- 1Anhui Province Key Laboratory of Aquaculture & Stock Enhancement, Fishery Institute of Anhui Academy of Agricultural Sciences, Hefei, China
- 2Key Laboratory of Freshwater Aquatic Genetic Resources, Ministry of Agriculture and Rural Affairs, Shanghai Ocean University, Shanghai, China
The color of crayfish (Procambarus clarkii) shells not only affects their value, but also has a significant impact on individual size. Astaxanthin is a common carotenoid that has been found in many studies to have different functions, including regulating body color formation. In this study, the levels of different carotenoids were detected using high performance liquid chromatography (HPLC) and found a significant correlation between astaxanthin content and shell color. An astaxanthin gene (PcASTA) was identified from P. clarkii, and it was expressed in tissues of crayfish with dark-red shells and green shells. The highest expression levels of PcASTA were found in the hepatopancreas, followed by the muscle. The expression of PcASTA in all tissues of red shell crayfish was higher than that of green shell crayfish, indicating that PcASTA may be involved in shell color formation in crayfish. Moreover, high water temperature and feeding β-carotene can lead to an increase in tissue expression of PcASTA and astaxanthin content in crayfish shells. The findings indicate that PcASTA may affect shell color in P. clarkii by being involved in astaxanthin synthesis and transformation.
1 Introduction
The red swamp crayfish (Procambarus clarkii) is a high-quality economic freshwater shrimp that is native to south central USA and northeastern Mexico. It is popular among consumers because of its attractive color, delicious flavor, and high nutritional value (high-quality protein, low lipid content, and rich minerals) (Amine et al., 2008). The red swamp crayfish was introduced from Japan in the 1930s and it has become the most important cultured crustacean with the largest aquaculture area and the highest total yield in China(Bureau of Fisheries and Fishery Management, 2021).
Crayfish belong to crustacean aquatic animals, and the shells are usually composed of an epicuticle, pigmented layer, calcified layer, and uncalcified layer. The growth of crustaceans is not continuous, but typically presents a discontinuous trapezoidal growth pattern, where the weight increases significantly after each molting (Freeman, 1990; Chengcong et al., 2019). As the number of molts increases during crayfish growth, the weight also gradually increases, the shell hardness increases, and the color becomes red. Once the crayfish shell turns dark red, it becomes strong, molting becomes difficult, and the individual size stabilizes as growth is hindered. In the market, individual shell color affects the price of crayfish; typically, red shell crayfish are most popular.
The shell color of aquatic crustaceans has a large impact on their commercial value. Such as greenish brown Astacus pallipes, orange-red Carcinus maenas, purple Hyriopsis cumingii and dark-red crayfish usually have higher market prices (Quarmby et al., 1977; Shahidi and Brown, 1998; Chen et al., 2021; Chen et al., 2022a). Studies have shown that the shell color of aquatic crustaceans is influenced by a variety of factors (e.g., pigments, metal elements, genetic, environmental factors) (Li et al., 2014b; Amaya and Nickell, 2015; Chen et al., 2017; Wang et al., 2020; Chen et al., 2021; Chen et al., 2022b).
Although there are many factors that affect the color of crustacean shells, some studies suggested that carotenoids are a key influencing factor (Shahidi and Brown, 1998; Niu et al., 2014). Carotenoids are a type of natural fat-soluble pigments that are synthesized by algae, plants, and microorganisms. Animals (including aquatic crustacean) cannot synthesize carotenoids on their own. They are obtained only by feeding and then converted into different types of carotenoids in the body (Miki et al., 1982; Yabuzaki, 2017). Carotenoids play a role in pigmentation, wound healing, and immune reactions in aquatic animals (Shahidi and Brown, 1998). More than 750 different carotenoids have been discovered, including canthaxanthin, echinenone, lutein, and astaxanthin, among others (Maoka, 2009). Shahidi and Brown suggested that astaxanthin is most widely distributed in aquatic animals, and is closely related to body color and shell color (Shahidi and Brown, 1998). Studies found that adding astaxanthin to feed is beneficial to improve shell color in aquatic crustaceans (Menasveta et al., 1993; Boonyaratpalin et al., 2001). Moreover, researchers have shown that aquatic crustaceans can convert a variety of carotenoids into astaxanthin, but the relevant regulatory genes and specific mechanisms by which that occurs are not clear.
In this study, the levels of different carotenoids in dark-red shell and green shell P. clarkii were determined. A new astaxanthin gene (PcASTA) was identified in P. clarkii for the first time. The expression levels of PcASTA in different tissues were also determined by qPCR. The content of astaxanthin in shells and PcASTA expression in different tissues was also determined in red shell P. clarkii. The findings suggested that PcASTA may affect shell color by affecting the synthesis and deposition of astaxanthin.
2 Materials and methods
2.1 Experimental animals
Two hundred red swamp crayfish (100 red shell crayfish and 100 green shell crayfish; weight = 24.67 ± 0.57 g; length = 6.57 ± 0.67 cm) were obtained from a crayfish breeding base in Chaohu City, Zhejiang Province, China. Before experimentation, all crayfish were acclimated for 1 week in tanks containing aerated fresh water.
2.2 Carotenoid content
The tail shell (TS) and cephalothorax shell (CS) were collected from six red shell crayfish (three males and three females) and six green crayfish (three males and three females), respectively. The TS and CS were then cleaned with 1 × PBS (phosphate buffered saline). All shells were frozen at −80°C for 24 h, and then a vacuum freeze-drying machine (Model FD-ID-50, Shanghai, China) was used to freeze-dry shells. The lyophilized (48 h) shells were ground into fine powder at room temperature, and ground TSs and CSs of the same shell color were mixed separately. Each sample (0.4 g) was mixed with 2 mL of acetone and cleaned by ultrasound for 15 min. After centrifugation at 4000 rpm for 10 min at 4°C, supernatants were collected and the insoluble fraction was subjected to the procedures described above 4-5 times (until the supernatant is colorless). All supernatants were collected into fresh tubes and diluted to 15 mL with acetone. A spectrophotometer (NanoDrop 2000c, Thermo Scientific, USA) was used to determine the O.D. of each sample at 470 nm. The extraction and determination processes were completed in the dark. Purified astaxanthin (purity: 95.2%, Dr. ehrenstorfer) was used as the standard with which a standard curve was established.
High performance liquid chromatography (HPLC) (Agilent 1260, Agilent Technologies, USA) with a YMC carotenoid separation column (YMC-carotenoid TM, YMC, Japan) was used to separate and quantify carotenoids in each sample. Briefly, 2.9 mL NaOH-methanol solution (0.02 mol/L) was added to 2 mL samples and let stand at 4°C for 12 h after sealing. Then, 0.6 mol/L phosphoric acid methanol solution and 100 mg PSA (Pressure Swing Adsorption) adsorbent was added and filtered using a 0.2 μm microporous filter membrane. Finally, 20 μL of treated sample was used for testing. Two mobile phases, methanol (A) and methyl tert butyl ether (B) were used for step-by-step elution, with a 1.0 mL/min flow rate and column temperature of 25°C. Five purified carotenoid standards (astaxanthin, zeaxanthin, lutein, echinenone and β-carotene) were used for qualitative and quantitative analysis.
2.3 RNA extraction and full-length cDNA cloning of the astaxanthin gene
The hepatopancreas (0.15 g) was collected from red shelled crayfish and immediately frozen in liquid nitrogen and stored at −80°C until use. Total RNA was extracted using TRIZOL reagent (Invitrogen, Carlsbad, CA, USA), according to the manufacturer’s instructions. Then, a spectrophotometer (NanoDrop 2000c, Thermo, USA) and electrophoresis with 1% agarose gels were used to determined quantity, purity and integrity of RNA. The hepatopancreas cDNA was obtained using the SMART RACE cDNA amplification kit (Clontech, Dalian, China). Based on the sequence characteristics of astaxanthin gene in relevant study and combined with the genomic information of P. clarkii, we obtained the predicted astaxanthin sequence (XM_045755678.1) from the NCBI database (He-Ling et al., 2012). Then the full-length cDNA of PcASTA was cloned with 5’- and 3’-RACE, and specific primers (Table 1) were designed based on the predicted sequence. The PCR protocol used was as follows: 5 min at 94°C; 32 cycles at 94°C for 30 s, 58°C for 30 s, and 72°C for 1 min, and a 10 min final extension at 72°C. The PCR products were sequenced using the Sanger method (Sangon, Shanghai, China).
2.4 Sequence analysis
The NCBI ORF Finder tool (http://www.ncbi.nlm.nih.gov/projects/gorf/) was used to identify the open reading frame (ORF) of PcASTA(Rong, 2006). The homology analyses of the PcASTA nucleotide and protein sequences were performed using the BLAST program (http://www.ncbi.nlm.gov/blast)(Rong, 2006). The structural domain of the amino acid sequence was identified using the Simple Modular Architecture Research Tool (http://smart.embl-heidelberg.de/). Amino acid sequence composition, molecular weight, isoelectric point, and other physical parameter information were obtained using the online Protparam (http://www.expasy.org/tools/protparam) tool (Gasteiger et al., 2005). Multiple sequence alignment of the astaxanthin gene was performed using Clustalx software (Thompson et al., 1997). MEGA 5.2 was used to construct a phylogenetic tree with the maximum likelihood method, and the aligned sequences were bootstrapped until 1000 replicates were performed (Kumar et al., 2008).
2.5 Tissue expression of PcASTA
First, different tissues (hepatopancreas, muscle, gonad, intestine, tail shell membrane and cephalothorax shell membrane) were obtained from eight red shell crayfish (four females and four males) and eight green shell crayfish (four females and four males). Matched tissues were then mixed and total RNA was extracted according to the methods described above. The PrimeScript™ RT reagent kit with gDNA Eraser (Takara, Dalian, China) was used to synthesize cDNA for qPCR analysis. Specific primers (Table 1) were designed based on the sequence of PcASTA. The 18s sequence (Table 1) was used as the internal reference. qPCR was carried out in a quantitative thermal cycler (Bio-Rad CFX-96; Bio-Rad, USA), with the following parameters: 3 min at 95°C; then 40 cycles of 95°C for 5 s, 57.5°C for 30 s and 72°C for 30 s. Finally, tissue-specific expression of PcASTA was analyzed using the comparative CT method (2−ΔΔCt).
2.6 Impact of β-carotene
Sixty healthy red shell P. clarkii were divided into two groups (30 crayfish per group), where one group was fed a common commercial crayfish diet (3% of biomass), and the other was fed the commercial crayfish diet with added β-carotene (80 mg/kg). After two weeks of breeding at room temperature (28 ± 2°C), eight crayfish (four females and four males) were randomly selected from each group and the shells and tissues were taken according to the methods described above. The content of astaxanthin in shells and the relative expression of PcASTA in the tissues were also detected according to the methods described above.
2.7 Temperature stress
Sixty healthy red shell P. clarkii were divided into two groups (30 crayfish per group), and two groups were cultured at a water temperature of 20°C (group A) and 30°C (group B) for 7 days, respectively. The shells and tissues were collected according to the methods described above. The content of astaxanthin in shells and the relative expression of PcASTA in the tissues were detected according to the methods described above.
2.8 Statistical analysis
Data are shown as the mean ± SD. One-way ANOVA was performed using SPSS 17.0 (SPSS, Chicago, IL, USA) to determine whether there were any significant differences between groups. A p-value < 0.05 was considered statistically significant.
3 Results
3.1 Analysis of carotenoid content in different color shells
As shown in Figure 1, five different carotenoids were detected in red and green shell crayfish. The total carotenoid content in red shells (TS and CS) was significantly higher than that in green shells (TS and CS). The content of astaxanthin in all shrimp shells was significantly higher than in other carotenoids. The content of lutein and β-carotene was also higher, while the content of zeaxanthin and echinenone were the lowest. Moreover, the content of various carotenoids in the TS was higher than that in the CS of the same-color crayfish.
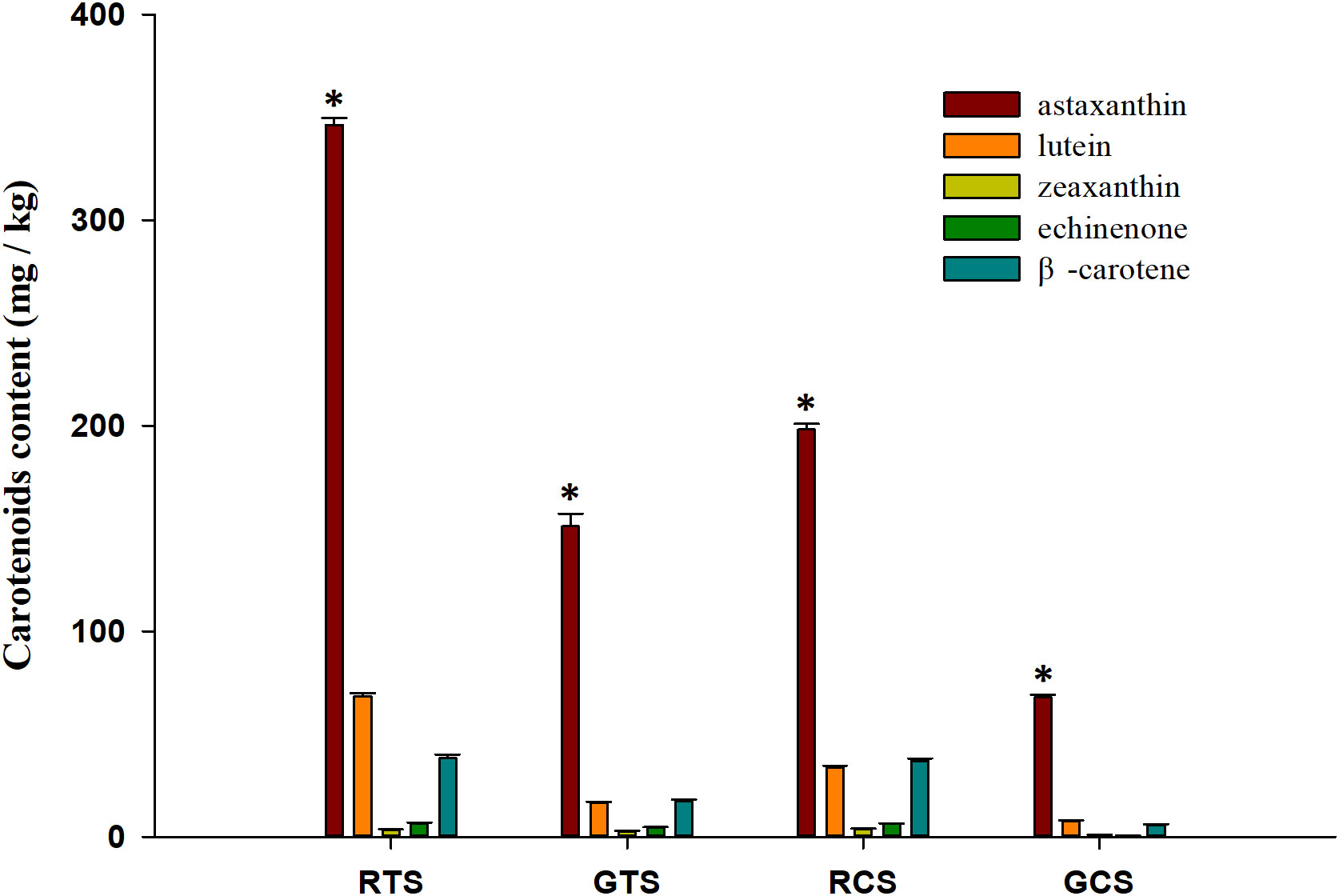
Figure 1 Different carotenoids contents in shells. RTS, red tail shell; GTS, green tail shell; RCS, red cephalothorax shell; GCS, green cephalothorax shell. “*” indicate significant differences in astaxanthin content compared to other carotenoids in each shell (p < 0.05).
3.2 Cloning and sequence analysis of PcASTA
The full-length cDNA of PcASTA (Accession No. OR514599) was obtained by 3’- and 5’- RACE (Figure S1). The sequence contained a 27-bp 5’-UTR, a 556-bp 3’-UTR, and a 747-bp open reading frame (ORF). The ORF encoded a polypeptide of 248 amino acids with a calculated molecular weight of 27.6 kDa and a theoretical isoelectric point of 4.67. It also contained a conserved zinc metallopeptidase domain (HEXXHXXGFXHEXXRXDRD). A phylogenetic tree was constructed and indicated that the amino acid sequence of PcASTA belonged to the same clade as astaxanthin from Astacus astacus, with a confidence level of 100% (Figure 2).
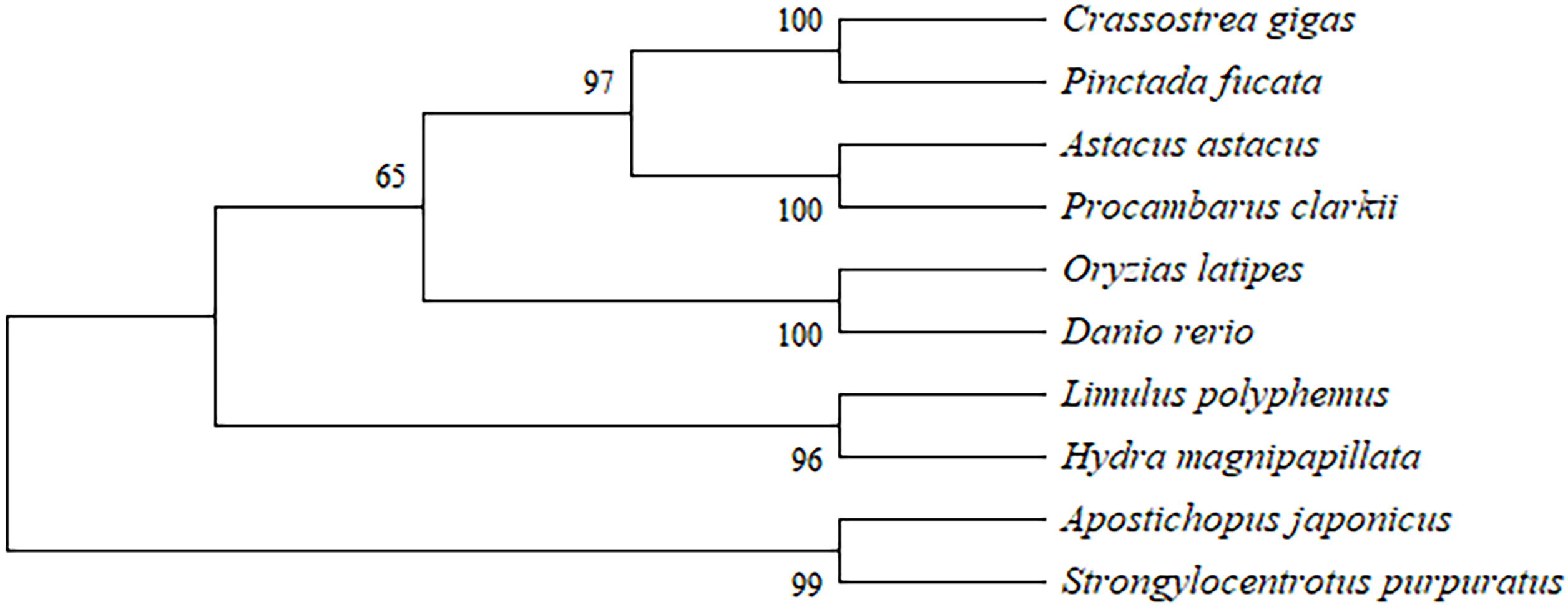
Figure 2 Phylogenetic analysis based on multiple sequence alignment. GenBank accession numbers: Crassostrea gigas (AAQ13464), Pinctada fucata (AAX56337), Astacus astacus (CAA64981), Procambarus clarkii (OR514599), Oryzias latipes (NP_001098208), Danio rerio (XP_001342763), Limulus polyphemus (CAQ16892), Hydra magnipapillata (XP_002164184), Apostichopus japonicus (HQ655812), and Strongylocentrotus purpuratus (XP_786540). Numbers are the bootstrap values for 1000 trials.
3.3 Tissue expression analysis
qPCR was used to detect the expression levels of PcASTA in different tissues of red and green shell crayfish. As shown in Figure 3, PcASTA was expressed in all tissues from both types of crayfish. In green shell crayfish, the highest level of PcASTA was in hepatopancreas, followed by muscle. Expression in those tissues was significantly higher than that in other tissues (p < 0.05). The levels of expression in the remaining tissues followed the order: tail shell membrane < gonad < intestine < cephalothorax shell membrane. In red shell crayfish, the hepatopancreas also had the highest level of expression, followed by muscle. Expression in both tissues was significantly higher than that in the other tissues (p < 0.05). The levels of expression in the remaining tissues were similar to that in green shell crayfish (tail shell membrane < intestine < gonad < cephalothorax shell membrane).
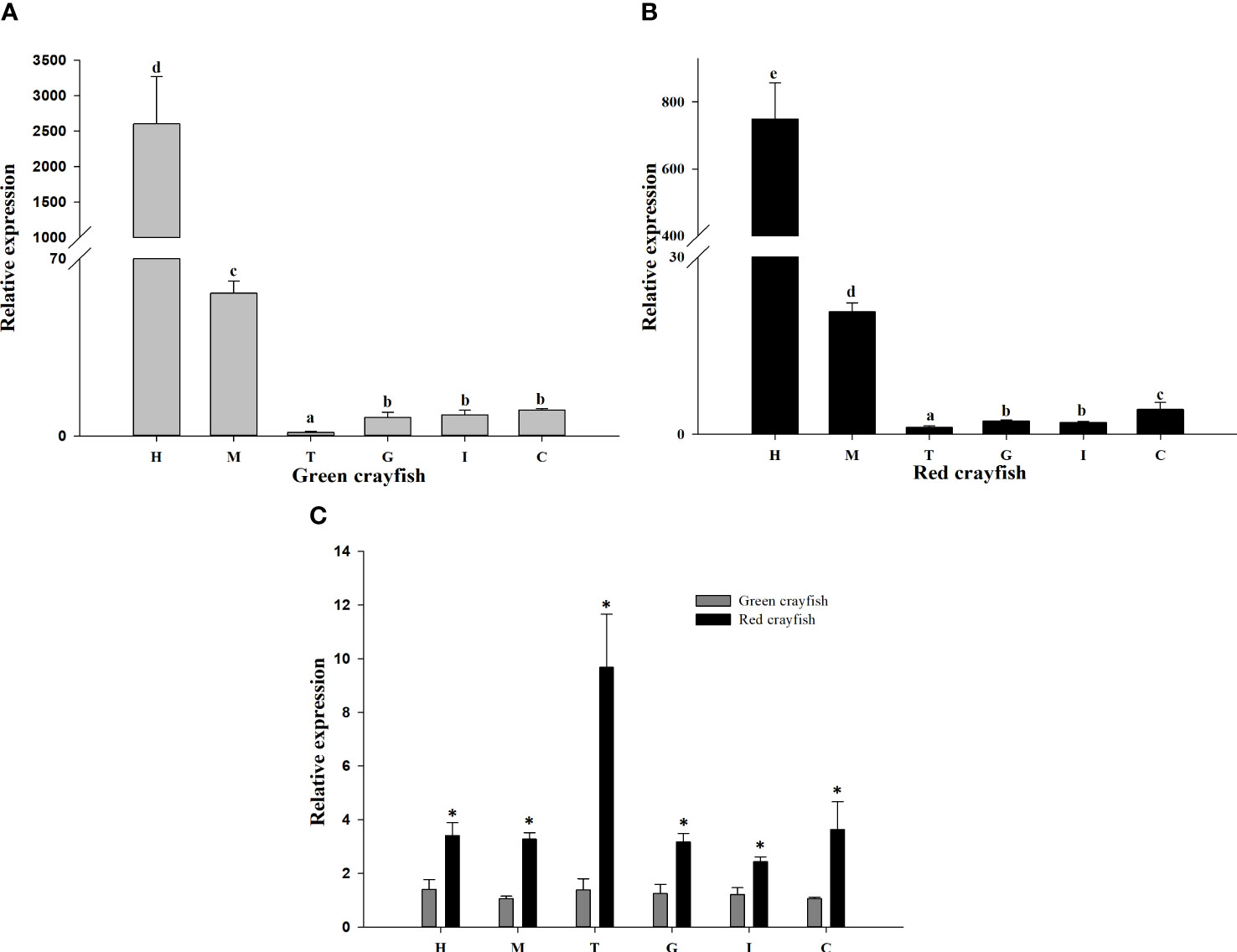
Figure 3 Relative expression of PcASTA. The relative expression of PcASTA in various tissues from green (A) and red (B) crayfish. Comparisons of PcASTA expression in green and red crayfish (C). H, hepatopancreas; M, muscle; T, tail shell membrane; G, gonad; I, intestine; C, cephalothorax shell membrane. Bars with different letters indicate significant differences in PcASTA expression levels between different tissues (p < 0.05). “*” indicates significant differences in PcASTA expression levels between the same tissues of two color crayfish (p < 0.05).
Comparing the expression levels of PcASTA in different tissues of the two colors of crayfish, it was observed that expression was significantly higher in all tissues from red crayfish compared to green crayfish (p < 0.05). Moreover, the most significant difference in expression was in tail shell membrane.
3.4 The impact of β-carotene
The astaxanthin content in shells and the expression levels of PcASTA were detected in crayfish fed a commercial diet containing β-carotene. As shown in Figure 4A, the astaxanthin content of CS and TS among crayfish fed a diet containing β-carotene was significantly higher than in crayfish fed a commercial diet (p < 0.05).
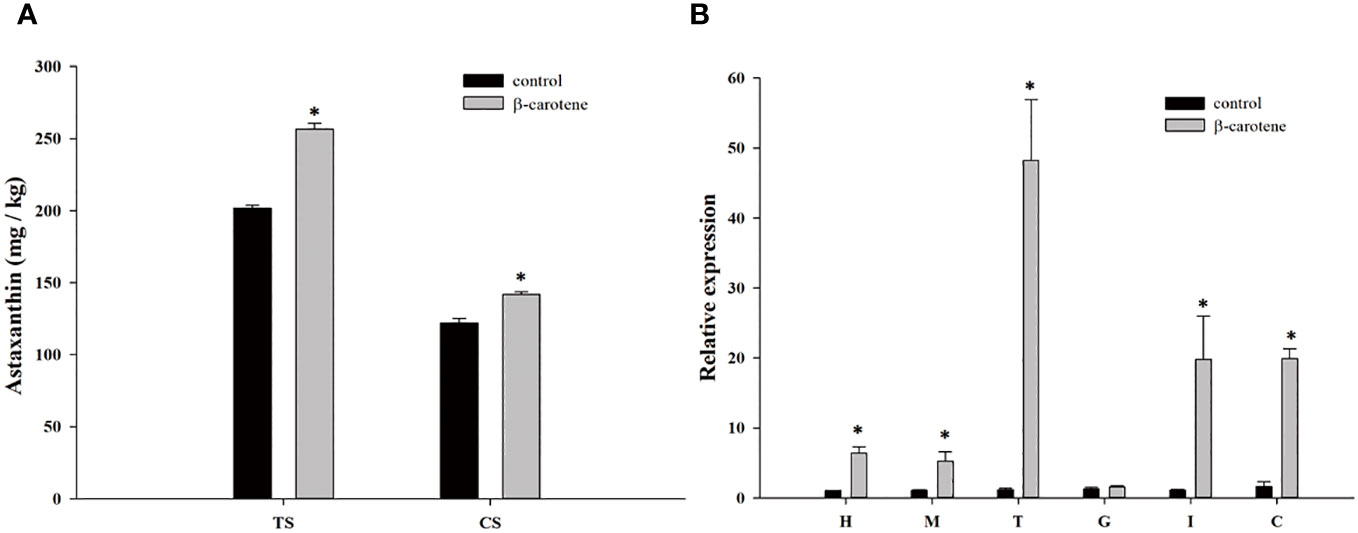
Figure 4 Astaxanthin content in shells (A) and the expression level of PcASTA in different tissues (B) following fed β-carotene. TS, tail shell; CS, cephalothorax shell; H, hepatopancreas; M, muscle; T, tail shell membrane; G, gonad; I, intestine; C, cephalothorax shell membrane. “*” indicates significant differences in astaxanthin content between the same shells and PcASTA expression levels between the same tissues of two groups (p < 0.05), the same as below.
As shown in Figure 4B, the expression of PcASTA in all tissues tested (except gonad) in crayfish fed a diet containing β-carotene was significantly higher than in crayfish fed a commercial diet (p < 0.05).
3.5 The impact of temperature
The astaxanthin content in shells was evaluated in crayfish cultured at different water temperatures. As shown in Figure 5A, the astaxanthin content of the TS in crayfish from group B was significantly higher than that of group A (p < 0.05). However, the astaxanthin content in the CS of group B crayfish was higher than that of group A crayfish, but the difference was not significant.
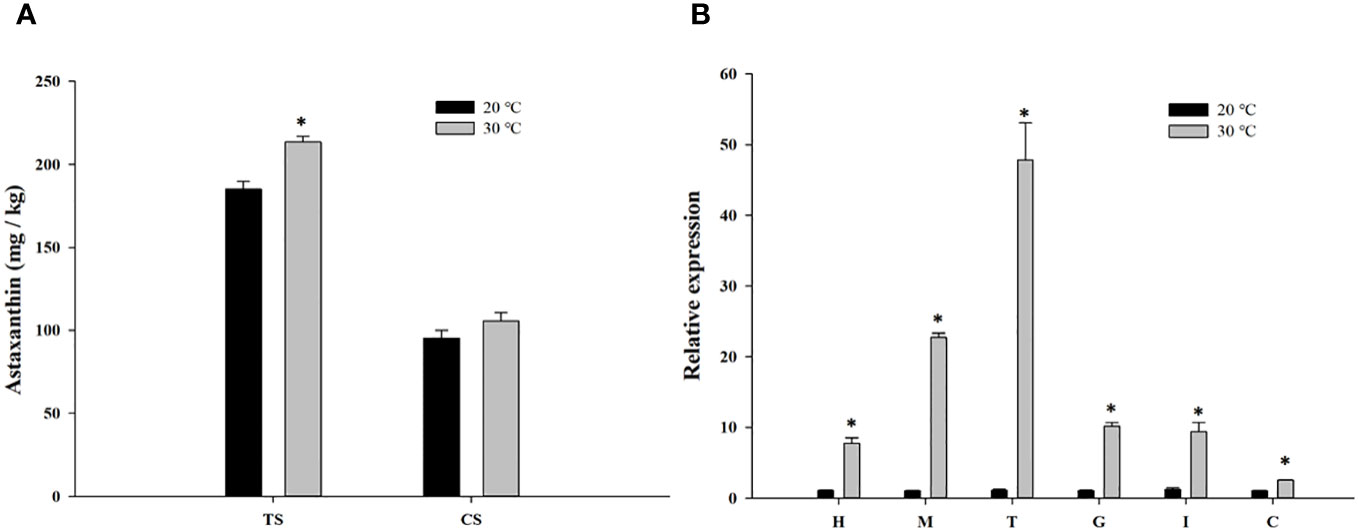
Figure 5 Astaxanthin content in shells (A) and the expression level of PcASTA in different tissues (B) following cultured in different temperature. TS, tail shell; CS, cephalothorax shell; H, hepatopancreas; M, muscle; T, tail shell membrane; G, gonad; I, intestine; C, cephalothorax shell membrane. “*” indicates significant differences in astaxanthin content between the same shells and PcASTA expression levels between the same tissues of two groups (p < 0.05).
The expression of PcASTA in different tissues from crayfish in groups A and B were also detected (Figure 5B). PcASTA was expressed in all tissues, but the expression levels in each tissue from group B crayfish was significantly higher than that of group A crayfish (p < 0.05).
4 Discussion
Carotenoids are natural pigments, and play important roles in color formation in aquatic animals. Some studies have found that carotenoids not only affect muscle color, but also affect the shell color of shellfish (Li et al., 2010; Li et al., 2014b). In this study, different carotenoids were detected in red and green shell crayfish. We found that all types of carotenoids in the TS and CS of red shell crayfish were higher than in green shell crayfish, which means that carotenoids may affect shell color. Although animals can only obtain carotenoids (including astaxanthin) through their diet, Wade et al. indicated that crustaceans can convert one carotenoid to another (Wade et al., 2017). Some studies found that β-carotene and zeaxanthin were converted to astaxanthin through a complex process (Tanaka et al., 1976; Boonyaratpalin et al., 2001). The results also showed that astaxanthin was the carotenoid with the highest levels in shells, and its content in different color shells was also significantly different. Astaxanthin is a common carotenoid, which mainly exists in crustaceans and algae (Shahidi and Brown, 1998). Some studies indicated that astaxanthin is not only an antioxidant, but also a colorant (Shimidzu et al., 1996; Boonyaratpalin et al., 2001). Vogt et al. revealed that astaxanthin is the main colorant in aquatic animals, and the astaxanthin gene was first detected in the hepatopancreas of crayfish (Vogt et al., 1989). Those data indicated that astaxanthin may be the most important factor affecting the shell color of crayfish.
Although many studies have shown that the astaxanthin plays a role in the shell color formation of aquatic crustaceans, the specific molecular mechanisms by which that occurs are unclear. In this study, the astaxanthin gene (PcASTA) from P. clarkii was successfully cloned. The amino acid sequence contained a zinc metallopeptidase domain, which belongs to the conserved sequence of the astaxanthin superfamily. The ‘HEXXH’ motif is essential for zinc binding, and the biological function of zinc metallopeptidase requires the binding of zinc to this site (Bode et al., 1992). Moreover, phylogenetic analyses showed that the PcASTA amino acid sequence belonged to the same clade as astaxanthin sequences from A. astacus. Therefore, the results suggested that PcASTA belongs to the astaxanthin superfamily. Astaxanthin genes have been found in many species, but their role in color formation remains unclear.
One study suggested a close relationship between the astaxanthin gene and sea cucumber body color (He-Ling et al., 2012). In this study, astaxanthin gene expression was the highest in the hepatopancreas of crayfish of two different colors. Studies have shown that the hepatopancreas is an important organ for metabolism and the storage of carotenoids (Schumaker et al., 1994; Vershinin, 1996). Some researchers also suggested that the expression levels of carotenoid-related genes were closely related to the levels of carotenoids in tissues (Schumaker et al., 1994; Li et al., 2014a). The high levels of PcASTA expression might be due to its involvement in metabolism and the storage of carotenoids in the hepatopancreas. Further comparing the expression levels of astaxanthin genes in the tissues of two different color crayfish, it was found that the expression levels of PcASTA in tissues from red shell crayfish were significantly higher than in green shell crayfish. The difference was greatest in the CS membrane, and there was also a significant difference in the TS membrane.
Simkiss et al. indicated that the shell membrane provided a mineralization template and participates in calcification during the formation of crustacean shells (Simkiss and Wilbur, 1989). Shechter et al. found a large amount of uncalcified chitin in the shell membranes of red claw crayfish (Shechter et al., 2008). Chitin is synthesized in crustaceans, and then forms a hard shell through a series of complex processes such as transportation, deposition, and calcification. Thus, chitin is indispensable for the formation of crustacean shells (Muzzarelli, 2013). These results suggested that PcASTA may be involved in shell color formation in P. clarkii.
Crustaceans can convert one carotenoid to another, and Matsuno indicated that the major conversion pathway of carotenoids to astaxanthin in crustaceans was the conversion of β-carotene to astaxanthin (Matsuno, 2001; Wade et al., 2017). Some studies have found a significant increase in astaxanthin content in shrimp and crab after feeding on β-carotene (Campbell, 1969; Boonyaratpalin et al., 2001). In this study, the astaxanthin content in the CS and TS increased after feeding on β-carotene, and the expression levels of PcASTA also increased. Those results suggest that crayfish can also convert β-carotene to astaxanthin, and PcASTA may play an important role in that process. Moreover, we also found that high temperatures caused an increase in the expression of PcASTA and the levels of astaxanthin in shells. Cheesman et al. found that high temperatures cause esterified astaxanthin to transform into free astaxanthin in crustaceans, thus, darkening the shell color (Cheesman et al., 1967). Carla et al. found that the absorption and metabolism of carotenoids in animals were also affected by temperature (De Carvalho and Caramujo, 2017). Therefore, we hypothesize that PcASTA may affect shell color in P. clarkii by participating in the synthesis and transformation of astaxanthin.
In conclusion, this study for the first time indicated that PcASTA may be an important gene in the synthesis of astaxanthin, affecting the shell color of P. clarkii. These findings will help to understand the mechanisms of shell color formation, and thus, improve the crayfish farming industry.
Data availability statement
The datasets presented in this study can be found in online repositories. The names of the repository/repositories and accession number(s) can be found in the article/Supplementary Material.
Ethics statement
The animal study was approved by Institutional Animal Care and Use Committee (IACUC) of Anhui Academy of Agricultural Sciences, Hefei, China. The study was conducted in accordance with the local legislation and institutional requirements.
Author contributions
XC: Conceptualization, Data curation, Methodology, Writing – original draft, Writing – review & editing. JC: Investigation, Project administration, Software, Writing – original draft. LH: Investigation, Resources, Software, Writing – review & editing. BW: Methodology, Software, Writing – review & editing. CW: Investigation, Validation, Writing – original draft. JH: Conceptualization, Project administration, Supervision, Writing – review & editing. ZB: Project administration, Supervision, Writing – review & editing.
Funding
The author(s) declare financial support was received for the research, authorship, and/or publication of this article. This study was financially supported by Open Funding Project of the Key Laboratory of Freshwater Aquatic Genetic Resources, Ministry of Agriculture and Rural Affairs, Talent Project of Anhui Academy of Agricultural Sciences (QNYC-202215) and Key R&D Program of Anhui Province (202104a06020006).
Acknowledgments
We thank International Science Editing (http://www.internationalscienceediting.com) for editing this manuscript.
Conflict of interest
The authors declare that the research was conducted in the absence of any commercial or financial relationships that could be construed as a potential conflict of interest.
Publisher’s note
All claims expressed in this article are solely those of the authors and do not necessarily represent those of their affiliated organizations, or those of the publisher, the editors and the reviewers. Any product that may be evaluated in this article, or claim that may be made by its manufacturer, is not guaranteed or endorsed by the publisher.
Supplementary material
The Supplementary Material for this article can be found online at: https://www.frontiersin.org/articles/10.3389/fmars.2023.1343126/full#supplementary-material
References
Amaya E., Nickell D. (2015). Using feed to enhance the color quality of fish and crustaceans. Feed feeding practices aquaculture, 269–298. doi: 10.1016/B978-0-08-100506-4.00011-8
Amine T. M., Aiad A. S., Abu El-Nile M. O. (2008). Evaluation of chemical quality and nutrition value of fresh water cray fish (Procambarus clarkii). J. High Institute Public Health 38(Proceedings 2nd Int. Conf. High Institute Public Health" AlexHealth 2008"), 1–13. doi: 10.21608/jhiph.2008.22496
Bode W., Gomis-Rüth F. X., Huber R., Zwilling R., Stöcker W. (1992). Structure of astacin and implications for activation of astacins and zinc-ligation of collagenases. Nature 358 (6382), 164–167. doi: 10.1038/358164a0
Boonyaratpalin M., Thongrod S., Supamattaya K., Britton G., Schlipalius L. (2001). Effects of β-carotene source, Dunaliella salina, and astaxanthin on pigmentation, growth, survival and health of Penaeus monodon. Aquaculture Res. 32, 182–190. doi: 10.1046/j.1355-557x.2001.00039.x
Bureau of Fisheries and Fishery Management. (2021). China fisheries statistical yearbook in 2021 (Beijing, China: Chinese Agricultural Press).
Campbell S. A. (1969). Carotenoid metabolism in the commensal crab Pinnotheres pisum. Comp. Biochem. Physiol. 30 (5), 803–812. doi: 10.1016/0010-406X(69)90035-8
Cheesman D. F., Lee W. L., Zagalsky P. F. (1967). Carotenoproteins in invertebrates. Biol. Rev. 42 (1), 131–160. doi: 10.1111/j.1469-185X.1967.tb01343.x
Chen X., He J., Zhang M., Bai Z., Li J. (2022b). Identification of a coproporphyrinogen-III oxidase gene and its correlation with nacre color in Hyriopsis cumingii. PloS One 17 (3), e0265318. doi: 10.1371/journal.pone.0265318
Chen H., Ji H., Pan C., Zhang D., Su W., Liu S., et al. (2022a). Purification and characterisation of two novel pigment proteins from the carapace of red swamp crayfish (Procambarus clarkii). Foods 11 (1), 35. doi: 10.3390/foods11010035
Chen X., Liu X., Bai Z., Zhao L., Li J. (2017). HcTyr and HcTyp-1 of Hyriopsis cumingii, novel tyrosinase and tyrosinase-related protein genes involved in nacre color formation. Comp. Biochem. Physiol. Part B: Biochem. Mol. Biol. 204, 1–8.
Chen X., Zhang M., Bai Z., He J., Li J. (2021). The mantle exosome proteins of Hyriopsis cumingii participate in shell and nacre color formation. Comp. Biochem. Physiol. Part D: Genomics Proteomics 39, 100844. doi: 10.1016/j.cbd.2021.100844
Chengcong Y., Zhenyan D., Aimin W., Fei L., Hongyan T., Wenping Y. (2019). Research progress of molting for crustacean aquatic animals. J. Yancheng Institute Technology(Natural Sci. Edition) 32 (4), 42–46.
De Carvalho C. C. C. R., Caramujo M. J. (2017). Carotenoids in aquatic ecosystems and aquaculture: A colorful business with implications for human health. Front. Mar. Sci. 4. doi: 10.3389/fmars.2017.00093
Freeman J. A. (1990). Molt increment, molt cycle duration, and tissue growth in Palaemonetes pugio Holthuis larvae. J. Exp. Mar. Biol. Ecol. 143 (1), 47–61. doi: 10.1016/0022-0981(90)90110-X
Gasteiger E., Hoogland C., Gattiker A., Duvaud S.E., Wilkins M. R., Appel R. D., et al. (2005). “Protein Identification and Analysis Tools on the expasy Server,” in The Proteomics Protocols Handbook. Ed. Walker. J. M. (Totowa, NJ: Humana Press), 571–607.
He-Ling Z., Hong-Sheng Y., Huan Z., Shi-Lin L. (2012). Cloning and expression of astacin gene in albino and normal sea cucumber (Apostichopus japonicus Selenka). Mar. Sci. 36 (3), 22–28.
Kumar S., Nei M., Dudley J., Tamura K. (2008). MEGA: A biologist-centric software for evolutionary analysis of DNA and protein sequences. Briefings Bioinf. 9 (4), 299–306. doi: 10.1093/bib/bbn017
Li X., Bai Z., Luo H., Liu Y., Wang G., Li J. (2014a). Cloning, differential tissue expression of a novel hcApo gene, and its correlation with total carotenoid content in purple and white inner-shell color pearl mussel Hyriopsis cumingii. Gene 538 (2), 258–265. doi: 10.1016/j.gene.2014.01.046
Li X., Bai Z., Luo H., Wang G., Li J. (2014b). Comparative analysis of total carotenoid content in tissues of purple and white inner-shell color pearl mussel, Hyriopsis cumingii. Aquaculture Int. 22 (5), 1577–1585. doi: 10.1007/s10499-014-9766-6
Li N., Hu J., Wang S., Cheng J., Hu X., Lu Z., et al. (2010). Isolation and identification of the main carotenoid pigment from the rare orange muscle of the Yesso scallop. Food Chem. 118 (3), 616–619. doi: 10.1016/j.foodchem.2009.05.043
Maoka T. (2009). Recent progress in structural studies of carotenoids in animals and plants. Arch. Biochem. biophysics 483 (2), 191–195. doi: 10.1016/j.abb.2008.10.019
Matsuno T. (2001). Aquatic animal carotenoids. Fisheries Sci. 67 (5), 771–783. doi: 10.1046/j.1444-2906.2001.00323.x
Menasveta P., Worawattanamateekul W., Latscha T., Clark J. (1993). Correction of black tiger prawn (Penaeus monodon Fabricius) coloration by astaxanthin. Aquacultural Eng. 12 (4), 203–213. doi: 10.1016/0144-8609(93)90012-Z
Miki W., Yamaguchi K., Konosu S. (1982). Comparison of carotenoids in the ovaries of marine fish and shellfish. Comp. Biochem. Physiol. B Comp. Biochem. 71 (1), 7–11. doi: 10.1016/0305-0491(82)90167-5
Niu J., Wen H., Li C.-H., Liu Y.-J., Tian L.-X., Chen X., et al. (2014). Comparison effect of dietary astaxanthin and β-carotene in the presence and absence of cholesterol supplementation on growth performance, antioxidant capacity and gene expression of Penaeus monodon under normoxia and hypoxia condition. Aquaculture 422, 8–17. doi: 10.1016/j.aquaculture.2013.11.013
Quarmby R., Nordens D., Zagalsky P., Ceccaldi H., Daumas R. (1977). Studies on the quaternary structure of the lobster exoskeleton carotenoprotein, crustacyanin. Comp. Biochem. Physiol. Part B: Comp. Biochem. 56 (1), 55–61. doi: 10.1016/0305-0491(77)90222-X
Rong O. U. (2006). A new pubMed search tool——NCBI search toolbar and its applications. Res. Med. Educ. 05 (05), 474–475.
Schumaker V. N., Phillips M. L., Chatterton J. E. (1994). “Apolipoprotein B and Low-Density Lipoprotein Structure: Implications for Biosynthesis of Triglyceride-Rich Lipoproteins,” in Advances in Protein Chemistry. Eds. Anfinsen C. B., Edsall J. T., Richards F. M., Eisenberg. D. S. (Academic Press), 45, 205–248.
Shahidi F., Brown J. A. (1998). Carotenoid pigments in seafoods and aquaculture. Crit. Reveiws Food Sci. 38 (1), 1–67. doi: 10.1080/10408699891274165
Shechter A., Berman A., Singer A., Freiman A., Grinstein M., Erez J., et al. (2008). Reciprocal changes in calcification of the gastrolith and cuticle during the molt cycle of the red claw crayfish cherax quadricarinatus. Biol. Bull. 214 (2), 122–134. doi: 10.2307/25066669
Shimidzu N., Goto M., Miki W. (1996). Carotenoids as singlet oxygen quenchers in marine organisms. Fisheries Sci. 62 (1), 134–137. doi: 10.2331/fishsci.62.134
Simkiss K., Wilbur K. M. (1989). Biomineralization : cell biology and mineral deposition. Q. Rev. Biol. 1 (3), 257–264.
Tanaka Y., Matsuguchi H., Katayama T., Simpson K. L., Chichester C. O. (1976). The biosynthesis of astaxanthin. XVIII. The metabolism of the carotenoids in the prawn, Penaeus japonicus bate.:The Metabolism of the Carotenoids in the Prawn, Penaeus japonicus Bate. NIPPON SUISAN GAKKAISHI 42 (2), 197–202.
Thompson J. D., Gibson T. J., Plewniak F., Jeanmougin F., Higgins D. G. (1997). The CLUSTAL_X windows interface: flexible strategies for multiple sequence alignment aided by quality analysis tools. Nucleic Acids Res. 25 (24), 4876–4882. doi: 10.1093/nar/25.24.4876
Vershinin A. (1996). Carotenoids in mollusca: approaching the functions. Comp. Biochem. Physiol. Part B: Biochem. Mol. Biol. 113 (1), 63–71. doi: 10.1016/0305-0491(96)00104-6
Vogt G., Stöcker W., Storch V., Zwilling R. (1989). Biosynthesis of Astacus protease, a digestive enzyme from crayfish. Histochemistry 91 (5), 373–381. doi: 10.1007/BF00493824
Wade N. M., Gabaudan J., Glencross B. D. (2017). A review of carotenoid utilisation and function in crustacean aquaculture. Rev. Aquaculture 9 (2), 141–156. doi: 10.1111/raq.12109
Wang Z., Adzigbli L., Zheng Z., Yang C., Deng Y. (2020). How cultured pearls acquire their colour. Aquaculture Res. 51 (10), 3925–3934. doi: 10.1111/are.14765
Keywords: Procambarus clarkii, carotenoid, astaxanthin, shell, color
Citation: Chen X, Chen J, Huang L, Wu B, Wu C, He J and Bai Z (2024) PcASTA in Procambarus clarkii, a novel astaxanthin gene affecting shell color. Front. Mar. Sci. 10:1343126. doi: 10.3389/fmars.2023.1343126
Received: 23 November 2023; Accepted: 22 December 2023;
Published: 11 January 2024.
Edited by:
Ji Hyung Kim, Gachon University, Republic of KoreaCopyright © 2024 Chen, Chen, Huang, Wu, Wu, He and Bai. This is an open-access article distributed under the terms of the Creative Commons Attribution License (CC BY). The use, distribution or reproduction in other forums is permitted, provided the original author(s) and the copyright owner(s) are credited and that the original publication in this journal is cited, in accordance with accepted academic practice. No use, distribution or reproduction is permitted which does not comply with these terms.
*Correspondence: Jixiang He, aGVqaXhpYW5nYWhAc2luYS5jb20=; Zhiyi Bai, enliYWlAc2hvdS5lZHUuY24=