- Ocean Governance Research Group, Research Institute for Sustainability – Helmholtz Centre Potsdam, Potsdam, Germany
The model pathways of the Intergovernmental Panel for Climate Change (IPCC) for the timely achievement of global climate targets, especially the target of limiting global warming to 1.5°C compared to pre-industrial levels, suggest the need for safeguarding and enhancing the global carbon sink. Experts argue that the deployment of so-called negative emissions technologies for large-scale carbon dioxide removal holds potential for keeping the temperature in line with limits set by the Paris Agreement. Ocean-based negative emissions technologies (ONETs) intend to enhance carbon sequestration and storage in the ocean, e.g., by changing the ocean’s physical or biogeochemical properties. But in addition to these intended effects, ONETs may also cause unintentional impacts on the ocean’s condition and on related coastal and marine ecosystem services that are relevant for the attainment of a range of global policy goals. This article links potential direct and indirect, intentional and unintentional impacts of eight ONETs on the marine environment to the regulations and policy goals of international environmental agreements of the current global ocean governance regime. The results thereof outline a direct, implicit and indirect governance framework of ONETs. Hereby, a broader perspective of the concept of (global) ocean governance is adopted to outline a wider network that goes beyond the explicit regulation of ONETs within the realm of ocean governance. This first-order assessment derives gaps and challenges in the existing governance framework, as well as needs and opportunities for comprehensive governance of the technologies. It is determined that while the inclusion of ONETs in the global climate strategy may be deemed necessary for reaching net zero emission targets in the future, a range of potential trade-offs with other policy goals may need to be considered or dealt with when deploying ONETS for climate mitigation. Further, foresight-oriented and adaptive governance mechanisms appear imperative to bridge gaps resulting from extensive uncertainties and unknowns linked to ONET deployment in a changing ocean and. The identified ONET governance framework reiterates current challenges in ocean governance, for instance related to fragmentation, but also represents an opportunity for a synergistic and integrated approach to future governance.
1 Introduction
During the international climate negotiations in 2015, Parties to the United Nations Framework Convention on Climate Change (UNFCCC) finalised the Paris Agreement with the ambition of limiting global warming to ‘well below 2°C, preferably to 1.5°C’ (UNFCCC, 2015 - Art. 2(a)). Scientific articles on a wide range of geographical regions, ecosystems, and economic sectors reiterate the imperative of limiting warming to 1.5°C for a thriving planet, especially for marine, coastal and island ecosystems and communities (King et al., 2017; Giardino et al., 2018; McWhorter et al., 2022). The Intergovernmental Panel on Climate Change (IPCC) 2018 Special Report on “Global Warming of 1.5°C”, based on the assessment of 6,000 peer-reviewed publications, put forward emissions pathways consistent with global ambitions of limiting global warming to 1.5°C above pre-industrial levels (IPCC, 2018). All proposed pathways indicate that a global transition towards carbon neutrality is pertinent by 2050, but that residual greenhouse gas emissions from hard-to-abate activities would need to be counterbalanced (IPCC, 2022). Carbon dioxide removal (CDR) from the atmosphere as additional element in the global strategy towards reaching carbon neutrality by mid-century and ultimately limiting global warming, especially to 1.5°C, has been put forward by many studies, as well as the IPCC, for achieving global climate ambition agreed upon in the Paris Agreement (IPCC, 2022). The ocean’s significant role in the earth’s climate system, having sequestered 26% of total CO2 emissions in the last decade (Friedlingstein et al., 2022), indicates a promising potential for such approaches.
Human interventions have been suggested for the large-scale reduction of atmospheric CO2 levels in the near-term, to offset emissions from sources that will likely not achieve carbon-neutrality by mid-century and to sustain these efforts for the long-term (IPCC, 2022). Additional CO2 sequestered from the atmosphere and stored long-term via CDR has the opposite effect to producing emissions, and can be described as achieving ‘negative emissions’ (IPCC, 2018). So-called ‘negative emissions technologies’ (NETs) can broadly be defined as human interventions in nature that remove and store greenhouse gases from the atmosphere with the aim of climate change mitigation. Scientists and private stakeholders have proposed a wide range of technologies that intervene in the ocean as part of the global carbon cycle to increase the ocean’s carbon sequestration potential and storage capacity (Minx et al., 2018; GESAMP, 2019). Approaches include chemical enhancement of carbon storing properties of ocean water (e.g., ocean alkalinisation), a shift in the water column to increase uptake of carbon (e.g., artificial up- and downwelling), or the conservation and restoration of coastal and marine ecosystems with carbon storing properties (e.g., mangroves). These activities intentionally induce a change in the ocean’s biogeochemical and physical condition, or in marine ecosystems, for the purpose of higher carbon uptake and storage.
In comparison to terrestrial NETs, such as bioenergy with carbon capture and storage (BECCS), ocean-based interventions are subject to the interconnectedness of the marine environment, and of the ocean and human society, and consequentially demand international coordination in governance (Boucher et al., 2014; Minx et al., 2018). In addition to mitigating climate change, a change in the ocean’s condition due to ONET deployment may alter the marine environment to an extent where ecosystem service provision within and across jurisdictional boundaries is compromised (GESAMP, 2019). Governance of ONETs must consider the trade-offs between climate mitigation and impaired ecosystem services when assessing the modalities of allowing or restricting such technologies. The fragmented global governance framework in place creates additional challenges for a streamlined approach to governance of emerging ocean uses that potentially affect multiple areas of international cooperation (Biermann et al., 2009; Boyes and Elliott, 2014; Haas et al., 2021). Complexity within the global ocean governance framework is increased by the high uncertainties and many unknowns related to ocean and climate change (Payne et al., 2016), and related to the response of the marine ecosystem to many of such technological interventions (Renforth and Henderson, 2017).
This paper presents a review of direct, implicit and indirect linkages between eight selected ONETs and international environmental agreements (IEAs) including their underlying policy goals for the ocean, and ultimately outlines a global governance framework surrounding ONETs. The review builds on an analysis of potential direct and indirect, intentional and unintentional impacts of eight ONETs on the marine environment and links these to the regulations and policy goals of relevant international environmental agreements of the current ocean governance. It highlights main challenges for the comprehensive governance of ONETs and identifies synergistic opportunities for future governance. Building on a first-order assessment of interactions of ONETs with the ocean, and of potential effects of ONETs deployment on ocean condition and ecosystem service (ES) provision, the type and directionality of linkages between ONETs and relevant IEAs is investigated to identify and map the existing governance framework relevant for a comprehensive approach to regulating and managing ONET deployment. Drawing from recent publications that explore the challenges of ocean governance in light of rapidly increasing human uses of the ocean and the deteriorating condition of the marine environment (Brodie Rudolph et al., 2020; Singh and Ort, 2020; Spalding and de Ycaza, 2020; Stephenson et al., 2021), a broader perspective of (global) ocean governance is adopted in this study. This broader perspective attempts to bring together legal aspects of regulating human activities in the ocean for environmental protection and conservation (Singh and Jaeckel, 2018; Singh and Ort, 2020) with an understanding of the concept of environmental governance as it has developed in the social and political sciences. Pattberg and Widerberg (2015) describe global environmental governance as the system of institutions, actors, processes and governing instruments required to address environmental issues. For the purpose of this study, focus is placed on IEAs as governing instruments and core components of the governance system while widening the scope to include agreements beyond the direct and explicit regulation. Ostrom’s Institutional Analysis and Development (IAD) Framework (Ostrom, 1999; McGinnis, 2011; Ostrom, 2011; McGinnis and Ostrom, 2014) has been instrumental in guiding the study towards such a broader understanding of governance in the context of ONETs and the quest for comprehensive “governance” of ONET deployment, albeit without applying the IAD framework strictly for the assessments conducted.
2 Methodology
The research presented here considers eight ocean-based negative emissions technologies (ONETs), pre-selected as a study base by the EU-funded Horizon 2020 project OceanNETs1 which the research presented here is part of. Negative emissions technologies are considered ‘ocean-based’ in that their deployment interacts with one or more components of the ocean, such as the seafloor, ocean water or marine biomass (Boucher et al., 2014). These ONETs are a non-exhaustive representation of recent and emerging technological and scientific studies within the field (Fuss et al., 2018; Gattuso et al., 2018; Keller, 2018; GESAMP, 2019). The selected ONETs range in their status towards deployment from theoretical studies (artificial downwelling), to lab-based (direct electrochemical CO2 removal from seawater; terrestrial biomass dumping) and mesocosm experimentation (ocean alkalinisation), field testing (artificial upwelling; ocean fertilisation) and fully operational (blue carbon ecosystems). The presented research is limited to the assessment of ONETs’ interactions with the ocean (including coastal zones) during deployment whereby freshwater and terrestrial impacts, as well as related governance frameworks, are beyond the scope of this study. Impacts of technologies on the marine environment during other lifecycle phases, such as initial implementation (e.g., via shipping of materials, installation of infrastructure) are acknowledged in this study, but as the scientific literature currently provides limited information in this regard, the focus is on impacts linked to the operation of the technologies.
The identification and analysis of the governance framework surrounding selected ONETs encompassed four steps (see Figure 1). As a first step, a knowledge base was created for each of the eight technologies and captured in overview tables. A short online questionnaire was developed to gather expert knowledge on key aspects of ONET deployment that are relevant to the regulation and governance of ONETs governance. The questionnaire was administered with members from the OceanNETs project consortium and comprised of six questions pertaining to 1) the interaction between the eight selected ONETs and the ocean, 2) potential area and scale of deployment, 3) engaged maritime zone (coastal waters, exclusive economic zone, high seas) and parts of the ocean and water column (deep sea, surface waters etc.), 4) possible co-benefits, 5) possible environmental or socio-economic concerns, and 6) relevant factors to consider for ocean governance. The information gathered via the questionnaire served as starting point for an extensive literature review, which was carried out for each of the technologies. Both the GESAMP (2019) report of Working Group 41 “High Level Review of a wide Range of proposed Marine Geoengineering Techniques” and the National Academies of Sciences, Engineering, and Medicine (2022) report “A Research Strategy for Ocean-based Carbon Dioxide Removal and Sequestration” served as primary resources for the literature review. Variation in availability of scientific publications between the eight ONETs affected the review, with a more extensive literature base available for some, e.g., ocean alkalinisation and fertilisation, and limited available scientific knowledge for technologies in early research stages, e.g., artificial downwelling (Fuss et al., 2018; Minx et al., 2018; Nemet et al., 2018; GESAMP, 2019).
A qualitative description of the impact of each ONET on the ocean’s condition and its ES was carried out next (step 2). Building on the data compiled with the initial questionnaire and literature review in step 1, the potential impacts of the selected ONETs on the ocean were mapped along Essential Ocean Variables (EOVs), as defined for the Global Ocean Observing System to provide a structured framework for coordinating measurements and describing the ocean condition (Lindstrom et al., 2012; Miloslavich et al., 2018; Tanhua et al., 2019; Global Ocean Observing System, 2021; Satterthwaite et al., 2021). EOVs comprise a list of physical, biochemical and biological/ecosystem variables (Global Ocean Observing System, 2021). For this first-order mapping, EOVs were employed as they allow to structure possible physical, chemical, and biological/ecosystem changes in ocean condition induced by ONETs. The mapping exercise produced a qualitative description of each ONET’s effects on the EOVs. Impacts were differentiated as i) direct intentional impact on the ocean’s condition for higher carbon uptake and ii) potential direct unintentional impacts, and further grouped by (potential) impacts on the ocean’s biochemical, physical, and biological/ecosystem condition, e.g., an unintentional release of trace metals into seawater via ocean alkalinization (see Tables S1 to S8, Annex I, Supplementary Material). The impact assessment of “blue carbon ecosystems” was limited to coastal ecosystems, specifically to mangroves, salt marshes and seagrass meadows.
Based on these findings, and to further determine the potential indirect impact of ONETs on the environment and society, a second literature review was conducted on potential impact of ONETs on coastal and marine ES. For this purpose, the electronic scientific research database Science Direct was consulted using a key word search, combining the identified direct effects on the ocean (e.g., “coral reef recovery”) with the key words “ecosystem service*”, “marine”, “coast*” and “ocean”. For this mapping exercise, the Common International Classification of Ecosystem Services, Version 5.1 (Haines-Young and Potschin, 2018) was utilised to select relevant coastal and marine ES and describe the potential impacts of ONETs on the supply of these, grouped into provisioning services, regulation and maintenance services, and cultural services. These findings were determined as either indirect intentional impact (i.e., climate regulation) or potential indirect unintentional impact (e.g., enhancing or implicating food provision). The tables in Annex I of the Supplementary Material summarize the results of this qualitative first-order mapping of direct and indirect intentional and potential unintentional impacts of the assessed ONETS on ocean condition and ES. Table 1 provides an overview of the scheme developed for classifying the (potential) impacts.
In step 3, an identification of the governance framework relevant to ONETs was carried out. Results from step 2 determined that all ONETs may impact the ocean’s condition in a highly complex and transboundary manner if deployed, depending on scale and characteristics. The international environmental governance regime would function as overarching governance arena, comprised of legal frameworks and norms as well as the institutional arrangements and procedures in place to implement the goals set out in the individual frameworks. Results of the impact assessment determined that ONETs would further likely indirectly impact a wide range of global policy goals, such as protection of cultural heritage or food security. A total of 452 multilateral agreements, provided by the International Environmental Agreements Database Project (Mitchell, 2002-2022), were screened for relevance to the direct and indirect governance of ONETs. International agreements were selected for further analysis if identified as (explicitly, implicitly or indirectly) regulating or governing, or being relevant for the governance of potential impacts of ONETs on the ocean. Regional agreements (e.g., Antarctic Treaty System) were excluded to limit the scope of the study. Eight international frameworks were identified to form the governance framework for ONETs (see Table 2).
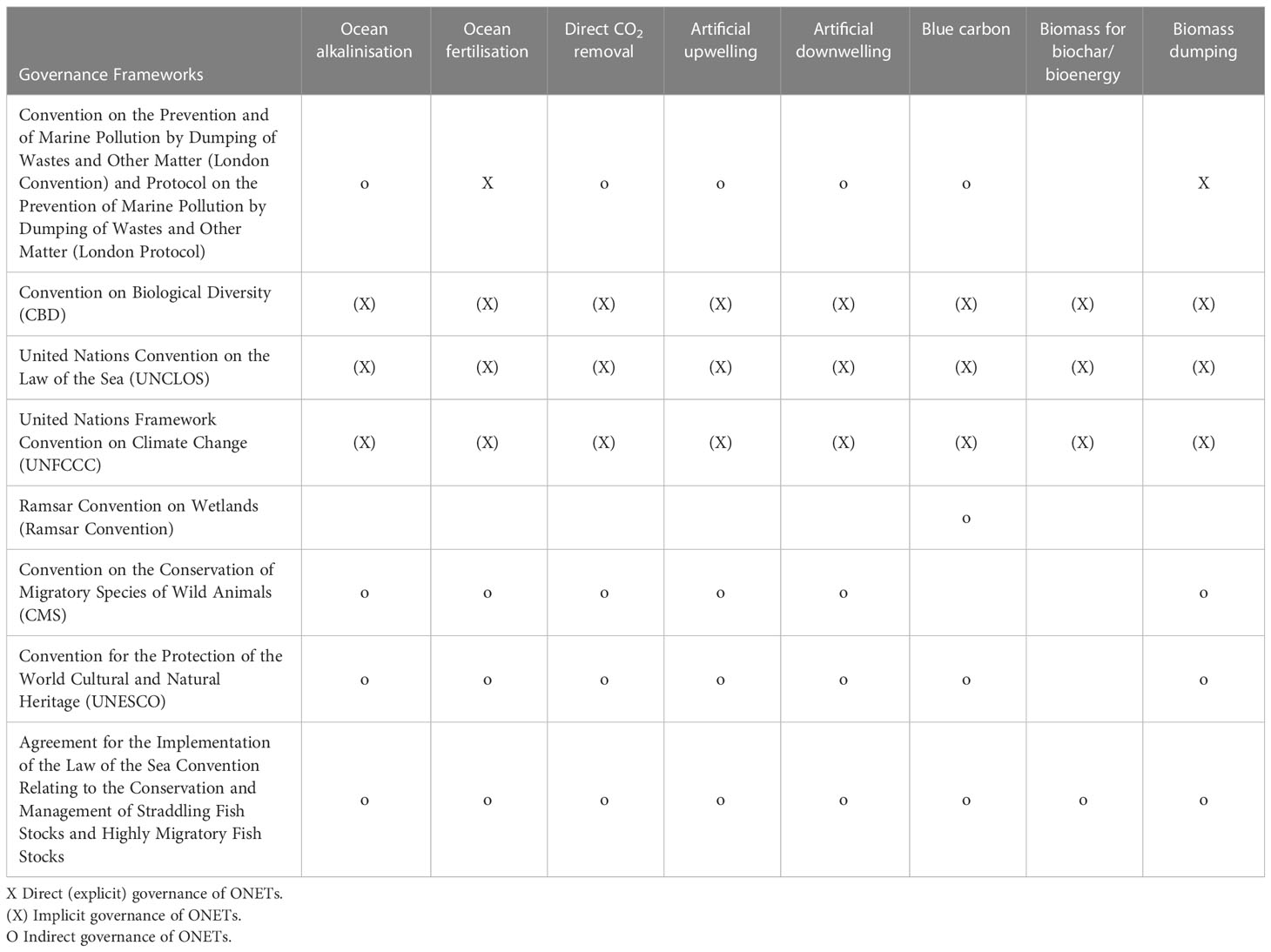
Table 2 Overview of direct (explicit), implicit and indirect governance of eight ONETs for reviewed governance frameworks.
Step 4, the analysis of selected governance frameworks, involved a review of the legislative text of each agreement including amendments, decisions, resolutions or supplementary documents. Table 3 provides an overview of the scheme developed for classifying and describing the different frameworks in terms of their relation to ONETs, i.e., whether they provide direct regulation or explicit guidelines for dealing with ONETs, implicitly establish a framework for governing ONETs, or comprise an indirect governance framework by addressing indirect and unintentional effects of ONETs on the marine environment. First it was determined if texts explicitly govern, i.e., regulate or in an explicit and direct way address, either geoengineering in the ocean or a specific ONET (e.g., Resolution LC-LP.1, 2008, on the Regulation of Ocean Fertilization) framed as ‘direct and explicit governance’ of ONETs. Next, it was determined which frameworks govern the direct impacts of ONETs on ocean condition (i.e., United Nations Convention on the Law of the Sea, UNCLOS; Convention on Biological Diversity, CBD), as well as the indirect intentional impact of ONETs on the ocean for regulating climate change (i.e., UNFCCC), framed as ‘direct implicit governance’ (see Table 2 and Figure 2). Finally, the identified international agreements were analysed in terms of whether they address governance of the potential indirect, unintentional impact of ONETs on the marine environment, via possible impacts on marine and coastal ES (e.g., UN Fish Stocks Agreement).
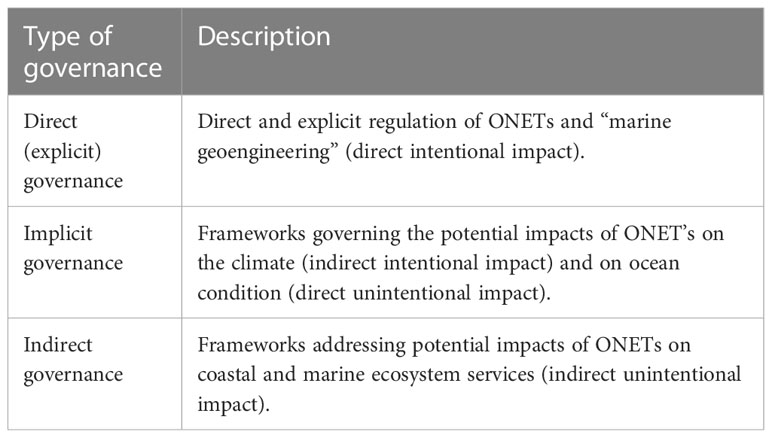
Table 3 Classification scheme applied for analysing the governance framework of ocean-based negative emission technologies (ONETs).
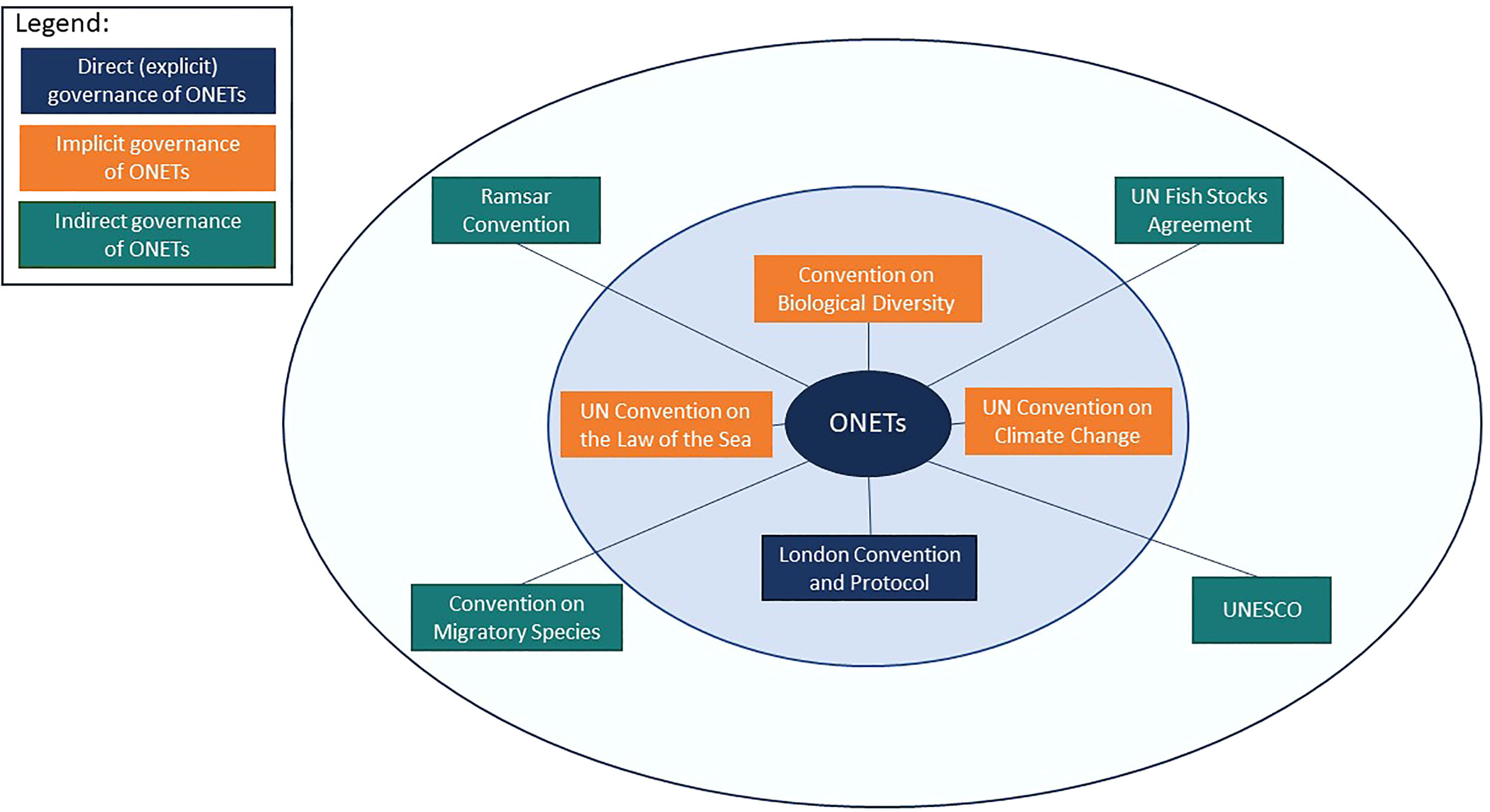
Figure 2 ONET governance framework. The “inner” circle represents direct explicit governance of ONETs and implicit governance of potential impacts of ONETs on ocean condition and climate regulation. The “outer” circle presents indirect governance of ONETs via governance of potentially impacted marine and coastal ecosystem services.
3 Results
3.1 Impacts of ONETs on ocean condition and coastal/marine ecosystem services
The assessment of ONETs’ impact on the ocean’s condition, conducted along Essential Ocean Variables (EOVs), identified a range of potential direct and indirect impacts on the ocean for each of the eight ONETs (see Supplementary Material, Tables S1–8). An impact of an ONET on the ocean was determined direct if a change in the ocean’s physical, biogeochemical, or biological condition was cited in literature. The analysis distinguishes between intentional (i.e., increase in carbon uptake and storage) and unintentional (e.g., increase in trace metals) potential changes in ocean condition. These findings were further reviewed to identify potential impacts on marine and coastal ES and to distinguish between the indirect intentional impact of ONETs on the ES of climate regulation and indirect unintentional impacts on other marine and coastal ES, such as food provision. Tables S1–8 of the supplementary material provides a list of the potential direct and indirect impacts of ONETs on ocean condition and coastal/marine ES as determined from the literature.
3.1.1 Ocean alkalinisation
Ocean alkalinisation, also known as ocean alkalinity enhancement or enhanced weathering, intends to change the ocean’s geochemical condition by adding alkaline substances (e.g., olivine) into the ocean to increase carbon uptake from the atmosphere (Renforth and Henderson, 2017; GESAMP, 2019). Such a change in seawater geochemistry encourages a range of impacts on the ocean’s condition, specifically, an increase in ocean alkalinity and a consequent limitation or reduction in acidification levels via the change in ocean water pH (Ilyina et al., 2013; Feng et al., 2016), which could enable a regional improvement in the state of otherwise deteriorating organisms, such as coral reefs without implicating the ocean’s primary productivity (Albright et al., 2016; Feng et al., 2016; Gore et al., 2019; Hall-Spencer and Harvey, 2019). Ocean alkalinisation could hence indirectly strengthen the range of ES these habitat-forming coastal organisms provide for society, as the species richness within coral reefs generates important fishing grounds (Fisher et al., 2015; Grafeld et al., 2017) as well as many cultural ES (Motuhi et al., 2016; Spalding et al., 2017; Woodhead et al., 2019). It has further been assessed that non-calcifying macro-algae would decline under alkalinisation (Koch et al., 2013) which could reduce harmful algal blooms in coastal waters (Hartmann et al., 2013) and indirectly counter related lost fishing opportunities for society (Moore et al., 2019) as well as reduce associated negative impacts on the health of people living in coastal areas (Manganelli et al., 2012). Further direct impacts of ocean alkalinisation on the ocean’s biological condition include a potential shift in phytoplankton species composition (Köhler et al., 2013; Gagern, 2019), the ultimate impact of which is difficult to assess, but could ultimately impact fish stocks and other ES provided by wild aquatic animals as phytoplankton make up the base of aquatic food webs (Naselli-Flores and Padisák, 2023). However, inadvertent effects of alkalinity enhancement on phytoplankton communities have been assessed as temporary in nature and deemed as low in relevance in comparison to the achieved climatic benefits (Ferderer et al., 2022). The possible release of trace metals from silicate minerals during the process of ocean alkalinisation (Hartmann et al., 2013) could have a variety of indirect impacts on ES and potentially cause toxicity in various marine organisms (Jin et al., 2021). However, a recent paper by (2022) suggests that dissolved nickel may not have a strong effect on phytoplankton. Finally, the transport of alkaline materials, including for deployment in the ocean, may require an expansion of the global shipping fleet and traffic (Caserini et al., 2021), which would potentially augment linked impacts on the ocean, such as unintentional transport of invasive alien species via ships, enhanced pollution and noise (Keller et al., 2011).
3.1.2 Ocean fertilisation
Ocean fertilisation for CDR constitutes the addition of either micronutrients, most prominently iron, or macronutrients such as nitrogen compounds for the purpose of increasing phytoplankton stocks in the ocean for enhanced carbon sequestration and potential long-term carbon storage as phytoplankton sink to the seafloor (Boyd et al., 2007; Secretariat of the Convention on Biological Diversity, 2009; Moore et al., 2013; GESAMP, 2019). Temporary lowering of CO2 concentrations in surface waters via fertilisation allows for a temporary change in surface pH until equilibrium with atmospheric CO2 is re-established. However, sinking of organic material from the increased productivity and its re-mineralisation in the waters beneath the surface mixed layer causes an increase of CO2 in deeper waters, which could cause further acidification of the deep sea and negatively impact related biodiversity (Cao and Caldeira, 2010). Ocean fertilisation relies on use of limiting nutrients which thereby may be trapped and become unavailable for biological production in other regions (“nutrient robbing”) (Shepherd, 2009; Oschlies et al., 2010a; National Academies of Sciences, Engineering, and Medicine, 2022). The increase in phytoplankton caused by iron fertilisation has been proposed as a measure to enhance fish stocks (GESAMP, 2019) as these could offer new feeding grounds (Galbraith et al., 2019). Simultaneously, the intentional elevation of nutrient levels through fertilisation has been reported to cause a depletion of mid-seawater oxygen levels and has been linked to an increase in harmful algae blooms (Lampitt et al., 2008). Such events have, however, not been reported for the open ocean where ocean fertilisation would likely take place (Silver et al., 2010). While uncertainty remains with regards to potential indirect impacts of ocean fertilisation on the biogeochemical condition of the ocean (Yoon et al., 2018), it has been deemed near-inevitable that entire ecosystems, from the upper ocean to the seafloor, will be altered (Williamson et al., 2012).
3.1.3 Direct electrochemical CO2 removal from seawater with carbon capture and storage
In this approach, seawater is physically extracted from the ocean via pumps and electrochemically processed to remove dissolved inorganic carbon as CO2 gas or calcium carbonate (Ocean Visions, 2021), after which the extracted carbon is transported to sites for long-term storage and the basified seawater can be added back into the ocean (GESAMP, 2019; Sharifian et al., 2021). The ocean’s alkalinity is enhanced and an increased uptake of atmospheric CO2 in surface waters is achieved in an effort to restore a carbonate equilibrium between the ocean and the atmosphere (de Lannoy et al., 2018; GESAMP, 2019; Sharifian et al., 2021). The intentional impact on the ocean’s biogeochemical condition is equal to that of ocean alkalinisation, and consequently, direct and indirect unintentional impacts on ocean condition and ES, respectively, are similar (see Section 3.1.1). However, as the technological approach is still in early stages of development, impact studies have not been published to date (GESAMP, 2019) and there remain many unknowns with regards to the unintentional impact of removing large amounts of dissolved organic carbon from seawater (Ocean Visions, 2021). In order to correctly assess the level of potential impacts of ONETs on the ocean environment, it is necessary to have an understanding of the scale of deployment, including number of ships needed to achieve set CO2 drawdown targets as well as number of intake structures. Depending on the scale of deployment, ES provision may be subject to process and infrastructure-associated disturbances of the seabed during construction of the intake structure as is the case for other seawater-pumping technologies (i.e., desalination, power-plant cooling, material extraction) (Lattemann and Höpner, 2008; Eisaman et al., 2018).
3.1.4 Artificial upwelling
For artificial upwelling, pipes or wave pumps are utilised to pump up cold, nutrient-rich water from the deep sea with the purpose to fertilize surface waters and stimulate phytoplankton growth (GESAMP, 2019). As with ocean fertilisation, the newly generated stock of phytoplankton should fix additional CO2 in its biomass and potentially store carbon for a period of at least several hundred to 1,500 years as phytoplankton sinks to the ocean floor. Addition of nutrients to the upper ocean similarly causes an enhancement of biological production (Oschlies et al., 2010b) and could impact associated ES and industries, such as fishing or mussel farming (Handå et al., 2014; Casareto et al., 2017; Ortiz et al., 2022). In addition to an impact on phytoplankton in the upper ocean, increased carbon drawdown may have an impact on deep-sea biodiversity and linked ES (Pan et al., 2016; Levin et al., 2023). Artificial upwelling of ocean water also impacts the ocean’s physical condition by locally cooling down surface water temperatures, which could impact ocean circulation and reduce extreme events such as hurricanes, as well as mitigate thermal stress on vulnerable ocean ecosystems, e.g., coral reefs (Schneider et al., 2017; Sawall et al., 2020). Simultaneously, the decrease in pH achieved through upwelling could aggravate ocean acidification and compromise marine organisms further (Pan et al., 2016). In terms of infrastructure for artificial upwelling, Yool et al. (2009) calculated that a great quantity of pumps, up to several millions, would be needed to achieve the intentional impact on climate change regulation. Beyond the ecological implications of initial installation, the implemented large-scale infrastructure may interfere with activities such as fishing and shipping, endanger marine wildlife via risk of entanglement, or cause debris and pollution as pump material malfunctions and maintenance services to fix broken pipes may transport invasive alien species (Barboza et al., 2019; National Academies of Sciences, Engineering, and Medicine, 2022). In addition, simulations show that the termination of the technology would likely result in higher global mean air temperatures as stored heat would be released back to the surface ocean and atmosphere (Oschlies et al., 2010b).
3.1.5 Artificial downwelling
The downward transport of carbon-saturated surface waters into deeper ocean water for long-term carbon storage, termed artificial downwelling, intends for an alteration of the ocean’s physical condition via a shift in the water column, e.g., by using pipes (GESAMP, 2019). Such large-scale shifts in the water column will change the density structure of the ocean and will likely change ocean circulation over tens of kilometres (National Academies of Sciences, Engineering, and Medicine, 2022). Along with the intentional transport of CO2, installed pipes may transport surface heat, fertilising nutrients, as well as oxygen into sub-surface waters (Stigebrandt et al., 2015). Artificial downwelling may concurrently improve upon ocean cycling and be utilised to mitigate hypoxia (Liu et al., 2020), but impact assessments have thus far not been undertaken to conclude the extent of such impacts for artificial downwelling for the purpose of CO2 removal (GESAMP, 2019). The scale of space and infrastructure needed to deploy the technology would reflect that of artificial upwelling, and similar impacts on marine ES can be expected (National Academies of Sciences, Engineering, and Medicine, 2022). Finally, the likeliness of deployment of this approach for CDR has been identified as relatively low as estimated costs for deployment are high while many uncertainties with regards to enduring effectiveness remain (Zhou and Flynn, 2005).
3.1.6 Blue carbon ecosystems
A variety of components of marine and coastal ecosystems (e.g., mangroves, macroalgae) can sequester carbon (known as ‘blue carbon’) from the atmosphere and, if left undisturbed, store it in their biomass and sediments indefinitely (Nellemann et al., 2009). The restoration and sustainable management, including conservation and protection, of coastal blue carbon ecosystems (specifically mangroves, seagrass meadows and saltmarshes) can have additional benefits such as reduce wave height and coastal erosion, which can ultimately protect coastal regions against flooding and storm surges (Krauss et al., 2003; Shepard et al., 2011; Hochard et al., 2019). Moreover, certain coastal blue carbon ecosystems have the ability to reduce deoxygenation of ocean water, reduce nutrients and filter trace metals in coastal waters (Sousa et al., 2010; Marques et al., 2011; Laffoley and Baxter, 2019), and hence have the ability to restore many regulatory ES provided by the ocean (Unsworth et al., 2012). The new habitat provided for many marine species by restoring and sustainably managing coastal blue carbon ecosystems additionally serves the spill-over effect benefitting provisioning ES via e.g., fisheries and mussel farms (Cullen-Unsworth and Unsworth, 2013). Coastal blue carbon ecosystems also provide extensive cultural ES to society, as they provide opportunities for recreational and tourism uses (Uddin et al., 2013; Queiroz et al., 2017), educational and scientific value (Friess et al., 2020), can be part of spiritual interactions of local communities (Uddin et al., 2013) and embody environmental heritage for future generations (Mitra, 2020). While a wide range of co-benefits has been identified for the implementation and restoration of coastal blue carbon ecosystems, recent publications highlight that a potential release of methane and nitrous oxide, especially linked to the formation of new sites, can offset climate mitigation benefits attributed to blue carbon (Rosentreter et al., 2021; Zhang et al., 2022). An assessment of carbon stocks in previously less studied seagrass meadow regions determined that for some cases, CO2 released via carbonate sediment production may even offset CO2 sequestration and storage benefits of the coastal blue carbon ecosystem (Howard et al., 2018). In addition, carbon burial rates may differ greatly across blue carbon sites of the same ecosystem type, and the cost-effectiveness of blue carbon for the primary purpose of CDR has thus been determined as highly uncertain (Williamson and Gattuso, 2022). It should also be noted that environmental and economic trade-offs may arise when the safeguarding and expansion of carbon-rich coastal ecosystems means to forgo obtained ES, such as mangrove forests for local fuel provision (Wylie et al., 2016).
3.1.7 Marine biomass for biochar or bioenergy with carbon capture and storage
In this approach, macroalgae are cultivated in coastal and near-shore areas for uptake of carbon and harvested for later processing on land to biochar or biofuel in conjunction with carbon capture and storage (Nellemann et al., 2009; GESAMP, 2019). The ‘ocean-based’ extent of this ONET constitutes the large-scale implementation and operation of seaweed farms, which have been proposed both for the nearshore and offshore environment, depending on potential conjunctions with already established industries (e.g., aquaculture, offshore windfarms) (GESAMP, 2019). Extensive macroalgae farms in coastal areas may dampen wave energy (Mork, 1996) and consequently better protect shorelines from coastal erosion (Hasselström et al., 2018). Macroalgae have the ability to sequester eutrophying nutrients (Kim et al., 2015) and passively remove trace metals from ocean water (Davis et al., 2003), creating a positive impact on regulation of ocean water condition (Hasselström et al., 2018). Macroalgae cultivation can also increase release of trace gases that contain sulphur or halogens (Leedham et al., 2013), impacting the ocean’s net regulation of atmospheric composition and condition via emission of halocarbons (Phang et al., 2015). The implementation of seaweed farms forms new habitats for various marine species and can provide significant value for food web dynamics between organisms and ecosystem (Hasselström et al., 2018) as well as catch composition for fisheries, and even increase catches via spill-over effect in comparison to catches around vegetation-free seafloors (Eklöf et al., 2013). Seaweed farms may release particulate or dissolved organic matter into the ocean that impact microbial production, oxygen consumption and food supply (Levin et al., 2023). Algae cultivation may additionally impact physical and experiential interactions with the environment in cases where seaweed farms replace recreational areas (Cabral et al., 2016).
3.1.8 Terrestrial biomass dumping
In this approach, carbon-rich terrestrial biomass is dumped into the ocean via ships, from where it sinks to the ocean floor to be stored upon marine sediments indefinitely, along with the stored carbon (Metzger et al., 2002). During the descent of biomass to the seafloor, particulate or dissolved organic matter could be released and alter microbial production, oxygen consumption and food supply in the mesopelagic realm (Levin et al., 2023). The physical impact of biomass onto the seafloor may cause physical resuspension and disturb deep-sea marine sediments and organisms (Strand and Benford, 2009; Levin et al., 2023), and disturb methane reservoirs (Kvenvolden, 1993; Thurber et al., 2014). Terrestrial biomass as food source on the seafloor may attract a large number of predators and change species interactions in the deep sea, and potentially impact commercial fishing (Levin et al., 2023). Introduction of terrestrial organic matter may cause reduced oxygen-levels and potential increases in hydrogen sulphide, methane, nitrous oxide and nitrogen and phosphorus compounds on the seafloor via decay of by-products entering ocean water (Metzger and Benford, 2001), further impacting the deep sea’s processes of bioturbation and bioremediation (Thurber et al., 2014). Many unknowns remain with regards to impacts of large-scale terrestrial biomass dumping on the ocean floor and biodiversity (Metzger et al., 2002; Strand and Benford, 2009).
3.2 International governance framework surrounding ONETs
Based on the analysis of ONETs’ intentional and potential unintentional impacts on ocean condition and related ES, this paper outlines a governance framework surrounding the technologies that encompasses aspects beyond the explicit regulation of ONETs and marine geoengineering. The analysis presented here distinguishes between
● direct governance that has explicitly been adopted by the international community for the purpose of regulating ONETs (section 3.2.1);
● implicit governance of potential impacts of ONET’s on ocean condition, including impacts on the ocean for regulating climate change (section 3.2.2); and
● indirect governance which addresses potential indirect and unintentional impacts of ONETs on coastal and marine ecosystem services (section 3.2.3).
In total, eight international governance frameworks have been identified to make up the extended governance landscape surrounding ONETs (see also Table 2 and Figure 2, and Tables S9 to S16 of the supplementary material).
3.2.1 Direct (explicit) governance of ONETs
Scientific research and potential future deployment of ONETs, or “marine geoengineering”, is directly and explicitly regulated by the Convention on the Prevention of Marine Pollution by Dumping of Wastes and Other Matter and the 1996 Protocol on the Prevention of Marine Pollution by Dumping of Wastes and Other Matter. Table S9 of the supplementary material provides a detailed analysis.
3.2.1.1 Convention and Protocol on the Prevention of Marine Pollution by Dumping of Wastes and Other Matter
The Convention on the Prevention of Marine Pollution by Dumping of Wastes and Other Matter (London Convention, LC), and the 1996 Protocol on the Prevention of Marine Pollution by Dumping of Wastes and Other Matter (London Protocol, LP) meant to modernise and completely replace the Convention in time, is the only international agreement to explicitly govern ONETs, namely marine geoengineering and, for the time being, specifically ocean fertilisation (see Table 2 and Table S9, Supplementary Material). The non-binding LC/LP Resolution on the Regulation of Ocean Fertilisation (2008) acknowledges that “the scope of the London Convention and Protocol includes ocean fertilisation activities” and that ocean fertilisation “should be considered as contrary to the aims of the Convention and Protocol and not currently qualify for any exemption from the definition of dumping [ … ]” (IMO, 2008). The LC sets forth that Contracting Parties prevent the pollution of the sea by dumping, i.e. the deliberate disposal, of any wastes or other matters that may “create hazards to human health, to harm living resources and marine life, to damage amenities or to interfere with other legitimate uses of the sea” (IMO, 1972 - Art. 1 and 3). This indicates that ONETs with harmful impacts to the condition of the ocean via dumping, such as ocean alkalinisation, by which the addition of alkaline matter can release trace metals into ocean water and further impact biogeochemical cycles (Hartmann et al., 2013; Hauck et al., 2016), are governed by the framework. Further, dumping of terrestrial biomass on the seafloor is covered by Annex 1 of the London Protocol via the category of wastes “Organic material of natural origin”, and by Annex 1 of the London Convention LP as “Uncontaminated organic material of natural origin”, which requires therefore permitting under the national regulations that implement the Convention or Protocol.
An Amendment was made to the London Protocol on marine geoengineering activities in 2013, which allows for ‘legitimate’ research activities and mandates “Contracting Parties shall not allow the placement of matter into the sea from vessels, aircraft, platforms or other man-made structures at sea for marine geoengineering activities” as listed in the related Annex, but the Amendment is not yet in force. While the Annex is limited to the previously addressed ocean fertilisation (IMO, 2013 - Art. 6bis), a process is underway to add further types of marine geoengineering approaches to the Annex, which allows for the Amendment to give regulatory structure for potential future regulation of ONETs that would otherwise be considered dumping. Additional ONET activities could accordingly be subject to indirect regulation if they encompass “placement” of material into the ocean, e.g., ocean alkalinisation, terrestrial biomass.
3.2.2 Implicit governance of ONETs
The results of the impact assessment determined that ONETs aim to directly alter the ocean’s physical, biogeochemical or biological/ecosystem condition in a variety of ways with the intention to reduce atmospheric carbon via enhanced sequestration and storage within the ocean. Three governance frameworks were identified as relevant for the implicit governing ONETs via these possible impacts on the marine environment: the Convention on Biological Diversity, the United Nations Convention on the Law of the Sea, and the United Nations Framework Convention on Climate Change. Tables S10, S11 and S12 of the supplementary material provide detailed analyses for these frameworks.
3.2.2.1 Convention on Biological Diversity
The Convention on Biological Diversity (CBD) positioned itself concurrently to the LC/LP proceedings on ocean fertilisation in support of the resulting Resolution on the Regulation of Ocean Fertilisation (LC-LP.1 2008) and urged its own Parties to apply “the precautionary approach as well as customary international law” relevant to geoengineering “ (UNEP, 2016) (see also Table 2 and Table S10, Supplementary Material). In accordance with the precautionary approach the CBD further urges that risks to the environment, biodiversity as well as socio-cultural impacts must be considered. The CBD implicitly promotes coastal blue carbon ecosystem management by encouraging parties to “implement ecosystem management activities, including the [ … ] conservation of mangroves, salt marshes and seagrass beds” to support the objectives of the UNFCCC (UNEP, 2010a - para 8(n)). Further, the global targets for 2030 of the Kunming-Montreal Global Biodiversity Framework (Convention on Biological Diversity, 2022), and particularly Targets 8 and 11, are indicative of implicit support of blue carbon ecosystems, as well as of marine biomass for biochar or bioenergy with carbon capture and storage. By urging to increase resilience “through mitigation, adaptation, and disaster risk reduction actions, including through nature-based solution and/or ecosystem-based approaches, while minimizing negative and fostering positive impacts of climate action on biodiversity” (Convention on Biological Diversity, 2022 - Target 8), the newly adopted framework can also be interpreted as cautioning that any climate action taken should keep negative impacts on (marine) biodiversity to a minimum.
3.2.2.2 United Nations Convention on the Law of the Sea
The United Nations Convention on the Law of the Sea (UNCLOS) does not explicitly govern ONETs and the initial debates surrounding ONETs have occurred largely outside of UNCLOS proceedings (Redgwell, 2012). But UNCLOS governs the direct impacts of ONETs on the ocean’s condition, which is why it is considered in this assessment as a component of the implicit governance framework of ONETs (Table 2 and Figure 2). UNCLOS mandates the general protection and preservation of the marine environment (United Nations, 1982 - Art. 192) across the entirety of the ocean, including the high seas, from its 168 parties and consequentially regulates ONETs that impact either. UNCLOS requires States that have permitted activities that could cause harm to the marine environment via pollution to take adequate measures for monitoring the (potential) effects of such activities on the marine environment and to keep these under surveillance (United Nations, 1982 - Art. 204), further demanding that States assess and report on potential harmful effects of activities permitted in Article 206 of UNCLOS (United Nations, 1982), and address pollution from seabed activities by e.g. adopting appropriate regulations (United Nations, 1982 – Article 208). States are further required to take necessary measures against harmful pollution to the ocean from the use of technologies under their jurisdiction, which may be relevant for ONET activities in the future (United Nations, 1982 – Art. 196), it must be noted however, that the mentioned provisions are untested in practice.
3.2.2.3 United Nations Framework Convention on Climate Change
The socio-environmental issue of climate change as “common concern of humankind” has been regulated on the global scale by the United Nations Framework Convention on Climate Change (UNFCCC) since 1992 and includes the ocean within its definition of the climate system via the hydrosphere. Limiting global warming as set out by the Paris Agreement in 2015, the IPCC put forward mitigation pathways compatible with 1.5°C. These pathways include CO2 removal (i.e., CDR) and underline that global efforts for emissions reductions need to be supplemented by additional removal of atmospheric CO2 (e.g., via NETs) to stay below 1.5°C global warming (Rogelj et al., 2018). Further, in its sixth assessment report chapter on climate change mitigation, the IPCC notes that “[T]he deployment of CDR to counterbalance hard-to-abate residual emissions is unavoidable if net zero CO2 or GHG emissions are to be achieved” (Intergovernmental Panel on Climate Change, 2022). The UNFCCC convention acts as governance framework surrounding the indirect intentional impact of ONETs to reduce atmospheric carbon and accordingly implicitly governs ONETs. Official UNFCCC negotiations have to this date not explicitly included geoengineering or negative emissions technologies. Increasing regulatory attention has been received for blue carbon ecosystems since the topic was first raised at COP15 (Nellemann et al., 2009). While the term itself has not been taken up within the framework, the 2013 Wetlands Supplement for National GHG Inventories (IPCC, 2014) provides guidance on specified management activities in coastal areas of mangroves, tidal marshes and seagrass meadows, which belong to the most prominent coastal blue carbon ecosystems. The UNFCCC REDD+ mechanism has further provided market funding for individual coastal blue carbon ecosystems e.g., mangroves. The UN Ocean and Climate Change Dialogue of 2022, mandated by COP26, further urged for the ocean to be included in climate solutions and specifically supported the integration of coastal blue carbon ecosystems into UNFCCC processes, such as Nationally Determined Contributions (UNFCCC, 2022).
3.2.3 Indirect governance of ONETs
The results of the impact assessment determined a range of environmental international agreements to further consider in the wider context of ONET governance, as their policy objectives may be indirectly (positively or negatively) impacted by ONET deployment. Linking the technologies’ identified unintentional indirect impacts on marine and coastal ES with relevant international environmental governance frameworks results in an indirect governance framework for ONETs (see Figure 2). Policy goals underlying the frameworks identified as relevant in this section are potentially impacted by such indirect unintentional impacts of ONETs on the marine environment and should be taken into consideration for comprehensive future regulation and management of ONET deployment to ensure policy coherence, to minimise possible negative effects, and maximise possible co-benefits.
In total, four IEAs were identified as part of the indirect governance framework surrounding ONETs (see also Table 2 and Figure 2, and Tables S13-to S16 of the supplementary material):
● Convention on Wetlands of International Importance Especially as Waterfowl Habitat (Ramsar Convention) (Table S13)
● Convention on the Conservation of Migratory Species of Wild Animals (CMS) (Table S14)
● Convention for the Protection of the World Cultural and Natural Heritage (UNESCO) (Table S15)
● Agreement for the Implementation of the Law of the Sea Convention Relating to the Conservation and Management of Straddling Fish Stocks and Highly Migratory Fish Stocks (UN Fish Stocks Agreement) (Table S16)
The results of the analysis presented below are grouped by Haines-Young and Potschin (2018) ES sections (provisioning ES; regulation & maintenance ES; cultural ES).
3.2.3.1 Indirect ONET governance relevant for provisioning ecosystem services
Several ONETs were identified as potentially impacting wild and cultivated animals or plants for nutrition, materials or energy (see Tables S1-S8, supplementary material). For example, ocean alkalinisation can counter ocean acidification, by which habitat-forming coastal habitats could be restored and which could enable a positive spill-over of economically relevant species for global fisheries (Grafeld et al., 2017). In 2018, global fisheries production reached highest ever recorded levels, and with nearly 40 million people globally employed in the fisheries sector the importance of fish stocks for the world economy is evident (FAO, 2020). The UN Agreement for the Implementation of UNCLOS relating to the Conservation and Management of Straddling Fish Stocks and Highly Migratory Fish Stocks (UN Fish Stocks Agreement) regulates fisheries resources within and beyond the EEZ and sets out principles for the conservation and management of specific fish stocks based on the precautionary approach and the best available scientific information. The Agreement has the objective of optimum utilisation of fisheries resources and additionally mandates a general protection of biodiversity in the marine environment (UN, 1995 - Art. 5(g)), offering indirect governance of ONETs impact on fish stocks. The UN Fish Stocks Agreement mandates States to “adopt, where necessary, conservation and management measures [ … ] to [restore] populations [ … ] above levels at which their reproduction may become seriously threatened” and encourages Parties to “promote and conduct scientific research and develop appropriate technologies in support of fishery conservation and management” (UN, 1995 - Art. 5 (e and k)). ONETs with positive impacts on economically relevant species could be considered as technological conservation measure to increase stocks of species to ‘support fishery conservation’ as mandated by Art. 5(k). For example, growth limitation of marine fish due to low iron availability may be overcome via iron fertilisation, potentially enhancing catches (Galbraith et al., 2019). Especially coastal blue carbon ecosystems as habitats for a wide range of species has been assessed to impact provisioning ES with positive benefits for fisheries and mussel farms (Cullen-Unsworth and Unsworth, 2013). Furthermore, the Convention on Wetlands of International Importance Especially as Waterfowl Habitat (Ramsar Convention) aims to conserve and restore wetlands, and specifically promotes the “conservation, restoration, and sustainable management of coastal blue carbon ecosystems” for their many benefits to society (Ramsar Convention on Wetlands, 2018), one of which can be food provision.
3.2.3.2 Indirect ONET governance relevant for regulation and maintenance ecosystem services
Artificial downwelling has been linked to mitigating hypoxia in ocean waters (ES: ‘lifecycle maintenance, habitat and gene pool protection’), supporting the objective of all of the above IEAs (Ramsar Convention, CMS, UNESCO, UN Fish Stocks Agreement) to conserve the marine environment. At the same time, the potential shifts in water temperature via artificial up- and downwelling may negatively impact species vulnerable to such changes in ocean condition. The Convention on the Conservation of Migratory Species of Wild Animals (CMS) provides a list of migratory endangered species for which Parties to the CMS are asked “to prevent, remove, compensate for or minimize, as appropriate, the adverse effects of activities or obstacles that seriously impede or prevent the migration of the species; and to the extent feasible and appropriate, to prevent, reduce or control factors that are endangering or are likely to further endanger the species, including strictly controlling the introduction of, or controlling or eliminating, already introduced exotic species” (CMS, 1979 - Art. 3). Marine migratory species included in Appendix I of the CMS may be especially vulnerable to changes in ocean condition, e.g., temperature shifts via artificial upwelling and downwelling. The level of uncertainty and persisting knowledge gaps in ocean connectivity are a key constraint and ONET-related pressures to individual migratory species would need assessing.
3.2.3.3 Indirect ONET governance relevant for cultural ecosystem services
Marine and coastal ecosystems offer a wide range of cultural ES, such as intellectual and representative or physical and experiential interactions with the environment. Especially restoration of coastal blue carbon ecosystems can safeguard the cultural value diverse coastal species for future generations (Mitra, 2020). The Convention for the Protection of the World Cultural and Natural Heritage (UNESCO Convention) aims for Parties to avoid any deliberate measures which might directly or indirectly damage the cultural and natural heritage (UNESCO, 1972 - Art. 6). In addition, Convention has recognised coastal blue carbon ecosystems as ‘natural features of outstanding universal value’ and includes 21 marine sites in part for their carbon storing abilities on the UNESCO World Heritage List (UNESCO, 2020).
4 Discussion
ONETs have been proposed as human response to climate change, to enhance uptake of atmospheric CO2 in the ocean and mitigate climate-induced pressures on the planet, including e.g., ocean acidification (Harvey, 2008; Hartmann et al., 2013; Taylor et al., 2016). Future international climate change policy based on IPCC reports is likely set to rely on the inclusion of ONETs as part of the portfolio to achieve net zero emission targets (IPCC, 2022), though the degree to which CDR will be a necessary component to reach global emission reductions targets is still being debated (Buck et al., 2023; Lund et al., 2023). The assumptions made by the IPCC have generated scientific and economic interest in the topic, which in turn may promote the streamlining of ocean-based negative emissions into national climate mitigation strategies (Boettcher et al., 2021). The results presented in this article emphasise the need for inclusion of the ocean environment in CDR decision-making together with potential interconnected impacts to humanity. The presented review of eight ONETs highlights the possible range of biogeochemical, physical and ecosystem changes they could cause to the ocean. A variety of co-benefits and potential negative impacts on ES, as well as persisting uncertainties and unknowns, has been identified and emphasises the complexity of the challenge that ONETs pose for comprehensive ocean governance.
Previous studies have detailed the initial international policy response to ocean fertilisation experiments, and later marine geoengineering in general, and have reflected that ONET deployment will place new demands on the international law and governance system (Galaz, 2012; Williamson et al., 2012; Bodle et al., 2014; Magnan et al., 2016; Gattuso et al., 2018; McGee et al., 2018; Boettcher et al., 2021). This paper builds on this research as it reflects on how ONETs are situated within the broader global ocean governance framework when considering the technologies’ potential intended and unintended impacts on the coastal and marine environment, as well as linked ES. The results of this study suggest a comprehensive approach to governing marine CDR that considers both co-benefits and trade-offs between climate and ocean protection as well as other policy goals. An ocean-centric perspective on ONET governance as additional maritime activity rather than regulation of potential environmental harm, as it is currently governed under the LC/LP, offers a more holistic entry-point to the topic, putting potential trade-offs and synergies within the ocean realm (and beyond) forward for consideration in addition to significant climate mitigation benefits, rather than directly compromising between climate action and other goals of the international community. This approach also reflects propositions made by Dooley et al. (2021) to navigate decision-making based on the impacts of CDR activities on the environment rather than primarily as a contribution to climate mitigation. Careful consideration of the range of potential impacts of ONETs, in addition to the intended climate effects, will allow decision-makers to navigate the pathway towards potential future deployment of the technologies in a social-ecological system-based manner that is cognisant of the overall complexity.
The LC/LP, administered by the International Maritime Organisation (IMO) as a specialised agency of the United Nations, is the explicit regulatory instrument for ocean fertilisation and other future endeavours into marine geoengineering. The regulatory approach includes a detailed “Assessment Framework for Scientific Research involving Ocean Fertilisation” that demands careful inspection of proposals for scientific research for e.g., impacts on the environment. A case-by-case review alone of proposed ONET activity may, however, be unfit for purpose considering the outlined demands for comprehensive approaches. Recognising the interaction of a single ONET with the ocean, though a necessary assessment, will not provide an adequate knowledge base for comprehensive decision-making. Effective (and large-scale) ocean-based CDR for limiting global warming is expected to involve a portfolio of ONETs, rather than following a single-technology approach (Minx et al., 2018). The interdependent nature of the ocean (and climate) system with and between technologies therefore poses an overarching challenge within which ONET governance must operate. A comprehensive assessment framework for ONETs would need to consider the sum of all parts, i.e., encompass cumulative direct and indirect impacts of each added ONET activity to the ocean system, and within potential limitations in availability of ocean data (Moltmann et al., 2019), which poses added challenges for decision-making. A comprehensive governance framework accordingly requires, besides the integration of relevant frameworks and processes (see Figure 2 for a proposed wider framework), integration of strategic foresight mechanisms to bridge gaps resulting from extensive uncertainties and unknowns linked to ONET deployment in a changing ocean and climate.
Furthermore, ONET governance can be deemed decision-making under “deep uncertainty” (Constantino and Weber, 2021), and can lead to policy paralysis where inaction can erroneously appear like the leading way forward (Polasky et al., 2020). While risk characterisation mandated by the LC Assessment Framework includes an assessment of uncertainty associated with proposed activities, an integrated foresight-oriented approach of anticipatory governance may be better suited to regulating ONET activities in a holistic manner, especially as the future state of the world and the ocean environment itself holds many uncertainties. Governance and decision making with regards to global climate change mitigation is simultaneously fuelled by an urgency to act (Orlove et al., 2020), which may impact effective ONET governance as significant components of “good” governance, such as general transparency and early stakeholder engagement, may be skipped as CDR becomes more relevant to the global climate change strategy (Moon et al., 2017).
The global ocean governance framework is set up in a fragmented sectoral regime of institutional frameworks that is often criticised for being in itself unfit for purpose as it does not match well with the spatial and temporal specificities of the ocean as a fluid, interconnected realm (Young et al., 2007; Mahon et al., 2015; Wright et al., 2016; Grip, 2017; Watson-Wright and Luis Valdés, 2019). These issues also come to a fore in the context of marine CDR and possible future and large-scale deployment of ONETs. For instance, the Parties of the CBD have restated the Resolution made under the LC/LP to limit ocean fertilisation activities to those of a research character until associated risks are appropriately assessed and considered. Further, the CBD has positioned itself against geoengineering activities with potential adverse effects to biodiversity in general (UNEP, 2010b). The dual approach within the current global ocean governance framework, directly regulating ocean fertilisation and potentially further marine CDR technologies in the sense of avoiding environmental impacts from a specific source (LC/LP), as well as treating ONETs as potential threat to conservation of marine living resources (CBD), indicates the complexities of ONET governance within a fragmented framework. These two frameworks have different mandates (LC/LP: prevention of pollution; CBD: conservation), geographic scope (LC/LP: the global ocean; CBD: for components of biological diversity, areas under national jurisdiction; for processes and activities, both within and beyond national jurisdiction), differing parties to their convention, a different level of binding force for relevant decisions and resolutions (LC/LP: binding, CBD: non-binding), and their processes are not integrated. Besides such duplicity of efforts, one of several prevailing issues of current ocean governance (Singh and Ort, 2020), there are also persisting gaps in the direct governance framework that require attention. Emerging ONETs that alter ocean condition for higher carbon uptake by means other than dumping of substances, e.g., artificial upwelling, are not (yet) explicitly considered within LC/LP. Such emerging technologies may thus not be adequately addressed by the existing governance structures, or not addressed at all, unless they are considered in the Annex of the 2013 Amendment to the London Protocol which is yet to be further detailed - and yet to enter into force.
The United Nations’ 2030 Agenda for Sustainable Development (United Nations, 2015) commits the global community to transform current trajectories in multiple topical areas, including climate action (SDG 13) and sustainable use and conservation of the ocean (SDG 14). The 2030 Agenda now is at the mid-point of its implementation and, as the High-Level Political Forum on Sustainable Development (HLPF) stressed when calling for the 2023 SDG Summit, “a new phase of accelerated progress towards the SDGs” appears indispensable to achieve the goals set (ECOSOC, 2023). In the preamble of the ambitious resolution the UN General Assembly adopted in 2015 (United Nations, 2015), the global community is reminded that the 17 sustainable development goals (SDGs) and their individual targets form an indivisible and integrated whole that seeks to balance all dimensions of sustainable development. ONETs offer a promising pathway towards accelerated progress for climate change mitigation, a field in which necessary transformative action on the national level is still greatly lacking. But it must not be forgotten that none of the goals can be achieved in isolation, which accounts for the climate goals SDG 13 as well as for the ocean goal SDG 14 (Griggs et al., 2017). And, furthermore, no goal should be compromised for another one, unless the global community makes a deliberate and well-informed decision in such a direction. Healthy oceans are linked to food security and livelihoods, among other things (Schmidt et al., 2017), and although climate change has negative effects on ocean “health”, actions to combat climate change should not compromise other objectives, including those that concern global ocean governance, such as the protection and preservation of the marine environment. The 2030 Agenda, as aspirational as it is with its overarching goal to leave no one behind, demands consideration of the interests and needs of humankind as a whole and in an equitable manner. Steering ONET governance forward under the principles set by the 2030 Agenda requires a balanced consideration of all of humanity’s goals and decision-making on the basis of synergies rather than trade-offs. An added difficulty is provided as global ocean and climate governance frameworks so far fail to effectively integrate the overlaps between each other’s regimes (Gallo et al., 2017; Spalding and de Ycaza, 2020), even though the interlinkages between the ocean and climate system are fundamental both in tackling climate change as well as ocean degradation. Further, international ocean governance is set up in a patchwork approach and objectives, whether seemingly opposing (e.g., blue growth and biodiversity conservation) or working in the same direction, are sometimes regulated by separate agreements and entities that fail to integrate the other’s provisions to governance (Stephenson et al., 2021). This disconnection is reflected in the indirect governance framework developed for ONETs (see Figure 2) as it is made up of sectoral as well as conservation regulation. Strengthening of policy coherence and integration within the identified ONET governance framework for climate mitigation, ocean preservation and other linked policy goals may pre-emptively alleviate pressure nodes in future decision-making regarding ONET deployment.
In contrast, blue carbon as a nature-based solution, which in the past has been considered as a comparatively low-risk or “low-regret” option for marine CDR (Gattuso et al., 2021), has successfully mainstreamed the ocean’s ability to store additional carbon into global climate policy (e.g., REDD+). The identified governance framework for ONETs is positioned comparatively favourable towards restoration and management of coastal blue carbon ecosystems. Blue carbon is sometimes excluded from the negative emissions technologies discourse altogether and rather discussed as nature-based solution of high feasibility (Howard, 2016), with a wide range of local to global co-benefits linked to implementation (Hilmi et al., 2021). Albeit this universal understanding has recently been challenged (Williamson and Gattuso, 2022), and the current governance framework may not be nuanced enough to appropriately identify potential negative impacts of activities related to blue carbon.
In addition to lacking comprehensiveness, the current direct governance framework surrounding ONETs can be described as largely reactive and lacking foresight. It would be beneficial to determine how best to approach ONET governance in a manner in which trade-offs are minimised and benefits are encouraged as well as distributed fairly across the globe, and in anticipation of future challenges. Emerging technologies such as ONETs require consideration as an additional human use of an already exhausted ocean space (Halpern et al., 2019), for which protective regulation has failed on multiple scales since the adoption of UNCLOS (Zondervan et al., 2013). Furthermore, the challenges surrounding ONETs, and the identified gaps and open questions of current regulation and governance, call for a comprehensive approach to governing the deployment of such technologies in the ocean. Customary international law in this context offers very relevant elements (GESAMP, 2019), including through principles such as such as the precautionary approach and the no harm principle. Equity and fairness are rightly reflected upon critically in contemporary discussions on ocean governance (Bennett, 2018; Bennett, 2022), and with the emerging topic of ONETs lies the opportunity to integrate such knowledge advances. Codes of conduct have been put forward to give guidance on how to best govern CDR, such as the Oxford Principles for geoengineering (Rayner et al., 2013) that include public participation and transparency as key pillars for future governance. A comprehensive approach therefore would not only encompass a wider framework of direct, implicit and indirect governance elements (Figure 2), key principles of ocean governance and codes of conduct for marine geoengineering, but also take up principles of “good” governance such as coherence, equity and inclusiveness (CEC, 2001; Chang, 2010) in order to establish a meaningful and widely acceptable framework for navigating the future deployment of ONETs. The draft agreement on the conservation of marine biodiversity in areas beyond national jurisdiction (United Nations, 2023), negotiated under the United Nations Convention the Law of the Sea, may be critical towards closing gaps in ocean governance of the high seas and further addressing concepts such as benefit sharing and the common heritage for humankind in the ocean realm. Pending final editing, signing and ratification, the new treaty could be supportive of action that helps reduce climate change impacts on marine ecosystems. The exact standards, guidelines, and processes of the treaty, including for environmental impact assessments and related UN processes, including LC/LP, are still to be further detailed.
5 Conclusion
The assessment of interactions of ONETS with the marine environment and subsequent analysis of related international environmental agreements, as presented here, rendered a unique perspective on the direct, implicit and indirect governance framework that is pertinent for ONETs and potential future deployment at global scales. A closer look at current regulation and governance of ocean-based CDR through the lens of ocean governance reveals a number of challenges and open questions that must be addressed before the perceived, advocated or mandated urgency to act and include (marine) CDR in the global climate mitigation strategy, or interests of individual actors and stakeholders, become the driving force. The assessment presented here points to the need for integration and coherence between the frameworks currently addressing ONETs, whether directly, implicitly or indirectly, and to the need for an overall comprehensive governance approach. There are many examples where the technological enhancement of activities that engage the ocean for society’s benefits has in fact led to negative outcomes for the complex ecosystem, such as offshore oil and gas rigs and bottom-trawlers that have disrupted the ocean floor for economic gain (Zacharias, 2014). While strengthening efforts towards limiting global warming is central for the sustainable development of the planet (Fuso Nerini et al., 2019), global governance of ONETs must appropriately consider the wide range of impacts on the ocean’s condition and linked marine and coastal ES. This includes nuanced assessment of widely endorsed blue carbon ecosystems. On the other hand, ONETs as a solution to climate change may potentially equally benefit the marine environment, as it would society in the long term. A case-by-case assessment of ONETs’ impacts on the ocean environment, which would be the practice under current direct regulation through LC/LP, may not be appropriate for good decision-making. A robust assessment of potential accumulative unintended impacts of ONETs on the ocean, and of potential long-term effects, must be streamlined into governance and decision-making processes.
Nonetheless, as with trade-offs related to the absence of climate mitigation measures or mitigation limited to emissions reductions, the risks associated with deployment of ocean-based NETs must be assessed and weighed in accordance with their trade-offs and benefits to humankind, the marine environment, and other goals set out in the 2030 Agenda for Sustainable Development such as food security (SDG 1) and conservation and sustainable use of life below water (SDG 14). Known impacts on ocean condition and related ES have been presented in this study and reveal a range of potential trade-offs that the governance framework surrounding ONETs needs to recognise and consider to potentially decide in favour of global climate ambitions over other policy goals. A robust and foresight-oriented governance framework that aims for integration between the different frameworks in place, or sets up a new, overarching structure that allows for rigorous oversight and integration of a suite of principles aligned with “good” governance seems mandatory. Ocean governance in general, but also existing (direct) ONET regulation, tend to be reactive. The findings of this assessment, though, strongly suggest that decision-making needs to get ahead of the issue instead of lagging behind these emerging technologies in order to ensure best possible outcomes for people and planet. The deep uncertainty that surrounds ONETs, their intended and unintended impacts on the marine environment, and their real-world contribution to combatting climate change, should be cause for precaution - but not for paralysis in policy making, as the urgency of the climate crisis demands decision-making within appropriate time frames. Still, robust and “good” governance approaches should lead the way and future decision-making should not be rushed in favour of quick fixes. Climate action via long-term emission reductions has a tendency to be very slow when economically unappealing.
Global ocean governance presents many complexities and is further challenged by the climate issue. Spalding and de Ycaza, 2020) like many other authors critically emphasise that there is a general lack of comprehensiveness in ocean governance in light of the challenges the ocean, and with it humanity, is facing. New emerging technologies such as the set of ONETs discussed in this paper introduce further challenges and a possibly quickly moving “target” which policy and decision-making, but also public deliberation, must keep up with – and, better yet, get ahead of the debate and technological developments. With regards to comprehensive governance of ONETs, it may be required to identify pathways “through” the prescribed ocean governance framework rather than attempting to eliminate the weaknesses of the system. Research is needed not only into technical feasibility or cost-effectiveness of ONETs, but also into what “good” governance of ONETs could or should look like – including from an ocean and environmental perspective. Therefore, the identified ONET governance framework not only reiterates current challenges in ocean governance, but also represents an opportunity for a synergistic and integrated approach to future governance. Consideration of governance of land-sea interactions of ONETs was beyond the scope of research, as well other aspects such as carbon accounting, but is of course also highly relevant for future research activities towards good governance of ONETs. Concrete elements of comprehensive ONET governance based on the findings presented in this study are subject to follow-up research and can be used as starting point for developing pathways towards future ONET governance on the global to regional level.
Data availability statement
The original contributions presented in the study are included in the article/Supplementary Material. Further inquiries can be directed to the corresponding author.
Ethics statement
Ethical review and approval was not required for the study on human participants in accordance with the local legislation and institutional requirements. The research and activities carried out by the researchers as part of the EU funded OceanNETs project comply with ethical principles and relevant national, EU and international legislation, such as the Charter of Fundamental Rights of the European Union and the European Convention on Human Rights.
Author contributions
LR and BN conceptualised the study; LR performed the impact mapping; LR and BN performed the governance framework analysis; LR wrote the manuscript with contributions from BN. All authors contributed to the article and approved the submitted version.
Funding
The authors acknowledge funding under the EU Horizon 2020 research and innovation programme under grant agreement № 869357 (project OceanNETs). The work of BN was further supported by the German Federal Ministry of Education and Research (BMBF) through its Research for Sustainable Development program (FONA), and the Federal State of Brandenburg.
Acknowledgments
The authors herby thank colleagues from the OceanNETs project consortium for providing knowledge on ocean-based negative emissions technologies. The authors would like to specifically thank Alexander Proelss and Rob Steenkamp for providing their legal expertise. The authors would like to further thank Sebastian Unger and Ben Boteler for their guidance in research development and participation in extensive discussions on ocean governance. And, last but not least, we are grateful to the reviewers of the manuscript for their thorough comments and suggestions for this work.
Conflict of interest
The authors declare that the research was conducted in the absence of any commercial or financial relationships that could be construed as a potential conflict of interest.
Publisher’s note
All claims expressed in this article are solely those of the authors and do not necessarily represent those of their affiliated organizations, or those of the publisher, the editors and the reviewers. Any product that may be evaluated in this article, or claim that may be made by its manufacturer, is not guaranteed or endorsed by the publisher.
Supplementary material
The Supplementary Material for this article can be found online at: https://www.frontiersin.org/articles/10.3389/fmars.2023.995130/full#supplementary-material
Footnotes
- ^ See https://www.oceannets.eu/, last accessed 03 January 2023.
References
Albright R., Caldeira L., Hosfelt J., Kwiatkowski L., Maclaren J. K., Mason B. M., et al. (2016). Reversal of ocean acidification enhances net coral reef calcification. Nature 531 (7594), 362–365. doi: 10.1038/nature17155
Barboza L. G. A., Cózar A., Gimenez B. C. G., Barros T. L., Kershaw P. J., Guilhermino L. (2019). “Chapter 17 - macroplastics pollution in the marine environment,” in World Seas: an Environmental Evaluation (Second Edition). Ed. Sheppard C. (Elsevier Ltd.: Academic Press), 305–328.
Bennett N. J. (2018). Navigating a just and inclusive path towards sustainable oceans. Mar. Policy 97, 139–146. doi: 10.1016/j.marpol.2018.06.001
Bennett N. J. (2022). Mainstreaming equity and justice in the ocean. Front. Mar. Sci. 9. doi: 10.3389/fmars.2022.873572
Biermann F., Davies O., van der Grijp N. (2009). Environmental policy integration and the architecture of global environmental governance. Int. Environ. Agreements: Politics Law Economics 9 (4), 351. doi: 10.1007/s10784-009-9111-0
Bodle R., Oberthür S., Donat L., Homann G., Sina S., Tedsen E. (2014). Options and proposals for the international governance of geoengineering. Umweltbundesamt Dessau-Roßlau. Climate Change | 14/2014. Available at: https://www.umweltbundesamt.de/publikationen/options-proposals-for-the-international-governance.
Boettcher M., Brent K., Buck H. J., Low S., McLaren D., Mengis N. (2021). Navigating potential hype and opportunity in governing marine carbon removal. Front. Climate 3 (47). doi: 10.3389/fclim.2021.664456
Boucher O., Forster P. M., Gruber N., Ha-Duong M., Lawrence M. G., Lenton T. M., et al. (2014). Rethinking climate engineering categorization in the context of climate change mitigation and adaptation. Wiley Interdiscip. Reviews: Climate Change 5 (1), 23–35. doi: 10.1002/wcc.261
Boyd P. W., Jickells T., Law C. S., Blain S., Boyle E. A., Buesseler K. O., et al. (2007). Mesoscale iron enrichment experiments 1993-2005: synthesis and future directions. Sci. (New York N.Y.) 315 (5812), 612–617. doi: 10.1126/science.1131669
Boyes S. J., Elliott M. (2014). Marine legislation–the ultimate 'horrendogram': international law, European directives & national implementation. Mar. pollut. Bull. 86 (1-2), 39–47. doi: 10.1016/j.marpolbul.2014.06.055
Brodie Rudolph T., Ruckelshaus M., Swilling M., Allison E. H., Osterblom H., Gelcich S., et al. (2020). A transition to sustainable ocean governance. Nat. Commun. 11 (1), 3600. doi: 10.1038/s41467-020-17410-2
Buck H. J., Carton W., Lund J. F., Markusson N. (2023). Why residual emissions matter right now. Nat. Climate Change 13 (4), 351–358. doi: 10.1038/s41558-022-01592-2
Cabral P., Levrel H., Viard F., Frangoudes K., Girard S., Scemama P. (2016). Ecosystem services assessment and compensation costs for installing seaweed farms. Mar. Policy 71, 157–165. doi: 10.1016/j.marpol.2016.05.031
Cao L., Caldeira K. (2010). Can ocean iron fertilization mitigate ocean acidification? Climatic Change 99 (1), 303–311. doi: 10.1007/s10584-010-9799-4
Casareto B. E., Niraula M. P., Suzuki Y. (2017). Marine planktonic ecosystem dynamics in an artificial upwelling area of Japan: Phytoplankton production and biomass fate. J. Exp. Mar. Biol. Ecol. 487, 1–10. doi: 10.1016/j.jembe.2016.11.002
Caserini S., Pagano D., Campo F., Abbà A., De Marco S., Righi D., et al. (2021). Potential of maritime transport for ocean liming and atmospheric CO2 removal. Front. Climate 3. doi: 10.3389/fclim.2021.575900
CEC. (2001). “European governance - A white paper,” in Commission of the European Communities COM 428 final 2001. C 287/01(442). Available at: https://eur-lex.europa.eu/legal-content/EN/TXT/?uri=CELEX%3A52001DC0428
Chang Y. C. (2010). International legal obligations in relation to good ocean governance. Chin. J. Int. Law 9 (3), 589–605. doi: 10.1093/chinesejil/jmq024
Constantino S. M., Weber E. U. (2021). Decision-making under the deep uncertainty of climate change: The psychological and political agency of narratives. Curr. Opin. Psychol. 42, 151–159. doi: 10.1016/j.copsyc.2021.11.001
Convention on Biological Diversity. (2022). “Kunming-montreal global biodiversity framework,” in Convention on Biological Diversity (Montreal, Canada: Convention on Biological Diversity).
Convention on the Conservation of Migratory Species of Wild Animals. (1979). “Convention text,” in Convention on the Conservation of Migratory Species of Wild Animal. (Bonn: CMS)
Cullen-Unsworth L., Unsworth R. (2013). Seagrass meadows, ecosystem services, and sustainability. Environment 55 (3), 14–28. doi: 10.1080/00139157.2013.785864
Davis T. A., Volesky B., Mucci A. (2003). A review of the biochemistry of heavy metal biosorption by brown algae. Water Res. 37 (18), 4311–4330. doi: 10.1016/S0043-1354(03)00293-8
de Lannoy C.-F., Eisaman M. D., Jose A., Karnitz S. D., DeVaul R. W., Hannun K., et al. (2018). Indirect ocean capture of atmospheric CO2: Part I. Prototype of a negative emissions technology. Int. J. Greenhouse Gas Control 70, 243–253. doi: 10.1016/j.ijggc.2017.10.007
Dooley K., Harrould-Kolieb E., Talberg A. (2021). Carbon-dioxide removal and biodiversity: A threat identification framework. Global Policy 12 (S1), 34–44. doi: 10.1111/1758-5899.12828
ECOSOC. (2023). The 2023 SDG Summit – the High-level Political Forum on Sustainable Development under the auspices of the General Assembly (New York: United Nations) (Accessed 23.05.2023).
Eisaman M. D., Rivest J. L., Karnitz S. D., de Lannoy C.-F., Jose A., DeVaul R. W., et al. (2018). Indirect ocean capture of atmospheric CO2: Part II. Understanding the cost of negative emissions. Int. J. Greenhouse Gas Control 70, 254–261. doi: 10.1016/j.ijggc.2018.02.020
Eklöf J., Torre-Castro M., Nilsson C., Rönnbäck P. (2013). How do seaweed farms influence local fishery catches in a seagrass-dominated setting in Chwaka Bay, Zanzibar? Aquat. Living Resour. 19, 137–147. doi: 10.1051/alr:2006013
FAO. (2020). “The state of world fisheries and aquaculture,” in The State of World Fisheries and aquaculture (SOFIA) (Rome, Italy: Food and Agriculture Organization of the United Nations).
Feng E. Y., Keller D. P., Koeve W., Oschlies A. (2016). Could artificial ocean alkalinization protect tropical coral ecosystems from ocean acidification? Environ. Res. Lett. 11 (7). doi: 10.1088/1748-9326/11/7/074008
Ferderer A., Chase Z., Kennedy F., Schulz K. G., Bach L. T. (2022). Assessing the influence of ocean alkalinity enhancement on a coastal phytoplankton community. Biogeosciences Discuss. 2022, 1–36. doi: 10.5194/bg-2022-17
Fisher R., O’Leary R. A., Low-Choy S., Mengersen K., Knowlton N., Brainard R. E., et al. (2015). Species richness on coral reefs and the pursuit of convergent global estimates. Curr. Biol. 25 (4), 500–505. doi: 10.1016/j.cub.2014.12.022
Friedlingstein P., Jones M. W., O'Sullivan M., Andrew R. M., Bakker D. C. E., Hauck J., et al. (2022). Global carbon budget 2021. Earth System Sci. Data 14 (4), 1917–2005. doi: 10.5194/essd-14-1917-2022
Friess D. A., Yando E. S., Alemu I J. B., Wong L. W., Soto S. D., Bhatia N. (2020). Ecosystem services and disservices of mangrove forests and salt marshes. Oceanography Mar. Biol. 58, 107–141. doi: 10.1201/9780429351495-3
Fuso Nerini F., Sovacool B., Hughes N., Cozzi L., Cosgrave E., Howells M., et al. (2019). Connecting climate action with other Sustainable Development Goals. Nat. Sustainability 2 (8), 674–680. doi: 10.1038/s41893-019-0334-y
Fuss S., Lamb W. F., Callaghan M. W., Hilaire J., Creutzig F., Amann T., et al. (2018). Negative emissions—Part 2: Costs, potentials and side effects. Environ. Res. Lett. 13 (6), 063002. doi: 10.1088/1748-9326/aabf9f
Gagern A. (2019). Ocean Alkalinity Enhancement: Current state of knowledge and potential role of philanthropy. September 9th, 2019 Meeting Proceedings, Half Moon Bay, California. Available at: https://oursharedseas.com/wp-content/uploads/2020/02/Ocean-Alkalinity-Enhancement.pdf.
Galaz V. (2012). Geo-engineering, governance, and social-ecological systems: critical issues and joint research needs. Ecol. Soc. 17 (1). doi: 10.5751/ES-04677-170124
Galbraith E., Le Mézo P., Hernandez G., Bianchi D., Kroodsma D. (2019). Growth limitation of marine fish by low iron availability in the open ocean. Front. Mar. Sci. 6. doi: 10.3389/fmars.2019.00509
Gallo N. D., Victor D. G., Levin L. A. (2017). Ocean commitments under the Paris Agreement. Nat. Climate Change 7 (11), 833–838. doi: 10.1038/nclimate3422
Gattuso J.-P., Magnan A. K., Bopp L., Cheung W. W. L., Duarte C. M., Hinkel J., et al. (2018). Ocean solutions to address climate change and its effects on marine ecosystems. Front. Mar. Sci. 5 (337). doi: 10.3389/fmars.2018.00337
Gattuso J.-P., Williamson P., Duarte C. M., Magnan A. K. (2021). The potential for ocean-based climate action: negative emissions technologies and beyond. Front. Climate 2 (37). doi: 10.3389/fclim.2020.575716
GESAMP. (2019). “High level review of a wide range of proposed marine geoengineering techniques,” in Rep. Stud. GESAMP. Eds. Boyd P., M.G. C. (London SE1 7SR: IMO/FAO/UNESCO-IOC/UNIDO/WMO/IAEA/UN/UN Environment/ UNDP/ISA Joint Group of Experts on the Scientific Aspects of Marine Environmental Protection).
Giardino A., Nederhoff K., Vousdoukas M. (2018). Coastal hazard risk assessment for small islands: assessing the impact of climate change and disaster reduction measures on Ebeye (Marshall Islands). Regional Environ. Change 18 (8), 2237–2248. doi: 10.1007/s10113-018-1353-3
Global Ocean Observing System. (2021). Essential Ocean Variables. Available at: https://www.goosocean.org/index.php?option=com_content&view=article&id=14&Itemid=114 (Accessed 21 January 2023).
Gore S., Renforth P., Perkins R. (2019). The potential environmental response to increasing oceanalkalinity for negative emissions. Mitigation Adaptation Strategies Global Change 24 (7), 1191–1211. doi: 10.1007/s11027-018-9830-z
Grafeld S., Oleson K. L. L., Teneva L., Kittinger J. N. (2017). Follow that fish: Uncovering the hidden blue economy in coral reef fisheries. PloS One 12 (8), e0182104. doi: 10.1371/journal.pone.0182104
Griggs D. J., Nilsson M., Stevance A. -S., McCollum D. (Eds.) (2017). A Guide to SDG Interactions: from Science to Implementation. (Paris: International Council for Science).
Grip K. (2017). International marine environmental governance: A review. Ambio 46 (4), 413–427. doi: 10.1007/s13280-016-0847-9
Haas B., Mackay M., Novaglio C., Fullbrook L., Murunga M., Sbrocchi C., et al. (2021). The future of ocean governance. Rev. Fish Biol. Fish 32(1), 1–18. doi: 10.1007/s11160-020-09631-x
Haines-Young R., Potschin M. B. (2018). Common International Classification of Ecosystem Services (CICES) V5.1 and Guidance on the Application of the Revised Structure. (Nottingham, UK: Fabis Consulting Ltd.)
Hall-Spencer J. M., Harvey B. P. (2019). Ocean acidification impacts on coastal ecosystem services due to habitat degradation. Emerging topics Life Sci. 3 (2), 197–206. doi: 10.1042/ETLS20180117
Halpern B. S., Frazier M., Afflerbach J., Lowndes J. S., Micheli F., O’Hara C., et al. (2019). Recent pace of change in human impact on the world’s ocean. Sci. Rep. 9 (1), 11609. doi: 10.1038/s41598-019-47201-9
Handå A., McClimans T. A., Reitan K. I., Knutsen Ø., Tangen K., Olsen Y. (2014). Artificial upwelling to stimulate growth of non-toxic algae in a habitat for mussel farming. Aquaculture Res. 45 (11), 1798–1809. doi: 10.1111/are.12127
Hartmann J., West A. J., Renforth P., Köhler P., de la Rocha C. L., Wolf-Gladrow D. A., et al. (2013). Enhanced chemical weathering as a geoengineering strategy to reduce atmospheric carbon dioxide, supply nutrients, and mitigate ocean acidification. Rev. Geophysics 51 (2), 113–149. doi: 10.1002/rog.20004
Harvey L. D. D. (2008). Mitigating the atmospheric CO2 increase and ocean acidification by adding limestone powder to upwelling regions. J. Geophysical Res. 113 (C4). doi: 10.1029/2007JC004373
Hasselström L., Visch W., Gröndahl F., Nylund G., Pavia H. (2018). The impact of seaweed cultivation on ecosystem services - a case study from the west coast of Sweden. Mar. pollut. Bull. 133. doi: 10.1016/j.marpolbul.2018.05.005
Hauck J., Köhler P., Wolf-Gladrow D., Völker C. (2016). Iron fertilisation and century-scale effects of open ocean dissolution of olivine in a simulated CO2 removal experiment. Environ. Res. Lett. 11 (2), 24007. doi: 10.1088/1748-9326/11/2/024007
Hilmi N., Chami R., Sutherland M. D., Hall-Spencer J. M., Lebleu L., Benitez M. B., et al. (2021). The role of blue carbon in climate change mitigation and carbon stock conservation. Front. Climate 3. doi: 10.3389/fclim.2021.710546
Hochard J. P., Hamilton S., Barbier E. B. (2019). Mangroves shelter coastal economic activity from cyclones. Proc. Natl. Acad. Sci. U.S.A. 116 (25), 12232–12237. doi: 10.1073/pnas.1820067116
Howard J. (2016) Supporting Coastal Conservation and Community Development in Kaimana, Indonesia by valuing Blue Carbon. Available at: https://birdsheadseascape.com/conservation-science/supporting-coastal-conservation-and-community-development-in-kaimana-indonesia-by-valuing-blue-carbon-by-jennifer-howard/ (Accessed 25.08.2021).
Howard J. L., Creed J. C., Aguiar M. V. P., Fourqurean J. W. (2018). CO2 released by carbonate sediment production in some coastal areas may offset the benefits of seagrass “Blue Carbon” storage. Limnology Oceanography 63 (1), 160–172. doi: 10.1002/lno.10621
Ilyina T., Wolf-Gladrow D., Munhoven G., Heinze C. (2013). Assessing the potential of calcium-based artificial ocean alkalinization to mitigate rising atmospheric CO2 and ocean acidification. Geophysical Res. Lett. 40 (22), 5909–5914. doi: 10.1002/2013GL057981
IMO. (1972). Convention on the Prevention of Marine Pollution by Dumping of Wastes and Other Matter 1972 (London: I.M. Organization).
IMO. (2008). Resolution LC-LP.1 (2008) on the Regulation of Ocean Fertilization (I.M. Organization).
IMO. (2013). Resolution LP.4(8) on the Amendment to the London Protocol to Regulate the Placement of Matter for Ocean Fertilization and other Marine Geoengineering Activities (not in force) (I.M. Organization).
Intergovernmental Panel on Climate Change. (2022). “Climate change 2022: mitigation of climate change. Summary for policy makers,” in Working Group III contribution to the Sixth Assessment Report of the Intergovernmental Panel on Climate Change (Geneva, Switzerland: Intergovernmental Panel on Climate Change), 36 pages.
IPCC. (2014). 2013 Supplement to the 2006 IPCC Guidelines for National Greenhouse Gas Inventories: Wetlands. Eds. Hiraishi T., Krug T., Tanabe K., Srivastava N., Baasansuren J., Fukuda M., et al (Switzerland: IPCC).
IPCC. (2018). Global Warming of 1.5°C. An IPCC Special Report on the impacts of global warming of 1.5°C above pre-industrial levels and related global greenhouse gas emission pathways, in the context of strengthening the global response to the threat of climate change, sustainable development, and efforts to eradicate poverty [Masson-Delmotte V.Zhai P.Pörtner H.-O.Roberts D.Skea J.Shukla P. R. (eds.)]. (Cambridge, UK and New York, NY, USA: Cambridge University Press). doi: 10.1017/9781009157940.001
IPCC. (2022). “Climate change 2022: mitigation of climate change contribution,” in Contribution of Working Group III to the Sixth Assessment Report of the Intergovernmental Panel on Climate Change. (Geneva, Switzerland: IPCC)
Jin P., Zhang J., Wan J., Overmans S., Gao G., Ye M., et al. (2021). The combined effects of ocean acidification and heavy metals on marine organisms: A meta-analysis. Front. Mar. Sci. 8. doi: 10.3389/fmars.2021.801889
Keller R. P., Drake J. M., Drew M. B., Lodge D. M. (2011). Linking environmental conditions and ship movements to estimate invasive species transport across the global shipping network. Diversity Distributions 17 (1), 93–102. doi: 10.1111/j.1472-4642.2010.00696.x
Kim J., Kraemer G., Yarish C. (2015). Use of sugar kelp aquaculture in Long Island Sound and the Bronx River Estuary for nutrient extraction. Mar. Ecol. Prog. Ser. 531, 155–166. doi: 10.3354/meps11331
King A., Karoly D., Henley B. (2017). Australian climate extremes at 1.5 °C and 2 °C of global warming. Nat. Climate Change 7. doi: 10.1038/nclimate3296
Koch M., Bowes G., Ross C., Zhang X. H. (2013). Climate change and ocean acidification effects on seagrasses and marine macroalgae. Global Change Biol. 19 (1), 103–132. doi: 10.1111/j.1365-2486.2012.02791.x
Köhler P., Abrams J. F., Völker C., Hauck J., Wolf-Gladrow D. A. (2013). Geoengineering impact of open ocean dissolution of olivine on atmospheric CO2, surface ocean pH and marine biology. Environ. Res. Lett. 8 (1). doi: 10.1088/1748-9326/8/1/014009
Krauss K. W., Allen J. A., Cahoon D. R. (2003). Differential rates of vertical accretion and elevation change among aerial root types in Micronesian mangrove forests. Estuarine Coast. Shelf Sci. 56 (2), 251–259. doi: 10.1016/S0272-7714(02)00184-1
Kvenvolden K. A. (1993). Gas hydrates—geological perspective and global change. Rev. Geophysics 31 (2), 173–187. doi: 10.1029/93RG00268
Laffoley D., Baxter J. M. (2019). “Ocean deoxygenation : everyone’s problem : causes, impacts, consequences and solutions,” in Full report (Gland, Switzerland: IUCN), 580 pp. doi: 10.2305/IUCN.CH.2019.14.en
Lampitt R. S., Achterberg E. P., Anderson T. R., Hughes J. A., Iglesias-Rodriguez M. D., Kelly-Gerreyn B. A., et al. (2008). Ocean fertilization: a potential means of geoengineering? Philos. Trans. R. Soc. A: Mathematical Phys. Eng. Sci. 366 (1882), 3919–3945. doi: 10.1098/rsta.2008.0139
Lattemann S., Höpner T. (2008). Environmental impact and impact assessment of seawater desalination. Desalination 220 (1), 1–15. doi: 10.1016/j.desal.2007.03.009
Leedham E. C., Hughes C., Keng F. S. L., Phang S. M., Malin G., Sturges W. T. (2013). Emission of atmospherically significant halocarbons by naturally occurring and farmed tropical macroalgae. Biogeosciences 10 (6), 3615–3633. doi: 10.5194/bg-10-3615-2013
Levin L. A., Alfaro-Lucas J. M., Colaço A., Cordes E. E., Craik N., Danovaro R., et al. (2023). Deep-sea impacts of climate interventions. Science 379 (6636), 978–981. doi: 10.1126/science.ade7521
Lindstrom E., Gunn J., Fischer A., McCurdy A., Glover L. K. (2012). A Framework for ocean observing (Paris, France: I.O. Commission).
Liu S., Zhao L., Xiao C., Fan W., Cai Y., Pan Y., et al. (2020). Review of artificial downwelling for mitigating hypoxia in coastal waters. Water 12 (10), 2846. doi: 10.3390/w12102846
Lund J. F., Markusson N., Carton W., Buck H. J. (2023). Net zero and the unexplored politics of residual emissions. Energy Res. Soc. Sci. 98, 103035. doi: 10.1016/j.erss.2023.103035
Magnan A. K., Colombier M., Bille R., Joos F., Hoegh-Guldberg O., Portner H. O., et al. (2016). Implications of the paris agreement for the ocean. Nat. Climate Change 6 (8), 732–735. doi: 10.1038/nclimate3038
Mahon R., Fanning L., Gjerde K. M., Young O., Reid M., Douglas S. (2015). “Transboundary waters assessment Programme (TWAP) assessment of governance arrangements for the ocean, Volume 2: Areas beyond National Jurisdiction,” in IOC Technical Series (Paris: UNESCO-IOC).
Manganelli M., Scardala S., Stefanelli M., Palazzo F., Funari E., Vichi S., et al. (2012). Emerging health issues of cyanobacterial blooms. Ann. Ist Super Sanita 48 (4), 415–428. doi: 10.4415/ann_12_04_09
Marques B., Lillebø A. I., Pereira E., Duarte A. C. (2011). Mercury cycling and sequestration in salt marshes sediments: An ecosystem service provided by Juncus maritimus and Scirpus maritimus. Environ. pollut. 159 (7), 1869–1876. doi: 10.1016/j.envpol.2011.03.036
McGee J., Brent K., Burns W. (2018). Geoengineering the oceans: an emerging frontier in international climate change governance. Aust. J. Maritime Ocean Affairs 10 (1), 67–80. doi: 10.1080/18366503.2017.1400899
McGinnis M. D. (2011). An introduction to IAD and the language of the ostrom workshop: A simple guide to a complex framework. Policy Stud. J. 39 (1), 169–183. doi: 10.1111/j.1541-0072.2010.00401.x
McGinnis M., Ostrom E. (2014). Social-ecological system framework: initial changes and continuing challenges. Ecol. Soc. 19. doi: 10.5751/ES-06387-190230
McWhorter J. K., Halloran P. R., Roff G., Skirving W. J., Perry C. T., Mumby P. J. (2022). The importance of 1.5°C warming for the Great Barrier Reef. Glob Chang Biol. 28 (4), 1332–1341. doi: 10.1111/gcb.15994
Metzger R. A., Benford G. (2001). Sequestering of atmospheric carbon through permanent disposal of crop residue. Climatic Change 49 (1), 11–19. doi: 10.1023/A:1010765013104
Metzger R. A., Benford G., Hoffert M. I. (2002). To bury or to burn: optimum use of crop residues to reduce atmospheric CO2. Climatic Change 54 (3), 369–374. doi: 10.1023/A:1016136202309
Miloslavich P., Bax N. J., Simmons S. E., Klein E., Appeltans W., Aburto-Oropeza O., et al. (2018). Essential ocean variables for global sustained observations of biodiversity and ecosystem changes. Global Change Biol. 24 (6), 2416–2433. doi: 10.1111/gcb.14108
Minx J. C., Lamb W. F., Callaghan M. W., Fuss S., Hilaire J., Creutzig F., et al. (2018). Negative emissions—Part 1: Research landscape and synthesis. Environ. Res. Lett. 13 (6), 063001. doi: 10.1088/1748-9326/aabf9b
Mitchell R. B. (2002-2022). International Environmental Agreements Database Project (Version 2020.1). Available at: http://iea.uoregon.edu/ (Accessed 14.07.2022).
Mitra A. (2020). “Mangrove forests in india,” in Exploring Ecosystem Services (Switzerland: Springer Cham), 1–32.
Moltmann T., Turton J., Zhang H.-M., Nolan G., Gouldman C., Griesbauer L., et al. (2019). A global ocean observing system (GOOS), delivered through enhanced collaboration across regions, communities, and new technologies. Front. Mar. Sci. 6. doi: 10.3389/fmars.2019.00291
Moon K., Blackman D., Brewer T. D., Sarre S. D. (2017). Environmental governance for urgent and uncertain problems. Biol. Invasions 19 (3), 785–797. doi: 10.1007/s10530-016-1351-7
Moore S. K., Cline M. R., Blair K., Klinger T., Varney A., Norman K. (2019). An index of fisheries closures due to harmful algal blooms and a framework for identifying vulnerable fishing communities on the U.S. West Coast. Mar. Policy 110, 103543. doi: 10.1016/j.marpol.2019.103543
Moore C. M., Mills M. M., Arrigo K. R., Berman-Frank I., Bopp L., Boyd P. W., et al. (2013). Processes and patterns of oceanic nutrient limitation. Nat. Geosci. 6 (9), 701–710. doi: 10.1038/ngeo1765
Mork M. (1996). “Wave attenuation due to bottom vegetation,” in Waves and Nonlinear Processes in Hydrodynamics. Eds. Grue J., Weber B.G. &J. E. (Dordrecht: Springer Netherlands), 371–382.
Motuhi S.-E., Mehiri M., Payri C. E., La Barre S., Bach S. (2016). Marine natural products from new caledonia—A review. Mar. Drugs 14 (3), 58. doi: 10.3390/md14030058
Naselli-Flores L., Padisák J. (2023). Ecosystem services provided by marine and freshwater phytoplankton. Hydrobiologia 850, 2691–2706. doi: 10.1007/s10750-022-04795-y
National Academies of Sciences, Engineering, and Medicine. (2022). A Research Strategy for Ocean-based Carbon Dioxide Removal and Sequestration (Washington, DC: The National Academies Press).
Nellemann C., Corcoran E., Duarte C. M., Valdés L., De Young C., Fonseca L., et al. (2009). Blue Carbon. A Rapid Response Assessment (Birkeland Trykkeri AS, Norway: U.N.E.P. GRID-Arendal).
Nemet G. F., Callaghan M. W., Creutzig F., Fuss S., Hartmann J., Hilaire J., et al. (2018). Negative emissions—Part 3: Innovation and upscaling. Environ. Res. Lett. 13 (6), 063003. doi: 10.1088/1748-9326/aabff4
Ocean Visions. (2021). Electrochemical CDR - State of Technology. Available at: https://www2.oceanvisions.org/roadmaps/electrochemical-cdr/state-of-technology/ (Accessed 30.06.2022).
Orlove B., Shwom R., Markowitz E., Cheong S.-M. (2020). Climate decision-making. Annu. Rev. Environ. Resour. 45 (1), 271–303. doi: 10.1146/annurev-environ-012320-085130
Ortiz J., Arístegui J., Taucher J., Riebesell U. (2022). Artificial upwelling in singular and recurring mode: consequences for net community production and metabolic balance. Front. Mar. Sci. 8. doi: 10.3389/fmars.2021.743105
Oschlies A., Koeve W., Rickels W., Rehdanz K. (2010a). Side effects and accounting aspects of hypothetical large-scale Southern Ocean iron fertilization. Biogeosciences 7 (12), 4017–4035. doi: 10.5194/bg-7-4017-2010
Oschlies A., Pahlow M., Yool A., Matear R. J. (2010b). Climate engineering by artificial ocean upwelling: Channelling the sorcerer's apprentice. Geophysical Res. Lett. 37 (4). doi: 10.1029/2009GL041961
Ostrom E. (1999). “Institutional rational choice: an assessment of the institutional analysis and development framework,” in Theories of the policy process. Ed. Sabatier P. A. (Boulder, CO: Westview Press), 35–71.
Ostrom E. (2011). Background on the institutional analysis and development framework. Policy Stud. J. 39 (1), 7–27. doi: 10.1111/j.1541-0072.2010.00394.x
Pan Y., Fan W., Zhang D., Chen J., Huang H., Liu S., et al. (2016). Research progress in artificial upwelling and its potential environmental effects. Sci. China Earth Sci. 59 (2), 236–248. doi: 10.1007/s11430-015-5195-2
Pattberg P., Widerberg O. (2015). “Global environmental governance,” in Encyclopedia of Global Environmental Governance and Politics. Eds. Pattberg P., Zelli F. (Cheltenham, UK: Edward Elgar Publishing), 28–35.
Payne M. R., Barange M., Cheung W. W. L., MacKenzie B. R., Batchelder H. P., Cormon X., et al. (2016). Uncertainties in projecting climate-change impacts in marine ecosystems. ICES J. Mar. Sci. 73 (5), 1272–1282. doi: 10.1093/icesjms/fsv231
Phang S.-M., Keng F., Kaur P., Lim Y. K., Abd. Rahman N., Leedham E., et al. (2015). Can seaweed farming in the tropics contribute to climate change through emission of short-lived halocarbons? Malaysian J. Sci. 34, 8–19. doi: 10.22452/mjs.vol34no1.2
Polasky S., Crépin A.-S., Biggs R., Carpenter S. R., Folke C., Peterson G., et al. (2020). Corridors of clarity: four principles to overcome uncertainty paralysis in the anthropocene. BioScience 70 (12), 1139–1144. doi: 10.1093/biosci/biaa115
Queiroz L. D. S., Rossi S., Calvet-Mir L., Ruiz-Mallén I., García-Betorz S., Salvà-Prat J., et al. (2017). Neglected ecosystem services: Highlighting the socio-cultural perception of mangroves in decision-making processes. Ecosystem Serv. 26, 137–145. doi: 10.1016/j.ecoser.2017.06.013
Ramsar Convention on Wetlands. (2018). 13th Meeting of the Conference of the Contracting Parties to the Ramsar Convention on Wetlands “Wetlands for a Sustainable Urban Future” Dubai, United Arab Emirates, 21-29 October 2018. Available at: https://www.ramsar.org/sites/default/files/documents/library/xiii.14_blue_carbon_e.pdf.
Rayner S., Heyward C., Kruger T., Pidgeon N., Redgwell C., Savulescu J. (2013). The Oxford Principles. Climatic Change 121 (3), 499–512. doi: 10.1007/s10584-012-0675-2
Redgwell C. (2012). UNCLOS and climate change. Proc. ASIL Annu. Meeting 106, 406–409. doi: 10.5305/procannmeetasil.106.0406
Renforth P., Henderson G. (2017). Assessing ocean alkalinity for carbon sequestration. Rev. Geophysics 55 (3), 636–674. doi: 10.1002/2016rg000533
Rogelj J., Shindell D., Jiang K., Fifita S., Forster P., Ginzburg V., et al. (2018). “Mitigation pathways compatible with 1.5°C in the context of sustainable development,” in In: Global Warming of 1.5°C. An IPCC Special Report on the impacts of global warming of 1.5°C above pre-industrial levels and related global greenhouse gas emission pathways, in the context of strengthening the global response to the threat of climate change, sustainable development, and efforts to eradicate poverty. Eds. Masson-Delmotte V., Zhai P., Pörtner H.-O., Roberts D., Skea J., Shukla P. R., et al. (Cambridge, UK and New York, NY, USA: Cambridge University Press).
Rosentreter J. A., Al-Haj A. N., Fulweiler R. W., Williamson P. (2021). Methane and nitrous oxide emissions complicate coastal blue carbon assessments. Global Biogeochemical Cycles 35 (2), e2020GB006858. doi: 10.1029/2020GB006858
Satterthwaite E. V., Bax N. J., Miloslavich P., Ratnarajah L., Canonico G., Dunn D., et al. (2021). Establishing the foundation for the global observing system for marine life. Front. Mar. Sci. 8 (1508). doi: 10.3389/fmars.2021.737416
Sawall Y., Harris M., Lebrato M., Wall M., Feng E. Y. (2020). Discrete pulses of cooler deep water can decelerate coral bleaching during thermal stress: implications for artificial upwelling during heat stress events. Front. Mar. Sci. 7. doi: 10.3389/fmars.2020.00720
Schmidt S., Neumann B., Waweru Y., Durussel C., Unger S., Visbeck M. (2017). “SDG14 Conserve and sustainably use the oceans, seas and marine resources for sustainable development,” in A Guide to SDG Interactions: from Science to Implementation. Eds. Griggs D. J., Nilsson M., McCollum A.-S. S. &D. (Paris: International Council for Science), 174–218.
Schneider W., Donoso D., Garcés-Vargas J., Escribano R. (2017). Water-column cooling and sea surface salinity increase in the upwelling region off central-south Chile driven by a poleward displacement of the South Pacific High. Prog. Oceanography 151, 38–48. doi: 10.1016/j.pocean.2016.11.004
Secretariat of the Convention on Biological Diversity. (2009). Scientific Synthesis of the Impacts of Ocean Fertilization on Marine Biodiversity. Montreal, Technical Series No. 45, 53 pages
Sharifian R., Wagterveld R. M., Digdaya I. A., Xiang C., Vermaas D. A. (2021). Electrochemical carbon dioxide capture to close the carbon cycle. Energy Environ. Sci. 14 (2), 781–814. doi: 10.1039/D0EE03382K
Shepard C. C., Crain C. M., Beck M. W. (2011). The protective role of coastal marshes: a systematic review and meta-analysis. PloS One 6 (11), e27374. doi: 10.1371/journal.pone.0027374
Shepherd J. G. (2009). Geoengineering the Climate: Science, Governance and Uncertainty. Ed. Society T. R. (London: The Royal Society).
Silver M. W., Bargu S., Coale S. L., Benitez-Nelson C. R., Garcia A. C., Roberts K. J., et al. (2010). Toxic diatoms and domoic acid in natural and iron enriched waters of the oceanic Pacific. Proc. Natl. Acad. Sci. United States America 107 (48), 20762–20767. doi: 10.1073/pnas.1006968107
Singh P., Jaeckel A. (2018). Future Prospects of Marine Environmental Governance. In: Salomon M., Markus T. (eds) Handbook on Marine Environment Protection. (Cham: Springer). doi: 10.1007/978-3-319-60156-4_32
Singh P. A., Ort M. (2020). “Law and policy dimensions of ocean governance,” in YOUMARES 9 - The Oceans: Our Research, Our Future. Eds. Liebich J. S. ,. V., Bode-Dalby M. (Cham: Springer).
Sousa A. I., Lillebø A. I., Pardal M. A., Caçador I. (2010). Productivity and nutrient cycling in salt marshes: Contribution to ecosystem health. Estuarine Coast. Shelf Sci. 87 (4), 640–646. doi: 10.1016/j.ecss.2010.03.007
Spalding M., Burke L., Wood S. A., Ashpole J., Hutchison J., zu Ermgassen P. (2017). Mapping the global value and distribution of coral reef tourism. Mar. Policy 82, 104–113. doi: 10.1016/j.marpol.2017.05.014
Spalding A. K., de Ycaza R. (2020). Navigating shifting regimes of ocean governance: from UNCLOS to sustainable development goal 14. Environ. Soc. 11 (1), 5–26. doi: 10.3167/ares.2020.110102
Stephenson R. L., Hobday A. J., Allison E. H., Armitage D., Brooks K., Bundy A., et al. (2021). The quilt of sustainable ocean governance: patterns for practitioners. Front. Mar. Sci. 8 (120). doi: 10.3389/fmars.2021.630547
Stigebrandt A., Liljebladh B., de Brabandere L., Forth M., Granmo Å., Hall P., et al. (2015). An experiment with forced oxygenation of the deepwater of the anoxic by fjord, western sweden. AMBIO 44 (1), 42–54. doi: 10.1007/s13280-014-0524-9
Strand S. E., Benford G. (2009). Ocean sequestration of crop residue carbon: recycling fossil fuel carbon back to deep sediments. Environ. Sci. Technol. 43 (4), 1000–1007. doi: 10.1021/es8015556
Tanhua T., McCurdy A., Fischer A., Appeltans W., Bax N., Currie K., et al. (2019). What we have learned from the framework for ocean observing: evolution of the global ocean observing system. Front. Mar. Sci. 6. doi: 10.3389/fmars.2019.00471
Taylor L. L., Quirk J., Thorley R. M. S., Kharecha P. A., Hansen J., Ridgwell A., et al. (2016). Enhanced weathering strategies for stabilizing climate and averting ocean acidification. Nat. Climate Change 6 (4), 402–406. doi: 10.1038/nclimate2882
Thurber A. R., Sweetman A. K., Narayanaswamy B. E., Jones D. O. B., Ingels J., Hansman R. L. (2014). Ecosystem function and services provided by the deep sea. Biogeosciences 11 (14), 3941–3963. doi: 10.5194/bg-11-3941-2014
Uddin M. S., de Ruyter van Steveninck E., Stuip M., Shah M. A. R. (2013). Economic valuation of provisioning and cultural services of a protected mangrove ecosystem: A case study on Sundarbans Reserve Forest, Bangladesh. Ecosystem Serv. 5, 88–93. doi: 10.1016/j.ecoser.2013.07.002
UN. (1995). Agreement for the implementation of the provisions of the united nations convention on the law of the sea of 10 december 1982 relating to the conservation and management of straddling fish stocks and highly migratory fish stocks (New York; USA: U. Nations).
UNEP. (2010a). Decision X/33 Biodiversity and Climate Change - paragraph 8 item (n) (Japan: Nagoya, Aichi Prefecture).
UNEP. (2010b). Decision X/33 Biodiversity and Climate Change - paragraph 8 item (w) (Japan: Nagoya, Aichi Prefecture).
UNESCO. (1972). Convention Concerning the Protection of the World Cultural and Natural Heritage (Paris, France: S.a.C.O. United Nations Educational).
UNESCO. (2020). UNESCO Marine World Heritage: Custodians of the globe’s blue carbon assets (Paris, France).
UNFCCC. (2015). “Adoption of the paris agreement,” in FCCC/CP/2015/L.9/Rev.1 (Paris, France: Framework Convention on Climate Change).
UNEP. (2016). Decision Adopted by the Conference of the Parties to the Convention on Biological Diversity, XIII/14. Climate-related geoengineering, Cancun, Mexico, 4-17 December 2016. Available at: https://www.cbd.int/doc/decisions/cop-13/cop-13-dec-14-en.pdf.
UNFCCC. (2022). Ocean and Climate Change Dialogue 2022. Available at: https://unfccc.int/event/ocean-and-climate-change-dialogue-2022 (Accessed 14.07.2022).
United Nations. (1982). “United nations convention on the law of the sea,” in A/CONF.62/122, reprinted in 21 I.L.M. 1261. (Montego Bay, Jamaica: United Nations).
United Nations. (2015). “Transforming our world: the 2030 Agenda for Sustainable Development. UNGA Resolution A/RES/70/1,” in Resolution adopted by the General Assembly on 25 September 2015 70/1 (New York: United Nations General Assembly).
United Nations. (2023). Draft agreement under the United Nations Convention on the Law of the Sea on the conservation and sustainable use of marine biological diversity of areas beyond national jurisdiction (New York, U.S: U.N.G. Assembly).
Unsworth R. K. F., Collier C. J., Henderson G. M., McKenzie L. J. (2012). Tropical seagrass meadows modify seawater carbon chemistry: Implications for coral reefs impacted by ocean acidification. Environ. Res. Lett. 7 (2), 1–9. doi: 10.1088/1748-9326/7/2/024026
Watson-Wright W., Luis Valdés J. (2019). “Fragmented governance of our one global ocean,” in The Future of Ocean Governance and Capacity Development I.O.I.-. Canada(Leiden, The Netherlands:Brill | Nijhoff), 16–22.
Williamson P., Gattuso J.-P. (2022). Carbon removal using coastal blue carbon ecosystems is uncertain and unreliable, with questionable climatic cost-effectiveness. Front. Climate 4. doi: 10.3389/fclim.2022.853666
Williamson P., Wallace D. W. R., Law C. S., Boyd P. W., Collos Y., Croot P., et al. (2012). Ocean fertilization for geoengineering: A review of effectiveness, environmental impacts and emerging governance. Process Saf. Environ. Prot. 90 (6), 475–488. doi: 10.1016/j.psep.2012.10.007
Woodhead A. J., Hicks C. C., Norström A. V., Williams G. J., Graham N. A. J. (2019). Coral reef ecosystem services in the Anthropocene. Funct. Ecol. 33 (6), 1023–1034. doi: 10.1111/1365-2435.13331
Wright G., Rochette J., Greiber T. (2016). “Sustainable development of the oceans: closing the gaps in the international legal framework,” in Legal Aspects of Sustainable Development (Cham: Springer), 549–564.
Wylie L., Sutton-Grier A. E., Moore A. (2016). Keys to successful blue carbon projects: Lessons learned from global case studies. Mar. Policy 65, 76–84. doi: 10.1016/j.marpol.2015.12.020
Yool A., Shepherd J. G., Bryden H. L., Oschlies A. (2009). Low efficiency of nutrient translocation for enhancing oceanic uptake of carbon dioxide. J. Geophysical Research: Oceans 114. doi: 10.1029/2008JC004792
Yoon J. E., Yoo K. C., Macdonald A. M., Yoon H. I., Park K. T., Yang E. J., et al. (2018). Reviews and syntheses: Ocean iron fertilization experiments – past, present, and future looking to a future Korean Iron Fertilization Experiment in the Southern Ocean (KIFES) project. Biogeosciences 15 (19), 5847–5889. doi: 10.5194/bg-15-5847-2018
Young O. R., Osherenko G., Ekstrom J., Crowder L. B., Ogden J., Wilson J. A., et al. (2007). Solving the crisis in ocean governance: place-based management of marine ecosystems. Environment: Sci. Policy Sustain. Dev. 49 (4), 20–32. doi: 10.3200/envt.49.4.20-33
Zacharias M. (2014). Marine policy: an introduction to governance and international law of the oceans (London: Routledge).
Zhang C., Zhang Y., Luo M., Tan J., Chen X., Tan F., et al. (2022). Massive methane emission from tree stems and pneumatophores in a subtropical mangrove wetland. Plant Soil 473 (1), 489–505. doi: 10.1007/s11104-022-05300-z
Zhou S., Flynn P. C. (2005). Geoengineering downwelling ocean currents: A cost assessment. Climatic Change 71 (1), 203–220. doi: 10.1007/s10584-005-5933-0
Keywords: negative emissions technologies, carbon dioxide removal, ocean governance, ocean alkalinisation, ocean fertilisation, blue carbon
Citation: Röschel L and Neumann B (2023) Ocean-based negative emissions technologies: a governance framework review. Front. Mar. Sci. 10:995130. doi: 10.3389/fmars.2023.995130
Received: 15 July 2022; Accepted: 11 July 2023;
Published: 16 August 2023.
Edited by:
Catarina Frazão Santos, University of Lisbon, PortugalReviewed by:
Miranda Boettcher, German Institute for International and Security Affairs (SWP), GermanyChris Vivian, Centre for Environment, Fisheries and Aquaculture Science (CEFAS), United Kingdom
Michael Kenneth Orbach, Duke University, United States
Copyright © 2023 Röschel and Neumann. This is an open-access article distributed under the terms of the Creative Commons Attribution License (CC BY). The use, distribution or reproduction in other forums is permitted, provided the original author(s) and the copyright owner(s) are credited and that the original publication in this journal is cited, in accordance with accepted academic practice. No use, distribution or reproduction is permitted which does not comply with these terms.
*Correspondence: Lina Röschel, bGluYS5yb2VzY2hlbEByaWZzLXBvdHNkYW0uZGU=