher levels of BPA have also been recorded in canned fish from the Greek market, although no direct comparison can be made as the species name or the group of the organisms was not mentioned (Tzatzarakis et al., 2017). Arditsoglou and Voutsa (2012) collected mussels, seawater, and sediment from Aegean Sea, and although BPA was present in seawater and sediment, was absent in mussels’ tissue. BPA has been known as EDC, thus similar to PAEs measures have been taken for its mitigation (EU, 2011, 2018). Most recently, a meta-analysis study presented that BPA concentrations’ range was greater within fish species – compared to the rest of the biota groups- and had significantly negatively impacted various biological responses (Wu and Seebacher, 2020).
The variability within the distribution of the plastic additives was also primarily explored with PCAs production based on presence and absence approach. Highlighting the most important findings, DIDP, DEHP and BPA was positively related to each other in both areas, as well as DINP were also positively related to this group in NAS. The observed findings could come from the molecular structure and chemical properties of each individual plastic additive and the inter-species differences in metabolic transformation (Gobas et al., 2003), as DEHP, DIDP and DINP are high molecular weight PAEs with low solubility. In contrary, although BPA has a low octanol-water partition coefficient (logKow =3.4) (Oehlmann et al., 2008) is considered a moderately hydrophobic compound with a tendency to bioconcentrate, as it has been verified that residuals remain within the organism in greater concentration than the initial (Gatidou et al., 2010). Furthermore, differences in feeding behavior and preferences as well as in metabolic efficiency among different individuals or species could greatly influence the accumulation potential of PAEs and BPA, as it was also underlined in other similar studies (Net et al., 2015; Hu et al., 2016; Sala et al., 2022). The fat content of each individual or species which is dependent on catching season, size, tissue analyzed (Hernández-Martínez et al., 2013) and geographical location could also affect the accumulation capacity of lipophilic chemicals such as PAEs and BPA (Savoca et al., 2023). DBP and in metabolic product MNBP were also positively related in the analyzed specimen within both fisheries divisions. In general, the detection of phthalate metabolites suggests that fish were exposed to phthalate metabolites from water or sediment, as phthalate parents can be transformed by microorganisms (Gao and Wen, 2016), or recently exposed to phthalate parents that are not yet fully incorporated and metabolized (Fourgous et al., 2016; Guerranti et al., 2016). However, further research is needed as the metabolism of phthalates in fish is still lacking information (Guerranti et al., 2016). In parallel, monoesters presence in fish tissues could also be attributed to the PAEs leaching from ingested plastic particles (Guerranti et al., 2016). It becomes evident that a great complexity characterizes the accumulation process of these contaminants which explains the observed diverse patterns of plastic additives concentrations between the different species and suggests that some additional factors should be taken into consideration in future studies.
The Estimated Daily Intake (EDI) and the Target Hazard Quotient (THQ) were estimated aiming to identify a potential adverse effect through the consumption by humans of the selected commercially important fishes. The concentration found only in the muscle samples was used (see Supplementary Table S1, Supplementary Material), as it was considered a more realistic scenario. Furthermore, different scenarios of concentrations levels (P5, P50, P95) were also examined taking also into consideration the mean fish intake for children and adults. The EDI of DEHP, DIDP, and BPA had values below the Tolerable Daily Intake (TDI) within all different concentrations considered (Figure 3). In the majority of EDI estimations, NAS had slightly greater values than IOS estimations, corresponding to the expected results by the overall findings on the plastic additives occurrences as described above. EDI for DBP was estimated only for NAS samples as it was not present in muscle samples of IOS, producing EDI values below TDI, similar to the other PAEs. The consumption of the referenced portions of contaminated with PAEs fish flesh was unlikely to cause adverse effects to humans. The current results are in agreement with recent studies based on samples collected from the local fishers of the Tyrrhenian Sea (Squillante et al., 2023), and different fish markets in Spain (Castro et al., 2023). Although commonly used PAEs have high octanol-water partition coefficients, fishes exhibit a higher metabolic capacity to PAEs than other biota groups within marine environments and thus biomagnification is not generally expected to occur (Staples et al., 1997; Van Wezel et al., 2000; Oehlmann et al., 2008). In contrast to PAEs, the EDI for BPA was below the TDI for every scenario of exposure presented in the flesh of the fish (Figure 3), although BPA had a greater likelihood than PAEs to prompt a negative effect. Similar findings of BPA-related risk were previously reported for fish collected from the Portuguese coast (Barboza et al., 2020). Most recently, BPA has been recognized as substance of very high concern and the tolerable level of BPA toxicity in humans was significantly reduced from 4.0 μg/kg to 0.2 ng/kg (EFSA, 2023). Considering the new proposed TDI value, THQ of BPA for both NAS and IOS indicated a substantially higher likelihood of exposure to effect even in the scenario of low concentration. In recognition of the potential risk that plastic additives pose to human health, PAEs and BPA are subject to regulatory monitoring, while their use in food and beverage contact products has been set at specific levels in EU countries to keep their leaching from packaging into food at acceptable levels (EU, 2011, 2018). The scenario of consuming the fish as a whole was also examined, producing similar findings for PAEs, but greater values, even in low exposure for BPA in the NAS division, highlighting that the removal of the internal organs before cooking is crucial. Last but not least, organisms are expected to be exposed simultaneously to various contaminants and their cumulative impact have been proven able of producing toxic effects (Gao et al., 2018). Future efforts on integrated exposure estimation through different pathways and ongoing update and adaption on chemicals prioritization tools will further highlight mitigation measures to the risk of the exposure (Aurisano et al., 2021).
Within this work, phthalates and bisphenol A were detected in the tissues of E.encrasicolus, S.pilchardus, B.boops and M.barbatus collected from the marketplace of two important fishing divisions of the Mediterranean Sea, the Aegean and the Ionian Sea. Different patterns of concentrations of plastic additives occurred between the different species, and while the habitat of the fish seems to be a possible factor influencing their variability. DEHP had the highest mean concentration and was detected in the majority of the samples analyzed for each species for both the muscle and the GIT. Overall, the muscle had in most cases a lower mean concentration than the GIT for each contaminant analyzed, statistically significant differences were recorded for DEHP and the species B.boops (NAS) and S.pilchardus (IOS). DIDP and DINP that have been used as alternatives for DEHP in plastics production, even though acceptable limits have been set for various applications were also detected. DIDP was present in the majority of the samples collected at both divisions, while no statistically significant differences were observed at the spatial scale or between the species. In contrary, DINP was recorded only in the GIT of E.encrasicolus (NAS). The rest of the examined PAEs were detected partially in the analyzed samples. It should be mentioned than MNBP and its parent substance DBP were co-presented in S.pilchardus and B.boops (NAS) in higher frequencies. Despite the fact that monoesters (MNBP, MNOP) were present in low percentages, their detection indicates that further research is needed to better describe the relationship with their parent diesters within the fish. BPA was detected in all species analyzed within both divisions, with the exception of S. pilchardus from IOS, and the mean concentrations followed those of DEHP or DIDP for each species. The risk assessment of human exposure to the PAEs and BPA via fish consumption showed no adverse effects for both groups considered (children and adults) at both sites. In the case of small pelagic fish, the effect was also assessed for the whole-fish consumption scenario and the risk was higher for BPA. Future research on plasticizers accumulation in different marine matrices, regional variability, marine species, especially those used for human consumption, and their metabolic transformation in different tissues will provide a better understanding of their impact. Investigations on conditions better simulating the marine environment (e.g. distribution and degradation, simultaneous exposure mixtures of contaminants) will further contribute in understanding plastic additives issue.
The original contributions presented in the study are included in the article/Supplementary Material. Further inquiries can be directed to the corresponding authors.
Ethical approval was not required for the study involving animals in accordance with the local legislation and institutional requirements. Under this study, we used commercially available fish samples obtained from the local fish markets of the study area. Thus the fish were no longer alive by the time they were purchased.
DM: Conceptualization, Data curation, Formal analysis, Investigation, Methodology, Software, Visualization, Writing – original draft, Writing – review & editing. MB: Conceptualization, Data curation, Formal analysis, Funding acquisition, Investigation, Methodology, Project administration, Resources, Supervision, Validation, Writing – original draft, Writing – review & editing. RP: Conceptualization, Data curation, Investigation, Methodology, Project administration, Resources, Supervision, Validation, Writing – original draft, Writing – review & editing. ES: Investigation, Methodology, Validation, Writing – review & editing. MC: Data curation, Investigation, Methodology, Writing – review & editing. CS: Investigation, Methodology, Writing – review & editing. GF: Investigation, Methodology, Writing – review & editing. SI: Investigation, Methodology, Writing – review & editing. RV: Data curation, Formal analysis, Investigation, Methodology, Resources, Validation, Writing – review & editing. FT: Investigation, Writing – review & editing. EK: Writing – review & editing, Conceptualization, Formal analysis, Investigation, Project administration, Resources, Supervision, Writing – original draft.
The author(s) declare financial support was received for the research, authorship, and/or publication of this article. This research for University of Catania was funded by “fondi di ateneo 2020-2022, Università di Catania, linea OpenAccess”. The research work for Dimitra Marmara was supported by the Hellenic Foundation for Research and Innovation (HFRI) under the HFRI PhD Fellowship grant (Fellowship Number: 1331).
Author RV was employed by SIALAB srl.
The remaining authors declare that the research was conducted in the absence of any commercial or financial relationships that could be construed as a potential conflict of interest.
All claims expressed in this article are solely those of the authors and do not necessarily represent those of their affiliated organizations, or those of the publisher, the editors and the reviewers. Any product that may be evaluated in this article, or claim that may be made by its manufacturer, is not guaranteed or endorsed by the publisher.
The Supplementary Material for this article can be found online at: https://www.frontiersin.org/articles/10.3389/fmars.2024.1334237/full#supplementary-material
Alimi O. S., Farner Budarz J., Hernandez L. M., Tufenkji N. (2018). Microplastics and nanoplastics in aquatic environments: aggregation, deposition, and enhanced contaminant transport. Environ. Sci. Technol. 52, 1704–1724. doi: 10.1021/acs.est.7b05559
Alkan N., Alkan A., Castro-Jiménez J., Royer F., Papillon L., Ourgaud M., et al. (2021). Environmental occurrence of phthalate and organophosphate esters in sediments across the Gulf of Lion (NW Mediterranean Sea). Sci. Total Environ. 760, 143412. doi: 10.1016/j.scitotenv.2020.143412
Andrady A. L. (2011). Microplastics in the marine environment. Mar. Pollut. Bull. 62, 1596–1605. doi: 10.1016/j.marpolbul.2011.05.030
Andrady A. L. (2015). “Persistence of plastic litter in the oceans. In Marine anthropogenic litter,” in Marine Anthropogenic Litter. Eds. Bergmann M., Gutow L., Klages M. (Springer, Cham), 57–72. doi: 10.1007/978-3-319-16510-3
Andrady A. L., Neal M. A. (2009). Applications and societal benefits of plastics. Philos. Trans. R. Soc. B: Biol. Sci. 364, 1977–1984. doi: 10.1098/rstb.2008.0304
Arditsoglou A., Voutsa D. (2012). Occurrence and partitioning of endocrine-disrupting compounds in the marine environment of Thermaikos Gulf, Northern Aegean Sea, Greece. Mar. Pollut. Bull. 64, 2443–2452. doi: 10.1016/j.marpolbul.2012.07.048
Aurisano N., Huang L., Milà i Canals L., Jolliet O., Fantke P. (2021). Chemicals of concern in plastic toys. Environ. Int. 146, 106194. doi: 10.1016/j.envint.2020.106194
Barboza L. G. A., Cunha S. C., Monteiro C., Fernandes J. O., Guilhermino L. (2020). Bisphenol A and its analogs in muscle and liver of fish from the North East Atlantic Ocean in relation to microplastic contamination. Exposure and risk to human consumers. J. Hazard. Materials 393, 122419. doi: 10.1016/j.jhazmat.2020.122419
Baudena A., Ser-Giacomi E., Jalón-Rojas I., Galgani F., Pedrotti M. L. (2022). The streaming of plastic in the Mediterranean Sea. Nat. Commun. 13, 2981. doi: 10.1038/s41467-022-30572-5
Blasi M. F., Avino P., Notardonato I., Di Fiore C., Mattei D., Gauger M. F. W., et al. (2022). Phthalate esters (PAEs) concentration pattern reflects dietary habitats (δ13C) in blood of Mediterranean loggerhead turtles (Caretta caretta). Ecotoxicol. Environ. Saf. 239, 113619. doi: 10.1016/j.ecoenv.2022.113619
Calafat A. M., Ye X., Silva M. J., Kuklenyik Z., Needham L. L. (2006). Human exposure assessment to environmental chemicals using biomonitoring. Int. J. androl. 29, 166–171. doi: 10.1111/j.1365-2605.2005.00570.x
Castro Ó., Borrull S., Borrull F., Pocurull E. (2023). High production volume chemicals in the most consumed seafood species in Tarragona area (Spain): Occurrence, exposure, and risk assessment. Food Chem. Toxicol. 173, 113625. doi: 10.1016/j.fct.2023.113625
Cerkvenik-Flajs V., Fonda I., Gombač M. (2018). Analysis and occurrence of bisphenol A in mediterranean mussels (Mytilus galloprovincialis) sampled from the Slovenian Coastal Waters of the North Adriatic Sea. Bull. Environ. Contam. Toxicol. 101, 439–445. doi: 10.1007/s00128-018-2415-4
Chang B., Yang C., Cheng C., Yuan S. (2004). Biodegradation of phthalate esters by two bacteria strains. Chemosphere 55, 533–538. doi: 10.1016/j.chemosphere.2003.11.057
Corrales J., Kristofco L. A., Steele W. B., Yates B. S., Breed C. S., Williams E. S., et al. (2015). Global assessment of bisphenol A in the environment: review and analysis of its occurrence and bioaccumulation. Dose-response 13, 1559325815598308. doi: 10.1177/1559325815598308
Cózar A., Sanz-Martin M., Martì E., González-Gordillo J. I., Ubeda B., Gálvez J. Á., et al. (2015). Plastic accumulation in the mediterranean sea. PloS One 10, e0121762. doi: 10.1371/journal.pone.0121762
ECHA. (2018). Seven new substances added to the Candidate List, entry for Bisphenol-A updated to reflect its endocrine disrupting properties for the environment. Available online at: https://echa.europa.eu/-/seven-new-substances-added-to-the-candidate-list-entry-for-bisphenol-a-updated-to-reflect-its-endocrine-disrupting-properties-for-the-environment (Accessed December 13, 2023).
ECHA. (2023). Bisphenols (European Chemicals Agency). Available online at: https://echa.europa.eu/hot-topics/bisphenols (Accessed December 13, 2023).
EEA. (2023). Chemicals signals. Available online at: https://www.eea.europa.eu/publications/zero-pollution/health/signals/chemicals (Accessed December 13, 2023).
EFSA. (2012). Guidance on selected default values to be used by the EFSA Scientific Committee, Scientific Panels and Units in the absence of actual measured data. EFSA J. 10, 2579. doi: 10.2903/j.efsa.2012.2579
EFSA. (2014). Dietetic Products, Nutrition, and Allergies. Scientific Opinion on health benefits of seafood (fish and shellfish) consumption in relation to health risks associated with exposure to methylmercury. EFSA J. 12, 3761. doi: 10.2903/j.efsa.2014.3761
EFSA. (2019). Panel on Food Contact Materials, Enzymes and Processing Aids, Silano, V., Barat Baviera, J. M., Bolognesi, C., Chesson, A., Cocconcelli, P. S., Crebelli, R. et al. Update of the risk assessment of di-butylphthalate (DBP), butyl-benzyl-phthalate (BBP), bis (2-ethylhexyl) phthalate (DEHP), di-isononylphthalate (DINP) and di-isodecylphthalate (DIDP) for use in food contact materials. EFSA J. 17, e05838. doi: 10.2903/j.efsa.2019.5838
EFSA. (2020). Norwegian Institute of Public Health, Gkrillas, A., Dirven, H., Andreassen, M., Papadopoulou, E., Hjertholm, H., Husøy, T. Risk assessment of phthalates based on aggregated exposure from foods and personal care products and comparison with biomonitoring data. EFSA J. 18, e181105. doi: 10.2903/j.efsa.2020.e181105
EFSA. (2023). Panel on Food Contact Materials, Enzymes and Processing Aids (CEP), Lambré, C., Barat Baviera, J. M., Bolognesi, C., Chesson, A., Cocconcelli, P. S., Crebelli, R., et al. Re-evaluation of the risks to public health related to the presence of bisphenol A (BPA) in foodstuffs. EFSA J. 21, e06857. doi: 10.2903/j.efsa.2023.6857
Eriksen M., Lebreton L. C., Carson H. S., Thiel M., Moore C. J., Borerro J. C., et al. (2014). Plastic pollution in the world’s oceans: more than 5 trillion plastic pieces weighing over 250,000 tons afloat at sea. PloS One 9, e111913. doi: 10.1371/journal.pone.0111913
Errico S., Nicolucci C., Migliaccio M., Micale V., Mita D. G., Diano N. (2017). Analysis and occurrence of some phenol endocrine disruptors in two marine sites of the northern coast of Sicily (Italy). Mar. Pollut. Bull. 120, 68–74. doi: 10.1016/j.marpolbul.2017.04.061
EU. (2000). Commission Directive 2000/60 of the European Parliament and of the Council od 23 October 2000 establishing a framework for Community action in the field of water policy. Off. J. Eur. Union. Available at: http://data.europa.eu/eli/dir/2000/60/oj.
EU. (2011). Commission Directive 2011/8, Amending Directive 2002/72/EC as regards the restriction of use of Bisphenol A in plastic infant feeding bottles Text with EEA relevance. Off. J. Eur. Union. Available at: http://data.europa.eu/eli/dir/2011/8/oj.
EU. (2018). Commission Regulation 2018/213, On the use of bisphenol A in varnishes and coatings intended to come into contact with food and amending Regulation (EU) No 10/2011 as regards the use of that substance in plastic food contact materials. Off. J. Eur. Union. Available at: http://data.europa.eu/eli/reg/2018/213/oj.
Eurostat. (2023). Eurostat Data browser. Available online at: https://ec.europa.eu/eurostat/databrowser (Accessed December 13, 2023).
FAO. (2022). The State of World Fisheries and Aquaculture 2022. Towards Blue Transformation (Rome: FAO). doi: 10.4060/cc0461en
FAO. (2023). Fisheries and Aquaculture Division (FAO Major Fishing Areas). Available online at: https://fao.org (Accessed June 6, 2023).
Fernadez M. A. (2019). Populations collapses in marine invertebrates due to endocrine disruption: A cause for concern? Front. Endocrinol. 10. doi: 10.3389/fendo.2019.00721
Flint S., Markle T., Thompson S., Wallace E. (2012). Bisphenol A exposure, effects, and policy: a wildlife perspective. J. Environ. Manage. 104, 19–34. doi: 10.1016/j.jenvman.2012.03.021
Forner-Piquer I., Mylonas C. C., Fakriadis I., Papadaki M., Piscitelli F., Di Marzo V., et al. (2019). Effects of diisononyl phthalate (DiNP) on the endocannabinoid and reproductive systems of male gilthead sea bream (Sparus aurata) during the spawning season. Arch. Toxicol. 93, 727–741. doi: 10.1007/s00204-018-2378-6
Fourgous C., Chevreuil M., Alliot F., Amilhat E., Faliex E., Paris-Palacios S., et al. (2016). Phthalate metabolites in the European eel (Anguilla Anguilla) from Mediterranean coastal lagoons. Sci. Total Environ. 569, 1053–1059. doi: 10.1016/j.scitotenv.2016.06.159
Froese R., Pauly D. (2023). FishBase (World Wide Web electronic publication). Available online at: www.fishbase.org (Accessed December 13, 2023).
Gao D. W., Wen Z. D. (2016). Phthalate esters in the environment: a critical review of their occurrence, biodegradation, and removal during wastewater treatment processes. Sci. Total Environ. 541, 986–1001. doi: 10.1016/j.scitotenv.2015.09.148
Gao H., Xu R., Cao W., Di Q., Li R., Lu L., et al. (2018). Combined effects of simultaneous exposure to six phthalates and emulsifier glycerol monosterate on male reproductive system in rats. Toxicol. Appl. Pharmacol. 341, 87–97. doi: 10.1016/j.taap.2018.01.013
Gatidou G., Vassalou E., Thomaidis N. S. (2010). Bioconcentration of selected endocrine disrupting compounds in the Mediterranean mussel, Mytilus galloprovincialis. Mar. Pollut. Bull. 60, 2111–2116. doi: 10.1016/j.marpolbul.2010.07.003
Gobas F. A. P. C., Mackintosh C. E., Webster G., Ikonomou M., Parkerton T. F., Robillard K. (2003). “Bioaccumulation of Phthalate Esters in Aquatic Food-Webs,” in Series Anthropogenic Compounds. The Handbook of Environmental Chemistry, vol. 3Q . Ed. Staples C. A. (Springer, Berlin, Heidelberg). doi: 10.1007/b11467
Godoi F. G. A., Forner-Piquer I., Randazzo B., Habibi H. R., Lo Nostro F. L., Moreira R. G., et al. (2021). Effects of di-isononyl phthalate (DiNP) on follicular atresia in zebrafish ovary. Front. Endocrinol. 12. doi: 10.3389/fendo.2021.677853
Grigoriadou A., Schwarzbauer J., Georgakopoulos A. (2008). Molecular indicators for pollution source identification in marine and terrestrial water of the industrial area of Kavala city, North Greece. Environ. Pollut. 151, 231–242. doi: 10.1016/j.envpol.2007.01.053
Guerranti C., Cau A., Renzi M., Badini S., Grazioli E., Perra G., et al. (2016). Phthalates and perfluorinated alkylated substances in atlantic bluefin tuna (Thunnus thynnus) specimens from mediterranean sea (Sardinia, Italy): levels and risks for human consumption. J. Environ. Sci. Health B. 51, 661–667. doi: 10.1080/03601234.2016.1191886
Helsel R. D. (2012). Statistics for censored environmental data using minitab and R. 2nd ed (Hoboken, New Jersey: John Wiley & Sons, Inc.). doi: 10.1002/9781118162729
Hermabessiere L., Dehaut A., Paul-Pont I., Jazeque R., Lacroix C., Soudant P., et al. (2017). Occurrence and effects of plastic additives on marine environments and organism: a review. Chemosphere 182, 781–793. doi: 10.1016/j.chemosphere.2017.05.096
Hernández-Martínez M., Gallardo-Velázquez T., Osorio-Revilla G., Almaraz-Abarca N., Ponce-Mendoza A., Vásquez-Murrieta M. S. (2013). Prediction of total fat, fatty acid composition and nutritional parameters in fish fillets using MID-FTIR spectroscopy and chemometrics. LWT-Food Sci. Technol. 52, 12–20. doi: 10.1016/j.lwt.2013.01.001
Hidalgo-Serrano M., Borrull F., Marcé R. M., Pocurull E. (2022). Phthalate esters in marine ecosystems: Analytical methods, occurrence and distribution. TrAC Trends Anal. Chem. 151, 116598. doi: 10.1016/j.trac.2022.116598
Hu X., Gu Y., Huang W., Yin D. (2016). Phthalate monoesters as markers of phthalate contamination in wild marine organisms. Environ. Pollut. 218, 410–418. doi: 10.1016/j.envpol.2016.07.020
Jambeck J. R., Geyer R., Wilcox C., Siegler T. R., Perryman M., Andrady A., et al. (2015). Plastic waste inputs from land into the ocean. Science 347, 768–771. doi: 10.1126/science.1260352
Jebara A., Albergamo A., Rando R., Potortì A. G., Lo Turco V., Mansour H. B., et al. (2021). Phthalates and non-phthalate plasticizers in Tunisian marine samples: Occurrence, spatial distribution and seasonal variation. Mar. Pollut. Bull. 163, 111967. doi: 10.1016/j.marpolbul.2021.111967
Julian P., Helsel D. (2021). NADA2: Data Analysis for Censored Environmental Data. R package version 1.0.2. Available online at: https://github.com/SwampThingPaul/NADA2 (Accessed May 8, 2023).
Kaandorp M. L., Dijkstra H. A., van Sebille E. (2020). Closing the Mediterranean marine floating plastic mass budget: inverse modeling of sources and sinks. Environ. Sci. Technol. 54, 11980–11989. doi: 10.1021/acs.est.0c01984
Karami A., Golieskardi A., Ho Y. B., Larat V., Salamatinia B. (2017). Microplastics in eviscerated flesh and excised organs of dried fish. Sci. Rep. 7, 5473. doi: 10.1038/s41598-017-05828-6
Kim J. J., Kumar S., Kumar V., Lee Y. M., Kim Y. S., Kumar V. (2019). Bisphenols as a legacy pollutant, and their effects on organ vulnerability. Int. J. Environ. Res. Public Health 17, 112. doi: 10.3390/ijerph17010112
Klečka G. M., Staples C. A., Clark K. E., van der Hoeven N., Thomas D. E., Hentges S. G. (2009). Exposure analysis of bisphenol A in surface water systems in North America and Europe. Environ. Sci. Technol. 43, 6145–6150. doi: 10.1021/es900598e
Langston W. J. (2020). Endocrine disruption and altered sexual development in aquatic organisms: an invertebrate perspective. J. Mar. Biol. Assoc. United Kingdom 100, 495–515. doi: 10.1017/S0025315420000533
Lee L. (2017). NADA: nondetects and data analysis for environmental data R package version 1.6-1. Available online at: https://CRAN.R-project.org/package=NADA (Accessed May 8, 2023).
Liubartseva S., Coppini G., Lecci R., Clementi E. (2018). Tracking plastics in the Mediterranean: 2D Lagrangian model. Mar. Pollut. Bull. 129, 151–162. doi: 10.1016/j.marpolbul.2018.02.019
Lusher A., Bergmann M., Gutow L., Klages M. (2015). “Microplastics in the marine environment: distribution, interactions and effects,” in Marine anthropogenic litter (Springer International Publishing, Cham), 245–307. doi: 10.1007/978-3-319-16510-3_10
Míguez J., Herrero C., Quintás I., Rodríguez C., Gigosos P. G., Mariz O. C. (2012). A LC–MS/MS method for the determination of BADGE-related and BFDGE-related compounds in canned fish food samples based on the formation of [M+ NH4]+ aducts. Food Chem. 135, 1310–1315. doi: 10.1016/j.foodchem.2012.05.099
Net S., Sempéré R., Delmont A., Paluselli A., Ouddane B. (2015). Occurrence, fate, behavior and ecotoxicological state of phthalates in different environmental matrices. Environ. Sci. Technol. 49, 4019–4035. doi: 10.1021/es505233b
Oehlmann J., Oetken M., Schulte-Oehlmann U. (2008). A critical evaluation of the environmental risk assessment for plasticizers in the freshwater environment in Europe, with special emphasis on bisphenol A and endocrine disruption. Environ. Res. 108, 140–149. doi: 10.1016/j.envres.2008.07.016
Paluselli A., Aminot Y., Galgani F., Net S., Sempere R. (2018b). Occurrence of phthalate acid esters (PAEs) in the northwestern Mediterranean Sea and the Rhone River. Prog. Oceanogr. 163, 221–231. doi: 10.1016/j.pocean.2017.06.002
Paluselli A., Fauvelle V., Schmidt N., Galgani F., Net S., Sempéré R. (2018a). Distribution of phthalates in marseille bay (NW Mediterranean Sea). Sci. total Environ. 621, 578–587. doi: 10.1016/j.scitotenv.2017.11.306
Panio A., Fabbri Corsarini S., Bruno A., Lasagni M., Labra M., Saliu F. (2020). Determination of phthalates in fish fillets by liquid chromatography tandem mass spectrometry (LC-MS/MS): A comparison of direct immersion solid phase microextraction (SPME) versus ultrasonic assisted solvent extraction (UASE). Chemosphere 255, 127034. doi: 10.1016/j.chemosphere.2020.127034
Poopal R. K., Zhang J., Zhao R., Ramesh M., Ren Z. (2020). Biochemical and behavior effects induced by diheptyl phthalate (DHpP) and Diisodecyl phthalate (DIDP) exposed to zebrafish. Chemosphere 252, 126498. doi: 10.1016/j.chemosphere.2020.126498
R Core Team. (2022). R: a language and environment for statistical computing. R Foundation for Statistical Computing, version 4.2.1 (Vienna, Austria) (Accessed July 10, 2022).
Rios-Fuster B., Alomar C., González G. P., Martínez R. M. G., Rojas D. L. S., Hernando P. F., et al. (2022). Assessing microplastic ingestion and occurrence of bisphenols and phthalates in bivalves, fish and holothurians from a Mediterranean marine protected area. Environ. Res. 214, 114034. doi: 10.1016/j.envres.2022.114034
Rowdhwal S. S. S., Chen J. (2018). Toxic effects of Di-2-ethylhexyl phthalate: an overview. Biomed. Res. Int. 1750368. doi: 10.1155/2018/1750368
Sala B., Gimenez J., Fernandez-Arribas J., Bravo C., Lloret-lloret E., Esteban A., et al. (2022). Organophosphate ester plasticizers in edible fish from the Mediterranean sea. Mar. Pollut. Hum. Expos. 292, 118377. doi: 10.1016/j.envpol.2021.118377
Salvaggio A., Tiralongo F., Krasakopoulou E., Marmara D., Giovos I., Crupi R., et al. (2019). Biomarkers of exposure to chemical contamination in the commercial fish species lepidopus caudatus (Euphrasen 1788): A particular focus on plastic additives. Front. Physiol. 10. doi: 10.3389/fphys.2019.00905
Sánchez-Avila J., Tauler R., Lacorte S. (2012). Organic micropollutants in coastal waters from NW Mediterranean Sea: Sources distribution and potential risk. Environ. Int. 46, 50–62. doi: 10.1016/j.envint.2012.04.013
Savoca D., Barreca S., Lo Coco R., Punginelli D., Orecchio S., Maccotta A. (2023). Environmental aspect concerning phthalates contamination: analytical approaches and assessment of biomonitoring in the aquatic environment. Environments 10, 99. doi: 10.3390/environments10060099
Schmidt N., Castro-Jiménez J., Oursel B., Sempéré R. (2021). Phthalates and organophosphate esters in surface water, sediments and zooplankton of the NW Mediterranean Sea: exploring links with microplastic abundance and accumulation in the marine food web. Environ. Pollut. 272, 115970. doi: 10.1016/j.envpol.2020.115970
Squadrone S., Berti G., Griglione A., Falsetti S., Nurra N., Sartor R. M., et al. (2023). Phthalate diester occurrence in marine feed and food (Mediterranean Sea). Environ. Sci. Pollut. Res., 1–6. doi: 10.1007/s11356-023-28361-8
Squillante J., Scivicco M., Ariano A., Nolasco A., Esposito F., Cacciola N. A., et al. (2023). Occurrence of phthalate esters and preliminary data on microplastics in fish from the Tyrrhenian sea (Italy) and impact on human health. Environ. Pollut. 316, 120664. doi: 10.1016/j.envpol.2022.120664
Staples C. A., Peterson D. R., Parkerton T. F., Adams W. J. (1997). The environmental fate of phthalate esters: a literature review. Chemosphere 35, 667–749. doi: 10.1016/S0045-6535(97)00195-1
Suaria G., Avio C. G., Mineo A., Lattin G. L., Magaldi M. G., Belmonte G., et al. (2016). The Mediterranean Plastic Soup: synthetic polymers in Mediterranean surface waters. Sci. Rep. 6, 37551. doi: 10.1038/srep37551
Tsiaras K., Hatzonikolakis Y., Kalaroni S., Pollani A., Triantafyllou G. (2021). Modeling the pathways and accumulation patterns of micro- and macro-plastics in the mediterranean. Front. Mar. Sci. doi: 10.3389/fmars.2021.743117
Tunçer S., Artüz O. B., Demirkol M., Artüz M. L. (2018). First report of occurrence, distribution, and composition of microplastics in surface waters of the sea of Marmara, Turkey. Mar. Pollut. Bull. 135, 283–289. doi: 10.1016/j.marpolbul.2022.113331
Tzatzarakis M. N., Karzi V., Vakonaki E., Goumenou M., Kavvalakis M., Stivaktakis P., et al. (2017). Bisphenol A in soft drinks and canned foods and data evaluation. Food Additives Contam.: Part B 10, 85–90. doi: 10.1080/19393210.2016.1266522
UNEP. (2016). Marine plastic debris and microplastics – Global lessons and research to inspire action and guide policy change (Nairobi: United Nations Environment Programme). Available at: https://wedocs.unep.org/20.500.11822/7720.
UNEP and BRS Secretariat, United Nations Environment Programme and Secretariat of the Basel, Rotterdam and Stockholm Conventions. (2023). Chemicals in plastics: a technical report (Geneva: United Nations Environment Programme). Available at: https://www.unep.org/resources/report/chemicals-plastics-technical-report.
Uren-Webster T. M., Lewis C., Filby A. L., Paull G. C., Santos E. M. (2010). Mechanisms of toxicity of di (2-ethylhexyl) phthalate on the reproductive health of male zebrafish. Aquat. Toxicol. 99, 360–369. doi: 10.1016/j.aquatox.2010.05.015
van der Lingen C. D., Bertrand A., Bode A., Brodeur R., Cubillos L. A., Espinoza P., et al. (2009). “Trophic dynamics,” in Climate Change and Small Pelagic Fish (Cambridge University Press, Cambridge).
Van Wezel A., Van Vlaardingen P., Posthumus R., Crommentuijn G., Sijm D. (2000). Environmental risk limits for two phthalates, with special emphasis on endocrine disruptive properties. Ecotoxicol. Environ. Saf. 46, 305–321. doi: 10.1006/eesa.2000.1930
Vered G., Kaplan A., Avisar D., Shenkar N. (2019). Using solitary ascidians to assess microplastic and phthalate plasticizers pollution among marine biota: A case study of the Eastern Mediterranean and Red Sea. Mar. Pollut. Bull. 138, 618–625. doi: 10.1016/j.marpolbul.2018.12.013
Wang H., Jiang L., Gu S., Wang X. (2021). Migration of bisphenol A from polyvinyl chloride plastics to solvents of different polarities and packaged food in China. Pack. Technol. Sci. 34, 127–137. doi: 10.1002/pts.2545
WHO. (2012). State of the science of endocrine disrupting chemicals 2012: summary for decision-makers (United Nations Environment Programme and the World Health Organization).
Wickham H. (2016). ggplot2: Elegant Graphics for Data Analysis (Houston, Texas, USA: Springer-Verlag New York). Available at: https://ggplot2.tidyverse.org
Wiesinger H., Wang Z., Hellweg S. (2021). Deep dive into plastic monomers, additives, and processing aids. Environ. Sci. Technol. 55, 9339–9351. doi: 10.1021/acs.est.1c00976
Wu N. C., Seebacher F. (2020). Effect of the plastic pollutant bisphenol A on the biology of aquatic organisms: A meta-analysis. Glob. Change Biol. 26, 3821–3833. doi: 10.1111/gcb.15127
Yang W. K., Chiang L. F., Tan S. W., Chen P. J. (2018). Environmentally relevant concentrations of di (2-ethylhexyl) phthalate exposure alter larval growth and locomotion in medaka fish via multiple pathways. Sci. Total Environ. 640, 512–522. doi: 10.1016/j.scitotenv.2018.05.312
Yılmaz D., Kalay M., Dönmez E., Yılmaz N. (2016). Assessment of biological effects of environmental pollution in Mersin Bay (Turkey, northeastern Mediterranean Sea) using Mullus barbatus and Liza ramada as target organisms. Environ. Pollut. 208, 361–370. doi: 10.1016/j.envpol.2015.10.002
Yuan S., Huang I., Chang B. (2010). Biodegradation of dibutyl phthalate and di-(2-ethylhexyl) phthalate and microbial community changes in mangrove sediment. J. Hazard. Materials 184, 826–831. doi: 10.1016/j.jhazmat.2010.08.116
Yuen B. B. H., Qiu A. B., Chen B. H. (2020). Transient exposure to environmentally realistic concentrations of di-(2-ethylhexyl)-phthalate during sensitive windows of development impaired larval survival and reproduction success in Japanese medaka. Toxicol. Rep. 7, 200–208. doi: 10.1016/j.toxrep.2020.01.009
Keywords: phthalates, bisphenol A, plasticizers, Mediterranean Sea, principal component analysis, fish
Citation: Marmara D, Brundo MV, Pecoraro R, Scalisi EM, Contino M, Sica C, Ferruggia G, Indelicato S, Velardita R, Tiralongo F and Krasakopoulou E (2024) Plastic additives in commercial fish of Aegean and Ionian Seas and potential hazard to human health. Front. Mar. Sci. 11:1334237. doi: 10.3389/fmars.2024.1334237
Received: 06 November 2023; Accepted: 13 February 2024;
Published: 12 March 2024.
Edited by:
Richard M. K. Yu, The University of Newcastle, AustraliaReviewed by:
Steven Jing-liang Xu, Hong Kong Metropolitan University, ChinaCopyright © 2024 Marmara, Brundo, Pecoraro, Scalisi, Contino, Sica, Ferruggia, Indelicato, Velardita, Tiralongo and Krasakopoulou. This is an open-access article distributed under the terms of the Creative Commons Attribution License (CC BY). The use, distribution or reproduction in other forums is permitted, provided the original author(s) and the copyright owner(s) are credited and that the original publication in this journal is cited, in accordance with accepted academic practice. No use, distribution or reproduction is permitted which does not comply with these terms.
*Correspondence: Dimitra Marmara, ZG1hcm1hcmFAbWFyaW5lLmFlZ2Vhbi5ncg==; ZGVtaW1hcm1hcmFAZ21haWwuY29t
Disclaimer: All claims expressed in this article are solely those of the authors and do not necessarily represent those of their affiliated organizations, or those of the publisher, the editors and the reviewers. Any product that may be evaluated in this article or claim that may be made by its manufacturer is not guaranteed or endorsed by the publisher.
Research integrity at Frontiers
Learn more about the work of our research integrity team to safeguard the quality of each article we publish.