- Guangdong Provincial Key Laboratory of Marine Disaster Prediction and Prevention, Shantou University, Shantou, China
Some marine mammals can adjust acoustic parameters of their sound signals in response to changes in sunlight and ambient noise. Evidence was found in several toothed whales; however, relatively little research has focused on true seals. Spotted seals (Phoca largha) are highly vociferous during the breeding season. Four major underwater call types, drums, growls, knocks, and sweeps, have been identified in the acoustic repertoire of wild spotted seals in Liaodong Bay, China. This study investigated the potential effects of light (day and night) and noise (105–135 dB re 1 μPa, broadband 50–8,000 Hz) conditions on the centroid frequency (CF), root-mean-square bandwidth (BW), duration (DU), and received level (RL) parameters of their underwater vocalisations using a series of generalised linear models (GLMs). Results showed that there were significant interaction effects of light and noise on knock CFs and the BWs for all call types. Specifically, knock CFs decreased and BWs for all call types became narrower when noise increased at night but kept stable during daytime. No significant interactions were found in other acoustic parameters. However, the results of univariate GLM analyses showed that the spotted seals could lengthen their vocalisations at night and increase the vocalisation RLs in response to higher noise levels (the Lambard effect), regardless of call type. To our knowledge, this is the first study to demonstrate that the spotted seals, a poorly studied species in Chinese waters, have some capabilities to adjust their vocalisations in response to changes in light and noise conditions. The results are helpful to understand how seals interact with their habitats and useful when developing mitigation strategies to minimise disturbance of light and noise from human activities in Liaodong Bay, China.
1 Introduction
Most marine mammals rely on sounds for underwater communication (Au and Hastings, 2008). Some species have been shown to be capable of adjusting their sound signals to optimise communication dependent on natural sunlight (referred to as light hereafter) and ambient noise (excluding the conspecific and other biotic underwater sounds, referred to as noise hereafter) (Brumm, 2013). There are a number of studies that aim to explore how toothed whales (odontocetes) adjust their ultrasonic click and whistle sounds in response to changes in light or noise (e.g., Foote et al., 2004; Soldevilla et al., 2010; Deconto and Monteiro-Filho, 2016; Fouda et al., 2018; Ogawa and Kimura, 2023). True seals (Phocidae) are known to be quite vocal underwater, especially during the breeding seasons, and their vocalisations play an important role in coordinating social and sexual behaviour (Costa and McHuron, 2022). However, to my knowledge, only a few studies exist on the noise effects on the acoustic parameter adjustments of true seals (Matthews et al., 2020; Fournet et al., 2021; Yang et al., 2022) and little is known about the light impacts. In order to address this research gap, it is valuable to examine the effects of light and noise on acoustic parameter changes in underwater vocalisations of true seals.
Underwater true seals emit vocalisations mainly in low-frequency ranges (a few hundreds of Hz to a few kHz), which are highly overlapping with the predominant frequencies of ambient noise due to wind and shipping sources (Wenz, 1962; Terhune, 2019; Costa and McHuron, 2022). Ambient noise with high intensity can mask the seal vocalisations and further disturb their behaviours. According to the hypothesis of acoustic adaptation (Morton, 1975), the seals probably use different compensation strategies in noisy conditions to reduce masking, for example, shifting the vocalisation frequency and timing or raising the sound amplitude (the Lombard effect) (Brumm, 2013; Luo et al., 2018). Nevertheless, recent findings about true seals do not support this hypothesis. Both harbour (Phoca vitulina) and bearded (Erignathus barbatus) seals had no or limited ability to adjust amplitude, duration, or frequency parameters of their vocalisations to compensate for ambient noise masking (Matthews et al., 2017; Fournet et al., 2021). Notably, these two studies selected only seal vocalisations with high (50–70 dB) signal-to-noise ratio (SNR) for analyses, and the bearded seal study only used the daytime data. More studies are needed on this topic to reduce bias in vocalisation sample selections.
Many true seals, including harbour, bearded, ribbon (Histriphoca fasciata), Weddell (Leptonychotes weddellii), leopard (Hydrurga leptonyx), and crabeater (Lobodon carcinophagus) seals, were generally more vocally active during nighttime than during daytime (Thomas and DeMaster, 1982; Rouget et al., 2007; Frouin-Mouy et al., 2016; Nikolich et al., 2018; Otsuki et al., 2018, Shabangu and Charif, 2021). Although the precise function of seal underwater vocalisations is currently unknown, they are suspected to be primarily related to foraging, mating, courtship, territorial defence, and other social contacts (Hayes et al., 2004; Charrier et al., 2013). Visual acuity in true seals is quite good underwater, at least at the sea surface, and visual cues play an important role in mediating the seal behaviours in good light conditions (Costa and McHuron, 2022). But during nighttime, when the seals’ sight was limited, they may adjust their vocalisation parameters to improve the probability of successful communication. Furthermore, light and noise can be co-sensed by underwater vocalising seals, and it is therefore curial to study their interaction impacts on seal vocal behaviours. During daytime, seals are not likely to adjust their vocalisation parameters in response to increasing ambient noise because they can supplement sensing with visual cues. But this may be different during nighttime. This pattern is true for some bird and dolphin species (Brumm and Zollinger, 2013; Deconto and Monteiro-Filho, 2016). However, no information is currently available regarding examination of potential interaction effects of light and noise on true seal vocalisations.
Spotted seals are the only wild pinniped species that reproduce in Chinese waters (Rugh et al., 1997; Han et al., 2010; Yang et al., 2023). They are sea-ice obligates and migrate annually to the breeding area in the Liaodong Bay (approximately 38°43′–40°58′N and 119°50′–122°18′E), China, where they reside from late October to early May depending on the presence of sea ice (Won and Yoo, 2004; Han et al., 2010). The abundance of wild spotted seals in Liaodong Bay has decreased from approximately 4,500 individuals in 1990 to <500 individuals in 2020, raising concerns for the conservation status of this species (Li et al., 2017; Wang, 2023). The spotted seals are highly vocal during the breeding season, particularly when they mate and forage under water (Beier and Wartzok, 1979; Yang et al., 2017; Sills and Reichmuth, 2022). The underwater vocal repertoire of the wild spotted seal population in Liaodong Bay includes at least four call types, i.e., drums, growls, knocks, and sweeps (Yang et al., 2017). These acoustic signals create an opportunity to test how spotted seals adjust their underwater vocalisations under different light and noise conditions. This study recorded underwater vocalisations from a group of wild spotted seals in Liaodong Bay during the breeding season. The aim of this study was to examine the potential interaction effects of light and noise on the vocalisation parameters of spotted seals in Liaodong Bay, China.
2 Materials and methods
2.1 Data collection
Underwater sounds were recorded in calm or moderate sea states (Beaufort 0–3) during 7 days (23–24 March 2014, 1–3 April 2016, and 30 March–1 April 2018) at a haul-out site for spotted seals in the Liao River Estuary in the north of Liaodong Bay, China (Figure 1). The chosen recording days are immediately after ice melt and within the breeding season of the spotted seals (Han et al., 2010). On average, 150–200 spotted seals of different genders and ages hauled out on the tidal mudflat were counted on each of the seven recording days. The recording site is located in a remote rural estuary area. No fisheries, tourism, shipping, and other human activities were observed during data collection. The underwater ambient noise sources are primarily involved in the wind changing and distant shipping (>5 km outside the recording site).
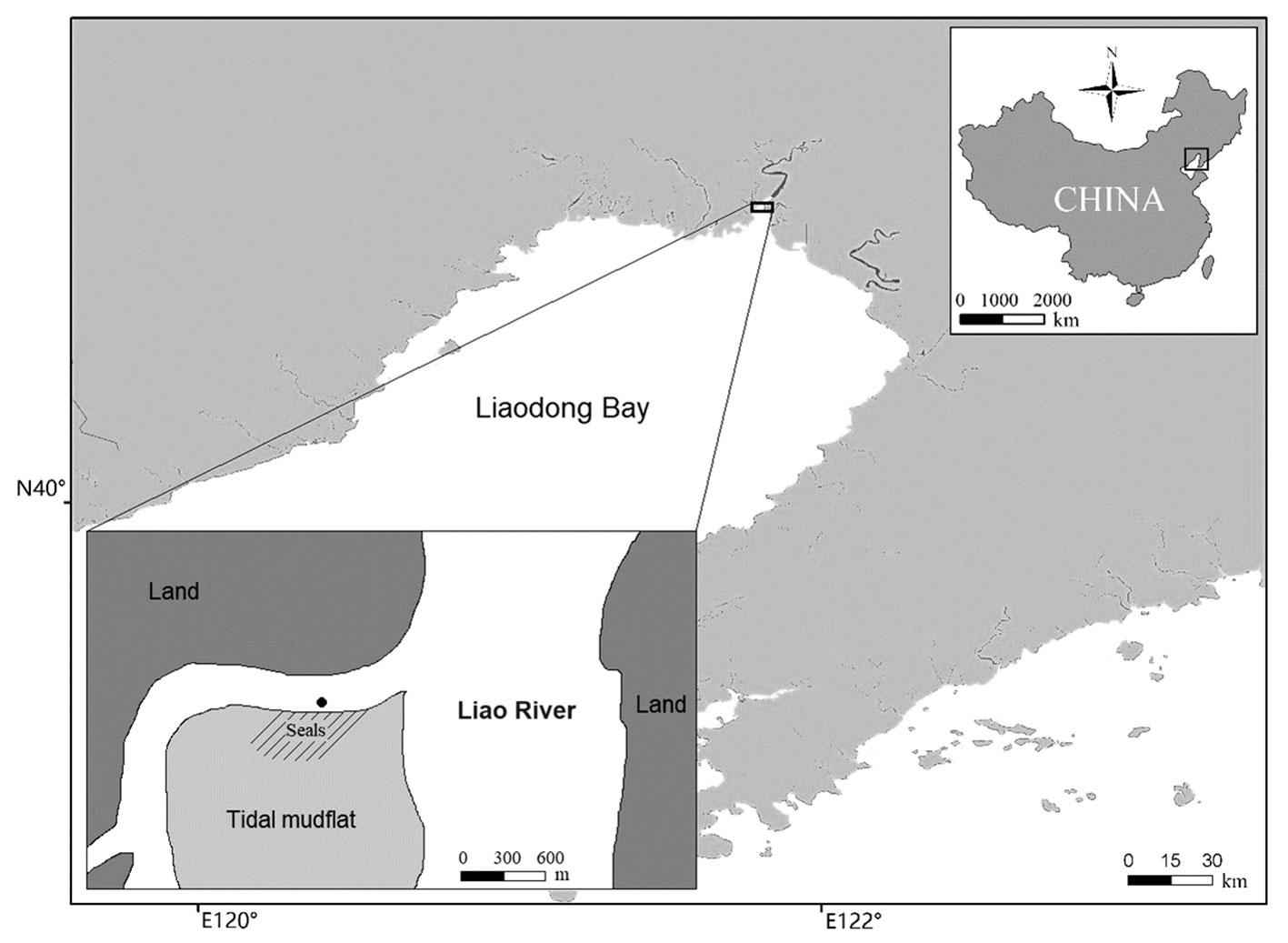
Figure 1 Study area of spotted seal (Phoca largha). Underwater vocalisations were recorded using a DSG-Ocean Acoustic Datalogger in Liao River estuary in Liaodong Bay, NE China. Black dot indicates the position (40°54′N, 121°49′E) of the DSG and shadowed seals’ area indicates the tidal mudflat for seal haul-out.
Sound data were continuously recorded using a calibrated autonomous sound recorder (DSG-Ocean Acoustic Datalogger; Loggerhead Instruments, Sarasota, FL, USA) sampling at 80 kHz with 16-bit resolution. The DSG contains an omnidirectional hydrophone with a sensitivity of -180.6 dB re 1 V/μPa and a flat frequency response ( ± 3 dB) between 20 Hz and 25 kHz. The DSG was moored to a 10-kg 3-fluke anchor with a rope at approximately 5 m above the muddy seafloor in 10–20-m water depths (depending on the tide). A surface buoy was connected to the DSG with rope for retrieval. Seal counts and visual observation were only performed during daytime, while underwater sounds were recorded in both daylight and darkness.
2.2 Data analysis
Sound recordings were first filtered with a 10-pole Butterworth band-pass filter with cutoff frequencies between 50 and 8,000 Hz in Adobe Audition 3.0 (Adobe Systems Inc., San Jose, CA, USA). The low cutoff frequency was at 50 Hz to remove unwanted low-frequency flow noise. The high cutoff frequency was chosen because all of the energy in the spotted seal underwater vocalisations is found below 8,000 Hz (Beier and Wartzok, 1979; Yang et al., 2017; Sills and Reichmuth, 2022). To examine how acoustic parameters change in different light conditions (containing daytime and nighttime in this study), 60 1-min segments of sound recordings were randomly selected from each of the 7 days during daytime (8:00 a.m. to 2:00 p.m.) and during nighttime (10:00 p.m. to 4:00 a.m.). All sound segments were visually examined using spectrograms (Hanning window, FFT size = 1,024 points, frequency overlap = 50%) and aurally reviewed in Adobe Audition to manually identify and extract the spotted seal vocalisations. Four call types (i.e., drum, growl, knock, and sweep) with clearly distinct vocal contours (Yang et al., 2017) and SNR values >6 dB (Matthews, 2017) were extracted in each 1-min segment. Given that the spotted seals produce knock vocalisations in trains (see Figure 4 in Yang et al., 2017), only the highest-amplitude knock in each of the knock trains was used for further analyses. To minimise the data nonindependence, only one vocalisation of each of the four call types was selected in each 1-min segment.
Acoustic parameter analyses were made using custom-written routines in Matlab R2016a (MathWorks, Natick, MA, USA) according to the measurement methods of dolphin click parameters (Yang et al., 2020; Yang et al., 2021). Four acoustic parameters including the centroid frequency (CF), root-mean-square (rms) bandwidth (BW), duration (DU), and received level (RL) were determined for each selected seal vocalisation. The CF (defined as the frequency separating the spectrum into equal energy halves on a linear scale) and BW (defined as the standard deviation of a linear spectrum around CF) parameters were calculated from the power spectrum using equations given in Au and Hastings (2008). It was noted that the peak frequency and -3 dB bandwidth were not considered here because they are unstable parameters in broadband sound analyses (Kinsler et al., 1999). DU was determined as the time interval corresponding to 95% of accumulated energy (Madsen et al., 2004). The RL of each seal vocalisation was calculated as rms sound pressure within the signal duration. Since previous hearing studies showed that true seals are able to interpret and respond to sound signals less than 0.5 s (Reichmuth et al., 2012), rms ambient noise levels (NLs) were calculated for the 1-s period prior to the selected seal vocalisations in accordance with the study of Fournet et al. (2021).
2.3 Statistical analysis
All statistical analyses were performed using R version 4.0 (R Core Team, 2020) and R Studio version 1.2.5042 (R Studio Team, 2019). A series of generalised linear models (GLMs) with Gaussian error structures was fitted using the “glm” function in the R’s standard stats package (Mauro et al., 2020). Specifically, four different models were built with the CF, BW, DU, and RL as response variables. A backward selection process was used, starting off with initial models that contained a three-way interaction among call type (drum, growl, knock, and sweep), light condition (daytime and nighttime), and NL (continuous variable). If a significant three-way interaction was observed, a two-way interaction analysis between light condition and NL for each of the four call types would be made separately. If there was no interaction, a univariate GLM analysis would be carried out for the light condition and NL, respectively. The alpha criterion for statistical testing was set at p-value <0.05.
3 Results
In total, 661 high-SNR (>6 dB) spotted seal underwater vocalisations (including 182 drums, 242 growls, 112 knocks, and 125 sweeps) and the preceding ambient noise recordings during daytime and nighttime were selected and analysed. Table 1 presents the minimum, maximum, median, and 25% and 75% quartiles of the CF, BW, DU, and RL parameters of the seal vocalisations and the NLs.
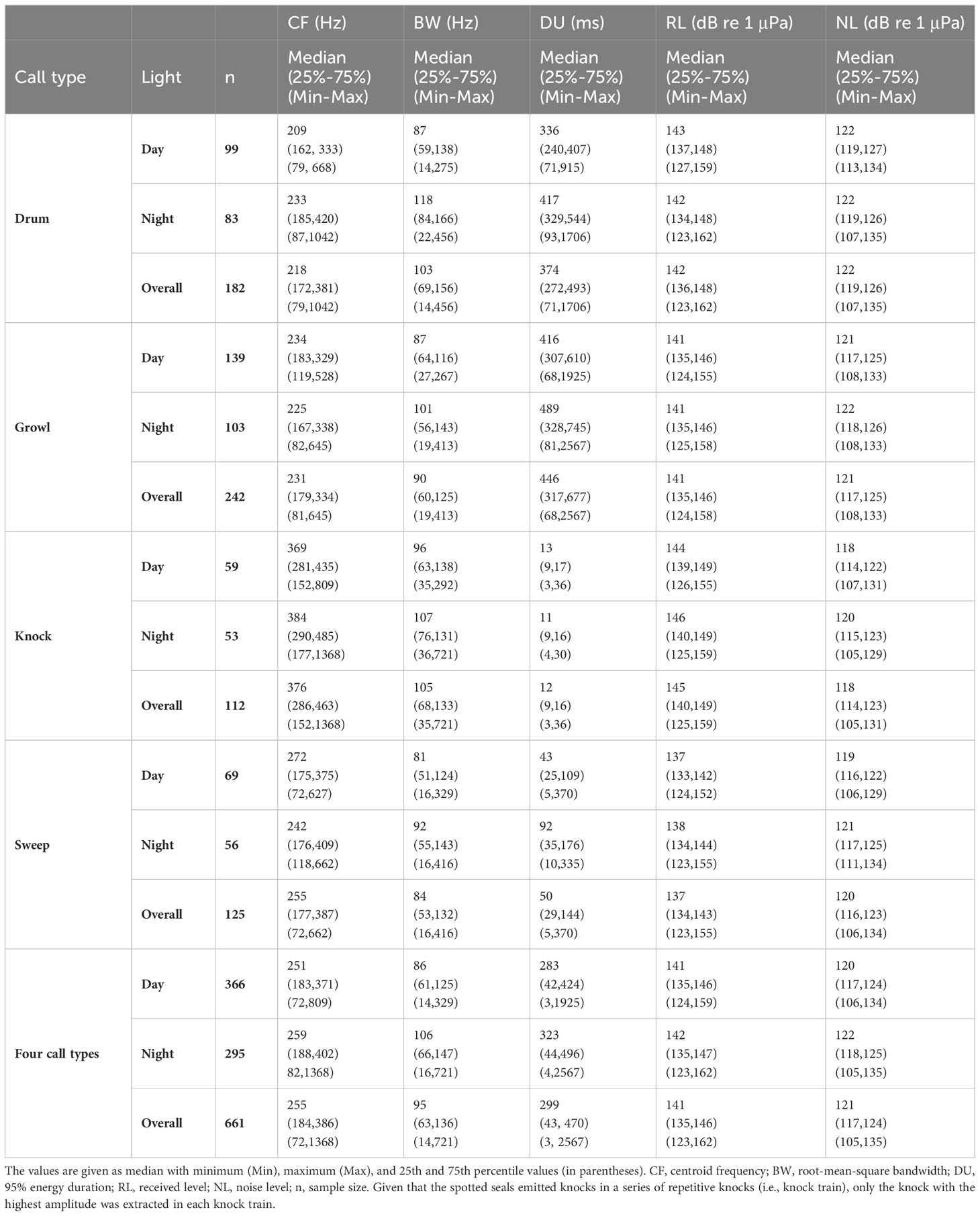
Table 1 Acoustic parameters of four call types (drum, growl, knock, and sweep) of spotted seals (Phoca largha) in Liaodong Bay, China.
Four initial GLMs that contained a three-way interaction between light condition (daytime and nighttime) NL, and call type were run for the four acoustic parameters. A significant interaction was detected only in the CFs (t = -2.91, p < 0.05, Table 2). Then, the dataset was split into four groups based on the call type to examine the interaction effects of light condition and NL on the CFs separately. Specifically, for the knocks, the interaction of light condition and NL significantly affected their CFs (t = -2.07, p < 0.05, Table 3). The knock CFs significantly decreased during nighttime when the noise enhanced (t = -4.01, p < 0.05) and kept stable during daytime (t = -1.31, p > 0.05) (Table 4, Figure 2). For the remaining three call types (i.e., drum, growl, and sweep), no significant interaction effects between light condition and NL were detected (p > 0.05 for all, Table 3). The univariate GLM results showed that there were significant differences for the drum and growl CFs between daytime and nighttime (drum: t = 2.54, p < 0.05; growl: t = -0.27, p < 0.05), but no significant differences between two light conditions were detected for the sweep CFs (t = 0.31, p > 0.05) (Table 3, Figure 3). Furthermore, the CFs were significantly decreased with NLs for both drum (t = -4.27, p < 0.05) and growl (t = 6.41, p < 0.05) vocalisations; however, this was not the case for sweeps (t = -1.09, p > 0.05) (Table 3, Figure 3).
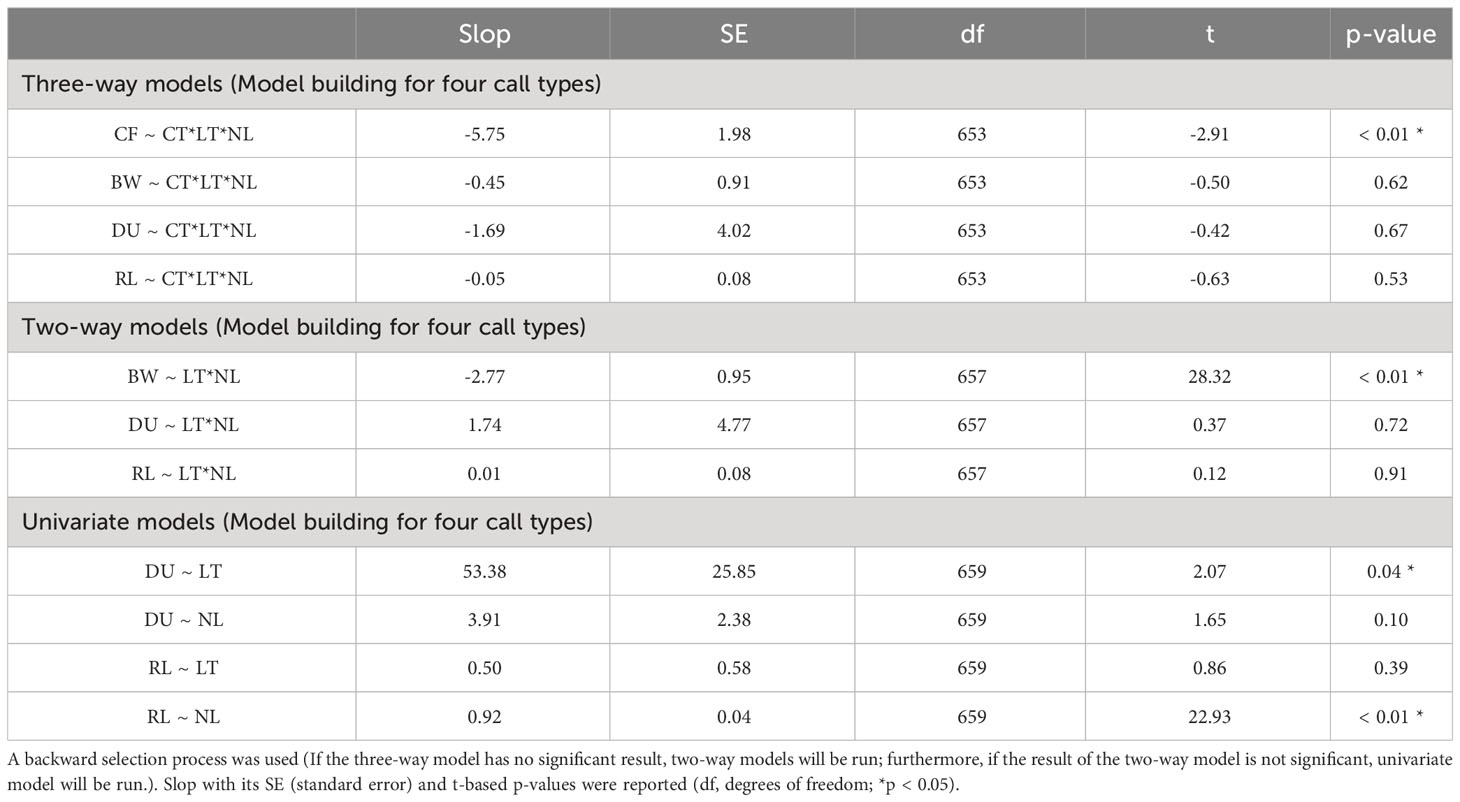
Table 2 Results of the generalised linear models for interaction effects of call type (CT), light (LT), and noise (NL) conditions on the CF (centroid frequency), BW (root-mean-square bandwidth), DU (95% energy duration), and RL (received level) parameters of underwater vocalisations from spotted seals (Phoca largha) in Liaodong Bay, China.
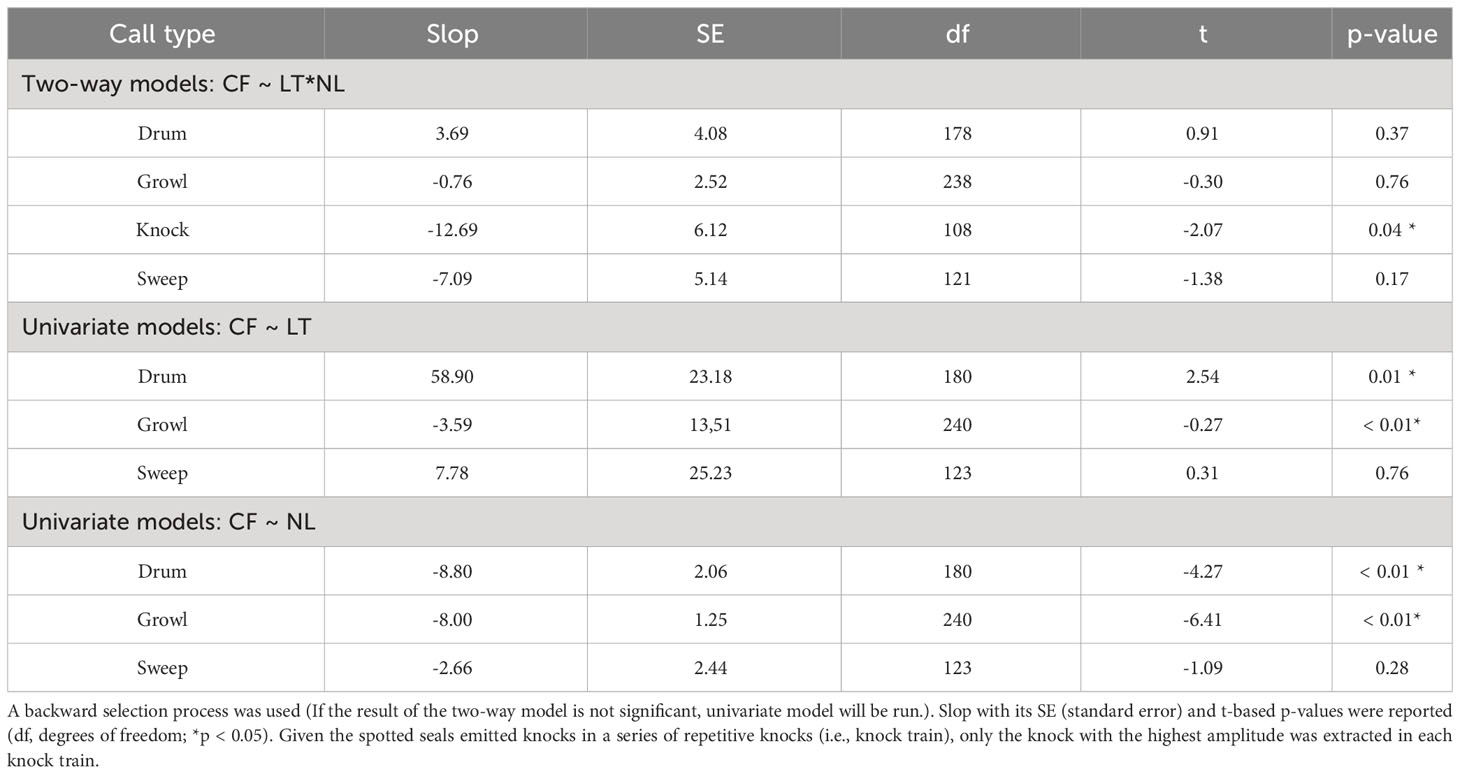
Table 3 Results of the generalised linear models for interaction effects of light (LT) and noise (NL) conditions on the CF (centroid frequency) of the four call types (drum, growl, knock, and sweep) from spotted seals (Phoca largha) in Liaodong Bay, China.
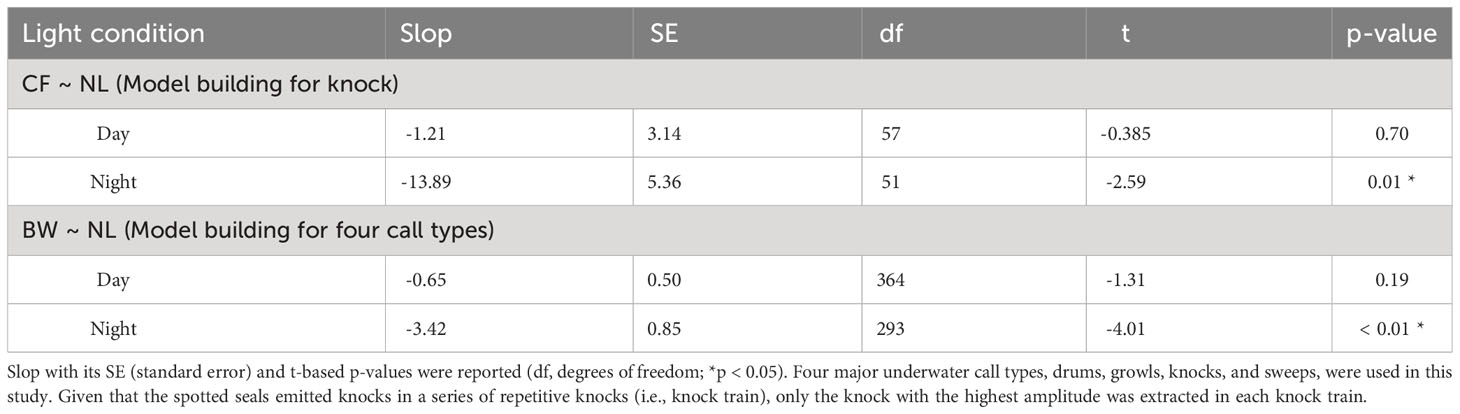
Table 4 Results of the generalised linear models for noise (NL) effects in different light conditions (day and night) on the knock CF (centroid frequency), four call types’ BW (root-mean-square bandwidth) of spotted seals (Phoca largha) in Liaodong Bay, China.
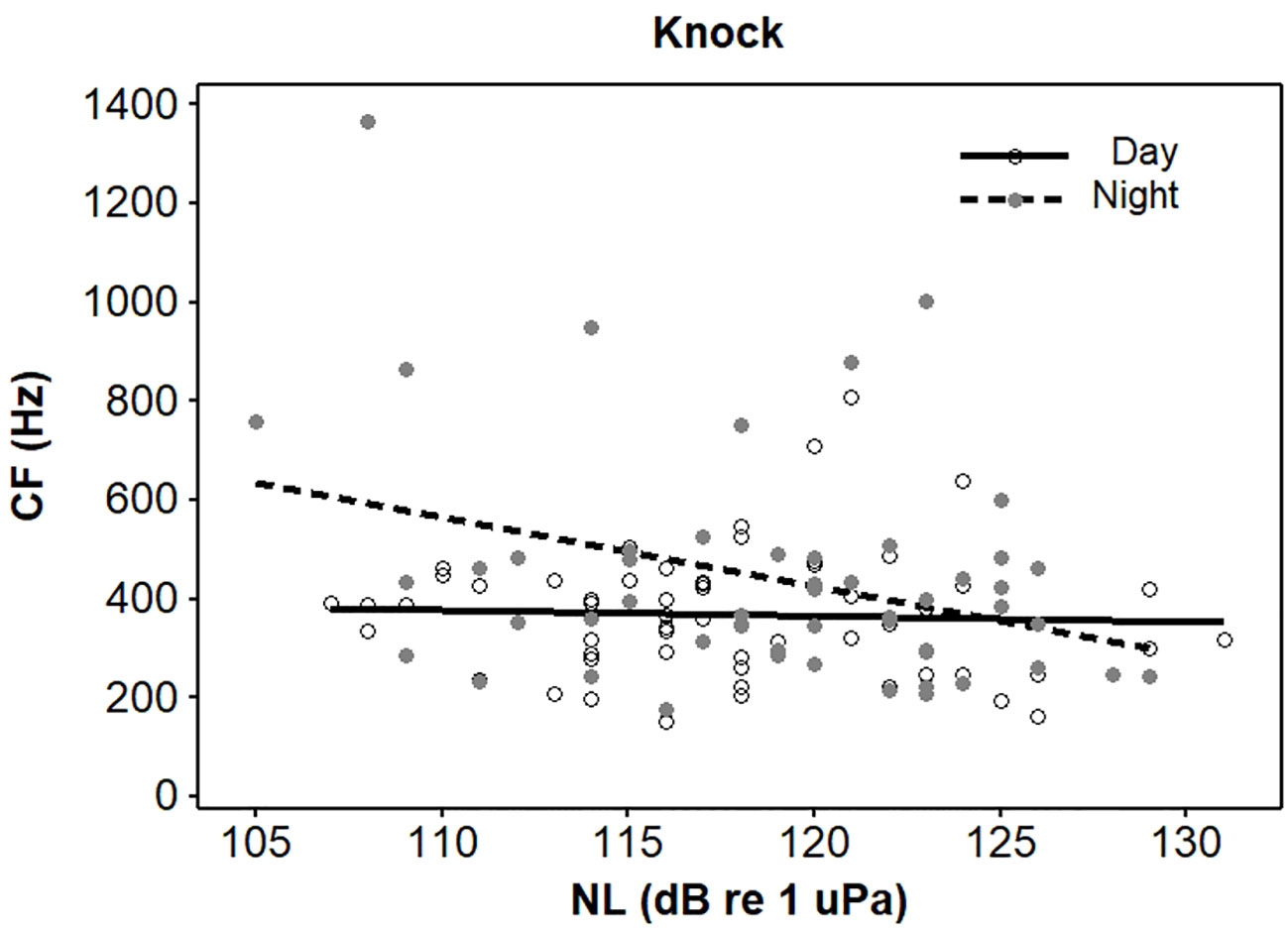
Figure 2 Interaction effect of light (day and night) and noise on centroid frequencies (CFs) of knock vocalisations emitted from spotted seals (Phoca largha) in Liaodong Bay, China.
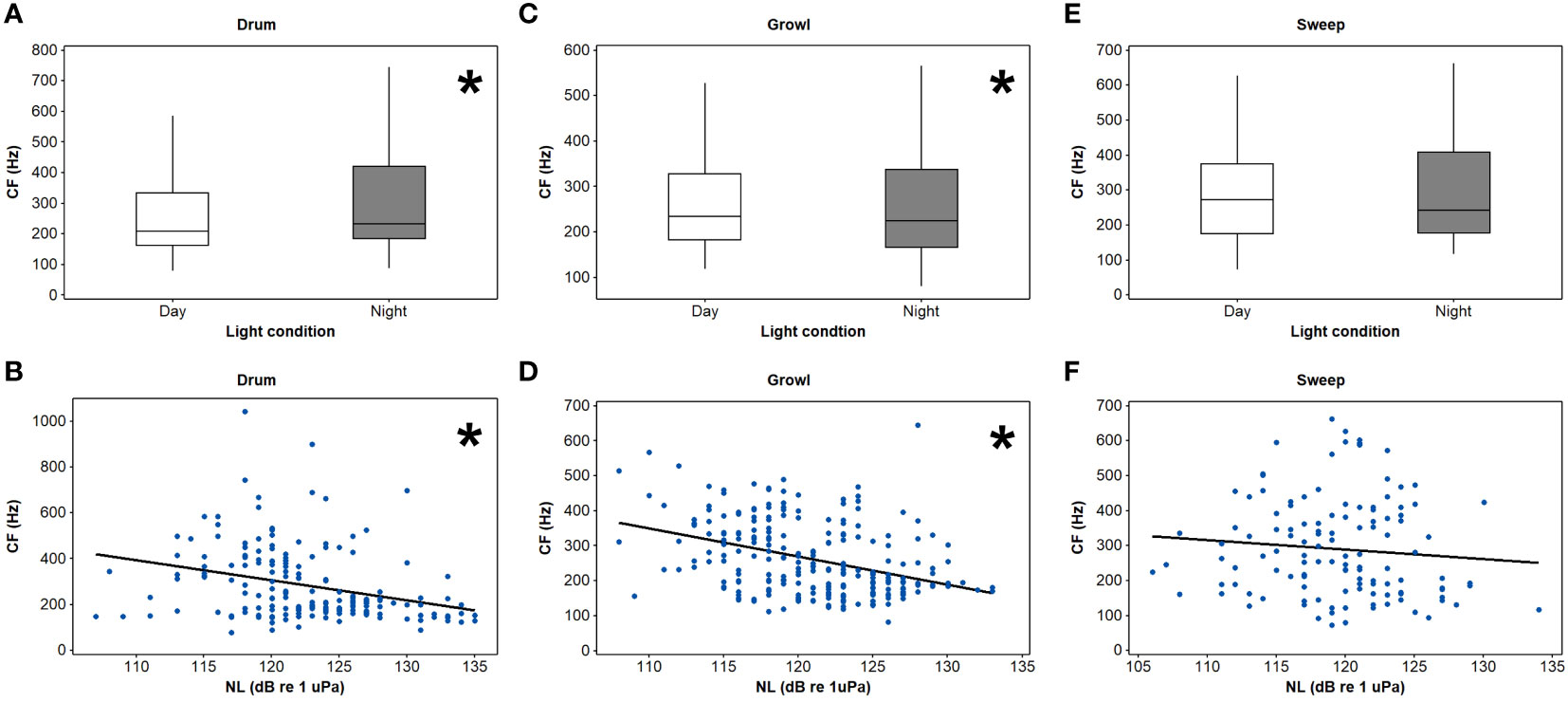
Figure 3 Centroid frequency (CF) differences between day and night and the relationships between CF and noise levels (NLs) for drum (A, B), growl (C, D), and sweep (E, F) vocalisations of spotted seals (Phoca largha) in Liaodong Bay, China. * indicates statistical significance (p < 0.05).
As for the BW, there was a significant two-way interaction between light condition and NL (t = 28.32, p < 0.05; Table 2). During nighttime, the BWs significantly decreased when NLs increased (t = -2.59, p < 0.05), while noise had no significant influence on the BWs during daytime (t = -0.39, p > 0.05) (Table 4, Figure 4).
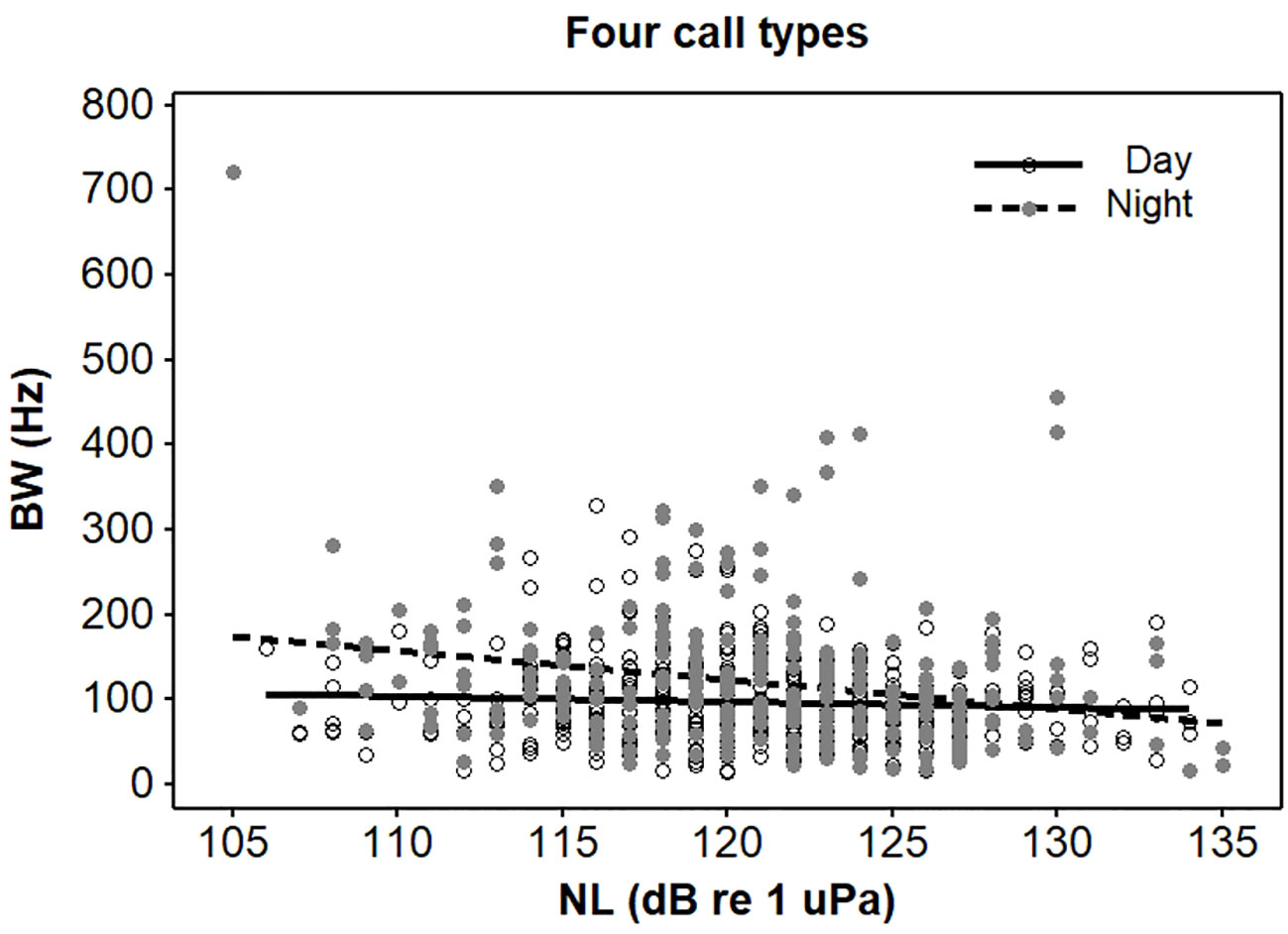
Figure 4 Interaction effect of light (day and night) and noise on root-mean-square bandwidths (BWs) of four call types emitted from spotted seals (Phoca largha) in Liaodong Bay, China.
Neither a significant three-way interaction between light condition, NL, and call type nor a significant two-way interaction between light condition and NL was observed for the DU and RL parameters (p > 0.05 for all, Table 2). For all four call types, the results of univariate GLM analyses showed that the spotted seals produced significantly longer underwater vocalisations during nighttime than daytime (t = 2.07, p < 0.05), and light condition had no significant impact on the RLs (t = 0.86, p > 0.05), while the RLs were significantly increased with the NLs (t = 22.93, p < 0.05), and the noise effect on the DUs was not significant (t = 0.86, p > 0.05) (Table 2, Figure 5).
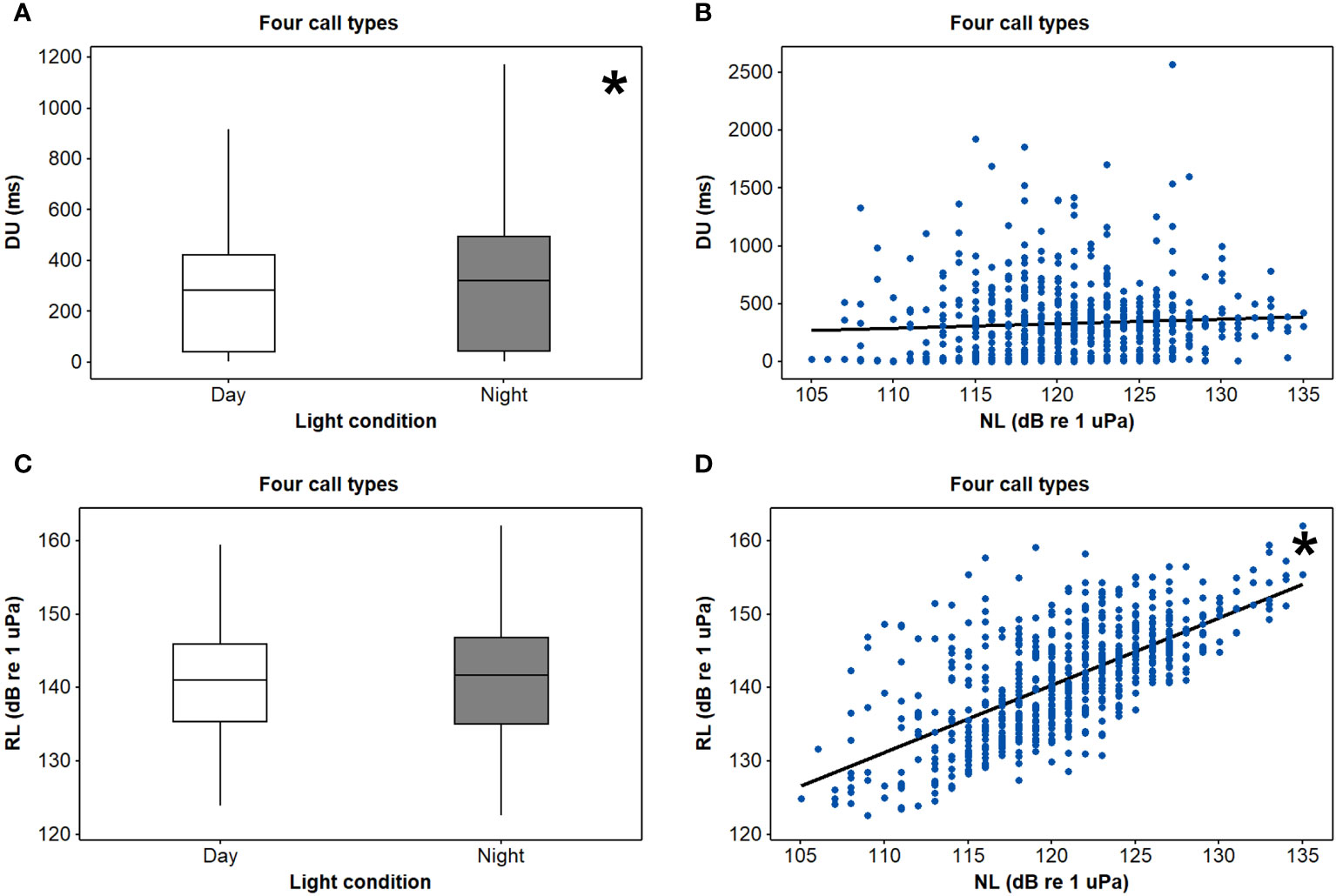
Figure 5 Differences of (A) 95% energy duration (DU) and (C) received level (RL) between day and night and the relationships between (B) DUs or (D) RLs and noise levels (NLs) for four call types of spotted seals (Phoca largha) in Liaodong Bay, China. * indicates statistical significance (p < 0.05).
4 Discussion
Understanding how seals in the wild adjust their acoustic signals in response to changes in multiple environmental factors is a great challenge, since the semiaquatic seals often lived in remote and inaccessible areas and they had complex sensory adaptations and abilities (Costa and McHuron, 2022). The present study is the first attempt to investigate the interaction effects of light and noise on the acoustic parameters of underwater vocalisations recorded using a broadband hydrophone system from the wild spotted seals in Liaodong Bay, China. The results of regression models demonstrated that there were significant interaction effects of light and noise on the knock CFs and all call type BWs, but not on DUs and RLs. Furthermore, regardless of call type, univariate GLM analyses showed that this species had longer vocalisations during nighttime than during daytime and might have the capability to raise the vocalisation intensity in response to the ambient noise that ranged from 105 to 135 dB re 1 μPa.
The present study proved that the knock CFs and BWs of the four call types significantly decreased with the increasing NLs during nighttime but they did not change during daytime (Figures 2, 4). Previous bearded seal study only used the vocalisation data recorded during daytime, and this species had limited ability to adjust its vocalisation frequency and bandwidth parameters in different noise conditions (Fournet et al., 2021). Both knock CF ranges (here, range was defined as the difference between maximum and minimum) and BW ranges of the four call types were much higher during nighttime compared to during daytime (Table 1). This provided enough dynamic ranges for seals to adjust their frequencies at night.
Ambient noise of the present study was mainly a result of fluctuations in wind and distant shipping, and the NLs had no significant difference between day and night for each and all of the four call types (Table 1, Mann–Whitney U tests, p > 0.05 for all). Light difference between day and night would be the primary reason for the frequency adjustments. During nighttime, the seals had relatively poor visibility, leading them to rely more heavily on acoustic cues for communication (Brumm, 2013). Therefore, it was reasonable to speculate that the occurrence of vocal adjustment during nighttime would be beneficial for the seal communication with insufficient visual cues.
Furthermore, in order to convey more information to conspecifics during nighttime, it was highly possible that the seals would exhibit a broader frequency range, longer duration, and higher sound intensity. The results of univariate GLM analyses showed that there was no significant linear relationship between the sound intensity of spotted seal vocalisations and light conditions (Figure 5C), which made the seals receive vocalisations with relatively stable SNRs. On the other hand, the present data not only proved that all call types had longer durations during nighttime (Figure 5A) but also showed that most BWs were higher during nighttime under the same noise condition regardless of call type (Figure 4). The duration and bandwidth results are in good consistency with numerous animal species, e.g., fish (Feng and Bass, 2016), bird (McCracken and Sheldon, 1997), bat (McGowan and Kloepper, 2020), and dolphins (Bittencourt et al., 2016).
It is important to highlight that the acoustic parameters of a seal’s vocalisations are highly influenced by its underwater behaviour (Mizuguchi et al., 2016). Sound recordings were carried out at the end of the sea ice season (in late March or early April), when adult spotted seals were mainly in their moulting stage and hauled-out more frequently during the warm period (Burns, 2009; Han et al., 2010). On average, the air temperature difference between day and night was approximately 20°C–25°C during the seal sound recordings. Spotted seals might therefore spend more time on the mudflat haul-outs during the daytime to reduce heat loss associated with the moulting behaviour (Paterson et al., 2012). This suggested that more vocalising spotted seals could be recorded during nighttime than during daytime. Some harbour seals (the closest sister species to spotted seals), especially females, spend more time in foraging underwater during nighttime than other time of day; males spend more time in the water to increase their chances of encountering females (Matthews et al., 2017). This may increase the probability of recording more underwater vocalisations associated with seal foraging and mating activities during nighttime. If this is true for the spotted seals, these behavioural variances could be another reason to explain the acoustic parameter changes between day and night.
The underwater vocalisations of spotted seals covering a range from several tens of Hz to 1,000 or more Hz could be completely or partially masked by loud ambient noise with a similar range. Surprisingly, the spotted seals decreased the CF and BW parameters of their vocalisations with increasing NLs (Figures 2–4). Such frequency-shift pattern was very different from toothed whales, which generally increased the frequency parameters of their communication whistles as noise increased (Papale et al., 2015). For example, to avoid masking, the common bottlenose dolphins (Tursiops truncatus) produced relatively high-frequency (4–18 kHz) whistles through phonic lips and the melon, which had very few or no frequency overlap with the ambient noise (Fouda et al., 2018; van Ginkel et al., 2018). Unlike the whistles, the underwater vocalisations produced by the spotted seals var expansion of the tracheal membrane were mainly approximately hundreds of frequencies (Table 1), which highly overlapped the frequency range of ambient noise (Martin et al., 2017). Increasing the vocalisation frequencies would have few contributions to avoid noise masking for the seal species. Alternatively, the seals adjusted their vocalisations by decreasing the frequency and bandwidth parameters when noise increasing. The seal vocalisations with lower frequency propagate better under water and induce more closely spaced harmonics, which are often easily identified by conspecifics (Au and Hastings, 2008). This frequency finding was in line with the study of Torres Borda et al. (2021), which showed that captive harbour seal pups produced in-air vocalisations with lower fundamental frequency in response to noise increasing.
The seal vocalisation frequencies tend to be decreased when the animal moves away from the hydrophone (a phenomenon known as the Doppler effect) (Siderius and Porter, 2006). It is assumed that the underwater distribution of vocalising seals around the hydrophone is random; thus, there is no reason to deduce that the decrease in seal frequency is a result of the seals moving away from the hydrophone. Consequently, the Doppler effect would be unsuitable to explain why the spotted seals decreased their vocalisation frequencies when the noise was increasing.
According to the RL analyses, the spotted seals in Liaodong Bay, China, exhibited the Lombard effect in that they increased the intensity of their vocalisations in increased ambient noise (Figure 5D). Spotted seal vocalisations had a median RL of 141 dB re 1 μPa (regardless of the call type), and the RLs increased by 0.92 dB per dB increase in NLs (univariate GLM, slop = 0.92, p < 0.05; Table 2). The difference between the highest and lowest NLs measured in this study was approximately 20 dB. The predicted change in RLs would be approximately 18 dB, which was larger than the RL interquartile range (11 dB; Table 1). Taken together, this indicated that the adjustment of vocalisation RLs in response to the ambient noise changes might mainly result from the seals’ Lombard response rather than the natural variance of vocalisation intensity.
An approximately 1:1 correlation between the increase in RLs and increase in NLs was found in this study. This finding was consistent with my recent study on spotted seal growls (Yang et al., 2022) and some previous studies on cetacean species, e.g., humpback (Megaptera novaeangliae) (Dunlop et al., 2014), killer (Orcinus orca) (Holt et al., 2011), and belugas (Delphinapterus leucas) (Scheifele et al., 2005) whales. However, Matthews et al. (2020) showed that the source level of harbour seal roars increased by only 0.16 dB for every 1-dB increase in ambient noise, and this adjustment did not sufficiently compensate for increasing noise. Bearded seals did increase their vocalisation intensity until ambient NL reached a behavioural threshold, above which the seal would stop increasing the vocalisation source levels (Fournet et al., 2021).
Previous studies on frogs and bats had revealed that it was more likely to detect the Lombard effect when using vocalisations with lower SNRs (for a review, see Luo et al., 2018). The harbour and bearded seal studies selected the vocalisations with very high SNR values ranging from 50 to 70 dB, which is much higher than the SNRs used in this study (approximately 20 dB on average, estimated in Table 1). This might be the reason why the Lombard effect was detected in spotted seals here. It would be of interest of future work to investigate the SNR impacts when estimating the presence of the Lombard effect in different seal species.
An additional finding of this study was that the noise had no significant effect on the durations of spotted seal vocalisations (Figure 5B). This was also true for other phocids, including harbour (Matthews et al., 2020), Weddell (Terhune, 2016), and bearded (Fournet et al., 2021) seals. The maximum durations of seals’ underwater vocalisations were generally brief (less than a few seconds) probably due to the limitation of their physical ability, and therefore, the seals could not lengthen their vocalisations further in noisy conditions.
Although the sample size in the present study is relatively small and limited to a single recording site, the results clearly indicated that there were significant interaction effects of light and noise on the two frequency-related parameters (i.e., CF and BW) of spotted seal vocalisations and that their vocalisation DU and RL parameters were influenced by light and noise, respectively. Further research with an appropriate sampling design and a large sample size is needed to confirm the interaction effects of light and noise without having statistical significance on acoustical parameters, such as the DU and RL parameters here.
In summary, the present study highlights that the spotted seals, a poorly studied species in Chinese waters, have some capabilities to adjust their vocalisations in response to changes of light and noise conditions. An increased knowledge regarding the seal vocalisation adjustments was helpful to understand how seals interact with their habitats (Yang et al., 2023). Results from this study could therefore be useful when developing evidence-based management and conservation strategies to minimise disturbance to spotted seals from anthropogenic activities in both Liaodong Bay and elsewhere.
Data availability statement
The raw data supporting the conclusions of this article will be made available by the authors, without undue reservation.
Ethics statement
The animal study was approved by Ministry of Agriculture and Rural Affairs of the People’s Republic of China. The study was conducted in accordance with the local legislation and institutional requirements.
Author contributions
LY: Conceptualization, Data curation, Formal Analysis, Funding acquisition, Investigation, Methodology, Software, Validation, Visualization, Writing – original draft, Writing – review & editing.
Funding
The author(s) declare financial support was received for the research, authorship, and/or publication of this article. This work was supported by the National Nature Science Foundation of China (42276141), Guangdong National Nature Science Foundation (2022A1515010587), and Shantou University STU Scientific Research Initiation Grant (NTF21033).
Acknowledgments
I would like to thank the staff from Liaoning Ocean and Fisheries Science Research Institute (China) for field assistance. I also thank the handling editor and two reviewers for their valuable comments on the article.
Conflict of interest
The author declares that the research was conducted in the absence of any commercial or financial relationships that could be construed as a potential conflict of interest.
Publisher’s note
All claims expressed in this article are solely those of the authors and do not necessarily represent those of their affiliated organizations, or those of the publisher, the editors and the reviewers. Any product that may be evaluated in this article, or claim that may be made by its manufacturer, is not guaranteed or endorsed by the publisher.
Footnotes
- ^ This article was formatted in British English.
References
Beier J. C., Wartzok D. (1979). Mating behaviour of captive spotted seals (Phoca largha). Anim. Behav. 27, 772–781. doi: 10.1016/0003-3472(79)90013-7
Bittencourt L., Lima I. M. S., Andrade L. G., Carvalho R. R., Bisi T. L., Lailson-Brito J., et al. (2016). Underwater noise in an impacted environment can affect Guiana dolphin communication. Mar. pollut. Bull. 114, 1130–1134. doi: 10.1016/j.marpolbul.2016.10.037
Brumm H., Zollinger S. A. (2013). “Avian vocal production in noise,” in Animal communication and noise. (Berlin, Germany: Springer).
Burns J. J. (2009). Harbor Seal and Spotted Seal: Phoca vitulina and P. largha InandEncyclopedia of Marine Mammals. 2nd ed. Eds. Perrin W. F., Würsig B., Thewissen J. G. M. (London: Academic Press).
Charrier I., Mathevon N., Aubin T. (2013). Bearded seal males perceive geographic variation in their trills. Behav. Ecol. Sociobiol. 67, 1679–1689. doi: 10.1007/s00265-013-1578-6
Costa D. P., McHuron E. A. (2022). Ethology and behavioral ecology of phocids (Cham, Switzerland: Springer).
Deconto L. S., Monteiro-Filho E. A.. (2016). Day and night sounds of the Guiana dolphin, Sotalia guianensis (Cetacea: Delphinidae) in southeastern Brazil. Acta Ethol. 19, 61–68. doi: 10.1007/s10211-015-0223-y
Dunlop R. A., Cato D. H., Noad M. J. (2014). Evidence of a Lombard response in migrating humpback whales (Megaptera novaeangliae). J. Acoust. Soc Am. 136, 430–437. doi: 10.1121/1.4883598
Feng N. Y., Bass A. H. (2016). “Singing” fish rely on circadian rhythm and melatonin for the timing of nocturnal courtship vocalization. Curr. Biol. 26, 2681–2689. doi: 10.1016/j.cub.2016.07.079
Foote A. D., Osborne R. W., Hoelzel A. R. (2004). Whale-call response to masking boat noise. Nature 428, 910–910. doi: 10.1038/428910a
Fouda L., Wingfield J. E., Fandel A. D., Garrod A., Hodge K. B., Rice A. N., et al. (2018). Dolphins simplify their vocal calls in response to increased ambient noise. Biol. Lett. 14, 20180484. doi: 10.1098/rsbl.2018.0484
Fournet M. E. H., Silvestri M., Clark C. W., Klinck H., Rice A. N. (2021). Limited vocal compensation for elevated ambient noise in bearded seals: implications for an industrializing Arctic Ocean. ProC.R. Soc Biol. Sci. Ser. B 288, 20202712. doi: 10.1098/rspb.2020.2712
Frouin-Mouy H., Mouy X., Martin B., Hannay D. (2016). Underwater acoustic behavior of bearded seals (Erignathus barbatus) in the northeastern Chukchi Sea 2007–2010. Mar. Mammal Sci. 32, 141–160. doi: 10.1111/mms.12246
Han J., Sun F., Gao X., He C., Wang P., Ma Z., et al. (2010). Low microsatellite variation in spotted seal (Phoca largha) shows a decrease in population size in the Liaodong Gulf colony. Ann. Zool. Fenn. 47, 15–27. doi: 10.5735/086.047.0102
Hayes S. A., Kumar A., Costa D. P., Mellinger D. K., Harvey J. T., Southall B. L., et al. (2004). Evaluating the function of the male harbour seal, Phoca vitulina, roar through playback experiments. Anim. Behav. 67, 1133–1139. doi: 10.1016/j.anbehav.2003.06.019
Holt M. M., Noren D. P., Emmons C. K. (2011). Effects of noise levels and call types on the source levels of killer whale calls. J. Acoust. Soc Am. 130, 3100–3106. doi: 10.1121/1.3641446
Kinsler L. E., Frey A. R., Coppens A. B., Sanders J. V. (1999). Fundamentals of Acoustics (New York: John Wiley and Sons).
Li H., Man W., Li X., Ren C., Wang Z., Li L., et al. (2017). Remote sensing investigation of anthropogenic land cover expansion in the low-elevation coastal zone of Liaoning Province, China. Ocean Coast. Manag 148, 245–259. doi: 10.1016/j.ocecoaman.2017.08.007
Luo J., Hage S. R., Moss C. F. (2018). The Lombard effect: From acoustics to neural mechanisms. Trends. Neurosci. 41, 938–949. doi: 10.1016/j.tins.2018.07.011
Madsen P. T., Kerr I., Payne R. (2004). Source parameter estimates of echolocation clicks from wild pygmy killer whales (Feresa attenuata)(L). J. Acoust. Soc Am. 116, 1909–1912. doi: 10.1121/1.1788726
Martin K., Tucker M. A., Rogers T. L. (2017). Does size matter? Examining the drivers of mammalian vocalizations. Evolution 71, 249–260. doi: 10.1111/evo.13128
Matthews L. P. (2017). Harbor Seal (Phoca vitulina) Reproductive Advertisement Behavior And The Effects Of Vessel Noise (Syracuse, US: PhD dissertation, Syracuse University).
Matthews L. P., Fournet M. E. H., Gabriele C., Klinck H., Parks S. E. (2020). Acoustically advertising male harbour seals in southeast Alaska do not make biologically relevant acoustic adjustments in the presence of vessel noise. Biol. Lett. 16, 20190795. doi: 10.1098/rsbl.2019.0795
Matthews L. P., Gabriele C. M., Parks S. E. (2017). The role of season, tide, and diel period in the presence of harbor seal (Phoca vitulina) breeding vocalizations in Glacier Bay National Park and Preserve, Alaska. Aquat. Mamm. 43, 537–546. doi: 10.1578/AM.43.5.2017.537
Mauro M., Pérez-Arjona I., Perez E. J. B., Ceraulo M., Bou-Cabo M., Benson T., et al. (2020). The effect of low frequency noise on the behaviour of juvenile Sparus aurata. J. Acoust. Soc Am. 147, 3795–3807. doi: 10.1121/10.0001255
McCracken K. G., Sheldon F. H. (1997). Avian vocalizations and phylogenetic signal. Proc. Natl. Acad. Sci. 94, 3833–3836. doi: 10.1073/pnas.94.8.3833
McGowan K. A., Kloepper L. N. (2020). Different as night and day: wild bats modify echolocation in complex environments when visual cues are present. Anim. Behav. 168, 1–6. doi: 10.1016/j.anbehav.2020.07.025
Mizuguchi D., Tsunokawa M., Kawamoto M., Kohshima S. (2016). Underwater vocalizations and associated behavior in captive ringed seals (Pusa hispida). Polar Biol. 39, 659–669. doi: 10.1007/s00300-015-1821-x
Morton E. S. (1975). Ecological sources of selection on avian sounds. Am. Nat. 109, 17–34. doi: 10.1086/282971
Nikolich K., Frouin-Mouy H., Acevedo-Gutiérrez A. (2018). Clear diel patterns in breeding calls of harbor seals (Phoca vitulina) at Hornby Island, British Columbia. Canada. Can. J. Zool. 96, 1236–1243. doi: 10.1139/cjz-2018-0018
Ogawa M., Kimura S. S. (2023). Variations in echolocation click characteristics of finless porpoise in response to day/night and absence/presence of vessel noise. PloS One 18, e0288513. doi: 10.1371/journal.pone.0288513
Otsuki M., Akamatsu T., Nobetsu T., Mizuguchi D., Mitani Y. (2018). Diel changes in ribbon seal Histriophoca fasciata vocalizations during sea ice presence in the Nemuro Strait, Sea of Okhotsk. Polar Biol. 41, 451–456. doi: 10.1007/s00300-017-2203-3
Papale E., Gamba M., Perez-Gil M., Martin V. M., Giacoma C. (2015). Dolphins adjust species-specific frequency parameters to compensate for increasing background noise. PloS One 10, e0121711. doi: 10.1371/journal.pone.0121711
Paterson W., Sparling C., Thompson D., Pomeroy P., Currie J., McCafferty D. (2012). Seals like it hot: Changes in surface temperature of harbour seals (Phoca vitulina) from late pregnancy to moult. J. Therm. Biol. 37, 454–461. doi: 10.1016/j.jtherbio.2012.03.004
R Core Team. (2020) R: a language and environment for statistical computing [computer software]. Vienna: R Foundation for Statistical Computing. 2020
R Studio Team. (2019) RStudio: Integrated Development for R. Boston: RStudio, Inc.; 2019. http://www.rstudio.com/.
Reichmuth C., Ghoul A., Southall B. L. (2012). Temporal processing of low-frequency sounds by seals (L) a. J. Acoust. Soc Am. 132, 2147–2150. doi: 10.1121/1.4746030
Rouget P., Terhune J. M., Burton H. (2007). Weddell seal underwater calling rates during the winter and spring near Mawson Station, Antarctica. Mar. Mammal Sci. 23, 508–523. doi: 10.1111/j.1748-7692.2007.00129.x
Rugh D. J., Sheldon K. E., Withrow D. E. (1997). Spotted seals, Phoca largha, in Alaska. Mar. Fish Rev. 59, 1–18.
Scheifele P. M., Andrew S., Cooper R., Darre M., Musiek F., Max L. (2005). Indication of a Lombard vocal response in the St. Lawrence River beluga. J. Acoust. Soc Am. 117, 1486–1492. doi: 10.1121/1.1835508
Shabangu F. W., Charif R. A. (2021). Short moan call reveals seasonal occurrence and diel-calling pattern of crabeater seals in the Weddell Sea, Antarctica. Bioacoustics 30, 543–563. doi: 10.1080/09524622.2020.1819877
Siderius M., Porter M. B. (2006). Modeling techniques for marine-mammal risk assessment. IEEE J. Oceanic Eng. 31, 49–60. doi: 10.1109/JOE.2006.872211
Sills J. M., Reichmuth C. (2022). Vocal behavior in spotted seals (Phoca largha) and implications for passive acoustic monitoring. Front. Remote Sens. 3, 862435. doi: 10.3389/frsen.2022.862435
Soldevilla M. S., Wiggins S. M., Hildebrand J. A. (2010). Spatio-temporal comparison of Pacific white-sided dolphin echolocation click types. Aquat. Biol. 9, 49–62. doi: 10.3354/ab00224
Terhune J. M. (2016). Weddell seals do not lengthen calls in response to conspecific masking. Bioacoustics 25, 75–88. doi: 10.1080/09524622.2015.1089791
Terhune J. M. (2019). The underwater vocal complexity of seals (Phocidae) is not related to their phylogeny. Can. J. Zool. 97, 232–240. doi: 10.1139/cjz-2018-0190
Thomas J., DeMaster D. (1982). An acoustic technique for determining diurnal activities in leopard (Hydrurga leptonyx) and crabeater (Lobodon carcinophagus) seal. Can. J. Zool. 60, 2028–2031. doi: 10.1139/z82-260
Torres Borda L., Jadoul Y., Rasilo H., Salazar Casals A., Ravignani A. J. (2021). Vocal plasticity in harbour seal pups. Philos. T. R. Soc B, 376, 20200456. doi: 10.1098/rstb.2020.0456
van Ginkel C., Becker D. M., Gowans S., Simard P. (2018). Whistling in a noisy ocean: bottlenose dolphins adjust whistle frequencies in response to real-time ambient noise levels. Bioacoustics 27, 391–405. doi: 10.1080/09524622.2017.1359670
Wang Z. (2023). Enforce China’s plan to protect spotted seals. Science 381, 1163–1163. doi: 10.1126/science.adj9396
Wenz G. M. (1962). Acoustic ambient noise in the ocean: Spectra and sources. J. Acoust. Soc Am. 34 (12), 1936–1956. doi: 10.1121/1.1909155
Won C., Yoo B. H. (2004). Abundance, seasonal haul-out patterns and conservation of spotted seals Phoca largha along the coast of Bak-ryoung Island, South Korea. Oryx 38, 109–112. doi: 10.1017/S0030605304000171
Yang L., Sharpe M., Temple A. J., Berggren P. (2021). Characterization and comparison of echolocation clicks of white-beaked dolphins (Lagenorhynchus albirostris) off the Northumberland coast, UK. J. Acoust. Soc Am. 149, 1498–1506. doi: 10.1121/10.0003560
Yang L., Sharpe M., Temple A. J., Jiddawi N., Xu X., Berggren P. (2020). Description and classification of echolocation clicks of Indian Ocean humpback (Sousa plumbea) and Indo-Pacific bottlenose (Tursiops aduncus) dolphins from Menai Bay, Zanzibar, East Africa. PloS One 15, e0230319. doi: 10.1371/journal.pone.0230319
Yang L., Xu X., Berggren P. (2022). Spotted seal Phoca largha underwater vocalisations in relation to ambient noise. Mar. Ecol. Prog. Ser. 683, 209–220. doi: 10.3354/meps13951
Yang L., Xu X., Zhang P., Han J., Li B., Berggren P. (2017). Classification of underwater vocalizations of wild spotted seals (Phoca largha) in Liaodong Bay, China. J. Acoust. Soc Am. 141, 2256–2262. doi: 10.1121/1.4979056
Keywords: acoustic communication, adaptive changes, vocalisation, marine mammal, pinnipeds
Citation: Yang L (2024) The interaction effects of light and noise on the acoustic parameters of underwater vocalisations of wild spotted seals (Phoca largha) in Liaodong Bay, China1. Front. Mar. Sci. 10:1342134. doi: 10.3389/fmars.2023.1342134
Received: 21 November 2023; Accepted: 15 December 2023;
Published: 10 January 2024.
Edited by:
Xuelei Zhang, Ministry of Natural Resources, ChinaReviewed by:
Songzuo Liu, Harbin Engineering University, ChinaZhaolong Cheng, Chinese Academy of Fishery Sciences (CAFS), China
Copyright © 2024 Yang. This is an open-access article distributed under the terms of the Creative Commons Attribution License (CC BY). The use, distribution or reproduction in other forums is permitted, provided the original author(s) and the copyright owner(s) are credited and that the original publication in this journal is cited, in accordance with accepted academic practice. No use, distribution or reproduction is permitted which does not comply with these terms.
*Correspondence: Liangliang Yang, bGx5YW5nQHN0dS5lZHUuY24=