- 1Microbial Oceanography Laboratory, The Marine Science Institute, University of the Philippines, Quezon City, Philippines
- 2Microplastics Quantification, Identification, and Biodegradation (MicroQuIB) Facility, Bolinao Marine Laboratory, The Marine Science Institute, Bolinao, Philippines
- 3Institute of Environmental Science and Meteorology, University of the Philippines, Quezon City, Philippines
Introduction: Microplastic pollution has become a global issue, eliciting attention not just from the scientific community but also both from the public and governmental bodies. Drawing data-driven policies and interventions, however, remain difficult due to the severely lacking baseline information from different environments such as beaches. One of the challenges in doing baseline studies is the lack of harmonized methodologies that will allow for comparison of results, integration of data, and its effective translation to evidence-based policies. Emphasis on quality control measures among baselining efforts through the proper implementation of experimental controls is also lacking.
Methodology: To address these gaps, we compared methodologies for preparing the sediment matrix for experimental controls, as well as evaluated protocols for extracting microplastics from tropical beach sediments. Beach sediments were collected, dried, sieved, and spiked with known amounts of microplastics of different polymer types. The removal and extraction efficiencies of the protocols being compared were evaluated.
Results and discussion: Our results showed that subjecting beach sediments to a furnace at 550° C for 4 hours is the most efficient way to remove plastic contamination, implying its applicability for preparing experimental controls. Meanwhile, a modified version of Masura et al. (2015), one of the widely cited methodologies for microplastics extraction, exhibited the highest mean extraction efficiency (99.05 ± 0.82%) among the protocols being compared. Results of this work will be useful in identifying methods that can be adopted and utilized for research and baselining efforts not just in the Philippines but also in Southeast Asia. This will also be helpful in the harmonization of methods, data reporting, and even skills as implemented through the regional and national action plans to address marine plastic pollution.
1 Introduction
Accumulating evidence demonstrates the severe impacts of the different forms of plastics pollution in aquatic and marine environments (Sigler, 2014; MacLeod et al., 2021). Among the different scales by which plastics can affect the environment, smaller fragments measuring less than 5 mm also known as ‘microplastics’ (Arthur et al., 2009) tend to be more problematic as they are less visible, highlighting their potential to be ingested, transported, and accumulate unnoticed (Padervand et al., 2020). In fact, the presence of microplastics has already been documented in zooplankton (Frias et al., 2014; Desforges et al., 2015), fish (Sequeira et al., 2020), corals (Ding et al., 2019), and other marine organisms (Guzzetti et al., 2018). Because of their small size, one of the growing concerns is how they get transferred across the trophic levels - from the very small organisms such as plankton (Setälä et al., 2014) to the apex predators that include humans (Nelms et al., 2018) - highlighting their widespread bioavailability. Additionally, their buoyancy and passive drifting allow them to be transported to places far from where they originated (Ryan, 2015). In fact, microplastics have been detected in the remotest regions of the Arctic (Lusher et al., 2015) and Antarctic (Waller et al., 2017), and even in the air (Gasperi et al., 2018). This is concerning, since microplastics in the natural environment have been observed to coexist with entities adsorbed on its surface including but not limited to known harmful substances such as heavy metals (Brennecke et al., 2016), polycyclic aromatic hydrocarbons (PAHs) (Sharma et al., 2020), persistent organic pollutants (POPs) (Bakir et al., 2014), and even pathogenic microorganisms (Kirstein et al., 2016). With the still relatively limited studies on the direct effects of microplastics on organisms, scientists postulate that surface modification of microplastics may be a potentially greater risk to the environment than the particle itself (Franzellitti et al., 2019; Campanale et al., 2020).
Undoubtedly, the problem of microplastics pollution warrants immediate and proper action and intervention to help reduce its negative effects. Policies for its mitigation, however, will necessitate the availability of data that can provide insights on the development of sustainable, appropriate, and long-term solutions. This entails counting, surveying, and baselining of plastics pollution across different temporal and spatial scales. Nevertheless, the lack of standardization in size range definitions, as well as the lack of harmonization in sampling and processing methodologies, hinders the translation of these scientific efforts into functioning policies, and paints a suboptimal illustration of the plastic pollution problem (Silva et al., 2018; Hartmann et al., 2019; Galarpe et al., 2021).
As scientists continue to advance our understanding of microplastics pollution, the race towards developing methods for extracting microplastics endures (Cutroneo et al., 2021). Several protocols are currently being employed to extract microplastics from different environmental matrices such as water (e.g., freshwater (Pashaei et al., 2023) and wastewater (Mhiret Gela and Aragaw, 2022)), sediments (e.g., soil (Scheurer and Bigalke, 2018) and riverine (Osorio et al., 2021)), and biota (e.g., fish (Tien et al., 2020)) with varying efficiencies. In water samples, the general procedure involves an initial volume reduction step through filtration, followed by extraction. Meanwhile, biota samples necessitate the removal of tissues integrated with microplastics, most commonly through digestion, prior to extraction (Karlsson et al., 2017). However, for sediments, the resemblance in sizes and at times in density of some microplastics and sediment particles presents difficulties in size- or density-based preconcentration (Nuelle et al., 2014; Quinn et al., 2017). Density separation facilitates the segregation between microplastics and non-plastics through the addition of a solution, usually a salt solution, causing the flotation of microplastics and sinking of non-plastics (Hidalgo-Ruz et al., 2012). Therefore, an extracting solution denser than most plastic types, at the same time less dense than most non-plastic materials, is desired to effectively discriminate between plastics and non-plastics. Several studies have investigated the use of various salt solutions for density separation; Mathalon & Hill (2014) used saturated sodium chloride (NaCl) solution to extract microplastics from intertidal sediments and in mussel samples (Mathalon and Hill, 2014). Meanwhile, other studies incorporated the use of heavier salts such as zinc chloride (ZnCl2) to improve extraction efficiency (Osorio et al., 2021; Kukkola et al., 2022; Mhiret Gela and Aragaw, 2022). Water and sediment samples with considerable amounts of interfering organic matter are also treated with agents for organic matter digestion such as strong alkalis, acids, or oxidizing agents (Prata et al., 2019b). Some studies have also tested methods other than density separation; for instance, Bellasi et al. (2021) suggested the use of oil-based extraction as a greener alternative. With the still ongoing pursuit of harmonization in protocols for processing microplastics, issues regarding reproducibility of methods and comparability of results remain to be addressed on the global, regional, and local scale (Rochman et al., 2017; Braaten et al., 2021; Galarpe et al., 2021; Omeyer et al., 2022).
The lack of comparable, field-derived baselines primarily driven by the challenge of unharmonized protocols is especially relevant in Southeast Asia, whose countries are consistently ranked among the top plastic polluting countries in the world in reference to the widely cited modeling studies (Jambeck et al., 2015; Law et al., 2020; Meijer et al., 2021). As a matter of fact, the availability of field-derived data in the region is only starting to build up in recent years (Curren et al., 2021; Alindayu et al., 2023). In the Philippines, for example, studies on the occurrence and abundance of microplastics in coastal ecosystems (i.e., beach, mangroves, seagrass beds (Paler et al., 2019; Gaboy et al., 2022; Navarro et al., 2022)), freshwater ecosystems (i.e., lakes, riverine systems (Osorio et al., 2021; Arcadio et al., 2022)), and even ambient air (Romarate et al., 2022) are now becoming increasingly available. Still, progress in the region remains slow in the absence of harmonized methodologies validated on tropical beach sediments. The difficulty of studying beach sediments lies in the complexity and heterogeneity of the matrix which is also dependent on environmental conditions (Constant et al., 2019). In addition, the interactions between sediment particles and microplastics (i.e., aggregation, adsorption) make them more challenging to effectively separate compared to water samples (Li et al., 2019). To date, most of the published studies that compared methods for extraction were conducted in the developed regions outside the tropics characterized by significantly different sets of habitat types, and environmental and socioeconomic conditions (Gabisa and Gheewala, 2022). This lack of reference methodology for tropical beach sediments has led to different groups employing varying methods for sampling and extracting microplastics, resulting in difficulties in integration and interpretation of data, and comparison of results (Galarpe et al., 2021; Omeyer et al., 2022). Additionally, there is not much emphasis being given on the appropriate implementation of experimental controls in the form of spiked samples and blanks (Dawson et al., 2023). Spiked samples and sample blanks are important quality assurance/control (QA/QC) measures to assess the performance of the extraction protocol and to account for extraneous microplastic contamination, respectively; both of which require beach sediments that are free from microplastic contamination (Dawson et al., 2023). However, given that there is still no standard or recommended way of preparing uncontaminated beach sediments, the quality of the resulting data remains difficult to ascertain in the absence of experimental controls. Thus, this study aims to contribute to addressing these gaps by comparing methodologies for matrix preparation for experimental controls and extraction of microplastics from tropical beach sediments. As of this writing, there are no existing studies in literature that have compared methods for preparing uncontaminated beach sediments for controls, making this the first to do such work. This study is also the first to compare protocols for extraction of microplastics from beach sediments in the Philippines which are presumed to be likewise applicable to other countries in Southeast Asia and other tropical coastal environments. The insights from this study will be helpful to the current efforts to harmonize methodologies not just in the Philippines but also in the region.
2 Materials and methods
2.1 Preparation of sediments for controls
The beach sediments used in the comparison of protocols were collected from Guiguiwanen Beach beside the Bolinao Marine Laboratory of The Marine Science Institute, University of the Philippines in Bolinao, Pangasinan (Figure 1) using a small metal shovel and a metal container. The top 5 cm were collected, dried in an oven at 80°C, sieved through a 5.6-mm mesh metal sieve, and labeled as ‘sieved beach sediments’.
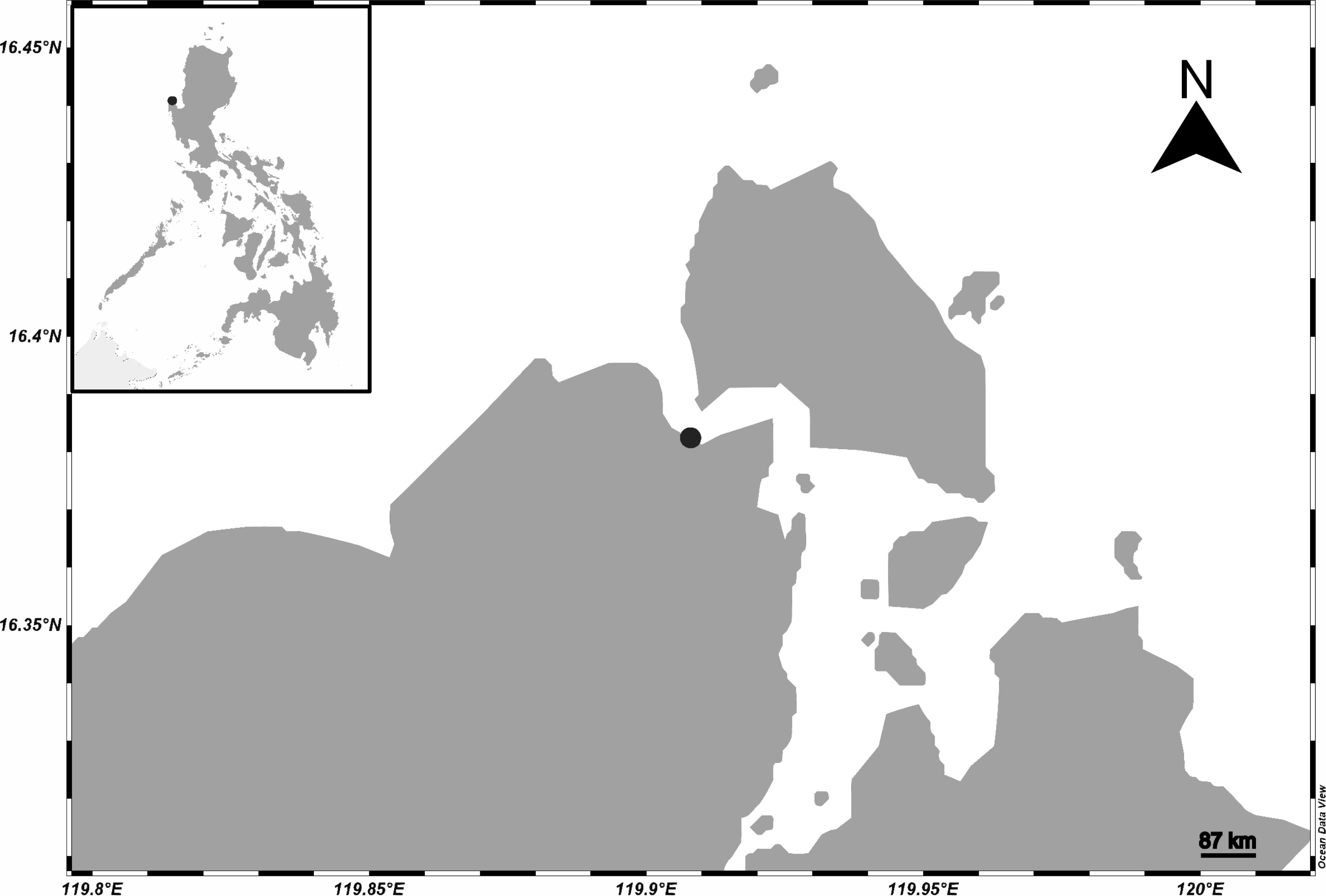
Figure 1 Sampling location for beach sediments used in comparison of methodologies. [inset] Map of the Philippines with the sampling location indicated with a black dot. (Figure generated through ODV; Schlitzer, 2023).
Sediments free from plastic contamination, hereinafter referred to as ‘clean’ beach sediments, are essential components of experimental controls for microplastics quantification (i.e., blanks and spiked samples). To determine the most efficient way to prepare ‘clean’ beach sediments, three methodologies were compared. One hundred grams (100 g) of sieved beach sediments were transferred to beakers and aluminum pans, which were then spiked by adding a known number of different common plastic types reported in Philippine-based surveys (PlastiCount Pilipinas, 2022). These include polystyrene (PS) foam, polypropylene (PP) fragment, low-density polyethylene (LDPE) film, high-density polyethylene (HDPE) fragment, polyamide (PA/nylon) fiber, and polyethylene terephthalate (PET) fragment. The microplastics used for spiking were prepared from commonly available consumer plastic items. These were cut and shredded to varying sizes ranging from 1-5 mm and their polymer type verified through Attenuated Total Reflectance - Fourier Transform Infrared (ATR-FTIR) spectroscopy using the Bruker ATR-IR Polymers, Plastics and Additives Library, Kunststoff-Institut Lüdenscheid database and ATR-FTIR Polymer Library (Bruker Optics).
Ten microplastics from each of the six plastic categories were added to each sediment sample (Han et al., 2019). Here, three methodologies for preparing ‘clean’ beach sediments were tested and compared. Method 1 involved the addition of saturated NaCl (ρ = 1.2 g/mL) followed by subsequent vigorous stirring for 2 minutes (Bridson et al., 2020). The mixture was allowed to settle for at least 5 hours before decanting the supernatant to remove floating plastics. The addition of extracting solution, allotment of time for settling, and decantation of the supernatant were repeated thrice. Saturated NaCl solution was used as the density separation solution as it is a more economical and practical approach; higher density solutions are usually expensive and are not cost-efficient for the sole purpose of preparing ‘clean’ beach sediments. On the other hand, Method 2 involved subjecting the beach sediments in aluminum pans to a furnace at 550°C for 4 h (Schütze et al., 2022). Lastly, Method 3 employed a density separation method using saturated NaCl (single extraction) followed by subjecting the beach sediments to a furnace at 550°C for 4 hours (Shim et al., 2016), as shown in Figure 2. Each procedure was performed in seven replicates.
2.2 Comparison of methods for extraction
The methodologies by Thompson et al. (2004), Coppock et al. (2017), and Masura et al. (2015) are commonly used approaches for extracting microplastics from beach sediments but with noted variabilities. To optimize and validate the efficiency of the methods when applied to tropical beach sediments, the three methodologies were compared as shown in Figure 3.
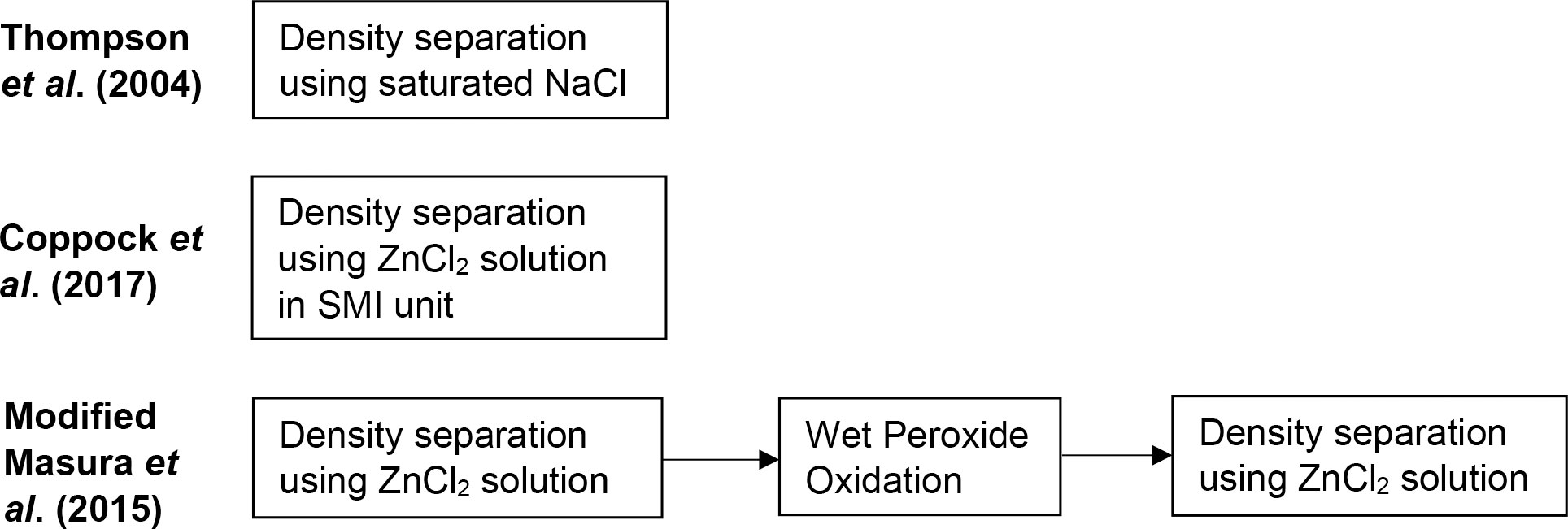
Figure 3 General overview of methodologies being compared for extracting microplastics from beach sediments.
The most efficient protocol for the preparation of ‘clean’ beach sediments as determined in the previous section was employed. For this part, 100 g of ‘clean’ beach sediments were transferred to a beaker. Ten microplastics from each of the seven plastic categories, namely: polystyrene (PS) foam, polypropylene (PP) fragment, low-density polyethylene (LDPE) film, high-density polyethylene (HDPE) fragment, polyamide (PA/nylon) fiber, polyethylene terephthalate (PET) fragment, and polyvinyl chloride (PVC) shavings were added to each sediment sample (Figure 4). A small amount (~0.02 g) of dried seaweed (Sargassum spp.) and driftwood were also added to the mixture to simulate the presence of some naturally occurring organic material in environmental samples. Each methodology employed triplicate samples.
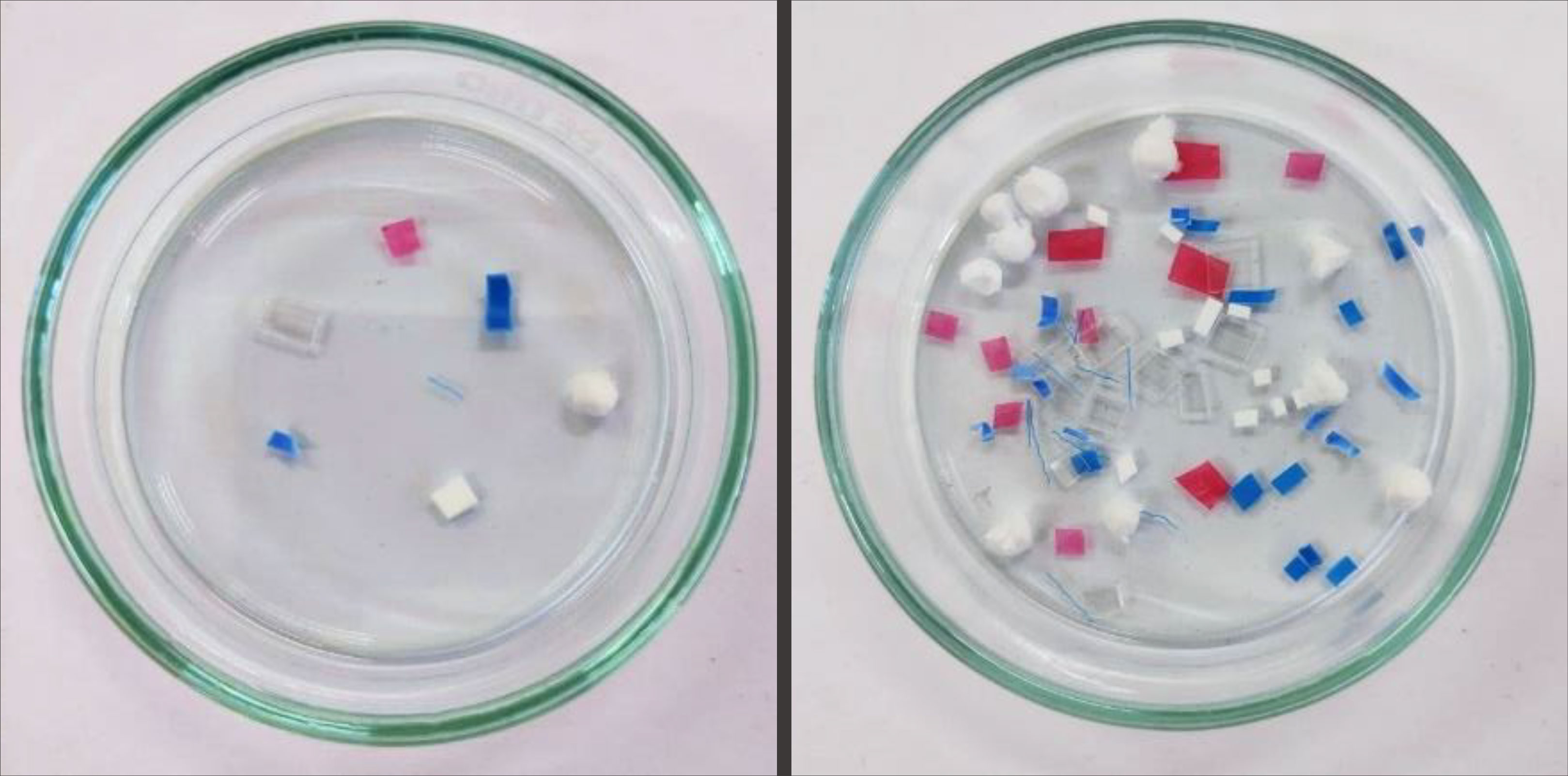
Figure 4 Microplastics used for spiking: clear PET fragment, red LDPE film, blue PP fragment, blue PA/nylon fiber, white PS foam, white HDPE fragment, and blue PVC shavings.
Thompson et al. (2004) employed density separation using saturated NaCl (ρ = 1.2 g/mL). For this, saturated NaCl was added to the spiked sediment samples and the mixture was stirred for 30 seconds. After allowing the sediments to settle for 2 minutes, the supernatant was filtered, and the residue was collected and counted.
Coppock et al. (2017) made use of a Sediment-Microplastic Isolation (SMI) unit, shown in Supplementary Figure 1, to facilitate the density separation using a solution of ZnCl2 (ρ = 1.5 g/mL). The SMI unit was initially primed through repeated washing using ZnCl2 solution. Spiked sediment samples were then added to the SMI unit followed by the addition of ZnCl2 solution. A magnetic stir bar was also added to agitate the mixture for 5 minutes followed by the settling process for another 5 minutes. To remove possible trapped air bubbles in the sediments, three short pulses were introduced through the magnetic stir bar. The mixture was then left to stand. When the supernatant was clear with sediments, the valve was closed, and the mixture in the upper half of the SMI unit containing the floating particles was transferred to a beaker and subsequently filtered. The residue was collected and counted.
The procedure by Masura et al. (2015) with slight modifications as sugested by Besley et al. (2017) was employed for the third set of spiked samples. The general workflow involved an initial two-round density separation step through the addition of ZnCl2 solution (ρ = 1.5 g/mL), followed by wet peroxide oxidation to remove organic matter, then another two-round density separation using ZnCl2 to facilitate the isolation of microplastics from the remaining sediments. The detailed flowchart is shown in Supplementary Figure 2.
2.3 Quality control measures
This study employed protocols to minimize and account for the possibility of contamination. As much as possible, the use of plastic materials was avoided during sampling and laboratory processing. Gloves were worn throughout the experiment. Laboratory work was performed in the Microplastics Quantification, Identification, and Biodegradation (MicroQuIB) facility in the Bolinao Marine Laboratory (BML) of the UP MSI, a dedicated clean facility for plastics research equipped with an air purifier to minimize the possibility of air contamination. Blanks for air contamination were also set up. Prior to the conduct of the experiment, the working table was wiped with a paper towel and 70% ethanol; lint remover was also used on the clothes and lab gowns of the researchers before entering the laboratory. Samples being processed were covered with aluminum foil or glass petri plates whenever possible. Lastly, reagents used in the experiment were pre-filtered through GF/C filters (1.2-µm pore size) prior to usage.
2.4 Statistical analyses
Efficiencies of methodologies were expressed through percent removal (for preparation of controls) and percent extraction efficiencies. To test for significant differences between treatments, one-way analysis of variance (ANOVA) was employed at α = 0.05 and implemented in PAST version 4.13 (Hammer et al., 2001). Tukey’s pairwise comparison was used as the post-hoc test.
3 Results and discussion
3.1 Preparation of sediments for controls
Experimental controls, both positive (spiked samples) and negative (blanks), are essential QA/QC measures in any type of experiment. In microplastics research, spiked samples are used to assess how well the methodology was performed (Miller et al., 2017). Spiking involves the addition of a known amount of microplastics to ‘clean’ beach sediments. Spiked samples then undergo the usual extraction protocol and the amount of microplastics recovered by the end is noted; a high recovery rate serves as an assurance of the quality of the conduct of the experiment (Primpke et al., 2022). Additionally, spike-recovery tests may also be used, as in this experiment, to validate the effectiveness of methods in extracting microplastics from environmental matrices (Way et al., 2022). On the other hand, blanks are employed to account for extraneous contamination from the environment, reagents, and equipment used in processing samples (Dawson et al., 2023). Sample blanks essentially contain everything in the sample matrix except the analyte, in this case, microplastics (Cantwell, 2019). This allows for a realistic estimation of contamination as the sediment samples are being processed. However, obtaining a reference material in the form of ‘clean’ beach sediments is very difficult and not commercially plausible (Silva et al., 2018; Cadiou et al., 2020). While some studies have employed their own protocol for ‘cleaning’ beach sediments (Shim et al., 2016; Bridson et al., 2020; Schütze et al., 2022), no controlled comparisons have yet been made that can inform future harmonization efforts. Thus, there is a need to optimize towards an efficient method for removing plastic contamination in beach sediments.
Results summarized in Figure 5 showed that two of the three protocols tested exhibited high efficiencies in removing plastic contamination in sediments, allowing for its use in experimental controls. Specifically, only Method 1 exhibited relatively low removal efficiencies, which also varied depending on polymer type. Method 1 operated on the principle of physical separation through density differences among the sediment matrix, extracting solution, and microplastics. The low average removal efficiency of nylon fibers (76.2%) was observed, which may be related to its morphology; the small diameter of fibers relative to its length makes it very difficult to extract, as seen in many spike-recovery tests (Crichton et al., 2017; Cashman et al., 2020). The density of nylon (1.13 – 1.15 g/mL) is also near the maximum density of saturated NaCl solution (1.2 g/mL) (Table 1), and the presence of additives could have increased the fibers’ density towards or beyond 1.2 g/mL, lowering its removal efficiency (Nuelle et al., 2014). Lastly, the fibers’ susceptibility to the effects of static electricity facilitates its adherence to the sides of the glass container, which could have resulted in a lower removal rate (Catarino et al., 2017). Meanwhile, PET fragments also recorded a very low average removal efficiency (18.1%), owing to its higher density (1.37 – 1.39 g/mL) than the extracting solution. It is for this reason that other extracting solutions with a higher density such as zinc chloride (ZnCl2), sodium iodide (NaI), and zinc bromide (ZnBr2) are generally considered for microplastics extraction, albeit deemed impractical for the sole purpose of preparing ‘clean’ beach sediments for controls due to their higher costs (Nuelle et al., 2014; Prata et al., 2019a). Removal efficiencies beyond 100% may be attributed to errors in counting during spike addition, since the thinness of LDPE films makes it easy to mistake two films as one. Also, some of the less rigid microplastics (i.e., LDPE films and PS foams) are prone to fragmentation during processing, resulting in higher counts after the extraction (Cashman et al., 2020).
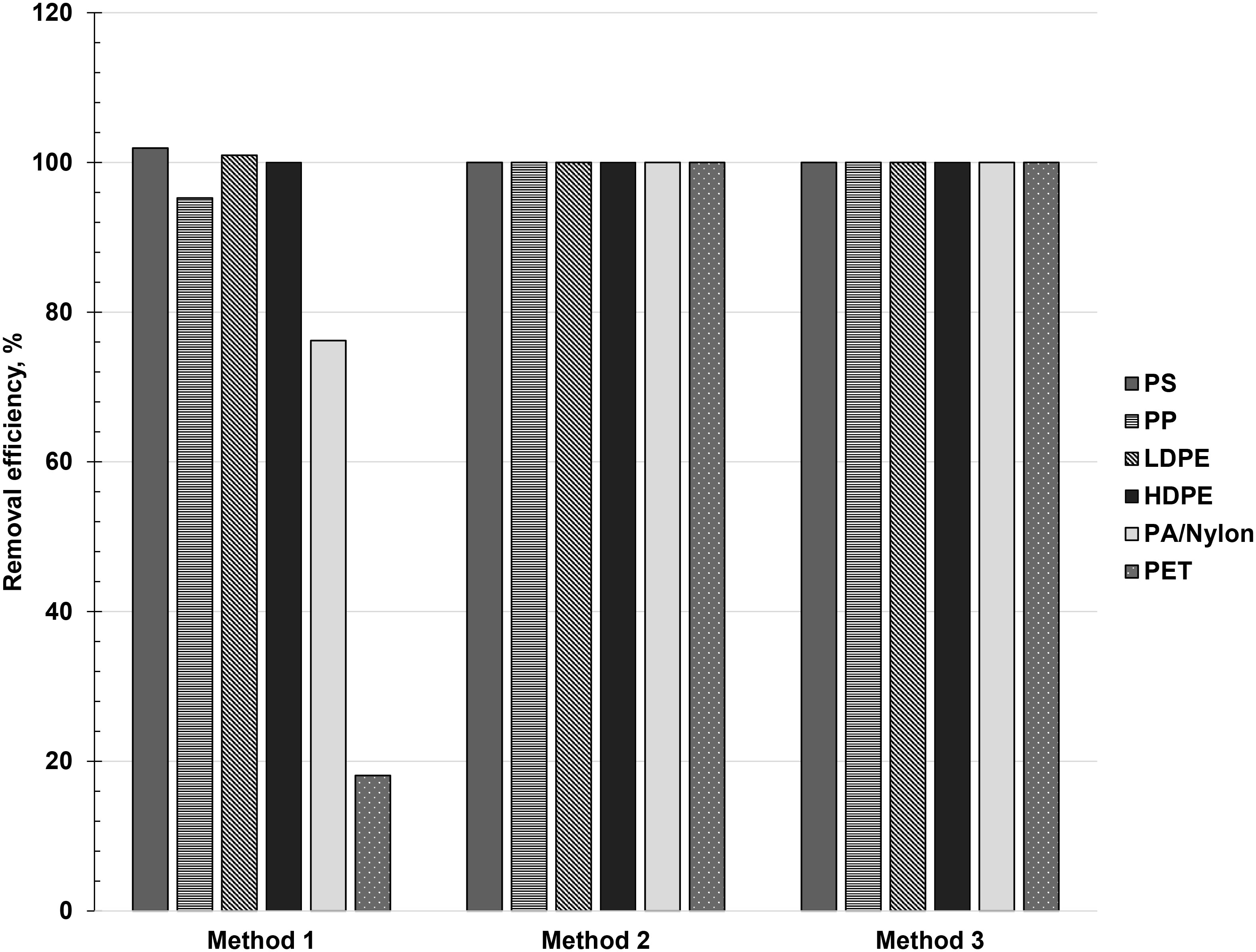
Figure 5 Removal efficiencies of Method 1 (density separation using saturated NaCl), Method 2 (furnace, 550°C for 4 h), and Method 3 (density separation using saturated NaCl + furnace, 550°C for 4 h) for preparation of ‘clean’ beach sediments.
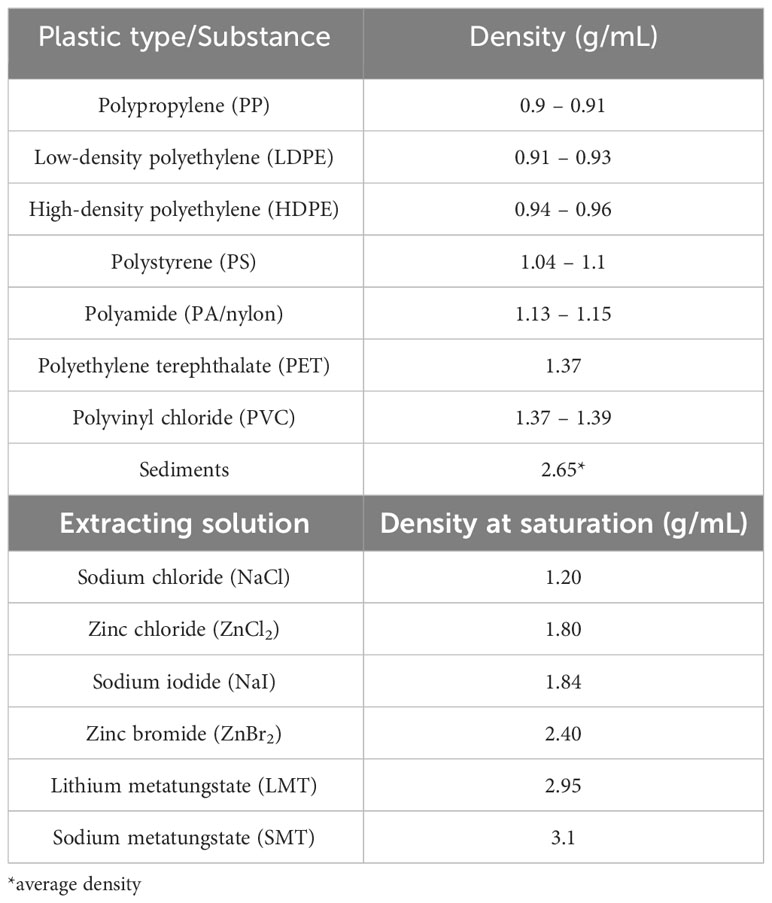
Table 1 Density values for different plastic types and extracting solutions (Hidalgo-Ruz et al., 2012; Barboza et al., 2019).
Methods 2 and 3, both of which relied on high temperatures in a furnace to facilitate the removal of microplastics from beach sediments, showed 100% removal efficiency for all polymer types (Figure 5). Thermal decomposition of the polymer types used in this experiment occurs at temperatures between 350°C and 500°C (Beyler and Hirschler, 2002; Dümichen et al., 2017; Johnson et al., 2020; Ni et al., 2020). However, to account for the possible increase in thermal decomposition temperature due to the presence of additives in plastics, this study increased the employed temperature up to 550°C. Since Method 2 showcased a 100% removal efficiency with just a single step compared to Method 3, it was considered as the most efficient way of preparing ‘clean’ beach sediments for experimental controls in microplastics extraction.
3.2 Comparison of methods for extraction
Once ‘clean’ beach sediments are available for experimental controls, extraction of microplastics from environmental samples, along with spike-recovery tests, can then be conducted. However, due to the high degree of variability among properties and composition of microplastics, it is also reasonable to expect certain differences in recoveries depending on the methodology employed. For instance, Cashman et al. (2020) in a similar study noted that microplastics larger in size are generally well-extracted, which may point towards observer bias as an important factor affecting counts among studies. Additionally, matrix characteristics such as organic matter content may also influence the quality of extraction (Cashman et al., 2020).
Here, the modified protocol of Masura et al. (2015) exhibited an extraction efficiency of 100% for all plastic types except PA/Nylon (93.33%), as shown in Figure 6. This observation seems to be likely associated with the use of a heavier extraction solution (ZnCl2, ρ = 1.5 g/mL) compared to Thompson et al. (2004), and the implementation of wet peroxide oxidation to digest organic matter. This is in congruence with the findings of other studies, suggesting that the removal of organic matter contributes to higher percent recovery during extraction, as organic matter can interact and aggregate with microplastics (Cashman et al., 2020; Constant et al., 2021). The main advantage of this methodology is that it significantly reduces the amount of contaminating organic matter that may complicate further processing and analyses of microplastics, without damaging the integrity of the polymers (Prata et al., 2019b). This is especially important if other methods aside from the traditional, microscopy-based counting (i.e., Nile Red staining and spectroscopic methods) will be employed, as the presence of organic matter, particularly biofilms, can interfere with the signals from the microplastics. As shown in Figure 7, notable amounts of organic matter (i.e., Sargassum spp. and driftwood) remained in samples that did not undergo a digestion step. Meanwhile, the sample that underwent wet peroxide oxidation showed less organic matter, mostly in the form of undigested driftwood. Hydrogen peroxide (H2O2) excels in digestion of plant organic matter, although studies have noted that animal tissues and cellulosic materials such as driftwood are not removed as efficiently (Dyachenko et al., 2017; Herrera et al., 2018; Prata et al., 2019b). For environmental samples containing substantial amounts of animal tissues, a digestion protocol with 10% KOH has been suggested (Prata et al., 2019b).
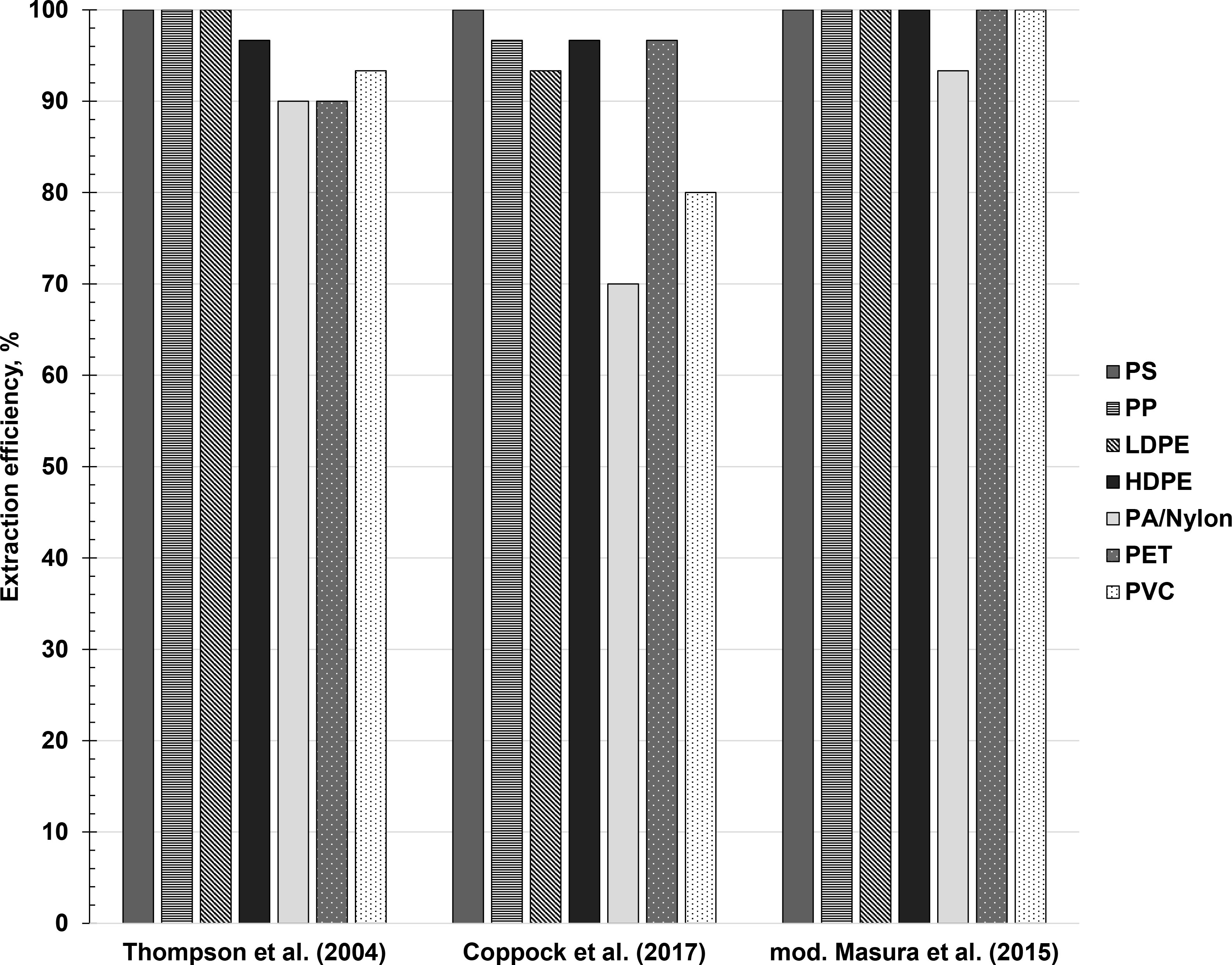
Figure 6 Extraction efficiencies of Thompson et al. (2004), Coppock et al. (2017), and modified Masura et al. (2015) across polymer types.
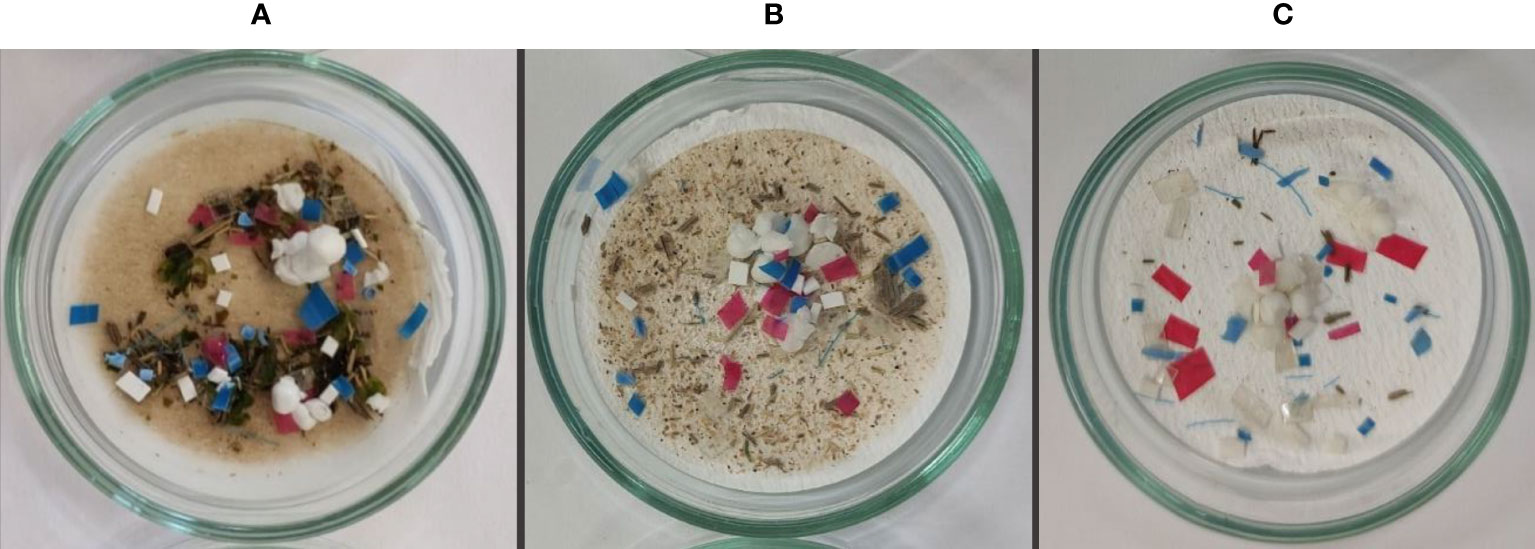
Figure 7 Microplastics extracted using (A) Thompson et al. (2004), (B) Coppock et al. (2017), and (C) modified Masura et al. (2015).
Meanwhile, the relatively high extraction efficiency for Thompson et al. (2004) despite only using saturated NaCl solution may be a result of the reduced heteroaggregation between the sediment matrix and the microplastics, as induced by ‘cleaning’ the beach sediment samples through a furnace prior to mixing with the microplastics. Generally, suspended sediments can form heteroaggregates with microplastics and facilitate their sinking, resulting in a lower extraction efficiency (Li et al., 2019). However, studies have shown that at temperatures beyond 500°C, the physical properties of the soil are altered and that its aggregative properties are disrupted, allowing for a more efficient extraction through decantation (Zihms et al., 2013). This was also visually noted during the performance of the experiment. Overall, these observations may point towards the potential influence of matrix properties (e.g., sediment grain size, organic matter content) on the efficiency of extraction, which was also validated by similar studies (Cashman et al., 2020; Constant et al., 2021).
Interestingly, the overall mean extraction efficiency for the protocol of Coppock et al. (2017) was lower (90.48 ± 2.97%) compared to Thompson et al. (2004) (95.71%) despite using a heavier extracting solution, albeit statistically insignificant (p = 0.09) (Supplementary Figure 3). This also manifested individually across polymer types such as PP (96.67% vs. 100%), LDPE (93.33% vs. 100%), PA/Nylon (70% vs. 90%), and PVC (80% vs. 93.33%). One possible explanation for the lower recovery of Coppock et al. (2017) may be related to the equipment and the interactions it facilitates through its use. For instance, it was visually noted that the unrecovered microplastics were either adhered to the inside walls of the SMI unit or trapped within the ball valve. This was the case despite priming the SMI unit with ZnCl2 solution prior to extraction, as suggested by Coppock et al. (2017), and despite repeated washing after extraction. The same observation was reported by Nel et al. (2019) wherein they suggested not utilizing the ball valve at all and detaching the upper chamber for a more thorough rinsing than when it is attached. The adherence of microplastics to the walls of the SMI unit may be a function of hydrophobic and/or electrostatic interactions and the morphology of the microplastics (Lechthaler et al., 2020; Al Harraq and Bharti, 2022). Among the microplastics used in recovery tests, LDPE films, PVC shavings, and nylon fibers had the highest surface area-to-volume ratio, making them more susceptible to the effects of hydrophobic and/or electrostatic forces (Enders et al., 2020). Thus, it is certainly possible that morphology plays an important role in facilitating interactions between the surface of the plastic and other components during processing. In some studies, modifications were introduced to the SMI unit mostly by replacing the original plastic PVC design into other materials (e.g., glass, aluminum) to avoid plastic contamination and hydrophobic interactions; however, aluminum-based SMIs are known to be susceptible to corrosion when ZnCl2 is used as the extracting solution (Nakajima et al., 2019; Lechthaler et al., 2020). On another note, a higher extraction efficiency for PET (96.67%) was observed for Coppock et al. (2017), which was expected given that PET is less dense than the ZnCl2 extracting solution. Comparing the overall mean extraction efficiencies among the three protocols tested (Supplementary Figure 3), the modified methodology of Masura et al. (2015) was considered the most effective protocol for microplastics extraction from beach sediments.
It is important to acknowledge that the microplastics used in these experiments were neither weathered nor biofouled, which could possibly be more representative of microplastics in the natural environment. However, a sufficient representation was achieved by sourcing microplastics from commonly available consumer plastic items, as listed among the most common plastic types documented in baselining surveys in the Philippines (PlastiCount Pilipinas, 2022). Also, the beach sediment type of the sampling site may not capture the diversity of beach sediment types in the tropics. Lastly, the effect of the furnace on the integrity of the sediment was beyond the scope of this study.
3.3 Implications on the harmonization of methods and future research
Global awareness on microplastics pollution only started in the 1970s, when researchers were supposedly sampling for Sargassum spp. yet came across a lot of small plastic pellets in their neuston nets (Carpenter and Smith, 1972). Since then, more and more studies have documented the presence of microplastics in different environments around the world, a testament to its ubiquity (Rochman, 2018). As a response, global policy-making bodies have listed marine plastic pollution among their priority concerns (Bank et al., 2021). The United Nations Sustainable Development Goal 14: Life Below Water (UN SDG 14) identified pollution by marine plastics under Target 14.1.1b, albeit only to a limited degree for microplastics (Walker, 2021). The United Nations Environment Assembly (UNEA) in March 2022 adopted a resolution to work towards an “international legally binding instrument” to combat plastic pollution, recognizing microplastics as part of the equation (United Nations Environment Assembly resolution 5/14, 2022). Meanwhile, the detailed G20 Report on Actions Against Marine Plastic Litter acknowledges the need to establish harmonized monitoring efforts to better assess the efficiency of countermeasures being employed (MoEFCC, 2023). While these are laudable achievements in terms of global policies, the translation of these frameworks into actions on the regional and local settings remains suboptimal, as microplastics research still suffer drawbacks due to the lack of harmonization in sampling, processing, and characterization (Hartmann et al., 2019).
For Southeast Asia and the Philippines, the field of plastics research is still considered in its infancy. A deeper look into the status of microplastics research in the Philippines reveals the considerably varied approaches among baselining efforts (Galarpe et al., 2021). Table 2 demonstrates how protocols for processing and characterizing microplastics from beach sediments differ among local researchers, which is also prevalent in other countries in the region (Omeyer et al., 2022).
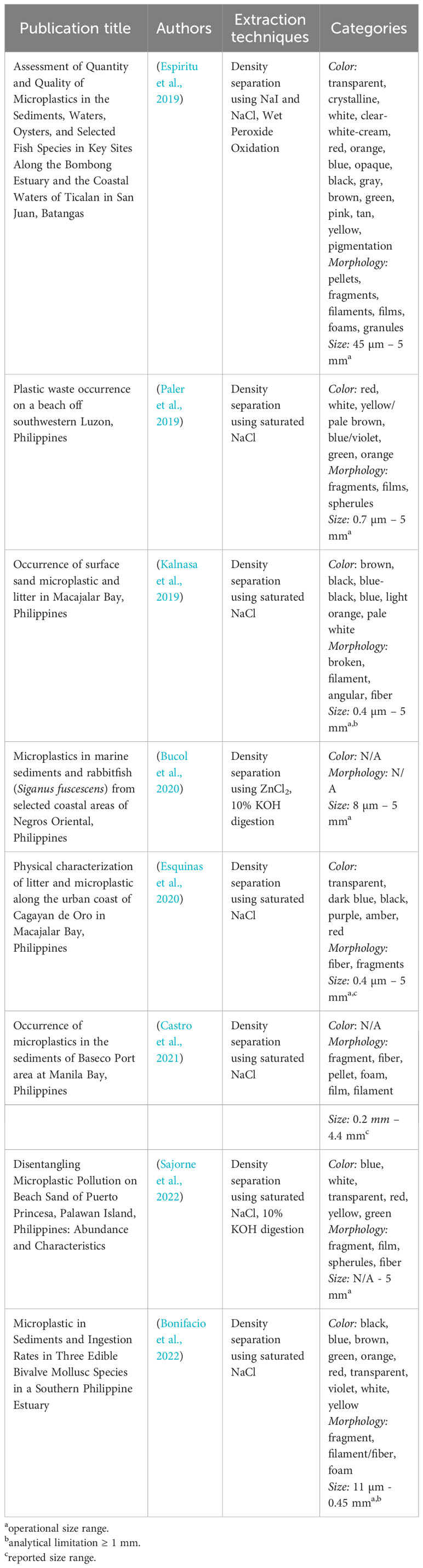
Table 2 Summary of publications and their processing and identification protocols for microplastics in beach sediments in the Philippines.
There are several challenges to the pursuit of harmonization in the Philippines, the most obvious one being the valid technological constraints (Galarpe et al., 2021). The cost of reagents and equipment for microplastics quantification and characterization is an important consideration. For instance, NaCl is a much cheaper option as the salt for density separation than ZnCl2 or NaI; however, using NaCl runs the risk of underestimating the heavier plastic types given its lower density at saturation (ρ = 1.2 g/mL). Other extracting reagents such as oil are also being explored as a cheaper and greener alternative to dense salt solutions, although extraction efficiencies remain variable across studies; not to mention its potential interference to subsequent spectroscopic analyses (Bellasi et al., 2021; Constant et al., 2021). Despite the array of options available for extraction solutions, it can be argued that harmonization in this aspect is still possible even in consideration of the cost. The data from the PlastiCount Pilipinas portal (https://plasticount.ph), which is the most comprehensive database of all plastics research in the Philippines to date (Alindayu et al., 2023), suggest a significantly higher abundance of lighter macroplastics such as PE and PP compared to the heavier plastics such as PET and PVC. Assuming that most of the microplastics in Philippine beaches originated from the fragmentation and degradation of these macroplastics (Van Emmerik and Schwarz, 2019), this data could possibly imply that using saturated NaCl as a density separation solution may provide a sufficient estimate of microplastics pollution, at least for the lighter plastic types. Therefore, the comparison between studies employing different extracting solutions (saturated NaCl vs. ZnCl2) can then still be valid even in just a few plastic types (i.e., plastics with ρ ≤ 1.2 g/mL), as long as the other steps in the protocol were done similarly and that limitations have been acknowledged and considered.
Another technological constraint is the limited access to well-designed and equipped laboratories for microplastics research. This is important, as air contamination of microplastics is a well-established phenomenon that is known to affect the quality of the results (Prata et al., 2021). Access to equipment for spectroscopic methods such as Fourier Transform Infrared (FTIR) spectroscopy and Raman spectroscopy, commonly employed to determine polymer composition, is also limited to very few laboratories or facilities. However, with the proper implementation of experimental controls, background contamination and procedural errors can be adequately accounted for. It is important to note that the harmonization being put forward is geared towards adopting a possible array of options, albeit limited, instead of a one size fits all-approach, in consideration of the diversity of local environmental contexts in the Philippines as well as the differences in resource availability and accessibility.
Given the understandable difficulties in harmonizing methodologies due to a lot of things that need to be considered, a more readily applicable aspect of harmonization can be implemented in terms of the categories used for data reporting. Microplastics extracted from environmental samples are usually described in terms of color, morphology/shape, size, and polymer type (Rocha-Santos and Duarte, 2015). In the Philippines, the most common categories employed in beach sediment studies are summarized in Table 2. Harmonization in the categories used in data reporting will allow for a more effective comparison among studies and integration of results; in fact, this is already recognized by other regions globally, such as the European Union, whose guidelines are currently being updated to incorporate harmonized categories for data reporting (Bäuerlein et al., 2023).
There is also the aspect of harmonization in expertise and skill. Even if methodologies and data reporting protocols are harmonized, a high degree of variability between the researchers’ skills due to differences in the degree of training, experience, and expertise in executing protocols could lead to difficulties in comparability of results (Cadiou et al., 2020; Kotar et al., 2022; Piccardo et al., 2022). This highlights the need to conduct interlaboratory calibration – a harmonization exercise commonly employed as part of QA/QC measures to evaluate the reliability of results across participating laboratories not just within the Philippines but also at the regional level (Tsangaris et al., 2021). To do this, an uncontaminated sediment sample and a sediment sample spiked with a known amount of microplastics, are sent to participating laboratories for processing and subsequent quantification. The results returned by the laboratories are then collated to assess reproducibility of methodologies and comparability of results. Studies that have conducted interlaboratory calibration noted that the operators’ experience seems to influence the quality of extraction, particularly in smaller size classes (Cadiou et al., 2020; Piccardo et al., 2022). An interlaboratory study by Cadiou et al. (2020) revealed that underestimation due to losses of microplastics during handling and processing may be a much greater source of error than overestimation due to extraneous contamination among the participating laboratories. These kinds of insights emphasize the importance of interlaboratory comparisons and consequently, the necessity of an efficient methodology to prepare ‘clean’ beach sediments, which this study was able to achieve.
The challenges faced by microplastics research in the Philippines is a problem shared with other developing countries not just in Southeast Asia but also worldwide (Hartmann et al., 2019; Curren et al., 2021; Omeyer et al., 2022). The paucity of baselines brought about by unharmonized protocols impede our progress to a better understanding of the problem (Omeyer et al., 2022). As a consequence, efforts to address plastic pollution at the policy level suffer from the lack of a realistic direction. In the Philippines, for example, the overarching goal of the National Plan of Action for the Prevention, Reduction, and Management of Marine Litter (NPOA-ML) was set to “Zero waste to Philippine waters by 2040”, yet there is a clear recognition of the lack of comprehensive baselines with which the evaluation of progress is dependent on (Department of Environment and Natural Resources – Environmental Management Bureau, 2021). The problem of the lack of harmonization is likewise recognized on the regional level through Component II of the ASEAN Regional Action Plan for Combating Marine Debris in the ASEAN Member States (2021-2025) under “Research, Innovation, and Capacity Building” (ASEAN Secretariat, 2021). For this, the academe and research institutions will play huge roles in providing guidance and support in the form of science-based recommendations. In the Philippines, a network of plastics researchers in the Philippines was just recently launched as the Plastics Research Network (PlaReNet) Philippines (PlastiCount Pilipinas, 2022), with the objective of assisting in the harmonization of plastics research efforts in the country and aiding the implementation of the NPOA-ML. On the regional level, an international network of experts on marine plastic pollution published a comprehensive set of research agenda and recommendations on tackling marine plastics in the region (Omeyer et al., 2022). This study hopes to contribute to these undertakings by providing information on the potential methodologies that may be considered for harmonized protocols as evaluated on tropical beach sediment samples.
4 Conclusion
Effective evidence-based policies to tackle plastic pollution necessitate comprehensive environmental baselines. However, baselining studies require reliable, reproducible, and efficient methodologies to generate high-quality data that are comparable and contextualized in the regional and local setting. In this study, we compared methods for the preparation of uncontaminated beach sediments for experimental controls, as well as evaluated the efficiencies of protocols for extracting microplastics from tropical beach sediments. The results of this study may be used to suggest potential methodologies for a more harmonized approach to baselining efforts in the Philippines and in Southeast Asia.
Microplastics research has been continuously developing over the recent years, although there is still a lot of work that needs to be done. While it is true that certain limitations exist slowing down progress, a corresponding opportunity can be taken advantage of as this also translates into ease of shifting to the harmonized protocols early on. Given that global, regional, and national action plans already recognize the importance of baselines and harmonization as evident through their implementing strategies, the next thing that needs to be done is to ensure its effective implementation with the support of the relevant research institutions.
Data availability statement
The raw data supporting the conclusions of this article will be made available by the authors upon request, without undue reservation.
Author contributions
JB: Conceptualization, Formal Analysis, Investigation, Methodology, Writing – original draft, Writing – review & editing. NG: Conceptualization, Methodology, Investigation, Visualization, Writing – review & editing. DO: Conceptualization, Funding acquisition, Resources, Supervision, Writing – review & editing.
Funding
The author(s) declare financial support was received for the research, authorship, and/or publication of this article. The authors are grateful for the financial support for this research extended through the Department of Science and Technology – National Research Council of the Philippines (DOST-NRCP)-funded Plastics in the marine environment, trophic systems, and aquaculture in the Philippines (PlasMics), DOST - Philippine Council for Industry, Energy, and Emerging Technology Research and Development (DOST-PCIEERD)-funded PlastiCount Pilipinas, DOST - Science Education Institute Accelerated Science and Technology Human Resource Development Program (DOST-SEI-ASTHRDP) Scholarship Grant, National Security Council (NSC)-funded Upgrading Capacity, Infrastructure, and Assets for Marine Scientific Research in the Philippines (UPGRADE-CIA) project, and United Kingdom Research and Innovation (UKRI)-funded Microbial Transformation of Plastics in South East Asian Seas: A Hazard and A Solution (MicroSEAP) project. The MicroSEAP project also funded the article processing charges for this publication.
Acknowledgments
The authors would like to thank the members of the Microbial Oceanography Laboratory of the University of the Philippines, The Marine Science Institute, especially Mark Prudente and Kim John Balboa who provided assistance during the conduct of the experiment, and the staff and administrative personnel of the Bolinao Marine Laboratory.
Conflict of interest
The authors declare that the research was conducted in the absence of any commercial or financial relationships that could be construed as a potential conflict of interest.
Publisher’s note
All claims expressed in this article are solely those of the authors and do not necessarily represent those of their affiliated organizations, or those of the publisher, the editors and the reviewers. Any product that may be evaluated in this article, or claim that may be made by its manufacturer, is not guaranteed or endorsed by the publisher.
Supplementary material
The Supplementary Material for this article can be found online at: https://www.frontiersin.org/articles/10.3389/fmars.2023.1285041/full#supplementary-material
References
Al Harraq A., Bharti B. (2022). Microplastics through the lens of colloid science. ACS Environ. Au. 2 (1), 3–10. doi: 10.1021/acsenvironau.1c00016
Alindayu R. C., Licnachan L. O., Luzadas R. L., Ignacio P. S., Onda D. F. (2023). Moving towards open data, public access, and information sharing to combat marine plastics pollution in the Philippines and the Southeast Asian region. Ocean. Coast. Manage. 243, 106771. doi: 10.1016/j.ocecoaman.2023.106771
Arcadio C. G. L. A., Navarro C. K. P., Similatan K. M., Inocente S. A. T., Ancla S. M. B., Banda M. H. T., et al. (2022). Microplastics in surface water of Laguna de Bay: First documented evidence on the largest lake in the Philippines. Environ. Sci. pollut. Res. doi: 10.1007/s11356-022-24261-550
Arthur C., Baker J., Bamford H. (Eds.) (2009) in Proceedings of the International Research Workshop on the Occurrence, Effects and Fate of Microplastic Marine Debris, , Sept 9-11, 2008 (NOAA Technical Memorandum NOS-OR&R-30).
ASEAN Secretariat (2021). ASEAN Regional Action Plan for Combating Marine Debris in the ASEAN Member States (Jakarta, Indonesia: Community Relations Division (CRD), ASEAN Secretariat).
Bakir A., Rowland S. J., Thompson R. C. (2014). Enhanced desorption of persistent organic pollutants from microplastics under simulated physiological conditions. Environ. pollut. 185, 16–23. doi: 10.1016/j.envpol.2013.10.007
Bank M. S., Swarzenski P. W., Duarte C. M., Rillig M. C., Koelmans A. A., Metian M., et al. (2021). Global Plastic Pollution Observation System to aid policy. Environ. Sci. Technol. 55 (12), 7770–7775. doi: 10.1021/acs.est.1c00818
Barboza L. G. A., Frias J. P. G. L., Booth A. M., Vieira L. R., Masura J., Baker J., et al. (2019). “Microplastics Pollution in the Marine Environment,” in World Seas: An Environmental Evaluation (Elsevier), 329–351. doi: 10.1016/B978-0-12-805052-1.00020-6
Bäuerlein P. S., Erich M. W., van Loon W. M. G. M., Mintenig S. M., Koelmans A. A. (2023). A monitoring and data analysis method for microplastics in marine sediments. Mar. Environ. Res. 183, 105804. doi: 10.1016/j.marenvres.2022.105804
Bellasi A., Binda G., Pozzi A., Boldrocchi G., Bettinetti R. (2021). The extraction of microplastics from sediments: An overview of existing methods and the proposal of a new and green alternative. Chemosphere 278, 130357. doi: 10.1016/j.chemosphere.2021.130357
Besley A., Vijver M. G., Behrens P., Bosker T. (2017). A standardized method for sampling and extraction methods for quantifying microplastics in beach sand. Mar. pollut. Bull. 114 (1), 77–83. doi: 10.1016/j.marpolbul.2016.08.055
Beyler C. L., Hirschler M. M. (2002). Thermal decomposition of polymers. SFPE. Handb. fire. Prot. Eng. 2 (7), 111–131.
Bonifacio P. S. P., Metillo E. B., Romano E. F. (2022). Microplastic in sediments and ingestion rates in three edible bivalve mollusc species in a Southern Philippine estuary. Water. Air. Soil pollut. 233 (11), 455. doi: 10.1007/s11270-022-05926-w
Braaten H. F. V., Karlsson M., Nordbø A. J., Hurley R., O’Neill C., Jaya R. K., et al. (2021). Plastic pollution in Indonesia and the Philippines: current status and upcoming knowledge needs. NIVA-rapport.
Brennecke D., Duarte B., Paiva F., Caçador I., Canning-Clode J. (2016). Microplastics as vector for heavy metal contamination from the marine environment. Estuarine. Coast. Shelf. Sci. 178, 189–195. doi: 10.1016/j.ecss.2015.12.003
Bridson J. H., Patel M., Lewis A., Gaw S., Parker K. (2020). Microplastic contamination in Auckland (New Zealand) beach sediments. Mar. pollut. Bull. 151, 110867. doi: 10.1016/j.marpolbul.2019.110867
Bucol L. A., Romano E. F., Cabcaban S. M., Siplon L. M. D., Madrid G. C., Bucol A. A., et al. (2020). Microplastics in marine sediments and rabbitfish (Siganus fuscescens) from selected coastal areas of Negros Oriental, Philippines. Mar. pollut. Bull. 150, 110685. doi: 10.1016/j.marpolbul.2019.110685
Cadiou J.-F., Gerigny O., Koren, Zeri C., Kaberi H., Alomar C., et al. (2020). Lessons learned from an intercalibration exercise on the quantification and characterisation of microplastic particles in sediment and water samples. Mar. pollut. Bull. 154, 111097. doi: 10.1016/j.marpolbul.2020.111097
Campanale, Massarelli, Savino, Locaputo, Uricchio (2020). A detailed review study on potential effects of microplastics and additives of concern on human health. Int. J. Environ. Res. Public Health 17 (4), 1212. doi: 10.3390/ijerph17041212
H Cantwell (2019) Blanks in Method Validation – Supplement to Eurachem Guide The Fitness for Purpose of Analytical Methods. Available at: https://eurachem.org.
Carpenter E. J., Smith K. L. (1972). Plastics on the sargasso sea surface. Science 175 (4027), 1240–1241. doi: 10.1126/science.175.4027.1240
Cashman M. A., Ho K. T., Boving T. B., Russo S., Robinson S., Burgess R. M. (2020). Comparison of microplastic isolation and extraction procedures from marine sediments. Mar. pollut. Bull. 159, 111507. doi: 10.1016/j.marpolbul.2020.111507
Castro L. J. A., Monsada A. M., Cruz K. D. (2021). Occurrence of microplastics in the sediments of Baseco Port area at Manila Bay, Philippines. IOP. Conf. Series.: Earth Environ. Sci. 958 (1), 12009. doi: 10.1088/1755-1315/958/1/012009
Catarino A. I., Thompson R., Sanderson W., Henry T. B. (2017). Development and optimization of a standard method for extraction of microplastics in mussels by enzyme digestion of soft tissues. Environ. Toxicol. Chem. 36 (4), 947–951. doi: 10.1002/etc.3608
Constant M., Billon G., Breton N., Alary C. (2021). Extraction of microplastics from sediment matrices: Experimental Comparative Analysis. J. Hazardous. Mater. 420, 126571. doi: 10.1016/j.jhazmat.2021.126571
Constant M., Kerhervé P., Mino-Vercellio-Verollet M., Dumontier M., Sànchez Vidal A., Canals M., et al. (2019). Beached microplastics in the northwestern Mediterranean Sea. Mar. pollut. Bull. 142, 263–273. doi: 10.1016/j.marpolbul.2019.03.032
Coppock R. L., Cole M., Lindeque P. K., Queirós A. M., Galloway T. S. (2017). A small-scale, portable method for extracting microplastics from marine sediments. Environ. pollut. 230, 829–837. doi: 10.1016/j.envpol.2017.07.017
Crichton E. M., Noël M., Gies E. A., Ross P. S. (2017). A novel, density-independent and FTIR-compatible approach for the rapid extraction of microplastics from aquatic sediments. Analytical. Methods 9 (9), 1419–1428. doi: 10.1039/C6AY02733D
Curren E., Kuwahara V. S., Yoshida T., Leong S. C. (2021). Marine microplastics in the ASEAN Region: A review of the current state of knowledge. Environ. pollut. 288, 117776. doi: 10.1016/j.envpol.2021.117776
Cutroneo L., Reboa A., Geneselli I., Capello M. (2021). Considerations on salts used for density separation in the extraction of microplastics from sediments. Mar. pollut. Bull. 166, 112216. doi: 10.1016/j.marpolbul.2021.112216
Dawson A. L., Santana M. F. M., Nelis J. L. D., Motti C. A. (2023). Taking control of microplastics data: A comparison of control and blank data correction methods. J. Hazardous. Mater. 443, 130218. doi: 10.1016/j.jhazmat.2022.130218
Department of Environment and Natural Resources – Environmental Management Bureau (2021) Philippine National Plan of Action for the Prevention, Reduction, and Management of Marine Litter. Available at: https://nswmc.emb.gov.ph.
Desforges J.-P. W., Galbraith M., Ross P. S. (2015). Ingestion of microplastics by zooplankton in the northeast pacific ocean. Arch. Environ. Contamination. Toxicol. 69 (3), 320–330. doi: 10.1007/s00244-015-0172-5
Ding J., Jiang F., Li J., Wang Z., Sun C., Wang Z., et al. (2019). Microplastics in the coral reef systems from xisha islands of south China sea. Environ. Sci. Technol. 53 (14), 8036–8046. doi: 10.1021/acs.est.9b01452
Dümichen E., Eisentraut P., Bannick C. G., Barthel A.-K., Senz R., Braun U. (2017). Fast identification of microplastics in complex environmental samples by a thermal degradation method. Chemosphere 174, 572–584. doi: 10.1016/j.chemosphere.2017.02.010
Dyachenko A., Mitchell J., Arsem N. (2017). Extraction and identification of microplastic particles from secondary wastewater treatment plant (WWTP) effluent. Analytical. Methods 9 (9), 1412–1418. doi: 10.1039/C6AY02397E
Enders K., Lenz R., Ivar do Sul J. A., Tagg A. S., Labrenz M. (2020). When every particle matters: A QuEChERS approach to extract microplastics from environmental samples. MethodsX 7, 100784. doi: 10.1016/j.mex.2020.100784
Espiritu E. Q., Dayrit S. S., Coronel A. O., Paz N. C., Ronquillo P. L., Castillo V. G., et al. (2019). Assessment of quantity and quality of microplastics in the sediments, waters, oysters, and selected fish species in key sites along the bombong estuary and the coastal waters of ticalan in San Juan, Batangas. Phil. J. Sci. 148 (4), 789–801.
Esquinas G. G. M. S., Mantala A. P., Atilano M. G., Apugan R. P., Galarpe V. R. K. R. (2020). Physical characterization of litter and microplastic along the urban coast of Cagayan de Oro in Macajalar Bay, Philippines. Mar. pollut. Bull. 154, 111083. doi: 10.1016/j.marpolbul.2020.111083
Franzellitti S., Canesi L., Auguste M., Wathsala R. H. G. R., Fabbri E. (2019). Microplastic exposure and effects in aquatic organisms: A physiological perspective. Environ. Toxicol. Pharmacol. 68, 37–51. doi: 10.1016/j.etap.2019.03.009
Frias J. P. G. L., Otero V., Sobral P. (2014). Evidence of microplastics in samples of zooplankton from Portuguese coastal waters. Mar. Environ. Res. 95, 89–95. doi: 10.1016/j.marenvres.2014.01.001
Gabisa E. W., Gheewala S. H. (2022). Microplastics in ASEAN region countries: A review on current status and perspectives. Mar. pollut. Bull. 184, 114118. doi: 10.1016/j.marpolbul.2022.114118
Gaboy S. M. M., Guihawan J. Q., Leopardas V. E., Bacosa H. P. (2022). Unravelling macroplastic pollution in seagrass beds of Iligan City, Mindanao, Philippines. Mar. pollut. Bull. 185, 114233. doi: 10.1016/j.marpolbul.2022.114233
Galarpe V. R. K. R., Jaraula C. M. B., Paler M. K. O. (2021). The nexus of macroplastic and microplastic research and plastic regulation policies in the Philippines marine coastal environments. Mar. pollut. Bull. 167, 112343. doi: 10.1016/j.marpolbul.2021.112343
Gasperi J., Wright S. L., Dris R., Collard F., Mandin C., Guerrouache M., et al. (2018). Microplastics in air: Are we breathing it in? Curr. Opin. Environ. Sci. Health 1, 1–5. doi: 10.1016/j.coesh.2017.10.002
Guzzetti E., Sureda A., Tejada S., Faggio C. (2018). Microplastic in marine organism: Environmental and toxicological effects. Environ. Toxicol. Pharmacol. 64, 164–171. doi: 10.1016/j.etap.2018.10.009
Hammer Ø., Harper D. A. T., Ryan P. D. (2001). PAST: paleontological statistics software package for education and data analysis. Palaeontol. Electronica. 4 (1), 9pp.
Han X., Lu X., Vogt R. D. (2019). An optimized density-based approach for extracting microplastics from soil and sediment samples. Environ. pollut. 254, 113009. doi: 10.1016/j.envpol.2019.113009
Hartmann N. B., Hüffer T., Thompson R. C., Hassellöv M., Verschoor A., Daugaard A. E., et al. (2019). Are we speaking the same language? Recommendations for a definition and categorization framework for plastic debris. Environ. Sci. Technol. 53 (3), 1039–1047. doi: 10.1021/acs.est.8b05297
Herrera A., Garrido-Amador P., Martínez I., Samper M. D., López-Martínez J., Gómez M., et al. (2018). Novel methodology to isolate microplastics from vegetal-rich samples. Mar. pollut. Bull. 129 (1), 61–69. doi: 10.1016/j.marpolbul.2018.02.015
Hidalgo-Ruz V., Gutow L., Thompson R. C., Thiel M. (2012). Microplastics in the marine environment: A review of the methods used for identification and quantification. Environ. Sci. Technol. 46 (6), 3060–3075. doi: 10.1021/es2031505
Jambeck J. R., Geyer R., Wilcox C., Siegler T. R., Perryman M., Andrady A., et al. (2015). Plastic waste inputs from land into the ocean. Science 347 (6223), 768–771. doi: 10.1126/science.1260352
Johnson A. C., Ball H., Cross R., Horton A. A., Jürgens M. D., Read D. S., et al. (2020). Identification and quantification of microplastics in potable water and their sources within water treatment works in england and wales. Environ. Sci. Technol. 54 (19), 12326–12334. doi: 10.1021/acs.est.0c03211
Kalnasa M. L., Lantaca S. M. O., Boter L. C., Flores G. J. T., Galarpe V. R. K. R. (2019). Occurrence of surface sand microplastic and litter in Macajalar Bay, Philippines. Mar. pollut. Bull. 149, 110521. doi: 10.1016/j.marpolbul.2019.110521
Karlsson T. M., Vethaak A. D., Almroth B. C., Ariese F., van Velzen M., Hassellöv M., et al. (2017). Screening for microplastics in sediment, water, marine invertebrates and fish: Method development and microplastic accumulation. Mar. pollut. Bull. 122 (1–2), 403–408. doi: 10.1016/j.marpolbul.2017.06.081
Kirstein I. V., Kirmizi S., Wichels A., Garin-Fernandez A., Erler R., Löder M., et al. (2016). Dangerous hitchhikers? Evidence for potentially pathogenic Vibrio spp. on microplastic particles. Mar. Environ. Res. 120, 1–8. doi: 10.1016/j.marenvres.2016.07.004
Kotar S., McNeish R., Murphy-Hagan C., Renick V., Lee C.-F. T., Steele C., et al. (2022). Quantitative assessment of visual microscopy as a tool for microplastic research: Recommendations for improving methods and reporting. Chemosphere 308, 136449. doi: 10.1016/j.chemosphere.2022.136449
Kukkola A. T., Senior G., Maes T., Silburn B., Bakir A., Kröger S., et al. (2022). A large-scale study of microplastic abundance in sediment cores from the UK continental shelf and slope. Mar. pollut. Bull. 178, 113554. doi: 10.1016/j.marpolbul.2022.113554
Law K. L., Starr N., Siegler T. R., Jambeck J. R., Mallos N. J., Leonard G. H. (2020). The United States’ contribution of plastic waste to land and ocean. Sci. Adv. 6 (44), eabd0288. doi: 10.1126/sciadv.abd0288
Lechthaler S., Hildebrandt L., Stauch G., Schüttrumpf H. (2020). Canola oil extraction in conjunction with a plastic free separation unit optimises microplastics monitoring in water and sediment. Analytical. Methods 12 (42), 5128–5139. doi: 10.1039/D0AY01574A
Li Y., Wang X., Fu W., Xia X., Liu C., Min J., et al. (2019). Interactions between nano/micro plastics and suspended sediment in water: Implications on aggregation and settling. Water Res. 161, 486–495. doi: 10.1016/j.watres.2019.06.018
Lusher A. L., Tirelli V., O’Connor I., Officer R. (2015). Microplastics in Arctic polar waters: The first reported values of particles in surface and sub-surface samples. Sci. Rep. 5 (1), 14947. doi: 10.1038/srep14947
MacLeod M., Arp H. P. H., Tekman M. B., Jahnke A. (2021). The global threat from plastic pollution. Science 373 (6550), 61–65. doi: 10.1126/science.abg5433
Masura J., Baker J., Foster G., Arthur C., Herring C. (2015). Laboratory Methods for the Analysis of Microplastics in the Marine Environment: Recommendations for quantifying synthetic particles in waters and sediments (NOAA Technical Memorandum NOS-OR&R-48).
Mathalon A., Hill P. (2014). Microplastic fibers in the intertidal ecosystem surrounding Halifax Harbor, nova scotia. Mar. pollut. Bull. 81 (1), 69–79. doi: 10.1016/j.marpolbul.2014.02.018
Meijer L. J. J., van Emmerik T., van der Ent R., Schmidt C., Lebreton L. (2021). More than 1000 rivers account for 80% of global riverine plastic emissions into the ocean. Sci. Adv. 7 (18), eaaz5803. doi: 10.1126/sciadv.aaz5803
Mhiret Gela S., Aragaw T. A. (2022). Abundance and characterization of microplastics in main urban ditches across the Bahir Dar City, Ethiopia. Front. Environ. Sci. 10. doi: 10.3389/fenvs.2022.831417
Miller M. E., Kroon F. J., Motti C. A. (2017). Recovering microplastics from marine samples: A review of current practices. Mar. pollut. Bull. 123 (1–2), 6–18. doi: 10.1016/j.marpolbul.2017.08.058
MoEFCC (2023). “G20 Report on Actions Against Marine Plastic Litter,” in Fifth Information Sharing Based on the G20 Implementation Framework, 1st Edition (New Delhi, India: Ministry of Environment, Forest and Climate Change), 575 pp.
Nakajima R., Tsuchiya M., Lindsay D. J., Kitahashi T., Fujikura K., Fukushima T. (2019). A new small device made of glass for separating microplastics from marine and freshwater sediments. PeerJ 7, e7915. doi: 10.7717/peerj.7915
Navarro C. K. P., Arcadio C. G. L. A., Similatan K. M., Inocente S. A. T., Banda M. H. T., Capangpangan R. Y., et al. (2022). Unraveling microplastic pollution in mangrove sediments of Butuan bay, Philippines. Sustainability 14 (21), 14469. doi: 10.3390/su142114469
Nel H., Krause S., Sambrook Smith G. H., Lynch I. (2019). Simple yet effective modifications to the operation of the Sediment Isolation Microplastic unit to avoid polyvinyl chloride (PVC) contamination. MethodsX 6, 2656–2661. doi: 10.1016/j.mex.2019.11.007
Nelms S. E., Galloway T. S., Godley B. J., Jarvis D. S., Lindeque P. K. (2018). Investigating microplastic trophic transfer in marine top predators. Environ. pollut. 238, 999–1007. doi: 10.1016/j.envpol.2018.02.016
Ni B.-J., Zhu Z.-R., Li W.-H., Yan X., Wei W., Xu Q., et al. (2020). Microplastics mitigation in sewage sludge through pyrolysis: the role of pyrolysis temperature. Environ. Sci. Technol. Lett. 7 (12), 961–967. doi: 10.1021/acs.estlett.0c00740
Nuelle M.-T., Dekiff J. H., Remy D., Fries E. (2014). A new analytical approach for monitoring microplastics in marine sediments. Environmental Pollution. 184, 161–169. doi: 10.1016/j.envpol.2013.07.027
Omeyer L. C. M., Duncan E. M., Aiemsomboon K., Beaumont N., Bureekul S., Cao B., et al. (2022). Priorities to inform research on marine plastic pollution in Southeast Asia. Sci. Total. Environ. 841, 156704. doi: 10.1016/j.scitotenv.2022.156704
Osorio E. D., Tanchuling M. A., Diola M. (2021). Microplastics occurrence in surface waters and sediments in five river mouths of Manila Bay. Front. Environ. Sci. 9. doi: 10.3389/fenvs.2021.719274
Padervand M., Lichtfouse E., Robert D., Wang C. (2020). Removal of microplastics from the environment. A review. Environ. Chem. Lett. 18 (3), 807–828. doi: 10.1007/s10311-020-00983-1
Paler Ma. K.O., Malenab Ma. C.T., Maralit J. R., Nacorda H. M. (2019). Plastic waste occurrence on a beach off southwestern Luzon, Philippines. Mar. pollut. Bull. 141, 416–419. doi: 10.1016/j.marpolbul.2019.02.006
Pashaei R., Sabaliauskaitė V., Suzdalev S., Balčiūnas A., Putna-Nimane I., Rees R. M., et al. (2023). Assessing the occurrence and distribution of microplastics in surface freshwater and wastewaters of Latvia and Lithuania. Toxics 11 (4), 292. doi: 10.3390/toxics11040292
Piccardo M., Priami G. S., Anselmi S., Bevilacqua S., Renzi M. (2022). Intra-laboratory calibration exercise for quantification of microplastic particles in fine-grained sediment samples: Special focus on the influence of User Experience. Microplastics 1 (3), 440–455. doi: 10.3390/microplastics1030032
PlastiCount Pilipinas (2022) PlastiCount Pilipinas. Available at: https://plasticount.ph (Accessed February 10, 2023).
Prata J. C., da Costa J. P., Duarte A. C., Rocha-Santos T. (2019a). Methods for sampling and detection of microplastics in water and sediment: A critical review. TrAC. Trends Analytical. Chem. 110, 150–159. doi: 10.1016/j.trac.2018.10.029
Prata J. C., da Costa J. P., Girão A. V., Lopes I., Duarte A. C., Rocha-Santos T. (2019b). Identifying a quick and efficient method of removing organic matter without damaging microplastic samples. Sci. Total. Environ. 686, 131–139. doi: 10.1016/j.scitotenv.2019.05.456
Prata J. C., Reis V., da Costa J. P., Mouneyrac C., Duarte A. C., Rocha-Santos T. (2021). Contamination issues as a challenge in quality control and quality assurance in microplastics analytics. J. Hazardous. Mater. 403, 123660. doi: 10.1016/j.jhazmat.2020.123660
Primpke S., Booth A. M., Gerdts G., Gomiero A., Kögel T., Lusher A., et al. (2022). Monitoring of microplastic pollution in the Arctic: Recent developments in polymer identification, quality assurance and control, and data reporting. Arctic. Sci., as–2022-0006. doi: 10.1139/as-2022-0006
Quinn B., Murphy F., Ewins C. (2017). Validation of density separation for the rapid recovery of microplastics from sediment. Analytical. Methods 9 (9), 1491–1498. doi: 10.1039/C6AY02542K
Rocha-Santos T., Duarte A. C. (2015). A critical overview of the analytical approaches to the occurrence, the fate and the behavior of microplastics in the environment. TrAC. Trends Analytical. Chem. 65, 47–53. doi: 10.1016/j.trac.2014.10.011
Rochman C. M. (2018). Microplastics research—from sink to source. Science 360 (6384), 28–29. doi: 10.1126/science.aar7734
Rochman C. M., Regan F., Thompson R. C. (2017). On the harmonization of methods for measuring the occurrence, fate and effects of microplastics. Analytical. Methods 9 (9), 1324–1325. doi: 10.1039/C7AY90014G
Romarate R. I. A., Ancla S. M. B., Patilan D. M. M., Inocente S. A. T., Pacilan C. J. M., Sinco A. L., et al. (2022). Breathing plastics in Metro Manila, Philippines: Presence of suspended atmospheric microplastics in ambient air. doi: 10.21203/rs.3.rs-1751821/v1
Ryan P. G. (2015). “A brief history of marine litter research,” in Marine anthropogenic litter. Eds. Bergmann M., Gutow L., Klages M. (Springer International Publishing), 1–25. doi: 10.1007/978-3-319-16510-3_1
Sajorne R. E., Cayabo G. D. B., Gajardo L. J. A., Mabuhay-Omar J. A., Creencia L. A., Bacosa H. P. (2022). Disentangling microplastic pollution on beach sand of Puerto Princesa, Palawan Island, Philippines: abundance and characteristics. Sustainability 14 (22), 15303. doi: 10.3390/su142215303
Scheurer M., Bigalke M. (2018). Microplastics in Swiss floodplain soils. Environ. Sci. Technol. 52 (6), 3591–3598. doi: 10.1021/acs.est.7b06003
Schlitzer R. (2023). Ocean data view. Available at: https://odv.awi.de.
Schütze B., Thomas D., Kraft M., Brunotte J., Kreuzig R. (2022). Comparison of different salt solutions for density separation of conventional and biodegradable microplastic from solid sample matrices. Environ. Sci. pollut. Res. 29 (54), 81452–81467. doi: 10.1007/s11356-022-21474-6
Sequeira I. F., Prata J. C., da Costa J. P., Duarte A. C., Rocha-Santos T. (2020). Worldwide contamination of fish with microplastics: A brief global overview. Mar. pollut. Bull. 160, 111681. doi: 10.1016/j.marpolbul.2020.111681
Setälä O., Fleming-Lehtinen V., Lehtiniemi M. (2014). Ingestion and transfer of microplastics in the planktonic food web. Environ. pollut. 185, 77–83. doi: 10.1016/j.envpol.2013.10.013
Sharma M. D., Elanjickal A. I., Mankar J. S., Krupadam R. J. (2020). Assessment of cancer risk of microplastics enriched with polycyclic aromatic hydrocarbons. J. Hazardous. Mater. 398, 122994. doi: 10.1016/j.jhazmat.2020.122994
Shim W. J., Song Y. K., Hong S. H., Jang M. (2016). Identification and quantification of microplastics using Nile Red staining. Mar. pollut. Bull. 113 (1–2), 469–476. doi: 10.1016/j.marpolbul.2016.10.049
Sigler M. (2014). The effects of plastic pollution on aquatic wildlife: current situations and future solutions. Water. Air. Soil pollut. 225 (11), 2184. doi: 10.1007/s11270-014-2184-6
Silva A. B., Bastos A. S., Justino C. I. L., da Costa J. P., Duarte A. C., Rocha-Santos T. A. P. (2018). Microplastics in the environment: Challenges in analytical chemistry - A review. Analytica. Chim. Acta 1017, 1–19. doi: 10.1016/j.aca.2018.02.043
Thompson R. C., Olsen Y., Mitchell R. P., Davis A., Rowland S. J., John A. W. G., et al. (2004). Lost at sea: where is all the plastic? Science 304 (5672), 838–838. doi: 10.1126/science.1094559
Tien C.-J., Wang Z.-X., Chen C. S. (2020). Microplastics in water, sediment and fish from the Fengshan River system: Relationship to aquatic factors and accumulation of polycyclic aromatic hydrocarbons by fish. Environ. pollut. 265, 114962. doi: 10.1016/j.envpol.2020.114962
Tsangaris C., Panti C., Compa M., Pedà C., Digka N., Baini M., et al. (2021). Interlaboratory comparison of microplastic extraction methods from marine biota tissues: A harmonization exercise of the Plastic Busters MPAs project. Mar. pollut. Bull. 164, 111992. doi: 10.1016/j.marpolbul.2021.111992
United Nations Environment Assembly resolution 5/14 (2022). End plastic pollution: towards an international legally binding instrument (UNEA/RES/5/14). Available at: https://undocs.org/en/UNEP/EA.5/Res.14.
Van Emmerik T., Schwarz A. (2019). Plastic debris in rivers. WIREs. Water 7 (1). doi: 10.1002/wat2.1398102
Walker T. R. (2021). (Micro)plastics and the UN sustainable development goals. Curr. Opin. Green Sustain. Chem. 30, 100497. doi: 10.1016/j.cogsc.2021.100497
Waller C. L., Griffiths H. J., Waluda C. M., Thorpe S. E., Loaiza I., Moreno B., et al. (2017). Microplastics in the Antarctic marine system: An emerging area of research. Sci. Total. Environ. 598, 220–227. doi: 10.1016/j.scitotenv.2017.03.283
Way C., Hudson M. D., Williams I. D., Langley G. J. (2022). Evidence of underestimation in microplastic research: A meta-analysis of recovery rate studies. Sci. Total. Environ. 805, 150227. doi: 10.1016/j.scitotenv.2021.150227
Keywords: microplastics, Southeast Asia, beach sediments, harmonization, Philippines
Citation: Bonita JDP, Gomez NCF and Onda DFL (2023) Assessing the efficiency of microplastics extraction methods for tropical beach sediments and matrix preparation for experimental controls. Front. Mar. Sci. 10:1285041. doi: 10.3389/fmars.2023.1285041
Received: 29 August 2023; Accepted: 16 October 2023;
Published: 03 November 2023.
Edited by:
Ilaria Corsi, University of Siena, ItalyCopyright © 2023 Bonita, Gomez and Onda. This is an open-access article distributed under the terms of the Creative Commons Attribution License (CC BY). The use, distribution or reproduction in other forums is permitted, provided the original author(s) and the copyright owner(s) are credited and that the original publication in this journal is cited, in accordance with accepted academic practice. No use, distribution or reproduction is permitted which does not comply with these terms.
*Correspondence: Deo Florence L. Onda, ZGZvbmRhQG1zaS51cGQuZWR1LnBo