Commentary: Demographic response of osprey within the lower Chesapeake Bay to fluctuations in menhaden stock
- 1Center for Conservation Biology, William & Mary, Williamsburg, VA, United States
- 2Department of Biology, William & Mary, Williamsburg, VA, United States
Forage fish support the largest fisheries in the world and play a vital role in marine food webs by transferring energy from plankton to consumers within higher trophic levels. Growing commercial demand for these species and concern for the impact of over harvest on predator populations has driven a paradigm shift in management objectives from maximizing economic return to the establishment of ecosystem-based limits on harvest rates. How well current harvest policy supports noncommercial species like piscivorous birds remains poorly understood. We investigated the relationship between osprey breeding performance within the lower Chesapeake Bay and a menhaden stock index during a period (1974-2021) when the menhaden index fluctuated over 35-fold. Reproductive rate (young/pair/year), brood provisioning (fish/10-h) and the proportion of menhaden in the diet all declined during the study period. Indicators of food stress including brood reduction and nest failure increased during the study period. The population transitioned from reproductive surplus (demographic source) to reproductive deficit (demographic sink). A significant relationship between reproductive rate and the menhaden index suggests that osprey population viability requires that the menhaden stock be restored to 1980s levels. Current ecological reference points based on the food requirements of predatory fish are unlikely to protect the osprey population. We suggest the establishment of menhaden or reproductive thresholds designed to allow osprey to meet demographic targets (1.15 young/pair/year).
1 Introduction
Forage fish are small pelagic schooling species (e.g. anchovy, sardine, capelin, herring, menhaden) that support the largest fisheries in the world by weight and play a significant role in marine ecosystems by transferring energy from plankton to upper trophic-level predators (Furness, 1982; Crawford et al., 2008; Pikitch et al., 2012). Forage fish account for 30% of global landings (17 million metric tons-yr) and demand for the protein and oils produced from these fish continues to rise, placing increasing pressure to harvest more (Alder et al., 2008; Food and Agriculture Organization [FOA], 2012; Pikitch et al., 2014). These fish account for $18.7 billion-yr in economic benefit, are important to many cultures and serve significant roles within aquatic ecosystems (Konar et al., 2019; Nissar et al., 2023). Public and scientific concern about the impact of over harvesting forage fish on predators including predatory fish, seabirds, and marine mammals has grown in recent decades (Pikitch et al., 2004; McLeod and Leslie, 2009; Link, 2010; Smith et al., 2011; Pikitch et al., 2018). This concern has driven a paradigm shift in stock management from single-species, maximum-sustained-yield models designed to optimize harvest to ecosystem-based approaches focused on the tradeoffs between commercial interests and the health of the broader ecosystem (Dickey-Collas et al., 2014; Rice and Duplisea, 2014; National Marine Fisheries Service [NMFS], 2016). However, evidence suggests that ecosystem-based estimates of sustainable harvest may still be too high to support many predator populations (Cury et al., 2011; Pikitch et al., 2012).
Atlantic menhaden (Brevoortia tyrannus) is a forage fish found in western Atlantic waters from Nova Scotia to Florida and supports the largest commercial fishery by weight along the east coast of the United States (Ahrenholz, 1991; National Marine Fisheries Service [NMFS], 2019). Most menhaden are landed using purse seines for the reduction fishery where fish are processed into fish meal and oil to be sold for animal feed, pet food, fertilizer, and dietary supplements (Southeast Data Assessment and Review [SEDAR], 2020). Due to the decline of other species, menhaden are also the focus of a growing bait industry where fish are caught by mixed gear, frozen, and sold as bait for use in other commercial and recreational fisheries. In addition to their commercial value, menhaden are forage fish for a community of consumers including predatory fish (Uphoff, 2003; Walter et al., 2003; Scharf et al., 2004), birds (McLean and Byrd, 1991; Viverette et al., 2007; Glass and Watts, 2009) and marine mammals (Smith et al., 2015).
Atlantic menhaden are regulated by the Atlantic States Marine Fisheries Commission (ASMFC) and harvest policy has evolved in recent decades from no management to maximum-sustained-yield to the development of models designed to evaluate the tradeoffs between commercial take and ecosystem services (Anstead et al., 2021). This change in approach has resulted in the establishment of the first harvest cap for the Chesapeake Bay (Atlantic States Marine Fisheries Commission [ASMFC], 2005), a reduction in the initial cap of more than 50% in less than 15 years and a consideration of local depletion policy. However, the state-of-the-art ecosystem-based model currently evaluates the maximum harvest of menhaden that would allow for maximum harvest of striped bass (Morone saxatilis) effectively trading off the yield of a commercially important forage fish against the yield of a commercially important predatory fish (Chagaris et al., 2020). As part of the transition to an ecosystem approach, scientists were tasked with developing ecological reference points that could be used to track tradeoffs between harvest and ecosystem services (Anstead et al., 2021). Reference points are either stock or predator-dependent conditions that facilitate the consideration of ecosystem services in harvest decisions. Noncommercial species are not among the list of ecological reference points designed to evaluate harvest impacts and the relationship between allowable harvest and the viability of these species remains poorly understood.
The osprey is an obligate piscivore (Poole, 1989; Poole et al., 2002) that is considered to be a sensitive indicator of fish stocks (Grove et al., 2009; Henny et al., 2010). Chesapeake Bay supports one of the largest osprey breeding populations in the world (Henny, 1983). The population experienced dramatic declines in the post-World War II era due to reproductive suppression (Truitt, 1969; Kennedy, 1971; Wiemeyer, 1971; Kennedy, 1977) induced by environmental contaminants (Via, 1975; Wiemeyer et al., 1975). The population reached a low of 1,450 breeding pairs by the early 1970s (Henny et al., 1974). During the 1970s and 1980s reproductive rates recovered (Watts and Paxton, 2007) and the population doubled in size by 1995 (Watts et al., 2004). The rate of recovery varied over an order of magnitude between geographic areas of the Bay related to the depth of the earlier decline and likely variation in prey availability (Watts and Paxton, 2007). Ospreys within portions of the Chesapeake are believed to be menhaden-dependent (McLean and Byrd, 1991). However, the demographic and dietary response of osprey within these areas to fluctuations in menhaden availability has not been evaluated.
Here we examine osprey reproductive performance, brood provisioning and diet over a period (1974-2021) during which menhaden stock fluctuated over 35-fold. We assess the relationship between menhaden stock and the demographic viability of osprey with respect to current harvest policy.
2 Methods
This study uses data from four generations of graduate students (Stinson, 1976; McLean, 1986; Glass, 2007; Academia, 2022) who studied different aspects of osprey foraging and nesting ecology within the study area (1974-2021). Although the objectives of these studies differed somewhat, there is a common methodology and set of data allowing for broad comparisons across time periods. The studies included six field seasons of osprey observations including 1974-1975 (Stinson, 1976), 1985 (McLean, 1986), 2006-2007 (Glass, 2007) and 2021 (Academia, 2022).
2.1 Study area
We conducted fieldwork with osprey within Mobjack Bay and vicinity. Mobjack Bay is a broad (186 km2) sub estuary of the lower Chesapeake Bay (Figure 1) that is formed by the convergence of four rivers (Severn, Ware, North, and East) all arising within the Coastal Plain of Virginia. Waters are polyhaline (18-30 ppt) and microtidal (amplitude < 1 m) with two tide cycles-d. The osprey population within the study area reached a low of 15 breeding pairs by the early 1970s (Kennedy, 1971), had recovered to 87 pairs by 1995 (Watts et al., 2004) and now includes approximately 100 breeding pairs (Watts, unpublished data). The osprey population nesting within the Chesapeake Bay is migratory. Osprey return to breeding territories from South America in early to mid-March, lay clutches in early April and fledge young from mid-June through late-July (Stinson, 1976). Within the study area osprey primarily nest on navigational aids, offshore duck blinds, boat houses, abandoned docks and nesting platforms erected by citizens.
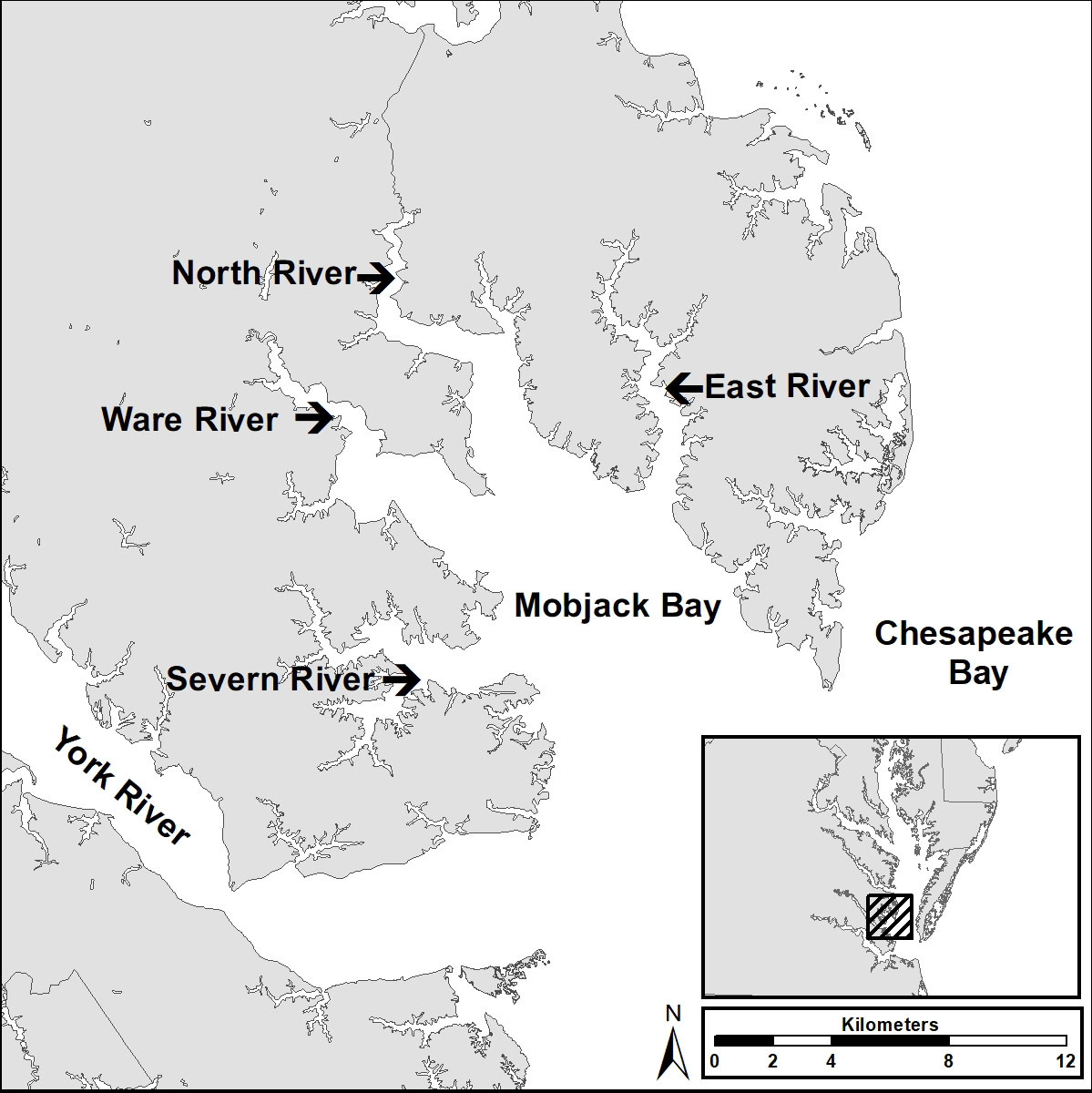
Figure 1 Map of the Mobjack Bay study area used to study osprey breeding performance, provisioning and diet within the lower Chesapeake Bay (1974-2021).
2.2 Osprey demography
The osprey is a long-lived species with associated high adult survival and relatively high reproductive potential (Poole et al., 2002). Annual adult survival has been shown to vary from 80 to 90% across populations (e.g., Henny and Wight, 1969; Spitzer et al., 1983; Postupalsky, 1989; Ryttmann, 1994; Wahl and Barbraud, 2014) with survival during the first year consistently lower and ranging from 40 to 60% (e.g., Henny and Wight, 1969; Wahl and Barbraud, 2014). Osprey exhibit delayed onset to reproduction. For growing populations, recruitment into the breeding population typically occurs during the fourth year with a smaller number entering the population during their third or fifth years (Kinkead, 1985). Age-at-first reproduction increases as populations reach carrying capacity (Spitzer, 1980; Poole, 1989). For the Chesapeake Bay, 1.15 (young/breeding pair/year) is the reproductive rate estimated to be required to replace adult mortality (Poole, 1989). The break-even rate likely fluctuates as the population of floaters (birds of breeding age that do not hold territories) expands and contracts through time. A reproductive rate above this range (demographic source) allows the population to grow to capacity or stabilize at capacity as the floater population expands. A reproductive rate below this range (demographic sink) is not sustainable and if maintained will result in a population decline in the absence of immigration.
We monitored focal osprey nests (N = 75, 68, 132, 68 for periods 1974-75, 1985, 2006-07 and 2021 respectively) weekly during the nestling period by boat to determine breeding performance. Each nest check used a mirror pole to examine nest contents in order to determine the number of eggs and young present. Weekly visits allowed us to determine clutch size and fledging rate. We considered a nest to be active if a breeding attempt was documented (eggs or young observed). We considered young that reached six weeks of age to be of near fledging age. We considered nests to be successful if they produced at least one young that survived to six weeks of age. We considered annual reproductive rate to be the mean number of young produced per active breeding pair. We consider brood reduction to be the difference between the number of young hatched and the number of young surviving to fledging age. We used a set of focal nests (N = 8, 7, 8, 13 for periods 1974-75, 1985, 2006-07, and 2021 respectively) during each period to quantify brood reduction.
2.3 Provisioning rate and diet
We observed osprey nests during the nestling period (May – July) to estimate fish delivery rates. Provisioning rate and diet were quantified using focal nests as outlined above during each time period. During the 1970s and 1980s we observed offshore nests (mean distance = 50 m) with binoculars and spotting scopes. In later years we used digital video systems mounted 1 m above the nest to estimate fish delivery rates. During all years, observations were made from dawn to dusk. All fish delivered to nests were recorded. During all periods except the 1970s an effort was made to identify delivered fish to species.
2.4 Male time budget
During the nestling period, male ospreys alternate between making hunting forays to provision young and perching on the nest or favored perches near the nest site. During the 1970s and 1980s, we quantified time budgets by recording the amount of time males perched near the nest. We used the time away from the nest as an estimate of hunting activity. This may overestimate actual hunting time.
2.5 Menhaden stock assessment
We used a hierarchical stock index to evaluate the relationship between menhaden abundance and osprey nesting parameters over the study period. No menhaden stock index has been developed that is specific to the Mobjack Bay study area. Twenty-four fishery-independent menhaden surveys are conducted along the Atlantic Coast (Southeast Data Assessment and Review [SEDAR], 2022). These surveys vary in objectives, methodology, and year of initiation. The majority of the surveys do not extend back to the 1970s. A hierarchical analysis has been used (Conn, 2010) to merge these efforts into a single index (1959-2021) and provide a posterior mean and confidence interval (Southeast Data Assessment and Review [SEDAR], 2022). We used the young-of-year abundance index (YOY) because it is the only index that fully covers the study period. Osprey use menhaden to provision young that typically range from 10 to 25 cm (mean = 19.1 ± 0.37 SE, N = 253), (Watts et al. unpub.). This size range suggests that osprey are primarily using menhaden in the 2 to 4-year age classes (Schueller et al., 2014). The Mid-Atlantic Adult Menhaden Relative Abundance Index (MAD) does not cover the study period. However, the hierarchical stock index used here is significantly correlated with both the more local Maryland geometric mean catch index (N = 63, Spearman Rank Correlation Coefficient = 0.61, p < 0.05) and the MAD (N = 37, Spearman Coefficient = 0.4, p < 0.05).
2.6 Statistical analysis
We evaluated trends in reproductive (all nests), provisioning and diet (focal nests) parameters across the study period using a one-way ANOVA with period (1974-75, 1985, 2006-07, 2021) as the grouping parameter and nests as samples. We evaluated each parameter for deviations from normality (Kolmogorov-Smirnov test) and homogeneity of variance (Levene test) to test for compliance with ANOVA assumptions. We used Tukey’s honestly significant difference test to make pairwise, post-hoc comparisons between time periods for parameters where ANOVA results were significant. We examined the relationship between period and the proportion of young lost using the Freeman-Halton extension of the Fisher’s exact test for a 2 X 4 contingency table (Freeman and Halton, 1951). We examined the relationship between mean reproductive rate and the Atlantic Menhaden Relative Abundance Index using a simple linear regression. The mean reproductive rate was used because we were interested in the relationship between population-wide performance and menhaden availability.
3 Results
Both the Atlantic menhaden relative abundance index (Figure 2) and osprey reproductive performance (Table 1) declined over the study period. The menhaden index declined from a high of 4.1 in 1980 to 1.6 in 1985 and then remained below 1.0 after 1990 and below 0.5 after 2005. Following the decline during the late 1970s through 1980s, menhaden appear to have reached a state change in the early 1990s and have remained low since that time. Osprey reproductive rates were above maintenance levels in 1975 and 1985 but by 2006 and 2021 productivity was well below that required to sustain the population. For successful nests, brood size fell from 2.0 in 1975 to 1.2 by 2021. The decline in reproductive rates over the study period was due to brood reduction. Clutch size did not differ between the time periods (Table 1). However, the percentage of young lost between hatching and fledging increased significantly (df = 3, χ2 statistic = 10.2, p < 0.01) from 5.3% (1 of 18) to 12.5% (2 of 16) to 75% (18 of 24) to 76% (19 of 25) for 1974-75, 1985, 2006-07 and 2021 respectively.
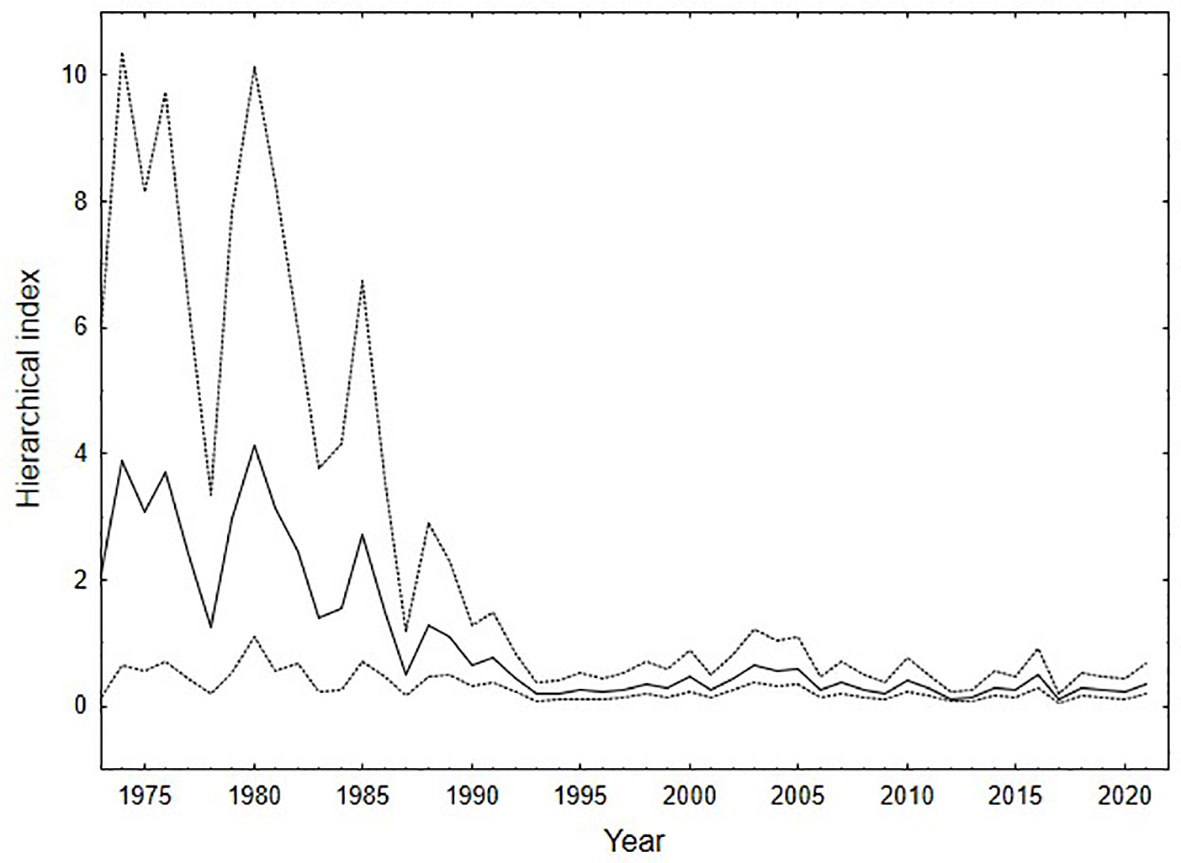
Figure 2 Chronosequence of Atlantic menhaden young of the year abundance index used as a proxy for menhaden availability within the study area. The black line indicates posterior mean and the gray lines represent 95% confidence intervals around the mean.

Table 1 Mean (± standard error) estimates of osprey reproductive rate, clutch size, brood size, nests monitored (N) and one-way ANOVA results from the lower Chesapeake Bay.
Reproductive rate was associated with provisioning rate (Figure 3). Significantly higher fish delivery rates were documented for nests with more young compared with those with fewer young or that failed (N = 36, df = 3, F-statistic = 4.8, p < 0.05). Post-hoc tests revealed that this significant result was driven by differences between nests that raised 3 young compared to those that failed (Tukey’s honestly significant difference, p = 0.03). A significant decline in provisioning rate (fish/10 hr) was observed over the study period (Table 2). An interesting finding is that although provisioning rate declined 34% between 1975 and 1985, the proportion of the time budget that males spent hunting increased from 57.0% to 70.1% suggesting that catch per unit effort declined substantially. Between 1985 and 2021 the mean contribution of menhaden to osprey diet declined significantly from 67.3% to 24.7% to 30.2% for 1985, 2006-07 and 2021 respectively (Table 1) with values for individual nests ranging from 48 to 83% and 12.5 to 45.5% for 1985 and the later years respectively. This change in diet composition resulted in a significant (83.3%) decline in the delivery rate of menhaden to nests (Table 2). Changes in mean reproductive rate coincide with declines in the menhaden index (Figure 4). The response of mean annual reproductive rate for osprey in the study area to menhaden availability (Atlantic Menhaden Relative Abundance Index) was strong and significant (F-statistic = 42.1, df = 1,4, p < 0.01, R2 = 0.91). The form of the relationship (reproductive rate = 0.5289 + (0.3529)(menhaden index)).
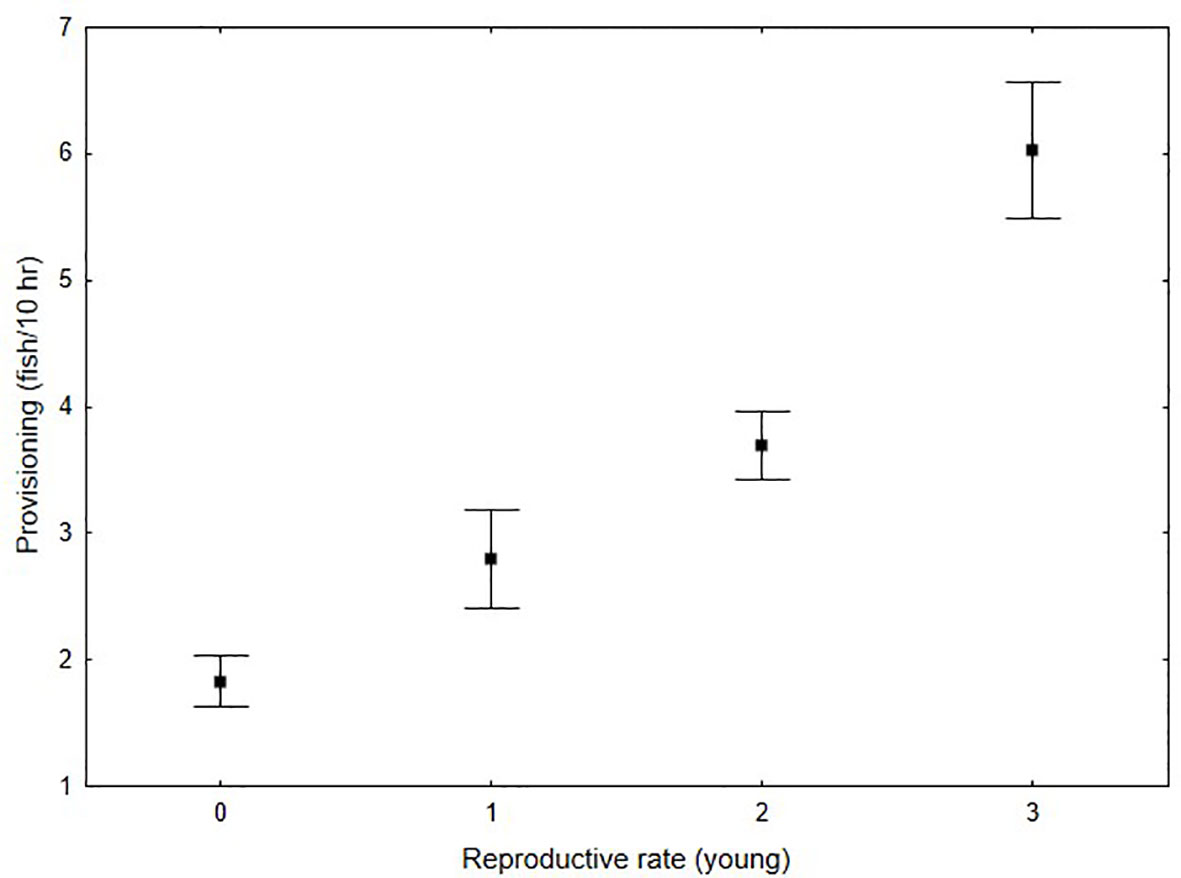
Figure 3 Relationship between mean provisioning rate and brood size (outcome of breeding attempt) for osprey pairs within the lower Chesapeake Bay. Focal nests for all time periods (1974-75, 1985, 2006-07, 2021) were combined. Squares indicate means and error bars represent standard errors around the mean.

Table 2 Mean (± standard error) estimates of osprey reproductive, provisioning and diet parameters, sample sizes (nests) and one-way ANOVA results from the lower Chesapeake Bay.
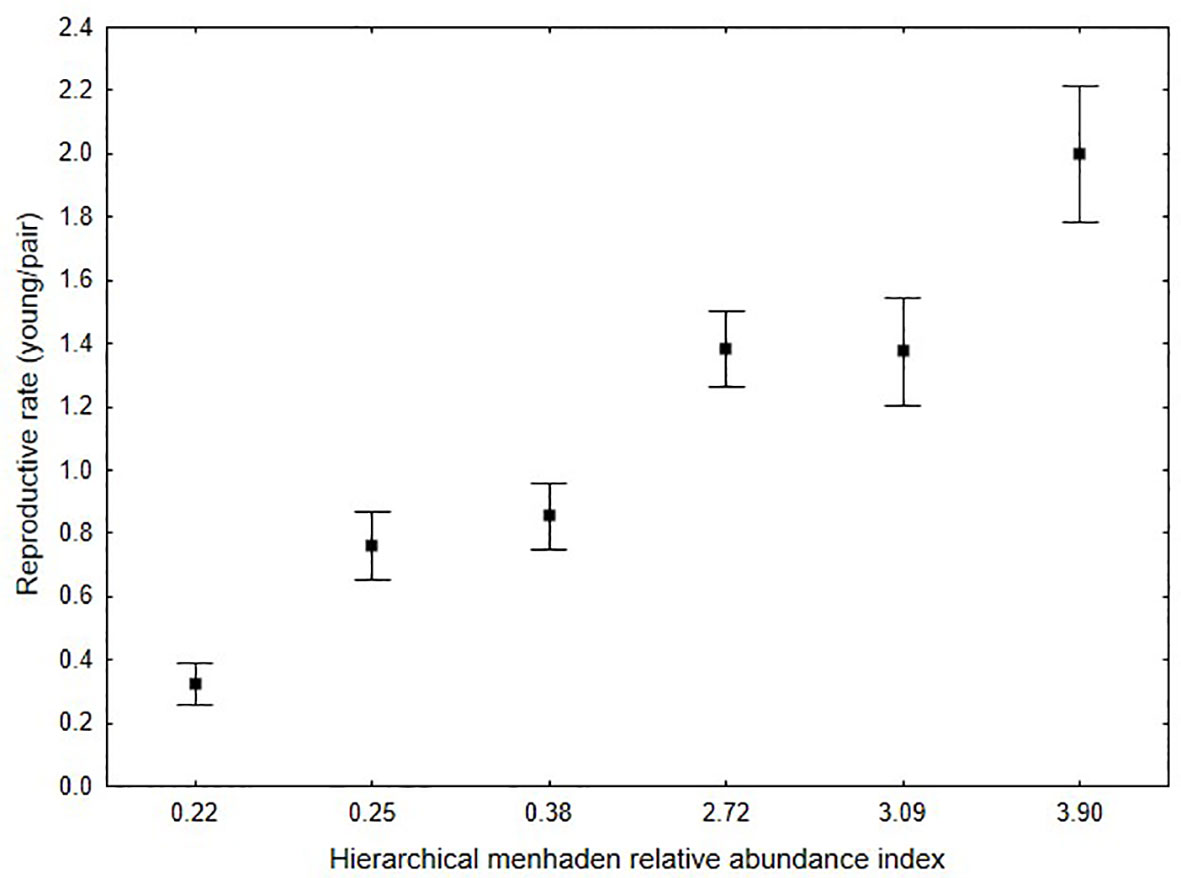
Figure 4 Relationship between mean reproductive rate (all nests monitored for each year) and menhaden stock index for osprey within the lower Chesapeake Bay. Estimated reproductive rate required for a stable population within the Chesapeake Bay is 1.15. Squares indicate means and error bars represent standard errors around the mean.
4 Discussion
The Atlantic menhaden has experienced several boom-and-bust cycles since large-scale harvest was initiated in the 1850s (Anstead et al., 2021). Although data are incomplete, the stock does not appear to recover to prior levels following each bust event resulting in a ratcheting down of the stock through time. The most recent bust event began in the late 1980s and through the 1990s the stock reached historically low levels. This event resulted in a narrowing of the commercial industry, a change in policy on both state and federal levels, and the introduction of an ecosystem-based approach to menhaden management (Anstead et al., 2021; Drew et al., 2021). Throughout this treatment, we have used the Atlantic Menhaden Relative Abundance Index as a proxy for menhaden availability within the study area because no fisheries-independent data exist on this local scale. One of the problems with using a range-wide abundance index is the inherent “masking” or averaging across spatial variation in abundance. We are unable to evaluate the correspondence between local menhaden abundance and the range-wide index. However, osprey nesting observations including a decline in both menhaden delivery rates and the importance of menhaden in the diet suggest that the trend within the study area has been consistent with the range-wide index.
Within a relatively short period of time the Mobjack Bay osprey population transitioned from reproductive surplus (demographic source) to reproductive deficit (demographic sink). The population likely crossed this tipping point during the early 1990s coincident with the bust event initiated in the late 1980s. The osprey is a long-lived species and populations are expected to absorb short-term perturbations in prey availability. However, menhaden within the Chesapeake Bay have not experienced a significant recovery back to 1980s levels since this initial decline. Based on internal demographics (local demographics without immigration) the Mobjack Bay osprey population is predicted to have experienced an ongoing decline over the past two decades. The fact that the population remains stable suggests that it is being “rescued” by ongoing immigration from other subpopulations that are producing a reproductive surplus. Subpopulations within lower salinity reaches of the Chesapeake have sustained the most rapid growth in recent decades (Watts et al., 2004; Watts and Paxton, 2007), have some of the highest rates of productivity (Glass, 2007), and could be the source of these immigrants. The combination of reproductive deficit and population stability highlight the fact that population surveys alone are not always the best indicator of local viability (Pulliam, 1988). Spatial variation in forage fish abundance may drive metapopulation dynamics for birds that are central-place foragers and depend on key species (Staudinger et al., 2020). Management strategies for fish stocks should be designed to minimize risks of local depletion in order to protect higher trophic-level consumers.
Food stress and subsequent brood reduction is widespread in ospreys (e.g., Poole, 1982; Eriksson, 1986; Hagan, 1986; Steidl and Griffin, 1991; Machmer and Ydenberg, 1998) and has been shown to lead to reproductive deficits and population decline (Bowman et al., 1989). Although ospreys are capable of catching a wide variety of fish and often have diverse diets (Poole, 1989) populations typically depend on one or two species during the nesting period (Nesbitt, 1974; Eriksson, 1986; Harmata et al., 2007). Osprey within Mobjack Bay appear to be menhaden-dependent. Eighteen fish species have been identified within the diet of osprey during the brooding period within this study area (McLean, 1986; Glass, 2007). However, menhaden accounted for nearly 75% of fish provided to broods by weight and energy content in 1985 (McLean and Byrd, 1991). None of the other species in the diet appear to be alternatives to menhaden in terms of energy density except for American eel (Anguilla rostrata) (McLean, 1986; Glass, 2007). American eels are energy dense but have accounted for <3% of the diet.
It seems plausible that menhaden represent a keystone species for osprey within the lower Chesapeake Bay in representing the only prey species with high energy density that is capable of reaching a population size required to allow osprey to reproduce above maintenance levels. A recent experimental menhaden-addition study within the Mobjack Bay study area demonstrated that an increase in provisioning rate resulted in a significantly higher reproductive rate for treatment nests when compared to control nests (Academia and Watts, 2023). Supplementation of nests with 63.4 g/d of menhaden pushed productivity to sustainable levels while control nests remained below maintenance. The study demonstrated that the pairs required 202.7 g/d of fish or 338.6 kcal/d in order to reach maintenance reproductive rates. Within the historical context presented here, osprey have not achieved these provisioning rates since the 1980s.
The energy demand of the osprey population is not on the scale that would be expected to regulate menhaden numbers. The osprey is a small-time menhaden consumer within the context of the broader Chesapeake Bay ecosystem where populations of predatory fish have the capacity to consume large quantities of menhaden (Uphoff, 2003; Chagaris et al., 2020). Even during the period when menhaden accounted for 75% of the osprey diet, the osprey population would have consumed only 0.004% of the commercial landings (McLean and Byrd, 1991). However, fluctuations in menhaden stock have the capacity to regulate osprey populations within areas where they are the primary prey. The overfishing and ultimate collapse of the menhaden population in southern New England has been implicated in the lack of osprey recovery back to pre-DDT levels within specific locations that were believed to have been heavily menhaden dependent during periods of high population density (Bierregaard et al., 2014).
Ospreys have limited capacity to compensate for low prey availability. Males hunt during the nestling period while females attend to broods (Poole, 1989, Poole et al., 2002). Males provision both the female and nestlings with the female consuming approximately 30% of fish provided (McLean, 1986). Regardless of energy demand male osprey hunt only 60-70% (Stinson, 1976; McLean, 1986) of the day which limits their ability to make adjustments to either high energy demand from broods or low prey densities (Stinson, 1978; Jamieson et al., 1983; Eriksson, 1986). When a male’s capacity to meet energetic needs is inadequate, sibling aggression and subsequent brood reduction is the behavioral mechanism that adjusts brood size to available food (Poole, 1982; Forbes, 1991; Machmer and Ydenberg, 1998). As provisioning declines below the needs of all brood mates, larger, more aggressive siblings monopolize available food leaving subordinates to starve. Continued reductions in provisioning will ultimately lead to nest failure. These behavioral mechanisms link prey availability to population viability. There appears to be an energetic state space where male ospreys operate (Green and Ydenberg, 1994) and, under low prey availability conditions, their ability to reach demographic viability may be constrained.
The relationship between mean reproductive rate and the Atlantic menhaden relative abundance index suggests that reaching the demographic target (1.15 young/pair) required for population maintenance would require an index value of 2.73. This index value has not been realized since 1985 (Figure 2). This finding is consistent with the independent finding from supplementation that suggests that provisioning rates will need to return to 1980s levels in order to reach stability. These findings are also consistent with those reported for 14 seabird species within seven marine ecosystems (Cury et al., 2011). Breeding seabirds that rely on forage fish showed a response to changes in prey abundance and breeding success began to decline when prey fell below 34.6% of the maximum observed prey abundance.
The development of ecological reference points to inform ecosystem-based fisheries management is in its infancy and understandably the current menhaden model and associated reference points focus on predatory fish of commercial interest (Chagaris et al., 2020; Anstead et al., 2021). Striped bass were chosen as a reference because of the assemblage of predatory fish within the ecosystem, they have been shown to be the most sensitive to menhaden (Buchheister et al., 2017). A stated assumption of this approach is that because striped bass are the most sensitive, setting harvest thresholds that consider their requirements will effectively protect predators (including birds) that are less sensitive. However, it seems unlikely that the striped bass “umbrella” will protect bird populations. Osprey likely fell below a critical demographic tipping point in the early 1990s. Striped bass did not exhibit definitive signs of food stress until the late 1990s and continue to be used as an indicator of menhaden to the present (Uphoff, 2003; Anstead et al., 2021). There is a clear mismatch in terms of energetic demand and associated menhaden consumption between predatory fish and piscivorous birds within the Bay. The three dominant fish predators including striped bass, bluefish (Pomatomus saltatrix), and weakfish (Cynoscion regalis) are capable of consuming large portions of menhaden and at times exceed the commercial take (Uphoff, 2003; Chagaris et al., 2020). If birds were added as equally weighted consumers to the ecosystem model their consumption would represent a rounding error in the broader consumption. Because ospreys have little impact on the menhaden population but require threshold menhaden densities in order to sustain a demographic surplus, the development of an ecological reference point based on either a biomass or reproductive rate threshold would be a better strategy for protecting this population. Biomass thresholds are used to ensure that predators have sufficient prey to meet population targets (Pikitch et al., 2012). Such harvest triggers are being used within other fisheries. For example, if breeding performance of the black-legged kittiwake (Rissa tridactula) falls below a predetermined threshold a change in management is triggered for the sandeel (Ammodytes marinus) (International Council for Exploration of the Seas, 2008). Similarly, if the body condition of Antarctic krill predators declines below a set threshold, a reduction in fisheries quota is triggered (Boyd, 2002).
A priority for future osprey-menhaden work within the lower Chesapeake Bay is the development of a catch-per-unit-effort (CPUE) model (prey capture/time spent hunting) for males provisioning broods. Stinson (1976) recorded the duration of male hunting forays and their success but did not record fish species captured. McLean (1986); Glass (2007), and Academia (2022) recorded the delivery rate of menhaden but did not link delivery rates to the duration and success of male hunting events. Development of a CPUE model for menhaden (and other fish species) would contribute to a better understanding of the state space where osprey may meet demographic requirements within the time available for hunting. A CPUE-demographic framework could lead to a simple monitoring program based on male hunting that would inform whether or not stock levels are adequate to sustain a viable osprey population.
Data availability statement
The raw data supporting the conclusions of this article will be made available by the authors, without undue reservation.
Ethics statement
The animal study was approved by Institutional Animal Care and Use Committee. The study was conducted in accordance with the local legislation and institutional requirements.
Author contributions
BW: Conceptualization, Formal analysis, Funding acquisition, Methodology, Project administration, Supervision, Validation, Writing – original draft. CS: Investigation, Writing – review & editing. PM: Investigation, Writing – review & editing. KG: Investigation, Writing – review & editing. MA: Formal analysis, Methodology, Validation, Writing – review & editing. MB: Investigation, Methodology, Supervision, Writing – review & editing.
Funding
The author(s) declare financial support was received for the research, authorship, and/or publication of this article. Funding for field projects was provided by the Virginia Department of Wildlife Resources, William & Mary, the Center for Conservation Biology, the International Osprey Foundation, the Virginia Society of Ornithology, the Williamsburg Bird Club and the Coastal Virginia Wildlife Observatory.
Acknowledgments
We thank all of the landowners who allowed access to their properties and osprey pairs, the field assistants who helped with field logistics and data collection and the graduate committee members who helped to guide research projects.
Conflict of interest
The authors declare that the research was conducted in the absence of any commercial or financial relationships that could be construed as a potential conflict of interest.
Publisher’s note
All claims expressed in this article are solely those of the authors and do not necessarily represent those of their affiliated organizations, or those of the publisher, the editors and the reviewers. Any product that may be evaluated in this article, or claim that may be made by its manufacturer, is not guaranteed or endorsed by the publisher.
References
Academia M. H. (2022). Food supplementation increases performance of ospreys in the lower Chesapeake Bay (Williamsburg, Virginia, USA: College of William and Mary). M.A. Thesis.
Academia M. H., Watts B. D. (2023). Food supplementation increases reproductive performance of ospreys in the lower Chesapeake Bay. Front. Mar. Sci. 10. doi: 10.3389/fmars.2023.1172787
Ahrenholz D. W. (1991). Population biology and life history of the North American menhadens. Brevoortia spp. Mar. Fisheries Rev. 53, 3–19.
Alder J., Campbell B., Karpouzi V., Kaschner K., Pauly D. (2008). Forage fish: From ecosystems to markets. Annu. Rev. Environ. Resour. 33, 153–166. doi: 10.1146/annurev.environ.33.020807.143204
Anstead K. A., Drew K., Chagaris D., Cieri M., Schueller A. M., McNamee J. E., et al. (2021). The path to an ecosystem approach for forage fish management: A case study of Atlantic menhaden. Front. Mar. Sci. 8. doi: 10.3389/fmars.2021.607657
Atlantic States Marine Fisheries Commission [ASMFC] (2005). Addendum II to Amendment 1 to the Interstate Fishery Management Plan for Atlantic Menhaden (Arlington, VA: Atlantic States Marine Fisheries Commission).
Bierregaard R. O. Jr., David A. B., Gibson L., Kennedy R. S., Poole A. F., Scheibel M. S., et al. (2014). Post-DDT recovery of osprey (Pandion haliaetus) populations in southern New England and Long Island, New York 1970-2013. J. Raptor Res. 48, 361–374. doi: 10.3356/JRR-OSPR-14-04.1
Bowman R., Powell G. V. N., Hovis J. A., Kline N. C., Wilmers T. (1989). Variations in reproductive success between subpopulations of the osprey (Pandion halietus) in south Florida. Bull. Mar. Sci. 44, 245–250.
Boyd I. L. (2002). Integrated environment-prey-predator interactions off South Georgia: implications for management of fisheries. Aquat. Conservation: Mar. Freshw. Ecosyst. 12, 119–126. doi: 10.1002/aqc.481
Buchheister A., Miller T. J., Houde E. D. (2017). Evaluating ecosystem-based reference points for Atlantic Menhaden. Mar. Coast. Fisheries 9, 457–478. doi: 10.1080/19425120.2017.1360420
Chagaris D., Drew K., Schueller A., Cieri M., Brito J., Buchheister A. (2020). Ecological reference points for Atlantic menhaden established using and ecosystem model of intermediate complexity. Front. Mar. Sci. 7. doi: 10.3389/fmars.2020.606417
Conn P. B. (2010). Hierarchical analysis of multiple noisy abundance indices. Can. J. Fisheries Aquat. Sci. 67, 108–120. doi: 10.1139/F09-175
Crawford R. J. M., Underhill L. G., Coetzee J. C., Fairweather T., Shannon L. J., Wolfaardt A. C. (2008). Influences of the abundance and distribution of prey on African penguins Spheniscus demersus off western South Africa. Afr. J. Mar. Sci. 30, 165–175. doi: 10.2989/AJMS.2008.30.1.17.467
Cury P. M., Boyd I. L., Bonhommeau S., Anker-Nilssen T., Crawford R. J. M., Furness R. W., et al. (2011). Global seabird response to forage fish depletion – one-third for the birds. Science 334, 1703–1706. doi: 10.1126/science.1212928
Dickey-Collas M., Engelhard G. H., Rindorf A., Raab K., Smout S., Aarts G., et al. (2014). Ecosystem-based management objectives for the North Sea: riding the forage fish rollercoaster. ICES J. Mar. Sci. 71, 128–142. doi: 10.1093/icesjms/fst075
Drew K., Cieri M., Schueller A. M., Buchheister A., Chagaris D., Nesslage G., et al. (2021). Balancing model complexity, data requirements, and management objectives in developing ecological reference points for Atlantic menhaden. Front. Mar. Sci. 8. doi: 10.3389/fmars.2021.608059
Eriksson M. O. G. (1986). Fish delivery, production of young, and nest density of osprey (Pandion haliaetus) in southwest Sweden. Can. J. Zoology 64, 1961–1965. doi: 10.1139/z86-295
Food and Agriculture Organization [FOA]. (2012). The state of the world fisheries and aquaculture (Italy: Food and Agriculture Organization of the United Nations Fisheries and Agriculture Department. Rome).
Forbes L. S. (1991). Hunger and food allocation among nestlings of facultatively siblicidal ospreys. Behav. Ecol. Sociobiology 29, 189–195. doi: 10.1007/BF00166400
Freeman G. H., Halton J. H. (1951). Note on the exact treatment of contingency, goodness of fit and other problems of significance. Biometrika 38, 141–149. doi: 10.1093/biomet/38.1-2.141
Furness R. W. (1982). Competition between fisheries and seabird communities. Adv. Mar. Biol. 20, 225–3007. doi: 10.1016/S0065-2881(08)60141-8
Glass K. A. (2007). Broad spatial trends in osprey provisioning, reproductive success, and population growth within the lower Chesapeake Bay (Williamsburg, VA, USA: College of William and Mary). M.A. Thesis.
Glass K. A., Watts B. D. (2009). Osprey diet composition and quality in high and low salinity areas of lower Chesapeake Bay. J. Raptor Res. 43, 27–36. doi: 10.3356/JRR-08-22.1
Green D. J., Ydenberg R. C. (1994). Energetic expenditure of male ospreys provisioning natural and manipulated broods. Ardea 82, 249–262.
Grove R. A., Henny C. J., Kaiser J. L. (2009). Osprey: worldwide sentinel for assessing and monitoring environmental contamination in rivers, lakes, reservoirs, and estuaries. J. Toxicol. Environ. Health 12, 15–44. doi: 10.1080/10937400802545078
Hagan J. M. (1986). Temporal patterns in pre-fledging survival and brood reduction in an osprey colony. Condor 88, 200–205. doi: 10.2307/1368916
Harmata P. J., Restani M., Harmata A. R. (2007). Settlement patterns, foraging behavior, and reproductive success of ospreys along a heterogeneous riverine corridor. Can. J. Zoology 85, 56–62. doi: 10.1139/z06-190
Henny C. J., Smith M. M., Stotts V. D. (1974). The 1973 distribution and abundance of breeding Ospreys in the Chesapeake Bay. Chesapeake Science 15, 125–133.
Henny C. J. (1983). “Distribution and abundance of ospreys in the United States,” in Biology and Management of Bald Eagles and Ospreys. Ed. Bird D. M. (Ste. Anne de Bellevue Quebec, CA: Harpell Press), 175–186.
Henny C. J., Grove R. A., Kaiser J. L., Johnson B. L. (2010). North American osprey populations and contaminants: Historic and contemporary perspectives. J. Toxicol. Environ. Health 13, 579–603. doi: 10.1080/10937404.2010.538658
Henny C. J., Wight H. M. (1969). An endangered osprey population: estimates of mortality and production. Auk 86, 188–198. doi: 10.2307/4083495
International Council for Exploration of the Seas (2008). Report of the ICES Advisory Committee 2008 (Denmark: International Council for Exploration of the Sea, Copenhagen).
Jamieson I. G., Seymour N. R., Bancroft R. P., Sullivan R. (1983). Sibling aggression in nestling ospreys in Nova Scotia. Can. J. Zoology 61, 466–469. doi: 10.1139/z83-063
Kennedy R. S. (1971). Population dynamics of Ospreys in Tidewater, Virginia 1970-1971 (Williamsburg, VA. USA: College of William and Mary). M.A. Thesis.
Kennedy R. S. (1977). The status of the osprey in tidewater Virginia 1970- 1971. Proc. North Am. Osprey Res. Conf. U.S. Natl. Park Serv. Trans. Proc. Ser. No. 2, 121–133.
Kinkead T. P. (1985). Age structure and dispersal of Chesapeake Bay ospreys (Williamsburg, VA. USA: College of William and Mary). M.A. Thesis.
Konar M., Qiu S., Tougher B., Vause J., Tlusty M., Fitzsimmons K., et al. (2019). Illustrating the hidden economic, social and ecological values of global forage fish resources. Resources Conserv. Recycling 151, 104456. doi: 10.1016/j.resconrec.2019.104456
Link J. (2010). Ecosystem-Based Fisheries Management: Confronting Tradeoffs (New York: Cambridge University Press).
Machmer M. M., Ydenberg R. C. (1998). The relative roles of hunger and size asymmetry in sibling aggression between nestling ospreys, Pandion haliaetus. Can. J. Zoology 76, 181–186. doi: 10.1139/z97-183
McLean P. K. (1986). The feeding ecology of the Chesapeake Bay Ospreys and the growth and behavior of their young (Williamsburg, VA USA: The College of William and Mary). M.A. Thesis.
McLean P. K., Byrd M. A. (1991). The diet of Chesapeake Bay ospreys and their impact on the local fishery. J. Raptor Res. 25, 109–112.
McLeod K. L., Leslie H. M. (2009). Why ecosystem-based management? In Ecosystem-Based Management for the Oceans. Eds. McLeod K., Leslie H. (Washington, D.C: Island Press), 1–12.
National Marine Fisheries Service [NMFS]. (2016). Ecosystem-based Fisheries Management Policy of the National Marine Fisheries Service National Oceanic and Atmospheric Administration NMFS Policy Directive 01-120 (NC, U.S.A: Beaufort).
National Marine Fisheries Service [NMFS]. (2019). Forecast for the 2019 Gulf and Atlantic Menhaden Purse-Seine Fisheries and Review of the 2018 Fishing Season (NC, U.S.A: Beaufort).
Nissar S., Bakhtiyar Y., Arafat M. Y., Andrabi S., Bhat A. A., Yousuf T. (2023). A review of the ecosystem services provided by the marine forage fish. Hydrobiologia 850, 2871–2902. doi: 10.1007/s10750-022-05033-1
Pikitch E. K., Boersma P. D., Boyd I. L., Conover D. O., Cury P. M., Essington T. E., et al. (2018). The strong connection between forage fish and their predators: a response to Hilborn et al., (2017). Fisheries Res. 198. doi: 10.1016/j.fishres.2017.07.022
Pikitch E. K., Boersma P. D., Boyd I. L., Conover D. O., Cury P., Essington T., et al. (2012). Little Fish, Big Impact: Managing a crucial link in ocean food webs (Washington, D.C: Lenfest Ocean Program), 108.
Pikitch E. K., Rountos K. J., Essington T. E., Santora C., Pauly D., Watson R., et al. (2014). The global contribution of forage fish to marine fisheries and ecosystems. Fish Fisheries. 15, 43–64. doi: 10.1111/faf.12004
Pikitch E. K., Santora C., Babcock E. A., Bakun A., Bonfil R., Conover D. O., et al. (2004). Ecosystem-based fishery management. Science 305, 346–347. doi: 10.1126/science.1098222
Poole A. F. (1982). Brood reduction in temperate and sub-tropical ospreys. Oecologia 53, 111–119. doi: 10.1007/BF00377144
Poole A. F. (1989). Ospreys: a natural and unnatural history (New York: Cambridge University Press).
Poole A. F., Bierregaard R. O., Martell M. S. (2002). “Osprey (Pandion haliaetus),” in The Birds of North America, No. 683. Eds. Poole A., Gill F. (Philadelphia: The Birds of North America, Inc).
Postupalsky S. (1989). “Osprey,” in Lifetime reproduction in birds. Ed. Newton I. (London: Academic Press), 297–313.
Pulliam H. R. (1988). Sources, sinks, and population regulation. Am. Nat. 132, 652–661. doi: 10.1086/284880
Rice J., Duplisea D. (2014). Management of fisheries on forage species: the test-bed for ecosystem approaches to fisheries. ICES J. Mar. Sci. 71, 143–152. doi: 10.1093/icesjms/fst151
Ryttmann H. (1994). Estimates of survival and population development of the osprey Pandion haliaetus, common buzzard Buteo buteo and sparrowhawk Accipiter nisus in Sweden. Ornis Svec. 4, 159–172.
Scharf F. S., Manderson J. P., Frabrizio M. C., Pessutti J. P., Rosendale J. E., Chant R. J., et al. (2004). Seasonal and interannual patterns of distribution and diet of bluefish within a Middle Atlantic Bight estuary in relation to abiotic and biotic factors. Estuaries 27, 426–436. doi: 10.1007/BF02803535
Schueller A. M., Williams E. H., Cheshire R. T. (2014). A proposed, tested, and applied adjustment to account for bias in growth parameter estimates due to selectivity. Fisheries Res. 158, 26–39. doi: 10.1016/j.fishres.2013.10.023
Smith A. D., Brown C. J., Bulman C. M., Fulton E. A., Johnson P., Kaplan I. C., et al. (2011). Impacts of fishing low-trophic level species on marine ecosystems. Science 333, 1147–1150. doi: 10.1126/science.1209395
Smith L. A., Link J. S., Cadrin S. X., Palka D. L. (2015). Consumption by marine mammals on the Northeast US continental shelf. Ecol. Appl. 25, 373–389. doi: 10.1890/13-1656.1
Southeast Data Assessment and Review [SEDAR]. (2020). SEDAR 69 – Atlantic menhaden benchmark stock assessment report (North Charleston, SC: SEDAR), 691.
Southeast Data Assessment and Review [SEDAR]. (2022). Atlantic States Marine Fisheries Commission – Atlantic Menhaden Stock Assessment Update (North Charleston, SC: National Oceanic and Atmospheric Administration).
Spitzer P. R. (1980). “Dynamics of a discrete coastal breeding population of ospreys in the northeastern USA 1969-1979,” (Ithaca, NY, USA: Cornell University). Ph.D. Thesis.
Spitzer P. R., Poole A. F., Scheibel M. (1983). “Initial population recovery of breeding ospreys between New York City and Boston,” in Biology and management of Bald Eagles and Ospreys. Ed. Bird D. M. (Ste. Anne de Bellevue, Quebec, CA: Harpell Press), 231–241.
Staudinger M. D., Goyert H., Suca J. J., Coleman K., Welch L., Llopiz J. K., et al. (2020). The role of sand lances (Ammodytes sp.) in the Northwest Atlantic Ecosystem: A synthesis of current knowledge with implications for conservation and management. Fish Fisheries. 21, 522–556. doi: 10.1111/faf.12445
Steidl R. J., Griffin C. R. (1991). Growth and brood reduction in mid-Atlantic coast ospreys. Auk 108, 363–370. doi: 10.1093/auk/108.2.363
Stinson C. H. (1976). The evolutionary and ecological significance of the clutch size of the osprey (Williamsburg, VA, USA: College of William and Mary). M.A. Thesis.
Stinson C. H. (1978). The influence of environmental conditions on aspects of the time budgets of breeding ospreys. Oecologia 36, 127–139. doi: 10.1007/BF00349803
Uphoff J. H. Jr. (2003). Predator-prey analysis of striped bass and Atlantic menhaden in upper Chesapeake Bay. Fisheries Manage. Ecol. 10, 313–322. doi: 10.1046/j.1365-2400.2003.00366.x
Via J. W. (1975). Eggshell thinning and pesticide residues in ospreys from the lower Chesapeake Bay (Williamsburg, VA, USA: College of William and Mary). M. A. Thesis.
Viverette C. B., Garman G. C., McIninch S., Markham A. C., Watts B. D., Macko S. A. (2007). Finfish-waterbird trophic interactions in tidal freshwater tributaries of the Chesapeake Bay. Waterbirds 30, 50–62. doi: 10.1675/1524-4695(2007)030[0050:FTIITF]2.0.CO;2
Wahl R., Barbraud C. (2014). The demography of a newly established osprey Pandion haliaetus population in France. Ibis 156, 84–96. doi: 10.1111/ibi.12114
Walter J. F. III, Overton A. S., Hl. Ferry K., Mathers M. E. (2003). Atlantic coast feeding habits of striped bass: a synthesis supporting a coast-wide understanding of trophic biology. Fisheries Manage. Ecol. 10, 349–360. doi: 10.1046/j.1365-2400.2003.00373.x
Watts B. D., Byrd M. A., Watts M. U. (2004). Status and distribution of breeding Ospreys in the Chesapeake Bay: 1995-96. J. Raptor Res. 38, 47–54.
Watts B. D., Paxton B. J. (2007). Ospreys of the Chesapeake Bay: Population recovery, ecological requirements, and current threats. Waterbirds 30, 39–49. doi: 10.1675/1524-4695(2007)030[0039:OOTCBP]2.0.CO;2
Wiemeyer S. N. (1971). Reproductive success of Potomac River osprey. Chesapeake Sci. 12, 278–280. doi: 10.2307/1350918
Keywords: osprey, menhaden, Chesapeake Bay, reproductive rate, diet, fisheries, ecosystem-based management
Citation: Watts BD, Stinson CH, McLean PK, Glass KA, Academia MH and Byrd MA (2024) Demographic response of osprey within the lower Chesapeake Bay to fluctuations in menhaden stock. Front. Mar. Sci. 10:1284462. doi: 10.3389/fmars.2023.1284462
Received: 28 August 2023; Accepted: 13 December 2023;
Published: 08 January 2024.
Edited by:
Ronald Ydenberg, Simon Fraser University, CanadaReviewed by:
Peter J. Auster, Mystic Aquarium, United StatesMarco Restani, St. Cloud State University, United States
Copyright © 2024 Watts, Stinson, McLean, Glass, Academia and Byrd. This is an open-access article distributed under the terms of the Creative Commons Attribution License (CC BY). The use, distribution or reproduction in other forums is permitted, provided the original author(s) and the copyright owner(s) are credited and that the original publication in this journal is cited, in accordance with accepted academic practice. No use, distribution or reproduction is permitted which does not comply with these terms.
*Correspondence: Bryan D. Watts, bdwatt@wm.edu