- 1Department of Estuarine and Delta Systems, Netherlands Institute for Sea Research and Utrecht University, Yerseke, Netherlands
- 2Department of Ecology, Environment and Plant Sciences, Stockholm University, Stockholm, Sweden
- 3The Centre for Environment, Fisheries and Aquaculture Science, Lowestoft, United Kingdom
- 4Wageningen Marine Research, Wageningen University & Research, IJmuiden, Netherlands
- 5Ghent University, Department of Biology, Marine Biology Research Group, Ghent, Belgium
- 6Unité mixte de recherche Dynamique et durabilité des écosystèmes (DECOD), Institut Français de Recherche pour l'Exploitation de la Mer (IFREMER), Laboratoire Ecologie et Modèles pour l'Halieutique (EMH), Nantes, France
- 7Hafok AB, Stenhamra, Sweden
- 8Hellenic Centre for Marine Research, Institute of Marine Biological Resources and Inland Waters, Heraklion, Crete, Greece
- 9The Lyell Centre, Heriot-Watt University, Edinburgh, United Kingdom
Introduction: The importance of the response-effect trait dichotomy in marine benthic ecology has garnered recent attention. Response traits, characterising species responses to environmental variations, have been a dominant focus in the development of ecological indicators for ecosystem health assessment. In contrast, effect traits, expressing effects of organism activities on the ecosystem, still do not benefit from an equal interest in spite of the complementary facet that they provide to complete our understanding of functional diversity and ecosystem vulnerability. In this study, we explore the consequences of disturbance by bottom trawl fisheries on benthic effect trait composition.
Methods: To this end, we used different contexts of environmental and trawling conditions from thirteen case studies in European waters and apply the same analytical procedure to derive a gradient that solely account for trawling-induced disturbance (Partial RLQ analysis).
Results: Bottom trawling was found to be a selective force of benthic effect trait composition in a majority of case studies. In general, tube-dwelling species were more typical of low trawling frequencies, whereas deep burrowing species were more resistant at high trawling frequencies. Although we report significantly deleterious effects of trawling on benthic ecosystem functions, the effect trait pattern along the gradient was never related to life span, a key response trait generally assumed to express recoverability following disturbance. Furthermore, we show that trends in species multi-functionality and community functional diversity can be negative or positive along the trawling intensity gradient.
Discussion: We discuss the relevance of these results in light of recent developments in the framework of response and effect trait dichotomy, and provide guidelines of trait data analysis in the context of trawl fisheries impact on the sea floor. Our findings emphasize the importance of fundamental concepts from functional ecology in this context and represent a first step toward an assessment of trawling effect more oriented on benthos-mediated biogeochemical processes.
1 Introduction
In sedimentary environments, benthic invertebrates provide important ecosystem functions such as bioturbation (Kristensen et al., 2012) and habitat creation (Auster and Langton, 1999; Thrush and Dayton, 2002). Several studies focusing on specific sea floor functions have directly or potentially evidenced that benthic species loss can be critical to ecosystem functioning (Solan et al., 2004; Olsgard et al., 2008; Godbold and Solan, 2013; Woodin et al., 2016; Garcia et al., 2021). Bottom trawl fisheries have long been regarded as the major threat to benthic ecosystem functions since there is no equivalent human physical disturbance on the sea floor in terms of spatial and temporal extents (Jennings and Kaiser, 1998; Thrush and Dayton, 2002; Crowder et al., 2008). While there is ample evidence of fisheries impacts on organism themselves (Jones, 1992; Kaiser et al., 2002; Sciberras et al., 2018), more comprehensive views on ecosystem-wide damages have been advocated so that the burden of proof becomes a stronger management incentive (Dayton, 1998; Agardy, 2000). Indeed, mortality of benthic species does not necessarily indicate irreversible losses of functional diversity if it is not accompanied by losses of irreplaceable functions (Micheli and Halpern, 2005; Crowder et al., 2008). Therefore, regardless of its direct effect on mortality of benthic species, management intervention on demersal trawling is likely to hinge on demonstrable evidence of its impacts on important ecosystem processes.
In the quest for methods to assess ecosystem health, marine benthic ecology has recently experienced a surge in ecological indicator development through the use of multiple biological traits (Beauchard et al., 2017; Villnäs et al., 2017; Miatta et al., 2021). Until now, most studies have focused on species vulnerability in the context of ecosystem degradation solely based on organism loss. For instance, it is now well established that life span is a relevant proxy to growth rate and to some degree reproductive success (Kindsvater et al., 2016). This trait can be used to express population recoverability following disturbance, as long-lived species are more vulnerable than short-lived ones due to their slower growth (Auster and Langton, 1999; Hiddink et al., 2017; Rijnsdorp et al., 2018). Biological traits that determine how species respond to disturbance and adapt to the environment in which they occur are often referred to as “response traits”. However, following disturbance, population reduction or species extirpation is all the more critical if these species provide crucial ecosystem functions, something that response traits do not directly express. Rather, “effect traits”, that depict effects of organisms on the ecosystem, do have a direct expression on ecosystem functions and thus are more appropriate to relate disturbance to ecosystem functioning. The consideration for this response-effect trait dichotomy, raised some time ago (Lavorel and Garnier, 2002), was recently bolstered in marine benthic ecology (Beauchard et al., 2023). Response and effect traits consist of two complementary facets to describe species assembly rules and functional diversity, i.e., what species are on the one hand, and what species do on the other hand (Beauchard, 2023).
The manipulation of effect trait data offers new research opportunities on sea floor ecosystem functioning in relation to disturbance. Current theoretical understanding assumes most benthic species perform several ecosystem functions (Snelgrove et al., 2014), notably through the concept of functional niche (Rosenfeld, 2002; Violle and Jiang, 2009), still poorly considered in marine benthic ecology. The functional niche of a species can be defined as the multidimensional extent that its trait modalities occupy in the Euclidean space defined by trait variables (Beauchard et al., 2023); the more divergent the trait modalities, the broader the niche, and consequently the more dissimilar or numerous the functions performed by the species are. In addition, the species position in the trait space can reveal peculiar and rare combinations of traits, irreplaceable after species loss through natural extinction or caused by long-lasting human pressure. Such trait combinations are known to expand the volume of the species community in the trait space, and ultimately to increase functional diversity as shown in the evolution of the marine benthos (Villéger et al., 2011). Hence, when derived from effect traits, functional niche and diversity become meaningful concepts appropriate to the assessment of ecosystem health, functioning and service supply: species loss and consequent collapse in diversity indicate potential ecosystem degradation.
These considerations open the door to new exploratory investigations in our understanding of bottom trawling effects on the marine ecosystem. Although life span is strongly related to species recoverability following disturbance, the loss of short-lived species as a result of chronic bottom trawling might be as critical as losing long-lived species from an ecosystem functioning perspective. The concept of functional niche lies at the basis of this possibility. Beauchard et al. (2023) recently provided evidence of possible independence between species life span and functional niche (in terms of type and breadth) in the marine benthos. Indeed, not all species are equally impacted by demersal fisheries as other attributes such as burrowing depth may enable some species to survive irrespective of their life span (Thrush et al., 1998; Bergman and van Santbrink, 2000; Duplisea et al., 2002; Tiano et al., 2020; Beauchard et al., 2021). For instance, if a set of functions are ensured by species of a similar degree of sensitivity to trawling gears (e.g. epibenthic), an impact on those functions should be expected beyond a certain trawling disturbance frequency that prevents recovery of these species (i.e., growth too slow). In contrast, trait composition characteristic of deeply buried species should positively covary with trawling intensity, whatever the life span of those species. Hence, depending on the local assemblage, various patterns in effect trait composition should be expected along a gradient of trawling disturbance, combining epibenthic and endobenthic species along the gradient. Additionally, species with large functional niche are those that contribute the most to functional diversity, and those species can occupy different depths in the sediment (Beauchard et al., 2023). Consequently, due to the potential mismatch with recovery potential, any trend in functional niche and diversity along a gradient of increasing trawling disturbance frequency should result, as trawling primarily affects the upper sediment layer with possibly lower impact on deep burrowing species.
In this study, we explore the likelihood of these considerations through thirteen case studies representing various environmental, sampling and demersal fishing contexts in European waters. The possible independence between life span on the one hand, and functional niche and diversity on the other hand, may suggest that impact on ecosystem function by bottom trawling is context dependent. By disentangling the influences of natural drivers and bottom trawling on benthic species composition, we hypothesize that bottom trawling can be a selective force of benthic effect trait composition (H1), irrespective of life span (H2). Furthermore, we hypothesize that bottom trawling can be a driver of functional niche and diversity, effect being case- or context-specific (H3).
2 Materials and methods
2.1 Case studies
Our hypotheses were tested through thirteen case studies for which similar information was available, enabling the application of the same analytical methodology (Figure 1). For each case study, we considered: bottom trawling intensity (mostly as swept area ratio (SAR, yr-1); Eigaard et al., 2017); habitat descriptors traditionally used in benthic ecology to explain species community composition such as depth and descriptors that account for hydrology and sediment type (Supplementary Material 1, Table S1); the distributions of benthic invertebrate taxa identified at the lowest taxonomic level; for the same taxa, effect trait data; see section “Data analysis” regarding the content of data matrices. A brief summary of the main features of each case study is provided hereafter and in Table 1.
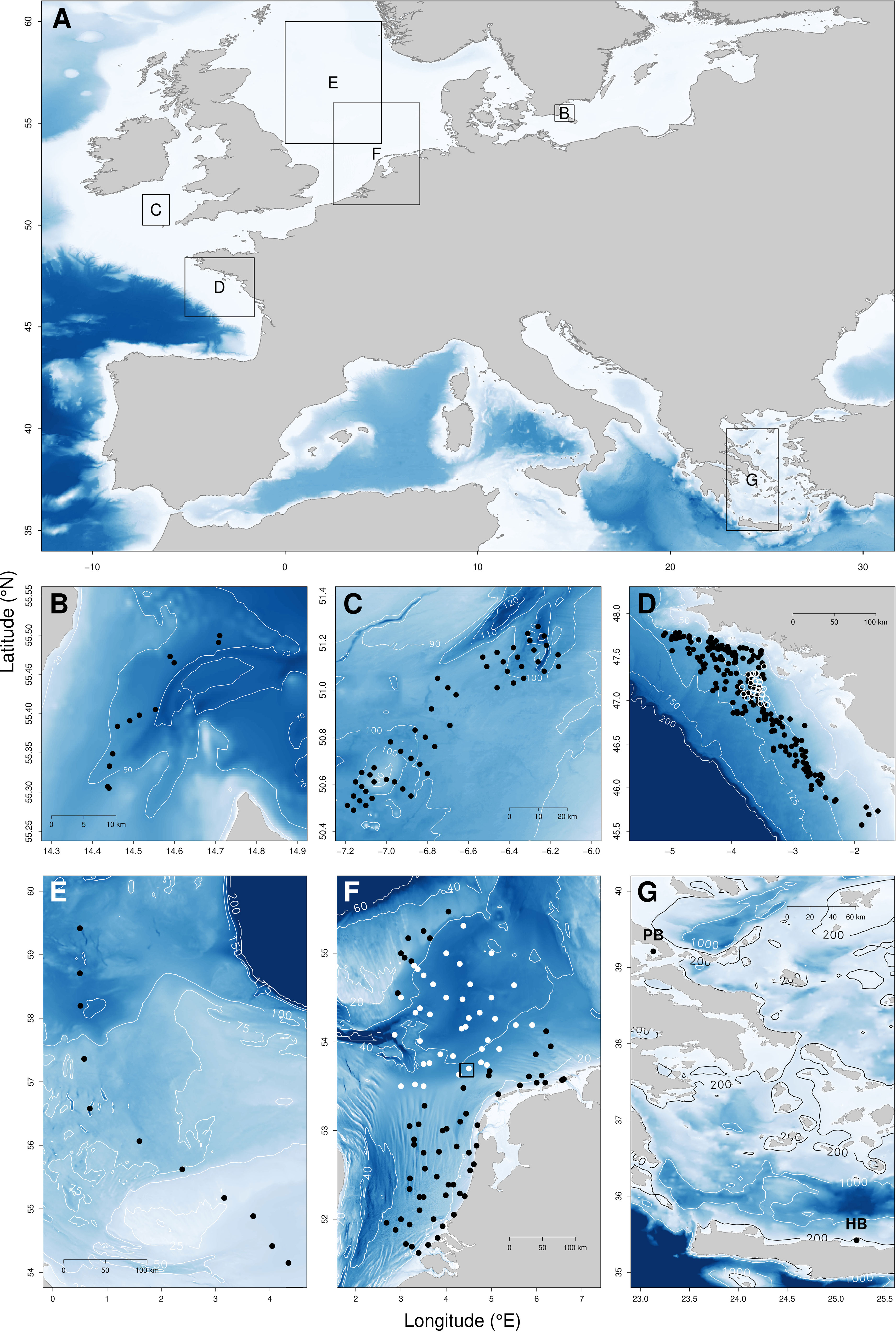
Figure 1 Study areas. (A) Locations of the case studies in European waters; black frames delineate panels from B to G where sample positions (dots) are mapped. (B) Baltic Sea (BS). (C) Celtic Sea case study (CS). (D) Bay of Biscay; black dots, BBL case; opened white circles, BBF case. (E) North Sea transect (NST). (F) Dutch sector of the North Sea (all dots, DSNS–WA); white dots, low dynamics case (DSNS – LD); black dots, high dynamics case (DSNS – HD); square in the middle, Frisian Front case (FF). (G) Eastern Mediterranean Sea (EMS); PB, Pagasitikos Bay case; HB, Heraklion Bay case; samples are aggregated at very small scale (45 and 50 for PB and HB respectively). Contour lines indicate depth in meters, with contrasts emphasized by blue background adapted to each area.
Baltic Sea (BS). Replicated sampling was done in an area of the southern Baltic commercially fished with otter trawls, mainly for cod. The fauna was sampled with a 0.1 m2 van Veen grab and sieved through a 1 mm mesh. Sediment cores (8 or 9 cm diameter) were analysed for % organic carbon, porosity, chlorophyll and grain size. Mean bottom water salinity, oxygen and nutrient concentrations were extracted for each study site from the Copernicus Marine Environment Monitoring Service (CMEMS)’s Baltic Sea Physics and Biogeochemical Reanalysis products (CMEMS, 2021).
Dutch sector of the North Sea (DSNS). The area extends from the southern to the central North Sea. It is regularly monitored by the Dutch Ministry of the Environment at 103 permanent stations sampled by box corer (0.07 m²). Samples are sieved through a 1 mm mesh. Three case studies are considered from DSNS given the large area and the possibility of different sub-scale patterns. Next to the whole area (“DSNS – WA” case study), two habitats of contrasting hydrodynamics are considered, low dynamics habitat (DSNS – LD) and high dynamics habitat (DSNS – HD). A detailed description is provided in Beauchard et al. (2022).
North Sea Transect (NST case study). Sediment box cores (0.07 m²) were sampled along a transect of 500 km, spanning from the Dutch coast to Fladen Grounds (northern North Sea); samples were sieved through 1 mm. Three replicates were taken at 11 stations, from 26 to 225 m deep, so large variations in community composition were expected. No sub-case studies were considered given the low number of stations; see De Borger et al. (2021) for details.
Frisian Front (FF). Before-after control-impact experiment (BACI) which took place in the muddy area of the Frisian Front (North Sea). Two adjacent locations, equally disturbed by commercial trawlers, were trawled with either tickler chain or pulse rigged beam trawl. Due to experimental constraints, only the tickler chain treatment was kept so that numbers of control and impacted samples were equitably represented (control, 7; impacted, 9). Sampling was performed using a box corer before sieving through 1 mm; see details in Tiano et al. (2020).
Celtic Sea (CS). Sampling stations were selected according to spatial and temporal heterogeneity. At each stations, five replicate box cores (0.08 m2) were collected and sub-sampled for the sediment particle size assessment and porosity at 0-5cm depth intervals. Subsequently, the bulk of these cores were sieved through 1 mm. A detailed description can be found in Thompson et al. (2017).
Bay of Biscay – LANGOLF survey (BBL). Sampling stations cover the muddy areas corresponding to the main Nephrops norvegicus fishing ground located in the central-northern part of the Bay of Biscay. The data was obtained from three annual trawling surveys. Although the fauna included burrowing species, the epi-megafauna represented a dominant component collected using a Nephrops twin otter-trawl with a 40 mm cod-end mesh. The area covered by each station was around 0.04 km². See Robert (2017) for a detailed description.
Bay of Biscay – FEBBE survey (BBF). This survey covers a restricted area of the central muddy area of the Bay of Biscay. Sampling stations were selected so that habitat variations be minimum. The sediment type was mainly muddy sand with 13 to 24% of mud content, while ensuring a marked gradient in trawling pressure (SAR of 2.9 to 19.0 year-1). Sediment samples were collected in May and August (five replicates per station on average) using a Day-Grab (0.1 m2) and sieved through 1 mm. See Robert (2017) for a detailed description.
Eastern Mediterranean Sea (EMS). Are included data from three sampling campaigns conducted in Pagasitikos Bay and Heraklion Bay. Pagasitikos Bay (EMS-PB) samples stem from a BACI experiment in a previously unfished area where they were taken before and two days after a trawling event (1000 × 50 m). In Heraklion Bay, two case studies were separated: a BACI experiment (EMS-HBB) of similar design to the EMS-PB case study and a control-impact survey (EMS-HBS) inside and outside a commercial fishing lane. In this last case, SAR data were not available so fishing intensity was represented as a categorical variable (0 for untrawled samples and 1 for trawled samples); however, trawling intensity is estimated very low in Heraklion Bay (0.5-1.0 SAR). In the three case studies, sediment samples were collected from trawled and control sites using a Smith-McIntyre grab (0.1 m2) and sieved through 0.5 mm. We considered a fourth case study as the combination the three data sets (EMS-WA).
2.2 Trawling intensity data
In most case studies, we used the vessel monitoring system (VMS) and logbook data from fishing vessels ≥ 12 m-long compiled by the International Council for the Exploration of the Sea (ICES, 2021) that exists in raster layers (0.05° × 0.05° resolution) of annual (2009-2020) fishing intensity data covering maritime areas of the OSPAR regional sea convention. The layers aggregate beam trawl, dredge, demersal seine and otter trawl gears. Yearly average trawling intensity values per sampling station were considered for the longest period preceding the benthic sampling for each case study data set. For the Baltic case study, yearly average SAR was calculated from VMS and logbook data for ≥ 12 m-long fishing vessels (otter trawlers) between 2016 and 2019 at a resolution of 0.002° × 0.002°. In EMS and BACI case studies, trawling intensity was represented as a binary variable (0 for untrawled sites and 1 for trawled sites). In none of our case studies were there closed seasons or clear bottom trawling seasonality, only switches from some to other commercial species. Hence, multiple trawling disturbances within a year were not necessarily aggregated within a restricted period so that we did not expect temporal opportunities for benthic recovery, especially since most areas had a SAR of > 1.
2.3 Trait data
We used the recently published data set from Beauchard et al. (2023) that provides 15 effect traits covering bioturbation and habitat creation (e.g. burrowing depth, sediment mixing and bioirrigation potentials, burrow and biogenic emergent structure types and sizes), and documented for 812 European benthic invertebrate species. These traits mainly express ecosystem engineering whereby engineer species indirectly affect the fitness of co-occurring species by modulating resource availability (Jones et al., 1997). As we investigated only sedimentary habitats, we discarded the trait “Bioerosion” that is only expressed in hard substrata. Forty-seven additional species representing substantial proportions of the occurrences in some case studies were documented for the same traits (Supplementary Material 2). The data set also includes life span, a key trait strongly related to growth rate and used to express species recoverability potential in assessment such as the Relative Benthic Status adopted by ICES to estimate seabed habitat condition (Hiddink et al., 2017; Pitcher et al., 2017; Rijnsdorp et al., 2018). Taxa identified only at the genus level or rarely above were attributed mean trait scores of species within the considered clade.
2.4 Data analysis
2.4.1 Functional niche breadth calculation
Using the 859 species, we recalculated species functional niches breadth following the procedure initially proposed and detailed in Beauchard et al. (2023). Each of the 15 traits is an 859 species × m modality matrix, and the 15 matrices enable the representation of each species through 15 positions within a same system of multivariate axes (Multiple Factor Analysis; Abdi et al., 2013). Then, the functional niche breadth consists of the variance of the 15 axis scores (sum of variances for several axes). As a result, the more dissimilar the traits, the broader the niche and ultimately the more multi-functional the species; computational details of the procedure are provided in Supplementary Materials 3. Calculation of functional diversity is described in the section on hypothesis testing.
2.4.2 Trawling-induced disturbance gradient
We built a multivariate space accounting for covariations between trawling intensity and effect traits to test our three hypotheses. The relationships between trawling intensity and trait composition were explored by combining the RLQ ordination technique and the Fourth-corner testing procedure (Dray et al., 2014). RLQ ordination enables sample, habitat descriptor (matrix R; samples × descriptors), species (matrix L; samples × species) and trait (matrix Q; species × traits) positioning within the same multivariate space (Dolédec et al., 1996), while the Fourth-corner testing provides a conservative assessment of the habitat-traits statistical relationships (Dray and Legendre, 2008; Peres-Neto et al., 2017). This analytical methodology is now widely used in community ecology, but we applied here a modified version given the possible confounding effects between habitat descriptors and trawling on faunal composition. Indeed, correlations between habitat descriptors and trawling intensity could indicate that fishing effort was not equally exerted on all habitats, with impacts possibly biased toward benthic communities of specific functional attributes. To control for such constraints, we applied the “Partial-RLQ” procedure (Wesuls et al., 2011) that consists in regressing trawling intensity and each taxon on habitat descriptors and in extracting residuals as new R and L matrices. In this way, we built a disturbance gradient perfectly correlated to trawling intensity while controlling for habitat variations. In all case studies, matrices L and Q were preliminarily processed by Correspondence Analysis and Fuzzy Correspondence Analysis (Chevénet et al., 1994) respectively, whereas matrix R was processed either by standardised Principal Component Analysis or Hill and Smith Analysis (Hill and Smith, 1976) depending on the presence of qualitative habitat descriptors next to quantitative ones. Following detrending, residual values in matrix L were rescaled by subtraction to the minimum value of the matrix in order to get null or positive values as negative ones cannot be processed by Correspondence Analysis; this does not affect data structure and relationships between taxa (columns) which are attributed the same importance (same column sum) as in Non-Symmetric Correspondence Analysis (Grimaret-Carpentier et al., 1998). The RLQ-Fourth-corner methodology provides unbiased R-Q correlations unlike the more popular community weighted mean (CWM), which can critically overestimate these correlations as CWM ignores biological variations within communities (Peres-Neto et al., 2017).
2.4.3 Hypothesis testing
The resulting multivariate RLQ system was composed of a single axis along which variable trait ordinations could be expected following H1 (trawling-induced trait selection); see Figure 2. H2 (independence of recoverability, expressed by life span, along the gradient) was then simply considered as the covariation between the axis score and life span, and similarly H3 with functional niche breadth and functional diversity (any trend expected), and tested through the Fourth-corner method. Functional diversity, defined for a species community, was calculated from the taxon axis scores; rather than using the raw abundance (matrix L) and trait data (matrix Q) as regularly encountered in the literature, we used the species scores from the RLQ axis to ensure diversity values solely consequent from traits selected by trawling effect. We limited our consideration of functional diversity to the species community extent along the partial RLQ axis through Rao’s quadratic entropy as the weighted sum of square distances between species positions (Rao, 1982). A high value for this index indicates that species within the community perform highly dissimilar functions and that species loss can entail substantial loss in ecosystem function. The significance of the RLQ pattern (H1) was assessed by the double random permutation procedure of Dray and Legendre (2008) combining model 2 (99999 row permutations of matrix L breaking the R-L link) and model 4 (column permutations breaking the L-Q link), recalculating the RLQ co-inertia at each iteration (i.e., multidimensional covariance). The same procedure was applied to relate life span (H2, Figure 2C), and functional niche and diversity to the partial RLQ axis (H3, Figures 2C, D).
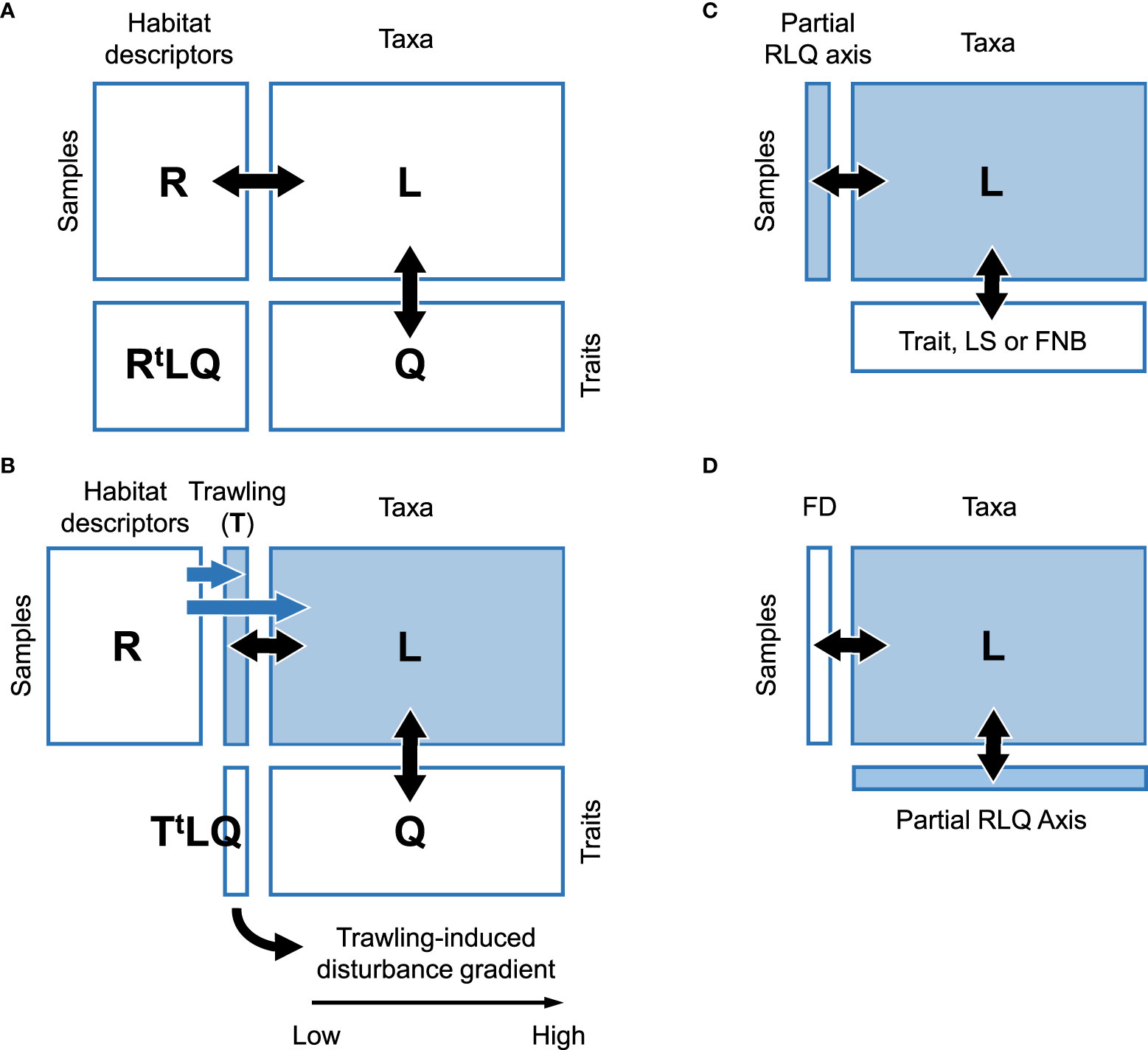
Figure 2 Summary of the data analytical procedure. (A) Typical full RLQ matrix (rectangles) disposition; black arrows indicate row or column matching; multivariate axes that simultaneously enable sample, habitat descriptor, taxa and trait positioning are obtained through the product AtA with A = RtLQ. (B) Partial RLQ; blue arrows indicate detrending of the trawling and L variables from the R variables through multiple regression (residual extraction, filled rectangles). As the matrix AtA with A = TtLQ contains only one value (sum of square covariances between the unique variable of T and Q variables through L abundances, i.e., presence-absence, individual or biomass density), the multivariate ordination results in a single axis, with increasing T values from left to right. The Fourth-corner method was primarily applied to test the significance of the relationship between matrices T and Q (H1); when significant, the procedure was applied again to test the significance of the relationships between the RLQ axis and each effect trait. (C) Relating effect trait (H1), life span (LS, H2) or functional niche breadth (FNB, H3) to the partial RLQ sample axis scores derived from (B) and testing through the Fourth-corner procedure. (D) Reciprocally, relating functional diversity (FD) to the partial RLQ taxon axis scores derived from (B) as part of H3.
2.4.4 Complementary analytical considerations
Given the magnitude of our data and analyses, we synthesized the interpretations of species and trait patterns by positioning functional species groups along the RLQ axis. We used the typology identified in Beauchard et al. (2023) that consists of 15 species groups of specific sets of functions. The analyses were carried out in R 4.3.1 (R Core Team, 2023) with the package “ade4” (Chessel et al., 2004; Dray et al., 2007); see Supplementary Material 3 for R code details. We used the command “rlq” for RLQ ordination, and the command “fourthcorner2” for testing the correlation between the RLQ axis (i.e., sample scores as the trawling-induced disturbance gradient) and each trait as a block of trait modality variables (co-inertia testing; Figure 2C). We also used the command “fourthcorner” to test the correlation between sample scores and functional niche breadth, and the correlation between axis species scores and the Rao index (as a single variable calculated per sample with the FD package; Laliberté et al., 2014; Figure 2D). Prior to partial RLQ analysis, we assessed potential confounding effect between matrix R and trawling intensity on matrix L through Redundancy Analysis with the commands “rda” and “RsquareAdj” of the “vegan” package (Oksanen et al., 2010). We then tested the significance of the full RLQ pattern (i.e., before detrending) in order to verify if trawling alone could induce a trait selection independently of natural forces (i.e., partial RLQ significance) in case of full RLQ non-significance. Indeed, as the coefficient of variation (CV = 100 × SD/mean trawling intensity; Table 1) indicated that variability in trawling intensity was not constant among case studies, we hypothesized that partial RLQ should be significant when trawling was sufficiently variable within a case study (i.e., high CV). When available, all faunal measurements, including presence-absence, individual and biomass densities, were used in matrix L to select the most significant RLQ patterns (i.e., lowest p-value).
3 Results
In most case studies, taxonomic composition was generally dependent on habitat descriptors, with a systematic relationship between trawling intensity and habitat descriptors that could induce confounding effects on trait composition (Table 2). The full RLQ pattern, without considering trawling intensity, was significant in only one case study (NST) while a majority of partial RLQ patterns were found significant (apart from non-significant EMS case studies), and mostly through faunal presence-absence.
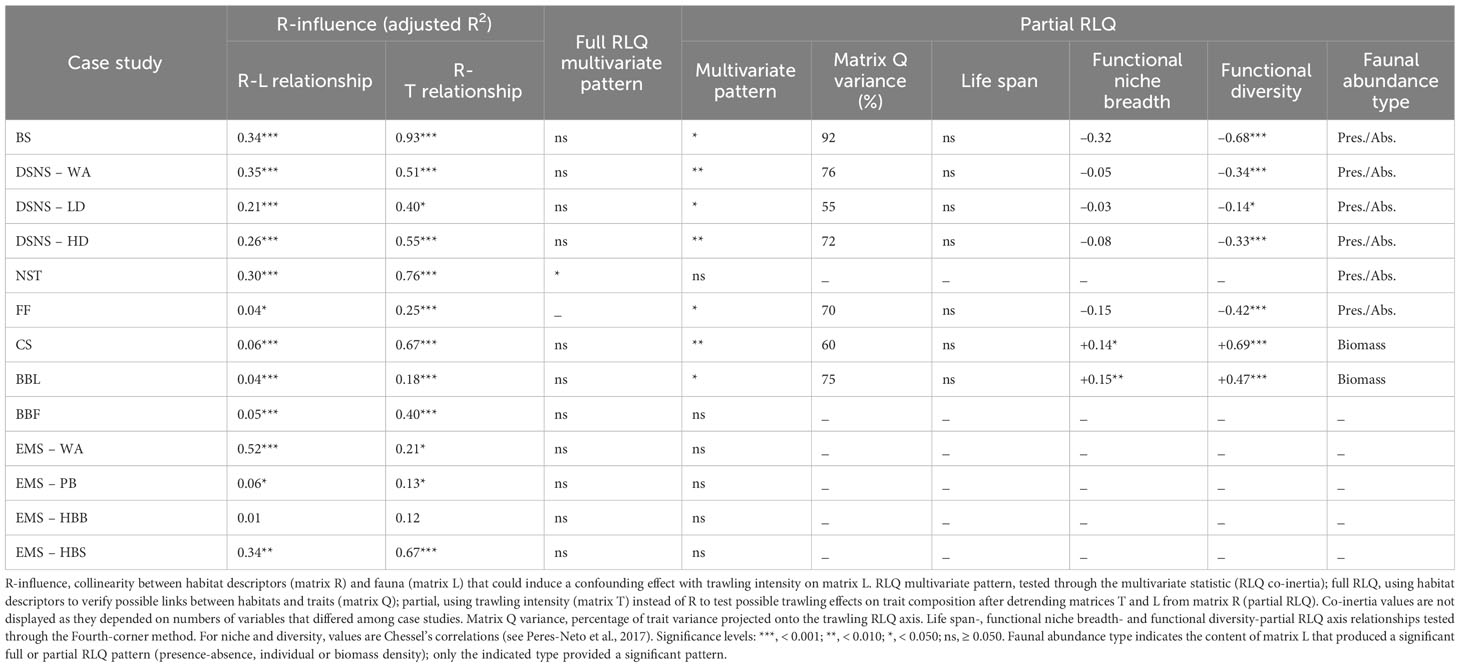
Table 2 Analytical results; see Figure 2 for theoretical support and text for case study abbreviations.
As expected, non-significant trawling effects corresponded to low trawling variability (low CV, Table 1; case studies NST and BBF). Significant RLQ patterns indicated that bottom trawling can be a selective force of benthic effect trait composition (H1). In no case was life span significantly related to the trawling-induced disturbance gradient (Figure 3), and functional niche breadth and diversity could exhibit positive, negative or non-significant trends (Table 2), hence validating H2 and H3 (Figures 4, 5).
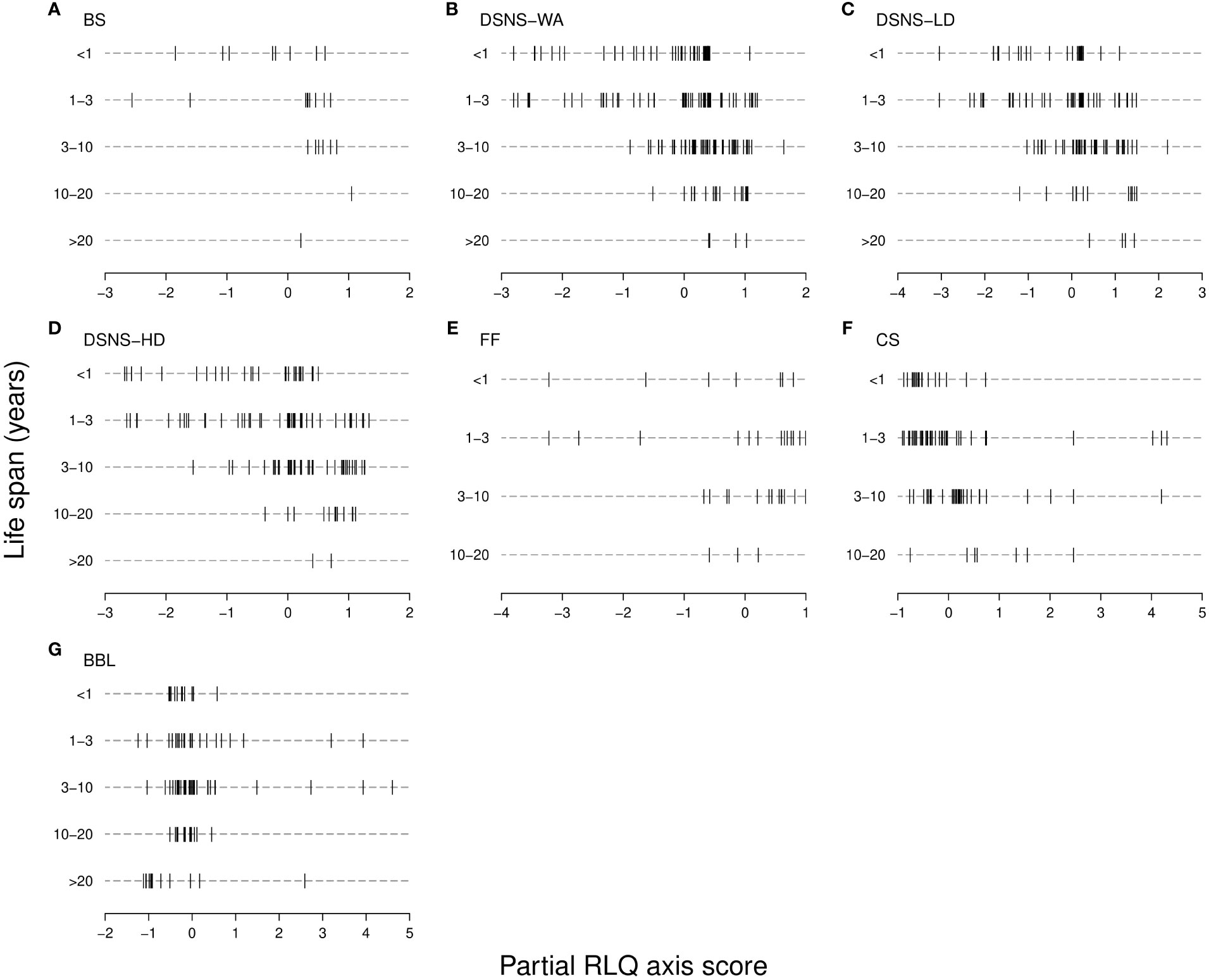
Figure 3 Relationship between life span and the partial RLQ axis; from (A-G), each of the case studies for which the partial RLQ pattern was found significant. In every case study (see Materials and Methods for abbreviations), the RLQ multivariate space consists of a single axis (horizontal) expressing an increase in trawling intensity from left to right, but associated with various effect trait compositions (see Table 3). Species (vertical segments) are grouped per age class. Life span is supposed to express recoverability following disturbance as long-lived species are generally slow-growing, thus accounting for vulnerability to trawling. If it would be the case, long-lived species, vulnerable to minimum disturbance frequency, would be positioned on the left side of the axis, while shorter-lived species would be positioned rather on the right as being the only ones remaining at highest disturbance frequency; no such a trend appears, and no individual age class was significantly correlated to the axis through the Fourth-corner procedure (p > 0.05). As the partial RLQ axis maximizes the covariation between trawling intensity and effect traits (expressing ecosystem functions), life span does not appear to be indicative of sea floor functional vulnerability.
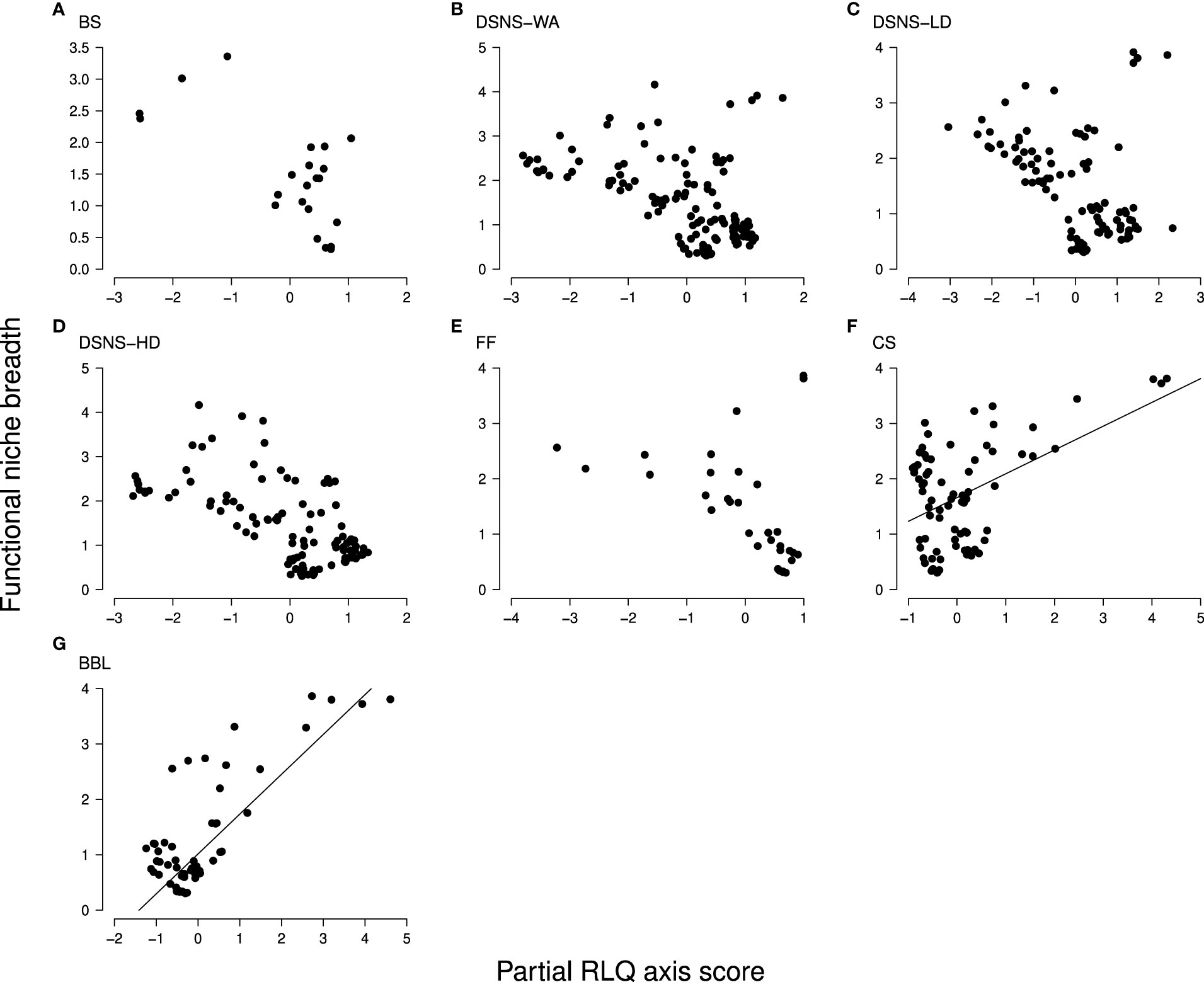
Figure 4 Trend in species functional niche breadth as species multi-functionality along the partial RLQ axis (black dots, species); from (A-G), each of the case studies for which the partial RLQ pattern was found significant (see Materials and Methods for abbreviations). Values can be compared between functional groups in Figure 6 that displays the same species positions along the x-axis: for instance, among species of broad functional niches, tubicolous species (e.g., SmallTub group) on the left side in BS, DSNS and FF case studies, and deep burrowing species (e.g., Deep3D group) on the right side in DSNS-WA, DSNS-LD, FF, and CS case studies. In every case study, the RLQ multivariate space consists of a single axis (horizontal) expressing an increase in trawling intensity from left to right, but associated with various effect trait compositions (Table 3). Trends can be indistinctly positive or negative and were found significant only for CS and BBL case studies (Table 2). In several cases (e.g. DSNS, FF and CS), the range of variation is function of trawling intensity. Only median-high values are found to be impacted under low trawling in DSNS-WA and FF case studies (tubicolous worms) while a wider range subsists at higher intensity where deep burrowing and surficial-resistant species occur. The opposite appears in CS and BBL cases: only deep burrowers subsist at high trawling intensity.
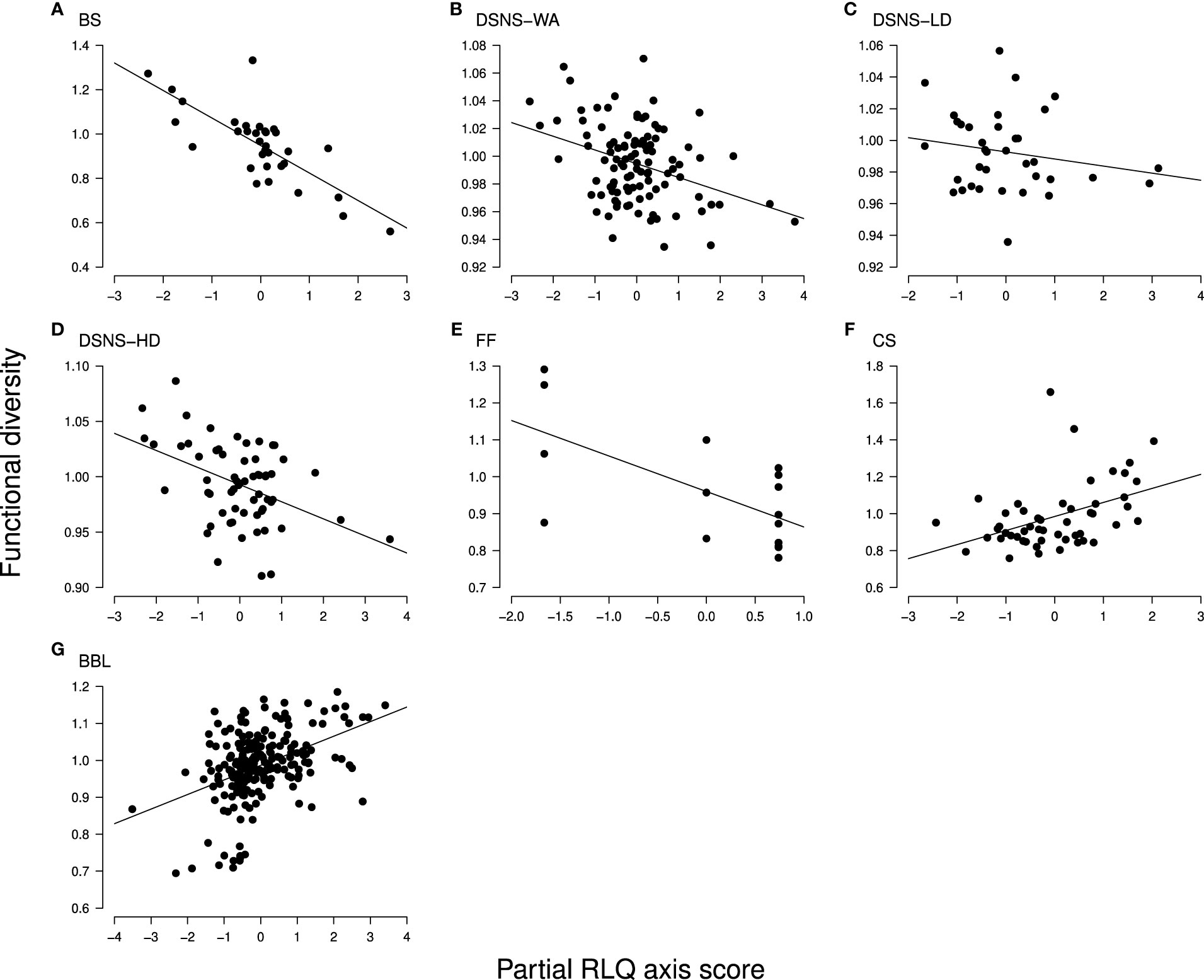
Figure 5 Trend in functional diversity (Rao’s index calculated from species positions) along the partial RLQ axis (black dots, species communities); from (A-G), each of the case studies for which the partial RLQ pattern was found significant (see Materials and Methods for abbreviations). In every case study, the RLQ multivariate space consists of a single axis (horizontal) expressing an increase in trawling intensity from left to right, but associated with various effect trait combinations (see Table 3). Trends can be indistinctly positive or negative and were found significant in all case studies (Table 2).
Various combinations of traits were found covariant with trawling intensity, showing case study-specificity in trait composition (Table 3). Although functional group composition varied among case studies, some aspects were quite common when looking at their distributions along the disturbance gradient (Figure 6). Tube dwelling species (“SmallTub” and “DeepTub”), when substantially present, were mostly positioned in the left side of the axis, corresponding to lower trawling intensity. Typical representatives of these groups were tubicolous worms (e.g. Lanice conchilega, Phoronis spp., Polydora spp., Spio spp., Streblospio spp., Thelepus cincinnatus) with characteristic traits like upward or downward material conveying, tube building and consequent sediment biostabilisation (Table 3). In contrast, deep burrowing groups (“SesBiot” and “Deep3D”) were mostly associated with intense trawling (right; except Deep3D in DSNS – HD where this group was rare). SesBiot group includes various sessile and deeply buried species such as Chaetopterus variopedatus, Lutraria lutraria and Mya arenaria or less deeply buried such as Amphiura spp. which can substantially irrigate the sediment through simple or complex burrow systems. Deep3D group includes mud shrimps (Callianassa subterranea, Gilvossius tyrrhenus, Upogebia spp.), lugworms (Arenicola spp.) and peanut worms (Golfingia elongata) that can achieve disproportionate sediment mixing (diffusion, upward conveying and regeneration) and irrigation rates. More intermediate along the disturbance gradient, the group “SurfDiff”, composed of many mobile epibenthic or shallow buried species (surficial biodiffusors), was represented in all case studies. Its position along the gradient could vary from slightly left (i.e., lower trawling intensity) when heterogeneously composed (crabs, ophiuroids, polychaetes and others; CS and BBL case studies) to slightly more to the right (i.e., higher trawling intensity) where there were great abundances of small crustaceans such as amphipods or hooded shrimps (BS, DSNS – HD).
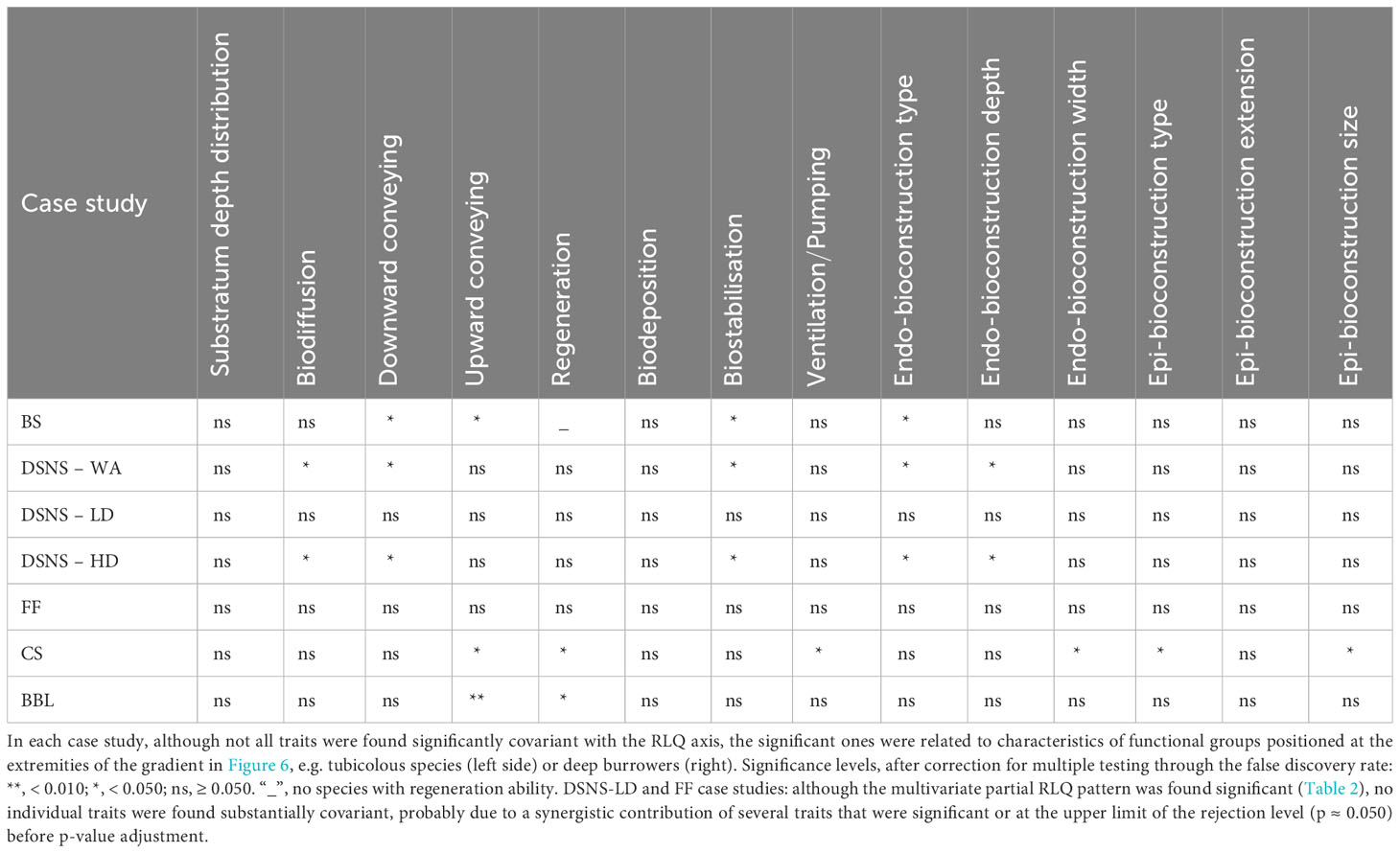
Table 3 Trait-partial RLQ axis relationships tested through the multivariate statistic (RLQ co-inertia) as part of H1; see Materials and Methods for case study abbreviations.
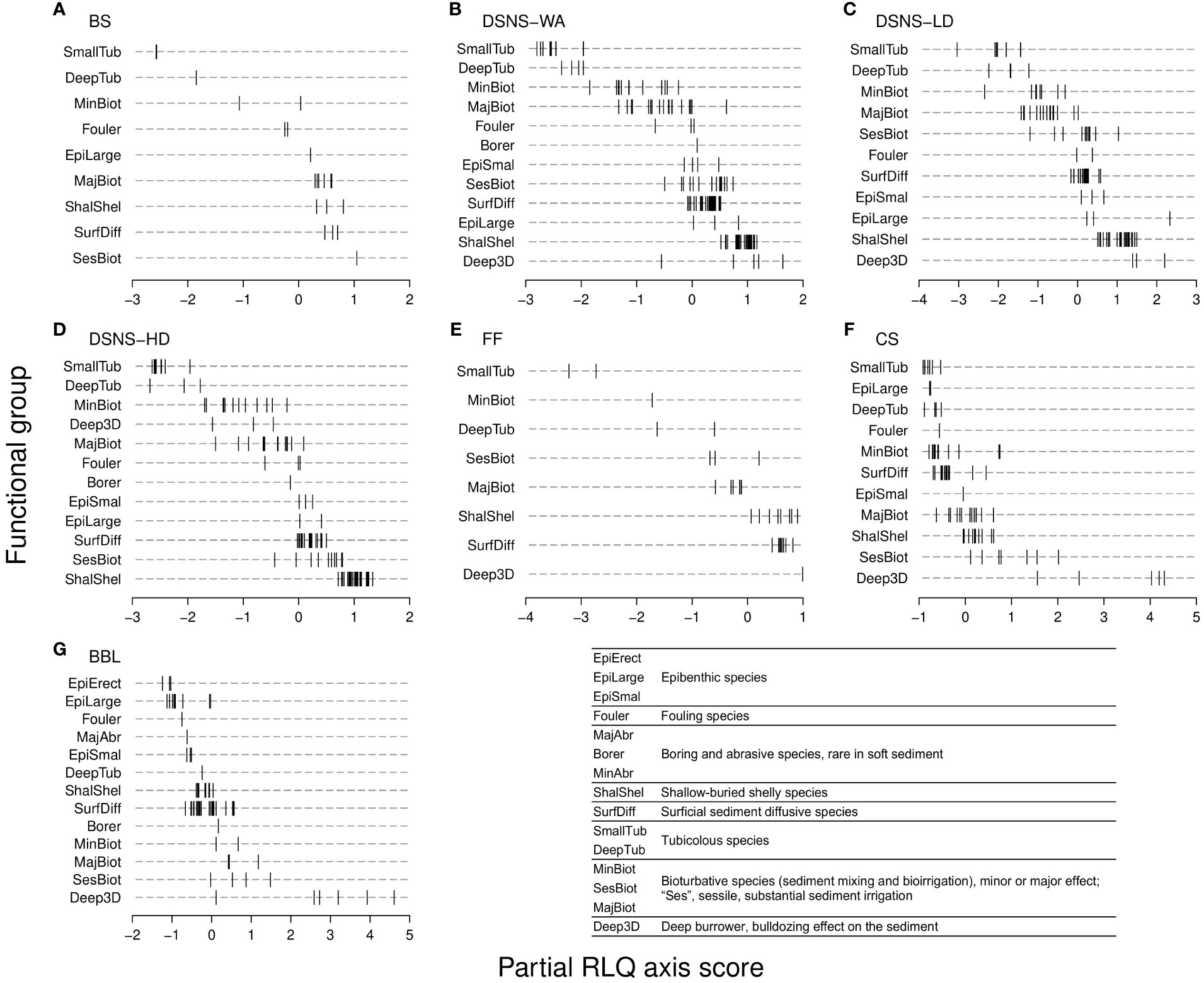
Figure 6 Functional group distributions along the partial RLQ axis (standardised) from (A-G), each of the case studies for which the partial RLQ pattern was found significant (see Materials and Methods for abbreviations). In every case study, the RLQ multivariate space consists of a single axis (horizontal) expressing an increase in trawling intensity from left to right, associated with various effect trait compositions (see Table 3). Vertical segments, species positions within functional groups; group distributions are arranged from left to right according to their mean species position. As the analysis controls for the effect of habitat, species positions are solely determined by trawling intensity. Hence, species positioned on the left side of the axis are those that disappear firstly, i.e., at lowest trawling intensity. Bottom right, brief description of functional groups; see Discussion, Supplementary Material 2 and Beauchard et al. (2023) for more details.
Based on epibenthic sampling, the BBL case study provided complementary outcomes regarding the epifauna (Figure 6G). Groups of large sessile species were found the most characteristic of low trawling intensity: on the one hand, large erect or arborescent species (group “EpiErect”) that include crinoids (Antedon petasus, Leptometra celtica) and sponges (Axinellidae), and, on the other hand, less morphologically complex but substantially large species (“EpiLarge”) such as oysters as reef builders (Neopycnodonte choclear, Ostrea edulis), sea pens (Funiculina quadrangularis, Pennatula phosphorea, Pteroeides griseum) and other corals (Alcyonium spp.). Other groups endowed with burrowing ability, less impacted, extended the gradient toward higher trawling frequencies: sessile (“SesBiot”; Chaetopterus sp.) and major bioturbators (“MajBiot”; Brissopsis lyrifera, Rissoides desmaresti), and deep gallery builders (“Deep3D”; Upogebia stellata) with possibly an epibenthic foraging mode (Alpheus glaber, Goneplax rhomboides, Homarus gammarus, Nephrops norvegicus).
4 Discussion
4.1 Key implications of functional ecology for bottom trawling impact studies
As already reported in other studies (Tillin et al., 2006; van Denderen et al., 2015; Pommer et al., 2016; Howarth et al., 2018; Beauchard et al., 2021; McLaverty et al., 2021; De la Torriente Diez et al., 2022; Tsikopoulou et al., 2022), our results show that bottom trawling can have a selective effect on benthic trait composition, likely because of fishing gear-induced mortality. However, the present study is novel due to the specific use of effect traits with a very strong emphasis on trait compositional variation that could be solely due to trawling effect by controlling for habitat variations (partial RLQ axis), as evidenced (H1). The absence of relation between life span and the partial RLQ axis (H2) is clear empirical support of different ecological implications between response and effect traits. Additionally, the significant RLQ patterns demonstrate that traits other than life span are involved in bottom trawling impact. Below, we discuss the causes of faunal vulnerability evidenced here and emphasize the importance of fundamental concepts from functional ecology in the framework of bottom trawling impact studies.
Functional group distributions provided important outcomes (Figure 6); most prominent was the opposition between tubicolous and deep burrowing species at the extremities of the trawling intensity gradient. This emphasizes the key role of burrowing depth in trawling impact, although the trait “Substratum depth distribution” was never found significantly covariant along the trawling gradient (Table 3). This is likely because some deep burrowing and very mobile species can exploit the overall sediment matrix from the sediment surface to several tens of centimetres deep (Pearson and Rosenberg, 1978; Rhoads and Boyer, 1982). Thus, they partly share their niche with other species that are more restricted to the upper sediment layer, while they exhibit a lower risk of gear impact when occurring in deeper layers; this finding is very similar to what can be observed under natural storm disturbance (Posey et al., 1996). Also, among groups found at intermediate trawling intensity such as surficial diffusors, expectations can be variable, as the species from this group in the CS and BBL case studies were more sensitive to trawling gear than some species in the BS and DSNS – HD cases. Crabs, ophiuroids and polychaetes found in CS and BBL case studies are larger than the abundant small crustaceans in BS and DSNS – HD cases, so body size could have been a possible cause of vulnerability (body mass being implicitly expressed in several effect traits); as previously shown in natural conditions, surficial and potentially resistant species to physical stress are necessarily A-strategists (adversity selection; Greenslade, 1983; Southwood, 1988) such as small crustaceans in the marine benthos (Beauchard et al., 2022). Outcomes from the BBL analysis strengthen the implications of traits other than life span in vulnerability to trawling impact: the charismatic coral living forms, typically exposed because of their large size and deprived of mobility. Hence, traits such as burrowing depth and body size can act as a survival traits to explain the vulnerability or resistance of some functional groups, irrespective of life span.
Responses of functional niche breadth and diversity to trawling intensity were shown to be case-specific (H3). The fact that functional diversity did respond positively to trawling intensity in some cases seems paradoxical since one would expect that diversity would decline in response to heavy and abrasive fishing gears like trawl doors, tickler chains and dredges (Grabowski et al., 2014). As a reminder, functional diversity was calculated from species RLQ axis scores so that its trend was necessarily consequent from trawling intensity, not from untrawled conditions. As surficial organisms are more sensitive to fishing gears, deep burrowers, as the remaining part of the fauna following disturbance, can maintain a major source of functional variation due to their rare combinations of traits (“functional originality”; Kondratyeva et al., 2019). Additionally, epibenthic and shallow buried organisms like tubicolous worms can co-occur with deep burrowers where both types increase functional diversity (Beauchard et al., 2023). Thus, since trawling removes some species, when diversity was positively correlated to the axis, its values could underestimate the values that would be observed in untrawled conditions where more groups could have co-occurred without necessarily generating a trend in diversity. In this case, the trawling-induced disturbance gradient (partial RLQ axis), controlling for habitat variations and accounting only for trawling effect, can return a biased image of trawling effect while diversity is really impaired. In a nutshell, bottom trawling never increases diversity; at the most, it creates a gradient by removing ecosystem functions in some places while it has less effects in some others. As shown in Figures 4, 5, niche and diversity follow very similar trends. In most cases, species of intermediate to broad functional niche occur only at low trawling intensity, which means that trawling can have significant effects on sea floor multi-functionality in reducing diversity everywhere along the gradient. As an ensuing question, beyond which disturbance frequency does the temporal removal of this multi-functionality become detrimental to ecosystem functioning?
The loss of tube dwelling species with increasing trawling intensity may have been less expected given the widely accepted fast recoverability of these organisms following natural or human-induced disturbance (Pearson and Rosenberg, 1978; Levin, 1984; Barry, 1989; Noji and Noji, 1991). If such species were truly resilient to trawling, their presence should have been expected rather at high disturbance level (as an important fraction of the remaining community), which is not the case since, when present, the group “SmallTub” (small tube dwellers) systematically occurred at lowest trawling frequency (Figure 6). Very brittle, occupying the upper centimeters of the sediments (with sometimes protruding tubes), and with a life cycle often reaching a year or more, these species cannot proliferate everywhere as the average SAR of commercial fisheries infers that trawl disturbance notably exceeds one per year in many areas. The disappearance of tube dwellers might be more critical than generally considered since these species ensure multiple functions, as shown by their substantially broad functional niches (Figure 4; e.g., sediment stabilisation and irrigation, advective transfer of materials, biodeposition, mound creation and habitat building through tube lawns). While the positive trend in diversity along the disturbance gradient in FF, CS and BBL case studies might be misleading, the negative trend in BS and DSNS case studies may be truly critical due to the loss of tubicolous species. Only a few studies reported impacts on tubicolous worms (Collie, 2000; Kollmann and Stachowitsch, 2001; Hermsen et al., 2003; Callaway et al., 2010; Tiano et al., 2022), and more recent studies provide evidence of their vulnerability to trawling (McLaverty et al., 2021), with especially critical consequences given the habitat opportunities offered by tube lawns (Collie, 2000; Callaway et al., 2010; Vitaliano et al., 2013). This suggests that even relatively low trawling frequencies can prevent the recovery of short-lived species, showing again the lack of conservatism in the use of life span alone to indicate ecosystem degradation.
Some deep burrowing species (e.g. Deep3D group) exhibit an apparent resistance to trawling disturbance, probably as they inhabit sediment depths beyond the sediment penetration of demersal trawls. The contribution of these species to ecosystem functioning may represent another important point of discussion. In addition to their engineering effect within the sediment matrix through complex burrow systems that they actively irrigate, they modify the sediment surface by producing large mounds consequent from upward sediment displacement: these burrow linings and mounds are known to be biogeochemical hotspots concentrating microorganisms (Ziebis et al., 1996; Murray et al., 2002). As already evidenced in the Frisian Front case study (Tiano et al., 2020), the alteration of these structures through bottom trawling might impair ecosystem functioning regardless of the sensitivity of the engineering organism to the trawling gear. This logic could also hold for every species producing mounds and pellets, including tubicolous species previously mentioned, and other functional implications of these biogenic structures (Mouritsen et al., 1998; Murray et al., 2002; Wild et al., 2005). Hence, from a conservation perspective, the direct trawling impact on important engineering species may not be the only concern, the impact on engineer functional effects should also be considered (i.e., notion of “extended phenotype”; Dawkins, 1982). This advocates for further studies on the possible links between effect traits and biogeochemical aspects such as nutrient fluxes and microbiological implications (Kristensen and Kostka, 2005; Laverock et al., 2010; Belley and Snelgrove, 2016; Deng et al., 2022) to verify possible effects of bottom trawling on these aspects via the impact on the fauna.
As our investigation was limited to effect traits expressing ecosystem engineering, it is worth noting that bottom trawling can concomitantly impact other benthic ecosystem functions (Jennings and Kaiser, 1998). Long-term benthos mortality can alter trophic networks, including benthic and pelagic components (Ramsay et al., 1997; Ramsay et al., 1998a). Within the benthos, carrion short-term exposure by trawling gears can promote scavenging in non-vulnerable species (either fast-growing, highly mobile, robust or endowed with regenerative ability; Ramsay and Kaiser, 1998b; Bergmann and Moore, 2001). Within the functional group typology, this might be particularly the case in surficial diffusors (SurfDiff) and a few shelly species (ShalShel): annelid (Phyllodoce mucosa), starfish (Asterias rubens), brittle stars (Ophiura spp.), decapods (Cancer pagurus, Crangon spp., Diogenes pugilator, Liocarcinus spp., Pagurus bernhardus, Palaemon serratus), amphipods (Hippomedon denticulatus, Perioculodes longimanus), isopods (Eurydice spp., Natatolana borealis), gastropods (Bela nebula, Epitonium clathratulum, Euspira spp.); within other groups, and marginally, major bioturbator (MajBiot; Hediste diversicolor) and lobsters (Deep3D; Homarus gammarus, Nephrops norvegicus). While bottom trawling can promote scavenger abundance, most of the scavenging surficial dwellers have lowest engineering multi-functionality (Beauchard et al., 2023). Hence, due to complex relations between the types of ecosystem functions, bottom trawling consequences on total benthic fluxes of materials and energy might occur through highly variable functional pathways and might be strongly context dependent.
4.2 Experimental and analytical considerations
In this multiple case study analysis, we gathered data sets already existing and from different experimental contexts. Although we used different sets of descriptors to best account for the generally inevitable habitat effect on community composition, the trawling RLQ analysis enabled us to compare these disparate data sets on a single axis along which only trawling intensity could account for functional variation. A posteriori, four experimental contexts could be distinguished, of which at least three were represented among the case studies.
(1) No or weak habitat-species community relationship (low R-L adjusted R2, Table 2) and homogeneous distribution of trawling intensity. A low variation in taxonomic composition cannot ensure a substantial variation in functions (i.e., trait composition), and low variation in trawling intensity reduces the experimental power to detect trawling effects on trait composition. FF, EMS-PB and EMS-HBB case studies, as controlled experiments were relevant representatives. This was also characteristic of BBL and BBF case studies, possibly due to lack of habitat preference in megabenthos (BBL) and extremely restricted and deliberately reduced habitat contrast (BBF).
(2) No or weak R-L relationship and variable trawling intensities. In spite of low functional variations over space, substantial variations in trawling intensity can provide an ideal context to identify the benthic functions most vulnerable to disturbance. The experimental power should be strengthened in the case of functionally more dissimilar species communities within any sample, like in the CS case study.
(3) Substantial R-L relationship and homogeneous trawling intensities. The NST case study was well illustrative of this context given its large ranges of sediment types and depths, and low CV in trawling intensity that likely prevented the detection of significant trawling effect.
(4) Substantial R-L relationship and variable trawling intensities. This context is experimentally the most complete as it enables all combinations of communities and trawling pressure to be tested. Although the strong R-L covariation and associated high CV in trawling intensity observed in several case studies could have represented such an ideal context, strong covariations between natural gradients and trawling intensity created a bias towards context (3) as trawling intensities were not evenly represented in all habitats. This concerned BS and the three DSNS case studies. Regarding the EMS-WA and EMS-HBS case studies, it may be characteristic of context (3) or (4) given the strong R-L relationship; in any case, the unavailability of quantitative SAR or possibly too low commercial SAR is important to draw attention to the insignificance of their RLQ patterns.
The experimental handling of trawling is important to infer effect on benthic functional composition. Although the range of variation in trawling intensity seems determinant, we cannot exclude potential shortcomings due to the large spatial resolution of the SAR data (0.050° × 0.050°) used in several case studies and that could have resulted in topographically heterogeneous areas. For instance, in spite of relatively impoverished community composition and important detrending, the BS case study provided a clear partial RLQ pattern with a large amount of biological variance projected on the axis. The much finer resolution of SAR values (0.002° × 0.002°) used in this case study may have enabled a more accurate quantification of disturbance. By contrast, BACI studies, which often do not examine multiple trawling frequencies, or quantification of commercial SAR at large spatial resolution (e.g. 0.050° × 0.050° vs 0.002° × 0.002°) may explain the absence of significant patterns in EMS case studies. In any case, we cannot exclude some other limitations in compositional variation within species communities that could have conjointly led to the absence of a significant pattern.
In general, changes in community composition across a significant R-L relationship constitute a prerequisite for potential changes in functional composition. Although substantial R-L relationships in several case studies fulfilled this requirement, we observed a large absence of full RLQ significance, possibly due to several reasons. Environmental variations have primarily a selective effect on response traits (“environmental filtering”; Weiher and Keddy, 1995), not necessarily on effect traits (Jones et al., 1994; Jones et al., 1997; Lavorel and Garnier, 2002), for which some functional redundancy may have occurred among communities. Nevertheless, as some relationships between habitat descriptors and effect traits could have been expected (Beauchard et al., 2023), sampling effort or technique may have been insufficient to capture the whole functional range of the fauna. Indeed, the faunal occurrence data in the DSNS case studies were considered in the limits of the period of trawling intensity availability (2009-2018), while analyses over the full monitoring period (1995-2018), including more taxa, resulted in significant full RLQ patterns (unpublished work). In any case, the absence of full RLQ significance acted as controlled treatment before a strong emphasis of the selective effect of trawling on trait composition through partial RLQ with trawling as the sole source of significant variation.
The importance of other technical aspects also deserves mention. In benthic community ecology, biomass is generally considered as the best faunal measurement as it accounts for the amount of active living tissues in organisms. While biomass might not be ideal to capture demographic dynamics in benthic communities (Beauchard et al., 2022), it seems intuitively more relevant through the specific use of effect traits when measuring effect rates such as sediment mixing and bioirrigation, closely related to body size (Kristensen and Kostka, 2005; Kristensen et al., 2012). However, biomass was not always the best measurement to detect significant trawling effects. One should keep in mind that our primary objective was to relate the response of effect trait composition to trawling disturbance, not the contribution of trait composition to in situ measurements of bioturbation rates. Therefore, the presences of functional types, reflecting dwelling modes within the sediment matrix, prevailed over quantities such as number of individual organisms or biomass through the all-or-nothing effect of trawling in six case studies. In general, the control of environmental forces (that can include trawling intensity) on species assembly is primarily exerted on species presence at a certain spatial scale, here well illustrated in the NST case study (full-RLQ, Table 2), density dependence being more relevant at smaller scale (Wilson, 2012). In all case studies, faunas were composed of species groups functionally dissimilar but sometimes heterogeneous in terms of species body size, whatever the abundance (i.e., individual or biomass density). Thus, in most significant cases, presence-absence was more relevant to account for a between-functional group trawling effect dominant over within group effects (Figure 6), these latter generating statistical noise when considering individual or biomass density. Hence, when available, it is important to consider all types of faunal measurements that can lead to variable levels of community pattern significance, even presence-absence as the least intuitive.
This overview of the thirteen case studies highlights that outcomes can be variable and uncertain when considering a single case study in biological trait analysis. Although some commonalities appeared in our analytical outcomes, case-specific experimental aspects can be determinant for highlighting significant RLQ patterns. In this respect, experimental extent (e.g. sampling surface area, number of sampling stations) is certainly not determinant as reported for benthic response traits (Beauchard et al., 2022), but rather other aspects can prevail (e.g. contrast in functional composition, range in disturbance frequency). At least, this scrutiny of experimental aspects could support a methodological basis for designing future studies combining variable levels of natural and trawling variations or for providing guidelines to interpret unexpected analytical outcomes.
4.3 Conclusions
The specific use of multiple effect traits offers interesting research opportunities that remain relatively unexplored in benthic ecology, both in a fundamental and applied context. We provided here a first empirical assessment of bottom trawling impact on ecosystem functions that benthic species are assumed to perform given the suites of effect traits they are endowed with. Impacts on the sea floor by bottom trawling have long been recognised, so that the validation of our first hypothesis (modification of trait composition) was strongly expected. We also provided empirical evidences of the absence of trend in life span along the trawling-induced disturbance gradient, supporting our second hypothesis. Although this outcome may have been theoretically less expected, it was in line with recent developments (Beauchard et al., 2023). The consideration of other traits, like burrowing depth, body size or body resistance may improve predictions in losses of ecosystem functions as already proposed (Tyler-Walters et al., 2009; Bolam et al., 2014; Beauchard et al., 2021). Next to growth and reproduction that life span can partly express, burrowing depth ensures survival, the third Darwinian fitness component needed for a theoretically-sound development of ecological indicators of disturbance. Importantly, we showed that bottom trawling could substantially impact species multi-functionality, and that gradients of trait composition could be misleading in the absence of consideration for fundamental concepts of functional community ecology (third hypothesis).
Our findings represent a first step toward a more accurate assessment of trawling effect on sea floor benthos-mediated biogeochemical processes. Using our analytical approach, correlating other measurements (e.g. nutrient fluxes) detrended from the effects of abiotic drivers on community composition with the trawling-induced disturbance gradient does not impose any technical constraint. Hence, this opens the door to deeper investigations regarding other possible properties of the functional groups in various environmental contexts, and we would urge similar analytical explorations with data from other sea areas.
Lastly, we showed that a non-significant trait pattern can mask a significant one, the difficulty being to capture and isolate the source of variation in trait composition. Beyond the support of our hypotheses, this work represents more than an analytical exercise. The use of traits in species community ecology can offer interesting research opportunities, and insignificant patterns should not spur us towards skepticism regarding trait data analysis. As demonstrated in this study, the absence of trends in single case studies can merely indicate that some experimental contexts are less appropriate than others to synecological hypothesis testing.
Data availability statement
The trait data can be found in Supplementary Material 2. Habitat descriptor and faunal occurrence data will be made available upon request, directed to b2xpdmllci5iZWF1Y2hhcmRAbmlvei5ubA==.
Ethics statement
The manuscript presents research on animals that do not require ethical approval for their study.
Author contributions
OB: Conceptualization, Data curation, Formal analysis, Investigation, Methodology, Writing – original draft. CB: Data curation, Writing – review & editing. SB: Data curation, Writing – review & editing. JT: Data curation, Writing – review & editing. CG: Data curation, Writing – review & editing. EB: Data curation, Writing – review & editing. PL: Data curation, Writing – review & editing. MB: Data curation, Writing – review & editing. IT: Data curation, Writing – review & editing. NP: Data curation, Writing – review & editing. CS: Data curation, Writing – review & editing. JC: Data curation, Writing – review & editing. KS: Data curation, Writing – review & editing. MS: Data curation, Supervision, Writing – review & editing.
Funding
The author(s) declare financial support was received for the research, authorship, and/or publication of this article. Financial support to OB and KS was provided by the European Union through the NECCTON project (New Copernicus Capability for Trophic Ocean Networks; grant agreement 101081273). KS thanks the European Marine Observation and Data Network (EMODnet Thematic Lot n° VI – Biology CINEA/EMFAF/2022/3.5.2/SI2.895681- EMODnet Biology) supported by the Directorate-General for Maritime Affairs and Fisheries (DG MARE).
Acknowledgments
This work was achieved as part of the ICES Working Group on Fisheries Benthic Impact and Trade-off (WGFBIT) based on developments from the Working Group on Biodiversity Science (WGBIODIV). We thank two reviewers for constructive comments on an earlier version of the manuscript.
Conflict of interest
The authors declare that the research was conducted in the absence of any commercial or financial relationships that could be construed as a potential conflict of interest.
The author(s) declared that they were an editorial board member of Frontiers, at the time of submission. This had no impact on the peer review process and the final decision.
Publisher’s note
All claims expressed in this article are solely those of the authors and do not necessarily represent those of their affiliated organizations, or those of the publisher, the editors and the reviewers. Any product that may be evaluated in this article, or claim that may be made by its manufacturer, is not guaranteed or endorsed by the publisher.
Supplementary material
The Supplementary Material for this article can be found online at: https://www.frontiersin.org/articles/10.3389/fmars.2023.1303909/full#supplementary-material
References
Abdi H., Williams L. J., Valentin D. (2013). Multiple factor analysis: principal component analysis for multitable and multiblock data sets. Wiley Interdiscip. Rev. Comput. Stat. 5, 149–179. doi: 10.1002/wics.1246
Agardy T. (2000). Effects of fisheries on marine ecosystems: a conservationist’s perspective. ICES J. Mar. Sci. 57, 761–765. doi: 10.1006/jmsc.2000.0721
Auster P. J., Langton R. W. (1999). The effects of fishing on fish habitat. Am. Fish. Soc Symp. 22, 150–187.
Barry J. P. (1989). Reproductive response of a marine annelid to winter storms: an analog to fire adaptation in plants? Mar. Ecol. Prog. Ser. 54, 99–107. doi: 10.3354/MEPS054099
Beauchard O. (2023). The importance of trait selection on the meaning of functional diversity in benthic studies. Front. Mar. Sci. 10. doi: 10.3389/fmars.2023.1195595
Beauchard O., Brind’Amour A., Schratzberger M., Laffargue P., Hintzen N. T., Somerfield P. J., et al. (2021). A generic approach to develop a trait-based indicator of trawling- induced disturbance. Mar. Ecol. Prog. Ser. 675, 35–52. doi: 10.3354/meps13840
Beauchard O., Mestdagh S., Koop L., Ysebaert T., Herman P. M. J. (2022). Benthic synecology in a soft sediment shelf: habitat contrasts and assembly rules of life strategies. Mar. Ecol. Prog. Ser. 682, 31–50. doi: 10.3354/meps13928
Beauchard O., Thompson M. S. A., Ellingsen K., Piet G. J., Laffargue P., Soetaert K. (2023). Assessing sea floor functional biodiversity and vulnerability. Mar. Ecol. Prog. Ser. 708, 21–43. doi: 10.3354/meps14270
Beauchard O., Veríssimo H., Queirós A. M., Herman P. M. J (2017). The use of multiple biological traits in marine community ecology and its potential in ecological indicator development. Ecol. Indic. 76, 81–96. doi: 10.1016/j.ecolind.2017.01.011
Belley R., Snelgrove P. V. R. (2016). Relative contributions of biodiversity and environment to benthic ecosystem functioning. Front. Mar. Sci. 3. doi: 10.3389/fmars.2016.00242
Bergman M. J. N., van Santbrink J. W. (2000). Mortality in megafaunal benthic populations caused by trawl fisheries on the Dutch continental shelf in the North Sea in 1994. ICES J. Mar. Sci. 57, 1321–1331. doi: 10.1006/jmsc.2000.0917
Bergmann M., Moore P. G. (2001). Mortality of Asterias rubens and Ophiura ophiura discarded in the Nephrops fishery of the Clyde Sea area, Scotland. ICES Mar. Sci. 58, 531–542. doi: 10.1006/jmsc.2001.1046
Bolam S. G., Coggan R. C., Eggleton J., Diesing M., Stephens D. (2014). Sensitivity of macrobenthic secondary production to trawling in the English sector of the Greater North Sea: a biological trait approach. J. Sea Res. 85, 162–177. doi: 10.1016/j.seares.2013.05.003
Callaway R., Desroy N., Dubois S. F., Fournier J., Frost M., Godet L., et al. (2010). Ephemeral bio-engineers or reef-building polychaetes: how stable are aggregations of the tube worm Lanice conChilega (Pallas 1766)? Integr. Comp. Biol. 50, 237–250. doi: 10.1093/icb/icq060
Chessel D., Dufour A. B., Thioulouse J. (2004). The ade4 package-I – One-table methods. R News 4, 5–10.
Chevénet F., Dolédec S., Chessel D. (1994). A fuzzy coding approach for the analysis of long-term ecological data. Freshw. Biol. 31, 295–309. doi: 10.1111/j.1365-2427.1994.tb01742.x
CMEMS (2021). Baltic sea biogeochemistry and physics reanalysis (Marine Data Store (MDS: E.U. Copernicus Marine Service Information (CMEMS). doi: 10.48670/moi-00012and10.48670/moi-00013
Collie J. (2000). Photographic evaluation of the impacts of bottom fishing on benthic epifauna. ICES J. Mar. Sci. 57, 987–1001. doi: 10.1006/jmsc.2000.0584
Crowder L. B., Hazen E. L., Avissar N., Bjorkland R., Latanich C., Ogburn M. B. (2008). The impacts of fisheries on marine ecosystems and the transition to ecosystem-based management. Annu. Rev. Ecol. Evol. Syst. 39, 259–278. doi: 10.1146/annurev.ecolsys.39.110707.173406
Dayton P. (1998). Reversal of the burden of proof in fisheries management. Science 279, 821–822. doi: 10.1126/science.279.5352.821
De Borger E., Braeckman U., Soetaert K. (2021). Rapid organic matter cycling in North Sea sediments. Cont. Shelf Res. 214, 104327. doi: 10.1016/j.csr.2020.104327
De la Torriente Diez A., González-Irusta J. M., Serrano A., Aguilar R., Sánchez F., Blanco M., et al. (2022). Spatial assessment of benthic habitats vulnerability to bottom fishing in a Mediterranean seamount. Mar. Policy 135, 104850. doi: 10.1016/j.marpol.2021.104850
Deng L., Meile C., Fiskal A., Bölsterlia D., Han X., Gajendra N., et al. (2022). Deposit-feeding worms control subsurface ecosystem functioning in intertidal sediment with strong physical forcing. PNAS Nexus 1, 1–16. doi: 10.1093/pnasnexus/pgac146
Dolédec S., Chessel D., Ter Braak C. J. F., Champely S. (1996). Matching species traits to environmental variables: a new three-table ordination method. Environ. Ecol. Stat. 3, 143–166. doi: 10.1007/BF02427859
Dray S., Choler P., Dolédec S., Peres-Neto P. R., Thuiller W., Pavoine S., et al. (2014). Combining the fourth-corner and the RLQ methods for assessing trait responses to environmental variation. Ecology 95, 14–21. doi: 10.1890/13-0196.1
Dray S., Dufour A. B., Chessel D. (2007) The ade4 Package – II: Two-table and K-table methods. R News. Available at: https://cran.r-project.org/doc/Rnews.
Dray S., Legendre P. (2008). Testing the species traits-environment relationships: the fourth-corner problem revisited. Ecology 89, 3400–3412. doi: 10.1890/08-0349.1
Duplisea D. E., Jennings S., Warr K. J., Dinmore T. A. (2002). A size-based model of the impacts of bottom trawling on benthic community structure. Can. J. Fish. Aquat. Sci. 59, 1785–1795. doi: 10.1139/f02-14
Eigaard O. R., Bastardie F., Hintzen N. T., Buhl-Mortensen L., Buhl-Mortensen P., Catarino R., et al. (2017). The footprint of bottom trawling in European waters: distribution, intensity, and seabed integrity. ICES J. Mar. Sci. 74, 847–865. doi: 10.1093/icesjms/fsw194
Garcia C., Solan M., Bolam S. G., Sivyer D., Parker R., Godbold J. A. (2021). Exploration of multiple post-extinction compensatory scenarios improves the likelihood of determining the most realistic ecosystem future. Environ. Res. Commun. 3, 045001. doi: 10.1088/2515-7620/abf468
Godbold J. A., Solan M. (2013). Long-term effects of warming and ocean acidification are modified by seasonal variation in species responses and environmental conditions. Philos. Trans. R. Soc London 368, 1–11. doi: 10.1098/rstb.2013.0186
Grabowski J. H., Bachman M., Demarest C., Eayrs S., Harris B. P., Malkoski V., et al. (2014). Assessing the vulnerability of marine benthos to fishing gear impacts. Rev. Fish. Sci. Aquac. 22, 142–155. doi: 10.1080/10641262.2013.846292
Greenslade P. J. M. (1983). Adversity selection and the habitat templet. Am. Nat. 122, 352–365. doi: 10.1086/284140
Grimaret-Carpentier C., Chessel D., Pascal J. P. (1998). Non-symmetric correspondence analysis: an alternative for species occurrence data. Plant Ecol. 138, 97–112. doi: 10.1023/A:1009708824434
Hermsen J. M., Collie J. S., Valentine P. C. (2003). Mobile fishing gear reduces benthic megafaunal production on Georges Bank. Mar. Ecol. Prog. Ser. 260, 97–108. doi: 10.3354/meps260097
Hiddink J. G., Jennings S., Sciberras M., Szostek C. L., Hughes K. M., Ellis N., et al. (2017). Global analysis of depletion and recovery of seabed biota after bottom trawling disturbance. Proc. Natl. Acad. Sci. U.S.A. 114, 8301–8306. doi: 10.1073/pnas.1618858114
Hill M. O., Smith A. J. E. (1976). Principal component analysis of taxonomic data with multi-state discrete characters. Taxon 25, 249–255. doi: 10.2307/1219449
Howarth L. M., Waggitt J. J., Bolam S. G., Eggleton J., Somerfield P. J., Hiddink J. G. (2018). Effects of bottom trawling and primary production on the composition of biological traits in benthic assemblages. Mar. Ecol. Prog. Ser. 602, 31–48. doi: 10.3354/meps12690
ICES (2021). “OSPAR request on the production of spatial data layers of fishing intensity/pressure,” in In report of the ICES advisory committee 2021. (Denmark:ICES Headquarters). doi: 10.17895/ices.advice.8297
Jennings S., Kaiser M. J. (1998). The effects of fishing on marine ecosystems. Adv. Mar. Biol. 34, 201–352. doi: 10.1016/S0065-2881(08)60212-6
Jones J. B. (1992). Environmental impact of trawling on the seabed: a review. N. Z. J. Mar. Freshw. Res. 26, 59–67. doi: 10.1080/00288330.1992.9516500
Jones C. G., Lawton J. H., Shachak M. (1994). Organisms as ecosystem engineers. Oikos 69, 373–386. doi: 10.2307/3545850
Jones C. G., Lawton J. H., Shachak M. (1997). Positive and negative effects of organisms as physical ecosystem engineers. Ecology 78, 1946–1957. doi: 10.1890/0012-9658(1997)078[1946:PANEOO]2.0.CO;2
Kaiser M. J., Collie J. S., Hall S. J., Jennings S., Poiner I. R. (2002). Modification of marine habitats by trawling activities: prognosis and solutions. Fish Fish. 3, 114–136. doi: 10.1046/j.1467-2979.2002.00079.x
Kindsvater H. K., Mangel M., Reynolds J. D., Dulvy N. K. (2016). Ten principles from evolutionary ecology essential for effective marine conservation. Ecol. Evol. 6, 2125–2138. doi: 10.1002/ece3.2012
Kollmann H., Stachowitsch M. (2001). Long-term changes in the benthos of the northern Adriatic Sea: a phototransect approach. Mar. Ecol. 22, 135–154. doi: 10.1046/j.1439-0485.2001.01761.x
Kondratyeva A., Grandcolas P., Pavoine S. (2019). Reconciling the concepts and measures of diversity, rarity and originality in ecology and evolution. Biol. Rev. Camb. Philos. Soc 94, 1317–1337. doi: 10.1111/brv.12504
Kristensen E., Kostka J. E. (2005). Macrofaunal burrows and irrigation in marine sediment: microbiological and biogeochemical interactions,” in Interactions between macro- and microorganisms in marine sediments. Eds. Kristensen E., Haese R. R., Kostka J. E. (Washington D. C., NW: American Geophysical Union), 125–157. doi: 10.1029/CE060p0125
Kristensen E., Penha-Lopes G., Delefosse M., Valdemarsen T., Quintana C. O., Banta G. T. (2012). What is bioturbation? The need for a precise definition for fauna in aquatic sciences. Mar. Ecol. Prog. Ser. 446, 285–302. doi: 10.3354/meps09506
Laliberté E., Legendre P., Shipley B. (2014) FD: measuring functional diversity from multiple traits, and other tools for functional ecology. Available at: https://cran.r-project.org/web/packages/FD/index.html.
Laverock B., Smith C. J., Tait K., Osborn A. M., Widdicombe S., Gilbert J. A. (2010). Bioturbating shrimp alter the structure and diversity of bacterial communities in coastal marine sediments. ISME J. 4, 1531–1544. doi: 10.1038/ismej.2010.86
Lavorel S., Garnier E. (2002). Predicting changes in community composition and ecosystem functioning from plant traits: revisiting the holy grail. Funct. Ecol. 16, 545–556. doi: 10.1046/j.1365-2435.2002.00664.x
Levin L. A. (1984). Life history and dispersal patterns in a dense infaunal polychaete assemblage: community structure and response to disturbance. Ecology 65, 1185–1200. doi: 10.2307/1938326
McLaverty C., Dinesen G. E., Gislason H., Brooks M. E., Eigaard O. R. (2021). Biological traits of benthic macrofauna show size-based differences in response to bottom trawling intensity. Mar. Ecol. Prog. Ser. 671, 1–19. doi: 10.3354/meps13790
Miatta M., Bates A. E., Snelgrove P. V. R. (2021). Incorporating biological traits into conservation strategies. Annu. Rev. Mar. Sci. 13, 421–443. doi: 10.1146/annurev-marine-032320-094121
Micheli F., Halpern B. S. (2005). Low functional redundancy in coastal marine assemblages. Ecol. Lett. 8, 391–400. doi: 10.1111/j.1461-0248.2005.00731.x
Mouritsen K. N., Mouritsen L. T., Jensen K. T. (1998). Change of topography and sediment characteristics on an intertidal mud-flat following mass-mortality of the amphipod Corophium volutator. J. Mar. Biol. Assoc. UK 78, 1167–1180. doi: 10.1017/S0025315400044404
Murray J. M. H., Meadows A., Meadows P. S. (2002). Biogeomorphological implications of microscale interactions between sediment geotechnics and marine benthos: a review. Geomorphology 47, 15–30. doi: 10.1016/S0169-555X(02)00138-1
Noji C. I. M., Noji T. T. (1991). Tube lawns of spionid polychaetes and their significance for recolonization of disturbed benthic substrates. Meeresforsch. 33, 235–246.
Oksanen J., Blanchet G., Kindt R., Legendre P. (2010) Vegan: community ecology package. Available at: https://cran.r-project.org/package=vegan.
Olsgard F., Schaanning M. T., Widdicombe S., Kendall M. A., Austen M. C. (2008). Effects of bottom trawling on ecosystem functioning. J. Exp. Mar. Biol. Ecol. 366, 123–133. doi: 10.1016/j.jembe.2008.07.036
Pearson T. H., Rosenberg R. (1978). Macrobenthic succession in relation to organic enrichment and pollution of the marine environment. Oceanogr. Mar. Biol. Annu. Rev. 16, 229–311.
Peres-Neto P. R., Dray S., ter Braak C. J. F. (2017). Linking trait variation to the environment: critical issues with community-weighted mean correlation resolved by the fourth-corner approach. Ecography 40, 806–816. doi: 10.1111/ecog.02302
Pitcher C. R., Ellis N., Jennings S., Hiddink J. G., Mazor T., Kaiser M. J., et al. (2017). Estimating the sustainability of towed fishing-gear impacts on seabed habitats: a simple quantitative risk assessment method applicable to data-limited fisheries. Methods Ecol. Evol. 8, 472–480. doi: 10.1111/2041-210X.12705
Pommer C. D., Olesen M., Hansen J. L. S. (2016). Impact and distribution of bottom trawl fishing on mud-bottom communities in the Kattegat. Mar. Ecol. Prog. Ser. 548, 47–60. doi: 10.3354/meps11649
Ramsay K., Kaiser M. J. (1998b). Demersal fishing disturbance increases predation risk for whelks (Buccinum undatum L.). J. Sea. Res. 39, 299–304. doi: 10.1016/S1385-1101(98)00005-7
Posey M., Lindberg W., Alphin T., Vose F. (1996). Influence of storm disturbance on an offshore benthic community. Bull. Mar. Sci. 59, 523–529.
Ramsay K., Kaiser M. J., Hughes R. N. (1998a). Responses of benthic scavengers to fishing disturbance by towed gears in different habitats. J. Exp. Mar. Biol. Ecol. 224, 73–89. doi: 10.1016/S0022-0981(97)00170-6
Ramsay K., Kaiser M. J., Moore P. G., Hughes R. N. (1997). Consumption of fisheries discards by benthic scavengers: utilization of energy subsidies in different marine habitats. J. Anim. Ecol. 66, 884–896. doi: 10.2307/6004
Rao C. R. (1982). Diversity and dissimilarity coefficients: a unified approach. Theor. Popul. Biol. 21, 24–43. doi: 10.1016/0040-5809(82)90004-1
R Core Team (2023). R: a language and environment for statistical computing (Vienna: R Foundation for Statistical Computing). Available at: https://www.R-project.org.
Rhoads D. C., Boyer L. F. (1982). “The effects of marine benthos on physical properties of sediments,” in Animal-sediment relations – the biogenic alteration of sediments. Eds. McCall P. L., Tevesz M. J. S. (New York and London: Academic Press), 3–52.
Rijnsdorp A. D., Bolam S. G., Garcia C., Hiddink J. G., Hintzen N. T., van Denderen P. D., et al. (2018). Estimating sensitivity of seabed habitats to disturbance by bottom trawling based on the longevity of benthic fauna. Ecol. Appl. 28, 1302–1312. doi: 10.1002/eap.1731
Robert A. (2017). Combined effects of natural and anthropogenic factors on benthic invertebrate communities of Norway lobster mudflats (Nephrops Norvegicus) in the Bay of Biscay. PhD thesis. (European University of Brittany, Rennes). Available at: https://archimer.ifremer.fr/doc/00611/72341/.
Rosenfeld J. S. (2002). Functional redundancy in ecology and conservation. Oikos 98, 56–162. doi: 10.1034/j.1600-0706.2002.980116.x
Sciberras M., Hiddink J. G., Jennings S., Szostek C. L., Hughes K. M., Kneafsey B., et al. (2018). Response of benthic fauna to experimental bottom fishing: a global meta-analysis. Fish Fish. 19, 698–715. doi: 10.1111/faf.12283
Snelgrove P. V. R., Thrush S. F., Wall D. H., Norkko A. (2014). Real world biodiversity–ecosystem functioning: a seafloor perspective. Trends Ecol. Evol. 29, 398–405. doi: 10.1016/j.tree.2014.05.002
Solan M., Bradley J., Cardinale B. J., Downing A. L., Engelhardt K. A. M., Ruesink J. L., et al. (2004). Extinction and ecosystem function in the marine benthos. Science 306, 1177–1180. doi: 10.1126/science.1103960
Thompson C. E. L., Silburn B., Williams M. E., Hull T., Sivyer D., Amoudry L. O., et al. (2017). An approach for the identification of exemplar sites for scaling up targeted field observations of benthic biogeochemistry in heterogeneous environments. Biogeochemistry 135, 1–34. doi: 10.1007/s10533-017-0366-1
Thrush S. F., Dayton P. K. (2002). Disturbance to marine benthic habitats by trawling and dredging: implications for marine biodiversity. Annu. Rev. Ecol. Evol. Syst. 33, 449–473. doi: 10.1146/annurev.ecolsys.33.010802.150515
Thrush S. F., Hewitt J. E., Cummings V. J., Dayton P. K., Cryer M., Turner S. J., et al. (1998). Disturbance of the marine benthic habitat by commercial fishing: impacts at the scale of the fishery. Ecol. Appl. 8, 866–879. doi: 10.1890/1051-0761(1998)008[0866:DOTMBH]2.0.CO;2
Tiano J. C., Depestele J., Van Hoey G., Fernandes J., van Rijswijk P., Soetaert K. (2022). Trawling effects on biogeochemical processes are mediated by fauna in high-energy biogenic-reef-inhabited coastal sediments. Biogeosciences 19, 2583–2598. doi: 10.5194/bg-19-2583-2022
Tiano J. C., van der Reijden K. J., O’Flynn S., Beauchard O., van der Ree S., van der Wees J., et al. (2020). Experimental bottom trawling finds resilience in large-bodied infauna but vulnerability for epifauna and juveniles in the Frisian Front. Mar. Environ. Res. 159, 104964. doi: 10.1016/j.marenvres.2020.104964
Tillin H. M., Hiddink J. G., Jennings S., Kaiser M. J. (2006). Chronic bottom trawling alters the functional composition of benthic invertebrate communities on a sea-basin scale. Mar. Ecol. Prog. Ser. 318, 31–45. doi: 10.3354/meps318031
Tsikopoulou I., Smith C. J., Papadopoulou K. N., Austen M. C. (2022). Linking species functional traits to specific biogeochemical processes under trawling pressure. Biology 11, 1378. doi: 10.3390/biology11101378
Tyler-Walters H., Roger S. I., Marshall C. E., Hiscock K. (2009). A method to assess the sensitivity of sedimentary communities to fishing activities. Aquat. Conserv. 19, 285–300. doi: 10.1002/aqc.965
van Denderen P. D., Bolam S. G., Hiddink J. G., Jennings S., Kenny A., Rijnsdorp A. D., et al. (2015). Similar effects of bottom trawling and natural disturbance on composition and function of benthic communities across habitats. Mar. Ecol. Prog. Ser. 541, 31–43. doi: 10.3354/meps11550
Villéger S., Novack-Gottshall P. M., Mouillot D. (2011). The multidimensionality of the niche reveals functional diversity changes in benthic marine biotas across geological time. Ecol. Lett. 14, 561–568. doi: 10.1111/j.1461-0248.2011.01618.x
Villnäs A., Hewitt J., Snickars M., Westerbom M., Norkko A. (2017). Template for using biological trait groupings when exploring large-scale variation in seafloor multifunctionality. Ecol. Appl. 28, 78–94. doi: 10.1002/eap.1630
Violle C., Jiang L. (2009). Towards a trait-based quantification of species niche. J. Plant Ecol. 2, 87–93. doi: 10.1093/jpe/rtp007
Vitaliano J., Packer D., Reid R., Guida V. (2013). Broad_scale, dense amphipod tube aggregations on the sea bed: implications for resource species that utilize benthic habitats. Fish. Oceanogr. 22, 61–67. doi: 10.1111/fog.12003
Weiher E., Keddy P. A. (1995). Assembly rules, null models, and trait dispersion: new questions from old patterns. Oikos 74, 159–164. doi: 10.2307/3545686
Wesuls D., Oldeland J., Dray S. (2011). Disentangling plant trait responses to livestock grazing from spatio-temporal variation: the partial RLQ approach. J. Veg. Sci. 23, 98–113. doi: 10.1111/j.1654-1103.2011.01342.x
Wild C., Røy H., Huettel M. (2005). Role of pelletization in mineralization of fine-grained coastal sediments. Mar. Ecol. Prog. Ser. 291, 23–33. doi: 10.3354/meps291023
Wilson J. B. (2012). Species presence/absence sometimes represents a plant community as well as species abundances do, or better. J. Veg. Sci. 23, 1013–1023. doi: 10.1111/j.1654-1103.2012.01430.x
Woodin S. A., Volkenborn N., Pilditch C. A., Lohrer A. M., Wethey D. S., Hewitt J. D., et al. (2016). Same pattern, different mechanism: Locking onto the role of key species in seafloor ecosystem process. Sci. Rep. 7, 43695. doi: 10.1038/srep26678
Keywords: benthic invertebrate, effect trait, ecosystem function, bottom trawling, vulnerability, functional niche breadth, functional diversity
Citation: Beauchard O, Bradshaw C, Bolam S, Tiano J, Garcia C, De Borger E, Laffargue P, Blomqvist M, Tsikopoulou I, Papadopoulou NK, Smith CJ, Claes J, Soetaert K and Sciberras M (2023) Trawling-induced change in benthic effect trait composition – A multiple case study. Front. Mar. Sci. 10:1303909. doi: 10.3389/fmars.2023.1303909
Received: 28 September 2023; Accepted: 06 December 2023;
Published: 22 December 2023.
Edited by:
Elva G. Escobar-Briones, National Autonomous University of Mexico, MexicoReviewed by:
Peter J. Auster, Mystic Aquarium, United StatesFelipe Amezcua, National Autonomous University of Mexico, Mexico
Copyright © 2023 Beauchard, Bradshaw, Bolam, Tiano, Garcia, De Borger, Laffargue, Blomqvist, Tsikopoulou, Papadopoulou, Smith, Claes, Soetaert and Sciberras. This is an open-access article distributed under the terms of the Creative Commons Attribution License (CC BY). The use, distribution or reproduction in other forums is permitted, provided the original author(s) and the copyright owner(s) are credited and that the original publication in this journal is cited, in accordance with accepted academic practice. No use, distribution or reproduction is permitted which does not comply with these terms.
*Correspondence: Olivier Beauchard, b2xpdmllci5iZWF1Y2hhcmRAbmlvei5ubA==