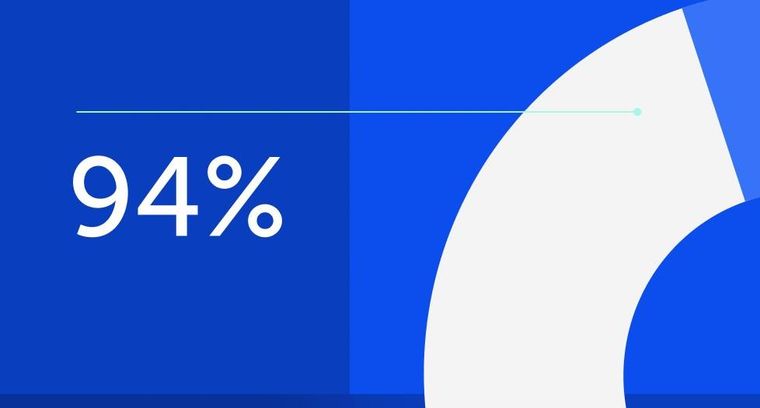
94% of researchers rate our articles as excellent or good
Learn more about the work of our research integrity team to safeguard the quality of each article we publish.
Find out more
ORIGINAL RESEARCH article
Front. Mar. Sci., 01 December 2023
Sec. Marine Ecosystem Ecology
Volume 10 - 2023 | https://doi.org/10.3389/fmars.2023.1278293
Domoic acid-producing blooms of the diatom genus Pseudo-nitzschia are pervasive in coastal environments globally. Domoic acid, a neurotoxin, accumulates via trophic transfer into marine food webs and is often associated with mass marine mammal mortality and stranding events. In Southern California, California sea lions (Zalophus californianus) are an indicator species for food web impacts of domoic acid because they are abundant secondary consumers, sensitive to domoic acid intoxication, and are actively monitored by stranding networks. However, domoic acid exposure may occur a distance from where a sea lion ultimately strands. This spatiotemporal variation complicates coupling domoic acid observations in water to strandings. Therefore, we sought to quantify whether monitoring data from four pier sites across the region, covering nearly 700 km of coastline from 2015-2019, could be used to predict adult and subadult sea lion strandings along the 68 km Orange County coastline surveyed by the Pacific Marine Mammal Center. We found that increased sea lion strandings were often observed just prior to an increase in particulate domoic acid at the piers, confirming that clusters of subadult and adult sea lion strandings with clinical signs of domoic acid intoxication serve as indicators of bloom events. In addition, domoic acid concentrations at Stearns Wharf, nearly 200 km from stranding locations, best predicted increased total sea lion strandings, and strandings of sea lions with domoic acid intoxication symptoms. Particulate domoic acid concentrations greater than 0.05 μg/L at Stearns Wharf were linked to stranding probabilities in Orange County ranging from 2.2% to 55% per week, and concentrations of 0.25 μg/L resulted in weekly stranding probabilities ranging from 16% to 81% depending on the stranding scenario modeled.
Harmful algal blooms (HABs) are a common water quality issue in many coastal and inland waterbodies globally and cause widespread impacts on human and animal health, recreation, fisheries and aquaculture (Broadwater et al., 2018; Moore et al., 2020; Wells et al., 2020; Anderson et al., 2021). One of the most pervasive HAB-forming organisms, particularly within the Southern California, is the diatom genus Pseudo-nitzschia, which contains over a dozen species capable of producing the neurotoxin domoic acid (Bates et al., 2018). Domoic acid accumulates via trophic transfer throughout pelagic and benthic food webs, resulting in significant risks to both human and ecosystem health (Bates et al., 1989; Bejarano et al., 2008b; Lefebvre et al., 2017). Domoic acid exposure causes sickness and mass mortality events in a variety of marine animals including sea lions, sea otters, whales and seabirds (Lefebvre et al., 2002a; Kvitek et al., 2008; Torres De La Riva et al., 2009; Gibble et al., 2021; Moriarty et al., 2021).
The first event in which domoic acid intoxication of marine animals was conclusively linked to a bloom of Pseudo-nitzschia occurred in 1998 in Monterey Bay, California. During this event, over 70 California sea lions (Zalophus californiaus) were treated for domoic acid intoxication, 48 of which died (Scholin et al., 2000). Domoic acid was detected not only in the blood and urine of the affected sea lions but also was detected in sardines, anchovies, and phytoplankton in the area (Scholin et al., 2000). Since this event, domoic acid related strandings of marine mammals have continued to be observed for more than two decades along the California coastline, impacting hundreds of marine animals (Gulland et al., 2002; Greig et al., 2005; Bejarano et al., 2008a; Bejarano et al., 2008b; Goldstein et al., 2008).
Marine mammals are good sentinels of coastal ecosystem health due to their high trophic status, distinctive life histories, and long term residence in coastal environments, which are traits that make them responsive to ecosystem changes (Rice and Rochet, 2005; Bossart, 2011). Within Southern California, California sea lions are an important indicator species due to their permanent residence in the region, abundance, and their upper trophic level status (Melin et al., 2012). California sea lions are also commonly rescued and rehabilitated by the California Marine Mammal Stranding Network (Greig et al., 2005). California sea lions strand for a variety of reasons including malnutrition, infectious diseases, human interactions (ie: gunshot wounds, fishing line entanglement), natural and anthropogenic changes in the environment (storms, climatic cycles, climate change), and exposure to algal toxins such as domoic acid (Greig et al., 2005; Simeone et al., 2015; Gulland et al., 2022).
Domoic acid is a water-soluble chemical analog of L-glutamate and is an agonist of glutamate receptors. It causes massive neuronal activation, leading to seizures, neuronal injury, and neuronal death over time (Silvagni et al., 2005). Domoic acid intoxication is most often diagnosed by clinical signs combined with a known bloom occurring in the region of the strandings. Diagnostic testing for domoic acid in feces, urine, stomach contents, as well as other fluids and tissues are available, but not always pursued due to funding limitations and the pathognomonic signs associated with acute domoic acid intoxication. California sea lions exhibit varying clinical presentations to domoic acid exposure based on whether the animal has had an acute intoxication or has developed chronic symptoms in response to multiple exposures over time, although the progression from acute to chronic is not well understood. California sea lions exposed acutely to domoic acid typically display a common suite of neurological symptoms including seizures, postictal behavior, head weaving, ataxia, lethargy, and abnormal scratching (Scholin et al., 2000; Gulland et al., 2002). Other symptoms include abortion, premature parturition of pups and death (Brodie et al., 2006). Acute cases are reported to strand in spatiotemporal clusters (Bejarano et al., 2008a). Domoic acid epileptic disease, also referred to as chronic epileptic syndrome in sea lions, is thought to be caused by one or more sublethal exposure events resulting in permanent brain damage (Ramsdell and Gulland, 2014). Sea lions with this syndrome show behavioral changes and intermittent seizures (suffering from seizures two weeks apart or after two weeks in rehabilitation) associated with hippocampal atrophy (Goldstein et al., 2008; Cook et al., 2015; Cook et al., 2016). These animals are often asymptomatic between seizures and strand individually, unlike acute domoic acid stranding events that present in stranding clusters with dozens of sea lions displaying DA intoxication clinical signs within a small geographic region. Adult females are most frequently associated with domoic acid related strandings in California, although all sexes and age classes of sea lions are susceptible to intoxication (Bejarano et al., 2008a).
The relationship between California sea lion stranding events and the presence of domoic acid in the environment is complex for multiple reasons. One major factor is the temporal and spatial variability of domoic acid in the environment, particularly during bloom events. The production of domoic acid by Pseudo-nitzschia is dynamic and varies based on environmental conditions, which can also influence bloom formation and duration (Trainer et al., 2020). Blooms are also heterogeneous spatially. The level of toxin present in Pseudo-nitzschia cells can vary across both horizontal and vertical gradients along the coast (Seegers et al., 2015; Bowers et al., 2018). The amount of toxin that is produced during a bloom event is also dependent on the Pseudo-nitzschia species present, since not all species of Pseudo-nitzschia produce toxins, and the toxin production rates of toxigenic species can vary considerably across species and strains (Trainer et al., 2012; Bowers et al., 2018). Another complicating factor is that domoic acid can enter the food web via multiple mechanisms. Domoic acid can enter both pelagic (Lefebvre et al., 1999; Lefebvre et al., 2002b) and benthic food webs (Vigilant and Silver, 2007; Smith et al., 2021), and marine mammal exposure is then a result of the consumption of contaminated prey items that are typically primary or secondary consumers. Recent work has also shown that sea lion prey organisms can differentially accumulate domoic acid (Bernstein et al., 2021), further complicating the linkages between the presence of domoic acid in water samples and impacts on upper trophic levels.
California sea lions are a useful indicator species for identifying pelagic food web impacts of domoic acid-producing blooms in the region. Previous studies along the California coastline have highlighted correlations between the presence of either domoic acid or Pseudo-nitzschia cells in the environment and sea lion stranding events. Significant, but weak, correlations between a monthly time series of sea lion strandings of all age classes and abundances of two different toxigenic Pseudo-nitzschia species and particulate domoic acid (pDA) concentrations were observed between 1998-2007 in Monterey Bay, California (Bargu et al., 2010). In Southern California, a significant, multi-species marine mammal stranding event was caused by a large domoic acid-producing bloom in 2002. Comparisons between stranding reports and Pseudo-nitzschia relative cell abundances, a semiquantitative measure of Pseudo-nitzschia abundance in relation to other phytoplankton, for the year of 2002 indicated significant correlations between strandings of multiple marine mammal species and increased relative abundances of Pseudo-nitzschia both immediately and with lags up to six weeks following peaks in Pseudo-nitzschia relative cell abundances (Torres De La Riva et al., 2009).
Here, we aim to identify a quantitative link between observations of domoic acid in water samples and marine mammal strandings to build towards the ability to predict negative ecosystem impacts from bloom events to better contextualize water concentrations of domoic acid. Predictions of domoic acid related marine mammal stranding events can also help Stranding Network locations, which are largely non-profit organizations, better prepare for rescuing and rehabilitating an influx of intoxicated animals. This study considered particulate domoic acid data collected routinely from 2015-2019 at four pier stations across the region and California sea lions stranding data from the same period collected along a 68 km stretch of the Southern California coastline by the Pacific Marine Mammal Center (PMMC). The goals of this study were to: 1) model the relationship between pier based domoic acid data and sea lion strandings 2) identify which pier(s) were most strongly linked with stranding events, and 3) to identify thresholds of domoic acid concentrations that are associated with an elevated risk of stranding events.
Harmful algal bloom monitoring for domoic acid concentrations and other related parameters has been conducted on a weekly basis since 2008 within Southern California as a part of the California Harmful Algal Bloom Monitoring and Alert Program (HABMAP; Kudela et al., 2015). Particulate domoic acid (pDA) concentrations from January 2015 to December 2019 were monitored at four pier locations within the Southern California (Figure 1): Scripps Pier in San Diego (n = 260), Newport Beach Pier on the San Pedro Shelf (n = 256), Santa Monica Pier in the Santa Monica Bay (n = 258), and Stearns Wharf along the Santa Barbara Channel (n = 258). The collection and analysis of pDA samples is described in Seubert et al. (2013). Briefly, surface water samples were collected weekly, typically on Mondays, at each HABMAP pier location. Subsamples were collected in duplicate for pDA analysis via gentle vacuum filtration of 188 - 200 mL of sample water onto glass fiber filters. Filters were stored at -20°C in the dark until analyzed. Filters were extracted in 3 mL of 10% methanol, sonicated for 15 seconds and centrifuged for 15 minutes at 4,000 rpm. The supernatant was analyzed via Mercury Science, Inc., Domoic Acid Enzyme-Linked ImmunoSorbant Assay (ELISA: Mercury Science, Durham, NC) according to the methods described in Litaker et al. (2008). The detection limit for pDA is 0.02 μg/L.
Figure 1 Locations of stranded adult and subadult California sea lion rescued between 2015 and 2019 in relation to pier monitoring stations. Stranding locations are shown in blue circles and the four weekly HABMAP pier monitoring stations are shown with orange squares.
In 2017, offshore water sampling was conducted in response to signs of a region-wide domoic acid-producing bloom at the pier monitoring locations and reports of California sea lions stranding with domoic acid intoxication clinical signs. Surface water samples were collected opportunistically in Santa Monica Bay on April 13 and April 17, 2017 and in the Santa Barbara Channel on May 22, May 25 and June 6, 2017. Samples were also collected on the San Pedro Shelf region on April 18 and 19, and May 10 and 11, 2017 from both surface waters and the chlorophyll maximum located in the subsurface waters (depths ~20m). A total of 73 discrete pDA samples were collected and analyzed as a part of these 2017 offshore sampling efforts following the same method applied to the pier monitoring samples.
Pacific Marine Mammal Center (PMMC) is a member of the West Coast Marine Mammal Stranding Network, which was established under the United States Marine Mammal Protection Act by the National Oceanic and Atmospheric Administration (NOAA) to respond to stranded marine mammals. PMMC rescues, rehabilitates, and releases sick and injured marine mammals along the Orange County coastline, which is approximately 68 km of the Southern California coastline (Figure 1). All marine mammal rescue and rehabilitation activities are conducted by PMMC under a Stranding Agreement with National Marine Fisheries Service/NOAA.
Patient case records from 2015-2019 for California sea lions (hereafter, sea lions) were reviewed and collated for each animal’s case presentation including sex, age class, seizure activity, postictal signs, comatose, abortion, and whether the sea lion was diagnosed by the attending veterinarian with acute domoic acid intoxication following the behavioral diagnostic criteria outlined by Gulland et al. (2002). This period was selected due to the accessibility of continuous case records with sufficient detail to synthesize the case characteristics described above. Notably, however, multiple veterinarians made diagnoses during the time series based on these criteria which may have introduced some variation in the diagnosis of domoic acid intoxication during the study period. Age classes were defined following Greig et al. (2005) using dentition, the straight-line length from nose to tail, pregnancy status, and sexual dimorphism to classify a sea lion’s age class. The following demographic categories are included in this study: juvenile/subadult male, subadult female, adult male, and adult female. Younger sea lions in the pup and yearling age classes were excluded as sea lions within these age classes are generally the most common patients at the center and often strand due to malnutrition (Bejarano et al., 2008a), and rarely present with acute or chronic domoic acid intoxication during blooms. Adult and subadult age classes are the most commonly affected by domoic acid intoxication (Bejarano et al., 2008a). Only live sea lions that were rescued on the Orange County coastline, brought to PMMC’s facility, and assessed by the attending veterinarian were included. Adult and subadult sea lions that died on the beach were excluded from the study cohort because an attending veterinarian could not assess clinical signs to diagnosis domoic acid intoxication prior to death.
Generalized linear autoregressive moving average (GLARMA) models were used to identify whether domoic acid concentration at each of four piers best predicted combined adult and subadult sea lion strandings along the Orange County coastline. The GLARMA model approach was selected for this analysis since it is well suited for use with observational, count based time series data in which counts are relatively small, have non-normal data distributions, and display serial dependence (Dunsmuir, 2015; Dunsmuir and Scott, 2015). The concentrations of domoic acid, which were measured at each pier monitoring location, as well as past stranding events, were explored as potential explanatory variables for the presence/absence of a stranding event. For the purposes of our analyses, we define a stranding event as the stranding of an individual adult or subadult sea lion. Cross correlation is a measurement of whether two different variables are related with each other such that changes in one variable precede changes in the other. Therefore, cross correlation functions were used to identify if domoic acid concentrations observed at pier locations led or lagged stranding events (Supplementary Figure 1).
Many environmental datasets are autocorrelated, meaning that measurements at one period of time are correlated with the value of that same variable that was measured previously in time. If autocorrelation is present in the dependent variable in a statistical analysis, it is necessary to address it statistically (Box et al., 2015). Therefore, we first investigated whether our sea lion stranding data is autocorrelated. In particular, we considered strandings from zero through six weeks prior to each time point, because strandings are autocorrelated with a local maximum at six weeks (Supplementary Figure 2A). Based on the autocorrelation patterns that we did observe in the stranding data (Supplementary Figure 2A), the six and one week moving average values of stranding events preceding each observation were incorporated into the model, so that effects of domoic acid exposure could be explored after subtracting out the autocorrelated signal. We also investigated whether partial autocorrelation was present in our sea lion stranding data. Partial autocorrelation identifies associations between current and previous stranding observations, controlling for (partialling out) shorter time lags (Supplementary Figure 2B).
Several stranding event scenarios were considered in our modeling efforts to determine if different demographics within the stranding cases yielded similar relationships to pier locations. These stranding event scenarios included total strandings (n = 153), strandings where the sea lion was diagnosed as a suspected domoic acid case based on the behavioral criteria described above (n = 60), strandings where the sea lion experienced a seizure (n = 50), and strandings of female sea lions (n = 119). The female only stranding scenario was included in addition to the symptom-based scenarios because previous studies have indicated that subadult and adult females have comprised a majority of acute domoic acid cases historically in the region (Bejarano et al., 2008a). The sample size was too small to model females that aborted fetuses while in treatment (n = 13). We first queried a combined model that explored the effects of domoic acid concentration at all four piers. We then dropped each pier with lowest predictive power, in sequence, from our model, to determine whether a model with data from fewer piers was more informative. Akaike’s information criterion (AIC) was used to compare the performance of each model with lower AIC values indicating better fit models than higher AIC values in each modeled stranding scenario. We also conducted a Wald Test, which tested whether the results of the GLARMA model provide a better fit to those of a linear model that ignores the effects of autocorrelation (our significance α threshold for this test was 0.05).
Lastly, the best-fit models were validated using forward prediction. In this approach the values at each time point were predicted by models that had been trained only on the previous stranding data, and by models which had been provided with both pDA concentration data and stranding data leading up to that time point. The models outputted a predicted value μt, at each time point, which is the λ value of the predicted poisson function most likely to return the observed number of strandings. The performance of each model, measured as the mean squared error (MSE; Eqn 1), was compared to the performance of a null model which was trained only on stranding data, but not pDA concentrations, and which was only provided with stranding information.
Where μ(t) is the predicted value of strandings at each timepoint t and OS(t) is the observed number of strandings at each time point.
While the GLARMA model identifies statistical associations accounting for autocorrelation in stranding patterns, we also wanted to identify the general relationship between pDA concentration and strandings, ignoring autocorrelation patterns. Such an approach would allow identification of the pDA thresholds that were associated with elevated stranding probabilities since there are no existing water thresholds with which to evaluate monitoring data for an increased risk of ecosystem impacts. A challenge of using GLARMA, and indeed any autocorrelation approach, is that the modeled probability is dependent not only on observed domoic acid concentrations, but also on previous occurrences of strandings. This means that model outputs are all contingent on previous stranding levels and thus make it difficult to determine thresholds of concern related to a specific pDA concentration. Therefore, Poisson Regression modeling was used to explore association between pDA concentrations and adult sea lion stranding events ignoring temporal autocorrelation of time. This analysis was conducted using observations from the strongest explanatory pier location, Stearns Wharf, which had been identified and previously found to be statistically significant in all of our modeling scenarios, using the GLARMA approach described above. Models were fit to identify the relationship between pDA concentration and the probability that at least one, or at least two sea lion stranding events occurred in a given week. This general linear model was conducted using the glm function, with Poisson family using the `stats` package in R. Probabilities of one and two stranding events were generated by converting the lambda (λ) parameter generated by R’s prediction function into probabilities of more than one and more than two strandings using the `dpois` function in the `stats` package in R.
Detectable pDA concentrations were observed within the Southern California region each year between 2015 and 2019 (Figures 2C–F), with a significant domoic acid-producing bloom of Pseudo-nitzschia was observed throughout the region in the spring of 2017. Detectable concentrations of domoic acid were observed at all pier locations routinely between March and early June, though concentrations varied substantially across piers during this event. Santa Monica Pier and Stearns Wharf had the highest observed domoic acid concentrations with multiple observations of pDA >1 μg/L, Newport Pier and Scripps Pier had lower concentrations of pDA that were generally<1 μg/L except for one observation in the first week of May at Scripps Pier of 2.07 μg/L. The highest concentration of domoic acid observed across pier stations peaked at 14.4 μg/L at Santa Monica Pier (Figure 2D). Offshore observations of pDA were more irregular than those at the piers, but these observations generally showed some marked spatial differences in pDA concentrations (Figure 3). The highest offshore concentrations were detected offshore of Stearns Wharf in the Santa Barbara Channel (17 μg/L) and offshore of Newport Pier on the San Pedro Shelf (10 μg/L). Offshore and subsurface concentrations were often higher, sometimes by an order of magnitude or more, than those observed at Newport Pier (Figure 3D). Offshore observations in the Santa Barbara Channel and Santa Monica Bay generally showed less of a distinct difference from the pier observations (Figures 3B–D), but these observations were limited to surface grabs and were patchier than observations offshore of Newport Pier (Figure 3D).
Figure 2 Time series of total adult and subadult California sea lions (A), stranding patients that exhibited domoic acid symptoms while in treatment at PMMC (B) and particulate domoic acid concentrations (μg/L) at Stearns Wharf (C), Santa Monica Pier (D), Newport Pier (E) and Scripps Pier (F). Domoic acid concentrations are plotted on a semi-log scale.
Figure 3 Total sea lion strandings per week during the 2017 event (A) and particulate domoic acid concentrations (B–D) observed at pier stations and offshore event sampling cruises. Geographic extent of three regions of interest (E) and specific sampling locations (F–H) during 2017 event response surveys (green circles indicate surface samples and blue crosses indicate subsurface samples) in relation to pier monitoring stations (orange squares). Domoic acid concentrations are plotted on a semi-log scale with observations below the detection limit intersecting the dashed line at 0.02 μg/L.
Observations of detectable domoic acid were more infrequent in the periods preceding and following the large bloom in 2017. In 2015, pDA was only detected at Stearns Wharf and Santa Monica Pier monitoring sites, with toxin detections ranging between 0.04 μg/L to 0.45 μg/L (Figures 2C, D). There was a period of fairly consistent detection of pDA between May and early July at Stearns Wharf, which was representative of a historically widespread bloom of Pseudo-nitzschia, which occurred across the U.S. West Coast (McCabe et al., 2016). However, this event was not observed at the other three piers to the south. In 2016, domoic acid was observed intermittently at Stearns Wharf, Newport Pier and Scripps Pier in the first half of the year at concentrations<0.50 μg/L. Following the 2017 bloom, domoic acid was least commonly observed at the pier locations in 2018, with only two instances of domoic acid detection at concentrations of<0.15 μg/L at Newport Pier. Domoic acid was detected at all four pier locations in the spring of 2019, although concentrations were all below 0.20 μg/L with the highest concentrations observed at Newport Pier of 0.19 μg/L (Figure 2E).
The Pacific Marine Mammal Center rescued and administered veterinary care to a total of 153 adult and subadult sea lions between 2015 and 2019 (Figure 2A). During this period, adult and subadult strandings generally comprised a minor percentage of the overall sea lions rescued each year ranging from 7% (38/528) to 12% (16/138) of sea lion cases, apart from 2017 where 54% (61/114) of total strandings were in this age demographic. Of the subadult and adult sea lions stranded in 2017, 80% (49/61) were diagnosed as suspected domoic acid cases. Temporally, 95% (58/61) of the sea lion strandings during this year coincided with when elevated domoic acid concentrations were observed in the environment with 56% (34/61) of the sea lions strandings occurring in April, 23% (14/61) in May, and 16% (10/61) in June (Figure 3A). Most of the adult and subadult sea lions that stranded during this period displayed various clinical signs consistent with domoic acid intoxication, ranging from postictal behavior (obtunded to comatose), seizure activity, and abortion. Additionally, 97% (56/58) of these stranded sea lions between April and June were females (Supplementary Figure 3A), 11 of which aborted a pup while in the rehabilitation hospital. All aborted pups were non-viable or stillborn, estimated to be 7 to 8 months in fetal gestation. Neurological or seizure activity was reported in 48% (28/58) of the stranded sea lions during the bloom, either on the beach or once back at PMMC.
In the years surrounding 2017 (2015, 2016, 2018, and 2019) PMMC responded to strandings of substantially fewer subadult and adult sea lions. Prior to the 2017 event, 38 subadult and adult sea lions were rescued in 2015, and 31 in in 2016. In the two years following the event, 7 subadult and adult sea lions were rescued in 2018, and 16 in 2019. Cases where sea lions were suspected domoic acid cases or observed to have seizures were also fewer in the years surrounding 2017. Of the total subadult and adult sea lion cases annually, 11% (4/38) were suspected domoic acid cases in 2015, 13% (4/31) in 2016, 14% (1/7) in 2018 and 13% (2/16) in 2019, compared to 80% of cases in 2017 (Figure 2B). Seizures followed a similar pattern in 2015 and 2016 and were observed in 13% (5/38) of cases in 2015 and 19% (6/31) of cases in 2016. Interestingly, a larger percentage of sea lions had reported neurological or seizure activity in 2018 and 2019, occurring in 43% (3/7) and 38% (6/16) of cases, respectively (Supplementary Figure 3B). These cases may represent chronic domoic acid patients, versus acute domoic acid intoxication associated with smaller, more ephemeral domoic acid events.
Total adult and subadult sea lion stranding events typically occurred in temporal clusters with multiple strandings occurring over a period of weeks along the Orange County coastline, followed by periods without strandings of adult and subadult sea lions (Figures 2A, B). This dynamic was particularly apparent in 2017 during the domoic acid-producing bloom of Pseudo-nitzschia that extended across most of the region. During this period, more than 50 sea lions stranded within a span of 11 weeks. Autocorrelation, but not partial autocorrelation, was observed in the overall stranding time series (Supplementary Figure 2), indicating that stranding in a given week is positively associated to previous stranding rates, with local autocorrelation maxima at one and six weeks.
Adult and subadult sea lion strandings on the Orange County coastline over the time series often preceded the observation of pDA at the piers. At all of the piers, except Newport Pier, the strongest observed cross correlations occurred with 1-3 week lead between pDA observations and total strandings, meaning that increased strandings were typically observed prior to an increase of pDA at the piers (Supplementary Figure 1). However, at all piers but Scripps Pier, the second most significant cross correlation observed was with no lag in pDA concentrations. Since our goal was to be able to estimate the risk of sea lion strandings with the pDA observations collected at the piers, and lagged values of pDA were not among the top cross correlations, we did not implement GLARMA models that considered previous values of pDA concentration at any pier. Instead, we used a GLARMA model that considered one- and six-week moving averages of stranding counts as inputs to account for these relationships. Wald tests confirmed that the model is better represented by processes that consider time lags in sea lion stranding counts than ones that did not (Wald = 26.01, p< 0.01).
The GLARMA model, fit to total strandings, was tested with pDA observations from all four piers within the region. Comparing AIC values of different models suggested that the total strandings scenario was most accurately predicted from models that include three piers (Supplementary Table 1). The best-fit model included pDA data from Stearns Wharf, Santa Monica Pier and Newport Pier, and the statistical significance denoted by the p-values, which decreased in significance from north to south. Interestingly, only pDA concentrations at Stearns Wharf and Santa Monica Pier were statistically significant (p< 0.05, Table 1), while Newport Pier observations improved the overall model fit (as measured by AIC values, Supplementary Table 1) but its coefficient was not statistically significant (p = 0.088; Table 1).
Table 1 Model statistics for the total adult and subadult sea lions stranding scenario (Total) and the sea lions with domoic acid intoxication symptoms scenario.
Strandings in the other three scenarios considered also generally appeared in temporal clusters, and models were best fit using multiple piers (Figure 2B, Supplementary Figure 2). The GLARMA model for stranded sea lions that presented with domoic acid intoxication symptoms, incorporating one- and six-week stranding lag data, demonstrated the best fit when data from both Stearns Wharf and Santa Monica Pier were included as predictors (Supplementary Table 1). The inclusion of pDA data from the other two pier locations weakened model performance based on the AIC values (Supplementary Table 1). Sea lions exhibiting seizures and stranded females were modeled separately (Supplementary Table 2). All model scenarios were better fit by GLARMA processes than by equivalent general linear models (Wald Tests for each p<0.01).
The forward prediction model, trained on all previous data, was able to predict total sea lion strandings from previous strandings and contemporaneous pDA data from Stearns Wharf, Santa Monica Pier and Newport Pier with a MSE of 2.84 (Figure 4). This was better than the null model, which was trained only on sea lion stranding data and without pDA data, which had an MSE of 41.1. Prior to 2016, there were not enough data to allow for model convergence. Furthermore, the model was “fooled” at the beginning of the domoic acid event in 2017 due to the negative lag term in the models built before 2017, resulting in substantial underprediction of the probability of additional strandings initially. However, from the second half of this event and forward, the model correctly predicted the rest of the dataset (MSE = 0.29) and was more accurate than the null model that did not consider pDA concentrations (null MSE = 0.34). Forward predictions of the other three stranding scenarios (sea lions with symptoms of domoic acid exposure, subadult/adult female sea lions, and sea lions with seizures) showed less ability to predict stranding events. The forecast models of subadult/adult female sea lions was also improved by inclusion of pDA data (pDA Model MSE = 3.90, Null Model MSE = 5.28), but not models of sea lions with domoic acid exposure symptoms (pDA Model MSE = 5.95, Null Model MSE = 2.25) or sea lions with seizures (pDA Model MSE = 0.384, Null Model MSE = 0.342) (Supplementary Figure 4).
Figure 4 GLARMA forecast model of total strandings for study period. The y-axis corresponds to observed strandings and μ the predicted Poisson lambda value of the GLARMA model, corresponding roughly to the forecasted number and (if<1) probability of strandings. The x-axis corresponds to time. Black dots are observed stranding events. The blue line is the GLARMA model fit to the training data and forecasts are shown in red. The red line begins in 2016 because forecast models based only on data before 2016 fail to converge on a solution (find a set of parameters that are able to forecast the data). The GLARMA forecast model is trained only with data preceding a given time point, and the domoic acid concentrations from that week. It then predicts the number of strandings for that week.
To better define the degree to which pDA observations are linked to negative ecosystem impacts, simple models comparing pDA concentration at Stearns Wharf to strandings were also developed. These models, which ignored the temporal autocorrelation relationships described above, indicated that the probability of sea lion strandings events were statistically related to observed pDA concentrations (All Strandings, Nagelkerke R2 = 0.440; DA Symptoms Nagelkerke R2 = 0.658; Females Nagelkerke R2 = 0.494; Seizures Nagelkerke R2 = 0.378; p-value for all models<< 0.001; Figure 5, Supplementary Figure 5). Baseline stranding probabilities were never zero in these models, even when pDA was below the methodological detection limit. This non-zero baseline indicates that there is always some probability of sea lion strandings each week. This is reasonable as sea lions strand for reasons other than domoic acid exposure. For instance, even in weeks with no pDA in the water and no recent strandings, strandings did still occur (Figure 2). The baseline stranding probability for an individual adult/subadult sea lion in the total strandings scenario (when pDA was set to the detection threshold of 0.02 μg/L) was 44% per week (95% confidence intervals (CI): 38% – 49%) while the baseline probability of two or more sea lions stranding was 12% per week (CI: 8% – 15%; Figure 5A). Baseline stranding probabilities of strandings with domoic acid intoxication symptoms were 13% per week (CI: 8.4% – 17%; Figure 5B) for individual sea lions, and 0.89% for two or more sea lions (CI: 0.36% – 1.6%).
Figure 5 Observations and Poisson regression models of the relationship between domoic acid concentration and stranding scenarios, total strandings (A) and sea lions exhibiting behavioral symptoms of domoic acid intoxication (B). Points indicate weekly observations. The x-axis corresponds to DA concentrations and the y-axis and point color reflect numbers of strandings. Points at or near the y-value of 0 correspond to weeks in which there were no strandings. Black points with y value at or near 1 correspond to weeks with one stranding. Blue points indicate weeks with two or more strandings. Black and blue bands indicate the probability, predicted by a Poisson regression model, of one or more (black) and two or more strandings (blue) at different domoic acid concentrations. Lines indicate the maximum likelihood probability and bands indicate two standard errors of that mean value.
Stranding probabilities increased with increasing concentrations of pDA in the environment. At a concentration of 0.05 μg/L the probability of a single sea lion stranding was 55% per week (CI: 49% – 60%). At a concentration of 0.25 μg/L the probability of a single sea lion stranding was 81% per week (CI: 75% – 86%) (Figure 5). Multi-sea lion stranding probabilities were >50% per week at pDA concentrations of 0.25 μg/L and >80% per week at 0.75 μg/L. As with the total strandings scenario, the probability of strandings with domoic acid intoxication symptoms increasing rapidly between 0.05 μg/L (stranding probability one sea lion with DA symptoms 20% per week; CI: 14-26%; two sea lions 2.2% per week, CI: 1.0%-3.7%) and 0.25 μg/L (stranding probability of one sea lion with DA symptoms 52% per week, CI: 41-60%; two sea lions with DA symptoms 16% per week, CI: 10%-24%) of pDA for both individual and multiple sea lions.
All best-fit models of adult and subadult sea lion strandings revealed a positive statistical link between stranding events and pDA concentrations at pier monitoring locations. Notably, however, we found that subadult and adult sea lion strandings were typically observed prior to increased concentrations of pDA at most piers monitoring sites. Using the region-wide routine monitoring data, we were also able to identify a consistent correlation between strandings in Orange County and domoic acid at Stearns Wharf in Santa Barbara, nearly 200 km to the north of the stranding locations, across each of the stranding scenarios tested. Our best-fit models for each of the different stranding scenarios showed a pattern of decreasing significance from north to south across the region, underscoring the importance of considering the large geographic regions covered by sea lions when considering algal toxin exposure (Bernstein et al., 2021). Our results do not necessarily imply that domoic acid related marine mammal stranding events are solely driven by Pseudo-nitzschia blooms in the northernmost regions of Southern California. In fact, along with the Santa Barbara Channel, the San Pedro Channel (Santa Monica Pier and Newport Pier are within this area) are considered subregional domoic acid hotspots within Southern California (Sandoval-Belmar et al., 2023). However, in our time series, pDA observations at Stearns Wharf provide the best indication of when marine mammal strandings caused by domoic acid intoxication may occur. We believe this link could indicate that either pDA observations from Santa Barbara are potentially more representative than the other pier locations of the pDA concentrations in offshore and/or subsurface waters where sea lions forage, that sea lions do not strand near the areas where they became intoxicated, that sea lions throughout the region consume prey that originated near Santa Barbara, or a combination of factors.
Our results suggest data from the nearshore pier locations can provide a reasonable approximation of when domoic acid related strandings are likely to occur, despite not fully capturing potential offshore and subsurface bloom dynamics. This finding is somewhat surprising in part because previous work has indicated that domoic acid observations collected at pier monitoring sites are often not fully representative of broader bloom spatial extent and magnitude within the region (Seegers et al., 2015; Smith et al., 2018). Indeed, we did also find that at most pier locations, strandings often lead observations of pDA by between 1-3 weeks, limiting how much early warning these locations could provide for domoic acid related strandings. Estimates of remotely sensed chlorophyll-a have suggested a notable disconnect often exists between primary production patterns nearshore and those 2-4 km offshore in California (Frolov et al., 2013). Previous studies of bloom ecology within the Southern California region have shown that that there are sometimes significant differences between onshore Pseudo-nitzschia abundances and domoic acid levels compared to those both offshore and subsurface (Seegers et al., 2015; Smith et al., 2018).
Offshore observations collected during the large bloom event in 2017 highlight some notable spatial heterogeneity in domoic acid concentrations, particularly on the San Pedro Shelf region offshore of the PMMC stranding response zone and Newport Pier (Figure 3). While these observations do not have the same temporal resolution as the weekly observations collected at the piers, they highlight the potential for an order of magnitude difference in toxin concentrations between the nearshore and offshore regions, which may have significant implications for the predictive power of specific pier monitoring sites throughout the region. Interestingly, the difference between nearshore and offshore observations was not as pronounced in the Santa Monica Bay (Figure 3B) and Santa Barbara Channel (Figure 3C) regions, albeit observations were less extensive than in the San Pedro Shelf region and only represent a single bloom event (Figure 3A). Previous reports in the Santa Barbara Channel have demonstrated that there can be significant differences between concentrations of pDA at Stearns Wharf and those offshore in the Channel, with instances of offshore pDA concentrations exceeding those observed at pier stations by one or two orders of magnitude (Umhau et al., 2018). However, when considered over a five-year period between 2009 and 2013, there were no significant differences between concentrations of pDA routinely sampled at offshore stations and those at Stearns Wharf (Umhau et al., 2018). This suggests that Stearns Wharf has good explanatory power because it is, on average, more representative of offshore domoic acid dynamics, which affect sea lions. Comparable pDA datasets to those presented in Umhau et al. (2018) do not currently exist offshore of Santa Monica Pier, Newport Pier, or Scripps Pier, therefore it is unclear how representative these locations are, on average, of more offshore conditions.
The life histories of sea lions contributes significantly to their domoic acid exposure and resulting stranding dynamics, particularly for acute exposure scenarios (Bargu et al., 2010). The main breeding colonies along the U.S. West Coast for sea lions are located on the Santa Barbara Channel Islands, including San Miguel, San Nicolas, Santa Barbara and San Clemente Islands (Lowry and Forney, 2005). San Miguel, which is about 75 km offshore of Stearns Wharf and the most northern of the island chain, is the largest of the sea lion rookeries in the region (Le Boeuf and Bonnell, 1980; Lowry et al., 2021). The distribution of sea lions along the coast varies based on age and sex (Melin et al., 2000; Bargu et al., 2010). Our study focused on subadult and adult sea lions, which are either on the onset of sexual maturity or are sexually mature (Sinai et al., 2014). The breeding season occurs between May and August, during which time sexually mature males and females reside on the rookeries. Males rarely leave the islands to forage during the breeding season in order to maintain their territories (Bargu et al., 2010). Males spend the remainder of the year foraging along broad stretches of the U.S. West Coast as far north as Washington state (Weise et al., 2006). Female sea lions give birth between June and July, and typically remain with their offspring for the duration of their lactation period, which typically lasts for about one year (Gerber et al., 2010). During this period, these females split their time between nursing and foraging both offshore and on the continental shelf around the rookeries (Melin et al., 2000). Non-lactating females, however, disperse along the California coast following the breeding season (Melin et al., 2000). Domoic acid-producing blooms exhibit a strong seasonality in Southern California, being most common in the spring and early summer (Smith et al., 2018), overlapping with the latter half of the maternal care period for lactating females and the beginning of the breeding season. Sea lions prey on several fish species known to accumulate domoic acid, including sardines and anchovies (Bernstein et al., 2021). Thus, the proximity of Stearns Wharf to the rookeries on the Channel Islands, and San Miguel in particular, may contribute to the consistent significance of the pDA observations at this location in our different stranding scenario models since a large proportion of the sea lion population resides and feeds in that area when bloom events are common in in Southern California. However, sea lions that spend time at the smaller rookeries located throughout the Southern California region also can strand along the Orange County coastline during bloom events, but likely make up a smaller proportion of the local population and thus contribute to the weaker relationships with the other pier locations.
Most of the adult and subadult sea lions rescued by PMMC during the study period with symptoms of domoic acid intoxication were female, highlighting that bloom timing and sea lion ecology influences stranding demographics. This was particularly apparent during the 2017 bloom event that initiated in April and ended in June. This overlapped with the period when many of the region’s female sea lions would be foraging around the Channel Islands and surrounding regions. These observations are consistent with a stranding demographics study that indicated that adult females were between 47%-82% of the domoic acid cases reported by members of the California Marine Mammal Stranding Network between 1998-2006 (Bejarano et al., 2008a). Bargu et al. (2010) has previously highlighted how stranding demographics in Monterey Bay, CA may be related to temporal bloom dynamics. A bloom in the Monterey Bay region in March and April of 2007 was linked to the stranding of adult and subadult male sea lions, who were likely exposed to the bloom as they migrated though the area from the north to the breeding sites in the south (Bargu et al., 2010). The timing of blooms may therefore result in stronger impacts on specific demographics of the population, which has important population level effects in marine mammals that are currently understudied (Bejarano et al., 2008b).
The present study focused on the stranding patterns of adult and subadult sea lions, which likely contributed to the clearer relationship with domoic acid concentrations in the water than in previous studies. The causes of sea lion strandings are multiple, with some of the most commonly reported causes along the California coast being malnutrition, leptospirosis infections, and trauma (Greig et al., 2005). Pups and yearlings are typically the most numerous age class encountered by stranding networks and they most often strand due to malnutrition (Greig et al., 2005). Furthermore, the domoic acid intoxication cases in this study were identified based on published behavioral diagnostic criteria (Gulland et al., 2002; Goldstein et al., 2008) and analytical confirmation of the toxin in patient fluids was not conducted. Although analytical confirmation of domoic acid in animal fluids or other diagnostic testing is ideal for confirming a diagnosis, the behavioral diagnostic criteria, particularly for acute cases, are quite distinctive from many of the other leading causes of stranding in older sea lions. Thus, by excluding younger age class sea lions from our analyses, we were able to minimize the inclusion of sea lions that stranded for reasons other than domoic acid toxicosis and effectively model the relationship between domoic acid presence in the environment and related stranding events for multiple stranding scenarios.
The results of the present study are most applicable to linking environmental observations of domoic acid to stranding events related to acute intoxications. Acute intoxication events typically involve temporally clustered strandings, while sea lions presenting with signs of chronic domoic acid exposure often strand more sporadically and might be decoupled from significant bloom events (Goldstein et al., 2008). Our models all looked for temporal correlations between domoic acid in the environment and strandings and therefore, describe strandings related to acute, rather than chronic, exposures. When examining routine environmental observations of domoic acid and toxigenic Pseudo-nitzschia species from 2004-2007 in Monterey Bay, Bargu et al. (2012) reported a weak relationship between stranded sea lions of all age classes with signs of both acute and chronic domoic acid exposure. The relationship was improved, however, when only sea lions with acute symptomatology were included in the analysis (Bargu et al., 2012). Goldstein et al. (2008) reported a four-month time lag between sea lions that stranded with signs of acute domoic acid exposure and those that stranded with signs of chronic exposure along the central and northern coast of California. These observations all point to the decoupling between bloom dynamics and sea lions that strand with chronic or low level domoic acid exposure, making these types of strandings much harder to model and predict.
Sea lion stranding data are challenging to analyze using many conventional time series approaches because they are autocorrelated count data. While ARIMA approaches (Shumway and Stoffer, 2010; Box et al., 2015), including general additive mixed models with autocorrelation functions (Cram et al., 2015) are conventional for time series data, these approaches expect the dependent variable to be normally distributed and so are not suitable for our count data, which are better modeled with a Poisson distribution. Similarly, while Poisson regression approaches can be used for count data (James et al., 2013), and while general additive models that assume Poisson or negative binomial distributed data are common for count data (Zuur et al., 2009), these approaches do not account for autocorrelated data. Hierarchical Bayesian approaches offer a possible solution to this problem (Tunaru, 2002), though we note that the ability to work with autocorrelated Poisson data was removed from the commonly used BRMS package (Buerkner, 2016), so implementation of such an approach likely requires additional validation. Random forest models may provide advantages for prediction (Kane et al., 2014) as they can handle nonlinear interactions between predictors (James et al., 2013), however general linear model based approaches have the advantage of returning coefficients for the different parameters and therefore being more interpretable. Therefore, we selected the GLARMA approach to model these data and anticipate that it will continue to be useful for future time series of sea lion strandings and similar phenomena.
An additional consideration is that pDA concentrations were sampled only at a single moment in time, whereas sea lions are likely responding to average pDA concentration in the environment, including rapid shifts in pDA concentrations that occur between sampling events. Thus, higher resolution sampling, or sampling using methods that integrate over time, might provide data that more closely relate to strandings. For example, the use of passive samplers like solid phase adsorption toxin tracking samplers, which have been shown to provide an early indication of the presence of domoic acid in shellfish compared to water grab samples (Lane et al., 2010), could address this issue. Thus, the variability of water grab samples could lead to somewhat lower sensitivity than would be found by other methods, and thus our relationships between pDA concentration and strandings could be underestimated.
One major goal of this study was to identify pDA concentrations of concern by identifying pDA levels that are associated with elevated stranding probabilities. Therefore, to identify such a threshold, we used a simple Poisson regression model to determine the probabilities of one or two strandings, at different domoic acid concentrations, ignoring previous stranding levels. This simpler modeling approach was reasonable given that the GLARMA models, along with multiple mechanistic and field studies, have demonstrated associations between domoic acid and strandings that were not purely due to autocorrelation. Ultimately, this allowed for the identification of potential pDA levels of concern independently of summarizing recent stranding histories, which increases the utility of observations of pDA for assessing the risk of negative ecosystem impacts based on an observation of a given concentration.
The spatial and temporal heterogeneity in domoic acid-producing blooms and sea lion habitat usage has previously made it difficult to link environmental observations to specific ecosystem impacts. Developing more quantitative linkages between environmental algal toxin concentrations and ecosystem effects is beneficial for both HAB ecologists and marine mammal stranding networks. The present study focused on a five-year period between 2015 and 2019, during which only one major domoic acid related stranding event occurred in 2017. The development of longer term stranding time series of domoic acid related stranding cases should be continued to refine our statistical models through the inclusion of multiple major events, which, unfortunately, are likely to occur in the future. Future studies should explore these relationships across longer timescales to better understand the additional influences of low frequency changes in the environment, such as shifting climate modes, which might cause variations in both bloom development, marine mammal behavior and domoic acid intoxication patterns. Our analysis was also limited to stranding events occurring along the Orange County coastline. Relationships between environmental concentrations of domoic acid and strandings over broader geographic areas should also be examined, since there is potentially substantial variability in both bloom dynamics and localized marine mammal ecology over larger regions.
The relationship between offshore and subsurface blooms in the region and marine mammal stranding is not well studied due to the sparsity of routine offshore HAB observations. Domoic acid has been detected in several different marine mammal species stranded in Southern California that are known to forage in offshore pelagic and benthic habitats (Torres De La Riva et al., 2009; Fire et al., 2010). In some cases, marine mammals, including sea lions, strand and display signs of acute domoic acid exposure in advance of observations of toxigenic Pseudo-nitzschia cells in the nearshore environment (Torres De La Riva et al., 2009; Bargu et al., 2012; Broadwater et al., 2018), pointing to the occurrence of offshore events that precede nearshore bloom observations. Using cross correlation functions, we also observed evidence of sea lion strandings slightly leading observations of increased pDA at most pier locations in our time series (Supplementary Figure 1), which could be attributed to sea lions being exposed to domoic acid related to offshore and/or subsurface blooms that were not yet detected at the pier locations. More routine observations of offshore and subsurface bloom dynamics regionally, such as those described in Frolov et al. (2013), should be prioritized. These types of observations would help to better resolve their connectivity (or lack thereof) to nearshore bloom dynamics and would likely improve the relationship and potential predictive power of future forecasting efforts. The statistical approaches employed in our study could be applied to future time series of offshore monitoring data to evaluate how it might improve forecasting of sea lion strandings from environmental monitoring data. Until these offshore and subsurface monitoring data are more readily available, comparing the routine pier monitoring observations with monitoring of sea lions strandings in Southern California is a useful approach to direct when it is necessary to perform offshore and subsurface monitoring for domoic acid. Pier based monitoring facilitates more rapid identification of these harder to detect bloom events and comparing pDA to strandings allows identification of when domoic acid contaminates ecologically and economically important fisheries.
We identified pDA concentrations of potential concern based on the probability that specific concentrations could result in ecosystem impacts, with 0.05 μg/L pDA and 0.25 μg/L pDA representing two potential levels of concern based on increasing risk of sea lion stranding events. Ultimately, developing these types of relationships can help to move towards the ability to forecast marine mammal stranding events related to HABs. The capacity to model and forecast domoic acid-producing blooms is increasing; it would be beneficial to link these improved forecasts to ecosystem impacts, such as marine mammal strandings. Currently, the California coast has an operational forecasting model, California Harmful Algal Risk Mapping (C-HARM), however C-HARM does not currently link to marine mammal strandings (Anderson et al., 2016). With additional research, C-HARM or similar model products could be expanded to also consider marine mammal stranding risks based on these pDA levels of concern, thereby linking the model outputs to ecosystem effects.
Harmful algal bloom observations made by Cal-HABMAP are publicly available on the program website: https://calhabmap.org/. California sea lion stranding records can be found at the following https://doi.org/10.5281/zenodo.8433164. Data analysis code can be found at: https://doi.org/10.6084/m9.figshare.24415654.
Ethical approval was not required for the studies on animals in accordance with the local legislation and institutional requirements because our study used existing data on marine mammal stranding demographic and case histories, which are collected as a part of routine work at the Pacific Marine Mammal Center.
JS: Conceptualization, Data curation, Formal Analysis, Funding acquisition, Methodology, Project administration, Writing – original draft, Writing – review & editing, Visualization. JC: Conceptualization, Formal Analysis, Methodology, Writing – original draft, Writing – review & editing, Data curation, Visualization. MB: Conceptualization, Data curation, Writing – review & editing, Visualization. VH: Conceptualization, Data curation, Writing – original draft, Writing – review & editing. DS: Conceptualization, Data curation, Formal Analysis, Methodology, Writing – review & editing, Visualization. AD: Conceptualization, Data curation, Formal Analysis, Methodology, Writing – original draft, Writing – review & editing.
The author(s) declare financial support was received for the research, authorship, and/or publication of this article. All funding to support the Pacific Marine Mammal Center’s rescue and rehabilitation efforts for California sea lions were made possible by generous donors and community support. This paper is a result of research funded by the National Oceanic and Atmospheric Administration (NOAA) National Centers for Coastal Ocean Science (NCCOS) Competitive Research Program under Ecosystem and Harmful Algal Bloom (ECOHAB) Award NA19NOS4780181 and with NCCOS HAB Event Response funding.
The authors wish to thank the principal investigators, field staff and students associated with Cal-HABMAP pier monitoring efforts funded by the Southern California Coastal Ocean Observing System for collecting sustained observations of domoic acid since 2008. We also thank the animal care staff, volunteers, and donors at the Pacific Marine Mammal Center who assisted and supported rescue and rehabilitation efforts of the stranded marine mammals for the duration of this study. We wish to acknowledge the National Centers for Coastal Ocean Science (NCCOS) HAB Event Response Program for supporting offshore sampling efforts in 2017. We thank Meredith D. A. Howard, David A. Caron, Raphael M. Kudela, and Clarissa Anderson for coordinating event response sampling and analysis efforts in 2017. We also thank the Orange County Sanitation District, City of Los Angeles Environmental Monitoring Division, and the Santa Barbara Channelkeepers for offshore field sampling assistance. This manuscript has been posted as a preprint on the bioRxiv server (https://doi.org/10.1101/2023.08.15.549094). This is NCCOS ECOHAB publication #ECO1078 and HAB Event Response publication #ER34.
The authors declare that the research was conducted in the absence of any commercial or financial relationships that could be construed as a potential conflict of interest.
All claims expressed in this article are solely those of the authors and do not necessarily represent those of their affiliated organizations, or those of the publisher, the editors and the reviewers. Any product that may be evaluated in this article, or claim that may be made by its manufacturer, is not guaranteed or endorsed by the publisher.
The Supplementary Material for this article can be found online at: https://www.frontiersin.org/articles/10.3389/fmars.2023.1278293/full#supplementary-material
Anderson C. R., Kudela R. M., Kahru M., Chao Y., Rosenfeld L. K., Bahr F. L., et al. (2016). Initial skill assessment of the California Harmful Algae Risk Mapping (C-HARM) system. Harmful Algae 59, 1–18. doi: 10.1016/j.hal.2016.08.006
Anderson D. M., Fensin E., Gobler C. J., Hoeglund A. E., Hubbard K. A., Kulis D. M., et al. (2021). Marine harmful algal blooms (HABs) in the United States: History, current status and future trends. Harmful Algae 102, 101975. doi: 10.1016/j.hal.2021.101975
Bargu S., Goldstein T., Roberts K., Li C., Gulland F. (2012). Pseudo-nitzschia blooms, domoic acid, and related California sea lion strandings in Monterey Bay, California. Mar. Mammal Sci. 28, 237–253. doi: 10.1111/j.1748-7692.2011.00480.x
Bargu S., Silver M., Goldstein T., Roberts K., Gulland F. (2010). Complexity of domoic acid-related sea lion strandings in Monterey Bay, California: foraging patterns, climate events, and toxic blooms. Mar. Ecol. Prog. Ser. 418, 213–222. doi: 10.3354/meps08816
Bates S. S., Bird C. J., Freitas A. S. W., Foxall R., Gilgan M., Hanic L. A., et al. (1989). Pennate diatom nitzschia pungens as the primary source of domoic acid, a toxin in shellfish from Eastern Prince Edward Island, Canada. Can. J. Fish. Aquat. Sci. 46, 1203–1215. doi: 10.1139/f89-156
Bates S. S., Hubbard K. A., Lundholm N., Montresor M., Leaw C. P. (2018). Pseudo-nitzschia, Nitzschia, and domoic acid: New research since 2011. Harmful Algae 79, 3–43. doi: 10.1016/j.hal.2018.06.001
Bejarano A. C., Gulland F. M., Goldstein T., St Leger J., Hunter M., Schwacke L. H., et al. (2008a). Demographics and spatio-temporal signature of the biotoxin domoic acid in California sea lion (Zalophus californianus) stranding records. Mar. Mammal Sci. 24, 899–912. doi: 10.1111/j.1748-7692.2008.00224.x
Bejarano A. C., VanDola F. M., Gulland F. M., Rowles T. K., Schwacke L. H. (2008b). Production and toxicity of the marine biotoxin domoic acid and its effects on wildlife: a review. Hum. Ecol. Risk Assessment: Int. J. 14, 544–567. doi: 10.1080/10807030802074220
Bernstein S., Ruiz-Cooley R. I., Kudela R., Anderson C. R., Dunkin R., Field J. C. (2021). Stable isotope analysis reveals differences in domoic acid accumulation and feeding strategies of key vectors in a California hotspot for outbreaks. Harmful Algae 110, 102117. doi: 10.1016/j.hal.2021.102117
Bossart G. D. (2011). Marine mammals as sentinel species for oceans and human health. Vet. Pathol. 48, 676–690. doi: 10.1177/0300985810388525
Bowers H. A., Ryan J. P., Hayashi K., Woods A. L., Marin R., Smith G. J., et al. (2018). Diversity and toxicity of Pseudo-nitzschia species in Monterey Bay: Perspectives from targeted and adaptive sampling. Harmful Algae 78, 129–141. doi: 10.1016/j.hal.2018.08.006
Box G. E., Jenkins G. M., Reinsel G. C., Ljung G. M. (2015). Time series analysis: forecasting and control (Hoboken, New Jersey: John Wiley & Sons).
Broadwater M. H., Van Dolah F. M., Fire S. E. (2018). “Vulnerabilities of marine mammals to harmful algal blooms,” in Harmful algal blooms: a desk compendium. Eds. Shumway S. E., Burkholder J. M., Morton S. L. (Hoboken, New Jersey: John Wiley & Sons), 191–222. doi: 10.1002/9781118994672.ch5
Brodie E. C., Gulland F. M., Greig D. J., Hunter M., Jaakola J., Leger J. S., et al. (2006). Domoic acid causes reproductive failure in California sea lions (Zalophus californianus). Mar. Mammal Sci. 22, 700–707. doi: 10.1111/j.1748-7692.2006.00045.x
Buerkner P. (2016). AR in negbinomial model · Issue 77 · paul-buerkner/brms (GitHub). Available at: https://github.com/paul-buerkner/brms/issues/77 (Accessed December 10, 2022).
Cook P. F., Reichmuth C., Rouse A., Dennison S., Van Bonn B., Gulland F. (2016). Natural exposure to domoic acid causes behavioral perseveration in Wild Sea lions: Neural underpinnings and diagnostic application. Neurotoxicol. Teratol. 57, 95–105. doi: 10.1016/j.ntt.2016.08.001
Cook P. F., Reichmuth C., Rouse A. A., Libby L. A., Dennison S. E., Carmichael O. T., et al. (2015). Algal toxin impairs sea lion memory and hippocampal connectivity, with implications for strandings. Science 350, 1545–1547. doi: 10.1126/science.aac5675
Cram J. A., Chow C.-E. T., Sachdeva R., Needham D. M., Parada A. E., Steele J. A., et al. (2015). Seasonal and interannual variability of the marine bacterioplankton community throughout the water column over ten years. ISME J. 9, 563–580. doi: 10.1038/ismej.2014.153
Dunsmuir W. T. (2015). “Generalized linear autoregressive moving average models,” in Handbook of discrete-valued time series, CRC monographs. Eds. Davis R., Holan S., Lund R., Ravishanker N. (Boca Raton, FL: CRC Press), Chapter 3, pp. 51–57.
Dunsmuir W. T. M., Scott D. J. (2015). The glarma package for observation-driven time series regression of counts. J. Stat. Softw. 67, 1–36. doi: 10.18637/jss.v067.i07
Fire S. E., Wang Z., Berman M., Langlois G. W., Morton S. L., Sekula-Wood E., et al. (2010). Trophic transfer of the harmful algal toxin domoic acid as a cause of death in a minke whale (Balaenoptera acutorostrata) stranding in Southern California. Aquat. Mammals 36, 342–350. doi: 10.1578/AM.36.4.2010.342
Frolov S., Kudela R. M., Bellingham J. G. (2013). Monitoring of harmful algal blooms in the era of diminishing resources: A case study of the U.S. West Coast. Harmful Algae 21–22, 1–12. doi: 10.1016/j.hal.2012.11.001
Gerber L. R., González-Suárez M., Hernández-Camacho C. J., Young J. K., Sabo J. L. (2010). The cost of male aggression and polygyny in California sea lions (Zalophus californianus). PloS One 5, e12230. doi: 10.1371/journal.pone.0012230
Gibble C. M., Kudela R. M., Knowles S., Bodenstein B., Lefebvre K. A. (2021). Domoic acid and saxitoxin in seabirds in the United States between 2007 and 2018. Harmful Algae 103, 101981. doi: 10.1016/j.hal.2021.101981
Goldstein T., Mazet J. A. K., Zabka T. S., Langlois G., Colegrove K. M., Silver M., et al. (2008). Novel symptomatology and changing epidemiology of domoic acid toxicosis in California sea lions (Zalophus californianus): an increasing risk to marine mammal health. Proc. R. Soc B. 275, 267–276. doi: 10.1098/rspb.2007.1221
Greig D. J., Gulland F. M. D., Kreuder C. (2005). A decade of live California sea lion (Zalophus californianus) strandings along the Central California coast: causes and trends 1991-2000. Aquat. Mammals 31, 11–22. doi: 10.1578/AM.31.1.2005.11
Gulland E. M., Haulena M., Fauquier D., Lander M., Zabka T., Duerr R., et al. (2002). Domoic acid toxicity in Californian sea lions (Zalophus californianus): clinical signs, treatment and survival. Vet. Rec. 150, 475–480. doi: 10.1136/vr.150.15.475
Gulland F. M. D., Baker J., Howe M., LaBrecque E., Leach L., Moore S. E., et al. (2022). A review of climate change effects on marine mammals in United States waters: Past predictions, observed impacts, current research and conservation imperatives. Climate Change Ecol. 3, 100054. doi: 10.1016/j.ecochg.2022.100054
James G., Witten D., Hastie T., Tibshirani R. (2013). An introduction to statistical learning (New York, New York: Springer).
Kane M. J., Price N., Scotch M., Rabinowitz P. (2014). Comparison of ARIMA and Random Forest time series models for prediction of avian influenza H5N1 outbreaks. BMC Bioinf. 15, 276. doi: 10.1186/1471-2105-15-276
Kudela R. M., Bickel A., Carter M. L., Howard M. D. A., Rosenfeld L. (2015). “The monitoring of harmful algal blooms through ocean observing: the development of the California harmful algal bloom monitoring and alert program in coastal ocean observing system,” in coastal ocean observing systems. Eds. Liu Y., Kerkering H., Weisberg R. H.. (New York, New York: Academic Press), 58–75. doi: 10.1016/B978-0-12-802022-7.00005-5
Kvitek R. G., Goldberg J. D., Smith G. J., Doucette G. J., Silver M. W. (2008). Domoic acid contamination within eight representative species from the benthic food web of Monterey Bay, California, USA. Mar. Ecol. Prog. Ser. 367, 35–47. doi: 10.3354/meps07569
Lane J. Q., Roddam C. M., Langlois G. W., Kudela R. M. (2010). Application of Solid Phase Adsorption Toxin Tracking (SPATT) for field detection of the hydrophilic phycotoxins domoic acid and saxitoxin in coastal California. Limnol. Oceanogr.: Methods 8, 645–660. doi: 10.4319/lom.2010.8.0645
Le Boeuf B. J., Bonnell M. L. (1980). Pinnipeds of the California Islands: abundance and distribution, In: Power D.M. (Ed.), The California Islands: Proceedings of a Multidisciplinary Symposium. Santa Barbara Museum of Natural History, Santa Barbara, CA, pp. 475–496.
Lefebvre K. A., Bargu S., Kieckhefer T., Silver M. W. (2002a). From sanddabs to blue whales: the pervasiveness of domoic acid. Toxicon 40, 971–977. doi: 10.1016/S0041-0101(02)00093-4
Lefebvre K. A., Kendrick P. S., Ladiges W., Hiolski E. M., Ferriss B. E., Smith D. R., et al. (2017). Chronic low-level exposure to the common seafood toxin domoic acid causes cognitive deficits in mice. Harmful Algae 64, 20–29. doi: 10.1016/j.hal.2017.03.003
Lefebvre K. A., Powell C. L., Busman M., Doucette G. J., Moeller P. D. R., Silver J. B., et al. (1999). Detection of domoic acid in northern anchovies and california sea lions associated with an unusual mortality event. Nat. Toxins 7, 85–92. doi: 10.1002/(SICI)1522-7189(199905/06)7:3<85::AID-NT39>3.0.CO;2-Q
Lefebvre K., Silver M., Coale S., Tjeerdema R. (2002b). Domoic acid in planktivorous fish in relation to toxic Pseudo-nitzschia cell densities. Mar. Biol. 140, 625–631. doi: 10.1007/s00227-001-0713-5
Litaker R. W., Stewart T. N., Eberhart B.-T. L., Wekell J. C., Trainer V. L., Kudela R. M., et al. (2008). Rapid enzyme-linked immunosorbent assay for detection of the algal toxin domoic acid. J. Shellfish Res. 27, 1301–1310. doi: 10.2983/0730-8000-27.5.1301
Lowry M. S., Forney K. A. (2005). Abundance and distribution of California sea lions (Zalophus californianus) in central and northern California during 1998 and summer 1999. Fishery Bulletin, 103.
Lowry M. S., Jaime E. M., Moore J. E. (2021). Abundance and distribution of pinnipeds at the Channel Islands in southern California, central and northern California, and southern Oregon during summer 2016−2019. NOAA Tech Mem NOAA-TM-NMFS-SWFSC-656. doi: 10.25923/6qhf-0z55
McCabe R. M., Hickey B. M., Kudela R. M., Lefebvre K. A., Adams N. G., Bill B. D., et al. (2016). An unprecedented coastwide toxic algal bloom linked to anomalous ocean conditions. Geophys Res. Lett. 43, 10–366. doi: 10.1002/2016GL070023
Melin S. R., Delong R. L., Thomason J. R., Vanblaricom G. R. (2000). Attendance patterns of California sea lion (Zalophus californianus) females and pups during the non-breeding season at San Miguel island. Mar. Mammal Sci. 16, 169–185. doi: 10.1111/j.1748-7692.2000.tb00911.x
Melin S. R., Orr A. J., Harris J. D., Laake J. L., DeLong R. L. (2012). California sea lions: an indicator for integrated ecosystem assessment of the California current system. California Cooperative Oceanic Fisheries Investigations Rep. 53, 140–152.
Moore S. K., Dreyer S. J., Ekstrom J. A., Moore K., Norman K., Klinger T., et al. (2020). Harmful algal blooms and coastal communities: Socioeconomic impacts and actions taken to cope with the 2015 U.S. West Coast domoic acid event. Harmful Algae 96, 101799. doi: 10.1016/j.hal.2020.101799
Moriarty M. E., Tinker M. T., Miller M. A., Tomoleoni J. A., Staedler M. M., Fujii J. A., et al. (2021). Exposure to domoic acid is an ecological driver of cardiac disease in southern sea otters. Harmful Algae 101, 101973. doi: 10.1016/j.hal.2020.101973
Ramsdell J., Gulland F. (2014). Domoic acid epileptic disease. Mar. Drugs 12, 1185–1207. doi: 10.3390/md12031185
Rice J. C., Rochet M.-J. (2005). A framework for selecting a suite of indicators for fisheries management. ICES J. Mar. Sci. 62, 516–527. doi: 10.1016/j.icesjms.2005.01.003
Sandoval-Belmar M., Smith J., Moreno A. R., Anderson C., Kudela R. M., Sutula M., et al. (2023). A cross-regional examination of patterns and environmental drivers of Pseudo-nitzschia harmful algal blooms along the California coast. Harmful Algae 126, 102435. doi: 10.1016/j.hal.2023.102435
Scholin C. A., Gulland F., Doucette G. J., Benson S., Busman M., Chavez F. P., et al. (2000). Mortality of sea lions along the central California coast linked to a toxic diatom bloom. Nature 403, 80–84. doi: 10.1038/47481
Seegers B. N., Birch J. M., Marin R., Scholin C. A., Caron D. A., Seubert E. L., et al. (2015). Subsurface seeding of surface harmful algal blooms observed through the integration of autonomous gliders, moored environmental sample processors, and satellite remote sensing in southern California: Harmful algal blooms subsurface seeding. Limnol. Oceanogr. 60, 754–764. doi: 10.1002/lno.10082
Seubert E. L., Gellene A. G., Howard M. D. A., Connell P., Ragan M., Jones B. H., et al. (2013). Seasonal and annual dynamics of harmful algae and algal toxins revealed through weekly monitoring at two coastal ocean sites off southern California, USA. Environ. Sci. pollut. Res. 20, 6878–6895. doi: 10.1007/s11356-012-1420-0
Shumway R. H., Stoffer D. S. (2010). Time series analysis and its applications: With R Examples (Cham, Switzerland: Springer International Publishing).
Silvagni P., Lowenstine L. J., Spraker T., Lipscomb T., Gulland F. (2005). Pathology of domoic acid toxicity in California sea lions (Zalophus californianus). Veterinary Pathol. 42, 184–191. doi: 10.1354/vp.42-2-184
Simeone C. A., Gulland F. M. D., Norris T., Rowles T. K. (2015). A systematic review of changes in marine mammal health in North America 1972-2012: the need for a novel integrated approach. PloS One 10, e0142105. doi: 10.1371/journal.pone.0142105
Sinai N. L., Dadaian R. H., Kass P. H., Verstraete F. J. M. (2014). Dental pathology of the California sea lion (Zalophus californianus). J. Comp. Pathol. 151, 113–121. doi: 10.1016/j.jcpa.2014.02.004
Smith J., Connell P., Evans R. H., Gellene A. G., Howard M. D., Jones B. H., et al. (2018). A decade and a half of Pseudo-nitzschia spp. and domoic acid along the coast of southern California. Harmful algae 79, 87–104. doi: 10.1016/j.hal.2018.07.007
Smith J., Shultz D., Howard M. D. A., Robertson G., Phonsiri V., Renick V., et al. (2021). Persistent domoic acid in marine sediments and benthic infauna along the coast of Southern California. Harmful Algae 108, 102103. doi: 10.1016/j.hal.2021.102103
Torres de la Riva G., Johnson C. K., Gulland F. M., Langlois G. W., Heyning J. E., Rowles T. K., et al. (2009). Association of an unusual marine mammal mortality event with Pseudo-nitzschia spp. blooms along the southern California coastline. J. wildlife Dis. 45, 109–121. doi: 10.7589/0090-3558-45.1.109
Trainer V. L., Bates S. S., Lundholm N., Thessen A. E., Cochlan W. P., Adams N. G., et al. (2012). Pseudo-nitzschia physiological ecology, phylogeny, toxicity, monitoring and impacts on ecosystem health. Harmful Algae 14, 271–300. doi: 10.1016/j.hal.2011.10.025
Trainer V. L., Moore S. K., Hallegraeff G., Kudela R. M., Clement A., Mardones J. I., et al. (2020). Pelagic harmful algal blooms and climate change: Lessons from nature’s experiments with extremes. Harmful Algae 91, 101591. doi: 10.1016/j.hal.2019.03.009
Tunaru R. (2002). Hierarchical Bayesian models for multiple count data. Austrian J. Stat 31 (2&3), 221–229.
Umhau B. P., Benitez-Nelson C. R., Anderson C. R., McCabe K., Burrell C. (2018). A time series of water column distributions and sinking particle flux of Pseudo-nitzschia and domoic acid in the Santa Barbara Basin, California. Toxins 10, 480. doi: 10.3390/toxins10110480
Vigilant V. L., Silver M. W. (2007). Domoic acid in benthic flatfish on the continental shelf of Monterey Bay, California, USA. Mar. Biol. 151, 2053–2062. doi: 10.1007/s00227-007-0634-z
Weise M. J., Costa D. P., Kudela R. M. (2006). Movement and diving behavior of male California sea lion (Zalophus californianus) during anomalous oceanographic conditions of 2005 compared to those of 2004. Geophys. Res. Lett. 33, L22S10. doi: 10.1029/2006GL027113
Wells M. L., Karlson B., Wulff A., Kudela R., Trick C., Asnaghi V., et al. (2020). Future HAB science: Directions and challenges in a changing climate. Harmful Algae 91, 101632. doi: 10.1016/j.hal.2019.101632
Keywords: harmful algal blooms, domoic acid, Pseudo-nitzschia, California sea lions, marine mammal strandings, food webs
Citation: Smith J, Cram JA, Berndt MP, Hoard V, Shultz D and Deming AC (2023) Quantifying the linkages between California sea lion (Zalophus californianus) strandings and particulate domoic acid concentrations at piers across Southern California. Front. Mar. Sci. 10:1278293. doi: 10.3389/fmars.2023.1278293
Received: 16 August 2023; Accepted: 10 November 2023;
Published: 01 December 2023.
Edited by:
Ibon Galparsoro, Technological Center Expert in Marine and Food Innovation (AZTI), SpainReviewed by:
Ailsa Hall, University of St Andrews, United KingdomCopyright © 2023 Smith, Cram, Berndt, Hoard, Shultz and Deming. This is an open-access article distributed under the terms of the Creative Commons Attribution License (CC BY). The use, distribution or reproduction in other forums is permitted, provided the original author(s) and the copyright owner(s) are credited and that the original publication in this journal is cited, in accordance with accepted academic practice. No use, distribution or reproduction is permitted which does not comply with these terms.
*Correspondence: Jayme Smith, amF5bWVzQHNjY3dycC5vcmc=
†These authors share first authorship
Disclaimer: All claims expressed in this article are solely those of the authors and do not necessarily represent those of their affiliated organizations, or those of the publisher, the editors and the reviewers. Any product that may be evaluated in this article or claim that may be made by its manufacturer is not guaranteed or endorsed by the publisher.
Research integrity at Frontiers
Learn more about the work of our research integrity team to safeguard the quality of each article we publish.