- 1School of Marine Biology, Xiamen Ocean Vocational College, Xiamen, China
- 2Xiamen Key Laboratory of Intelligent Fishery, Jimei University, Xiamen, China
- 3Fisheries College, Jimei University, Xiamen, China
- 4Haiyo Biotechnology Co., LTD, Xiamen, China
- 5Agricultural and Rural Development Service Center of Pingtan Comprehensive Pilot Zone, Fuzhou, China
- 6Department for Medical Research, E-Da Hospital, I-Shou University, Koahsiung City, Taiwan
Shrimp is an important aquaculture species worldwide. The use of antibiotics to suppress disease outbreaks has led to antibiotic resistance; however, probiotics or natural herbal additives can enhance the health of farmed shrimp. In this study, the effects of formulations containing natural herbs and probiotics on shrimp farming were explored. Following indoor shrimp farming, the shrimp were returned to outdoor natural ponds for 1 week in the presence of a fermented probiotic product. The gut microbiota was surveyed using 16S rRNA gene sequencing at 1, 2, 3, and 8 weeks after the natural pond release. The results showed that Vibrio-related bacterial genera increased significantly in the shrimp intestinal microbiota at 2 weeks and were particularly high at 3 weeks after natural pond release. The phyla Firmicutes, Bacteroidetes, Vibrio-related bacteria, and the genus Cetobacterium emerged as crucial bacteria linked to shrimp health and growth. Overall, the diversity of the shrimp intestinal microbiota was lower upon release into the natural pond. However, this outcome may be associated with dysbiosis or influenced by the natural environment. Further research is warranted to substantiate these findings. A perspective on the shrimp gut microbiota provides important information for aquaculture management and explains the implementation of control measures.
1 Introduction
Shrimp aquaculture is a vital economic cornerstone of many countries. Most early studies on shrimp aquaculture management have focused on shrimp disease and nutritional supplementation (Toma and James, 1975; Feingold, 2000; Walker and Winton, 2010; Lightner, 2011). However, in recent decades, with the advancements in next-generation sequencing and microbiota studies, research on the gut microbiota of aquatic species, including fish and shrimp, has dramatically increased (Diwan and Harke, 2022; El-Saadony et al., 2022; Turner et al., 2022; Vijayaram et al., 2022). The gut microbiota, particularly the interactions between the gut microbiota and the host, is a prominent research field (Adak and Khan, 2019).
Research on aquatic organisms, especially economically important aquaculture animals, has focused on disease-related topics, including pathogens such as Vibrio species (de Souza Valente et al., 2020; Gainza and Romero, 2020; Soo and Bhassu, 2022). Many non-pathogenic strains within the Vibrio genus are important for crustaceans due to their ability to produce chitinases (Sugita and Ito, 2006), which may support healthy gut function. The impact of other relevant microbial communities in the shrimp gut on the stability of the Vibrio species composition has also been evaluated (Holt et al., 2021; Sha et al., 2022). Another critical area of focus is the variation in the gut microbiota composition among different shrimp species and their interactions with the host (Holt et al., 2021; Wang et al., 2020), along with the gut microbiota in different sections of the shrimp gut (Holt et al., 2021). However, further studies are required to enhance the aquaculture efficiency and prevent shrimp diseases. Studies have also provided insights into the prevention of disease transmission through food (de Souza Valente et al., 2020). Therefore, a large focus of research in this field is enhancing aquaculture efficiency while also preventing shrimp diseases.
Probiotics, which are defined as live microorganisms, contribute to intestinal function and immune system development (Diwan and Harke, 2022; Ma et al., 2022). The intestine is the largest immune organ in the body (Wu and Wu, 2012). Probiotics facilitate efficient feed utilization, maintain physiological immune mechanisms, and improve growth efficiency, among other functions (Fuller, 1989). Thus, hosts lacking a healthy microbial community are prone to rapid death owing to infection, highlighting the indispensable symbiotic relationship between probiotics and hosts (Bhogoju and Nahashon, 2022; Nourizadeh et al., 2022). The use of probiotics in aquaculture has been extensively studied for their capacity to inhibit detrimental environmental and gut bacteria, stimulate growth, and optimize enzymatic systems (Balcazar et al., 2006; Martinez Cruz et al., 2012). The goal is to enhance the diversity of gut microbes to optimize the breakdown and absorption efficiency of organic matter, thereby further improving intestinal immune function (Ringo et al., 2022; Zeng et al., 2023). The enhancement of this mechanism also leads to improvements in the survival rates of aquaculture species and has the potential to reduce reliance on drugs in aquaculture (Martinez Cruz et al., 2012; Meng et al., 2023). For crustaceans, common probiotics include Bacillus S11, Pseudomonas sp. PM11, and Vibrio fluvialis PM17 (Farzanfar, 2006; Kumar et al., 2016). These probiotics are primarily used to enhance growth, resist pathogens, and increase the productivity of crustacean aquaculture. Thus, probiotics can be used as natural additives in healthy aquaculture systems.
Many reports have indicated that traditional Chinese herbal medicine possesses specific therapeutic and preventive capabilities against diseases (Zhai and Li, 2019; Zhang et al., 2021). Numerous herbs exhibit natural antipyretic and detoxifying effects and contain essential natural compounds, such as phenylethanoid glycosides, resins and their glycosides, pentacyclic triterpenoids, chlorogenic acid, iridoids, and essential oils, which play crucial roles in curing viral infections and enhancing resistance (Liao et al., 2022). Common medicinal herbs with such effects include Isatis indigotica, Datura stramonium, Pulsatilla chinensis, Eucalyptus leaves, Forsythia suspensa, Lonicera japonica, Acorus tatarinowii, and Gentiana macrophylla. These active substances in natural herbs are often subjected to fermentation and extraction using fly larval enzyme solutions and selenium-enriched yeast, producing liquid formulations containing natural compounds widely used in aquaculture for disease and antibacterial resistance (Zhang et al., 2021; Liao et al., 2022).
Penaeus vannamei, commonly known as white shrimp, is a significant species in mainland China. However, this species is often plagued by gill and white spot diseases, necessitating the use of medications to maintain the health of aquaculture organisms and inadvertently promoting the emergence of drug-resistant pathogens (Hassan et al., 2022; He et al., 2023; Tadese et al., 2022). Therefore, bioactive compounds that can be extracted from traditional Chinese medicine and have gained attention to recent years (Cai et al., 2022). Recently, probiotics have been frequently used as byproducts in feed additives (Martinez Cruz et al., 2012) due to their ability to decompose naturally (Diwan and Harke, 2022; Ma et al., 2022). In this study, we studied the impact of a fermented herbal probiotic on the gut microbiotas of white shrimp. The juveniles of white shrimp were grown in the presence of Chinese herbal fermented products for 7 days, after which they were released into natural cultivation ponds. Subsequently, temporal changes in the intestinal microbiota of white shrimp after culture in natural environments were analyzed to understand the impact period of adding fermented products to white shrimp. This study provides crucial reference data for environmentally friendly and healthy aquaculture practices.
2 Materials and methods
2.1 Probiotic fermentation product composition
The probiotic fermentation product contained the following mixture: 30 units humic acid; 8 units lactic acid bacteria; 5 units selenium-enriched yeast; 8 units Bacillus subtilis; 20 units brown sugar; 0.1–0.4 units vitamin complex; 0.1 unit vitamin complex, which contained vitamin C, vitamin B1, vitamin B2, vitamin B6, vitamin B12, niacin, pantothenic acid, folate, and choline; and 60 units pure freshwater. The herbal components of the Chinese herbal additive solution product include the roots of Isatis indigotica, Zornia cantoniensis, Pulsatilla chinensis, and leaves of Tasmanian Bluegum, Forsythiae Fructus, Lonicera japonica, Acorus gramineus, Gentiana cruciate. These components were fermented using a fermentation liquid and selenium yeast. All raw materials were purchased from the traditional Chinese medicine market.
2.2 Addition experiment
Various herbal additives, with the compositions described in Section 2.1, were added to the initial shrimp cultivation ponds in an indoor environment on day 8 after fermentation. The average size of the cultivated shrimps was approximately 5 cm. The aquaculture conditions and feeding regimen were as: three circular aquaculture tanks, each containing 2.5 tons of water, and 500 shrimp larvae per tank (individual averages were recorded during the initial experimental observation on July 10). The water temperature is maintained between 28–30 °C, with feeding occurring three times daily at 7:30, 12:30, and 19:30. The natural pond was 2 m long and 2 m wide with a maximum depth of 3 m. In natural ponds, the shrimp density was 200 individuals per m3. The salinity of water in the natural ponds was maintained at approximately 18 PSU. SDR1 (SDR1-1, SDR1-2, and SDR1-3) represents the sampling time point with the addition of the probiotic fermentation product for 7 days, SDR2 (SDR2-1, SDR2-2, and SDR2-3), SDR3 (SDR3-1, SDR3-2, and SDR3-3), SDR4 (SDR4-1, SDR4-2, and SDR4-3), and SDR5 (SDR5-1, SDR5-2, and SDR5-3) correspond to 1, 2, 3, and 8 weeks, respectively, after returning the shrimp to the natural cultivation ponds. Three specimens were collected at each time point, and five white shrimp guts were mixed. Therefore, 15 and 75 shrimp gut microbiota samples were used for microbiota analysis. The relative abundances of each sample are shown in Supplementary Figure S1.
2.3 Genomic DNA extraction
Genomic DNA (gDNA) was extracted from shrimp gut using a QIAamp DNA Microbiome Kit (Qiagen, Hilden, Germany) according to the manufacturer’s instructions. The concentration of extracted gDNA was determined using a NanoDrop 2000 spectrophotometer (Thermo Fisher Scientific, Waltham, MA, USA). The quality of the extracted gDNA was evaluated using electrophoresis separation (1.5% gel in Tris-acetate ethylenediaminetetraacetic acid buffer), and purified gDNA was stored at −20°C until partial 16S rRNA gene sequencing analysis.
2.4 16S rRNA gene sequencing, library construction, and microbial community analysis
The 16S rRNA gene targeting the V3-V4 region was amplified using the KAPA HiFi HotStart Ready Mix PCR kit. Amplicon PCR amplification was performed using the Illumina MiSeq system (Illumina, San Diego, CA, USA) following a comprehensive protocol for paired-end sequencing as described previously (Villanueva and Chen, 2019). After obtaining raw sequencing files from the next-generation sequencing platform, the data were analyzed using the Qiime2 software package to characterize microbial diversity at the phylum and genus levels (Bolyen et al., 2019). Briefly, sequence data were trimmed to remove chimeras and clustered into operational taxonomic units (OTUs) using a 97% threshold limit of similarity with respect to the RDP database. The confidence threshold for limiting taxonomic depth was set to 0.7. Additionally, beta diversity was measured based on the weighted UniFrac index, followed by a permutational multivariate analysis of variance using phyloseq (McMurdie and Holmes, 2013). The results were visualized using ggplot2 (Villanueva and Chen, 2019). The relative abundance of microbes at the genus and species levels was determined using QIIME2 v2019.1, and the results were visualized using the ggplot2 and Eulerr packages (Chen et al., 2011). The Chao and Shannon indices were used to determine the alpha diversity of the sampling group. Subsequently, the t-tests were conducted to compare the alpha-diversity of the shrimp gut microbiotas from the various timepoints. Statistical significance for Firmicutes, Bacteroidetes, Vibrio, and Cetobacterium between each pair of time points within five different time points was assessed using the Wilcoxon rank-sum test based on relative abundance ratios. The distribution pattern of the microbial community based on similarities and dissimilarities was further assessed through Statistical Analysis of Metagenomic Profiles (STAMP) (Parks et al., 2014). Principal coordinate analysis was conducted using canoco5.1 software (http://www.canoco5.com/, accessed on May 14, 2020). Additionally, linear discriminant analysis effect size (LEfSe) was performed using Galaxy software (http://huttenhower.sph.harvard.edu/lefse/) using a linear discriminant analysis score >2 and p-value < 0.05 to identify the differential abundance of microbiota among the experimental groups (all time points vs. all time points).
3 Results
3.1 Clustering, microbial community composition, and abundance analysis based on 16S rRNA V3-4 sequencing
According to the 97% OTU analysis, after 7 days, the fermented probiotic treatment (SDR1) showed the most unique features (33.9%) compared to the other groups, as shown in the Venn diagram (Figure 1A). However, a small portion (2.2%) of the OTUs was shared among the five groups, indicating a variable microbial community with temporal changes, particularly in natural shrimp cultivation ponds. Moreover, after being released at 1 week (SDR2) and 3 weeks (SDR4), the samples showed similar proportions for the individual abundance (3.3% and 2.7%, respectively) of OTUs, indicating the presence of dominant species at these time points.
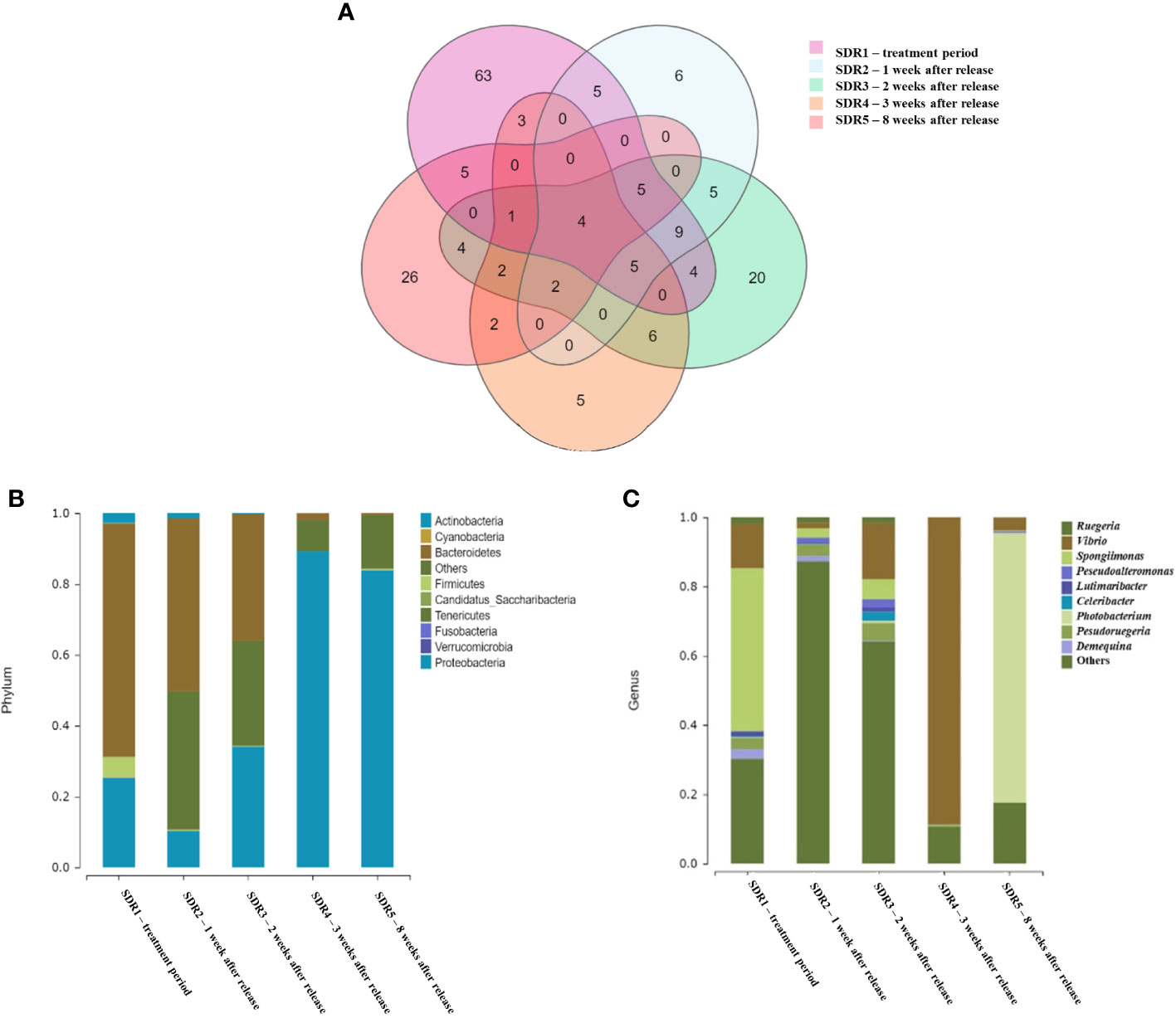
Figure 1 (A) Ven diagram of shared and unique operational taxonomic units between the five experimental groups. Bacterial taxonomic composition and abundance at the phylum (B) and genus (C) levels associated with shrimp gut cavity of five experimental groups.
Taxonomic analysis of the V3-4 region of 16S rRNA revealed striking differences in the composition and abundance of the microbial community at the taxonomic level in the shrimp gut cavity at five different time points. The relative abundances of the top ten phyla and genera associated with the gut cavities in each group are shown in Figures 1B, C. The relative abundances of each sample are shown in Supplementary Figure S2. Bacteroidetes was the most common phylum in the gut cavities of the SDR1(65.9%, treatment week) and SDR2 (48.8%, 1 week after release) groups. Proteobacteria was the most common phylum in the gut cavities of SDR4 (89.3%, 3 weeks after release) and SDR5 (83.8%, 8 weeks after release). Proteobacteria and Bacteroidetes were present in the same proportion (approximately 35%) 2 weeks after release (SDR3). The relative abundances of the top ten phyla in each sample were similar at the same time point, which was consistent with the results of the OTU analysis (Supplementary Figure S2). At the genus level, Spongiimonas, Photobacterium, and Vibrio were the most common genera in the shrimp gut cavity. Among them, Spongiimonas showed a high occurrence (47.0%) only during the treatment week (SDR1) and rapidly decreased in samples from later time points. The genera Vibrio and Photobacterium accounted for 88.8% 3 weeks after release (SDR4) and 77.9% 8 weeks after release (SDR5). Gammaproteobacteria was dominant in SDR4 (3 weeks after release). Bacterial diversity was higher in the initial stage than in the natural shrimp cultivation ponds.
3.2 Alpha diversity and beta diversity of microbiota in shrimp gut microbiome
We determined the Chao1 and Shannon diversity indices to explore community richness, taxon richness, and evenness of the shrimp gut microbiota at different time points (Figure 2). The average Chao1 index of the shrimp gut microbiota was the highest in the SDR1 group, but it also showed the highest standard deviation. After adding the fermentation product in the first week, the Chao1 index of SDR2 (1 week after release) significantly differed from that of SDR1 (treatment week). Over time, the standard deviations of the subsequent samples (SDR3–5) increased significantly. Shannon’s index showed that SDR3 (2 weeks after release) had the highest diversity, whereas the trend in Shannon’s index of other samples was similar to that of the Chao1 index, suggesting that the fermentation treatment increased the microbial diversity of the shrimp gut microbiota, whereas diversity decreased during natural shrimp pond cultivation. The Chao1 and Shannon’s diversity index value of each sample were shown in Supplementary Table S1.
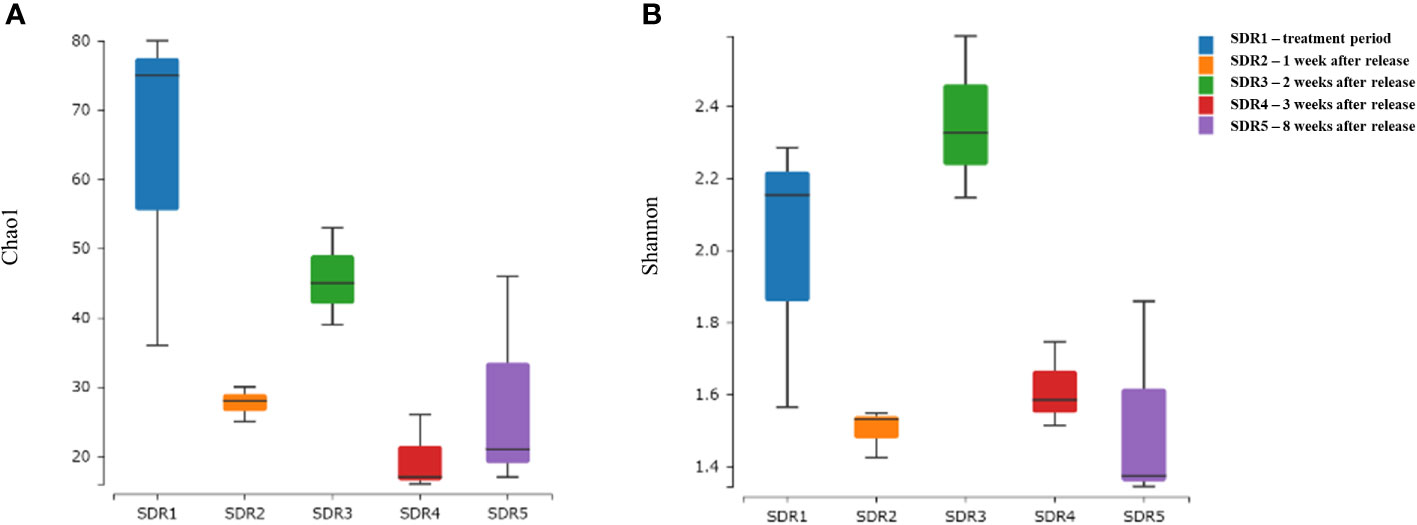
Figure 2 Box plots showing alpha diversity (A) Chao1 richness estimator and (B) Shannon Index variation between groups.
Principal coordinate analysis of beta diversity showed that the shrimp gut microbiota following the addition of fermentation products exhibited significant dispersion compared to that at other time points, especially over longer durations (Figure 3). However, after adding the fermentation product, the samples of shrimp released at 1 week and 2 weeks (SDR2 and SDR3) were distributed more closely together, whereas the samples released after 3 weeks (SDR4) and 8 weeks (SDR5) were similar, indicating that the addition of the fermentation product led to a more similar microbial community composition, which became stable after 3 weeks. Furthermore, the gut microbiota exhibited similar distributions at the same time points, suggesting that the gut microbiota of different shrimps at the same time points were similar and stably influenced by both the pond environment and the fermentation product. Furthermore, the adonis function based on permutational multivariate analysis of variance revealed a significant difference in beta diversity between the initial and subsequent shrimp samples (p < 0.05).
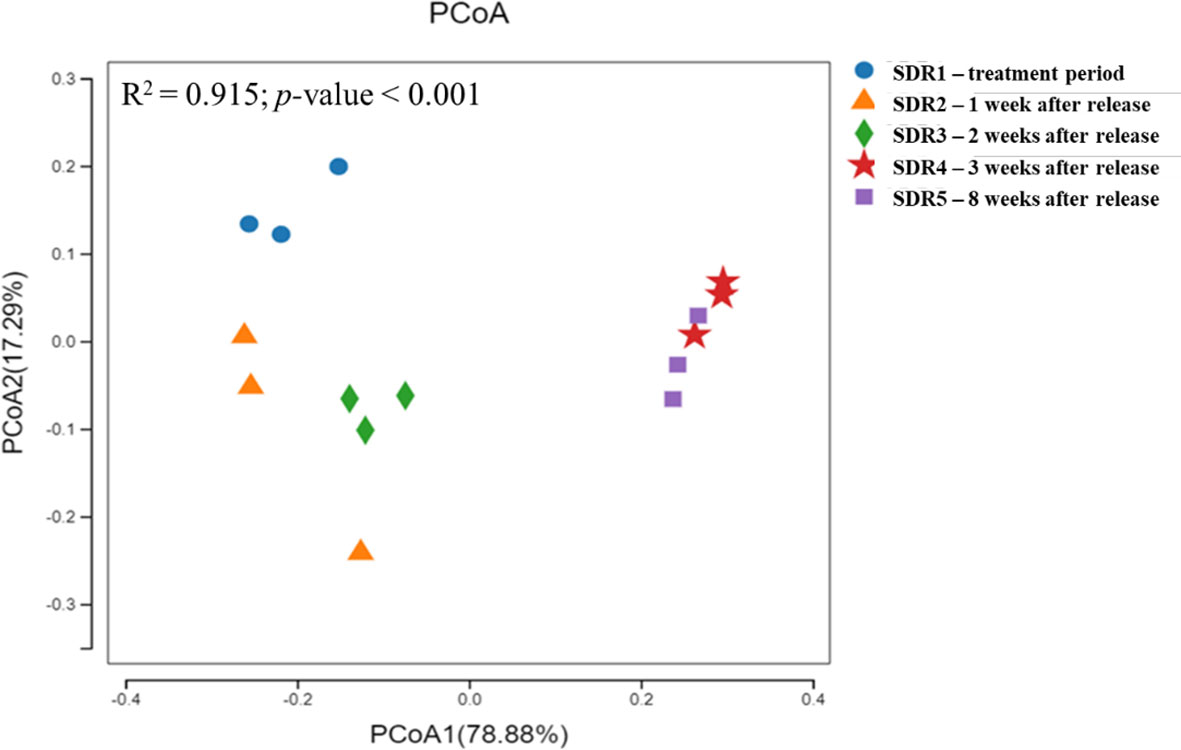
Figure 3 Principal coordinates analysis (PCoA) plot for each sample based on the weighted UniFrac metric.
3.3 Differential analysis at taxonomic levels associated with the gut cavity from five time point experiments
LEfSe analysis coupled with the logarithmic linear discriminant analysis score was performed to explore the significant differences in bacterial taxa between the gut microbiota of shrimp at different time points (Figure 4A). The LEfSe analysis revealed 34 significantly different bacteria at different taxonomic levels. Among these, only one significant bacterium was enriched in shrimp gut microbiotas 8 weeks after release (SDR5), whereas 15 bacteria were significantly enriched in shrimp guts 1 week after pond release (SDR2). These significantly enriched bacteria were typically more abundant in association with aquatic bacteria (Figure 4B), with significant differences between groups. At 3 and 8 weeks after pond release the most common bacterial genera in shrimp gut microbiotas were mostly related to Vibrio, whereas at 2 weeks post release, there was more Celeribacter and Donghicola, both belonging to the family Rhodobacteraceae. SDR2 (1 week after release) showed a higher abundance of genera associated with Actinomycetota and Rhodobacteraceae. However, the SDR1 (treatment week) sample subjected to fermentation product culture showed a diverse pattern, with phyla, classes, and genera such as Flavobacterium, Bacteroidetes, Clostridia, and Firmicutes.
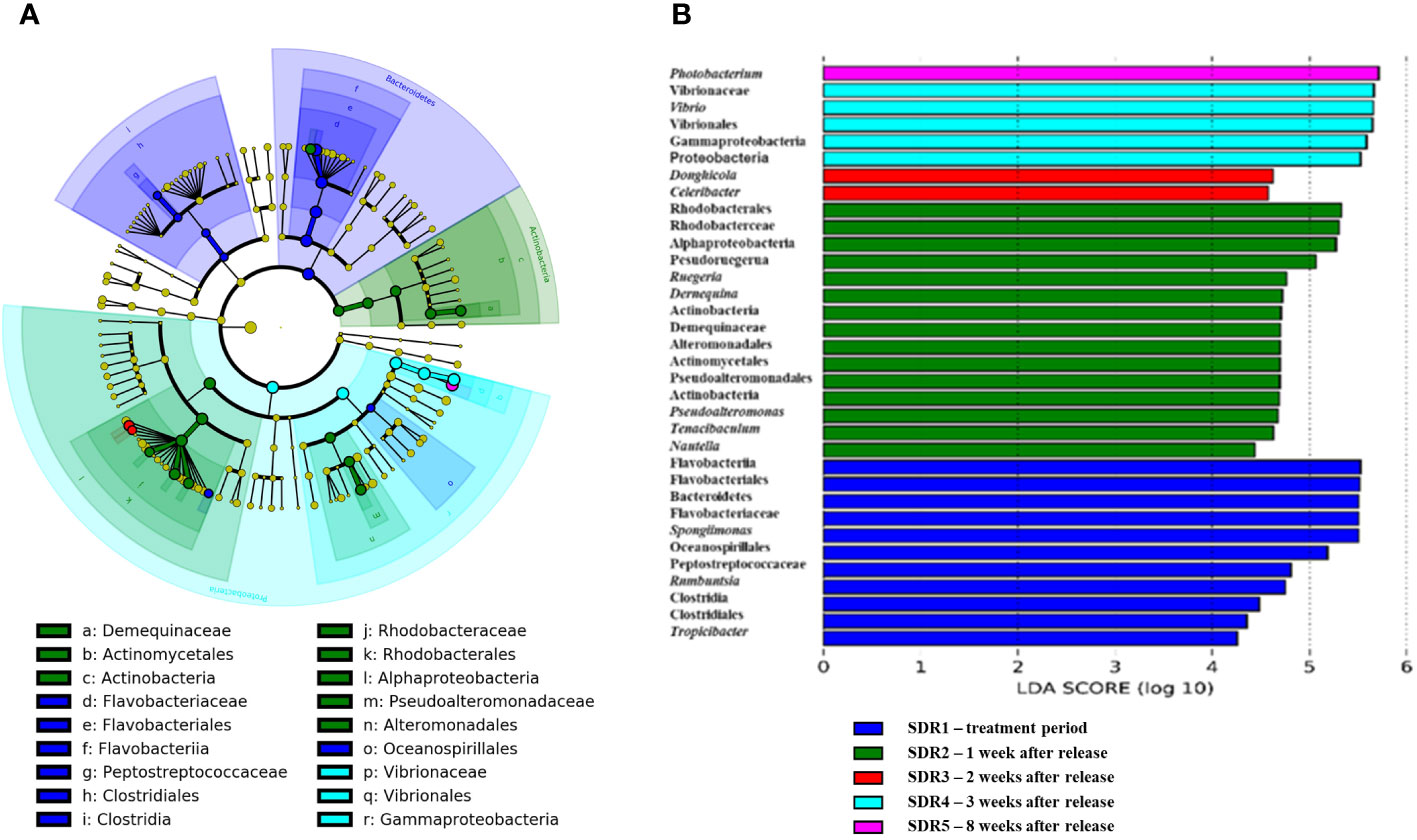
Figure 4 (A) Cladogram showing differentially abundant taxa at the species level associated with the gut microbiota of shrimp. (B) Bar graph showing the relative abundance of these differential abundant taxa related to the gut microbiota of shrimp at different time points.
3.4 Differential analysis of key bacteria associated with the gut cavity from five time point experiments
We conducted a statistical analysis (Wilcoxon rank-sum test) of the relative abundance ratios at five different time points based on important bacterial phyla (Firmicutes and Bacteroidetes) commonly used to assess nutrient absorption, as well as two important harmful and beneficial bacterial genera (Vibrio and Cetobacterium), as shown in Figure 5. The results indicated that the relative abundance of Firmicutes in SDR1 was significantly higher than that of other species, indicating that the abundance decreased over time after returning to the natural pond. In contrast, although Bacteroidetes showed a significantly higher abundance in SDR1 (treatment week) than in SDR3–5 (2 to 8 weeks after release), its decrease in relative abundance before SDR3 (2 weeks after release) was not as rapid as that of Firmicutes. This finding suggests that after the shrimp were removed from the fermentation solution, there was a lack of phyla such as Firmicutes in the natural pond, leading to a rapid decline in the shrimp intestinal microbiota and resulting in a drastic reduction in the Firmicutes to Bacteroidetes ratio.
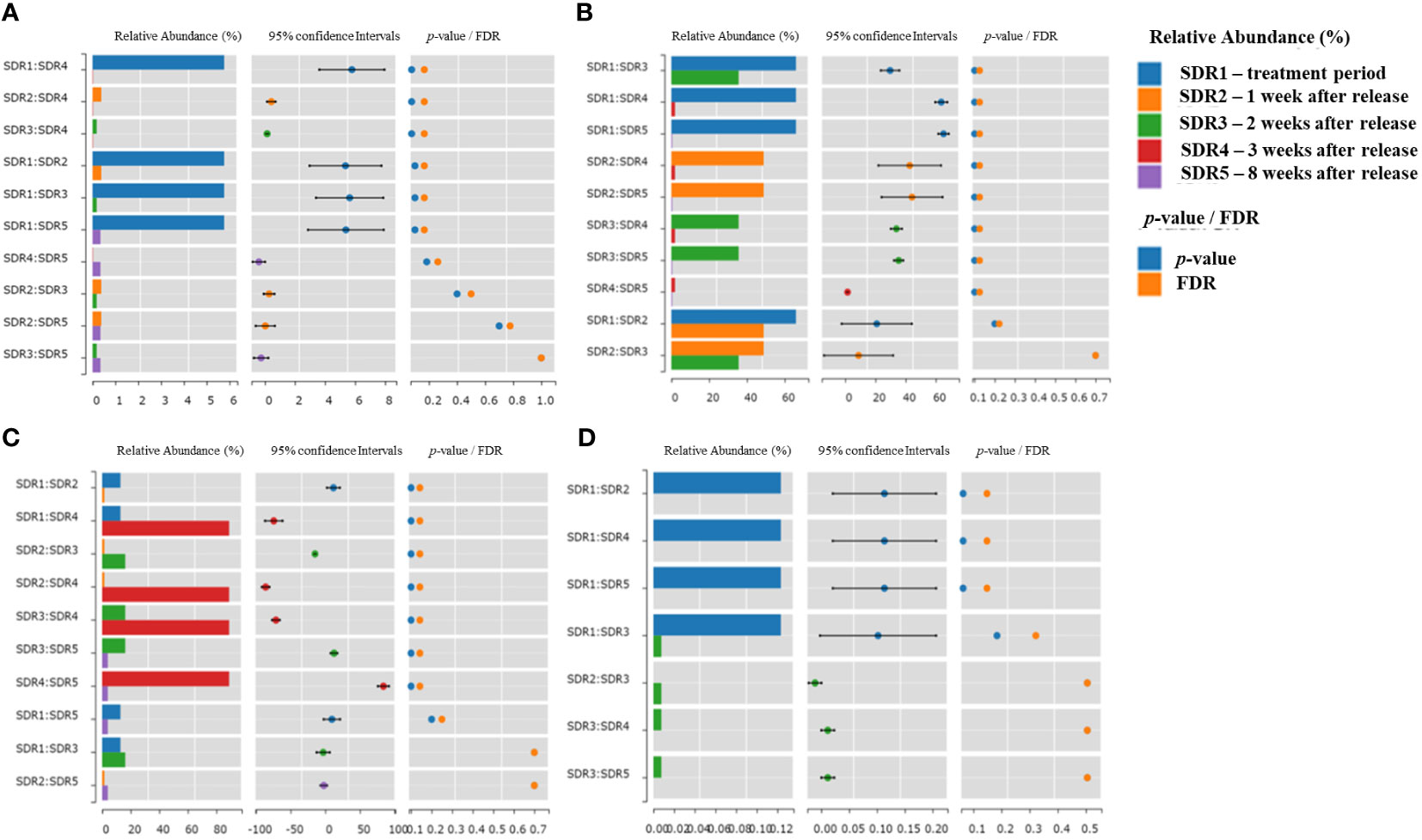
Figure 5 Relative abundance and statistical analysis of key bacterial phyla/genera at five different sampling time points. (A) Firmicutes, (B) Bacteroidetes, (C) Vibrio, (D) Cetobacterium.
Analysis of the relative abundance of the genus Vibrio, which is harmful to shrimp growth, revealed the highest proportion in SDR4 (3 weeks after release), with relatively fewer occurrences during the other periods. Although the relative abundance of Vibrio decreased in SDR5 (8 weeks after release), this period was dominated by the genus Photobacterium, which is also related to Vibrio bacteria. Hence, Vibrio became predominant after SDR4 treatment (3 weeks after release). The genus Cetobacterium contains potentially beneficial bacteria and was significantly more abundant in SDR1 (treatment week) than in other samples. These bacteria showed a low level of SDR3 (2 weeks after released) but were absent from SDR2, SDR4, and SDR5 (1, 3, and 8 weeks after release, respectively). This result indicated that Cetobacterium underwent abundant proliferation during fermentation solution cultivation and rapidly decreased after returning to the natural pond. Although some of these bacteria may have been present, they did not grow in the shrimp intestine over the long term.
4 Discussion
The highest number of OTUs was observed during the treatment week (SDR1). However, during SDR2 (1 week after release), when the shrimp were reintroduced into the natural pond, only six exclusive OTUs remained. Over time, SDR3 (2 weeks after release) showed 20 exclusive OTUs of SDR2 (1 week after release), and SDR4 (3 week after release) had only five exclusive OTUs after an additional week. After a more extended SDR5 (8 week after release) treatment period, the number of exclusive OTUs increased. This indicates that the shrimp gut microbiota undergoes sequential changes in the natural environment over time. However, abrupt changes may also occur, mainly related to the environment. These results are similar to those of other temporal studies (Landsman et al., 2019; Dai et al., 2020). Furthermore, because of the influence of fermentation fluid, there were significantly more exclusive OTUs in the gut microbiota of SDR1 (treatment week), which has also been observed in other shrimp populations cultured with probiotics or fermentation products (Sha et al., 2016; Cheng et al., 2017; Mazon-Suastegui et al., 2020). To compare relative abundance, we sequenced the V3-4 region of 16S rRNA and employed OTU-based taxonomic analysis. In previous studies, this method may have had limitations in analyzing bacteria with slight variation in the V3-4 region, leading to species bias (Holt et al., 2021). Therefore, in terms of relative abundance comparisons, we analyzed fewer species. According to García-López et al.’s study, comparative analyses of shrimp intestinal microbiota using 97% OTU, 99% OTU, or ASV methods demonstrated comparability at the family level (Garcia-Lopez et al., 2021). At the genus and species levels, 97% OTU exhibited differences compared to the other two methods. Surprisingly, 97% OTU, however, produced comparable α and β-diversity profiles. In summary, the use of ASV in 16S rRNA short fragment analysis tends to yield more species and diversity. Still, the validity remains subject to debate due to the short fragment length. On the other hand, the use of the 97% OTU classification method may underestimate species diversity but demonstrates significant richness, α-diversity, and β-diversity as expected. Consequently, the future mainstream approach in microbiota analysis will be using 16S full-length sequencing analysis in conjunction with ASV (Mou et al., 2023; Su et al., 2023).
Based on species analysis, Vibrio-related bacterial communities were consistently present in the shrimp gut microbiota throughout the various stages of this study. However, during the natural pond cultivation phase, a noticeable increase in the proportion of Vibrio was observed. Therefore, the diversity of samples from different cultivation times appeared more similar in the principal coordinate analysis. A high representation of Vibrio in the shrimp gut microbiota during conventional cultivation was commonly observed in many previous studies, particularly for the genera Vibrio and Photobacterium, which account for over 70% of the composition in both wild-caught and domesticated shrimp gut microbiota (Holt et al., 2021; Tzuc et al., 2014; Rungrassamee et al., 2016; Zheng et al., 2017). These two genera were also rich in shrimp gut microbiotas at 3 and 8 weeks after pond release, respectively. One primary reason for this result is that many species within the Vibrio spp. produce chitinase enzymes (Sugita and Ito, 2006), which may have contributed to the consistently high abundance of Vibrio in the shrimp gut microbiota. However, within Vibrio spp., there is a higher proportion of potentially harmful bacterial species, resulting in suppression of Vibrio-related bacterial communities in the shrimp gut microbiota, a research focus. In addition to their potential adverse effects on shrimp growth (Lavilla-Pitogo et al., 1998), many Vibrio species affect human health (Manilal et al., 2010; Lin et al., 2021), making them an important aspect of food safety in the farm-to-table chain (Yang et al., 2019). We showed that the fermentation products inhibited the proliferation of Vibrio and enhanced the overall microbial diversity. Additionally, during the initial 2 weeks of natural pond cultivation, the proportion of Vibrio remained relatively low. However, because we sequenced only the 16S V3-4 region, further identification of Vibrio species is necessary to provide more information related to food safety and host health.
Previous research has shown that in shrimp gut microbiota with slower growth rates, microbial diversity was significantly reduced compared to shrimp exhibiting faster growth (Holt et al., 2021; Xiong et al., 2017). The gut microbiota of the shrimp during the treatment week (SDR1) phase, when raised in a fermentation fluid environment, demonstrated higher diversity. However, after reintroduction to the natural pond, both alpha diversity and the total number of bacterial species and OTUs decreased significantly over time. The Shannon’s index of SDR3 (2 week after release) is high, but its Chao index is lower than that of SDR1 (treatment week), indicating that during the SDR3 period, although the richness is not as high as SDR1, the overall community diversity is high. Additionally, based on the microbial composition distribution diagram (Figure 1), it can be observed that SDR3 has a relatively similar composition distribution to SDR1(treatment week), but with a higher species diversity, potentially influenced by microbial communities in the natural environment. Additionally, during SDR4 (3 weeks after release), there was a notably higher presence of Gammaproteobacteria than during the other periods. Previous studies have indicated that a substantial decrease in bacterial diversity in slow-growing shrimp is linked to a sharp increase in the relative abundance of Gammaproteobacteria (Xiong et al., 2017). This is a critical concern because the presence of both Vibrio and Gammaproteobacteria 3 weeks after reintroduction into the natural pond may lead to sluggish shrimp growth. Another significant factor was the ratio of Firmicutes to Bacteroidetes. According to Fan and Li’s 2019 study of the white shrimp Litopenaeus vannamei in marine aquaculture, the Firmicutes to Bacteroidetes ratio was 0.34 to 6.04 for normal growth and 3.08 to 3.08 for slow growth (Fan and Li, 2019). Thus, when Firmicutes and Bacteroidetes were low and high, respectively, the growth rates tended to be slow. Our results demonstrated a substantial reduction in Firmicutes after reintroduction into the natural pond; although Bacteroidetes also decreased, the proportional difference was significant. Furthermore, both Firmicutes and Bacteroidetes were nearly absent from the later stages of natural pond cultivation, which may have impacted overall shrimp growth and is a cause for concern. Cetobacterium is considered beneficial because it stimulates the production of vitamin B12 in the gut, thereby enhancing host immune responses against pathogens (Qi et al., 2023). Our results revealed a high proportion of this genus in the gut microbiota of shrimp cultured in fermentation products. However, this proportion decreased significantly after reintroduction into natural ponds. This suggests that this genus cannot effectively establish itself in the shrimp gut in a typical natural pond environment, primarily because of environmental factors and the influence of existing microbial communities. Consequently, fermentation products can promote the growth of this genus in the shrimp gut, potentially suppressing the proliferation of pathogens. Thus, during SDR1 (treatment week), the proportion of Vibrio species was relatively low, and other species in the Enterobacteriaceae family were relatively scarce. Previous studies have indicated a significant correlation between Enterobacteriaceae species and shrimp diseases associated with algae and pond water (Gardiner et al., 2015; Zheng et al., 2016). Therefore, the relatively high presence of species from the Enterobacteriaceae family during SDR3 (2 weeks after release) is important. LEfSe outcomes showed that the Celeribacter and Donghicola were significant presented in SDR3, they are a genus of bacteria from the family of Rhodobacteraceae (Yoon et al., 2007). LEfSe was a powerful tool to identify the most differentially abundant taxa between groups.
Limitations of this study include the absence of microbial analysis of the original herbal additive and the lack of pretreatment shrimp intestinal microbial data before exposure to the herbal additive. However, the primary focus of this study was to investigate the changes in the intestinal microbial community of shrimp in conventional aquaculture after treatment with an herbal additive solution. This information serves as a reference for suggesting subsequent application times. The study involved a triplicate experimental design, with each experiment comprising mixed samples from the intestines of five shrimp species. Furthermore, the use of the V3-4 region may introduce bias into the classification. Future studies should consider utilizing the full-length 16S rRNA gene for a more comprehensive analysis.
5 Conclusions
After treatment with herbal additives, the microbiota of shrimp intestines exhibited higher compositional diversity and richness compared to those of shrimp release natural ponds. However, notable changes occurred in the microbiota upon returning the shrimp to the natural pond, characterized by a significant increase in the abundance of the Vibrio genus and the Rhodobacteraceae family, particularly after the third week. Further exploration of Vibrio species is warranted at this stage to ascertain their potential association with shrimp growth or assess their pathogenicity. It is crucial to note that the 16S V3-4 region sequencing performed in this study provides taxonomic resolution only at the genus level or coarser. For a more comprehensive analysis, especially for better understanding species that may not be distinctly identified in the V3-4 region, full-length 16S rRNA sequencing should be conducted. This limitation should be taken into consideration when interpreting the study’s findings. In conclusion, this study presents a temporal assessment of microbiota changes in shrimp reintroduced into natural ponds after herbal additive treatment. The results serve as a reference for evaluating shrimp health or determining optimal timing for additive administration in the future.
Data availability statement
The datasets presented in this study can be found in online repositories utilized in this study are available at Figshare (10.6084/m9.figshare.25294936).
Author contributions
X-YL: Conceptualization, Data curation, Funding acquisition, Methodology, Project administration, Supervision, Validation, Writing – original draft. Y-JS: Conceptualization, Funding acquisition, Investigation, Methodology, Project administration, Supervision, Validation, Writing – review & editing. X-JZ: Formal analysis, Investigation, Software, Validation, Writing – original draft. Y-SC: Resources, Writing – original draft. X-WZ: Resources, Writing – original draft. J-SC: Funding acquisition, Project administration, Software, Writing – original draft, Writing – review & editing.
Funding
The author(s) declare financial support was received for the research, authorship, and/or publication of this article. This research was funded by the Fujian Collaborative Innovation Center of Marine Biology Application Technology (grant number XTZX-HYSW- 1802 and XTZX-HYSW- 1804 and E-Da Hospital (grant numbers EDPJ111063 and EDPJ111066).
Conflict of interest
Author Y-SC was employed by the company Haiyo Biotechnology Co., LTD, Xiamen, China.
The remaining authors declare that the research was conducted in the absence of any commercial or financial relationships that could be construed as a potential conflict of interest.
Publisher’s note
All claims expressed in this article are solely those of the authors and do not necessarily represent those of their affiliated organizations, or those of the publisher, the editors and the reviewers. Any product that may be evaluated in this article, or claim that may be made by its manufacturer, is not guaranteed or endorsed by the publisher.
Supplementary material
The Supplementary Material for this article can be found online at: https://www.frontiersin.org/articles/10.3389/fmars.2024.1332585/full#supplementary-material
References
Adak A., Khan M. R. (2019). An insight into gut microbiota and its functionalities. Cell Mol. Life Sci. 76, 473–493. doi: 10.1007/s00018-018-2943-4
Balcazar J. L., de Blas I., Ruiz-Zarzuela I., Cunningham D., Vendrell D., Muzquiz J. L. (2006). The role of probiotics in aquaculture. Vet. Microbiol. 114, 173–186. doi: 10.1016/j.vetmic.2006.01.009
Bhogoju S., Nahashon S. (2022). Recent advances in probiotic application in animal health and nutrition: A review. Agriculture 12, 304. doi: 10.3390/agriculture12020304
Bolyen E., Rideout J. R., Dillon M. R., Bokulich N. A., Abnet C. C., Al-Ghalith G. A., et al. (2019). Reproducible, interactive, scalable and extensible microbiome data science using QIIME 2. Nat. Biotechnol. 37, 852–857. doi: 10.1038/s41587-019-0209-9
Cai X., J-s W., Long H., Ren W., Zhang X., Huang A.-y., et al. (2022). The probiotic effects, dose, and duration of lactic acid bacteria on disease resistance in Litopenaeus vannamei. Aquacult. Rep. 26, 101299. doi: 10.1016/j.aqrep.2022.101299
Chen H., Boutros P. (2011). VennDiagram: a package for the generation of highly-customizable Venn and Euler diagrams in R. BMC Bioinf. 12, 35. doi: 10.1186/1471-2105-12-35
Cheng A. C., Lin H. L., Shiu Y. L., Tyan Y. C., Liu C. H. (2017). Isolation and characterization of antimicrobial peptides derived from Bacillus subtilis E20-fermented soybean meal and its use for preventing Vibrio infection in shrimp aquaculture. Fish Shellfish Immunol. 67, 270–279. doi: 10.1016/j.fsi.2017.06.006
Dai W., Sheng Z., Chen J., Xiong J. (2020). Shrimp disease progression increases the gut bacterial network complexity and abundances of keystone taxa. Aquaculture 517, 734802. doi: 10.1016/j.aquaculture.2019.734802
de Souza Valente C., Rodiles A., Freire Marques M. R., Merrifield D. L. (2020). White spot syndrome virus (WSSV) disturbs the intestinal microbiota of shrimp (Penaeus vannamei) reared in biofloc and clear seawater. Appl. Microbiol. Biotechnol. 104, 8007–8023. doi: 10.1007/s00253-020-10816-4
Diwan A. D., Harke S. N. (2022). Gopalkrishna, Panche AN: Aquaculture industry prospective from gut microbiome of fish and shellfish: An overview. J. Anim. Physiol. Anim. Nutr. (Berl) 106, 441–469. doi: 10.1111/jpn.13619
El-Saadony M., Shehata A., Alagawany M., Abdel-Moneim A.-M., Selim D., Abdo M., et al. (2022). A review of shrimp aquaculture and factors affecting the gut microbiome. Aquacult. Int. 30, 2847–2869. doi: 10.1007/s10499-022-00936-1
Fan L., Li Q. X. (2019). Characteristics of intestinal microbiota in the Pacific white shrimp Litopenaeus vannamei differing growth performances in the marine cultured environment. Aquaculture 505, 450–461. doi: 10.1016/j.aquaculture.2019.02.075
Farzanfar A. (2006). The use of probiotics in shrimp aquaculture. FEMS Immunol. Med. Microbiol. 48, 149–158. doi: 10.1111/j.1574-695X.2006.00116.x
Feingold K. R. (2000). “The Effect of Diet on Cardiovascular Disease and Lipid and Lipoprotein Levels,” in Endotext. Eds. Feingold, et al. (MA: South Dartmouth).
Fuller R. (1989). Probiotics in man and animals. J. Appl. Bacteriol. 66, 365–378. doi: 10.1111/j.1365-2672.1989.tb05105.x
Gainza O., Romero J. (2020). Effect of mannan oligosaccharides on the microbiota and productivity parameters of Litopenaeus vannamei shrimp under intensive cultivation in Ecuador. Sci. Rep. 10, 2719. doi: 10.1038/s41598-020-59587-y
Garcia-Lopez R., Cornejo-Granados F., Lopez-Zavala A. A., Cota-Huizar A., Sotelo-Mundo R. R., Gomez-Gil B., et al. (2021). OTUs and ASVs produce comparable taxonomic and diversity from shrimp microbiota 16S profiles using tailored abundance filters. Genes (Basel) 12 (4), 564. doi: 10.3390/genes12040564
Gardiner M., Thomas T., Egan S. (2015). A glutathione peroxidase (GpoA) plays a role in the pathogenicity of Nautella italica strain R11 towards the red alga Delisea pulchra. FEMS Microbiol. Ecol. 91, fiv021. doi: 10.1093/femsec/fiv021
Hassan M. A., Fathallah M. A., Elzoghby M. A., Salem M. G., Helmy M. S. (2022). Influence of probiotics on water quality in intensified Litopenaeus vannamei ponds under minimum-water exchange. AMB Express 12, 22. doi: 10.1186/s13568-022-01370-5
He X., Abakari G., Tan H., Liu W., Luo G. (2023). Effects of different probiotics (Bacillus subtilis) addition strategies on a culture of Litopenaeus vannamei in biofloc technology (BFT) aquaculture system. Aquaculture 566, 739216. doi: 10.1016/j.aquaculture.2022.739216
Holt C. C., Bass D., Stentiford G. D., van der Giezen M. (2021). Understanding the role of the shrimp gut microbiome in health and disease. J. Invertebr. Pathol. 186, 107387. doi: 10.1016/j.jip.2020.107387
Kumar V., Roy S., Meena D. K., Sarkar U. K. (2016). Application of probiotics in shrimp aquaculture: importance, mechanisms of action, and methods of administration. Rev. Fish. Sci. Aquacult. 24, 342–368. doi: 10.1080/23308249.2016.1193841
Landsman A., St-Pierre B., Rosales-Leija M., Brown M., Gibbons W. (2019). Investigation of the potential effects of host genetics and probiotic treatment on the gut bacterial community composition of aquaculture-raised pacific whiteleg shrimp, litopenaeus vannamei. Microorganisms 7 (8), 217. doi: 10.3390/microorganisms7080217
Lavilla-Pitogo C. R., Leaño E. M., Paner M. G. (1998). Mortalities of pond-cultured juvenile shrimp, Penaeus monodon, associated with dominance of luminescent vibrios in the rearing environment. Aquaculture 164, 337–349. doi: 10.1016/S0044-8486(98)00198-7
Liao W., Huang L., Han S., Hu D., Xu Y., Liu M., et al. (2022). Review of medicinal plants and active pharmaceutical ingredients against aquatic pathogenic viruses. Viruses 14, 1281. doi: 10.3390/v14061281
Lightner D. V. (2011). Virus diseases of farmed shrimp in the Western Hemisphere (the Americas): a review. J. Invertebr. Pathol. 106, 110–130. doi: 10.1016/j.jip.2010.09.012
Lin I. C., Hussain B., Hsu B. M., Chen J. S., Hsu Y. L., Chiu Y. C., et al. (2021). Prevalence, genetic diversity, antimicrobial resistance, and toxigenic profile of vibrio vulnificus isolated from aquatic environments in Taiwan. Antibiot. (Basel) 10 (5), 505. doi: 10.3390/antibiotics10050505
Ma K., Chen S., Wu Y., Ma Y., Qiao H., Fan J., et al. (2022). Dietary supplementation with microalgae enhances the zebrafish growth performance by modulating immune status and gut microbiota. Appl. Microbiol. Biotechnol. 106, 773–788. doi: 10.1007/s00253-021-11751-8
Manilal A., Sujith S., Selvin J., Shakir C., Gandhimathi R., Kiran G. S. (2010). Virulence of vibrios isolated from diseased black tiger shrimp, Penaeus monodon, Fabricius. J. World Aquacult. Soc. 41, 332–343. doi: 10.1111/jwas.2010.41.issue-3
Martinez Cruz P., Ibanez A. L., Monroy Hermosillo O. A., Ramirez Saad H. C. (2012). Use of probiotics in aquaculture. ISRN Microbiol. 2012, 916845. doi: 10.5402/2012/916845
Mazon-Suastegui J. M., Salas-Leiva J. S., Medina-Marrero R., Medina-Garcia R., Garcia-Bernal M. (2020). Effect of Streptomyces probiotics on the gut microbiota of Litopenaeus vannamei challenged with Vibrio parahaemolyticus. Microbiologyopen 9, e967. doi: 10.1002/mbo3.967
McMurdie P., Holmes S. (2013). Phyloseq: an R package for reproducible interactive analysis and graphics of microbiome census data. PloS One 8, e61217. doi: 10.1371/journal.pone.0061217
Meng X., Cai H., Li H., You F., Jiang A., Hu W., et al. (2023). et al: Clostridium butyricum-fermented Chinese herbal medicine enhances the immunity by modulating the intestinal microflora of largemouth bass (Micropterus salmoides). Aquaculture 562, 738768. doi: 10.1016/j.aquaculture.2022.738768
Mou C. Y., Li Q., Huang Z. P., Ke H. Y., Zhao H., Zhao Z. M., et al. (2023). et al: PacBio single-molecule long-read sequencing provides new insights into the complexity of full-length transcripts in oriental river prawn, macrobrachium nipponense. BMC Genomics 24, 340. doi: 10.1186/s12864-023-09442-x
Nourizadeh R., Sepehri B., Abbasi A., Sayyed R. Z., Khalili L. (2022). Impact of Probiotics in Modulation of Gut Microbiome (Singapore: Springer).
Parks D. H., Tyson G. W., Hugenholtz P., Beiko R. G. (2014). STAMP: statistical analysis of taxonomic and functional profiles. Bioinformatics 30, 3123–3124. doi: 10.1093/bioinformatics/btu494
Qi X., Zhang Y., Zhang Y., Luo F., Song K., Wang G., et al. (2023). Vitamin B12 produced by Cetobacterium somerae improves host resistance against pathogen infection through strengthening the interactions within gut microbiota. Microbiome 11, 135. doi: 10.1186/s40168-023-01574-2
Ringo E., Harikrishnan R., Soltani M., Ghosh K. (2022). The effect of gut microbiota and probiotics on metabolism in fish and shrimp. Anim. (Basel) 12 (21), 301. doi: 10.3390/ani12213016
Rungrassamee W., Klanchui A., Maibunkaew S., Karoonuthaisiri N. (2016). Bacterial dynamics in intestines of the black tiger shrimp and the Pacific white shrimp during Vibrio harveyi exposure. J. Invertebr. Pathol. 133, 12–19. doi: 10.1016/j.jip.2015.11.004
Sha H., Li L., Lu J., Xiong J. (2022). High nutrient induces virulence in the AHPND-causing Vibrio parahaemolyticus, interpretation from the ecological assembly of shrimp gut microbiota. Fish Shellfish Immunol. 127, 758–765. doi: 10.1016/j.fsi.2022.07.016
Sha Y., Liu M., Wang B., Jiang K., Qi C., Wang L. (2016). Bacterial population in intestines of litopenaeus vannamei fed different probiotics or probiotic supernatant. J. Microbiol. Biotechnol. 26, 1736–1745. doi: 10.4014/jmb.1603.03078
Soo T. C. C., Bhassu S. (2022). Biochemical indexes and gut microbiota testing as diagnostic methods for Penaeus monodon health and physiological changes during AHPND infection with food safety concerns. Food Sci. Nutr. 10, 2694–2709. doi: 10.1002/fsn3.2873
Su H. Y., Hussain B., Hsu B. M., Lee K. H., Mao Y. C., Chiang L. C., et al. (2023). Bacterial community analysis identifies Klebsiella pneumoniae as a native symbiotic bacterium in the newborn Protobothrops mucrosquamatus. BMC Microbiol. 23, 213. doi: 10.1186/s12866-023-02936-4
Sugita H., Ito Y. (2006). Identification of intestinal bacteria from Japanese flounder (Paralichthys olivaceus) and their ability to digest chitin. Lett. Appl. Microbiol. 43, 336–342. doi: 10.1111/j.1472-765X.2006.01943.x
Tadese D. A., Song C., Sun C., Liu B., Liu B., Zhou Q., et al. (2022). et al: The role of currently used medicinal plants in aquaculture and their action mechanisms: A review. Rev. Aquacult. 14, 816–847. doi: 10.1111/raq.12626
Toma R. B., James W. H. (1975). Nutritional evaluation of protein from shrimp cannery effluent (shrimp waste protein). J. Agric. Food Chem. 23, 1168–1171. doi: 10.1021/jf60202a042
Turner J. W. Jr., Cheng X., Saferin N., Yeo J. Y., Yang T., Joe B. (2022). Gut microbiota of wild fish as reporters of compromised aquatic environments sleuthed through machine learning. Physiol. Genomics 54, 177–185. doi: 10.1152/physiolgenomics.00002.2022
Tzuc J. T., Escalante D. R., Rojas Herrera R., Gaxiola Cortes G., Ortiz M. L. (2014). Microbiota from Litopenaeus vannamei: digestive tract microbial community of Pacific white shrimp (Litopenaeus vannamei). Springerplus 3, 280. doi: 10.1186/2193-1801-3-280
Vijayaram S., Sun Y. Z., Zuorro A., Ghafarifarsani H., Van Doan H., Hoseinifar S. H. (2022). Bioactive immunostimulants as health-promoting feed additives in aquaculture: A review. Fish Shellfish Immunol. 130, 294–308. doi: 10.1016/j.fsi.2022.09.011
Villanueva R. A., Chen Z. (2019). ggplot2: elegant graphics for data analysis (2nd ed.). Measure.: Interdiscip. Res. Perspect. 17, 160–167. doi: 10.1080/15366367.2019.1565254
Walker P. J., Winton J. R. (2010). Emerging viral diseases of fish and shrimp. Vet. Res. 41, 51. doi: 10.1051/vetres/2010022
Wang Y., Al Farraj D. A., Vijayaraghavan P., Hatamleh A. A., Biji G. D., Rady A. M. (2020). Host associated mixed probiotic bacteria induced digestive enzymes in the gut of tiger shrimp Penaeus monodon. Saudi J. Biol. Sci. 27, 2479–2484. doi: 10.1016/j.sjbs.2020.07.010
Wu H. J., Wu E. (2012). The role of gut microbiota in immune homeostasis and autoimmunity. Gut Microbes 3, 4–14. doi: 10.4161/gmic.19320
Xiong J., Dai W., Zhu J., Liu K., Dong C., Qiu Q. (2017). The underlying ecological processes of gut microbiota among cohabitating retarded, overgrown and normal shrimp. Microb. Ecol. 73, 988–999. doi: 10.1007/s00248-016-0910-x
Yang C., Zhang X., Fan H., Li Y., Hu Q., Yang R., et al. (2019). Genetic diversity, virulence factors and farm-to-table spread pattern of Vibrio parahaemolyticus food-associated isolates. Food Microbiol. 84, 103270. doi: 10.1016/j.fm.2019.103270
Yoon J. H., Kang S. J., Oh T. K. (2007). Donghicola eburneus gen. nov., sp. nov., isolated from seawater of the East Sea in Korea. Int. J. Syst. Evol. Microbiol. 57, 73–76. doi: 10.1099/ijs.0.64577-0
Zeng S., Duan Y., Li X., Hu Y., Mo Z., Dan X., et al. (2023). Effects of Cryptocaryon irritants infection on the histopathology, oxidative stress, immune response, and intestinal microbiota in the orange-spotted grouper Epinephelus coioides. Fish Shellfish Immunol. 133, 108562. doi: 10.1016/j.fsi.2023.108562
Zhai Q., Li J. (2019). Effectiveness of traditional Chinese herbal medicine, San-Huang-San, in combination with enrofloxacin to treat AHPND-causing strain of Vibrio parahaemolyticus infection in Litopenaeus vannamei. Fish shellfish Immunol. 87, 360–370. doi: 10.1016/j.fsi.2019.01.008
Zhang Y., Bian Y., Cui Q., Yuan C., Lin Y., Li J., et al. (2021). Effect of dietary complex Chinese herbal medicine on growth performance, digestive enzyme activities in tissues and expression of genes involved in the digestive enzymes and antioxidant enzymes and bacterial challenge in Litopenaeus vannamei. Aquacult. Res. 52, 6741–6750. doi: 10.1111/are.15544
Zheng Y., Yu M., Liu J., Qiao Y., Wang L., Li Z., et al. (2017). Bacterial Community Associated with Healthy and Diseased Pacific White Shrimp (Litopenaeus vannamei) Larvae and Rearing Water across Different Growth Stages. Front. Microbiol. 8, 1362. doi: 10.3389/fmicb.2017.01362
Keywords: shrimp, fermentation product, 16S rRNA gene sequencing, gut microbiota, vibrio related bacteria
Citation: Lin X-Y, Shih Y-J, Zhang X-J, Cai Y-S, Zhou X-W and Chen J-S (2024) Perspective on intestinal microbiota temporal changes of herbal additives treated shrimp in a natural aquaculture setting. Front. Mar. Sci. 11:1332585. doi: 10.3389/fmars.2024.1332585
Received: 13 November 2023; Accepted: 20 February 2024;
Published: 05 March 2024.
Edited by:
Marcel Martinez-Porchas, National Council of Science and Technology (CONACYT), MexicoReviewed by:
Estefanía Garibay, National Council of Science and Technology (CONACYT), MexicoConnie A. Rojas, University of California, Davis, United States
Yale Deng, University of Minnesota Twin Cities, United States
Copyright © 2024 Lin, Shih, Zhang, Cai, Zhou and Chen. This is an open-access article distributed under the terms of the Creative Commons Attribution License (CC BY). The use, distribution or reproduction in other forums is permitted, provided the original author(s) and the copyright owner(s) are credited and that the original publication in this journal is cited, in accordance with accepted academic practice. No use, distribution or reproduction is permitted which does not comply with these terms.
*Correspondence: Jung-Sheng Chen, bmlja3kwNzEyMTRAZ21haWwuY29t