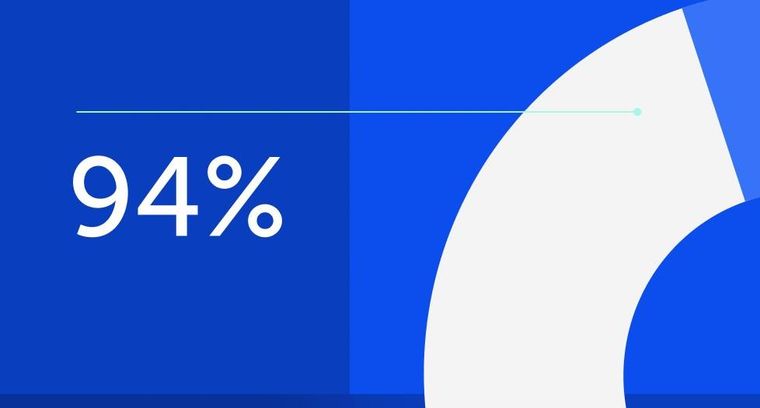
94% of researchers rate our articles as excellent or good
Learn more about the work of our research integrity team to safeguard the quality of each article we publish.
Find out more
EDITORIAL article
Front. Mar. Sci., 04 January 2024
Sec. Physical Oceanography
Volume 10 - 2023 | https://doi.org/10.3389/fmars.2023.1361265
This article is part of the Research TopicMulti-Scale Fluid Physics in Oceanic Flows: New Insights from Laboratory Experiments and Numerical SimulationsView all 16 articles
Editorial on the Research Topic
Multi-scale fluid physics in oceanic flows: new insights from laboratory experiments and numerical simulations
Oceanic flows are characterized by various physical processes that occur over a wide range of spatial-temporal scales, such as eddies, tides, waves, convections, plumes, and turbulent mixing. These flows have great impacts on the oceanic environment (physical, chemical, biological, and ecological) and the global climate, so a comprehensive understanding of their dynamical mechanisms is crucial. However, because of the often-harsh oceanic environment and associated technical difficulties, revealing the multi-scale, multi-physical dynamics of oceanic flows via field measurements remains a considerable challenge. In this context, laboratory experiments and numerical simulations, which can be conducted under precisely-controlled conditions with a relatively low cost, become useful tools for tackling this problem.
This Research Topic collects a total of fifteen articles that provide valuable insights into the multi-scale dynamics of oceanic flows, using laboratory experiments and numerical simulations. While the oceanic flows investigated in these articles involve a diverse range of physical processes, they can be broadly classified into two groups: (1) interaction between oceanic flows and topography and (2) air-sea interaction.
For the first group of studies, one well recognized example is the interaction between internal tides and topography, which is believed to play an important role in maintaining the meridional overturning circulation through contributing a large amount of diapycnal mixing. Thanks to the rapid development in computational power and techniques, this process can be numerically simulated in more detail than before. For example, compared to early studies that mostly used idealized topography and considered all the tidal constituents in the numerical models, modern high-resolution simulations allow researchers to adopt realistic topography in the models and study the influence of different tidal constituents separately. These issues are exactly the focuses of Tang et al. and Wang et al. Their studies provide a more detailed energy budget of internal tides and the effects of larger-scale currents on internal tides during the generation and propagation processes, which is important in developing ocean models with high accuracy.
Compared to the extensive studies of the interaction between internal tides and topography, how the bottom topography affects the meridional overturning in deep oceans has received less attention. By conducting novel laboratory experiments of rotating horizontal convection, Zhang et al. observed that even without spatial variation of mixing intensity, the existence of a meridional ridge can strongly alter both the pattern and the strength of the overturning circulation. This finding not only provides a fresh perspective to explore the overturning circulation via field measurements and modeling studies, but also raises a new question on the influence of bottom topography on the overturning circulation.
While the answer to the question above is subject to future studies, the effect of a sloping bottom on buoyant plumes is becoming clear. Through laboratory experiments on a rotating table, Yuan et al. revealed that bottom-attached river plumes trapped near the coast inhibit offshore freshwater transport on one hand, and promote coastal current transport on the other. The interaction between buoyant plumes and topography is also crucial in the deep sea. By investigating the dispersion of hydrothermal plumes in a complex flow field arising from the interaction with a pronounced seafloor, Xu and German showed that tidal forcing has a notable influence on the plume dispersion by inducing an anti-cyclonic circulation near the region with large topographic features. These findings are useful for modeling bio-geo-chemical processes in oceans.
The significant role of topography on oceanic flows can be found in more situations. Examples collected in this Research Topic include the influence of multiple islands on western boundary currents (Li et al.), the sub-meso-scale motions in coastal regions with shallow water (Si et al.), and the effect of large-scale suspended mussel farms on the tidally induced water circulation (Zhong et al.). These studies not only provide a more comprehensive understanding of the multi-scale dynamics of oceanic flows, but also reflect the current trend in this research field: moving towards more complex physical processes occurring at smaller scales.
The second major topic in this Research Topic is air-sea interaction. One important issue is the role of tropical cyclones, one of the key drivers of upper ocean dynamics. In contrast to previous studies that are mostly based on the statistics of multiple tropical cyclones, Chu et al. focused on the influence of an individual event on the ocean dynamics. Somewhat surprisingly, they found that even a low-intensity cyclone can pronouncedly alter the thermohaline structure by amplifying Kelvin waves in the equatorial regions. It is even more surprising to realize that, according to the large-eddy simulations by Falor et al., surface evaporation can result in a significant diurnal variation in the thermohaline structure by enhancing the irreversible mixing throughout the day. These findings can lead to a better prediction of the key variables in upper oceans and help to improve the parameterizations in ocean modeling.
Improving model parameterizations not only requires a better understanding of the dynamics driving air-sea interaction, but also calls for new methods to analyze the involved processes quantitatively. For example, it is notoriously difficult to quantify the relative contribution of wind forcing and surface buoyancy flux to the upper ocean dynamics. By proposing a new scaling method, Cen et al. found that the turbulent kinetic energy dissipation rate at the mixing layer base exhibits a universal similarity, in spite of variable dynamic states. The new scaling parameter, given by a nonlinear combination of wind-shear-driven dissipation rate and surface buoyancy flux, could potentially simplify model parameterizations. Another long-standing issue in the study of air-sea interaction is how to establish the wind-wave relation, which is often contaminated by the existence of swells. In the work by Gao et al., a probability-based approach was proposed to extract the swell information without knowing the wave spectrum a priori. Compared with previous empirical relations in the literature, this method is more practical in distinguishing swells from wind waves.
Besides new methods for data analysis, the present Research Topic also collects a few articles that propose novel perspectives to understand oceanic phenomena. One of such studies is the work of Zhang & Xie. Noticing the similarity between the amplitude equation of near-inertial waves and the Schrödinger equation, they interpreted the large-scale concentration of near-inertial waves in anticyclones as a combined effect of energy conservation and uncertainty principle. It is quite interesting and even astonishing to see that oceanic phenomena can be explained by the basic concepts of quantum mechanics. Another interesting study is carried out by Azminuddin et al. By using a Lagrangian particle tracking method, they quantitatively explored the destination of the New Guinea Coastal Undercurrent and the associated water mass transformation. Although their tracer release experiment method is not new, it is not until recently that researchers can use this technique to disclose the pathways of deep waters. This could help to solve the puzzle of upwelling that goes back decades: where and how deep-ocean waters rise back to the surface to keep the overturning circulation going?
Finally, we end this Editorial by highlighting the work of Moscoso et al.. They showed that even table-top experiments built with LEGO modules can reproduce the multi-scale dynamics that are essential in deep ocean convection. Although these experiments just confirm the existing theories and do not provide any new insight, they are very useful for popular science education. In particular, these low-cost and elegant experiments may inspire young people to pursue careers in physical oceanography in the future. In this context, we hope this Research Topic can not only stimulate further innovative investigations and the next generation of ocean models, but also act as a source for inspiring the next generation of physical oceanographers.
CC: Writing – review & editing. S-DH: Writing – original draft, Writing – review & editing. HJ: Writing – review & editing. YY: Writing – review & editing. S-QZ: Writing – review & editing.
We thank all the authors and reviewers for their valuable contributions to this Research Topic.
The authors declare that the research was conducted in the absence of any commercial or financial relationships that could be construed as a potential conflict of interest.
All claims expressed in this article are solely those of the authors and do not necessarily represent those of their affiliated organizations, or those of the publisher, the editors and the reviewers. Any product that may be evaluated in this article, or claim that may be made by its manufacturer, is not guaranteed or endorsed by the publisher.
Keywords: oceanic flows, multi-scale fluid dynamics, interaction between flow and topology, air-sea interaction, table-top experiment, numerical simulation
Citation: Cenedese C, Huang S-D, Jiang H, Yuan Y and Zhou S-Q (2024) Editorial: Multi-scale fluid physics in oceanic flows: new insights from laboratory experiments and numerical simulations. Front. Mar. Sci. 10:1361265. doi: 10.3389/fmars.2023.1361265
Received: 25 December 2023; Accepted: 27 December 2023;
Published: 04 January 2024.
Edited and Reviewed by:
Ming Li, University of Maryland, College Park, United StatesCopyright © 2024 Cenedese, Huang, Jiang, Yuan and Zhou. This is an open-access article distributed under the terms of the Creative Commons Attribution License (CC BY). The use, distribution or reproduction in other forums is permitted, provided the original author(s) and the copyright owner(s) are credited and that the original publication in this journal is cited, in accordance with accepted academic practice. No use, distribution or reproduction is permitted which does not comply with these terms.
*Correspondence: Claudia Cenedese, Y2NlbmVkZXNlQHdob2kuZWR1; Shi-Di Huang, aHVhbmdzZEBzdXN0ZWNoLmVkdS5jbg==; Houshuo Jiang, aHNqaWFuZ0B3aG9pLmVkdQ==; Yeping Yuan, eXlwaW5nQHpqdS5lZHUuY24=; Sheng-Qi Zhou, c3F6aG91QHNjc2lvLmFjLmNu
Disclaimer: All claims expressed in this article are solely those of the authors and do not necessarily represent those of their affiliated organizations, or those of the publisher, the editors and the reviewers. Any product that may be evaluated in this article or claim that may be made by its manufacturer is not guaranteed or endorsed by the publisher.
Research integrity at Frontiers
Learn more about the work of our research integrity team to safeguard the quality of each article we publish.