- 1IMC, International Marine Centre, Oristano, Italy
- 2NBFC, National Biodiversity Future Center, Palermo, Italy
Maritime Spatial Planning (MSP) promotes the sustainable human activities development and uses in the marine space, playing a role in their effective management. The enhancement of connectivity is crucial for the conservation of biodiversity and landscape planning. Ecological Corridors (ECs) are an important type of connectivity for biodiversity conservation in fragmented habitats. The EU Biodiversity Strategy 2030 includes ECs into the network of protected areas and allows for the creation of additional protected areas. MSP studies considering ECs remain still lacking, especially for the design of networks between Marine Protected Areas (MPAs) and Other Effective area-based Conservation Measures (OECMs). In this paper, knowledge, and tools for investigating marine ECs were reviewed, with a systematic bibliometric analysis to summarize the current scientific research. Previous studies integrating ecological connectivity into planning for marine conservation have focused on models of larval dispersal, adult movements, and dispersal of single species by using benthic habitat proxies. Few studies were found on ECs in marine environments: in the coral Caribbean reef systems in the Gulf of Mexico; within benthic habitats along the Pacific coast of Canada; between MPAs in British Columbia (Canada); and by analyzing migratory species in the Yangtze estuary (China). Commonly used approaches to project and map ECs in marine environments are least-cost and circuit theories allowing to incorporate movement with cost or resistance to movement, depending on species and preferred habitats. The systematic bibliometric analysis returned 25 studies, most of which were from North America (40%) and European countries (36%) and the largest share of papers (68%) from 2018 to 2022. This review pinpointed the need of integrating different disciplines to investigate connectivity and the need by policymakers and practitioners to recognize the importance of ecological connectivity, even there are significant challenges for integrating connectivity into policies, planning, and conservation.
1 Introduction
Maritime Spatial Planning (MSP) is a strategy defined by the EU Directive 2014/89/EU as an integrative public process that establish a framework whereby authorities in relevant Member States allocate human activity in maritime space. MSP goals include fostering the long-term expansion of the maritime economy, the development of marine regions, and the exploitation of marine resources by integrating ecological, economic, and social objectives (e.g.: Gilliland and Laffoley, 2008; Halpern et al., 2008; Stelzenmüller et al., 2010; Micheli et al., 2013; Kelly et al., 2014; Halpern et al., 2015; Da Luz Fernandes et al., 2018). MSP outcomes can result in plans, permits, strategies, planning concepts, guidelines, governance principles, and other administrative decisions about the spatio-temporal allocation of present and future human activities and uses in maritime space (https://maritime-spatial-planning.ec.europa.eu/msp-eu/introduction-msp1). Furthermore, MSP promotes the reduction of conflicts and the creation of synergies and cooperation between sectors and EU countries, and the creation of protected area networks to safeguard and maintain the environment by recognizing the effects and opportunities for space utilization, raising stakeholder awareness (https://maritime-spatial-planning.ec.europa.eu/msp-eu/introduction-msp1). MSP necessitates spatial information regarding marine resources, biodiversity, habitat, ecosystems, and human activities to effectively manage them (Ban et al., 2010; Da Luz Fernandes et al., 2018; Margules and Pressey, 2000; Pressey et al., 2007; Muñoz et al., 2017).
In this context, the protection and enhancement of natural connectivity is a challenging topic for biodiversity conservation and landscape planning (Ersoy et al., 2018; Fang et al., 2018; Pereira, 2018; Guzmán-Colón et al., 2020; Tulloch et al., 2021). Despite its ecological importance, connectivity in marine environments is challenging to assess due to limited data and the fact that it encompasses several ecological processes such as dispersal (by larvae, juveniles, and adults), oceanographic conditions, ontogenetic shifts, migration, nutrient flow, invasive species, anthropogenic impacts, or diseases (Gillanders et al., 2003; Robinson et al., 2005; Blowes and Connolly, 2012; Friesen et al., 2019).
Conservation in Europe is carried out with regulatory frameworks such as the Birds and Habitats Directives and the Marine Strategy Framework Directive (Leontiou et al., 2022) resulting in the designation of the Natura 2000 network, which currently covers almost 10% of the entire EU maritime area (more than 3,150 marine Natura 2000 sites, over 550,000 km2) (https://ec.europa.eu/2). In addition, the rate of Marine Protected Areas (MPAs) is increasing worldwide as a response to the UN Convention on Biological Diversity (2004) to effectively protect and conserve at least 10% of marine ecoregions through MPAs that are ecologically representative and well connected. Currently, a total of 18,384 MPAs and 818 Other Effective area-based Conservation Measures (OECMs) exist, covering the 8.26% of the world’s oceans, and less than a third of these are adequately connected (Saura et al., 2018; UNEP-WCMC, 2023).
Despite the EU’s concerted efforts in the identification of conservation zones, biodiversity loss continues at worrying rates (European Commission, 2021). At the European level, MPAs cover 12% of the seas, and only 1% are strictly protected. In this context, the action plan to foster the protection of EU marine ecosystem and reduce the impact of fishing activities was set through the new EU Biodiversity Strategy for 2030 (European Commission, 2021). This strategy established as main objective the protection of 30% of the EU seas, of which 10% strictly protected.
MPAs represent an important component for protecting ecosystems and endangered species, as well as for fisheries management, to ease anthropogenic stresses and guarantee the sustainable use of marine resources (Lubchenco et al., 2003; Lowry et al., 2009; Halpern et al., 2010). MPAs can help to reduce biodiversity loss by fostering population persistence, recovery, and expansion, as well as conserving community composition and the biological processes that control those ecosystems (Almany et al., 2009; Gaines et al., 2010; Speed et al., 2018). MPAs contribute significantly to climate change adaptation by improving ecosystem resilience and maintaining ecosystem services (Micheli et al., 2012; Carr et al., 2017). However, When MPA classification cannot be extended to key sites or when it is not in the best interests of local governments or landowners, these places can nevertheless be designated as OECMs (Diniz et al., 2022). An OECM is a geographically defined area, but not a protected area, governed and managed for biodiversity protection and conservation, and associated ecosystem services, as well as, when appropriate, cultural, spiritual, socioeconomic, and other important values (IUCN-WCPA, 2019). Moreover, OECMs can include a variety of players and governance types (for example, indigenous peoples, local communities, business actors, and governments) (IUCN-WCPA, 2019). Participation of these stakeholders in area-based conservation efforts is a necessary step toward achieving favorable socioeconomic and conservation outcomes (Maxwell et al., 2020). The potential role of OECMs in constructing ecologically representative and well-connected networks of MPAs is gaining traction (Woodley et al., 2012; Spalding et al., 2013; Borrini-Feyerabend et al., 2014; Jonas et al., 2014; Dunn et al., 2016; Laffoley et al., 2017; Diz et al., 2018; Rees et al., 2018).
1.1 The role of ecological corridors in the environmental conservation
Among the most well-known, prioritized, and historically implemented types of connectivity, Ecological Corridors (ECs) represent clearly defined geographical spaces (biological or physical strips), regulated throughout time in order to preserve or restore effective ecological connectivity allowing movement of species and related ecological processes such as energy and gene fluxes and nutrient cycles (Good, 1998; Benson et al., 2007; Van der Windt and Swart, 2008; Palmeri et al., 2017; Hilty et al., 2020; Velázquez et al., 2022).
According to the EU Biodiversity Strategy for 2030, Member States are called to create ECs between protected sites (European Commission, 2021). In addition, IUCN Guidelines for Conserving Connectivity through Ecological Networks and Corridors is meant to guide global connectivity conservation efforts to design, govern, and manage for effective ecological connectivity (Hilty et al., 2020; Zhao et al., 2022). The EU Biodiversity Strategy asks for ECs to be included in the network of protected areas as a method of creating a cohesive transboundary network of protected areas (Hilty et al., 2020). Ecologically, ECs play an important role in the reconnecting fragmented ecosystems, regulating climate (Pataki et al., 2011; Gratani and Varone, 2013) and water (Cettner et al., 2014; Nickel et al., 2014), and providing food (Barthel and Isendahl, 2013), with the common aim of fostering, protecting and conserving biodiversity, (Hilty et al., 2006; Kattwinkel et al., 2011; Klaus, 2013; Garmendia et al., 2016; Fung et al., 2017). From a sociocultural standpoint, ECs connect valuable locations, offer working and leisure areas, and cultivate a sense of place associated with cultural heritage, in addition to being key components in the growth of tourism (McHarg, 1969; Beger et al., 2015; Beyer et al., 2018; Hilty et al., 2020).
In this context, remains still lacking the volume in MSP scientific research on ECs, and in particular, into the design of networks between MPAs and OECMs, supporting connectivity of marine systems (Balbar and Metaxas, 2019). MPAs and OECMs as elements for the conservation of the biodiversity may not be enough for addressing threats, such as, for instance, global climate change (Bates et al., 2019). For this reason, further environmental attributes, such us connectivity through ECs, might be considered to achieve biodiversity protection goals.
The aim of this paper is to review the scientific literature to investigate the current approaches and tools applied for investigating marine ECs between MPAs and OECMs, in an MSP perspective, and to identify gaps and opportunities to improve ecological connectivity between current areas to promote marine ecosystem conservation.
2 An overview of bibliometric research of previous literature
Literature search was conducted in Scopus3 and checked in Google Scholar4 by using the searching terms: “ecological connectivity”, OR “ecological corridor*”, OR “ecological network”, AND “marine protected area*, OR “Natura 2000”, OR “Other effective area-based conservation measures”, OR “OECM*”, AND “marine”, OR “ocean”, OR “coastal water*”, OR “offshore”, OR “Mediterranean Sea”. Only peer reviewed English language articles were included in the analysis. Abstracts were subsequently examined manually to select only papers that analyzed connectivity in terms of ECs at sea among MPAs and OECMs.
The bibliographic review was completed on April 2023. The search returned a total of 25 studies, excluding duplicates, made up of 24 research articles and 1 review (Table S1). Of these, the largest share (n = 17, 68% of the total) was concentrated in the latest five years (2018-2022), while the first article dates back to 2009. Most articles were produced in North America (USA: 6, Canada: 4; 40%), followed by European countries (9, 36%), and Asian countries (3, 12%), while remaining papers were developed in South America and Oceania (3, 12%).
To highlight the main keywords on which considered papers have been focused, a total of 294 author keywords from the 25 investigated papers were collected and analyzed for their frequency and then plotted in a keyword cloud (Figure 1). Furthermore, most relevant keywords, found at least in five studies, are summarized in Table 1. Keywords were selected with the aim of collecting information first on global scale and then specifically on a Mediterranean scale to identify the existence of studies on marine ECs at two different scales (global and local). Only few manuscripts, that have been highlighted in this review have dealt with the topic, easily providing overall information covering the global oceanic scale. Because scientific literature on marine ECs is actually scarce, the focus on the Mediterranean would provide new information in anticipation of future studies.
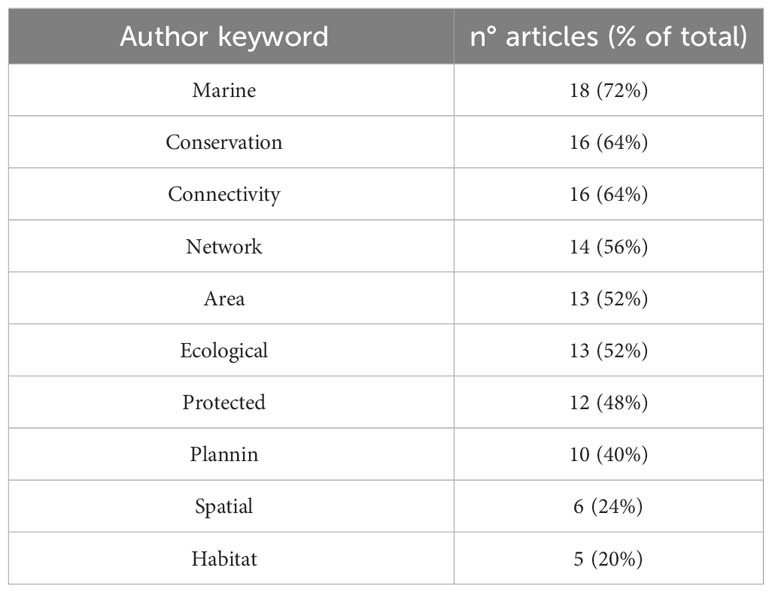
Table 1 Authors keywords, number of articles in which keywords are included, and proportion of these on the total of considered articles (%).
3 State of art of ECs connecting MPAs and OECMs: what do we know?
To maximize conservation effectiveness, the upcoming extension of MPAs needs the use of systematic conservation planning methodologies that account for connectivity (Balbar and Metaxas, 2019; Katsanevakis et al., 2020; Virtanen et al., 2020). Previous research has established advanced techniques for incorporating ecological connectivity into the selection of marine conservation priorities (Magris et al., 2016; Weeks, 2017; Daigle et al., 2020).
In contrast to terrestrial systems, where the underlying habitat structure associated with ECs is often rather static, in marine systems ECs are constantly changing due to water movements caused by hydrological and meteorological processes (Hastie et al., 2016).
Based on the information obtained from the systematic review, we report here on the state of art approaches and methodologies used to study ecological connectivity at sea. In particular, this section describes existing studies focused on the ecological connectivity in terms of larval dispersal, adults’ movement and migration, the importance of the tridimensional character of the marine environment, and common methodologies.
3.1 Larval dispersal
Research to investigate ecological connectivity in marine environments has focused mainly on models of larval dispersal by oceanic currents (Sanvicente-Añorve et al., 2014; Soria et al., 2014; Thomas Y. et al., 2014; Roberts et al., 2021), as a key driver of population connectivity (Treml et al., 2008; Andrello et al., 2015; Magris et al., 2016). Understanding dispersal trends between MPAs and OECMs is difficult since these phenomena are influenced by currents, season, time, and depth, all of which vary by area and species (Kinlan and Gaines, 2003; Basterretxea et al., 2012; Sayol et al., 2013). To deepen connectivity in seawaters by using larval dispersal, it is also important to use oceanographic data when addressing dispersion direction, since they constitute key variables for recruitment estimates (Schunter et al., 2011). Chemical tags (Hüssy et al., 2020), parentage analysis (Bode et al., 2019), or individual-based biophysical models are often used to evaluate larval, fragments or organisms’ dispersion distance in coastal marine ecosystems (Lett et al., 2020; Mari et al., 2020; Cecino and Treml, 2021) providing a spatial representativeness needed for conservation planning more easier than other techniques (Beger et al., 2022).
3.2 Adults’ movement and migration
In the past ten years, also adults’ movement has received a novel attention. It is well understood that knowledge on adult-mediated population connection is crucial for understanding the complicated dynamics of connectivity (Frisk et al., 2014; Pittman et al., 2014; Bryan-Brown et al., 2017; Holyoak et al., 2020; Keeley et al., 2021; Jetz et al., 2022). Connectivity analysis combines information about, for example, adult habitat preferences, resistance to mobility within and across habitat types, influence of oceanic currents, density dependency, and interactions between species where species-specific data are available (Rocha et al., 2002; Gillanders et al., 2003; Treml et al., 2008; Baggio et al., 2011; Caldwell and Gergel, 2013; Pittman et al., 2014; Magris et al., 2016; Allan et al., 2021). The most interesting species for assessing connectedness are those with modest adult mobility distances since the movement of widely dispersed species may limit the efficacy of conservation interventions (e.g.: MPAs) (Moffitt et al., 2009; Green et al., 2014; Friesen et al., 2021). Even if species with low dispersion distances might be confined, for example, within single MPAs (Kaplan et al., 2009; Carr et al., 2017; Friesen et al., 2021).
Furthermore, it was found that narrow coastal channels effectively function as ECs for mobile marine species migration, and earlier research suggests that marine predators may seek these habitats for feeding (e.g.: Tursiops truncatus and Phoca vitulina) (Brown and Mate, 1983; Thompson et al., 1991; Suryan and Harvey, 1998; Hastie et al., 2004; Wilson et al., 2007; Hastie et al., 2016; Krost et al., 2018). This because narrow coastal channels are characterized by hydrographic features (e.g.: current direction and velocity, tide) have been demonstrated to alter nutrient availability and movement, plankton retention, and fish aggregation, as well as potentially give greater feeding chances for predators (Benjamins et al., 2015).
Technological advancements in microelectronics for telemetry, spatial analytical approaches, and marine remote sensing favor the possibility of filling substantial information gaps in marine animal migrations (Pittman et al., 2014). In all cases, tagging highly mobile marine organisms with acoustic transmitters is the most successful and widely used approach for studying their movements in time and space (Pittman and McAlpine, 2003; Heupel et al., 2006).
In contrast, it is unclear how connectivity patterns, discovered using benthic habitat as a proxy, match with the effective population connectivity of a single species. (Friesen et al., 2019). By combining landscape characteristics with knowledge about a species’ capacity for dispersal (Calabrese and Fagan, 2004) or likelihood of dispersal between patches (Watson et al., 2010), this potential connectivity provides a more thorough understanding of species-specific connectivity patterns than a habitat proxy approach. However, connectivity is an important component in marine habitats, particularly for benthic species (Carr et al., 2003). Indeed, several physical drivers such as ocean currents are involved in connectivity (Brock et al., 2012). Surface currents can help to detect main connections and ECs which should be considered in MSP (Muñoz et al., 2015) and MPA network design (Schill et al., 2015).
3.3 Tridimensionality of marine environments
Another factor to consider is the tridimensional character of pelagic ecosystems, as well as the fact that most MSP techniques do not take this third vertical dimension into account (Muñoz et al., 2017). In fact, vertical connectivity can be attributed to physical (upwelling, downwelling, particle settling) or biological mechanisms (migration) (Robinson et al., 2010). For instance, the upwelling from the thermocline to the photic zone may promote the growth of phytoplankton, a component which is the basis of the pelagic food web (Sarhan et al., 2000; Granata et al., 2004). The deposition of particulate organic carbon connects surface primary production to benthic secondary production (Pfannekuche, 1993). Zooplankton diel vertical migration (DVM) contributes to the biological pump as a driver of carbon fluxes (Hernández-Leon et al., 2010; Ochoa et al., 2013; Ariza et al., 2015). Horizontal or vertical connectivity in marine ecosystems is essential for MSP as well as potential risk assessment of marine activities and discharges, however, there is currently a scarcity of knowledge on connection in these ecosystems (Sutton, 2013; Muñoz et al., 2015; Muñoz et al., 2017). Furthermore, in terms of connectivity direction, studies have primarily focused on horizontal or vertical one, but not both at the same time (Sutton, 2013; Schill et al., 2015; Muñoz et al., 2017). MSP and risk assessment necessitate the simultaneous consideration of all types of connectivity (physical, biological, horizontal, and vertical) (Muñoz et al., 2017).
3.4 ECs projection and mapping
There are, to the best of our knowledge, relatively few studies about the presence of EC in marine environments and among MPAs, while none including OECMs. Specifically, Ortiz-Lozano et al. (2013) studied EC across three coral reef systems in the southwest Gulf of Mexico. This study pinpointed that the found heterogeneity at a biogeographical and habitat level represents one of the main criteria to establish MPAs networks (Ortiz-Lozano et al., 2013). Pittman et al. (2014) provided direct evidence of ecological connectivity throughout an MPAs network in Caribbean reefs, considering movements of fish populations by using telemetric data. Subsequently, Friesen and coauthors (2019) incorporated connectivity within benthic habitats, as a proxy of adult movement due to the lack of information on population or individual mobility, into MPA planning in a case study conducted on Canada’s Pacific coast. In this paper, it was found a low interconnectedness among existing MPAs and the need to increase connectivity by prioritizing spaces that existing MPAs could use as steppingstones. In addition, Friesen et al. (2021) examined the ecological connectivity between MPAs considering two commercially important species in the Northern Shelf Bioregion in British Columbia (Canada). Lastly, even if not among MPAs, He et al. (2022) examined the importance of ECs to migratory species in the Yangtze estuary, the largest estuary in China, based on the variance in temporal and spatial density of three top fishery species to identify migratory ECs connecting optimum habitats that may be important in preserving population or community connectivity.
Common methodologies and tools for projecting and mapping ECs in terrestrial conservation planning have been established (Fenu and Pau, 2018; Liang et al., 2018; Bergès et al., 2020; Hilty et al., 2020). However, to our knowledge, in marine environments only two of these approaches were applied: least-cost theory and circuit theory (e.g.: McRae et al., 2008; McRae and Kavanagh, 2011; Pittman et al., 2014; Thomas C. J. et al., 2014; Friesen et al., 2021; Weeks, 2017). The two methods consider costs or resistance to migration based on species or habitat preferences (McRae et al., 2008; Correa Ayram et al., 2016). To run the lowest cost path, organisms need to be familiar with the landscape and its costs (Adriaensen et al., 2003; McClure et al., 2016). In this regard, least-cost path analysis calculates the single route between two regions with the lowest aggregate cost. On the other hand, circuit theory includes random walk theory similarly to random exploratory individual movements, assuming that organisms have not priori knowledge on the landscape (McRae et al., 2008; Dickson et al., 2019). Furthermore, the circuit theory technique examines the probability contributions of all feasible pathways in the landscape, allowing for the assessment of path redundancy and movement bottlenecks (Carroll et al., 2012; Dickson et al., 2019).
4 Discussion
4.1 Future perspectives and limitations of ECs connecting marine environments
MSP is one of the most significant processes for determining spatial priorities for the conservation through ecological connectivity (Beger et al., 2010). It is well understood that connectivity varies in space and time, making measurement and modeling difficult for conservation planning (Fahrig, 2003). Nevertheless, demographic growth and climate change present new problems and possibilities for incorporating connectivity into ecosystem-supportive planning (Simberloff, 1992; European Commission, 2015; Muñoz et al., 2017). Climate change is also expected to have a considerable influence on marine biological connectivity patterns (Harley et al., 2006; Andrello et al., 2015; Bruno et al., 2018; Friesen et al., 2021). Data on current and future ocean conditions, and the distribution of the species can be integrated to deepen how hotspots of connectivity as well as MPA networks interconnectedness may change over time (Heyman and Wright, 2011; Hooker et al., 2011; Piquer-Rodríguez et al., 2012; Friesen et al., 2019). Therefore, to integrate all available information on the ecological connectivity into MPAs planning is crucial (Carr et al., 2017). However, as stated earlier, direct integration of connectivity into MPA network planning is rare (Magris et al., 2014). To enhance connectivity between existing MPAs, planners should prioritize areas that can serve as steppingstones between existing ones. Connectivity regards the spatial structure of a network of MPAs and the potential capacity of organisms to move within each MPA of the network and in other suitable habitats outside to maintain itself. In some cases, to evaluate connectivity, only habitat inside MPAs have been regarded (Allison et al., 1998; Jonsson et al., 2020).
Connectivity can also depend on the movement between habitat patches within an MPA during various life stages (Berkström et al., 2022). Furthermore, connectivity within MPAs is important for species with reduced dispersal ranges and living in fragmented habitats, while connectivity between MPAs and the surrounding area is important for dispersal and genetic exchange between populations for larger areas (Andersson et al., 2008). Therefore, if any climate refugia exist, locating and protecting them can assist to preserve sensitive ecosystems (Brito-Morales et al., 2018). In this context, future studies should examine shifts in connectivity patterns in relation to climate change (Magris et al., 2014; Andrello et al., 2015). To better understand connectivity patterns of species regarding possible climate change implications, studies should evaluate vulnerability and movements throughout all life stages (Moffitt et al., 2009; Álvarez-Romero et al., 2018; Friesen et al., 2021). For instance, it is known that climate change is predicted to modify larval dispersion patterns, whether owing to decreasing planktonic larval duration or larval sensitivity to changing environmental features (O'Connor et al., 2007).
To our knowledge, there are very few MSP research that include horizontal, vertical, physical, and biological connectivity (Muñoz et al., 2017). This might be due to the existence of barriers across disciplines (oceanography, biology, and management) as well as the difficulty of developing a sample strategy that allows to study physical and biological connectivity simultaneously (Muñoz et al., 2017). Typically, benthic habitat data may be the only information available in MSP procedures where data are scarce (Brooks et al., 2004). Because of limitations of time and resources, conservation planners frequently use limited data to guide their decisions (Ban et al., 2009; Hansen et al., 2011). The biology of ecologically significant species (spawning and nursery grounds, reproductive periods, migration patterns) should be deepened to determine potential connectivity and offer a firm foundation for reserve siting, planning, and zoning (Fraschetti et al., 2018).
Faced with a future of increasing susceptibility to human activities (Halpern et al., 2019), successful marine biodiversity conservation necessitates a strategic planning approach to identifying places where numerous anthropogenic hazards coexist with ecological components (Halpern et al., 2008; Crain et al., 2009; Micheli et al., 2013).
Investigating connectivity in a managed area is crucial for population management as well as possible pollutant spread derived from human activities (Muñoz et al., 2015). In this context, currents have a role in connecting coastal managed areas environmentally, but that result administratively disconnected because differently managed by various local governments or nations (Muñoz et al., 2015). In case good connectivity is found between areas resulting under the jurisdiction of different administrations and countries, MSP requires administrative cooperation in that area as well as connectivity estimation and identification of ECs and the time necessary to cross them (Muñoz et al., 2015).
Lastly, encouraging is that policymakers and practitioners increasingly recognize the importance of ecological connectivity, even there are significant challenges for integrating connectivity into policies, planning, and conservation (Lausche, 2011; Keeley et al., 2019).
Data availability statement
The original contributions presented in the study are included in the article/Supplementary Material. Further inquiries can be directed to the corresponding author.
Author contributions
CP: conceptualization, data curation, validation, writing – original draft. EMDP: conceptualization, supervision, validation, Writing – review & editing.
Funding
The authors declare financial support was received for the research, authorship, and/or publication of this article. Project funded under the National Recovery and Resilience Plan (NRRP), Mission 4 Component 2 Investment 1.4 - Call for tender No. 3138 of 16 December 2021, rectified by Decree n.3175 of 18 December 2021 of Italian Ministry of University and Research funded by the European Union – NextGenerationEU. Project code CN_00000033, Concession Decree No. 1034 of 17 June 2022 adopted by the Italian Ministry of University and Research, CUP D33C22000960007, Project title “National Biodiversity Future Center - NBFC”.
Conflict of interest
The authors declare that the research was conducted in the absence of any commercial or financial relationships that could be construed as a potential conflict of interest.
Publisher’s note
All claims expressed in this article are solely those of the authors and do not necessarily represent those of their affiliated organizations, or those of the publisher, the editors and the reviewers. Any product that may be evaluated in this article, or claim that may be made by its manufacturer, is not guaranteed or endorsed by the publisher.
Supplementary material
The Supplementary Material for this article can be found online at: https://www.frontiersin.org/articles/10.3389/fmars.2023.1271397/full#supplementary-material
Footnotes
- ^ https://maritime-spatial-planning.ec.europa.eu/msp-eu/introduction-msp
- ^ https://ec.europa.eu/
- ^ https://www.scopus.com
- ^ https://scholar.google.com/
References
Adriaensen F., Chardon J. P., De Blust G., Swinnen E., Villalba S., Gulinck H., et al. (2003). The application of 'least-cost' modelling as a functional landscape model. Landsc. Urban Plan. 64, 233–247. doi: 10.1016/S0169-2046(02)00242-6
Allan J. C., Beazley K. F., Metaxas A. (2021). Ecological criteria for designing effective MPA networks for large migratory pelagics: Assessing the consistency between IUCN best practices and scholarly literature. Mar. Policy 127, 104219. doi: 10.1016/j.marpol.2020.104219
Allison G. W., Lubchenco J., Carr M. H. (1998). Marine reserves are necessary but not sufficient for marine conservation. Ecol. Appl. 8, S79–S92. doi: 10.2307/2641365
Almany G. R., Connolly S. R., Heath D. D., Hogan J. D., Jones G. P., McCook L. J., et al. (2009). Connectivity, biodiversity conservation and the design of marine reserve networks for coral reefs. Coral Reefs 28, 339–351. doi: 10.1007/s00338-009-0484-x
Álvarez-Romero J. G., Munguía-Vega A., Beger M., del Mar Mancha-Cisneros M., Suárez-Castillo A. N., Gurney G. G., et al. (2018). Designing connected marine reserves in the face of global warming. Glob. Change Biol. 24, e671–e691. doi: 10.1111/gcb.13989
Andersson Å., Korpinen S., Liman A. S., Nilsson P., Piekäinen H., Huggins A. (2008). “Ecological coherence and principles for MPA assessment, selection and design.” in BALANCE Technical Summary Report PART 3/4. (BALANCE, The Danish Forest and Nature Agency).
Andrello M., Mouillot D., Somot S., Thuiller W., Manel S. (2015). Additive effects of climate change on connectivity between marine protected areas and larval supply to fished areas. Divers. Distrib. 21, 139–150. doi: 10.1111/ddi.12250
Ariza A., Garijo J. C., Landeira J. M., Bordes F., Hernández-Leon S. (2015). Migrant biomass and respiratory carbon flux by zooplankton and micronekton in the subtropical northeast Atlantic Ocean (Canary Islands). Prog. Oceanogr. 134, 330–342. doi: 10.1016/j.pocean.2015.03.003
Baggio J. A., Salau K., Janssen M. A., Schoon M. L., Bodin O. (2011). Landscape connectivity and predator-prey population dynamics. Landsc. Ecol. 26, 33–45. doi: 10.1007/s10980-010-9493-y
Balbar A. C., Metaxas A. (2019). The current application of ecological connectivity in the design of marine protected areas. GECCO 17, e00569. doi: 10.1016/j.gecco.2019.e00569
Ban N. C., Gretchen J. A., Hansen M. J., Vincent A. C. J. (2009). Systematic marine conservation planning in data-poor regions: Socioeconomic data is essential. Mar. Policy 33 (5), 794–800. doi: 10.1016/j.marpol.2009.02.011
Ban N. C., Hussein M. A., Jeff A. A. (2010). Cumulative impact mapping: advances, relevance, and limitations to marine management and conservation, using Canada’s pacific waters as a case study. Mar. Policy 34, 876–886. doi: 10.1016/j.marpol.2010.01.010
Barthel S., Isendahl C. (2013). Urban gardens, agriculture, and water management: Sources of resilience for long-term food security in cities. Ecol. Econ. 86, 224–234. doi: 10.1016/j.ecolecon.2012.06.018
Basterretxea G., Jordi A., Catalán I. A., Sabatés A. (2012). Model-based assessment of local-scale fish larval connectivity in a network of marine protected areas. Fish. Oceanogr. 21, 291–306. doi: 10.1111/j.1365-2419.2012.00625.x
Bates A. E., Cooke R. S. C., Duncan M. I., Edgar G. J., Bruno J. F., Benedetti-Cecchi L., et al. (2019). Climate resilience in marine protected areas and the ‘Protection paradox’. Biol. Conserv. 236, 305–314. doi: 10.1016/j.biocon.2019.05.005
Beger M., Hedley S. G., Pressey R. L., Kerrie A. W., Peterson E. L., Dorfman D., et al. (2010). Conservation planning for connectivity across marine, freshwater, and terrestrial realms. Biol. Conserv. 143 (3), 565–575. doi: 10.1016/j.biocon.2009.11.006
Beger M., McGowan J., Treml E., Green A. L., White A. T., Wolff N. H., et al. (2015). Integrating regional conservation priorities for multiple objectives into national policy. Nat. Commun. 6, 8208. doi: 10.1038/ncomms9208
Beger M., Metaxas A., Balbar A. C., McGowan J. A., Daigle R., Kuempel C. D., et al. (2022). Demystifying ecological connectivity for actionable spatial conservation planning. Trends Ecol. Evol. 37, 1079–1091. doi: 10.1016/j.tree.2022.09.002
Benjamins S., Dale A. C., Hastie G. D., Waggitt J. J., Lea M. A., Scott B., et al. (2015). Confusions reigns? A review of marine megafauna interactions with tidal-stream environments. Oceanogr. Mar. Biol. 53, 1–54. doi: 10.1201/b18733-2
Benson R. L., Turo S., McCovey J. B. W. (2007). Migration and movement patterns of green sturgeon (Acipenser medirostris) in the Klamath and Trinity rivers, California, USA. Environ. Biol. Fishes 79, 269–279. doi: 10.1007/s10641-006-9023-6
Bergès L., Avon C., Bezombes L., Clauzel C., Duflot R., Foltête J.-C. (2020). Environmental mitigation hierarchy and biodiversity offsets revisited through habitat connectivity modelling. J. Environ. Manage. 256, 109950. doi: 10.1016/j.jenvman.2019.109950
Berkström C., Wennerström L., Bergström U. (2022). Ecological connectivity of the marine protected area network in the Baltic Sea, Kattegat and Skagerrak: Current knowledge and management needs. Ambio 51, 1485–1503. doi: 10.1007/s13280-021-01684-x
Beyer H. L., Kennedy E. V., Beger M., Chen C. A., Cinner J. E., Darling E. S., et al. (2018). Risk-sensitive planning for conserving coral reefs under rapid climate change. Conserv. Lett. 11, e12587. doi: 10.1111/conl.12587
Blowes S. A., Connolly S. R. (2012). Risk spreading, connectivity, and optimal reserve spacing. Ecol. Appl. 22, 311–321. doi: 10.2307/41416761
Bode M., Leis J. M., Mason L. B., Williamson D. H., Harrison H. B., Choukroun S., et al. (2019). Successful validation of a larval dispersal model using genetic parentage data. PloS Biol. 17, e3000380. doi: 10.1371/journal.pbio.3000380
Borrini-Feyerabend G. P., Bueno T., Hay-Edie B., Lang A., Rastogi A., Sandwith T. (2014). A primer on governance for protected and conserved areas, Stream on Enhancing Diversity and Quality of Governance (Gland, Switzerland: International Union for Conservation of Nature (IUCN).
Brito-Morales I., García Molinos J., Schoeman D. S., Burrows M. T., Poloczanska E. S., Brown C. J., et al. (2018). Climate velocity can inform conservation in a warming world. Trends Ecol. Evol. 33, 441–457. doi: 10.1016/j.tree.2018.03.009
Brock R. J., Kenchington E., Martínez-Arroyo A. (Eds.) (2012). Scientific Guidelines for Designing Resilient Marine Protected Area Networks in a Changing Climate (Montreal, Canada: Commission for Environmental Cooperation).
Brooks T. M., Da Fonseca G. A., Rodrigues A. S. (2004). Protected areas and species. Conserv. Biol. 18, 616–618. doi: 10.1111/j.1523-1739.2004.01836.x
Brown R. F., Mate B. R. (1983). Abundance movements and feeding habits of harbor seals (Phoca vitulina) at Netarts and Tillamook Bays, Oregon. Fish. Bull. 81, 291–301. doi: 10.2307/3536774
Bruno J. F., Bates A. E., Cacciapaglia C., Pike E. P., Amstrup S. C., van Hooidonk R., et al. (2018). Climate change threatens the world’s marine protected areas. Nat. Clim. Change 8, 499–503. doi: 10.1038/s41558-018-0149-2
Bryan-Brown D. N., Brown C. J., Hughes J. M., Connolly R. M. (2017). Patterns and trends in marine population connectivity research. Mar. Ecol. Prog. Ser. 585, 243–256. doi: 10.3354/meps12418
Calabrese J. M., Fagan W. F. (2004). A comparison-shopper’s guide to connectivity metrics. Front. Ecol. Environ. 2 (10), 529–536. doi: 10.1890/1540-9295(2004)002[0529:ACGTCM]2.0.CO;2
Caldwell I. R., Gergel S. E. (2013). Thresholds in seascape connectivity: influence of mobility, habitat distribution, and current strength on fish movement. Landsc. Ecol. 28, 1937–1948. doi: 10.1007/s10980-013-9930-9
Carr M. H., Neigel J. E., Estes J. A., Andelman S., Warner R. R., Largier J. L. (2003). Comparing marine and terrestrial ecosystems: Implications for the design of coastal marine reserves. Ecol. Appl. 13, 90–107. doi: 10.1890/1051-0761(2003)013[0090:CMATEI]2.0.CO;2
Carr M., Robinson S. P., Wahle C., Davis G., Kroll S., Murray S., et al. (2017). The central importance of ecological spatial connectivity to effective coastal marine protected areas and to meeting the challenges of climate change in the marine environment. Aquat. Conserv.: Mar. Freshw. Ecosyst. 21 (7), 6–29. doi: 10.1002/aqc.2800
Carroll C., McRae B. H., Brookes A. (2012). Use of linkage mapping and centrality analysis across habitat gradients to conserve connectivity of Gray Wolf populations in western North America. Conserv. Biol. 26, 78–87. doi: 10.1111/j.1523-1739.2011.01753.x
Cecino G., Treml E. A. (2021). Local connections and the larval competency strongly influence marine metapopulation persistence. Ecol. Appl. 31, e02302. doi: 10.1002/eap.2302
Cettner A., Ashley R., Hedstrom A., Viklander M. (2014). Sustainable development and urban stormwater practice. Urban Water J. 11, 185–197. doi: 10.1080/1573062X.2013.768683
Correa Ayram C. A., Mendoza M. E., Etter A., Salicrup D. R. P. (2016). Habitat connectivity in biodiversity conservation: a review of recent studies and applications. Prog. Phys. Geogr. 40, 7–37. doi: 10.1177/0309133315598713
Crain C. M., Halpern B. S., Beck M. W., Kappel C. V. (2009). Understanding and managing human threats to the coastal marine environment. Ann. N. Y. Acad. Sci. 1162, 39–62. doi: 10.1111/j.1749-6632.2009.04496.x
Daigle R., Metaxas A., Balbar A., Mcgowan J. A., Treml E. A., Kuempel C., et al. (2020). Operationalizing ecological connectivity in spatial conservation planning with Marxan Connect. Methods Ecol. Evol. 11, 570–579. doi: 10.1111/2041-210X.13349
Da Luz Fernandes M., Quintela A., Alves F. L. (2018). Identifying conservation priority areas to inform maritime spatial planning: a new approach. Sci. Total Environ. 639, 1088–1098. doi: 10.1016/j.scitotenv.2018.05.147
Dickson B. G., Albano C. M., Anantharaman R., Beier P., Fargione J., Graves T. A., et al. (2019). Circuit-theory applications to connectivity science and conservation. Conserv. Biol. 33, 239–249. doi: 10.1111/cobi.13230
Diniz M. F., Dallmeier F., Gregory T., Martinez V., Saldivar-Bellassai S., Benitez-Stanley M. A., et al. (2022). Balancing multi-species connectivity and socio-economic factors to connect protected areas in the Paraguayan Atlantic Forest. Landsc. Urban Plan. 222, 104400. doi: 10.1016/j.landurbplan.2022.104400
Diz D., Johnson D., Riddell M., Rees S., Battle J., Gjerde K., et al. (2018). Mainstreaming marine biodiversity into the SDGs: The role of other effective area-based conservation measures (SDG 14.5). Mar. Policy 93, 251–261. doi: 10.1016/j.marpol.2017.08.019
Dunn D. C., Maxwell S. M., Boustany A. M., Halpin P. N. (2016). Dynamic ocean management increases the efficiency and efficacy of fisheries management. PNAS 113, 668–673. doi: 10.1073/pnas.1513626113
Ersoy E., Jorgensen A., Warren P. H. (2018). Identifying multi-species connectivity corridors and the spatial pattern of the landscape. UFUG 40, 308–322. doi: 10.1016/j.ufug.2018.08.001
European Commission (2021). Directorate-General for Environment, EU biodiversity strategy for 2030 – Bringing nature back into our lives (Publications Office of the European Union), Belgium.
Fahrig L. (2003). Effects of habitat fragmentation on biodiversity. Annu. Rev. Ecol. Evol. Syst. 34 (1), 487–515. doi: 10.1146/annurev.ecolsys.34.011802.132419
Fang X., Hou X., Li X., Hou W., Nakaoka M., Yu X. (2018). Ecological connectivity between land and sea: a review. Ecol. Res. 33 (1), 51–61. doi: 10.1007/s11284-017-1549-x
Fenu G., Pau P. L. (2018). Connectivity analysis of ecological landscape networks by cut node ranking. Appl. Netw. Sci. 3, 22. doi: 10.1007/s41109-018-0085-0
Fraschetti S., Pipitone C., Mazaris A. D., Rilov G., Badalamenti F., Bevilacqua S., et al. (2018). Light and shade in marine conservation across european and contiguous seas. Front. Mar. Sci. 5. doi: 10.3389/fmars.2018.00420
Friesen S. K., Martone R., Rubidge E., Baggio J. A., Ban N. C. (2019). An approach to incorporating inferred connectivity of adult movement into marine protected area design with limited data. Ecol. Appl. 29 (4), e01890. doi: 10.1002/eap.1890
Friesen S. K., Rubidge E., Martone R., Hunter K. L., Peña M. A., Ban N. C. (2021). Effects of changing ocean temperatures on ecological connectivity among marine protected areas in northern British Columbia. Ocean Coast. Manage. 211, 105776. doi: 10.1016/j.ocecoaman.2021.105776
Frisk M. G., Jordaan A., Miller T. J. (2014). Moving beyond the current paradigm in marine population connectivity: Are adults the missing link? Fish Fish. 15, 242–254. doi: 10.1111/faf.12014
Fung E., Imbach P., Corrales L., Vilchez S., Zamora N., Argotty F., et al. (2017). Mapping conservation priori- ties and connectivity pathways under climate change for tropical ecosystems. Clim. Change 141 (1), 77–92. doi: 10.1007/s10584-016-1789-8
Gaines S. D., White C., Carr M. H., Palumbi S. R. (2010). Designing marine reserve networks for both conservation and fisheries management. PNAS 107, 18286–e18293. doi: 10.1073/pnas.0906473107
Garmendia E., Apostolopoulou E., Adams W. M., Bormpoudakis D. (2016). Biodiversity and green infrastructure in Europe: Boundary object or ecological trap? Land Use Policy 56, 315–319. doi: 10.1016/j.landusepol.2016.04.003
Gillanders B. M., Able K. W., Brown J. A., Eggleston D. B., Sheridan P. F. (2003). Evidence of connectivity between juvenile and adult habitats for mobile marine fauna: An important component of nurseries. Mar. Ecol. Prog. Ser. 247, 281–295. doi: 10.3354/meps247281
Gilliland P. M., Laffoley D. (2008). Key elements and steps in the process of developing ecosystem-based marine spatial planning. Mar. Policy 32 (5), 787–796. doi: 10.1016/j.marpol.2008.03.022
Good J. A. (1998). The potential role of ecological corridors for habitat conservation in Ireland: a review. Ir. Wildl. Man. 2, 72. pp.
Granata T. C., Estrada M., Zika U., Merry C. (2004). Evidence for enhanced primary production resulting from relative vorticity induced upwelling in the Catalan. Sci. Mar. 68 (S1), 113–119. doi: 10.3989/scimar.2004.68s1113
Gratani L., Varone L. (2013). Carbon sequestration and noise attenuation provided by hedges in Rome: The contribution of hedge traits in decreasing pollution levels. Atmos. pollut. Res. 4, 315–322. doi: 10.5094/APR.2013.035
Green A., Kertesz M., Peterson N., Retif S., Skewes T., Dunstan P., et al. (2014). “A regionalisation of Papua New Guinea’s marine environment,” in A technical report prepared for Papua New Guinea’s Department of Environment and Conservation with support from the Australian Government.
Guzmán-Colón D. K., Pidgeon A. M., Martinuzzi S., Radeloff V. C. (2020). Conservation planning for island nations: Using a network analysis model to find novel opportunities for landscape connectivity in Puerto Rico. GECCO 23, e01075. doi: 10.1016/j.gecco.2020.e01075
Halpern B. S., Frazier M., Afflerbach J., Lowndes J. S., Micheli F., O’Hara C., et al. (2019). Recent pace of change in human impact on the world’s ocean. Sci. Rep. 9 (1), 11609. doi: 10.1038/s41598-019-47201-9
Halpern B. S., Frazier M., Potapenko J., Casey K. S., Koenig K., Longo C., et al. (2015). Spatial and temporal changes in cumulative human impacts on the world’s ocean. Nat. Commun. 6, 7615. doi: 10.1038/ncomms8615
Halpern B. S., Lester S. E., McLeod K. L. (2010). Placing marine protected areas onto the ecosystem-based management seascape. PNAS 107 (43), 18312–18317. doi: 10.1073/pnas.090850310
Halpern B. S., Walbridge S., Selkoe K., Kappel C., Micheli F., Agrosa C., et al. (2008). A global map of human impact on Marine ecosystems. Science 319, 948–952. doi: 10.1126/science.1149345
Hansen G. J. A., Ban N. C., Jones M. L., Kaufman L., Hazel M. P., Yasué M., et al. (2011). Hindsight in marine protected area selection: A comparison of ecological representation arising from opportunistic and systematic approaches. Biol. Conserv. 144 (6), 1866–1875. doi: 10.1016/j.biocon.2011.04.002
Harley C. D. G., Randall H. A., Hultgren K. M., Miner B. G., Sorte C. J. B., Thornber C. S., et al. (2006). The impacts of climate change in coastal marine systems. Ecol. Lett. 9 (2), 228–241. doi: 10.1111/j.1461-0248.2005.00871.x
Hastie G. D., Russell D. J., Benjamins S., Moss S., Wilson B., Thompson D. (2016). Dynamic habitat corridors for marine predators; intensive use of a coastal channel by harbour seals is modulated by tidal currents. Behav. Ecol. Sociobiol. 70, 2161–2174. doi: 10.1007/s00265-016-2219-7
Hastie G. D., Wilson B., Wilson L. J., Parsons K. M., Thompson P. M. (2004). Functional mechanisms underlying cetacean distribution patterns: hotspots for bottlenose dolphins are linked to foraging. Mar. Biol. 144, 397–403. doi: 10.1007/s00227-003-1195-4
He Y., Zhao L., Liu S., Zhao X., Wang Y., Jiang X. (2022). Delineation of estuarine ecological corridors using the MaxEnt model to protect marine fishery biodiversity. Front. Mar. Sci. 9. doi: 10.3389/fmars.2022.966621
Hernández-Leon S., Franchy G., Moyano M., Menéndez I., Schmoker C., Putzeys S. (2010). Carbon sequestration and zooplankton lunar cycles: could we be missing a major component of the biological pump? Limnol. Oceanogr. 55 (6), 2503–2512. doi: 10.4319/lo.2010.55.6.2503
Heupel M., Semmens J., Hobday A. (2006). Automated acoustic tracking of aquatic animals: scales, design and development of listening station arrays. Mar. Freshw. Res. 57, 1. doi: 10.1071/MF05091
Heyman W. D., Wright D. J. (2011). Marine geomorphology in the design of marine reserve networks. Prof. Geogr. 63 (4), 429–442. doi: 10.1080/00330124.2011.585074
Hilty L. M., Arnfalk P., Erdmann L., Goodman J., Lehmann M., Wäger P. A. (2006). The relevance of information and communication technologies for environmental sustainability–a prospective simulation study. Environ. Model. Software 21 (11), 1618–1629. doi: 10.1016/j.envsoft.2006.05.007
Hilty J., Worboys G. L., Keeley A., Woodley S., Lausche B., Locke H., et al. (2020). “Guidelines for conserving connectivity through ecological networks and corridors,” in Best Practice Protected Area Guidelines Series No. 30. Gland, Switzerland (IUCN).
Holyoak M., Caspi T., Redosh L. W. (2020). Integrating disturbance, seasonality, multi-year temporal dynamics, and dormancy into the dynamics and conservation of metacommunities. Front. Ecol. Evol. 8. doi: 10.3389/fevo.2020.571130
Hooker S. K., Cañadas A., Hyrenbach K. D., Corriga C., Polovina J. J., Reeves R. R. (2011). Making protected area networks effective for marine top predators. Endanger. Species Res. 13, 203–218. doi: 10.3354/ESR00322
Hüssy K., Limburg K. E., de Pontual H., Thomas O. R. B., Cook P. K., Heimbrand Y., et al. (2020). Trace element patterns in otoliths: the role of biomineralization. Rev. Fish. Sci. 29, 445–477. doi: 10.1080/23308249.2020.1760204
IUCN-WCPA (2019). “Recognising and reporting other effective area-based conservation measures,” in IUCN, International Union for Conservation of Nature, Gland, Switzerland (IUCN). doi: 10.2305/IUCN.CH.2019.PATRS.3.en
Jetz W., McGowan J., Rinnan D. S., Possingham H. P., Visconti P., O’Donnell B., et al. (2022). Include biodiversity representation indicators in area-based conservation targets. Nat. Ecol. Evol. 6, 123–126. doi: 10.1038/s41559-021-01620-y
Jonas H. D., Barbuto V., Jonas H. C., Kothari A., Nelson F. (2014). New steps of change: looking beyond protected areas to consider other effective area-based conservation measures. PARKS 20, 111–128. doi: 10.2305/IUCN.CH.2014.PARKS-20-2.HDJ.en
Jonsson P. R., Moksnes P. O., Corell H., Bonsdorff E., Nilsson Jacobi M. (2020). Ecological coherence of Marine Protected Areas: New tools applied to the Baltic Sea network. Aquat. Conserv.: Mar. Freshw. 30, 743–760. doi: 10.1002/aqc.3286
Kaplan D. M., Botsford L. W., O’Farrell M. R., Gaines S. D. (2009). Model-based assessment of persistence in pro- posed marine protected area designs. Ecol. Appl. 19, 433–448. doi: 10.1890/07-1705.1
Katsanevakis S., Coll M., Fraschetti S., Giakoumi S., Goldsborough D., Mačíc V., et al. (2020). Twelve recommendations for advancing marine conservation in European and contiguous seas. Front. Mar. Sci. 7. doi: 10.3389/fmars.2020.565968
Kattwinkel M., Biedermann R., Kleyer M. (2011). Temporary conservation for urban biodiversity. Biol. Conserv. 144, 2335–2343. doi: 10.1016/j.biocon.2011.06.012
Keeley A. T. H., Beier P., Creech T., Jones K., Jongman R. H. G., Stonecipher G., et al. (2019). Thirty years of connectivity conservation planning: an assessment of factors influencing plan implementation. Environ. Res. Lett. 14, 103001. doi: 10.1088/1748-9326/ab3234
Keeley A. T. H., Beier P., Jenness J. S. (2021). Connectivity metrics for conservation planning and monitoring. Biol. Conserv. 255, 109008. doi: 10.1016/j.biocon.2021.109008
Kelly C., Gray L., Shucksmith R., Tweddle J. F. (2014). Review and evaluation of marine spatial planning in the Shetland Islands. Mar. Policy 46, 152–160. doi: 10.1016/j.marpol.2014.01.017
Kinlan B. P., Gaines S. D. (2003). Propagule dispersal in marine and terrestrial environments: a community perspective. Ecology 84, 2007–2020. doi: 10.1890/01-0622
Klaus V. H. (2013). Urban grassland restoration: A neglected opportunity for biodiversity conservation. Restor. Ecol. 21, 665–669. doi: 10.1111/rec.12051
Krost P., Goerres M., Sandow V. (2018). Wildlife corridors under water: an approach to preserve marine biodiversity in heavily modified water bodies. J. Coast. Conserv. 22, 87–104. doi: 10.1007/s11852-017-0554-0
Laffoley D., Dudley N., Jonas H., MacKinnon D., MacKinnon K., Hockings M., et al. (2017). An introduction to ‘other effective area-based conservation measures’ under Aichi Target 11 of the Con- vention on Biological Diversity: Origin, interpretation and emerging ocean issues. Aquat. Conserv.: Mar. Freshw. 27, 130–137. doi: 10.1002/aqc.2783
Leontiou S., Katsanevakis S., Vogiatzakis I. N. (2022). Accounting for functional connectivity in cross-realm conservation planning in a data poor context: The Cyprus case. J. Nat. Conserv. 70, 126304. doi: 10.1016/j.jnc.2022.126304
Lett C., Barrier N., Bahlali M. (2020). Converging approaches for modeling the dispersal of propagules in air and sea. Ecol. Modell. 415, 108858. doi: 10.1016/j.ecolmodel.2019.108858
Liang J., He X., Zeng G., Zhing M., Gao X., Xin L., et al. (2018). Integrating priority areas and ecological corridors into national network for conservation planning in China. Sci. Total Environ. 626, 22–29. doi: 10.1016/j.scitotenv.2018.01.086
Lowry G. K., White A. T., Christie P. (2009). Scaling up to networks of marine protected areas in the Philippines: biophysical, legal, institutional, and social considerations. Coast. Manage. 37 (3 – 4), 274–290. doi: 10.1080/08920750902851146
Lubchenco J., Palumbi S. R., Gaines S. D., Andelman S. (2003). Plugging a hole in the ocean: the emerging science of marine reserves. Ecol. Appl. 13 (1), S3–S7. doi: 10.1890/1051-0761(2003)013[0003:PAHITO]2.0.CO;2
Magris R. A., Pressey R. L., Weeks R., Ban N. C. (2014). Integrating connectivity and climate change into marine conservation planning. Biol. Conserv. 170, 207–221. doi: 10.1016/j.biocon.2013.12.032
Magris R. A., Treml E. A., Pressey R. L., Weeks R. (2016). Integrating multiple species connectivity and habitat quality into conservation planning for coral reefs. Ecography 39, 649–664. doi: 10.1111/ecog.01507
Margules C. R., Pressey R. L. (2000). Systematic conservation planning. Nature 405 (6783), 243–253. doi: 10.1038/35012251
Mari L., Melià P., Fraschetti S., Gatto M., Casagrandi R. (2020). Spatial patterns and temporal variability of seagrass connectivity in the Mediterranean Sea. Divers. Distrib. 26, 169–182. doi: 10.1111/ddi.12998
Maxwell S. L., Cazalis V., Dudley N., Hoffmann M., Rodrigues A. S. L., Stolton S., et al. (2020). Area-based conservation in the twenty-first century. Nature 586, 217–227. doi: 10.1038/s41586-020-2773-z
McClure M. L., Hansen A. J., Inman R. M. (2016). Connecting models to movements: testing connectivity model predictions against empirical migration and dispersal data. Landsc. Ecol. 31, 1419–1432. doi: 10.1007/s10980-016-0347-0
McRae B. H., Dickson B. G., Keitt T. H., Shah V. B. (2008). Using circuit theory to model connectivity in ecology, evolution, and conservation. Ecology 89, 2712–2724. doi: 10.1890/07-1861.1
McRae B. H., Kavanagh D. M. (2011). Linkage Mapper Connectivity Analysis Software (Seattle, USA: The Nature Conservancy).
Micheli F., Halpern B. S., Walbridge S., Ciriaco S., Ferretti F., Fraschetti S., et al. (2013). Cumulative human impacts on Mediterranean and black sea marine ecosystems: assessing current pressures and opportunities. PloS One 8, e79889. doi: 10.1371/journal.pone.0079889
Micheli F., Saenz-Arroyo A., Greenley A., Vazquez L., Espinoza Montes J. A., Rossetto M., et al. (2012). Evidence that marine reserves enhance resilience to climatic impacts. PloS One 7 (7), e40382. doi: 10.1371/journal.pone.0040832
Moffitt E. A., Botsford L. W., Kaplan D. M., O’Farrell M. R. (2009). Marine reserve networks for species that move within a home range. Ecol. Appl. 19, 1835–1847. doi: 10.1890/08-1101.1
Muñoz M., Reul A., Plaza F., Gómez-Moreno M. L., Vargas-Yañez M., Rodríguez V., et al. (2015). Implication of regionalization and connectivity analysis for marine spatial planning and coastal management in the Gulf of Cadiz and Alboran Sea. Ocean Coast. Manage. 118, 60–74. doi: 10.1016/j.ocecoaman.2015.04.011
Muñoz M., Reul A., Vargas-Yáñez M., Plaza F., Bautista B., García-Martínez M. C., et al. (2017). Fertilization and connectivity in the Garrucha Canyon (SE-Spain) implications for Marine Spatial Planning. Mar. Environ. Res. 126, 45–68. doi: 10.1016/j.marenvres.2017.02.007
Nickel D., Schoenfelder W., Medearis D., Dolowitz D. P., Keeley M., Shuster W. (2014). German experience in managing stormwater with green infrastructure. J. Environ. Plan. Manage. 57, 403–423. doi: 10.1080/09640568.2012.748652
O'Connor M. I., Bruno J. F., Gaines S. D., Halpern B. S., Lester S. E., Kinlan B. P., et al. (2007). Temperature control of larval dispersal and the implications for marine ecology, evolution, and conservation. PNAS 104, 1266–1271. doi: 10.1073/pnas.0603422104
Ochoa J., Maske H., Scheinbaum J., Candela J. (2013). Diel and lunar cycles of vertical migration extending to below 1000 m in the ocean and the vertical connectivity of depth-tiered populations. Limnol. Oceanogr. 58 (4), 1207–1214. doi: 10.4319/lo.2013.58.4.1207
Ortiz-Lozano L., Pérez-España H., Granados-Barba A., González-Gándara C., Gutiérrez-Velázquez A., Martos J. (2013). The Reef Corridor of the Southwest Gulf of Mexico: challenges for itsmanagement and conservation. Ocean Coast. Manage. 86, 22–32. doi: 10.1016/j.ocecoaman.2013.10.006
Palmeri L., Barausse A., Jorgensen S. E. (2017). Ecological processes handbook (Applied ecology and environmental management) (Florida: CRC Press), 341.
Pataki D. E., Carreiro M. M., Cherrier J., Grulke N. E., Jennings V., Pincetl S., et al. (2011). Coupling biogeochemical cycles in urban environments: Ecosystem services, green solutions, and misconceptions. Front. Ecol. Env. 9, 27–36. doi: 10.1890/090220
Pereira J. (2018). Multi-node protection of landscape connectivity: Habitat availability and topological reachability. Community Ecol. 19, 176–185. doi: 10.1556/168.2018.19.2.10
Pfannekuche O. (1993). Benthic response to the sedimentation of particulate organic matter at the BIOTRANS station, 47°N, 20°W. Deep Sea Res. Part II 40 (1–2), 135–149. doi: 10.1016/0967-0645(93)90010-K
Piquer-Rodríguez M., Kuemmerle T., Alcaraz-Segura D., Zurita-Milla R., Cabello J. (2012). Future land use effects on the connectivity of protected area networks in southeastern Spain. J. Nat. Conserv. 20 (6), 326–336. doi: 10.1016/j.jnc.2012.07.001
Pittman S. J., McAlpine C. A. (2003). Movements of marine fish and decapod crustaceans: process, theory and application. Adv. Mar. Biol. 44, 205–294. doi: 10.1016/s0065-2881(03)44004-2
Pittman S. J., Monaco M. E., Friedlander A. M., Legare B., Nemeth R. S., Kendall M. S., et al. (2014). Fish with chips: tracking reef fish movements to evaluate size and connectivity of Caribbean marine protected areas. PloS One 9, e96028. doi: 10.1371/journal.pone.0096028
Pressey R. L., Cabeza M., Watts M. E., Cowling R. M., Wilson K. A. (2007). Conservation planning in a changing world. Trends Ecol. Evol. 22, 583–892. doi: 10.1016/j.tree.2007.10.001
Rees S. E., Foster N. L., Langmead O., Pittman S., Johnson D. E. (2018). Defining the qualitative elements of Aichi Biodiversity Target 11 with regards to marine and coastal environment in order to strength global efforts for marine biodiversity conservation outlined in the United Nations Sustainable Development Goal 14. Mar. Policy 93, 241–250. doi: 10.1016/j.marpol.2017.05.016
Roberts K. E., Cook C. N., Beher J., Treml E. A. (2021). Assessing the current state of ecological connectivity in a large marine protected area system. Conserv. Biol. 35 (2), 699–710. doi: 10.1111/cobi.13580
Robinson C. L. K., Morrison J., Foreman M. G. G. (2005). Oceanographic connectivity among marine protected areas on the north coast of British Columbia, Canada. Can. J. Fish. Aquat. Sci. 62, 1350–1362. doi: 10.1139/f05-088
Robinson C., Steinberg D. K., Anderson T. R., Arístegui J., Carlson C. A., Frost J. R., et al. (2010). Mesopelagic zone ecology and biogeochemistry-a synthesis. Deep-Sea Res. II: Top. Stud. Oceanogr. 57 (16), 1504–1518. doi: 10.1016/j.dsr2.2010.02.018
Rocha L. A., Bass A. L., Robertson D. R., Bowen B. W. (2002). Adult habitat preferences, larval dispersal, and the comparative phylogeography of three Atlantic surgeonfishes (Teleostei: Acanthuridae). Mol. Ecol. 11, 243–252. doi: 10.1046/j.0962-1083.2001.01431.x
Sanvicente-Añorve L., Zavala-Hidalgo J., Allende-Arandía M. E., Hermoso-Salazar M. (2014). Connectivity patterns among coral reef systems in the southern Gulf of Mexico. Mar. Ecol. Prog. Ser. 498, 27–41. doi: 10.3354/meps10631
Sarhan T., García-Lafuente J., Vargas M., Vargas J. M., Plaza F. (2000). Upwelling mechanisms in the Northwestern Alboran sea. J. Mar. Syst. 23, 317–331. doi: 10.1016/S0924-7963(99)00068-8
Saura S., Bertzky B., Bastin L., Battistella L., Mandrici A., Dubois G. (2018). Protected area connectivity: Shortfalls in global targets and country-level priorities. Biol. Conserv. 219, 53–67. doi: 10.1016/j.biocon.2017.12.020
Sayol J. M., Orfila A., Simarro G., López C., Renault L., Galán A., et al. (2013). Sea surface transport in the Western Mediterranean Sea: a Lagrangian perspective. J. Geophys. Res. Oceans 118, 6371–6384. doi: 10.1002/2013JC009243
Schill S. R., Raber G. T., Roberts J. J., Treml E. A., Brenner J., Halpin P. N. (2015). No reef is an island: integrating coral reef connectivity data into the design of regional-scale marine protected area networks. PloS One 10 (12), e0144199. doi: 10.1371/journal.pone.0144199
Schunter C., Carreras-Carbonell J., Macpherson E., Tintoré J., Vidal-Vijande E., Pascual A., et al. (2011). Matching genetics with oceanography: directional gene flow in a Mediterranean fish species. Mol. Ecol. 20 (24), 5167–5181. doi: 10.1111/j.1365-294X.2011.05355.x
Simberloff D. (1992). “Conservation of pristine habitats and unintended effects of biological control,” in Selection Criteria and Ecological Consequences of Importing Natural Enemies; Conference Paper; Entomological Society of America, Annapolis, MD, USA. 103–117.
Soria G., Torre-Cosio J., Munguia-Vega A., Marinone S. G., Lavín M. F., Cinti A., et al. (2014). Dynamic connectivity patterns from an insular marine protected area in the Gulf of California. J. Mar. Syst. 129, 248–258. doi: 10.1016/j.jmarsys.2013.06.012
Spalding M., Meliane I., Milam A., Fitzgerald C., Hale L. (2013). Protecting marine spaces: global targets and changing approaches. Ocean Yearb. 27, 213–248. doi: 10.1163/22116001-90000160
Speed C. W., Cappo M., Meekan M. G. (2018). Evidence for rapid recovery of shark populations within a coral reef marine protected area. Biol. Conserv. 220, 308–319. doi: 10.1016/j.biocon.2018.01.010
Stelzenmüller V., Lee J., South A., Rogers S. I. (2010). Quantifying cumulative impacts of human pressures on the marine environment: a geospatial modelling framework. Mar. Ecol. Prog. Ser. 398, 19–32. doi: 10.3354/meps08345
Suryan R. M., Harvey J. T. (1998). Tracking harbor seals (Phoca vitulina richardsi) to determine dive behavior, foraging activity, and haul–out site use. Mar. Mammal Sci. 14, 361–372. doi: 10.1111/j.1748-7692.1998.tb00728.x
Sutton T. Y. (2013). Vertical ecology of the pelagic ocean: classical patterns and new perspectives. Rev. paper. J. Fish Biol. 83, 1508–1527. doi: 10.1111/jfb.12263
Thomas Y., Dumas F., Andréfouët S. (2014). Larval dispersal modeling of pearl oyster Pinctada margaritifera following realistic environmental and biological forcing in Ahe atoll lagoon. PloS One 9 (4), e95050. doi: 10.1371/journal.pone.0095050
Thomas C. J., Lambrechts J., Wolanski E., Traag V. A., Blondel V. D., Deleersnijder E., et al. (2014). Numerical modelling and graph theory tools to study ecological connectivity in the Great Barrier Reef. Ecol. Modell. 272, 160–174. doi: 10.1016/j.ecolmodel.2013.10.002
Thompson P. M., Pierce G. J., Hislop J. R. G., Miller D., Diack J. S. W. (1991). Winter foraging by common seals (Phoca vitulina) in relation to food availability in the inner Moray Firth, N.E. Scotland. J. Anim. Ecol. 60, 283–294. doi: 10.2307/5460
Treml E. A., Halpin P. N., Urban D. L., Pratson L. F. (2008). Modelling population connectivity by ocean currents, a graph-theoretic approach for marine conservation. Landsc. Ecol. 23 (1), 19–36. doi: 10.1007/s10980-007-9138-y
Tulloch V. J. D., Atkinson S., Possingham H. P., Peterson N., Linke S., Allan J. R. (2021). Minimizing cross-realm threats from land-use change: A national-scale conservation framework connecting land, freshwater and marine systems. Biol. Conserv. 254, 108954. doi: 10.1016/j.biocon.2021.108954
UNEP-WCMC (2023). Protected Planet: The World Database on other effective area-based conservation measures (WD-OECM) (Cambridge, UK: UNEP-WCMC and IUCN).
Van der Windt H. J., Swart J. A. A. (2008). Ecological corridors, connecting science and politics: the case of the Green River in the Netherlands. J. Appl. Ecol. 45, 124–132. doi: 10.1111/j.1365-2664.2007.01404.x
Velázquez J., Gülçin D., Vogt P., Rincón V., Hernando A., Gutiérrez J., et al. (2022). Planning restoration of connectivity and design of corridors for biodiversity conservation. Forests 13, 2132. doi: 10.3390/f13122132
Virtanen E. A., Moilanen A., Viitasalo M. (2020). Marine connectivity in spatial conservation planning: analogues from the terrestrial realm. Landsc. Ecol. 35, 1021–1034. doi: 10.1007/s10980-020-00997-8
Watson J. R., Mitarai S., Siegel D. A., Caselle J. E., Dong C., McWilliams J. C. (2010). Realized and potential larval connectivity in the Southern California Bight. Mar. Ecol. Prog. Ser. 401, 31–48. doi: 10.3354/meps08376
Weeks R. (2017). Incorporating seascape connectivity in conservation prioritisation. PloS One 12, e0182396. doi: 10.1371/journal.pone.0182396
Wilson M. F. J., O’Connell B., Brown C., Guinan J. C., Grehan A. J. (2007). Multiscale terrain analysis of multibeam bathymetry data for habitat mapping on the continental slope. Mar. Geod. 30, 3–35. doi: 10.1080/01490410701295962
Woodley S., Bertzky B., Crawhall N., Dudley N., Londoño J. M., MacKinnon K., et al. (2012). Meeting aichi target 11: What does success look like for protected area systems? PARKS 18, 23–34. doi: 10.2305/IUCN.CH.2012.PARKS-18-1.SW.en
Keywords: ecological corridors, connectivity, maritime spatial planning, marine protected areas, other effective area-based conservation measures, conservation
Citation: Podda C and Porporato EMD (2023) Marine spatial planning for connectivity and conservation through ecological corridors between marine protected areas and other effective area-based conservation measures. Front. Mar. Sci. 10:1271397. doi: 10.3389/fmars.2023.1271397
Received: 02 August 2023; Accepted: 20 September 2023;
Published: 05 October 2023.
Edited by:
Miriam Von Thenen, Leibniz Institute for Baltic Sea Research (LG), GermanyReviewed by:
Chiara Paoli, University of Genoa, ItalyMarcus Geoffrey Haward, University of Tasmania, Australia
Copyright © 2023 Podda and Porporato. This is an open-access article distributed under the terms of the Creative Commons Attribution License (CC BY). The use, distribution or reproduction in other forums is permitted, provided the original author(s) and the copyright owner(s) are credited and that the original publication in this journal is cited, in accordance with accepted academic practice. No use, distribution or reproduction is permitted which does not comply with these terms.
*Correspondence: Cinzia Podda, c.podda@fondazioneimc.it