- 1Biogéosciences, UMR 6282 CNRS, Université de Bourgogne, Dijon, France
- 2MARBEC, University of Montpellier, CNRS, Ifremer, IRD, Montpellier, France
- 3IFREMER Centre de Bretagne, DYNECO, Laboratoires d’Ecologie Benthique Côtière, Plouzané, France
- 4Fenner School of Environment & Society, Australian National University, Canberra, ACT, Australia
Sub-Antarctic coastal marine ecosystems harbor rich and diverse benthic communities. Despite their ecological uniqueness and vulnerability to global changes, studies on benthic communities remain limited. Using underwater video-imagery, we investigated the taxonomic and functional diversity of benthic communities associated with hard substrates at Baie du Marin (Ile de la Possession, Crozet archipelago). The Baie du Marin species richness and diversity were additively partitioned to evaluate spatial patterns of species through the following spatial scales: within images, among images within transects, and among transects. We analyzed imagery data from seven transects located at different sites inside Baie du Marin and covering contrasting natural rocky habitats and underwater artificial cable substrates. A total of 50 faunal (mainly represented by Echinodermata and Porifera phyla) and 14 algae (mainly represented by Rhodophyta phylum) taxa were identified. Rocky substrates were dominated by high densities of the polychaetes Parasabella sp. and Lanice marionensis, whereas submarine cables were dominated by high densities of the bivalve Kidderia sp. attached to macroalgae. Our results show contrasted distribution patterns in the faunal and algal assemblages within the Baie du Marin, with significant ecological differences between submarine cables and natural rocky substrates. Larger spatial scale (i.e., among transects) accounted for most of the bay richness and diversity, highlighting a high-level of habitat heterogeneity within the bay. Through a trait-based approach, our findings revealed that Crozet benthic communities are characterized by low functional richness, evenness, and redundancy, highlighting a potential vulnerability to current and future natural and anthropogenic changes. This study provides a novel bentho-ecological baseline for future assessments of natural and anthropogenic impacts on the marine environment of the Crozet archipelago; and for the conservation management of these remote habitats that make part of the French Southern Territories Marine Protected Area, recently inscribed on the UNESCO World Heritage list.
1 Introduction
Benthic ecosystems of sub-Antarctic islands host a unique and diverse biodiversity, characterized by high-level endemism (Branch et al., 1993; Chown et al., 2001; Barnes et al., 2006; Freeman et al., 2011; Hogg et al., 2011; Clark et al., 2019). While marine ecosystems of these isolated territories are of high conservation value (Chown et al., 2001), they remain understudied and vulnerable to multiple anthropogenic threats, including climate change (Hogg et al., 2011), biological invasion (Smith, 2002; Hogg et al., 2011; McCarthy et al., 2019), and increasing maritime traffic (i.e., fisheries, tourism activities and scientific research). These synergistic threats can lead to a shift in benthic communities caused by: (i) the loss of a wide range of habitats and associated diversity (including species subject to conservation measures) (Saucède et al., 2017); (ii) altered food web structure (Ehrenfeld, 2010; Kortsch et al., 2015); (iii) modified biotic interactions (Montoya and Raffaelli, 2010); and (iv) formation of a new range of habitats available for benthic and potentially alien species (McCarthy et al., 2019). Biodiversity loss and/or any substantial alteration of benthic assemblages can potentially impair whole ecosystem functioning and alter its properties (e.g., ecosystem services) (Cardinale et al., 2012). Shedding light on the taxonomic and functional diversity of polar nearshore marine benthic communities is therefore needed to provide a baseline for future conservation strategies.
Many studies on coastal marine ecosystems, encompassing those in sub-Antarctic and Antarctic regions, have reported a significant influence of substrate on the composition and distribution of benthic communities (Barnes et al., 2006; Freeman et al., 2011; Clark et al., 2019), and the potential relevance of substrate in conservation management. Hard substrates and habitat-forming species promote habitat heterogeneity and structural complexity, enhancing species diversity (MacArthur and MacArthur, 1961; Levin et al., 2010). Rocky habitats allow the development of benthic communities by providing an additional colonizing space, nutrient source or a protection against predation and unfavorable environmental conditions (Levin et al., 2010). Artificial hard substrates can act as a reef for benthic species by providing new habitats (Langhamer, 2012). The influence of submarine cables on benthic assemblages is subject of controversy; several studies have highlighted short term or minor ecological effects on benthic communities (Andrulewicz et al., 2003; Kogan et al., 2006; Dunham et al., 2015; Sherwood et al., 2016), whereas other studies have reported major effects including biological invasion risks due to new habitats provided by artificial structures (Connell and Glasby, 1999; Glasby et al., 2007). The characterization of hard substrate benthic communities is thus essential to assess the contribution of different habitat types to the regional diversity and to investigate the potential ecological effects of these artificial structures on these environments.
Diversity studies are fundamental for examining the structure of sub-Antarctic coastal marine ecosystems, particularly in addressing functional diversity for conservation management (Bremner, 2008; Cardinale et al., 2012; Lindegren et al., 2018). Ecosystem function is not directly linked to taxonomic richness, but is dependent on species characteristics – functional traits – that express these species (Hooper et al., 2005). In this regard, trait-based approach – defined as any morphological, physiological or phenological feature measurable at the individual level (Violle et al., 2007) – is a powerful tool to better comprehend the response of benthic diversity to environmental changes and how these translate to ecosystem functioning (Mason et al., 2005; Villéger et al., 2008; Laliberté and Legendre, 2010; Mouchet et al., 2010). Species functional traits are considered as suitable proxies of the ecological functions supported by an ecosystem (Villnäs et al., 2018). Trait-based approaches have previously proven to be efficient in understanding different ecological mechanisms that underlie the local diversity in a wide range of benthic ecosystems, including intertidal seagrass and subtidal maerl beds (Boyé et al., 2019), soft-sediment habitats (Hewitt et al., 2008), and estuarine systems (van Der Linden et al., 2012). A functional trait approach can illuminate ecosystem productivity, functional stability or resilience, and the application to polar regions could estimate the potential effects of biological invasion, climate change, and other stressor factors on ecosystem functions (Hewitt et al., 2016; Degen et al., 2018; Robinson et al., 2022).
Underwater imagery is a non-invasive method which is increasingly used to conduct diversity studies and investigate communities and habitats in polar sensitive environments (Foveau et al., 2017). Imaging techniques provide valuable quantitative and qualitative information on benthic communities and are an effective tool for monitoring species assemblages and ecosystem functioning (Friedlander et al., 2023). Whereas physical sampling provides point-based data, video-imagery allows for the acquisition of a large amount of ecological data over broader sampling regions with less sampling effort (Solan et al., 2003). Underwater imagery is a powerful method for designating the diversity of coastal benthic habitats, including algal (Amsler et al., 1995; Berov et al., 2016) and faunal communities of natural hard (van Rein et al., 2011; Almond et al., 2021) and artificial substrates such as submarine cables (Dunham et al., 2015; Taormina et al., 2020). Image-based techniques have continually proven their value in a wide range of scientific endeavors of marine ecosystems; such as the assessment of spatial pattern distribution of Antarctic benthic communities (Amsler et al., 1995; Teixidó et al., 2002; Gutt et al., 2019), characterizing sub-Antarctic nearshore marine communities (Clark et al., 2019), and examining species richness in kelp forests (Bravo et al., 2023; Friedlander et al., 2023). Alongside the increasing use of image-based techniques, the development of image and video annotation software, specific to benthic ecology, have increased the efficiency of marine imaging data analysis (Teixidó et al., 2011; Trygonis and Sini, 2012; Langenkämper et al., 2017; Zurowietz and Nattkemper, 2021). Therefore, underwater video-imagery analysis is a suitable approach to conservation studies and for monitoring the sub-Antarctic diversity of nearshore marine ecosystems.
The maritime domain surrounding the Crozet archipelago is managed as a nature reserve, which is part of the French Southern and Antarctic Lands Marine Protected Area (French Government, Decree No. 2016-1700), recently classified on the UNESCO World Heritage list. In 2016, a hydroacoustic monitoring station (HA04) was installed by the “Preparatory Commission for the Comprehensive Nuclear-Test-Ban Treaty Organization” (CTBT0) at Baie du Marin (Ile de la Possession, Crozet archipelago), requiring impact study of the benthic communities. In this context, the contract “Nearshore cable inspection and environmental survey at IMS hydroacoustic station HA04 Crozet, France”, was signed between the CTBTO and French Southern and Antarctic Lands (TAAF) for the inspection of submarine cables and ecological surveys of nearshore marine habitats in the Baie du Marin, which had never been studied before and for which our knowledge is currently limited. The present study aims (i) to describe and quantify the structure and composition of faunal and algal communities associated with hard substrates (rocky substrate vs. HA04 station submarine cables) at Baie du Marin; (ii) to investigate and compare the faunal taxonomic and functional diversity; and (iii) to assess the relative contribution of different spatial scales (α-diversity corresponding to the mean diversity within individual images; β1-diversity the compositional change among images within transects; and β2-diversity the compositional change among transects within the bay) to Baie du Marin richness and diversity.
2 Materials and methods
2.1 Study site
The Crozet archipelago (45°48’S – 46°26’S; 50°14’E – 52°15’E) consists of five main volcanic islands located in the Southern Ocean at 2400 km north of the Antarctic Continent and 2400 km southeast from the South African coast. These islands are divided into two groups with (i) a western group including Île aux Cochons, Îlots des Apôtres and Île des Pingouins; and (ii) an eastern group about 110 km away comprising the two largest islands of the archipelago, Île de l’Est and Île de la Possession. Ile de la Possession (46°25’S; 51°45’E) is the largest island of the Crozet archipelago – approximately 18 km wide by 15 km long – with a total surface area of ∼156 km2 and elevation of 934 m above sea level (a.s.l.) (Pic du Mascarin) (Figure 1A). The image-based sampling was focused on the Baie du Marin, a site located on the eastern coast of Île de la Possession.
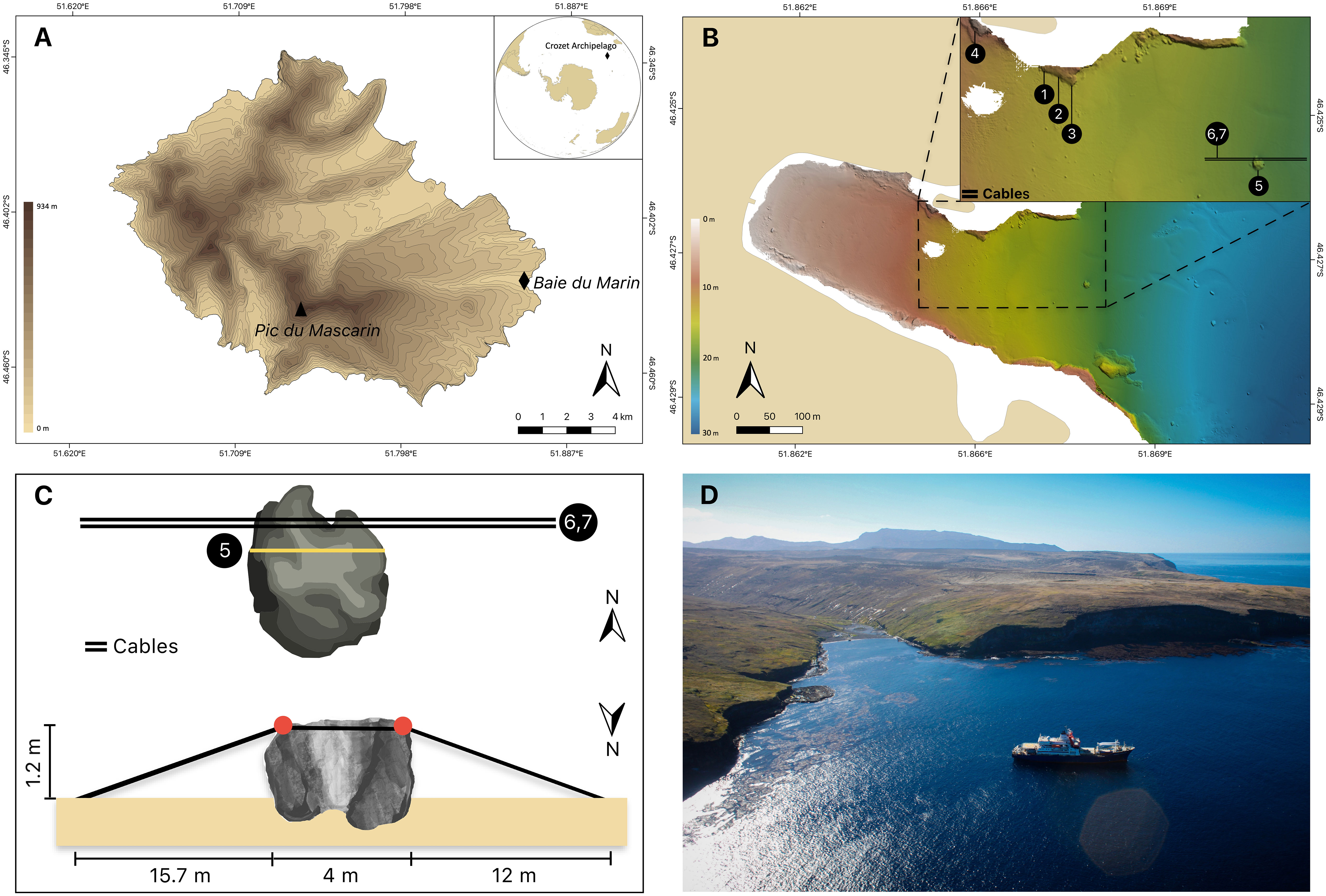
Figure 1 (A) Topographic map of Ile de la Possession (Crozet archipelago, Southern Ocean) with the location of the Baie du Marin (black diamond). (B) Bathymetric map of the Baie du Marin with a focus on the imagery sampling design, with (1) SD-CN1, (2) SD-CN2, (3) SD-CN3, (4) SD-CN4, (5) SD-Rock and (6,7) SD-Cable-2021 and SD-Cable-2022 respectively. (C) Schematic representation of the submarine cables and transects conducted on this area. Red circles: main points of contact between cables and rock. (D) Picture of the Baie du Marin. Photo courtesy of Comprehensive Nuclear-Test-Ban Treaty Organization, CTBTO-IPEV-TAAF cruise MD202/CTBTO-CRO R/V Marion Dufresne (2016).
The Baie du Marin (BDM; 46°25’54”S; 51°52’11”E) is a narrow inlet of c. 200 m width and 500 m length in its shallowest part (< 20 m depth) and opens to the ocean in a larger embayment of about 2 km wide at 40 m depth (Figures 1B–D). Due to the site geomorphology, the bay is exposed to ocean swell from the north. The coast is mainly composed of a rocky shore with a sandy beach located at the back of the bay. In general, the seabed is composed of coarse sand sediments, sometimes covered in organic detritus. In the inner inlet, down to 10 m depth, the bottom is a mix of coarse sands, pebbles and boulders. Rock and pebble areas are occasionally covered in stands of the giant kelp Macrocystis pyrifera, which are developed into loose patches along the shore between 10 and 20 m depth. Baie du Marin is characterized by the presence of a large colony of over 10,000 king penguins Aptenodytes patagonicus (Miller, 1778) and elephant seals Mirounga leonina (Linnaeus, 1758).
2.2 Imagery acquisition and data processing
Imagery acquisition took place from aboard the R/V Marion Dufresne II during the OP03-2021 and OP03-2022 logistical rotations conducted by TAAF (French Southern and Antarctic Lands) in response to Contract No. 2021-0882 with the CTBTO (Comprehensive Nuclear-Test-Ban Treaty Organization) for “Nearshore Cable Inspection and Environmental Survey at IMS Hydroacoustic Station HA04 Crozet, France” from 4th November to 9th November 2021 and 23rd November to 24th November 2022, respectively. Video-transects were recorded using a setting composed of three cameras, two GoPro HERO9® (5K video and 20-megapixel photos resolution; 80% field overlap) and an underwater camera Paralenz® Vaquita (4K resolution). The setting was also equipped with two lights and three 10 cm scaling lasers (spaced to 15 cm in 2022 setting) in order to estimate the filmed surface. Video-imagery was performed by scuba divers who maintained a regular swimming velocity, with the camera oriented parallel to the bottom at an average distance of 50 cm. Seven transects were conducted on different parts of the Baie du Marin (Figures 1B, C, 2, Supplementary Material 1). The first transect, SD-Rock, recorded benthic communities on the rock parallel to the cables at 19 m depth (Figure 2A). The following two transects (SD-Cable-2021 and SD-Cable-2022) recorded the suspended section of submarine cables to assess the potential influence of cables as substrate for benthic communities (Figures 2B, C). Finally, four transects – SD-CN1, SD-CN2, SD-CN3 and SD-CN4 – were recorded along the rock wall on the northern coast of the bay for approximately 200 m of the cables (Figures 2D–F). Amongst these four transects, three were carried out in 2022 (SD-CN1, SD-CN2 and SD-CN3) and one in 2021 (SD-CN4).
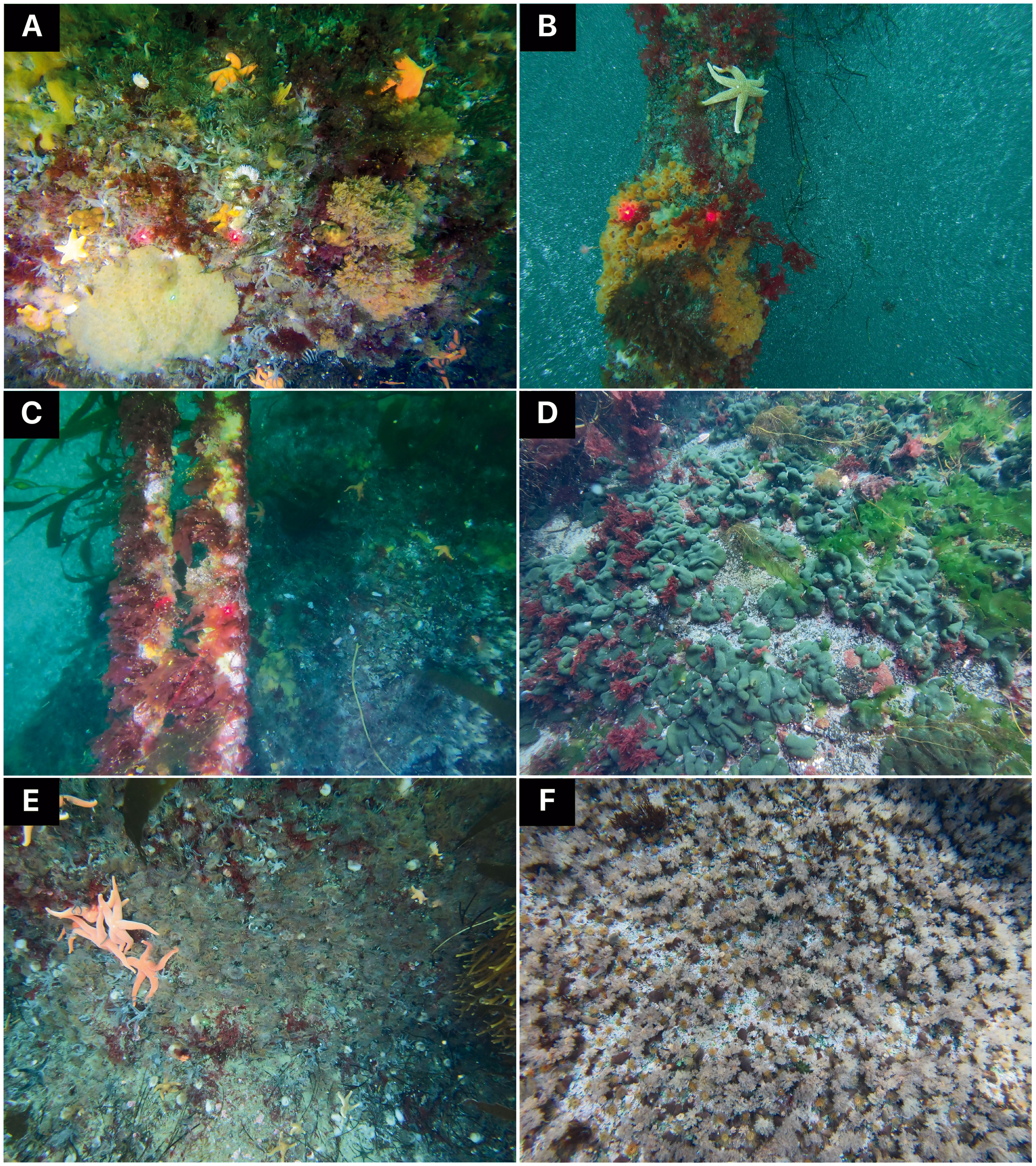
Figure 2 Benthic communities associated with hard substrates at different imagery sampling areas of the Baie du Marin, with: (A) rock located at the center of the bay; (B, C) submarine cables and (D–F) rocks located on the northern coast of the bay. Laser beams are 15 cm spaced for photos (A, C, D) and 10 cm spaced for photos (B, E, F). © Proteker.
Video-transects were divided in their entirety into individual images. All combined transects, a total of 161 images (representing c. 100 m2) were manually analyzed using the online open-source platform BIIGLE 2.0 (Benthic Image Indexing and Graphical Labelling Environment) (Langenkämper et al., 2017). For each image, all faunal organisms were scored and their counts were transformed into density (number of ind m−2), and algae cover was measured (m−2). In agreement with the taxonomists, organisms were identified to the lowest possible taxonomic level, by (i) submitting collected materials and macro-photography pictures captured during transect imaging acquisition; and (ii) through a systematic review of the images analyzed in BIIGLE 2.0.
2.3 Sampling effort, diversity metrics and benthic communities’ variation across transects
Diversity metrics, corresponding to Hill’s number of order q, were calculated for fauna and algae for each image of each transect and averaged in order to investigate the spatial variability of these indices across transects, with: species richness (q=0), Shannon diversity (q=1) and Simpson’s inverse (q=2) (Jost, 2006). In addition, Piélou’s evenness and “rare” species, defined as those represented by less than 1% of total species abundance, were also estimated. For each diversity metrics, non-parametric Kruskal-Wallis followed by Dunn’s post-hoc pairwise tests were conducted to test for significant differences among the seven transects at the image scale, and p-values were adjusted with a Bonferroni correction. To assess the sampling effort and to further explore how variation of diversity at the scale of the images scale-up at the scale of whole transects or the whole bay, rarefied diversity were computed from individual-based rarefaction curves for faunal communities (rarefied to the lowest number of individuals among transects: 1446 individuals) and image-based rarefaction curves for algal communities (rarefied to the lowest number of images among transects: 6 images). To investigate variation in benthic community composition across images and between transects, two Principal Coordinates ordination Analysis (PCoA) based on Hellinger-transformed data at the scale of the images were carried out on faunal and algal communities, respectively. Due to the absence of faunal taxa, eight images were excluded from the analyses (5 images of the SD-Cable-2021 transect and 3 of the SD-CN4 transect). A Redundancy Analysis (RDA) was carried out on these Hellinger-transformed data to ascertain how algal cover influences the distribution of faunal species. The Hellinger transformation was applied to minimize the greater weight given to rare species (Legendre and Gallagher, 2001). All statistical analyses were performed using R software (version 4.2.0, R Core Team, 2022). Rarefaction curves and diversity metrics were conducted using the package iNEXT (Hsieh et al., 2016). PCoA and RDA were realized using the package vegan (Oksanen et al., 2020).
2.4 Bay richness and diversity partitioning across spatial scales
The Baie du Marin (γ-diversity) faunal and algal richness (q=0) and diversity (q=1 and q=2) were additively partitioned to investigate spatial patterns of species through three hierarchical spatial components: (i) alpha (α-diversity) corresponding to the mean diversity within individual images; (ii) beta-1 (β1-diversity) the compositional change among images within transects; and (iii) beta-2 (β2-diversity) the compositional change among transects within the Baie du Marin (Figure 3). The significance of each spatial component was computed by comparing observed values to values draw from null distributions obtained by randomization (9999 permutations) of the original community matrix. The additive diversity partitioning analysis (Crist et al., 2003) was conducted using the PARTITION package available in GitHub.
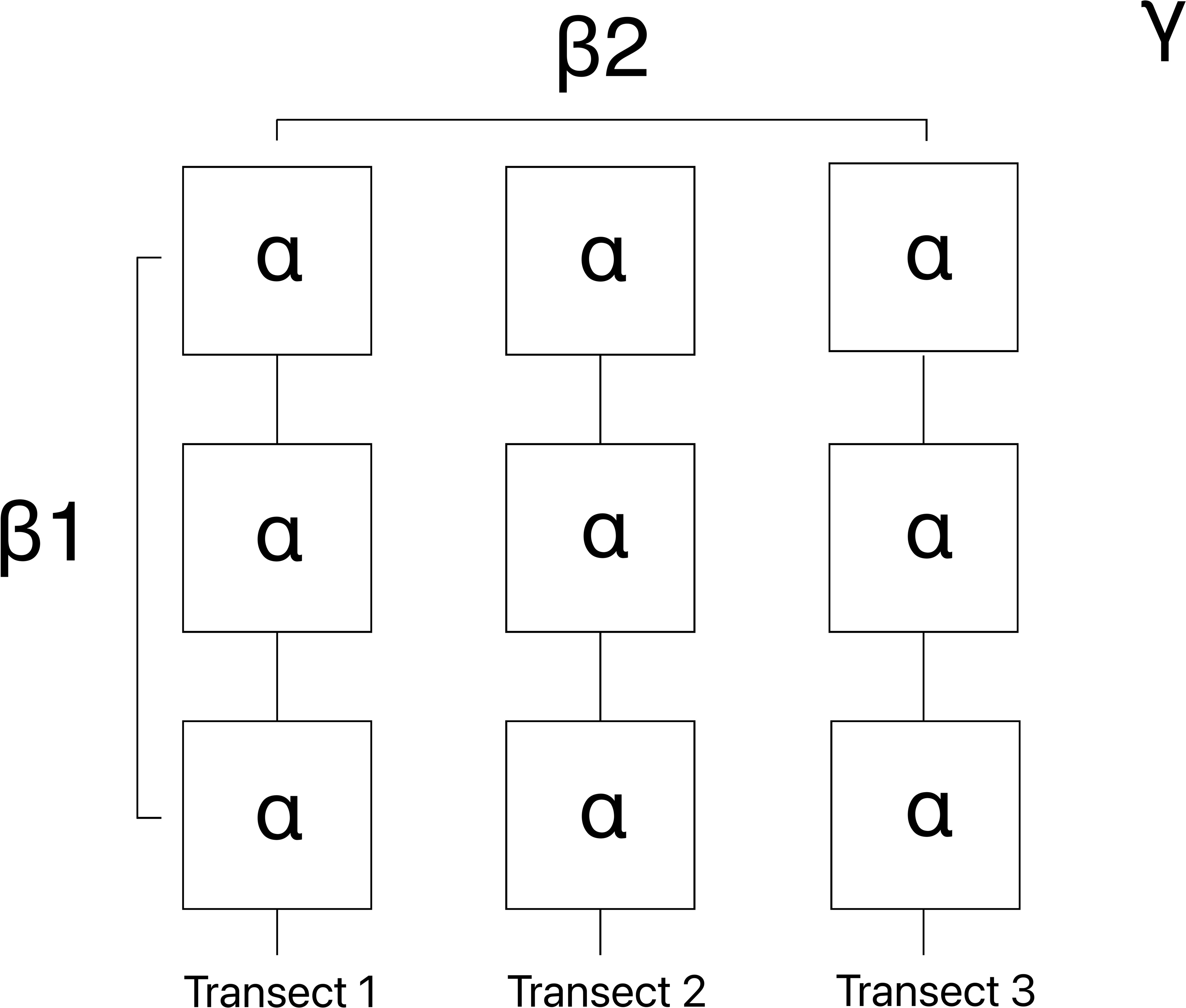
Figure 3 Schematic representation of the additive richness and diversity partitioning across the different spatial scale, with α-diversity (individual images), β1-diversity (compositional change among images within transects), β2-diversity (compositional change among transects within Baie du Marin) and γ-diversity (total richness and diversity at Baie du Marin).
2.5 Functional trait collection and analyses
The trait-based functional approach was focused on faunal taxa. Functional traits were gathered for the lowest taxonomic level possible and inferred from species of the same genus when data was missing. This ecological information was compiled from peer-reviewed literature, taxonomic expertise, and sample collection of the Université de Bourgogne. Traits were selected to reflect a wide array of ecological processes and therefore constituted suitable proxies of ecosystem functioning (Table 1). Faunal taxa were assigned 11 traits, which were divided into 37 modalities (Table 1, Supplementary Material 2.1 and 2.2). Taxa were scored for each trait modality based on their affinity using a fuzzy coding procedure (Chevenet et al., 1994), with a score ranging from 0 (no affinity) to 4 (exclusive affinity). This method allows for the integration of species plasticity towards certain traits (e.g., reproduction or feeding), and therefore the incorporation of the intra-specific variability in functional analysis.
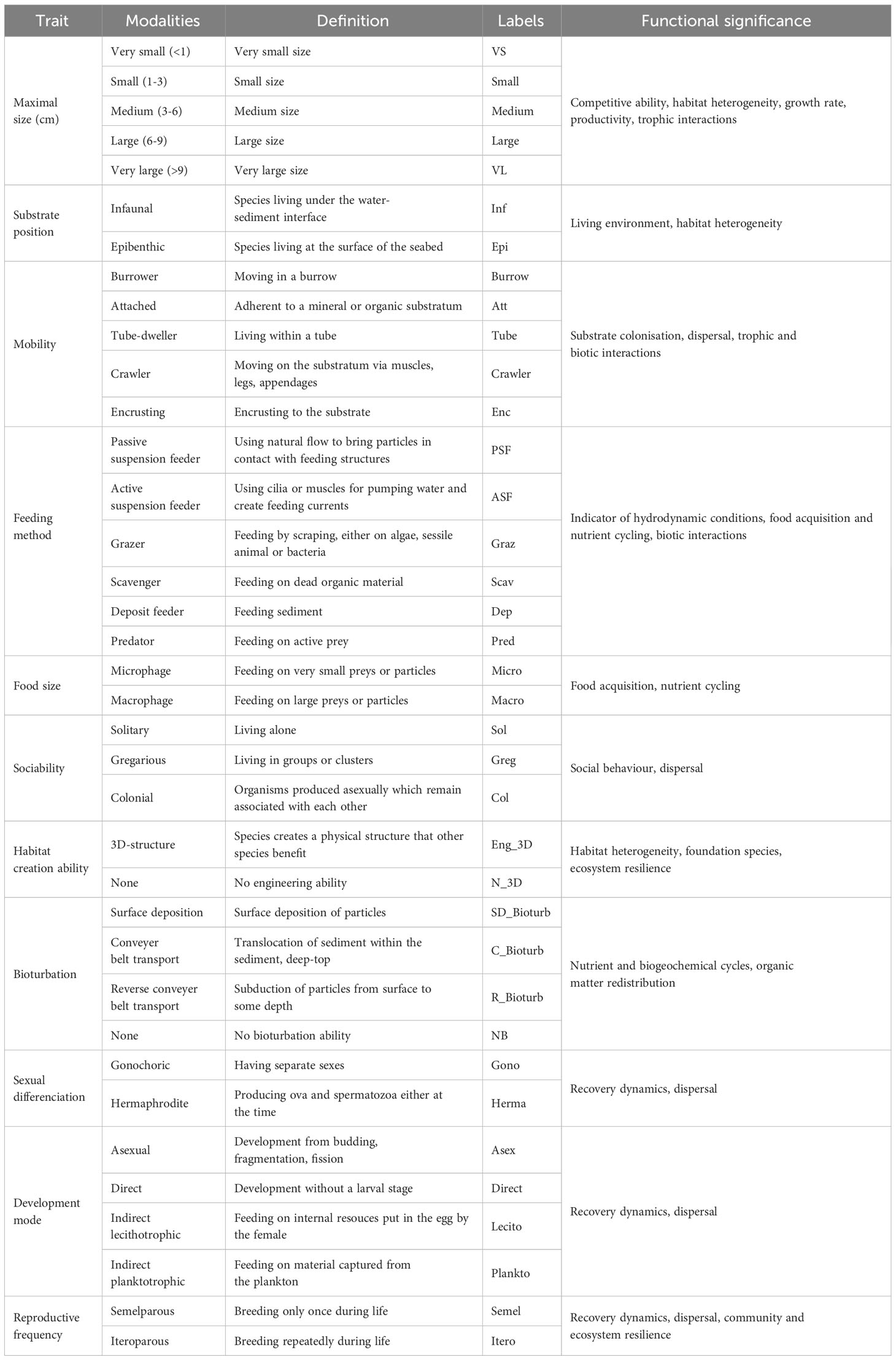
Table 1 Description of the functional traits and their modalities used to characterize faunal communities associated with hard substrate habitats and estimate their functional diversity.
The alpha functional diversity of faunal communities in each transect was characterized through complementary indices calculated using a subset of Principal Coordinates ordination Analysis axes (9 PCoA axes that accounted for 94% of the total variance of the functional/trait space) based on Euclidean distance of the standardized species-trait abundance matrix (Villéger et al., 2008; Laliberté and Legendre, 2010): (i) the functional richness (FRic) that corresponds to the amount of functional space – measured by the convex hull volume (Cornwell et al., 2006) – filled by the faunal community; (ii) the functional evenness (FEve) that describes the regularity of abundance distribution among species within the functional space and the functional distance between species; (iii) the functional divergence (FDiv) that measures the species abundances distribution in relation to the centroid of the functional space. FDiv indicates if abundant species are located close to the centroid (functional convergence) or in the external part of the functional space defined by the whole community (functional divergence); (iv) the functional dispersion (FDis), independent of species richness and defined as the mean-weighted distance of individual species in the functional space to the centroid (Laliberté and Legendre, 2010). High FDis values describe a high functional diversity within the community; (v) Rao’s quadratic entropy (RaoQ) which represents a combination between functional richness and divergence that is closely related to FDis (Laliberté and Legendre, 2010) and is equivalent to Simpson’s diversity index when all species are maximally different from each other (Botta-Dukát, 2005); and (vi) functional redundancy (FR), defined as the portion of functional traits shared by the species within a community or ecosystem. The functional space underlying these indices, and the distribution of species within it, was visualized using Principal Component Analysis (PCA).
Finally, functional beta diversity was explored through a PCA on the Hellinger-transformed trait modality densities. For that purpose, a species-trait abundance matrix was obtained by multiplying the faunal community matrix (abundances of the species within transects) with the species-trait matrix, containing the relative expression of trait modalities by species after standardization of scores to 1 for each trait and species. This procedure gives a weight at each modality in order to ensure that each trait has equal weight in the underlying functional analyses, regardless of the number of associated modalities. Functional analyses were conducted using the FD package (Laliberté et al., 2014) and PCA using the package vegan (Oksanen et al., 2020).
3 Results
3.1 Imagery sampling effort
A total of 50 faunal (representing 40,157 individuals) and 14 algal taxa were identified across the seven transects (Table 2). The Baie du Marin rarefaction curves (all transects combined) of the faunal and algal diversity show that a plateau is reached at about 50 taxa and 14 taxa, respectively, giving a fair representation of the benthic diversity in hard substrates along transects conducted at Baie du Marin (Figure 4; black curves). At the transect level, faunal rarefaction curves show that the sampling effort tends to reach an asymptote (with the exception of SD-CN3 and SD-Cable-2021 transects), indicating that enough images were collected to capture faunal diversity within transects (Figure 4A). For algal taxa, rarefaction curves at the transect level tend to reach a plateau, indicating sufficient sampling from images for algal diversity (Figure 4B).
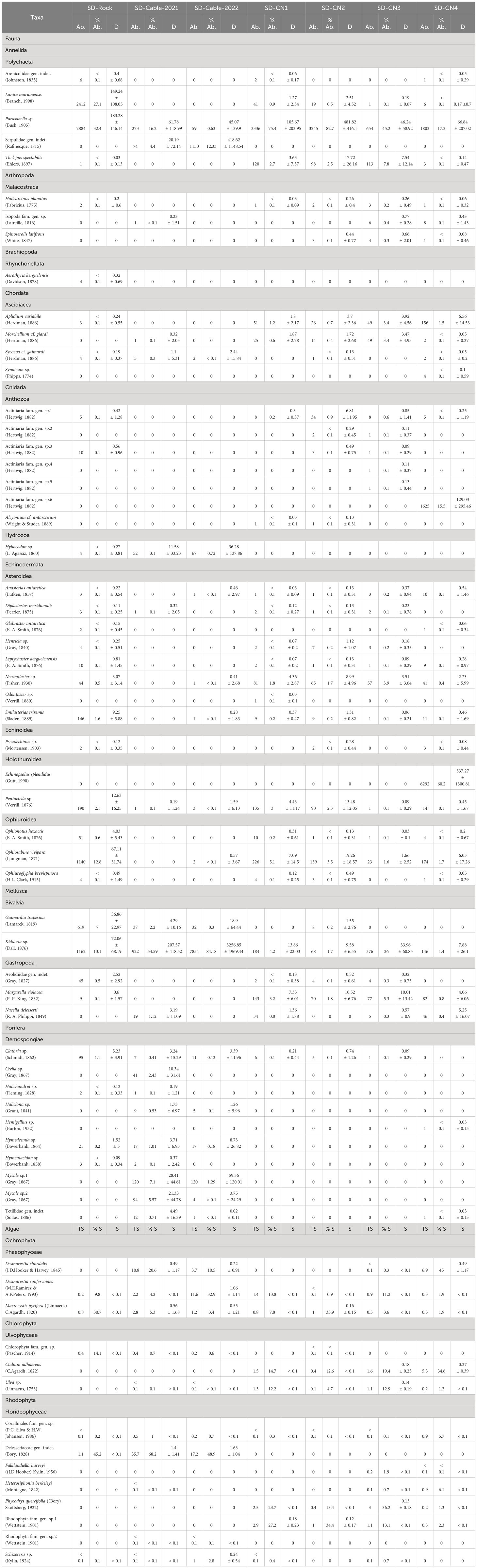
Table 2 Abundance (Ab.), relative abundance (% Ab.) and mean density (D in indiv m-2; mean ± standard deviation) of faunal taxa, as well as total surface cover (TS; m-2), relative surface cover (% S) and mean surface per square metre (S; m-2; mean ± standard deviation) of algal taxa identified at Baie du Marin using imagery transects.
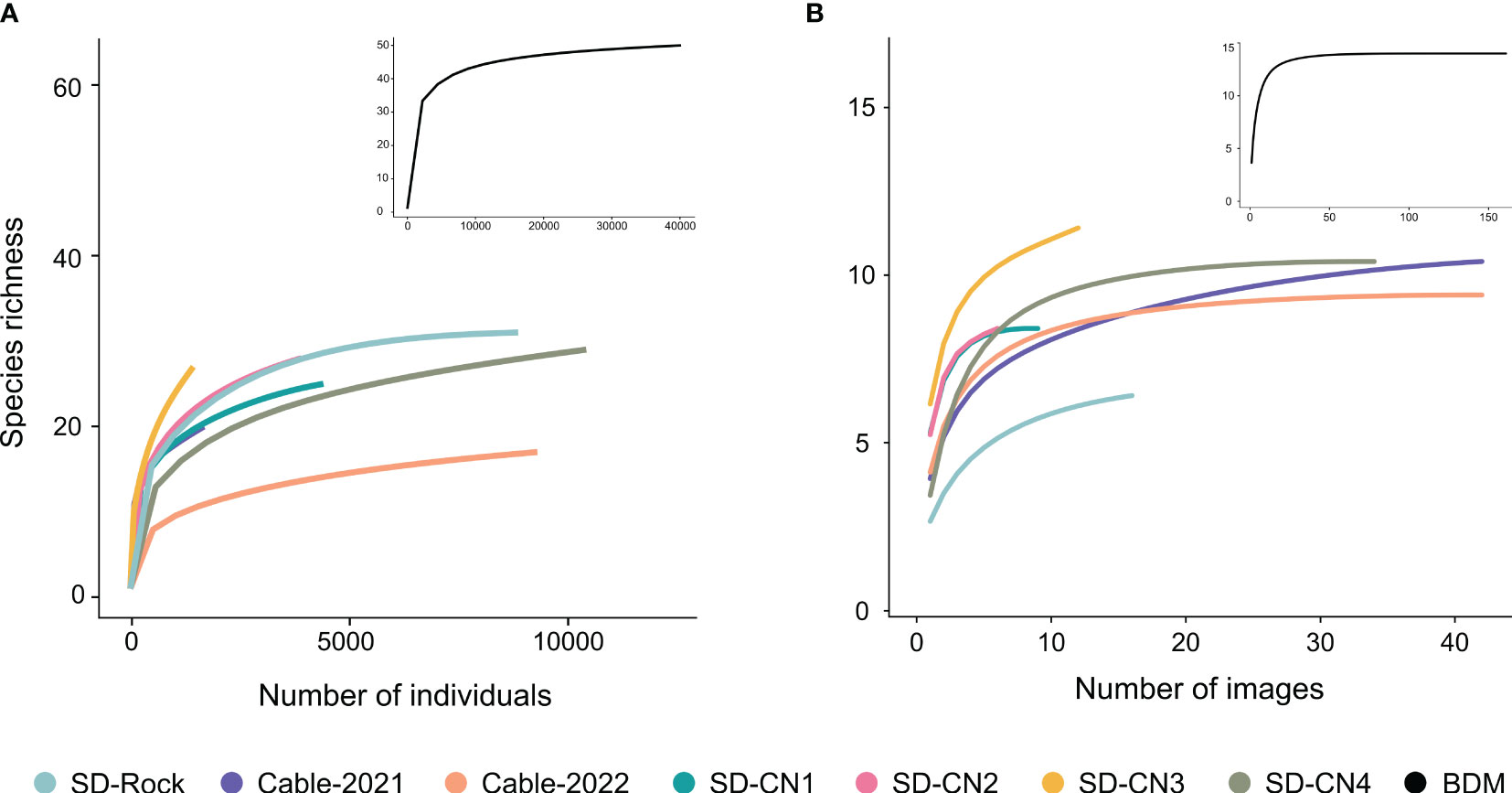
Figure 4 Rarefaction curves for (A) faunal and (B) algal communities within the seven transects at Baie du Marin. Black lines in the inset figures represent the rarefaction curve at the Baie du Marin scale considering all transects combined.
3.2 Crozet benthic communities’ description
The most diversified faunal phyla were Echinodermata (with 8 Asteroidea, 3 Ophiuroidea, 2 Holothuroidea and 1 Echinoidea) and Porifera (with 10 Demospongiae); and the most represented algal phylum was Rhodophyta (with 8 Florideophyceae). A high level of rare faunal taxa was found among transects, ranging from 50 to 82.4% (74.2 ± 10.6%) of the species (Table 3). Rare algal taxa were fewer, ranging from 10 to 60% (33.3 ± 15.5%). More specifically, the SD-Rock transect was dominated by the polychaetes Parasabella sp. (183.3 ± 146.1 ind m-2; 32.4%) and Lanice marionensis (149.2 ± 108.1 ind m-2; 27.1%), the bivalve Kidderia sp. (72.1 ± 68.2 ind m-2; 13.1%) and the ophiuroid Ophiosabine vivipara (67.1 ± 31.7 ind m-2; 12.8%). As for macroalgae, this transect was dominated by Delesseriaceae gen. indet. (45.2%), Macrocystis pyrifera (30.7%) and Chlorophyta sp. (14.1%). On the northern coast of BDM, the transect SD-CN1 was dominated by the high density of polychaete Parasabella sp. (105.7 ± 204 ind m-2; 75.4%), and to a lesser extent by the ophiuroid O. vivipara (7.1 ± 14.5 ind m-2; 5.1%) and the bivalve Kidderia sp. (13.9 ± 22 ind m-2; 4.2%), while algae were mainly represented by Rhodophyta sp.1 (27.2%), Phycodrys quercifolia (23.7%) and Codium adhaerens (14.6%). The SD-CN2 transect was highly dominated by the polychaete Parasabella sp. (481.8 ± 416.1 ind m-2; 82.7%), and to a lesser extent by the ophiuroid O. vivipara (19.3 ± 18.6 ind m-2; 3.5%) and the polychaete Thelepus spectabilis (17.7 ± 26.2 ind m-2; 2.5%); and by Rhodophyta sp.1 (34.4%), M. pyrifera (33.9%) and P. quercifolia (13.4%). Like the SD-CN1 and SD-CN2 transects, SD-CN3 was dominated by the polychaete Parasabella sp. (46.2 ± 58.9 ind m-2; 45.2%), the bivalve Kidderia sp. (34 ± 60.9 ind m-2; 26%) as well as the polychaete T. spectabilis (7.5 ± 12.1 ind m-2; 7.8%); while algae were mainly represented by P. quercifolia (36.2%), C. adhaerens (19.4%), Rhodophyta sp.1 (13.1%) and Ulva sp. (12.9%). Finally, SD-CN4 fauna was dominated by the holothuroid Echinopsolus splendidus (537.3 ± 1300.8 ind m-2; 60.2%), the polychaete Parasabella sp. (66.8 ± 207 ind m-2; 17.2%) and the actiniid Actiniaria sp.6 (129 ± 295.5 ind m-2; 15.5%), while algae were represented by Desmarestia chordalis (45%), C. adhaerens (34.6%), Heterosiphonia berkeleyi (6.1%) and Corallinales (5.7%). For submarine cables transects, the SD-Cable-2021 transect was dominated by the bivalve Kidderia sp. (207.6 ± 418.5 m-2; 54.6%), the polychaete Parasabella sp. (61.8 ± 119 ind m-2; 16.2%) and the sponge Mycale sp.1 (28.4 ± 44.6 ind m-2; 7.1%); and algae were represented by Delesseriaceae gen. indet. (68.2%), D. chordalis (20.6%) and M. pyrifera (5.3%). Finally, the SD-Cable-2022 transect was dominated by a high density of the bivalve Kidderia sp. (3256.9 ± 4.969.4 ind m-2; 84.2%) and to a lesser extent by the polychaete Serpulidae (418.6 ± 1148.5 ind m-2; 12.3%) and the sponge Mycale sp.1 (59.6 ± 120 ind m-2; 1.3%), while algae were represented by Delesseriaceae gen. indet. (48.9%), Desmarestia confervoides (32.9%) and D. chordalis (10.5%).
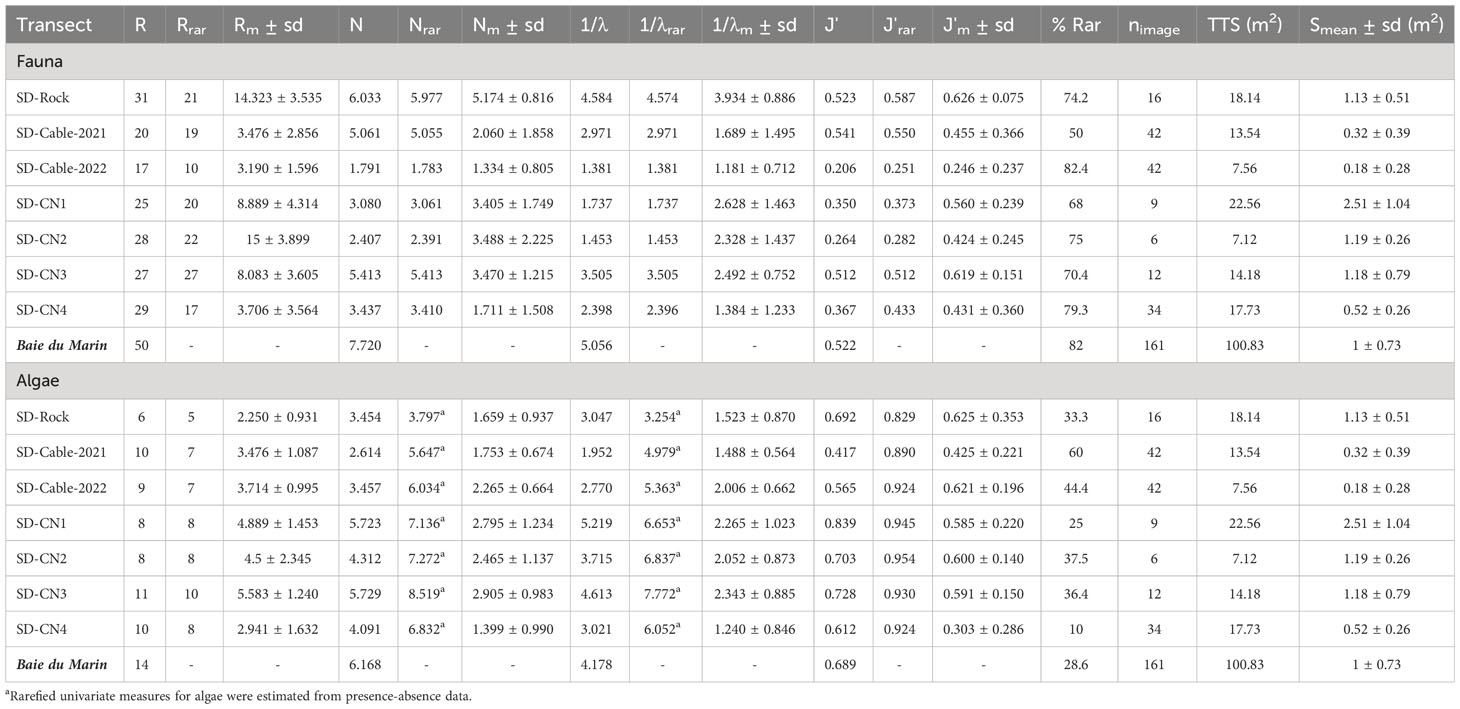
Table 3 Diversity indices of faunal and algal communities for each transect and for Baie du Marin (all transects combined). Diversity indices are taxonomic richness (R), rarefied taxonomic richness (Rrar), mean taxonomic richness by image (Rm), Shannon diversity (N), rarefied Shannon diversity (Nrar), mean Shannon diversity by image (Nm), Simpson diversity (1/λ), rarefied Simpson diversity (1/λrar), mean Simpson diversity by image (1/λm), Piélou’s evenness (J’), rarefied Piélou’s evenness (J’rar), mean Piélou’s evenness by image (J’m) and percentage of rare species (% Rar). The sampling effort is also provided with the number of images analyzed (nimage), transect total surface (TTS) and the mean image surface (Sm). Significance is given by asterisks: (***) p-value ≤ 0.001.
3.3 Taxonomic benthic diversity among and within transects
Baie du Marin hard substrates indicate a high-level of taxonomic richness and diversity for both fauna and algae composition (Table 3). With the exception of SD-Cable-2022, where species richness was low (Rrar = 10), the number of species was similar between transects (Rrar from 17 to 27 taxa). The highest level of diversity was found for the SD-Rock transect (Nrar = 5.977; 1/λrar = 4.574), followed by the SD-CN3 transect (Nrar = 5.413; 1/λrar = 3.505), and to a lesser extent by the SD-Cable-2021 transect (Nrar = 5.055; 1/λrar = 2.971; Table 3). Diversity values on the rock located at the center of the bay were greater (Nrar = 5.977; 1/λrar = 4.574) than those estimated from the northern coast (Nrar = 3.569 ± 1.3; 1/λrar = 2.273 ± 0.912). These patterns at the scale of transects are in line with the average richness and diversity per image. Along submarine cables transects, mean diversity indices per image were relatively low (SD-Cable-2021: Rm = 3.476 ± 2.856; Nm = 2.060 ± 1.858; 1/λm = 1.689 ± 1.595; SD-Cable-2022: Rm = 3.190 ± 1.596; Nm = 1.334 ± 0.805; 1/λm = 1.181 ± 0.712; Table 3). Images of the SD-Rock transect displayed high-levels of average richness and diversity (Rm = 14.323 ± 3.535; Nm = 5.174 ± 0.816; 1/λm = 3.934 ± 0.886), as well as a low spatial variability of these indices (Table 3). With the exception of the SD-CN4 transect, the mean diversity by image of transects conducted on the northern coast of the bay was similar (SD-CN1: Nm = 3.405 ± 1.749; 1/λm = 2.628 ± 1.463; SD-CN2: Nm = 3.488 ± 2.225; 1/λm = 2.328 ± 1.437; SD-CN3: Nm = 3.470 ± 1.215; 1/λm = 2.492 ± 0.752; Table 3). Overall, macrofaunal communities were characterized by low Piélou’s evenness values (J’rar = 0.427 ± 0.131), indicating high dominance of certain faunal taxa (Table 3). For algal communities, species richness was similar between transects and substrates (Rrar from 6 to 11 taxa; Table 3). Nevertheless, the highest value of species richness and diversity indices were found at the SD-CN3 transect (Rrar = 10; Nrar = 8.519; 1/λrar = 7.772), while lowest values were found on the SD-Rock transect (Rrar = 5; Nrar = 3.797; 1/λrar = 3.254). Algal diversity metrics were also lower on the submarine cables (Nrar = 5.841 ± 0.274; 1/λrar = 5.171 ± 0.272) compared to the rocky substrate of the northern coast (Nrar = 7.44 ± 0.743; 1/λrar = 6.829 ± 0.713; Table 3). Overall, the average richness and diversity by image along transects conducted on the northern coast of the Baie du Marin were higher than submarine cables and SD-Rock transects (Table 3). Piélou’s evenness values for algal communities were greater than for faunal communities (J’rar = 0.914 ± 0.042), indicating the greater distribution of algal cover across transects.
Taking into account the spatial heterogeneity of diversity metrics within transects (Figure 5), significant differences were identified in faunal species richness between rocky and submarine cables substrates, except for the SD-CN4 transect (Figure 5A). Overall, no significant variations in faunal species richness were detected between rocky substrate transects. However, the SD-Rock transect has shown significant differences of the faunal species richness and Shannon and Simpson diversity indices with submarines cables (SD-Cable-2021 and SD-Cable-2022) and SD-CN4 transects (Figures 5A, C, E). Globally, no significant differences in the Shannon and Simpson diversity indices were observed among transects conducted on the northern coast of the Baie du Marin (Figures 5C, E). As for species richness, no significant differences of Shannon and Simpson diversity indices were observed between submarine cables transects. For the algal component, no clear patterns were observed for the algal species richness and diversity between rocky and submarine cables transects (Figure 5). The SD-Rock highlighted significant variations of the algal species richness with submarine cables transects, but no significant differences in the Shannon and Simpson diversity indices (Figures 5B, D, F). On the northern coast of the bay, the algal species richness and Shannon and Simpson diversity indices were similar between the SD-CN1, SD-CN2 and SD-CN3 transects, but significant variations were observed between SD-CN4 with both SD-CN1 and SD-CN3 transects (Figures 5B, D, F).
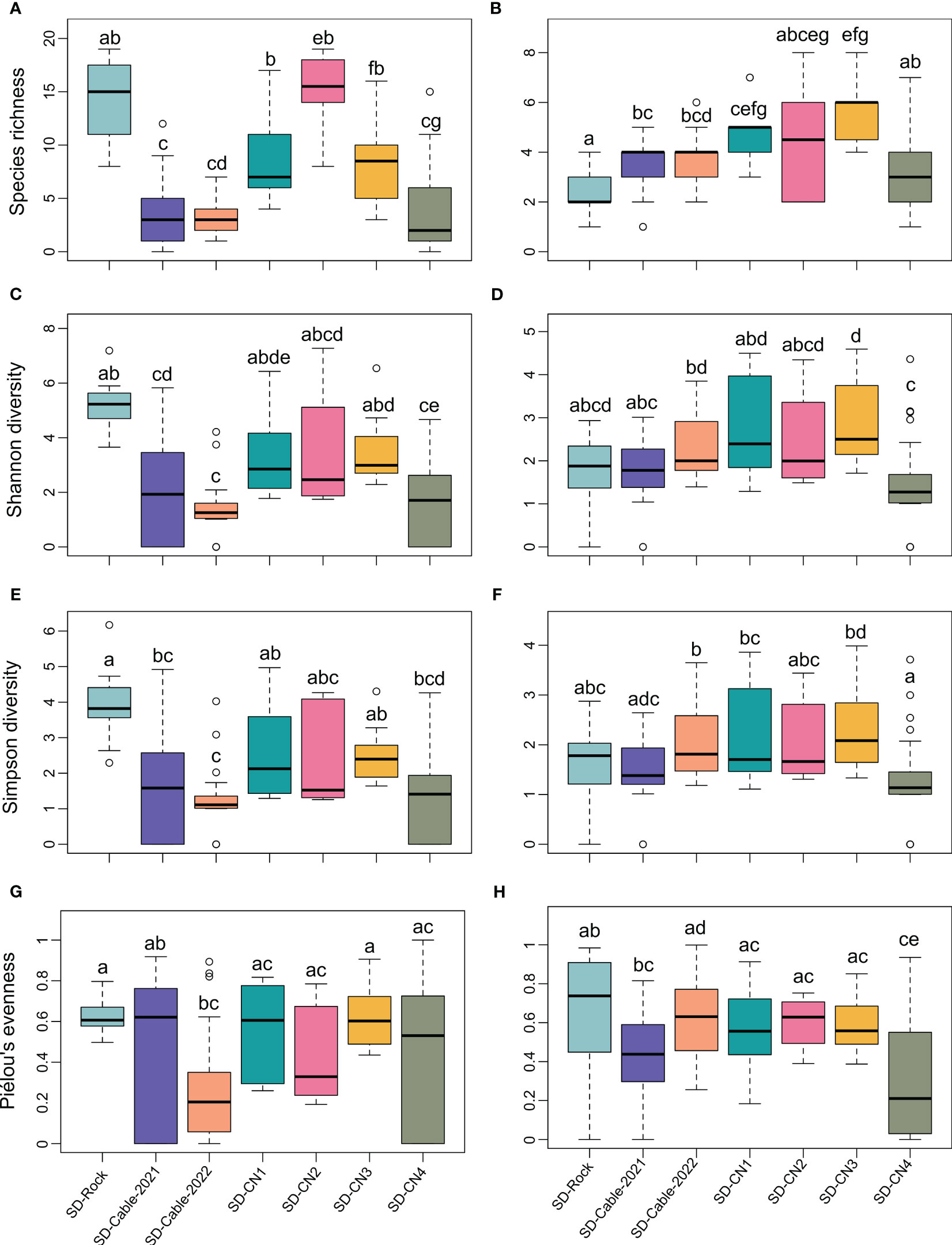
Figure 5 Diversity metrics comparison among the seven transects conducted at Baie du Marin at the image scale, with: species richness for fauna (A) and algae (B); Shannon diversity for fauna (C) and algae; Simpson diversity for fauna (D) and algae (E); and Piélou’s evenness for fauna (G) and algae (H), respectively. Each letter denotes a significantly different result according to the Dunn’s post-hoc pairwise tests.
3.4 Faunal and algal community variation among transects
Principal Coordinates ordination Analyses (PCoA) were performed to assess compositional patterns on faunal and algal communities between transects (Figure 6). For faunal communities, the PCoA highlighted distinct communities between natural (rocks) and artificial (submarine cables) hard substrates (Figure 6A). Although a section of the submarine cables goes through the rock, variation in faunal composition was highlighted between rock (SD-Rock) and submarine cables transects (SD-Cable-2021 and SD-Cable-2022). Overall, no clear differences were found between the four transects conducted on the northern coast of the Baie du Marin. However, faunal communities of the SD-Rock transect have displayed compositional and structural differences from the northern coast and submarine cable transects (Figure 6A). Interestingly, PCoA have shown that transect SD-CN4 harbors two distinct groups of fauna. Similar patterns were observed for algal communities (Figure 6B). With the exception of the SD-Rock, an important algal community variation was observed between natural and submarine cables substrates. This algal heterogeneity was found between transects from the center of the bay (SD-Rock, SD-Cable-2021 and SD-Cable-2022) and those on the northern coast of the bay (SD-CN1, SD-CN2, SD-CN3 and SD-CN4) (Figure 6B). However, no differences were observed between transects conducted on the northern part of the Baie du Marin.
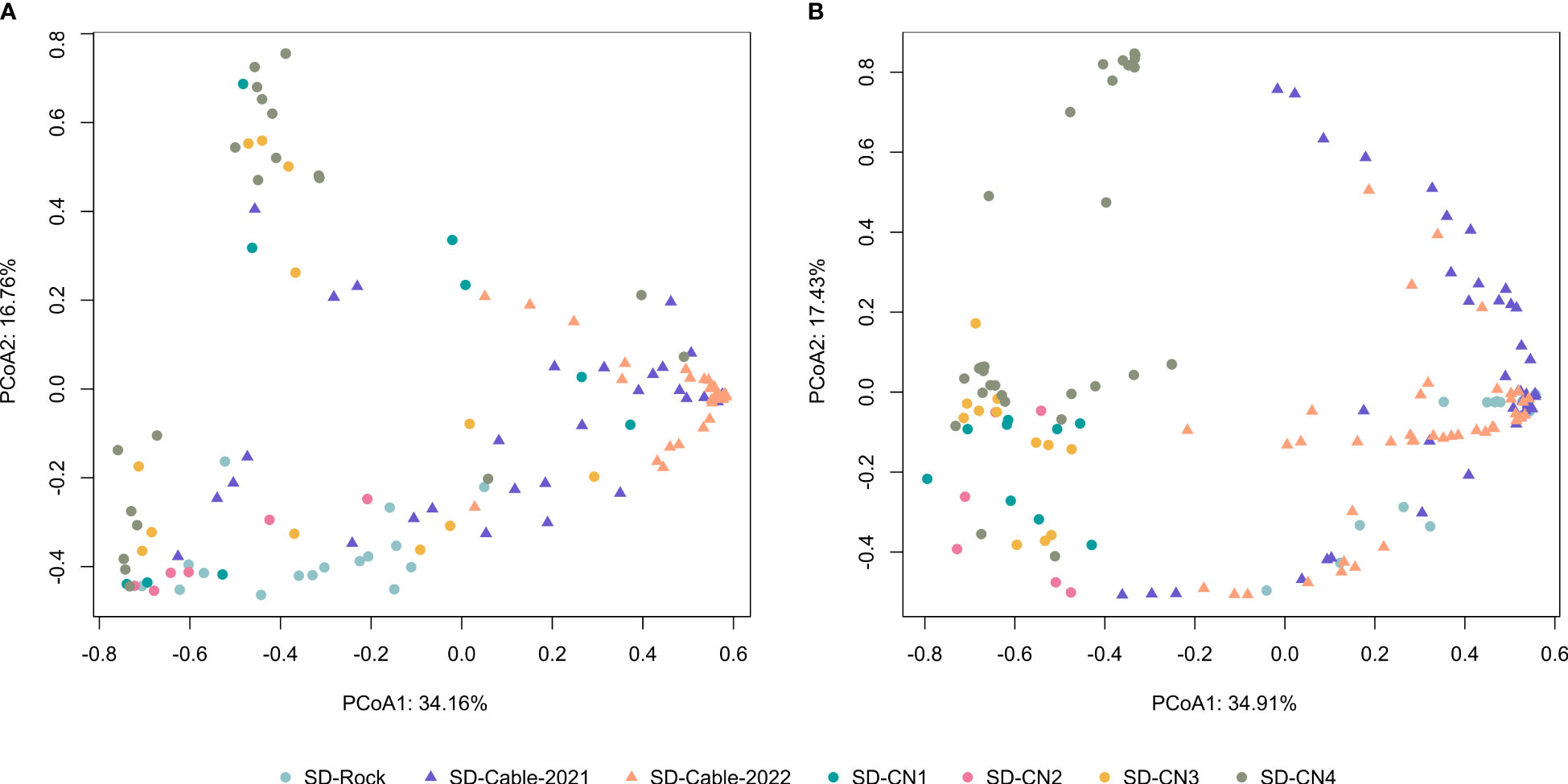
Figure 6 Principal Coordinates ordination Analysis (PCoA) based on Hellinger-transformed (A) faunal and (B) algal densities. The first two principal component analysis axes captured 50.92% and 52.34% of the total variance of Hellinger-transformed species faunal and algal composition, respectively.
3.5 Influence of algal cover on species diversity within transects
A Redundancy Analysis (RDA) was performed to characterize the influence of algal cover on faunal communities, and the algae distribution across transects (Figure 7). The RDA discerns four groups of co-occurring algae corresponding to: (i) Schizoseris sp., Rhodophyta sp.2, Desmarestia confervoides and Delesseriaceae gen. indet.; (ii) Macrocystis pyrifera and Chlorophyta sp.; (iii) Phycodrys quercifolia, Ulva sp., Codium adhaerens, Falklandiella harveyi and Rhodophyta sp.1; and (iv) Heterosiphonia berkeleyi, Desmarestia chordalis and Corallinales. Cables transects were generally characterized by the presence of algae in the group (i). The presence of a high surface cover generated by D. confervoides and Delesseriaceae gen. indet. was correlated to high densities of the bivalve Kidderia sp. The SD-Rock transect, characterized by high densities of the polychaetes Parasabella sp. and Lanice marionensis, as well as the ophiuroid Ophiosabine vivipara, was dominated by algae of the group (ii) and by P. quercifolia. Overall, transects conducted on the northern coast of the Baie du Marin have a greater levels of algal diversity, and more specifically to algae groups (iii) and (iv). The RDA highlighted that the distribution of the gastropod Margarella violacea is associated with the presence of C. adhaerens, A section of the SD-CN4 transect exhibited a high surface coverage of D. chordalis and Corallinales, while another section was mainly dominated by P. quercifolia. Corallinales are associated with the presence of high densities of the holothuroid Echinopsolus splendidus and the actiniid Actiniaria sp.6. The occurrence of the gastropod Nacella delesserti was also related to the presence of D. chordalis and H. berkeleyi algae.
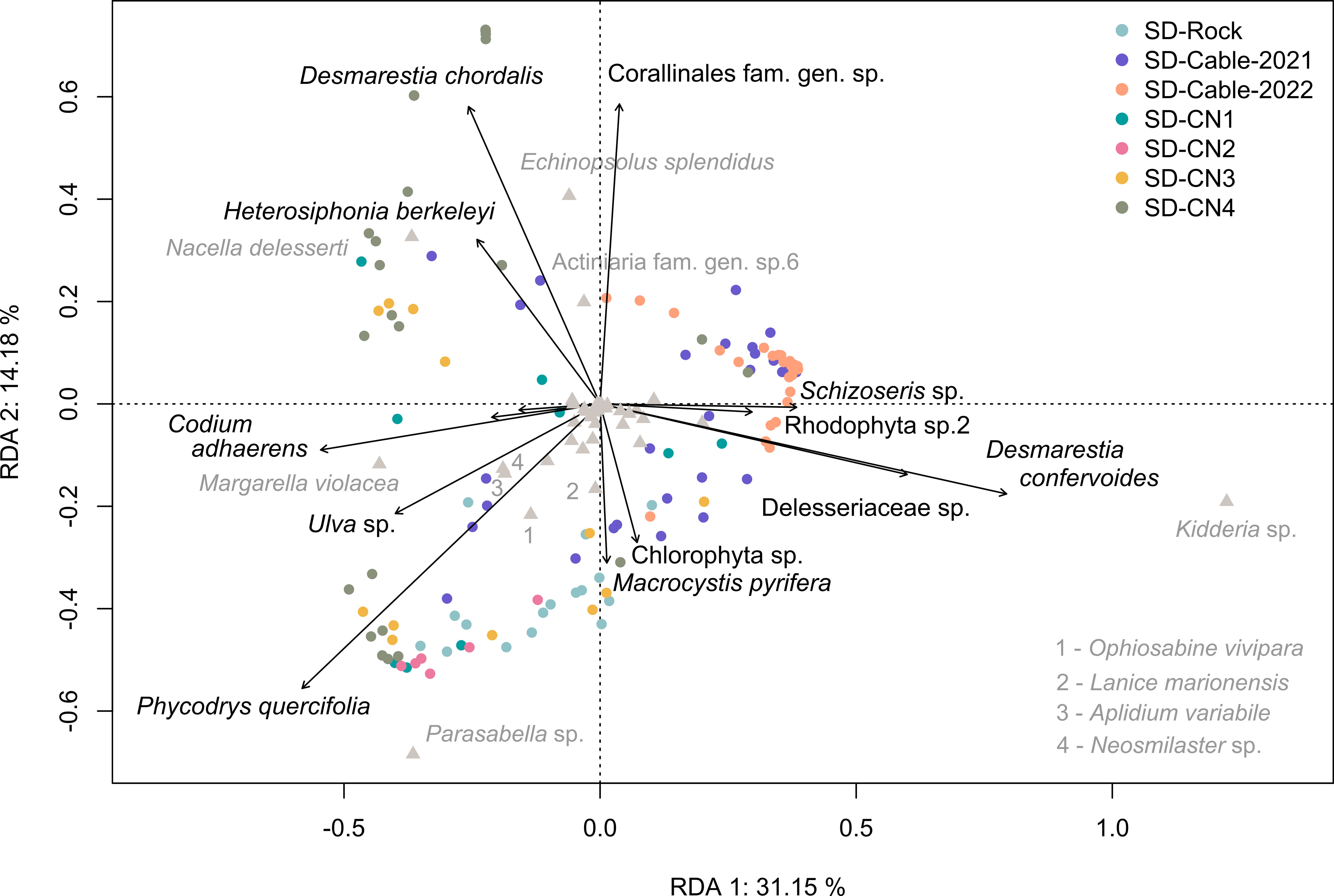
Figure 7 Redundancy analysis (RDA) between the Hellinger-transformed faunal communities and the algae surface cover across the different transects. The first two principal component analysis axes represented account together for 45.33% of the total variance.
3.6 Richness and diversity contributions across spatial scales
The additive partitioning of the Baie du Marin faunal richness (γ = 50) showed that the β2-diversity had the greatest contribution to total faunal richness (49.4%), followed by β1-diversity (38.8%) and α-diversity (11.8%) components (Figure 8A). The observed richness per image (αobs= 5.895) and among images within transects (β1obs = 19.390) were different and lower than expected if taxa were randomly distributed (Table 4; Figure 8A). The observed richness among transects was on the other hand, significantly higher than expected (β2obs = 24.714; p = 0.001). For Shannon diversity, β2-diversity (53.6%) contributed the most to the Baie du Marin diversity, followed by α-diversity (28%) and β1-diversity (18.3%) (Figure 8A). Alpha-diversity was lower than randomly expected by the null model. However, β1-diversity (β1obs = 1.415) and β2-diversity (β2obs = 4.14) were significantly higher than expected (p = 0.001). A similar pattern was observed for Simpson diversity (Table 4). For algal communities, the additive partitioning of the Baie du Marin richness (γ = 14) showed a similar contribution of β1-diversity (37.3%) and β2-diversity (36.7%), followed by α-diversity (26%) (Figure 8B). The additive partitioning shows that richness among images within transects (β1obs = 5.224) and richness among transects (β2obs = 5.143) were significantly greater than expected (p = 0.010) (Table 4). For Shannon diversity and Simpson diversity, similar patterns were observed with a greater contribution of β2-diversity (45.1% and 41.9% respectively) to the Baie du Marin diversity, followed by the contribution of β1-diversity (30.9% and 30.2% respectively) and α-diversity (24% and 27.9% respectively; Table 4; Figure 8B). Similar patterns of greater than expected β1 and β2 components were observed for both Shannon and Simpson diversity indices (Table 4).
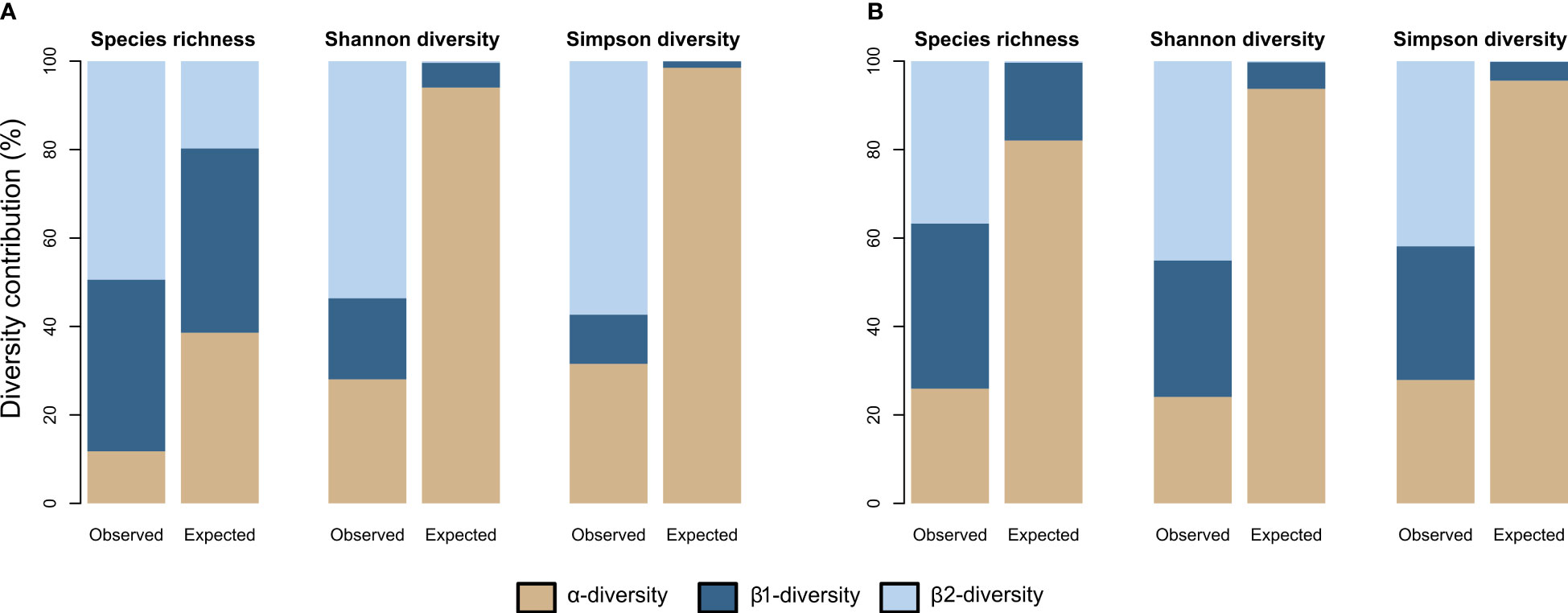
Figure 8 Observed and expected contribution, expressed in percentage values, of each spatial scale to the Baie du Marin richness (q=0) and diversity (q=1 and q=2) for (A) faunal and (B) algal communities.
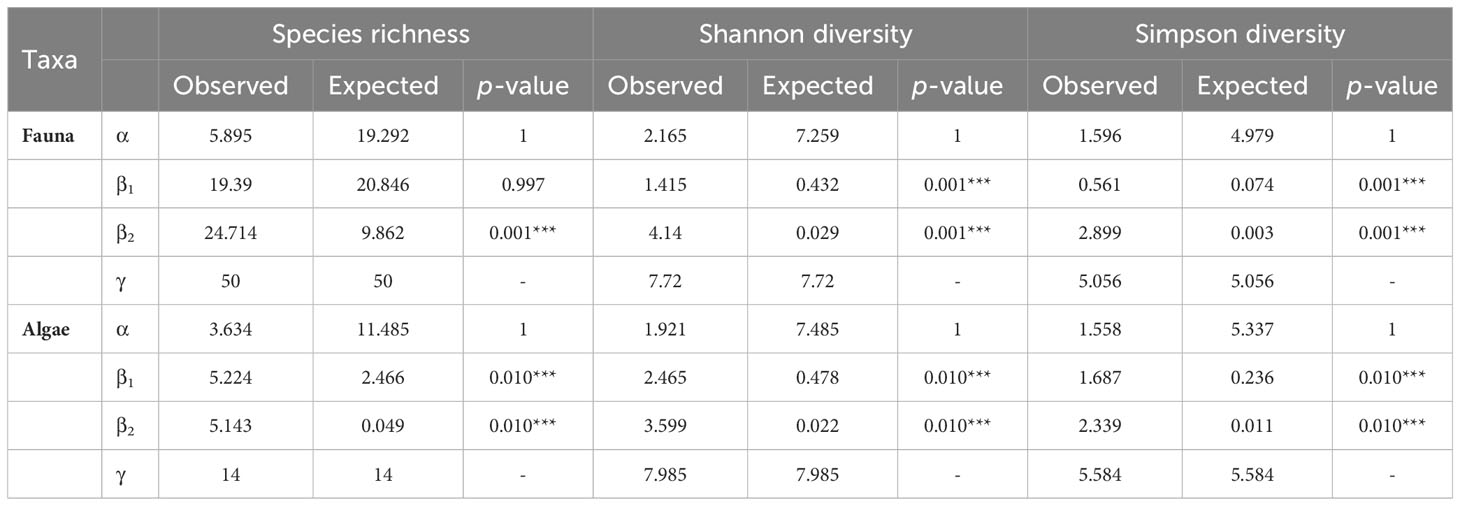
Table 4 Additive partitioning of the Baie du Marin richness and diversity of faunal and algal communities. α-diversity corresponds to the mean value per images, β1-diversity corresponds to the value among images within transects and β2-diversity to the value among transects. P-values were obtained by comparing observed values to expected values draw from a random distribution (9999 iterations).
3.7 Functional diversity of benthic faunal communities
The Principal Component Analysis (PCA) illustrated the trait distribution between faunal taxa (Figure 9) and trait densities within transects at Baie du Marin (Figure 10). PCA indicates that dominant species are characterized by a suspension-feeding (active or passive) mode. Active suspension feeders are mainly represented by bivalves and demosponges, while passive suspension feeders correspond to polychaetes and holothuroids (Figure 9). Despite lower abundances, a high diversity of predators, mainly represented by Asteroidea taxa, occurs within faunal assemblages. For trait densities distribution within transects, the PCA showed that submarine cables transects were characterized by attached, active suspension feeders and very small and solitary taxa with a direct development (Figure 10). In contrast, rock and northern coast transects display similar functional traits, dominated by habitat-forming and tube-dwellers taxa with a medium size and passive suspension feeders, as well as gregarious behavior, hermaphrodite reproduction, and lecithotrophic development mode (Figure 10).
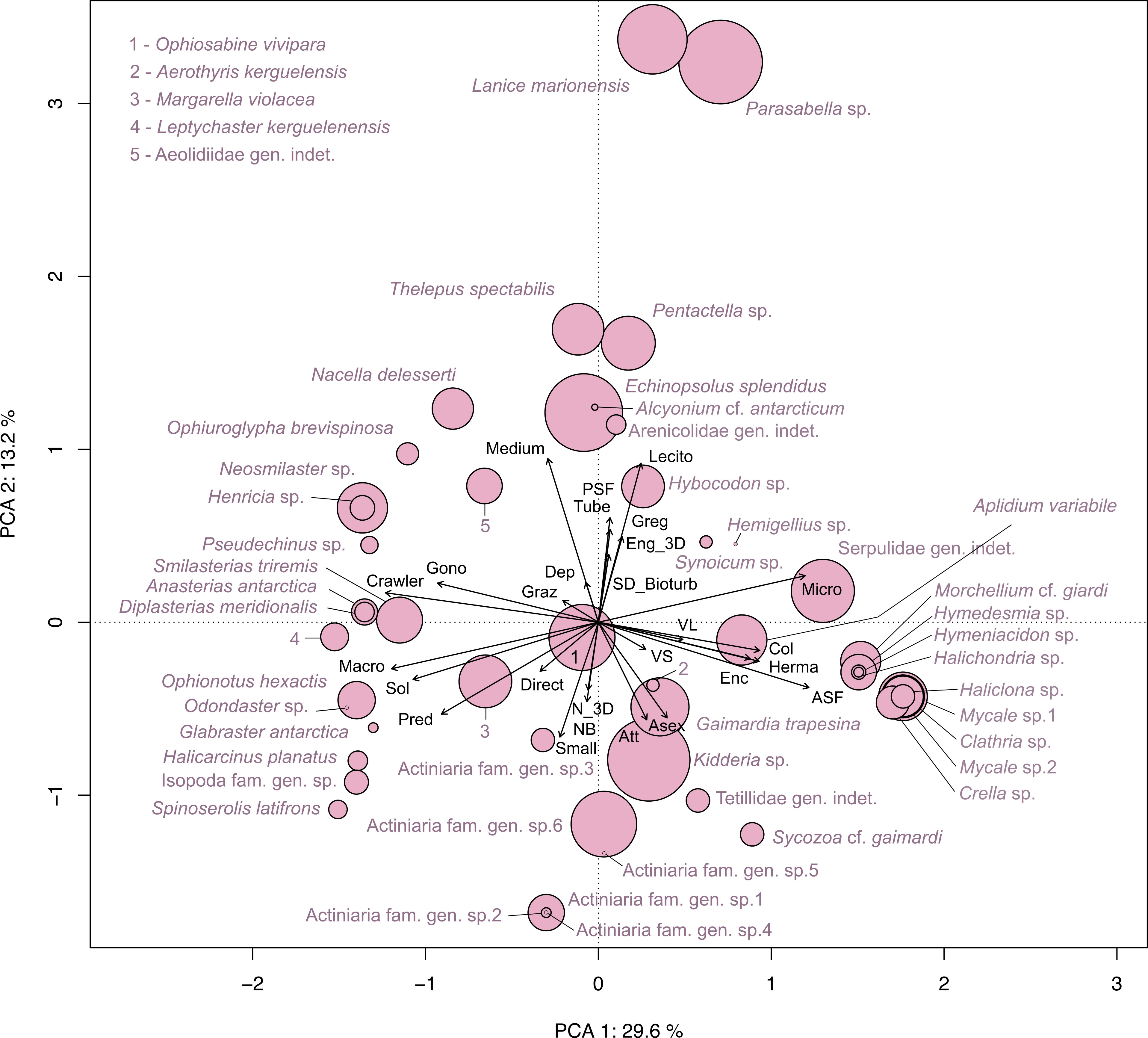
Figure 9 Principal Component Analysis (PCA) on functional trait distribution in faunal taxa identified within the Baie du Marin weighted by their abundances. The first two PCA axes jointly account for 42.8% of the total variance.
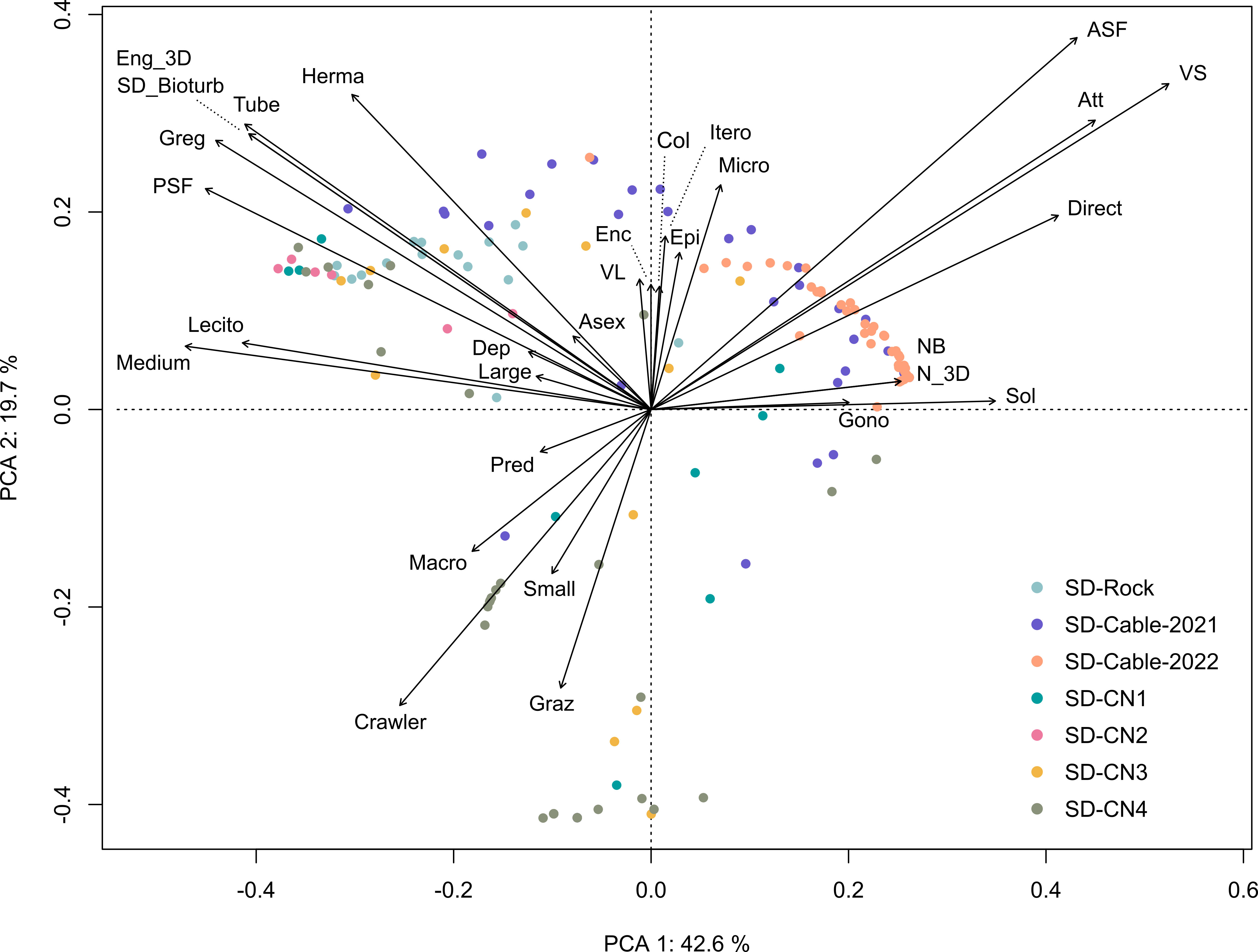
Figure 10 Principal Component Analysis (PCA) of the Hellinger-transformed trait modality densities within transects. The first two principal component analysis axes represented account together for 62.3% of the total variance of Hellinger-transformed trait composition.
Rocky substrate habitats displayed the greatest functional richness values, whereas transects on the submarine cables were characterized by lower levels of functional richness (Table 5; FRic). Faunal abundances were distributed in a similar pattern between transects (similar FEve and FDiv), with clustered abundances (low FEve) and higher abundances at the edges of the trait space (high FDiv) (Table 5; FEve and FDiv). The higher functional dispersion (FDis) and Rao’s quadratic entropy (RaoQ) values were found at the SD-CN3 transect, followed by SD-Rock and SD-Cable-2021 transects, whereas lower transect values were found at SD-Cable-2022, followed by SD-CN2 and SD-CN1 transects (Table 5; FDis and RaoQ). Collectively, all transects have shown a low functional redundancy (FR), ranging from 0.162 to 0.464 (0.301 ± 0.116) (Table 5; FR).
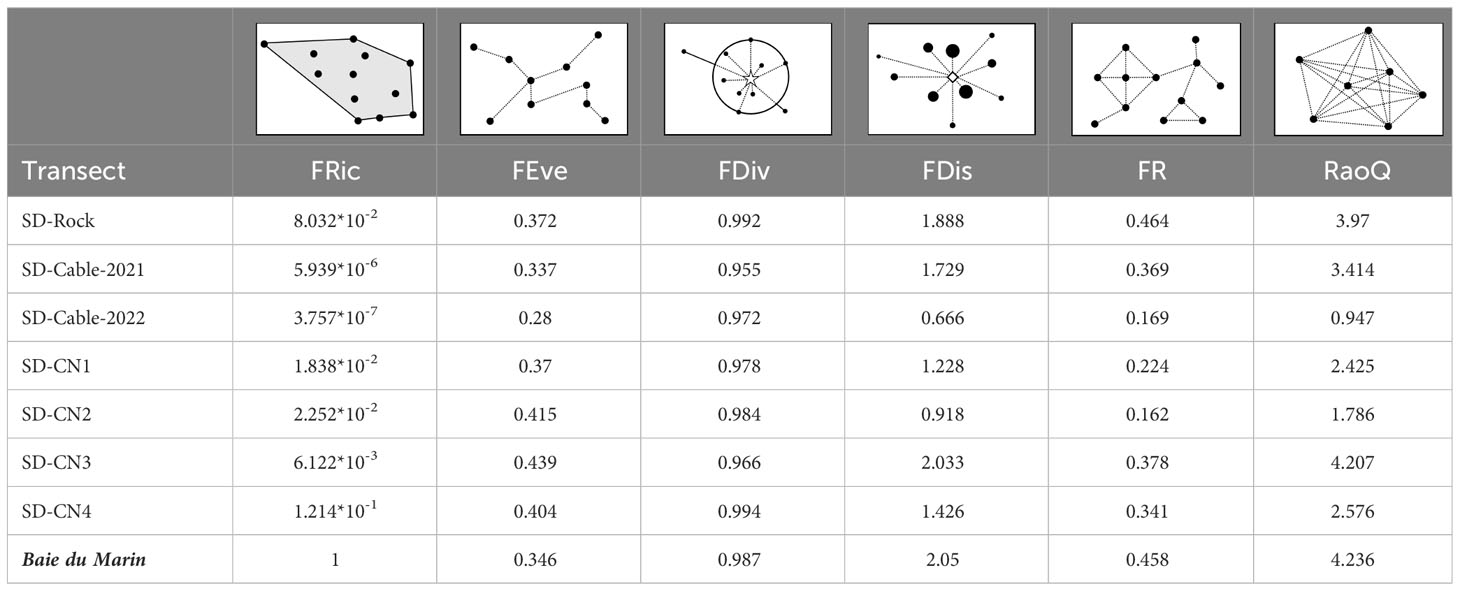
Table 5 Faunal functional diversity between transects conducted on hard substrates at Baie du Marin, with: functional richness (FRic), functional evenness (FEve), functional divergence (FDiv), functional redundancy (FR), Rao’s quadratic entropy (RaoQ), and their respective geometric representations.
4 Discussion
4.1 Description of hard substrate benthic communities
Crozet hard substrates have shown high faunal and algal diversity, highlighting the significant ecological value of these habitats for the preservation of marine polar ecosystems. Similarly to other Antarctic and sub-Antarctic islands (Downey et al., 2012), Demospongiae constitute a dominant component of Crozet marine benthic communities. Sponges provide a high habitat structural complexity and heterogeneity (Thrush et al., 2006), and also act as food resource and substrate for a great variety of faunal species (Gutt and Schickan, 1998; McClintock et al., 2008). The high diversity of echinoderms and more specifically sea stars (Asteroidea) is also characteristic of subtidal Southern Ocean benthic communities, where these organisms play an important role in trophic interactions and ecosystem functioning (McClintock, 1994; Le Bourg et al., 2022). Baie du Marin rocky substrates are covered by high densities of tube-dwellers polychaetes such as the terebellid Lanice marionensis, on which the Aeolidiidae nudibranchs feed, and the sabellid Parasabella sp. Interestingly, high-level densities of the polychaete Lanice marionensis were previously reported at Crozet, Marion and Prince Edward islands, but were not encountered at Kerguelen Islands (Sicinski and Gillet, 2002). These high polychaete densities found in Crozet benthic communities are often associated with high densities of the ophiuroid Ophiosabine vivipara. This brittle star is common on rocky substrates in the sub-Antarctic region, as reported at Prince Edwards Islands (Branch et al., 1993) and at Saint-Paul and Amsterdam Islands (O’Hara and Thuy, 2022). Important populations of the holothuroid Echinopsolus splendidus were also found on rocky substrates at shallow depths on the northern coast of the Baie du Marin. This species was also reported in the Antarctic region (O’Loughlin et al., 2011). Although some characteristic groups of sub-Antarctic rocky shores such as barnacles (e.g., genus Balanus) and mussels (e.g., genera Aulacomya and Mytilus) have been reported at Bounty Island (Freeman et al., 2011) and Kerguelen Islands (Arnaud, 1974), they appear to be absent in sampling areas of Crozet. The crab Halicarcinus planatus, only member of the Hymenosomatidae family that inhabits shallow subtidal waters of sub-Antarctic islands (Arnaud, 1974; Beckley and Branch, 1992; Branch et al., 1993), was identified at Baie du Marin. Extended stands of the giant kelp Macrocystis pyrifera are predominant in nearshore subtidal ecosystems of Kerguelen compared to the less widespread beds at Crozet. However, the composition of the marine vegetation identified at Crozet appears to be closely similar to algal taxa reported in coastal waters of the Kerguelen Islands, with a dominance of Rhodophyta species (Féral et al., 2021). As reported from the Beagle Channel at Tierra del Fuego (Adami and Gordillo, 1999), the bivalve Gaimardia trapesina is often found attached to the fronds of M. pyrifera. This bivalve is an emblematic species of nearshore Antarctic and sub-Antarctic waters. It has already been identified in numerous areas such as Antarctic Peninsula, Tierra del Fuego, Falkland Islands, Magellan Strait, South Georgia, Marion, Prince Edward, Kerguelen and Crozet islands (Dell, 1964; Branch et al., 1991; Adami and Gordillo, 1999; Puccinelli et al., 2018). High densities of the bivalve Kidderia sp. occur on fronds of Desmarestia confervoides and Delesseriaceae gen. indet. algae at Baie du Marin. Finally, Codium adhaerens habitat seemed to favor the presence of the grazer gastropod Margarella violacea.
Transects undertaken on the northern coast of the Baie du Marin highlighted a unique vertical zonation of benthic communities. From 0 to 3 m depth, the bedrock is mostly covered with crustose coralline algae and host high densities of the holothuroid Echinopsolus splendidus and actiniid Actiniaria sp.6. Like in the temperate sub-Antarctic islands where these calcifying algae dominate extensive areas with small invertebrates such as anemones and bryozoans (Freeman et al., 2011), or at Marion Island where they are abundant on boulders (Gon and Mostert, 1992), crustose coralline are characteristic of sub-Antarctic subtidal marine communities. From 3 to 7 m depth, the rocky plateau is dominated by a dense bed of canopy-forming D. chordalis, with corallines and Codium adhaerens covering the bottom. This canopy formed by algae could be hiding additional levels of benthic diversity, which could not be assessed using imaging techniques. From 7 to 8 m depth, the rock wall is inhabited by high abundance and diversity of faunal species, structurally dominated by high-level densities of the polychaete sabellid Parasabella sp. and ophiuroid Ophiosabine vivipara to a lesser extent. From 8 to 10 m depth, the rocky shore is mainly inhabited by C. adhaerens associated with the gastropod M. violacea. An important algal diversity is present in this area, including Phycodrys quercifolia, Ulva sp. and Macrocystis pyrifera. As reported at the Kerguelen Islands (Arnaud, 1974) and along the coastline of the sub-Antarctic Magellan region (Ríos et al., 2007), kelp holdfasts support high species richness and abundance of a wide range of invertebrate taxa compared with the surrounding environment. Approaching to the seafloor, the rocky substrate leaves place to vast sand stretches that are characteristic of the Baie du Marin.
4.2 Distribution of taxonomic diversity between transects and substrates
While species richness among rocky transects were substantially similar with each other and greater than transects performed on the submarine cables section, our results show compositional and structural differences between transects and substrates within the bay. High densities of the terebellid polychaete Lanice marionensis were found on rock located at the center of the bay. In contrast, benthic communities of the northern coast are dominated by high densities of the sabellid polychaete Parasabella sp. The presence of these passive suspension feeders polychaetes in high densities suggests a surrounding habitat rich in food sources, including phytoplankton, resuspended microphytobenthos, bacteria, and/or non-living organic particles (Jumars et al., 2015). We propose that particle capture and sediment deposition provided by these polychaetes could facilitate the presence in high abundance of the deposit feeder ophiuroid Ophiosabine vivipara. Structural and compositional differences in benthic communities between these areas could also reflect variation in environmental and hydrodynamic conditions within the bay. The greatest diversity found on the rock (SD-Rock) may also be attributed to the dense aggregations of the tube-building polychaete L. marionensis. As reported for Lanice conchilega (Rabaut et al., 2007; Van Hoey et al., 2008; Rabaut et al., 2009), this polychaete adds heterogeneity and complexity within the ecosystem, acting as a habitat-structuring species for a wide variety of organisms. In addition, hard substrates between the northern coast and the center of the bay were characterized by distinct macroalgal assemblages. Algae are a key component of benthic ecosystems and contribute greatly to the functioning of marine environments through their involvement in biogeochemical (especially carbon, nitrate and phosphorus) and nutrient cycles, both in water column and sediments. While algal physical characteristics can directly influence local conditions (e.g., light accessibility, oxygen concentration, particle deposition), their metabolism can also act on the ambient environment (Delille et al., 2009). Through physical structure and metabolism, algae generate important habitat modifications and create a multitude of ecological micro-niches that support high-levels of diversity, by providing a surface for larval settlement and colonization, refuges from predation and physical disturbance, food resources and nursery sites, thereby influencing directly or indirectly the faunal distribution and community structure in Antarctic (Gambi et al., 1994; Amsler et al., 2014) and sub-Antarctic (Graham et al., 2007; Andrade et al., 2016) ecosystems. In light of these results, we suggest that the interaction between rocky substrate and habitat-forming species (e.g., faunal and macroalgae physical architecture), increase habitat complexity and heterogeneity at Baie du Marin. This spatial segregation of environmental conditions and nutrient resources within these complex habitats allows for species coexistence and promotes high levels of taxonomic diversity.
Most studies assess the biological effects of cable installation as transient and relatively minor (Andrulewicz et al., 2003; Kogan et al., 2006; Dunham et al., 2015; Sherwood et al., 2016). For instance, in the case of the Acoustic Thermometry of Ocean Climate (ATOC)/Pioneer Seamount cable in the nearshore waters of California, a significant increase in the abundance of sea anemones on the cable was the main biological impact observed (Kogan et al., 2006). However, other studies have reported significant changes in species behavior due to submarine cables (Westerberg and Lagenfelt, 2008; Love et al., 2015; Love et al., 2017). At Crozet, our results show that the composition and structure of submarine cables faunal communities are different from the surrounding environment. Benthic species richness on submarine cables is similar between the two surveyed years. However, images of the suspended cables section in 2022 display a large algal development compared to images taken in 2021, which could explain the differences observed in diversity indices. Polychaetes Lanice marionensis and Parasabella sp. and ophiuroid Ophiosabine vivipara are abundant on the rock where the cables are laid, whereas only a few individuals are found on cables. Similarly to the Basslink High Voltage Direct Current (HVDC) cable in south-eastern Australia (Sherwood et al., 2016), a high diversity of sponges had colonized the cable surface. We suggest that suspended cables introduce heterogeneity within the ecosystem and promote the settlement of certain species. In addition to providing a new surface to colonize, we hypothesized that suspended cables are out of the reach for benthic consumers and thus influence biotic interactions by reducing predation pressure compared to the natural substrate on the seabed. This lowered predation pressure could facilitate the establishment of non-native and potential invasive species (Dumont et al., 2011). However, macroalgae diversity was similar between the rock and artificial substrates, but different from the northern part of the Baie du Marin. This difference may be explained by the depth variations between these areas. Overall, the suspended cables in the bay promoted the development of epifaunal biota in an area dominated by soft sediments, by providing a new colonization surface. This resulted in a composition and structure of faunal communities that differ from their surrounding environment.
Climate change and anthropogenic activities are major factors increasing the risk of biological invasion in polar marine ecosystems (McCarthy et al., 2019). Many studies have reported that the composition and structure of benthic communities differed between natural and artificial substrates, with a greater number of non-native species on artificial structures (Connell and Glasby, 1999; Glasby et al., 2007; Airoldi et al., 2015). Consequently, artificial structures may constitute a serious threat to the native biodiversity, highlighting the importance of monitoring programs to detect the presence of non-native species and prevent the spread of invasive species.
4.3 Richness and diversity partitioning: a high spatial heterogeneity
The greater contribution of the β2-diversity (among transects) to the Baie du Marin richness and diversity highlights that faunal species composition varied the most among transects. At larger spatial scales, β2-diversity was greater than expected under the null model, whereas values for finest scales (α and β1-diversity) were lower. These results indicate that faunal communities are not randomly distributed between transects, and that species distribution within these transects tends to be spatially structured as patches at a very small scale (approximately 1 m2). This aggregated distribution at fine spatial scales was also observed on Antarctic coastal habitats (Gili et al., 2001; Teixidó et al., 2002; Gutt et al., 2019; Rovelli et al., 2019) and could be the result of multiple ecological processes, including local environmental filtering (e.g., hydrodynamic conditions, bottom topography, substrate nature and inclination, depth) (Gutt et al., 1999; Cárdenas and Montiel, 2015), biotic interactions (e.g., competition, predation, facilitation) (Valdivia et al., 2014), and species biological traits (e.g., gregarious behavior, dispersal limitations). Our study’s results call for further studies to investigate the abiotic environment and species biology. In contrast to species richness, α-diversity has also shown a greater contribution to the Baie du Marin Shannon and Simpson diversity, which could be the result of the engineer species presence – such as habitat-formers Lanice marionensis and Parasabella sp. – that enhance species abundance and diversity. In addition to complex substrate micro-topography, biogenic structures provide a greater number of potential microhabitats at finer scales, promoting local variation of environmental conditions and food partitioning, and thus enabling high-level diversity (Jones et al., 1994). Similarly to faunal communities, β1-diversity and β2-diversity have shown closely matched and high-level contributions to the Baie du Marin algal richness. However, all spatial scales have shown a similar contribution to the Baie du Marin Shannon diversity. As reported in other studies (Gering et al., 2003; Valdivia et al., 2014; Gutt et al., 2019), these findings corroborate the importance of accounting for spatial scale in preserving sub-Antarctic habitats and their unique biodiversity for conservation policies. Multi-scale analyses are essential to ensure a better understanding of the local and regional processes that shape benthic assemblages (Lamy et al., 2018). These results provide useful information for decision-makers to design effective management strategies and sustain benthic diversity, ecosystem health, and resilience (Gering et al., 2003).
4.4 Functional diversity of Crozet benthic ecosystems
The trait-based approach constitutes an ecological bridge between the structure of species assemblages and ecosystem functioning (Mouchet et al., 2010). Faunal communities of Crozet shallow water habitats are dominated by suspension-feeders. This prevalent high diversity of suspension-feeders was also reported at the Kerguelen Islands (Améziane et al., 2011) and could play an important role in the recycling of nutrients in the water column (Orejas et al., 2000). Crozet benthic communities have shown a high number of species that have a lecithotrophic larval stage during development. Such species produce large eggs with rich and great amounts of food resources necessary for the effective embryonic and larval development (Pearse et al., 1991; Pearse and Lockhart, 2004). The predominance of lecithotrophy at Crozet is a life trait shared by many invertebrates in the Southern Ocean and in deep-sea environments (Lau et al., 2020). In addition, Crozet faunal communities show a high number of sessile organisms and if biological information on species lifespan are limited, we presume that these communities are likely characterized by slow growth rates, as reported in Southern Ocean marine ecosystems (Teixidó et al., 2004; Smale and Barnes, 2008). All these functional characteristics make these communities potentially vulnerable to changes in environmental conditions (Degen et al., 2018).
Overall, Crozet benthic communities have low values of functional richness (FRic), even more so for benthic communities located on the submarine cables substrate. Mason et al. (2005) reported that low FRic and low functional evenness (FEve) could indicate that some resources availability may be under- or not used by species. These low indices values, and consequently the dominance of some functional traits, could also be explained by the presence of few resources in sufficient abundance in which benthic communities are specialized (Boyé et al., 2019). While taxonomic results have shown that faunal communities present on the submarine cables differed from natural hard substrates, these functional characteristics could increase the establishment of non-native species and decrease the invasion resistance (Mason et al., 2005). Some recent studies have highlighted that the introduction of artificial substrates could facilitate biological invasions (Glasby et al., 2007; Macreadie et al., 2011; Adams et al., 2014). In addition, the low FEve and high functional divergence (FDiv) found in Crozet benthic communities are characteristic of communities where dominant species share similar traits that are generally different from all other species (e.g., sessile tube dwellers), and that a large part of functional trait space is occupied by rare species with rare traits. Therefore the distinctiveness of rare species functional traits, compared to the species pool, may have a significant role in the ecosystem functioning (Mouillot et al., 2013). The loss of species with distinctive traits may deeply affect ecosystem functioning, and several studies highlight the importance of considering rare species as a conservation priority (Ellingsen et al., 2007; Mouillot et al., 2013; Boyé et al., 2019). In addition to the low FEve and FDiv, benthic communities at Baie du Marin were characterized by low functional redundancy (FR). FR proposes that ecosystems characterized by high degree of species which share similar functional traits, are less susceptible to environmental upheavals or species loss (Schmera et al., 2017). Based on this concept, functional redundancy is a good proxy of ecosystem resilience. The low FEve and FR measured on faunal communities highlight a high vulnerability of the functional stability of Crozet benthic ecosystems. The taxonomic diversity found at Baie du Marin is associated with a redundancy of a few functions (e.g., suspension feeders, lecithotrophy), a pattern called functional over-redundancy (Mouillot et al., 2014). Depending on the interplay between species abundance and the rarity of functional traits, this disproportionate grouping of species in few particular functions leaves many ecological functions highly vulnerable (i.e., supported by just one or few species) (Mouillot et al., 2014; Boyé et al., 2019). As reported in the Western Antarctic Peninsula (Robinson et al., 2022), these results show that the functional diversity of Crozet benthic marine ecosystems may be highly vulnerable to species loss due to future environmental changes in sub-Antarctic region, including anthropogenic threats (Campos et al., 2013), biological invasion (McCarthy et al., 2019) and climate change (Auger et al., 2021).
5 Conclusion
This study provides an important description and analysis of the structure and distribution of nearshore subtidal benthic communities associated with hard substrates at Baie du Marin, and the first to assess the functional diversity of Crozet archipelago benthic marine ecosystems. Combined taxonomic and biological trait analyses provide a comprehensive understanding of marine ecosystem functioning and community structure. Our results show that the use of a non-destructive method, underwater imagery, is a powerful surveying technique to investigate patterns in marine organism distribution and sub-Antarctic ecosystems functioning. Overall, hard substrates constitute complex habitats harboring a rich faunal and algal diversity, with a high spatial heterogeneity at the Baie du Marin scale. The structure and composition of faunal communities on rocky shores were distinct from those of the submarine cables, where high sponge diversity occurs. No algal community differences were found between the rock located at the center of the bay and submarine cables. However, the algal composition of these sites differs from the northern part of the Baie du Marin. Further studies of environmental variables and biotic interactions that shape benthic communities would be a valuable contribution to improve our knowledge of the functioning of these ecosystems.
The high sensitivity of sub-Antarctic regions due to accelerating climate change is expected to impact coastal marine habitats of numerous sub-Antarctic islands – including the Crozet archipelago – through the multiple and synergistic effects of factors such as seawater temperature increase, ocean acidification, and extreme climatic events. Current taxonomic and functional results emphasize the high degree of vulnerability of Crozet marine communities to climate change and biological invasions. The low functional evenness and redundancy of Crozet benthic communities indicate that the functioning of these marine environments is highly vulnerable to diversity loss. These results highlight the need to use taxonomic and functional studies to advise conservation strategies. Future studies on the functioning of sub-Antarctic ecosystems are imperative to support an effective conservation of species and ecosystem functions, and for an enhanced resilience of these isolated territories against the current and future environmental changes.
Data availability statement
The original contributions presented in the study are publicly available. The taxonomical data can be found here: ZooBank with identifier "2BF9D61C-5167-4F2A-B0CD-1A36BB3F7CE7".
Ethics statement
The manuscript presents research on animals that do not require ethical approval for their study.
Author contributions
YL: Conceptualization, Formal analysis, Investigation, Methodology, Writing – original draft. LS: Formal analysis, Data curation, Investigation, Methodology, Writing – original draft. TL: Methodology, Formal analysis, Writing – original draft. AB: Methodology, Formal analysis, Writing – original draft. RD: Investigation, Supervision, Writing – original draft. TS: Funding acquisition, Conceptualization, Investigation, Methodology, Project administration, Resources, Supervision, Validation, Writing – original draft.
Funding
The author(s) declare financial support was received for the research, authorship, and/or publication of this article. This study is a contribution to the project No. 2021-0882 “Nearshore Cable Inspection and Environmental Survey at IMS Hydroacoustic Station HA04 Crozet, France” conducted by the French Southern and Antarctic Lands (TAAF) in response to the CTBTO solicitation and to the French Polar Institute project #1044 Proteker. YL was supported by TAAF-UB convention #2258.
Acknowledgments
We thank the captain and crew of the R/V Marion Dufresne II for assistance in the field, as well as the staff and scuba divers of the French Polar Institute and TAAF: S. Motreuil, C. Marschal, L. Le Gall, M. Guéné, L. Wauters, Y. Sabathé, S. Sémelin, and M.-F. Bernard. Technical assistance in imagery was also provided by D. Champagnac (Biogéosciences, Université de Bourgogne). Authors are particularly grateful to all taxonomists who have contributed to species identification from image data and provided information on functional traits: R. Downey (Porifera), M. Mackenzie (Holothuroidea), C.J. Sands and T. O’Hara (Ophiuroidea), M. Capa, N. Lavesque and S. Hourdez (Polychaeta), M. Tatián (Ascidiacea), C. Moreau (Asteroidea), S. Rosenfeld (Gastropoda), H. Wägele (Nudibranchia), and L. Le Gall (Algae). We also thank M. Zurowietz (Bielefeld University, Germany) for technical support on the BIIGLE 2.0 platform.
Conflict of interest
The authors declare that the research was conducted in the absence of any commercial or financial relationships that could be construed as a potential conflict of interest.
Publisher’s note
All claims expressed in this article are solely those of the authors and do not necessarily represent those of their affiliated organizations, or those of the publisher, the editors and the reviewers. Any product that may be evaluated in this article, or claim that may be made by its manufacturer, is not guaranteed or endorsed by the publisher.
Supplementary material
The Supplementary Material for this article can be found online at: https://www.frontiersin.org/articles/10.3389/fmars.2023.1291038/full#supplementary-material
References
Adami M. L., Gordillo S. (1999). Structure and dynamics of the biota associated with Macrocystis pyrifera (Phaeophyta) from the Beagle Channel, Tierra del Fuego. Sci. Mar. 63, 183–191. doi: 10.3989/scimar.1999.63s1183
Adams T. P., Miller R. G., Aleynik D., Burrows M. T. (2014). Offshore marine renewable energy devices as stepping stones across biogeographical boundaries. J. Appl. Ecol. 51, 330–338. doi: 10.1111/1365-2664.12207
Airoldi L., Turon X., Perkol-Finkel S., Rius M. (2015). Corridors for aliens but not for natives: effects of marine urban sprawl at a regional scale. Divers. Distrib. 21, 755–768. doi: 10.1111/ddi.12301
Almond P. M., Linse K., Dreutter S., Grant S. M., Griffiths H. J., Whittle R. J., et al. (2021). In-situ image analysis of habitat heterogeneity and benthic biodiversity in the Prince Gustav Channel, Eastern Antarctic Peninsula. Front. Mar. Sci. 8. doi: 10.3389/fmars.2021.614496
Améziane N., Eléaume M., Hemery L., Monniot F., Hemery A., Hautecoeur M., et al. (2011). Biodiversity of the benthos off Kerguelen Islands: overview and perspectives. Kerguelen Plateau Mar. Ecosyst. Fish., 157–167. doi: 10.26028/cybium/2011-35SP-016
Amsler C. D., McClintock J. B., Baker B. J. (2014). Chemical mediation of mutualistic interactions between macroalgae and mesograzers structure unique coastal communities along the western Antarctic Peninsula. J. Phycol. 50, 1–10. doi: 10.1111/jpy.12137
Amsler C. D., Rowley R. J., Laur D. R., Quetin L. B., Ross R. M. (1995). Vertical distribution of Antarctic peninsular macroalgae: cover, biomass and species composition. Phycologia 34, 424–430. doi: 10.2216/i0031-8884-34-5-424.1
Andrade C., Ríos C., Gerdes D., Brey T. (2016). Trophic structure of shallow-water benthic communities in the sub-Antarctic Strait of Magellan. Polar Biol. 39, 2281–2297. doi: 10.1007/s00300-016-1895-0
Andrulewicz E., Napierska D., Otremba Z. (2003). The environmental effects of the installation and functioning of the submarine SwePol Link HVDC transmission line: a case study of the Polish Marine Area of the Baltic Sea. J. Sea Res. 49, 337–345. doi: 10.1016/S1385-1101(03)00020-0
Arnaud P. M. (1974). “Contribution à la bionomie marine benthique des régions antarctiques et subantarctiques” in Téthys. 6, 468–653. C. d’Océanographie de M. M. Station marine d’Endoume.
Auger M., Morrow R., Kestenare E., Sallée J. B., Cowley R. (2021). Southern Ocean in-situ temperature trends over 25 years emerge from interannual variability. Nat. Commun. 12, 1–9. doi: 10.1038/s41467-020-20781-1
Barnes D. K. A., Linse K., Waller C., Morely S., Enderlein P., Fraser K. P. P., et al. (2006). Shallow benthic fauna communities of South Georgia Island. Polar Biol. 29, 223–228. doi: 10.1007/s00300-005-0042-0
Beckley L. E., Branch G. M. (1992). A quantitative scuba-diving survey of the sublittoral macrobenthos at subantarctic Marion Island. Polar Biol. 11, 553–563. doi: 10.1007/BF00237948
Berov D., Hiebaum G., Vasilev V., Karamfilov V. (2016). An optimised method for scuba digital photography surveys of infralittoral benthic habitats: a case study from the SW Black Sea Cystoseira-dominated macroalgal communities. Underw. Technol. 34, 11–20. doi: 10.3723/ut.34.011
Botta-Dukát Z. (2005). Rao’s quadratic entropy as a measure of functional diversity based on multiple traits. J. Veg. Sci. 16, 533–540. doi: 10.1111/j.1654-1103.2005.tb02393.x
Boyé A., Thiébaut É., Grall J., Legendre P., Broudin C., Houbin C., et al. (2019). Trait-based approach to monitoring marine benthic data along 500 km of coastline. Divers. Distrib. 25, 1879–1896. doi: 10.1111/ddi.12987
Branch M. L., Arnaud P. M., Cantera J., Gianakouras D. (1991). The benthic Mollusca and Brachiopoda of Marion and Prince Edward Islands: 1) Illustrated keys to the species 2) Records of the 1982-1989 University of Cape Town surveys. South Afr. J. Antarct. Res. 21, 45–64.
Branch G. M., Attwood C. G., Gianakouras D., Branch M. L. (1993). Patterns in the benthic communities on the shelf of the sub-Antarctic Prince Edward Islands. Polar Biol. 13, 23–34. doi: 10.1007/BF00236580
Bravo G., Kaminsky J., Bagur M., Alonso C. P., Rodríguez M., Fraysse C., et al. (2023). Roving diver survey as a rapid and cost-effective methodology to register species richness in sub-Antarctic kelp forests. Diversity 15, 354. doi: 10.3390/d15030354
Bremner J. (2008). Species’ traits and ecological functioning in marine conservation and management. J. Exp. Mar. Bio. Ecol. 366, 37–47. doi: 10.1016/j.jembe.2008.07.007
Campos L. S., Montone R. C., Moura R. B., Yoneshigue-Valentin Y., Kawall H. G., Convey P. (2013). “Anthropogenic impacts on sub-Antarctic and Antarctic islands and the adjacent marine environments,” in Adaptation and evolution in marine environments (Berlin, Heidelberg: Springer), 177–203. doi: 10.1007/978-3-642-27349-0_10
Cárdenas C. A., Montiel A. (2015). The influence of depth and substrate inclination on sessile assemblages in subantarctic rocky reefs (Magellan region). Polar Biol. 38, 1631–1644. doi: 10.1007/s00300-015-1729-5
Cardinale B. J., Duffy J. E., Gonzalez A., Hooper D. U., Perrings C., Venail P., et al. (2012). Biodiversity loss and its impact on humanity. Nature 486, 59–67. doi: 10.1038/nature11148
Chevenet F., Dolédec S., Chessel D. (1994). A fuzzy coding approach for the analysis of long-term ecological data. Freshw. Biol. 31, 295–309. doi: 10.1111/j.1365-2427.1994.tb01742.x
Chown S. L., Rodrigues A. S. L., Gremmen N. J. M., Gaston K. J. (2001). World heritage status and conservation of Southern Ocean Islands. Conserv. Biol. 15, 550–557. doi: 10.1046/j.1523-1739.2001.015003550.x
Clark G. F., Pastorino S., Marzinelli E. M., Turney C. S. M., Fogwill C. J., Johnston E. L. (2019). Nearshore marine communities at three New Zealand sub-Antarctic islands. Polar Biol. 42, 2193–2203. doi: 10.1007/s00300-019-02591-4
Connell S. D., Glasby T. M. (1999). Do urban structures influence local abundance and diversity of subtidal epibiota? A case study from Sydney Harbour, Australia. Mar. Environ. Res. 47, 373–387. doi: 10.1016/S0141-1136(98)00126-3
Cornwell W. K., Schwilk D. W., Ackerly D. D. (2006). A trait-based test for habitat filtering: convex hull volume. Ecology 87, 1465–1471. doi: 10.1890/0012-9658(2006)87[1465:ATTFHF]2.0.CO;2
Crist T. O., Veech J. A., Gering J. C., Summerville K. S. (2003). Partitioning species diversity across landscapes and regions: a hierarchical analysis of α, β, and γ diversity. Am. Nat. 162, 734–743. doi: 10.1086/378901
Degen R., Aune M., Bluhm B. A., Cassidy C., Kędra M., Kraan C., et al. (2018). Trait-based approaches in rapidly changing ecosystems: a roadmap to the future polar oceans. Ecol. Indic. 91, 722–736. doi: 10.1016/j.ecolind.2018.04.050
Delille B., Borges A. V., Delille D. (2009). Influence of giant kelp beds (Macrocystis pyrifera) on diel cycles of pCO2 and DIC in the Sub-Antarctic coastal area. Estuar. Coast. Shelf Sci. 81, 114–122. doi: 10.1016/j.ecss.2008.10.004
Dell R. K. (1964). Antarctic and subantarctic mollusca: amphineurea, scaphopoda and bivalvia. Discovery Rep. 33, 93–250.
Downey R. V., Griffiths H. J., Linse K., Janussen D. (2012). Diversity and distribution patterns in high southern latitude sponges. PloS One 7, e41672. doi: 10.1371/journal.pone.0041672
Dumont C. P., Harris L. G., Gaymer C. F. (2011). Anthropogenic structures as a spatial refuge from predation for the invasive bryozoan Bugula neritina. Mar. Ecol. Prog. Ser. 427, 95–103. doi: 10.3354/meps09040
Dunham A., Pegg J. R., Carolsfeld W., Davies S., Murfitt I., Boutillier J. (2015). Effects of submarine power transmission cables on a glass sponge reef and associated megafaunal community. Mar. Environ. Res. 107, 50–60. doi: 10.1016/j.marenvres.2015.04.003
Ehrenfeld J. G. (2010). Ecosystem consequences of biological invasions. Annu. Rev. Ecol. Evol. Syst. 41, 59–80. doi: 10.1146/annurev-ecolsys-102209-144650
Ellingsen K. E., Hewitt J. E., Thrush S. F. (2007). Rare species, habitat diversity and functional redundancy in marine benthos. J. Sea Res. 58, 291–301. doi: 10.1016/j.seares.2007.10.001
Féral J.-P., Verlaque M., Rosenfeld S., Poulin E., Chenuil A., Saucède T. (2021). The marine vegetation of the Kerguelen Islands: history of scientific campaigns, inventory of the flora and first analysis of its biogeographical affinities. Cryptogam. Algol. 42, 173–216. doi: 10.5252/cryptogamie-algologie2021v42a12
Foveau A., Haquin S., Dauvin J. C. (2017). Using underwater imagery as a complementary tool for benthos sampling in an area with high-energy hydrodynamic conditions. J. Mar. Biol. Oceanogr. 06, 1–7. doi: 10.4172/2324-8661.1000177
Freeman D., Cooper S., Funnell G., Neale D. (2011). Nearshore benthic community structure at the Bounty and Antipodes Islands, Subantarctic New Zealand. Polar Biol. 34, 1485–1499. doi: 10.1007/s00300-011-1006-1
Friedlander A. M., Ballesteros E., Caselle J. E., Hüne M., Adler A. M., Sala E. (2023). Patterns and drivers of benthic macroinvertebrate assemblages in the kelp forests of southern Patagonia. PloS One 18, 1–17. doi: 10.1371/journal.pone.0279200
Gambi M. C., Lorenti M., Russo G. F., Scipione M. B. (1994). Benthic associations of the shallow hard bottoms off Terra Nova Bay, Ross Sea: zonation, biomass and population structure. Antarct. Sci. 6, 449–462. doi: 10.1017/S0954102094000696
Gering J. C., Crist T. O., Veech J. A. (2003). Additive partitioning of species diversity across multiple spatial scales: implications for regional conservation of biodiversity. Conserv. Biol. 17, 488–499. doi: 10.1046/j.1523-1739.2003.01465.x
Gili J. M., Coma R., Orejas C., López-González P. J., Zabala M. (2001). Are Antarctic suspension-feeding communities different from those elsewhere in the world? Polar Biol. 24, 473–485. doi: 10.1007/s003000100257
Glasby T. M., Connell S. D., Holloway M. G., Hewitt C. L. (2007). Nonindigenous biota on artificial structures: could habitat creation facilitate biological invasions? Mar. Biol. 151, 887–895. doi: 10.1007/s00227-006-0552-5
Gon O., Mostert D. (1992). Aspects of the ecology of two notothenoiid fish species in the inshore zone of the sub-Antarctic Marion Island. S. Afr. J. Sci. 22, 59–67.
Graham M., Vásquez J. A., Buschmann A. (2007). Global ecology of the giant kelp Macrocystis: from ecotypes to ecosystems. Oceanogr. Mar. Biol. an Annu. Rev. 45, 39–88.
Gutt J., Arndt J., Kraan C., Dorschel B., Schröder M., Bracher A., et al. (2019). Benthic communities and their drivers: a spatial analysis off the Antarctic Peninsula. Limnol. Oceanogr. 64, 2341–2357. doi: 10.1002/lno.11187
Gutt J., Helsen E., Arntz W., Buschmann A. (1999). Biodiversity and community structure of the mega-epibenthos in the Magellan region (South America). Sci. Mar. 63, 155–170. doi: 10.3989/scimar.1999.63s1155
Gutt J., Schickan T. (1998). Epibiotic relationships in the Antarctic benthos. Antarct. Sci. 10, 398–405. doi: 10.1017/s0954102098000480
Hewitt J. E., Norkko J., Kauppi L., Villnäs A., Norkko A. (2016). Species and functional trait turnover in response to broad-scale change and an invasive species. Ecosphere 7, 1–11. doi: 10.1002/ecs2.1289/full
Hewitt J. E., Thrush S. F., Dayton P. D. (2008). Habitat variation, species diversity and ecological functioning in a marine system. J. Exp. Mar. Bio. Ecol. 366, 116–122. doi: 10.1016/j.jembe.2008.07.016
Hogg O. T., Barnes D. K. A., Griffiths H. J. (2011). Highly diverse, poorly studied and uniquely threatened by climate change: an assessment of marine biodiversity on South Georgia’s continental shelf. PloS One 6, e19795. doi: 10.1371/journal.pone.0019795
Hooper D. U., Chapin F. S. III, Ewel J. J., Hector A., Inchausti P., Lavorel S., et al. (2005). Effects of biodiversity on ecosystem functioning: a consensus of current knowledge. Ecol. Monogr. 75, 3–35. doi: 10.1890/04-0922
Hsieh T. C., Ma K. H., Chao A. (2016). iNEXT: an R package for rarefaction and extrapolation of species diversity (Hill numbers). Methods Ecol. Evol. 7, 1451–1456. doi: 10.1111/2041-210X.12613
Jumars P. A., Dorgan K. M., Lindsay S. M. (2015). Diet of worms emended: an update of polychaete feeding guilds. Ann. Rev. Mar. Sci. 7, 497–520. doi: 10.1146/annurev-marine-010814-020007
Kogan I., Paull C. K., Kuhnz L. A., Burton E. J., Von Thun S., Gary Greene H., et al. (2006). ATOC/Pioneer Seamount cable after 8 years on the seafloor: observations, environmental impact. Cont. Shelf Res. 26, 771–787. doi: 10.1016/j.csr.2006.01.010
Kortsch S., Primicerio R., Fossheim M., Dolgov A. V., Aschan M. (2015). Climate change alters the structure of arctic marine food webs due to poleward shifts of boreal generalists. Proc. R. Soc B Biol. Sci. 282, 20151546. doi: 10.1098/rspb.2015.1546
Laliberté E., Legendre P. (2010). A distance-based framework for measuring functional diversity from multiple traits. Ecology 91, 299–305. doi: 10.1890/08-2244.1
Lamy T., Reed D. C., Rassweiler A., Siegel D. A., Kui L., Bell T. W., et al. (2018). Scale-specific drivers of kelp forest communities. Oecologia 186, 217–233. doi: 10.1007/s00442-017-3994-1
Langenkämper D., Zurowietz M., Schoening T., Nattkemper T. W. (2017). BIIGLE 2.0 - Browsing and annotating large marine image collections. Front. Mar. Sci. 4. doi: 10.3389/fmars.2017.00083
Langhamer O. (2012). Artificial reef effect in relation to offshore renewable energy conversion: state of the art. Sci. World J. 2012, 1–8. doi: 10.1100/2012/386713
Lau S. C. Y., Wilson N. G., Silva C. N. S., Strugnell J. M. (2020). Detecting glacial refugia in the Southern Ocean. Ecography (Cop.). 43, 1639–1656. doi: 10.1111/ecog.04951
Le Bourg B., Saucède T., Charpentier A., Lepoint G., Michel L. N. (2022). What is the menu today in a subantarctic kelp food web from the Kerguelen Islands? Phytodetritus, phytoplankton and phytobenthos; not living kelp. Mar. Biol. 169, 1–16. doi: 10.1007/s00227-022-04105-z
Legendre P., Gallagher E. D. (2001). Ecologically meaningful transformations for ordination of species data. Oecologia 129, 271–280. doi: 10.1007/s004420100716
Levin L. A., Sibuet M., Gooday A. J., Smith C. R., Vanreusel A. (2010). The roles of habitat heterogeneity in generating and maintaining biodiversity on continental margins: an introduction. Mar. Ecol. 31, 1–5. doi: 10.1111/j.1439-0485.2009.00358.x
Lindegren M., Holt B. G., MacKenzie B. R., Rahbek C. (2018). A global mismatch in the protection of multiple marine biodiversity components and ecosystem services. Sci. Rep. 8, 1–8. doi: 10.1038/s41598-018-22419-1
Love M. S., Nishimoto M. M., Clark S., Bull A. S. (2015). Identical response of caged rock crabs (genera Metacarcinus and Cancer) to energized and unenergized undersea power cables in Southern California, USA. Bull. South. Calif. Acad. Sci. 114, 33–41. doi: 10.3160/0038-3872-114.1.33
Love M. S., Nishimoto M. M., Clark S., McCrea M., Bull A. S. (2017). The organisms living around energized submarine power cables, pipe, and natural sea floor in the inshore waters of Southern California. Bull. South. Calif. Acad. Sci. 116, 61–87. doi: 10.3160/soca-116-02-61-87.1
MacArthur R. H., MacArthur J. W. (1961). On bird species diversity. Ecology 42, 594–598. doi: 10.2307/1932254
Macreadie P. I., Fowler A. M., Booth D. J. (2011). Rigs-to-reefs: will the deep sea benefit from artificial habitat? Front. Ecol. Environ. 9, 455–461. doi: 10.1890/100112
Mason N. W., Mouillot D., Lee W. G., Wilson J. B. (2005). Functional richness, functional evenness and functional divergence: the primary components of functional diversity. Oikos 111, 112–118. doi: 10.1111/j.0030-1299.2005.13886.x
McCarthy A. H., Peck L. S., Hughes K. A., Aldridge D. C. (2019). Antarctica: the final frontier for marine biological invasions. Glob. Change Biol. 25, 2221–2241. doi: 10.1111/gcb.14600
McClintock J. B. (1994). Trophic biology of Antarctic shallow-water echinoderms. Mar. Ecol. Prog. Ser. 111, 191–202. doi: 10.3354/meps111191
McClintock J. B., Angus R. A., Ho C., Amsler C. D., Baker B. J. (2008). A laboratory study of behavioral interactions of the Antarctic keystone sea star Odontaster validus with three sympatric predatory sea stars. Mar. Biol. 154, 1077–1084. doi: 10.1007/s00227-008-1001-4
Montoya J. M., Raffaelli D. (2010). Climate change, biotic interactions and ecosystem services. Philos. Trans. R. Soc B Biol. Sci. 365, 2013–2018. doi: 10.1098/rstb.2010.0114
Mouchet M. A., Villéger S., Mason N. W. H., Mouillot D. (2010). Functional diversity measures: an overview of their redundancy and their ability to discriminate community assembly rules. Funct. Ecol. 24, 867–876. doi: 10.1111/j.1365-2435.2010.01695.x
Mouillot D., Bellwood D. R., Baraloto C., Chave J., Galzin R., Harmelin-Vivien M., et al. (2013). Rare species support vulnerable functions in high-diversity ecosystems. PloS Biol. 11, e1001569. doi: 10.1371/journal.pbio.1001569
Mouillot D., Villéger S., Parravicini V., Kulbicki M., Arias-González J. E., Bender M., et al. (2014). Functional over-redundancy and high functional vulnerability in global fish faunas on tropical reefs. Proc. Natl. Acad. Sci. U. S. A. 111, 13757–13762. doi: 10.1073/pnas.1317625111
O’Hara T. D., Thuy B. (2022). Biogeography and taxonomy of Ophiuroidea (Echinodermata) from the Iles Saint-Paul and Amsterdam in the southern Indian Ocean. Zootaxa 5124, 1–49. doi: 10.11646/zootaxa.5124.1.1
Oksanen A. J., Blanchet F. G., Friendly M., Kindt R., Legendre P., McGlinn D., et al. (2020). vegan: community ecology package. R package. Version 2.5-7.
O’Loughlin P. M., Paulay G., Davey N., Michonneau F. (2011). The Antarctic region as a marine biodiversity hotspot for echinoderms: diversity and diversification of sea cucumbers. Deep. Res. Part II Top. Stud. Oceanogr. 58, 264–275. doi: 10.1016/j.dsr2.2010.10.011
Orejas C., Gili J. M., Arntz W. E., Ros J. D., López P. J., Teixidó N., et al. (2000). Benthic suspension feeders, key players in Antarctic marine ecosystems? Contrib. to Sci. 1, 299–311.
Pearse J. S., Lockhart S. J. (2004). Reproduction in cold water: paradigm changes in the 20th century and a role for cidaroid sea urchins. Deep. Res. Part II Top. Stud. Oceanogr. 51, 1533–1549. doi: 10.1016/j.dsr2.2004.06.023
Pearse J. S., McClintock J. B., Bosch I. (1991). Reproduction of Antarctic benthic marine invertebrates: tempos, modes, and timing. Integr. Comp. Biol. 31, 65–80. doi: 10.1093/icb/31.1.65
Puccinelli E., von der Meden C. E. O., McQuaid C. D., Ansorge I. J. (2018). Biological characteristics of the rafting bivalve Gaimardia trapesina in the Southern Ocean. Mar. Biol. 165, 1–17. doi: 10.1007/s00227-018-3430-z
Rabaut M., Guilini K., Van Hoey G., Vincx M., Degraer S. (2007). A bio-engineered soft-bottom environment: the impact of Lanice conchilega on the benthic species-specific densities and community structure. Estuar. Coast. Shelf Sci. 75, 525–536. doi: 10.1016/j.ecss.2007.05.041
Rabaut M., Vincx M., Degraer S. (2009). Do Lanice conchilega (sandmason) aggregations classify as reefs? Quantifying habitat modifying effects. Helgol. Mar. Res. 63, 37–46. doi: 10.1007/s10152-008-0137-4
Ríos C., Arntz W. E., Gerdes D., Mutschke E., Montiel A. (2007). Spatial and temporal variability of the benthic assemblages associated to the holdfasts of the kelp Macrocystis pyrifera in the Straits of Magellan, Chile. Polar Biol. 31, 89–100. doi: 10.1007/s00300-007-0337-4
Robinson B. J. O., Barnes D. K. A., Grange L. J., Morley S. A. (2022). The extremes of disturbance reduce functional redundancy: functional trait assessment of the shallow Antarctic benthos. Front. Mar. Sci. 8. doi: 10.3389/fmars.2021.797112
Rovelli L., Attard K. M., Cárdenas C. A., Glud R. N. (2019). Benthic primary production and respiration of shallow rocky habitats: a case study from South Bay (Doumer Island, Western Antarctic Peninsula). Polar Biol. 42, 1459–1474. doi: 10.1007/s00300-019-02533-0
Saucède T., Guillaumot C., Michel L., Fabri-Ruiz S., Bazin A., Cabessut M., et al. (2017). Modeling species response to climate change in sub-Antarctic islands - Echinoids as a case study for the Kerguelen Plateau. Kerguelen Plateau Mar. Ecosyst. Fish., 95–116. doi: 10.5281/zenodo.3251680
Schmera D., Heino J., Podani J., Erős T., Dolédec S. (2017). Functional diversity: a review of methodology and current knowledge in freshwater macroinvertebrate research. Hydrobiologia 787, 27–44. doi: 10.1007/s10750-016-2974-5
Sherwood J., Chidgey S., Crockett P., Gwyther D., Ho P., Stewart S., et al. (2016). Installation and operational effects of a HVDC submarine cable in a continental shelf setting: Bass Strait, Australia. J. Ocean Eng. Sci. 1, 337–353. doi: 10.1016/j.joes.2016.10.001
Sicinski J., Gillet P. (2002). Biogeographical affinities of polychaetes from Îles Crozet. Antarct. Sci. 14, 353–363. doi: 10.1017/S0954102002000172
Smale D. A., Barnes D. K. A. (2008). Likely responses of the Antarctic benthos to climate-related changes in physical disturbance during the 21st century, based primarily on evidence from the West Antarctic Peninsula region. Ecography (Cop.). 31, 289–305. doi: 10.1111/j.0906-7590.2008.05456.x
Smith V. R. (2002). Climate change in the sub-Antarctic: an illustration from Marion Island. Clim. Change 52, 345–357. doi: 10.1023/A:1013718617277
Solan M., Germano J. D., Rhoads D. C., Smith C., Michaud E., Parry D., et al. (2003). Towards a greater understanding of pattern, scale and process in marine benthic systems: a picture is worth a thousand worms. J. Exp. Mar. Bio. Ecol. 285, 313–338. doi: 10.1016/S0022-0981(02)00535-X
Taormina B., Marzloff M. P., Desroy N., Caisey X., Dugornay O., Metral Thiesse E., et al. (2020). Optimizing image-based protocol to monitor macroepibenthic communities colonizing artificial structures. ICES J. Mar. Sci. 77, 835–845. doi: 10.1093/icesjms/fsz249
Teixidó N., Albajes-Eizagirre A., Bolbo D., Le Hir E., Demestre M., Garrabou J., et al. (2011). Hierarchical segmentation-based software for cover classification analyses of seabed images (Seascape). Mar. Ecol. Prog. Ser. 431, 45–53. doi: 10.3354/meps09127
Teixidó N., Garrabou J., Arntz W. E. (2002). Spatial pattern quantification of Antarctic benthic communities using landscape indices. Mar. Ecol. Prog. Ser. 242, 1–14. doi: 10.3354/meps242001
Teixidó N., Garrabou J., Gutt J., Arntz W. E. (2004). Recovery in Antarctic benthos after iceberg disturbance: trends in benthic composition, abundance and growth forms. Mar. Ecol. Prog. Ser. 278, 1–16. doi: 10.3354/meps278001
Thrush S., Dayton P., Cattaneo-Vietti R., Chiantore M., Cummings V., Andrew N., et al. (2006). Broad-scale factors influencing the biodiversity of coastal benthic communities of the Ross Sea. Deep. Res. Part II Top. Stud. Oceanogr. 53, 959–971. doi: 10.1016/j.dsr2.2006.02.006
Trygonis V., Sini M. (2012). PhotoQuad: a dedicated seabed image processing software, and a comparative error analysis of four photoquadrat methods. J. Exp. Mar. Bio. Ecol. 424–425, 99–108. doi: 10.1016/j.jembe.2012.04.018
Valdivia N., Díaz M. J., Holtheuer J., Garrido I., Huovinen P., Gómez I. (2014). Up, down, and all around: scale-dependent spatial variation in rocky-shore communities of Fildes Peninsula, King George Island, Antarctica. PloS One 9, 1–12. doi: 10.1371/journal.pone.0100714
van Der Linden P., Patrício J., Marchini A., Cid N., Neto J. M., Marques J. C. (2012). A biological trait approach to assess the functional composition of subtidal benthic communities in an estuarine ecosystem. Ecol. Indic. 20, 121–133. doi: 10.1016/j.ecolind.2012.02.004
Van Hoey G., Guilini K., Rabaut M., Vincx M., Degraer S. (2008). Ecological implications of the presence of the tube-building polychaete Lanice conchilega on soft-bottom benthic ecosystems. Mar. Biol. 154, 1009–1019. doi: 10.1007/s00227-008-0992-1
van Rein H., Schoeman D. S., Brown C. J., Quinn R., Breen J. (2011). Development of benthic monitoring methods using photoquadrats and scuba on heterogeneous hard-substrata: a boulder-slope community case study. Aquat. Conserv. Mar. Freshw. Ecosyst. 21, 676–689. doi: 10.1002/aqc.1224
Villéger S., Mason N. W. H., Mouillot D. (2008). New multidimensional functional diversity indices for a multifaceted framework in functional ecology. Ecology 89, 2290–2301. doi: 10.1890/07-1206.1
Villnäs A., Hewitt J., Snickars M., Westerbom M., Norkko A. (2018). Template for using biological trait groupings when exploring large-scale variation in seafloor multifunctionality. Ecol. Appl. 28, 78–94. doi: 10.1002/eap.1630
Violle C., Navas M. L., Vile D., Kazakou E., Fortunel C., Hummel I., et al. (2007). Let the concept of trait be functional! Oikos 116, 882–892. doi: 10.1111/j.0030-1299.2007.15559.x
Westerberg H., Lagenfelt I. (2008). Sub-sea power cables and the migration behaviour of the European eel. Fish. Manage. Ecol. 15, 369–375. doi: 10.1111/j.1365-2400.2008.00630.x
Keywords: Southern Ocean, sub-Antarctic, Crozet, benthos, community assembly, trait-based approach, underwater imagery, submarine cable
Citation: Lelièvre Y, Specq L, Lamy T, Boyé A, Downey RV and Saucède T (2023) Taxonomic and functional diversity of subtidal benthic communities associated with hard substrates at Crozet archipelago (sub-Antarctic, Southern Ocean). Front. Mar. Sci. 10:1291038. doi: 10.3389/fmars.2023.1291038
Received: 08 September 2023; Accepted: 22 November 2023;
Published: 19 December 2023.
Edited by:
Irini Tsikopoulou, Hellenic Center for Marine Research, GreeceReviewed by:
Kathryn Schoenrock, University of Galway, IrelandKrzysztof Pabis, University of Łódź, Poland
Giorgos Chatzigeorgiou, Hellenic Centre for Marine Research (HCMR), Greece
Copyright © 2023 Lelièvre, Specq, Lamy, Boyé, Downey and Saucède. This is an open-access article distributed under the terms of the Creative Commons Attribution License (CC BY). The use, distribution or reproduction in other forums is permitted, provided the original author(s) and the copyright owner(s) are credited and that the original publication in this journal is cited, in accordance with accepted academic practice. No use, distribution or reproduction is permitted which does not comply with these terms.
*Correspondence: Yann Lelièvre, eWFubi5sZWxpZXZyZTEwQGdtYWlsLmNvbQ==
†ORCID: Yann Lelièvre, orcid.org/0000-0003-3508-418X
Léa Specq, orcid.org/0009-0001-2548-2830
Thomas Lamy, orcid.org/0000-0002-7881-0578
Aurélien Boyé, orcid.org/0000-0002-5692-7660
Rachel Downey, orcid.org/0000-0001-9275-8879
Thomas Saucède, orcid.org/0000-0001-6056-4447