- 1Neoalgae Micro Seaweeds Products S.L., Group of Applied Research on Microalgae (GAREM) Group, Gijon, Spain
- 2Department of Construction and Manufacturing Engineering, University of Oviedo, Gijón, Spain
The nutraceutical market has experienced a significant growth over the last years, due to changing consumer preferences towards products of natural and organic origin. One of the main areas of interest in this field is microalgae-derived carotenoids, whose antioxidant activity protects against oxidative damage in human cells. The diatom Phaeodactylum tricornutum has been widely studied due to its high fucoxanthin content (antioxidant carotenoid). However, pilot and industrial scale experiments to realize continuous and stable production of P. tricornutum and extracted fucoxanthin are limited. For this reason, this research focuses on the study of the fucoxanthin production, going through culture, harvesting, scaling procedure and encapsulation stage. Finally, the optimal culture conditions for a fucoxanthin production of 2.5 mg/day/L were verified. Furthermore, the antioxidant capacity showed a decrease during storage, but still presented a capacity of 18 months, with improved performance when encapsulated with spirulina. The study carried out on encapsulated fucoxanthin-spirulina shows an inhibitory effect on lipogenesis at all concentrations tested in the 3T3-L1 cell line and a lipolytic efficacy at concentrations of 0.2 mg/mL and 0.1 mg/mL in the 3T3-L1 cell line.
1 Introduction
The global change in consumer mentality caused by the recent pandemic and environmental concerns has increased the demand for natural, sustainable and healthy products. In this new scenario, the concept of sustainability has become the most valued term by consumers (BIOECO ACTUAL, 2022). Microalgae are one of the materials most affected by this movement, due to their multiple benefits. These microorganisms are highly efficient in fixing CO2 and producing biomass by capturing sunlight. In addition, their biomass production capacity is four times greater than other vegetable sources (Zhuang et al., 2023). The need for nutrients such as nitrogen, phosphorus, carbon or potassium during cultivation also makes it possible to use the culture stage of these microorganisms in wastewater bioremediation treatments. Other advantages over other vegetable crops include the high lipid content of the biomass, the ability to grow throughout the year and the high growth rate (Cajamar ADN Agro, 2015).
According to the data published by Meticulous Market Research (Meticulous Market Research, 2023) under the title “Algae Product Market”, the global market for microalgae derived products is estimated to exceed $3.92 billion annually by 2030, with a Compound Annual Growth Rate (CAGR) of 5.4% during the forecast period between 2023 and 2030. This indicator refers to the profitability of the investments and allows an objective comparison of different sectors. For all this, and with this value in the microalgae market, capital operators promoted by private investment funds around the world are favored.
Dietary supplements are one of the consumer products affected by this trend. Related to this concept of sustainability, but already within the nutraceutical space, is the antioxidant market. A Google search finds 26,800.000 results related to antioxidant products, making it one of the most sought-after nutraceuticals claims in this sector and the most attractive to the end consumer.
The Panel on Dietary Antioxidants and Related Compounds of the Food and Nutrition Board (Cornelli, 2009), defined nutraceutical antioxidant as “a substance in food that significantly reduces the adverse effects of reactive oxygen species (ROS), reactive nitrogen species (RNS), or both on normal physiological function in humans”. The functional activity of the human body declines over the years, reducing the quality of life and increasing the likelihood of aged-related disease and mortality. Thus, treatments and daily habits that increase the accumulation of oxidative damage with age often exacerbate functional losses. On the other hand, treatments with nutraceutical compounds that reduce the accumulation of oxidative damage delay and attenuate the loss of functional capacity associated with the physical deterioration caused by aging.
These data provide a sufficient basis to establish a correlation between oxidative damage and aging, supporting the hypothesis that oxidative damage of aging is associated with age-related functional decline.
For all these reasons, it would be very valuable to be able to identify the mechanisms involved in this quality decline over the years. A key theory related to this fact of aging is the oxidative damage hypothesis, which states that functional losses associated with aging are caused by the accumulation of RONs damage (Markesbery, 1997).
Phaeodactylum tricornutum is widely used as a model organism due to its genomic sequence and ease of culture. This unicellular eukaryotic diatom microorganism belongs to the group Pennateae (Uzlasir et al., 2023). It is characterized by a length of 5-27 µm length and a width of 3-4 µm, and belongs to the family Phaeodactylaceae. Usually, its extraction focuses on the production of compounds with high added value, such as pigments and total phenolic compounds, but it is also a good source of proteins, carbohydrates and lipids, being one of the most reported algae in Europe for obtaining these compounds. Furthermore, Pagels, F. et al. (Pagels et al., 2022) reported P. tricornutum as the third most biomass producing microalgae, equal to Tetraselmis sp., and behind Isochrysis galbana. P tricornutum strain is known for its rapid growth rate and high yield of lipid content rich in fucoxanthin and n-3 long chain polyunsaturated fatty acids, with fucoxanthin levels at least ten times higher than in macroalgae (Kokkali et al., 2020; Lü et al., 2023).
This work focuses on the study of the development and production of fucoxanthin extract during scale-up and culture in a 400 L tubular photobioreactor, modifying the light intensity to detect potential differences in productivity, lipid content and fucoxanthin content. The antioxidant capacity over time and the hepaprotective capacity have also been demonstrated with an encapsulation process under the Spanish patent of the company Neoalgae. Also, the incorporation of the spirulina-fucoxanthin compound in a cosmetic with lipolytic function (Figure 1).
2 Materials and methods
2.1 Materials
Experimental work was carried out in three different locations: Neoalgae (Gijón, Spain), Bioquochem S. L. (BQC Redox Technologies: Llanera, Spain) and Gaiker Technological Center (Zamudio, Spain).
The algae studied was Phaeodactylum tricornutum (Figure 2), obtained from a collection of algae and protists in Scotland. Permeable reactive barrier (PBR) photobioreactors with capacity of 400 L were used to cultivate the selected microalgae (Aqualgae).
2.2 P. tricornutum cultivation process
2.2.1 Organism and culture conditions
Microalgae were grown in a mixture of artificial and natural seawater (ratio 1:9). Natural seawater was filtered and UV sterilized, and artificial seawater was prepared using tap water and NaCl (35 g/L NaCl). The mixture was then sterilized in an autoclave (121 °C, 20 min) if the final culture volume was less than 2 L, followed by bleaching (40 g/L NaClO) and sodium thiosulfate (Na2S2O3) sterilization protocol for large volumes (v/v). A modified Walne`s medium (Andersen, 2005) with a vitamin solution was used in this experiment (Table 1).
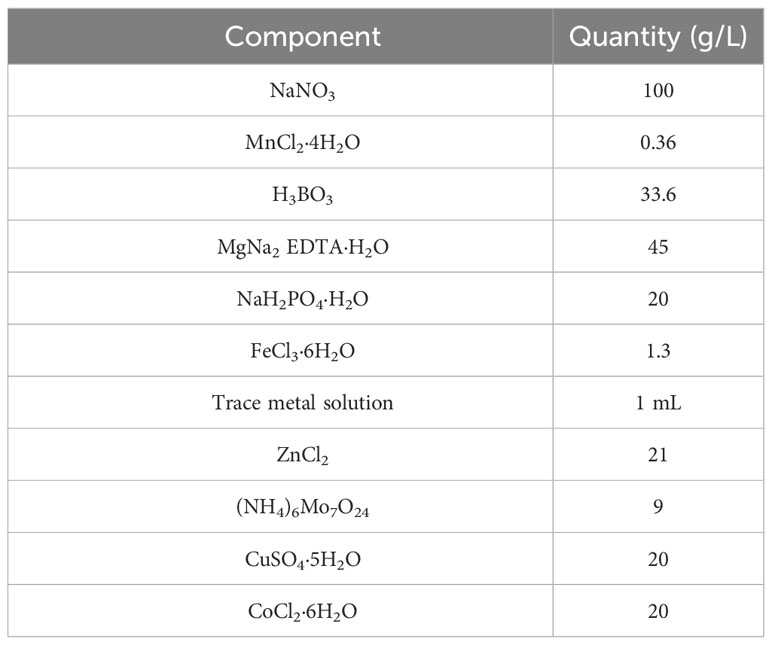
Table 1 Massive culture medium composition for the cultivation of marine phytoplankton commonly used for feeding various shellfish species.
2.2.2 Scale-up procedure
The scale-up procedure began in a 200 mL flask stored at 15 °C under 6500 lux intensity and a controlled photoperiod of 16 h light and 8 h dark, which allowed the microalgae to remain dormant. This process was carried out as batch system, harvesting (or using as inoculum) the whole volume from one culturing step to the next one. In a first step, 100 mL of dormant microalgae culture were diluted in 400 mL of previously sterilized culture medium. An aeration source was added, with a flow rate of 100 L·h-1, and the temperature was kept constant at 25 °C. In the second phase, the same culture conditions were used with a final volume of 2 L. In addition, no CO2 was added during the experiments and all tests were performed in triplicate. For this section of the culturing process, pH was uncontrolled, using sterilized fresh water at pH= 7 as starting point for culturing process.
The following stages of the scale-up process were developed in the same greenhouse with limited control over the culture parameters at Neoalgae facilities in Gijón (Spain) (Blanco-Vieites et al., 2023). In this section of the study, homogenization of the cultures was provided by aeration, keeping a constant flow rate of 100 L·h-1, as previous steps. Firstly, 2 L cultures were used as inoculum for 10 and 20 L plastic bottles (Nalgene, USA), which were maintained until late exponential growth phase was reached. Once cultures reached this section of the growth curve, a maximum of 100L, from 10 and 20 L bottles, of culture was used as inoculum for tubular PBRs of 400 L of working volume (shown in Figure 3). The closed tubular PBRs were placed in a greenhouse under ambient conditions. This section of the study was done under uncontrolled conditions of temperature, light intensity and photoperiod. Moreover, aeration was not necessary due to the fact that culture homogenization was develop through a commercial water pump. This cultivation process was carried out from December 2022 to April 2023, with the two differentiated tests of low and medium light intensity. As well as in previous steps of the scaling up process, starting pH equaled 7 and remained uncontrolled for the assay.
2.2.3 Harvesting
A GEA Westfalia Separator GMBH disc centrifuge (model OSE 5 – 91037) was used to collect the wet biomass produced. This equipment separates liquids from solids due to the high operating speed of the drum (8,000 – 9,000 rpm). Inside the drum discs, the fresh microalgae paste is concentrated with a solids content of 15 – 17%, depending on the concentration of the culture. In this case, 400 L of high solids culture were collected.
2.3 P. tricornutum derived antioxidant production
2.3.1 Lipid extraction
Total lipid extraction was performed according to the method described by Solovchenko, A. (Solovchenko, 2010) where 13.5 mL of chloroform, 6.5 mL of methanol and 5.25 mL of distilled water (68% NaCl) were mixed with 1 g of dry biomass. The mixture was stirred with a magnetic stirrer for 10 min and then centrifuged at 3,000 rpm for 10 min. The lower organic layer was transferred to a glass vial and the chloroform was removed in a rotary evaporator.
The final lipid mass was calculated as the difference between the weight of the empty vial and the vial after removal of the chloroform. Total lipid content (% dry mass) was the lipid mass divided by the initial algal biomass.
2.3.2 Fucoxanthin encapsulation process
The encapsulation process is covered by the European patent with application number: ES2728088A1 (Casado-Bañaes, 2019). This patent describes the incorporation of the fucoxanthin-rich oily extract into the broken spirulina (Arthrospira platensis) matrix, which is the carrier for obtaining powdered product. The process consists of an initial mixing phase, followed by disruption of the microalgae to obtain the encapsulant, and finally homogenization and dehydration of the product. The high percentage of oil (50% w/w) is stabilized only with spirulina, thus the stability of the final product is achieved without the presence of excipients. No binders, dispersants or stabilizers are used. Avoiding the use of excipients is advisable since the product obtained could be used as a nutraceutical.
The addition of a large amount of oil to a microalgae changes the composition of the final product, therefore increasing the oil ratio of a product changes the hydrophilic/lipophilic balance and this change hinders the stability of the product. However, the stability problems of Phaeodactylum oleoresin have been solved and a powder with a high oil content has been obtained, this process improves its physicochemical properties and makes it easier to handle.
For the preparation of the powdered ingredients (spirulina and P. tricornutum extract), a second mixing phase was carried out. The spirulina and oil extracts were prepared in a liquid medium (distilled water). The mixture of water/spirulina/oil extract was performed in optimal proportions 80:10:10 (80% water, 9.9% spirulina, 9.9% P. tricornutum and 0.25% tocopherol). The initial content of fucoxanthin in the material was 0.1 – 0.3%. These percentage may vary depending on the fucoxanthin content to be introduced in the final food supplement, either due to the increase in concentration or due to the fact that the percentage of fucoxanthin in the oil extract is lower and therefore it is necessary to adjust the proportions to obtain the percentage indicated in the food supplement.
Once the mixture was combined, it was lyophilized for dehydration. After this process, a powder product was obtained containing 49.4% spirulina, 49.4% P. tricornutum extract and 1.2% tocopherol. It was then vacuum packed under 48% vacuum for 4 seconds. Finally, it was frozen to preserve its properties.
2.3.3 Antioxidant capacity of fucoxanthin
The stability of the antioxidant capacity of fucoxanthin during storage was evaluated in three different periods of time (0, 12 and 18 months). This parameter was analyzed by the company Bioquochem S. L., according to an ABTS (2,2-azinobis-(3-ethylbenzothiazoline-6-sulfonate) antioxidant capacity test kit (KF01002, Bioquochem, Spain). The ABTS assay is based on the generation of the blue/green ABTS·+ chromophore (maximum absorbance 734 nm) (Vuolo et al., 2018). Subsequently, the antioxidant present in the sample reduces the ABTS, resulting in a discoloration of the solution proportional to the antioxidant activity/capacity and concentration. A vitamin C standard (vitamin C equivalent antioxidant capacity) was used to standardize and compare the TAC of the sample.
2.4 Lipolysis/lipogenesis study
This test focuses on evaluating the effectiveness of reducing the lipid content of a fat-burning cosmetic based on the encapsulated spirulina-fucoxanthin compound. This procedure was carried out in the facilities of the GAIKER Foundation.
The equipment used was the 3T3-L1 cell line (mouse fibroblast cell line, ARCC CL-173), with flow cytometry using fluorochrome (Bodipy) to mark the presence of lipids. The study was carried out in two different procedures: (a) lipolysis: the cell line was pretreated with an inducer of lipid production and exposed to the compound of interest during the last 24 hours; (b) lipogenesis: the cell line is pretreated with the compound of interest throughout the differentiation process. A single replicate was performed for each approach of the spirulina- fucoxanthin-derived product.
3T3-L1 cells were maintained in culture medium for at least two weeks before the start of the experiment to allow optimal cell growth and were subculture before reaching 70% confluence (cell/volume concentration).
For the lipolysis test, 20,000 cells/well were dispersed in preadipocyte expansion medium in 24 wall plates. After 48 hours, the culture medium was changed to differentiation medium and incubated for another 48 hours. Finally, the medium was changed to maintenance medium and 24 hours before the end of the experiment the cells were incubated in the presence and absence of the compound being studied. On the other hand, in the lipogenesis inhibition test, also 20,000 cells/well were dispersed in 24 well plates in full preadipocyte expansion. After 48 hours (or when the culture reached 100% confluence), the medium was changed to a fresh medium in the presence and absence of the compound of interest. The cells were incubated for another 48 hours and transferred to the differentiation medium, also in the presence and absence of the sample, for an incubation period of 48 hours. Finally, they were transferred to a maintenance medium and the evolution of the culture was monitored over the next 48 hours.
2.4.1 Screening test (dose selection)
The 96 well plates (48 lipolysis and 48 lipogenesis) were treated with 8 concentrations of the sample under study for 24 hours at 37 °C and 5% CO2. The sample was prepared in culture medium with the following concentrations: 20, 6.6, 2.2, 0.74, 0.25, 0.08, 0.027 and 0.009 mg/mL.
After incubation, the cells were washed with PBS and stained with MTT solution. These plates were incubated again at 37 °C for 2 hours, and then 100 µl DMSO/well was added. The absorbance was determined at 540 nm, and the percentage of cellular viability (%) was calculated according to Equation 1, where the OD(t) is the optical density of the culture after exposure to a sample and OD(c) is the optical density of the negative (untreated) control:
2.4.2 Efficacy test
For efficacy testing, cells were incubated with four different concentrations of the test sample for 24 hours: 0.2 mg/mL, 0.1 mg/mL, 0.05 mg/mL and 0.025 mg/mL. The anti-lipogenesis efficacy study verified the prevention of lipid production in the 3T3-1L cell culture, while the lipolytic efficacy study verified the ability of the compound to reduce the presence of lipids.
Lipid accumulation was determined using the fluorochrome Bodipy 493/503 (4,4-difluoro-1,3,5,7,8-Pentamethyl-4-Bora-3a,4a-Diaza-s-Indacene), which can be used as a natural lipid stain and as a marker for oils and other non-polar lipids due to its non-polar structure, fluorescence and long-wavelength absorption.
3 Results and discussion
3.1 Scale-up productivity
Figure 4 shows the P. Tricornutum microalgae production under low light intensity conditions from December 2022 to February 2023, while Figure 5 shows the equivalent for medium light intensity conditions from April 2023 to May 2023.
In the experiments carried out under low intensity conditions, the concentration of wet biomass production by culture was remarkably stable. On the other hand, those performed under medium intensity conditions were subjected to extreme environmental conditions due to the high temperatures experienced in Asturias (Spain) during the test period (Figure 6). This caused a significant decrease in the concentration of microalgae and, therefore, in the production of wet biomass from inside the tubular photoreactors. Apart from the stress period, the microalgal biomass production remained unstable in the same way. This phenomenon has been observed by other authors under high light culture conditions of P. Tricornutum (Remmers et al., 2017; Truong et al., 2023).
3.2 Lipid content
Analogous to the dry biomass production, the fucoxanthin productivity and content in the microalgae oil extract showed the same tendency. In the low light intensity test shown in Figure 7, the fucoxanthin content (g/day) remained relatively stable until day 95.
On the other hand, its production under medium light intensity growth conditions (Figure 8) showed an increasing trend until reaching the extreme temperature periods where the productivity falls to almost 0 mg fucoxanthin/day/L. Once the environmental stress conditions ceased, this phenomenon disappeared and the fucoxanthin content in the extract returned to maximum peaks (similar to those obtained at low light intensity) of 0.47 g/day and 2.3 mg fucoxanthin/day/L, respectively.
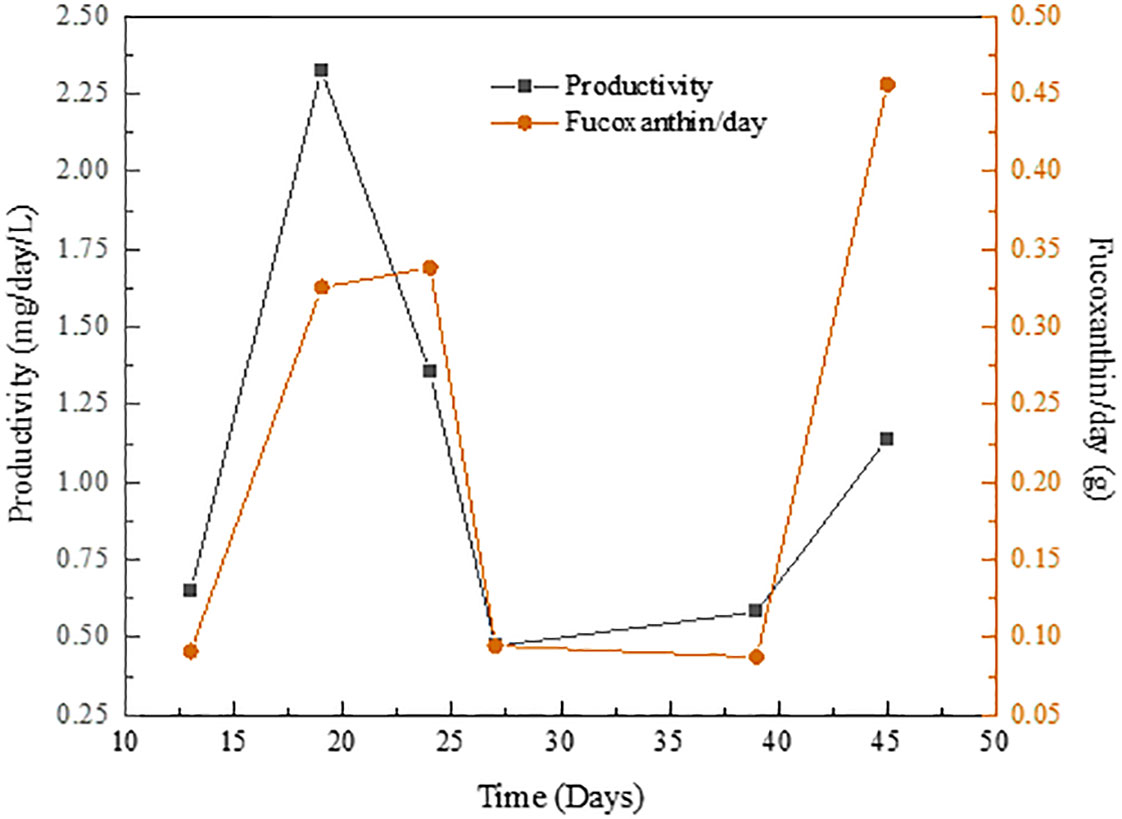
Figure 8 Fucoxanthin productivity from P. tricornutum growth in tubular PBR at medium light intensity.
Truong, T. Q., et al. (Truong et al., 2023) also evaluated the cell density and fucoxanthin content of P. tricornutum at different light intensities, which for them were high and low. In the study, they observed a drastic decrease in fucoxanthin content in the tests performed at high light intensity. The growth rate of P. tricornutum was similar to that reported in the current study: relatively stable at low intensity and more erratic at high intensity (medium intensity in the current study). Fucoxanthin production also decrease with increasing intensity.
3.3 Antioxidant capacity
The analysis of the antioxidant capacity of fucoxanthin present in the oily extract, shown in Figure 9, reveals a high oxidative capacity immediately after the spirulina encapsulation process (0 months). Over time, a decrease in its efficiency was observed, decreasing by 32% after 12 months of storage and up to 45% after 18 months. Despite the significant degradation over time, the antioxidant capacities of the compound remain remarkable after 18 months of storages.
3.4 Lipolysis/lipogenesis study
3.4.1 Screening test
Figures 10 and 11 show the percentage of cell viability of 3T3-L1 cells exposed to the indicated concentration of the tested (Figure 10) and reference (Figure 11) compounds, obtained from the absorbance data measurements during the screening test for the dose selection. SDS was the reference compound tested to determine the acceptability of the assay. C-1 and C-2 are untreated cells.
Based on the results of this study, was concluded that the optimal concentrations for the efficacy test would be 0.2, 0.1, 0.05 and 0.025 mg/mL.
3.4.2 Efficacy test
The results of the lipogenesis inhibition test are presented in Figure 12, which shows the percentage of fluorescence of 3T3-L1 cells at different concentrations. This clearly shows that the lipogenesis inhibitory activity is directly dependent on the spirulina-fucoxanthin content of the sample.
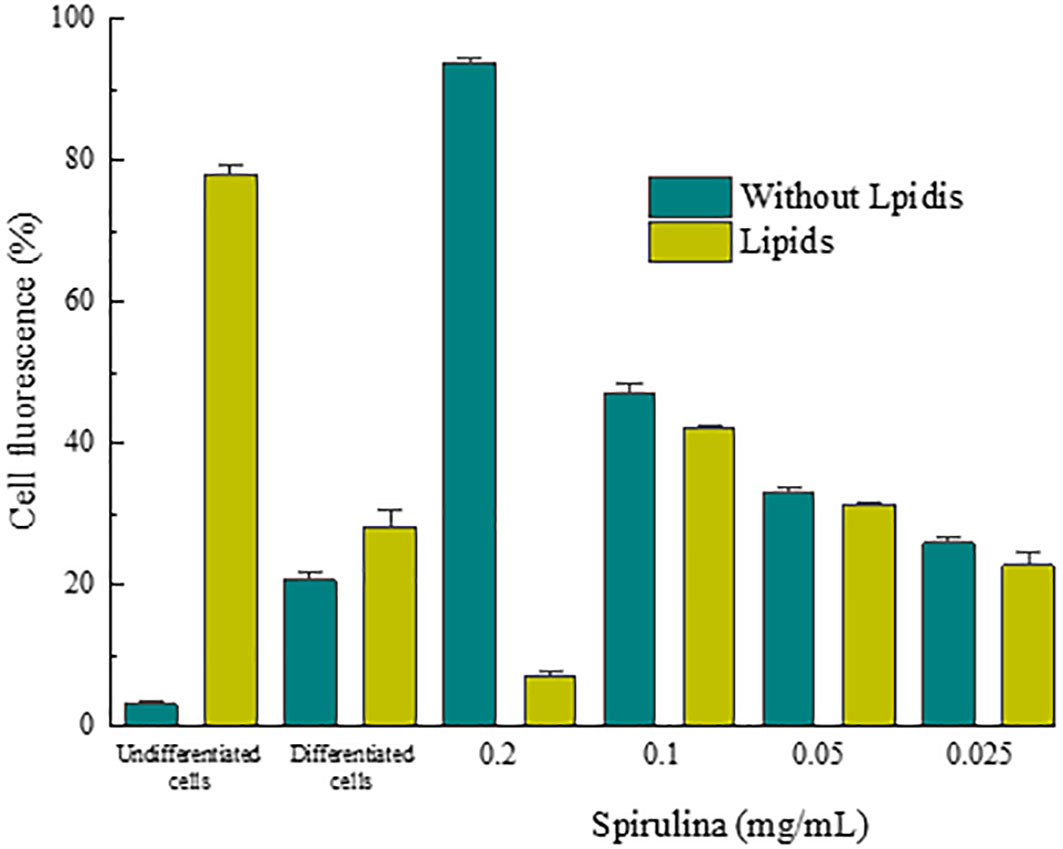
Figure 12 Percentage of fluorescence of the 3T3-L1 cells during the entire adipocyte differentiation process adipocytes to indicated concentrations of the material under study.
For the lipolytic effectiveness test, the data shown in Figure 13 were obtained. It represents the percentage of fluorescence of the 3T3-L1 cells in the same procedure as the previous test, where it is verified that the compound under study has a lipolytic activity, especially at a concentration of 0.2 and 0.1 mg/mL.
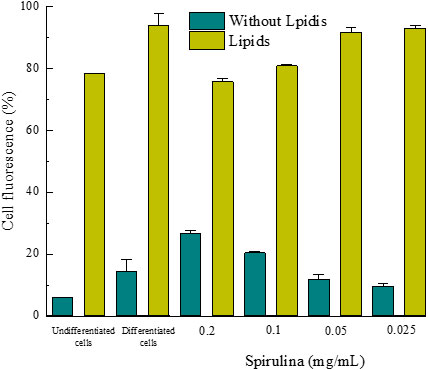
Figure 13 Percentage of fluorescence of 3T3-L1 cells differentiated to adipocytes to indicated concentrations of the material under study.
4 Conclusions
The availability of naturally occurring carotenoids in microalgae, such as fucoxanthin, provides an attractive source for the production of natural antioxidants. This, combined with the environmental and pollution control benefits of cultivating these microorganisms, makes them a potential asset to the global economy. In the present research, the culture of the Phaeodactylum tricornutum microalgae was successfully scale-up and the following points were concluded:
Fucoxanthin content in P. tricornutum was shown to be higher at low light intensities, and also appears to be more stable during the scale-up. Cultivation under medium light intensity does not remain stable but reaches production values similar to the previous one (2.5 mg/day/L). The instability of this test was also affected by the extreme temperature conditions of the site, reaching 40 °C, which caused a decrease in production of 0.5 mg/day/L.
The antioxidant capacity of fucoxanthin showed a significant decrease during the storage process. However, although the fucoxanthin-rich product is reduced, the antioxidant capacity is maintained after 18 months. Also, the antioxidant capacity of fucoxanthin encapsulated in spirulina was greater than that of the biomass. Thus, the antioxidant capacity of encapsulated fucoxanthin-spirulina is therefore greater than that of spirulina alone. This capacity is clearly due to the capabilities of fucoxanthin.
The fucoxanthin-spirulina material incorporated in a fat burning cosmetic showed a high inhibitory effect on lipogenesis at all concentrations tested, as well as a remarkable lipolytic function, especially at 0.2 and 0.1 mg/mL.
Data availability statement
The raw data supporting the conclusions of this article will be made available by the authors, without undue reservation.
Author contributions
JD-R: Conceptualization, Formal analysis, Methodology, Supervision, Validation, Visualization, Writing – original draft, Writing – review & editing. MÁ-G: Data curation, Project administration, Resources, Writing – review & editing. VC-B: Data curation, Investigation, Methodology, Writing – original draft. DS-M: Investigation, Methodology, Validation, Writing – original draft. CS-M: Formal analysis, Investigation, Writing – review & editing.
Funding
The author(s) declare financial support was received for the research, authorship, and/or publication of this article. This research was funded by REA in the Horizon Europe Framework Program within the funding scheme of Fair, healthy and environmentally-friendly food systems from primary pro-duction to consumption under the scope of the IMPRESS Project 101084437 (HORI-ZON-CL6-2022-FARM2FORK-02-two-stage).
Acknowledgments
The authors gratefully acknowledge the support of the Neoalgae Micro-Seaweed Products (Spain) Bioquochem S.L. (Spain) and Gaiker Technological Center (Spain) for this work.
Conflict of interest
GAREM Group is a research group inside Neoalgae. JD-R, MÁ-G, VC-B and DS-M were employed by Neoalgae Micro Seaweeds Products S.L.
The remaining author declares that the research was conducted in the absence of any commercial or financial relationships that could be construed as a potential conflict of interest.
The reviewer, MB-V, declares a past co-authorship with author, JD-R, with no conflict of interest.
Publisher’s note
All claims expressed in this article are solely those of the authors and do not necessarily represent those of their affiliated organizations, or those of the publisher, the editors and the reviewers. Any product that may be evaluated in this article, or claim that may be made by its manufacturer, is not guaranteed or endorsed by the publisher.
References
Andersen R. A. (2005). Algal culturing techniques. Ed. Andersen R. A. (New York: Elsevier Academic Press) 578.
BIOECO ACTUAL (2022) 2022: Tendencias y perspectivas de los productos naturales y ecológicos. Available at: https://www.bioecoactual.com/2022/07/20/2022-tendencias-y-perspectivas-de-los-productos-naturales-y-ecologicos/ (Accessed October 13, 2023).
Blanco-Vieites M., Casado V., Battez A. H., Rodríguez E. (2023). Culturing Arthrospira maxima in mining wastewater: Pilot-scale culturing and biomass valorization into C-phycocyanin and crude lipid extract. Environ. Technol. Innov. 29, 102978. doi: 10.1016/j.eti.2022.102978
Casado-Bañaes V. (2019). Procedimiento de microencapsulacion de aceites en microorganismos, producto obtenido por ese procedimiento y usos del mismo. ES2728088A1. Available at: https://patentimages.storage.googleapis.com/4c/59/09/8d2697c440d44d/ES2728088A1.pdf
Cornelli U. (2009). Antioxidant use in nutraceuticals. Clin. Dermatol. 27, 175–194. doi: 10.1016/j.clindermatol.2008.01.010
Kokkali M., Martí-Quijal F. J., Taroncher M., Ruiz M. J., Kousoulaki K., Barba F. J. (2020). Improved Extraction Efficiency of Antioxidant Bioactive Compounds from Tetraselmis chuii and Phedoactylum tricornutum Using Pulsed Electric Fields. Molecules 25, 3921. doi: 10.3390/molecules25173921
Lü L., Ma J. R., Li S. X., Xing H. Z., Yang Y. H., Miyashita K. (2023). Characterization and antioxidant evaluation of four kinds of marine oils in vitro. J. Oleo Sci. 72, 389–397. doi: 10.5650/jos.ess22362
Markesbery W. R. (1997). Oxidative stress hypothesis in Alzheimer’s disease. Free Radic. Biol. Med. 31, 134–147. doi: 10.1016/S0891-5849(96)00629-6
Meticulous Market Research (2023) Algae products market. 0–250. Available at: https://www.meticulousresearch.com/product/algae-products-market-3579 (Accessed October 13, 2023).
Pagels F., Amaro H. M., Tavares T. G., Amil B. F., Guedes A. C. (2022). Potential of microalgae extracts for food and feed supplementation—A promising source of antioxidant and anti-inflammatory compounds. Life 12 (11), 1901. doi: 10.3390/life12111901
Remmers I. M., Martens D. E., Wijffels R. H., Lamers P. P. (2017). Dynamics of triacylglycerol and EPA production in Phaeodactylum tricornutum under nitrogen starvation at different light intensities. PloS One 12 (4), e0175630. doi: 10.1371/journal.pone.0175630
Solovchenko A. (2010). Photoprotection in plants. 1st (Berlin, Heidelberg: Springer Berlin Heidelberg). doi: 10.1007/978-3-642-13887-4
Truong T. Q., Park Y. J., Koo S. Y., Choi J. H., Enkhbayar A., Song D. G., et al. (2023). Interdependence of fucoxanthin biosynthesis and fucoxanthin-chlorophyll a/c binding proteins in Phaeodactylum tricornutum under different light intensities. J. Appl. Phycol 35, 25–42. doi: 10.1007/s10811-022-02856-2
Uzlasir T., Selli S., Kelebek H. (2023). Effect of Salt Stress on the Phenolic Compounds, Antioxidant Capacity, Microbial Load, and In Vitro Bioaccessibility of Two Microalgae Species (Phaeodactylum tricornutum and Spirulina platensis). Foods 12 (17), 3185. doi: 10.3390/foods12173185
Vuolo M. M., Lima V. S., Maróstica Junior M. R. (2018). Phenolic compounds: structure, classification, and antioxidant power. Bioactive Compounds: Health Benefits Potential Appl. 33–50. doi: 10.1016/B978-0-12-814774-0.00002-5
Keywords: phaeodactylum tricornutum, microalgae, fucoxanthin, antioxidant, spirulina, Lipolysis, lipogenesis
Citation: Delgado-Ramallo JF, Álvarez-Gil M, Casado-Bañares V, Suárez-Montes D and Sanjurjo-Muñíz C (2024) Phaeodactylum tricornutum as a stable platform for pilot scale production and investigation of the viability of spirulina fucoxanthin as a commercial lipolysis active novel compound. Front. Mar. Sci. 11:1325159. doi: 10.3389/fmars.2024.1325159
Received: 31 October 2023; Accepted: 02 January 2024;
Published: 22 January 2024.
Edited by:
Weiqi Fu, Zhejiang University, ChinaReviewed by:
Jichang Han, Ningbo University, ChinaMario Blanco-Vieites, University of Santiago de Compostela, Spain
Copyright © 2024 Delgado-Ramallo, Álvarez-Gil, Casado-Bañares, Suárez-Montes and Sanjurjo-Muñíz. This is an open-access article distributed under the terms of the Creative Commons Attribution License (CC BY). The use, distribution or reproduction in other forums is permitted, provided the original author(s) and the copyright owner(s) are credited and that the original publication in this journal is cited, in accordance with accepted academic practice. No use, distribution or reproduction is permitted which does not comply with these terms.
*Correspondence: Jesús Fidel Delgado-Ramallo, fdelgado@neoalgae.es