- 1Marine Science Program, Biological and Environmental Science and Engineering Division (BESE), King Abdullah University of Science and Technology (KAUST), Thuwal, Saudi Arabia
- 2KAUST Red Sea Research Center, King Abdullah University of Science and Technology (KAUST), Thuwal, Saudi Arabia
- 3Marine Conservation Program, NEOM, Saudi Arabia
- 4Center of Carbonate Research, Department of Marine Geosciences, Rosenstiel School of Marine and Atmospheric Science, University of Miami, Miami, FL, United States
- 5OceanX, New York, NY, United States
- 6National Center for Wildlife Development, Riyadh, Saudi Arabia
- 7Computational Bioscience Research Center (CBRC), Division of Biological and Environmental Science and Engineering (BESE), King Abdullah University of Science and Technology, Thuwal, Saudi Arabia
Coral-dwelling gall crabs (Cryptochiridae) live in an obligate symbiosis with reef-building corals from shallow to deep waters. In particular, crabs of the genus Opecarcinus are known to occur across the tropical belt in association with the scleractinian family Agariciidae, down to a depth of 89 m. The Red Sea is a semi-enclosed basin that has long been recognized as a region of high marine biodiversity and endemism, with more than 230 decapod species recorded so far. There, nine Opecarcinus species are recorded from shallow and mesophotic corals. However, the occurrence of Opecarcinus below 30 m depth is documented only in the Saudi Arabian coast of the Northern Red Sea, and the rest of the Saudi Arabian Red Sea has remained unstudied. In this work, we assess the diversity and distribution of Opecarcinus from the mesophotic waters of the Saudi Arabian Red Sea. Through Remotely Operated Vehicles (ROV) and Submarines explorations, we collected 10 gall crab specimens associated with Agariciidae corals at mesophotic depths between 47 and 89 m. Two mitochondrial and one nuclear markers were used to obtain crab phylogenetic tree showing that three of the Opecarcinus species known from Red Sea shallow-water corals can also live at mesophotic depths. This is the first assessment of the depth distribution of the genus Opecarcinus in the Red Sea. It provides further evidence of the depth range adaptability of the Opecarcinus-Agariciidae association and highlights the need for a more in-depth investigation of the largely overlooked mesophotic coral-associated fauna.
Introduction
The gall crab family Cryptochiridae Paulson, 1875, is a widely distributed yet much-overlooked family on coral reefs (Simon-Blecher and Achituv, 1997; Hoeksema and van der Meij, 2013; Nogueira et al., 2014), living in obligate symbiosis with at least 66 tropical scleractinian coral genera from the Atlantic to the Indo-Pacific Ocean (Castro, 2015). Cryptochirids are primarily found in shallow-water reefs but can occur below 600 m depth (Kropp and Manning, 1987; Zibrowius and Gili, 1990; Manning, 1991). So far, only the Atlantic genus Detocarcinus Kropp and Manning, 1987, the circumtropical genus Opecarcinus Kropp and Manning, 1987, the Indo-Pacific genera Zibrovia Kropp & Manning, 1996, and Luciades Kropp and Manning, 1996 (probably a junior synonym of Opecarcinus – Xu et al., 2022) inhabit Mesophotic Coral Ecosystems (~30-150 m light-dependent tropical and subtropical reef communities – see Pyle and Copus, 2019 for an overview). In particular, the genera Opecarcinus and Luciades occur in the mesophotic in association with the agariciid genera Agaricia Lamarck, 1801(the former), exclusively occurring in the Atlantic, and the Indo-Pacific Leptoseris Milne Edwards and Haime, 1849 (both the two genera) (Kropp and Manning, 1996; Komatsu and Takeda, 2013; van der Meij et al., 2015; Xu et al., 2022; Vimercati et al., 2023). The ability of Opecarcinus to inhabit Agariciidae Gray, 1847 corals both in shallow and mesophotic reefs was linked to its depth range adaptability (van der Meij et al., 2015; van Tienderen and van der Meij, 2016) and to the fact that Opecarcinus’ host-specificity can influence its depth range (Vimercati et al., 2023). However, further studies are still required to understand their mesophotic diversity and geographic distribution.
The Red Sea is a young ocean basin (Ligi et al., 2012; Bonatti et al., 2015; Rasul et al., 2015; Augustin et al., 2021) characterized by high water temperature and salinity (Edwards, 1987; Roder et al., 2013). These environmental parameters in the Red Sea change gradually along latitude and depth (Sofianos and Johns, 2003; Raitsos et al., 2013; Rowlands et al., 2014, Rowlands et al., 2016; Chaidez et al., 2017; Berumen et al., 2019; Manasrah et al., 2019), making this body of water an optimal natural system to study the distribution of reef organisms (Berumen et al., 2019). As a result of its past and present geological configuration and its ecological conditions, the Red Sea has been recognized as a biodiversity hotspot with a high degree of endemism rate for shallow water organisms (Sheppard et al., 1992; Veron, 1995; Hughes et al., 2002; Obura, 2012; DiBattista et al., 2016a, DiBattista et al., 2016b; Berumen et al., 2019). However, our knowledge of the biota inhabiting Red Sea MCEs remains scarce due to logistical difficulties in reaching these environments (Hinderstein et al., 2010; Berumen et al., 2013, 2019). Studies on Red Sea MCEs are mainly limited to anthozoan corals of the Eilat coast of the Gulf of Aqaba (see, for example, Fricke and Hottinger, 1983; Fricke and Schuhmacher, 1983; Fricke and Knauer, 1986; Schlichter et al., 1986; Mass et al., 2007; Stambler et al., 2008; Alamaru et al., 2009; Einbinder et al., 2009; Nir et al., 2011), with some more recent explorations carried out along the Saudi Arabian Red Sea (see, for example, Ziegler et al., 2015; Chimienti et al., 2022; Terraneo et al., 2023; Vimercati et al., under revision; Vicario et al., submitted). To shed light on the biology and the ecology of MCEs, a focus on overlooked reef-associated invertebrates, such as cryptochirids, is crucial. In fact understanding how the diversity and ecology of coral-associated invertebrates change with increasing depth can be useful to establish the reef-associated invertebrates’ roles within coral reef ecosystems, especially in regard to their impact on reef resilience, and can inform the steps needed for their conservation (Stella et al., 2011; van der Schoot and Hoeksema, 2024). In this context, Red Sea scleractinian corals are known to host nine cryptochirid species ascribed to the genus Opecarcinus, mostly occurring in shallow water (< 30 m) (Xu et al., 2022), with the notable exception of the depth generalist Opecarcinus identified by the preliminary code SET4 (Xu et al., 2022), recovered on one Leptoseris cf. mycetoseroides Wells, 1954, specimen at 89 m depth only in the North Red Sea (Vimercati et al., 2023). Hence, the diversity, distribution, and host association of coral-dwelling Opecarcinus along the mesophotic Red Sea latitudinal gradient remains unknown, mainly due to the lack of exploration and targeted sampling beyond SCUBA diving depths.
During different recent research cruises from 2020 to 2022, Remotely Operated Vehicle (ROV) and manned submersible vehicle sampling allowed us to collect 10 gall crab specimens from MCE corals in four distinct regions spanning the Saudi Arabian Red Sea coastline. This study assesses Opecarcinus mesophotic diversity and distribution along the Saudi Arabian Red Sea. We provide a phylogeny reconstruction for the genus Opecarcinus and report new geographic and depth records and coral host associations.
Materials and methods
Crab sampling
A total of 10 gall crab specimens belonging to the genus Opecarcinus were collected during the Red Sea Deep Blue Expedition in 2020 (n=2) and the Red Sea Decade Expedition in 2022 (n=8) on board the M/V OceanXplorer along the Saudi Arabian Red Sea MCEs (Table 1). Sampling of the host corals took place in four regions spanning the latitudinal range of the Saudi Arabian Red Sea from the North Red Sea (NEOM area) (two sites), Al Wajh (one site), Central Red Sea (one site), and South Red Sea (four sites) (Figures 1A, B). The Opecarcinus specimens were extracted from their dwellings using pliers and tweezers from coral colonies of the genus Leptoseris and Pavona Lamarck, 1801, sampled between 47 and 89 m in depth using an Argus Mariner XL ROV and a triton 3303/3 submersible with a Schilling T4 hydraulic manipulator. An example of an ongoing sampling is shown in Figures 1C–E. The ROV and the submersible dives were video-recorded, and frame grabs of the crab-inhabited coral colonies were extracted from the videos using the open-source software MPC-HC (Media Player Classic – Home Cinema) and Adobe Premier software PRO™, respectively, to obtain host and crab dwelling images in situ. Kongsberg HIPaP 501 USBL (Ultra-Short Baseline), Sonardyne Sprin INS (Inertial Navigation System), and Sonardyne Ranger Pro 2 USBL provided the underwater vehicles’ geographic and depth position. The collected gall crabs were preserved in 80% ethanol for future molecular analyses and examined and imaged under a Leica M205A stereomicroscope. The host coral and gall crab specimens are deposited at the King Abdullah University of Science and Technology (KAUST), Thuwal, Saudi Arabia.
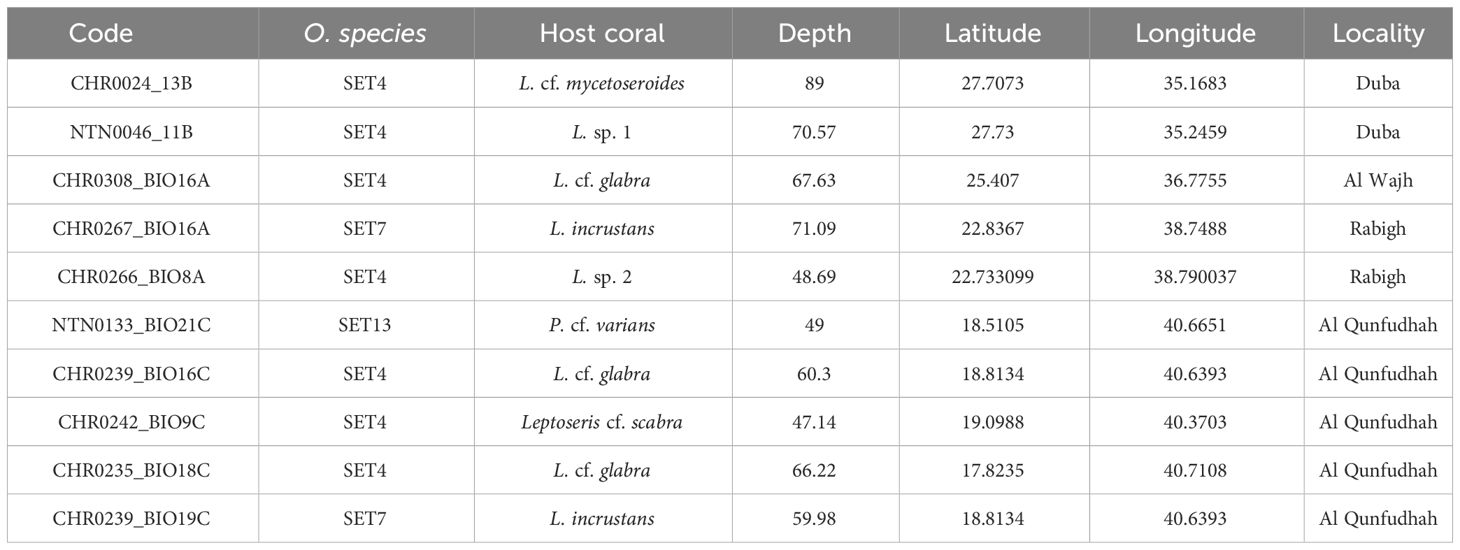
Table 1 List of the 10 Opecarcinus specimens collected in the Saudi Arabian Red Sea for this study, including the voucher code, species, host coral species, sampling depth, latitude, longitude, and locality.
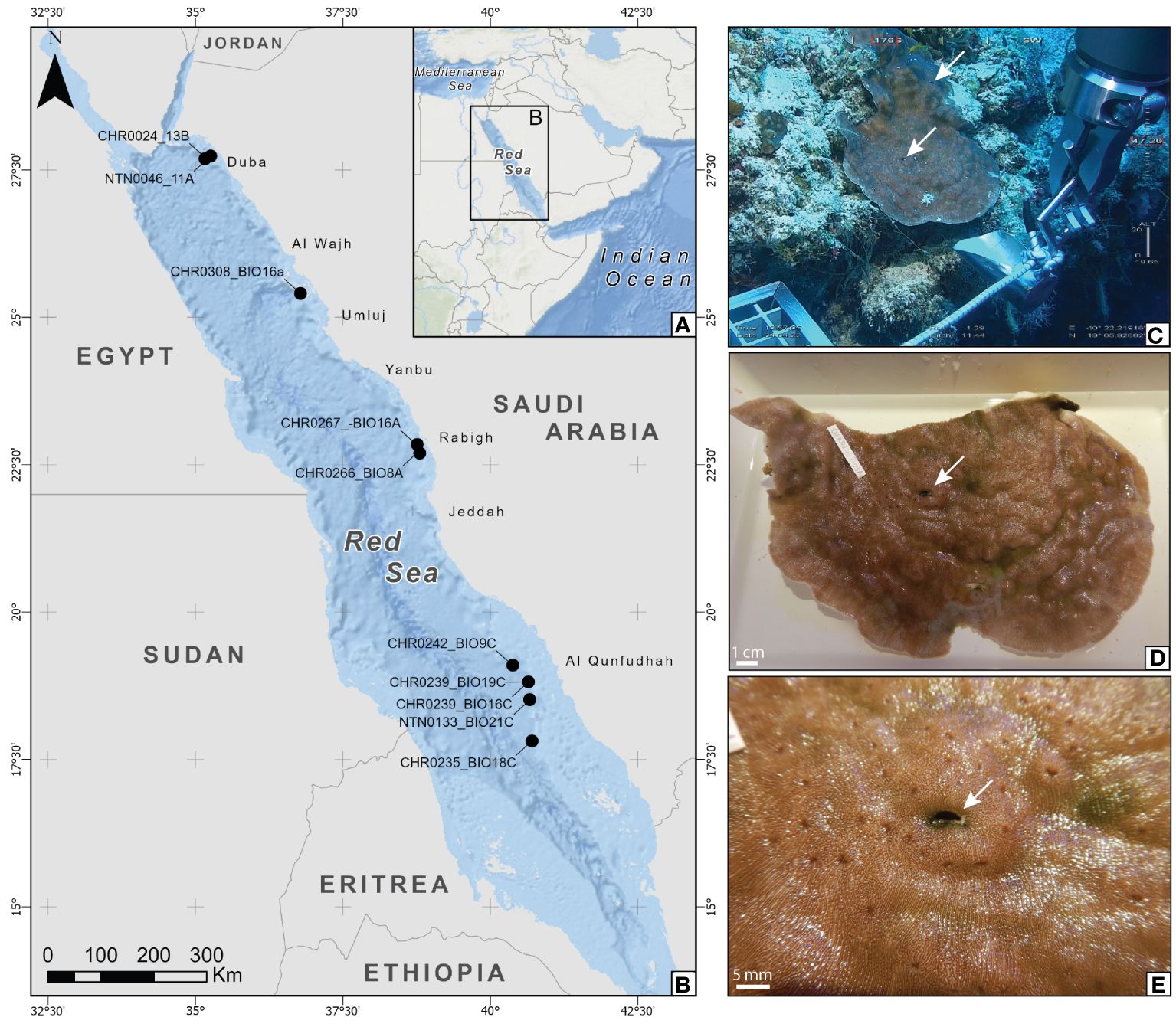
Figure 1 (A, B) Map of the Red Sea (A) showing the sampling area for this study (B) where the sampling site for each specimen is shown. CHR codes refer to ROV dives, and NTN code refers to submarine dives. (C–E) Sampling of CHR0242_BIO9C in association with Leptoseris cf. scabra at 47.14 m depth. (C) Sampling of the coral colony. (D, E) Gall crab (Opecarcinus SET4) dwelling in L. cf. scabra. The arrows indicate the dwellings. Map created using ArcGIS® software by Esri. ArcGIS® and ArcMap™ are the intellectual property of Esri and are used herein under license. Copyright © Esri. All rights reserved. For more information about Esri® software, please visit www.esri.com.
DNA extraction, amplification, and sequence analyses
High-quality DNA was extracted from the fifth pereiopod muscle using Dneasy ® Blood and Tissue kit (Qiagen Inc., Hilden, Germany) following the manufacturer’s protocol. DNA was amplified using the universal primers LCO1490/HCO2198 (Folmer et al., 1994) for the COI, the primers 16L2/16H10 (Schubart, 2009) for the 16S rRNA, and finally, the primers H3F/H3_R_SET (Colgan et al., 2000; van der Meij, 2015b) for the histone H3. The DNA amplification was performed in a 15 μL PCR volume of Multiplex Master Mix (Qiagen Inc., Hilden, Germany), 0.2 μL of each primer, and <0.1 ng of DNA. The PCR protocol for each primer followed the one of van der Meij (2015b). PCR samples were purified using Illustra™ Exostar™ 1-Step (GE Healthcare Life Sciences) following the manufacturer’s protocol and directly sequenced in forward and reverse directions using an ABI 3730xl DNA analyzer (Applied Biosystems, Foster City, CA, USA) at KAUST Bioscience Core Lab.
Forward and reverse sequences were assembled and edited using Geneious Prime 2019 (Biomatters), and manually checked using BioEdit Sequence Alignment Editor v7.2 (Hall, 1999). The phylogenetic analysis was performed on a concatenated dataset of the three genes (COI, 16S, and H3). Two sequences of Pseudohapalocarcinus ransoni were added to the alignment due to its phylogenetic position within the genus Opecarcinus (see Xu et al., 2022). Moreover, 2 sequences of Hapalocarcinus marsupialis sensu lato Stimpson, 1859, were used as outgroup (Supplementary Table S1), following van der Meij and Nieman (2016). All sequences were concatenated using Geneious Prime 2019 (Biomatters) and aligned using MAFFT 7.520 (Katoh et al., 2013) under the E-INS0i strategy. General statistics concerning the obtained sequences were calculated with MEGA X (Kumar et al., 2018) and DnaSP6.11.1 (Librado and Rozas, 2009) PartitionFinder 2 (Lanfear et al., 2017) was used to calculate the sequence evolution best-fit substitution model for Bayesian Inference (BI) and Maximum Likelihood (ML) and to perform a partitioning scheme comparison. We used linked branch lengths, the greedy search algorithm for nucleotide sequence, and considered 7 partitions: 16S, the three codon positions of COI, and the three codon positions of H3. PartitionFinder 2 selected for RaxML the evolutionary model GTR+G for COI_pos1, COI_pos2, H3_pos1, H3_pos2, and H3_pos2, and the model GTR+I+G for COI_pos3 and 16S. For MrBayes, the evolutionary models GTR+G for COI_pos1, COI_pos2, and H3_pos2, F81+I for COI_pos3, GTR+I+G for 16S, F81+G for H3_pos1, and GTR for H3_pos3 were selected. The BI and ML analyses were run using MrBayes v3.2.7a (Ronquist et al., 2012) and RaxML v8.2.12 (Stamatakis, 2014), respectively, on the CIPRES server (Miller et al., 2011). In particular, the BI analyses were performed using four Markov chain Monte Carlo chains for 10 million generations, saving a tree every 1000th generation. Tracer 1.7.1 (Rambaut et al., 2018) was employed at the end of the runs to verify effective sampling size and unimodal posterior distribution stationarity. The first 25% of trees sampled were discarded as burn-in. The ML topology was derived using default parameters on the CIPRES server, with a multiparametric bootstrap analysis of 1000 replicates.
Results
Phylogenetic analyses
In total, 23 sequences were obtained for COI (9), 16S (10), and H3 (4) markers (Supplementary Table S1). A total of 129 additional sequences for COI (55), 16S (41), and H3 (34) were retrieved from GenBank, based on previous molecular studies (van der Meij and Nieman, 2016; van Tienderen and van der Meij, 2016; Xu et al., 2022; Vimercati et al., 2023), and added to the alignment as reference sequences (Supplementary Table S1). Of these sequences, 31 were chosen from the Saudi Arabian Red Sea to cover the currently known Opecarcinus diversity from the basin (van der Meij and Nieman, 2016; Xu et al., 2022; Vimercati et al., 2023) (Supplementary Table S1). The final three loci concatenated alignment consisted of 1625 bp (625 bp for the COI, 646 bp for the 16S, and 354 bp for the H3), including 1210 conservative sites and 405 variable sites, of which 368 were parsimony-informative, and 36 were singletons. Moreover, DNAsp6 retrieved 281 mutations, not considering the 48 ambiguous bases that occurred in the GenBank sequences.
The phylogenetic tree reconstruction inferred from Bayesian Inference (BI) (Figure 2) included six of the nine previously described Opecarcinus species (Shaw and Hopkins, 1977; Kropp, 1989; van der Meij, 2014b) and 19 species reported by Xu et al. (2022), up to 15 of which are formally undescribed Opecarcinus species identified by preliminary codes, which are awaiting formal description (Xu et al., 2022; Vimercati et al., 2023; Xu et al., in prep). Pseudohapalocarcinus ransoni Fize & Serène, 1956, nested together with Opecarcinus, in agreement with previous reconstructions (van der Meij and Klaus, 2015; van der Meij and Nieman, 2016; Xu et al., 2022) (Figure 2). Moreover, coherently with Xu et al. (2022), material from the Red Sea was retrieved in 10 distinct clades, namely nine Opecarcinus and one of P. ransoni (Figure 2). The 10 mesophotic Red Sea specimens sampled in this study were recovered in 3 distinct and well-supported molecular lineages (Figure 2). In particular, seven gall crab specimens (one already published in Vimercati et al., 2023, and six from this study) (Supplementary Table S1) were retrieved with Opecarcinus SET4 (Xu et al., 2022) (Figure 2). Moreover, two mesophotic Red Sea specimens clustered together with the available sequences of Opecarcinus SET7 (Xu et al., 2022) (Figure 2). Finally, one specimen was recovered with Opecarcinus SET13 (Xu et al., 2022) (Figure 2).
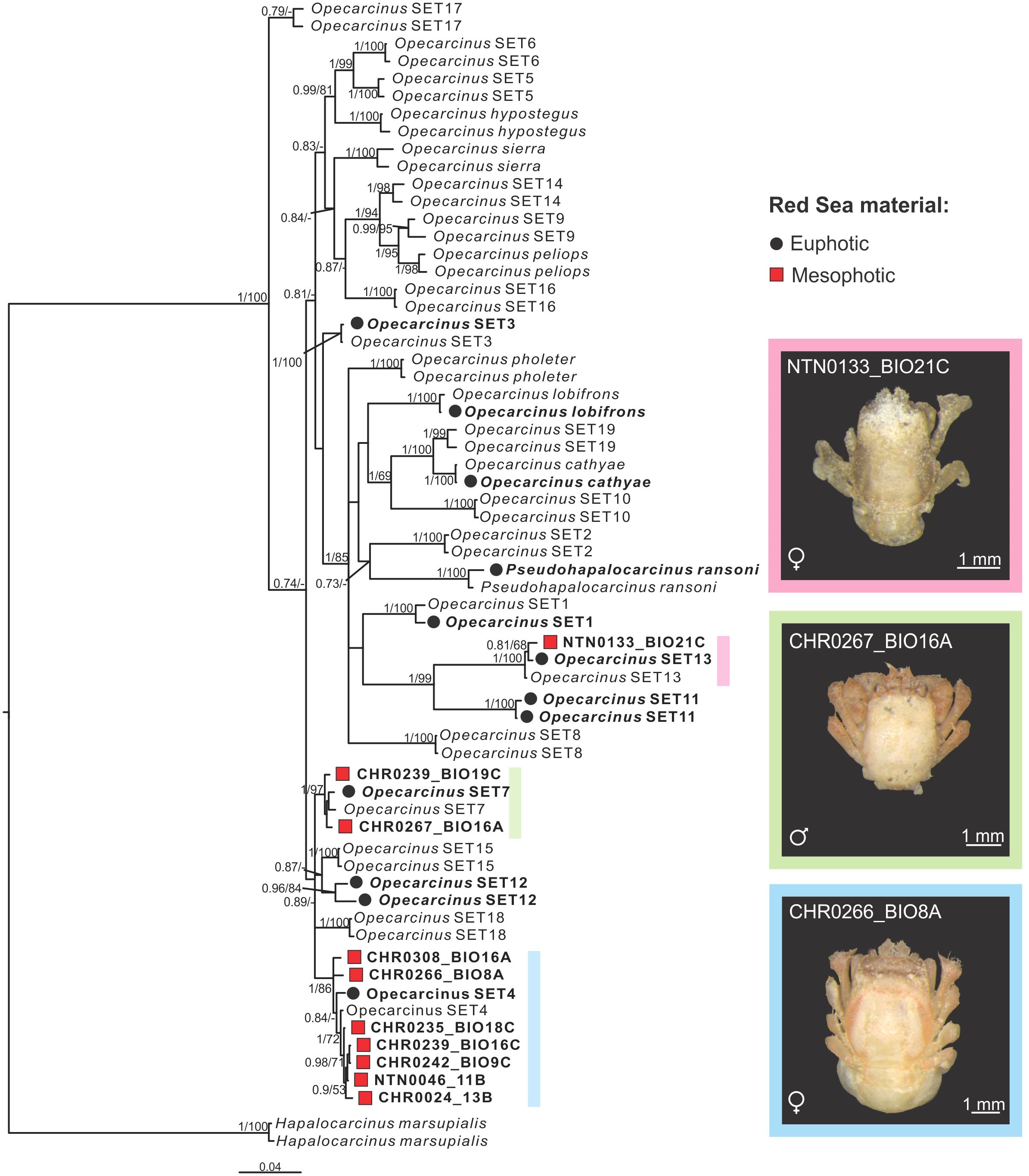
Figure 2 Mesophotic Opecarcinus phylogenetic reconstruction inferred from Bayesian Inference (BI) analysis of the combined mitochondrial (COI,16S) and nuclear (H3) datasets. Node values are posterior Bayesian Probability and Maximum Likelihood (ML) bootstrap values. Posterior Bayesian probabilities below 0.7 and ML bootstrap values below 50% are indicated by a dash (-). Red Sea samples are in bold. Black dots represent the currently known Opecarcinus species from the Red Sea (van der Meij and Nieman, 2016; Xu et al., 2022), while red squares highlight the mesophotic specimens collected for this study from the basin. Species without dots are representative of other Indo-Pacific localities. Hapalocarcinus marsupialis was used as an outgroup. Insert photographs by SV.
New locality, depth, and host records
For the first time, Opecarcinus SET4 was collected from Al Wajh and the Central Red Sea and Opecarcinus SET7 from the Central Red Sea (Table 1). Opecarcinus SET13 was sampled in the South Red Sea, where it has been previously reported inhabiting euphotic scleractinians (Xu et al., 2022) (Table 1).
The current Red Sea depth distribution range of Agariciidae-associated Opecarcinus is 89 m for Opecarcinus SET4 (Vimercati et al., 2023). In this work, we further extended the known depth distribution of Opecarcinus SET7 to 71 m depth and Opecarcinus SET13 to 49 m depth (Table 1).
Moreover, our study provides the first evidence of new host records for Opecarcinus in the Red Sea. In particular, Opecarcinus SET4 was sampled from dwellings in the coralla of different Leptoseris species, namely L. cf. mycetoseroides (Vimercati et al., 2023), Leptoseris cf. scabra Vaughan, 1907, Leptoseris sp. 1 (previously identified as Leptoseris cf. fragilis Milne Edwards & Haime, 1849, sensu Terraneo et al., 2017), Leptoseris sp. 2 (previously identified as Leptoseris sp. sensu Terraneo et al., 2017), and Leptoseris cf. glabra Dinesen, 1980 (Table 1). Opecarcinus SET7 was observed in association with Leptoseris incrustans (Quelch, 1986) (Table 1). Finally, Opecarcinus SET13 was retrieved on a mesophotic colony identified as Pavona cf. varians Verrill, 1864 (Table 1).
Discussion
During different recent research cruises from 2020 to 2022, we surveyed the Saudi Arabian mesophotic and deep reefs ranging from depths of 45 to 600 m, collecting 10 cryptochirid specimens at depths between 47 and 89 m (Figures 1A, B). Our phylogeny reconstruction analyses of the combined mitochondrial (COI, 16S) and nuclear (H3) datasets retrieved the collected material in 3 distinct and well-supported molecular clades, already published as O. SET4, O. SET7, O. SET13 by Xu et al. (2022). The records of mesophotic coral-dwelling gall crabs examined in this work significantly extend the previously known depth distribution range of Opecarcinus in the Red Sea. Moreover, the three species were found in association with previously unreported Agariciidae hosts, thus also allowing us here to extend the known host range for this gall crab genus.
Mesophotic Opecarcinus diversity and host coral abundance
Currently, the genus Opecarcinus includes at least 25 species, 9 of which are described (van der Meij, 2014b; DecaNet eds., 2023), up to 15 of which are currently devoid of a formal taxonomic description and identified by preliminary codes (Xu et al., 2022). Although it occurs in the tropical belt spanning the Atlantic and the Indo-Pacific regions (e.g., Scott, 1985, Scott, 1987; Kropp and Manning, 1987; Kropp, 1989; van der Meij, 2014b; Chan et al., 2020), most of the studies on its depth distribution focus on the Caribbean (van der Meij et al., 2015; van Tienderen and van der Meij, 2016) (Figure 3), with only 10 mesophotic records in the Indo-Pacific (two of them belonging to the genus Luciades) (Shen, 1936; Kropp and Manning, 1996; Komatsu and Takeda, 2013; Xu et al., 2022; Vimercati et al., 2023) (Figure 3). In particular, the single Atlantic Opecarcinus species follows the depth distribution of its host corals (van Tienderen and van der Meij, 2016). This cryptochirid species has a higher prevalence at greater depths (van Tienderen and van der Meij, 2016), where the coral genus Agaricia forms dense high-cover assemblages (Bongaerts et al., 2013, Bongaerts et al., 2015; Hoeksema et al., 2017). Hence, the Red Sea Opecarcinus mesophotic diversity reported in this study is unsurprising if we consider the associated host corals and their depth distribution and abundance in MCEs. In fact, Leptoseris is one of the most abundant scleractinian genera in MCEs (Fricke et al., 1987; Hinderstein et al., 2010; Rooney et al., 2010; Kahng et al., 2014, Kahng et al., 2017; Loya et al., 2019). The strict association between gall crabs and scleractinian corals and the cryptochirid host specificity at host species, genera, or families (e.g., van der Meij, 2015a, 2015b; van der Meij et al., 2015; Bähr et al., 2021; Xu et al., 2022; van der Schoot and Hoeksema, 2024) led to hypothesize that the number of host corals could predict the number of cryptochirids (van der Meij, 2015a; van der Schoot and Hoeksema, 2024). Moreover, Kohn and Leviten (1976) showed that habitat availability affects the abundance and diversity of coral-associated invertebrates. In this context, the high occurrence of the genus Leptoseris in the Red Sea MCEs could explain the mesophotic gall crab diversity found in this work.
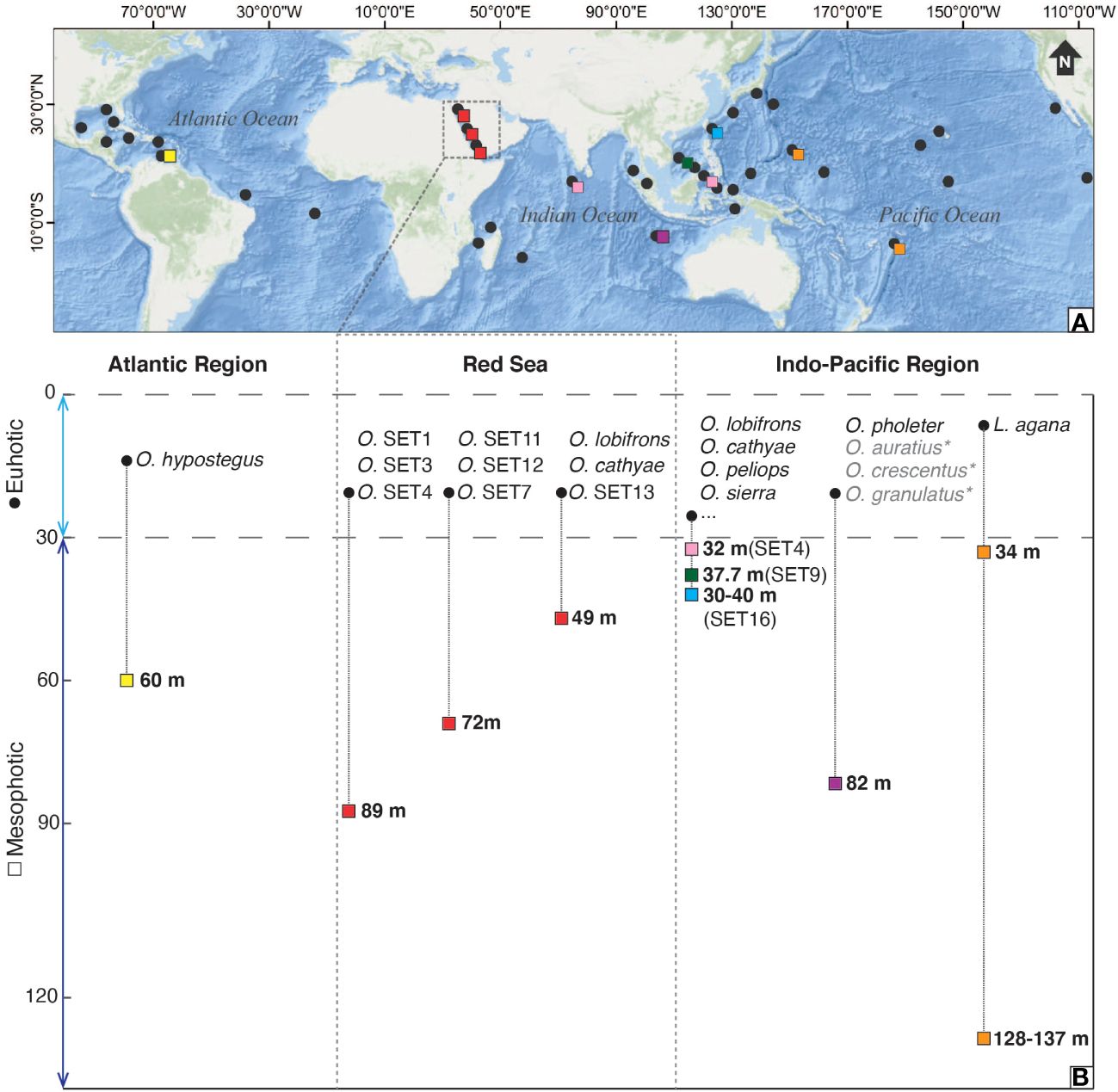
Figure 3 (A) Map of the Atlantic and Indo-Pacific Oceans (Red Sea included) showing the Opecarcinus and Luciades distribution in shallow waters (black dots) and the mesophotic deepest records in Curaçao (yellow square), in the Red Sea (red square), in Christmas Island (purple square), in Maldives and Lembeh (pink squares), in Spratly Islands (green square), in Taiwan (light blue square), and in Guam and Tonga (orange squares) based on literature distribution records (Kropp and Manning, 1987; Kropp, 1989; Kropp and Manning, 1996; Komatsu and Takeda, 2013; van der Meij et al., 2018; van Moorsel and van der Meij, 2018; Chan et al., 2020; Xu et al., 2022; Vimercati et al., 2023) and this study. (B) List of Opecarcinus and Luciades species with their photic (0-30 m) and mesophotic (~30-150 m) depth distribution in 3 main regions: the Atlantic Region, the Red Sea, and the Indo-Pacific Region. The formally undescribed Opecarcinus species identified by preliminary codes occurring in the Indo-Pacific (namely O. SET1-10, O. SET12-19) (Xu et al., 2022), are reported in (A) but not listed in (B), where they are indicated by three dots (…). Species in grey refer to the 3 Opecarcinus accepted species not analyzed molecularly in this study: Opecarcinus auratius Kropp, 1989, Opecarcinus crescentus (Edmondson, 1925), and Opecarcinus granulatus (Schen, 1936). Map created using ArcGIS® software by Esri. ArcGIS® and ArcMap™ are the intellectual property of Esri and are used herein under license. Copyright © Esri. All rights reserved. For more information about Esri® software, please visit www.esri.com.
New coral-gall crab associations in the Saudi Arabian Red Sea mesophotic zone
Unlike the Atlantic generalist gall crab species Troglocarcinus corallicola Verrill, 1908, most cryptochirids show host-specificity, inhabiting a single coral species or several closely related ones (Castro, 2015). In the Red Sea, Xu et al. (2022) reported Opecarcinus in association with 3 Agariciidae genera, namely Gardineroseris Scheer and Pillai, 1974, Pavona, and Leptoseris. Coral identification was mostly at the genus level, possibly due to the objective challenges posed by the unresolved taxonomic scenario of the family Agariciidae (Luck et al., 2013; Terraneo et al., 2017; Benzoni, 2022). In this study, most of the coral specimens collected didn’t match the morphology of any previously described nominal species. Consequently, we here report on new coral-gall crab associations from the Red Sea mesophotic zone, with the coral hosts identified at the species level for the first time (Table 1). Moreover, the genus Pavona has previously been reported down to 52 m in the Red Sea (Fricke and Schuhmacher, 1983; Scheer and Pillai, 1983), but our study marks the first record of a gall crab-Pavona association at a depth below 30 m.
MCEs Opecarcinus diversity in the Saudi Arabian Red Sea: new insights and implications for conservation
To date, in the Red Sea, specialists have recorded the occurrence of more than 40 cryptochirid species in association with scleractinian hosts (van der Meij, 2015a; van der Meij et al., 2018; Bähr et al., 2021; Xu et al., 2022), with the Red Sea identified as a center of biodiversity for gall crabs, second only to the Coral Triangle (van der Meij, 2015a; Bravo et al., 2021). For this work, 10 cryptochirid specimens were collected at depths between 47 and 89 m (Figures 1A, B), thus reporting unknown cryptochirid biodiversity at these depths (Figure 3). Moreover, half of them were retrieved from the South Red Sea (Table 1) (Figures 1A, B). A previous study on Red Sea shallow waters reported a higher cryptochirid abundance in the South compared to the central part of the basin, suggesting that the South’s high productivity could facilitate the settlement of coral-dwelling crabs (van der Meij, 2015a). However, in our study, the difference in distribution could be related to a very limited number of specimens collected compared to other studies focusing on shallow water (see van der Meij, 2015a; Xu et al., 2022). In this context, it is important to highlight that assessing the mesophotic coral associates’ diversity and distribution poses significant challenges due to the logistical difficulties and costs associated with mesophotic explorations (Hinderstein et al., 2010; Berumen et al., 2013, 2019). However, different studies focused on the importance of increasing our knowledge of coral-associated invertebrates (see Stella et al., 2011, for an overview; Hoeksema, 2017; Montano, 2020, Bravo et al., 2021; Montano, 2022), in light of current global and local threats coral reefs are facing (Hughes et al., 2003, Hughes et al., 2017; Hoegh-Guldberg et al., 2007; Burke et al., 2011; Cinner et al., 2016). Given the limited knowledge of reef-affiliated invertebrates, it is, thus, crucial to continue to investigate MCEs’ biodiversity to enhance our understanding of these low-light environments and to implement more effective conservation measures.
Conclusions
In conclusion, this study represents the first assessment of the diversity and distribution of the genus Opecarcinus in mesophotic corals along the Saudi Arabian Red Sea. We collected 10 specimens along the basin, with half of them sampled in the South Red Sea. We found 3 depth generalist species currently under taxonomic description, reconfirming that the genus Opecarcinus host specificity influences the depth range (Vimercati et al., 2023). Further investigation of mesophotic reefs is necessary to understand the role of depth on reef-affiliated invertebrates and to unravel the mesophotic diversity and depth zonation of cryptic reef-associated taxa, such as the gall crabs. Moreover, it is crucial to deepen our understanding of cryptochirids concerning their bathymetrical ranges and phylogenetic relationships of their host taxa to comprehend the evolutionary history of these mesophotic associations (García-Hernández et al., 2020) and to understand the role they play in coral reef ecosystems (Stella et al., 2011), in light of the current climate change scenario and consequent reef degradation.
Data availability statement
The sequence data supporting this study’s findings is openly available in GenBank of NCBI at https://www.ncbi.nlm.nih.gov/genbank, under accession numbers listed in Table S1.
Ethics statement
The manuscript presents research on animals that do not require ethical approval for their study.
Author contributions
SV: Conceptualization, Data curation, Formal analysis, Investigation, Methodology, Software, Validation, Visualization, Writing – original draft, Writing – review & editing. TT: Conceptualization, Supervision, Validation, Writing – review & editing. FM: Writing – review & editing. AA: Data curation, Funding acquisition, Project administration, Resources, Writing – review & editing. MR: Data curation, Resources, Writing – review & editing. VP: Data curation, Funding acquisition, Project administration, Resources, Writing – review & editing. MQ: Funding acquisition, Project administration, Resources, Writing – review & editing. CD: Funding acquisition, Project administration, Resources, Writing – review & editing. FB: Conceptualization, Data curation, Funding acquisition, Resources, Supervision, Validation, Project administration, Writing – review & editing.
Funding
The author(s) declare financial support was received for the research, authorship, and/or publication of this article. This work was supported by funding from NEOM, National Center for Wildlife (NCW). Research reported in this publication was supported by the King Abdullah University of Science and Technology (KAUST) (award #FCC/1/1973-50-01 and baseline to F.B., BAS/1/1090-01-01).
Acknowledgments
We thank NEOM for facilitating and coordinating the Red Sea Deep Blue expedition and, specifically, in addition to A. A. E., Thamer Habis, Justin Myner, Paul Marshall, Giacomo Palavicini, Peter Mackelworth, and Abdulaziz Alghamdi. We thank the National Center for Wildlife (NCW), Mohammed Qurban, Carlos M. Duarte, Jennifer E. Thompson, and Naira C. Pluma Guerrero for facilitating and coordinating the Red Sea Decade Expedition. We want to thank OceanX and the crew of OceanXplorer for their operational and logistical support for the duration of this expedition. In particular, we would like to acknowledge the ROV and submersible teams for sample collection and OceanX for support of scientific operations on board OceanXplorer. We also thank OceanX Media for documenting and communicating this work with the public. We also wish to thank Natalie Dunn and Susanne Bahr for contributing to part of the sampling efforts and Megan Nolan for helping provide the map in Figure 3. Finally, we thank the KAUST Bioscience Core Lab for helping with the Sanger Sequencing.
Conflict of interest
The authors declare that the research was conducted in the absence of any commercial or financial relationships that could be construed as a potential conflict of interest.
Publisher’s note
All claims expressed in this article are solely those of the authors and do not necessarily represent those of their affiliated organizations, or those of the publisher, the editors and the reviewers. Any product that may be evaluated in this article, or claim that may be made by its manufacturer, is not guaranteed or endorsed by the publisher.
Supplementary material
The Supplementary Material for this article can be found online at: https://www.frontiersin.org/articles/10.3389/fmars.2024.1305396/full#supplementary-material
References
Alamaru A., Loya Y., Brokovich E., Yam R., Shemesh A. (2009). Carbon and nitrogen utilization in two species of Red Sea corals along a depth gradient: Insights from stable isotope analysis of total organic material and lipids. Geochim. Cosmochim. Acta 73, 5333–5342. doi: 10.1016/j.gca.2009.06.018
Augustin N., van der Zwan F. M., Devey C. W., Brandsdóttir B. (2021). 13 million years of seafloor spreading throughout the Red Sea Basin. Nat. Commun. 12, 2427. doi: 10.1038/s41467-021-22586-2
Bähr S., Johnson M. L., Berumen M. L., Hardenstine R. S., Rich W. A., van der Meij S. E. (2021). Morphology and reproduction in the Hapalocarcinus marsupialis Stimpson 1859 species complex (Decapoda: Brachyura: Cryptochiridae). J. Crustac. Biol. 41, ruab052. doi: 10.1093/jcbiol/ruab052
Benzoni F. (2022). Re-discovery of the type material of Leptoseris fragilis (Cnidaria, Anthozoa, Scleractinia) from the upper mesophotic in La Réunion, a taxonomic puzzle. Zootaxa 5178, 596–600. doi: 10.11646/zootaxa.5178.6.8
Berumen M. L., Hoey A. S., Bass W. H., Bouwmeester J., Catania D., Cochran J. E. M., et al. (2013). The status of coral reef ecology research in the Red Sea. Coral Reefs 32, 737–748. doi: 10.1007/s00338-013-1055-8
Berumen M. L., Voolstra C. R., Daffonchio D., Agusti S., Aranda M., Irigoien X., et al. (2019). “The Red Sea: environmental gradients shape a natural laboratory in a nascent ocean,” in Coral Reefs of the Red Sea. Eds. Voolstra C., Berumen M. (Springer, Cham), 1–10. doi: 10.1007/978-3-030-05802-9_1
Bonatti E., Cipriani A., Lupi L. (2015). “The Red Sea: birth of an ocean,” in The Red Sea: The Formation, Morphology, Oceanography and Environment of a Young Ocean Basin. Eds. Rasul N. M. A., Stewart I. C. F. (Springer Earth System Sciences, Berlin Heidelberg), 29–44. doi: 10.1007/978-3-662-45201-1_2
Bongaerts P., Frade P. R., Hay K. B., Englebert N., Latijnhouwers K. R., Bak R. P., et al. (2015). Deep down on a Caribbean reef: lower mesophotic depths harbor a specialized coral-endosymbiont community. Sci. Rep. 5, 7652. doi: 10.1038/srep07652
Bongaerts P., Frade P. R., Ogier J. J., Hay K. B., Van Bleijswijk J., Englebert N., et al. (2013). Sharing the slope: depth partitioning of agariciid corals and associated Symbiodinium across shallow and mesophotic habitats (2-60 m) on a Caribbean reef. BMC Evol. Biol. 13, 205. doi: 10.1186/1471-2148-13-205
Bravo H., Xu T., van der Meij S. E. T. (2021). “Conservation of Coral-Associated Fauna,” in Earth Systems and Environmental Sciences (Elsevier). doi: 10.1016/B978-0-12-821139-7.00121-5
Burke L., Reytar K., Spalding M., Perry A. (2011). Reefs at risk revisited (Washington, DC: World Resources Institute).
Castro P. (2015). ““Symbiotic brachyura,“,” in Decapoda: Brachyura, Treatise on Zoology – Anatomy, Taxonomy, Biology, vol. 9C-I . Eds. Castro P., Davie P., Guinot D., Schram R., von Vaupel Klein J. C. (Drill, Leiden: Boston), 543–581. doi: 10.1163/9789004190832_012
Chaidez V., Dreano D., Agusti S., Duarte C. M., Hoteit I. (2017). Decadal trends in Red Sea maximum surface temperature. Sci. Rep. 7, 8144. doi: 10.1038/s41598-017-08146-z
Chan B. K., Wong K. J., Cheng Y. R., Thiel M., Poore G. (2020). “Biogeography and host usage of coral-associated crustaceans: barnacles, copepods, and gall crabs as model organisms,” in The Natural History of the Crustacea: Evolution and Biogeography of the Crustacea, vol. 8 . Eds. Thiel M., Poore G. C. (Oxford, UK, Oxford University Press), 183–215.
Chimienti G., Terraneo T. I., Vicario S., Marchese F., Purkis S. J., Eweida A. A., et al. (2022). A new species of Bathypathes (Cnidaria, Anthozoa, Antipatharia, Schizopathidae) from the Red Sea and its phylogenetic position. ZooKeys 1116, 1–22. doi: 10.3897/zookeys.1116.79846
Cinner J. E., Huchery C., MacNeil M. A., Graham N. A., McClanahan T. R., Maina J., et al. (2016). Bright spots among the world’s coral reefs. Nature 535, 416–419. doi: 10.1038/nature18607
Colgan D. J., Ponder W. F., Eggler P. E. (2000). Gastropod evolutionary rates and phylogenetic relationships assessed using partial 28S rDNA and histone H3 sequences. Zool. Scr. 29, 29–63. doi: 10.1046/j.1463-6409.2000.00021.x
DecaNet (2023). Opecarcinus Kropp & Manning 1987 (World Register of Marine Species). Available at: https://www.marinespecies.org/aphia.php?p=taxdetails&id=240955. DecaNet.
DiBattista J. D., Howard Choat J., Gaither M. R., Hobbs J. P. A., Lozano-Cortés D. F., Myers R. F., et al. (2016b). On the origin of endemic species in the Red Sea. J. Biogeogr. 43, 13–30. doi: 10.1111/jbi.12631
DiBattista J. D., Roberts M. B., Bouwmeester J., Bowen B. W., Coker D. J., Lozano-Cortés D. F., et al. (2016a). A review of contemporary patterns of endemism for shallow water reef fauna in the Red Sea. J. Biogeogr. 43, 423–439. doi: 10.1111/jbi.12649
Einbinder S., Mass T., Brokovich E., Dubinsky Z., Erez J., Tchernov D. (2009). Changes in morphology and diet of the coral Stylophora pistillata along a depth gradient. Mar. Ecol. Prog. Ser. 381, 167–174. doi: 10.3354/meps07908
Folmer O., Black M., Hoeh W., Lutz R., Vrijenhoek A. R. (1994). Conserved primers for PCR amplification of mitochondrial DNA from different invertebrate phyla. Mol. Mar. Biol. Biotechnol. 3, 294–299.
Fricke H., Hottinger L. (1983). Coral bioherms below the euphotic zone in the Red Sea. Mar. Ecol. Prog. Ser. 11, 113–117. doi: 10.3354/meps011113
Fricke H. W., Knauer B. (1986). Diversity and spatial pattern of coral communities in the Red Sea upper twilight zone. Oecologia 71, 29–37. doi: 10.1007/BF00377316
Fricke H. W., Schuhmacher H. (1983). The depth limits of Red Sea stony corals: an ecophysiological problem (a deep diving survey by submersible). Mar. Ecol. 4, 163–194. doi: 10.1111/j.1439-0485.1983.tb00294.x
Fricke H. W., Vareschi E., Schlichter D. (1987). Photoecology of the coral Leptoseris fragilis in the Red Sea twilight zone (an experimental study by submersible). Oecologia 73, 371–381. doi: 10.1007/BF00385253
García-Hernández J. E., de Gier W., van Moorsel G. W., Hoeksema B. W. (2020). The scleractinian Agaricia undata as a new host for the coral-gall crab Opecarcinus hypostegus at Bonaire, southern Caribbean. Symbiosis 81, 303–311. doi: 10.1007/s13199-020-00706-8
Hall T. A. (1999). BioEdit: a user-friendly biological sequence alignment editor and analysis program for Windows 95/98/NT. Nucleic Acids Symp. Ser. 41, 95–98.
Hinderstein L. M., Marr J. C. A., Martinez F. A., Dowgiallo M. J., Puglise K. A., Pyle R. L., et al. (2010). Theme section on “Mesophotic coral ecosystems: characterization, ecology, and management”. Coral Reefs 29, 247–251. doi: 10.1007/s00388-010-0614-5
Hoegh-Guldberg O., Mumby P. J., Hooten A. J., Steneck R. S., Greenfield P., Gomez E., et al. (2007). Coral reefs under rapid climate change and ocean acidification. Science 318, 1737–1742. doi: 10.1126/science.1152509
Hoeksema B. W. (2017). The hidden biodiversity of tropical coral reefs. Biodiversity 18, 8–12. doi: 10.1080/14888386.2017.1307787
Hoeksema B. W., Bongaerts P., Baldwin C. C. (2017). High coral cover at lower mesophotic depths: a dense Agaricia community at the leeward side of Curaçao, Dutch Caribbean. Mar. Biodivers. 47, 67–70. doi: 10.1007/s12526-015-0431-8
Hoeksema B. W., van der Meij S. E. T. (2013). Gall crab city: an aggregation of endosymbiotic crabs inhabiting a colossal colony of Pavona clavus. Coral Reefs 32, 59. doi: 10.1007/s00338-012-0954-4
Hughes T. P., Baird A. H., Bellwood D. R., Card M., Connolly S. R., Folke C., et al. (2003). Climate change, human impacts, and the resilience of coral reefs. Science 301, 929–933. doi: 10.1126/science.10850
Hughes T. P., Bellwood D. R., Connolly S. R. (2002). Biodiversity hotspots, centres of endemicity, and the conservation of coral reefs. Ecol. Lett. 5, 775–784. doi: 10.1046/j.1461-0248.2002.00383.x
Hughes T. P., Kerry J. T., Álvarez-Noriega M., Álvarez-Romero J. G., Anderson K. D., Baird A. H., et al. (2017). Global warming and recurrent mass bleaching of corals. Nature 543, 373–377. doi: 10.1038/nature21707
Kahng S. E., Copus J. M., Wagner D. (2014). Recent advances in the ecology of mesophotic coral ecosystems (MCEs). Curr. Opin. Environ. Sustain 7, 72–81. doi: 10.1016/j.cosust.2013.11.019
Kahng S., Copus J. M., Wagner D. (2017). “Mesophotic coral ecosystems,” in Marine Animal Forests: The Ecology of Benthic Biodiversity Hotspots. Eds. Rossi S., Bramanti L., Gori A., Orejas C. (Springer International Publishing, New York, NY), 185–206. doi: 10.1007/978-3-319-21012-4_4
Katoh K., Standley D. M. (2013). MAFFT multiple sequence alignment software version 7: improvements in performance and usability. Mol. Biol. Evol. 30, 772–780. doi: 10.1093/molbev/mst010
Kohn A. J., Leviten P. J. (1976). Effect of habitat complexity on population density and species richness in tropical intertidal predatory gastropod assemblages. Oecologia 25, 199–210. doi: 10.1007/BF00345098
Komatsu H., Takeda M. (2013). Second record of Luciades agana Kropp and Manning 1996 (Crustacea, Decapoda, Cryptochiridae) from Tonga, South Pacific. Bull. Natl. Mus. Nat. Sci. Ser. A 39, 11–14.
Kropp R. K. (1989). A revision of the Pacific species of gall crabs, genus Opecarcinus (Crustacea: Cryptochiridae). Bull. Mar. Sci. 45, 98–129.
Kropp R. K., Manning R. B. (1987). The Atlantic gall crabs, family Cryptochiridae (Crustacea: Decapoda: Brachyura). Smithson. Contrib. Zool 462, 1–21. doi: 10.5479/si.00810282.462
Kropp R. K., Manning R. B. (1996). Crustacea Decapoda: two new genera and species of deep water gall crabs from the Indo-west Pacific (Cryptochiridae). Résultats Des. Campagnes MUSORSTOM 15, 531–539.
Kumar S., Stecher G., Li M., Knyaz C., Tamura K. (2018). MEGA X: molecular evolutionary genetics analysis across computing platforms. Molecul. Biol. Evol. 35, 1547–1549. doi: 10.30651/jkm.v4i2.2203
Lanfear R., Frandsen P. B., Wright A. M., Senfeld T., Calcott B. (2017). PartitionFinder 2: new methods for selecting partitioned models of evolution for molecular and morphological phylogenetic analyses. Mol. Biol. Evol. 34, 772–773. doi: 10.1093/molbev/msw260
Librado P., Rozas J. (2009). DnaSP v5: a software for comprehensive analysis of DNA polymorphism data. Bioinformatics 25, 1451–1452. doi: 10.1093/bioinformatics/btb187
Ligi M., Bonatti E., Bortoluzzi G., Cipriani A., Cocchi L., Caratori Tontini F., et al. (2012). Birth of an ocean in the Red Sea: initial pangs. Geochemistry Geophys. Geosystems 13, Q08009. doi: 10.1029/2012GC004155
Loya Y., Puglise K. A., Bridge T. C. (2019). Mesophotic coral ecosystems Vol. 12 (New York: Springer). doi: 10.1007/978-3-319-92735-0
Luck D. G., Forsman Z. H., Toonen R. J., Leicht S. J., Kahng S. E. (2013). Polyphyly and hidden species among Hawai‘i’s dominant mesophotic coral genera, Leptoseris and Pavona (Scleractinia: Agariciidae). PeerJ 1, e132. doi: 10.7717/peerj.132
Manasrah R., Abu-Hilal A., Rasheed M. (2019). “Physical and chemical properties of seawater in the Gulf of Aqaba and Red Sea,” in Oceanographic and Biological Aspects of the Red Sea. Eds. Rasul N. M. A., Stewart I. C. F. (Springer, Cham), 41–73. doi: 10.1007/978-3-319-99417-8_3
Manning R. B. (1991). Crustacea Decapoda: Cecidocarcinus zibrowii, a new deep-water gall crab (Cryptochiridae) from New Caledonia. Résultats Des. Campagnes MUSORSTOM 9, 515–520.
Mass T., Einbinder S., Brokovich E., Shashar N., Vago R., Erez J., et al. (2007). Photoacclimation of Stylophora pistillata to light extremes: metabolism and calcification. Mar. Ecol. Prog. Ser. 334, 93–102. doi: 10.3354/meps334093
Miller M. A., Pfeiffer W., Schwartz T. (2011). “The CIPRES science gateway: a community resource for phylogenetic analyses,” in Proceedings of the 2011 TeraGrid Conference: extreme digital discovery. (Association for Computing Machinery), 1–8. doi: 10.1145/2016741
Montano S. (2020). The extraordinary importance of coral-associated fauna. Diversity 12, 357. doi: 10.3390/d12090357
Montano S. (2022). Diversity of coral-associated fauna: An urgent call for research. Diversity 14, 765. doi: 10.3390/d14090765
Nir O., Gruber D. F., Einbinder S., Kark S., Tchernov D. (2011). Changes in scleractinian coral Seriatopora hystrix morphology and its endocellular Symbiodinium characteristics along a bathymetric gradient from shallow to mesophotic reef. Coral Reefs 30, 1089–1100. doi: 10.1007/s00338-011-0801-z
Nogueira M. M., Menezes N. M., Johnsson R., Neves E. (2014). The adverse effects of cryptochirid crabs (Decapoda: Brachyura) on Siderastrea stellata Verril 1868 (Anthozoa: Scleractinia): causes and consequences of cavity establishment. Cah. Biol. Mar. 55, 155–162.
Obura D. (2012). The diversity and biogeography of Western Indian Ocean reef-building corals. PloS One 7, e45013. doi: 10.1371/journal.pone.0045013
Pyle R. L., Copus J. M. (2019). “Mesophotic coral ecosystems: Introduction and overview,” in Mesophotic Coral Ecosystems. Eds. Loya Y., Puglise K. A., Bridge T. C. L. (Springer, New York, NY, USA), 3–27. doi: 10.1007/978-3-319-92735-0_1
Raitsos D. E., Pradhan Y., Brewin R. J., Stenchikov G., Hoteit I. (2013). Remote sensing the phytoplankton seasonal succession of the Red Sea. PloS One 8, e64909. doi: 10.1371/journal.pone.0064909
Rambaut A., Drummond A. J., Xie D., Baele G., Suchard M. A. (2018). Posterior summarization in Bayesian phylogenetics using Tracer 1.7. Syst. Biol. 67, 901–904. doi: 10.1093/sysbio/syy032
Rasul N. M. A., Stewart I. C. F., Nawab Z. A. (2015). “Introduction to the Red Sea: its origin, structure, and environment,” in The Red Sea: The Formation, Morphology, Oceanography and Environment of a Young Ocean Basin. Eds. Rasul N. M. A., Stewart I. C. F. (Springer Berlin Heidelberg, Berlin), 1–28.
Roder C., Berumen M. L., Bouwmeester J., Papathanassiou E., Al-Suwailem A., Voolstra C. R. (2013). First biological measurements of deep-sea corals from the Red Sea. Sci. Rep. 3, 2802. doi: 10.1038/srep02802
Ronquist F., Teslenko M., van der Mark P., Ayres D. L., Darling A., Höhna S., et al. (2012). MrBayes 3.2: efficient Bayesian phylogenetic inference and model choice across a large model space. Syst. Biol. 61, 539–542. doi: 10.1093/sysbio/sys029
Rooney J., Donham E., Montgomery A., Spalding H., Parrish F., Boland R., et al. (2010). Mesophotic coral ecosystems in the Hawaiian Archipelago. Coral Reefs 29, 361–367. doi: 10.1007/s00338-010-0596-3
Rowlands G., Purkis S., Bruckner A. (2014). Diversity in the geomorphology of shallow-water carbonate depositional systems in the Saudi Arabian Red Sea. Geomorphology 222, 3–13. doi: 10.1016/j.geomorph.2014.03.014
Rowlands G., Purkis S., Bruckner A. (2016). Tight coupling between coral reef morphology and mapped resilience in the Red Sea. Mar. pollut. Bull. 105, 575–585. doi: 10.1016/j.marpolbul.2015.11.027
Schlichter D., Fricke H. W., Weber W. (1986). Light harvesting by wavelength transformation in a symbiotic coral of the Red Sea twilight zone. Mar. Biol. 91, 403–407. doi: 10.1007/BF00428634
Schubart C. D. (2009). “Mitochondrial DNA and Decapod Phylogenies: The Importance of Pseudogenes and Primer Optimization,” in Crustacean Issues 18: Decapod Crustacean Phylogenetics. Eds. Martin J. W., Crandall K. A., Felder D. L. (Taylor & Francis/CRC Press, Boca Raton, Florida), 47–65.
Scott P. J. B. (1985). Aspects of living coral associates in Jamaica. Proc. 5th Int. Coral Reef Congr. 5, 345–350.
Scott P. J. B. (1987). Associations between corals and macro-infaunal invertebrates in Jamaica, with a list of Caribbean and Atlantic coral associates. Bull. Mar. Sci. 40, 271–286.
Shaw J. K., Hopkins T. S. (1977). The distribution of the family Hapalocarcinidae (Decapoda, Brachyura) on the Florida middle ground. Proc. 3d Internatl. Coral Reef Symp. 1, 177–183.
Shen C. J. (1936). Notes on the family Hapalocarcinidae (Coral-infesting crabs) with the description of two new species. Hong Kong Naturalist. 5, 21–26.
Sheppard C., Price A., Roberts C. (1992). Marine Ecology of the Arabian Region: Patterns and Processes in Extreme Tropical Environments (London: Academic Press).
Simon-Blecher N., Achituv Y. (1997). Relationship between the coral pit crab Cryptochirus coralliodytes Heller and its host coral. J. Exp. Mar. Biol. Ecol. 215, 93–102. doi: 10.1016/S0022-0981(97)00002-6
Sofianos S. S., Johns W. E. (2003). An oceanic general circulation model (OGCM) investigation of the Red Sea circulation: 2. Three-dimensional circulation in the Red Sea. J. Geophys. Res. 527 Oceans 108, 3066. doi: 10.1029/2001JC001184
Stamatakis A. (2014). RAxML version 8: a tool for phylogenetic analysis and post-analysis of large phylogenies. Bioinform. 30, 1312–1313. doi: 10.1093/bioinformatics/btu033
Stambler N., Levy O., Vaki L. (2008). Photosynthesis and respiration of hermatypic zooxanthellate Red Sea corals from 5-75-m depth. Isr. J. Plant Sci. 56, 45–53. doi: 10.1560/IJPS.56.1-2.45
Stella J., Pratchett M., Hutchings P., Jones G. (2011). Coral-associated invertebrates. Oceanogr. Mar. Biol.: Annu. Rev. 49, 43–104. doi: 10.1201/b11009-3
Terraneo T. I., Arrigoni R., Benzoni F., Tietbohl M. D., Berumen M. L. (2017). Exploring the genetic diversity of shallow-water Agariciidae (Cnidaria: Anthozoa) from the Saudi Arabian Red Sea. Mar. Biodivers. 47, 1065–1078. doi: 10.1007/s12526-017-0722-3
Terraneo T. I., Ouhssain M., Castano C. B., Aranda M., Hume B. C., Marchese F., et al. (2023). From the shallow to the mesophotic: a characterization of Symbiodiniaceae diversity in the Red Sea NEOM region. Front. Mar. Sci. 10. doi: 10.3389/fmars.2023.1077805
van der Meij S. E. T. (2014b). A new species of Opecarcinus Kropp & Manning 1987 (Crustacea: Brachyura: Cryptochiridae) associated with the stony corals Pavona clavus (Dana 1846) and P. bipartita Nemenzo 1980 (Scleractinia: Agariciidae). Zootaxa 3869, 44–52. doi: 10.11646/zootaxa.3869.1.4
van der Meij S. E. T. (2015a). Evolutionary diversification of coral-dwelling gall crabs (Cryptochiridae). [PhD thesis]. Naturalis Biodiversity Center, Faculty of Science, Leiden University.
van der Meij S. E. T. (2015b). Host relations and DNA reveal a cryptic gall crab species (Crustacea: Decapoda: Cryptochiridae) associated with mushroom corals (Scleractinia: Fungiidae). Contrib. Zool. 84, 39–57. doi: 10.1163/18759866-08401004
van der Meij S. E. T., Benzoni F., Berumen M. L., Naruse T. (2018). New distribution records of the gall crab Opecarcinus cathyae van der Meij 2014 (Decapoda: Brachyura: Cryptochiridae) from the Red Sea, Maldives and Japan. Mar. Biodivers. 48, 1611–1613. doi: 10.1007/s12526-016-0598-7
van der Meij S. E. T., Klaus S. (2015). Origin and diversification of coral-dwelling 1073 gall crabs (Decapoda: Cryptochiridae). [PhD thesis]. (Naturalis Biodiversity Center, Faculty of Science, Leiden University), 79–86.
van der Meij S. E. T., Nieman A. M. (2016). Old and new DNA unweave the phylogenetic position of the eastern Atlantic gall crab Detocarcinus balssi (Monod 1956) (Decapoda: Cryptochiridae). J. Zoolog. Syst. Evol. Res. 54, 189–196. doi: 10.1111/jzs.12130
van der Meij S. E. T., Van Tienderen K. M., Hoeksema B. W. (2015). A mesophotic record of the gall crab Opecarcinus hypostegus from a Curaçaoan reef. Bull. Mar. Sci. 91, 205–206. doi: 10.5343/bms.2014.1072
van der Schoot R. J., Hoeksema B. W. (2024). Host specificity of coral-associated fauna and its relevance for coral reef biodiversity. Int. J. Parasitol. 54, 65–88. doi: 10.1016/j.ijpara.2023.09.002
van Moorsel G. W. N., van der Meij S. E. T. (2018). The stony coral Agaricia tenuifolia Dana 1848 as a new gall crab host (Decapoda: Cryptochiridae). Nauplius 26, e2018002. doi: 10.1590/2358-2936e2018002
van Tienderen K. M., van der Meij S. E. T. (2016). Occurrence patterns of coral-dwelling gall crabs (Cryptochiridae) over depth intervals in the Caribbean. PeerJ 4, e1794. doi: 10.7717/peerj.1794
Veron J. E. N. (1995). Corals in space and time: the biogeography and evolution of the Scleractinia (Ithaca, New York: Cornell University Press).
Vimercati S., van der Meij S. E. T., Terraneo T. I., Chimienti G., Marchese F., Eweida A. A., et al. (2023). A red sea depth record of the coral-dwelling crab opecarcinus (Decapoda: cryptochiridae) in the mesophotic zone. Diversity 15, 723. doi: 10.3390/d15060723
Xu T., Bravo H., Paulay G., van der Meij S. E. T. (2022). Diversification and distribution of gall crabs (Brachyura: Cryptochiridae: Opecarcinus) associated with Agariciidae corals. Coral Reefs 41, 699–709. doi: 10.1007/s00338-021-02163-1
Zibrowius H., Gili J. M. (1990). Deep-water scleractinia (Cnidaria: anthozoa) from Namibia, South Africa, and walvis ridge, southeastern atlantic. Sci. Mar. 54, 19–46.
Keywords: mesophotic coral ecosystems (MCEs), crustacea, agariciidae, species diversity, phylogeny
Citation: Vimercati S, Terraneo TI, Marchese F, Eweida AA, Rodrigue M, Pieribone V, Qurban M, Duarte CM and Benzoni F (2024) Diversity and distribution of coral gall crabs associated with Red Sea mesophotic corals. Front. Mar. Sci. 11:1305396. doi: 10.3389/fmars.2024.1305396
Received: 01 October 2023; Accepted: 13 March 2024;
Published: 28 March 2024.
Edited by:
Nicolas James Pilcher, Marine Research Foundation, MalaysiaReviewed by:
Bert W. Hoeksema, Naturalis Biodiversity Center, NetherlandsTao Xu, University of Groningen, Netherlands
Copyright © 2024 Vimercati, Terraneo, Marchese, Eweida, Rodrigue, Pieribone, Qurban, Duarte and Benzoni. This is an open-access article distributed under the terms of the Creative Commons Attribution License (CC BY). The use, distribution or reproduction in other forums is permitted, provided the original author(s) and the copyright owner(s) are credited and that the original publication in this journal is cited, in accordance with accepted academic practice. No use, distribution or reproduction is permitted which does not comply with these terms.
*Correspondence: Silvia Vimercati, silvia.vimercati@kaust.edu.sa