- 1Norwegian Polar Institute, Fram Centre, Tromsø, Norway
- 2Akvaplan-niva AS, Fram Centre, Tromsø, Norway
- 3Centre d’Etudes Biologiques de Chizé (CEBC), UMR 7372 CNRS-La Rochelle Université, Villiers-en-bois, France
- 4Norwegian Institute for Nature research, NINA, Oslo, Norway
- 5Norwegian Institute for Nature Research, NINA, Trondheim, Norway
Sea ice, a central component of polar ecosystems, is undergoing profound changes due to climate change. In particular, the Arctic is experiencing unprecedented warming at quicker rates than other regions. This alarming trend of sea ice loss has dire consequences, with spill-over effects on the entire ecosystem, from phytoplankton to top predators. The complex and dynamic nature of sea ice gives rise to diverse habitats, each with the potential to affect larger ecosystems in different ways. However, our understanding of the relative importance of different ice types for higher trophic levels remains limited. To address this knowledge gap, we conducted a comprehensive study of the effects of drift ice, landfast ice, and total sea ice extent (landfast ice + drift ice) on the body condition of six species of polar-breeding seabirds using long-term monitoring data (2003-2021) from Kongsfjorden, Svalbard. These species fell into two categories: Arctic species (Little Auk Alle alle, Brünnich’s Guillemot Uria lomvia, and Glaucous Gull Larus hyperboreus) and “boreal” (or north temperate) species (Black-legged Kittiwake Rissa tridactyla, Arctic Skua Stercorarius parasiticus, and Great Skua Stercorarius skua). We found that the presence and extent of different types of sea ice may have different effects on seabird body condition. Though we did not find any relationship between total sea-ice extent and seabird body condition, drift ice and landfast ice extent did produce significant effects. For Arctic species, these effects were positive. For boreal species, the relationship between body condition and drift and landfast ice was more complex. Our study suggests that the use of a non-specific sea ice variable may mask the effects of sea ice on Arctic wildlife, highlighting the importance of not considering sea ice to be uniform and simple habitat.
Introduction
Arctic sea-ice is disappearing fast due to climate change, and the ten lowest maxima of Arctic sea-ice extent have been recorded in the last 20 years (https://nsidc.org). Sea ice plays a crucial role in polar ecosystems by providing habitat structure, food resources, and shelter from predators for many species (Arrigo, 2017; Meyer et al., 2017; Thomas, 2017; Kohlbach et al., 2022). Sea ice primary production may be regionally and seasonally important compared to open ocean production, and contributes significantly to the total primary production of the marine ecosystem (e.g. in the Central Artic Ocean; Wiedmann et al., 2020). Alterations in sea ice distribution and properties are expected to cause significant ecological consequences for wildlife, such as changes in seasonal distributions and migration patterns, or modifications of life history traits (Carmack and Wassmann, 2006; Tedesco et al., 2019). This can ultimately lead to a decline in populations of ice-dependent species and alter entire ecosystems (Constable et al., 2014; Will et al., 2020).
The cycle of sea ice formation and melting has a direct impact on food webs and the biogeochemical cycles of the upper ocean (Vancoppenolle et al., 2013; Bluhm et al., 2017). Sea ice structures are complex and dynamic, resulting in a wide range of habitats based on thickness, age and dynamic processes such as rafting and ridging (Petrich and Eicken, 2017). Ice algae mainly grow in the bottom skeletal layer of the sea ice where light is sufficient and nutrients from the upper ocean can be exchanged with sea ice brines (Vancoppenolle et al., 2013). Dynamic processes such as surface flooding can also provide nutrients to other parts of the sea ice and lead to surface or internal ice algal growth (Arrigo, 2017). The resulting habitats are crucial for the survival and reproduction of a diverse array of marine invertebrates or fish species (e.g. the polar cod, Boreogadus saida, a key Arctic species, Huserbråten et al., 2019) and play a significant role in maintaining the overall health of marine ecosystems (Lannuzel et al., 2020).
The ecological consequences of sea ice alteration has been the subject of an increasing number of research projects in recent years (Post et al., 2013; Ardyna and Arrigo, 2020). However, due to the complex structure of sea ice, a large number of variables should ideally be considered to accurately describe sea ice structural characteristics (van Leeuwe et al., 2018). Landfast ice and drift ice are two types of sea ice that are commonly found in the polar regions. Landfast ice is the sea-ice anchored to the coast, an ice shelf, or the seafloor, and does not move with ocean currents. Drift ice, on the other hand, is free-floating sea-ice that moves with ocean currents. This free movement results in a dynamic formation and complex internal structure for drift ice, with the prominent formation of ridges and rafted ice floes. Drift ice and landfast ice are different in nature and their potential impacts on upper ocean food webs are not yet fully understood. These two types of sea ice have different physical and chemical properties, as well as different movements and persistence in the ocean, which all impact the organisms that inhabit and depend on them. For example, drift ice is typically much thinner than landfast ice, which allows more light penetration and results in higher sympagic primary production (Lizotte and Sullivan, 1992; Thomas, 2017). On the other hand, landfast ice typically accumulates more algal biomass than drift ice (Meiners et al., 2012; Meiners et al., 2018), which has greater algal biomass losses and higher zooplankton grazing (Arrigo, 2017). Organisms such as copepods and amphipods are, for example, not abundant in landfast ice until it warms and melts, and the brine volume increases. This allows grazers to have access to the sympagic biomass (Stoecker et al., 2000). Therefore, drift ice may represent a more important foraging habitat for grazers and, consequently, for higher trophic level organisms that prey on them. However, the relative importance of drift and landfast ice for upper trophic levels is not well understood, highlighting the need for further studies to better understand the role of sea ice in polar ecosystems.
Seabirds are good bioindicators of changes in the environment (Durant et al., 2009; Smit et al., 2021). In the Arctic, several seabird species are known to be affected by sea ice changes (Descamps and Ramírez, 2021; Divoky et al., 2021; Varpe and Gabrielsen, 2022; Sauser et al., 2023). However, the sea ice variables most commonly used to demonstrate such effects are sea ice extent (SIE) or sea ice concentration (SIC), which do not consider differences between ice types. To better understand the impact of sea ice on seabirds, it is important to know whether the presence of different sea-ice types can impact seabirds.
In this study, we analysed the relationship between the presence of different types of sea ice and the individual body condition of a high Arctic seabird community in Svalbard. While body condition is an indicator of physiological state and health (Murphy, 1996) and is known to be sensitive to changes in environmental conditions (e.g. Gardner et al., 2016), our primary focus is to investigate the specific impacts of different types of sea ice. In polar seabirds, changes in average sea ice extent or concentration have already been shown to impact the body condition of several species through a bottom-up effect (e.g. Jean-Gagnon et al., 2018 on common eiders Somateria mollissima; Sauser et al., 2018 on snow petrels Pagodroma nivea; Amélineau et al., 2019 on little auks Alle alle; Tarroux et al., 2020 on Antarctic petrels Thalassoica antarctica) but to our knowledge, the relative importance of landfast ice versus drift ice has never been tested. We used data from six seabird species (little auk Alle alle, Brünnich’s guillemot Uria lomvia, black legged kittiwake Rissa tridactyla, glaucous gull Larus hyperboreus, Arctic skua Stercorarius parasiticus, great skua Stercorarius skua) to assess the effect of changes in the extent of landfast ice, drift ice, and total sea ice (i.e. landfast ice + drift ice) on body condition. We predict larger effects on seabird body condition due to changes in drift ice compared to landfast ice, as drift ice is associated with higher zooplankton concentrations, on which seabird prey depends directly or indirectly (Søreide et al., 2010; Kohlbach et al., 2017). The species analysed in this study can be divided into two categories: the endemic Arctic species (glaucous gull, little auk, Brünnich guillemot), which breed exclusively in the Arctic, and the north temperate (or so-called “boreal”) species (kittiwake, Arctic skua, great skua), which have a wider distribution and are also common in temperate environments (Descamps and Strøm, 2021). Arctic species are probably better adapted to variations in sea ice, but in the current context of rapid sea ice retreat and atlantification of the region west of Svalbard (De Rovere et al., 2022), we believe that the magnitude of these changes is pushing Arctic species to the limits of their capacity. Boreal species, which also live in the Atlantic region, may be less sensitive to these changes.
Materials and methods
Study site and species
The study was conducted between 2003 and 2021 in Kongsfjorden, located in western Spitsbergen, Svalbard. The little auk is a small alcid (ca. 160 g), feeding exclusively on zooplankton and mainly on the lipid-rich copepod Calanus glacialis (Lønne and Gabrielsen, 1992; Mehlum and Gabrielsen, 1993; Descamps et al., 2022). The little auk foraging ecology is known to be associated with sea-ice (Jakubas et al., 2012; Amélineau et al., 2019). The Brünnich’s guillemot is a large alcid (ca. 1000g). It is a diving species, feeding mainly on fish down to 200 m depths (Mehlum and Gabrielsen, 1993; Elliott and Gaston, 2008). The black-legged kittiwake is a small larid (ca. 320g). It is a surface feeder that targets small fish and zooplankton (Vihtakari et al., 2018). Both of these species are known to forage partly on ice-associated fish, such as polar cod Boreogadus saida (Mehlum and Gabrielsen, 1993; Vihtakari et al., 2018) and their population dynamics have shown positive associations with sea-ice extent (Descamps and Ramírez, 2021). The glaucous gull is a large larid (ca. 1400g), a dietary generalist feeding on a wide variety of prey from both the marine and terrestrial food webs (Weslawski et al., 1994), including polar cod (Erikstad, 1990; Weslawski et al., 1994). The Arctic skua is a medium skua (ca. 450g), with a kleptoparasitic foraging strategy, stealing food mostly from Arctic terns and kittiwakes (Phillips et al., 1996). The great skua is a large skua (ca. 1300g), a dietary generalist feeding on discards and small fish, as well as other birds’ eggs, chicks or adults (Church et al., 2019). The importance of sea-ice in the foraging ecology of the two latter species is unknown but is expected to be low.
Body condition index
For each species, the body mass and structural size (total head length) of breeding individuals were measured each year from a sample of individuals (details in Supplementary Table S1). Various proxies of body condition have been proposed in the literature, and none is perfect for all species and contexts (Labocha and Hayes, 2012). In this study, we chose a simple and widely used body condition index (BCI), defined as the residual from a linear regression of individual body mass against structural size (Supplementary Table S1).These residuals are associated with relative fat mass in adults, making them a good proxy for fat stores (Kraft et al., 2019). We log-transformed body mass and structural size prior to the analyses to remove the heteroscedastic nature of the data (Schulte-Hostedde et al., 2005). Bird identity was included as a random factor in all models to take into account the lack of independence in our data (as a given individual may have been measured in several years). Variation in the condition of birds during the breeding season is well-documented and can be non-linear and species-specific (e.g. Moe et al., 2002; Rodríguez et al., 2017). To account for these intra-seasonal variations, we included the date of capture (expressed as the number of days since May 1st) in our models. Specifically, we included the date of capture as a polynomial variable of order one to three, depending on the species, in order to capture any potential non-linear relationships (see details in Supplementary Table S2).
Sea ice data
We focused on sea-ice in April and May, as this is the period of maximum sea-ice extent and is expected to be a good proxy of the general sea-ice conditions in a given year (details in Descamps and Ramírez, 2021). The spring phytoplankton bloom occurs during this period (Hodal et al., 2012) and is associated with the breeding performance of seabirds (e.g. Ramírez et al., 2017). Sea ice extent in Kongsfjorden was estimated from visual observations from the mountain Zeppelinfjellet each spring from 2003 to 2021 (see details about the procedure in Pavlova et al., 2019; Gerland et al., 2022). The dataset provides a daily ice coverage within the fjord, distinguishing between landfast and drift ice. For each observation day, the coverage of both landfast and drift ice was calculated as a percentage of the total fjord area covered. To determine the total sea ice extent for a given day, we summed the percentages of both types of ice. From these daily values we then determined the annual maximum for each ice category. Our sea ice data are limited to the fjord but although all species can also feed outside the fjord, they all use the fjord, to some extent, as a foraging area.
The drift ice in Kongsfjorden consists mainly of first-year ice derived from the local landfast ice, which limits its comparison with other ice-dominated regions such as Antarctica or the Canadian Arctic. However, Kongsfjorden drift- and landfast-ice still differ in structure (Svendsen et al., 2002). Moreover, under the influence of factors such as winds and ocean currents, additional structural differences can occur. Depending on the meteorological and oceanographic conditions, the drift ice may leave the fjord quickly or remain for a longer period of time. If it stays longer, it may undergo different changes than landfast ice, especially if it is exposed to warmer water. The size of the floe plays an important role in these dynamics, and under the influence of swell, drift ice often breaks up into narrow strips (Pavlova et al., 2019). These sections are particularly vulnerable to warming and melting, which in turn leads to changes in the internal structure of the ice, such as brine channels, and ultimately affects the habitat provided by the ice and associated fauna (Leu et al., 2006).
Statistical analyses
All analyses were performed in R (R Core Team, 2022).
We assessed the temporal trends in sea ice by using linear models (lm function) for each sea ice type (i.e. landfast ice, drift ice, total sea ice extent). We also tested the correlations between the different ice types (cor.test function). To assess the trend in body condition, we constructed a generalised mixed linear model (lmer function) using individual level data with year defined as a continuous variable. We also included the interaction between year and bird category (i.e boreal or Arctic); bird identity and species were included as random factors. To determine whether including the interaction term between year and species category improves the fit of the models, we followed an information-theoretic approach and compared the AIC (Akaike’s Information Criterion, Burnham and Anderson, 2002) of the models. If the difference in AIC values between two models was <2, the models were considered to have similar support, and as models were nested, the simplest was preferred.
We used a similar model selection approach to test for the effect of different sea ice types on birds’ BCI, testing sea ice type separately. We built generalised mixed additive models (gamm function, with a maximum likelihood (ML) estimation procedure, Zuur et al., 2009) with bird identity and species included as random factors. The model used BCI as a response variable and sea ice extent (landfast ice, drift ice or total ice extent) as continuous predictors. To account for non-linear relationships between BCI and covariates, and to limit model over-fitting, we chose an “optimal” number of knots (k, sensu Zuur et al., 2009) based on a visual comparison of the smoothers. The smooth term was set to a maximum of k = 3. To examine the interaction with bird category, we used the same model, adding the category parameter as a main effect and specifying for the sea-ice smoothing parameter that a replicate should be produced for each region (argument ‘by’).
Results
All sea ice types show strong interannual variability in period 2003-2021, ranging from 8 to 46% of drift ice, from 3 to 99% of landfast ice, and from 23 to 100% of total sea ice extent (Table 1; Figure 1). However, none of the sea-ice types considered exhibited a significant trend over the study period (Table 1; Figure 1). Drift ice and landfast ice were not correlated (R2 = 0.23, p-value= 0.35).
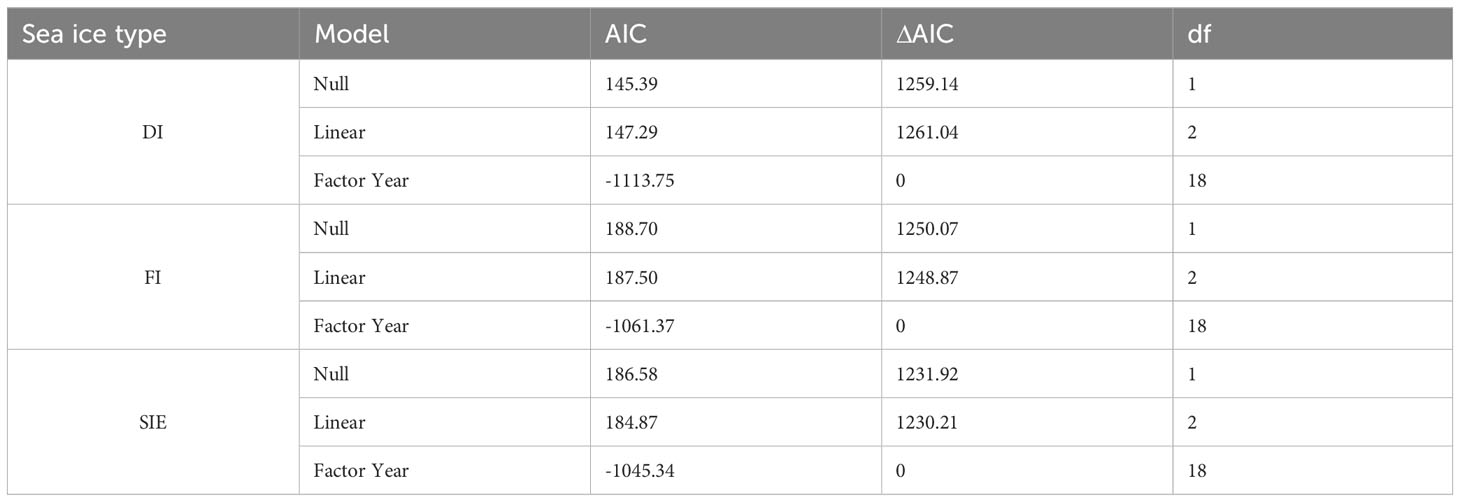
Table 1 Long-term trend in maximum drift ice (DI), maximum fast ice (FI) and maximum total sea ice extent (SIE).
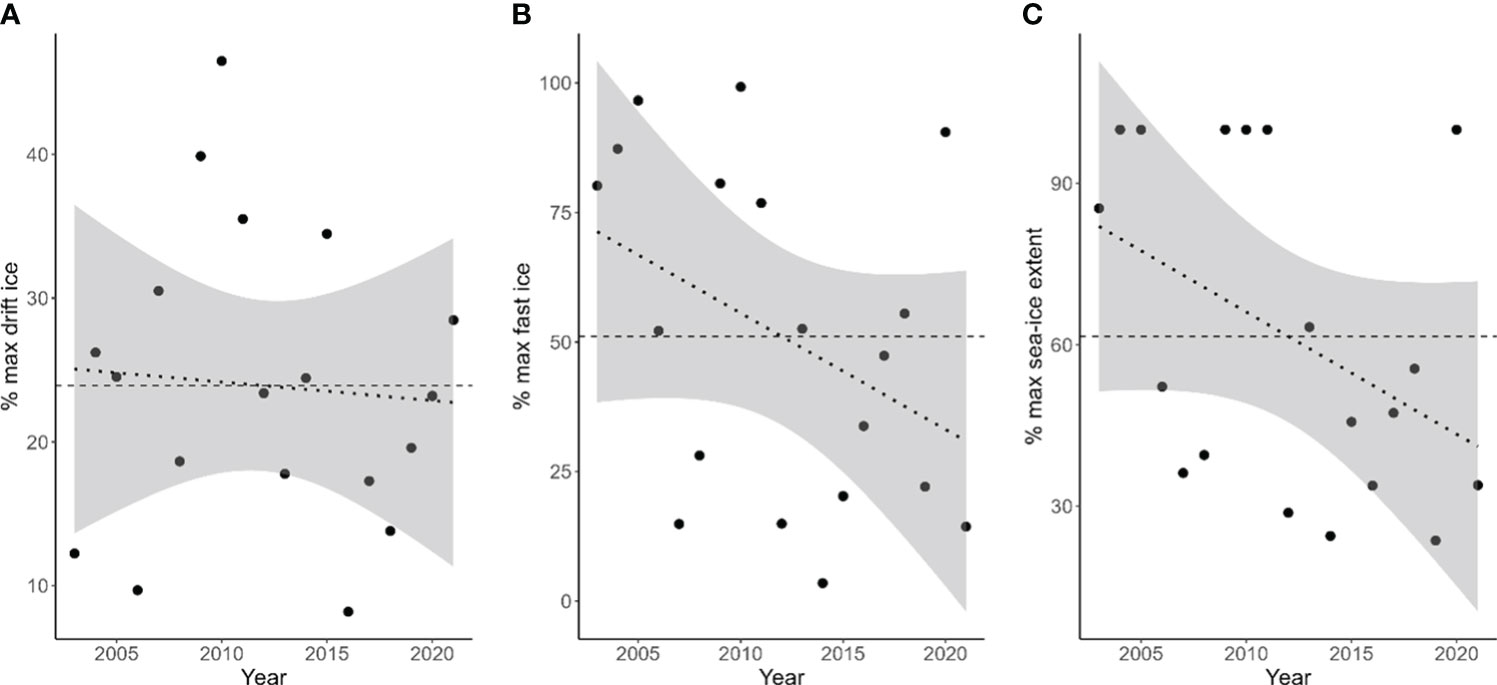
Figure 1 Interannual variation of the three sea ice types [(A): drift ice, (B): fast ice, (C): total sea ice extent] from 2003 to 2021. The horizontal dashed line represents the average per sea ice type over the entire study period. The doted lines represent the trends, along with the 95% confidence intervals, for each ice type. The trends shown are not statistically significant.
The body condition of seabirds breeding in Kongsfjorden showed large interannual variations, and was different for Arctic and boreal species (Table 2; Figure 2). However, there was no significant trend in body condition over the study period (Table 2).
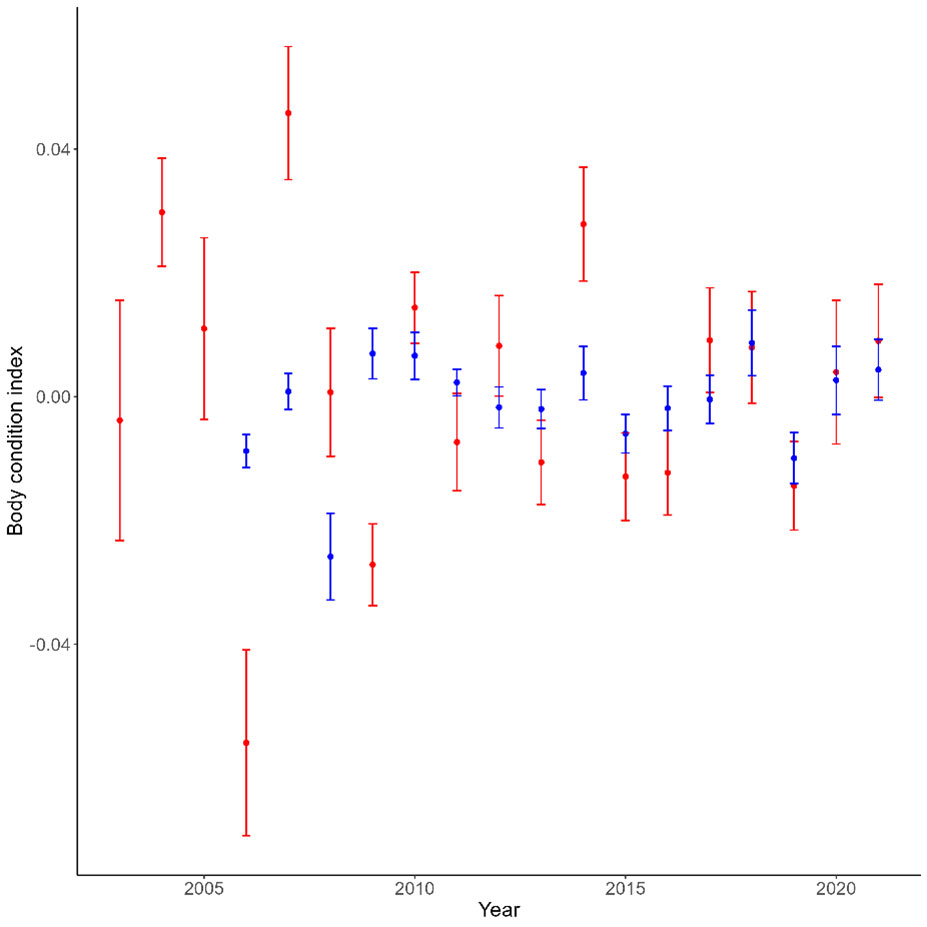
Figure 2 Interannual variations in the body condition of seabirds breeding in Kongsfjorden, Svalbard. Blue and red symbols represent the average condition (and associated SE) for Arctic (blue) and boreal (red) species respectively.
Changes in total sea ice extent in the fjord had no significant effect on seabird body condition (Table 3). However, variations in landfast and drift ice showed significant effects, and these effects differed for Arctic and boreal species (Table 3; Figure 3). For Arctic species, there was a positive linear relationship between body condition and drift ice presence, whereas landfast ice had a non-linear effect. Landfast ice presence was related to relative stability in body condition up to 50% ice cover, after which it showed a positive trend (Figure 3). For boreal species, the impacts of drift ice presence produced a bell-shaped curve with a positive effect up to 30% ice cover, after which it had a negative effect. Conversely, the impacts of landfast ice presence produced an inverted bell-shaped curve with a negative effect on body condition up to 50% ice cover, after which it had a positive effect (Figure 3).
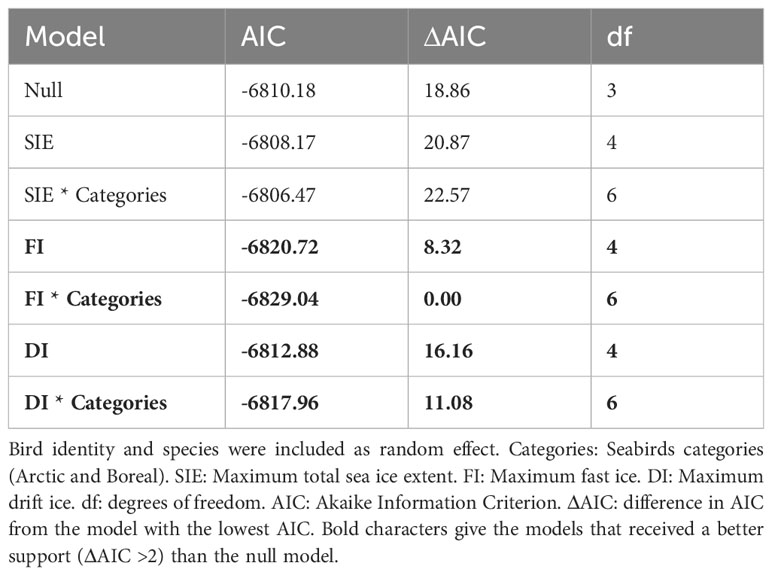
Table 3 Results of the model testing for the relationship between body condition index and sea ice extent for seabirds breeding in Kongsfjorden 2003-2021.
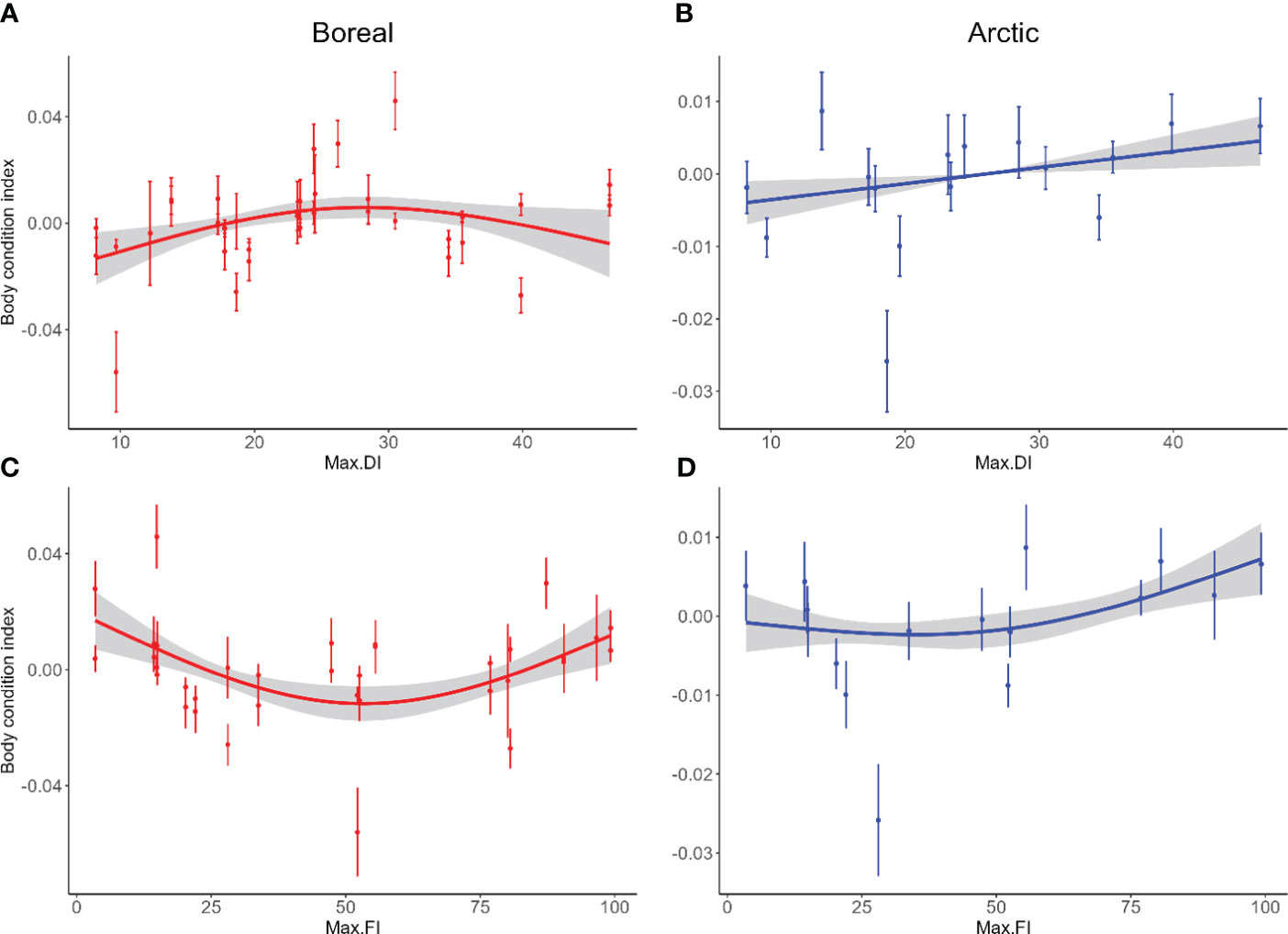
Figure 3 Relationship between maximum sea ice extent (in percentage) and the body condition of Boreal (A, C) and Arctic (B, D) seabirds breeding in Kongsfjorden, Svalbard. Max.DI, Maximum drift ice; Max.FI, Maximum fast ice. Plain lines correspond to estimated relationships from a general additive mixed model with associated 95% confidence intervals. Dots correspond to average body condition with standard error.
Discussion
Temporal variations in sea ice
From nearly 20 years of sea ice observations in Kongsfjorden, we found that while the total maximum sea ice extent varied strongly from year to year, ranging from 24 to 100% (Figure 1C), there was no significant trend. The landfast and drift ice extents also had large interannual variability with no significant trend. While climate change has been shown to have a significant impact on Arctic sea ice and is associated with a strong decline over the 20th century (Stroeve et al., 2012; Hetzinger et al., 2019), we did not observe any significant trends over the study period. It is worth noting, however, that maximum sea ice extent was higher on average in the early years. Our 20-year study period is probably too short to detect a significant decline in sea-ice due to large inter-annual variability, though trends would likely become significant when considering longer time-series. This highlights the need for continued monitoring of sea ice in the Arctic to better measure the impact of climate change on this critical ecosystem component.
Variations in body condition and effects of sea ice on Arctic and boreal species
The body condition of seabirds plays a crucial role in their survival and breeding success (Chastel et al., 1995; Ozgul et al., 2010; Cornioley et al., 2017). Seabirds rely on their body reserves to supply their energy requirements during prolonged periods of foraging, migration, and breeding (Williams, 1966). Having an adequate body condition, is essential to meet the energetic demands of seabirds during various life cycle stages, including breeding and moulting, emphasizing the importance of sufficient nutritional intake. In this study, we observed significant interannual variation in the body condition index (BCI) of seabirds breeding in Kongsfjorden. Temporal changes in BCI can reflect shifts in prey availability, ecosystem productivity, and thus environmental conditions (Montevecchi, 1993; Einoder, 2009). Sea ice has been shown to influence the body condition of several seabird species (e.g., Jean-Gagnon et al., 2018; Amélineau et al., 2019). However, the relationship between sea ice and body condition in seabirds is complex and can be influenced by factors such as sea ice structure (e.g., landfast ice and drift ice), primary and secondary productivity of the ice (Kohlbach et al., 2017; Koch et al., 2023), and the foraging strategies of the species (Amélineau et al., 2016).
We found that the body condition of seabirds breeding in Kongsfjorden is not significantly associated with total sea ice extent, but did have a significant relationship with landfast and drift ice, though the direction of this relationship differed between Arctic and boreal species. As predicted, both landfast and drift ice had an overall positive effect on the BCI of endemic Arctic species. Drift ice exhibits a positive linear relationship, whereas the relationship between BCI and landfast ice appears to be less straightforward, with a relatively stable BCI up to 50% coverage followed by a positive relationship thereafter. While limited in Konsfjorden, landfast and drift ice have distinct biological characteristics (Arrigo, 2017). Landfast ice should accumulates more algal biomass, but drift ice supports greater primary production (Arrigo, 2014; Arrigo, 2017). This suggests that zooplankton consumption might be higher in drift ice, making it a prey source for higher trophic levels (Thomas, 2017).
The impact of sea ice on seabird body condition is also influenced by the life history characteristics and foraging strategies of the species. Some seabird species may exhibit adaptive foraging behaviours and switch to alternative prey sources when sea ice conditions change, while others may rely more heavily on a specific prey species directly affected by sea ice variations. Studies conducted in the Western Antarctic Peninsula have documented significant population declines of key species, including Antarctic krill, silverfish, and Adélie penguins, in response to decreasing sea ice extent (Trivelpiece et al., 2011). Conversely, some species like salps or gentoo penguins appear to benefit from the loss of sea ice, possibly due to their greater adaptability in finding food sources (Forcada, 2008; Massom and Stammerjohn, 2010). Our study suggests that boreal species may be only partially dependent on ice-associated prey, coupled with a greater ability to flexibly switch to alternative prey sources. For example, a study of the diet of Kongsfjorden kittiwakes showed a decrease in consumption of Arctic prey (i.e. ice-associated prey) in recent years concurrently with the emergence of new Atlantic prey in their diet (Vihtakari et al., 2018). However, despite a potentially greater dietary flexibility, our results still indicated that landfast ice and drift ice may impact the body condition of boreal species. Our results also revealed an intriguing pattern in which the effect of sea ice appeared to be reversed depending on the type of ice studied. Drift ice had a bell-shaped effect on the body condition of boreal species, while landfast ice had a U-shaped effect. The mechanisms underlying the relationship between boreal species and the two types of sea ice remain unclear.
Overall, our results suggest that the two types of sea ice may play different roles in the availability of food resources. Additionally, the total sea ice extent (landfast plus drift ice), has no detectable effect on seabird condition. This suggests that the effects of a specific type of sea ice may be masked by the use of a non-specific sea ice variable, and highlights the need for research that considers different types of sea ice to determine the effects of sea ice changes on wildlife.
Limitations, implications and research avenues
The sea ice system in Kongsfjorden is heavily influenced by the advection of Atlantic waters. Sea ice in Kongsfjorden, regardless of its type, primarily originates from local sources and is associated with relatively low biomass. The differences between landfast- and drift ice in this fjord, remain small which may limit our ability to directly extrapolate our results to other ice systems. Furthermore, our study being correlational, we cannot firmly conclude that the sea-ice effects observed here represent causal relationships. It could be that sea-ice changes in Kongsfjorden are simply a proxy of a larger scale phenomenon that would be the true driver of the observed changes in seabird condition. Further studies linking bird foraging behaviour, prey biomass and sea-ice conditions in the fjord would be needed to confirm whether or not sea-ice changes in Kongsfjorden explain, at least partly, changes in seabird condition. It is also important to contextualize our findings by considering the size of sea-ice effects. Despite the statistical significance of our results, the impact of sea ice on the BCI, as measured in this study, appears to be relatively small. For example, a 30% increase in drift ice cover in Kongsfjorden would only increase the BCI of Arctic species by 0.008 points. For species such as the little auk, which weighs on average 150 grams, this increase would correspond to a mere 3-gram gain, and for larger species such as the glaucous gull, which weighs on average 1400 grams, the increase would be around 16 grams. These modest effect sizes suggest that sea ice may not be a major driver of BCI variability among seabirds breeding in Kongsfjorden. Moreover, it is worth noting that our study focused on breeding birds, which are typically individuals in good body condition at the start of the breeding season. Individuals most affected by sea ice changes may have skipped breeding, which would thus bias the sample of individuals included in our study and underestimate the real effects of sea-ice changes.
Until now, most sea ice monitoring has been carried out from satellites, which severely limits our understanding of sea ice structure. It is difficult to determine the exact nature of the sea ice from “above”. While we recognise that wider sea ice conditions beyond our study area can have a significant influence, our localised, direct observations aim to identify specific sea ice features that are important for bioindicators such as seabirds.
Conclusions
Our study provides new insights about the relationship between sea ice and top or meso predators breeding in the high Arctic, the region where the changes in sea ice are the most significant and have far-reaching effects on the entire Arctic ecosystem (Lannuzel et al., 2020). It sheds light on the complex connections between sea ice, prey availability and seabirds. By separately examining the effects of drift ice, landfast ice, and total sea-ice extent on seabird body condition, our analyses showed that the effects of sea ice vary depending on the ice variable considered. Importantly, the use of a general variable like total sea-ice extent did not capture the effects of specific types of sea ice on seabirds. These findings demonstrate the significance of considering the changes and variability of the distinct components that constitute the sea ice zone. It is not merely a question of treating the sea ice zone as an undefined mass bounded by an ice edge. The effects of changes in different types of sea ice described above highlight the need to consider specific sea ice variables when assessing their effects on the wider ecosystem.
Data availability statement
Data concerning Brünnich's guillemot, glaucous gull and little auk are now available in open access on the NPI data centre: 10.21334/npolar.2024.beff2e75. Data on Arctic skua and great skua are available on request to researchers (B. Moe/ S.A. Hanssen) and black legged kittiwake are available on request to researchers (O. Chastel).
Ethics statement
The animal study was reviewed and approved by Mattilsynet (Norwegian Food Safety Anthority, in charge of animal welfare). The study was conducted in accordance with the local legislation and institutional requirements.
Author contributions
CS: Conceptualization, Formal Analysis, Investigation, Methodology, Writing – original draft, Writing – review & editing. PB: Data curation, Writing – review & editing. OC: Data curation, Writing – review & editing. GG: Data curation, Writing – review & editing. SH: Data curation, Writing – review & editing. EL: Data curation, Writing – review & editing. BM: Data curation, Writing – review & editing. SM: Investigation, Writing – review & editing. KS: Data curation, Writing – review & editing. SD: Conceptualization, Data curation, Funding acquisition, Investigation, Methodology, Project administration, Writing – review & editing.
Funding
The author(s) declare financial support was received for the research, authorship, and/or publication of this article. This study was funded by the project FACE-IT (The Future of Arctic Coastal Ecosystems—Identifying Transitions in Fjord Systems and Adjacent Coastal Areas) and the programs SEAPOP (www.seapop.no) and MOSJ (www.mosj.no). FACE-IT has received funding from the European Union’s Horizon 2020 research and innovation program under grant agreement No 869154. The long-term study of kittiwakes in the Kongsfjord was supported by Institut Polaire Français (IPEV project no. 330) and the BNP Paribas Foundation (project SENSEI).
Conflict of interest
The authors declare that the research was conducted in the absence of any commercial or financial relationships that could be construed as a potential conflict of interest.
Publisher’s note
All claims expressed in this article are solely those of the authors and do not necessarily represent those of their affiliated organizations, or those of the publisher, the editors and the reviewers. Any product that may be evaluated in this article, or claim that may be made by its manufacturer, is not guaranteed or endorsed by the publisher.
Supplementary material
The Supplementary Material for this article can be found online at: https://www.frontiersin.org/articles/10.3389/fmars.2024.1326658/full#supplementary-material
References
Amélineau F., Grémillet D., Bonnet D., Bot T. L., Fort J. (2016). Where to forage in the absence of sea ice? Bathymetry as a key factor for an arctic seabird. PloS One 11, e0157764. doi: 10.1371/journal.pone.0157764
Amélineau F., Grémillet D., Harding A. M. A., Walkusz W., Choquet R., Fort J. (2019). Arctic climate change and pollution impact little auk foraging and fitness across a decade. Sci. Rep. 9, 1014. doi: 10.1038/s41598-018-38042-z
Ardyna M., Arrigo K. R. (2020). Phytoplankton dynamics in a changing Arctic Ocean. Nat. Clim. Change 10, 892–903. doi: 10.1038/s41558-020-0905-y
Arrigo K. R. (2014). Sea ice ecosystems. Annu. Rev. Mar. Sci. 6, 439–467. doi: 10.1146/annurev-marine-010213-135103
Arrigo K. R. (2017). “Sea ice as a habitat for primary producers,” in Sea Ice (Oxford, United Kingdom: John Wiley & Sons), 352–369. doi: 10.1002/9781118778371.ch14
Bluhm B. A., Swadling K. M., Gradinger R. (2017). “Sea ice as a habitat for macrograzers,” in Sea Ice (Oxford, United Kingdom: John Wiley & Sons), 394–414. doi: 10.1002/9781118778371.ch16
Burnham K. P., Anderson D. R. (2002). A practical information-theoretic approach. Model. selection multimodel inference 2, 70–71. doi: 10.2307/3803155
Carmack E., Wassmann P. (2006). Food webs and physical–biological coupling on pan-Arctic shelves: Unifying concepts and comprehensive perspectives. Prog. Oceanography 71, 446–477. doi: 10.1016/j.pocean.2006.10.004
Chastel O., Weimerskirch H., Jouventin P. (1995). Body condition and seabird reproductive performance: A study of three petrel species. Ecology 76, 2240–2246. doi: 10.2307/1941698
Church G. E., Furness R. W., Tyler G., Gilbert L., Votier S. C. (2019). Change in the North Sea ecosystem from the 1970s to the 2010s: great skua diets reflect changing forage fish, seabirds, and fisheries. ICES J. Mar. Sci. 76, 925–937. doi: 10.1093/icesjms/fsy165
Constable A. J., Melbourne-Thomas J., Corney S. P., Arrigo K. R., Barbraud C., Barnes D. K. A., et al. (2014). Climate change and Southern Ocean ecosystems I: how changes in physical habitats directly affect marine biota. Glob Change Biol. 20, 3004–3025. doi: 10.1111/gcb.12623
Cornioley T., Jenouvrier S., Börger L., Weimerskirch H., Ozgul A. (2017). Fathers matter: male body mass affects life-history traits in a size-dimorphic seabird. Proc. R. Soc. B: Biol. Sci. 284, 20170397. doi: 10.1098/rspb.2017.0397
De Rovere F., Langone L., Schroeder K., Miserocchi S., Giglio F., Aliani S., et al. (2022). Water masses variability in inner kongsfjorden (Svalbard) during 2010–2020. Front. Mar. Sci. 9, 741075. doi: 10.3389/fmars.2022.741075
Descamps S., Ramírez F. (2021). Species and spatial variation in the effects of sea ice on Arctic seabird populations. Divers. Distrib 27, 2204–2217. doi: 10.1111/ddi.13389
Descamps S., Strøm H. (2021). As the Arctic becomes boreal: ongoing shifts in a high-Arctic seabird community. Ecology 102. doi: 10.1002/ecy.3485
Descamps S., Wojczulanis-Jakubas K., Jakubas D., Vihtakari M., Steen H., Welcker J., et al. (2022). Consequences of Atlantification on a zooplanktivorous Arctic seabird. Front. Mar. Sci. 933, e03485. doi: 10.3389/fmars.2022.878746
Divoky G. J., Brown E., Elliott K. H. (2021). Reduced seasonal sea ice and increased sea surface temperature change prey and foraging behaviour in an ice-obligate Arctic seabird, Mandt’s black guillemot (Cepphus grylle mandtii). Polar Biol. 44, 701–715. doi: 10.1007/s00300-021-02826-3
Durant J., Hjermann D., Frederiksen M., Charrassin J., Le Maho Y., Sabarros P., et al. (2009). Pros and cons of using seabirds as ecological indicators. Clim. Res. 39, 115–129. doi: 10.3354/cr00798
Einoder L. D. (2009). A review of the use of seabirds as indicators in fisheries and ecosystem management. Fisheries Res. 95, 6–13. doi: 10.1016/j.fishres.2008.09.024
Elliott K. H., Gaston A. J. (2008). Mass-length relationships and energy content of fishes and invertebrates delivered to nestling Thick-billed Murres Uria lomvia in the Canadian Arctic 1981-2007. Mar. Ornithology 36, 25–34.
Erikstad K. E. (1990). Winter diets of four seabird species in the Barents Sea after a crash in the capelin stock. Polar Biol. 10, 619–627. doi: 10.1007/BF00239373
Forcada J. (2008). “The impact of climate change on antarctic megafauna,” in Impacts of Global Warming on Polar Ecosystems (Bilbao: Fundación BBVA), 83–112.
Gardner J. L., Amano T., Sutherland W. J., Clayton M., Peters A. (2016). Individual and demographic consequences of reduced body condition following repeated exposure to high temperatures. Ecology 97, 786–795. doi: 10.1890/15-0642.1
Gerland S., Pavlova O., Marnela M., Divine D., Kohler J., Renner A. H. H., et al. (2022). Sea ice extent variability in Kongsfjorden, Svalbard during 2003-2021, based on visual observations from the mountain Zeppelinfjellet. doi: 10.21334/NPOLAR.2022.D6D31F5B
Hetzinger S., Halfar J., Zajacz Z., Wisshak M. (2019). Early start of 20th-century Arctic sea-ice decline recorded in Svalbard coralline algae. Geology 47, 963–967. doi: 10.1130/G46507.1
Hodal H., Falk-Petersen S., Hop H., Kristiansen S., Reigstad M. (2012). Spring bloom dynamics in Kongsfjorden, Svalbard: nutrients, phytoplankton, protozoans and primary production. Polar Biol. 35, 191–203. doi: 10.1007/s00300-011-1053-7
Huserbråten M. B. O., Eriksen E., Gjøsæter H., Vikebø F. (2019). Polar cod in jeopardy under the retreating Arctic sea ice. Commun. Biol. 2, 1–8. doi: 10.1038/s42003-019-0649-2
Jakubas D., Iliszko L., Wojczulanis-Jakubas K., Stempniewicz L. (2012). Foraging by little auks in the distant marginal sea ice zone during the chick-rearing period. Polar Biol. 35, 73–81. doi: 10.1007/s00300-011-1034-x
Jean-Gagnon F., Legagneux P., Gilchrist G., Bélanger S., Love O. P., Bêty J. (2018). The impact of sea ice conditions on breeding decisions is modulated by body condition in an arctic partial capital breeder. Oecologia 186, 1–10. doi: 10.1007/s00442-017-4002-5
Koch C. W., Brown T. A., Amiraux R., Ruiz-Gonzalez C., MacCorquodale M., Yunda-Guarin G. A., et al. (2023). Year-round utilization of sea ice-associated carbon in Arctic ecosystems. Nat. Commun. 14, 1964. doi: 10.1038/s41467-023-37612-8
Kohlbach D., Schaafsma F. L., Graeve M., Lebreton B., Lange B. A., David C., et al. (2017). Strong linkage of polar cod (Boreogadus saida) to sea ice algae-produced carbon: Evidence from stomach content, fatty acid and stable isotope analyses. Prog. Oceanography 152, 62–74. doi: 10.1016/j.pocean.2017.02.003
Kohlbach D., Smik L., Belt S. T., Hop H., Wold A., Graeve M., et al. (2022). A multi-trophic marker approach reveals high feeding plasticity in Barents Sea under-ice fauna. Prog. Oceanography 208, 102895. doi: 10.1016/j.pocean.2022.102895
Kraft F.-L. O. H., Driscoll S. C., Buchanan K. L., Crino O. L. (2019). Developmental stress reduces body condition across avian life-history stages: A comparison of quantitative magnetic resonance data and condition indices. Gen. Comp. Endocrinol. 272, 33–41. doi: 10.1016/j.ygcen.2018.11.008
Labocha M. K., Hayes J. P. (2012). Morphometric indices of body condition in birds: a review. J. Ornithol 153, 1–22. doi: 10.1007/s10336-011-0706-1
Lannuzel D., Tedesco L., van Leeuwe M., Campbell K., Flores H., Delille B., et al. (2020). The future of Arctic sea-ice biogeochemistry and ice-associated ecosystems. Nat. Clim. Change 10, 983–992. doi: 10.1038/s41558-020-00940-4
Leu E., Falk-Petersen S., Kwaśniewski S., Wulff A., Edvardsen K., Hessen D. O. (2006). Fatty acid dynamics during the spring bloom in a High Arctic fjord: importance of abiotic factors versus community changes. Can. J. Fish. Aquat. Sci. 63, 2760–2779. doi: 10.1139/f06-159
Lizotte M. P., Sullivan C. W. (1992). Photosynthetic capacity in microalgae associated with Antarctic pack ice. Polar Biol. 12, 497–502. doi: 10.1007/BF00238188
Lønne O. J., Gabrielsen G. W. (1992). Summer diet of seabirds feeding in sea-ice-covered waters near Svalbard. Polar Biol. 12, 685–692. doi: 10.1007/BF00238868
Massom R. A., Stammerjohn S. E. (2010). Antarctic sea ice change and variability – Physical and ecological implications. Polar Sci. 4, 149–186. doi: 10.1016/j.polar.2010.05.001
Mehlum F., Gabrielsen G. W. (1993). The diet of High-Arctic seabirds in coastal and ice-covered, pelagic areas near the Svalbard archipelago. Polar Res. 12, 1–20. doi: 10.3402/polar.v12i1.6698
Meiners K. M., Vancoppenolle M., Carnat G., Castellani G., Delille B., Delille D., et al. (2018). Chlorophyll-a in Antarctic landfast sea ice: A first synthesis of historical ice core data. J. Geophysical Research: Oceans 123, 8444–8459. doi: 10.1029/2018JC014245
Meiners K. M., Vancoppenolle M., Thanassekos S., Dieckmann G. S., Thomas D. N., Tison J.-L., et al. (2012). Chlorophyll a in Antarctic sea ice from historical ice core data. Geophysical Res. Lett. 39, L21602. doi: 10.1029/2012GL053478
Meyer B., Freier U., Grimm V., Groeneveld J., Hunt B. P. V., Kerwath S., et al. (2017). The winter pack-ice zone provides a sheltered but food-poor habitat for larval Antarctic krill. Nat. Ecol. Evol. 1, 1853–1861. doi: 10.1038/s41559-017-0368-3
Moe B., Langseth I., Fyhn M., Gabrielsen G. W., Bech C. (2002). Changes in body condition in breeding kittiwakes Rissa tridactyla. J. Avian Biol. 33, 225–234. doi: 10.1034/j.1600-048X.2002.330304.x
Montevecchi W. A. (1993). “Birds as indicators of change in marine prey stocks,” in Birds as Monitors of Environmental Change. Eds. Furness R. W., Greenwood J. J. D. (Dordrecht: Springer Netherlands), 217–266. doi: 10.1007/978-94-015-1322-7_6
Murphy M. E. (1996). “Nutrition and Metabolism,” in Avian Energetics and Nutritional Ecology. Ed. Carey C. (Boston, MA: Springer US), 31–60. doi: 10.1007/978-1-4613-0425-8_2
Ozgul A., Childs D. Z., Oli M. K., Armitage K. B., Blumstein D. T., Olson L. E., et al. (2010). Coupled dynamics of body mass and population growth in response to environmental change. Nature 466, 482–485. doi: 10.1038/nature09210
Pavlova O., Gerland S., Hop H. (2019). “Changes in Sea-Ice Extent and Thickness in Kongsfjorden, Svalbard, (2003–2016),” in The Ecosystem of Kongsfjorden, Svalbard Advances in Polar Ecology. Eds. Hop H., Wiencke C. (Cham: Springer International Publishing), 105–136. doi: 10.1007/978-3-319-46425-1_4
Petrich C., Eicken H. (2017). “Overview of sea ice growth and properties,” in Sea Ice (Oxford, United Kingdom: John Wiley & Sons), 1–41. doi: 10.1002/9781118778371.ch1
Phillips R. A., Caldow R. W. G., Furness R. W. (1996). The influence of food availability on the breeding effort and reproductive success of Arctic Skuas Stercorarius parasiticus. Ibis 138, 410–419. doi: 10.1111/j.1474-919X.1996.tb08059.x
Post E., Bhatt U. S., Bitz C. M., Brodie J. F., Fulton T. L., Hebblewhite M., et al. (2013). Ecological consequences of sea-ice decline. Science 341, 519–524. doi: 10.1126/science.1235225
Ramírez F., Tarroux A., Hovinen J., Navarro J., Afán I., Forero M. G., et al. (2017). Sea ice phenology and primary productivity pulses shape breeding success in Arctic seabirds. Sci. Rep. 7, 4500. doi: 10.1038/s41598-017-04775-6
R Core Team (2022). 2020. R: A language and environment for statistical computing (Vienna, Austria: R Foundation for Statistical Computing). Available at: https://www.R-project.org/.
Rodríguez A., Moffett J., Revoltós A., Wasiak P., McIntosh R. R., Sutherland D. R., et al. (2017). Light pollution and seabird fledglings: Targeting efforts in rescue programs: Assessing Condition Of Rescued Seabirds. Jour. Wild. Mgmt. 81, 734–741. doi: 10.1002/jwmg.21237
Sauser C., Angelier F., Blévin P., Chastel O., Gabrielsen G. W., Jouanneau W., et al. (2023). Demographic responses of Arctic seabirds to spring sea-ice variations. Front. Ecol. Evol. 11. doi: 10.3389/fevo.2023.1107992
Sauser C., Delord K., Barbraud C. (2018). Increased sea ice concentration worsens fledging condition and juvenile survival in a pagophilic seabird, the snow petrel. Biol. Lett. 14, 20180140. doi: 10.1098/rsbl.2018.0140
Schulte-Hostedde A. I., Zinner B., Millar J. S., Hickling G. J. (2005). Restitution of mass–size residuals: validating body condition indices. Ecology 86, 155–163. doi: 10.1890/04-0232
Smit K. P., Bernard A. T. F., Lombard A. T., Sink K. J. (2021). Assessing marine ecosystem condition: A review to support indicator choice and framework development. Ecol. Indic. 121, 107148. doi: 10.1016/j.ecolind.2020.107148
Søreide J. E., Leu E., Berge J., Graeve M., Falk-Petersen S. (2010). Timing of blooms, algal food quality and Calanus glacialis reproduction and growth in a changing Arctic. Global Change Biol. 16, 3154–3163. doi: 10.1111/j.1365-2486.2010.02175.x
Stoecker D. K., Gustafson D. E., Baier C. T., Black M. M. (2000). Primary production in the upper sea ice. Aquat. Microbial Ecol. 21, 275–287. doi: 10.3354/ame021275
Stroeve J. C., Kattsov V., Barrett A., Serreze M., Pavlova T., Holland M., et al. (2012). Trends in Arctic sea ice extent from CMIP5, CMIP3 and observations. Geophysical Res. Lett. 39, L16502. doi: 10.1029/2012GL052676
Svendsen H., Beszczynska-Møller A., Hagen J. O., Lefauconnier B., Tverberg V., Gerland S., et al. (2002). The physical environment of Kongsfjorden–Krossfjorden, an Arctic fjord system in Svalbard. Polar Res. 21, 133–166. doi: 10.3402/polar.v21i1.6479
Tarroux A., Cherel Y., Fauchald P., Kato A., Love O. P., Ropert-Coudert Y., et al. (2020). Foraging tactics in dynamic sea-ice habitats affect individual state in a long-ranging seabird. Funct. Ecol. 34, 1839–1856. doi: 10.1111/1365-2435.13632
Tedesco L., Vichi M., Scoccimarro E. (2019). Sea-ice algal phenology in a warmer Arctic. Sci. Adv. 5, eaav4830. doi: 10.1126/sciadv.aav4830
Trivelpiece W. Z., Hinke J. T., Miller A. K., Reiss C. S., Trivelpiece S. G., Watters G. M. (2011). Variability in krill biomass links harvesting and climate warming to penguin population changes in Antarctica. Proc. Natl. Acad. Sci. 108, 7625–7628. doi: 10.1073/pnas.1016560108
Vancoppenolle M., Meiners K. M., Michel C., Bopp L., Brabant F., Carnat G., et al. (2013). Role of sea ice in global biogeochemical cycles: emerging views and challenges. Quaternary Sci. Rev. 79, 207–230. doi: 10.1016/j.quascirev.2013.04.011
van Leeuwe M. A., Tedesco L., Arrigo K. R., Assmy P., Campbell K., Meiners K. M., et al. (2018). Microalgal community structure and primary production in Arctic and Antarctic sea ice: A synthesis. Elementa: Sci. Anthropocene 6, 4. doi: 10.1525/elementa.267
Varpe Ø., Gabrielsen G. W. (2022). Aggregations of foraging black guillemots (Cepphus grylle) at a sea-ice edge in front of a tidewater glacier. Polar Res. 41. doi: 10.33265/polar.v41.7141
Vihtakari M., Welcker J., Moe B., Chastel O., Tartu S., Hop H., et al. (2018). Black-legged kittiwakes as messengers of Atlantification in the Arctic. Sci. Rep. 8, 1178. doi: 10.1038/s41598-017-19118-8
Weslawski J. M., Stempniewicz L., Galaktionov K. (1994). Summer diet of seabirds from the Frans Josef Land archipelago, Russian Arctic. Polar Res. 13, 173–181. doi: 10.1111/j.1751-8369.1994.tb00447.x
Wiedmann I., Ershova E., Bluhm B. A., Nöthig E.-M., Gradinger R. R., Kosobokova K., et al. (2020). What feeds the benthos in the arctic basins? Assembling a carbon budget for the deep arctic ocean. Front. Mar. Sci. 7. doi: 10.3389/fmars.2020.00224
Will A., Takahashi A., Thiebot J.-B., Martinez A., Kitaiskaia E., Britt L., et al. (2020). The breeding seabird community reveals that recent sea ice loss in the Pacific Arctic does not benefit piscivores and is detrimental to planktivores. Deep Sea Res. Part II: Topical Stud. Oceanography 181–182, 104902. doi: 10.1016/j.dsr2.2020.104902
Williams G. C. (1966). Natural selection, the costs of reproduction, and a refinement of Lack’s principle. Am. Nat. 100, 687–690. doi: 10.1086/282461
Keywords: sea ice, seabird, Arctic species, boreal species, body condition
Citation: Sauser C, Blévin P, Chastel O, Gabrielsen GW, Hanssen SA, Lorentzen E, Moe B, Moreau S, Sagerup K and Descamps S (2024) Ice type matters: impacts of landfast and drift ice on body condition in a high Arctic seabird community. Front. Mar. Sci. 11:1326658. doi: 10.3389/fmars.2024.1326658
Received: 23 October 2023; Accepted: 23 January 2024;
Published: 08 February 2024.
Edited by:
Gregory J. Robertson, Environment and Climate Change Canada (ECCC), CanadaReviewed by:
Lech Stempniewicz, University of Gdansk, PolandJan Marcin Weslawski, Polish Academy of Sciences, Poland
Copyright © 2024 Sauser, Blévin, Chastel, Gabrielsen, Hanssen, Lorentzen, Moe, Moreau, Sagerup and Descamps. This is an open-access article distributed under the terms of the Creative Commons Attribution License (CC BY). The use, distribution or reproduction in other forums is permitted, provided the original author(s) and the copyright owner(s) are credited and that the original publication in this journal is cited, in accordance with accepted academic practice. No use, distribution or reproduction is permitted which does not comply with these terms.
*Correspondence: Christophe Sauser, Y2hyaXN0b3BoZS5zYXVzZXJAZ21haWwuY29t