- 1Key Laboratory of Tropical Marine Bio-resources and Ecology, South China Sea Institute of Oceanology, Chinese Academy of Sciences, Guangzhou, China
- 2Southern Marine Science and Engineering Guangdong Laboratory (Guangzhou), Guangzhou, China
- 3Sanya Institute of Ocean Eco-Environmental Engineering, Hainan, China
- 4Nansha Marine Ecological and Environmental Research Station, Chinese Academy of Sciences, Sansha, China
- 5Guangdong Provincial Key Laboratory of Applied Marine Biology, Guangzhou, China
- 6Marine Biology Research Station at Daya Bay, Chinese Academy of Sciences, Shenzhen, China
Coral atolls are widely recognized as oases of remarkable biodiversity and productivity within the oligotrophic open ocean. However, considerable debate surrounds the net carbon metabolism of planktonic communities in oceanic coral atolls and their contribution to the overall carbon budget of the ocean. Zhongsha Atoll, situated in the central South China Sea, represents one of the largest submerged reef atolls globally. Despite its significance, the ecological environment and characteristics of community production and metabolism at Zhongsha Atoll have been scarcely studied, and it remains uncertain whether these features differ from those observed in barrier-type reefs. In this study, we examined the gross primary production (GPP), community respiration (CR), and net community production (NCP) of the planktonic community in Zhongsha Atoll and its surrounding waters from 22 June to 6 July 2020. We also analyzed the potential influences of their distribution patterns. Our findings revealed that CR did not vary significantly with depth and it was considerably higher than GPP. As a result, the waters at the euphotic depth of Zhongsha Atoll were found to be heterotrophic, with negative NCP. Additionally, the correlation analysis demonstrated a negative relationship between NCP and CR. The NCP values were -35.28 ± 26.73 and -53.18 ± 31.77 mg C m-3 d-1 for the surface and chlorophyll maximum (DCM) layers, respectively. The NCP for the upper water column was -3023 mg C m-2 d-1. The waters above the reef flat (FL) exhibited higher primary productivity, with GPP in the surface and DCM layer being 1.61 and 2.71 times greater, respectively, than in the surrounding oceanic regions. However, the FL displayed a greater level of heterotrophy due to its stronger CR. In conclusion, the carbon metabolism of the planktonic community in submerged Zhongsha Atoll acts as a source of atmospheric CO2, and the distribution of coral reefs considerably contributes to the efficiency of carbon cycling within the atoll.
1 Introduction
Coral reefs have been described as oases of biodiversity and productivity in oligotrophic oceans, covering less than 0.1% of the ocean surface (Spalding et al., 2001), but providing habitat for 25% of marine species and are valued at more than $375 billion annually through the promotion of tourism, fisheries and marine industries (Worm et al., 2006). The ecosystems are also hotspots for marine carbon sinks. The role of coral reefs in the carbon budget is of great importance in light of current climate challenges and the enormous potential for carbon storage in the oceans (Hoegh-Guldberg et al., 2017; Zhou et al., 2023).
However, the contribution of oceanic coral atolls to the source/sink of atmospheric CO2 has been controversial (Gattuso et al., 1999). The balance of the carbon budget is jointly regulated by the two major processes of primary production and respiratory metabolism. Although coral reef ecosystems have a strong carbon sequestration function through the photosynthesis of symbiotic zooxanthellae and phytoplankton, and the formation of coral calcium carbonate skeletons, the respiratory and metabolic processes of the ecosystems are also active due to the high biodiversity and rapid organic matter turnover rates (Tanaka et al., 2018). Metabolic carbon emission processes mainly include respiration and excretion by plankton, corals and benthos, carbon dioxide released by coral calcification, and microbial metabolic processes associated with the decomposition of organic matter (Crossland et al., 1991; Sorokin, 1991; Ware et al., 1992). In previous studies, carbon sink assessment has been primarily based on phytoplankton biomass and carbon isotope conversion efficiency (Azevedo et al., 2006; Duarte and Regaudie-De-Gioux, 2009). Indeed, it is necessary to take into account the carbon emitted during metabolic processes to further determine the net carbon production (Tambutté et al., 2011).
The production and metabolism of the planktonic community is of great importance for the material cycling and energy flow in coral reef ecosystems. Numerous studies have observed high plankton biomass and productivity in the vicinity of coral atolls, even radiating outwards for tens of kilometers (Gove et al., 2016; Richardson, 2019). Particularly for submerged atolls, the water column is an important component within the boundary of the coral reef ecosystem and contributes significantly to the carbon sink. Furthermore, plankton is critical to the nutrient supply of coral reef ecosystems (Wyatt et al., 2010). Particulate organic matter derived from plankton and its cellular metabolites is primarily fed by filter feeders and larvae in coral reef ecosystems (Ribes et al., 2005; Pawlik et al., 2018), and regulates benthic communities after sedimentation (Furnas et al., 2005). On the other hand, marine plankton are valuable indicators of environmental stability because they respond quickly to environmental changes due to their short-life cycle and rapid carbon turnover rate (Furnas et al., 2005; Glacio Souza et al., 2022). Therefore, planktonic metabolism plays a pivotal role in the carbon pump of coral atolls by influencing the carbon budget of the ecosystem, and the balance between production and metabolism is effective in assessing the stability and health of the ecosystem (Bell et al., 2014; Ke et al., 2016; Xiang et al., 2022).
The global coral reef ecosystem is currently confronted with various crises, exhibiting diverse types and extents of damage. Extensive research has been conducted on the detrimental effects of human activities and terrestrial inputs on nearshore coral reefs. However, an alarming deficiency exists in terms of scientific investigation focusing on coral reefs situated far offshore (Liu et al., 2020; Garcia-Corral et al., 2021). Although far offshore coral atolls are relatively isolated from terrestrial disturbances, they are not immune to ecological damage. The impacts of global climate change, including reduced coral cover and coral bleaching, are still evident in these remote ecosystems (Chen et al., 2009; Liu et al., 2020; Garcia-Corral et al., 2021). The South China Sea is one of the world’s largest marginal seas. Its total area of coral reefs is nearly 5% of the world’s (Huang et al., 2014), mainly distributed in the Nansha Islands, Xisha Islands, Dongsha Islands, Zhongsha Islands, Hainan Island and the South China mainland along the coast. Several studies conducted on offshore coral atolls in the South China Sea have highlighted notable differences in the ecological environment of the reef flats and lagoons within these atolls compared to the external surroundings. These investigations have reported high levels of primary productivity within the reef flats and lagoons (Ke et al., 2018; Fan et al., 2021). However, it is important to note that these atolls typically possess enclosed and relatively shallow lagoons, but the lagoon of Zhongsha Atoll is distinct in that it is encompassed by intermittent marginal reefs with water depths reaching up to 100 m (Huang et al., 2020). Therefore, Zhongsha Atoll has unique geographical structure and environmental characteristics. However, there is a scarcity of studies that delve into its ecological environment and the carbon metabolism of its planktonic community. Consequently, the potential contribution of its metabolic equilibrium to the marine carbon sink/source remains unclear.
In light of these considerations, this study employed field observations and experiments to comprehensively analyze the distribution patterns, regional variations, and influencing factors related to gross primary production (GPP), community respiration (CR), and net community production (NCP) of the plankton community within Zhongsha Atoll and its surrounding waters. These analyses were conducted after establishing a clear understanding of the fundamental ecological environment characteristics and distribution patterns. Moreover, the carbon metabolic flux of the plankton community in Zhongsha Atoll was assessed, aiming to ascertain its potential contribution to the overall carbon source-sink dynamics within the ecosystem.
2 Materials and methods
2.1 Study area and sample collection
Zhongsha Atoll is located in the center of the South China Sea, which is the main component of the Zhongsha Islands and the largest coral atoll among the various islands and reefs in the South China Sea. It is characterized by an oval shape with a NE-SW orientation and covers a total area of 23500 km2. An attribute of Zhongsha Atoll is its submerged nature, with approximately 20 reefs scattered throughout the area. These reefs, ranging from 10 to 26 m below the sea level, remain unexposed even during low tide. The lagoon within the atoll exhibits a water depth of ~70 m (Huang et al., 2020).
Seawater samples from Zhongsha Atoll (113.50°~115.20° E and 15.00°~16.50° N) and its adjacent waters were collected during the period of June 22 to July 6, 2020 for environmental and biological analysis (Figure 1). All samples were collected during the daytime. According to the geographical locations and depths of various sampling stations, the stations were classified into four categories: reef flats (FL), lagoon (LA), outer reef slopes (SL), and deep-sea regions (DS). The bottom depth was less than 50 m for FL, while for LA and SL, it averaged at 80 m and 280 m respectively. The DS had a depth exceeding 500 m.
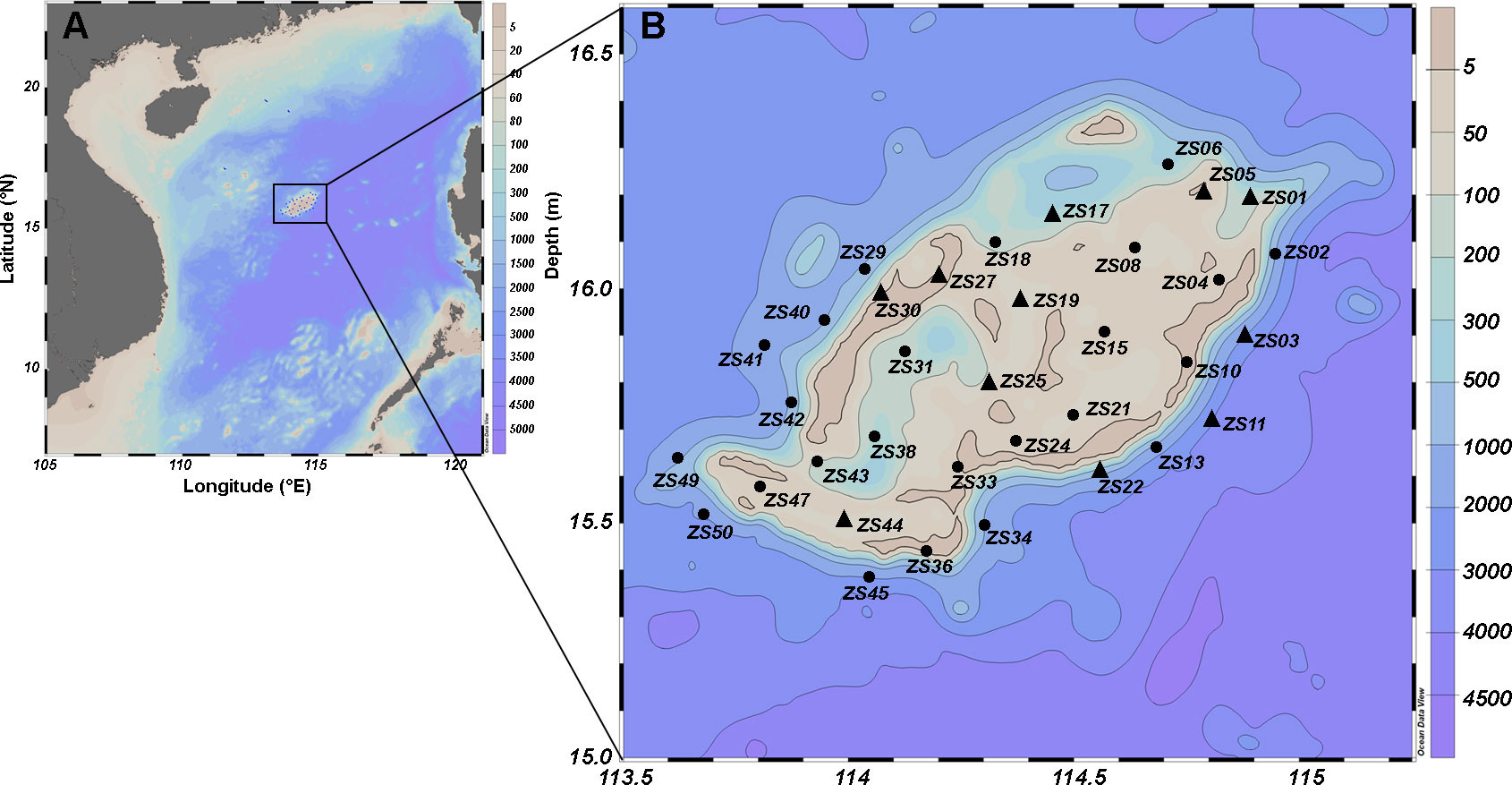
Figure 1 Map of the Zhongsha Atoll (A) in the central South China Sea (B), showing the location of sampling stations.
2.2 Environments and chlorophyll a measurements
The vertical profiles of temperature, salinity, and dissolved oxygen (DO) were monitored in situ by a multi-parameter water quality monitor Sonde (YSI 6600; Yellow Springs Instruments, Yellow Springs, OH, USA). The euphotic depth was estimated by Secchi disk (Koenings and Edmundson, 1991). Concentrations of nitrate (NO3-), nitrite (NO2-), ammonia (NH4+), silicate (SiO32-) and soluble reactive phosphorus (SRP) were measured with an automatic nutrient analyzer (AA3; Seal Instruments, Hamburg, Germany) according to the standard colorimetric techniques (Grasshoff et al., 1983). The dissolved inorganic nitrogen (DIN) content was calculated as the sum of NO3-, NO2-and NH4+.
For the size-fractioned chlorophyll a (Chl a) measurement, 500 mL water samples (prefiltered through a 200 μm mesh) were sequentially filtered through 20 μm nylon membrane (Millipore), 3 μm polycarbonate membrane (Millipore), and 0.7 μm (GF/F filter, Whatman) pore size membrane filters to collect filter samples for micro-, nano- and pico-sized Chl a, respectively. To measure the total Chl a, water samples (prefiltered through a 200 μm mesh) were filtered through 0.7 μm pore size membrane filters directly. All the filters were stored at -20°C, then measured by Turner Designs 10 fluorometer after extracting in the dark for 24 h at 4°C in 10 ml acetone (90% v/v) following the method of Parsons et al. (1984).
2.3 Planktonic community metabolism and primary production
The euphotic zone, where all photosynthesis takes place, plays a crucial role in controlling oceanic primary productivity and air-sea CO2 exchange. The water column integrated primary production, extending from the surface to the deep chlorophyll maximum (DCM) layer, typically constitutes 30-70% of the total carbon fixation within the euphotic zone (Hanson et al., 2007; Macías et al., 2014; Siswanto et al., 2005; Takahashi and Hori, 1984). In this study, based on the in situ observation and previous research of Nansha Islands (Cai et al., 2000; Wu et al., 2001), we determined the planktonic metabolism of the surface and the DCM layer to characterize the metabolic dynamics of Zhongsha Atoll (Figure 1B, stations marked by triangles).
To estimate the planktonic metabolism, we employed the light-dark bottle method (Serret et al., 1999). Specifically, seawater was collected from the surface and DCM layers by Niskin water sampler, then carefully transferred through silicon tubing into a total of six bottles: three designated as light bottles and three as dark bottles (light bottle covered by aluminum foil). These bottles were 340 mL narrow-mouth borosilicate glass bottles. All incubated bottles were carefully arranged in a culture tank on the deck, ensuring temperature control through the use of a chiller. In order to simulate the solar radiation corresponding to the sampling water depths in the natural environment, neutral grey blackout fabric was utilized.
The dissolved oxygen concentrations were measured at the start (0 h) and after a 24-hour incubation period using the Presens precision sensing Microx 4 Oxygen meter, with a detection limit of 0.01 mg L-1 and a detection accuracy of ±0.025 mg L-1. Gross primary production (GPP), community respiration (CR), and net community production (NCP) were calculated as follows: CR (mg O2 L−1 d−1) = DOD0h − DOD24h; NCP (mg O2 L−1 d−1) = DOL24h − DOL0h; GPP (mg O2 L−1 d−1) =NCP+CR= DOD0h+ DOL24h − DOD24h − DOL0h. The DOD0h and DOD24h are used to denote the DO concentrations at the beginning (0 h) and after a 24-hour incubation period in the dark bottles, respectively. Similarly, DOL24h and DOL0h represent the DO concentrations at the beginning (0 h) and after a 24-hour incubation period in the light bottles, respectively. Gross primary productivity (GPP), community respiration (CR), and net community production (NCP) were quantified in carbon units, with the conversion based on a photosynthetic quotient (PQ) of 1.3 and a respiratory quotient (RQ) of 1 (Gazeau et al., 2005; Hopkinson and Smith, 2005).
2.4 Data analysis
The depth-integrated Chl a concentration and planktonic metabolism were calculated as follows:
where ci is the concentration of Chl a (or community metabolism parameters including GPP, CR and NCP) in the ith depth, zi is the sample depth of ci (Estrada et al., 1993).
The map and contour figures were constructed using the software Surfer 11.0 (Golden Software Inc., Golden, CO, USA). The map of sampling stations and spatial distribution plots were drawn using Ocean Data View (Schlitzer, 2020). Pearson’s correlations between variables were analyzed using PASW Statistics for Windows (v18.0, SPSS Inc.). The planktonic metabolism, environmental and biological parameters were subjected to redundancy analysis (RDA) via CANOCO 5.0 software (Microcomputer Power, Ithaca, New York, USA).
3 Results
3.1 Environmental variables
The water temperature in Zhongsha atoll exhibited a distinct vertical pattern, with higher temperatures observed at the surface (30.67 ± 0.31°C) and lower temperatures in deeper layers (dropping to 26.12 ± 1°C at 75m, as shown in Figures 2, S1). Spatial variability was evident at 75 m depth, with temperature differences exceeding 4°C between different stations. The salinity displayed an opposite trend to temperature, with lower values observed at the surface (34.08 ± 0.04°C) and higher values in deeper layers (34.32 ± 0.11 at 75 m). Similar spatial heterogeneity was observed in salinity, particularly in the deeper layers where a difference of 0.4 (ranging from 34.18 to 34.58) was observed at 75 m depth, compared to a difference of 0.23 (ranging from 33.92 to 34.15) at the surface.
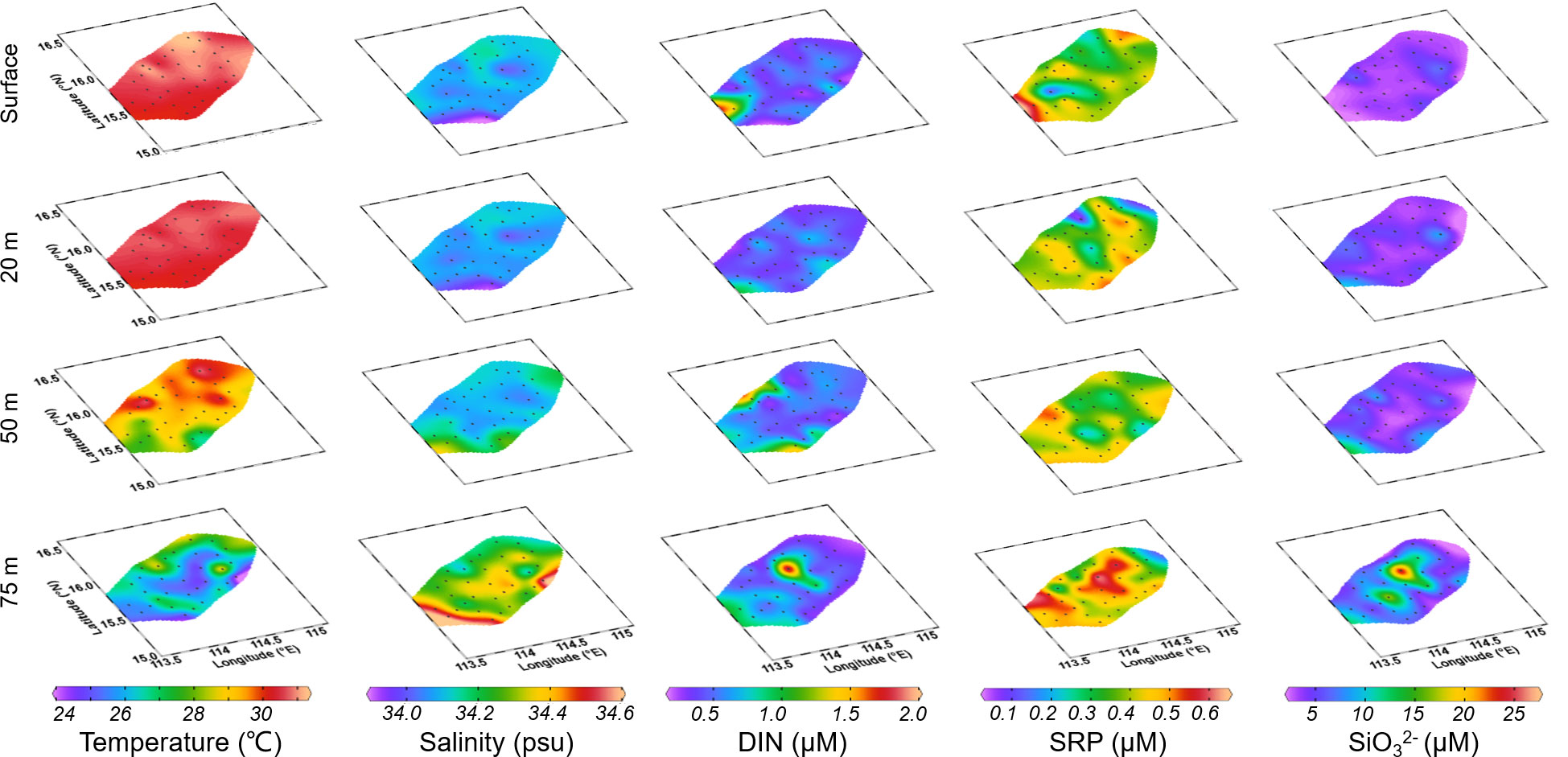
Figure 2 Distribution of temperature (°C), salinity, dissolved inorganic nitrogen (DIN, μM), soluble reactive phosphorus (SRP, μM) and silicate (SiO32-, μM) in the surface and 20, 50, 75 m depth layer.
The distribution pattern of inorganic nutrient concentration was similar to that of salinity, which were distributed in a uniform pattern with lower concentrations in the mixed layer, but concentrations increased in the deeper water layer (75 m depth). The concentrations of DIN, SRP and SiO32- in the surface layer were 0.5 ± 0.22, 0.38 ± 0.09 and 3.69 ± 1.00 μmol L-1, respectively, and increased to 0.6 ± 0.32, 0.46 ± 0.09 and 8.07 ± 4.58 μmol L-1, respectively, at 75 m depth. Notably, within the lagoon, nutrient concentrations were observed to be higher than those outside the atoll, particularly at a depth of 75 m.
3.2 Phytoplankton biomass and size structure
The average Chl a concentration at the surface, 20 m, and 50 m depths were 0.15 ± 0.05, 0.17 ± 0.08, and 0.27 ± 0.16 μg L-1, respectively. The concentration increased to 0.56 ± 0.23 μg L-1 at the DCM (75 m depth, Figure S1). The high concentration of Chl a corresponded to the low temperature and high salinity in the center of Zhongsha Atoll (Figure 3).
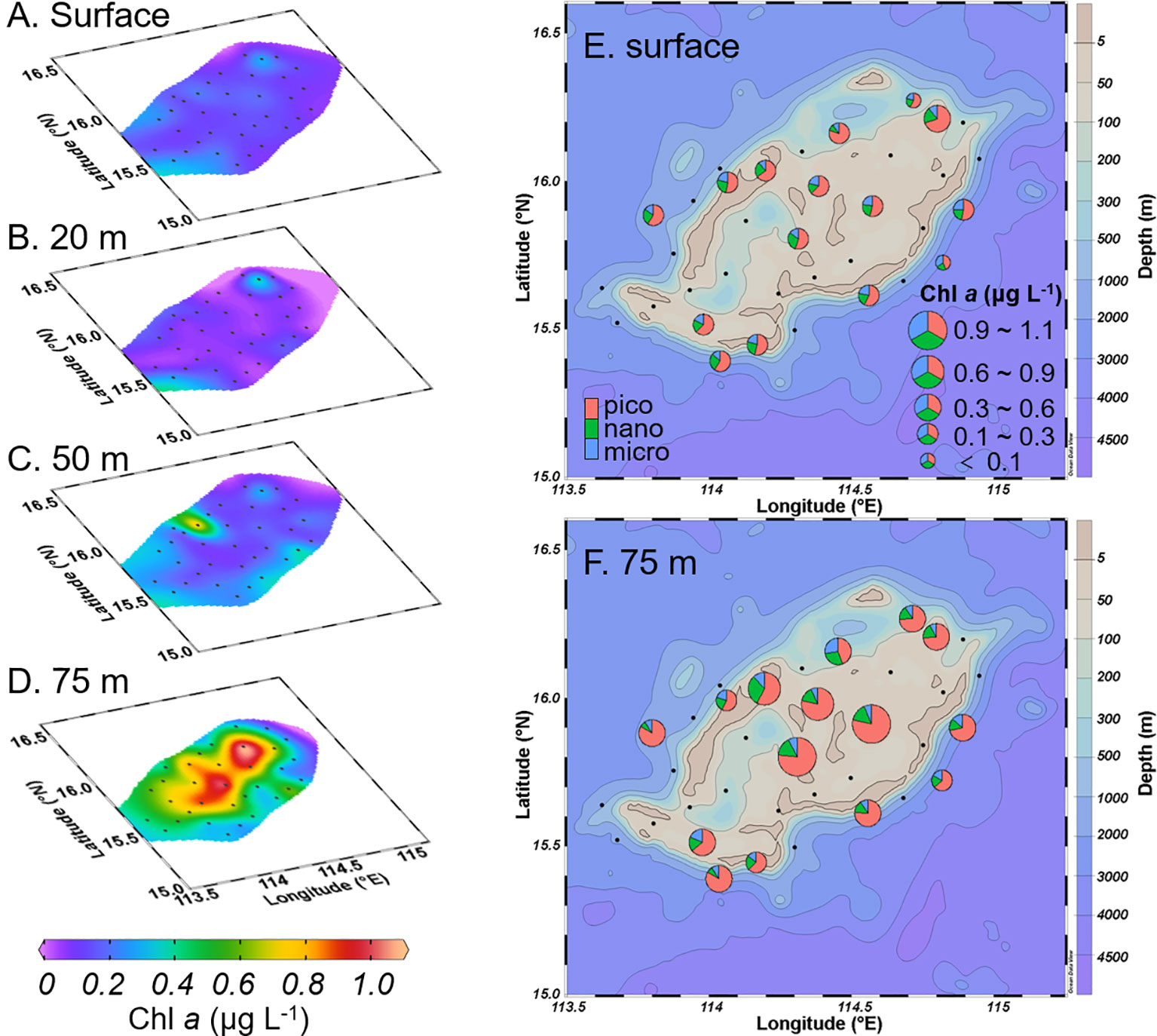
Figure 3 Distribution of chlorophyll a (Chl a, μg L-1) at surface (A), 20 m (B), 50 m (C) and 75 m (D) depth layers, and its size structure at surface (E) and 75 m (F) depth layers. Notice, the (E, F) share the same legend.
The size-fractionated Chl a analysis revealed that the phytoplankton biomass was predominantly composed of pico-sized cells, followed by the nano-size fraction. At the surface, the contribution of the pico-fraction to the total biomass ranged from 44.2% to 79.9% (with an average of 59.0 ± 8.0%). Notably, the waters outside the atoll exhibited a greater contribution from picoplankton compared to the regions inside the atoll. In the DCM layer, the picoplankton size fraction accounted for 44.5% to 83.6% (with an average of 69.8 ± 10.7%) of the total biomass, with the highest composition of pico-sized cells observed in the region within the atoll.
The depth-integrated Chl a from the surface to the DCM layer exhibited a comparable distribution and size-fraction pattern to the Chl a concentration in the DCM layer (Figure 4). Notably, a higher concentration of Chl a was found within the atoll, with picoplankton making a substantial contribution. The pico-fraction was the prominent component of the integrated Chl a, accounting for 55.1% to 76.1% (with an average of 67.6 ± 6.8%).
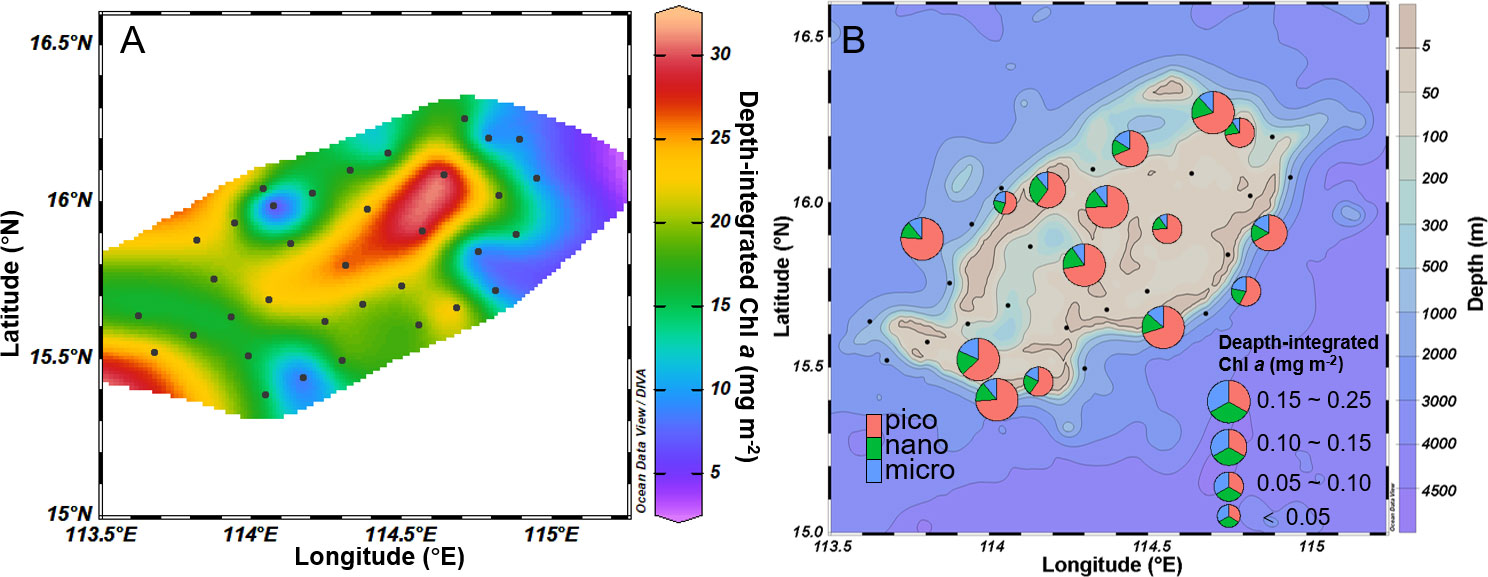
Figure 4 Depth-integrated chlorophyll a (mg m-2) (A) and its size structure (B) from surface to 75 m deep layer.
3.3 Planktonic community metabolism and primary production
The planktonic community production and respiratory metabolism exhibited distinct variations with respect to depth (Figure 5). Gross primary production (GPP) decreased significantly (F=4.54, p< 0.05) as depth increased, with the surface exhibiting a significantly higher productivity (32.6 ± 16.8 mg C m-3 d-1) compared to the DCM layer (16.4 ± 18.6 mg C m-3 d-1). Conversely, there was no significant difference in community respiration (CR) between the surface (68.3 ± 29.2 mg C m-3 d-1) and DCM layer (69.6 ± 36.0 mg C m-3 d-1). Both the surface and DCM layers exhibited significantly higher CR than GPP (F=12.98, p<0.01 and F=18.91, p<0.001, respectively), indicating an overall heterotrophic status of the NCP in Zhongsha Atoll, which averaged -35.3 ± 26.7 and -53.2 ± 31.8 mg C m-3 d-1 in surface waters and the DCM layer, respectively, while the integrated NCP for the entire water column was -3023 ± 1666 mg C m-2 d-1.
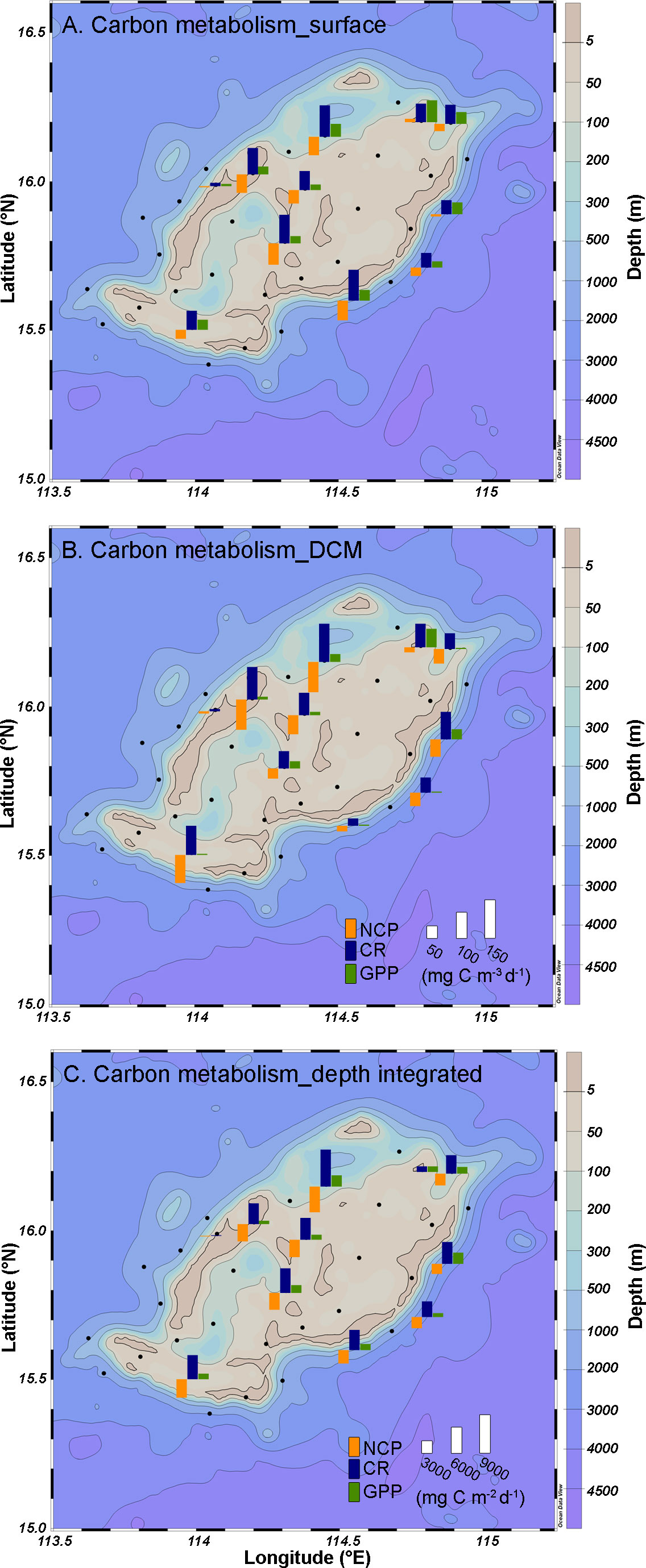
Figure 5 The carbon metabolism of net community production (NCP), community respiration (CR) and gross primary production (GPP) at surface (A), subsurface chlorophyll maximum layer (B) and depth integrated (C). Notice, the (A, B) share the same legend and scale.
The contributions of community production and respiratory metabolism to NCP were examined using correlation analysis (Figure 6). The inverse correlation of CR with NCP was highly significant in the surface (Pearson correlation coefficient R2 = 0.669, p<0.01) and more significant in the DCM layer (Pearson correlation coefficient R2 = 0.737, p<0.001). However, no significant correlation was found between GPP and NCP, neither in the surface nor in the DCM layer. RDA was conducted to elucidate the associations between community metabolic parameters and environmental as well as biological variables (Figure 7). The results showed that the first axis of RDA accounted for the largest proportion of data interpretation, and NCP and CR always pointed to both ends of RDA axis 1, indicating a strong negative correlation between NCP and CR, especially at the DCM layer. In addition, the total Chl a was closely related to GPP at the surface, whereas it was more related to CR in the depth-integrated results.
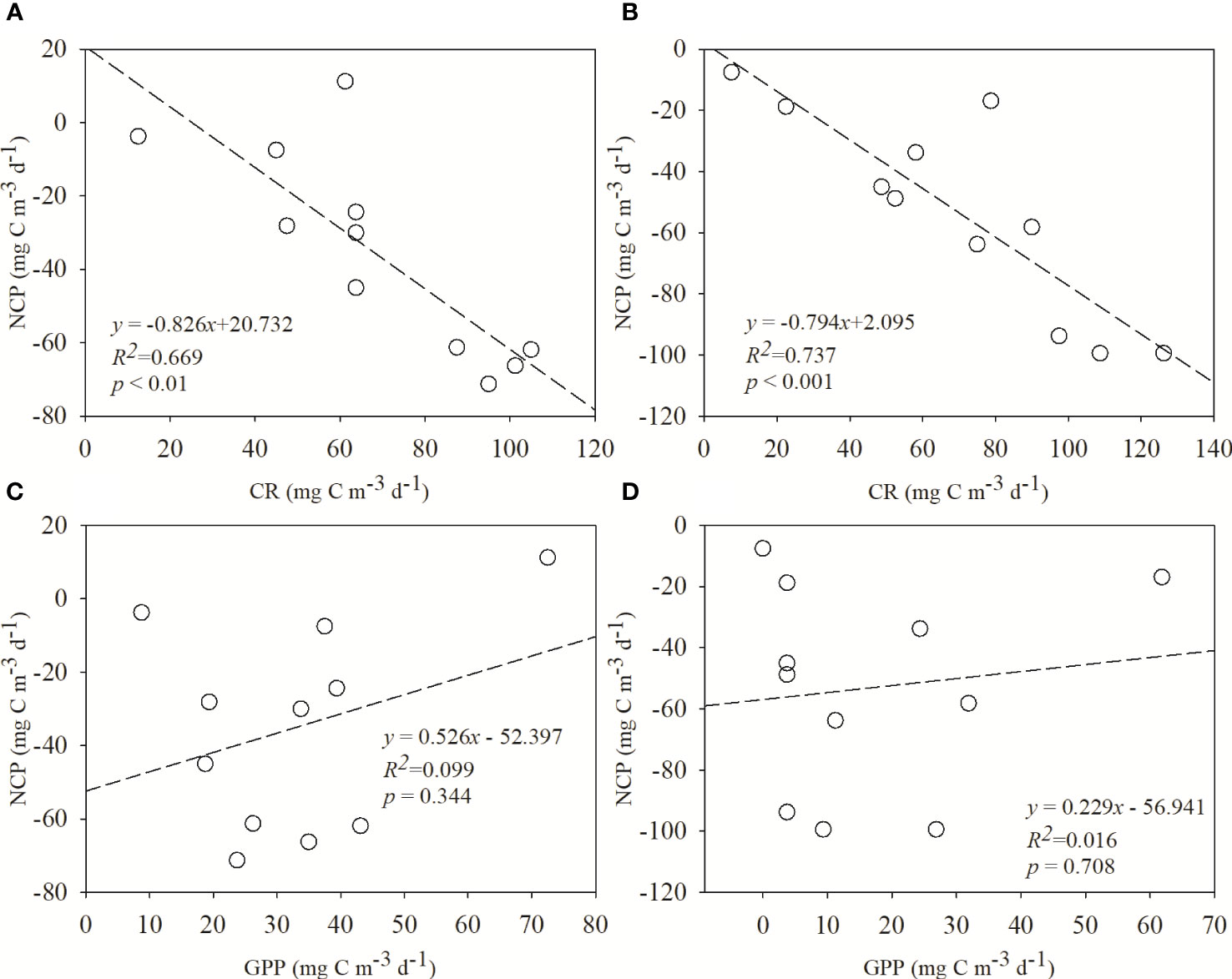
Figure 6 Regression analysis of net community productivity (NCP) with community respiration (CR) (A, B) or gross primary production (GPP) (C, D) at surface (A, C) and deep chlorophyll maximum (DCM) layers (B, D).
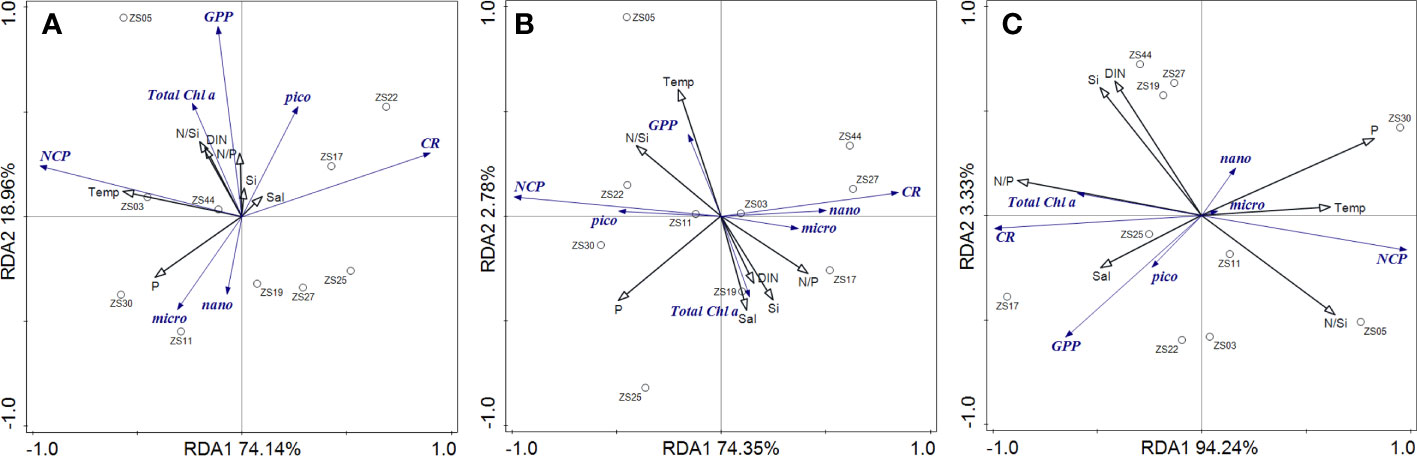
Figure 7 Redundancy analysis (RDA) of planktonic metabolism and environmental and biological factors at surface (A), deep chlorophyll maximum layer (B) and depth integrated (C).
The planktonic community metabolism varied among different regions of the Zhongsha Atoll (Figure 8). The primary production and metabolic rates were relatively high in both FL and SL regions. Specifically, GPP was highest in FL, measuring 49.4 ± 23.1 mg C m-3 d-1. This value was 1.61 times greater than that recorded in the DS region (30.6 ± 8.0 mg C m-3 d-1). In contrast, the LA region exhibited the lowest GPP of 25.4 ± 6.2 mg C m-3 d-1. Additionally, the surface NCP was the highest in the FL region, with an average value of -25 ± 36.3 mg C m-3 d-1. Furthermore, in the depth of the DCM layer, FL demonstrated the highest GPP of 35.6 ± 26.3 mg C m-3 d-1. This value was remarkably 2.7 times higher than that observed in DS (13.1 ± 13.3 mg C m-3 d-1). The GPP values in SL and LA were quite similar, measuring 15.3 ± 11.6 mg C m-3 d-1 and 13.1 ± 8.5 mg C m-3 d-1, respectively. FL also exhibited the highest CR rate in the DCM layer, with a value of 93.8 ± 15 mg C m-3 d-1. Consequently, the NCP in FL demonstrated a significant negative value of -58.1 ± 41.3 mg C m-3 d-1.
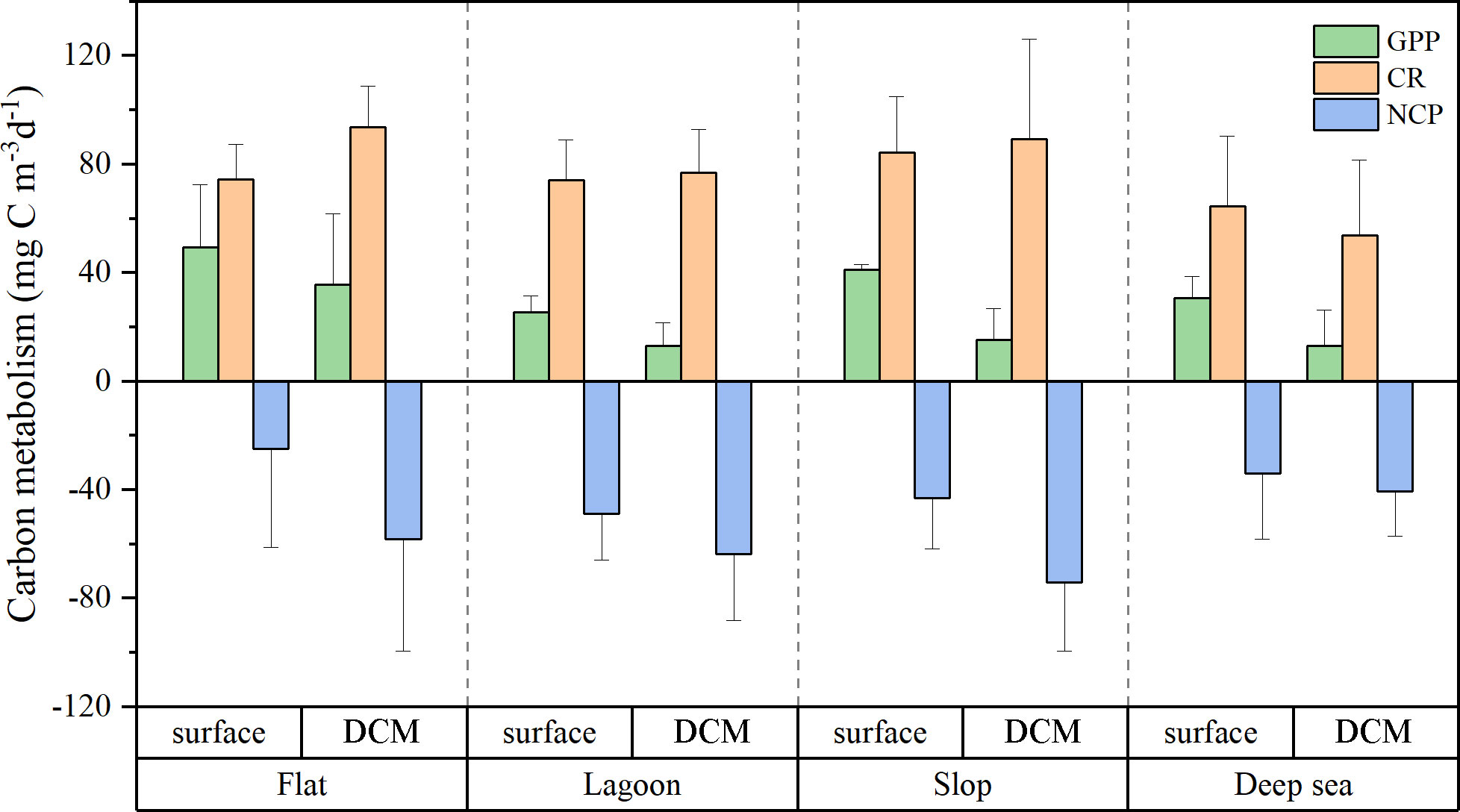
Figure 8 Average planktonic community carbon metabolism at surface and at the deep chlorophyll maximum (DCM) of different areas (flat, lagoon, slope and deep sea) of the Zhongsha Atoll.
4 Discussion
4.1 Ecological environment characteristics of Zhongsha Atoll during the summer monsoon
The present study reveals that the hydrological conditions of Zhongsha Atoll align with those of the deep-sea region outside the reef. As Zhongsha Atoll is completely submerged and the tops of the reef flats lie mostly 10-26 m below the surface, the upper waters experience mixing across the atoll (Figure S1). Additionally, the vertical variations in ecological conditions within the lagoon demonstrate a similar trend to the deep-sea area of the South China Sea (Li et al., 2017; Tai et al., 2020), where the mixed layer depth is approximately 50 m and the peak Chl a concentration occurs at around 75 m depth (Figure S1). Hence, the overall environmental characteristics of Zhongsha Atoll are largely influenced by the surrounding water conditions and currents.
During the study period, a receding cyclonic eddy was observed outside the southwestern end of the atoll (Wang et al., 2022). Generally, cyclonic eddies in the ocean often enhance primary production and phytoplankton biomass (Hu et al., 2014). Our observations indicate that the southwest region of the atoll exhibited low temperature, high salinity, and high Chl a concentration (Figure 2). However, the influence of the eddy on the atoll was limited to the outermost southwest edge and did not encompass the entire atoll. Conversely, due to variations in the widths and depths of the gaps between the reefs of Zhongsha Atoll, the level of exchange between the lagoon and sea water varied spatially (Huang et al., 2020), resulting in heterogeneity in temperature, salinity, and nutrient concentrations in the deeper layers of the lagoon (Figure 2).
The size structure of phytoplankton in Zhongsha Atoll was predominantly composed of the pico-fraction, which aligns with the composition of the phytoplankton community in the offshore waters of the South China Sea. Pico-phytoplankton typically contributes the most to phytoplankton biomass and primary production in the nutrient-poor open sea, accounting for as much as 70% of Chl a (Ke et al., 2014; Jiang et al., 2017). In our observations, pico-plankton constituted approximately 80% of the total Chl a in Zhongsha Atoll. This pattern has also been observed in other oceanic atolls, such as the Nansha Islands in the South China Sea (Huang, 2020) and the Great Astrolabe Lagoon of Fiji (Charpy and Blanchot, 1999), all of which exhibit similar ecological characteristics. Conversely, Chl a in nearshore coral reef ecosystems primarily consists of larger plankton. For instance, the nano-phytoplankton contributes between 49% and 73% of Chl a in the Great Barrier Reef Lagoon (Revelante and Gilmartin, 1982), and the presence of larger plankton is further promoted by external nutrient inputs (Bell et al., 2014). The comparison of Chl a concentration of different coral reefs also shows a similar pattern. Zhongsha Atoll displays a noticeably lower overall level of Chl a concentration compared to the neighboring shallow atolls in the South China Sea (Table 1). Ke et al. (2018) proposed a Chl a concentration threshold of 0.5 μg L-1 to assess nutrient enrichment in an open ocean atoll, based on findings from Huangyan Atoll. Our study revealed an average surface Chl a concentration of 0.15 μg L-1, indicating that the ecological condition of the Zhongsha Atoll aligns more closely with the oligotrophic nature of the South China Sea (Table 1).
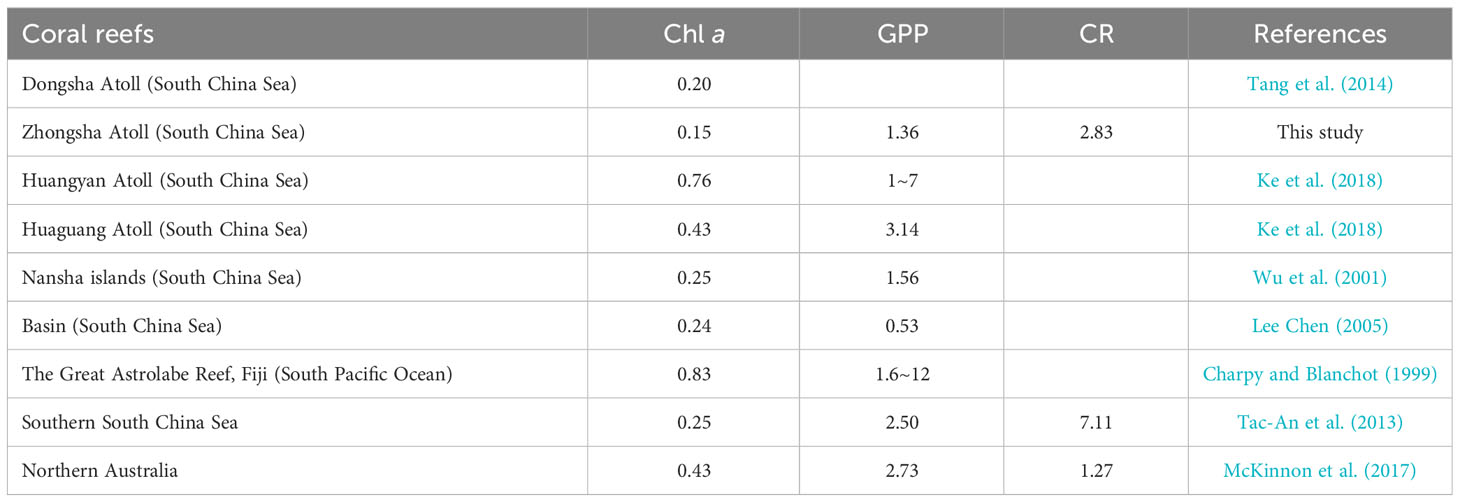
Table 1 Comparison of the surface chlorophyll a concentration (Chl a, μg L-1), planktonic community primary production (GPP, mg C m-3 h-1) and respiration metabolism (CR, mg C m-3 h-1) of Zhongsha Atoll investigated in this study with some reported islands or coral reefs.
4.2 Distribution pattern of planktonic community production and metabolism across Zhongsha Atoll
Extensive research has been conducted on the primary production of coral reef ecosystems, leading to a consensus that coral reefs are highly productive (Gattuso et al., 1999; Wu et al., 2001; Wang et al., 2007; Pan et al., 2012). However, the carbon dioxide dynamics in coral reef waters remain controversial (Ware et al., 1992; Gattuso et al., 1999; Nugraha and Insafitri, 2023). This study provides insights into the balance between community production and metabolism in the epipelagic zone of Zhongsha Atoll, revealing a heterotrophic state (Figure 5). Specifically, our findings indicate that the water column inside the atoll acts as a source of atmospheric CO2, and the annual net production of carbon dioxide in the upper water column, as determined by the calculated daily carbon flux derived from NCP, is estimated to be -1.10 ×103 t C km-2 y-1. The primary production of the open ocean or reefs in the central part of the South China Sea typically reaches its maximum near the surface (0-25 m), and gradually decreases with increasing depth (Liu et al., 2002; Deng et al., 2022). This suggests that the water column-integrated estimates of primary productivity in our study, which are based on the surface layer and the DCM layer, are likely to closely reflect the actual conditions. On the other hand, this study reveals that the vertical distribution of CR exhibits less variability compared to GPP. However, it is noteworthy that CR values consistently remain significantly higher than the corresponding GPP values across different depths. This observation is in accordance with the published findings of Regaudie-de-Gioux et al. (2009) and Regaudie-de-Gioux and Duarte (2013), thus further affirming the heterotrophic state of seawater surrounding the Zhongsha Atoll. Moreover, the results of both correlation analysis and RDA clearly revealed a noteworthy negative correlation between NCP and CR. In contrast, the correlation between NCP and GPP exhibited comparatively weaker strength. These findings suggest that the spatial distribution of NCP in Zhongsha Atoll is primarily subject to negative regulation by CR.
Although previous studies have reported that the phytoplankton biomass and primary productivity of lagoons remains constant with depth and is higher than that of the reef flats, it should be noted that these findings are specific to atolls with shallow lagoon less than 20 m depth (Wu et al., 2001; Ke et al., 2016; Ke et al., 2018). In the present study, Zhongsha Atoll features a deep lagoon, therefore the nutrient cycling processes mediated by corals and symbiotic zooxanthellae in the benthic environment have a lesser influence on the waters of the upper layer. As a result, unlike the shallow reef lagoons, the lagoon of Zhongsha Atoll displayed a distinct vertical variation in terms of primary production. Specifically, a substantial decrease in primary production was observed from the surface waters to the deeper regions of the lagoon. This pattern is similar to that of its adjacent deep-sea waters (Figure 8), as well as findings from the southern South China Sea, where primary production is predominantly concentrated within the upper 25 meters, gradually diminishing to negligible levels at depths ranging from 100 to 150 m (Wu et al., 2001). Moreover, Marra et al. (2020) also documented a general decline in global marine primary production beyond a depth of 50 meters. On the other hand, the vertical variation of CR in the lagoon of Zhongsha Atoll was found to be insignificant. This could be attributed to multiple factors, such as the deposition of organic materials from the upper water layer to the deeper waters, the transportation of these materials from the reef flat to the deeper waters of the lagoon, and the dynamic metabolism of the coral community in the bottom layer of the lagoon. These combined factors contribute to the maintenance or even elevation of plankton respiration rates compared to those observed in the surface layer. In contrast to the shallow lagoon, the lagoon of Zhongsha Atoll is characterized by a larger and deeper water column that lacks autotrophic processes. As a result, the DCM layer exhibits a more active and heterotrophic community metabolism. Consequently, the interplay between the promotion of heterotrophic metabolism and the restriction of primary production culminates in the creation of an anoxic environment within the deeper layers of the Atoll. As a result, the ecosystem functions as a CO2 source (Alldredge et al., 2013).
4.3 Influence of coral reef distribution on plankton production and metabolism in submerged atoll waters
In general, primary production and Chl a are higher around coral atolls than in the distant seawater. Although the overall ecological environment of Zhongsha Atoll did not show significant differences from the surrounding oligotrophic waters, it still exhibits more active productivity in the reef flat and reef slope (Figure 8). This indicates that corals also promote carbon fixation in the oceanic submerged atoll. The comparison of coral reefs in different regions and types also shows that although some atolls are lower in Chl a concentration, the level of primary productivity is still higher than that of the open sea of the South China Sea (Table 1), which suggests the importance of coral reefs to the primary production in the ocean. However, these regions are not only more productive but also more active in respiratory metabolism, with a production to respiratory metabolism ratio in the reef flat of 0.74, making it more heterotrophic compared to other areas. Similar results were reported by Tac-An et al. (2013), where surface water production from an deep sea coral reef in the southern South China Sea was about 1.30 times higher compared to a nutrient-limited marine area, but the primary production was only half of the respiratory metabolism. The occurrence of active metabolic processes in submerged reef flats can be largely attributed to the availability of sufficient light throughout the water column, promoting primary production in this shallow water region. This, in turn, leads to a constant turnover of biological activity. Additionally, coral reef systems are characterized by a rich abundance of biological resources, with the production of organic matter excreted by corals constituting approximately 30-40% of photosynthesis (Crossland et al., 1980). The decomposition and mineralization of this organic matter promote microbial metabolism, leading to increased material cycling and further attracting a larger population of zooplankton (Naumann et al., 2011; Huang et al., 2022). Consequently, these factors contribute to a more vigorous plankton community metabolism and enhanced material cycling within the coral reef ecosystem.
Carbon cycling processes in coral atolls are influenced by various factors, including seasonal variations in the hydrographic environment, the composition of benthic communities, and the health of coral reefs (Gattuso et al., 1996; Zhao et al., 2016; Zhang et al., 2023). The benthic community of Zhongsha Atoll is predominantly composed of reef-building corals (Huang et al., 2023). Previous studies have indicated that coral reef ecosystems can act as a source of atmospheric CO2 when the benthic community is dominated by reef-building corals (Ware et al., 1992; Kraines et al., 1996; Suzuki and Kawahata, 2004), and the present study further substantiates this phenomenon. Therefore, despite potential variations in planktonic carbon metabolism across different regions of Zhongsha Atoll, the overall ecosystem demonstrates a consistent heterotrophic nature and acts as a carbon source.
In conclusion, the ecological environment of Zhongsha Atoll during the study period closely resembled the characteristics of its surrounding open sea. This similarity can be attributed to the exchange of water with the deep-sea waters outside the atoll. The net carbon production of its planktonic community manifested as a source of CO2, and heterotrophic metabolism was stronger from the surface water to the DCM layer. The shallow-water areas of the atoll, including reef flats and reef slopes, displayed higher primary productivity compared to lagoons and the open ocean outside the atoll. However, heterotrophic metabolic processes were also more active in these areas. These findings suggest that the distribution of reef-building corals plays a significant role in carbon turnover efficiency and material cycling in the waters around coral atolls. It is important to note that this study was conducted during the early stages of the summer monsoon period. Therefore, further comprehensive and multidisciplinary analysis is necessary to comprehend the potential impacts of seasonal environmental variability and mesoscale physical processes on the metabolism of the planktonic community within the atoll. Such analysis will provide deeper insights into the role of coral atoll primary production and metabolism in the ocean’s carbon budget.
Data availability statement
The raw data supporting the conclusions of this article will be made available by the authors, without undue reservation.
Author contributions
CX: Data curation, Formal Analysis, Writing – original draft. YZ: Data curation, Writing – review & editing. GL: Data curation, Investigation, Writing – review & editing. XS: Funding acquisition, Supervision, Writing – original draft, Writing – review & editing. YH: Investigation, Writing – original draft. ZW: Investigation, Data curation, Writing – original draft. CZ: Investigation, Writing – original draft. LH: Writing – review & editing.
Funding
The author(s) declare financial support was received for the research, authorship, and/or publication of this article. This work was supported by the Hainan Provincial Joint Project of Sanya Yazhou Bay Science and Technology City, Grant No: 2021CXLH0011, Science and Technology Basic Resources Investigation Program of China, Grant No: 2018FY100105, the National Key Research and Development Program of China, Grant No: 2022YFC3103802, the Guangzhou Basic and Applied Basic Research No: 2023A04J0900, the National Natural Science Foundation of China No: 41890853; Science and Technology Planning Project of Guangdong Province, China (2023B1212060047).
Conflict of interest
The authors declare that the research was conducted in the absence of any commercial or financial relationships that could be construed as a potential conflict of interest.
The reviewer WG, declared a past co-authorship with the author, LG.
Publisher’s note
All claims expressed in this article are solely those of the authors and do not necessarily represent those of their affiliated organizations, or those of the publisher, the editors and the reviewers. Any product that may be evaluated in this article, or claim that may be made by its manufacturer, is not guaranteed or endorsed by the publisher.
Supplementary material
The Supplementary Material for this article can be found online at: https://www.frontiersin.org/articles/10.3389/fmars.2023.1296052/full#supplementary-material
References
Alldredge A., Carlson C., Carpenter R. (2013). Sources of organic Carbon to coral reef flats. Oceanography 26 (3), 108–113. doi: 10.5670/oceanog.2013.52
Azevedo I. C., Duarte P. M., Bordalo A. A. (2006). Pelagic metabolism of the Douro estuary (Portugal) – Factors controlling primary production. Estuar. Coast. Shelf Sci. 69 (1-2), 133–146. doi: 10.1016/j.ecss.2006.04.002
Bell P. R., Elmetri I., Lapointe B. E. (2014). Evidence of large-scale chronic eutrophication in the Great Barrier Reef: quantification of chlorophyll a thresholds for sustaining coral reef communities. Ambio 43 (3), 361–376. doi: 10.1007/s13280-013-0443-1
Cai P., Huang Y., Shen G., Li W., Liu G., Chen M., et al. (2000). The relationship between primary productivity and vertical stability of water column in the South China Sea. Acta Oceanologica Sin. 22, 137–140.
Charpy L., Blanchot J. (1999). Picophytoplankton biomass, community structure and productivity in the Great Astrolabe Lagoon, Fiji. Coral Reefs 18 (3), 255–262. doi: 10.1007/s003380050190
Chen T., Yu K., Shi Q., Li S., Price G. J., Wang R., et al. (2009). Twenty-five years of change in scleractinian coral communities of Daya Bay (northern South China Sea) and its response to the 2008 AD extreme cold climate event. Sci. Bull. 54 (12), 2107–2117. doi: 10.1007/s11434-009-0007-8
Crossland C. J., Barnes D. J., Borowitzka M. A. (1980). Diurnal lipid and mucus production in the staghorn coral Acropora acuminata. Mar. Biol. 60 (2-3), 81–90. doi: 10.1007/bf00389151
Crossland C. J., Hatcher B. G., Smith S. V. (1991). Role of coral reefs in global ocean production. Coral Reefs 10 (2), 55–64. doi: 10.1007/bf00571824
Deng L., Zhou W., Xu J., et al. (2022). Estimation of vertical size-fractionated phytoplankton primary production in the northern South China Sea. Ecol. Indic. 135, 108546. doi: 10.1016/j.ecolind.2022.108546
Duarte C. M., Regaudie-De-Gioux A. (2009). Thresholds of gross primary production for the metabolic balance of marine planktonic communities. Limnol. Oceanogr. 54 (3), 1015–1022. doi: 10.4319/lo.2009.54.3.1015
Estrada M., Marrase C., Latasa M., Berdalet E., Delgado M., Riera T. (1993). Variability of deep Chlorophyll maximum characteristics in the northwestern mediterranean. Mar. Ecol. Prog. Ser. 92 (3), 289–300. doi: 10.3354/meps092289
Fan L.-F., Qiu S.-Q., Chou W.-C. (2021). Carbonate chemistry of the Dongsha Atoll Lagoon in the northern South China Sea. Terrestrial Atmospheric Oceanic Sci. 32 (3), 399–409. doi: 10.3319/tao.2021.05.15.02
Furnas M., Mitchell A., Skuza M., et al. (2005). In the other 90%: phytoplankton responses to enhanced nutrient availability in the Great Barrier Reef Lagoon. Mar. pollut. Bull. 51 (1-4), 253–265. doi: 10.1016/j.marpolbul.2004.11.010
Garcia-Corral L. S., Duarte C. M., Agusti S. (2021). Plankton community metabolism in western Australia: estuarine, coastal and oceanic surface waters. Front. Mar. Sci. 7. doi: 10.3389/fmars.2020.582136
Gattuso J. P., Frankignoulle M., Smith S. V. (1999). Measurement of community metabolism and significance in the coral reef CO2 source-sink debate. Proc. Natl. Acad. Sci. U.S.A. 96 (23), 13017–13022. doi: 10.1073/pnas.96.23.13017
Gattuso J. P., Frankignoulle M., Smith S. V., Ware J. R., Wollast R. (1996). Coral reefs and carbon dioxide. Science 271 (5253), 1298–1298. doi: 10.1126/science.271.5253.1298-a
Gazeau F., Borges A. V., Barron C., Duarte C. M., Iversen N., Middelburg J. J. (2005). Net ecosystem metabolism in a micro-tidal estuary (Randers Fjord, Denmark): evaluation of methods. Mar. Ecol. Prog. Ser. 301, 23–41. doi: 10.3354/meps301023
Glacio Souza A., Diana P., João C., José William Alves da S., Jefferson S., Renato Teixeira M., et al. (2022). “Plankton: environmental and economic importance for a sustainable future,” in Plankton Communities. Eds. Leonel P., Ana Marta G. (Rijeka: IntechOpen), Ch. 2. doi: 10.5772/intechopen.100433
Gove J. M., McManus M. A., Neuheimer A. B., Polovina J. J., Drazen J. C., Smith C. R., et al. (2016). Near-island biological hotspots in barren ocean basins. Nat. Commun. 7, 10581. doi: 10.1038/ncomms10581
Grasshoff K., Ehrhardt M., Kremling K. (1983). Methods of Seawater Analysis (New York: Verlag Chemie).
Hanson C. E., Pesant S., Waite A. M., Pattiaratchi C. B. (2007). Assessing the magnitude and significance of deep chlorophyll maxima of the coastal eastern Indian Ocean. Deep Sea Res. Part II Top. Stud. Oceanogr. 54 (8-10), 884–901. doi: 10.1016/j.dsr2.2006.08.021
Hoegh-Guldberg O., Poloczanska E. S., Skirving W., Dove S. (2017). Coral reef ecosystems under climate change and ocean acidification. Front. Mar. Sci. 4. doi: 10.3389/fmars.2017.00158
Hopkinson C. S., Smith E. M. (2005). “Estuarine respiration: an overview of benthic, pelagic, and whole system respiration,” in Respiration in Aquatic Ecosystems. Eds. del Giorgio P., Williams P. (Oxford: Oxford University Press). doi: 10.1093/acprof:oso/9780198527084.003.0008
Hu Z. F., Tan Y. H., Song X. Y., Zhou L. B., Lian X. P., Huang L. M., et al. (2014). Influence of mesoscale eddies on primary production in the South China Sea during spring inter-monsoon period. Acta Oceanologica Sin. 33 (3), 118–128. doi: 10.1007/s13131-014-0431-8
Huang L. (2020). A Study on Ecological Processes of Nansha Islands Sea Area I (Beijing: Science Press).
Huang X., Betzler C., Wu S., Bernhardt A., Eagles G., Han X., et al. (2020). First documentation of seismic stratigraphy and depositional signatures of Zhongsha atoll (Macclesfield Bank), South China Sea. Mar. Pet. Geol. 117, 104349. doi: 10.1016/j.marpetgeo.2020.104349
Huang D., Chen J., Xu L., Wang X., Ning J., Li Y., et al. (2022). Larval fish assemblages and distribution patterns in the Zhongsha atoll (Macclesfield bank, South China sea). Front. Mar. Sci. 8. doi: 10.3389/fmars.2021.787765
Huang H., Jiang L., Lin Q. (2023). Reef-building Corals in the Zhongsha Islands (Beijing: Science Press).
Huang D., Licuanan W. Y., Hoeksema B. W., Chen C. A., Ang P. O., Huang H., et al. (2014). Extraordinary diversity of reef corals in the South China Sea. Mar. Biodivers 45 (2), 157–168. doi: 10.1007/s12526-014-0236-1
Jiang X., Li J. J., Ke Z. X., Xiang C. H., Tan Y. H., Huang L. M. (2017). Characteristics of picoplankton abundances during a Thalassiosira diporocyclus bloom in the Taiwan Bank in late winter. Mar. pollut. Bull. 117 (1-2), 66–74. doi: 10.1016/j.marpolbul.2017.01.042
Ke Z. X., Liu H. J., Wang J. X., Liu J. X., Tan Y. H. (2016). Abnormally high phytoplankton biomass near the lagoon mouth in the Huangyan Atoll, South China Sea. Mar. pollut. Bull. 112 (1-2), 123–133. doi: 10.1016/j.marpolbul.2016.08.028
Ke Z., Tan Y., Huang L., Liu H., Liu J., Jiang X., et al. (2018). Spatial distribution patterns of phytoplankton biomass and primary productivity in six coral atolls in the central South China Sea. Coral Reefs 37 (3), 919–927. doi: 10.1007/s00338-018-1717-7
Ke Z. X., Tan Y. H., Ma Y. N., Huang L. M., Wang S. B. (2014). Effects of surface current patterns on spatial variations of phytoplankton community and environmental factors in Sunda shelf. Cont. Shelf Res. 82, 119–127. doi: 10.1016/j.csr.2014.04.017
Koenings J. P., Edmundson J. A. (1991). Secchi disk and photometer estimates of light regimes in Alaskan lakes: Effects of yellow color and turbidity. Limnol. Oceanogr. 36 (1), 91–105. doi: 10.4319/lo.1991.36.1.0091
Kraines S., Suzuki Y., Yamada K., Komiyama H. (1996). Separating biological and physical changes in dissolved oxygen concentration in a coral reef. Limnol. Oceanogr. 41 (8), 1790–1799. doi: 10.4319/lo.1996.41.8.1790
Lee Chen Y.-l. (2005). Spatial and seasonal variations of nitrate-based new production and primary production in the South China Sea. Deep Sea Res. Part I: Oceanographic Res. Papers 52 (2), 319–340. doi: 10.1016/j.dsr.2004.11.001
Li J., Jiang X., Li G., Jing Z., Zhou L., Ke Z., et al. (2017). Distribution of picoplankton in the northeastern South China Sea with special reference to the effects of the Kuroshio intrusion and the associated mesoscale eddies. Sci. Total Environ. 589, 1–10. doi: 10.1016/j.scitotenv.2017.02.208
Liu K. K., Chao S. Y., Shaw P. T., Gong G. C., Chen C. C., Tang T. Y. (2002). Monsoon-forced chlorophyll distribution and primary production in the South China Sea: observations and a numerical study. Deep Sea Res. Part I: Oceanographic Res. Papers 49 (8), 1387–1412. doi: 10.1016/s0967-0637(02)00035-3
Liu S., Su H., Pan Y. F., Xu X. R. (2020). Spatial and seasonal variations of antibiotics and antibiotic resistance genes and ecological risks in the coral reef regions adjacent to two typical islands in South China Sea. Mar. pollut. Bull. 158, 111424. doi: 10.1016/j.marpolbul.2020.111424
Macías D., Stips A., Garcia-Gorriz E. (2014). The relevance of deep chlorophyll maximum in the open Mediterranean Sea evaluated through 3D hydrodynamic-biogeochemical coupled simulations. Ecol. Model 281, 26–37. doi: 10.1016/j.ecolmodel.2014.03.002
Marra J. F., Barber R. T., Barber E., Bidigare R. R., Chamberlin W. S., Goericke R., et al. (2020). A database of ocean primary productivity from the 14C method. Limnology Oceanography Lett. 6 (2), 107–111. doi: 10.1002/lol2.10175
McKinnon A. D., Duggan S., Logan M., Lønborg C. (2017). Plankton respiration, production, and trophic state in tropical coastal and shelf waters adjacent to northern Australia. Front. Mar. Sci. 4, 346. doi: 10.3389/fmars.2017.00346
Naumann M. S., Orejas C., Wild C., Ferrier-Pages C. (2011). First evidence for zooplankton feeding sustaining key physiological processes in a scleractinian cold-water coral. J. Exp. Biol. 214 (Pt 21), 3570–3576. doi: 10.1242/jeb.061390
Nugraha W. A., Insafitri I. (2023). Reefs as net carbon sources or net carbon sink? AIP Conf. Proc. 2634 (1), 020075. doi: 10.1063/5.0111323
Pan X., Wong G. T. F., Shiah F.-K., Ho T.-Y. (2012). Enhancement of biological productivity by internal waves: observations in the summertime in the northern South China Sea. J. Oceanogr. 68 (3), 427–437. doi: 10.1007/s10872-012-0107-y
Parsons T. R., Maita Y., Lalli C. M. (1984). A manual of chemical and biological methods for seawater analysis (Oxford: Pergamon Press).
Pawlik J. R., Loh T. L., McMurray S. E. (2018). A review of bottom-up vs. top-down control of sponges on Caribbean fore-reefs: what's old, what's new, and future directions. PeerJ 6, e4343. doi: 10.7717/peerj.4343
Regaudie-de-Gioux A., Duarte C. M. (2013). Global patterns in oceanic planktonic metabolism. Limnol. Oceanogr. 58 (3), 977–986. doi: 10.4319/lo.2013.58.3.0977
Regaudie-de-Gioux A., Vaquer-Sunyer R., Duarte C. M. (2009). Patterns in planktonic metabolism in the Mediterranean Sea. Biogeosciences 6 (12), 3081–3089. doi: 10.5194/bgd-6-8569-2009
Revelante N., Gilmartin M. (1982). Dynamics of phytoplankton in the great barrier reef lagoon. J. Plankton Res. 4 (1), 47–76. doi: 10.1093/plankt/4.1.47
Ribes M., Coma R., Atkinson M. J., Kinzie R. A. (2005). Sponges and ascidians control removal of particulate organic nitrogen from coral reef water. Limnol. Oceanogr. 50 (5), 1480–1489. doi: 10.4319/lo.2005.50.5.1480
Richardson T. L. (2019). Mechanisms and pathways of small-phytoplankton export from the surface ocean. Ann. Rev. Mar. Sci. 11, 57–74. doi: 10.1146/annurev-marine-121916-063627
Schlitzer R. (2020) Ocean Data View. Available at: http://odv.awi.de (Accessed October 20, 2021).
Serret P., Fernández E., Sostres J. A., Anadón R. (1999). Seasonal compensation of microbial production and respiration in a temperate sea. Mar. Ecol. Prog. Ser. 187, 43–57. doi: 10.3354/meps187043
Siswanto E., Ishizaka J., Yokouchi K. (2005). Estimating chlorophyll-a vertical profiles from satellite data and the implication for primary production in the kuroshio front of the East China Sea. J. Oceanogr. 61 (3), 575–589. doi: 10.1007/s10872-005-0066-7
Sorokin Y. I. (1991). Parameters of productivity and metabolism of coral reef ecosystems off central Vietnam. Estuar. Coast. Shelf Sci. 33 (3), 259–280. doi: 10.1016/0272-7714(91)90056-h
Spalding M. D., Green E. P., Ravilious C. (2001). World Atlas of Coral Reefs. Prepared at the UNEP World Conservation Monitoring Center (Berkley, USA: University of California Press).
Suzuki A., Kawahata H. (2004). “Reef water CO2 system and Carbon production of coral reefs: topographic control of system-level performance,” in Global Environmental Change in the Ocean and on Land. Eds. Shiyomi M., Kawahata H., Koizumi H. (Tokyo: Terraphub), 229–248.
Tac-An N., Minh-Thu P., Cherbadji I. I., Propp M. V., Odintsov V. S., Propp L. H. (2013). Primary production of coral ecosystems in the Vietnamese coastal and adjacent marine waters. Deep Sea Res. Part II: Topical Stud. Oceanography 96, 56–64. doi: 10.1016/j.dsr2.2013.05.020
Tai J.-H., Chou W.-C., Hung C.-C., Wu K.-C., Chen Y.-H., Chen T.-Y., et al. (2020). Short-term variability of biological production and CO2 system around Dongsha atoll of the northern south China sea: impact of topography-flow interaction. Front. Mar. Sci. 7. doi: 10.3389/fmars.2020.00511
Takahashi M., Hori T. (1984). Abundance of picophytoplankton in the subsurface chlorophyll maximum layer in subtropical and tropical waters. Mar. Biol. 79 (2), 177–186. doi: 10.1007/bf00951826
Tambutté S., Holcomb M., Ferrier-Pagès C., Reynaud S., Tambutté É., Zoccola D., et al. (2011). Coral biomineralization: From the gene to the environment. J. Exp. Mar. Biol. Ecol. 408 (1-2), 58–78. doi: 10.1016/j.jembe.2011.07.026
Tanaka Y., Suzuki A., Sakai K. (2018). The stoichiometry of coral-dinoflagellate symbiosis: carbon and nitrogen cycles are balanced in the recycling and double translocation system. ISME J. 12 (3), 860–868. doi: 10.1038/s41396-017-0019-3
Tang D., Ye H. J., Sui Y., Afanasyev Y. D., Wang S. (2014). “Typhoon impacts on subsurface marine ecosystems,” in Typhoon Impact and Crisis Management. Eds. Tang D., Sui G. (Berlin, Heidelberg: Springer Berlin Heidelberg), 219–240. doi: 10.1007/978-3-642-40695-9_10
Wang Y. H., Dai C. F., Chen Y. Y. (2007). Physical and ecological processes of internal waves on an isolated reef ecosystem in the South China Sea. Geophys. Res. Lett. 34 (18), L18609. doi: 10.1029/2007GL030658
Wang Y., Ju X., Du J., Liu C., Wang D., Zhou R., et al. (2022). Characteristics of the temperature and salinity distribution in adjacent sea areas of Zhongsha Islands during spring-summer transition period. Adv. Mar. Sci. 40 (3), 408–422. doi: 10.12362/j.issn.1671-6647.20210810001
Ware J. R., Smith S. V., Reaka-Kudla M. L. (1992). Coral reefs: sources or sinks of atmospheric CO2? Coral Reefs 11 (3), 127–130. doi: 10.1007/bf00255465
Worm B., Barbier E. B., Beaumont N., Duffy J. E., Folke C., Halpern B. S., et al. (2006). Impacts of biodiversity loss on ocean ecosystem services. Science 314 (5800), 787–790. doi: 10.1126/science.1132294
Wu C., Zhang J., Huang L. (2001). Primary productivity in some coral reeflagoons and their adjacent sea areasof nansha islands in spring. J. Trop. Oceanography 20 (3), 59–67.
Wyatt A. S. J., Lowe R. J., Humphries S., Waite A. M. (2010). Particulate nutrient fluxes over a fringing coral reef: relevant scales of phytoplankton production and mechanisms of supply. Mar. Ecol. Prog. Ser. 405, 113–130. doi: 10.3354/meps08508
Xiang C., Li Y., Ke Z., Li G., Huang Y., Su X., et al. (2022). Effects of daily nitrogen and phosphorus input on planktonic community metabolism in a semi-enclosed bay by mesocosm experiment. Acta Oceanologica Sin. 41 (8), 99–110. doi: 10.1007/s13131-022-1986-4
Zhang S., Bai Y., He X., Yu S., Song Z., Gong F., et al. (2023). The carbon sink of the Coral Sea, the world's second largest marginal sea, weakened during 2006–2018. Sci. Total Environ. 872, 162219. doi: 10.1016/j.scitotenv.2023.162219
Zhao M., Yu K., Shi Q., Yang H., Riegl B., Zhang Q., et al. (2016). The coral communities of Yongle atoll: status, threats and conservation significance for coral reefs in South China Sea. Mar. Freshw. Res. 67 (12), 1888–1896. doi: 10.1071/mf15110
Keywords: coral atoll, planktonic carbon metabolism, primary production, community respiration, net carbon production
Citation: Xiang C, Zhong Y, Li G, Song X, Huang Y, Wang Z, Zheng C and Huang L (2023) Planktonic carbon metabolism of an underwater coral atoll in the oligotrophic sea: a case study of Zhongsha Atoll, Central South China Sea. Front. Mar. Sci. 10:1296052. doi: 10.3389/fmars.2023.1296052
Received: 18 September 2023; Accepted: 10 November 2023;
Published: 01 December 2023.
Edited by:
Martin F. Soto-Jimenez, National Autonomous University of Mexico, MexicoReviewed by:
Frederico Pereir Brandini, University of São Paulo, BrazilWanchun Guan, Wenzhou Medical University, China
Copyright © 2023 Xiang, Zhong, Li, Song, Huang, Wang, Zheng and Huang. This is an open-access article distributed under the terms of the Creative Commons Attribution License (CC BY). The use, distribution or reproduction in other forums is permitted, provided the original author(s) and the copyright owner(s) are credited and that the original publication in this journal is cited, in accordance with accepted academic practice. No use, distribution or reproduction is permitted which does not comply with these terms.
*Correspondence: Xingyu Song, c29uZ3h5QHNjc2lvLmFjLmNu