- 1Department of Physical, Earth and Environmental Sciences, University of Siena, Siena, Italy
- 2Department of Integrative Marine Ecology (EMI), Genoa Marine Centre (GMC), Stazione Zoologica Anton Dohrn–National Institute of Marine Biology, Ecology and Biotechnology, Genoa, Italy
- 3Museo di Storia Naturale di Calci, University of Pisa, Pisa, Italy
- 4Istituto Zooprofilattico Sperimentale Lazio e Toscana, Sezione di Pisa, Pisa, Italy
- 5ARPAT (Agenzia Regionale Protezione Ambiente Toscana), Livorno, Italy
- 6Consorzio per il Centro Interuniversitario di Biologia Marina ed Ecologia Applicata “G. Bacci” (CIBM), Scoglio della Regina, Livorno, Italy
- 7Acquario di Livorno, Costa Edutainment S.p.A, Livorno, Italy
- 8Department of Life Sciences, University of Siena, Siena, Italy
- 9Accademia dei Fisiocritici, Siena, Italy
- 10Ente Parco Regionale della Maremma, Grosseto, Italy
- 11Centro Interuniversitario per la Ricerca sui Cetacei (CIRCE), Department of Physical, Earth and Environmental Sciences, University of Siena, Siena, Italy
Since 2013, loggerhead sea turtle (Caretta caretta, Linnaeus 1758) nesting has been observed further north along the Italian coast, reaching the Tuscan coast (NW Mediterranean Sea). The four nesting events that occurred in Tuscany in the summer of 2019 spurred the scientific community to monitor these occurrences more carefully, following them from egg deposition to hatching. This provided an opportunity to collect samples for conducting multidisciplinary investigations, including the toxicological investigations of the biological material collected from the four nests. The aim of this study was to conduct an initial assessment of persistent organic pollutants in the eggs laid in Tuscany, aiming to establish a baseline on this topic for subsequent nesting events that have occurred until today. Organochlorine compounds (OCs), specifically polychlorinated biphenyls (PCBs), dichlorodiphenyltrichloroethane (DDT) and its metabolites, and hexachlorobenzene (HCB), were analyzed and detected in unhatched eggs, embryos, and chorio-allantoic membranes (CAMs). OCs were detected in all samples, with PCBs > DDTs ≫ HCB. A significant spatial variation in pollutant levels and profiles among sea turtle nesting locations was found. Embryos showed higher levels of contamination than egg contents regardless of the developmental stages. Depth of the laying chamber and egg mass were not significant factors in OC bioaccumulation. For the first time in the Mediterranean Sea, this study assessed the role of CAM in the transfer of contaminants to the embryo. Overall, the OC levels found were lower compared to the results from other studies conducted worldwide on loggerhead sea turtle biological material. This was the first assessment of nest biological material for the North-Western coast of Italy.
1 Introduction
The Mediterranean Sea is known as a Large Marine Ecosystem (Med-LME) (Piroddi et al., 2020) with unique characteristics in terms of human history, biogeography, biology, and biodiversity. Even though it represents less than 1% of the global ocean surface, it is considered one of the hotspots for marine biodiversity with ~17,000 marine species, one-fifth of which are endemic, and more than 8% are classified as threatened species (IUCN, 2018; Crosti et al., 2020). Representative of these species is the loggerhead sea turtle, Caretta caretta (Linnaeus, 1758), which is the most abundant sea turtle in the Mediterranean basin, and it is an omnivorous, long-living, and opportunistic species (Casale et al., 2018). In the past (1996–2015), the Red List of the International Union for Conservation of Nature (IUCN) assessed C. caretta’s conservation status at the global level as Endangered; from 2015, this species is classified as Vulnerable (Casale and Tucker, 2017). The Mediterranean subpopulation (Wallace et al., 2011; Wallace et al., 2010) showed an overall increase over the past three generations, with an estimated adult population of 21,414 individuals and 7,200 average annual number of nests; therefore, it is considered Least Concern under the current IUCN Red List criteria (Casale, 2015). Nevertheless, the conservation of this marine species is threatened by various anthropic pressures coming from both land and sea, such as bycatch (e.g., pelagic longline fishery), intense tourism, habitat reduction of coastal habitats for turtles (e.g., nesting beaches), marine debris and pollution, pathogens (e.g., Salmonella spp.), and climate change (Casale et al., 2018; Fichi et al., 2016; Novillo et al., 2017; Casini et al., 2018; Lucchetti et al., 2019). Thus, monitoring of Mediterranean loggerhead sea turtle results is a priority in order to avoid downgrading to higher risk IUCN Red List categories as in the past (Casale et al., 2018). As far as the pressure of chemical pollution is concerned, organochlorine compounds (OCs) are among the major contaminants in the Mediterranean basin (Roscales et al., 2011). The Stockholm Convention adopted in 2001 severely limited, but preferentially banned, the production and use of 12 key persistent organic pollutants (POPs) including some OCs (Lallas, 2001). After the review of the Stockholm Convention in 2019, an additional 31 contaminants were banned. Additionally, other countries that had not yet ratified the convention can also ratify it at a later date, such as Italy, which has made it enter into force on 2023 (Multilateral Treaties Deposited with the Secretary-General, 2024). As a result, high levels of these persistent pollutants, particularly in the Mediterranean Sea (Hoffman et al., 2002; Giesy et al., 2014), continue to have harmful effects on large marine vertebrates including cetaceans, turtles, and elasmobranchs (Fossi and Marsili, 2003; Fossi et al., 2003; D’Ilio et al., 2011), disrupting their immunological, reproductive, and endocrine systems (Keller et al., 2004b; Keller et al., 2006; Cocci et al., 2018; Montes et al., 2020). Loggerhead sea turtles are considered highly exposed to the accumulation of these chemical compounds due to their longevity, omnivorous diet, and wide spatial distribution (Lazar et al., 2011; Camacho et al., 2013; Camacho et al., 2014; Storelli and Zizzo, 2014). Indeed, sea turtles are appropriate sentinels for long-term accumulation of POPs in oceanic, neritic, and coastal ecosystems due to large migrations and fidelity to specific foraging and nesting locations (Alava et al., 2006; Keller, 2013b).
Many studies have focused on the ecotoxicological status of adult C. caretta specimens (Casini et al., 2018; Campani et al., 2013; Caliani et al., 2014; Caliani et al., 2019; Bucchia et al., 2015; Savoca et al., 2018; Bianchi et al., 2022), and despite that there are some studies on nest monitoring and/or on hatching egg success (Clabough et al., 2022; Margaritoulis et al., 2022), the toxicological status of the biological material sampled in the nesting chambers has not been extensively investigated. Given this, it was strongly advised to determine POPs in biological material such as egg contents, embryonic annexes, and embryos that have been collected from the nest chamber in order to assess any potential negative direct impacts on sea turtle reproductive success and developing embryos. Moreover, monitoring pollution in nest biological material may be used as a proxy to biomonitor to track POP contamination in the Mediterranean population of loggerhead sea turtles (Muñoz and Vermeiren, 2020; Muñoz et al., 2021; Savoca et al., 2021). In light of this, the present study intends to examine for the first time the levels of polychlorinated biphenyls (PCBs), dichlorodiphenyltrichloroethane (DDT) and its metabolites (DDE and DDD), and hexachlorobenzene (HCB) in the biological material from four C. caretta nests laid along the coast of Tuscany (Italy) in 2019. Thus, employing sea turtles’ egg contents and their progeny as a case study of long-lived wildlife species trends would enable the identification of patterns in the internal distribution of POPs and the assessment of contributing factors (Muñoz et al., 2021). The uncommon and extraordinary loggerhead sea turtle nesting events that were initially documented in 2013 near Capalbio (Grosseto, Italy) and from which time nesting events were constantly being tracked over the following nesting seasons led researchers to choose the Tuscan coast as a sampling site (Mancusi et al., 2023). The presence of OCs was thus assessed in i) egg contents, ii) embryos, and iii) chorio-allantoic membranes (CAMs) known as the embryonic annexe, important for nutrient transfer, gas exchange, and metabolite storage (Cobb and Wood, 1997). The influence of the following factors on OC accumulation was considered: i) sampling site, ii) developmental stage of egg contents, iii) depth of the nest chamber, and iv) biological matrix.
2 Materials and methods
2.1 Study area and sample collection
The study area (i.e., Tuscan coast) was situated along the North Tyrrhenian Sea where C. caretta nested, and it was located within the Tuscan Archipelago and inside the Pelagos Marine Mammal Sanctuary in the Mediterranean Sea (Notarbartolo-di-Sciara et al., 2008).
The data analyzed refer to the C. caretta laying season of 2019 (June–October). From the first to last nesting event, the four nest sites were located as follows: the two northernmost nests in Marina di Cecina beach (Cecina, LI) (43.297878 N, 10.492796 E) and Rimigliano beach (San Vincenzo, LI) (43.025700 N, 10.523845 E) and the two southernmost nests in Le Marze beach (Marina di Grosseto, GR) (42.747063 N, 10.929739 E) and Riva del Sole beach (Castiglione della Pescaia, GR) (42.768852 N, 10.848411 E), named, respectively, NEST 1, NEST 2, NEST 3, and NEST 4 (Figure 1). First of all, NEST 1 was laid in the night between June 24 and 25, followed by NEST 2 in the night between July 8 and 9, NEST 3 in the night between July 17 and 18, and NEST 4 in the night between July 30 and 31.
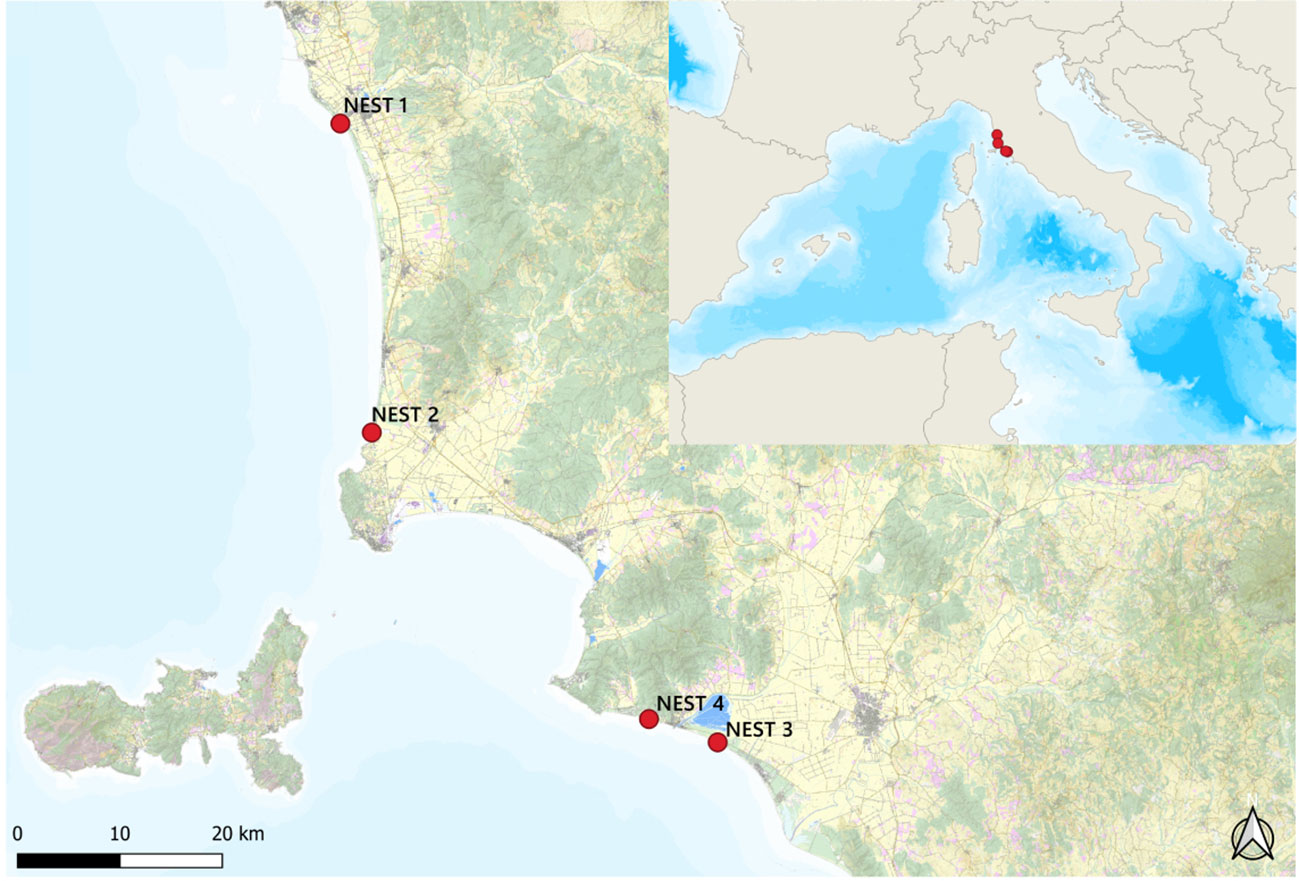
Figure 1 The four nesting sites of 2019. Nests are reported in order of egg deposition events, from the north to the south: Marina di Cecina (NEST 1), Rimigliano (NEST 2), Riva del Sole (NEST 4), and Le Marze (NEST 3). Map made by QGIS program 3.28.0 version.
Loggerhead sea turtle nesting events were reported to local authorities such as the Coast Guard or ARPAT (Regional Agency for Environmental Protection Tuscany) by tourists, scientists, stakeholders, or bathers that either have sighted and filmed directly sea turtle females laying eggs or have found traces on the beach. Once the eggs’ presence in the sighted nests was confirmed, the site was protected by a protection gate. Nests were monitored from the first hours of egg laying until the hatching, and each nest had a different nest incubation period as prescribed: NEST 1 = 74 days, NEST 2 = 57 days, NEST 3 = 60 days, NEST 4 = 58 days. Before excavation, at least 72 hours after the last hatchling emergence must be waited following standard procedures described in Miller et al. (2003) and Miller (1999). Excavation for sample collection was conducted for each nest on the following dates: September 8 for NEST 1, August 31 for NEST 2, September 21 for NEST 3, and October 10 for NEST 4.
At that time, the site was checked for any hatchlings that were stuck in the sand during the emergence. Gloves were used to excavate the nest chamber to avoid any contamination. Unhatched eggs including pipped (embryos not completely developed), eggshells of hatched eggs, dead hatchlings, and sand were collected. The maximum chamber depth and chamber floor width were also measured. The total number of eggs (hatched and unhatched) was recorded, and the diameter of each was measured using a stainless steel Vernier caliper. The samples were placed in a wide-neck plastic jar but wrapped in aluminum foil, thus isolated from the plastic walls of the jar itself; each sample was marked with the identification code of each nest. Samples were placed in isolated freezer bags (4 °C) to avoid decomposition and transferred to the laboratory. Samples described in Table 1 were divided among different research groups for several investigations such as morphological, parasitological, genetic, and ecotoxicological studies, thanks to the collaboration with ARPAT and IZSLT (Istituto Zooprofilattico Sperimentale delle Regioni Lazio e Toscana).

Table 1 Samples collected from the Tuscan loggerhead sea turtle nests laid along the Tuscan coast during summer of 2019.
In the laboratory, samples were stored at −20 °C. Following an overnight thawing in a cold room (4 °C), the eggs were carefully rinsed with water, and a small brush was used to remove sand particles; eggs were subsequently weighed, measured, and separated into eggshell and egg content (yolk/embryo and albumen). Eggs retaining the entire, intact eggshell were selected to isolate the CAM (only five eggs from NEST 4 met this requirement) by a dissection technique conducted using appropriate scissors, scalpels, and tweezers. For this purpose, each egg was set in a Petri dish under a stereo microscope and opened by tapping the eggshell. The embryo tissues appeared very degenerated in all samples. However, the CAM, adhering to the testaceous membrane, was isolated and rinsed with phosphate-buffered saline (PBS) at pH 6.8 in a volume of 7–15 mL. Small fragments of the membrane were observed under a light microscope (Motic Panthera U Trino) to ascertain the presence of remnants of blood vessels as a marker of the embryonic annex. It was not possible to analyze the chorio-allantoic membrane of pipped at the 27th and 28th stages because when using these samples (egg content + embryo) for other investigations, it was not possible to isolate the CAM with accuracy. For toxicological analyses, the following samples were selected: 13 random eggs from each nest (NEST 1, NEST 2, NEST 3, and NEST 4), five embryos and five eggs at the 27th and 28th developmental stages in NEST 1, 10 eggs with different depths in NEST 3, nine eggs with different mass (g) in NEST 2, and five CAMs isolated from five eggs sampled from NEST 4.
2.2 Environmental parameter analyses
The environmental parameters monitored during nest monitoring and post-hatching included the continuous tracking of temperature throughout the egg incubation phase and the granulometric analysis of the sampled sand (Table 2).
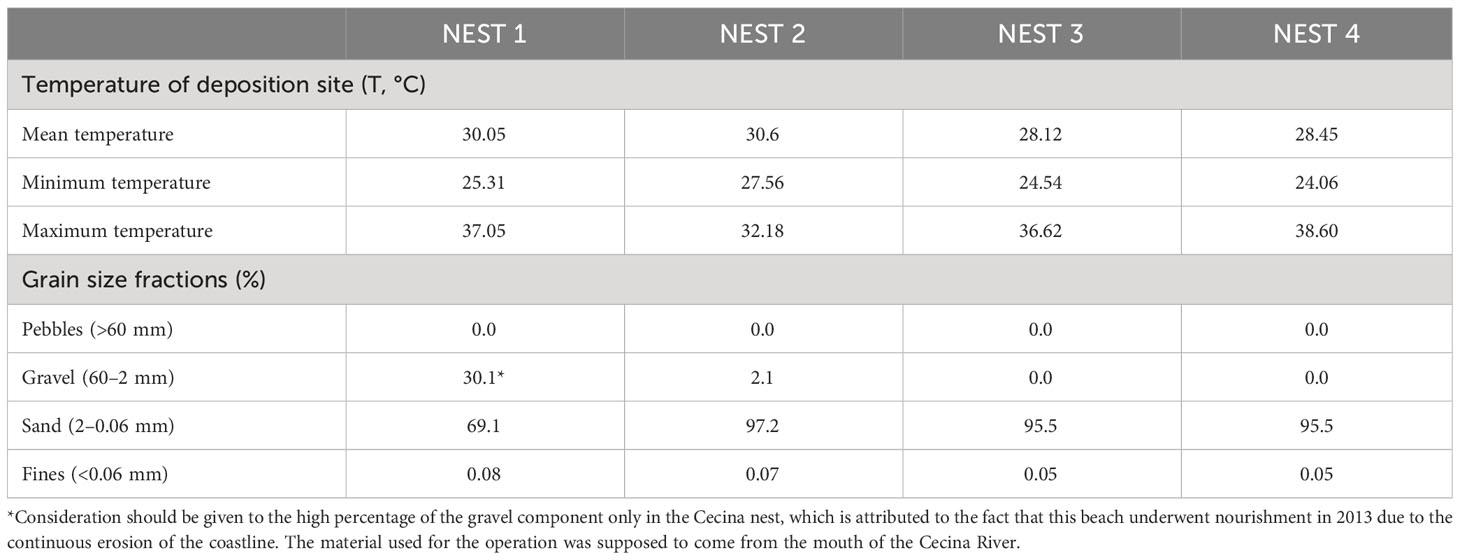
Table 2 Environmental parameters such as sand temperature of deposition site during entire incubation period and granulometry features of sand sampled from the deposition site.
For temperature monitoring, data loggers were deployed, one fixed at a depth of 50 cm and one mobile, closer to the surface, at 30 cm, checked every 15 days. These two instruments were used to control the temperature in the vicinity of the nesting chamber throughout the incubation period.
As for the analyses conducted on sediments, the grain size of the sand from the nesting site was assessed. To separate the different grain size fractions present in the sample, a sieving analysis was performed using standard sieves. The analysis of sandy or gravelly granular materials, in which the quantity of clays and silts (passing) is negligible or non-existent, was achieved through a dry sieving operation. In this type of analysis, the sample used was dried in an oven at a temperature of 105 °C–110 °C. The dry and loose materials was poured from the top into a stack of sieves, each with decreasing mesh sizes from top to bottom and equipped with a bottom closing pan. The mechanical sieves underwent regular shaking, and the various fractions of the material were retained by the different sieves according to their particle size. Once the sieving was completed, the various retained grain size fractions from each sieve and the bottom pan were weighed.
2.3 Analytical methodology
Analyses for HCB, DDTs, and PCBs were performed according to the analytical method of U.S. Environmental Protection Agency (EPA) 8081/8082 with some modifications (Marsili and Focardi, 1996). Samples (egg contents, embryos, and CAMs) were lyophilized in an Edwards® freeze drier for 3 days, and approximately 1 g was finely shredded using sterile Swann-Morton® Disposable Scalpels. Specifically, aliquots of 1 g of egg content (E “Egg content”), 0.50 g of embryo (B “Body”), and 0.06 g of CAM were extracted using n-hexane for pesticide residue analysis (PESTINORM, VWR Chemicals, Radnor, PA, USA). VWR cellulose thimbles (i.d. 25 mm, e.d. 27 mm, length 100 mm) used for extraction of the samples were preheated for approximately 30 min to 110 °C and pre-extracted for 9 h in a Soxhlet apparatus with n-hexane in order to remove any organochlorine contamination. Each sample was spiked with a surrogate compound (2,4,6-trichlorobiphenyl—IUPAC number 30) prior to extraction; this compound was quantified, and its recovery was calculated. After 9-h extraction with n-hexane, samples were purified with sulfuric acid 95% (VWR Chemicals), and then the supernatant solution was purified using adsorption chromatography with Florisil (60-100 mesh, Merck, Darmstadt, Germany) and dried at 130 °C overnight. Decachlorobiphenyl (DecaPCB—IUPAC number 209) was used as an internal standard, which was added to each sample prior to the gas chromatography analysis.
High-resolution capillary gas chromatography analyses were performed using an Agilent 6890N series gas chromatograph equipped with a 63Ni Electron Capture Detector (ECD) and an automatic injector on-column. The column used was an SBP-5 bonded phase capillary column (30-m length, 0.25-mm internal diameter, and 25-μm film thickness). The carrier gas was nitrogen with a head pressure of 15.5 psi (splitting ratio 50/1). The scavenger gas was argon/methane (95/5) at 40 mL/min. The oven temperature was 100 °C for the first 10 min, after which it was increased to 280 °C at 5 °C/min. The injector and detector temperatures were 200 °C and 280 °C, respectively. A mixture of specific isomers was used to calibrate the system, evaluate recovery, and confirm the results. The standard injection was prepared with 50 ng/mL of HCB, 100 ng/mL of DDT (pp′DDT, pp′DDD, pp′DDE, op′DDD, and op′DDE), 200 ng/mL of op′DDT, 500 ng/mL of PCB 30, 125 ng/mL of PCB 209, and 2 μg/mL of Aroclor 1260. For the evaluation of the linearity in the instrumental response and the instrumental sensitivity, the following quantities of the standard were injected: 1 μL, 2 μL, and 4 μL. Capillary gas chromatography revealed 29 PCB congeners (IUPAC numbers 95, 99, 101, and 118—pentachlorobiphenyls; 128, 135, 138, 144, 146, 149, 151, 153, and 156—hexachlorobiphenyls; 170, 171, 172, 174, 177, 178, 180, 183, and 187—heptachlorobiphenyls; 194, 195, 196, 199, 201, and 202—octachlorobiphenyls; 206—nonachlorobiphenyls). Total PCBs (PCBs) were quantified as the sum of all congeners. Total DDTs (DDTs) were calculated as the sum of the isomers op′DDT, pp′DDT, op′DDD, pp′DDD, op′DDE, and pp′DDE. The limit of detection (LOD) was calculated by measuring replicates (n = 20) of blank samples, determining the mean value and standard deviation (SD), and calculating the LOD as the mean + 2 SD. The LOD for all compounds analyzed was 0.1 ng/kg (ppt).
The extracted organic material (EOM%; lipid content) was calculated gravimetrically after extraction with n-hexane. OC results, normalized on a lipid basis, were expressed in ng/g lipid weight (l.w.), unless otherwise specified.
2.4 Statistical analyses
Statistical analysis was carried out using RStudio software®. Data were checked for normality distribution using the Shapiro–Wilk test (Shapiro and Wilk, 1965). Both parametric and non-parametric tests were applied (Hedeker and Gibbons, 1997). Two parametric tests such as T-test groups devised by Gosset in 1908 and the Kruskal–Wallis (Friedman, 1937) test were conducted regarding PCBs and DDTs in the egg contents of four nests, investigating the differences between the two groups. Differences among groups were tested using the Mann–Whitney–Wilcoxon test (Wilcoxon, 1945; Mann and Whitney, 1947). The p-value was fixed for each test.
3 Results and discussion
3.1 OCs in egg contents from four nests
The three studied POPs (HCB, DDTs, and PCBs) were detected in all biological materials (egg contents, embryos, and CAMs), and all samples showed the same pattern of relative abundance for target contaminants: PCBs > DDTs ≫ HCB. This reflects the accumulation trend of these xenobiotics that was reported for Mediterranean loggerhead sea turtle’s adult specimens, with PCBs always having the highest levels, especially in the north-western part of the basin (Casini et al., 2018; Lazar et al., 2011; Storelli et al., 2007). Only six PCB congeners (IUPAC numbers 28, 52, 101, 138, 153, and 180) were detected in egg content collected from nests laid along the coasts of the Campania region (SW Italy) (Esposito et al., 2023).
The results of PCBs, DDTs, and HCB in the egg content of the four nests are shown in Table 3. The Shapiro–Wilk test revealed that PCBs and DDTs were distributed parametrically both considering all nests together and in individual nests (p > 0.05); however, this was not the case for HCB. The non-parametric Kruskal–Wallis test showed significant differences between nests for both PCBs and DDTs (p < 0.001), while HCB was not statistically different in the four nests. Non-parametric Kolmogorov–Smirnov test (p < 0.05) indicated that DDTs and PCBs were significantly different between NEST 1 and NEST 3 and between NEST 1 and NEST 4, while NEST 2 was different in comparison to NEST 4.
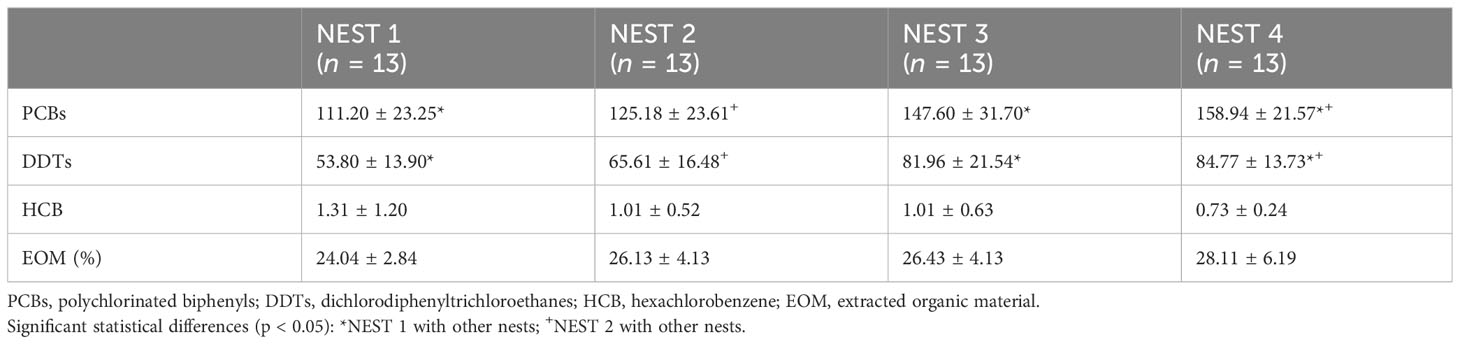
Table 3 Mean values ± standard deviation (SD) expressed in ng/g l.w. for PCB, DDT, and HCB levels and extracted organic matter investigated in 13 egg contents from Caretta caretta of NEST 1, NEST 2, NEST 3, and NEST 4 collected in summer 2019 in Tuscany (Italy).
Regarding fingerprints of the four nests, within each single, the differences among the 13 eggs were evaluated using the coefficient of variability (CV) calculated using the SD/arithmetic mean ratio for each compound. The results are reported in Supplementary Table 1; 10 compounds, specifically pp′DDE and PCBs 196, 187, 183, 170, 153, 149 + 118, 146, 138, and 101, showed a CV < 0.4 for all four nests; only PCB 177 had a CV > 0.6 in all nests, indicating high variability of this compound in hatching eggs. The others exhibited a different pattern among the nests. Indeed, NEST 1 had a greater number of compounds (10) with variability between high and very high in the 13 eggs of the clutch, while NEST 4 had the lowest variability (CV between low and medium variability). In general, in all nests, the eggs showed a low variability in their fingerprint: the percentage of compounds with low and medium variability ranged from 68% in NEST 1 to 90% in NEST 4.
Supplementary Table 2 shows the mean values (and SD) of PCB congener percentages and DDT metabolite percentages calculated on total PCBs and on total DDTs, respectively, found in the eggs of the four nests, compared with the Aroclor 1260. The non-parametric Kruskal–Wallis test showed significant differences (p < 0.05) among the four nests for most of the compounds detected, except for pp′DDT, PCB 201, PCB 199, PCB 195, PCB 180, PCB 177, PCB 156 + 171 + 202, PCB 151, PCB 144 + 135, and PCB 128. The statistical differences among the nests, evaluated using the Kolmogorov–Smirnov test (p < 0.05), were reported in Supplementary Table 2, marked with different symbols. In this case, too, it was noted that between NEST 1 and NEST 2 (southern Tuscany) and between NEST 3 and NEST 4 (northern Tuscany), there were really few differences: six compounds between NEST 1 and NEST 2 and three compounds between NEST 3 and NEST 4. This result was extremely interesting, as it showed a toxicological segmentation between the two areas. In fact, with the Kolmogorov–Smirnov test, most of the DDT metabolites and PCB congeners were significantly different (Supplementary Figures 1, 2).
The prevalent PCB congener percentage in all nests (Supplementary Table 2) was PCB 153, which ranged from 21.30% ± 4.30% in NEST 1 to 26.30% ± 3.18% in NEST 4, markedly higher than in Aroclor 1260 (9.39%). PCB 153 was the most abundant in all animals, probably because this congener is particularly persistent, as it has chlorines in positions 2, 4, and 5 of both rings of the biphenyl (Wolff et al., 1982; Bush et al., 1984; Safe et al., 1985) and no adjacent, unsubstituted carbons in the ortho–meta position (Clark and Krynitsky, 1980; Clark and Krynitsky, 1985). It is also crucial to toxicology since, among the OCs under investigation, PCB 153, with pp′DDE, PCB 118, and pp′DDT, is regarded as carcinogenic, mutagenic, and teratogenic organochlorine compounds (T-OCs), endocrine-disrupting chemicals (EDC-OCs), and immunosuppressant organochlorine compounds (IS-OCs) (Grattarola et al., 2023). Also, pp′DDE had the higher percentage among the DDT metabolites ranging between 60% in NEST 1 and 75% in NEST 3. For PCBs, elevated percentages were also reported by PCB 138 and PCB 180 congeners. These congeners, with PCB 153 and PCB 101 and the other two not evaluated in this study (PCB 28 and PCB 52), all non-dioxin-like PCBs (NDL-PCBs), are highly present in environmental matrices and animal tissues and also in abiotic substrates, and then, they are considered “PCBs indicators” by the European Food Safety Authority (EFSA European Food Safety, 2005; EFSA European Food Safety, 2010; Stenberg and Andersson, 2008; Elnar et al., 2012). Detectable PCB 101 was present in all samples but in very low percentages. These indicator PCBs were also among the compounds with the lowest variability among individual hatching eggs (Supplementary Table 2), demonstrating how they can be seen as powerful indicators in the present study.
The DDT/PCB relationship, shown in Table 4, determines which sector, agricultural or industrial, accounts for a large part of the total concentration of organochlorine compounds. Ratios less than 1 indicate an increased influence of industrial pollution or high population densities on organochlorine accumulation in samples. The ratios higher than 1 suggest that agriculture pollution would be crucial in determining the level of reached chlorinated hydrocarbons (Aguilar et al., 1999). In all samples analyzed, this ratio was much lower than 1, confirming a significant impact due to industrial contaminants for the sea turtles in this part of the Mediterranean basin (Marsili and Focardi, 1996; Santos-Neto et al., 2014). Regarding DDTs, a typical technical DDT is composed of pp′DDT (77.1%), the nearly inactive op′DDT (14.9%), pp′DDD (0.3%), op′DDD (0.1%), pp′DDE (4.0%), op′DDE (0.1%) and unidentified compounds (3.5%) (WHO Environmental Health Criteria 9, 1979), so the op′DDT+op′DDE+op′DDD)/DDT (∑op′DDT/DDT) ratio indicates whether the contamination comes from a classical pesticide or a technical DDT. Given that contamination from op′DDT is generally below 20%–30%, our results may suggest an agricultural type of contamination because the percentage of all op′DDT isomers occurred below 20%–30% (Nowell et al., 2019), although it is known that contamination can result from various sources (Thiombane et al., 2018).
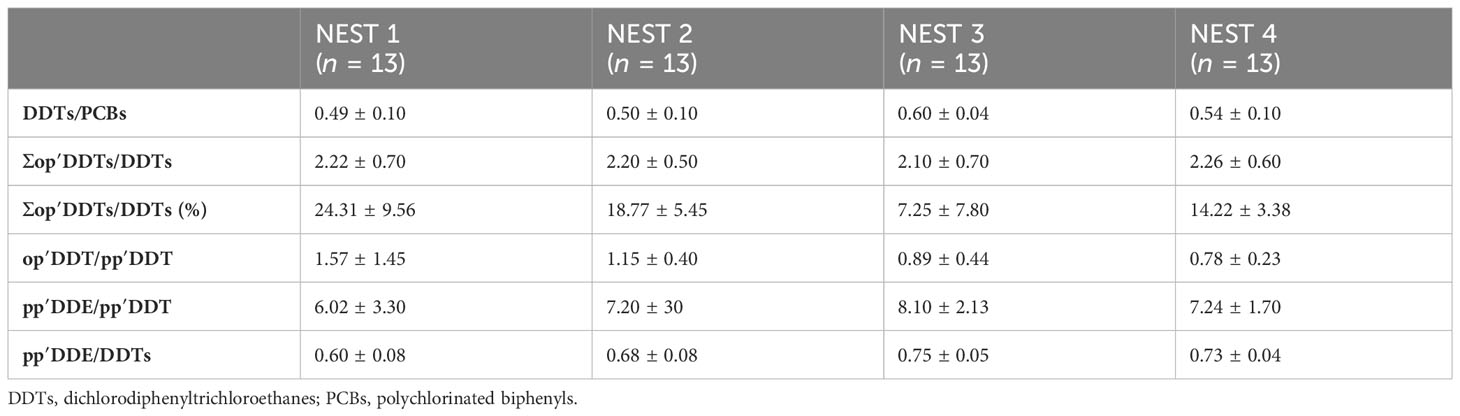
Table 4 Mean values ± standard deviation and ratio values (i.e., DDTs/PCBs, Σop′DDTs/DDTs, percentage of Σop′DDTs/DDTs, op′DDT/pp′DDT, pp′DDE/pp′DDT, and pp′DDE/DDTs) investigated in egg contents from Caretta caretta NEST 1, NEST 2, NEST 3, and NEST 4 collected in summer 2019 in Tuscany (Italy).
Additionally, a recent DDT release in the environment could be associated with the production of another pesticide, which was Dicofol, whose usage has only been recently regulated since 2019 listed in Annex A of the Stockholm Convention on Persistent Organic Pollutants (Regulation 2022/64). During Dicofol production, the conversion from op′DDT to op′dicofol was much less effective than the reaction that generates pp′dicofol from pp′DDT. For this reason, a typical Dicofol-based formulation had op′DDT/pp′DDT values greater than 1 (Qiu et al., 2005). Considering that in the environment o,p′ isomer degrades faster, the DDT “Dicofol type” can be established by ratio value op′DDT/pp′DDT > 0.25 (Qiu et al., 2005; Qiu and Zhu, 2010). In our samples, this ratio was much higher than 0.25; so even if from one side this could be related to a Dicofol type, which was banned only since 2019, from another side, this could be justified due to different types of metabolism and different partitions of contamination during oogenesis, which only further studies could support. DDE and DDD are also the main environmental metabolites and degradation products of DDT, with similar characteristics. The ratio pp′DDE/pp′DDT clearly indicates an old contamination (Walker et al., 2012), and in the technical DDT, this ratio was 0.05. A high ratio of pp′DDE to pp′DDT in the analyzed samples has been associated with a lack of exposure to newly applied DDT (Sereda et al., 2009; Wong et al., 2005), and therefore, there were recent deposits of insecticide into that ecosystem and also the degradation of most of the active substance (pp′DDT) to pp′DDE (Aguilar, 1984). In all samples analyzed, the pp′DDE/pp′DDT ratio had a value much larger than 0.05 (Table 4). pp′DDE/DDT ratio, having a similar meaning to the pp′DDE/pp′DDT ratio, can also indicate the efficiency of the metabolic processes (Borrell and Aguilar, 1987). In fact, the pp′DDE/DDT ratio indicates the relative abundance of metabolized forms of DDT, and it was used to estimate the time and intensity of DDT exposure, observing the efficiency of the species metabolism. Considering that pp′DDE levels increased over time as the animals continued to be exposed, a value >0.6 indicated that the contamination was not recent and that most of the pp′DDT had already been metabolized to pp′DDE in the past (Aguilar, 1984). Lastly, we could assume that the contamination of the samples was not due to a recent input of the insecticide into the ecosystem.
Unfortunately, a good comparison of these results with literature data from toxicological analyses conducted on C. caretta eggs was not permitted due to few available studies on this topic, different analytical methods, and different compositions of Aroclor (Table 5). Some studies that made comparisons revealed that despite their strict regulation for both PCBs (Cobb and Wood, 1997; Alava et al., 2011) and DDTs (Keller, 2013b; Esposito et al., 2023; Alava et al., 2011; Hillestad et al., 1974; Alam and Brim, 2000; Keller, 2013a; Keller et al., 2004a), there had been no decrease in these xenobiotics over the years mostly if we look at the results of the studies conducted in the Mediterranean basin. Esposito et al. (2023) carried out the only other investigation into the presence of organochlorines in eggs sampled from Italian nests. First of all, the results of this study showed that DDTs and HCB could be identified by instrumental gas chromatography, but the eggs examined in Campania showed that these contaminants were under the limit of detection, unfortunately without stating the limit. For PCBs, Esposito and colleagues (Esposito et al., 2023) examined only the six indicator congeners having values much lower than our findings (Table 5).
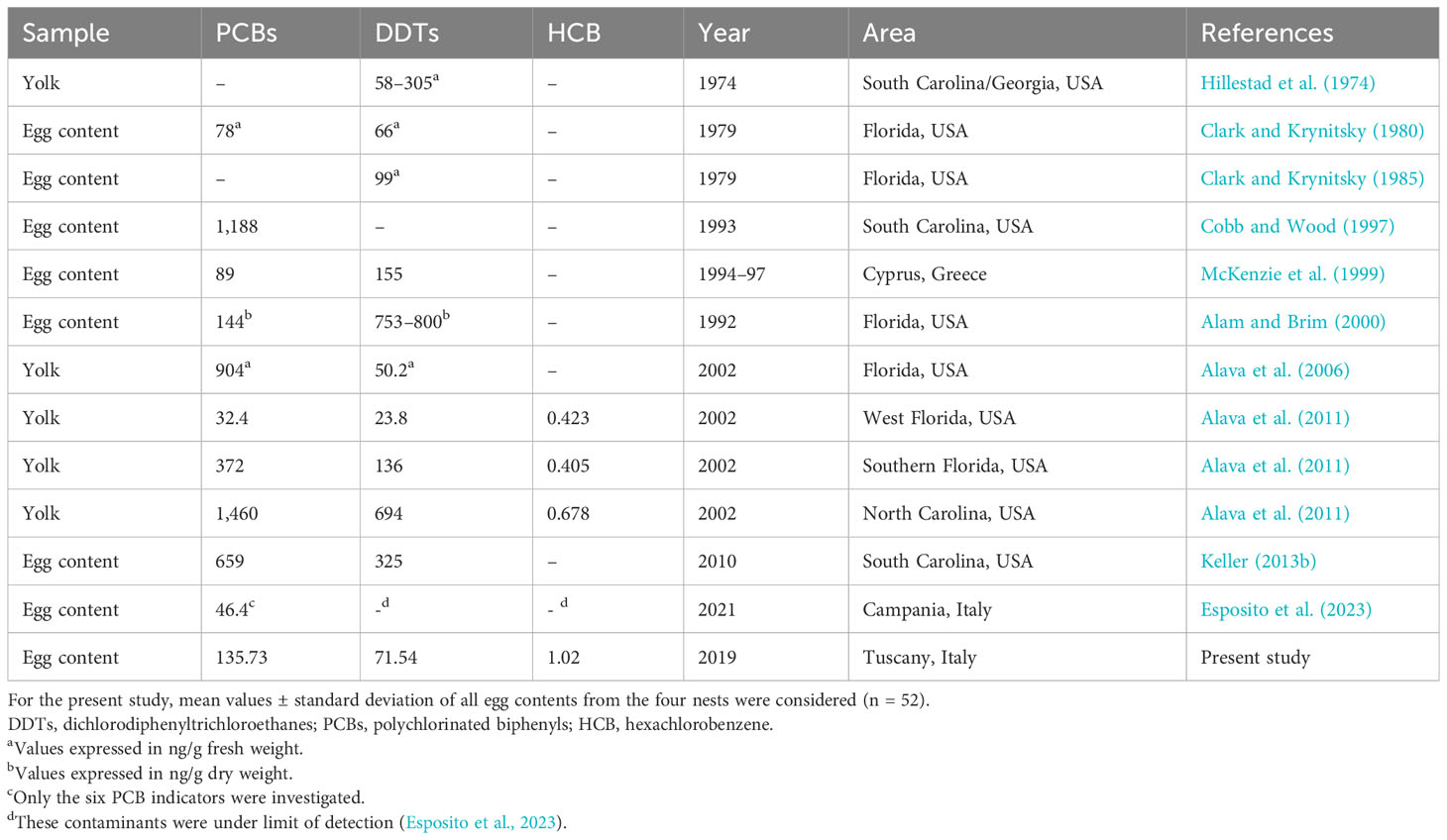
Table 5 Toxicological studies carried out on Caretta caretta eggs showing type of biological material, PCBs, DDTs, and HCB average values expressed in ng/g lipid weight unless otherwise specified, periods of sampling, study areas, and references.
Our results on HCB could only be compared with those of the study by Alava et al. (2011), who investigated this fungicide in loggerhead sea turtle eggs, where HCB was higher in our samples than in the yolks analyzed from eggs from three different nests on the east coast of the USA. Considering maternal transfer of persistent organic pollutants to sea turtle eggs, only a few studies have directly investigated health effects on developing embryos and newborn hatchlings in other turtle species such as green sea turtle and snapping turtles (Bishop et al., 1994; Van de Merwe et al., 2010; de Solla et al., 2007). However, the ability of females to transfer a substantial portion of their POPs to their offspring (Yordy et al., 2010) and the capacity of POPs, known as endocrine-disrupting contaminants, to impair the development of embryos have also been documented in reptiles, so they are also attributable to loggerhead sea turtle species (Guillette et al., 1995).
Another aspect to pay attention to is the comparison of OCs between biological material from a nest such as eggs, pipped or hatchlings, and tissues sampled from adult specimens. For instance, there is a study (Casini et al., 2018) conducted on the blood of adult specimens, some of which were sampled from the same nesting area of the present study, showing that contaminant levels were much higher (PCBs 590.54 ± 802.41 ng/g l.w., DDTs 173.11 ± 107.12 ng/g l.w., and HCB 9.04 ± 5.01 ng/g l.w.), compared to those found in the eggs of these investigations. This supports that the C. caretta, being a long-lived (up to 70–80 years) carnivorous reptile, is subject to bioaccumulation and biomagnification of persistent toxic compounds during its entire lifespan, leading these compounds to be much higher in adult specimens than in egg samples.
3.2 The influence of developmental stage on OC accumulation
Eggs collected from NEST 1 were analyzed of egg contents and embryos at two different developmental stages (Miller et al., 2017): 27 (embryo volume < yolk volume) and 28 (yolk volume = embryo volume) days with respect to the egg contents (including embryonic annexes). Samples from both developmental stages (n = 10 egg content for the 27th and 28th stages and n = 10 embryos for the 27th and 28th stages) showed that OC levels in the embryos were higher than in egg contents; this difference was also statistically significant at both the 27th and 28th stages as it was shown by a t-test with p < 0.05 for DDTs and PCBs. The PCBs’ relation between egg contents and embryos at the 27th and 28th stages was ≃1.6/1.7, whereas the relation of DDTs was ≃2 (Figure 2). The differences between egg contents and embryos were due to the lipophilicity of the two POPs. Indeed, it was demonstrated that the rate of diffusion between tissues and blood flow was specific and depended on the lipophilic properties of the compound: substances with higher lipophilicity were released more slowly from adipose tissue during lipid mobilization and were reabsorbed in greater amounts from lipid-rich tissues (Yordy et al., 2010; Jackson et al., 2017). Furthermore, considering that the Kow values of PCBs and DDTs were, respectively, 7.0–7.6 and 6.0–6.51, this could explain the faster release of DDTs from egg contents into the embryos (Levitt, 2010). However, additional molecular descriptors such as the number of polar groups may further influence the extent of diffusion limitation for individual compounds (Zhao et al., 2002). All DDT metabolites were more abundant in the embryos than in the egg contents at both the 27th and 28th stages, apart from the pp′DDE metabolite, which was statistically more abundant in the egg contents than in the embryo. Although the pp′DDE metabolite increased over time (O’Shea et al., 1980), a study conducted in rats has shown that the transplacental transfer of pp′DDE was slower to be present at concentrations of two to three orders of magnitude in the maternal tissues rather than fetal ones (You et al., 1999). Although the tissues of sea turtles differed from those of mammals, we could hypothesize that this metabolite had similar behavior in the reptiles because the egg portion was a tissue derived from maternal contribution, as was the placenta in mammals. In the literature, there were no specific toxicological studies that focused on evaluating OCs on embryos at different developmental stages. The only information that could be compared came from a study by Alava et al. (2006), in which C. caretta eggs were analyzed at different developmental stages and confirmed a significant increase in contaminant concentrations as embryonic development progresses (Van de Merwe et al., 2010).
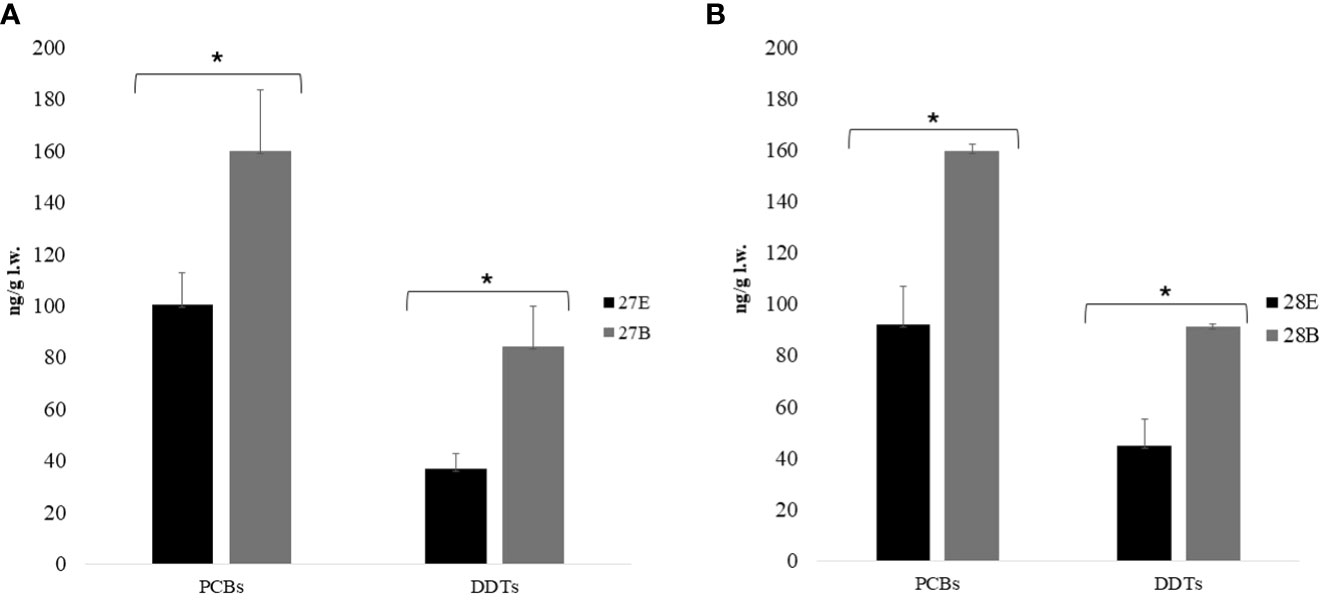
Figure 2 Distribution of mean values and standard deviation (ng/g l.w.) of PCBs and DDTs quantified in egg content and embryo at 27th (A) and 28th (B) development stages (n = 5 for each sample for both stages). At stage 27, PCBs’ mean values ± standard deviation for egg contents was 100.42 ± 12.56 ng/g l.w., while for embryos, it was 160.05 ± 23.52 ng/g l.w., and at stage 27, DDTs’ mean values ± standard deviation for egg contents was 37.03 ± 5.62 ng/g l.w., and for embryos, it was 84.49 ± 15.44 ng/g l.w. At stage 28, PCBs’ mean values ± standard deviation for egg contents was 92.28 ± 14.87 ng/g l.w. for egg contents, whereas for embryos, it was 159.92 ± 2.45 ng/g l.w., and at stage 28, DDTs’ mean values ± standard deviation for egg contents was 44.93 ± 10.14 ng/g l.w., while for embryos, it was 91.43 ± 0.91 ng/g l.w. The symbol * means statistical differences occurred. PCBs, polychlorinated biphenyls; DDTs, dichlorodiphenyltrichloroethanes; E, Egg content; B, Body.
3.3 Contamination of eggs with different mass
The eggs collected from NEST 2 nest allowed us to highlight a difference in contamination based on the mass of the egg, which was divided into four groups with the following average mass: 14.5 g (n = 2), 22.54 g (n = 2), 27.05 g (n = 3), and 32.2 g (n = 2 eggs). The analyses showed the same pattern for all groups of egg contaminants: PCBs > DDTs ≫ HCB. In the separate groups by mass, there were no significant differences for PCBs, DDTs, or HCB levels, so their amount could not be correlated with the increase in mass. These results were in contrast with those of two studies on leatherback turtle eggs that found a decrease in PCB and DDT concentrations with decreasing egg mass (Guirlet et al., 2010; Stewart et al., 2011). The low number of samples in our study (two to three eggs for each group) did not allow us to better investigate this parameter.
3.4 Contamination of eggs laid at different depths
During the excavation of NEST 3, it was possible to identify groups of eggs laid at different depths thanks to the good state of preservation of the laying chamber. Groups of eggs at different depths were selected for toxicological tests on the eggs found at depths of 24 cm (n = 2), 27 cm (n = 3), 32 cm (n = 3), and 38 cm (n = 2). In a study conducted on Chelydra serpentina, POP concentrations were found to be higher in eggs laid first (deeper) than in eggs laid last, although the differences were not statistically significant (Bishop et al., 1994). In the present study, PCBs, DDTs, and HCB levels were not statistically different between eggs laid at different depths, with the only exception that HCB in eggs at 24-cm depth occurred at higher levels than those at 38-cm depth.
3.5 OC accumulation between egg contents and chorio-allantoic membranes
CAM was another “egg” component that could accumulate high levels of POPs, as previously studied in birds and other reptiles (Cobb and Wood, 1997; Bargar et al., 1999; Bargar et al., 2003; Pastor et al., 1996). The membrane remained attached to the shell of the hatched egg, providing an easily retrievable sample from which we could obtain information on the differential contamination status in other compartments of the same egg (Keller, 2013b). However, in the present study, the CAM was isolated in five of the total 13 unhatched eggs from NEST 4. In both CAM and egg content, contaminant levels followed the same ranking: PCBs > DDTs ≫ HCB. PCBs, DDTs, and HCB were higher in CAMs than in egg contents from which they were isolated. The percentage partitioning between the two classes of PCB and DDT contaminants did not differ significantly between membranes and egg contents. In CAM, PCBs were 56.75% ± 6.0% and DDTs were 42.47% ± 6.1%, while in egg contents, PCBs were 65.91% ± 2.3% and DDTs were 32.28% ± 1.8%. The value of HCB was also higher in CAMs (0.78% ± 0.14%) than in egg content (0.34% ± 0.08%) as well as for other contaminants, but this difference was not significant. The embryo tissues, appearing highly degenerated, did not allow assigning a certain stage of development. However, the lack of significant differences in organic contaminants between CAM and egg content could be compatible with an early embryonic development stage. The only two studies conducted on the CAM of C. caretta and Alligator mississippiensis showed that the highest contaminant concentrations were pentachlorobiphenyls (congeners with IUPAC numbers 82–127) and hexachlorobiphenyls (congeners with IUPAC numbers 128–169), respectively (Cobb and Wood, 1997; Bargar et al., 1999). The studies on the CAMs were still preliminary, but these first results have highlighted the importance of this assessment in studying the role of this embryonic annex in the transfer of contaminants to the embryo, especially due to the CAM’s role in respiration, nutrient transport, and waste storage during embryonic development (Cobb and Wood, 1997). In particular, the role of waste storage should be further explored, as it may provide a mechanism for the removal of POPs from the developing embryo.
Our results showed statistical differences not only between two pairs of nests (NEST 1–NEST 2 vs. NEST 4–NEST 3) but also within the same nest, as in NEST 1, which had significant differences in contaminant values between the egg contents and the embryos. Considering that the two northernmost nests differ from the two southernmost ones, it is possible to hypothesize that the eggs from the two nests could have been laid by the same mother. This was confirmed by the genetic analyses conducted by IZSLT, revealing two different mitochondrial haplotypes: one was CC-A2.1, which was predominantly a Mediterranean strain but also Atlantic (most common strain), and another was CC-A3.1, which was a newly identified strain (Tolve et al., 2024).
In light of this, egg incubation, which lasts between 42 and 91 days, embryonic development, decomposition, bacterial metabolism, and desiccation, as well as exposure to sand, tidal water intrusion, or disturbance, were all factors that could potentially alter the contaminant levels originally contained in the egg. According to our findings, there was no evidence of a direct correlation of contaminants levels between egg contents and hatchlings’ success. Furthermore, despite global efforts to reduce or prohibit the production and use of POPs, these harmful substances persisted at significant levels in the biological samples of the loggerhead sea turtles under study. This ongoing presence continued to adversely affect organisms, causing harmful effects in both maternal and offspring tissues. For example, some DDT isomers and PCB congeners may still have harmful effects with estrogenic and anti-androgenic capacities (i.e., pp′DDT, op′DDT, pp′DDE, op′DDE, and PCB IUPAC numbers 95, 99, 101, and 153) as well as hazards that can affect both female and male reproductive processes (Fossi and Marsili, 2003). This reality induces scientists to reach the hazard levels for this species that led to the assessment of the toxicological risk for this marine organism, as it has already been evaluated for the Stenella coeruleoalba in the Mediterranean subpopulation (Marsili et al., 2004). Overall, this study shows that C. caretta can be used as a bioindicator for the Marine Strategy Framework Directive’s (MSFD, 2008/56/CE) Descriptor 1 on biodiversity and for Descriptor 10 on marine litter and may also be appropriate as a bioindicator for pollutants for Descriptor 8 on contaminants.
Data availability statement
The raw data supporting the conclusions of this article will be made available by the authors, without undue reservation.
Ethics statement
The animal study was approved by CITES IT 025 IS CITES IT 007 (Accademia dei Fisiocritici Onlus Prot. n. 161/2019). The study was conducted in accordance with the local legislation and institutional requirements.
Author contributions
ICe: Writing – original draft, Visualization, Resources, Investigation, Formal analysis, Conceptualization. FCap: Writing – review & editing, Visualization. LMi: Writing – review & editing, Visualization. GC: Writing – review & editing, Visualization. CA: Writing – review & editing, Visualization. MZ: Writing – review & editing, Visualization, Investigation. GT: Writing – review & editing, Visualization, Investigation. CM: Writing – review & editing, Visualization, Investigation. AN: Writing – review & editing, Visualization. EF: Writing – review & editing, Visualization. GR: Writing – review & editing, Visualization. AP: Writing – review & editing, Visualization. FB: Writing – review & editing, Visualization. DM: Writing – review & editing, Visualization, Investigation. FCan: Writing – review & editing, Visualization. CC: Writing – review & editing, Visualization. LT: Writing – review & editing, Visualization. LV: Writing – review & editing, Visualization. SV: Writing – review & editing, Visualization. ICa: Writing – review & editing, Visualization. LMa: Writing – review & editing, Validation, Supervision, Project administration, Methodology, Funding acquisition, Formal analysis, Data curation, Conceptualization.
Funding
The author(s) declare financial support was received for the research, authorship, and/or publication of this article. This work was supported by the Tuscan Region within the NATura Network Toscana (NAT.NE.T) scientific convention for Directive 92/43/CEE Habitat subscribed between the Tuscan Region and three Tuscan universities (Florence, Pisa, and Siena) in May 2019, and the framework was approved with D.G.R. n.592 of 05/06/2019.
Acknowledgments
We are deeply indebted to all the individuals who contributed to this study and to the volunteers who assisted the working group during the nesting events. We thank Parco della Maremma for their fundamental contribution to the monitoring of the nesting sites and the presence of the loggerhead turtle on the Tuscan coasts. Thanks to Accademia dei Fisiocritici Onlus for supporting in fieldwork with all necessary permits (National ident. code CITES IT 025 IS and International ident. Cod. CITES IT 007). Many thanks to Mr. Tommaso Capanni, who created the graphical abstract. Thanks to all the staff of the Tuscan Region for supporting the scientific team in all tasks conducted.
Conflict of interest
The authors declare that the research was conducted in the absence of any commercial or financial relationships that could be construed as a potential conflict of interest.
The author(s) declared that they were an editorial board member of Frontiers, at the time of submission. This had no impact on the peer review process and the final decision.
Publisher’s note
All claims expressed in this article are solely those of the authors and do not necessarily represent those of their affiliated organizations, or those of the publisher, the editors and the reviewers. Any product that may be evaluated in this article, or claim that may be made by its manufacturer, is not guaranteed or endorsed by the publisher.
Supplementary material
The Supplementary Material for this article can be found online at: https://www.frontiersin.org/articles/10.3389/fmars.2024.1327137/full#supplementary-material
References
Aguilar A. (1984). Relationship of DDE/ΣDDT in marine mammals to the chronology of DDT input into the ecosystem. Can. J. Fish. Aquat. Sci. 41, 840–844. doi: 10.1139/f84-100
Aguilar A., Borrell A., Pastor T. (1999). Biological factors affecting variability of persistent pollutant levels in cetaceans. J. Cetacean Res. Manage., 83–116. doi: 10.47536/jcrm.v1i1
Alam S. K., Brim M. S. (2000). Organochlorine, PCB, PAH, and metal concentrations in eggs of loggerhead sea turtles (Caretta caretta) from northwest Florida, USA. J. Environ. Sci. Health B. 35, 705–724. doi: 10.1080/03601230009373303
Alava J. J., Keller J. M., Kucklick J. R., Wyneken J., Crowder L., Scott G. I. (2006). Loggerhead Sea turtle (Caretta caretta) egg yolk concentrations of persistent organic pollutants and lipid increase during the last stage of embryonic development. Sci. Total Environ. 367, 170–181. doi: 10.1016/j.scitotenv.2006.02.029
Alava J. J., Keller J. M., Wyneken J., Crowder L., Scott G., Kucklick J. R. (2011). Geographical variation of persistent organic pollutants in eggs of threatened loggerhead sea turtles (Caretta caretta) from southeastern United States. Environ. Toxicol. Chem. 30, 1677–1688. doi: 10.1002/etc.553
Bargar T. A., Scott G. I., Cobb G. P. (2003). Chorio-allantoic membranes indicate avian exposure and biomarker responses to environmental contaminants: a laboratory study with white leghorn chickens (Gallus domesticus). Environ. Sci. Technol. 37, 256–260. doi: 10.1021/es0257873
Bargar T. A., Sills-McMurry C., Dickerson R. L., Rhodes W. E., Cobb G. P. (1999). Relative distribution of polychlorinated biphenyls among tissues of neonatal American alligators (Alligator mississippians). Arch. Environ. Contam. Toxicol. 37, 364–368. doi: 10.1007/s002449900526
Bianchi L., Casini S., Vantaggiato L., Di Noi A., Carleo A., Shaba E., et al. (2022). A novel ex vivo approach based on proteomics and biomarkers to evaluate the effects of chrysene, MEHP, and PBDE-47 on loggerhead sea turtles (Caretta caretta). Int. J. Environ. Res. Public Health 19, 4369. doi: 10.3390/ijerph19074369
Bishop C. A., Brown G. P., Brooks R. J., Lean D. R. S., Carey J. H. (1994). Organochlorine contaminant concentrations in eggs and their relationship to body size, and clutch characteristics of the female common snapping turtle (Chelydra serpentina serpentina) in Lake Ontario, Canada. Arch. Environ. Contam. Technol. 27, 82–87. doi: 10.1007/BF00203891
Borrell A., Aguilar A. (1987). Variations in DDE percentage correlated with total DDT burden in the blubber of fin and sei whales. Mar. pollut. Bull. 18, 70–74. doi: 10.1016/0025-326X(87)90570-4
Bucchia M., Camacho M., Santos M. R., Boada L. D., Roncada P., Mateo R., et al. (2015). Plasma levels of pollutants are much higher in loggerhead turtle populations from the Adriatic Sea than in those from open waters (Eastern Atlantic Ocean). Sci. Total Environ. 523, 161–169. doi: 10.1016/j.scitotenv.2015.03.047
Bush B., Snow J., Koblintz R. (1984). Polychlorobiphenyl (PCB) Congeners,p,P′-DDE, and hexachlorobenzene in maternal and 1210 fetal cord blood from mothers in upstate New York. Arch. Environ. Contam. Toxicol. 13, 517–527, 1211. doi: 10.1007/BF01056331
Caliani I., Campani T., Giannetti M., Marsili L., Casini S., Fossi M. C. (2014). First application of comet assay in blood cells of Mediterranean loggerhead sea turtle (Caretta caretta). Mar. Environ. Res. 96, 68–72. doi: 10.1016/j.marenvres.2013.09.008
Caliani I., Poggioni L., D’Agostino A., Fossi M. C., Casini S. (2019). An immune response-based approach to evaluate physiological stress in rehabilitating loggerhead sea turtle. Vet. Immunol. Immunopathol. 207, 18–24. doi: 10.1016/j.vetimm.2018.11.013
Camacho M., Luzardo O. P., Boada L. D., Jurado L. F. L., Medina M., Zumbado M., et al. (2013). Potential adverse health effects of persistent organic pollutants on sea turtles: evidence from a cross-sectional study on Cape Verde loggerhead sea turtles. Sci. Total Environ. 458, 283–289. doi: 10.1016/j.scitotenv.2013.04.043
Camacho M., Orós J., Henríquez-Hernández L. A., Valerón P. F., Boada L. D., Zaccaroni A., et al. (2014). Influence of the rehabilitation of injured loggerhead turtles (Caretta caretta) on their blood levels of environmental organic pollutants and elements. Sci. Total Environ. 487, 436–442. doi: 10.1016/j.scitotenv.2014.04.062
Campani T., Baini M., Giannetti M., Cancelli F., Mancusi C., Serena F., et al. (2013). Presence of plastic debris in loggerhead turtle stranded along the Tuscany coasts of the Pelagos Sanctuary for Mediterranean Marine Mammals (Italy). Mar. pollut. Bull. 74, 225–230. doi: 10.1016/j.marpolbul.2013.06.053
Casale P. (2015). Caretta caretta (Mediterranean subpopulation). IUCN Red List Threatened Species 2015, e.T83644804A83646294. doi: 10.2305/IUCN.UK.2015-4.RLTS.T83644804A83646294.en
Casale P., Broderick A. C., Camiñas J. A., Cardona L., Carreras C., Demetropoulos A., et al. (2018). Mediterranean Sea turtles: current knowledge and priorities for conservation and research. Endanger. Species Res. 36, 229–267. doi: 10.3354/esr00901
Casale P., Tucker A. D. (2017). Caretta caretta, loggerhead turtle. IUCN Red List Threatened Species 2017, e.T3897A119333622. doi: 10.2305/IUCN
Casini S., Caliani I., Giannetti M., Marsili L., Maltese S., Coppola D., et al. (2018). First ecotoxicological assessment of Caretta caretta (Linnaeus, 1758) in the Mediterranean Sea using an integrated nondestructive protocol. Sci. Total Environ. 631, 1221–1233. doi: 10.1016/j.scitotenv.2018.03.111
Clabough E. B., Kaplan E., Hermeyer D., Zimmerman T., Chamberlin J., Wantman S. (2022). The secret life of baby turtles: A novel system to predict hatchling emergence, detect infertile nests, and remotely monitor sea turtle nest events. PloS One 17 (10), e0275088. doi: 10.1371/journal.pone.0275088
Clark D. R. Jr., Krynitsky A. J. (1980). Organochlorine residues in eggs of loggerhead and green sea turtles nesting at Merritt Island, Florida–July and August 1976. Pesticides Monit. J. 14, 7–10.
Clark D. R., Krynitsky A. J. (1985). DDE residues and artificial incubation of loggerhead sea turtle eggs. Bull. Environ. Contam. Toxicol. 34, 121–125. doi: 10.1007/BF01609712
Cobb G. P., Wood P. D. (1997). PCB concentrations in eggs and chorio-allantoic membranes of loggerhead sea turtles (Caretta caretta) from the Cape Romain National Wildlife Refuge. Chemosphere 34, 539–549. doi: 10.1016/S0045-6535(96)00386-4
Cocci P., Mosconi G., Bracchetti L., Nalocca J. M., Frapiccini E., Marini M., et al. (2018). Investigating the potential impact of polycyclic aromatic hydrocarbons (PAHs) and polychlorinated biphenyls (PCBs) on gene biomarker expression and global DNA methylation in loggerhead sea turtles (Caretta caretta) from the Adriatic Sea. Sci. Total Environ. 619, 49–57. doi: 10.1016/j.scitotenv.2017.11.118
Crosti R., Arcangeli A., Campagnuolo S., Castriota L., Falautano M., Maggio T., et al. (2020). Assessing worth of marine protected areas for the protection of threatened biodiversity using IUCN Red List and Red List Index. A pilot study in six Mediterranean areas. Ecol. Indicators 119, 106765. doi: 10.1016/j.ecolind.2020.106765
de Solla S. R., Fernie K. J., Letcher R. J., Chu S. G., Drouillard K. G., Shahmiri S. (2007). Snapping turtles (Chelydra serpentina) as bioindicators in Canadian areas of concern in the Great Lakes Basin. 1. Polybrominated diphenyl ethers, polychlorinated biphenyls, and organochlorine pesticides in eggs. Environ. Sci. Technol. 41, 7252–7259. doi: 10.1021/es0710205
D’Ilio S., Mattei D., Blasi M. F., Alimonti A., Bogialli S. (2011). The occurrence of chemical elements and POPs in loggerhead turtles (Caretta caretta): an overview. Mar. pollut. Bull. 62, 1606–1615. doi: 10.1016/j.marpolbul.2011.05.022
EFSA European Food Safety (2005). Opinion of the scientific committee on a request from EFSA related to A harmonised approach for risk assessment of substances which are both genotoxic and carcinogenic. EFSA J. 3, 282. doi: 10.2903/j.efsa.2005.282
EFSA European Food Safety (2010). Results of the monitoring of non-dioxin-like PCBs in food and feed. EFSA J. 8, 1701. doi: 10.2903/j.efsa.2010.1701
Elnar A. A., Diesel B., Desor F., Feidt C., Bouayed J., Kiemer A. K., et al. (2012). Neurodevelopmental and behavioral toxicity via lactational exposure to the sum of six indicator non-dioxin-like-polychlorinated biphenyls (å6 NDL-PCBs) in mice. Toxicology 299, 44–54. doi: 10.1016/j.tox.2012.05.004
Esposito M., Canzanella S., Iaccarino D., Pepe A., Di Nocera F., Bruno T., et al. (2023). Trace elements and persistent organic pollutants in unhatched loggerhead turtle eggs from an emerging nesting site along the southwestern coasts of Italy, Western Mediterranean Sea. Animals 13, 1075. doi: 10.3390/ani13061075
Fichi G., Cardeti G., Cersini A., Mancusi C., Guarducci M., Di Guardo G., et al. (2016). Bacterial and viral pathogens detected in sea turtles stranded along the coast of Tuscany, Italy. Vet. Microbiol. 185, 56–61. doi: 10.1016/j.vetmic.2016.02.003
Fossi M. C., Marsili L. (2003). Effects of endocrine disruptors in aquatic mammals. Pure Appl. Chem. 75, 2235–2247. doi: 10.1351/pac200375112235
Fossi M. C., Marsili L., Neri G., Natoli A., Politi E., Panigada S. (2003). The use of a non-lethal tool for evaluating toxicological hazard of organochlorine contaminants in Mediterranean cetaceans: new data 10 years after the first paper published in MPB. Mar. pollut. Bull. 46, 972–982. doi: 10.1016/S0025-326X(03)00113-9
Friedman M. (1937). The use of ranks to avoid the assumption of normality implicit in the analysis of variance. Am. Stat. Assoc. 32, 675–701. doi: 10.1080/01621459.1937.10503522
Giesy J. P., Solomon K. R., Mackay D., Anderson J. (2014). Evaluation of evidence that the organophosphorus insecticide chlorpyrifos is a potential persistent organic pollutant (POP) or persistent, bioaccumulative, and toxic (PBT). Environ. Sci. Eur. 26, 29. doi: 10.1186/s12302-014-0029-y
Grattarola C., Minoia L., Giorda F., Consales G., Capanni F., Ceciarini I., et al. (2023). Health status of stranded common bottlenose dolphins (Tursiops truncatus) and contamination by immunotoxic pollutants: A threat to the Pelagos Sanctuary—Western Mediterranean Sea. Diversity 15, 569. doi: 10.3390/d15040569
Guillette L. J. Jr., Crain D. A., Rooney A. A., Pickford D. B. (1995). Organization versus activation: the role of endocrine-disrupting contaminants (EDCs) during embryonic development in wildlife. Environ. Health Perspect. 103, 157–164. https://ehp.niehs.nih.gov/doi/10.1289/ehp.95103s7157.
Guirlet E., Das K., Thomé J. P., Girondot M. (2010). Maternal transfer of chlorinated contaminants in the leatherback turtles, Dermochelys coriacea, nesting in French Guiana. Chemosphere 79, 720–726. doi: 10.1016/j.chemosphere.2010.02.047
Hedeker D., Gibbons R. D. (1997). Application of random-effects pattern-mixture models for missing data in longitudinal studies. psychol. Methods 2, 64–78. doi: 10.1037/1082-989X.2.1.64
Hillestad H. O., Reimold R. J., Stickney R. R., Windom H. L., Jenkins J. H. (1974). Pesticides, heavy metals, and radionuclide uptake in loggerhead sea turtles from South Carolina and Georgia. Herpetol. Rev. 5, 75.
Hoffman D. J., Rattner B. A., Burton G. A. Jr., Cairns J. Jr. (Eds.) (2002). Handbook of ecotoxicology (Boca Raton (FL): CRC press). doi: 10.1201/9781420032505
IUCN (2018) The IUCN Red List of Threatened Species. Version 2018-2. Available online at: http://www.iucnredlist.org.
Jackson E., Shoemaker R., Larian N., Cassis L. (2017). Adipose tissue as a site of toxin accumulation. Compr. Physiol. 7, 1085. doi: 10.1002/cphy.c160038
Keller J. M. (2013a). “Exposure to and Effects of Persistent Organic Pollutants”. in The Biology of Sea Turtles, Volume III. Eds. Wyneken J., Lohmann K. J., Musick J. A. (Boca Roaton, FL: CRC Press), 285–328.
Keller J. M. (2013b). Forty-seven days of decay does not change persistent organic pollutant levels in loggerhead sea turtle eggs. Environ. Toxicol. Chem. 32, 747–756. doi: 10.1002/etc.2127
Keller J. M., Kucklick J. R., Harms C. A., McClellan-Green P. D. (2004a). Organochlorine contaminants in sea turtles: correlations between whole blood and fat. Environ. Toxicol. Chem. 23, 726–738. doi: 10.1897/03-254
Keller J. M., Kucklick J. R., Stamper M. A., Harms C. A., McClellan-Green P. D. (2004b). Associations between organochlorine contaminant concentrations and clinical health parameters in loggerhead sea turtles from North Carolina, USA. Environ. Health Perspect. 112, 1074–1079. doi: 10.1289/ehp.6923
Keller J. M., McClellan-Green P. D., Kucklick J. R., Keil D. E., Peden-Adams M. M. (2006). Effects of organochlorine contaminants on loggerhead sea turtle immunity: comparison of a correlative field study and in vitro exposure experiments. Environ. Health Perspect. 114, 70–76. doi: 10.1289/ehp.8143
Lallas P. L. (2001). The Stockholm Convention on persistent organic pollutants. Am. J. Int. Law. 95, 692–708. doi: 10.2307/2668517
Lazar B., Maslov L., Romanić S. H., Gračan R., Krauthacker B., Holcer D., et al. (2011). Accumulation of organochlorine contaminants in loggerhead sea turtles, Caretta caretta, from the eastern Adriatic Sea. Chemosphere 82, 121–129. doi: 10.1016/j.chemosphere.2010.09.015
Levitt D. G. (2010). Quantitative relationship between the octanol/water partition coefficient and the diffusion limitation of the exchange between adipose and blood. BMC Clin. Pharmacol. 10, 1. doi: 10.1186/1472-6904-10-1
Lucchetti A., Bargione G., Petetta A., Vasapollo C., Virgili M. (2019). Reducing Sea turtle bycatch in the Mediterranean mixed demersal fisheries. Front. Mar. Sci. 6, 387. doi: 10.3389/fmars.2019.00387
Mancusi C., Caruso C., Terracciano G., Raimondi G., Venturi L., Tonelli L., et al. (2023). Nesting of Caretta caretta in Tuscany area (North Western Mediterranean Sea): insights of a recent colonization phenomenon. J. Herpetol. in press.
Mann H. B., Whitney D. R. (1947). On a test of whether one of two random variables is stochastically larger than the other. Ann. Math. Stat 18 (1), 50–60. doi: 10.1214/aoms/1177730491
Margaritoulis D., Lourenço G., Riggall T. E., Rees A. F. (2022). Thirty-eight years of loggerhead turtle nesting in Laganas Bay, Zakynthos, Greece: A review. Chelonian Conserv. Biol. 21 (2), 143–157. doi: 10.2744/CCB-1531.1
Marsili L., D’Agostino A., Bucalossi D., Malatesta T., Fossi M. C. (2004). Theoretical models to evaluate hazard due to organochlorine compounds (OCs) in Mediterranean striped dolphin (Stenella coeruleoalba). Chemosphere 56, 791–801. doi: 10.1016/j.chemosphere.2004.03.014
Marsili L., Focardi S. (1996). Organochlorine levels in subcutaneous blubber biopsies of fin whales (Balaenoptera physalus) and striped dolphins (Stenella coeruleoalba) from the Mediterranean Sea. Environ. pollut. 91, 1–9. doi: 10.1016/0269-7491(95)00037-R
McKenzie C., Godley B. J., Furness R. W., Wells D. E. (1999). Concentrations and patterns of organochlorine contaminants in marine turtles from Mediterranean and Atlantic waters. Mar. Environ. Res. 47 (2), 117–135. doi: 10.1016/S0141-1136(98)00109-3
Miller J. D. (1999). “Determining hatch size and clutch success,” in Research and Management Techniques for the Conservation of Sea Turtles. Eds. Eckert K. L., Bjorndal K. A., AbreuGrobois F. A., Donnelly M. (IUCN/SSC Marine Turtle Specialist Group Publication, Washington, DC, USA), 124–129.
Miller J. D., Limpus C. J., Godfrey M. H. (2003). “Nest site selection, oviposition, eggs, development, hatching, and emergence of loggerhead turtles,” in Loggerhead Sea Turtles. Eds. Bolten A. B., Witherington B. E. (Smithsonian Press, Washington, DC), 125–143.
Miller J. D., Mortimer J. A., Limpus C. J. (2017). A field key to the developmental stages of marine turtles (Cheloniidae) with notes on the development of Dermochelys. Chelonian Conserv. Biol. 16, 111–122. doi: 10.2744/CCB-1261.1
Montes A. D. N., Reyes G. E., Ramírez R. F., Romero P. R. (2020). Persistent organic pollutants in Kemp’s Ridley Sea turtle Lepidochelys kempii in Playa Rancho Nuevo Sanctuary, Tamaulipas, Mexico. Sci. Total Environ. 739, 140176. doi: 10.1016/j.scitotenv.2020.140176
Multilateral Treaties Deposited with the Secretary-General. (2024). United Nations, New York. Available at: https://treaties.un.org/Pages/ViewDetails.aspx?src=TREATY&mtdsg_no=XXVII-15&chapter=27&clang=_en on [07-03-2024].
Muñoz C. C., Hendriks A. J., Ragas A. M., Vermeiren P. (2021). Internal and maternal distribution of persistent organic pollutants in sea turtle tissues: a meta-analysis. Environ. Sci. Technol. 55, 10012–10024. doi: 10.1021/acs.est.1c02845
Muñoz C. C., Vermeiren P. (2020). Maternal transfer of persistent organic pollutants to sea turtle eggs: A meta-analysis addressing knowledge and data gaps toward an improved synthesis of research outputs. Environ. Toxicol. Chem. 39, 9–29. doi: 10.1002/etc.4585
Notarbartolo-di-Sciara G., Agardy T., Hyrenbach D., Scovazzi T., Van Klaveren P. (2008). The Pelagos sanctuary for Mediterranean marine mammals. Aquat. Conserv.: Mar. Freshw. 18, 367–391. doi: 10.1002/aqc.855
Novillo O., Pertusa J. F., Tomás J. (2017). Exploring the presence of pollutants at sea: monitoring heavy metals and pesticides in loggerhead turtles (Caretta caretta) from the western Mediterranean. Sci. Total Environ. 598, 1130–1139. doi: 10.1016/j.scitotenv.2017.04.090
Nowell L. H., Capel P. D., Dileanis P. D. (2019). Pesticides in stream sediment and aquatic biota: distribution, trends, and governing factors (Boca Raton (FL): CRC Press). doi: 10.1201/9780429104435
O’Shea T. J., Brownell R. L. Jr., Clark D. R. Jr., Walker W. A., Gay M. L., Lamont T. G. (1980). Organochlorine pollutants in small cetaceans from the Pacific and south Atlantic Oceans, November 1968-June 1976. Pestic. Monit. J. 14, 35–46.
Pastor D., Albaigés J., Ruiz X., Jover L. (1996). The use of chorio-allantoic membranes as predictors of egg organochlorine burden. Environ. Toxicol. Chem. 15, 167–171. doi: 10.1002/etc.5620150215
Piroddi C., Colloca F., Tsikliras A. C. (2020). The living marine resources in the Mediterranean Sea large marine ecosystem. Environ. Dev. 36, 100555. doi: 10.1016/j.envdev.2020.100555
Qiu X., Zhu T. (2010). Using the o, p′-DDT/p, p′-DDT ratio to identify DDT sources in China. Chemosphere 81, 1033–1038. doi: 10.1016/j.chemosphere.2010.08.049
Qiu X., Zhu T., Yao B., Hu J., Hu S. (2005). Contribution of dicofol to the current DDT pollution in China. Environ. Sci. Technol. 39, 4385–4390. doi: 10.1021/es050342a
Regulation 2022/643. Commission Delegated Regulation (EU) 2022/643 of 10 February 2022 amending Regulation (EU) No 649/2012 of the European Parliament and of the Council as regards the listing of pesticides, industrial chemicals, persistent organic pollutants and mercury and an update of customs codes. Available at: https://eur-lex.europa.eu/eli/reg_del/2022/643/oj.
Roscales J. L., González-Solís J., Muñoz-Arnanz J., Jiménez B. (2011). Geographic and trophic patterns of OCs in pelagic seabirds from the NE Atlantic and the Mediterranean: a multi-species/multi-locality approach. Chemosphere 85, 432–440. doi: 10.1016/j.chemosphere.2011.07.070
Safe S., Bandiera S., Sawyer T., Zmudzka B., Mason G., Romkes M., et al. (1985). Effects of structure on binding to the 2,3,7,8-TCDD receptor protein and AHH induction–halogenated biphenyls. 1206 Environ. Health Perspect. 1985, 21–33. doi: 10.1289/ehp.856121
Santos-Neto E. B., Azevedo-Silva C. E., Bisi T. L., Santos J., Meirelles A. C. O., Carvalho V. L., et al. (2014). Organochlorine concentrations (PCBs, DDTs, HCHs, HCB and MIREX) in delphinids stranded at the northeastern Brazil. Sci. Total Environ. 472, 194–203. doi: 10.1016/j.scitotenv.2013.10.117
Savoca D., Arculeo M., Barreca S., Buscemi S., Caracappa S., Gentile A., et al. (2018). Chasing phthalates in tissues of marine turtles from the Mediterranean Sea. Mar. pollut. Bull. 127, 165–169. doi: 10.1016/j.marpolbul.2017.11.069
Savoca D., Arculeo M., Vecchioni L., Cambera I., Visconti G., Melfi R., et al. (2021). Can phthalates move into the eggs of the loggerhead sea turtle Caretta caretta? The case of the nests on the Linosa Island in the Mediterranean Sea. Mar. pollut. Bull. 168, 112395. doi: 10.1016/j.marpolbul.2021.112395
Sereda B., Bouwman H., Kylin H. (2009). ComparingWater, bovine milk, and indoor residual spraying as possible sources of DDT and pyrethroid residues in breast milk. J. Toxicol. Environ. Health Part A 72, 842–851. doi: 10.1080/15287390902800447
Shapiro S. S., Wilk M. B. (1965). An analysis of variance test for normality (complete samples). Biometrika 52, 591–611. doi: 10.1093/biomet/52.3-4.591
Stenberg M., Andersson P. L. (2008). Selection of non-dioxin-like PCBs for in vitro testing on the basis of environmental abundance and molecular structure. Chemosphere 71, 1909–1915. doi: 10.1016/j.chemosphere.2008.01.007
Stewart K. R., Keller J. M., Templeton R., Kucklick J. R., Johnson C. (2011). Monitoring persistent organic pollutants in leatherback turtles (Dermochelys coriacea) confirms maternal transfer. Mar. pollut. Bull. 62, 1396–1409. doi: 10.1016/j.marpolbul.2011.04.042
Storelli M. M., Barone G., Marcotrigiano G. O. (2007). Polychlorinated biphenyls and other chlorinated organic contaminants in the tissues of Mediterranean loggerhead turtle Caretta caretta. Sci. Total Environ. 373, 456–463. doi: 10.1016/j.scitotenv.2006.11.040
Storelli M. M., Zizzo N. (2014). Occurrence of organochlorine contaminants (PCBs, PCDDs and PCDFs) and pathologic findings in loggerhead sea turtles, Caretta caretta, from the Adriatic Sea (Mediterranean Sea). Sci. Total Environ. 472, 855–861. doi: 10.1016/j.scitotenv.2013.11.137
Thiombane M., Petrik A., Di Bonito M., Albanese S., Zuzolo D., Cicchella D., et al. (2018). Status, sources, and contamination levels of organochlorine pesticide residues in urban and agricultural areas: a preliminary review in central–southern Italian soils. Environ. Sci. pollut. Res. 25, 26361–26382. doi: 10.1007/s11356-018-2688-5
Tolve L., Iannucci A., Garofalo L., Ninni A., Capobianco Dandona A., Ceciarini I., et al. Whole mitochondrial genome sequencing provides new insights into the phylogeography of loggerhead turtles (Caretta caretta) in the Mediterranean Sea. Mar. Biol. unpublished results 171(19). doi: 10.1007/s00227-023-04325-x
Van de Merwe J. P., Hodge M., Whittier J. M., Ibrahim K., Lee S. Y. (2010). Persistent organic pollutants in the green sea turtle Chelonia mydas: Nesting population variation, maternal transfer, and effects on development. Mar. Ecol. Prog. Ser. 403, 269–278. doi: 10.3354/meps08462
Walker C. H., Sibly R. M., Hopkin S. P., Peakall D. B. (2012). Principles of ecotoxicology (Boca Raton (FL): CRC press).
Wallace B. P., DiMatteo A. D., Bolten A. B., Chaloupka M. Y., Hutchinson B. J., Abreu-Grobois F. A., et al. (2011). Global conservation priorities for marine turtles. PloS One 6, e24510. doi: 10.1371/journal.pone.0024510
Wallace B. P., DiMatteo A. D., Hurley B. J., Finkbeiner E. M., Bolten A. B., Chaloupka M. Y., et al. (2010). Regional management units for marine turtles: a novel framework for prioritizing conservation and research across multiple scales. PloS One 5, e15465. doi: 10.1371/journal.pone.0015465
WHO Environmental Health Criteria 9 (1979). DDT and Its Derivatives; Environmental health criteria (Geneva: Albany, N.Y: World Health Organization; 1079 obtainable from WHO Publications Centre USA).
Wilcoxon F. (1945). Some uses of statistics in plant pathology. Biometr. Bull. 1, 41–45. doi: 10.2307/3002011
Wolff M. S., Thornton J., Fischbein A., Lilis R., Selikoff I. J. (1982). Disposition of polychlorinated biphenyl congeners in 1208 occupationally exposed persons. Toxicol. Appl. Pharmacol. 62, 294–306. doi: 10.1016/0041-008X(82)90128-4
Wong M. H., Leung A. O. W., Chan J. K. Y., Choi M. P. K. (2005). A review on the usage of POP pesticides in China, with emphasis on DDT loadings in human milk. Chemosphere 60, 740–752. doi: 10.1016/j.chemosphere.2005.04.028
Yordy J. E., Wells R. S., Balmer B. C., Schwacke L. H., Rowles T. K., Kucklick J. R. (2010). Partitioning of persistent organic pollutants between blubber and blood of wild bottlenose dolphins: implications for biomonitoring and health. Environ. Sci. Technol. 44, 4789–4795. doi: 10.1021/es1004158
You L., Gazi E., Archibeque-Engle S., Casanova M., Conolly R. B., Heck H. D. A. (1999). Transplacental and lactational transfer of p, p′-DDE in Sprague–Dawley rats. Toxicol. Appl. Pharmacol. 157, 134–144. doi: 10.1006/taap.1999.8673
Keywords: Caretta caretta, Mediterranean Sea, nests, maternal transfer, persistent organic pollutants (POPs), PCBs, DDTs
Citation: Ceciarini I, Capanni F, Minoia L, Consales G, Amico C, Zuffi MAL, Terracciano G, Mancusi C, Neri A, Franchi E, Raimondi G, Prestanti A, Bonucci F, Marchini D, Cancelli F, Caruso C, Tonelli L, Venturi L, Ventrella S, Caliani I and Marsili L (2024) Could persistent organic pollutants affect future generations of sea turtles by maternal transfer? First results for Caretta caretta nests along the North-Western coast of Italy. Front. Mar. Sci. 11:1327137. doi: 10.3389/fmars.2024.1327137
Received: 24 October 2023; Accepted: 29 February 2024;
Published: 18 March 2024.
Edited by:
Carlos Gravato, University of Lisbon, PortugalReviewed by:
Paolo Cocci, University of Camerino, ItalyPeriyadan K. Krishnakumar, King Fahd University of Petroleum and Minerals, Saudi Arabia
Copyright © 2024 Ceciarini, Capanni, Minoia, Consales, Amico, Zuffi, Terracciano, Mancusi, Neri, Franchi, Raimondi, Prestanti, Bonucci, Marchini, Cancelli, Caruso, Tonelli, Venturi, Ventrella, Caliani and Marsili. This is an open-access article distributed under the terms of the Creative Commons Attribution License (CC BY). The use, distribution or reproduction in other forums is permitted, provided the original author(s) and the copyright owner(s) are credited and that the original publication in this journal is cited, in accordance with accepted academic practice. No use, distribution or reproduction is permitted which does not comply with these terms.
*Correspondence: Francesca Capanni, ZnJhbmNlc2NhLmNhcGFubmlAdW5pc2kuaXQ=