- 1Key Laboratory of Environmental Change and Resource Use in Beibu Gulf, Nannning Normal University, Nanning, China
- 2School of Civil Engineering and Architecture, Guangxi University, Nanning, China
- 3First Institute of Oceanography, Ministry of Natural Resources, Qingdao, China
Mangrove forest is a critical primary producer, biological habitat, and carbon sink in the subtropical-tropical coast zone, and the natural variation of mangrove coverage deserves study for a better understanding of the dynamics of mangrove coastal evolution. In this study, multispectral Landsat images from 1985 to 2018 are used to reconstruct the change in the coverage of mangrove (dominant species is Aegiceras corniculatum) and salt marsh (dominant species is Cyperus malaccensis) in the Nanliu River estuary. Tidal flat elevation measuring and 210Pb dating is used to study the substrate elevation when mangroves first colonize salt marsh. Historical temperature records, river discharge records, and the time series N/P concentration in sediment are analyzed. It is found that the mangrove forests have expanded rapidly in salt marsh since the mid-1980s. The change in factors such as accommodation space, cold event frequency, and nutrient supply cannot explain the origin of mangrove expansion. A low river discharge episode lasting for 8 years since 1986 is considered to have triggered the mangrove expansion in this area, as previously established salt marsh plants died due to germination restriction caused by high salinity and mangroves colonized the salt marsh habitat during this period. This case proves again that estuarine wetlands are very sensitive to salinity variation.
1 Introduction
Mangrove forests are widely distributed in tropical-subtropical coasts and are significant to the global ecosystem, as they provide ecological functions (Clough, 1998), coastal protection (Mazda et al., 1996; Magi et al., 1997; Vermaat and Thampanya, 2006; Bouillon et al., 2008; Donato et al., 2011), carbon sequestration, and protect the coast from storm surges or tsunamis (Chmura et al., 2003; Bouillon et al., 2008; Daniel M. Alongi., 2008). Globally, the total area of mangrove forests decreased rapidly in the 20th century largely due to human destruction (Giri et al., 2011a). The loss of mangrove forest will lead to a primary productivity decrease, seawater quality degradation, and interred carbon being released into the coastal zone. In addition to human destruction, mangrove forests are sensitive to climate change, sea level fluctuation, and nutrient supply. Many studies have shown that a change in winter temperature, rainfall, sea level, or even severe hurricane can result in a variation in mangrove coverage (Eslami-Andargoli et al., 2009; Cavanaugh et al., 2014; Lovelock et al., 2015; Duke et al., 2022). For a specific region, the responses of mangrove coverage to such impacting factors deserve our attention and study, which will help us in better understanding the dynamics of mangrove coast evolution and in guiding mangrove wetland restoration projects.
By 2020, the total area of mangrove forest in China is about 29000 ha, of which the mangrove forests in Guangxi Province (in South China, adjacent to the Beibu Gulf of South China Sea) comprise about 7300 ha. Most of the mangrove forests in this region are located in estuaries or on the coast adjacent to estuaries. Although human activities such as shrimp pool construction have reclaimed many mangrove wetlands during past decades, the total area of mangrove forests has still increased in this region since the mid-1980s (Wang et al., 2023). Given regional mangrove forest expansion in recent years is rare around the world, revealing the dynamics of mangrove forest expansion in this region is meaningful.
The Nanliu River is the biggest river flowing into the Beibu Gulf from Guangxi province. There are large patches of mangrove forests on the inter-tidal zone off river mouths (on the north edge of Lianzhou Bay), which have expanded significantly during past decades (Figure 1). So, the Nanliu River estuary is an ideal place for the study of mangrove coverage dynamics in the context of climate change and human activities. Some studies have been carried out to study the variation in wetland coverage during past decades in this area, but these studies did not distinguish the salt marsh from the mangrove forest, or the imaging time of satellite images is not old enough to determine the starting time of mangrove expansion, thus their conclusion about the driving force of mangrove expansion may be questionable (Wang et al., 2016; Liu et al., 2017). In view of this, satellite remote sensing images interpretation, field survey, 210pb dating, and sediment record analysis are systematically used in this study to reveal the starting time of mangrove expansion in this area. Moreover, with the regional temperature record and river discharge record, the relationship between mangrove expansion and accommodation space gain, cold events frequency, estuary salinity, and nutrient supply is studied. The aim of this study is to reveal the driving force of mangrove expansion in this region.
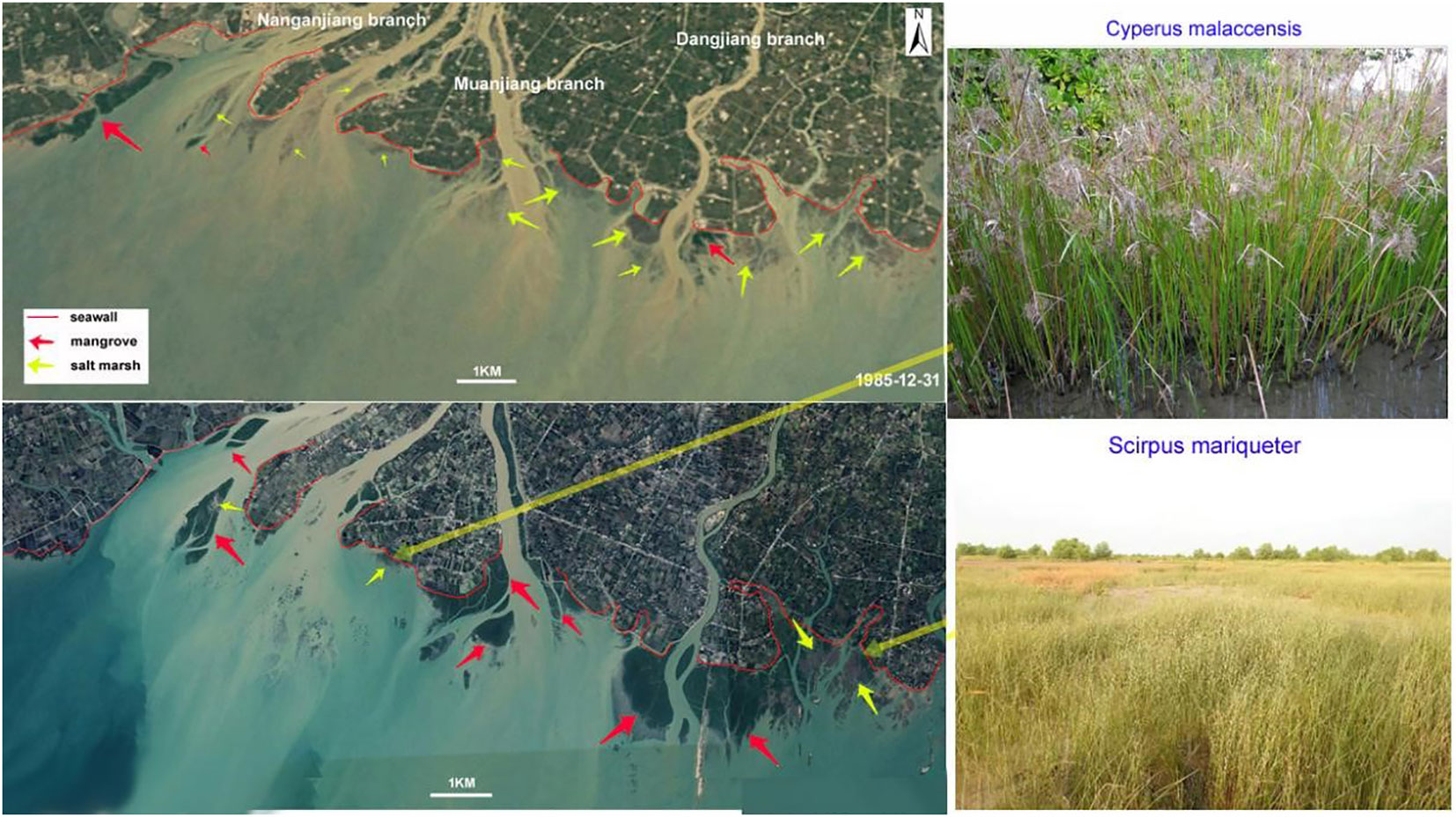
Figure 1 The spatial distribution of mangrove forest (dark green in color) and salt marsh (brown or light green in color) in the satellite images from 1985 and 2018 and the site photos (taken in 2020) of the salt marsh.
2 Study area and method
2.1 Geological and climatic context
The study area is in the estuary of Nanliu River, which flows into the Beibu Gulf of the Souh China Sea (Figure 2) and has a total length of 287 km and an annual discharge of 5.6×109 m3. In the estuary, there are three main branches: the Nanganjiang River, Muanjiang River, and Dangjiang River (Figure 2). On the tidal flat off river mouths, there are a large number of mangrove patches and a few salt marsh patches. The dominant mangrove species is Aegiceras corniculatum (Linn.) Blanco and the dominant species in the salt marshes is Cyperus malaccensis. The average annual rainfall is about 1600mm in this region over the past 60 years. Controlled by a tropical monsoon climate, the rainfall in the wet season (May to October) can account for approximately 80% of the total annual rainfall (Huang and Baoqing, 2015). The average annual temperature is 22°C in this region. In winter, strong cold air masses from northern Asia can lead to cold events during which the temperature can be reduced by 10°C. Diurnal tides are dominant in this area, the average tidal range is 2.5m and the maximum tidal range is 5.8m (Li et al., 2001). In summer or autumn, passing cyclones can result in energetic waves and storm surges, the maximum wave height record is about 2.0m and the maximum water addition exceeds 2.0m.
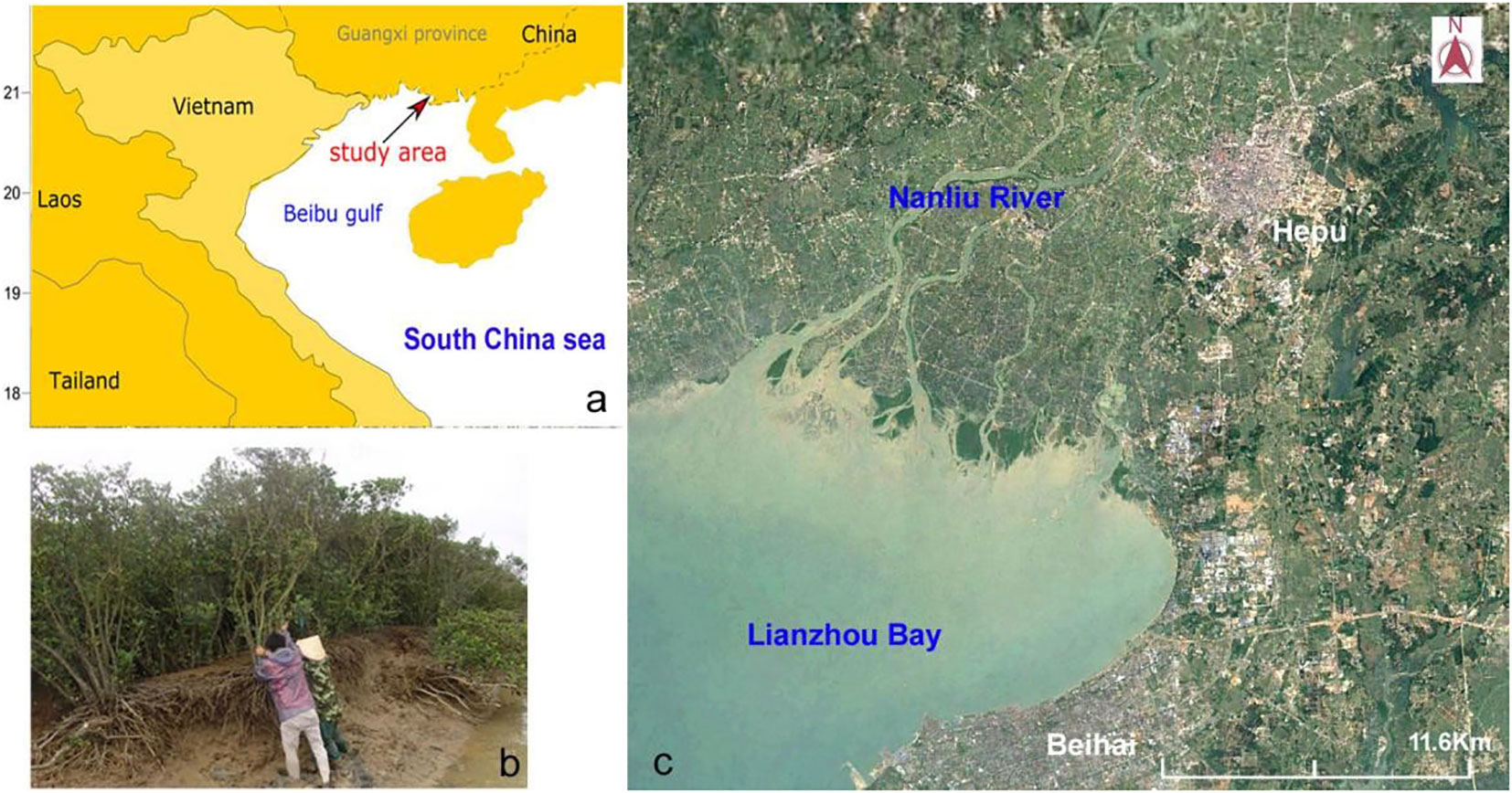
Figure 2 Location of the study area (A), distribution of mangrove forests in Nanliu River estuary (B), and the typical Aegiceras corniculatum plant in this area (C).
2.2 Method
In a field survey carried out in 2013, the elevation of a tidal flat along a main tidal creek in a mangrove forest off the river mouth of the Dangjiang branch was measured with a real-time kinematic GPS device (error< ± 3° cm). A Sediment core (109°5’44.99”E, 21°34’45.30”N) were collected at site C3 in this mangrove forest (Figure 3), which was sliced into sediment samples of 2cm in width. With part of the sediment samples, we separated plant debris from sediments by using a water sieve. The grain size of sediment samples was measured with a Master-2000 Laser particle sizer, and the organic carbon content of sediment samples was measured with a SHIMADZU TOC-5000 Total Organic Carbon Analyzer. The total nitrogen in sediment was measured with the Potassium dichromate sulfuric acid digestion Kjeldahl method. The total phosphorus concentration in sediment was determined spectrophotometrically with a Skalar Nutrient Analyzer. The 210Pb/226Ra radioactivity of some sediment samples was measured with a mass spectrometer in the State Key Lab of Lake Sciences of China. The excess 210Pb activity in sediment samples was obtained by subtracting the 226Ra activity from the total 210Pb activity. The measuring error of 210Pb radioactivity is ±9%~ ± 17%.
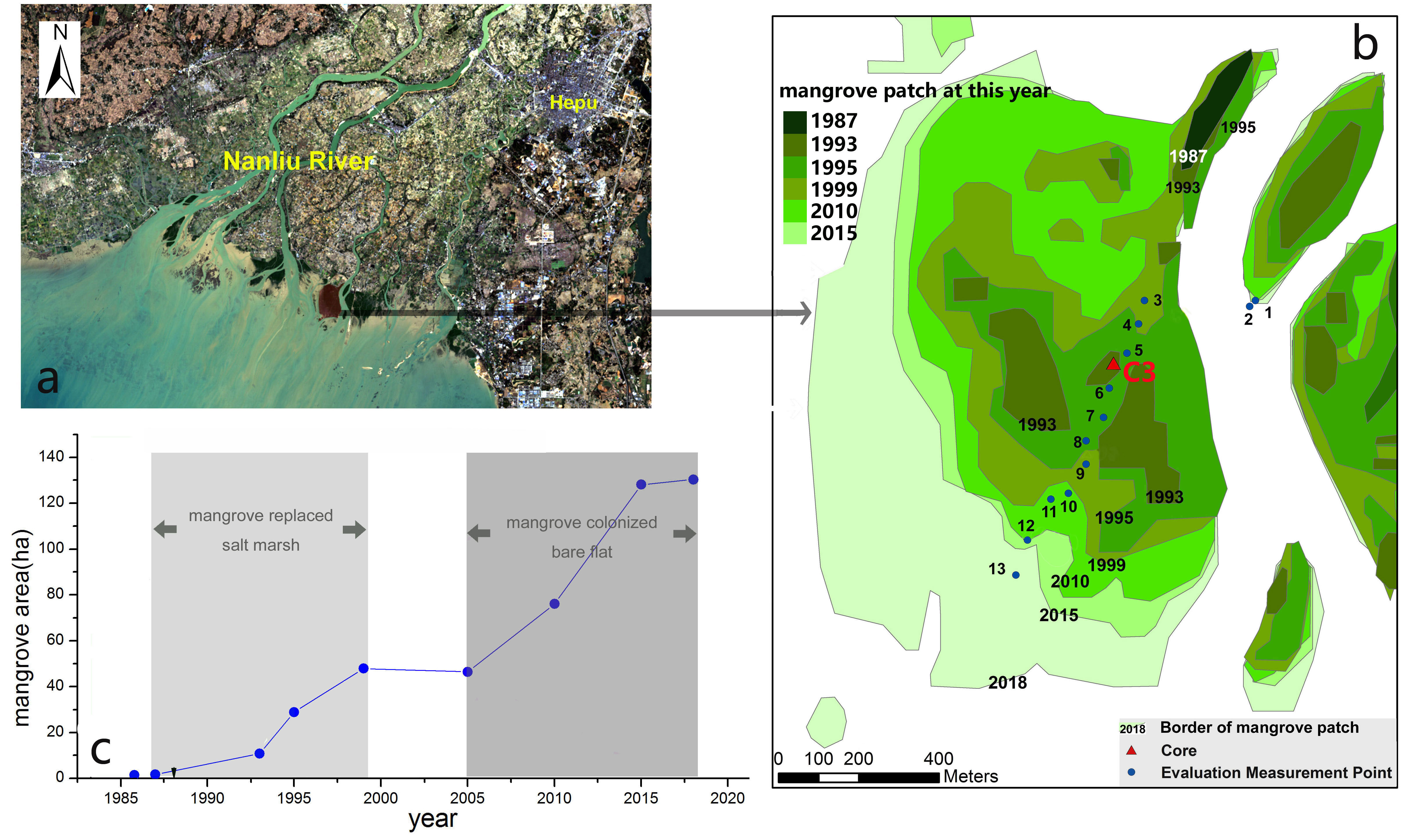
Figure 3 The expansion history (B) and change in area (C) for a mangrove patch off the river mouth of the Dangjiang branch (A) from 1987 to 2018.
Multispectral images collected by Landsat TM, ETM+, and OLI were used in this paper to reconstruct the change in mangrove coverage since the mid-1980s, most of them were collected in autumn (October to December) and winter (January to February). They were all downloaded from the United States Geological Survey (USGS) website (https://earthexplorer.usgs.gov/). The pixel side length of these Landsat multispectral images is 30 m. The downloaded images are listed in Table 1. Two main pre-processing steps were performed prior to classifying the Landsat images for recognizing the mangrove forest. These steps include a radiometric calibration and an atmospheric correction. Both the radiometric calibration and the atmospheric correction were carried out using ENVI software, i.e., the Radiometric Calibration tool for the radiometric calibration and the FLAASH Atmospheric Correction tool for the atmospheric correction. Thereafter, the Spectral Angle Mapper (SAM) classification tool in ENVI software was used to classify the Landsat images for mangrove forest recognition. The SAM is a physically based spectral classification proposed by Kruse et al. (1993). Their algorithm determines the spectral similarity between two spectra by calculating the angle between the spectra and treating them as vectors in a space with dimensionality equal to the number of bands. In this area, the spectra of mangrove forests are significantly different from that of salt marsh especially in in autumn and winter when the leaves of salt marsh plants are withered, for example, we can distinguish the mangrove forests (dark green) and salt marsh (light brown) even just by eye in the satellite images (Figure 1). The end member spectra of mangrove forests, seawater, sand, and other vegetation were extracted directly from the Landsat multispectral images (in 2018-02-01) as the average spectra of a region of interest (ROI). The angle between the end member spectrum vector and each pixel vector was then compared. The smallest of the 84 angles represents a closer match to the reference spectrum. By using this SAM classification tool, 85 mangrove forest pixels were counted, and the area of the mangrove forest was then calculated using the following equation:
where S, N, and r represent the area of the mangrove forest, the number of mangrove forest pixels, and the pixel side length of the Landsat multispectral images (30 m), respectively.
3 Results
3.1 The history of mangrove expansion determined by satellite remote sensing
Based on the interpretation of satellite remote sensing images, it is revealed that the history of mangrove expansion comes mainly at the cost of salt marsh in the Nanliu River estuary from 1987 to 2018 (Figure 4). In 1987, The wetlands off of river mouths were mainly salt marshes. There were only a few mangrove patches with a total area of 72 ha (Figure 5). From 1987 to 2010, the mangroves expanded significantly, and most of the salt marshes were replaced. From 2010 to 2018, some mangrove patches expanded onto bare flats and the total mangrove area increased to 622 ha by 2018. The average expanding rate of mangroves is about 18 ha/a from 1987 to 2018.
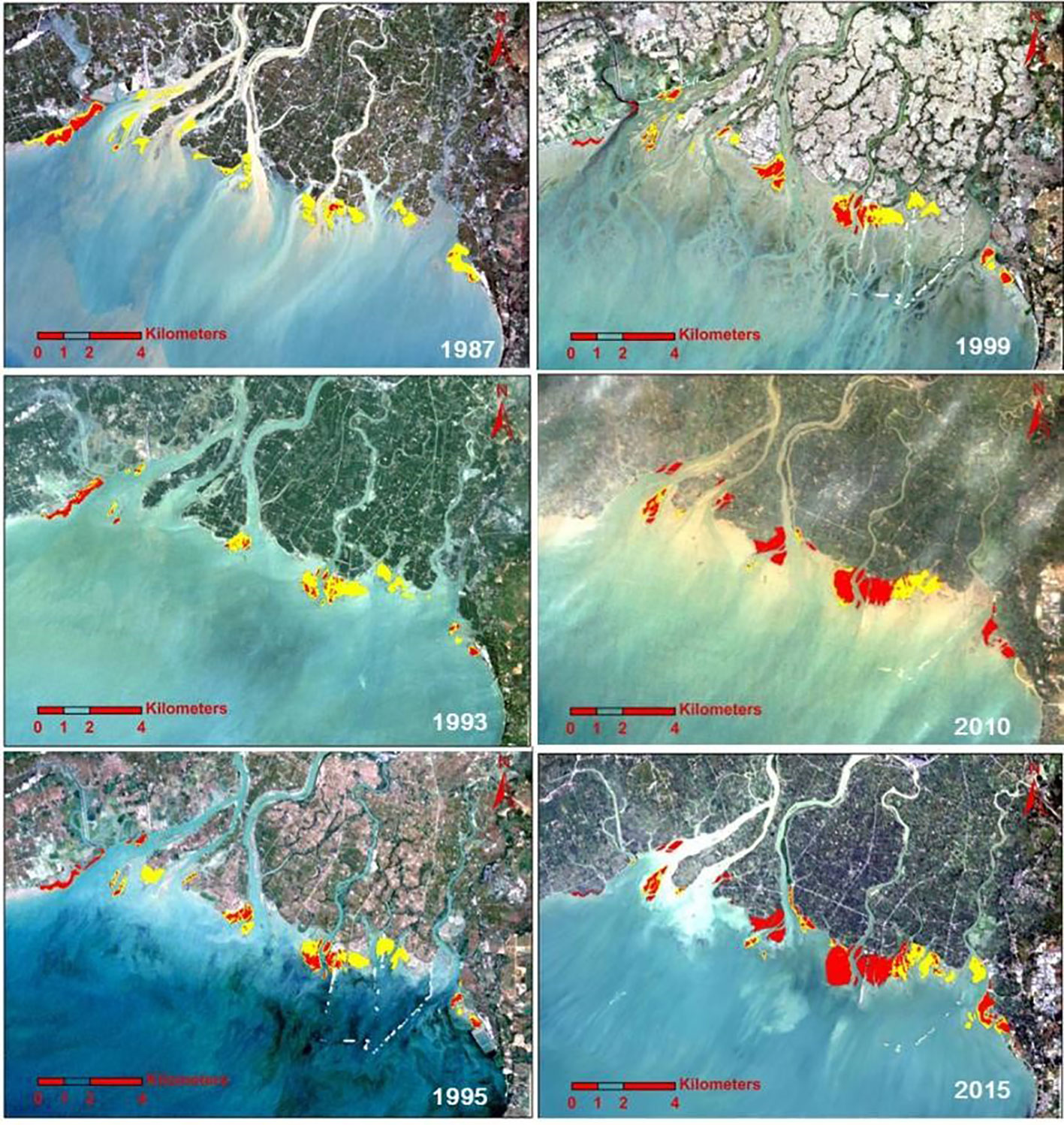
Figure 4 The spatial distribution of mangroves (red area) and salt marsh (yellow area) in satellite images from different years.
To study the natural mangrove expansion, the expansion history of a mangrove patch off the river mouth of the Dangjiang branch is studied, which is completely free from human interference. As is shown in Figure 3B, in 1987, there was only one small mangrove patch with an area of 1.71 ha. In 1993, two new mangrove patches emerged in the salt marsh and the total mangrove area reached 10.85 ha (Figures 3B, C). By 1999, the mangrove area reached 50 ha and all the salt marsh was replaced. From 1999 to 2005, the increase in mangrove area stopped, indicating an intermittent mangrove expansion on the bare tidal flats. From 2005 to 2018, the mangrove area increased rapidly again, indicating a mangrove colonization on bare flats in the period of 2002-2013. According to the expansion rate of the mangroves during 1987~1993, we can infer that the starting time of mangrove expansion in the salt marsh was within the time span of 1985-1987, in other words, the mangrove expanded since the mid-1980s in this area.
3.2 Evidence from 210Pb dating and plant debris
Sediment records and 210Pb dating are also used to study the transition of dominant species in wetlands and the substrate elevation at the time when mangroves first colonized.
In the upper part (0-42cm) of sediment core C3 collected in a mangrove patch which first emerged after the mid-1980s, the excess 210Pb specific radioactivity declined exponentially with accumulative mass, this indicates an approximately constant sedimentation flux, and 210Pbex concentration (Figure 6A). The CS-CF modal thus was used to calculate the sedimentation rate, which is 0.65 g.cm-2.a-1 in the depth range of 0-20cm and is 0.92 g.cm-2.a-1 in the depth range of 20-42cm. With the sedimentation rates obtained by 210Pb dating, we can determine the substrate elevation in 1986 (Figure 6B), which is 32cm below the tidal flat surface in 2013 (when the core was collected). The substrate elevation in 1986 was 66cm above the threshold for mangrove survival (Figure 7), indicating that accommodation space gain is not the reason for mangrove forest expansion since the mid-1980s. In this core, specific residual debris of mangrove plants (thin woody twigs and petioles) can be found at depths of less than 20cm; below 20cm, only salt marsh plant debris (thin and long leaves) can be found (Figure 8). This indicates that a transition of salt marsh to mangrove have taken place decades ago at this site.
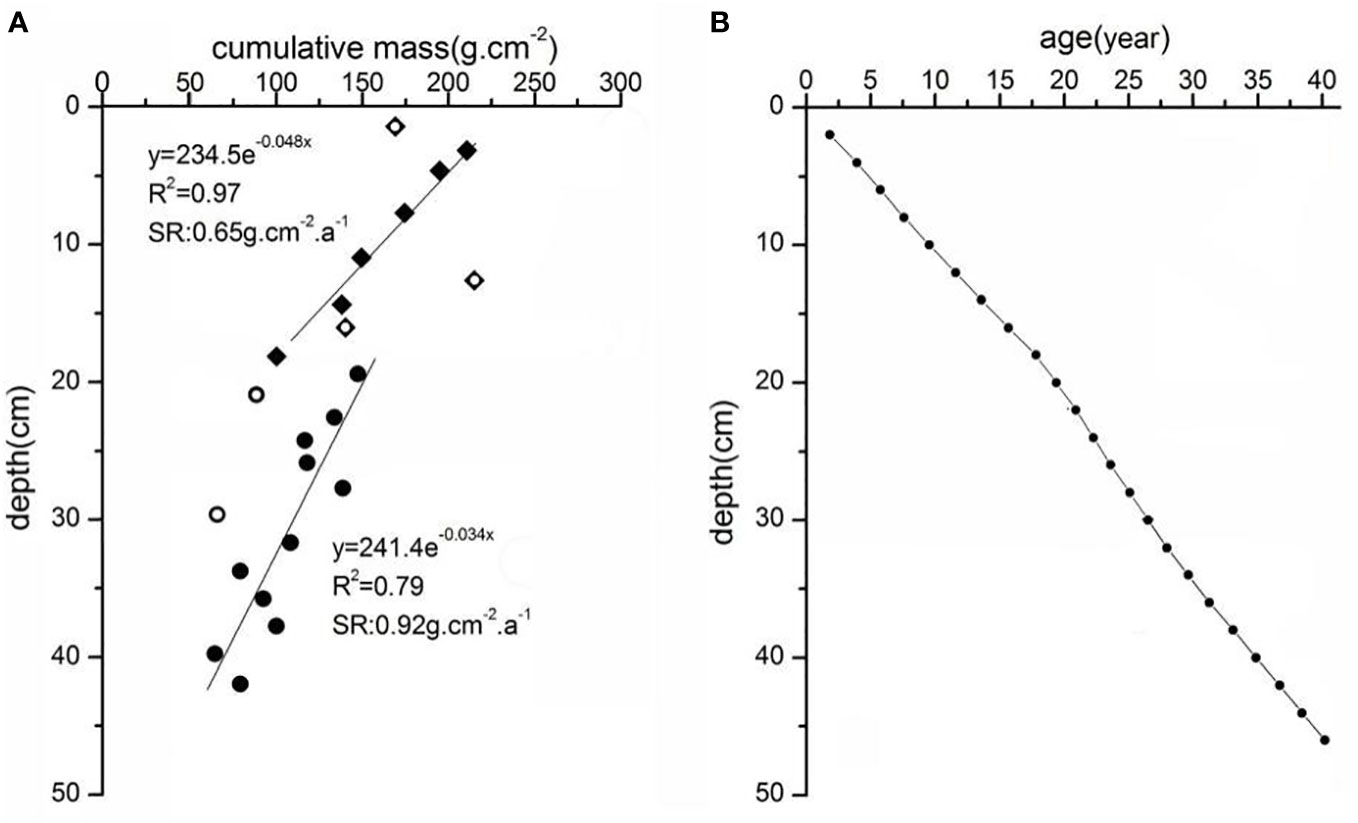
Figure 6 The variation of excess 210Pb radioactivity with cumulative mass in the upper part of core C3 (A), the year-depth curve in sediment core C3 (B).
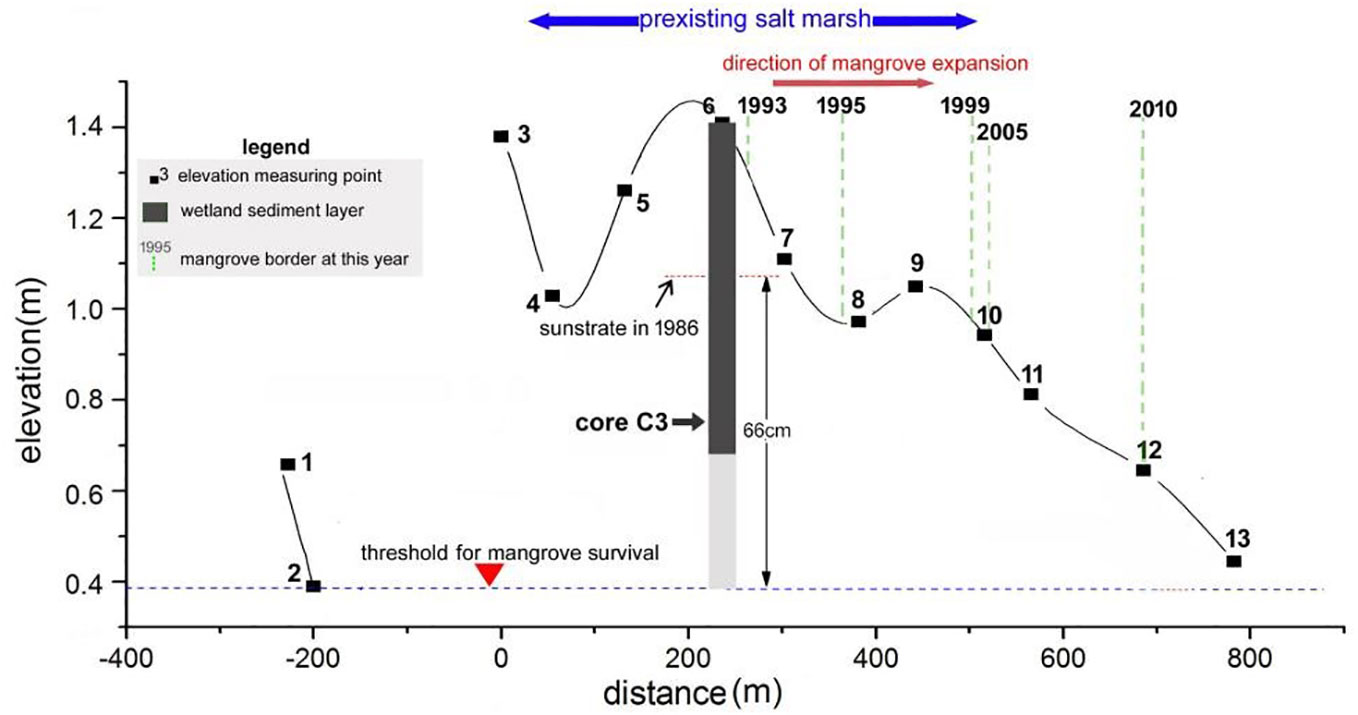
Figure 7 The elevation (national 1985 elevation of China) of mangrove tidal flat surface in 2013, the elevation of wetland substrate in 1986, and the threshold for mangrove survival.
3.3 The variation in winter temperature, estuary salinity, and nutrient supply during past decades
Environment factors such as air temperature (Lugo and Patterson-Zucca, 1977; Liu et al., 2014; Chen et al., 2017), salinity, and nutrient availability can influence the survival of mangrove seedlings and thus the expansion of mangroves. The time series of substitutes for these environmental factors shall be studied to reveal key factors triggering mangrove expansion in this area (Mo, 2017).
Extreme cold events can kill mangrove seedlings or even young mangroves in the subtropical zone. In South China, January and February are the coldest months. Recently, only one lethal cold event was reported in early 2008 (Chen et al., 2010). From 10 January to 10 February 2008, low temperatures lasted for 30 days, during which 70%-100% of seedlings of alien species such as Rhizophora stylosa, B.gymnorhiza, Sonneratia caseolaris died, while there was no report of Aegiceras corniculatum seedling being frozen to death in this area. The monthly average temperature in February 2008 was 11.2°C and represents the lowest value in recent years (Figure 9). As is shown in Figure 9, possible lethal cold events have been rare during the past sixty years in this area, from 1953 to 1988, there were only two possible lethal cold events (in 1968 and 1977) for Aegiceras corniculatum seedlings. As the time span between lethal cold events is long enough for the mangrove saplings to grow, the frequency of lethal cold events in this area did not reach a level of restraining mangrove expansion before the mid-1980s. The change in the frequency of lethal cold events thus cannot explain the starting of Aegiceras corniculatum forest expansion since the mid-1980s.
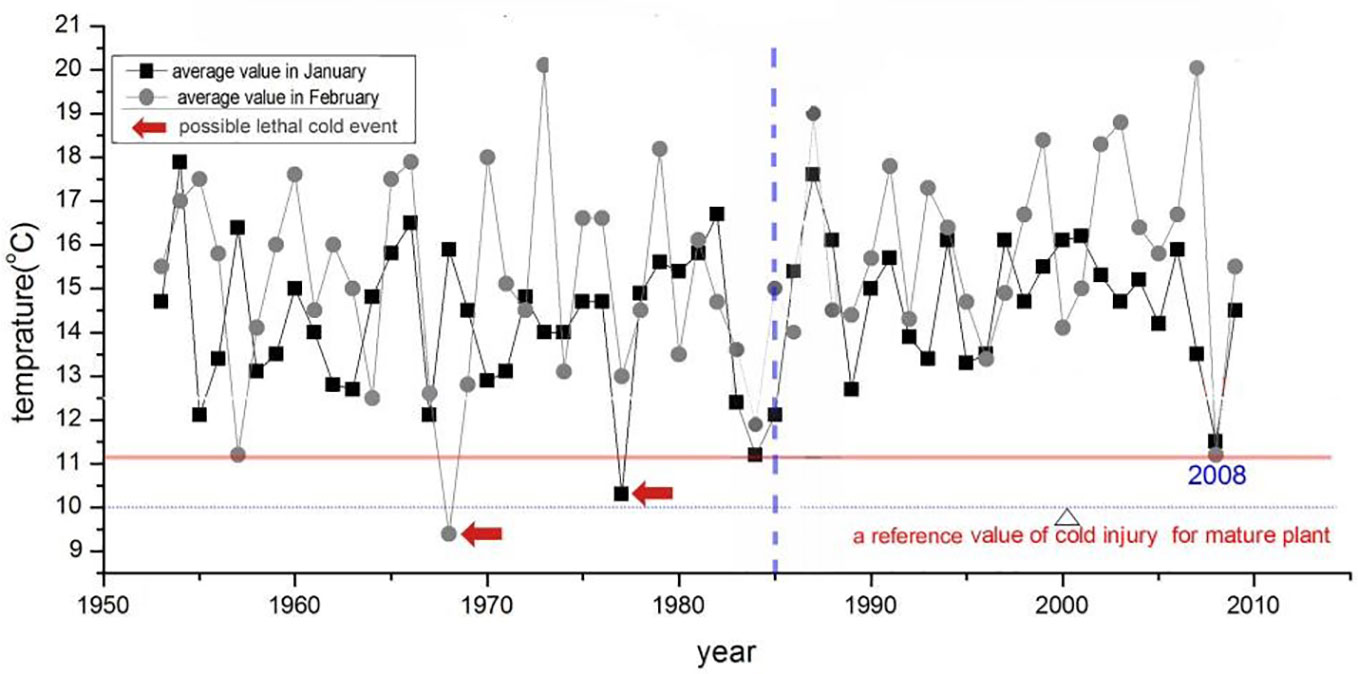
Figure 9 Time series of average air temperature in January and February from 1953 to 2009 in this area (recorded at the Beihai weather station).
Salinity is a key environmental factor affecting mangrove seedling survival and salt marsh plant rejuvenation. Suitable high salinity may promote the expansion of mangroves in salt marsh. In this area, the transition of salt marsh to mangrove began in the mid-1980s, which had just encountered an unprecedented low river discharge episode lasting for 8 years (Figure 10A). The average river discharge during this episode was only 120m3/s, lower by 25% than the average value of past 50 years. The salinity in the estuary must have increased significantly during this period. Given the occurrence time of the two events is highly consistent, the possibility of mangrove expansion triggered by low river discharge episodes is high.
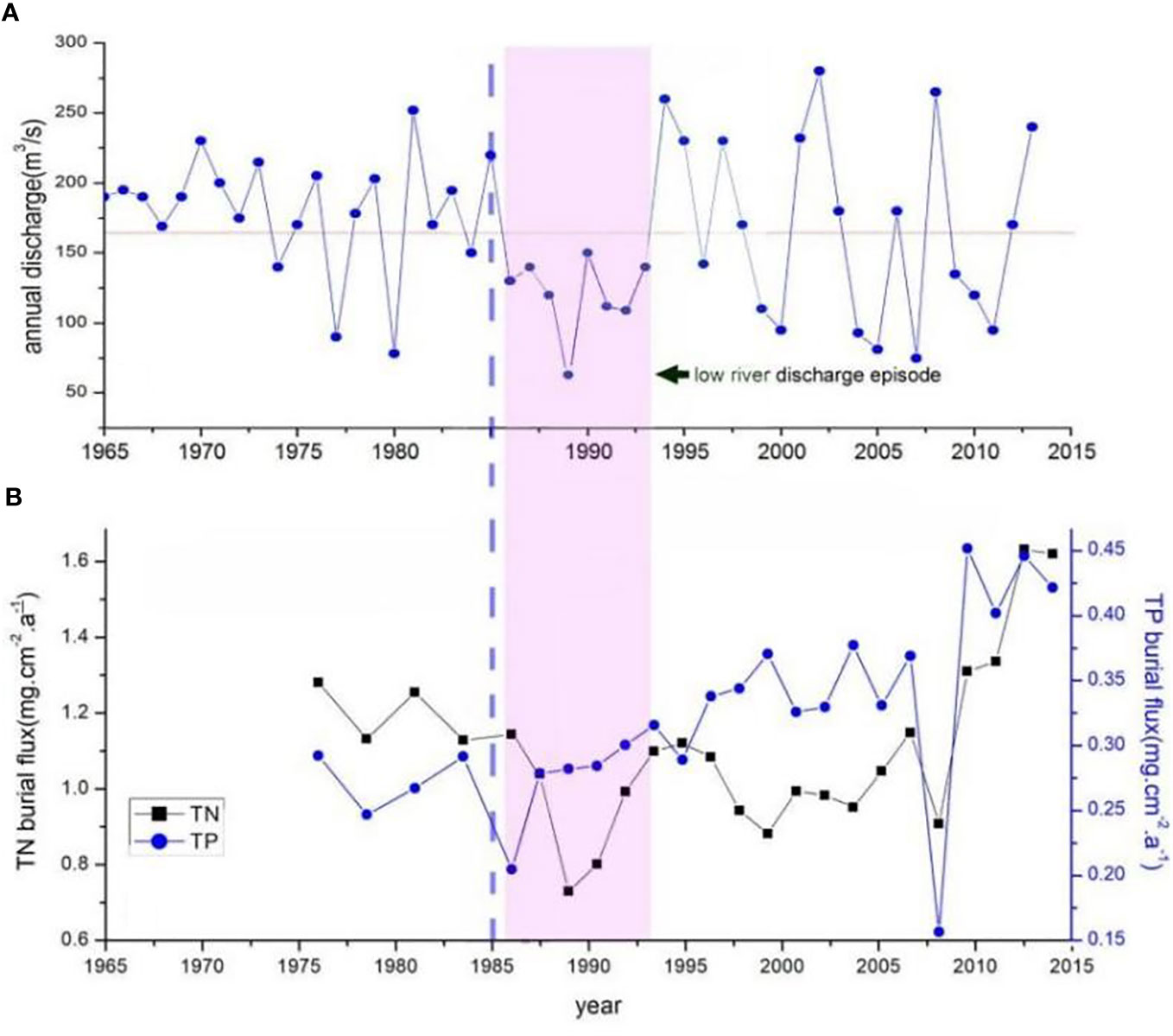
Figure 10 (A) time series of Nanliu River discharge (recorded at the Chang-Le hydrological station) from 1965 to 2013, (B) time series of total N/P burial flux in the sediment core C3.
The nutrient availability may affect the seedling establishment and survival indirectly in some environments. The nutrient status of the maternal tree and the provisioning of propagules may have a large influence on early seedling growth. A large size propagule with more material storage can enhance its survival probability under threats such as high salinity, cold events, shellfish attachment, and pests gnawing. Based on the chronology framework determined by 210Pb dating in sediment core C3, the time series of total phosphorus/nitrogen burial flux is revealed (Figure 10B). No significant increasing trend in the total phosphorus/nitrogen burial flux is found during the 1980s, indicating the nutrient supply did not increase at the time of mangrove forest expansion starting. The significant increase in phosphorus burial flux took place after 1990, while the significant increase in nitrogen burial flux took place after 2005. It is obvious that the beginning of mangrove expansion in salt marsh has no relationship with the change in nutrient status.
4 Discussion
4.1 Accommodation space gain and hydrodynamics
The elevation of accommodation space for mangroves is generally between the local mean sea level and the mean high tide level. Mangrove forests may expand due to accommodation space gain. The seaward expansion on the bare tidal flats is usually caused by tidal flat accretion (Young and Harvey, 1996), and the landward expansion to salt marsh may occur due to local sea level rise. According to this study, the mangroves expanded seaward in salt marsh in the period from the mid-1980s to late 1990s. Given the fact that the substrate elevation in salt marsh is usually not lower than that in mangroves, substrate elevation thus is not likely the limitation factor for mangrove expansion in this area before the mid-1980s. Actually, at a site where mangroves have first colonized salt marsh since the mid-1980s, the substrate elevation at that time is 66cm above the threshold for mangrove survival. Given the sea level fluctuation on the decadal scale only has a magnitude of centimeters in this region, sea level fluctuation itself can not impact the mangrove seedling establishment in the salt marsh. It seems that the seaward mangrove expansion into the salt marsh is not directly caused by tidal flat accretion. Hydrodynamics are another factor that may impact mangrove seedling establishment.
Storm surges that come with Typhoons may erode the mangrove seedlings on bare tidal flats in this area (Liu et al., 2017). On a macro tidal coast, strong tides and currents may be unfriendly for mangrove seedlings too. However, the mangrove expansion in this area first took place in salt marsh, where the hydrodynamic energy is dissipated significantly by dense plants. The possibility of hydrodynamic action restricting mangrove seedlings’ establishment is low. The period of the mid-1980s experienced the most frequent storm surge disasters (1984,1985,1986). What is more, according to the sedimentation rate obtained via 210Pb dating, we can infer that the substrate elevation in the salt marsh had reached the threshold for mangrove survival about 60 years before the time when the mangrove expansion first took place. Why the mangrove expansion did not occur during such a long period with suitable temperature for mangrove growth? During this long period, the study area must have experienced some span of time with a lower tidal range. In view of this, we can conclude that the hydrodynamic is not a key factor that can impact the salt marsh-mangrove transition in this area.
4.2 Salinity is critical in driving salt marsh-mangrove transition
In recent decades, cases of mangrove forest expansion have been reported around the world. Despite the cases caused by accommodation space gain, climate change is the main driving force for mangrove expansion. With global warming, some salt marshes have been replaced by mangroves in the areas with higher latitudes (Snedaker, 1995; Comeaux et al., 2012; Cavanaugh et al., 2014). Warmer winters and mangrove seedling supply are undoubtedly the prerequisites of mangrove expansion into salt marshes. However, the transition of salt marsh to mangrove has not occurred in many regions with suitable air temperatures for mangrove species. For instance, the frequency of lethal cold events in the study area did not reach a level of preventing mangrove expansion before the mid-1980s, but the mangrove expansion did not happen at that time.
Compared to air temperature, the environmental salinity may be more critical in driving the wetland vegetation succession. For example, mangrove seedlings are difficult to grow under the stress of high salinity in arid regions; excessive soil salinity caused by drought events and sea level drops can even kill adult mangrove plants (Duke et al., 2022). Salinity decline in estuaries coming with rainfall increase can enhance the survival rate of mangrove seedlings and lead to mangrove forest expansion in salt marshes in these arid regions (Eslami-Andargoli et al., 2009). In contrast, as the salinity tolerance of dominant species in salt marsh in wet regions usually is lower than mangrove species, a significant reduction of river discharge may result in dieback events of salt marsh plants and mangrove invasion. The dominant species in salt marsh in the Nanliu River estuary are Cyperus malaccensis and Spartina. The suitable germination salinity for Cyperus malaccensis is 5ppt and will be inhibited in germination when salinity exceeds 15ppt (Chen Y S., 2011) while the optimal salinity for the physiological function of mangrove seedlings growth ranges from 3ppt to 27ppt (Ye et al., 2005). During the low river discharge episode from 1986 to1993, the high salinity in the estuary is inferred to result in the death of Cyperus malaccensis due to rejuvenation ceasing, therefore, mangrove seedlings with higher salinity tolerance colonized salt marsh habitat, which led to a rapid mangrove forest expansion.
4.3 Subsequent impacts of mangrove forest expansion
Mangroves of the same area have higher productivity than salt marsh, which will provide more biomass in a wetland. It is undoubtedle that the mangrove expansion will enhance bio-diversity in the coastal zone. As mangroves need more nutrients than salt marsh species to maintain their high productivity, mangrove forest expansion may be beneficial to reduce the nutrient level in eutrophic coasts. However, in the estuaries where nutrients are not excessive, the decline of nutrient exporting with mangrove expansion may cause a reduction in algae biomass and lead to population decline of some fish species that only consume algae. In estuaries with poor mixing, more organic debris inputting from wetlands caused by mangrove forest expansion may intensify hypoxia in the underlying water body. Mangrove forest has a higher carbon sequestration rate than salt marsh, so mangrove forest expansion thus will improve the carbon pool function of the coast zone. However, methane production in mangrove wetlands usually is higher than that in salt marshes. Given the fact that the greenhouse effect of methane is about 60 times that of carbon dioxide, it is still uncertain that the mangrove forest expansion will mitigate or intensify the greenhouse effect. In sum, the effects of mangrove forest expansion on coastal ecology and the greenhouse effect still needs more studies.
5 Conclusions
In the Nanliu River estuary, mangrove forests expanded rapidly and replaced most of the salt marsh since the mid-1980s, the total mangrove area has increased from 72ha to 622ha in the period of 1987~2018. The mangrove forest expansion during this period cannot be attributed to accommodation space gain, cold event decrease, or nutrient supply increase. A low river discharge episode (1986~1993) is inferred to trigger this expansion directly. During this period, the original salt marsh dominant species (Cyperus malaccensis) died gradually due to germination restriction under high salinity and mangroves (Aegiceras corniculatum) colonized the salt marsh habitat gradually. This study shows that wetlands in estuaries are sensitive to salinity change, and that significant change in rainfall in drainage basins may result in a rapid transition of dominant plant species.
Data availability statement
The raw data supporting the conclusions of this article will be made available by the authors, without undue reservation.
Author contributions
TL: Writing – original draft, Writing – review & editing. RH: Writing – original draft. YS: Data curation, Methodology, Writing – review & editing. YL: Writing – original draft. ZS: Writing – original draft.
Funding
The author(s) declare financial support was received for the research, authorship, and/or publication of this article. This study was financed by a Major Project of Guangxi Science and Technology (No. AA23023016).
Conflict of interest
The authors declare that the research was conducted in the absence of any commercial or financial relationships that could be construed as a potential conflict of interest.
Publisher’s note
All claims expressed in this article are solely those of the authors and do not necessarily represent those of their affiliated organizations, or those of the publisher, the editors and the reviewers. Any product that may be evaluated in this article, or claim that may be made by its manufacturer, is not guaranteed or endorsed by the publisher.
References
Alongi D. M. (2008). Mangrove forests: Resilience, protection from tsunamis, and responses to global climate change. Estuarine Coast. Shelf Sci. 76, 1–13. doi: 10.1016/j.ecss.2007.08.024
Bouillon S., Borges A. V., Castaneda-Moya E., Diele K., Dittmar T., Duke N. C., et al. (2008). Mangrove production and carbon sinks: A revision of global budget estimates. Global Biogeochem Cycles 22, 1–8. doi: 10.1029/2007GB003052
Cavanaugh K. C., Kellner J. R., Forde A. J., et al. (2014). Poleward expansion of mangroves is a threshold response to decreased frequency of extreme cold events. Proc. Natl. Acad. Sci. 111, 723–727. doi: 10.1073/pnas.1315800111
Chen L., Wang W., Li Q. Q., Zhang Y., Yang S., Osland M. J., et al. (2017). Mangrove species’ responses to winter air temperature extremes in China. Ecosphere 8, e01865. doi: 10.1002/ecs2.1865
Chen L. Z., Wang W. Q., Zhang Y. H., et al. (2010). Damage to mangroves from extreme cold in early 2008 in southern China. Chin. J. Plant Ecol 34, 186–194.
Chen Y.-S. (2011). Effects of salinity stress on germination of two cyperus malaccensis. J. Anhui Agri (Sci) 39, 10396–110397.
Chmura G. L., Anisfeld S. C., Cahoon D. R., Lynch J. C. (2003). Global carbon sequestration in tidal, saline wetlandsoils. Global Biogeochem Cycles 17, 1–12. doi: 10.1029/2002GB001917
Clough B. (1998). Mangrove forest productivity and biomass accumulation in Hinchlnbrook channel, north Queensland. Australian Institute of Marine Science. 2, 191–198. doi: 10.1023/A:1009979610871
Comeaux R. S., Allison M. A., Bianchi. T. S. (2012). Mangrove expansion in the Gulf of Mexico with climate change: Implications for wetland health and resistance to rising sea levels. Estuarine Coast. Shelf Sci. 96, 81–95. doi: 10.1016/j.ecss.2011.10.003
Donato D. C., Kauffman J. B., Murdiyarso D., Kurnianto S., Stidham M., Kanninen M. (2011). Mangroves among the most carbon-rich forests in the tropics. Nat. Geosci. 4, 293–297. doi: 10.1038/ngeo1123
Duke N. C., Mackenzie J. R., Canning A., Hutley L. B., Bourke A. J., Kovacs J. M., et al. (2022). ENSO-driven extreme oscillations in mean sea level destabilise critical shoreline mangroves—An emerging threat. PloS Climate 1, e0000037. doi: 10.1371/journal.pclm.0000037
Eslami-Andargoli L., Dale P., Sipe N., Chaseling J. (2009). Mangrove expansion and rainfall patterns in Moreton Bay, southeast Queensland, Australia. Estuary Coast. Shelf Sci. 85, 292–298. doi: 10.1016/j.ecss.2009.08.011
Giri C., Ochieng E., Tieszen L. L., Zhu Z., Singh A., Loveland T., et al. (2011a). Status and distribution of mangrove forests of the world using earth observation satellite data. Glob Ecol. Biogeogr 20, 154–159. doi: 10.1111/j.1466-8238.2010.00584.x
Huang Y., Baoqing H. (2015). Analysis of change trend of annual runoff of the nanliujiang river based on wavelet transform. J. Guangxi Teachers Educ. Univ. 32, 110–114.
Kruse F. A., Lefkoff A. B., Boardman J. W., Heidebrecht K. B., Shapiro A. T., Barloon P. J., et al. (1993). The spectral image processing system (SIPS)—interactive visualization and analysis of imaging spectrometer data. Remote Sens. Environ. 44, 145–163. doi: 10.1016/0034-4257(93)90013-N
Li S., Huayong X., Mingjian. C. (2001). Study on hydrology and hydrodynamic environment of Guangxi offshore (Beijing: China Ocean Press), 10.
Liu K., Liu L., Liu H., Li X., Wang S. (2014). Exploring the effects of biophysical parameters on the spatial pattern of rare cold damage to mangrove forests. Remote Sens. Environ. 150, 20–33. doi: 10.1016/j.rse.2014.04.019
Liu T., Yancheng. T., Ying L. (2017). Mangrove swamp expansion controlled by climate since 1988: a case study in the Nanliu River Estuary, Guangxi, Southwest China. Acta Oceanol Sin. 36, 11–17. doi: 10.1007/s13131-017-1097-9
Lovelock C. E., Cahoon D. R., Friess D. A., Guntenspergen G. R., Krauss K. W., Reef R., et al. (2015). The vulnerability of Indo-Pacific mangrove forests to sea-level rise. Nature 526, 559–563. doi: 10.1038/nature15538
Lugo A. E., Patterson-Zucca C. (1977). The impact of low temperature stress on mangrove structure and growth. Trop. Ecol. 18, 149–161.
Magi M., Kogo M., Hong P. N. (1997). Mangroves as a coastal protection from waves in the Tong King delta Vietnam. Mangroves Salt Marshes 1, 127–135.
Mazda Y., Blasco F., Saenger P., Janodet E. (1996). Mangroves as indicators of coastal change. Catena 27, 258–2178.
Mo L. (2017). Study on Extreme Events of Temperature and Precipitation over Guangxi Zhuang Autonomous Region 1960-2015 (Lanzhou: Lanzhou University), 23.
Snedaker S. (1995). Mangroves and climate change in the Florida and Caribbean region: scenarios and hypotheses. Hydrobiologia 295, 43–49. doi: 10.1007/BF00029109
Vermaat J. E., Thampanya U. (2006). Mangroves mitigate tsunami damage: a further response. Estuarine Coast. Shelf Sci. 69, 1–3. doi: 10.1016/j.ecss.2006.04.019
Wang Z., Liu K., Cao J., Peng L., Wen. X. (2023). Annual change analysis of mangrove forests in China during 1986–2021 based on google earth engine. Forest 13, 1489. doi: 10.3390/f1309
Wang J., Liu J., Tiezhu S., Guofeng. W. (2016). Remotely dynamic monitoring o f mangrove in Lianzhou Gulf, Guangxi, during between 1990 a n d 2015. J. For. Environ. 36, 455–460.
Ye Y., Tam N. F. Y., Lu C. Y., et al. (2005). Effects of salinity on germination, seedling growth and physiology of three salt secreting mangrove species. Aquat. Bot. 83, 193–205. doi: 10.1016/j.aquabot.2005.06.006
Keywords: mangrove forest, wetland landscape, satellite remote sensing, climate change, Beibu Gulf
Citation: Liu T, Huang R, Sun Y, Liu Y and Song Z (2024) Rapid mangrove expansion triggered by low river discharge episode in Nanliu river estuary, Beibu Gulf of China. Front. Mar. Sci. 11:1285518. doi: 10.3389/fmars.2024.1285518
Received: 30 August 2023; Accepted: 08 February 2024;
Published: 08 March 2024.
Edited by:
Jamie Ruprecht, University of New South Wales, AustraliaReviewed by:
Qiang Yao, Louisiana State University, United StatesDandan Duan, Hainan Normal University, China
Copyright © 2024 Liu, Huang, Sun, Liu and Song. This is an open-access article distributed under the terms of the Creative Commons Attribution License (CC BY). The use, distribution or reproduction in other forums is permitted, provided the original author(s) and the copyright owner(s) are credited and that the original publication in this journal is cited, in accordance with accepted academic practice. No use, distribution or reproduction is permitted which does not comply with these terms.
*Correspondence: Yonggen Sun, c3lnQGZpby5vcmcuY24=