- 1Red Sea Research Center, King Abdullah University of Science and Technology (KAUST), Thuwal, Saudi Arabia
- 2KAUST Reefscape Restoration Initiative (KRRI), King Abdullah University of Science and Technology (KAUST), Thuwal, Saudi Arabia
- 3Red Sea Protectorate, Egyptian Environmental Affairs Agency (EEAA), Egyptian Ministry of Environment, Red Sea, Egypt
- 4Marine Science Program, Division of Biological and Environmental Science and Engineering, King Abdullah University of Science and Technology (KAUST), Thuwal, Saudi Arabia
Sexual reproduction is a crucial process for reef building coral populations to maximize genetic diversity and recover from large scale disturbances. Mass spawning events by Acropora species represent critical opportunities for populations to persist, and a process that is increasingly exploited to actively restore degraded reefs. However, the timing and predictive capacity of coral spawning throughout the broad thermal and environmental regime of the Red Sea – a region also undergoing significant development and active reef restoration – remains patchy. We, therefore, conducted three parallel reef surveys in the central Red Sea (Al-Fahal Reef, Thuwal - Saudi Arabia) and the eastern (Shushah Island - Saudi Arabia) and western (Hurghada – Egypt) coast of the northern Red Sea. Surveys assessed the gravidity of gonads, spawning timing, alignment with the lunar cycle of 21 Acropora spp. (total n= 572 colonies) around the full moons of April and May 2023. Consistent with past observations, synchronous spawning was observed for Acropora spp. in both the central and northern Red Sea during April and May, respectively. Interestingly, corals spawned on the full moon in both Shushah and Thuwal sites. In contrast, corals in Hurghada were independent of the lunar cycle and spawned 7-9 nights before the full moon in May. By integrating our 2023 observations with the historical spawning events in Hurghada and Thuwal (2002-2022), we found that the deviation of spawning timing from the full moon day was correlated with absolute Sea Surface Temperature (SST) (earlier spawning before the full moon day, lower SST) and warming rate (earlier spawning, more rapid warming) in 6-weeks prior to spawning. As such, temperature pattern is likely one of the primary factors governing gamete release, among other factors, that likely influence spawning day within the lunar month. These correlations between SST metrics and spawning timing suggest a potential framework to predict future Acropora spp. spawning dates. Our observations demonstrate the importance of parallel efforts across borders to collect critical data needed to inform management strategies aimed at conserving and restoring coral reefs in this ecologically diverse region.
Background
Reef building corals rely on sexual reproduction to recover from disturbances and maintain critical population sizes (Baums et al., 2019). Some coral taxa have evolved as “brooders” whereby fertilized eggs are nurtured internally to release fully formed coral larvae, whilst others are “broadcasters” by releasing millions of eggs and sperm into the water for external fertilization during synchronized spawning events. Reproductive synchronization allows cross-fertilization amongst coral colonies to enhance genetic diversity and gene flow within coral populations (Nakajima et al., 2010; van der Ven et al., 2022), which in turn maximizes the transmission of adaptive traits among coral conspecifics (Baums, 2008; Baums et al., 2013). Coral spawning hence plays a pivotal role in enhancing the adaptability and resilience of corals against environmental stressors (van Oppen et al., 2015, 2017). Effective reef management strategies, therefore, depend on understanding sexual reproduction processes, including spawning timing and magnitude (Baird and Guest, 2009) as well as gamete (or larval) connectivity (King et al., 2023). Recent acceleration of intervention-based management has particularly catalyzed global efforts to improve knowledge in coral reproduction (Randall et al., 2020) needed to select for improved coral adaptive properties (Voolstra et al., 2021), such as heat stress tolerance (Buerger et al., 2020; Howells et al., 2021), but also overcoming the risk of genetic bottlenecks from asexual-based coral propagation alone (Chan et al., 2018; Baums et al., 2019; Harrison et al., 2021).
Successful and synchronized coral spawning events can be governed by environmental cues, such as water temperature, wind and current speed, as well as lunar cycles and photoperiod (e.g (Van Woesik et al., 2006; Keith et al., 2016; Monfared et al., 2023). Timing of coral spawning is sensitive to water temperature, where changes or rapid fluctuations in seawater temperatures can stimulate early or delayed, and thus disrupting, spawning timing (Keith et al., 2016; Lin and Nozawa, 2023). Lunar cycle, specifically the full moon phase, is commonly associated with coral spawning whereby moonlight intensity and duration coordinate the release of gametes amongst different colonies, enhancing the chances of successful cross fertilization (Kaniewska et al., 2015; Lin et al., 2021). Consequently, anthropogenically driven environmental perturbations, such as altered thermal profiles under ocean warming (e.g., Levitan et al., 2014) as well as artificial lighting through urbanization (Davies et al., 2023) can disrupt this synchronization and thus, dilute gametes concentration and decrease the probability of their fertilization success. Databases that capture widespread patterns of observed reproductive timing in situ (e.g., Baird et al., 2021) have aided forecasting of spawning timings over space and time (Van Woesik et al., 2006; Keith et al., 2016), and, in turn, a means to deliberately tune spawning times in ex situ coral husbandry (Craggs et al., 2017). Even so, knowledge of spawning timing remains patchy and undefined for many species globally, particularly in places such as the Red Sea, where repeated observations over time are required to empirically track change and hence validate predictions (e.g., Levitan et al., 2014; Neely et al., 2020).
The Red Sea spans 18° in latitude and harbors unique and diverse coral reefs that live in contrasting thermal and environmental regimes along the latitudinal gradient (Osman et al., 2018; Berumen et al., 2019) and between west and east coasts (Eladawy et al., 2022). Such diverse environmental conditions drive large variability in spawning timing, based on periodic observations across a few key sites over the past few decades (summarized in Ziegler et al., 2019). For example, spawning of Acropora species was recorded in late April and early May on the Egyptian Red Sea coast (Hurghada and Marsa Alam Hanafy et al., 2010; Attalla, 2016). In contrast, Acropora species on the central east coast of the Red Sea have been recorded to spawn from early/mid-April (Thuwal and Jeddah; Bouwmeester et al., 2015). In general, multi-species spawning around the full moon seems to be a common event for different coral genera, including different Acropora species (Hanafy et al., 2010; Bouwmeester et al., 2015, 2015; Kotb et al., 2018). Whilst observations, to date, have not been conducted systematically, collective evidence indicate that spring-time spawning starts earlier at south toward north in later days/months as waters warm up (Ziegler et al., 2019), and thus spawning in Eilat, at the Gulf of Aqaba (northern Red Sea), is delayed until summer (Shlesinger and Loya, 1985; Bouwmeester et al., 2015). Spawning of Pocillopora verrucosa occurred during the day around the new moon during the daytime in May/June, with synchronization between the east and west coast of the central Red Sea (Saudi Arabia and Sudan - (Bouwmeester et al., 2011), and again later (June/July) in the Gulf of Aqaba (Shlesinger and Loya, 1985). Patterns in temperature-regulated spawning timing are indeed compelling (see also Keith et al., 2016), but for the Red Sea remains confounded by inter-annual patchiness of past observations, and variability of the full moon month within each calendar year (Ziegler et al., 2019). As such, the ability to predict finer scale variation in spawning timing within and between Red Sea regions remain limited without more consistent and collective observations for any given year.
Coral restoration activities – including development of large ex situ coral husbandry facilities and collection of spawning gametes, are expanding rapidly throughout the Red Sea, but with limited knowledge of reproductive timing. Such knowledge gaps further limit the accuracy of reef connectivity models of larval dispersal and successful population connectivity (Cowen and Sponaugle, 2009; Donahue et al., 2015), which is important for the long-term resilience of reefs throughout the region (Hock et al., 2017), particularly where coastal development continues to accelerate (Aguilera et al., 2020). This is indeed the case for the rapidly developing NEOM region in the north-east Red Sea, Saudi Arabia (north-eastern Red Sea - Figure 1), where spawning has not been previously documented (Ziegler et al., 2019). We therefore conducted a parallel effort among three research teams from two countries (Egypt and Saudi Arabia) to observe in situ spawning timings – targeted for species of Acropora – in 2023 across three sites that represent the central Red Sea as well as the east and west coasts of the northern Red Sea (Figure 1). We also aim to understand the role of temperature as a key factor that determines the date of spawning within the lunar cycle using remote sensing data during past in situ spawning events. We observed synchronous, yet distinct coral spawning timing patterns across the Red Sea. This study highlights the potential impact of ocean warming and coastal development on coral reproduction, emphasizing the value of collaborative monitoring for effective coral reef conservation and restoration in the region.
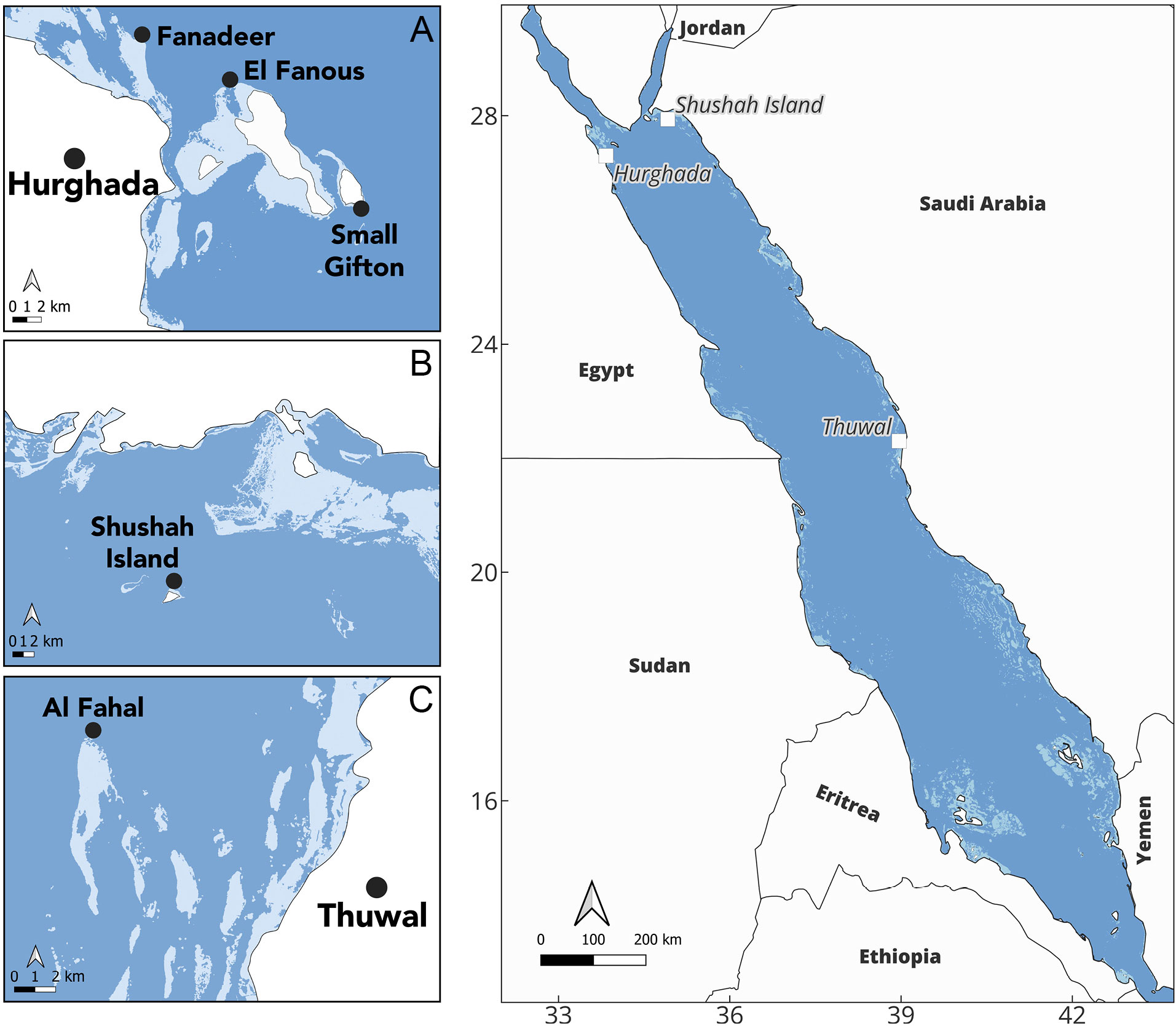
Figure 1 Map shows the study site locations along the central and northern Red Sea with the coral reef distribution extracted from the Allen Coral Atlas (shown in light blue). The coral spawning surveys took place at three distinct sites along the Red Sea: (A) the north-western coast of Red Sea at the Egypt, where we conducted surveys at three reefs in Hurghada: Small Gifton, Fanadeer, and El Fanous, (B) Shushah Island in the north-eastern of the Red Sea (Saudi Arabia), where they share similar latitude with Hurghada, and (C) Al Fahal Reef at Thuwal, which situated in the central coast of Saudi Arabia.
Materials and methods
Study sites and general survey approach
Different Acropora species were sampled for in situ gametogenic analysis, periodically, prior to and after the full moon of April (4th-7th) and May (4th-5th) 2023, when broadcast spawning is expected for the central and northern Red Sea based on the existing literature (Hanafy et al., 2010; Bouwmeester et al., 2015; Attalla, 2016). In parallel, in situ spawning observations were conducted over 3-4 nights during the full moon phase, commencing pre-sunset (ca. 17:30) to 23:30 local time. Survey sites were Hurghada, encompassing Small Gifton Island (27.185895N, 33.983358E) and Fanadeer (27.299142N, 33.831940E), located in the north-western Red Sea (Egypt) (Figure 1A), Shushah Island (27.93799N, 34.912887E), located in the north-eastern Red Sea (NEOM, Saudi Arabia) (Figure 1B), and Al Fahal Reef, at the Coral Probiotics Village (22.305072N, 38.964480E), located in the central Red Sea (Thuwal, Saudi Arabia) (Figure 1C). All sites are located offshore (5-20km from the shoreline) and were selected to include diverse coral assemblages with high abundance and diversity of Acropora colonies.
SCUBA diving surveys for gametes status were conducted randomly at all three sites in both months, targeting colonies of different Acropora species occurring at 3-9m depth across reef areas of approximately 100m2. Most surveys were conducted during daytime, except for Al Fahal reef where surveys were conducted at night. Acropora colonies were randomly selected based on the availability in the surveyed site. They were first photographed for later species identification ensuring images would depict the colony growth form, and the radial and axial polyps shape and arrangement. Branches were selected towards the center of the colony and removed using pliers at the middle of the branch to maximize locations that are likely gravid (Wallace, 1985). Branches (3-5cm) were then carefully removed, and macro-photographs of the broken side for both branch and colony were taken (Olympus TG6 with Auto white balance setting, see Figure 2). Images were then used to classify gametogenesis (Figure 2). Oocyte bundle maturity status follows a distinct transition in egg size and coloration, where the most matured oocytes exhibit distinct red coloration (Vargas-Ángel et al., 2006; Chiu et al., 2020). We classified observations where gametes were denoted; i) “immature” when oocytes were white, ii) “mature or gravid” as pink or red, ii) “absent” when oocytes are absent or undetectable (Figure 2), to account for differences in oocyte coloration reports across research groups/sites. These data were then used to determine the percentage of colonies encountered that were gravid over time. Depth, date, and time for each sample were also documented. In addition, night-time SCUBA surveys for gamete bundle setting and release were similarly conducted haphazardly in the same areas as daytime surveys, except for Hurghada where additional sites were surveyed only at night (Fanadeer and El Fanous). Divers typically conducted repetitive surveys one hour after the sunset from 19:00-22:30 local time (sunset approximately at 18.00), to enable constant observation at sites using red filtered torches. Colonies observed with (a) gamete bundles at the polyp opening (“setting” and ready to release) or (b) gamete bundles release were photographed for later visual identification and the time of observation was noted.
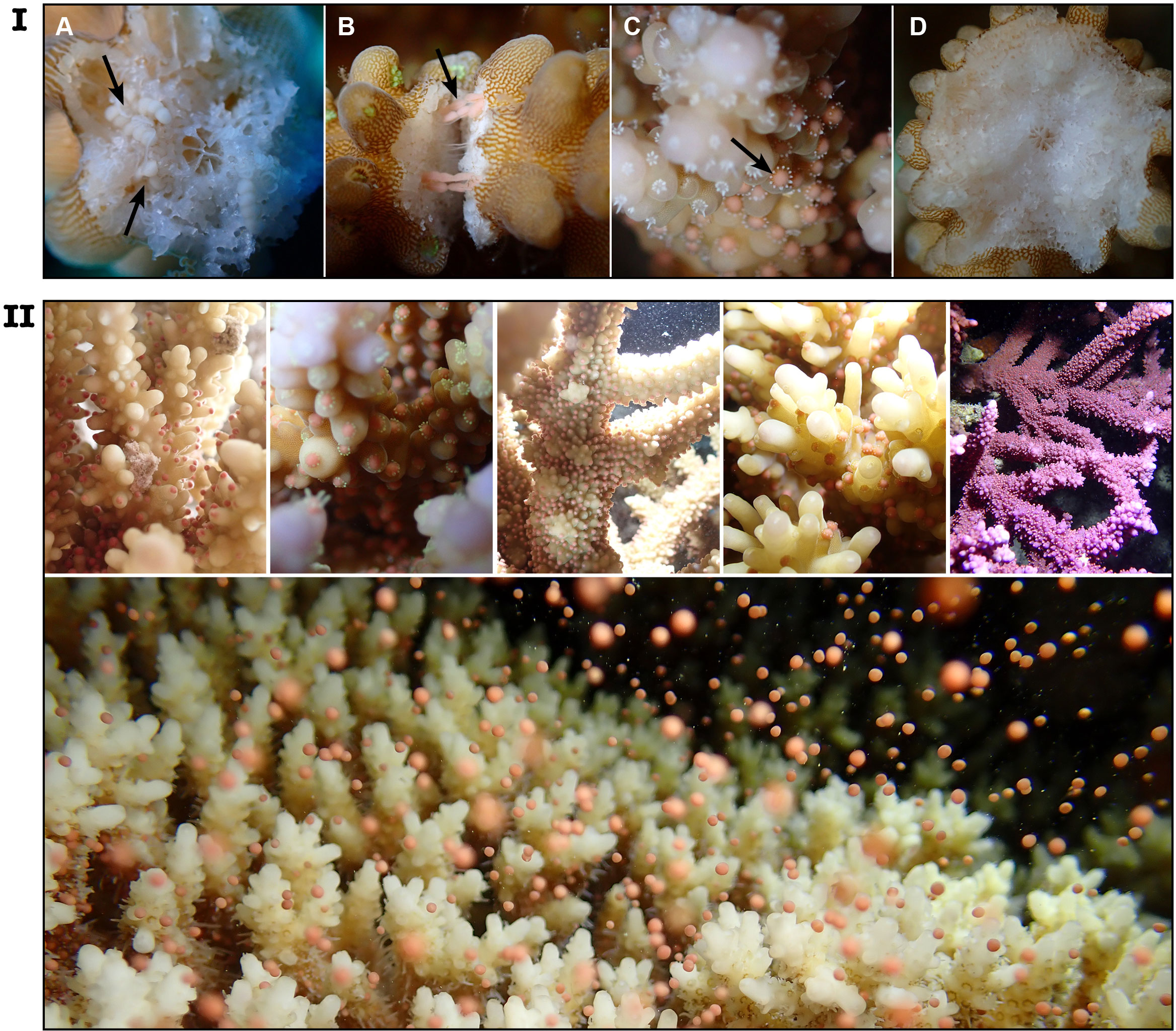
Figure 2 The panel presents in situ photographs illustrating various stages of gametogenesis and in situ spawning events in Acropora species observed during our surveys. Panel (I) provides a visual representation of different stages of gametogenesis (indicated by black arrow) and demonstrates the following; (A) gametes with a white coloration, indicating the presence of visible but immature oocytes, (B) development of gametes transitioning into a pink/red hue, as a sign of maturity, (C) gametes situated at the mouth of the coral polyp, showing their readiness for release gametes into water, (D) absence of visible gametes suggesting that all gametes have been released or lack of any oocyte development. Panel (II) exhibits setting and release of gametes from different mother Acropora species along the Red Sea, forming distinct gamete bundles. Photo credits: Eslam O. Osman, Alexander Stead.
Location-specific gametogenesis and spawning survey methodologies
According to literature, coral in the central Red Sea spawn during the full moon nights of early April, while spawning in the northern Red Sea occurs in late April or early May (Ziegler et al., 2019). Consequently, the surveys started earlier in Shushah Island due to the unknown spawning time and Thuwal, which typically starts earlier in April. General survey approaches were employed consistently across the three sites in May 2023. However, slightly different methods occurred between sites only in April, primarily due to location-specific operational conditions and site accessibility, using the procedures above. Differences resulting from this variation across sites are summarized as follows:
i. Thuwal (Al-Fahal Reef) – Spawning timing had been previously documented in Thuwal for species of Acropora during April/May (Bouwmeester et al., 2015, 2016). Therefore, visual surveys were conducted in April (4th-7th) two nights before and one night after the full moon (6th April), with the aim of exclusively identifying setting colonies and/or gamete bundle release. No gametogenesis surveys were conducted in April. In contrast, gametogenesis surveys in May were conducted following the above methods and spanned two nights; one night before the full moon (4th May) and the full moon night (5th May).
ii. Shushah Island – Gametogenesis surveys were conducted at regular intervals throughout April to early June since spawning timing for this region was unknown. Surveys typically spanned 50-100 colonies of 10-15 different notional species of Acropora. Nighttime setting/release surveys were also conducted during the full moon nights in April (4th-7th) and May (4th-5th).
iii. Hurghada – Past field observations documented spawning of Acropora spp. in Hurghada typically occurs around the full moon in late April (after 20th) (Hanafy et al., 2010; Ibrahim et al., 2021) and/or early May (Attalla, 2016). Therefore, gametogenesis surveys were conducted on 19th April (i.e., 15 days before the May full moon) on various Acropora morphologies (2-3 colonies each) that have previously been documented to spawn (Kotb et al., 2018). Unfortunately, no photographs could be taken for those colonies, and thus no later identification could be performed (see “Discussion” below). However, most investigated colonies exhibited mature oocytes on 19th April, and hence, it was anticipated that spawning would occur around the next full moon nights, as previously documented. Therefore, a second survey was conducted the following full moon in May (4th and 5th) to evaluate gametogenesis, where nine (n=26 colonies) and eleven Acropora species (n=25 colonies) were examined at small Gifton and Fanadeer, respectively. Surveys for gamete setting and release were also conducted at Al-Fanous without gametogenesis check (Figure 1C).
Identification of Acropora colonies
Acropora identification is challenging due to a combination of high species diversity and morphological plasticity of the traditional diagnostic characters (Wallace, 1999; Wallace et al., 2012). In the Red Sea, the situation is even more complex due to a high endemism rate as corals may exhibit characteristics or morphs that lead to different species compared to their morphologically similar, but genetically distinct, Indo-Pacific counterparts (Arrigoni et al., 2016; Dibattista et al., 2016; Berumen et al., 2019). However, boundaries were solved recently for a set of Acropora species through a novel genomics approach (Ramírez-Portilla et al., 2022) that opened new research avenues to understand the genus diversity (Bridge et al., 2023). In this study, Acropora species boundaries detection and identification were based on colony growth form, axial and radial corallites shape, and their arrangement from in situ imaging following the currently available taxonomic nomenclature including type morphology (Wallace, 1999). For colonies with morphologies matching the description of taxa originally described from the Red Sea, the full identification was provided. For colonies closely resembling the morphology of presumably widespread species with type locality in the wider Indo-Pacific region, the non-taxonomic identifier cf. was used to acknowledge a level of uncertainty (Sigovini et al., 2016). Finally, in the case of distinct morphologies that not matching the morphological traits of any species currently considered valid, an open nomenclature qualifier was used followed by sensu and the published reference that previously reported the morphology.
At Shushah Island, a wide variety of species and color morphs were observed as a result of the extensive sampling of colonies. To streamline the taxonomy process, we therefore initially grouped notional species before taxonomic assessment using representative in situ photographs for each notional species. We further assigned color morphs after the taxonomy to ensure a cautious and precise taxonomic assignment for various morphospecies. Specifically, Acropora sp. 1 was a binned group with apparent growth variations (Figure S1), Acropora cf. humilis was a binned group of all apparent morphological variations for this form (Figure S2), Acropora cf. downingi was a binned group of tabular and complex branched morphologies (Figure S3). In addition, the three main color morphs of Acropora hemprichi (beige, brown and blue) were retained as separate groups to evaluate uniformity in spawning timing amongst the variations for this group.
Sea surface temperature variability and prior spawning observations
To investigate the potential impact of seawater temperature on maturation and/or spawning timing at each site, in situ seawater temperature was measured throughout the spawning period at Al Fahal and Shushah Island, but not at Hurghada. At Al-Fahal reef, a multiparameter CTD sensor (Ocean Seven 310, Idronaut, Italy) recorded seawater temperature at regular intervals of time (30 minutes). At Shushah Island, Onset® HOBO loggers (Pendant® UA-002-64) were deployed to measure seawater temperature at intervals of 2 minutes. Both temperature loggers were positioned at a depth of 7-10 meters during the months of February through June 2023. To ensure a comparable seawater temperature metric across all sites, where loggers varied in brands and were unavailable at Hurghada, we acquired weekly sea surface temperature (SST) data from the Aqua MODISA (The Moderate Resolution Imaging Spectroradiometer) satellite (4 microns at night, 4km resolution). This data covers the period from February to June 2023 and was obtained from Giovanni website (https://giovanni.gsfc.nasa.gov/giovanni/).
In addition, we searched the literature for previous spawning timing reports specific to the areas of interest for our current observations (e.g., those summarized in Ziegler et al., 2019). Our search was limited to the period between 2002 to the present (see Table S1). This time frame was chosen because remote sensing SST data was not accessible prior to 2002, and the period after 2000 exhibits a more distinct SST profile compared to the period before (Osman et al., 2018; Eladawy et al., 2022). We also extracted historical remote sensing SST data, as above, for these sites and periods corresponding to the observed in situ spawning. This data covered a period of 6-weeks for each recorded spawning event at Hurghada (n=6 spawning event observations) and Thuwal (n=4 spawning event observations – see Table S1). This represents an average timeframe which oocyte maturation occurs progressively, and spawning time can be influenced by environmental variables (Sakai et al., 2020).
Data analysis
Gravidity categories were conservatively assigned as “present” or “absent” to account for differences in photograph coloration due to inconsistent white balancing. Percentage of gravid colonies for each species and/or site were plotted as a heatmap. All sea surface temperature (SST) were derived from remote sensing to have a valid comparison among sites, while data obtained from loggers were used only to validate the remote sensing SST trends. Remote sensing SST in 2023 was extracted and subsequently tabulated and plotted against daily average of in situ temperature data. For historical SST data during previous spawning events, we employed two standardized metrics to evaluate the historical thermal profiles for each site: i) Absolute SST mean over a six-week period was calculated to examine the variations in the minimum temperature required to trigger spawning at each site, ii) Warming rate was calculated from a linear regression of SST and time for the 6-week period prior to each spawning event to test whether differences in average rate of heating corresponded with the timing of spawning. We tested whether the difference in SST metrics (absolute SST and warming rate) was significantly different between Hurghada and Thuwal using a generalized linear model (GLM) in “R” (R Developement Core Team, 2017). Further, the absolute differences between SST at the time of spawning and the SST recorded six weeks prior (ΔSST) were calculated. In addition, absolute SST and warming rates were plotted against deviation from full moon night of historical spawning events to understand the correlation (using “ggpmisc” package) and whether rapid changes in SST prior to spawning can predict spawning time. All data were plotted using “ggplot2” package in “R” language.
Results
Here, we describe the reproductive status of 572 colonies belonging to 21 Acropora morphological species across three sites located along the central Red Sea as well as the east and west coast of the northern Red Sea. Of these species, Acropora eurystoma (Klunzinger, 1879) and Acropora maryae (Veron, 2002) are considered endemic to the Red Sea, while four distinct Acropora morphs were not identified to species level because they do not match the typical morphology of any currently recognized nominal species. We surveyed 13 Acropora species each at Hurghada and Shushah Island, and 12 Acropora species at Thuwal. Only two species, Acropora hemprichii (Ehrenberg, 1834) and Acropora sp. 1 sensu Al Tawaha et al. (2019), were examined across all sites (see Figure 3), whereas other species were site-specific (Figure 3). The remaining 12 Acropora morphospecies showed oocyte maturity, but were not observed spawning (Table S2) and thus, spawning timings of these taxa are broadly estimated based on oocyte maturity ranking. Whilst several Acropora species were examined across sites, they showed differences in spawning time (Figure 4).
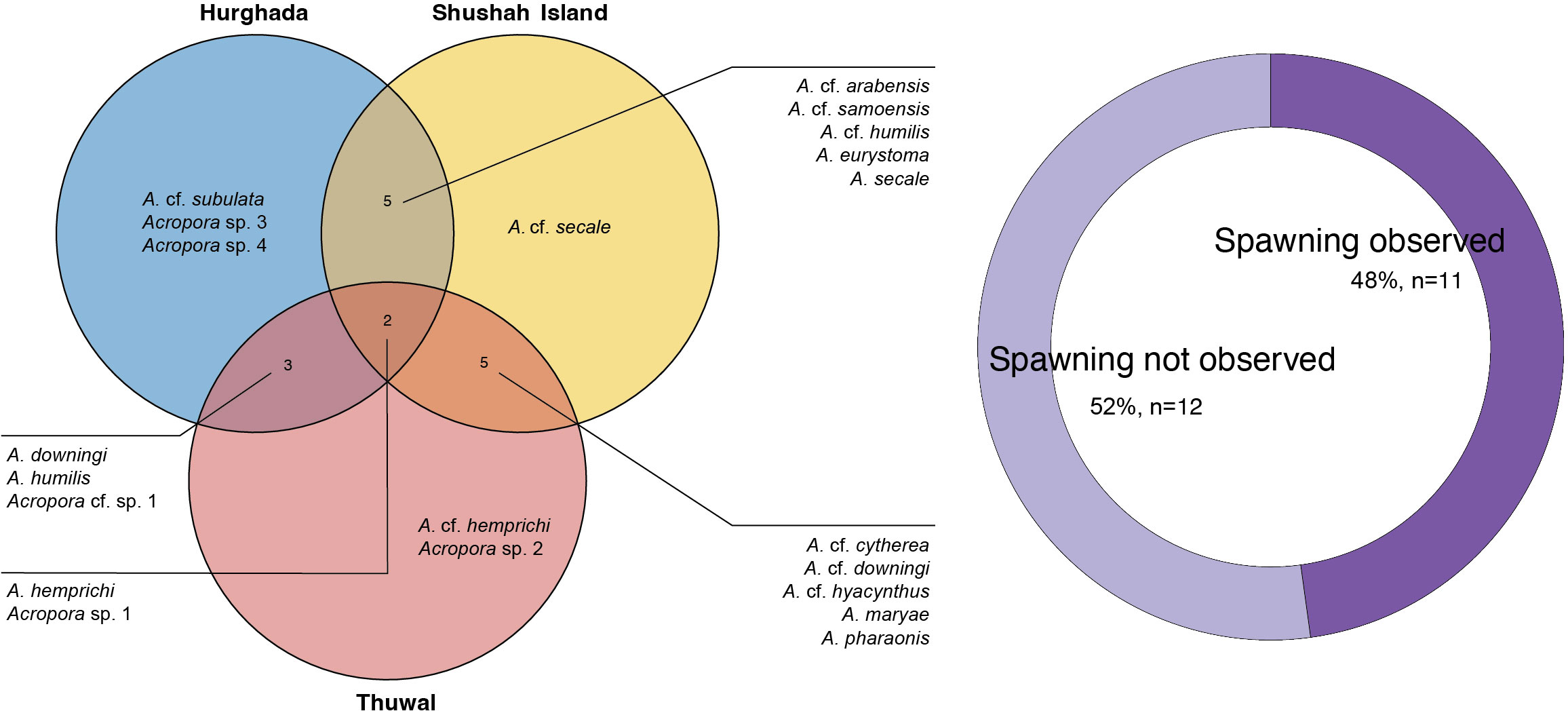
Figure 3 Panel represents information about the number of species that were examined for gametogenesis at three studied sites across the central and northern Red Sea. Venn diagram (left) depicts the distribution of Acropora species at each site, where most of the species were found in common among sites, particularly Acropora hemprichii and Acropora sp. 1 that were found in all sites. However, there were also instances where certain Acropora species were exclusively observed in specific sites. Donut plot (right) illustrates the percentage of observed coral species that underwent in situ spawning compared to the species that were assessed for gametogenesis but were not observed during the spawning event.
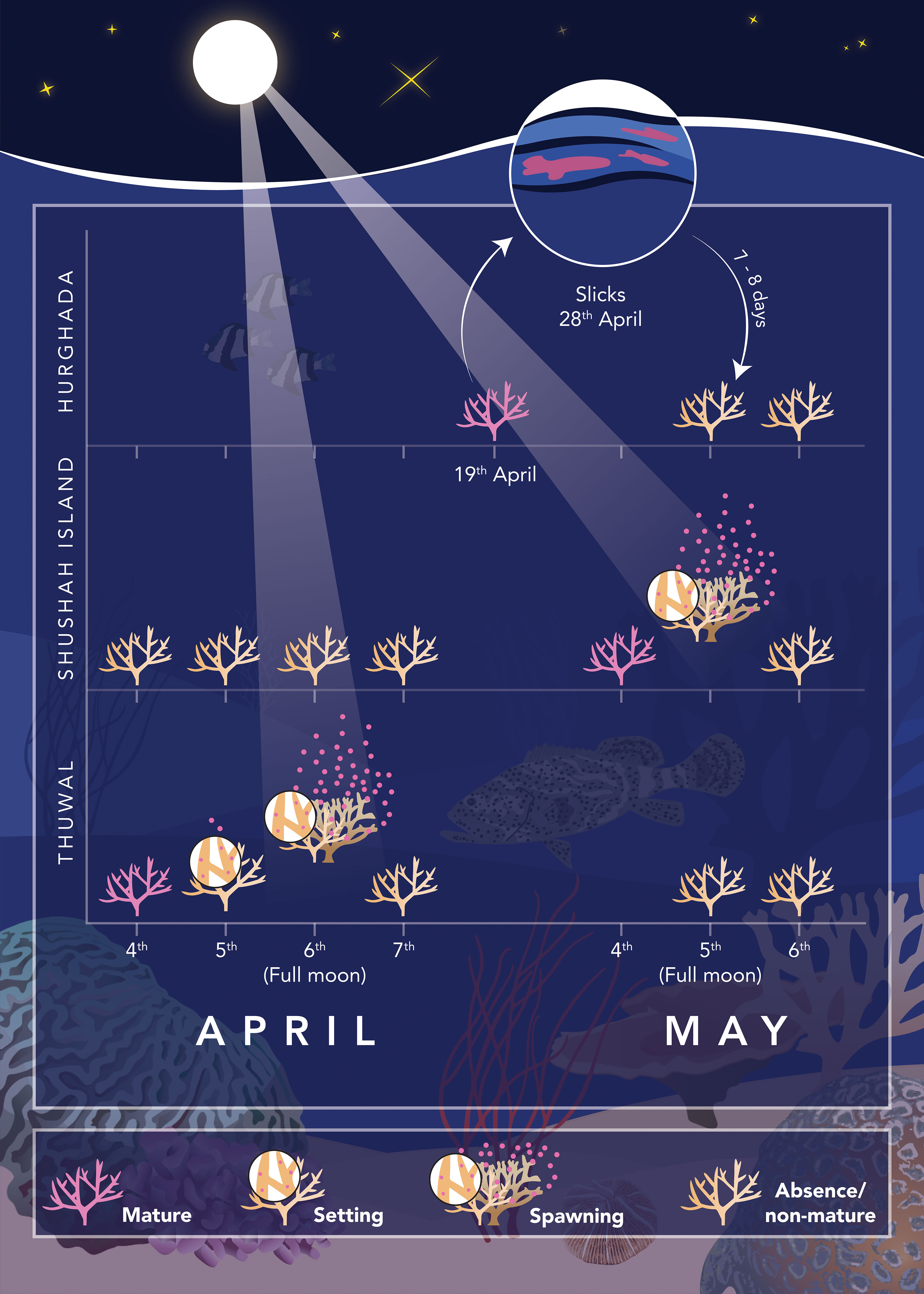
Figure 4 Schematic illustration shows the temporal pattern of Acropora spp. spawning timing across the three surveyed sites located along the central and northern (east and west) coasts of the Red Sea. The data reveals that the spawning event was initially observed in the central Red Sea (Thuwal on Al Fahal Reef) during the Full Moon nights of April. Some coral released few gametes in the night before the full moon (April 5th), but the main spawning event was observed during the full moon night (April 6th). In contrast, there was no coral spawning witnessed in the northern Red Sea during April. In situ spawning at Shushah Island was observed during the Full Moon of May 5th. However, there was no gametogenesis check during moon night of April at Hurghada, but it takes placed on 19th April where all investigated Acropora were mature and ready to release. Gametogenesis checks confirmed that corals at Hurghada had already completed spawning before the full moon in May, as no in situ spawning was observed at that location. This observation aligned with slick observations on the coast of Hurghada on April 28th, indicating that corals in Hurghada had released gametes 7-9 days before the full moon in May.
Gametogenesis and in situ observation of coral spawning
Thuwal (Al-Fahal Reef)
Spawning was first observed at Al-Fahal reef (Central Red Sea), during the full moon nights of April (5th and 6th). Six Acropora species (total n=16 colonies) were observed in situ “setting” and subsequently releasing gamete bundles between 22:30 to 23:30hrs: A. hemprichii, A. cf. hemprichii, A. cf. downingi, A. sp 1., A. sp. 2, and Acropora cf. sp1 (Figure S4, Table S2). The main spawning event occurred during the full moon night (6th April), with several colonies (A. hemprichii, A. cf. downingi, and A. sp. 1) “dribbling” some gamete bundles one night (5th April) before full moon (Figure 4). No spawning was observed on April 7th indicating that spawning at Al Fahal reef was predominantly synchronized across multiple species. Gametogenesis surveys were initiated during the full moon nights of May. Observations from 37 colonies belonging to 11 Acropora species (5th-6th May) demonstrated that 75% of the investigated colonies (n=28) were not gravid with undetected oocytes, confirming they had already fully spawned. Notably, A. cf. humilis (n=5 colonies) consistently exhibited white oocytes, consistent with lack of prior in situ spawning observations for this species in April. Furthermore, few colonies of A. hemprichii and A. sp. 2, (n=2 colonies each), two species that already had been observed to release gametes in April, exhibited pale pink gonads. As such, the primary spawning event for this site appears to have occurred in April for most species of Acropora species, but few colonies and species retained later spawning.
Shushah Island
Random gametogenesis surveys at Shushah Island (northeast Red Sea) demonstrated that several coral species exhibited a cumulative increase in gravidity extent (% of corals surveyed) throughout April-June (Figure 5). While spawning has not been observed in Shushah Island during April, in situ observations confirmed spawning on the full moon night of May (5th) and a complete absence of oocytes from subsequent gametogenesis surveys 14 days after May full moon (19th May) (Figures 4, 5, Table S2); specifically, A. eurystoma, A. cf. cytherea, and A. sp. 1. Colonies of A. cf. downingi also generally followed this pattern, but with a single positive observation for gravidity (of a single colony surveyed) on the 19th May likely skewing the perceived timings (see Table S3). Peak % gravidity extent was also observed pre full moon (3rd) and with spawning observed around full moon for A. hemprichii (beige), A. cf. humilis, and A. secale. However, subsequent gametogenesis surveys on May 19th indicated that some colonies were still gravid (i.e., A. cf. humilis, and A. secale) or could not confirm their gravidity status (no observations, A. hemprichii (beige)). Furthermore, gametogenesis surveys for these three species confirmed complete absence of oocytes by mid-June. Spawning was also observed around the full moon of May for species A. pharaonis, A. maryae, A. cf. secale, but gametogenesis surveys for these taxa were few and therefore May peak spawning could not be confirmed. In all of these cases, spawning was observed for multiple colonies only on the full moon (May 5th) (and not the day before) and at either 19:30 (A. eurystoma) or 23:00 onwards (all others) suggesting mass and synchronous multi-species spawning. Unfortunately, site restrictions beyond this time prevented us to further confirm if additional spawning for these species continued during this lunar period (Figure 5).
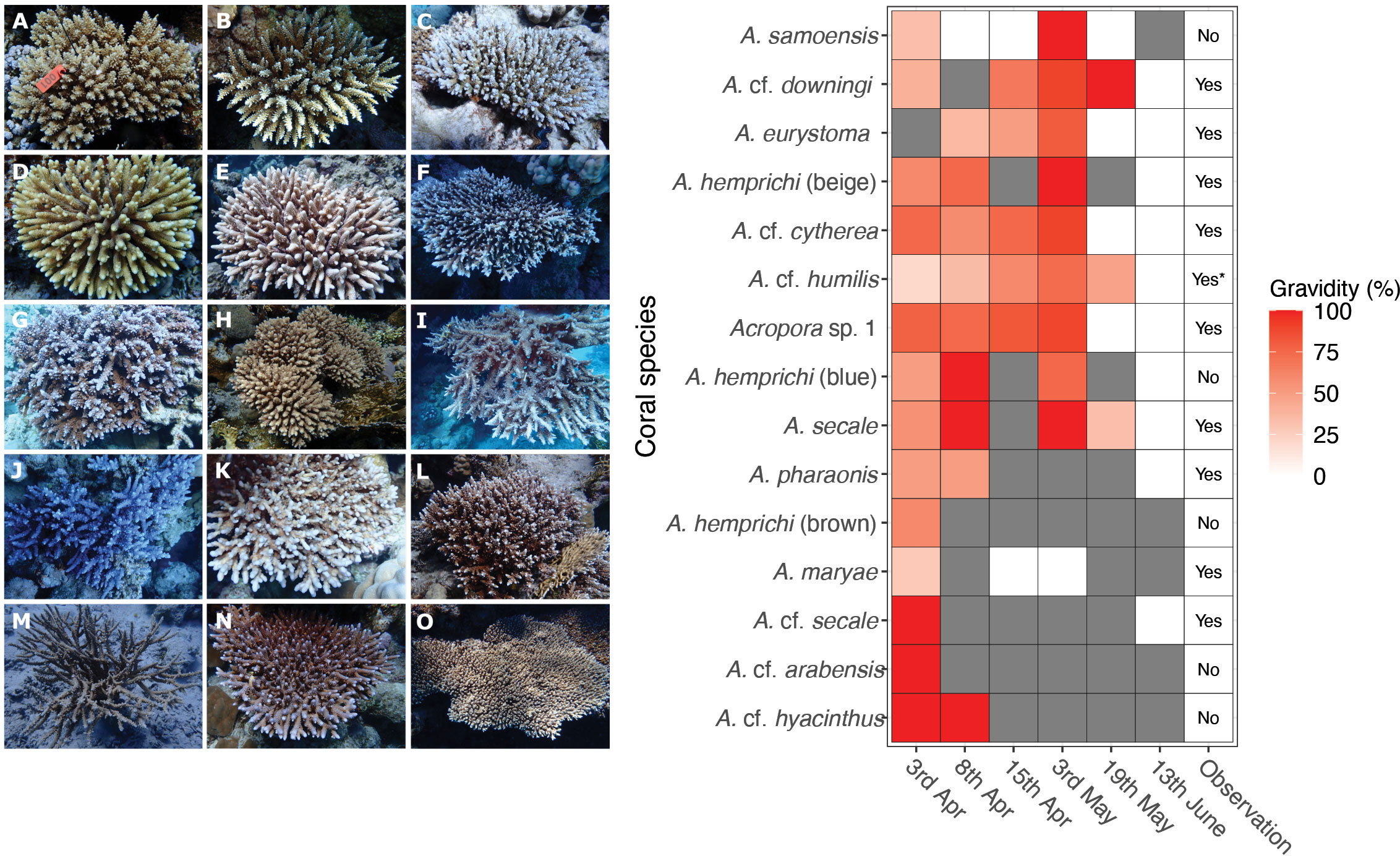
Figure 5 Panel displays in situ photographs of Acropora species surveyed at Shushah Island, along with their respective gravidity statuses recorded at different time intervals. In situ photograph (Left) for each of the Acropora species (n=12 include three morphotypes for A. hemprichii) examined at Shushah Island, while heatmap (Right) illustrates time series of gravidity checks, with colors denoting the percentage of oocytes gravidity, over six time points. Notably, certain time points exhibited lack of observations for some species (indicated by the gray color), while in situ spawning for some colonies were not observed as indicated in the observation column. Species are: (A) A. secale, (B) A. cf. secale, (C) Acropora sp. 1, (D) A. cf. arabensis, (E) A. cf. humilis, (F) A. cf. cytherea, (G) A. hemprichii (beige), (H) A. eurystoma, (I) A. cf. downingi, (J), A. hemprichii (blue), (K) A. maryae, (L) A. hemprichii (brown), (M) A. pharaonis, (N) A. samoensis, (O) A. cf. hyacinthus.
Several species that were included in the gametogenesis surveys were not observed to spawn in May (i.e., A. cf. arabensis), despite it appeared gravid before the 6th of April full moon, or after full moon (Acropora cf. hyacinthus - see Figure 5). A. hemprichii (brown), A. hemprichii (blue) and A. samoensis were also not observed to spawn. In the case of A. hemprichii (brown, blue), this was likely confounded by patchy gametogenesis survey data; however, A. samoensis was observed to be highly gravid pre full moon (3rd May) and then entirely absent of ooctyes post May full moon (19th) suggesting this species – and potentially others – simply went unrecorded for setting or release on the 4th and 5th May. Finally, whilst A. cf. humilis was observed to spawn in May, these observations were few (2 colonies), and where A. cf. cytherea represents a likely cluster complex of different digitate species of Acropora. The remaining high gravidity (~50% from 19 colonies) on May 19th but absence of gravidity after the June full moon indicates that species likely spawned between May 19th but before the full moon in June.
Hurghada
Survey efforts at Hurghada (northwest Red Sea) did not observe any in situ spawning (i.e., egg setting or release) events during the full moon of May at any site (Small Gifton, Fanadeer, and Al Fanous). Gametogenesis surveys in mid-April (19th) at Small Gifton indicated that a majority of the investigated Acropora species (n=12 species, 2-3 colonies each) were gravid and ready to spawn. Notably, no photographs were captured for these species because of technical issues, and hence no standard taxonomic identification could be performed. These data were therefore not included in our formal analysis in terms of total number of investigated species, but site and local knowledge suggest these taxa were A. cf. gemmifera, A. cf. digitifera, A. cf. valida, A. cf. nasuta, A. cf. selago, A. cf. latistella, A. cf. cytherea, A. cf. clathrata, A. cf. squarrosa, A. cf. hemprichii, A. cf. formosa, A. cf. acuminata. All exhibited gravid oocytes and therefore ready for spawning. A subsequent survey that captured photographs and therefore taxonomy during the May full moon (5th and 6th) was achieved for 46 colonies belong to 13 species at two sites (21 in Small Gifton and 25 in Fanadeer – see Table S2), and revealed that none of them were gravid, thereby indicating that Acropora spp. had already released their eggs before the full moon, regardless the precise spawning day. This outcome was coincided with reported mass spawn slicks off the coast of Hurghada (see Figure S5) observed 7-10 nights (28th April) before the full moon of May (5th), which may have come from Acropora species that presumably spawned 1-2 days prior to the slicks being recorded (Figure 4). Thus, spawning at Hurghada did not appear to coincide with the full moon, in contrast to the spawning observations on the east coast site (Shushah Island) at the full moon.
Thermal profile across surveyed sites
As expected, sea surface temperatures generally increased from February to June, with the central Red Sea displaying higher temperatures compared to the northern Red Sea sites (Figure 6). Notably, in situ temperature data and remote sensing data exhibited remarkably similar patterns (Figure 6), indicating that remote sensing provided a reliable proxy to cross compare sites, where in situ temperature measurements were lacking for Hurghada (Figure 6). Based on remote sensing SST, mean (±SD) temperatures for April were 26.8 ± 0.9°C at Al Fahal reef versus 23.6 ± 0.4°C and 22.8 ± 0.3°C for Shushah Island and Hurghada, respectively. By May, mean SST increased to 28.3 ± 0.9°C at Al Fahal, 25.3 ± 0.7°C at Shushah Island, and 24.3 ± 0.6°C at Hurghada. The warming rate in 2023 for 6-weeks prior to the spawning date at Shushah Island (0.43°C per week, range= 22.8-25°C, ΔSST=2.2°C) was higher than Hurghada (0.24°C per week, range= 22.5-23.9°C, ΔSST=1.4°C). It should be noted that the linear regression model returned a stronger fit for Shushah (R2 = 0.99) compared to Hurghada (R2 = 0.7), suggesting SST increases at Shushah were more consistent over time over the 6-week period (Figure 6).
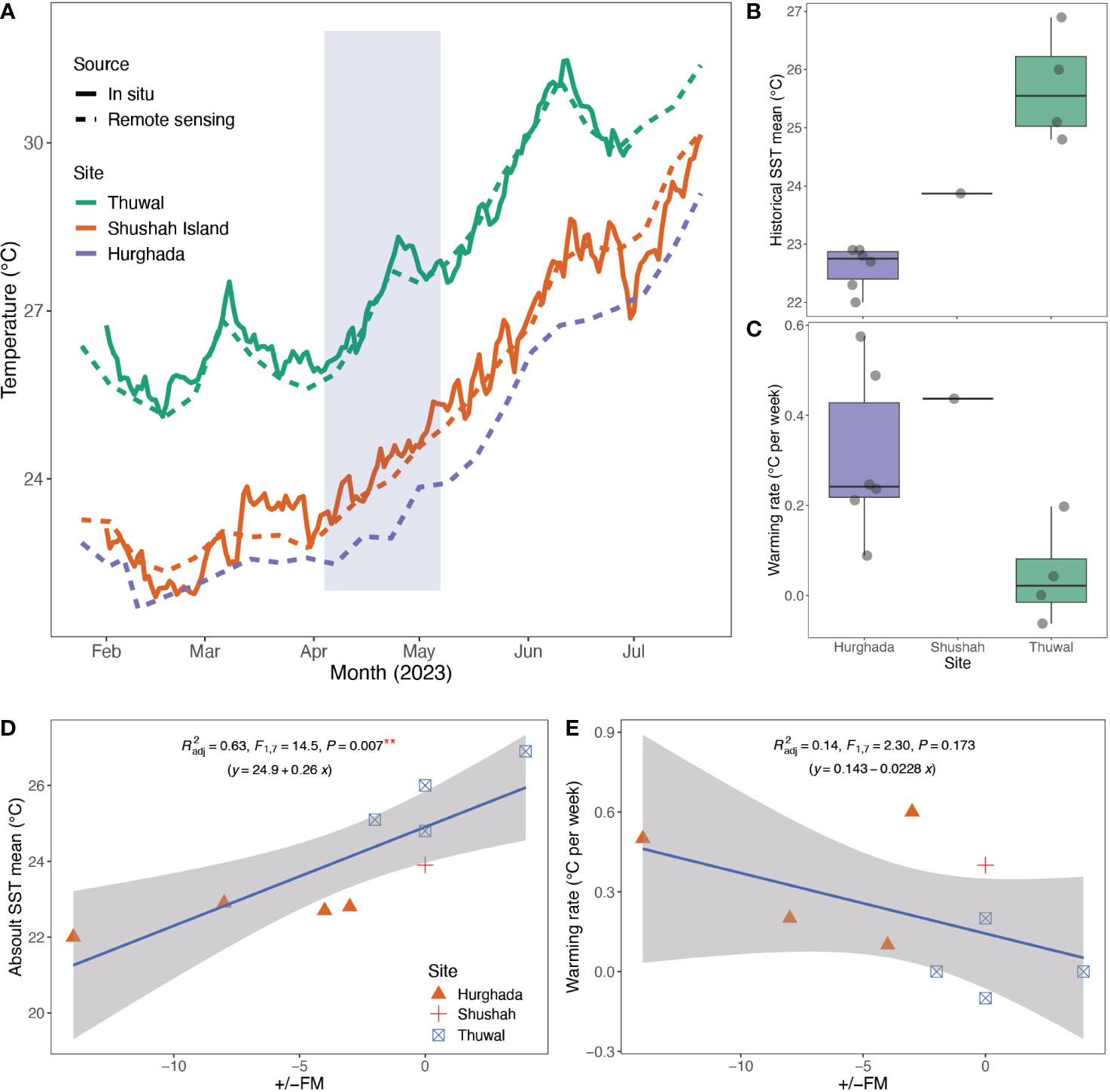
Figure 6 Panel presents the profile of Sea Surface Temperature (SST) at the study sites. (A) SST were collected at each study site from February to July 2023, and data were obtained through; i) remote sensing using weekly data from the Aqua MODISA satellite, and ii) daily average in situ temperature measurements for Shushah Island (HOBO logger) and Thuwal (Multiparameter CTD sensor), but unfortunately, data was lacking for Hurghada. The data revealed that SST was higher in April in the central Red Sea (Thuwal) compared to the northern region (Shushah Island and Hurghada). Notably, Shushah Island exhibited higher temperatures than Hurghada, even though spawning in Hurghada commenced before the full moon in May, while Shushah Island initiated spawning during the full moon night (blue shade show the spawning range). This difference in spawning behavior at Hurghada coincided with a rapid increase in temperature, while Shushah Island showed a more gradual increase in sea surface temperature. (B) The boxplot illustrates historical remote sensing data (2002-2023) collected from the Thuwal, Shushah, and Hurghada regions include our observations in 2023, where frequent in situ spawning events have been observed. This data represents the mean temperature for the 6-weeks leading up to the observed spawning events. The historical data reveals that Thuwal tends to experience higher temperatures at the time of spawning compared to Hurghada, where coral spawning occurs at lower temperatures. (C) Boxplot illustrates the warming trends in the three sites over 6-weeks prior spawning observations that showed higher warming rates in Hurghada than Thuwal. (D) Point plots show the relationship between historical absolute SST and warming rates (E) versus deviation of spawning day from the full moon (+/-FM) for the historical spawning events along the Red Sea. Correlation points for Marsa Allam and Dahab were removed as the exact spawning day was not in situ observed.
Historical spawning records indicate that spawning in Hurghada typically occurs from late April (20th) to early May (8th), regardless of the full moon phase. Spawning timing for Hurghada have shown a deviation ranging from -3 to -18 days before the full moon night, based on four in situ observations (Table S1). In contrast, corals at Thuwal predominantly spawn in early April, with the latest recorded date being April 25th in 2013. The deviation from the full moon night for Thuwal spawning ranges from -2 to +4 days based on four in situ observations (see Table S1). Analysis of SST for 6-weeks prior to these historical spawning events confirmed that mean temperature was always significantly higher for Thuwal (25.7 ± 1°C, n=4) compared to Hurghada (22.6 ± 0.4°C, n=6 – GLM, df=1, F=54.6, p<0.001), aligning to our observations during 2023 (Figure 6). However, the warming rate for Hurghada (0.3 ± 0.2°C per week) was significantly higher than for Thuwal (0.04 ± 0.1°C per week – GLM, df=1, F= 6.4, p<0.04). Our observations for warming rate versus spawning SST at Shushah Island in 2023 were more similar to the historical patterns for Hurghada (Figure 6). Thus, it appears spawning timings coincide with slower warming prior to a relatively high SST in the central Red Sea versus more rapid warming to a relatively lower spawning SST in the northern Red Sea. Indeed, a significant positive correlation was observed between absolute historical SST with the deviation from full moon night (Lm, adj. R2 = 0.55, F=10.8, p=0.01), while a markedly negative correlation between the warming rate and deviation from full moon night, although this latter trend was not significant (Lm, adj. R2 = 0.15, F=2.4, p=0.2, see Figure 6).
Discussion
Coral sexual reproduction events and notably mass multi-species synchronized spawning events are essential processes for maintaining the health and recovery of coral reef ecosystems (Baums, 2008; Baums et al., 2019). Such “mass” spawning fosters genetic diversity (Nakajima et al., 2010) and can aid active recovery of degraded reef areas (Randall et al., 2020; Humanes et al., 2021) to overcome population bottlenecks. Whilst this process is often synchronized with lunar cycles, other environmental cues fine tune the exact spawning timing, particularly for corals that thrive across large thermal regimes, such as western Australia (Gilmour et al., 2016), the Great Barrier Reef (Sakai et al., 2020), and presumably the Red Sea. Past spawning observations of Red Sea corals have been restricted to few sites and time points, thereby constraining understanding of spawn time variability – a factor increasingly critical to aid restoration efforts (Craggs et al., 2017; Randall et al., 2020) and wider reef management strategies (Romero-Torres et al., 2017; Doropoulos et al., 2019). Our study combined parallel observations in the same year from the central, as well as east and west northern Red Sea for the first time, covering over 572 colonies belonging to 21 Acropora species to investigate gametogenesis and spawning patterns along the Red Sea. Our 2023 data confirmed temporal variation in spawning timing between the central and northern regions of the Red Sea, with events occurring during the full moon nights of April in Thuwal (central), and in May for Shushah Island (East-North); however, the spawning in Hurghada (West-North) was 7-9 days before full moon of May (potentially reflecting more variable SST 6-weeks prior compared to the other sites). Integration of our 2023 data with historical observations identified that earlier spawning timing across the central and northern Red Sea correlates with cooler absolute SSTs that have experienced more rapid warming 6-weeks prior. However, the variation in spawning time we observed in our study highlights dynamic coral spawning timings for this region, and most likely through several governing factors at play.
Our 2023 data showed that spawning was later in the northern Red Sea than for central Red Sea (Table S1), a pattern consistent with past observations (reviewed in Ziegler et al., 2019). Factors governing synchronization of coral spawning can operate across different timeframes (hours versus days or months). Most notably, seawater temperature plays a significant role in governing gamete maturation for corals and many other reef organisms (Hue et al., 2020), thereby determining the spawning month (Harrison et al., 1984; Babcock et al., 1986). High or low seawater temperatures can expedite or delay oocyte maturation, affecting the month and/or day of spawning. Consequently, different coral morphospecies and locations possess distinct temperature thresholds crucial for promoting oocyte maturation and spawning (Lin and Nozawa, 2023). Such a notion presumably explains general differences in earlier spawning timings observed for the warmer central versus cooler northern Red Sea regions both in our 2023 dataset and historically (Figure 6). Also, this general role of absolute SST in governing spawning month is consistent with previous in situ spawning records from other locations in the Red Sea (Bouwmeester et al., 2015, 2015; Kotb et al., 2018), where some species exhibit spawning patterns in April/May (e.g., A. lamarcki, A. parapharaonis, A. plantaginea) versus A. humilis in May/June (Bouwmeester et al., 2015; Ziegler et al., 2019), as per our 2023 dataset from Shushah Island and Thuwal. In our study, immature eggs of A. humilis were documented in May, combined with lack of spawning observations during our in situ surveys, indicate that they consistently spawn after other Acropora taxa in later month(s). Thus, A. humilis may require higher temperatures for gonad maturation, and hence, late spawning in the upcoming month(s). Thus, increases of SST as expected for the Red Sea under climate change scenarios (Eladawy et al., 2022) may be expected to shift these current reproductive cycle/timings and influence larval dispersion pattern. In fact, dispersion of coral larvae relies on water movement and circulation that can vary among months. As such, shifting the timing of coral spawning may affect coral connectivity, population recovery, and shift community composition. This highlights the importance of continued and coordinated temporal monitoring to coral spawning time and synchrony. In addition, this will require further improvements in taxonomic identification that allow comparability and data integration to ensure detailed understanding for reproduction patterns of coral conspecifics along the Red Sea latitudinal gradient.
Interestingly, corals at both Thuwal and Shushah Island locations spawned on the night of full moon (April and May, respectively). Spawning observations during and/or around the full moon nights seems a relatively common phenomenon that has been documented in various bioregions (Baird et al., 2021), including the Red Sea (Bouwmeester et al., 2015). Moonlight serves as a natural signal to coordinate among coral colonies to synchronize the release of their gametes. For example, genomic analysis showed the moonlight induce and upregulate genes related to Circadian clock genes in A. millepora, that may play a role in regulating and synchronizing spawn timing (Brady et al., 2016). Lin et al. (2021) showed that shaded corals around full moon delayed spawning suggesting that moon light is a key factor triggering coral spawning in Dipsastraea speciosa. However, observations have revealed instances where spawning timing deviates from the night of full moon to several days before or after full moon. In these cases, the timing of gamete release remains highly specific to each coral species. For example, spawning of different coral species (e.g., A. humilis, Porites lutea, and Porites solida) in the Red Sea showed deviation from the full moon (Hanafy et al., 2010; Bouwmeester et al., 2015; Attalla, 2016). Similarly, coral spawning along the Great barrier Reef (GBR) have been consistently offset by 3-4 days after full moon, but the exact factors leading to this deviation are unclear. However, Sakai et al. (2020) observed that high SST (and low wind speed) for 1-2 month before spawning day is strongly correlated with deviation from full moon night in Acropora species. This aligns with our analysis where a significant correlation between absolute SST and deviation from night of full moon was observed in previous historical spawning events (see Figure 6). Indeed, Keith et al. (2016) suggested that rapid shift in SST was the primary cue for coral spawning across Indo-Pacific sites. This aligns with our findings where corals on west coast of the northern Red Sea (i.e., Hurghada) showed rapid shift in SST and spawned few days before the full moon, although the exact date of spawning was not observed.
By collectively examining 2023 and historical spawning events across our sites, variation of spawning time relative to the full moon can be explained by differences in SST dynamics (absolute versus prior warming rates). Such trends provide an intriguing hypothesis to further validate – and means to potentially predict – spawning timing for other sites throughout the Red Sea. However, we noted that substantial residual variation was evident, in particular for the correlation between warming rate and spawning timing (Figure 6). It is possible such variation still reflects challenges in integrating data sets across sites and investigators over time. However, factors other than SST also can affect the timing of spawning, e.g., onset of darkness (Babcock et al., 1986), the duration of regionally calm weather (Van Woesik, 2010), food availability (Fadlallah, 1983), twilight chromaticity (Boch et al., 2011), and rainfall (Mendes and Woodley, 2002), that may introduce inter-annual (or inter-site) variance. Clearly, our dataset is currently unable to tease apart such factors, but regardless, it would suggest that several factors, primarily temperature, displayed a distinct trend for north versus south Red Sea, but to a lesser extent east versus west.
Temporal variation of spawning between west and east northern Red Sea (Hurghada vs Shushah Island) was an unexpected result, particularly they have the same latitude. Spawning at Hurghada in the northwest Red Sea for 2023 occurred approximately 7-9 days prior to full moon, however in situ spawning has not been observed at Hurghada. It is important to acknowledge that the observed slicks along Hurghada coast are assumed to originate from Acropora, and may potentially include gametes from other coral species, however the identification of the spawned coral species from slicks poses a limitation. Despite this, our gametogenesis surveys revealed that Acropora species released their eggs before the full moon, despite not knowing the exact spawning dates. This observation previously reported in 2009, 2012, and 2013 where spawning was documented 3-5 before the full moon (Hanafy et al., 2010; Attalla, 2016 – see Table S1), and in contrast to spawning on full moon at Shushah in the northeast in 2023. Similarly, anecdotal observations in 2022 further confirmed that spawning slicks were seen after the full moon (16th April) by 14 days (April 29th), which is the new moon (Ahmed Ghallab, personal observation). Such earlier spawning of corals at Hurghada compared to Shushah Island could be attributed to two potential factors. Firstly, the fluctuation and rapid changes in seawater temperature in Hurghada (see Figure 6) perhaps induced corals to release their gametes. Whilst Hurghada exhibited a lower warming rate (0.3°C per week) compared to Shushah Island (0.4°C per week) 6-weeks prior to the 2023 spawning, this warming rate was less consistent over time for Hurghada compared to Shushah (in terms of explaining warming rate by a linear regression). Intriguingly, differences in SST (ΔSST) in 3-weeks prior to spawning were similar between sites (1.3°C vs 1.4°C Shushah vs Hurghada), highlighting higher rapid warming the last few weeks before spawning (Figures 4, 6). Keith et al. (2016) demonstrated that a rapid increase in SST is a central feature in explaining spawning timing, where elevated SST expedites gamete development (Harrison et al., 1984; Babcock et al., 1986). Presumably retaining oocytes until the full moon nights under increasing SST would have imposed higher metabolic cost, and perhaps prompting coral hosts to release their gametes earlier to mitigate this demand (Leinbach et al., 2021). Our historical data suggests a correlation between warming rates and spawning timing (Figure 6), but with the current limited dataset was not significant.
In our 2023 dataset spawning timings curiously appeared to reflect a trade off in absolute temperature and prior warming rate, specifically, slower warming but higher absolute temperatures in the central Red Sea (Thuwal) versus faster warming but lower absolute temperatures (Hurghada and Shushah Island). Constant, but variable, historical high warming data in Hurghada (since 2008) may likely adjust the biological rhythm of spawning that govern cellular processes to promote spawning, however lack of repeated in situ spawning observation at Hurghada is a limitation and this is yet to be resolved in coming years. Overall, our observations align with previous research findings, where spawning day appear correlated to SST (Lin and Nozawa, 2023). Similarly, colonies of the same coral species at similar latitudes in the Great Barrier Reef, but separated by 60 km, exhibit different spawning days because of earlier SST warming (Willis et al., 1985).
Secondly, we posit that artificial light present in Hurghada (northwest Red Sea), as a result of intensive urban development, could have disrupted the natural spawning timing of corals, as compared to Shushah Island (northeast Red Sea) where such pollution is currently absent. Hurghada is a well-developed city known for experiencing significant light pollution along its coastline (Davies et al., 2023), and light pollution has been observed to disrupt gametogenesis in several Acropora species in the Indo-Pacific Ocean (Ayalon et al., 2021). Although all our survey sites were located approximately 5-15 km from the shoreline, it is possible that proximity of the Hurghada sites closer to a well-developed city with light pollution might impact the offshore reefs. Such a hypothesis warrants further investigation, especially with the accelerated development of the NEOM coastline. Coastal fringing reefs could be more susceptible to the effects of this light interference than offshore reefs. However, we do not possess in situ records of coral spawning in fringing reefs versus offshore areas to understand whether light pollution influences coral spawning, and therefore, it is a logical point for future assessments of spawning timings.
In conclusion, timing of coral spawning in the Red Sea is clearly complex and influenced by various factors that differ across its geographical span, including temperature, lunar cycles, and potentially the presence of light pollution, amongst others. Our parallel observations in 2023 of spawning behavior of Acropora corals in the central and northern Red Sea suggests distinct temporal patterns, with each site (and species) having specific temperature thresholds for oocyte maturation. These variations in temperature thresholds likely result in variations in Acropora (including multi-species) mass spawning times amongst Red Sea locations, and thus the reproductive season in the Red Sea spans from April to June for various coral species. Despite the importance of lunar cycles and moonlight in coral spawning, observations have shown that some corals (e.g., Hurghada) are likely independent of the lunar cycle and do not strictly spawn during full moon night(s). Our data suggests that such temporal and spatial patterns of coral spawning appear highly governed by SST, thereby providing a potentially useful means to predict (and model) future spawning timing for Acropora species. However, such trends similarly raise questions as to how future ocean warming might impact this timing and in turn larval dispersal pattern and connectivity of coral populations throughout the Red Sea. Effective reef management – through both preservation of source versus sink reefs as well as intervention activities that look to boost coral abundance through exploiting the sexual reproduction process – rests on resolving factors that fine tune spawning timings. Thus, continued understanding the dynamics of coral spawning throughout the region is urgent. Notably, along the Red Sea coastline where ongoing development persists, and climate change continuously influences the dynamics and temperatures of the sea surface over time.
Data availability statement
The original contributions presented in the study are included in the article/Supplementary Material. Further inquiries can be directed to the corresponding authors.
Ethics statement
The animal study was approved by King Abdullah University for Science and Technology. The study was conducted in accordance with the local legislation and institutional requirements.
Author contributions
EOO: Conceptualization, Data curation, Formal analysis, Methodology, Project administration, Visualization, Writing – original draft, Writing – review & editing. DS: Conceptualization, Supervision, Writing – original draft, Writing – review & editing. TA: Methodology, Writing – review & editing. MC: Methodology, Writing – review & editing. NC: Methodology, Writing – review & editing. IE-S: Methodology, Writing – review & editing. AG: Methodology, Writing – review & editing. EG: Methodology, Writing – review & editing. NG-B: Methodology, Writing – review & editing. JG: Methodology, Writing – review & editing. PP: Methodology, Writing – review & editing. MR-S: Writing – review & editing. ES: Writing – review & editing. AS: Writing – review & editing. SY: Writing – review & editing. FB: Writing – review & editing. RP: Writing – review & editing.
Funding
The author(s) declare financial support was received for the research, authorship, and/or publication of this article. The conducted survey received separate funding at each site. Survey at KAUST was funded by the KAUST baseline fund to RP, while the survey at Shushah Island was supported through ongoing work of the KAUST Reefscape Restoration Initiative (KRRI). Furthermore, the work at Hurghada was funded by the Egyptian Environmental Affairs Agency (EEAA). EOO is partially funded by postdoctoral fellowship provided by Ocean Science and Solutions Applied Research Institute (OSSARI), Education, Research, and Innovation (ERI) Sector, NEOM, Tabuk, Saudi Arabia. This research was also supported in part by a grant #NGS-60324R-20 from the National Geographic Society provided to EOO.
Acknowledgments
The authors express gratitude to James Geust, Jesscia Bouwmeester, and Sebastian Schmidt-Roach for their valuable contributions to the sampling design. Additionally, special thanks go to Barbara Ribeiro, Adam Barno, Yusuf El-khaled, Walter Rich (KAUST), and Sean Bull, Gareth Lloyd, Zac Forsman, and the KRRI Coastline dive team (Shushah) Ibrahim Alhamad, Wael Alsobhi, Ma’an Banjar, Mahmoud Gaber, Shadi Magdi, Ahmed Maghrabi, Adel Mustafa, Mohammed Saeed for their tireless support to the fieldwork through logistical support. The graphic design of Figure 3 was courtesy of Hend Nawwar (KAUST). The team is also thankful for all the support received from CMOR (Ramzi Aljahdali and Waleed Aljahdali) and the diving facility (Krasimir Todorov and Saeedullah Soomro) at KAUST to enable night dives. Also, we would like to thank Allen Coral Atlas for enabling visualization of coral reefs distribution along the Red Sea.
Conflict of interest
The authors declare that the research was conducted in the absence of any commercial or financial relationships that could be construed as a potential conflict of interest.
The author(s) declared that they were an editorial board member of Frontiers, at the time of submission. This had no impact on the peer review process and the final decision.
Publisher’s note
All claims expressed in this article are solely those of the authors and do not necessarily represent those of their affiliated organizations, or those of the publisher, the editors and the reviewers. Any product that may be evaluated in this article, or claim that may be made by its manufacturer, is not guaranteed or endorsed by the publisher.
Supplementary material
The Supplementary Material for this article can be found online at: https://www.frontiersin.org/articles/10.3389/fmars.2024.1333621/full#supplementary-material
References
Aguilera M. A., Tapia J., Gallardo C., Núñez P., Varas-Belemmi K. (2020). Loss of coastal ecosystem spatial connectivity and services by urbanization: Natural-to-urban integration for bay management. J. Environ. Manage. 276, 111297. doi: 10.1016/J.JENVMAN.2020.111297
Al Tawaha M., Benzoni F., Eid E., Abu Awali A. (2019). The hard corals of Jordon: A field Guild. R. Mar. Conserv. Soc. Jordan (Amman, Jordon), 432.
Arrigoni R., Benzoni F., Terraneo T. I., Caragnano A., Berumen M. L. (2016). Recent origin and semi-permeable species boundaries in the scleractinian coral genus Stylophora from the Red Sea. Sci. Rep. 6. doi: 10.1038/srep34612
Attalla T. (2016). Some Ecological Aspects on coral reefs communities of the Red Sea- PhD thesis, Suez Canal University, Egypt.
Ayalon I., Rosenberg Y., Benichou J. I. C., Campos C. L. D., Sayco S. L. G., Nada M. A. L., et al. (2021). Coral gametogenesis collapse under artificial light pollution. Curr. Biol. 31, 413–419.e3. doi: 10.1016/J.CUB.2020.10.039
Babcock R. C., Bull G. D., Harrison P. L., Heyward A. J., Oliver J. K., Wallace C. C., et al. (1986). Synchronous spawnings of 105 scleractinian coral species on the Great Barrier Reef. Mar. Biol. 90, 379–394. doi: 10.1007/BF00428562
Baird A. H., Guest J. R. (2009). Spawning synchrony in scleractinian corals: Comment on Mangubhai & Harrison, (2008). Mar. Ecol. Prog. Ser. 374, 301–304. doi: 10.3354/MEPS07838
Baird A. H., Guest J. R., Edwards A. J., Bauman A. G., Bouwmeester J., Mera H., et al. (2021). An Indo-Pacific coral spawning database. Sci. Data 8, 1–9. doi: 10.1038/s41597-020-00793-8
Baums I. B. (2008). A restoration genetics guide for coral reef conservation. Mol. Ecol. 17, 2796–2811. doi: 10.1111/J.1365-294X.2008.03787.X
Baums I. B., Baker A. C., Davies S. W., Ea A., Grottoli G., Kenkel C. D., et al. (2019). Considerations for maximizing the adaptive potential of restored coral populations in the western Atlantic. Ecol. Appl. 29, e01978. doi: 10.1002/EAP.1978
Baums I. B., Devlin-Durante M. K., Polato N. R., Xu D., Giri S., Altman N. S., et al. (2013). Genotypic variation influences reproductive success and thermal stress tolerance in the reef building coral, Acropora palmata. Coral. Reefs. 32, 703–717. doi: 10.1007/s00338-013-1012-6
Berumen M. L., Arrigoni R., Bouwmeester J., Terraneo T. I., Benzoni F. (2019). Corals of the Red Sea. 123–155. doi: 10.1007/978-3-030-05802-9_7
Boch C. A., Ananthasubramaniam B., Sweeney A. M., Doyle F. J., Morse D. E. (2011). Effects of light dynamics on coral spawning synchrony. Biol. Bull. 220, 161–173. doi: 10.1086/BBLv220n3p161
Bouwmeester J., Baird A. H., Chen C. J., Guest J. R., Vicentuan K. C., Berumen M. L. (2015). Multi-species spawning synchrony within scleractinian coral assemblages in the Red Sea. Coral. Reefs. 34, 65–77. doi: 10.1007/S00338-014-1214-6/FIGURES/3
Bouwmeester J., Berumen M. L., Baird A. H. (2011). Daytime broadcast spawning of Pocillopora verrucosa on coral reefs of the central Red Sea. Galaxea. J. Coral. Reef. Stud. 13, 23–24. doi: 10.3755/galaxea.13.23
Bouwmeester J., Gatins R., Giles E. C., Sinclair-Taylor T. H., Berumen M. L. (2016). Spawning of coral reef invertebrates and a second spawning season for scleractinian corals in the central Red Sea. Invertebrate. Biol. 135, 273–284. doi: 10.1111/IVB.12129
Brady A. K., Willis B. L., Harder L. D., Vize P. D. (2016). Lunar phase modulates circadian gene expression cycles in the broadcast spawning coral Acropora millepora. Biol. Bull. 230, 130–142. doi: 10.1086/BBLV230N2P130/ASSET/IMAGES/LARGE/Z1N0021636910005.JPEG
Bridge T. C. L., Cowman P. F., Quattrini A. M., Bonito V. E., Sinniger F., Harii S., et al. (2023). A tenuis relationship: traditional taxonomy obscures systematics and biogeography of the ‘Acropora tenuis’ (Scleractinia: Acroporidae) species complex. Zoological J. Linn. Soc. doi: 10.1093/ZOOLINNEAN/ZLAD062
Buerger P., Alvarez-Roa C., Coppin C. W., Pearce S. L., Chakravarti L. J., Oakeshott J. G., et al. (2020). Heat-evolved microalgal symbionts increase coral bleaching tolerance. Sci. Adv. 6, eaba2498. doi: 10.1126/SCIADV.ABA2498
Chan W. Y., Peplow L. M., Menéndez P., Hoffmann A. A., van Oppen M. J. H. (2018). Interspecific hybridization may provide novel opportunities for coral reef restoration. Front. Mar. Sci. 5. doi: 10.3389/FMARS.2018.00160/BIBTEX
Chiu Y. L., Shikina S., Yoshioka Y., Shinzato C., Chang C. F. (2020). De novo transcriptome assembly from the gonads of a scleractinian coral, Euphyllia ancora: molecular mechanisms underlying scleractinian gametogenesis. BMC Genomics 21, 1–20. doi: 10.1186/S12864-020-07113-9/TABLES/5
Cowen R. K., Sponaugle S. (2009). Larval dispersal and marine population connectivity. Ann. Rev. Mar. Sci. 1, 443–466. doi: 10.1146/ANNUREV.MARINE.010908.163757
Craggs J., Guest J. R., Davis M., Simmons J., Dashti E., Sweet M. (2017). Inducing broadcast coral spawning ex situ: Closed system mesocosm design and husbandry protocol. Ecol. Evol. 7, 11066. doi: 10.1002/ECE3.3538
Davies T. W., Levy O., Tidau S., de Barros Marangoni L. F., Wiedenmann J., D’Angelo C., et al. (2023). Global disruption of coral broadcast spawning associated with artificial light at night. Nat. Commun. 14, 1–7. doi: 10.1038/s41467-023-38070-y
Dibattista J. D., Roberts M. B., Bouwmeester J., Bowen B. W., Coker D. J., Lozano-Cortés D. F., et al. (2016). A review of contemporary patterns of endemism for shallow water reef fauna in the Red Sea. J. Biogeography 43 (3), 423–439. doi: 10.1111/JBI.12649
Donahue M. J., Karnauskas M., Toews C., Paris C. B. (2015). Location isn’t everything: timing of spawning aggregations optimizes larval replenishment. PloS One 10 (6), e0130694. doi: 10.1371/JOURNAL.PONE.0130694
Doropoulos C., Elzinga J., ter Hofstede R., van Koningsveld M., Babcock R. C. (2019). Optimizing industrial-scale coral reef restoration: comparing harvesting wild coral spawn slicks and transplanting gravid adult colonies. Restor. Ecol. 27, 758–767. doi: 10.1111/REC.12918
Eladawy A., Nakamura T., Shaltout M., Mohammed A., Nadaoka K., Fox M. D., et al. (2022). Appraisal of coral bleaching thresholds and thermal projections for the northern Red Sea refugia. Front. Mar. Sci. 9. doi: 10.3389/FMARS.2022.938454/BIBTEX
Ehrenberg C. G. (1834). Beiträge zur physiologischen Kenntniss der Corallenthiere im allgemeinen, und besonders des rothen Meeres, nebst einem Versuche zur physiologischen Systematik derselben. Abhandlungen der Königlichen Akademie der Wissenschaften Berlin. 1, 225–380.
Fadlallah Y. H. (1983). Sexual reproduction, development and larval biology in scleractinian corals - A review. Coral. Reefs. 2, 129–150. doi: 10.1007/BF00336720/METRICS
Gilmour J., Speed C. W., Babcock R. (2016). Coral reproduction in western Australia. PeerJ 2016, 2010. doi: 10.7717/PEERJ.2010/SUPP-5
Hanafy M. H., Aamer M. A., Habib M., Rouphael A. B., Baird A. H. (2010). Synchronous reproduction of corals in the Red Sea. Coral. Reefs. 29, 119–124. doi: 10.1007/S00338-009-0552-2/TABLES/5
Harrison R. L., Babcock R. C., Bull G. D., Oliver J. K., Wallace C. C., Willis B. L. (1984). Mass spawning in tropical reef corals. Science 223, 1186–1189. doi: 10.1126/SCIENCE.223.4641.1186
Harrison P. L., dela Cruz D. W., Cameron K. A., Cabaitan P. C. (2021). Increased coral larval supply enhances recruitment for coral and fish habitat restoration. Front. Mar. Sci. 8. doi: 10.3389/FMARS.2021.750210/BIBTEX
Hock K., Wolff N. H., Ortiz J. C., Condie S. A., Anthony K. R. N., Blackwell P. G., et al. (2017). Connectivity and systemic resilience of the Great Barrier Reef. PloS Biol. 15, e2003355. doi: 10.1371/JOURNAL.PBIO.2003355
Howells E. J., Abrego D., Liew Y. J., Burt J. A., Meyer E., Aranda M. (2021). Enhancing the heat tolerance of reef-building corals to future warming. Sci. Adv. 7, eabg6070. doi: 10.1126/SCIADV.ABG6070/SUPPL_FILE/SCIADV.ABG6070_TABLE_S5.ZIP
Hue T., Chateau O., Lecellier G., Kayal M., Lanos N., Gossuin H., et al. (2020). Temperature affects the reproductive outputs of coral-eating starfish Acanthaster spp. after adult exposure to near-future ocean warming and acidification. Mar. Environ. Res. 162, 105164. doi: 10.1016/J.MARENVRES.2020.105164
Humanes A., Beauchamp E. A., Bythell J. C., Carl M. K., Craggs J. R., Edwards A. J., et al. (2021). An experimental framework for selectively breeding corals for assisted evolution. Front. Mar. Sci. 8. doi: 10.3389/FMARS.2021.669995/BIBTEX
Ibrahim R. M., Shaban W. M., Ali A. H. A. M., Morsy G. H., Abdel-Salam H. A. (2021). Gametogenic development and synchronous spawning of the acroporid corals Acropora cytherea and Acropora tenuis in the Red Sea. Egyptian. J. Aquat. Biol. Fisheries. 25, 419–436. doi: 10.21608/EJABF.2021.164595
Kaniewska P., Alon S., Karako-Lampert S., Hoegh-Guldberg O., Levy O. (2015). Signaling cascades and the importance of moonlight in coral broadcast mass spawning. ELife 4, e09991. doi: 10.7554/ELIFE.09991.001
Keith S. A., Maynard J. A., Edwards A. J., Guest J. R., Bauman A. G., van Hooidonk R., et al. (2016). Coral mass spawning predicted by rapid seasonal rise in ocean temperature. Proc. R. Soc. B.: Biol. Sci. 283, 20160011. doi: 10.1098/RSPB.2016.0011
King S., Saint-Amand A., Walker B. K., Hanert E., Figueiredo J. (2023). Larval dispersal patterns and connectivity of Acropora on Florida’s Coral Reef and its implications for restoration. Front. Mar. Sci. 9. doi: 10.3389/FMARS.2022.1038463/BIBTEX
Klunzinger C. B. (1879). Die Korallthiere des Rothen Meeres, 2. Theil: Die Steinkorallen. Erster Abschnitt: Die Madreporaceen und Oculinaceen (Gutmann, Berlin) 1-88, 1–10.
Kotb M. M. A., Hanafy M. H., Monir T. (2018). Synchronized spawning and fecundity of Acropora species in the Red Sea. Indian J. Geo. Mar. Sci. 47, 2023–2041.
Leinbach S. E., Speare K. E., Rossin A. M., Holstein D. M., Strader M. E. (2021). Energetic and reproductive costs of coral recovery in divergent bleaching responses. Sci. Rep. 11, 1. doi: 10.1038/s41598-021-02807-w
Levitan D. R., Boudreau W., Jara J., Knowlton N. (2014). Long-term reduced spawning in Orbicella coral species due to temperature stress. Mar. Ecol. Prog. Ser. 515, 1–10. doi: 10.3354/MEPS11063
Lin C. H., Nozawa Y. (2023). The influence of seawater temperature on the timing of coral spawning. Coral. Reefs. 42, 417–426. doi: 10.1007/S00338-023-02349-9/FIGURES/5
Lin C. H., Takahashi S., Mulla A. J., Nozawa Y. (2021). Moonrise timing is key for synchronized spawning in coral Dipsastraea speciosa. Proc. Natl. Acad. Sci. United. States America 118, e2101985118. doi: 10.1073/PNAS.2101985118/SUPPL_FILE/PNAS.2101985118.SAPP.PDF
Mendes J. M., Woodley J. D. (2002). Timing of reproduction in Montastraea annularis: relationship to environmental variables. Mar. Ecol. Prog. Ser. 227, 241–251. doi: 10.3354/MEPS227241
Monfared M. A. A., Sheridan K., Dixon S. P., Gledhill M., Berre T. (2023). Coral spawning patterns of Acropora across two Maldivian reef ecosystems. PeerJ 11, e16315. doi: 10.7717/PEERJ.16315
Nakajima Y., Nishikawa A., Iguchi A., Sakai K. (2010). Gene flow and genetic diversity of a broadcast-spawning coral in northern peripheral populations. PloS One 5, 11149. doi: 10.1371/JOURNAL.PONE.0011149
Neely K. L., Lewis C. L., Macaulay K. A. (2020). Disparities in Spawning Times Between in situ and ex situ Pillar Corals. Front. Mar. Sci. 7. doi: 10.3389/FMARS.2020.00643/BIBTEX
Osman E. O., Smith D. J., Ziegler M., Kürten B., Conrad C., El-Haddad K. M., et al. (2018). Thermal refugia against coral bleaching throughout the northern Red Sea. Global Change Biol. 24, 474–484. doi: 10.1111/gcb.13895
Ramírez-Portilla C., Baird A. H., Cowman P. F., Quattrini A. M., Harii S., Sinniger F., et al. (2022). Solving the Coral Species Delimitation Conundrum. Systematic Biol. 71 (2), 461–475. doi: 10.1093/SYSBIO/SYAB077
Randall C. J., Negri A. P., Quigley K. M., Foster T., Ricardo G. F., Webster N. S., et al. (2020). Sexual production of corals for reef restoration in the Anthropocene. Mar. Ecol. Prog. Ser. 635, 203–232. doi: 10.3354/MEPS13206
R Developement Core Team (2017). R: A language and environment for statistical computing. R. Foundation. Stat. Computing. 1, 409. doi: 10.1007/978-3-540-74686-7
Romero-Torres M., Acosta A., Treml E. A. (2017). The regional structure of spawning phenology and the potential consequences for connectivity of coral assemblages across the Eastern Tropical Pacific. ICES. J. Mar. Sci. 74, 613–624. doi: 10.1093/ICESJMS/FSW218
Sakai Y., Hatta M., Furukawa S., Kawata M., Ueno N., Maruyama S. (2020). Environmental factors explain spawning day deviation from full moon in the scleractinian coral Acropora. Biol. Lett. 16, 20190760. doi: 10.1098/RSBL.2019.0760
Shlesinger Y., Loya Y. (1985). Coral community reproductive patterns: red sea versus the great barrier reef. Science 228, 1333–1335. doi: 10.1126/SCIENCE.228.4705.1333
Sigovini M., Keppel E., Tagliapietra D. (2016). Open Nomenclature in the biodiversity era. Methods Ecol. Evol. 7 (10), 1217–1225. doi: 10.1111/2041-210X.12594
van der Ven R. M., Ratsimbazafy H. A., Kochzius M. (2022). Large-scale biogeographic patterns are reflected in the genetic structure of a broadcast spawning stony coral. Coral. Reefs. 41, 611–624. doi: 10.1007/S00338-022-02220-3/TABLES/3
van Oppen M. J. H., Gates R. D., Blackall L. L., Cantin N., Chakravarti L. J., Chan W. Y., et al. (2017). Shifting paradigms in restoration of the world’s coral reefs. Global Change Biol. 23, 3437–3448. doi: 10.1111/GCB.13647
van Oppen M. J. H., Lukoschek V., Berkelmans R., Peplow L. M., Jones A. M. (2015). A population genetic assessment of coral recovery on highly disturbed reefs of the Keppel Island archipelago in the southern Great Barrier Reef. PeerJ 2015, e1092. doi: 10.7717/PEERJ.1092/SUPP-12
Van Woesik R. (2010). Calm before the spawn: global coral spawning patterns are explained by regional wind fields. Proc. R. Soc. B.: Biol. Sci. 277, 715–722. doi: 10.1098/RSPB.2009.1524
Van Woesik R., Lacharmoise F., Köksal S. (2006). Annual cycles of solar insolation predict spawning times of Caribbean corals. Ecol. Lett. 9, 390–398. doi: 10.1111/J.1461-0248.2006.00886.X
Vargas-Ángel B., Colley S. B., Hoke S. M., Thomas J. D. (2006). The reproductive seasonality and gametogenic cycle of Acropora cervicornis off Broward County, Florida, USA. Coral. Reefs. 25, 110–122. doi: 10.1007/S00338-005-0070-9/TABLES/4
Veron J. E. N. (2002). New species described in Corals of the World. Aust. Institute Mar. Sci., 206.
Voolstra C. R., Suggett D. J., Peixoto R. S., Parkinson J. E., Quigley K. M., Silveira C. B., et al. (2021). Extending the natural adaptive capacity of coral holobionts. Nat. Rev. Earth Environ. 2 (11), 747–762. doi: 10.1038/s43017-021-00214-3
Wallace C. C. (1985). Reproduction, recruitment and fragmentation in nine sympatric species of the coral genus Acropora. Mar. Biol. 88, 217–233. doi: 10.1007/BF00392585/METRICS
Wallace C. (1999). Staghorn Corals of the World: A Revision of the Genus Acropora. Staghorn Corals World. doi: 10.1071/9780643101388
Wallace C. C., Done B. J., Muir P. R. (2012). Revision and catalogue of worldwide staghorn corals acropora and isopora (scleractinia: Acroporidae) in the museum of tropical Queensland. Memoirs Queensland Museum 57 (1), 1–257. doi: 10.17082/J:2204-1478-56-2.2013-42
Willis B. L., Babcock R. C., Harrison P. L., Oliver J. K., Wallace C. C. (1985) Patterns in the mass spawning of corals on the Great Barrier Reef from 1981 to 1984. 343–348. Available at: https://researchportal.scu.edu.au/esploro/outputs/conferencePaper/Patterns-in-the-mass-spawning-of/991012822175002368.
Keywords: sexual reproduction, gametes release, coral reproduction, spawning, moonlight, spawning synchrony, restoration
Citation: Osman EO, Suggett DJ, Attalla TM, Casartelli M, Cook N, El-Sadek I, Gallab A, Goergen EA, Garcias-Bonet N, Glanz JS, Pereira PH, Ramirez-Sanchez M, Santoro EP, Stead A, Yoder S, Benzoni F and Peixoto RS (2024) Spatial variation in spawning timing for multi-species Acropora assemblages in the Red Sea. Front. Mar. Sci. 11:1333621. doi: 10.3389/fmars.2024.1333621
Received: 05 November 2023; Accepted: 05 February 2024;
Published: 04 March 2024.
Edited by:
Nicolas James Pilcher, Marine Research Foundation, MalaysiaReviewed by:
Masaya Morita, University of the Ryukyus, JapanJessica Bouwmeester, University of Hawaiʻi at Mānoa, United States
Copyright © 2024 Osman, Suggett, Attalla, Casartelli, Cook, El-Sadek, Gallab, Goergen, Garcias-Bonet, Glanz, Pereira, Ramirez-Sanchez, Santoro, Stead, Yoder, Benzoni and Peixoto. This is an open-access article distributed under the terms of the Creative Commons Attribution License (CC BY). The use, distribution or reproduction in other forums is permitted, provided the original author(s) and the copyright owner(s) are credited and that the original publication in this journal is cited, in accordance with accepted academic practice. No use, distribution or reproduction is permitted which does not comply with these terms.
*Correspondence: Eslam O. Osman, ZXNsYW0ub3NtYW5Aa2F1c3QuZWR1LnNh; David J. Suggett, ZGF2aWQuc3VnZ2V0dEBrYXVzdC5lZHUuc2E=
†These authors have contributed equally to this work