- 1National Institute of Aquatic Resources, Technical University of Denmark, Kgs. Lyngby, Denmark
- 2Department of Mathematics and Statistics, University of Strathclyde, Glasgow, United Kingdom
Motivated by the global rise in demand for marine products, there is increased interest in exploitation of the mesopelagic zone. However, the feasibility of this potential fishery remains uncertain, partly due to limited biological data and knowledge on sustainability of exploitation, and due to questions related to its economic viability. Consequently, there is a demand for better insights into these factors before any commencement of a fishery. Here we use the DISPLACE individual-vessel based bio-economic model to evaluate economic and biological trade-offs of a fishery on Maurolicus muelleri and Benthosema glaciale, the main potential target fish species in the mesopelagic zone of the Northeast Atlantic. We found that a fish price between 1.6-5.0 €/kg is necessary for mesopelagic resources to assure a profitable mesopelagic fishery, which is twice that expected for species with similar fat content. These high fish prices are necessary to cover the high fuel consumption costs due to the distant fishing grounds. Furthermore, the distance of the fishing grounds makes the fuel tank capacity a limiting factor for the fishery. A first evaluation of preliminary harvest control strategies indicated that a low fishing mortality of F = 0.2 year-1 resulted in the highest potential profitability of the fishery. Restriction of gear mesh sizes did not significantly affect the profitability of the fishery. We show that the current ecological uncertainties, especially regarding the life-history, species-specific and spatio-temporal abundance and distribution estimates have a significant impact on the estimates of potential viability of the fishery. It is therefore of the utmost importance to gain more insights in those factors before investments are made into the development of such fishery.
1 Introduction
In this study, we examine the economic and biological trade-offs and interactions associated with a mesopelagic fishery targeting sub-populations of Maurolicus muelleri (Gmelin, 1789, Mueller’s pearlside) and Benthosema glaciale (Reinhardt, 1837, glacier lantern fish) in the Northeast Atlantic Ocean. We aim to identify ecological and economic thresholds for transitioning between fisheries in which Danish large-scale pelagic fishing vessels are currently involved.
1.1 Motivation for a potential mesopelagic fishery
The mesopelagic zone has gained considerable interest due to its potential as a new fisheries resource and the role mesopelagic organisms may play in carbon sequestration in the deep ocean (St. John et al., 2016; Grimaldo et al., 2020; Standal & Grimaldo, 2020; Fjeld et al., 2023; Schadeberg et al., 2023). Estimates of global mesopelagic fish biomass range between 1 and 15 Gt, though with significant uncertainty due to sampling challenges such as trawl avoidance and non-fish sources of acoustic energy (Gjosaeter, 1986; Kaartvedt et al., 2012; Irigoien et al., 2014; Anderson et al., 2019; Proud et al., 2019). The most recent biomass estimates using different modelling approaches result in biomass estimates of 2.4 Gt (Anderson et al., 2005) and 3.8-8.3 Gt (Proud et al., 2019). In the Northeast Atlantic Ocean, M. muelleri and B. glaciale are the primary target species for trial and potential mesopelagic fishing due to their abundance and nutritional value (Gjosaeter and Kawaguchi, 1980; Alvheim et al., 2020; Grimaldo et al., 2020). These species are mainly intended to be utilized for the fish meal and fish oil industry as well as for nutraceutical production (St. John et al., 2016; Prellezo, 2019; Grimaldo et al., 2020; Olsen et al., 2020; Paoletti et al., 2021). Although the suggested abundances and nutritional value of the species might be indicative for potential mesopelagic exploitation, the harvest should be ecologically sustainable and economically viable (van der Meer et al., 2023).
1.2 Uncertainty in mesopelagic biomass and population dynamics to support a potential mesopelagic fishery
To date there is limited knowledge of the species- and stock-specific abundance and distribution of M. muelleri and B. glaciale in the Northeast Atlantic Ocean. M. muelleri is more abundant near continental shelf breaks and seamounts, while B. glaciale is primarily found in the open ocean (Gjosaeter and Kawaguchi, 1980; Boehlert et al., 1994). An acoustics survey in Norwegian waters reported a mesopelagic biomass estimate ranging between 10-35 g/m2, and indicated that M. muelleri was the most abundant fish species (Gjosaeter, 1986). A spawning stock size of M. muelleri of 12 g/m2 was estimated based on a study of the distribution of fish eggs and larvae in the Northeast Atlantic (Gjosaeter and Kawaguchi, 1980). Furthermore, acoustic surveys reported biomasses of M. muelleri ranging between 71-162 thousand tonnes in the Bay of Biscay and 248 thousand tonnes in Icelandic waters (Jónsson et al., 2010; Sobradillo et al., 2019). A study using an Isaacs-Kidd midwater trawl reported a biomass between 0.1-2.4 g/m2, of which 97% was B. glaciale, which provides the only species-specific mentioning of biomass of B. glaciale in the Northeast Atlantic region (Gjosaeter and Kawaguchi, 1980).
As with their abundances and distributions, the growth and mortality rates of both species, and their associated spatial variability, remain uncertain (Vastenhoud et al., 2023). M. muelleri belongs to the family of hatchetfish (Sternoptychidae), while B. glaciale belongs to the family of lanternfish (Myctophidae). Both M. muelleri and B. glaciale are small size species, with a maximum length of approximately 7 and 10 cm, respectively (Gjosaeter, 1981b, 1981a). Similar to many mesopelagic organisms, both species perform extensive diel vertical migrations (DVM), as a tradeoff between growth and survival (Halliday, 1970; Kawaguchi and Mauchline, 1982; Kaartvedt et al., 2009; Dypvik et al., 2012; Staby et al., 2013; Prihartato et al., 2015).
1.3 Feasibility, challenges and uncertainty of a potential fishery
Detailed biological knowledge of stock-specific abundances, distribution patterns and life-histories are crucial in the evaluation of the sustainability of potential mesopelagic exploitation and the long-term economic viability of such a fishery. Previous mesopelagic trial fisheries conducted in the Northeast Atlantic region, the Oman Sea, the Arabian Sea, and the Southern Ocean have shown limited economic viability and poor catches (Valinassab et al., 2007; Marine Research Institute, 2015; Roberts et al., 2020; Standal and Grimaldo, 2020). For instance, the former Soviet Union reports indicated annual catches of lanternfish in the Southern Ocean ranging from 500-2,500 tonnes in 1980, peaking at 78,000 tonnes in 1990, but later the fishery was deemed economically unviable (Kock, 2000). Experimental fisheries targeting lanternfish in the Oman Sea reported daily catch rates of 24-28 tonnes or annual catches of 2,400-2,800 tonnes assuming 100 fishing days per year (Valinassab et al., 2007). Similarly, a trial fishery in Iceland targeting M. muelleri reported initial landings of 46,000 tonnes in 2009, followed by decreasing landings and eventual abandonment of the trial fishery in 2016 (Marine Research Institute, 2015). An experimental trial fishery off the west coast of Norway in June-July 2019 reported 1192 tonnes of M. muelleri (Standal and Grimaldo, 2020). Despite the variable and lower-than-expected catch rates observed in previous trial fisheries, the perceived potential of a mesopelagic fishery remains substantial. The Danish fishing industry estimates that catch rates of 200-500 tonnes of mesopelagic fish per haul, corresponding to approximately 40,000-100,000 tonnes annually assuming two long hauls per 24 hours and 100 fishing days per year, are required to obtain an economic viable fishery (Paoletti et al., 2021). These projected catch rates exceed those reported in previous trial fisheries in the region.
The substantial catch rates are necessary to cover the anticipated high operating costs of a potential mesopelagic fishery, associated with the use of adequately large and small meshed pelagic trawls operated at significant depths to filter large water volumes (Paoletti et al., 2021). Fuel costs, along with labor costs, constitute the primary contributors to fishing expenses, and fishing fleets face challenges when fuel prices are high (Abernethy et al., 2010; Cheilari et al., 2013). Optimizing trawl efficiency through gear adaptations, implementing herding mechanisms with artificial lights, or employing continuous pumping systems at the cod-end can help reduce operating costs (Paoletti et al., 2021; Underwood et al., 2021; Grimaldo et al., 2022). However, these gear optimizations require significant investments, which are already considerable due to necessary investment into new on-board conservation systems and methods or new fishing vessels (Paoletti et al., 2021). These expenses are required to increase the threshold for trip duration, which is currently limited to 3-5 days due to the rapid degradation of the species (Paoletti et al., 2021).
In addition to necessary catch volumes, the economic viability of a mesopelagic fishery highly depends on fish prices, which are currently uncertain. Prices for mesopelagic catch are expected to reflect those of fish with similar fat content used for industrial purposes, such as summer herring (Paoletti et al., 2021). Fish prices are likely to increase with higher fat content (Rana et al., 2009). Previously reported prices given current use for fish meal and oil range between 0.3-0.4 €/kg for M. muelleri in Norway, and in Denmark, prices are expected to range between 0.3-0.55 €/kg (Paoletti et al., 2021).
1.4 Management and governance of a potential mesopelagic fishery
In the Northeast Atlantic Ocean, most commercial fish stocks are managed through mechanisms such as individual vessel quotas (IVQs), access regulations, or total allowable catch (TAC). The absence of TACs, due to the lack of assessments and determination of MSY (Maximum Sustainable Yield) reference levels on stock basis in particular, may drive and limit the potential expansion of mesopelagic activities as fishing rights should be sought before the fishery becomes regulated or closed (Standal and Grimaldo, 2020). In an initial step, the potential mesopelagic fishery could be regulated by implementing fleet capacity and effort restrictions, which could involve limitations on the number of vessels, fishing duration, horsepower, or fishing gears and mesh sizes (Standal and Grimaldo, 2020). However, due to the complex role of mesopelagic species in the marine food web and the potential bycatch of other commercially important species, an ecosystem-based management approach and framework should be developed, following a precautionary approach (UN, 1982; FAO, 1995; UN, 1995; UN, 2002; ICES, 2019a). In that context other strategies to reduce ecosystem impacts and attain ecosystem-based fisheries management such as balanced harvesting could also be explored (Zhou et al., 2019). Ideally, this management framework should also consider the potentially significant ecosystem and global climate associated role of mesopelagic species in carbon sequestration to the deep ocean as part of the biological carbon pump (BCP) (Boyd et al., 2019). Processes involved in carbon and nutrient cycling within the mesopelagic zone play a crucial role in the annual sequestration of 2 to 6 billion metric tons of carbon (Buesseler and Boyd, 2009; Hoagland et al., 2019; Pinti et al., 2023). The potential diminishment of this regulating ecosystem service, for example due to climate change or exploitation, could attribute to significant economic losses by increased mitigation and adaptation costs (Barange et al., 2017). Considering the potentially high social opportunity costs of this fishery and the trade-offs between regulating, cultural, supporting and provisioning ecosystem services of the mesopelagic ecosystem (Hoagland et al., 2019), the final management framework and governance of the potential harvest in the mesopelagic should not be a purely scientific question, but also a legal and social consideration (Schadeberg et al., 2023).
1.5 Previous bio-economic modelling in a mesopelagic context
To assess the potential viability of a mesopelagic fishery, it is crucial to evaluate not only the ecological impacts and dynamics but also the economic viability and their interconnectedness to potential exploitation. Previous studies have utilized various bio-economic modeling approaches, including food-web models, to assess the potential viability and associated trade-offs of mesopelagic exploitation in different regions (Prellezo, 2019; Paoletti et al., 2021; Dowd et al., 2022; Kourantidou and Jin, 2022). These studies have identified economic potential in the exploitation of mesopelagic species given assumptions on costs and prices but have also highlighted potential impacts on stocks of epipelagic predators due to complex food web interactions, as well as knowledge gaps related to ecological processes (Prellezo, 2019; Paoletti et al., 2021; Dowd et al., 2022; Kourantidou and Jin, 2022).
Consideration of region- and fleet-specific differences in fisheries dynamics is particularly important in the context of mesopelagic fisheries, as motivations and opportunities may vary (Bastardie et al., 2021). Irish pelagic fishing vessels are currently not occupied during the full year, and mesopelagic species are considered an opportunity during seasons where the vessels would else be inactive (Groeneveld et al., 2022). In the Bay of Biscay, a potential mesopelagic fishery could represent a seasonal opportunity for demersal trawlers and seiners to compensate for effort limitations caused by discard bans in their current fisheries (Prellezo, 2019), while in Denmark a mesopelagic fishery would likely involve construction of new vessels since existing large-scale vessels are already to high extent occupied year-round (Prellezo, 2019; Paoletti et al., 2021). Participation in trial fisheries can also be motivated by the race to obtain historical fishing rights before the closure of an unregulated fishery (Standal and Grimaldo, 2020).
Integrated ecological-economic fisheries models (IEEFMs) are valuable tools for assessing the sustainability and impacts of potential mesopelagic exploitation and anticipating the effects of management actions on ecological, economic, and social dynamics (Nielsen et al., 2018). The individual-vessel-based bio-economic DISPLACE model, which considers the activity of vessels and their interaction with stocks at a highly spatially and seasonal disaggregated scale, is particularly useful for marine management (Bastardie et al., 2014). DISPLACE has previously been used as tool for Management Strategy Evaluation (MSE), for example to evaluate marine spatial plans or fishing impacts on the seafloor, and has been applied in different regions including the North Sea, Ionian Sea, the Baltic Sea and the Mediterranean Sea (Bastardie et al., 2014; Bastardie et al., 2017; Bastardie et al., 2020; Maina et al., 2021; Rufener et al., 2023).
This model is well-suited for studying the sustainability of potential mesopelagic fisheries as it considers spatial population dynamics and the variability of individual vessels, providing a finer-scale understanding of spatial and temporal mesopelagic fishery dynamics. The model has already been implemented and validated for the Danish fishery, which serves as a basis for the potential mesopelagic fishery. This dynamic simulation model complements static approaches, such as the one used by Paoletti et al. (2021). In the current study, the model is utilized to assess sensitivity to different ecological and economic drivers and to evaluate different management scenarios according to sustainability, and it can be iteratively updated in the future as more knowledge becomes available.
1.6 Objectives
In this study we analyze economic and biological trade-offs related to the potential mesopelagic exploitation, and we evaluate candidate strategies to manage those risks. With the DISPLACE individual-vessel based bio-economic model (Bastardie et al., 2014, 2021) we evaluate different scenarios for a mesopelagic fishery targeting sub-populations of M. muelleri and B. glaciale associated to the case of large-scale Danish pelagic fishery (Paoletti et al., 2021). Initially we evaluate the profitability of a mesopelagic fishery and compare this to the existing fisheries that the selected vessels are participating in to identify incentives to switch to a mesopelagic fishery. Subsequently we consider ranges and variability in (i) abundances of the target species in the different regions, (ii) fishing costs (fixed/variable costs and fuel prices) and (iii) fish prices. We evaluate the outcomes of the scenarios in terms of fleet and vessel-specific catches, revenue, and profit, and compare those to the fisheries that the vessels are currently participating in. On that basis we identify thresholds for switching between fisheries. The following research questions (RQ) are addressed:
● RQ 1: Do these populations occur in adequately high densities to support economically viable exploitation of M. muelleri and B. glaciale in the Northeast Atlantic?
● RQ 2: Are the costs of a mesopelagic fishery too high for a fishery to be viable for different populations?
● RQ 3: Are the fish prices adequately high to secure a long-term viable mesopelagic fishery, also considering the necessary investment?
Furthermore, we explore under the assumption of a viable fishery the impact of different Harvest Control Rules (HCRs), on the stock-specific biological sustainability and the economic viability of a mesopelagic fishery, according to (i) spatially explicit fishing mortality levels according to different F levels and (ii) fishing gear selectivity ranges (L50 and L75), considering the selectivity-driven, region-specific size distributions.
● RQ 4: Is it possible to establish levels of fishing mortality (F) that will result in economic viability?
● RQ 5: Can regulating gear mesh sizes achieve biological sustainability of a mesopelagic fishery?
2 Materials and methods
2.1 The DISPLACE bio-economic model
The DISPLACE model (Bastardie et al., 2014) integrates the decision-making processes of the fishers when stock conditions, economic viability, or fisheries management changes, allowing to simultaneously evaluate the economic and ecological sustainability of a fishery. The model combines an individual based model (IBM) for fishing vessels that cover allocation of actual fishing effort and includes movements to or from a fishing area, between fishing areas, or rest at ports, with a spatially explicit, size-structured population model (SM Section 4). The movements of vessels between model nodes follows the shortest path between departure and destination areas, in this case between ports and fishing grounds and vice versa (Bastardie et al., 2014). A vessel depletes a stock at a node each time step t (hourly), according to the stock distribution, the catch rate of a specific vessel at a fishing ground, and the selectivity of the gear used. The core model is written in C++ and is openly available online (Bastardie, 2023).
2.2 Study area and delineation of populations
The model settings reflect the current Danish large-vessel pelagic fleet, which is the most likely Danish fleet segment to switch to a mesopelagic fishery in the future (Paoletti et al., 2021). We parametrized five spatially explicit regional mesopelagic populations: three populations of Maurolicus muelleri in (i) the Norwegian Sea (MA1.nor), (ii) the Icelandic waters and Irminger Sea (MA2.ice) and (iii) the Bay of Biscay (MA3.bob), and two populations of Benthosema glaciale in (iv) the Norwegian Sea (BH1.nor) and (v) the Icelandic waters and Irminger Sea (BH2.ice). The division into different populations is necessary to account for the differences in survey-specific density data from the different regions of the two species. In the further parametrization and the results, we refer to the abbreviated mesopelagic population names, and we use stocks or populations to refer to those five components. The populations are assigned to three boxes, with a fine-resolution grid of 30 by 30 km, while the area connecting the populations to each other and to land have a coarse 200 by 200 km resolution (Figure 1). The departure and landing harbors were selected where defined based on the landing history of the selected fishing vessels and according to distance to fishing grounds (See SM Section 2.1 for the list of harbor names).
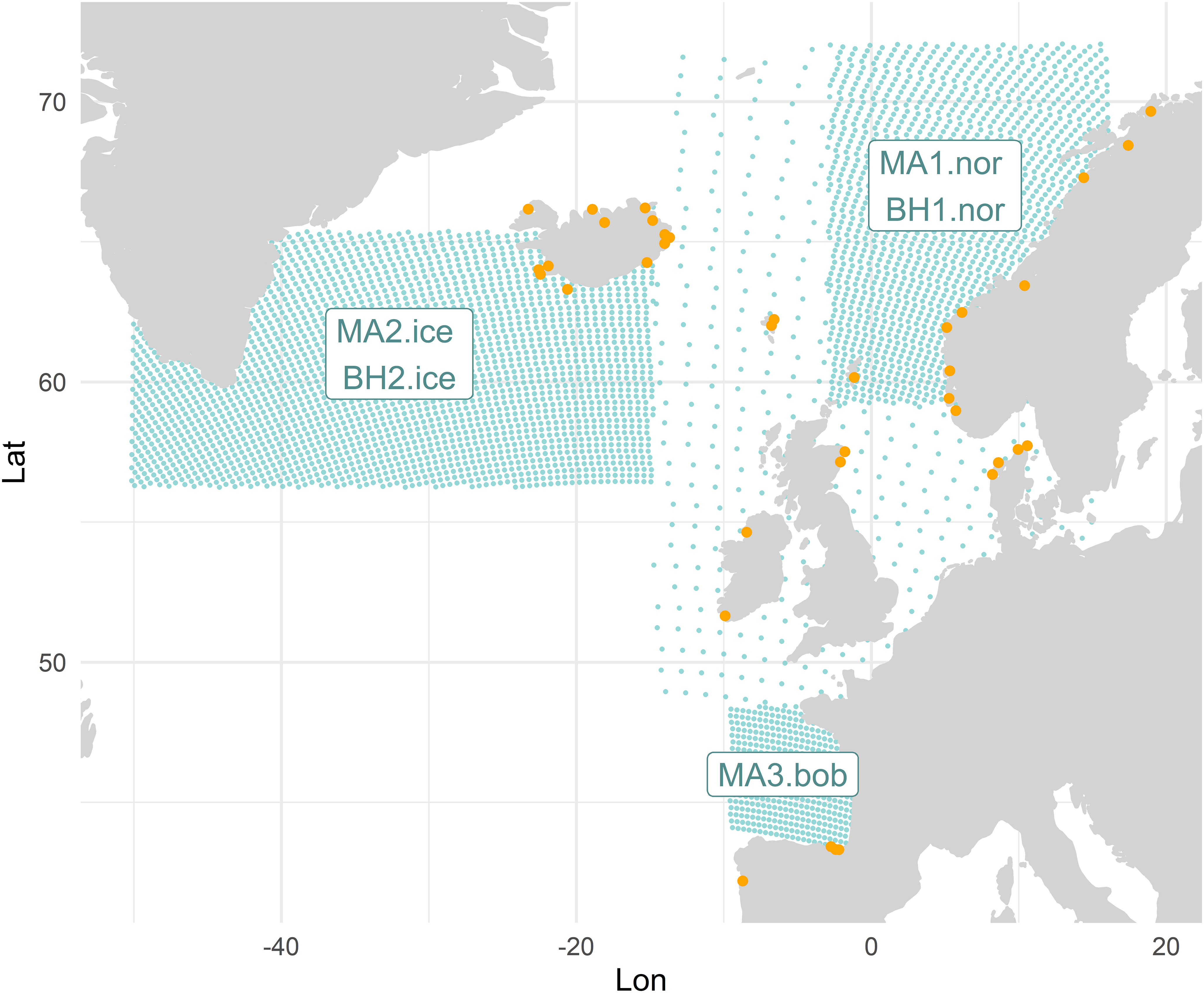
Figure 1 Map of the study area. Green points indicate the model nodes, the departure and landing harbors are indicated by the orange points.
2.3 Population dynamic parameters
The population dynamics model is length-based. On each node, there is a population with a length distribution informed by research survey data. The individual growth is applied each quarter according to a von Bertalaffy Growth Function (VBGF). A natural mortality rate is applied each month, and populations regenerate at the beginning of each year taking into account spawning biomass and recruitment to the stock. The spatial distribution of each stock is assumed to be fixed throughout the year.
Length distributions of the populations originate from length-frequency data from field campaigns of the EU-H2020 MEESO project (www.meeso.org) and other scientific surveys (SM Section 1.1). The data covers the North Atlantic region, from the Bay of Biscay in the South (43°N) up to Svalbard (82°N) and between 16°W and 35°E. The length distributions were binned into size groups of 0.5 cm and the allometric length-weight relationship parameters for each species are used to convert length to weight categories and vice versa (SM Table 5).
Population-specific growth parameters of the VBGF and natural mortality rates originate from (Vastenhoud et al., 2023) (SM Table 6). In the population dynamics model of DISPLACE, fish demography is modelled according to a transition matrix, and somatic growth is stochastic: the asymptotic length Linf is subsampled from a normal distribution with mean Linf and coefficient of variance (CV) of 0.2 cm. The von Bertalanffy growth coefficient K is randomly sampled from a normal distribution with mean K and a CV of 0.1 (year-1). The natural mortality rate M (year-1) is population-specific and constant for all size classes. The proportion mature at the midpoint of each size class follows a logistic curve where the probability of being mature is given by:
indicates the length at which 50% of the population is mature, while r indicates the maturation rate. For the mesopelagic populations r = 2 cm-1, to reflect the fast maturation of both species (Gjosaeter, 1981a; Salvanes and Stockley, 1996). The L50 of each species (3.9 cm for M. muelleri and 4.5 cm for B. glaciale) originated from previously reported literature (Gjosaeter, 1981a, 1981b).
We applied a Beverton-Holt stock-recruitment relationship between the spawning stock biomass (SSB) and recruitment (R) (Beverton, 1992), where recruitment is defined as the number of new fish available to the fishery in the next year. The Beverton-Holt stock-recruitment relationship is appropriate if there is a maximum abundance dependent on food availability or space, or if predators can adjust their predatory activity directly to changes in the abundance of prey (Wootton, 1990), and is therefore appropriate in the current case of small-sized mesopelagic species. The relationship assumes recruitment reaches an asymptote at high spawner abundance according to:
Fisheries management and the estimation of biological reference points and sustainability depends heavily on the stock-recruitment relationship, and in cases where there is insufficient data to directly estimate spawner-recruit relationships a common method is to use the steepness parameter h (Mangel et al., 2010). For the current case there is no information regarding spawner-recruit relationships for any of the species, and we estimated and using the steepness parameter h, life-history parameters related to growth, mortality and maturity from the FishLife package (Thorson, 2020), and the total biomass of the regional populations of each species (Mangel et al., 2010) (See SM Section 1.3). An overview of the stock-recruitment parameters used in this study can be found in SM Table 2.
2.4 Mesopelagic abundance estimates
Estimates of relative abundance per km2 for each stock originate from a literature review (SM Section 1.2). We define the stock abundance available to the fishery as the product of the area size of each region where the depth is greater than 100m (Norwegian Sea, Icelandic waters and Irminger Sea and Bay of Biscay, in km2) and the densities presented in SM Table 1. To obtain the size of the areas in km2 we extracted bathymetry data from the NOAA ETOPO 2022 database (NOAA National Centers for Environmental Information, 2022) on a 1 minute resolution using the ‘marmap’ package (Pante and Simon-Bouhet, 2013) in R (RStudio Team, 2020). The area in km2 was defined using the raster package (Hijmans and van Etten, 2012) in R.
A rough estimate of the total biomass in each region can be obtained by multiplying the total abundance of each population with the mean individual weight (M. muelleri: 1.9 g (Gjosaeter, 1986), B. glaciale: 2.1 g (Gjosaeter, 1973)) (Table 1).
2.5 Estimating population spatial distributions
We estimated spatial distributions of the populations using General Additive Models (GAMs) (Figure 2). See SM Section 1.4 for a detailed description of the spatial distribution modelling.
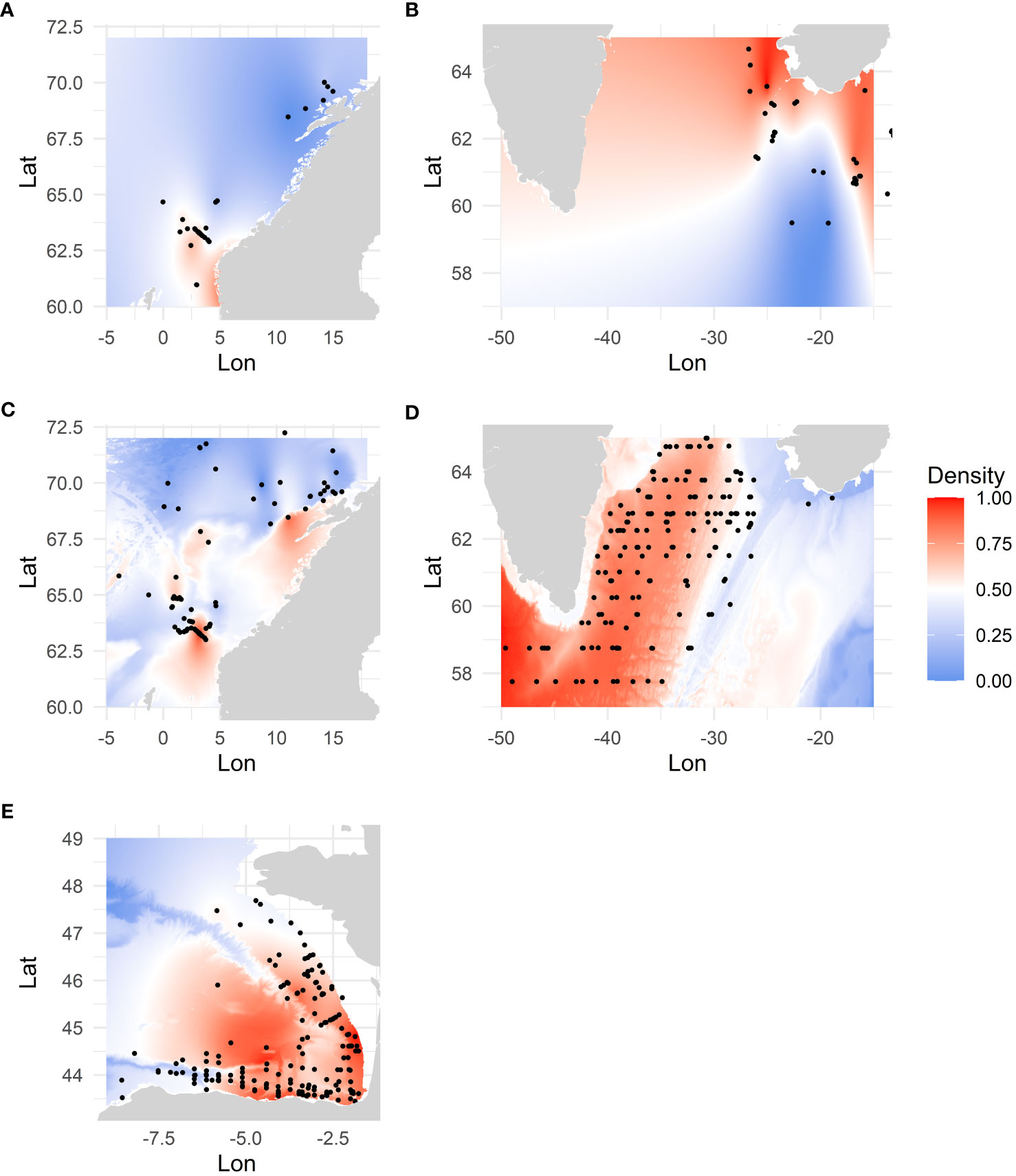
Figure 2 Estimated density probabilities resulting from the species distribution modelling for the different populations (A: MA1.nor, B: MA2.ice, C: BH1.nor, D: BH2.ice, E: MA3.bob). Grey points indicate the locations of the survey hauls used as response variable in the models.
2.6 Fishery dynamic parameters
We selected six large-scale Danish fishing vessels that participated in the blue whiting fishery from 2015 to 2019. These vessels were chosen due to their technological capacity to transition to an offshore, mesopelagic fishery (Paoletti et al., 2021). While five of these vessels are currently discontinued (Fiskeristyrelsen, 2023), we included them in our analysis to assess vessel-specific differences and variability if the entire blue whiting fishery were to switch to a mesopelagic fishery (See also SM Section 2.2). The selected vessels are equipped with refrigerated seawater (RSW) systems for conservation, which impose a maximum duration between the first catch and landing of 3-5 days (Paoletti et al., 2021). A plausible vessel-specific fuel tank size was provided by the Danish Pelagic Producers Organization (DPPO). Consequently, the fishing trips are limited to a maximum of 5 days after the first catch. The fishing speed is constrained to 2.3 knots according to (Grimaldo et al., 2022), while the steaming speed is set to 12.4 knots based on an analysis of vessel speed data from the actual Vessel Monitoring Systems (VMS) (See SM Section 2.3). We defined five population-specific métiers (fisheries), wherein the fishing arena of each métier corresponds to the distribution limits of the respective population (Figure 3). All vessels can participate in each métier. We assume catch rates of 400 tonnes per haul, corresponding to catch rates from the sandeel fishery, based on expectations of the Danish fishing industry (Paoletti et al., 2021).
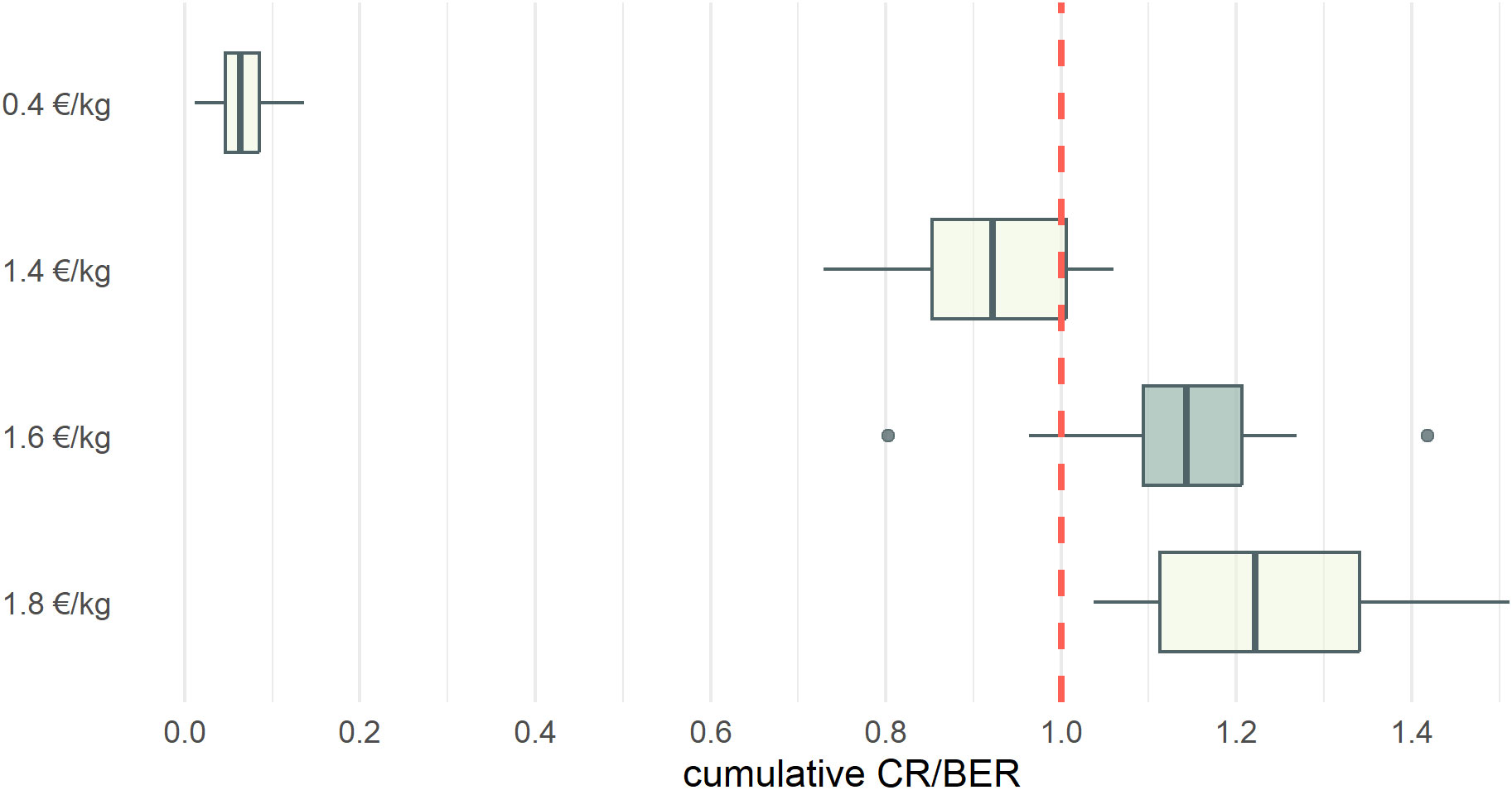
Figure 3 Boxplots of the revenue to break even revenue ratio (CR/BER) for the simulations according to different fish prices. The red dotted line indicates the level from which the fishery becomes economically viable in the short-term. The profitable baseline, used in the comparison with all further scenarios, is indicated in dark grey.
The economic parameters are for each vessel parametrized based on data from the EU Scientific, Technical and Economic Committee for Fisheries (STECF). We extracted aggregated data from the ‘DNK NAO TM 40XX NGI’ fleet segment for 2012 to 2018 (European Commission, Joint Research Centre and Scientific, Technical and Economic Committee for Fisheries, 2020). The selected data includes, but is not limited to, the six selected vessels described above. Details of the cost structure are described in SM Section 2.4.
2.7 Fish and fuel prices
The Danish pelagic fishing industry expects prices of mesopelagic resources to be similar to the prices of summer herring given the fat content (0.3-0.55 €/kg or 3.5-6 NOK/kg (Paoletti et al., 2021)), and we accordingly defined fish prices in all harbors at 0.4 €/kg for the baseline scenario. Fish meal and fish oil prices fluctuate according to the trends in the production of the products in Peru, which is correlated with the El Niño climatic oscillation (EU, 2021; Groeneveld et al., 2022). Generally, the prices for fish meal and fish oil are dependent on the species and volumes landed, and range between 0.19-0.28 €/kg for sprat and 0.38-0.73 €/kg for herring in Denmark (Groeneveld et al., 2022). The fuel price corresponds to the average marine gasoil price in Denmark in May 2023 (0.571 €/l, EUMOFA, (2023)).
2.8 Gear selectivity
A stretched mesh size of 10 mm has been suggested as most suitable for mesopelagic resources (Valinassab, 1998; Groeneveld et al., 2022). The mesopelagic populations are depleted according to a logistic selectivity ogive, reflecting a trawl-type selectivity pattern. The selectivity parameter estimates indicating the length at which an individual has respectively 50% or 75% chance to be caught originate from estimates from (Vastenhoud et al., 2023) ( and ).
2.9 Potential depletion from other countries
To make a realistic assumption regarding the population depletion from other countries, we first simulated a ‘no depletion’ scenario without depletion from other countries. We then estimated the Maximum Sustainable Yield (MSY) for the different populations (SM Section 3). We assumed that the difference between MSY and the catch from the Danish mesopelagic fleet corresponds to the total mesopelagic catch from the other fishing countries. Those ‘residual’ catches are proportionally assigned to other countries according to their distance to the different fishing grounds, and used in all further analyses (SM Table 11).
2.10 Scenarios
We evaluated alternative scenarios to identify factors affecting the ecological sustainability and the economic viability of a potential mesopelagic fishery (Table 2). Each scenario is simulated 20 times over a 10-year period (2023-2033).
We first ran the baseline scenario, and subsequently increased fish prices until an economically viable fishery was reached. That scenario was then assigned as ‘Profitable baseline’, from which we compared the profitability to the current profitability of the different métiers, to identify switching opportunities (Section 3.1). We then investigated scenarios where we compared the profitable baseline with increasing abundances to investigate whether increasing densities could impact the viability of a Danish mesopelagic fishery (Section 3.2). We also investigated an increase in fixed costs with 50%, to reflect the investment in new fishing vessels, gear and conservation methods (Paoletti et al., 2021), and increases in variable costs of 50% due to the increase of fuel use related to the large and small-meshed mesopelagic fishing nets (Paoletti et al., 2021) and an increase in fuel price of 50% (Section 3.3). For each of the scenarios we identified the increase or decrease in fish price necessary to cover the cost increase. Regarding the management strategies we investigated a range of fishing mortalities, and the effect of gear restrictions with a multiplier on the gear selectivity curve parameters (Section 3.4).
3 Results
Using a fish price of 0.4 €/kg, which corresponds to the expected price paid for mesopelagic resources (see section 2.7), the costs related to the fishery are too high for the fishery to be viable. Scenarios with increasing fish prices indicated that the fishery becomes profitable with a fish price of 1.6 €/kg, according to the Current Revenue to Break-Even Revenue ratio (CR/BER) (Figure 3, Table 3). This economic indicator shows the short-term profitability of the fishery considering both fixed and variable costs. If the ratio is greater than 1 then the fishery is economically viable in the short term, as there is sufficient cash flow generated to cover the costs, while a ratio below 1 indicates that the fishery is not viable in the short and mid-term. The formulae for the calculation of CR/BER, the gross value added (GVA) and other economic indicators can be found in SM Section 4 (SM Table 13). Accordingly, we defined the scenario with a fish price of 1.6 €/kg as the ‘Profitable baseline’. All subsequent scenarios were compared with the profitable baseline, and effects were defined as ‘large positive effect’ with an increase of > 25% relative to the profitable baseline, a ‘small positive effect’ with an increase of 5-25%, ‘no real effect’ when the difference between scenarios was between -5 - 5%, a ‘small negative effect’ with a decrease of 5-25% and a ‘large negative effect’ with a decrease of < 25%.
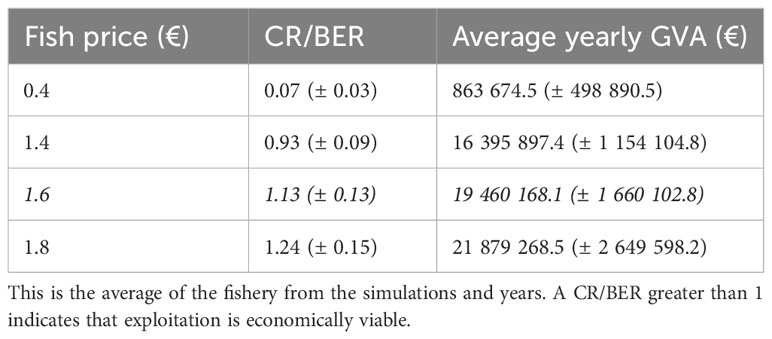
Table 3 The mean and standard deviation of the revenue to break even revenue ratio (CR/BER) and the average yearly gross value added (GVA) for the simulations according to different fish price scenarios.
3.1 Switching opportunities from current activities
To evaluate thresholds to switch from a current activity to a potential mesopelagic fishery, we compared the average yearly economic return (gross value added GVA) from the mesopelagic fishery to the average yearly profits estimated for the different pelagic fishing activities in (Paoletti et al., 2021). We compared the GVAs with the 5-year (2015-2019) averages of the 70-80m vessel size class (SM Section 6, based on the data from Supplementary Table 4 in Paoletti et al., 2021). According to a fish price of 0.4 €/kg, which is expected by the Danish pelagic fishery, a mesopelagic fishery would not be viable under current circumstances. However, the average yearly GVA (€ 835 404.1) of such fishery is higher compared to the OTM/PS Herring (industrial) métier. The average yearly profit (GVA) of an economically viable mesopelagic fishery (€ 19 460 168 with a fish price of 1.6 €/kg) is higher than the average yearly profits of the OTB Sandeel (€ 11 962 473), OTM/PTM Sprat (€ 3 766 162) and OTM/PS Herring (industrial, € 782 090) métiers. Switching from those métiers could be considered if the mesopelagic catches would generate fish prices of 1.6 €/kg. However, the average fish prices of those current industrial fisheries are significantly lower compared to the fish prices necessary for an economically viable fishery.
3.2 Abundance
Increased abundances mainly affected the landings indicators, but not for all populations and only with a small positive effect (Table 4, Figure 4). Only with a population abundance increase of 200% is there a positive effect of the CPUE at fishing (6.02% increase) and on the total landings (7.08% increase). This indicates that the fishery is mainly limited by effort: an increase in abundance leads to an increase in CPUE, but not enough to also generate a positive impact on the economic indicators. This should also be seen in context of the general low densities of the two species in relation to exploitation given current state-of-the-art abundance estimates in literature and commercial trial fisheries. The main limiting factor regarding the fishing trips is the size of the fuel tank, and to a lesser extent the storage capacity (SM Figure 11).
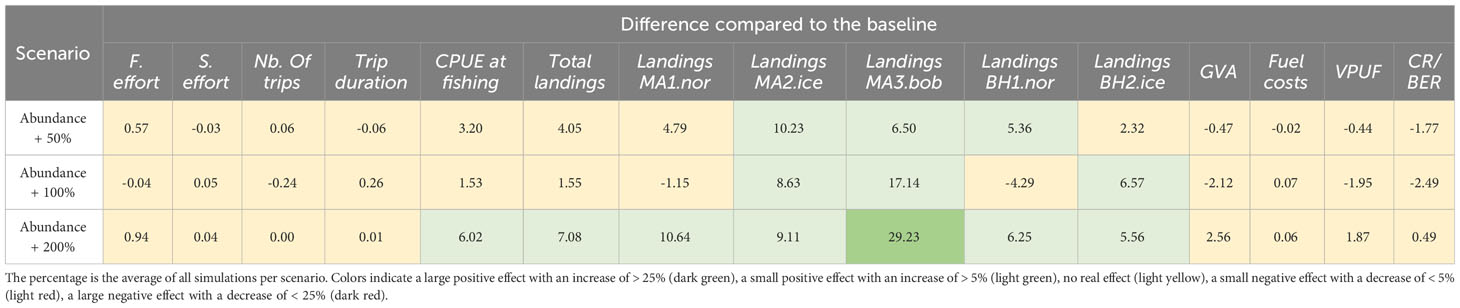
Table 4 The percentage difference compared to the profitable baseline for each of the abundance scenarios.
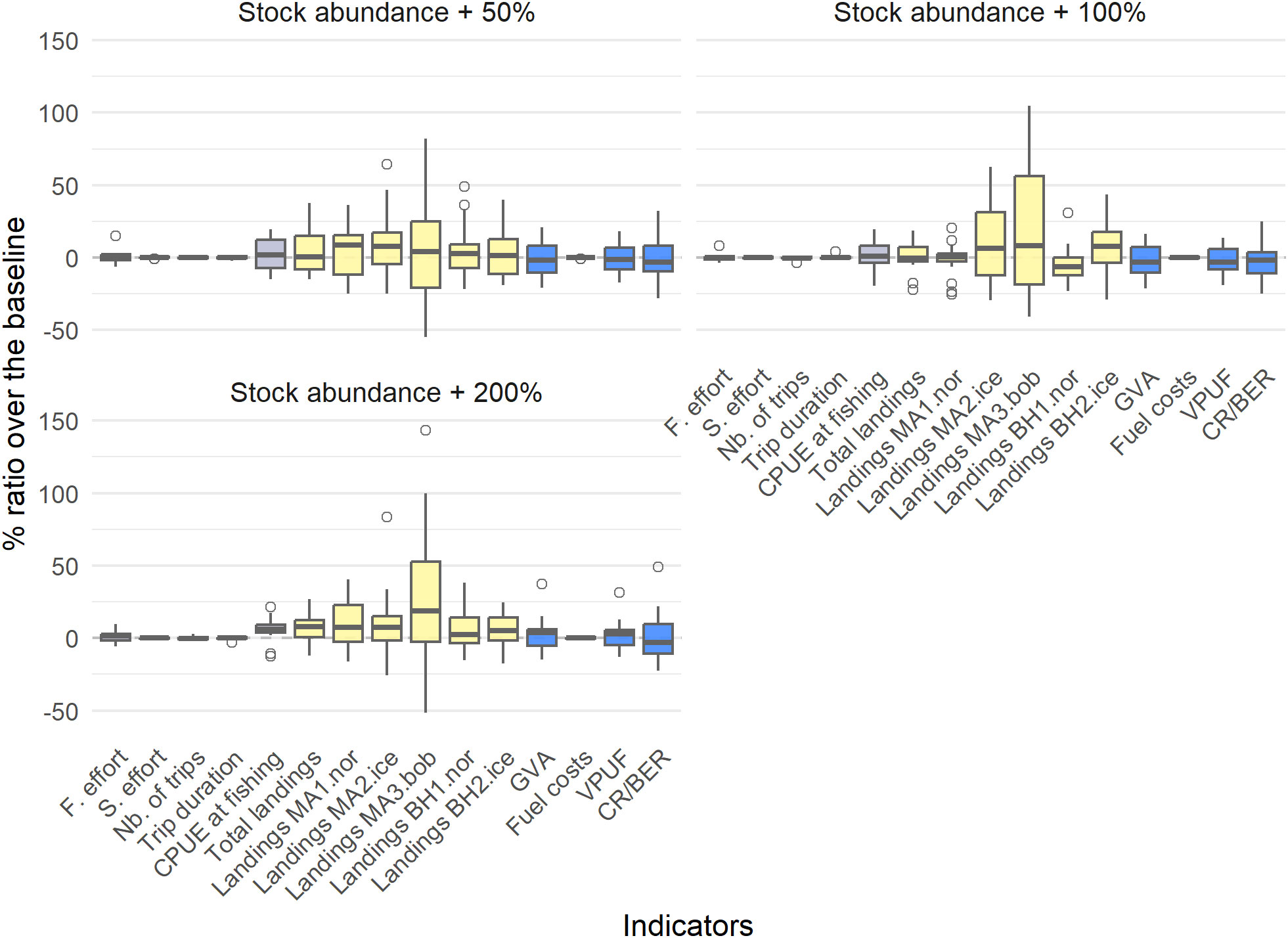
Figure 4 The percentage difference of the abundance scenarios compared to the profitable baseline, with boxplots indicating the different simulations. Grey indicates trip-related indicators, yellow indicates biological indicators and blue indicates economic indicators.
3.3 Fishing costs (fixed & variable) and fuel price
Increasing the fixed costs, in this case the vessel value, by 50% did not result in a significant impact on most of the fishery dynamics nor in an impact on the catch efficiency or landings (Figure 5, Table 5). However, the increase greatly impacted the CR/BER (32.59% decrease). This indicates that, as the fishing dynamics do not adapt to such investment costs, a larger revenue from catches is necessary to break even to repay the investments in new vessels or the switch from other fisheries. We also identified large impacts of fuel consumption on the fishery dynamics, while an increase in fuel price only impacted the costs related to fuel but did not change the dynamics. An increase in fuel consumption of 50% results in a large negative effect on fishing effort (30.63% decrease) and all indicators related to catches and landings. This results in a reduction of the GVA of 85.32%, and a reduction in CR/BER of 89.41%. The main reason for such a negative impact of more fuel consumed per hour is the necessity to return to port due to reaching the vessels fuel tank capacity (SM Section 5.2). Increasing the fuel price has a lesser impact on the different indicators: it has a small negative effect on the GVA (9.37% decrease) and on the CR/BER (12.24% decrease) and causes a large increase in fuel costs (50.09% increase).
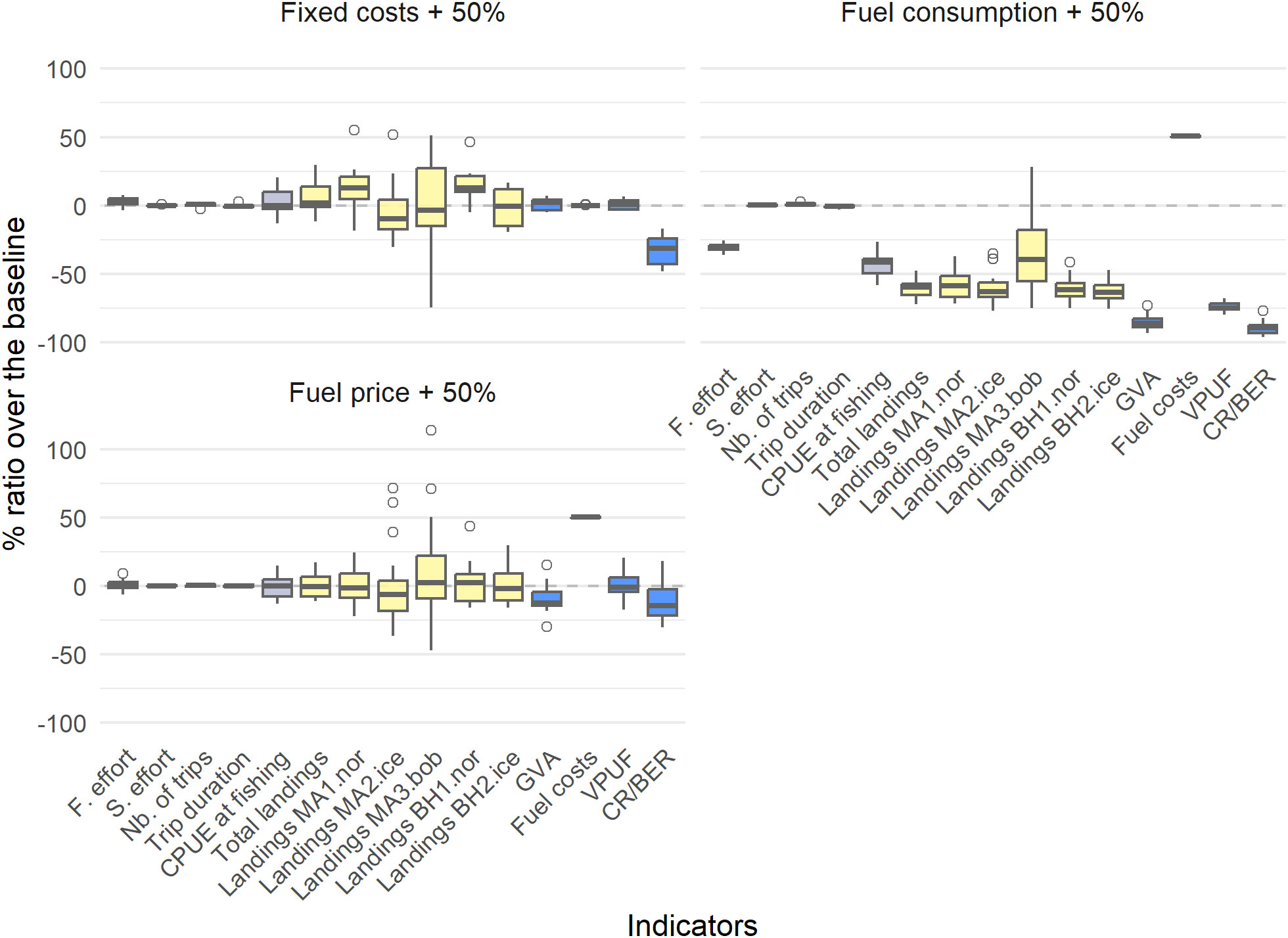
Figure 5 The percentage difference of the cost (fixed and fuel consumption) and fuel price scenarios compared to the profitable baseline, with boxplots indicating the different simulations. Grey indicates trip-related indicators, yellow indicates biological indicators and blue indicates economic indicators.
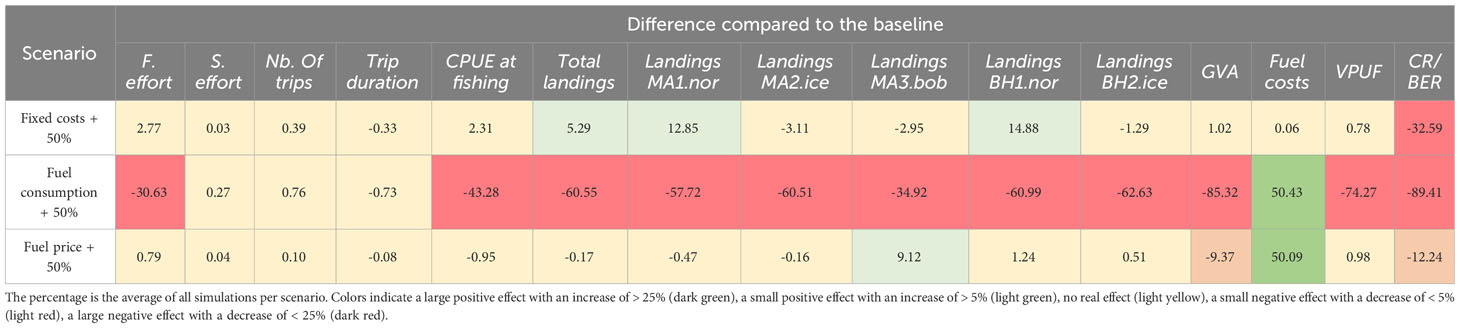
Table 5 The percentage difference compared to the profitable baseline for each of the cost (fixed and fuel consumption) and fuel price scenarios.
While for an economically viable fishery considering an increase of fixed costs of 50%, a fish price of 2.6 €/kg is necessary, an increase in fuel consumption of 50% requires fish price of 5.0 €/kg or higher. An increase in fuel price of 50% requires fish prices of 1.8 €/kg (Figure 6).
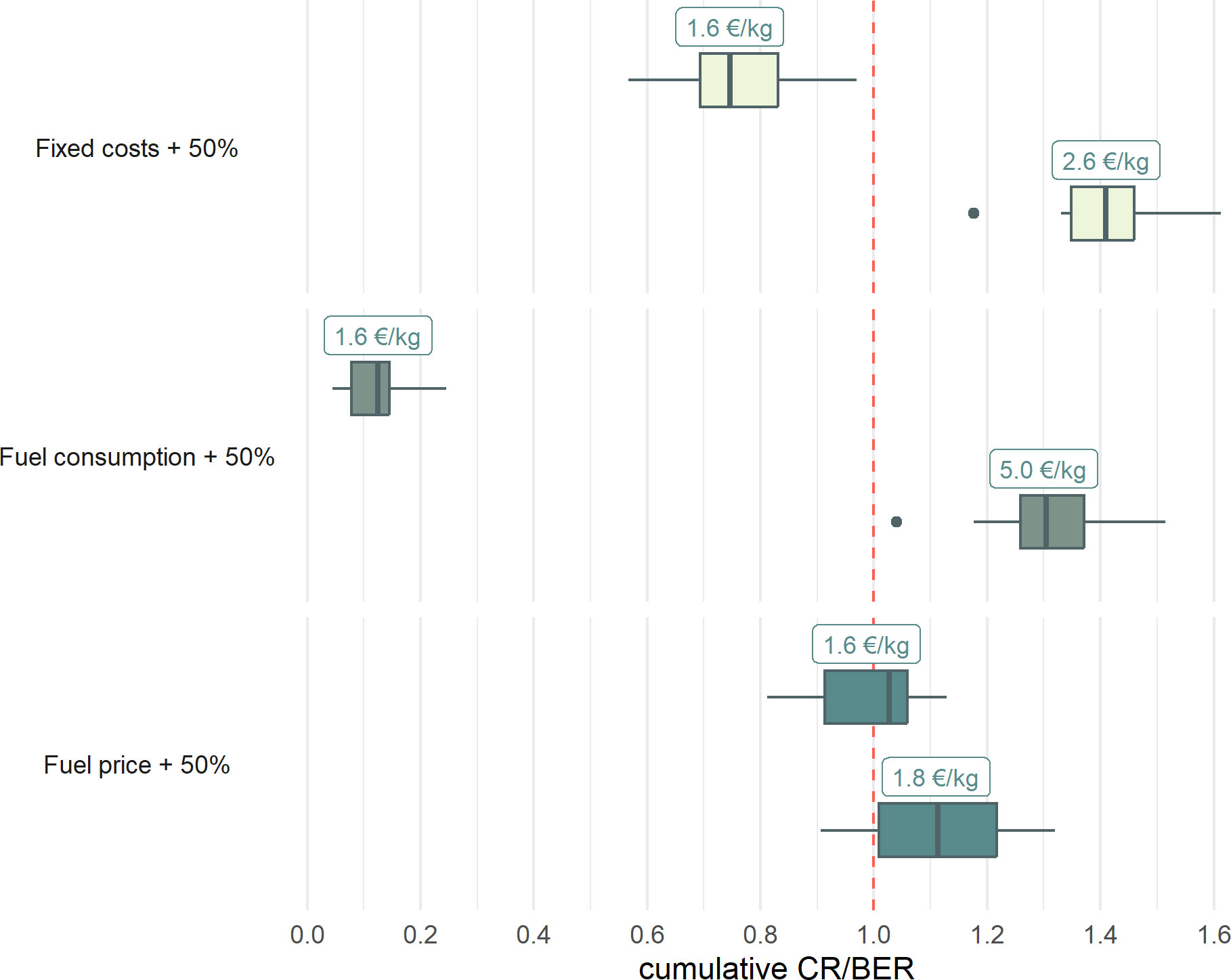
Figure 6 Box plots of the CR/BER ratio of the cost increase scenarios, with the red dotted line indicating the level from which the fishery becomes economically viable in the short-term. For each scenario the top boxplot shows the CR/BER ratio with a fish price of 1.6 €/kg, the bottom boxplots show the CR/BER for the fish price from which the cost increase scenario becomes viable.
3.4 Management strategies
3.4.1 Fishing mortality
Varying levels of fishing mortality between 0.2 – 1.0 (year-1) generally resulted in a reduction of catch efficiency (CPUE at fishing) and landings, but in a small increase in the CR/BER ratio compared to the profitable baseline (Table 6, Figure 7). A fishing mortality of F = 0.2 resulted in the highest profitability, with an increase of 16.62% compared to the profitable baseline. This fishing mortality also resulted in the highest GVA (15.32% increase compared to the baseline). Increasing the levels of fishing mortality above F = 0.6 results in decreasing catch efficiency (14.88% decrease for F = 0.8 and 16.64% decrease for F = 1.0) for small increases in the profitability of the fishery (CR/BER increase of 7.02% for F = 0.8 and 8.07% for F = 1.0).
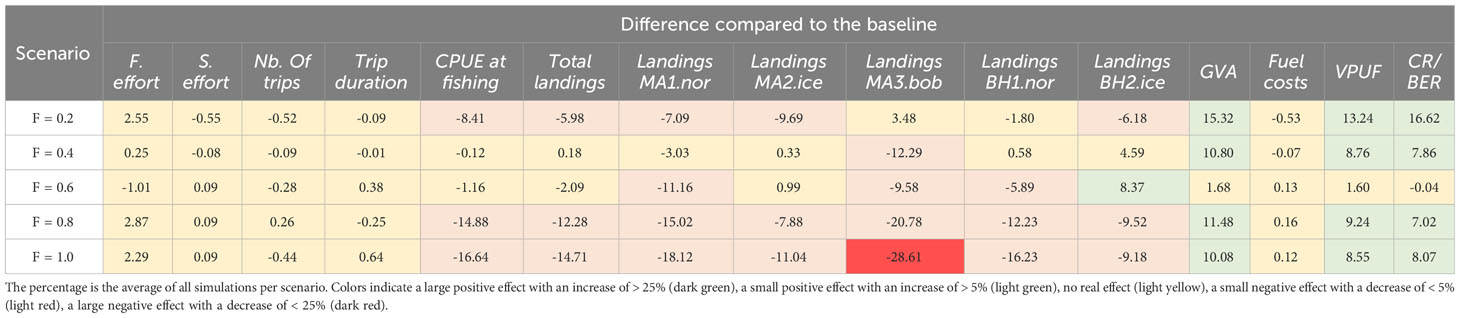
Table 6 The percentage difference compared to the profitable baseline for each of the fishing mortality scenarios and according to potential harvest rules.
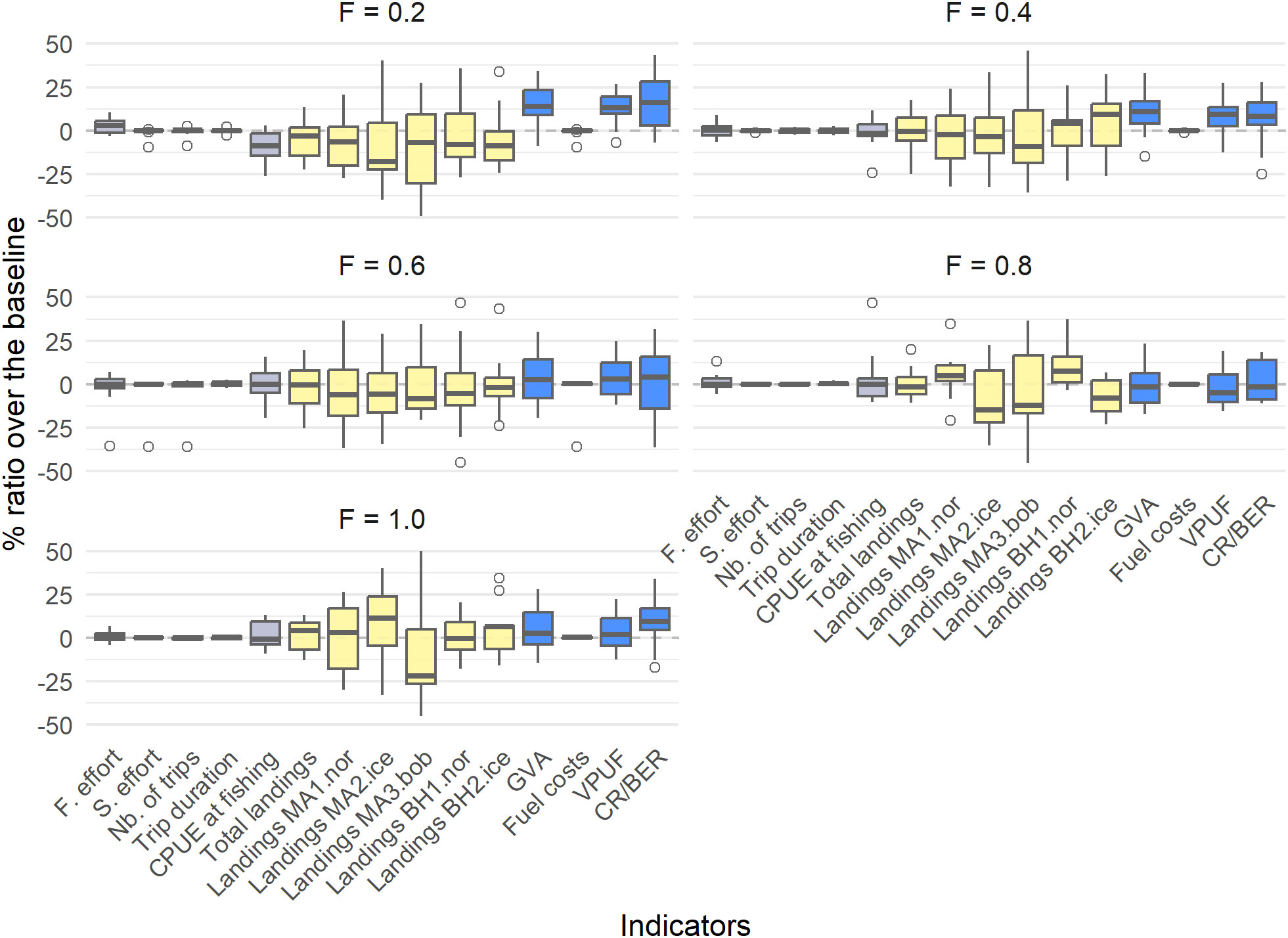
Figure 7 The percentage difference of the fishing mortality scenarios compared to the profitable baseline, with boxplots indicating the different simulations. Grey indicates trip-related indicators, yellow indicates biological indicators and blue indicates economic indicators.
3.4.2 Gear selectivity
Increasing gear selectivity parameters L50 and L75 had a small positive effect on the CR/BER ratio (5.63% increase) when increasing the selectivity parameters with 10%, e.g. increased trawl mesh size, together with increases in landings of M. muelleri and B. glaciale in Iceland (9.99% and 6.41% increase, respectively) (Figure 8, Table 7). Further increases in the mesh sizes did not have a large positive or negative effect on the fisheries dynamics or on the economic indicators (see the definition of effect thresholds at the beginning of this section). With an increase in the selectivity parameters of 20-30%, there was a decrease of 9.03% in the landings of M. muelleri in the Bay of Biscay.
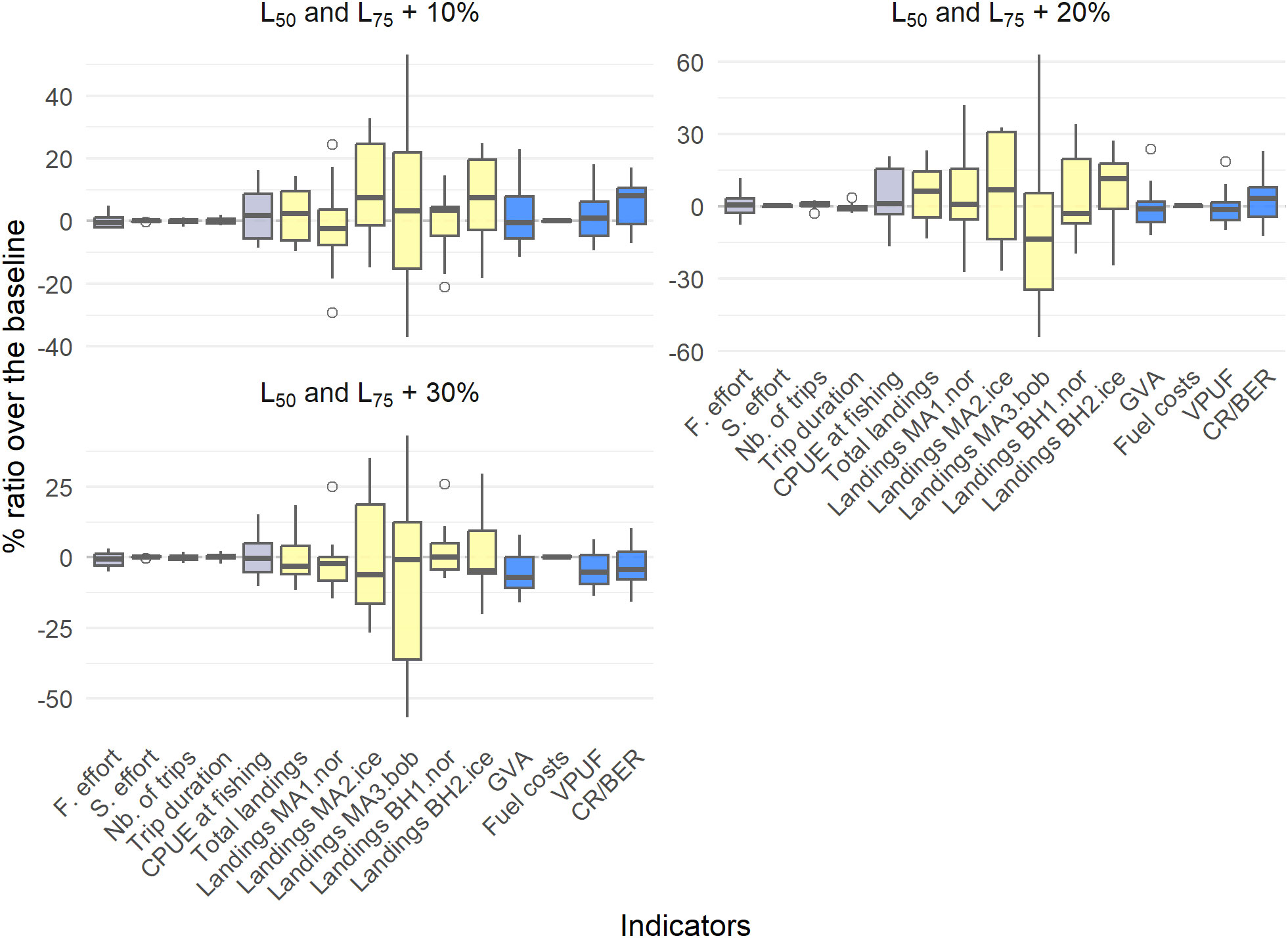
Figure 8 The percentage difference of the selectivity scenarios compared to the profitable baseline, with boxplots indicating the different simulations. Grey indicates trip-related indicators, yellow indicates biological indicators and blue indicates economic indicators.
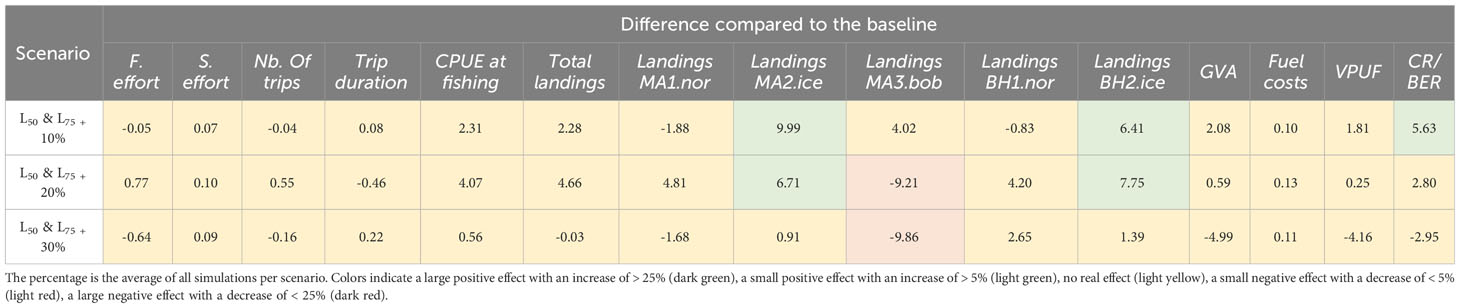
Table 7 The percentage difference compared to the profitable baseline for each of the selectivity scenarios.
4 Discussion
In this study we evaluated the effect of ecological and economic variability on the economic viability of a potential mesopelagic fishery targeting M. muelleri and B. glaciale in the Northeast Atlantic Ocean. We identified thresholds for switching—or potentially increasing capacity with new vessels—of the current large-scale Danish pelagic fleet from their existing activities to a potential mesopelagic fishery.
We found that fish prices of at least 1.6 €/kg are necessary for a mesopelagic fishery to be viable in the case of the Danish large-vessel pelagic fleet. In parallel, the mesopelagic fish abundance up to 200% compared to the current baseline slightly increases the viability of the fishery due to higher catch efficiency. The mesopelagic fish currently perceived abundances are therefore insufficient for an economically viable fishery with a baseline fish price of 0.4 €/kg. Investment into a new fishing vessel to exploit the mesopelagic resource (reflected in our simulations by increasing the vessel value by 50%), would require a minimum fish price of €2.6 to assure economic viability, while an increase in fuel consumption during fishing and fuel prices with 50% requires mesopelagic fish price to increase to €5.0 and €1.8, respectively.
Overall, the profitability of a mesopelagic fishery with the expected fish price of 0.4 €/kg is low compared to the existing fisheries that the Danish large-vessel fleet is involved in. However, if fish prices can be increased to 1.6 €/kg, the threshold required for an economically viable mesopelagic fishery, the average yearly profit of such economically viable mesopelagic fishery is higher than the average yearly profits of the sandeel, sprat and industrial herring fisheries. In that case those fisheries would be candidate fisheries to switch from. However, the highest reported average fish price of target species of the métier is 1.01 €/kg (for Atlantic mackerel), and it is unlikely that a potential mesopelagic fishery would generate higher fish prices than mackerel.
In terms of management strategies, we found that varying the levels of fishing mortality indicated a threshold of F = 0.2 year-1 to result in a viable fishery, which also generates the highest profitability compared to other scenario with increasing fishing mortality. We did not identify large overall effects of varying mesh sizes and selectivity on the profitability of the fishery, but the effects on the landings from the specific populations varied, where increases in mesh sizes led to a small increase followed by a small decrease in landings of M. muelleri in certain regions.
A previous study investigating the potential viability of a mesopelagic fishery performed by the Danish large-vessel pelagic fleet indicated maximum profits per trip between 1.2-1.6 million € per trip of 3-5 days and with a maximum on-board storage capacity of 3000 tonnes (Paoletti et al., 2021). The storage capacity can be increased when building new vessels, which could result in larger profits. In this study, however, the storage capacity was not the limiting factor but rather the fuel tank capacity as a result from the distant fishing grounds. In the case of the Spanish fleet an economically viable fishery was possible, but only for a narrow price-cost margin (Prellezo, 2019). A break-even point of 450 €/tonnes (0.45 €/kg) was required utilizing the full storage capacity (50 tonnes), up to 700 €/tonnes (0.7 €/kg) with the maximum reported price. In the latter case the catch per trip can be lower (32 tonnes), which might be more realistic for the vessels considered (Prellezo, 2019). This study also indicated that this mesopelagic fishery would not be viable if the fuel costs were doubled, which corresponds with the findings of the current study where both an increase in fuel price and fuel consumption resulted in a strong negative impact on the vessel economics.
A larger scale study investigating the viability of a mesopelagic fishery also considering the connection with fisheries on epipelagic species indicated a small window of viability under the condition of high fish prices and low costs related to the mesopelagic fishery (Kourantidou and Jin, 2022). Under the conditions of this study a potential mesopelagic fishery would be viable from a price of 2 $/kg (1.84 €/kg), indicating that a higher price is required than what would be expected according to an industrial fishery with similar oil contents, which corresponds to our results where different profitability scenarios required fish prices ranging between €1.6-5.0.
4.1 Assumptions, limitations and future improvements
The DISPLACE model works with high spatial-temporal resolution and high level of detail for both the dynamics of the fisheries and the harvested stocks. Since we are considering an extremely data-limited situation we have made assumptions that can be explored within DISPLACE which allowed us to consider large-scale patterns and drivers of the potential mesopelagic fishery performed by suitable Danish large pelagic vessels.
4.1.1 Ecological assumptions
For M. muelleri and B. glaciale region and species-specific biomass, abundance and distribution estimates are limited. In the Mediterranean Sea, mesopelagic biomass estimates ranged between 0.10 and 1.08 tonnes/km2 (Clavel-Henry et al., 2020). Biomass estimates in two Norwegian fjords indicated an average of 11.2 tonnes/km3 of B. glaciale and 4.0 tonnes/km3 of M. muelleri, but with large differences in species composition between the fjords (Alvheim et al., 2020). Those estimates have a similar order of magnitude compared to the abundance estimates used in this study (see also Section 4.3.4). In the current study we do not consider distributions on a fine scale, nor do we consider seasonality in the distributional patterns, due to the absence of species distribution information of the species considered here. Seasonal distributions might be affected by the spawning of the species, or by seasonal distribution changes of predators such as juvenile albacore tuna (Thunnus alalunga) (Pusineri et al., 2005). Sensitivity studies regarding the size and distance of the distribution patches of both species indicated that those, as expected, have a large effect on the final profitability of the fishery, where increasing patch size and distance between patches resulted in a decrease in the overall profitability of the fishery. The concentration of mesopelagic biomass into hotspots could be related to concentration by currents or advective processes (Benitez-Nelson et al., 2007; Sutton et al., 2008; Godø et al., 2012; Gjeitsund Thorvaldsen et al., 2023). Illuminating the drivers of those processes and understanding the schooling behaviour of the species is a crucial future step and necessary for making the distinction between density concentrations and overall abundance of the species (Hidalgo and Browman, 2019; Grimaldo et al., 2020). To further determine key gaps in the ecological assumptions in the evaluation of the economic viability of a mesopelagic fishery we conducted sensitivity studies regarding the life-history parameters (see SM Section 5.1). Especially the natural mortality M had a high impact on the estimates of profitability of the fishery, and the growth parameters K and Linf to a lesser extent. It is therefore important to gain more insight into the species-specific distributions and spatio-temporal abundances and their drivers as well as in the population-specific life-history parameters in the evaluation of both the ecological sustainability and the economic viability of a potential mesopelagic fishery.
In our study, the stock recruitment relationship is not based on measurements of recruitment, but on identified life-history parameters and total stock biomass. That is because we do not currently have an appropriate estimate of recruitment for the species under investigation. Using this method, the parameters of the stock-recruitment relationship are mainly dependent on M, Linf and h (SM Section 1.3). Improved understanding of the drivers of this relationship requires long-term demographic studies of the target species of interest, preferably combined with experimental studies of the quantitative effects of abiotic and biotic factors on the life-history of the species (Wootton, 1990). Especially M. muelleri is a short-lived species, and similar to other short-lived species, its life-history characteristics, including large inter-annual variations in recruitment, might pose significant challenges to its management (ICES, 2019b). While there is to date no information available for the appropriate parametrization of these dynamics in the DISPLACE model, in the short term this might be captured by increasing the coefficient of stochastic variation of the stock-recruitment relationship.
4.1.2 Fishery dynamics assumptions
Also the fishery dynamics were parametrized according to the currently available information, which did not allow for a high level of detail. While we here focused on evaluating fishery dynamics, economy and switching opportunities by the Danish large vessel fleet on an annual scale, it is important to note that these opportunities may vary seasonally. While sprat and herring are harvested throughout the year, other pelagic fisheries show distinct seasonality (Paoletti et al., 2021). For example, blue whiting and horse mackerel are predominantly targeted during the first quarter, sandeel fishing is mainly conducted in the second quarter, and Atlantic mackerel and Norway pout are primarily targeted in the fourth quarter of the year. The mesopelagic fishery conducted by other countries may also exhibit seasonal variability (Prellezo, 2019). We, however, assume a fixed annual fraction is depleted by other countries. We also assume that the catches from other countries are divided equally to all other countries, but it is possible that there is variability in this where the country closest to the population will take a larger share. This also might result in a reduction of the current share taken by the Danish fishery in our evaluations.
To account for the vertically migrating behaviour of the species, the Danish pelagic fishing industry expects a fishing pattern consisting of two long tows per 24h period, where heaving and setting takes place during dawn and dusk while the fish are migrating (Paoletti et al., 2021). Therefore the fuel consumption could differ between the haul during the night in shallower water layers compared to hauls taking place during the day, potentially affecting the fishing behaviour and associated costs. Further, there is currently a maximum fishing trip limit of 3-5 days between the initial catch of mesopelagic species and landings due to the rapid degradation of the species (Paoletti et al., 2021). To extend trip durations, various on-board conservation methods such as freezing, silage production, or thermic separation could be implemented (Paoletti et al., 2021). While we accounted for the possible investment costs associated with the installation of those new conservation methods by increasing the fixed costs of the fishery, it remains uncertain what the specific investment costs will be.
Also regarding the cost structure of the vessels there is uncertainty. In this study the cost structure of the fishing vessels analyzed is based on data from EU Data Collection Framework (EU DCF) analyzed by the STECF every year (STECF Annual Economic Report database (European Commission, Joint Research Centre and Scientific, Technical and Economic Committee for Fisheries)). The data is aggregated according to fleet segment, encompassing a broader range of vessels beyond the ones analyzed. Within the selected fleet segment, which includes pelagic vessels larger than 40m, the number of vessels varied between 11 and 22 during the period from 2013 to 2018, while the minimum vessel size considered in this study is 69.95m. The larger vessels in this segment generally incur higher fixed and variable costs, though they also tend to have higher profitability per trip due to their extended trip durations and larger storage capacity. On the other hand, more recently built pelagic fishing vessels might be more energy-efficient, thereby reducing the environmental impact per kilo of catch and reducing the fuel limitation of the potential mesopelagic fishery (SM Section 2.2). It is important to acknowledge that various other socio-economic uncertainties influence the cost structure and fishing patterns of these vessels. For example, the implications of Brexit will impact fishing rights, quota trades between countries, and the distribution of fisheries. Additionally, fluctuations in energy prices have a significant impact on the energy-intensive potential of the mesopelagic fishery (Pelletier et al., 2014). It is also uncertain how many fishing vessels would finally enter the potential mesopelagic harvest, and how that would reflect in the average total cost of the fishery. Further, technological advancements, such as the introduction of herding mechanisms, might enhance the efficiency of the fishery even with low densities, thereby reducing operating costs (Grimaldo et al., 2020; Paoletti et al., 2021; Underwood et al., 2021).
4.1.3 Selectivity of fishing gears
The uncertainty in mesopelagic abundance estimates is indicative of the challenges of sampling the mesopelagic zone: trawl avoidance of mesopelagic fish might result in an underestimation of mesopelagic biomass when using trawl sampling (Kaartvedt et al., 2012), while the acoustic energy of acoustic biomass estimates in the mesopelagic zone is not solely due to backscattering of the swim bladders of fish but also from e.g. siphonophores (Proud et al., 2019). Those challenges also affect the selectivity of a potential mesopelagic fishery, and the catch efficiency and selectivity of mesopelagic trawls are also affected by the avoidance behavior and mesh selection (Grimaldo et al., 2022). Several studies have reported gear avoidance of mesopelagic species at towing speeds of 2-3 knots, which is suggested as one of the main factors of the lower trawl-based estimates of mesopelagic biomass (Gjosaeter and Kawaguchi, 1980; Kaartvedt et al., 2012; Davison et al., 2015; Underwood et al., 2020; Grimaldo et al., 2022). Fish avoidance can be reduced by increasing the towing speed, which however also increases drag resistance and associated costs related to fuel. We accounted for the increase in fuel consumption due to the drag resistance in one of the scenarios, however the final fuel consumption will be dependent on the new gear designed to target the mesopelagic species.
4.2 Management of a mesopelagic fishery
While the United States has banned commercial fisheries targeting species in the mesopelagic zone in the Pacific Ocean (PFMC, 2016), the Blue Growth Strategy from the European Union (EU) is open to the exploration of new marine resources such as mesopelagic species. To date there is, however, no management framework in place to regulate mesopelagic resource exploitation in the Northeast Atlantic (Standal and Grimaldo, 2020). Given the complex role of mesopelagic species in the marine food web and the potential bycatch of other commercial species, an ecosystem-based management framework should be developed following a precautionary approach (UN, 1982; FAO, 1995; Salomon et al., 2014; ICES, 2019a; van der Meer et al., 2023).
Management strategy evaluation (MSE) allows for the evaluation of effectiveness of management strategies, in this case according to different harvest control rules (HCR) and gear restrictions. For a more thorough evaluation of management accurate stock assessments according to MSY are necessary. But although the body of scientific knowledge regarding the mesopelagic ecosystem is increasing, data availability remains a challenge in the evaluation of the sustainability of a potential mesopelagic fishery. Specifically, information on species and region-specific biomass densities and variability therein, population dynamic parameters and stock delineation is crucial, and the current knowledge only allows for the assessment of ranges in variability. Further, DISPLACE is a model shaped to consider multiple stocks and possible wasted resources (former “discards”) resulting from technical interactions: currently there is insufficient information in the parametrization of this to include bycatch considerations that would be dynamic. If this study provides an exploration of different management scenarios, in the next step all possible bycatch species should also be considered for a full and integrated management system evaluation (MSE) besides the MSY and PA sustainability for the target stocks. Mesopelagic trial fisheries showed variability in bycatch rates of other mesopelagic and commercial species such as krill Meganyctiphanes norvegica, Norway pout (Trisopterus esmarkii), cod (Gadus morhua) and saithe (Pollachius virens) (Marine Research Institute, 2015; Grimaldo et al., 2020; Standal and Grimaldo, 2020; Grimaldo et al., 2022). This variability may be extensive and dependent on target-species and area (Staby and Aksnes, 2011; Fock et al., 2019; Grimaldo et al., 2020)). Through their diel vertical migrations, mesopelagic species contribute both to the transport of nutrients to deeper waters as well as to the transfer of energy up the food chain (Costello and Breyer, 2017). Optimally, a management framework should also consider the potentially important role of the mesopelagic species in the sequestration of carbon to the deep ocean, as part of the biological carbon pump (BCP).
4.3 Conclusion
The current uncertainties, especially regarding the life-history, species-specific and spatio-temporal abundance estimates indicate that caution should be taken in the case of a mesopelagic fishery and investments therein. This study shows that a potential mesopelagic fishery performed by the case of the Danish large-vessel pelagic fleet would only be viable with fish prices above 1.6 €/kg for mesopelagic resources, which is much higher compared to what has been observed and expected to date for species with similar fat contents. Due to the large distances to offshore fishing areas for the Danish fleet, the fuel tank capacity and the current fuel costs are the main limiting factors of this potential fishery. A first evaluation of preliminary harvest control strategies indicated that a low fishing mortality of F = 0.2 year-1 resulted in the highest profitability of the fishery. Restriction of gear mesh sizes did not, initially, result in significant positive or negative effects on the species considered. As we do not have the information to specifically evaluate scenarios of bycatch, larger mesh sizes should therefore be considered for a potential mesopelagic fishery in order to minimize impacts on potential bycatch species.
Data availability statement
The data analyzed in this study is subject to the following licenses/restrictions: The data underlying this article will be shared on reasonable request to the corresponding author. Requests to access these datasets should be directed to Berthe M. J. Vastenhoud,Ym1qdkBhcXVhLmR0dS5kaw==.
Author contributions
BV: Conceptualization, Data curation, Formal analysis, Investigation, Methodology, Software, Validation, Visualization, Writing – original draft, Writing – review & editing. FB: Conceptualization, Data curation, Formal analysis, Investigation, Methodology, Software, Validation, Visualization, Writing – original draft, Writing – review & editing. KA: Formal analysis, Investigation, Methodology, Software, Validation, Writing – review & editing. DS: Formal analysis, Investigation, Methodology, Software, Validation, Writing – review & editing. JN: Conceptualization, Formal analysis, Funding acquisition, Investigation, Methodology, Software, Validation, Writing – original draft, Writing – review & editing.
Funding
The author(s) declare financial support was received for the research, authorship, and/or publication of this article. This study was financed by the EU H2020 MEESO Project “Ecologically and Economically Sustainable Mesopelagic Fisheries” Grant Agreement No. 817669.
Acknowledgments
We express our gratitude to the contributors of biological data within the H2020 MEESO project. Special thanks to Paula Alvarez, Dorleta Garcia and Guillermo Boyra (AZTI) for providing length-frequency data in the Bay of Biscay, and to Sigurður Þór Jónsson and Klara Jakobsdóttir (MFRI), as well as Thor Klevjer and Webjørn Melle (IMR), for their invaluable contributions in providing length-frequency data in Icelandic waters and the Irminger Sea, and the Norwegian Sea, respectively. The data and parameters, as detailed and published in Vastenhoud et al. (2023), were used in parameterizing the initial length distributions, determining growth, mortality, and gear selectivity parameters, and modeling spatial distributions in the DISPLACE model. We also thank Claus R. Sparrevohn and Emil Bundgaard Holm (DPPO) for their contribution to the vessel-specific parametrization of the fuel tank capacity, and Michael Gallagher (BIM) for the selection of Irish landing harbors.
Conflict of interest
The authors declare that the research was conducted in the absence of any commercial or financial relationships that could be construed as a potential conflict of interest.
The author(s) declared that they were an editorial board member of Frontiers, at the time of submission. This had no impact on the peer review process and the final decision.
Publisher’s note
All claims expressed in this article are solely those of the authors and do not necessarily represent those of their affiliated organizations, or those of the publisher, the editors and the reviewers. Any product that may be evaluated in this article, or claim that may be made by its manufacturer, is not guaranteed or endorsed by the publisher.
Supplementary material
The Supplementary Material for this article can be found online at: https://www.frontiersin.org/articles/10.3389/fmars.2023.1285793/full#supplementary-material
References
Abernethy K. E., Trebilcock P., Kebede B., Allison E. H., Abernethy N. K. D., Trebilcock K. E., et al. (2010). Fuelling the decline in UK fishing communities? ICES J. Mar. Sci. 67, 1076–1085. doi: 10.1093/ICESJMS/FSP289
Alvheim A. R., Kjellevold M., Strand E., Sanden M., Wiech M. (2020). Mesopelagic species and their potential contribution to food and feed security—a case study from Norway. Foods 9, 344. doi: 10.3390/foods9030344
Anderson C. I. H., Brierley A. S., Armstrong F. (2005). Spatio-temporal variability in the distribution of epi- and meso-pelagic acoustic backscatter in the Irminger Sea, North Atlantic, with implications for predation on Calanus finmarchicus. Mar. Biol. 146, 1177–1188. doi: 10.1007/s00227-004-1510-8
Anderson T. R., Martin A. P., Lampitt R. S., Trueman C. N., Henson S. A., Mayor D. J., et al. (2019). Quantifying carbon fluxes from primary production to mesopelagic fish using a simple food web model. ICES J. Mar. Sci. 76, 690–701. doi: 10.1093/icesjms/fsx234
Barange M., Butenschön M., Yool A., Beaumont N., Fernandes J. A., Martin A. P., et al. (2017). The cost of reducing the North Atlantic Ocean biological carbon pump. Front. Mar. Sci. 3. doi: 10.3389/FMARS.2016.00290
Bastardie F. (2023). DISPLACE. Available at: https://displace-project.org/.
Bastardie F., Angelini S., Bolognini L., Fuga F., Manfredi C., Martinelli M., et al. (2017). Spatial planning for fisheries in the Northern Adriatic: working toward viable and sustainable fishing. Ecosphere 8, e01696. doi: 10.1002/ECS2.1696
Bastardie F., Brown E. J., Andonegi E., Arthur R., Beukhof E., Depestele J., et al. (2021). A review characterizing 25 ecosystem challenges to be addressed by an ecosystem approach to fisheries management in Europe. Front. Mar. Sci. 7. doi: 10.3389/fmars.2020.629186
Bastardie F., Danto J., Rufener M. C., van Denderen D., Eigaard O. R., Dinesen G. E., et al. (2020). Reducing fisheries impacts on the seafloor: A bio-economic evaluation of policy strategies for improving sustainability in the Baltic Sea. Fish Res. 230, 105681. doi: 10.1016/J.FISHRES.2020.105681
Bastardie F., Nielsen J. R., Miethe T. (2014). DISPLACE: A dynamic, individual-based model for spatial fishing planning and effort displacement - integrating underlying fish population models. Can. J. Fish. Aquat. Sci. 71, 366–386. doi: 10.1139/cjfas-2013-0126
Benitez-Nelson C. R., Bidigare R. R., Dickey T. D., Landry M. R., Leonard C. L., Brown S. L., et al. (2007). Mesoscale eddies drive increased silica export in the subtropical Pacific Ocean. Sci. (1979) 316, 1017–1021. doi: 10.1126/science.1136221
Beverton R. J. H. (1992). Patterns of reproductive strategy parameters in some marine teleost fishes. J. Fish Biol. 41, 137–160. doi: 10.1111/j.1095-8649.1992.tb03875.x
Boehlert G. W., Wilson C. D., Mizun0 K. (1994). Populations of the sternoptychid fish Maurolicus muelleri on seamounts in the Central North Pacific! Pac. Sci. 48, 57–69.
Boyd P. W., Claustre H., Levy M., Siegel D. A., Weber T. (2019). Multi-faceted particle pumps drive carbon sequestration in the ocean. Nature 568, 327–335. doi: 10.1038/s41586-019-1098-2
Buesseler K. O., Boyd P. W. (2009). Shedding light on processes that control particle export and flux attenuation in the twilight zone of the open ocean. Limnol. Oceanogr. 54, 1210–1232. doi: 10.4319/lo.2009.54.4.1210
Cheilari A., Guillen J., Damalas D., Barbas T. (2013). Effects of the fuel price crisis on the energy efficiency and the economic performance of the European Union fishing fleets. Mar. Policy 40, 18–24. doi: 10.1016/J.MARPOL.2012.12.006
Clavel-Henry M., Piroddi C., Quattrocchi F., Macias D., Christensen V. (2020). Spatial distribution and abundance of mesopelagic fish biomass in the Mediterranean sea. Front. Mar. Sci. 7. doi: 10.3389/fmars.2020.573986
Costello M. J., Breyer S. (2017). Ocean depths: the mesopelagic and implications for global warming. Curr. Biol. 27, R36–R38. doi: 10.1016/J.CUB.2016.11.042
Davison P., Lara-Lopez A., Anthony Koslow J. (2015). Mesopelagic fish biomass in the southern California current ecosystem. Deep Sea Res. 2 Top. Stud. Oceanogr. 112, 129–142. doi: 10.1016/j.dsr2.2014.10.007
Dowd S., Chapman M., Koehn L. E., Hoagland P. (2022). The economic tradeoffs and ecological impacts associated with a potential mesopelagic fishery in the California Current. Ecol. Appl. 32, 1–17. doi: 10.1002/eap.2578
Dypvik E., Klevjer T. A., Kaartvedt S. (2012). Inverse vertical migration and feeding in glacier lanternfish (Benthosema glaciale). Mar. Biol. 159, 443–453. doi: 10.1007/s00227-011-1822-4
EU (2021). European Commission Directorate-General for Maritime Affairs and Fisheries, Fishmeal and fish oil: production and trade flows in the EU (Luxembourg: Publications Office of the European Union). doi: 10.2771/062233
EUMOFA (2023). Monthly marine gasoil prices. Available at: https://www.eumofa.eu/macroeconomic (Accessed June 6, 2023).
European Commission, Joint Research Centre, Scientific, Technical and Economic Committee for Fisheries, Carvalho N., Guillen J., Prellezo R. (2020). The 2020 annual economic report on the EU fishing fleet (STECF 20-06) (Publications Office). Available at: https://data.europa.eu/doi/10.2760/500525.
Fiskeristyrelsen (2023). Fiskeristyrelsen. Available at: https://fartoej.fiskeristyrelsen.dk/fdweb/app/vessel-find (Accessed April 14, 2023).
Fjeld K., Tiller R., Grimaldo E., Grimsmo L., Standal I. B. (2023). Mesopelagics–New gold rush or castle in the sky? Marine Policy, 147. doi: 10.1016/j.marpol.2022.105359
Fock H. O., Czudaj S., Bartolino V. (2019). Size structure changes of mesopelagic fishes and community biomass size spectra along a transect from the equator to the Bay of Biscay collected in 1966-1979 and 2014-2015. ICES J. Mar. Sci. 76, 755–770. doi: 10.1093/icesjms/fsy068
Gjeitsund Thorvaldsen K., Neuenfeldt S., Mariani P., Nielsen J. R. (2023). Hiding in plain sight: Predator avoidance behaviour of mesopelagic Maurolicus muelleri during foraging - in submission. Mar. Ecol. Prog. Ser. SPF2, SPF2av5. doi: 10.3354/meps14424
Gjosaeter J. (1973). Age, growth, and mortality of the myctophid fish, Benthosema glaciale (Reinhardt), from Western Norway. Sarsia 52, 1–14. doi: 10.1080/00364827.1973.10411227
Gjosaeter J. (1981a). Growth, production and reproduction of the myctophid fish Benthosema glaciale from western Norway and adjacent seas. Serie Havundersokelser - Fiskeridirektoratets Skrifter 17, 79–108.
Gjosaeter J. (1981b). Life history and ecology of Maurolicus muelleri (Gonostomatidae) in Norwegian waters. Serie Havundersokelser - Fiskeridirektoratets Skrifter 17, 109–131.
Gjosaeter J. (1986). Estimates of the abundance of mesopelagic fish off southern Norway and west of the British Isles 1971-1976. Flødevigen rapportserie 1, 1–22.
Gjosaeter J., Kawaguchi K. (1980). A Review Of The World Resources Of Mesopelagic Fish (Rome: FAO Fisher).
Godø O. R., Samuelsen A., Macaulay G. J., Patel R., Hjøllo S. S., Horne J., et al. (2012). Mesoscale eddies are oases for higher trophic marine life. PloS One 7, e30161. doi: 10.1371/journal.pone.0030161
Grimaldo E., Grimsmo L., Alvarez P., Herrmann B., Tveit G. M., Tiller R., et al. (2020). Investigating the potential for a commercial fishery in the Northeast Atlantic utilizing mesopelagic species. ICES J. Mar. Sci. 77, 2541–2556. doi: 10.1093/icesjms/fsaa114
Grimaldo E., Herrmann B., Brčić J., Cerbule K., Brinkhof J., Grimsmo L., et al. (2022). Prediction of potential net panel selectivity in mesopelagic trawls. Ocean Eng. 260, 111964. doi: 10.1016/j.oceaneng.2022.111964
Groeneveld R., Richter A., Sen S. (2022). Should we fish the mesopelagic? An economic analysis for four EU fishing fleets. Report of H2020 MEESO Deliverable 6.3 (Confidential).
Halliday R. G. (1970). Growth and vertical distribution of the glacier lanternfish, Benthosema glaciale, in the Northwestern Atlantic. J. Fish. Res. Board Canada 27, 105–116. doi: 10.1139/F70-011
Hidalgo M., Browman H. I. (2019). Developing the knowledge base needed to sustainably manage mesopelagic resources. ICES J. Mar. Sci. 76, 609–615. doi: 10.1093/icesjms/fsz067
Hijmans R. J., van Etten J. (2012). raster: Geographic analysis and modeling with raster data. R package version 2.0-12. Available at: http://cran.r-project.org/package=raster.
Hoagland P., Jin D., Holland M., Kostel K., Taylor E., Renier N., et al. (2019). Value beyond view: illuminating the human benefits of the ocean twilight zone (Woods Hole, MA: Woods Hole Oceanographic Institution). doi: 10.1575/1912/25013
ICES (2019a). “Advice basis,” in Report of the ICES Advisory Committee, section 1.2 (Copenhagen: ICES). doi: 10.17895/ices.advice
ICES (2019b). Workshop on data-limited stocks of short-lived species (WKDLSSLS). ICES Scientific Reports 1:73, 166. doi: 10.17895/ices.pub.5549
Irigoien X., Klevjer T. A., Røstad A., Martinez U., Boyra G., Acuña J. L., et al. (2014). Large mesopelagic fishes biomass and trophic efficiency in the open ocean. Nat. Commun. 5, 3271. doi: 10.1038/ncomms4271
Jónsson S., Bárðarson B., Mariano Burgos J. M., Gunnarson L., Gunnarsdóttir S. (2010). Report on cruise B3-2010 of RV Bjarni Sæmundsson in Icelandic waters in winter 2010. Marine and Freshwater Research Institute, Iceland, 22 pp. Available at: https://download.pangaea.de/reference/110911/attachments/CruiseReportB03_2010final.pdf.
Kaartvedt S., Røstad A., TA K., Staby A. (2009). Use of bottom-mounted echo sounders in exploring behavior of mesopelagic fishes. Mar. Ecol. Prog. Ser. 395, 109–118. doi: 10.3354/meps08174
Kaartvedt S., Staby A., Aksnes D. L. (2012). Efficient trawl avoidance by mesopelagic fishes causes large underestimation of their biomass. Mar. Ecol. Prog. Ser. 456, 1–6. doi: 10.3354/MEPS09785
Kawaguchi K., Mauchline J. (1982). Biology of myctophid fishes (Family myctophidae) in the rockall trough. Northeastern Atlantic Ocean Biol. Oceanogr. 1, 337–373. doi: 10.1080/01965581.1982.10749447
Kourantidou M., Jin D. (2022). Mesopelagic–epipelagic fish nexus in viability and feasibility of commercial-scale mesopelagic fisheries. Nat. Resour. Model. 35, e12350. doi: 10.1111/nrm.12350
Maina I., Kavadas S., Vassilopoulou V., Bastardie F. (2021). Fishery spatial plans and effort displacement in the eastern Ionian Sea: A bioeconomic modelling. Ocean Coast. Manag. 203, 105456. doi: 10.1016/J.OCECOAMAN.2020.105456
Mangel M., Brodziak J., Dinardo G. (2010). Reproductive ecology and scientific inference of steepness: a fundamental metric of population dynamics and strategic fisheries management. Fish Fish. 11, 89–104. doi: 10.1111/j.1467-2979.2009.00345.x
Marine Research Institute (2015). State of marine stocks in Icelandic waters 2014/2015 and prospects for the quota year 2015/2016. Mar. Res. Iceland 182, 217.
Nielsen J. R., Thunberg E., Holland D. S., Schmidt J. O., Fulton E. A., Bastardie F., et al. (2018). Integrated ecological-economic fisheries models-Evaluation, review and challenges for implementation. Fish Fish. 19, 1–29. doi: 10.1111/faf.12232
NOAA National Centers for Environmental Information (2022). ETOPO 2022 15 Arc-Second Global Relief Model (NOAA National Centers for Environmental Information). doi: 10.25921/fd45-gt74
Olsen R. E., Strand E., Melle W., Nørstebø J. T., Lall S. P., Ringø E., et al. (2020). Can mesopelagic mixed layers be used as feed sources for salmon aquaculture? Deep Sea Res. 2 Top. Stud. Oceanogr. 180, 104722. doi: 10.1016/j.dsr2.2019.104722
Pante E., Simon-Bouhet B. (2013). marmap: A package for importing, plotting and analyzing bathymetric and topographic data in R. PloS One 8, e73051. doi: 10.1371/journal.pone.0073051
Paoletti S., Nielsen J. R., Sparrevohn C. R., Bastardie F., Vastenhoud B. M. J. (2021). Potential for mesopelagic fishery compared to economy and fisheries dynamics in current large scale Danish pelagic fishery. Front. Mar. Sci. 8. doi: 10.3389/fmars.2021.720897
Pelletier N., André J., Charef A., Damalas D., Green B., Parker R., et al. (2014). Energy prices and seafood security. Global Environ. Change 24, 30–41. doi: 10.1016/j.gloenvcha.2013.11.014
PFMC (2016). Pacific Management Council - CPS FMP Amendment 15: Unfished and unmanaged forage fish protections. Available at: https://www.federalregister.gov/documents/2016/04/04/2016-07516/fisheries-off-west-coast-%0Astates-comprehensive-ecosystem-based-amendment-1-amendments-to-the-fishery#sectno-reference-660.5.
Pinti J., DeVries T., Norin T., Serra-Pompei C., Proud R., Siegel D. A., et al. (2023). Model estimates of metazoans’ contributions to the biological carbon pump. Biogeosciences 20, 997–1009. doi: 10.5194/BG-20-997-2023
Prellezo R. (2019). Exploring the economic viability of a mesopelagic fishery in the Bay of Biscay. ICES J. Mar. Sci. 76, 771–779. doi: 10.1093/icesjms/fsy001
Prihartato P. K., Aksnes D. L., Kaartvedt S. (2015). Seasonal patterns in the nocturnal distributionand behavior of the mesopelagic fish Maurolicus muelleri at high latitudes. Mar. Ecol. Prog. Ser. 521, 189–200. doi: 10.3354/meps11139
Proud R., Handegard N. O., Kloser R. J., Cox M. J., Brierley A. S., Demer D. (2019). From siphonophores to deep scattering layers: Uncertainty ranges for the estimation of global mesopelagic fish biomass. ICES J. Mar. Sci. 76, 718–733. doi: 10.1093/icesjms/fsy037
Pusineri C., Vasseur Y., Hassani S., Meynier L., Spitz J., Ridoux V. (2005). Food and feeding ecology of juvenile albacore, Thunnus alalunga, off the Bay of Biscay: a case study. ICES J. Mar. Sci. 62, 116–122. doi: 10.1016/j.icesjms.2004.09.004
Rana K. J., Siriwardena S., Hasan M. R. (2009). Impact of rising feed ingredient prices on aquafeeds and aquaculture production. FAO Fish. Aquac. Tech. Pap. 541, 1–63.
Roberts C. M., Hawkins J. P., Hindle K., Wilson R. W., O’leary B. C. (2020). Entering the Twilight Zone: The ecological role and importance of mesopelagic fishes. Blue Mar. Foundation, 1–29.
RStudio Team (2020). RStudio: Integrated Development for R (Boston, MA: RStudio, Inc.). Available at: https://www.rstudio.com/.
Rufener M.-C., Nielsen J. R., Kristensen K., Bastardie F. (2023). Closing certain essential fish habitats to fishing could be a win-win for fish stocks and their fisheries – Insights from the western Baltic cod fishery. Fish Res. 268, 106853. doi: 10.1016/j.fishres.2023.106853
Salomon M., Markus T., Dross M. (2014). Masterstroke or paper tiger – The reform of the EU’s Common Fisheries Policy. Mar. Policy 47, 76–84. doi: 10.1016/j.marpol.2014.02.001
Salvanes A. G. V., Stockley B. M. (1996). Spatial variation of growth and gonadal developments of Maurolicus muelleri in the Norwegian Sea and in a Norwegian fjord. Mar. Biol. 126, 321–332. doi: 10.1007/BF00347456
Schadeberg A., Kraan M., Groeneveld R., Trilling D., Bush S. (2023). Science governs the future of the mesopelagic zone. NPJ Ocean Sustainability 2, 2. doi: 10.1038/s44183-023-00008-8
Sobradillo B., Boyra G., Martinez U., Carrera P., Peña M., Irigoien X. (2019). Target Strength and swimbladder morphology of Mueller’s pearlside (Maurolicus muelleri). Sci. Rep. 9, 1–14. doi: 10.1038/s41598-019-53819-6
Staby A., Aksnes D. L. (2011). Follow the light-diurnal and seasonal variations in vertical distribution of the mesopelagic fish Maurolicus muelleri. Mar. Ecol. Prog. Ser. 422, 265–273. doi: 10.3354/MEPS08938
Staby A., Srisomwong J., Rosland R. (2013). Variation in DVM behaviour of juvenile and adult pearlside Maurolicus muelleri linked to feeding strategies and related predation risk. Fish Oceanogr. 22, 90–101. doi: 10.1111/fog.12012
Standal D., Grimaldo E. (2020). Institutional nuts and bolts for a mesopelagic fishery in Norway. Mar. Policy 119, 104043. doi: 10.1016/j.marpol.2020.104043
St. John M. A., Borja A., Chust G., Heath M., Grigorov I., Mariani P., et al. (2016). A dark hole in our understanding of marine ecosystems and their services: Perspectives from the mesopelagic community. Front. Mar. Sci. 3. doi: 10.3389/fmars.2016.00031
Sutton T. T., Porteiro F. M., Heino M., Byrkjedal I., Langhelle G., Anderson C. I. H., et al. (2008). Vertical structure, biomass and topographic association of deep-pelagic fishes in relation to a mid-ocean ridge system. Deep Sea Res. Part II: Topical Stud. Oceanogr. 55, 161–184. doi: 10.1016/j.dsr2.2007.09.013
Thorson J. T. (2020). Predicting recruitment density dependence and intrinsic growth rate for all fishes worldwide using a data-integrated life-history model. Fish Fish. 21, 237–251. doi: 10.1111/FAF.12427
UN (1982). United Nations Convention on the Law of the Sea (UNCLOS). Available at: http://www.un.org/Depts/los/convention_agreements/convention_overview_convention.htm.
UN (1995). United Nations Conference on Straddling Fish Stocks and Highly Migratory Fish Stocks. Available at: http://www.un.org/Depts/los/convention_agreements/convention_overview_fish_stocks.htm.
UN (2002). World Summit on Sustainable Development (WSSD). Available at: https://sustainabledevelopment.un.org/milesstones/wssd.
Underwood M. J., García-Seoane E., Klevjer T. A., Macaulay G. J., Melle W. (2020). An acoustic method to observe the distribution and behaviour of mesopelagic organisms in front of a trawl. Deep Sea Res. 2 Top. Stud. Oceanogr. 180. doi: 10.1016/J.DSR2.2020.104873
Underwood M. J., Utne Palm A. C., Øvredal J. T., Bjordal Å. (2021). The response of mesopelagic organisms to artificial lights. Aquac. Fish 6, 519–529. doi: 10.1016/J.AAF.2020.05.002
Valinassab T. (1998). Trial fishing for lantern fishes (myctophids) in the Gulf of Oman, (1989–1990). FAO Fish. Circular (Rome: FAO), 935.
Valinassab T., Pierce G. J., Johannesson K. (2007). Lantern fish (Benthosema pterotum) resources as a target for commercial exploitation in the Oman Sea. J. Appl. Ichthyol. 23, 573–577. doi: 10.1111/j.1439-0426.2007.01034.x
van der Meer J., Callier M., Fabi G., van Hoof L., Nielsen J. R., Raicevich S. (2023). The carrying capacity of the seas and oceans for future sustainable food production: Current scientific knowledge gaps. Food and Energy Security, 1–28. doi: 10.1002/fes3.464
Vastenhoud B. M. J., Mildenberger T. K., Kokkalis A., Paoletti S., Alvarez P., Garcia D., et al. (2023). Growth and natural mortality of Maurolicus muelleri and Benthosema glaciale in the Northeast Atlantic Ocean. Front. Mar. Sci, 10. doi: 10.3389/fmars.2023.1278778
Keywords: mesopelagic fishery, ecological sustainability, economic viability analysis, Danish large-scale pelagic fishery, DISPLACE bio-economic model, Maurolicus muelleri, Benthosema glaciale, Northeast Atlantic Ocean
Citation: Vastenhoud BMJ, Bastardie F, Andersen KH, Speirs DC and Nielsen JR (2023) Economic viability of a large vessel mesopelagic fishery under ecological uncertainty. Front. Mar. Sci. 10:1285793. doi: 10.3389/fmars.2023.1285793
Received: 30 August 2023; Accepted: 09 November 2023;
Published: 15 December 2023.
Edited by:
Trevor Hutton, Commonwealth Scientific and Industrial Research Organisation (CSIRO), AustraliaReviewed by:
Gorka Merino, Technology Center Expert in Marine and Food Innovation (AZTI), SpainPatrice Guillotreau, Institut de Recherche Pour le Développement (IRD), France
Copyright © 2023 Vastenhoud, Bastardie, Andersen, Speirs and Nielsen. This is an open-access article distributed under the terms of the Creative Commons Attribution License (CC BY). The use, distribution or reproduction in other forums is permitted, provided the original author(s) and the copyright owner(s) are credited and that the original publication in this journal is cited, in accordance with accepted academic practice. No use, distribution or reproduction is permitted which does not comply with these terms.
*Correspondence: Berthe M. J. Vastenhoud, Ym1qdkBhcXVhLmR0dS5kaw==