- 1Department of Water Resources and Harbor Engineering, College of Civil Engineering, Fuzhou University, Fuzhou, China
- 2Fujian Institute of Geological Survey, Fuzhou, China
- 3State Key Laboratory of Estuarine and Coastal Research, East China Normal University, Shanghai, China
- 4Institute of Estuarine and Coastal Research/State and Local Joint Engineering Laboratory of Estuarine Hydraulic Technology, School of Marine Engineering and Technology, Sun Yat-sen University, Guangzhou, China
Understanding the evolution and driving factors of sedimentation and erosion at the mouths of small and medium-sized mountain streams during various periods is essential for regional spatial utilization, development, and sustainable economic growth. This is particularly important when considering the combined impact of climate change and human activities. This paper presents an analysis of the changes in sedimentation and erosion of mouth isobaths and underwater deltas over different periods using nautical chart data (1950-2019) and analyzes the factors driving changes in sedimentation and erosion during different periods from 1950 to 2020 based on the runoff-sediment discharge of the Minjiang River (MR) and extreme climate factors such as typhoons, especially the driving factors that caused a sudden change in the sedimentation and erosion process between 1998 and 2005. The results indicate that runoff-sediment characteristics are crucial in driving sedimentation and erosion changes. In the past 70 years, the underwater delta of the MRE has mainly experienced four stages: deposition (1950-1992) -erosion (1993-1998) -deposition (1998-2011) -erosion (2011-2019). Taking the impoundment operation of the Shuikou Reservoir in 1993 as the node, the sediment load of the Minjiang River into the sea began to decrease sharply, and then the estuary quickly showed a state of erosion. The change of sediment flux into the sea is the main driving factor for the evolution of erosion and deposition in the Minjiang River Estuary (MRE). The critical value of the erosion-deposition transition is about 570.3 × 104 t/yr. After the estuary entered a state of erosion in 1993-1998, significant siltation suddenly occurred in 1998-2005. The reason for the siltation in this period may be related to the frequent transit typhoons and flood events in 1998-2005. Therefore, the change of river sediment supply into the sea is the main driving factor controlling the erosion and deposition evolution of the Minjiang River estuary on a long time scale of more than 10 years, which reflects the influence of large-scale human activities on the river and estuary area in the past half century. Extreme climate events are the incentive to adjust the estuary landform in the short term. Extreme climate events will not fundamentally change the process of estuarine geomorphological evolution under the background of sediment supply reduction.
Highlights
1. Taking the impoundment operation of Shuikou Reservoir in 1993 as the node the long-term siltation state of the subaqueous delta in the Minjiang Estuary changed to erosion the critical value of the transition is about 570.3 × 104 t/yr.
2. The change of sediment load into the sea is the main driving factor for the adjustment of estuarine geomorphic evolution in the past 70 years.
3. The sudden significant siltation in 1998-2005 may be caused by frequent typhoons and frequent floods in the Minjiang Estuary during this period.
1 Introduction
The estuary is a sensitive zone where land and sea intersect, serving as both the destination for river material and the starting point for ocean matter. It is also a core area where the population gathers, the economy thrives, and navigation and harbor projects are concentrated. In recent decades, under the influence of global warming, sea level rise, and strong human activities in the basin-estuary system, the characteristics of riverine sediment discharge into the sea have undergone significant changes, resulting in geomorphic responses such as underwater delta erosion and shoreline retreat, as well as problems such as saline intrusion, sewage backflow, and ecological degradation (Milliman and Meade, 1983; Nienhuis et al., 2020; Syvitski et al., 2022). Clarifying the response characteristics and driving mechanisms of estuarine sedimentary geomorphology is not only of great significance for a profound understanding of the characteristics of land-sea interactions and changes in estuarine ecological environment but also provides a useful reference for ensuring navigation safety and implementing important projects such as coastal protection (Xue, 2023).
In the past decades, numerous studies have recognized the significant impact of human activities, particularly dam construction, on reducing sediment supply in rivers (Wu et al., 2016; Li, 2017; Maloney et al., 2018; Guo et al., 2021). This has resulted in a global trend of many deltaic estuaries shifting from an accretionary to a erosional regime. The construction of large reservoirs in the Mississippi River (Maloney et al., 2018), Nile (Stanley, 1996), Mekong (Anthony et al., 2015), and Yangtze Rivers (Li et al., 2017; Guo et al., 2021) has led to a significant decrease in sediment discharge, resulting significant erosion of estuarine subaqueous deltas. However, the response of the estuarine geomorphology of the Yangtze River to sediment reduction has a time lag effect of about 30 years (Zhu et al., 2019; Luan et al., 2021). Due to the influence of water conservancy projects in Yellow River basin, the runoff and sediment into the sea have decreased sharply, and the trend of estuarine delta deposition has been significantly slowed down (Wang et al., 2016; Ji et al., 2022). Although there is a consensus globally that reduced sediment supply to river deltas increases the risk of coastal erosion, due to the complexity of geomorphic evolution mechanisms, there is still a large difference in understanding the factors that drive the response process of different estuarine sedimentary geomorphologies.
The estuarine system acts as a filter to transport material from the river to the ocean, which can effectively regulate the sediment load (Dürr et al., 2011). When sediment is transported by rivers into estuaries, sedimentation and sorting often occur due to the widening of the cross section and the change of velocity (FitzGerald et al., 2015). Meanwhile, the strong riverbed shear stress caused by the combination of wave-induced seabed agitation, nearshore current caused by wave and wind, and tidal currents leads to a resuspension-redeposition process, and this process is repeated (Hayes, 1975; Wright and Nittrouer, 1995; FitzGerald et al., 2015). In particular, intense waves generated by passing typhoons often cause drastic changes in the bed surface (Zhang, 2006). For example, in 2011, the Susquehanna River discharged 6.7 million tons of sediment into a bay under the influence of Tropical Storm Lee. Most of the sediment is deposited within the bay within 20 days. Long-term simulations of the storm showed that due to asymmetric tidal resuspension during the receding flood, as well as estuary circulation and tidal advection, most of the sediment brought by the flood was redistributed and accumulated in the region with the highest turbidity while the rest was transported and deposited in the central part of the bay (Cheng et al., 2013). The adjustment process of river deltaic landform evolution occurs under the coupling effect of multiple factors. Studies such as construction of the Three Gorges Dam (Luan, 2017) and artificial diversion of the Yellow River (Wang et al., 2022) caused estuary erosion indicate that high-intensity human disturbance (such as cascade hydropower development) in the basin leads to a reduction in sediment supply to the sea, gradually becoming the main driving factor for the widespread development of estuarine deltas into erosion. However, Wang et al. (2020) found that about 0.9-4.7×108 m3 of sediment carried by typhoon into the estuary made up for the erosion of the estuary to a certain extent. Rodriguez et al. (2020) found that in the North American region, although many rivers have interrupted the transport pathway of sediment due to dam construction, sediment deposition in coastal sedimentary centers has significantly increased. This may be attributed to the additional sediment supplied by human-induced landscape changes. Key scientific issues such as the impact of reservoir sediment trapping (Nittrouer and Viparelli, 2014), the contribution of climate factors (such as floods, storm surges, etc.) (Darby et al., 2016; Mei et al., 2018), and future trend changes (Boudet et al., 2017; Li, 2020) still exist for intense discussion. The complex dynamic mechanism of estuarine geomorphology restricts us to systematically understand the evolution of estuarine geomorphology and reasonably predict the future.
In recent years, more and more research has focused on small and medium-sized rivers (especially mountain streams) (Gao et al., 2009; Chen et al., 2016; Liu et al., 2016; Yang and Yin, 2018; Tang et al., 2021). This is not only because their material flux to the coast may exceed that of the world’s major river systems (Milliman et al., 2008; Hilton et al., 2011), but also because the spatial scale of mountain streams is smaller, and their runoff and sediment flux to the sea are significantly influenced by extreme events. Mountain streams have a sedimentary system characterized by “instantaneous large flux-extreme event influence-rapid material transformation,” and they are extremely sensitive to climate change and human activities (Bi et al., 2015; Yang et al., 2016; Yang and Yin, 2018). In a global context, the reduction of sediment discharges into the sea due to the construction of reservoirs and dams in river basins has become an inevitable trend (Vörösmarty et al., 2003; Syvitski et al., 2009). Compared with the estuaries of major rivers, changes in runoff and sediment dynamics caused by human activities or climate factors can often be quickly manifested in the geomorphic changes of small and medium-sized mountain stream estuaries, which makes it easier to capture the geomorphic response processes and changes in their driving factors. In the context of continuous erosion caused by the decrease in sediment discharges into the sea, the construction of embankments and related engineering projects in the Qiantang River Estuary, has enhanced flood advantage and increased sediment transport towards the land. As a result, the sediment deposition rate in the estuary has increased (Xie et al., 2017). Analysis of sediment cores from the intertidal zone of the Yalu River Estuary reveals that the estuarine sedimentary environment is significantly affected by the decrease of sediment from the basin (Wang et al., 2011; Shi et al., 2017). In addition, large-scale reclamation in the estuarine region of the Yalu River has significantly reduced tidal inundation, leading to severe siltation in the western channel (Wang et al., 2011). While the phenomenon of delta erosion due to the reduction of sediment discharges into the sea is widespread globally, the factors driving the morphological evolution of different river deltas differ significantly across different regional conditions and timescales.
The MR, as a typical small and medium-sized mountainous river in Southeast China, has a relatively small basin and sedimentary system (Li et al., 2008; Chen et al., 2010; Wang et al., 2020; Wang et al., 2022). It exhibits rapid responses to various factors in terms of underwater delta evolution (Gao et al., 2012; Lu et al., 2023). Previous researchers found that in the first half of the 20th century, the MRE showed strong sediment accretion. From the late 1980s to the end of the 20th century, the erosion became dominant (Xu et al., 2009; Chen et al., 2010; Lai et al., 2023). Wang et al. (2020) revealed that human activities such as deforestation caused an increase in the sedimentation rate at the estuary from 1963 to 1986, followed by a rapid decrease in sedimentation rate caused by the construction of the reservoirs in the upstream. Lu et al. (2023) found that the response of the MRE to the decrease in sediment flux shifted from deposition to erosion within a time scale of less than 10 years. The rapid response of the MRE landform evolution to driving factors is helpful for the rapid and clear identification of changes in driving factors at different time scales, especially against the backdrop of continuous reduction in sediment input to the sea and increasing human activities and the combined effects of extreme climate events. The identification of the driving factors behind the increased sedimentation rate in the estuary during 1998-2005 is particularly significant. The study of the driving factors in the MRE enriches the case studies of sedimentary evolution in small and medium-sized river estuaries worldwide and helps to expand our understanding of the evolution of deltaic estuaries under the coupled effects of human activities and extreme climate factors. Based on the relationship between the sediment flux into the sea and the amount of erosion and deposition in the estuary, this paper discusses the significant variation stages and their driving factors in the process of erosion and deposition evolution, and reveals the coupling effect of human activities and climate change on the geomorphic evolution of the MRE.
2 Study area
The MR (Figure 1B) originated from the eastern foot of Wuyi Mountain and the southern foot of Xianxialing at the junction of Fujian Province and Jiangxi Province. It is controlled by the fault structure to form a typical lattice water system. It generally runs northwest-southeast across the central and northern parts of Fujian Province and flows into the Taiwan Strait through Fuzhou. The total length is 2,872 km, the total length of the mainstream is nearly 577 km, and the basin area is 60,992 km2, accounting for half of the total area of Fujian Province (Wang et al., 2022). Among them, the upper reaches of Nanping are upstream, the middle reaches from Nanping to Anrenxikou are middle reaches, and the lower reaches below Anrenxikou are downstream. The basin is located in the subtropical marine monsoon climate zone. The annual precipitation is between 1400 and 2400 mm, and the precipitation gradually decreases from the upstream to the downstream, from the northwest mountain area to the southeast coast. The MR Basin is rich in water and less in sediment, and the runoff is large. The average annual runoff is 605.5 × 108 m3. The suspended sediment concentration of the river is small, and the average annual sediment transport is only 750 × 104 t. Since the construction of the Shuikou Dam (Construction began in 1987, the first unit was put into operation in 1993, and all units were completed and put into operation in 1996), the sediment transport has decreased significantly, and the average annual sediment transport since 1993 is only 248 × 104 t (Lai et al., 2023).
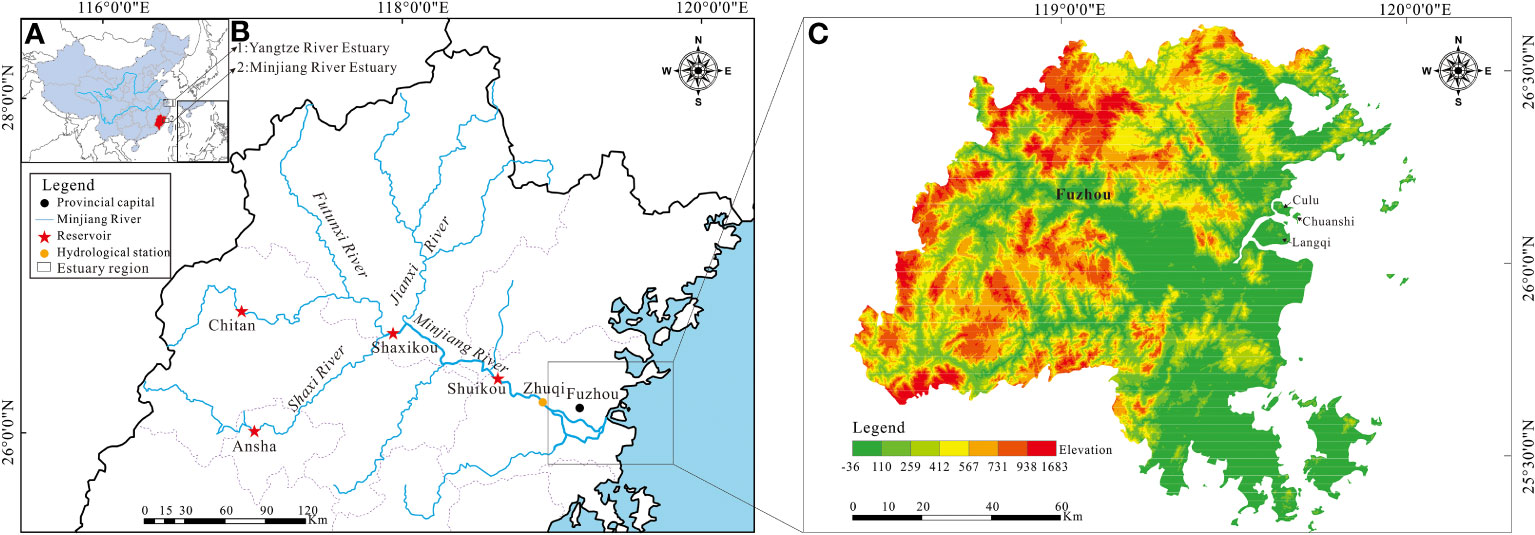
Figure 1 Location of the Minjiang River and the Minjiang River Estuary. (A) Location of Fujian Province; (B) Water system distribution map of Minjiang River Basin; (C) The surface elevation of Fuzhou and the location map of Minjiang River Estuary.
The MRE (Figure 1C) is a strong tidal river estuary with multiple channels. The maximum observed tidal range is 7.04 m, with an average tidal range of 4.46 m (Meihua station), making it the main driving force shaping the estuary. The flood tide has a certain role in the re-transportation and re-deposition of sediments. The tidal wave is a standing wave and the tide belongs to a regular semidiurnal tide. The tidal flow in the estuary is generally a reciprocating flow that is consistent with the river channel. The offshore tidal flow of the estuary has certain characteristics of rotational flow (Wang et al., 2020). The MRE has numerous branches and a complex river network. Before entering the sea, it is blocked by Langqi Island and is divided into the Meihua waterway in the south and the Changmen waterway in the north. After the northern branch leaves the Changmen waterway, it is blocked by Cuolu Island, and Chuanshi Island, and is divided into four waterways such as Wuzhu and Chuanshi. The offshore area faces the sea, and the wave action is significant, with both prevailing and strong wave directions being northeastward. Exogenous sediments play an important role during typhoons and winter gales. (Chen et al., 1998). In addition, the sea area at the mouth of the MR is one of the regions in southeastern coastal China that is most severely affected by typhoons, with an average of 5.7 typhoons each year, and a maximum storm surge of 2.52 m (Chen et al., 2015).
3 Materials and methods
3.1 Data sources
The MR’s annual discharge and sediment load into the sea were measured at the Zhuqi hydrological station (46 km from the river mouth), which covers over 90% of the river basin area (Figure 1B). The annual runoff (1950-2019) and sediment discharge (1950-2019) were obtained from the Zhuqi Station are sourced from the “China Sediment Bulletin” (2002-2019) published by the Ministry of Water Resources of the People’s Republic of China, and the data provided by the Hydrological and Water Resources Survey Bureau of Fujian Province (1950-2001). The sand excavation data from 1993-1996, 1998-2006, 2007-2008, and 2009-2019 were sourced from Hu et al.’s (2015) “River Evolution under the Joint Action of Reservoirs and River Sand Mining”, Lin Lin (2009) “Research and Exploration of Sand Mining in the Lower Reaches of the Minjiang River”, and the “Annual River Sand Mining Implementation Plan for the Fuzhou section of the lower reaches of the Minjiang River” published by the Fuzhou Water Resources Bureau. Underwater elevation data of the MRE were obtained from the “Minjiang Estuary Nautical Charts” published by the Navigation Assurance Department of the People’s Liberation Army Navy (PLA) in 1950, 1955, 1975, 1986, 1989, 1992, 1998, 2005, 2011, and 2019 (a total of 10 charts) (Table 1). Typhoon data was obtained from the Zhejiang Provincial Department of Water Resource’s website on typhoon path (https://typhoon.slt.zj.gov.cn/slt.htm), with the number of typhoons passing through or landing in Fujian province each year counted. Typhoons that pass through the study area were considered as extreme events that significantly affect the MRE.
3.2 Methods
Spatial analysis was performed using ArcGIS 10.8 to investigate the interannual variations and changes in siltation and erosion during different periods of the isobaths (2 m, 5 m, 10 m) of the MRE, to analyze the siltation and erosion changes over the past 70 years. The paper maps were scanned and the coordinate system and georeferencing were defined using ArcGIS software, and water depth points were digitized. The Kriging interpolation method was used to interpolate the discrete data into a regular grid of 30 m ×30 m, forming a digital elevation model (DEM) of the MRE and extracting the isobaths (2 m, 5 m, 10 m). The DEM data from adjacent years were overlaid and analyzed, and the region analysis was used to calculate the siltation and erosion. To facilitate comparisons, this paper converted the coordinate and depth datum (Wang and Wang, 1995), unifying the coordinate system (WGS84) and the depth datum (the theoretical Lowest Astronomical Tide) of all of the nautical charts.
Runoff mainly comes from rainfall, while sediment is transported through runoff. The relationship between runoff and sediment in the watershed directly affects the siltation and erosion of the estuary. Generally, as the runoff increases, the concentration of suspended sediment also increases, but the rate of increase varies greatly depending on time and other factors.
The sediment-discharge relationship curve refers to the power-law function relationship curve that describes the relationship between flow rate (Q) and suspended sediment concentration (SSC). It not only represents the soil erosion intensity and sediment transport capacity of the watershed but also reflects the changes in the sediment-carrying capacity of the water flow (Asselman, 2000; Syvitski, 2000). It has been applied in the study of the relationship between discharge and sediment concentration in many rivers such as the Yangtze and Yellow Rivers (Xie et al., 2021; Zhang et al., 2021). Its expression can be represented as:
Taking the logarithm of the equation simultaneously yields another linear expression:
In the equation, SSC refers to sediment concentration (kg/m3), Q refers to flow rate (m3/s), and a and b are parameters obtained from fitting the runoff-sediment relationship curve. The coefficient a represents the sediment yield characteristics of the watershed, reflecting the variations in soil erosion intensity and sediment transport under human intervention, and can reflect the amount of sediment supply (Asselman, 2000; Syvitski et al., 2000; Xia et al., 2021). Coefficient b represents the erosional dynamics, indicating the sediment-carrying capacity of the river, i.e., the magnitude of the river’s erosive power, reflecting the correlation between the river’s erosional capacity and climate patterns, channel morphology, and Watershed erosion degree (Syvitski et al., 2000; Yang et al., 2007; Ganju et al., 2008; Xia et al., 2021). A larger b value means that as the water volume increases, the erosional capacity also increases.
4 Results and discussion
4.1 Analysis of the variation of isobaths and the sedimentation and erosion of MRE during different periods in the past 70 years
The distribution of isobaths can serve as an important indication of the topographical features of an estuary. The changes in isobaths during different periods can reveal the sedimentation and erosion characteristics of the underwater terrain. This paper extracted the 2 m, 5 m, and 10m isobaths from a total of 7 nautical charts from 1975, 1986, 1992, 1998, 2005, 2011, and 2019 to explore the migration characteristics of isobaths in the MRE.
The 2 m isobath showed a clear seaward progradation trend from 1975 to 1992 (Figure 2A), with the most significant deposition occurring on the side of Chuanshi Island in the Chuanshi Channel, where the deposition on the side of Langqi Island was also evident. At the outlet of Meihua Channel, the 2 m isobath moved southward and became narrower. However, from 1998 to 2019, the 2 m isobath mainly showed erosion and retreat, with a significant turning point in 1998. The 2 m isobath in the Chuanshi Channel significantly retreated towards the channel, and the eastern 2 m isobath of Chuanshi Island also showed obvious retreat and became more complex. The isobaths near Langqi Island on the Chuanshi Channel side were significantly eroded, and were closer to Langqi Island than in 1975, with an increased distance between the isobaths. However, in 2005, there was significant seaward progradation in some areas of the 2 m isobath, mainly appearing on the eastern side of Langqi Island, the Langqi Island side of Chuanshi Channel, and the outlet of Meihua Channel, while the changes in other years were not significant.
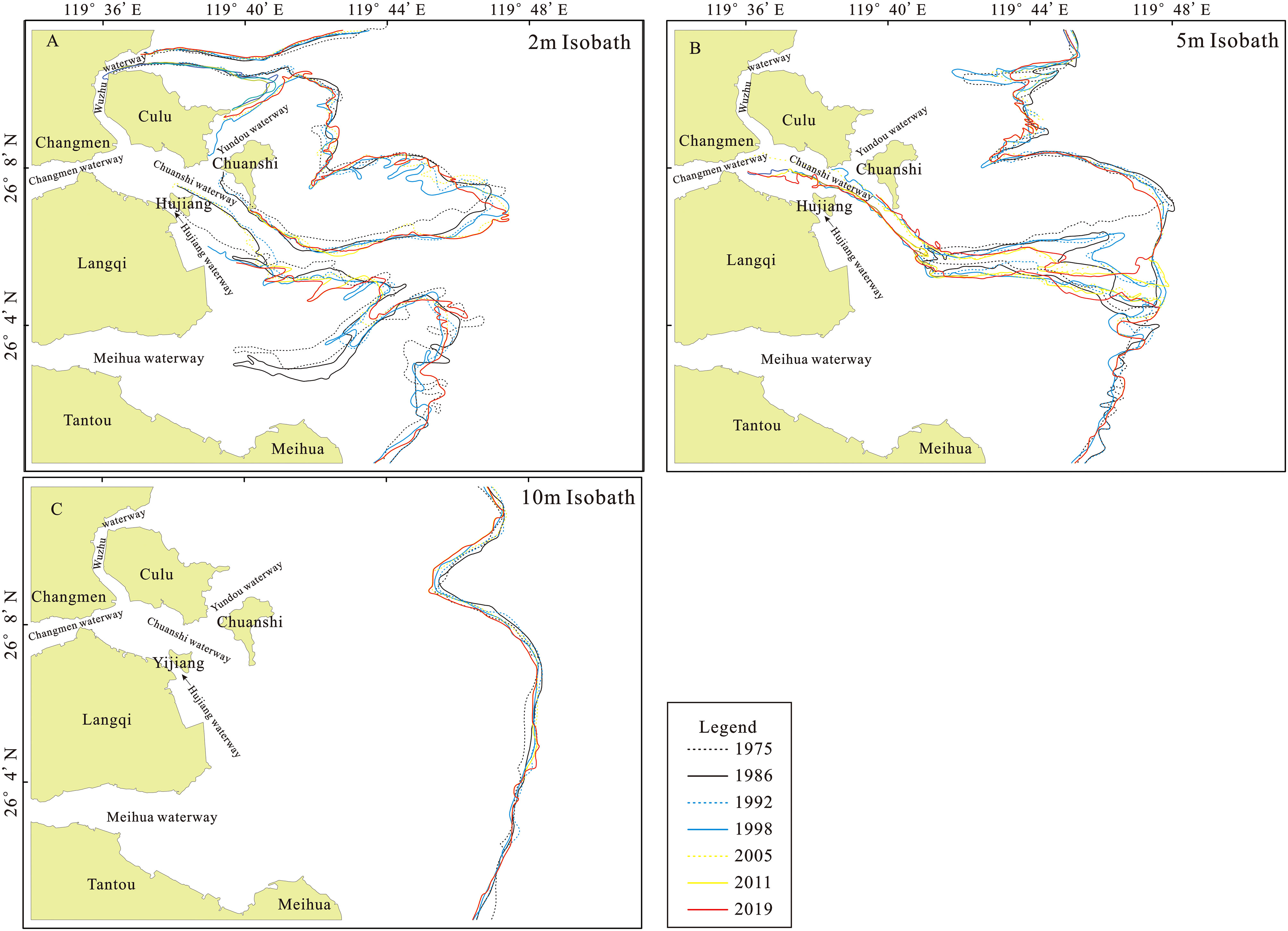
Figure 2 Isobath Distribution Map of the Minjiang River Estuary (1975-2019). (A) 2 m Isobath; (B) 5 m Isobath; (C) 10 m Isobath.
The changes in the 5 m isobath mainly occurred in the area near the outlet of the Chuanshi Channel, particularly on the side of Chuanshi Island, while the changes in other areas were less significant (Figure 2B). The interannual changes of the 5 m isobath were generally consistent with those of the 2 m isobath. From 1975 to 1986, the 5 m isobath of Chuanshi Island on the Chuanshi Channel significantly prograded seaward to as much as 4000 m. In 1992, there was significant erosion on the Langqi Island side of the Chuanshi Channel, and deposition on the Chuanshi Island side, while no significant changes occurred in other areas. In 1998, the 5 m isobath suffered significant erosion and retreat, with the 5 m isobath in the Chuanshi Channel significantly broken and extending towards the channel. The 5 m isobath in the Wuzhu Channel also retreated significantly, and the isobath at the outlet of the Meihua Channel became more complex. However, in 2005, the 5 m isobath reverted to progradation, with the most significant progradation occurring on the Chuanshi Island side of the Chuanshi Channel, while the changes in other years were not significant.
The changes in the 10m isobath mainly occurred north of the outlet of the Meihua Channel (Figure 2C). From 1976 to 1985, the 10m isobath significantly prograded, while from 1985 to 1992, the 10 m isobath did not show significant changes, with coexisting local erosion and deposition. In 1998, the 10 m isobath retreated over a long distance due to erosion. In 2005, except for the outlet of the Wuzhu Channel, the isobaths in other areas showed progradation, while from 2005 to 2019, they retreated again due to erosion. In 2019, compared with 1975, the 10m isobath retreated due to erosion between the Wuzhu Channel and the Chuanshi Channel, while significant progradation occurred east of Langqi Island.
The changes in different isobaths occurred in different locations. Overall, the changes in the 2 m isobath mainly occurred on the east side of Chuanshi Island and both sides of the Meihua Channel. The changes in the 5 m isobath mainly occurred at the outlet of the Chuanshi Channel, while the changes in the 10m isobath mainly occurred north of the Meihua Channel. The interannual changes of the isobaths showed similar trends and displayed significant periodic characteristics. That is, before the construction of the dam at the estuary, the dominant trend was seaward deposition, while after the construction of the dam, the dominant trend was erosion and retreat, with a conspicuous isobath retreat occurring from 1992 to 1998.
The interannual variation of the isobaths qualitatively characterizes the underwater topographical changes in the MRE. According to the nautical chart data from 1950 to 2019, the evolution of the deltaic sedimentation in the estuary was divided into seven intervals to quantitatively analyze the variation of sedimentation during different periods. As shown in Table 2, the first interval from 1950 to 1975 was dominated by sedimentation, with a net sedimentation volume of 3.10×108 m3. The average annual net sedimentation volume was 1.24×107 m3/yr, and the average sedimentation depth was 0.539 m, with an average annual sedimentation rate of 0.022 m/yr. The second interval from 1975 to 1986 had a net sedimentation volume of 0.86×108 m3, with an average annual volume of 0.78×107 m3/yr, an average sedimentation depth of 0.150 m, and an average annual sedimentation rate of 0.014 m/yr, which was significantly lower than the previous interval. The third interval from 1986 to 1992 exhibited a slight sedimentation trend, with a net sedimentation volume of 0.16×108 m3, an average annual sedimentation volume of 0.27×107 m3/yr, an average sedimentation depth of 0.028 m, and an average annual sedimentation rate of 0.005 m/yr, which was significantly lower than the previous two intervals. The fourth interval from 1992 to 1998 showed severe erosion, with a net erosion volume of -1.16×108 m3, an average annual erosion volume of -1.94×107 m3/yr, an average erosion depth of -0.203 m, and an average annual erosion rate of -0.034 m/yr. The fifth interval from 1998 to 2005 experienced a short period of widespread sedimentation, with a net sedimentation volume of 1.05×108 m3, an average annual sedimentation volume of 1.50×107 m3/yr, an average sedimentation depth of 0.183 m, and an average annual sedimentation rate of 0.026 m/yr, which was significantly higher than the previous few sedimentation periods. After a moderate sedimentation period from 2005 to 2011, the estuary of MR exhibited erosion again in the seventh interval from 2011 to 2019, with a net erosion volume of -0.53×108 m3, an average annual erosion depth of -0.012 m/yr, although this interval showed a slightly larger sedimentary area compared to the eroded area.
In the past 70 years, the MRE delta has changed sedimentation (1950-1992), erosion (1992-1998), sedimentation (1998-2011), and erosion (2011-2019). Overall, before the construction of the Shuikou dam in 1993, sedimentation occurred at a rate of 0.017 m/yr, with a net sedimentation volume of 4.08 × 108 m3. After the dam was built (1993-2019), there was a slight overall trend of erosion, with a net erosion volume of -0.36 × 108 m3 and an annual erosion depth of 0.002 m. However, the significant erosion from 1992-1998 suddenly shifted to significant sedimentation from 1998-2005, the cause of which still requires further analysis.
4.2 Changes of runoff-sediment relationship in MR
The characteristics of runoff and sediment are the most fundamental driving factors affecting the evolution of erosion and deposition. Since the 1960s, there has been a significant decrease in the sediment concentration of water flow. Concerning the main stream of the MR, the data we have collected and previous publications have shown that the annual discharge of the river has remained relatively stable since the 1960s. However, there has been a significant decreasing trend in annual sediment yield (Wang et al., 2022).
From the perspective of runoff and sediment matching, as shown in Figure 3B, the R2 value was around 0.5-0.8 during the 1960s-1980s. However, in the 1990s, the water and sediment relationship became completely chaotic, with an R2 value of only 0.15. This fully reflects the changes in sediment discharge into the sea over the past 70 years. Although the sediment yield was continuously decreasing during the 1960s-1980s, the decrease was relatively small compared to the 1990s. The construction of Shuikou Reservoir in 1993 intercepted a large amount of sediment, resulting in a sudden decrease in sediment yield during the 1990s to only 57.5% of that in the 1980s, while the runoff volume remained almost unchanged, leading to a complete disruption of the water and sediment matching. In the 2010s, the water and sediment relationship basically returned to normal, with an R2 value of 0.83.
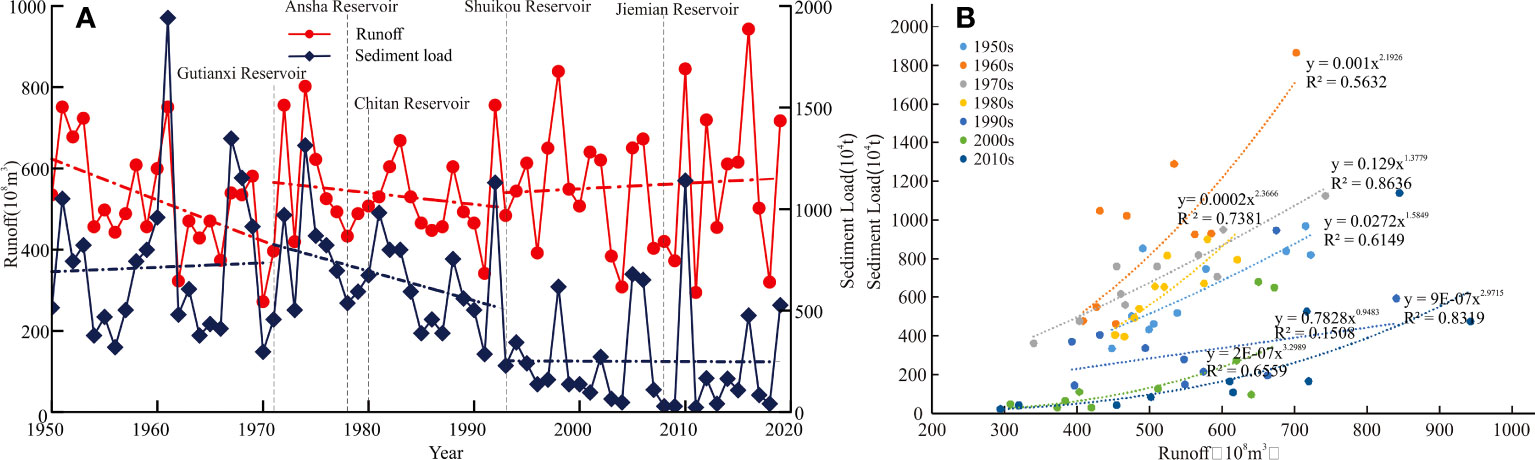
Figure 3 (A) The runoff and sediment load of Minjiang River (Zhuqi station) from 1950 to 2019. (B) The rating curve of Zhuqi station in the Minjiang River (1950-2019).
Generally speaking, the specific parameters of the runoff and sediment relationship curve can reflect changes in erosion and deposition potential, among which parameter a reflects the amount of sediment supply and parameter b indicates erosion potential (Müller and Förstner, 1968; Syvitski et al., 2000; Ganju et al., 2008). Parameter a increased compared to before in the 1970s and increased sharply to 0.7828 in the 1990s, while it showed an overall decreasing trend in other decades. An increase in coefficient a indicates that the influence of external factors, such as human disturbances, on the sediment yield characteristics of the Minjiang River Basin has intensified. Chen (2023), in their attribution analysis of runoff-sediment changes in the Minjiang River Basin, found that human activities primarily controlled sediment transport during the period from 1970 to 2020. Furthermore, human activities during this period led to a decrease in sediment transport. In the 1970s, the extensive construction of water conservancy projects and drastic changes in land use types in the Minjiang River Basin resulted in a reduction in sediment transport. In the 1990s, the main factor was the water storage and operation of the Shuikou Reservoir, which intercepted a large amount of sediment. Parameter b significantly decreased in the 1990s and reached its highest value of 3.2989 in the 2000s. After the construction of the Shuikou Dam, sediment accumulation occurred upstream of the dam, and a large amount of clear water was discharged downstream, increasing the possibility of erosion in the downstream river channel. Although the sharp decrease in sediment supply was not immediately reflected in the riverbed section, the MRE delta is sensitive to the amount of sediment supplied from upstream. The construction of the Shuikou Dam in 1993 intercepted a large amount of sediment, causing the delta to immediately shift from “sedimentation” to “erosion” (Table 2).
4.3 Response of subaqueous delta in MRE to incoming sediment
The sediment supplied from the watershed is the main material source for the development of the subaqueous delta in the MRE. Over the past 70 years, the subaqueous delta has undergone seven stages of sedimentary evolution, namely “significant accumulation (1950-1975) - accumulation (1975-1986) - slight erosion and deposition (1986-1992)- significant scour (1992-1998) - significant accumulation (1998-2005) - slight erosion and deposition (2005-2011) - significant scour (2011-2019)” (Table 2). The first four stages of rapid geomorphic evolution in the MRE, namely “significant accumulation, accumulation, slight erosion and deposition, and significant scour,” match the changes in sediment discharge into the sea from the MR during this period (Figure 4A). From 1950 to 1975, the sediment yield of the MR was 3.68×108 m3, with a net erosion and deposition rate of the subaqueous delta of 3.10×108 m3/a; from 1975 to 1986, sediment yield was 1.39×108 m3, with a net erosion and deposition rate of 0.86×108 m3; from 1986 to 1992, sediment yield was 0.69×108 m3, with a net sediment accumulation rate of 0.16×108 m3; and from 1992 to 1998, the sediment yield decreased to 0.33×108 m3. During this period, there was a transition from sediment accumulation to scouring, with a huge net sediment accumulation rate of -1.16×108 m3. However, with the continued decrease of sediment discharge into the sea, there was a transition from significant scouring to significant accumulation during 1998-2005, with a huge net sediment accumulation rate of 1.05×108 m3, which far exceeded the amount of sediment discharged into the sea. From 2005 to 2011, the sediment discharge into the sea from the MR slightly increased to 0.38×108 m3, and the net erosion and deposition rate during this period was 0.17×108 m3; from 2011 to 2019, the sediment discharge into the sea from the MR decreased to 1.63×107 m3, and the net erosion and deposition rate during this period was -0.36×108 m3.
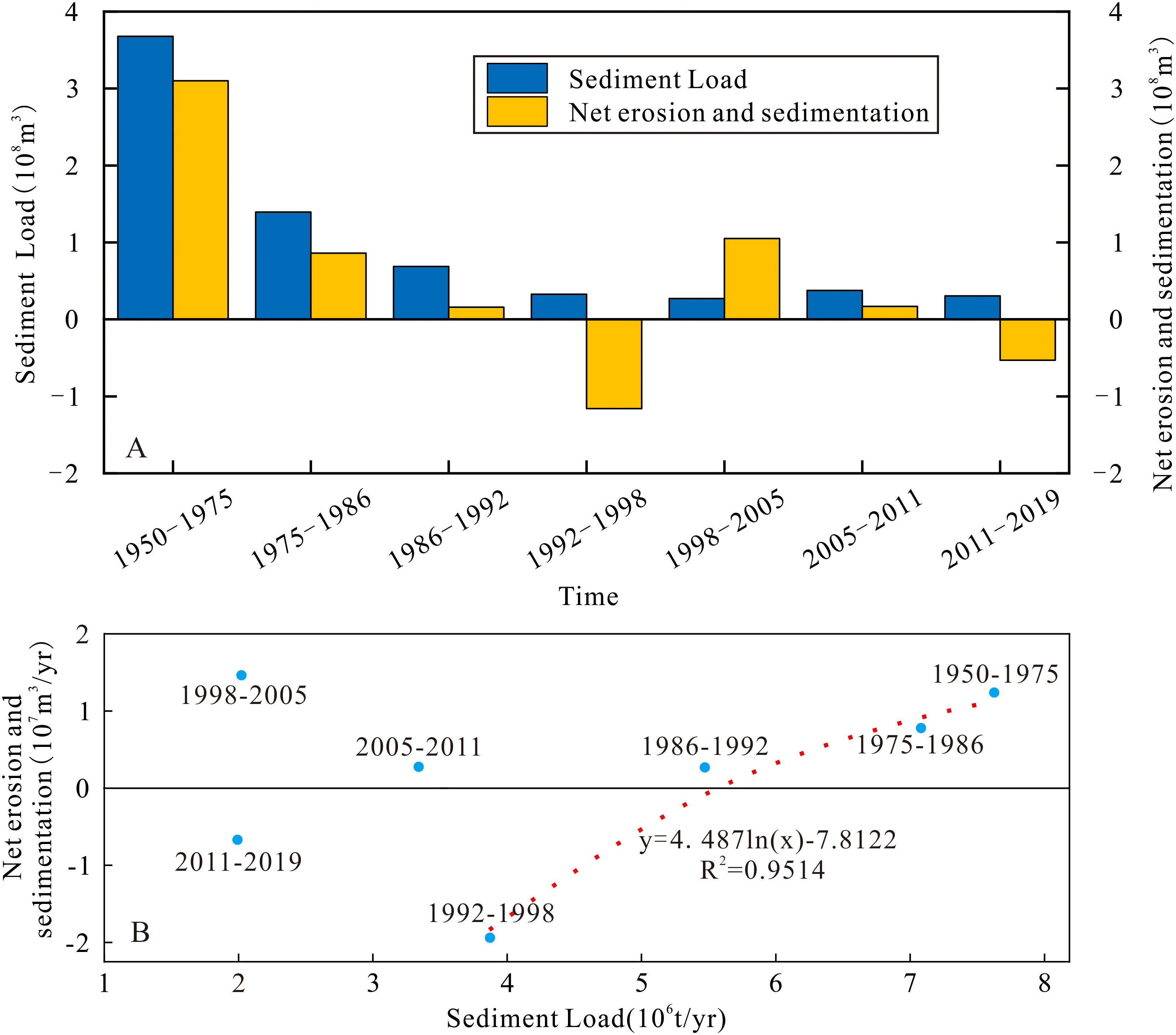
Figure 4 (A) Comparison between net sedimentation and sediment discharge in the Minjiang River Estuary’s underwater delta; (B) Logarithmic relationship between annual average sediment transport and annual net sedimentation in the Minjiang River Estuary.
Overall, except for the period of 1998-2005 where a significant variation occurred, the evolution of sedimentation and erosion in the MRE underwater delta was largely consistent with the variations in sediment discharge into the sea from the MR. This is especially true for the first four stages. Through linear fitting of the sediment discharge and net sediment transport data from the first four stages (1950-1998) (Figure 4B), a relationship equation was obtained:
From this equation, the critical value for the transition of sedimentation and erosion in the underwater delta was calculated to be 5.703×106 t/yr. When the sediment discharge into the MRE remains consistently below this value, the estuarine subaqueous delta will be characterized by erosion as the main feature. The sediment discharge in the 1980s was 6.32×106 t/yr, while in the 1990s it was 3.64×106 t/yr, indicating that erosion mainly occurred after the impoundment of the Shuikou Reservoir. Therefore, the main trend of the evolution of the MRE subaqueous delta over the past 70 years has changed from accretion (1950-1992) to erosion (1993-1997, 2011-2019) (Figure 4B). The variation in sediment flux into the sea (Figure 3A) is the primary driving factor in the dynamic changes of the MRE subaqueous delta. Referring to the research experience on the morphological changes of river mouth subaqueous landforms caused by the construction of large and medium-sized reservoirs in other basins, after the operation of the Shuikou Reservoir in 1993, the MRE subaqueous delta would remain in an erosional state for a relatively long period. However, the MRE subaqueous delta only maintained a significant erosional state for five years from 1993 to 1998 before rapidly transitioning back to an accretion state. Moreover, the amount of accretion during this period was much greater than the cumulative sediment flux into the sea from 1993 to 1998, indicating that there must be other factors driving the change in erosional and accretion states during this period.
4.4 The impact of ocean dynamic factors on the sedimentary changes in the MRE
The ocean dynamic factors that affect the geomorphology of the MRE mainly include tidal currents, waves, and coastal currents. The MR is a small-to-medium-sized mountain-stream river-shelf system, with a gentle shelf slope. After the river sediment enters the estuary, it is not quickly transported away but accumulates in the estuary area (Wang et al., 2022). The diffusion of sediment discharged into the sea is mainly controlled by tidal currents and waves. In the MRE, the suspended sediment concentration is generally lower in the surface layer compared to the bottom layer, and the suspended sediment concentration gradually decreases downstream along the river channel. Furthermore, there are significant variations within the tidal cycle. Due to the fine-grained nature of the suspended sediment particles in the estuary, under the interaction of tidal currents and river runoff, the suspended sediment primarily diffuses towards the mouth of the river. During this diffusion process, some sediment deposits in the shallow water areas along the shores and estuarine branches, while the majority of the sediment accumulates on the slope of the delta and in the prodelta (Liu et al., 2001). The sea surface outside the MR mouth is vast, with strong wave action, mainly composed of mixed waves consisting of wind waves and swell waves. The average annual wave height is 1.1 m, and the maximum wave height is 6.5 m. However, studies have not found significant changes in wave and tidal dynamics in the MRE, even though ebb tidal currents are the primary driving force shaping the estuary (Xu et al., 2014). The shaping of the subaqueous landforms in the MRE by tidal currents and waves mainly involves the transport and deposition of sediment from the river to the sea and the resuspension-redeposition of sediment within the estuarine area.
In addition, the coastal currents in the MRE are mainly Zhejiang-Fujian Coastal Current and Taiwan Warm Current (Yi et al., 2020) (Figure 5). The Zhejiang-Fujian coastal current has obvious seasonality, which is strong in winter and weak in summer. In winter, the Zhejiang-Fujian coastal current flows southward and northward in summer (Yi et al., 2020), and in winter, the Zhejiang-Fujian coastal current can carry the Yangtze River into the sea (Changjiang Water Resources Commission, 2000-2020). The sediment is transported southward and deposited along the coast. In addition to the surface of the Taiwan Warm Current, which is easily affected by the air front, the flow direction of the middle and lower layers is stable and always northward. Studies have shown that the muddy sedimentary area in the northeast corner of the MRE belongs to the southern end of the Zhejiang-Fujian Coastal Current and belongs to the distal mud of the Yangtze River Subaqueous Delta (Chen et al., 2010). Some scholars esteem that the siltation in 1998-2005 was caused by the sediment brought by the Zhejiang-Fujian coastal current in the case of the continuous decrease of the sediment volume from the MR into the sea (Chen et al., 2010; Lu, 2010). However, due to the increasing human activities in the Yangtze River Basin (especially the construction of the Three Gorges Dam), the sediment flux from the Yangtze River to the sea has also declined sharply. The survey found that the sediment flux from the Yangtze River Basin in the 2000s has decreased by 75% compared with that in the 1960s, resulting in the erosion of its underwater delta (Wang et al., 2020). Affected by the decrease of sediment load from the Yangtze River to the sea, the sediment carried southward by the Zhejiang-Fujian Coastal Current will also continue to decrease, and there is a time lag of 10-14 years (Liu et al., 2021). In addition, some studies have found that in recent years, the Zhejiang-Fujian Coastal Current has been cut off in the Wenling area of Zhejiang Province in winter (Chen and Jiang, 2017). Therefore, the sudden change of sedimentation in the MRE during the period from 1998 to 2005 is unlikely to be caused by the sediment brought by the Zhejiang-Fujian Coastal Current. Although the Taiwan Warm Current is the backbone of the Fujian-Zhejiang offshore current, it (except the surface layer) flows almost all year round along the isobath to the northeast (Hu et al., 1980). The material of the Taiwan River into the sea is carried northward by the strengthened Taiwan Warm Current in summer and is affected by the Zhejiang-Fujian Coastal Current and the ‘water barrier ‘effect of the Taiwan Warm Current shear front near the water depth of 50m (Pan et al., 1985; Liu et al., 2021), which is difficult to spread westward. At the same time, the study of Yu et al. (2002) also pointed out that the Pliocene-Pleistocene sediments from Taiwan were transported westward, which was blocked by the convex structure and could not be transported westward to the central Taiwan Strait. Therefore, it is less likely that the exogenous sediment transported by marine power will cause significant siltation in the underwater delta of the MRE during 1998-2005.
4.5 The impact of extreme weather such as typhoons on the sedimentation and erosion changes in the MRE
Globally, the increasing frequency of typhoons and flood events has a substantial impact on the evolution of river delta systems. Extreme events such as typhoons and floods disrupt the hydraulic dynamics and sediment sources in deltaic regions, leading to rapid and significant changes in the sedimentary environment of river subaqueous deltas (Carlin and Dellapenna, 2014). Typhoons have a strong impact on coastal landforms, submarine topography, and sediment transport in the river mouth. Although the duration of typhoon’s action is relatively short, its energy concentration and strength can cause significant changes in the seabed topography and landform in a short period. The impact of a strong typhoon often exceeds the changes throughout the season under normal tides. Research has found that while typhoons bring floods and disasters to delta cities, they also bring the material for delta development - sediment. This can largely compensate for the insufficient sediment supply to the river delta caused by the reduction of upstream sediment (Wang et al., 2020). However, Hurricane Sandy in 2012 only caused minor estuarine morphological change in Barnegat Inlet, New Jersey, and induced a sediment deposition of 3 cm across the near shore and inner shelf of the Mid-Atlantic Bight (Miles et al., 2015; Miselis et al., 2016). Therefore, whether erosion or deposition occurs during TCs is still undetermined, particularly in mega-deltas experiencing a reduction in riverine sediment inputs. Taking the Yangtze River as an example, in the past 20 years, there have been 0-2 typhoons annually affecting delta development at the mouth of the Yangtze River (Figure 1A). Rough estimates suggest that they can transport 8.91-46.83×106 m3 of sediment from the sea to the South Basin and nearby waters, which is equivalent to 9.2%-48.4% of the annual average sediment discharge into the sea (after the Three Gorges reservoir was built) (Wang et al., 2020). As sediment supply from the river and the coastal currents of the Zhejiang-Fujian coast continue to decrease, there has been a transformation from erosion to deposition in the underwater delta of the MR. The sea-derived sediment brought by typhoons may be one of the reasons for this transformation. Due to its geographical location, according to relevant studies and statistics from 1950 to 2010, the marine area of the MRE experienced an average of 5.7 typhoons per year, making it one of the most significantly affected areas by typhoons in China (Chen et al., 2015). During 1998-2005, frequent typhoons passed through Fuzhou, such as OTTO in 1998, Feiyan and Taozhi in 2001, and Haitang and Talim in 2005. Through the analysis of typhoon tracks, we found that three typhoons were passing through the MRE (research area) during 1950-1975 and 1998-2005, while there was only one typhoon passing through or no typhoon passing through in other periods (Figure 6). Accumulated sediment load into the sea from 1998 to 2005 was 0.27×108 m3, while the net sedimentation and erosion at the river mouth was 1.05×108 m3. It is unclear where the sediment, which caused the underwater delta to silt up by about 0.8×108 m3, came from. Combining relevant research, it is speculated that there are two main possibilities for the source of sediment: one is from the sediment in the MR channel below the Zhuqi station, and the other is from the sea-derived sediment brought by typhoons. The MRE later transformed again from deposition to erosion, which indicates that the impact of extreme weather on sedimentation in the river mouth is likely to be temporary and cannot change the overall transformation process from sedimentation to erosion in the river mouth under the context of decreasing sediment supply into the sea.
During the flood, the rapid water flow caused severe erosion and undercutting of the upper reaches of the delta, and a large amount of sediment was transported to the outside of the delta (Wang et al., 2020). About 40% of the sediment of the Yangtze River is deposited in the estuary every year, and the rest of the sediment escapes to the sea to form an underwater delta (Chen et al., 1985; Wang et al., 2020). Due to the inflow of a large amount of sediment carried by high-flow runoff in the flood season, significant siltation may occur in the offshore area. However, the intensity of sediment deposition is affected by the size of seasonal runoff (Dai et al., 2015). However, some studies have shown that only extreme floods, rather than normal high flow in the flood season, can induce erosion in the upper reaches of the delta (Gallagher and Ross, 2018). The MR is located in the subtropical monsoon region, where floods are relatively severe in the basin. According to incomplete statistics, there were 20 major floods in the MR from 1949 to 1998, with an average occurrence rate of 2.5 years, including 9 catastrophic floods, with an average occurrence rate of 5.6 years. In these years, the peak flow at the Zhuqi hydrological station exceeded 18000 m3/s (Ye, 2003). From 1998 to 2005, the drought and flood disasters in the MR were extremely severe, except for the years of continuous drought in 2003-2004, large floods occurred in other years, with the peak flow at the Zhuqi station exceeding 10000 m3/s, and in 2000 and 2002, exceeding 20000 m3/s. In June 1998 and June 2005 respectively, the first and second largest floods in the MR basin since the recorded data occurred. The peak flow at the Zhuqi station reached 33800 m3/s and 30600 m3/s respectively (Ye, 2003; Wang, 2014). Large and catastrophic floods in the mainstream of the MR occurred frequently during this period, which must have caused severe erosion in the downstream river channel, carrying a large amount of downstream river sediment into the sea, causing sedimentation in the river mouth and possibly promoting significant siltation in the MRE’s underwater delta from 1998 to 2005. In recent years, although there have been frequent floods above the warning level in the MR basin, the impacts have been relatively mild, and there have been no catastrophic floods.
In summary, after the operation of the Shuikou Reservoir in 1993, the sediment discharge into the MRE remained at a low level for an extended period. The estuary transitioned from a predominantly depositional state to an erosional state. However, from 1998 to 2005, there was a significant accretion in the subaqueous delta of the MRE. An analysis of flood and typhoon events (Table 3) in the MR watershed between 1950 and 2019 reveals that the period from 1998 to 2005 coincided with frequent flood events in the watershed and numerous typhoons passing through the estuarine waters. These extreme climate events triggered a change in the estuarine sedimentation regime during that period. However, the impact of floods and typhoons on delta sedimentation is short-term. The accretion in the MRE during 1998-2005 was followed by a sharp decrease in the accretion from 2005-2011, with only 0.17× 108 m3 of sediment accumulation. Then, from 2011 to 2019, the estuary reverted back to an erosional state. This suggests that the variation in sediment flux into the sea remains the primary driving factor in the dynamic changes of the subaqueous delta of the MRE, while extreme climate events can modify the short-term evolution of the delta. The driving factors for estuarine delta evolution vary across different time scales. It is important to note that the evidence provided so far does not fully support this viewpoint, and further empirical data and evidence are required to substantiate this observation.
4.6 Effects of human activities on the changes of erosion and deposition in the MRE
Human activities are the main cause of the increase and decrease of sediment input in the estuary area. In the past 70 years, the sediment into the sea of the MR has shown a significant decreasing trend. From the 1950s to the 1980s, the average annual sediment into the sea of the MRE has been maintained at more than 6 million tons, and the underwater delta in the estuary area has been strongly silted up. However, since the 1960s, 8 large reservoirs (Table 4), 19 medium-sized reservoirs (Figure 7A) and 688 small reservoirs have been built in the basin (Zhuang and Shi, 2010). The interception effect of the reservoir on the upstream sediment has gradually reduced the sediment into the sea, especially since the completion and operation of the Shuikou Reservoir in 1993. The sediment volume of the MR into the sea has dropped sharply. According to the measured data of the Zhuqi Station, the average sediment concentration of the river after the completion of the Shuikou Hydropower Station is 0.04 kg/m3, which is about 60% lower than the 0.11 kg/m3 before the dam construction. Therefore, there was a severe erosion phenomenon during the 1990s. Large-scale reservoir construction within the watershed is a decisive factor in the sharp decline of sediment discharge into the MRE, leading to adjustments in estuarine geomorphology.
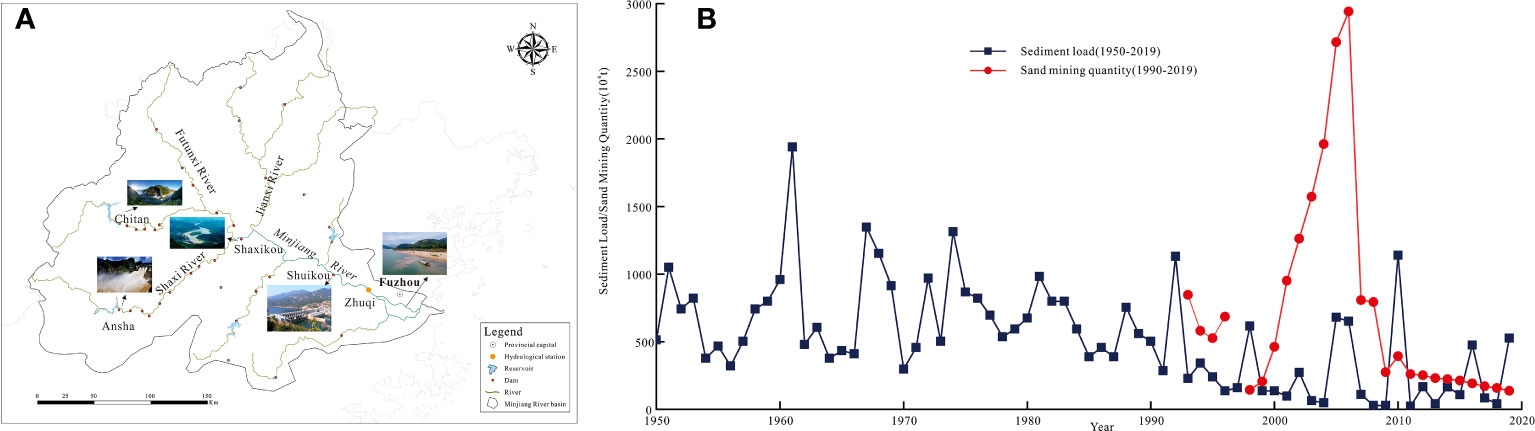
Figure 7 (A) Distribution of large and medium-sized reservoirs and dams in the Minjiang River Basin.; (B) The sediment load of Minjiang River (1950-2019) and the amount of sand mining in the lower reaches of Minjiang River (1990-2019).
In addition, human activities in the estuary are also one of the reasons that affect the erosion and deposition changes in the estuary area, mainly including reclamation, river regulation projects and sand mining (Dai et al., 2015). In the past 70 years, the influence of reclamation on the MRE area has become more and more serious. The influence of reclamation on the underwater delta of the MRE is very limited, while the influence on the onshore delta is huge, which promotes the change of the coastline. The MRE has carried out three phases of navigation channel regulation projects, and a large number of spur dikes and spur dikes have been built (Table 5). The first phase of the navigation regulation project of MR was from 1981 to 1987, and a guide dam was built at the southern end of Chuanshi Island, which was 2.35 km long. The second phase of the regulation project was completed in 1998, and the inner sand was comprehensively regulated. A shallow dam and an inverted fish mouth dam were built at the iron mouth, three spur dikes were built at the north dike, and a section of the dike (about 1.3 km long) and two spur dikes were built in the southeast of Hujiang Island. Since the second phase of the regulation project, dikes, spur dikes and other buildings have not only made the water flow to the channel, but deepened the sea channel, the pattern of the deep channel of the shoal in the inner sand section is stable, and most of the 5 m isobaths are in a state of penetration, but the building intercepts a large amount of sediment into the sea, causing siltation at the iron mouth. Some scholars find that the transition from erosion to siltation in the MRE from 1998 to 2005 is especially due to the interception of the transit sediment by the spur dike on the guide dam, which plays a role in promoting siltation (Lu, 2010). However, the impact of buildings such as spur dikes on the subaqueous delta of the estuary is mainly concentrated in the vicinity of the building, and the impact on the evolution of the entire estuary is very limited. In the case of continuous reduction of sediment into the sea, the average annual amount of erosion and deposition is as high as 1.50×107 m3/yr, even higher than the average annual amount of erosion and deposition from 1950 to 1975 (1.24×107 m3/yr), and the construction of dams along the coast is far from this effect.
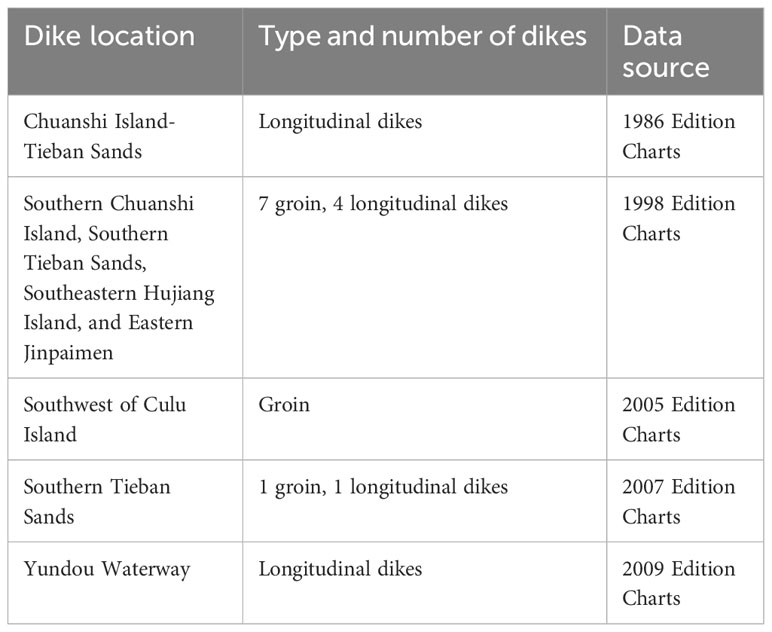
Table 5 Minjiang River Estuary barrage sand channel improvement groin, longitudinal dikes construction.
The sand mining activities in the downstream river channel of the MR and the estuarine region have also had a significant impact on the evolution of the subaqueous delta. The extensive mining or dredging of sediment has led to erosion of the riverbed in the estuary and a reduction in sediment deposition (Lu et al., 2023). Since the 1980s, with the rapid development of engineering construction and urbanization, the demand for sand and gravel has been continuously increasing. At the same time, sand mining technologies have significantly improved, leading to the flourishing development of the sand mining industry in the downstream section of the MR. According to incomplete statistics, before 1990, the annual sand mining volume in the downstream section of the MR was generally below 3 million tons. However, in the late 1990s, illegal sand mining became rampant, and the sand mining volume in the downstream section of the river dramatically increased (Figure 7B). The average annual sand mining volume exceeded 10 million tons, with the highest annual mining volume reaching 15 million tons. The volume of sand excavated per year was several times higher than the sediment load coming into the river basin, resulting in severe riverbed incisions in the MR and posing risks to the river channel. It is worth noting that the sharp increase in sand mining volume from the late 1990s to the mid-2000s was closely related to the decrease in sediment discharge into the MRE and the abrupt changes in sedimentation dynamics in the river mouth area. With the introduction of policies and enhanced management of sand mining in the river channels by the Fuzhou municipal government, sand mining activities in the downstream section of the Minjiang River have gradually been regulated in recent years. The impact of sediment mining or dredging on the geomorphic evolution of the estuary is temporary, especially in the estuarine region. In the short term, it can directly cause drastic changes in the subaqueous geomorphology of the estuary. However, when analyzed over a longer time scale, with the standardization and scientific management of sand mining activities, the potential adjustment of the subaqueous geomorphology of the entire estuarine region caused by sand mining activities is likely to be relatively small.
5 Conclusion
Before 1993, the underwater delta showed a clear accumulation trend. However, after the impoundment of the Shuikou Reservoir in 1993, the sediment discharge into the sea decreased, leading to a predominance of erosion in the estuary. The main driving factor influencing the evolution of sedimentation and erosion is the supply of sediment into the sea, with an annual average sediment flux of about 570.3×104 t/yr being the critical threshold for the transition between sedimentation and erosion. After the completion of the dam, the underwater delta changed from significant erosion in 1993-1998 to significant deposition in 1998-2005. During this period, the net accumulation of sediment reached 1.05 × 108 m3, far more than the accumulation of 1975-1992 before the construction of the dam. However, from 1998 to 2005, the total sediment discharge of MR into the sea was only 0.27 × 108 m3. From 1998 to 2005, frequent transit typhoons and frequent flood events may be the cause of additional siltation.
While the driving factors of MRE morphological evolution at longer timescales have been well understood, further empirical data and research are needed to confirm the specific impacts of typhoons and flood events on the erosion and deposition dynamics of the MRE. In addition, the future trends of MRE morphological evolution remain an ongoing concern, especially under the coupling effects of human activities and extreme climate events. On the whole, this work provides an excellent example of the rapid response of small and medium-sized mountain estuaries to extreme weather events and the geomorphological evolution of underwater deltas under the background of sediment reduction.
Data availability statement
The raw data supporting the conclusions of this article will be made available by the authors, without undue reservation.
Author contributions
XLai: Conceptualization, Data curation, Formal Analysis, Funding acquisition, Methodology, Resources, Supervision, Visualization, Writing – original draft, Writing – review & editing. JJia: Data curation, Formal Analysis, Methodology, Visualization, Writing – original draft, Writing – review & editing. YH: Data curation, Formal Analysis, Methodology, Visualization, Writing – original draft, Writing – review & editing. BJ: Conceptualization, Supervision, Writing – review & editing. CL: Supervision, Writing – review & editing. XLin: Conceptualization, Supervision, Writing – review & editing. PX: Conceptualization, Supervision, Writing – review & editing. CC: Conceptualization, Supervision, Writing – review & editing. JJiang: Conceptualization, Supervision, Writing – review & editing. YL: Writing – review & editing, Conceptualization, Supervision. WW: Conceptualization, Supervision, Writing – review & editing.
Funding
The author(s) declare financial support was received for the research, authorship, and/or publication of this article. This research was funded by the National Natural Science Foundation of China (No. U22A20585, and No. 52109118) and the Guangdong Basic and Applied Basic Research Foundation (NO. 2023A1515030088).
Conflict of interest
The authors declare that the research was conducted in the absence of any commercial or financial relationships that could be construed as a potential conflict of interest.
Publisher’s note
All claims expressed in this article are solely those of the authors and do not necessarily represent those of their affiliated organizations, or those of the publisher, the editors and the reviewers. Any product that may be evaluated in this article, or claim that may be made by its manufacturer, is not guaranteed or endorsed by the publisher.
References
Anthony E. J., Brunier G., Besset M., Goichot M., Dussouillez P., Nguyen V. L. (2015). Linking rapid erosion of the Mekong River delta to human activities. Sci. Rep. 5 (01), 14745. doi: 10.1038/srep14745
Asselman N. (2000). Fitting and interpretation of sediment rating curves. J. Hydrology 234 (3-4), 228–248. doi: 10.1016/S0022-1694(00)00253-5
Bi L., Yang S., Li C., Guo Y., Wang Q., Liu J. T., et al. (2015). Geochemistry of river-borne clays entering the East China Sea indicates two contrasting types of weathering and sediment transport processes. Geochemistry Geophysics Geosystems 16 (09), 3034–3052. doi: 10.1002/2015GC005867
Boudet L., Sabatier F., Radakovitch O. (2017). Modelling of sediment transport pattern in the mouth of the Rhone delta: Role of storm and flood events. Estuarine Coast. Shelf Sci. 198, 568–582. doi: 10.1016/j.ecss.2016.10.004
Carlin J., Dellapenna T. (2014). Event-driven deltaic sedimentation on a low-gradient, low-energy shelf: The Brazos River subaqueous delta, northwestern Gulf of Mexico. Mar. Geology 353, 21–30. doi: 10.1016/j.margeo.2014.03.017
Changjiang Water Resources Commission (2000-2020). Changjiang sediment bulletin (Wuhan: Changjiang Press).
Chen F., Wang H., Zheng Z., Zhang P., Ye Y. (1998). The formation and evolution of underwater delta in the Minjiang estuary: I. Environmental factors and geomorphological development of underwater delta formation. J. Appl. Oceanography (04), 396–401. doi: CNKI:SUN:TWHX.0.1998-04-006
Chen H. (2023). The influence of the sediment flux of Minjiang River into the sea on climate change and human activities has been studied in the past 70 years (Fuzhou: Fuzhou University). [dissertation/master’s thesis].
Chen J., Tang J., Li D. (2015). The impact of materials entering the sea on the mouth of the Minjiang River and coastal areas. Beijing: Science Press, 10–95.
Chen J., Yu X., Li D., Lai Z., Xu X., Huang C. (2010). Evolution and genesis of seabed geomorphology in the past years at the mouth of the Minjiang River. Mar. Eng. 28 (02), 82–89. doi: CNKI:SUN:HYGC.0.2010-02-012
Chen R., Jiang X. (2017). Remote sensing analysis of recent changes in the transport of suspended sediment from the Yangtze River estuary to the Zhejiang and Fujian coasts. Mar. Sci. 41 (03), 89–101. doi: 10.11759/hykx20160920002
Chen X., Liu J., Guo L., Liu X., Yin P. (2016). Short-scale sedimentary dynamics in the coastal waters of the Dagu River estuary in Jiaozhou Bay. Mar. geology Quaternary geology 36 (06), 45–50. doi: CNKI:SUN:HYDZ.0.2016-06-007
Chen Y., Peng G., Jiao W. (1985). Radiocarbon dates from the East China Sea and their geological implications. Quaternary Res. Interdiscip. J. Seattle Wash 24 (2), 197–203. doi: 10.1016/0033-5894(85)90006-7
Cheng P., Li M., Li Y. (2013). Generation of an estuarine sediment plume by a tropical storm. J. Geophysical Research: Oceans 118 (2), 856–868. doi: 10.1002/jgrc.20070
Dai Z., Liu J., Wen W. (2015). Morphological evolution of the south passage in the Changjiang (Yangtze River) estuary, China. Quaternary Int. 380-381, 314–326. doi: 10.1016/j.quaint.2015.01.045
Darby S. E., Hackney C. R., Leyland J., Kummu M., Lauri H., Parsons D. R., et al. (2016). Fluvial sediment supply to a mega-delta reduced by shifting tropical-cyclone activity. Nature 539 (7628), 276–279. doi: 10.1038/nature19809
Dürr H. H., Laruelle G. G., van Kempen C. M., Slomp C. P., Meybeck M., Middelkoop H. (2011). Worldwide typology of nearshore coastal systems: defining the estuarine filter of river inputs to the oceans. Estuaries Coasts 34, 441–458. doi: 10.1007/s12237-011-9381-y
FitzGerald D. M., Georgiou I., Miner M. (2015). “Estuaries and tidal inlets,” in Coastal Environments and Global Change Wiley online books. Eds. Masselink G., Gehrels R. (Chichester, UK, UK: John Wiley & Sons, Ltd), 268–298. doi: 10.1002/9781119117261.ch12
Gallagher J., Ross D. (2018). Sediment geochronology for bar-built estuaries subject to flood deposition and erosion: A robust multiproxy approach across an estuarine zone. Holocene 28, 341–353. doi: 10.1177/0959683617729441
Ganju N. K., Knowles N., Schoellhamer D. H. (2008). Temporal downscaling of decadal sediment load estimates to a daily interval for use in hindcast simulations. J. Hydrology 349 (03-04), 512–523. doi: 10.1016/j.jhydrol.2007.11.026
Gao J., Jun L., Harry W., Bai F., Cheng Y., Wang Y. (2012). Rapid changes of sediment dynamic processes in Yalu River Estuary under anthropogenic impacts. Int. J. Sediment Res. 27 (1), 37–49. doi: 10.1016/S1001-6279(12)60014-6
Gao J., Li J., Wang Y., Bai F., Li J., Cheng Y. (2009). Composition and distribution of heavy minerals in sediments in estuaries and coastal waters of the Yalu River and their sedimentary kinetics. Acta Oceanologica Sinica 31 (03), 84–94. doi: 10.3321/j.issn:0253-4193.2009.03.010
Guo L., Zhu C., Xie W., Xu F., Wu H., Wan Y., et al. (2021). Changjiang Delta in the Anthropocene: Multi-scale hydro-morphodynamics and management challenges. Earth-Science Rev. 223, 103850. doi: 10.1016/j.earscirev.2021.103850
Hayes M. O. (1975). “Morphology of sand accumulation in estuaries,” in Estuarine research. Ed. Cronin L. E. (London, UK and New York, USA: Academic Press, Inc), 3–22.
Hilton R. G., Galy A., Hovius N., Horng M. J., Chen H. (2011). Efficient transport of fossil organic carbon to the ocean by steep mountain rivers: An orogenic carbon sequestration mechanism. Geology 39 (01), 71–74. doi: 10.1130/G31352.1
Hu D., Lv L., Xiong Q., Ding Z., Sun S. (1980). A study on upwelling along the coast of Zhejiang. Sci. Bull. (03), 131–133. doi: CNKI:SUN:KXTB.0.1980-03-009
Hu C., Wang E., Wang X. (2015). Analysis of river course evolution under the combined action of reservoir and river sand mining. J. Water Resour. Water Eng. 03), 178–183. doi: 10.11705/j.issn.1672-643X.2015.03.37
Ji H., Chen S., Jiang C., Fan Y., Fu Y., Li P., et al. (2022). Damming-induced hydrogeomorphic transition in downstream channel and delta: A case study of the yellow river, China. Water 14 (13), 2079. doi: 10.3390/w14132079
Lai X., Hou Y., Jia J., Chen C., Su Y., Jiang J., et al. (2023). Rapid change of erosion-deposition evolution in the Minjiang estuary, Southeast China. Ocean Coast. Manage. 238, 106574. doi: 10.1016/j.ocecoaman.2023.106574
Li S. (2017). Study on the change process of water and sediment in South Asian tropical rivers flowing into the sea. [dissertation/master’s thesis]. (Shanghai: East China Normal University).
Li B. (2020). Review of the dynamic mechanism of geomorphological evolution in estuarine delta of the world. Shanghai Land Resour. 41 (04), 93–97. doi: 10.3969/j.issn.2095-1329.2020.04.018
Li D., Chen J., Wang A., Yu X. (2008). Research progress on sedimentary dynamics in the mouth of the Minjiang River. Mar. Bull. 27 (02), 111–116. doi: 10.3969/j.issn.1001-6392.2008.02.017
Li X., Liu J. P., Saito Y., Nguyen V. L. (2017). Recent evolution of the Mekong Delta and the impacts of dams. Earth-Science Rev. 175, 1–17. doi: 10.1016/j.earscirev.2017.10.008
Lin L. (2009). Research and discussion on sand mining in the lower reaches of the Minjiang River. Water Sci. Technol. (02), 21–23. doi: CNKI:SUN:SLKI.0.2009-02-008
Liu S., Gao J., Xu X., Shi Y., Shu Z., Wu H., et al. (2021). Response of sediment particle size components in argillaceous areas along Zhejiang and Fujian to the decrease of sediment transport into the Yangtze River. Acta Oceanologica Sin. 43 (03), 105–115. doi: 10.12284/hyxb2021025
Liu J. T., Hsu R. T., Hung J. J., Chang Y. P., Wang Y. H., Rendle-Bühring R. H., et al. (2016). From the highest to the deepest: The Gaoping River–Gaoping Submarine Canyon dispersal system. Earth-Science Rev. 153, 274–300. doi: 10.1016/j.earscirev.2015.10.012
Liu C., Jia H., Chen X. (2001). Sedimentary structure and sedimentation of Minjiang River 11. Oceans Lakes 32 (2), 8. doi: 10.3321/j.issn:0029-814X.2001.02.009
Lu H. (2010). Study on seabed topography and geomorphological features in the Minjiang estuary and adjacent seas. (Xiamen: Third Institute of Oceanography, State Oceanic Administration). [dissertation/master’s thesis].
Lu H., Wang C., Cai F., Wu C., Bao J., Zheng Y., et al. (2023). Morphological change and its response to human activities in the Estuary of Minjiang River, China since last early century. Earth Surface Processes Landforms 48 (10), 1940–1955. doi: 10.1002/esp.5595
Luan H. (2017). Establishment and application of prediction model for interdecadal erosion evolution in the Yangtze River estuary. [dissertation/master’s thesis]. Shanghai: East China Normal University.
Luan H., Ding P., Yang S., Wang Z. (2021). Accretion-erosion conversion in the subaqueous Yangtze Delta in response to fluvial sediment decline. Geomorphology 382, 107680. doi: 10.1016/j.geomorph.2021.107680
Maloney J. M., Bentley S. J., Xu K., Obelcz J., Georgiou I. Y., Miner M. D. (2018). Mississippi River subaqueous delta is entering a stage of retrogradation. Mar. Geology 400, 12–23. doi: 10.1016/j.margeo.2018.03.001
Mei X., Dai Z., Wei W., Li W., Wang J., Sheng H. (2018). Secular bathymetric variations of the North Channel in the Changjiang (Yangtze) Estuary, China 1880–2013: Causes and effects. Geomorphology 303, 30–40. doi: 10.1016/j.geomorph.2017.11.014
Miles T., Seroka G., Kohut J., Schofield O., Glenn S. (2015). Glider observations and modeling of sediment transport in Hurricane Sandy. J. Geophysical Research: Oceans 120, 1771–1791. doi: 10.1002/2014JC010474
Milliman J. D., Farnsworth K. L., Jones P. D., Xu K. H., Smith L. C. (2008). Climatic and anthropogenic factors affecting river discharge to the global ocean 1951–2000. Global planetary Change 62 (03-04), 187–194. doi: 10.1016/j.gloplacha.2008.03.001
Milliman J. D., Meade R. H. (1983). World-wide delivery of river sediment to the oceans. J. Geology 91 (01), 1–21. doi: 10.1086/628741
Miselis J., Andrews B., Nicholson R., Defne Z., Ganju N., Navoy A. (2016). Evolution of mid-Atlantic coastal and back-barrier estuary environments in response to a hurricane: Implications for barrier-estuary connectivity. Estuaries Coasts 39, 916–934. doi: 10.1007/s12237-015-0057-x
Müller G., Förstner U. (1968). General relationship between suspended sediment concentration and water discharge in the Alpenrhein and some other rivers. Nature 217 (5125), 244–245. doi: 10.1038/217244a0
Nienhuis J. H., Ashton A. D., Edmonds D. A., Hoitink A. J. F., Kettner A. J., Rowland J. C., et al. (2020). Global-scale human impact on delta morphology has led to net land area gain. Nature 577 (7791), 514–518. doi: 10.1038/s41586-019-1905-9
Nittrouer J. A., Viparelli E. (2014). Sand as a stable and sustainable resource for nourishing the Mississippi River delta. Nat. Geosci. 7 (05), 350–354. doi: 10.1038/ngeo2142
Pan Y., Xu D., Xu J. (1985). Front structure, changes and causes of upwelling zone along Zhejiang coast. Acta Oceanologica Sin. (Chinese edition) 07 (04), 401–411.
Rodriguez A., McKee B., Miller C., Bost M., Atencio A. (2020). Coastal sedimentation across North America doubled in the 20th century despite river dams. Nat. Commun. 11 (1), 3249. doi: 10.1038/s41467-020-16994-z
Shi Y., Liu Z., Gao J., Yang Y., Wang Y. (2017). The response of sedimentary record to catchment changes induced by human activities in the western intertidal flat of Yalu River Estuary, China. Acta Oceanologica Sin. 36, 54–63. doi: 10.1007/s13131-016-0941-7
Stanley D. J. (1996). Nile delta: extreme case of sediment entrapment on a delta plain and consequent coastal land loss. Mar. Geology 129 (03-04), 189–195. doi: 10.1016/0025-3227(96)83344-5
Syvitski J., Ángel J. R., Saito Y., Overeem I., Vörösmarty C. J., Wang H., et al. (2022). Earth’s sediment cycle during the Anthropocene. Nat. Rev. Earth Environ. 3 (03), 179–196. doi: 10.1038/s43017-021-00253-w
Syvitski J. P. M., Kettner A. J., Overeem I., Hutton E. W. H., Hannon M. T., Brakenridge G. R., et al. (2009). Sinking deltas due to human activities. Nat. Geosci 2, 681–686. doi: 10.1038/ngeo629
Syvitski J. P., Morehead M. D., Bahr D. B., Mulder T. (2000). Estimating fluvial sediment transport: the rating parameters. Water Resour. Res. 36 (09), 2747–2760. doi: 10.1029/2000WR900133
Tang R., Dai Z., Zhou X., Li S. (2021). Tropical cyclone-induced water and suspended sediment discharge delivered by mountainous rivers into the Beibu Gulf, South China. Geomorphology 389, 107844. doi: 10.1016/j.geomorph.2021.107844
Vörösmarty C. J., Meybeck M., Fekete B., Sharma K., Green P., Syvitski J. P. M. (2003). Anthropogenic sediment retention: major global impact from registered river impoundments. Glob Planet Change 39, 169–190. doi: 10.1016/S0921-8181(03)00023-7
Wang H., Zhang Z., Hu Y. (2011). The impact of water conservancy projects in the Yalu River basin on estuarine deltas. J Ecol. 30 (8), 1799–1804.
Wang Y. (2014). Temporal variation characteristics of precipitation runoff in Minjiang River Basin and identification of flood and drought events. [master’s thesis]. (Fuzhou: Fujian Normal University).
Wang J., Dai Z., Mei X., Fagherazzi S. (2020). Tropical cyclones significantly alleviate mega-deltaic erosion induced by high riverine flow. Geophysical Res. Lett. 47 (19), e2020GL089065. doi: 10.1029/2020GL089065
Wang S., Fan Y., Liu M., Wu X., Wang H., Bi N. (2022). Spatial-temporal variation characteristics of silting in the abandoned estuary of Qingshuigou of the Yellow River. Mar. Geology Frontier 38 (5), 1–11. doi: 10.16028/j.1009-2722.2021.082
Wang S., Fu B., Piao S., Lü Y., Ciais P., Feng X., et al. (2016). Reduced sediment transport in the Yellow River due to anthropogenic changes. Nat. Geosci. 9 (01), 38–41. doi: 10.1038/ngeo2602
Wang J., Wang X. (1995). Application of modern historical charts in port channel engineering. Mar. Bull. 014 (003), 74–80. doi: CNKI:SUN:HUTB.0.1995-03-009
Wang A., Ye X., Xu X., Xie J., Tao S., Lai Z., et al. (2022). Source-sink process of subtropical small and medium-sized mountain and stream river-wide shelf system: A case study of the Minjiang-East China Sea shelf system. J. Sedimentology 40 (06), 1615–1634. doi: 10.14027/j.issn.1000-0550.2022.135
Wright L. D., Nittrouer C. A. (1995). Dispersal of river sediments in coastal seas: six contrasting cases. Estuaries 18, 494–508. doi: 10.2307/1352367
Wu C. S., Yang S., Huang S., Mu J. (2016). Delta changes in the Pearl River estuary and its response to human activities, (1954–2008). Quaternary Int. 392, 147–154. doi: 10.1016/j.quaint.2015.04.009
Xia S., Zhang H., Hao J., Yang J. (2021). Study on the relationship characteristics and driving factors of water and sediment at the small river dam station of the Fujiang River of the Yangtze River. Resour. Environ. Yangtze River Basin 30 (7), 11. doi: 10.11870/cjlyzyyhj202107008
Xie D., Pan C., Wu X., Gao S., Wang Z. (2017). Local human activities overwhelm decreased sediment supply from the Changjiang River: Continued rapid accumulation in the Hangzhou Bay-Qiantang Estuary system. Mar. Geology 392, 66–77. doi: 10.1016/j.margeo.2017.08.013
Xie F., Zhao G., Mu X., Gao P., Sun W. (2021). Changes in the relationship between water and sediment in the mainstream of the Yellow River in the past 70 years. Sci. Soil Water Conserv. China 05), 1–9. doi: 10.16843/j.sswc.2021.05.001
Xu Y., Cai F., Lu H., Wu C., Zheng Y., Bao J. (2014). Characteristics of linear sedimentary sand outside the Minjiang estuary and Jiulongjiang estuaries in Fujian. Acta Oceanologica Sinica 36 (05), 142–151. doi: 10.3969/j.issn.0253-4193.2014.05.016
Xu X., Chen J., Lai Z. (2009). Evolution of seabed topography and geomorphology in the Minjiang estuary in the past hundred years with the support of GIS. Taiwan strait 28 (04), 577–585. doi: CNKI:SUN:TWHX.0.2009-04-022
Xue C. (2023). Sedimentary records and coastal system response of the Yellow River diversion event from 1128 to 1855. [dissertation/master’s thesis]. Shanghai: East China Normal University. doi: 10.27149/d.cnki.ghdsu.2023.000116
Yang G., Chen Z., Yu F., Wang Z., Zhao Y., Wang Z. (2007). Sediment rating parameters and their implications: Yangtze River, China. Geomorphology 85 (3-4), 166–175. doi: 10.1016/j.geomorph.2006.03.016
Yang S., Bi L., Li C., Wang Z., Dou Y. (2016). Major sinks of the Changjiang (Yangtze River)-derived sediments in the East China Sea during the late Quaternary. Geological Society London Special Publications 429 (01), 137–152. doi: 10.1144/SP429.6
Yang S., Yin P. (2018). Sediment source and sink process of small and medium-sized rivers under the influence of natural environment change and human activities. Mar. geology Quaternary geology 38 (01), 1–10. doi: 10.16562/j.cnki.0256-1492.2018.01.001
Ye J. (2003). GIS-based flood risk analysis and zoning research in Minjiang River Basin. [dissertation/master’s thesis]. Fuzhou: Fujian Normal University.
Yi S., Wu C., Lin J., Hu X., Luo W. (2020). Development characteristics of sand wave group in the coastal waters of Minjiangkou-Sanshawankou, Fujian Province, its genesis and its influence on the application of marine engineering. Geology China 47 (5), 1554–1566. doi: 10.12029/gc20200519
Yu F., Zang J., Guo B., Hu Y. (2002). Some phenomena of the Kuroshio intrusion into shelf area and the shelf circulation of the East China Sea. Adv. Mar. Sci. 20 (03), 21–28. doi: 10.3969/j.issn.1671-6647.2002.03.004
Zhang J. (2006). Numerical simulation of estuarine coastal hydrodynamics and suspended sediment movement. [dissertation/master’s thesis]. Shanghai: Shanghai Jiao Tong University.
Zhang G., Yu Z., Yi L., Lei X., Qian F., Li J., et al. (2021). Changes in the relationship between water and sediment in the basin above Cuntan Station of the main stream of the Yangtze River in the past 70 years and its driving factors. J. Soil Water Conserv. 01), 79–84. doi: 10.13870/j.cnki.stbcxb.2021.01.011
Zhu C., Guo L., van Maren D., Tian B., Wang X., He Q., et al. (2019). Decadal morphological evolution of the mouth zone of the Yangtze Estuary in response to human interventions. Earth Surface Processes Landforms 44 (12), 2319–2332. doi: 10.1002/esp.4647
Keywords: driving factors, extreme climate, human activities, sediment, Minjiang River estuary
Citation: Lai X, Jia J, Hou Y, Jiang B, Lin C, Lin X, Xu P, Chen C, Jiang J, Liu Y and Wei W (2023) Analysis of the driving factors of the change of erosion-deposition in the Minjiang Estuary, Southeast China. Front. Mar. Sci. 10:1287169. doi: 10.3389/fmars.2023.1287169
Received: 01 September 2023; Accepted: 30 October 2023;
Published: 20 November 2023.
Edited by:
Achilleas G. Samaras, Democritus University of Thrace, GreeceCopyright © 2023 Lai, Jia, Hou, Jiang, Lin, Lin, Xu, Chen, Jiang, Liu and Wei. This is an open-access article distributed under the terms of the Creative Commons Attribution License (CC BY). The use, distribution or reproduction in other forums is permitted, provided the original author(s) and the copyright owner(s) are credited and that the original publication in this journal is cited, in accordance with accepted academic practice. No use, distribution or reproduction is permitted which does not comply with these terms.
*Correspondence: Beihan Jiang, jiangbeihan@fzu.edu.cn