- Division of Natural Sciences, Pepperdine University, Malibu, CA, United States
Marine protected areas (MPAs) aim to protect habitats and ecosystems to promote the diversity and health of marine populations. To evaluate the health of fish populations within and outside of MPAs in Southern California, we used flight initiation distance (FID). FID is the distance at which an individual will flee from a perceived predator and is a direct measure of boldness. Lower FIDs are indicative of bolder populations. Lower FID values indicate that fish will have the opportunity to dedicate less energy to fleeing from predators which could then be used for increased foraging. Data was collected from eight locations along the Southern California coast, four in protected areas and four in non-protected areas. FIDs were measured with a sonar-based range finder. All fish species that recreational fishermen could catch were targeted, with a focus on six core species spearfishermen catch commonly. Results showed that all fish sampled in MPAs have significantly lower FIDs than those in non-protected areas. An ANOVA showed no significant difference between different locations of the same protected status. The six core species had lower average FIDs in protected areas, three species had significant differences between protected and non-protected populations, and there was no significant difference in FIDs between species. These results show that Southern California MPAs are promoting healthier fish communities with respect to boldness behavior. This data and methods can be implemented in future projects to expand the range of metrics measured to inform management tools for California’s MPAs and MPAs at large.
1 Introduction
Long-term overfishing has led to the collapse of fisheries and the degradation of marine ecosystems around the world (Jackson et al., 2001). To protect ocean habitats and the communities they support, many countries have established marine protected areas (MPAs). MPAs limit or prevent take of marine life within their boundaries in order to allow populations to recover to healthy levels. Fish populations in California have followed the same global trends, as catch normalized by fishing pressure peaked before World War II and has declined since then (Free et al., 2022). Healthy fish populations are important for robust coastal ecosystems and the California economy. In California alone commercial and recreational fishing generated over 24 billion dollars and employed over 140,000 people in 2016 (Fisheries Economics of the United States, 2016 | NOAA Fisheries, 2021). Fish populations decline led to California’s passage of the Marine Life Protection Act (MLPA) in 1999 which sought to create a network of MPAs to increase coherence and effectiveness in protecting the state’s marine ecosystems, and marine natural heritage, as well as to improve recreational, educational and study opportunities provided by marine ecosystems (Marine Life Protection Act, 1999; Saarman and Carr, 2013; California Department of Fish and Wildlife, 2023). Over the next 13 years, MPAs were created and implemented along the California coast by different agencies and with varying restrictions. In 2012 the California MPA Network (124 MPAs) was completed.
With 2022 marking the tenth year of the full implementation of the California MPA Network, there has been a decadal management review evaluating the effectiveness of the network in meeting the MLPA goals (Wertz et al., 2022; The First 10 Years: Measuring the Success of California’s Underwater Parks, 2023). Fish within the boundaries of MPAs are larger and more abundant than those outside of protected areas (Hamilton et al., 2021). Most protected areas also had greater biomass of target species than reference sites outside of MPAs (Carr et al., 2021). MPAs in Southern California have had the largest positive impact on populations within their boundaries, with greater biomass increases seen in these MPAs than in ones farther north (Sullivan-Stack et al., 2022). Reasons for this are that the Southern California MPAs were established earlier, meaning there has been a longer period to see change (Sullivan-Stack et al., 2022), and that more temperate ecosystems are more responsive to change than cooler areas (Tanaka et al., 2021). There has been a lot of work done looking at the abundance and size of marine life in protected areas, but the effects of protection status on behavior have been limited to one study focused only on barred sand bass (Paralabrax nebulifer) around Catalina Island (Mason and Lowe, 2010).
In order to evaluate the effectiveness of MPAs along the Southern California coast in promoting healthier communities of fish targeted by fishermen, we used flight initiation distance (FID). Flight initiation distance is a commonly used metric in behavioral ecology that measures the balance of current and future fitness of potential prey in response to predation events (Ydenberg and Dill, 1986). FID represents the optimization of current and future fitness; it is a metric of how an animal can best survive in the present and be able to produce and care for offspring in the future (Dowling and Bonier, 2018). It has been extensively used to evaluate the effects of various anthropogenic impacts on species from reptiles to mammals (Blumstein et al., 2003; Legagneux and Ducatez, 2013; McGowan et al., 2014; Breck et al., 2019). These studies have shown that increased urbanization leads to acclimatization by birds, reptiles, and mammals, showing shorter FIDs in locations where there is more human presence and that these changes can vary by species, not just phyla (Blumstein et al., 2003; McGowan et al., 2014; Breck et al., 2019). It has also been shown that birds will modify their behavior and have longer FIDs in response to consistent threats in an area (Legagneux and Ducatez, 2013).
An animal’s response to threats affects many different levels of animal behavior, from social structures to foraging habits (McArthur et al., 2014; Heathcote et al., 2017). These fear responses vary with the landscape, varying levels of predation and the availability of hiding places change behavioral responses, leading to the concept of a landscape of fear (Laundre et al., 2010), where prey’s perception of predation risk is different in different areas. These fear responses come largely from the threat of predation, so predator presence is especially important in constructing the landscape of fear. Importantly, even non-consumptive interactions between predator and prey can drastically alter prey behavior as prey will exhibit strong flight responses and dedicate less energy to foraging even with just a threat of predation (Matassa and Trussell, 2011). Even without actual predation, the effect of potential predator interactions can affect community composition across trophic levels (Matassa and Trussell, 2011). Not only is decreasing population sizes a problematic result of increased hunting, but changing the behavior of prey by manipulating this landscape of fear can have similarly negative effects. It has also been found that in some cases human interactions can elicit greater behavioral responses than the presence and absence of natural predators. Ciuti et al. (2012) found that the effect size of human disturbance was greater than changes from habitat type or natural predators for elk in the Rocky Mountains. This means that the effects of human predation could impact species’ behavior and by extension community interactions and composition much more than would occur from predation only by natural predators. Protected areas in both terrestrial and aquatic environments aim to limit the effects of human predation to reduce the significant changes that can result and to allow for healthy communities across trophic levels to thrive (Geldmann et al., 2013).
Fishing, especially spearfishing, has been shown to increase fear responses of fish (Lima and Dill, 1990; Handegard et al., 2003). However, many different factors can influence fish flight responses. One of the ways that fish try to combat threats of predation is through schooling (Larsson, 2012), but studies around the effect of schooling report mixed results, with some showing that schooling fish allow a closer approach (Stankowich and Blumstein, 2005), while others say there is no effect (Huijbers et al., 2011; Samia et al., 2019). Fish FID responses have also varied with the presence of hunting gear such as a speargun (Januchowski-Hartley et al., 2012; Tran et al., 2016). The diver’s starting distance from the fish (i.e., distance between the diver and the fish when the fish was first spotted), (Stamoulis et al., 2019), habitat complexity (Nunes et al., 2015), and fish body length (Gotanda et al., 2009) have also been shown to affect how different species respond to potential human predation. FID has been used to measure the effects of fishing pressures on fish behavior, especially comparing protected and non-protected areas (Benevides et al., 2018). In studies conducted along the coasts of Barbados, Brazil, and Rapa Nui fish in protected areas had lower FIDs than those in areas where fishing was allowed (Gotanda et al., 2009; Benevides et al., 2018; Petit et al., 2022). FIDs have begun to be used as a metric of the effectiveness of MPAs as they show behavioral characteristics associated with higher fitness. They can be beneficial in several different ways. First, they can be used as a metric of the effectiveness of protection. Part of the purpose of protection of fishing areas can be to change fish behavior to allow fish to be easier to catch in non-protected areas (Cinner et al., 2006). FID can be used to see if fish are becoming less wary, and as a result, easier to catch in fished areas around MPAs.
Additionally, changes in behavior can indicate the success of protection in promoting healthier communities of fished species. An increase in predator presence can prompt an increase in prey fish wariness (Milinski, 1986; Kindinger and Albins, 2017). As prey wariness increases, these fish dedicate less energy to foraging (Kindinger and Albins, 2017), which can reduce growth rates (Lima and Dill, 1990). As one of the metrics of the success of an MPA is the increase of fish size in its bounds (Turnbull et al., 2021), MPAs with less wary fish due to decreased fishing pressure should end up having larger fish as they can dedicate more energy to foraging. It has been shown that fish with traits correlated to increased foraging behavior, such as greater boldness and activity rates, are more likely to be caught by fishermen (Biro and Post, 2008). It has also been observed that populations outside of protected areas begin to evolutionarily self-select traits that lead to slower growth rates, while those inside MPAs exhibit behaviors leading to faster growth (Sørdalen et al., 2022). Larger fish, in turn, lead to more and greater viability of offspring (Qvarnström and Price, 2001; Birkeland and Dayton, 2005; Pew Charitable Trusts, 2016), allowing populations to recover to pre-fishing levels. When bolder individuals are allowed to grow without the threat of human predation, they increase the health of the population through greater size and reproduction rates.
Another way that FID has been used is to measure the efficacy of MPAs in preventing marine take (Benevides et al., 2018; Nunes et al., 2018). Once a baseline FID is established for the species in an area, FID, along with other quantitative data, can be used over time to evaluate whether the restrictions are being followed. If in some protected areas FIDs are more consistent with fish in non-protected areas, that can indicate that the regulations in place are not being followed. As FIDs can be influenced by a host of factors, from habitat complexity (Nunes et al., 2015) to fish size (Gotanda et al., 2009) it is not enough to show the effectiveness of protection on its own. However, when used with other metrics of population health, such as biomass and biodiversity, it can inform decisions on the management and enforcement of regulations in protected areas.
Most studies using fish FID were conducted around the Pacific Islands, with some along the eastern coast of North and South America (Nunes et al., 2018). No studies have used FID of fish along the west coast of North or South America (Nunes et al., 2018). In California, unreliable visibility and surf conditions are likely reasons for this. Higher concentrations of nutrients in the water can lead to increased algae presence (Anderson et al., 2002; Smith, 2003; Anderson et al., 2008), which contributes to lower visibility in the water. To our knowledge, this study is the first to use FID as a metric to evaluate the effectiveness of MPAs in fostering healthy fish populations in such a setting. FID has been used to evaluate the effectiveness of MPAs in Brazil and other countries (Gotanda et al., 2009; Benevides et al., 2018; Petit et al., 2022). It represents a tool to increase the scope of management strategies for protected areas in California. If well implemented, FID can be paired with more commonly collected data, such as fish biomass, size, counts, or landings to evaluate the effectiveness of MPAs. Using FID will allow managers to evaluate not only the physical changes in abundance and sizes but also the changes in fish behavior.
This study aims to evaluate the effectiveness of MPAs in Southern California in promoting healthy fish communities and to test the efficacy of FID as a metric of fish community health in Southern California waters. We hypothesized that fish populations would be healthier within the boundaries of MPAs, resulting in smaller FIDs for fish inside protected areas. To test this hypothesis FID was measured for fish species that are usually caught by spearfishermen within and outside of MPAs in Southern California.
2 Methods
2.1 Locations
The data was collected at eight different locations, four protected areas and four non-protected areas (Figure 1). The protected sites are all in State Marine Reserves (SMRs) or State Marine Conservation Areas (SMCAs). SMRs prevent the take of any living, geological, or cultural marine resource within their boundaries, while SMCAs may allow restricted take within their boundaries. The two SMCAs we sampled are Point Dume SMCA and Laguna Beach SMCA, which allow for the take of pelagic fish and incidental take of organisms during dredging, respectively. Big Dume Beach and El Matador Beach are at opposite ends of a continuous stretch of protected coast spanning two MPAs. The Point Dume SMR protects 19.50 km2 of coastal water around Point Dume. Point Dume SMCA extends over six kilometers west from the western boundary of the SMR and encloses 41.23 km2. The Point Dume SMCA allows the take of only pelagic fish, none of which were sampled in our study. Big Dume Beach is in Point Dume SMR and El Matador beach is in Point Dume SMCA. Woods Cove and Tablerock Beach are in a section of Orange County Coast protected by four separate protected areas. Woods Cove is in the Laguna Beach SMR, which covers 7.09 kilometers of coast and encloses 17.40 km2 of ocean. As an SMR, there is no take allowed within the boundaries. Tablerock is in the Laguna Beach SMCA and covers a stretch of ocean on the southern side of the SMR. It encloses 8.00 km2, and only incidental catch related to coastal management is allowed. All non-protected sites are along the Malibu coastline. Latigo is the farthest west at Latigo Point. Puerco is 4.5 km east of Latigo alongshore and off of Malibu Bluffs Park along Malibu Road. Surfrider is 2 km east of Puerco near the Malibu Lagoon, and Big Rock is 7 km east of Surfrider at the end of Big Rock Road. None of these areas have any protected status and spearfishing is common at all of the non-protected sites.
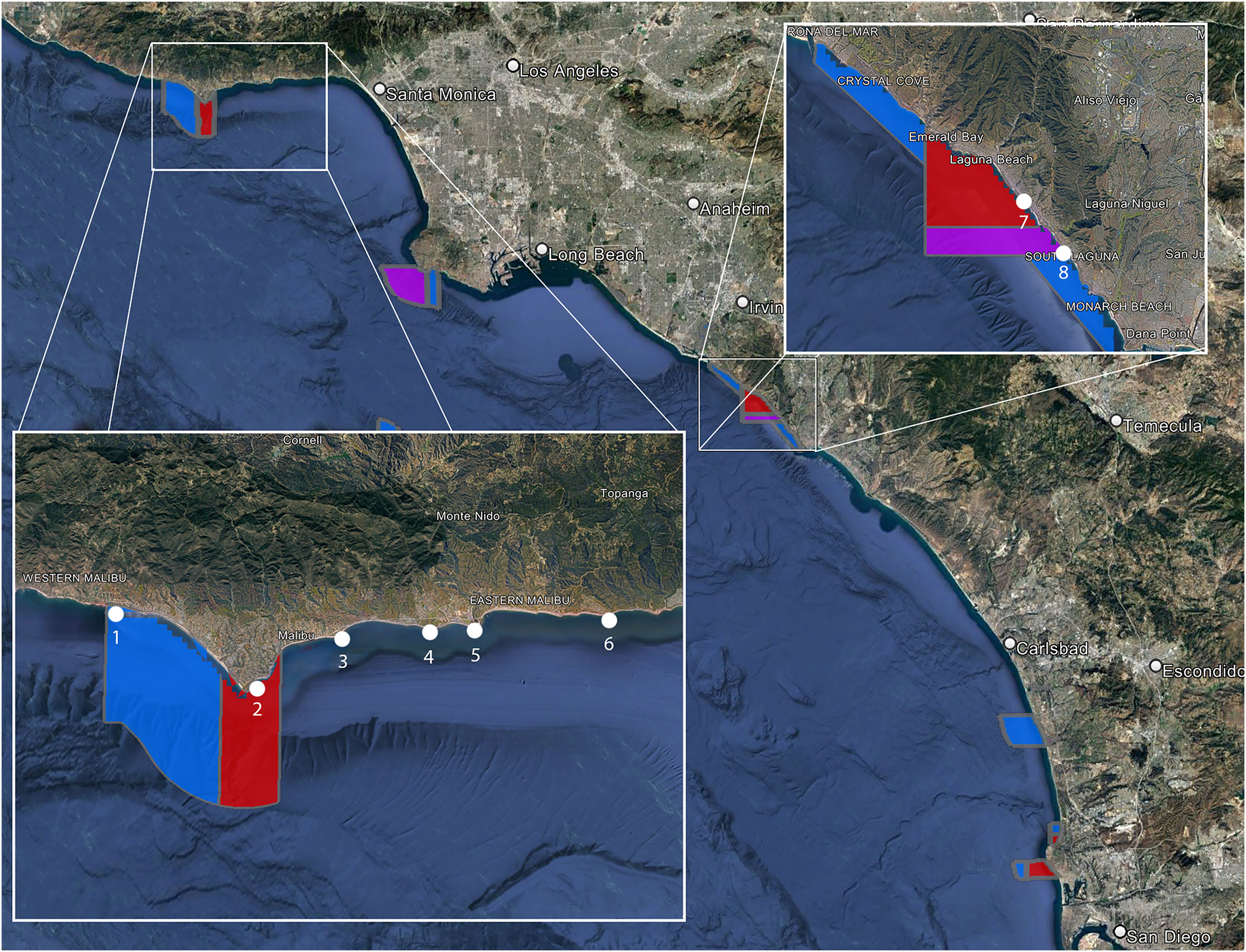
Figure 1 Red sites are Marine Reserves that prevent any take within their boundaries. Blue sites are conservation areas, which allow some take (varies from site to site). Purple areas are State Marine Conservation Areas that don’t allow any take within their boundaries. El Matador (1), Big Dume Beach (2), Woods Cove (7), and Tablerock Beach (8) are all in MPAs. Latigo (3), Puerco (4), Surfrider (5), and Big Rock (6) are all non-protected areas.
The reef fish we targeted have high site fidelity. These fish only leave the natural reefs they are found on originally to populate artificial reefs introduced in the area (Matthews, 1985). Since all of our sites were natural sites, we do not expect the movement of these fish between sites. In addition to this, many of our core species exhibit high site fidelity, and any movement that is seen between sites occurs at much shorter distances (i.e., 1 km) than those between any of our sites (Hixon, 1981; Valle, 1989; Lowe et al., 2003) (shortest proximity between our sites is 2 km). All sampling was conducted in October and November of 2021 and between May and July of 2022 (Table 1).
2.2 Fish species
Any fish encountered larger than 10 cm, indicating adult specimens, were targeted for FID measurements. The dataset includes 23 fish species (Supplemental Table 1). The only species not targeted was Hypsypops rubicundus, commonly known as the Garibaldi, because it is protected across the state and unlike the other fish observed is highly territorial. All of the species were included for all statistical tests expect the tests looking at the individual six core species (section 2.4). The following six core species were tested individually for differences between protected and non-protected populations (section 2.4): black perch (Embiotoca jacksoni), kelp bass (Paralabrax clathratus), opaleye (Girella nigricans), sargo (Anisotremus davidsonii), silver surfperch (Hyperprosopon ellipticum), and zebraperch (Kyphosus azureus). These core species were subsetted to have at least 10 total approaches with a minimum of three approaches in both protected and non-protected areas. Topsmelt (Atherinops affinis) also meets these requirements, but spearfishermen do not commonly catch it, so it was not included in our core species. The six core species are all taken by spear and line fishermen (Porzio, 2014; Stenstrom, 2018); therefore, they are good indicators of the effectiveness of fish population protection in MPAs. All of the core species are common along Southern California shores (Miller and Lea, 1972).
2.3 Sampling procedures
The data is a compilation of individual fish approaches (n=185) collected by snorkeling and freediving. Only one FID data point was collected per dive. Dive depths varied between two and eight meters. The diver swam along the bottom until they saw a target fish. The diver would swim straight at the fish at a constant pace of about one m/sec. FID was taken when the fish began to swim away from the diver faster than it was being approached, increasing the distance between it and the diver. FID measures the closest distance that the diver was able to get to the fish. Distance between the diver and the fish was taken with a RICANK Depth Finder, which is usually used to measure the distance to an object (i.e., seafloor or a fish) when ice fishing. The depth finder reports distances from 0.8 to 90 meters down to the tenth of a meter accuracy. In some cases, the distance directly to the fish could not be obtained, so the distance to the nearest rocks behind the fish was measured instead. Sometimes, the fish was too close to the diver for the range finder to get a reading, so a visual estimate was taken. This only occurred on some distances under 0.8 meters. All sampling sites were rocky bottom reefs with kelp beds; hence the distance to the nearest hard substrate behind the fish was comparable to the distance to the fish itself. In order to prevent sampling of the same fish multiple times, divers would swim unidirectionally east or west along the shore at least 10 meters away from the previous sampling location before sampling another fish of the same species. FID, species name or description, and location were recorded by the diver for all approaches. For 99 of the 185 total data points (i.e., 54%), schooling behavior was also recorded and defined as four or more fish of the same species swimming as a group and recorded by the diver. The FID of schooling fish was determined for the closest fish in the school that the diver was able to approach. Species were identified on the dive by the diver. Fish that could not be identified in the field were identified using A Field Guide to Coastal Fishes From Alaska to California (Kells et al., 2016) right after the dive based on the fish’s morphological characteristics. Fish that could not be identified in the field or with the field guide afterward were not included. There were only three individuals that could not be identified out of 188 total approaches (i.e., 1.5%), leaving the final data set at 185 individuals.
2.4 Statistical analysis
Our first two-way ANOVA was conducted with all FID data from all approaches (n=185) in order to see how a community responds to protected status (Table 2; Figure 2A). We conducted a two-way ANOVA using species and protected status as factors in order to account for differences in community composition in protected and non-protected areas.
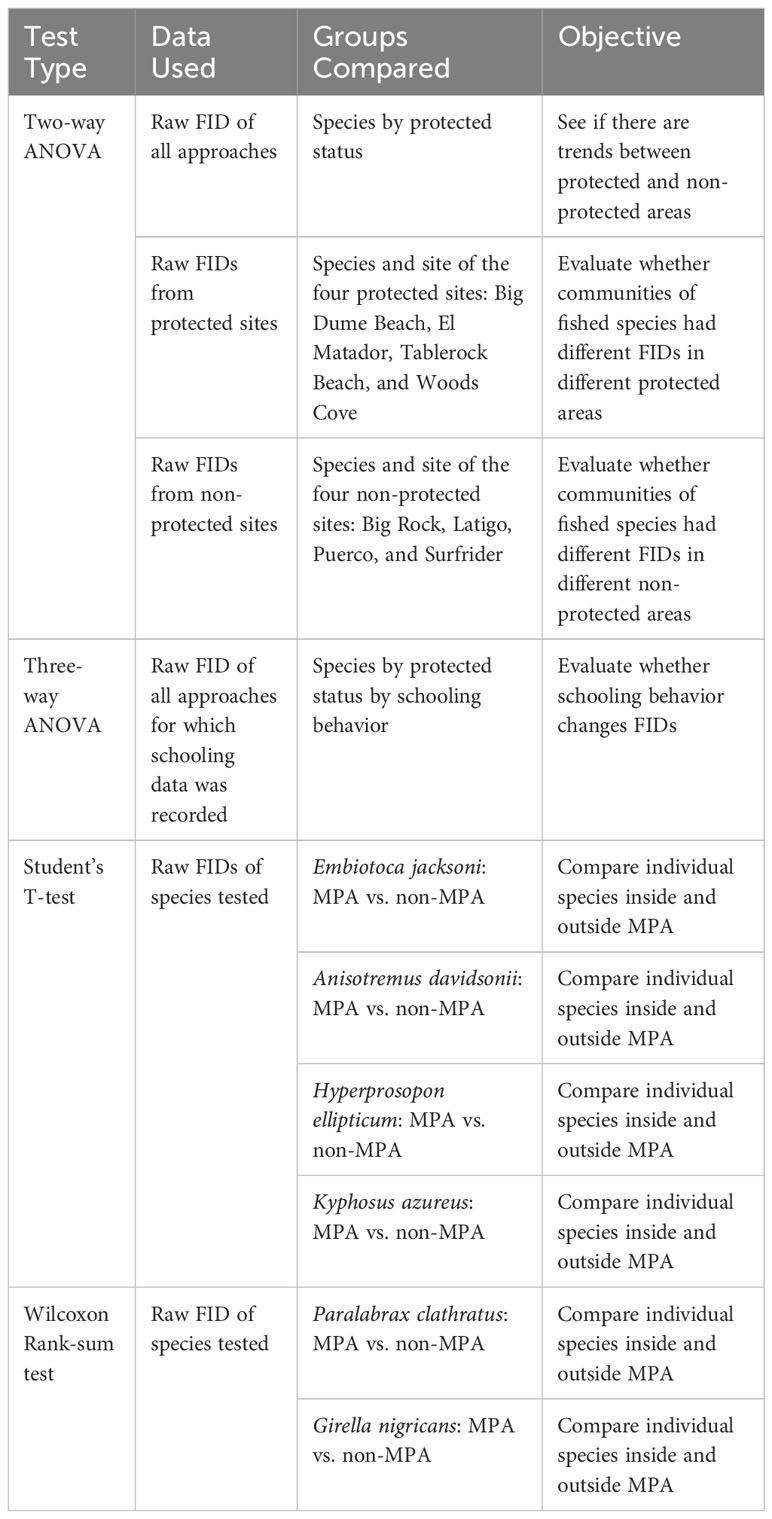
Table 2 All of the statistical tests conducted with the data used per analysis, the groups compared in each analysis, and the objective of the statistical test.
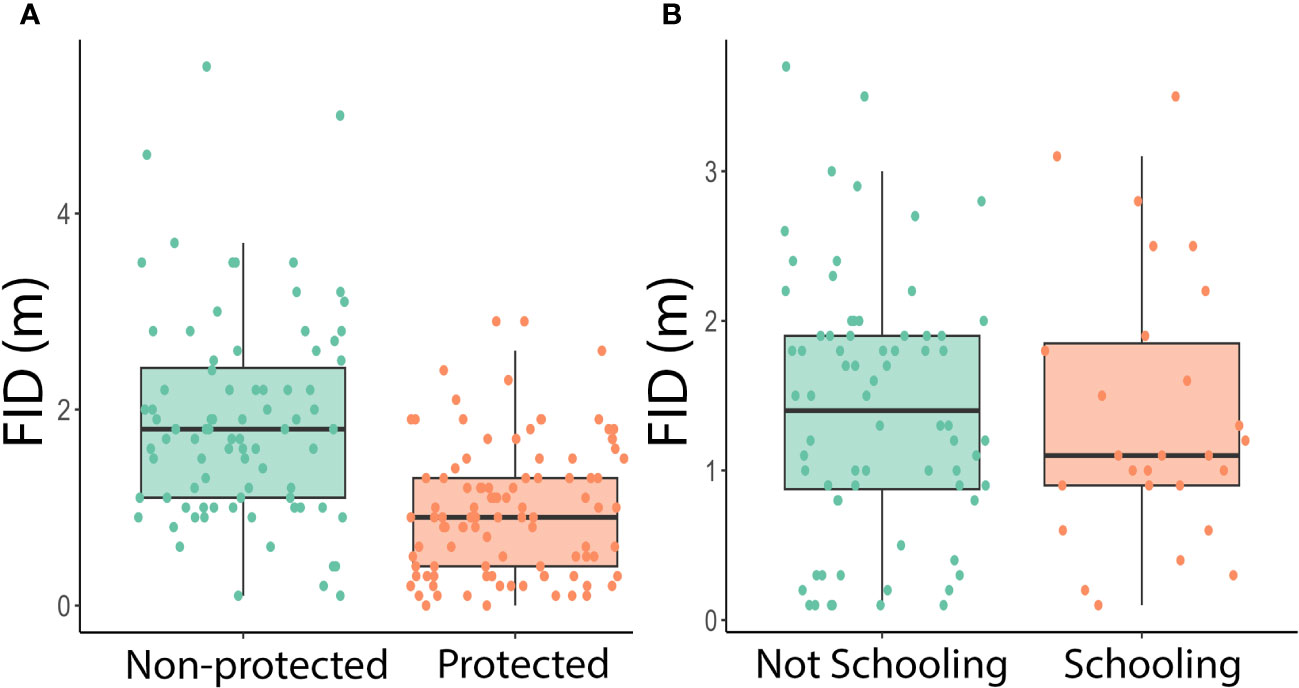
Figure 2 (A) Boxplot of protected status against FID in meters. Each data point represents one approach of an individual fish in a protected or non-protected area. A two-way ANOVA showed a significant difference between fish in protected and non-protected areas (Two-way ANOVA: species: df = 23, F = 1.88, p = 0.0141 protected: df = 1, F = 47.5, p = 1.39x10-10). (B) Boxplot of schooling behavior against FID in meters. Each data point represents a fish approached in the field for which we collected schooling data. A three-way ANOVA found there was no significant difference between schooling and non-schooling fish (Three-way ANOVA: species: df = 12, F = 1.81, p = 0.0642 schooling: df = 1, F = 0.399, p =0.530 protected: df = 1, F = 29.1, p = 9.03 x 10-7).
Next, we looked at the subset of the data (n=99) which includes schooling data to test whether differences in FID could be associated with schooling behavior. We used a three-way ANOVA with species, protected status, and schooling behavior as factors in order to determine whether coastal fish in Southern California vary in FID with different schooling behaviors (Table 2; Figure 2B).
We then used two two-way ANOVAs to evaluate whether fish at different sites with the same protected status (e.g, Big Dume Beach and El Matador - both protected areas) had different FIDs. We conducted two two-way ANOVAs on populations of fish within and outside of protected areas, respectively. The first two-way ANOVA used the variables of species and site on a subset of the overall data which only included approaches in MPAs (n=101, Table 2; Figure 3). The second two-way ANOVA used the same variables on a subset of the overall dataset which only included approaches in non-protected areas (n=84, Table 2; Figure 3). These tests evaluated whether populations in different sites of the same protected status had different behaviors.
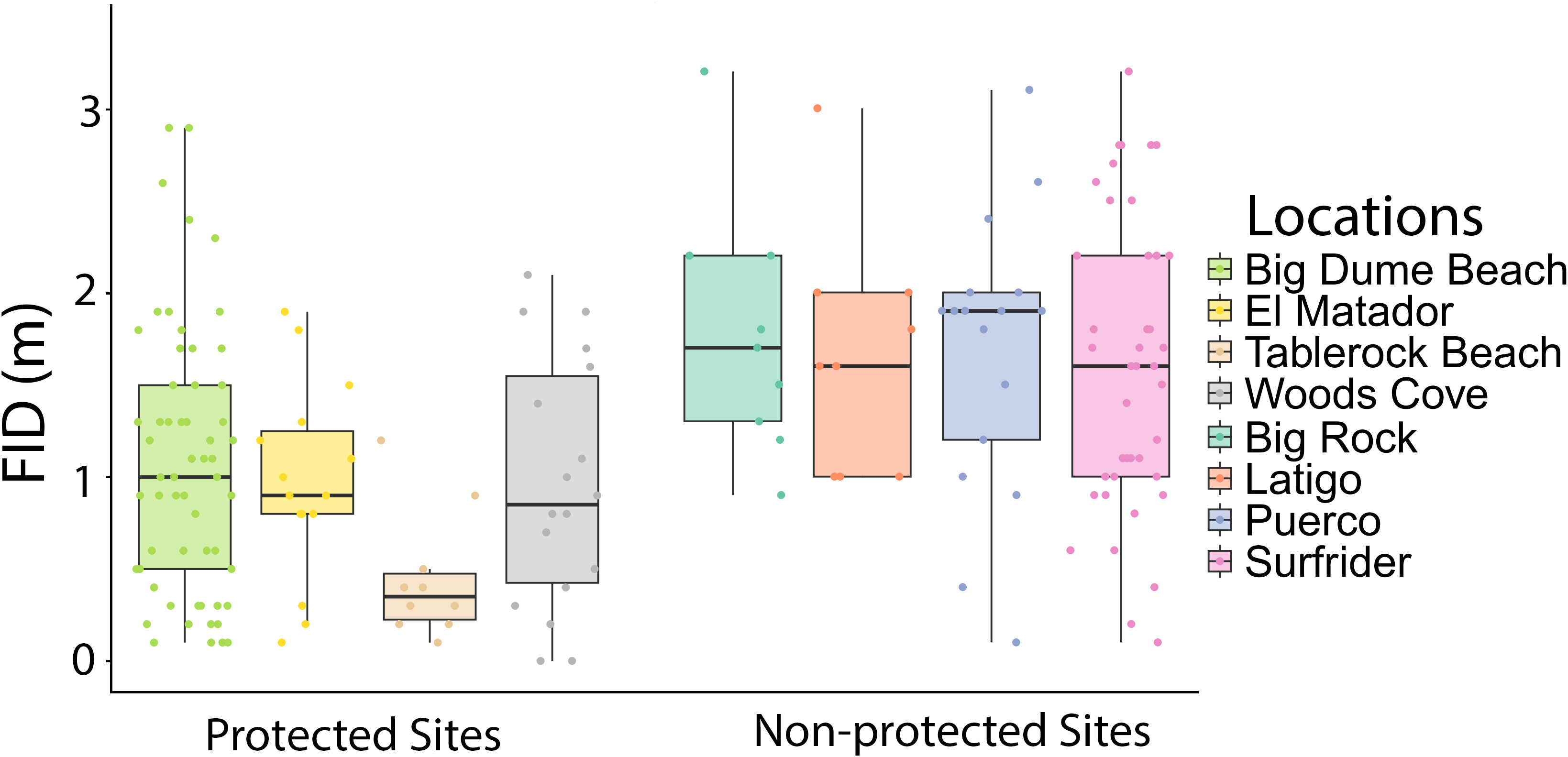
Figure 3 Boxplot of FID in meters by site, with protected and non-protected sites grouped together. There was no significant difference between sites of the same protected status according to two two-way ANOVA tests, one for protected areas and one for non-protected areas (Two-way ANOVAs in: MPA sites: species: df=20, F=1.50, p=0.0821 site: df=3, F=0.571, p=0.636; Two-way ANOVA in non-MPA sites: species: df=12, F=1.55, p=0.133 site: df=3, F=0.742, p=0.531).
We performed two Wilcoxon rank-sum tests and four Student’s T-tests on a subset of the data set that only included six of the species sampled. We chose these six species based on three criteria: (1) these six species had at least three approaches in each protected status, (2) these species had at least ten approaches total, and (3) all six species are commonly taken by spearfishermen in Southern California. The six species included in this test were black perch (Embiotoca jacksoni), kelp bass (Paralabrax clathratus), opaleye (Girella nigricans), sargo (Anisotremus davidsonii), silver surfperch (Hyperprosopon ellipticum), and zebra perch (Kyphosus azureus). With this group, we performed individual Student’s T-tests or Wilcoxon rank-sum tests with each species, based on whether the data passed Normality assumptions. These tests compared the FIDs of individuals approached inside MPAs to those approached outside of MPAs. For Embiotoca jacksoni, Anisotremus davidsonii, Hyperprosopon ellipticum, and Kyphosus azureus we performed Student’s T-tests because their populations both inside and outside MPAs were Normally distributed. We performed Wilcoxon rank-sum tests for Paralabrax clathratus and Girella nigricans because the data for these species was not Normally distributed. For all analyses, p-values less than 0.05 were considered statistically significant. All statistical tests described in this section are detailed in Table 2. All data analysis was done in Rstudio (Version 2023.06.2 561).
3 Results
There were 185 total approaches and FIDs recorded, 101 in protected areas and 84 in non-protected areas. There were at least nine approaches for each site, with Big Dume having the most approaches of a protected site (n=60) and Surfrider having the most data of a non-protected area (n=45) (Table 3). The two-way ANOVA conducted by species and protected status found that there was a significant difference with regards to species and protected status (Two-way ANOVA: species: df=23, F=1.88, p=0.0141 protected: df=1, F=47.5, p=1.39x10-10, Figure 2A). The three-way ANOVA looking at the effect of schooling found no relationship between schooling behavior and FID, with or without the effect of protected status considered (Three-way ANOVA: species: df=12, F=1.81, p=0.0642 schooling: df=1, F=0.399, p=0.530 protected: df=1, F=29.1, p=9.03x10-7, Figure 2B). The two two-way ANOVAs evaluating differences in FID between sites of the same protected status found that there were no differences within the four protected sites or the four non-protected sites (Two-way ANOVA in MPA sites: species: df=20, F=1.50, p=0.0821 site: df=3, F=0.571, p=0.636; Two-way ANOVA in non-MPA sites: species: df=12, F=1.55, p=0.133 site: df=3, F=0.742, p=0.531, Figure 3).
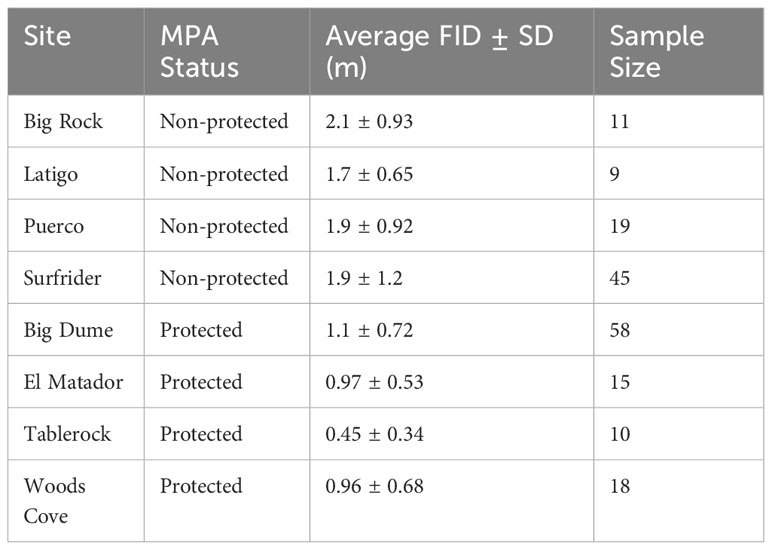
Table 3 Average flight initiation distance (FID) ± standard deviation (SD) in meters (m) for the eight sampling sites.
For the six core species, Embiotoca jacksoni, Anisotremus davidsonii, and Hyperprosopon ellipticum had under ten approaches in protected areas, with seven, seven, and eight approaches, respectively (Table 4). Only Embiotoca jacksoni and Anisotremus davidsonii had under ten approaches outside of MPAs, with six and three respectively, while Hyperprosopon ellipticum had ten approaches in non-protected areas (Table 4). Paralabrax clathratus had the most approaches, with 21 in MPAs and 19 in non-protected areas (Table 4). Girella nigricans had 12 in protected areas and 21 outside of protected areas, while Kyphosus azureus had 13 in MPAs and ten in non-protected areas (Table 4). All species had lower average FIDs in protected areas. When tests were performed between individuals in protected and non-protected areas for each species, there were only significant differences between Paralabrax clathratus (Pc), Girella nigricans (Gn), and Kyphosus azureus (Ka) (Students T-test: Ka t=3.35, df=21, p=0.0031; Wilcoxon Rank-Sum Test: Gn W=204, p=0.0035 Pc W=357, p=2.04x10-5, Figure 4) (Table 5). There were no significant differences between populations in protected and non-protected areas for Embiotoca jacksoni (Ej), Anisotremus davidsonii (Ad), and Hyperprosopon ellipticum (He) (Student’s T-test: Ej t=0.941, df=11, p=0.367; Ad t=0.724, df=8, p=0.490; He t=1.04, df=17, p=0.313, Figure 4) (Table 5).
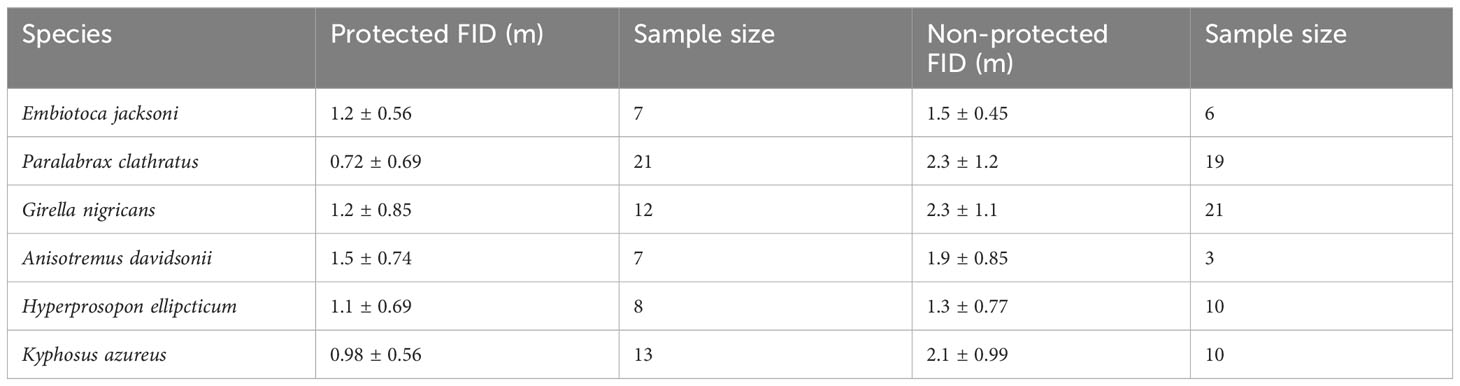
Table 4 Average fid (m) with standard deviations for the six core species in both protected and non-protected areas.
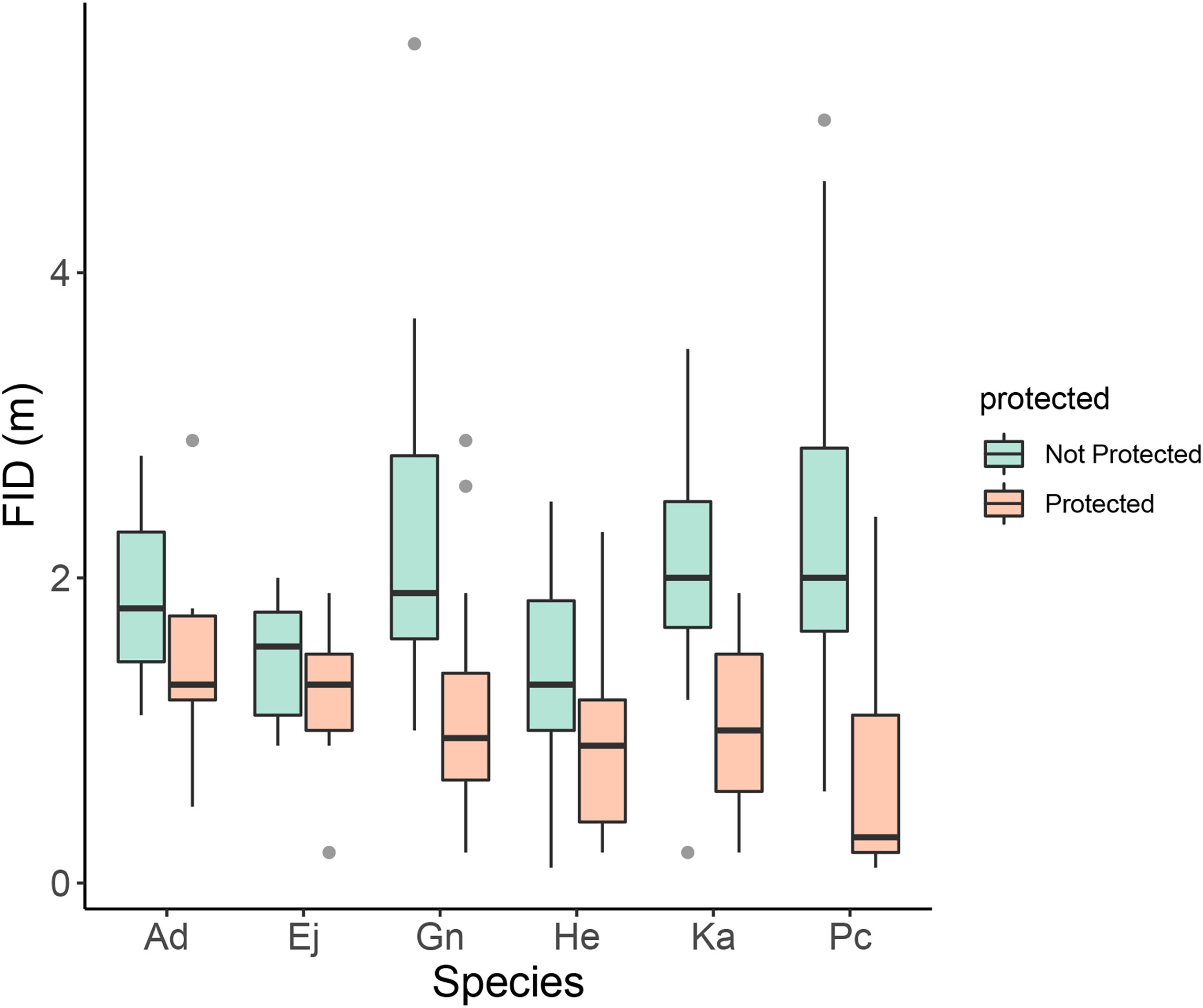
Figure 4 Boxplot of FID in meters by species and protected status. Ad, Anisotremus davidsonii; Ej, Embiotoca jacksoni; Gn, Girella nigricans; He, Hyperprosopon ellipticum; Ka, Kyphosus azureus; and Pc, Paralabrax clathratus. There were significant differences between individuals inside and outside of MPAs for three species: Paralabrax clathratus (Pc), Kyphosus azureus (Ka), and Girella nigricans (Gn) (Student’s T-test: Ka t=3.35, df=21, p=0.0031; Wilcoxon Signed Rank-Sum Test: Gn W=204, p=0.0035 Pc W=357, p=2.04x10-5). There were no significant differences for Embiotoca jacksoni (Ej), Anisotremus davidsonii (Ad), and Hyperprosopon ellipticum (He) (Student’s T-test: Ej t=0.941, df=11, p=0.367; Ad t=0.724, df=8, p=0.490; He t=1.04, df=17, p=0.313).
4 Discussion
The purpose of this project was to evaluate the effectiveness of Southern California MPAs in promoting healthier fish communities. Using FID as a metric for health, we hypothesized that fish in MPAs would have lower FIDs than those in non-protected areas, indicating higher levels of fish boldness. Across the four protected areas studied, fish had lower FIDs than fish in the four non-protected sites to which they were compared.
There was no significant difference in FID between schooling fish (n=27) and solitary fish (n=72). These results go against a study that found that schooling decreased FIDs in reef fish (Stankowich and Blumstein, 2005), but are consistent with results found by other studies which showed schooling did not affect the FIDs of fish (Huijbers et al., 2011; Samia et al., 2019). Adult fish are less likely to school in the first place, as schooling can lead to more competition for resources, which larger fish tend to avoid (Hoare et al., 2000). It could be that individuals engaged in what we defined as schooling behavior (i.e., four or more individuals in a group), were not functioning as a group but just happened to be found in the same place.
Fish in protected areas had shorter FIDs on average than those in non-protected areas (Figure 2A). This is consistent with our hypothesis that fishing pressures in non-protected areas would lead to increased wariness in fish populations and increases in fish FIDs outside of MPAs. The increase in human-based predator presence can lead to increased responsiveness from potential prey (Milinski, 1986). This increased vigilance of fish found outside MPAs can lead prey to change their foraging behavior to dedicate less energy to foraging and more to finding safety (Lima and Dill, 1990). Fish in protected areas are not forced to deal with extra predatory pressures from humans, suggesting they can dedicate more energy to foraging and will not be forced to flee from potential predators at such a large distance. This means that fish in protected areas may have increased fitness. Decreased foraging behavior can lead to slower growth rates of individuals and populations in the long term (Lima and Dill, 1990), which can be correlated to lower community health (DeAngelis et al., 1990). These results are consistent with other metrics of fish community health inside of MPAs, such as increases in abundance and biomass (Carr et al., 2021; Hamilton et al., 2021). And the increases in bold behavior of fish inside of MPAs should lead to increases in the other metrics of fish community health (Biro and Post, 2008; Sørdalen et al., 2022). As such, FID represents a new and useful tool for evaluating the effectiveness of the MPA network in Southern California. The evaluation of fish behavior can add another dimension to monitoring methods used on the California MPA network, which historically have used physical metrics to assess effectiveness. This work is significant since behavioral studies have been constrained to one species in one location (Mason and Lowe, 2010).
When comparing FIDs of the six core species in protected versus non-protected areas, only Paralabrax Clathratus, Kyphosus azureus, and Girella nigricans had significant differences in FID between populations (Table 5). All three species are considered important game fish and are targeted by both line and spear fishermen along the Southern California coast (Sommer et al., 1995; Stenstrom, 2018; Fishbase - Girella nigricans, 2023; Fishbase - Kyphosus azureus, 2023; Fishbase - Paralabrax clathratus, 2023). All three of the other species are taken by fishermen, but Hyperprosopon ellipticum and Embiotica jacksoni tend to be smaller than the other fish in the core group and are bottom feeders, meaning that their flesh is considered to be lower quality than the other fish in this grouping (Fishbase - Embiotoca jacksoni, 2023; Fishbase - Hyperprosopon ellipticum, 2023). As a result, Hyperprosopon ellipticum and Embiotica jacksoni could be targeted less in non-protected areas, meaning that they would experience less predatory pressure from the lack of protection. Anisotremus davidsonii also had no significant differences between populations in protected and non-protected areas (Table 5). Anisotremus davidsonii is consistently targeted by spearfishermen, as it tends to be larger than Hyperprosopon ellipticum and Embiotica jacksoni and has meat that is valued more highly (Fishbase - Anisotremus davidsonii, 2023). The lack of differences between protected and non-protected populations could be due to smaller sampling sizes, as there were only seven approaches in protected areas and three in non-protected areas, which were the smallest sample sizes of the core species (Table 4).
Fish behavior was consistent across different sites for both protected and non-protected areas (Figure 3). There were no significant differences between sites of the same protected status; however, Tablerock had an average FID that was less than half of the FIDs of the other protected areas (Table 3). Access to Tablerock Beach is difficult and the location is primarily known by Laguna Beach locals for being secluded and calm (California Beaches - Table Rock Beach, 2023). This could be the reason why the fish there seem to be less responsive to people.
There was no difference between the two sites that were partially protected and the two completely protected sites (Figure 3). There is debate about the effectiveness of partially protected marine reserves around the world (Zupan et al., 2018). While they are established with the same goal of promoting healthier marine communities, their effectiveness in accomplishing this goal has been mixed (Sciberras et al., 2013; Turnbull et al., 2021). There is some evidence that they provide increased benefit to populations when compared to completely open areas (Lester and Halpern, 2008; Sciberras et al., 2013; Sciberras et al., 2015), but other data has suggested that they are no better than completely unregulated sites (Hall et al., 2021; Turnbull et al., 2021). There are many types of partially protected areas, and this variation can be correlated to the variation in effectiveness (Zupan et al., 2018). Higher levels of protection resulted in greater biomass and abundance in partially protected areas (Zupan et al., 2018). For behavioral responses, there was no difference between fully and partially protected sites within our study. However, it is important to note that the partially protected sites in this study, Tablerock Beach and El Matador, both heavily limit the species that can be captured within their boundaries, and none of the species sampled in this study were allowed to be taken by fishermen in those areas (California Code of Regulations, 2023). That could mean these function as completely protected areas for the species studied. However, a study on the response of non-target fish to protection status found that even species that spear fishermen do not target respond with shorter FIDs in MPAs versus non-protected areas (Tran et al., 2016). This same study also found that a non-target species responded differently in the presence of a speargun, with longer FIDs when approached with a spear than without one (Tran et al., 2016). It is also important to evaluate the effects of spearfishing and protection on non-target species. This work could contribute to understanding the effectiveness and drawbacks of partially protected areas.
Expanding this study to more MPAs along the California coast would be beneficial, especially to the northern part of the state. Since MPAs in that area have shown slower recovery rates than those in Southern California (Sullivan-Stack et al., 2022), understanding how MPAs affect fish behavior in their boundaries could lend useful information to site managers. It would also be useful to see how protection status changes the behavior of fish over time, not only in comparison to unprotected sites. Since the MPAs in Northern California were implemented more recently (Sullivan-Stack et al., 2022), current studies could act as a baseline, and future studies incorporating FID would give insights on changes in behavior over time, further broadening our understanding of MPA effectiveness. Models have shown that it could take over a decade for changes in biomass and abundance to become noticeable (Kaplan et al., 2019), but there is no timeline for how long behavioral changes could take. FID should also be used to evaluate the effects of MPAs on the areas around them. MPAs have been shown to increase the amount of fish around them (i.e., spillover effect), which allows for the increased catch of fishermen (McClanahan and Mangi, 2000; Ziegler et al., 2022). There is also evidence that behavioral changes are observed in areas adjacent to MPAs, with a study showing that for a certain distance outside of an MPA, fish continued to have lower FIDs than fish that weren’t near protected areas (Januchowski-Hartley et al., 2012). However, this study was done in a tropical setting, and this type of work should also be done in temperate settings to see whether the pattern holds true in these types of ecosystems. By evaluating fish wariness in fish spilling over MPAs, managers will have more metrics to ensure connectivity between MPAs in the MPA network along the California coastline. Finally, this study shows the feasibility of using fish FIDs on the west coast of the Americas, which has not been done before (Nunes et al., 2018). FID is a useful tool for evaluating the effects of fishing on fish behavior and can be used by site managers to evaluate and help create effective marine reserve networks.
It could also be beneficial to see how acclimatization to human interactions changes FIDs of fish in non-fished areas. Terrestrial species have been shown to adapt to human interactions and have lower FIDs in areas with high human interaction (Blumstein et al., 2003; McGowan et al., 2014; Breck et al., 2019). These species are not being hunted by humans in urban areas, meaning that there is no need for increased FIDs due to the threat of human predation. It would be helpful to see if fish in MPAs with high human traffic behave the same way. While some studies have looked at how the use of an area affects fish FIDs (Benevides et al., 2018), there are still gaps in the literature such as how this can change with degrees of use and in different environments. Because our study focused on human predation as the changing factor of FID, we were not able to speak to the effects of general human interactions on fish FID.
Data availability statement
The raw data supporting the conclusions of this article will be made available by the authors, without undue reservation.
Ethics statement
Ethical approval was not required for the study involving animals in accordance with the local legislation and institutional requirements because fish were observed in their natural environment and were not harmed by data collector’s presence.
Author contributions
LH: Conceptualization, Data curation, Formal Analysis, Investigation, Methodology, Project administration, Validation, Visualization, Writing – original draft. FL: Data curation, Funding acquisition, Project administration, Supervision, Writing – review & editing.
Funding
The author(s) declare financial support was received for the research, authorship, and/or publication of this article. Funding was received from the NSF grants: DBI 1950350, DBI 2226092, and DEB 2312723. This study was also funded by the Pepperdine University’s Seaver Dean’s Office and Division of Natural Sciences.
Acknowledgments
We would also like to thank all those who helped with data collection including Leon Yang, Conor Kramer, Bryan Kaleta, Julian Jacobs, Sydney Soquet, Caleb Nieleback, Max Sprute, and Haley Hoidal. We would finally like to thank Dr. Helen Holmlund, Dr. Javier Monzon, Mharie Brigham, and Julian Jacobs for reviewing the manuscript and providing edits.
Conflict of interest
The authors declare that the research was conducted in the absence of any commercial or financial relationships that could be construed as a potential conflict of interest.
Publisher’s note
All claims expressed in this article are solely those of the authors and do not necessarily represent those of their affiliated organizations, or those of the publisher, the editors and the reviewers. Any product that may be evaluated in this article, or claim that may be made by its manufacturer, is not guaranteed or endorsed by the publisher.
Supplementary material
The Supplementary Material for this article can be found online at: https://www.frontiersin.org/articles/10.3389/fmars.2023.1270603/full#supplementary-material
Supplementary Table 1 | The number of approaches per species for all locations.
References
Anderson D. M., Burkholder J. M., Cochlan W. P., Glibert P. M., Gobler C. J., Heil C. A., et al. (2008). Harmful algal blooms and eutrophication: Examining linkages from selected coastal regions of the United States. Harmful Algae 8, 39–53. doi: 10.1016/j.hal.2008.08.017
Anderson D. M., Glibert P. M., Burkholder J. M. (2002). Harmful algal blooms and eutrophication: Nutrient sources, composition, and consequences. Estuaries 25, 704–726. doi: 10.1007/BF02804901
Benevides L. J., Pinto T. K., Nunes J., de A. C. C., Sampaio C. L. S. (2018). Fish escape behavior as a monitoring tool in the largest Brazilian multiple-use Marine Protected Area. Ocean Coast. Manage. 152, 154–162. doi: 10.1016/j.ocecoaman.2017.11.029
Birkeland C., Dayton P. K. (2005). The importance in fishery management of leaving the big ones. Trends Ecol. Evol. 20, 356–358. doi: 10.1016/j.tree.2005.03.015
Biro P. A., Post J. R. (2008). Rapid depletion of genotypes with fast growth and bold personality traits from harvested fish populations. Proc. Natl. Acad. Sci. 105, 2919–2922. doi: 10.1073/pnas.0708159105
Blumstein D. T., Anthony L. L., Harcourt R., Ross G. (2003). Testing a key assumption of wildlife buffer zones: is flight initiation distance a species-specific trait? Biol. Conserv. 110, 97–100. doi: 10.1016/S0006-3207(02)00180-5
Breck S. W., Poessel S. A., Mahoney P., Young J. K. (2019). The intrepid urban coyote: a comparison of bold and exploratory behavior in coyotes from urban and rural environments. Sci. Rep. 9, 2104. doi: 10.1038/s41598-019-38543-5
California Beaches - Table Rock Beach (2023) Calif. Beaches. Available at: https://www.californiabeaches.com/beach/table-rock-beach/ (Accessed March 16, 2023).
California Code of Regulations (2023). Available at: https://govt.westlaw.com/calregs/Document/IB4CC1D80249B11ED98DDA91C363C43D9?transitionType=Default&contextData=%28sc.Default%29 (Accessed February 2, 2023).
California Department of Fish and Wildlife (2023). Available at: https://wildlife.ca.gov/Conservation/Marine/MPAs/MLPA (Accessed March 10, 2023).
Carr M. H., Caselle J. E., Cavanaugh K., Freiwald J., Kroeker K., Pondella D., et al (2021) Monitoring and Evaluation of Kelp Forrest Ecosystems in the MLPA Marine Protected Area Network. Available at: https://caseagrant.ucsd.edu/system/files/2022-06/Kelp%20Forest%20Technical%20Report%20Narrative_v2.pdf.
Cinner J., Marnane M. J., McClanahan T. R., Almany G. R. (2006). Periodic closures as adaptive coral reef management in the indo-pacific. Ecol. Soc 11. doi: 10.5751/ES-01618-110131
Ciuti S., Northrup J. M., Muhly T. B., Simi S., Musiani M., Pitt J. A., et al. (2012). Effects of humans on behaviour of wildlife exceed those of natural predators in a landscape of fear. PloS One 7, e50611. doi: 10.1371/journal.pone.0050611
DeAngelis D. L., Barnthouse L. W., Van Winkle W., Otto R. G. (1990). A critical appraisal of population approaches in assessing fish community health. J. Gt. Lakes Res. 16, 576–590. doi: 10.1016/S0380-1330(90)71446-3
Dowling L., Bonier F. (2018). Should I stay, or should I go: Modeling optimal flight initiation distance in nesting birds. PloS One 13, e0208210. doi: 10.1371/journal.pone.0208210
Fishbase - Anisotremus davidsonii (2023) (Fishbase). Available at: https://www.fishbase.se/summary/Anisotremus-davidsonii.html (Accessed March 20, 2023).
Fishbase - Embiotoca jacksoni (2023) (Fishbase). Available at: https://www.fishbase.se/summary/3628 (Accessed March 20, 2023).
Fishbase - Girella nigricans (2023) (Fishbase). Available at: https://www.fishbase.se/summary/Girella-nigricans.html (Accessed March 20, 2023).
Fishbase - Hyperprosopon ellipticum (2023) (Fishbase). Available at: https://www.fishbase.se/summary/3632 (Accessed March 20, 2023).
Fishbase - Kyphosus azureus (2023) (Fishbase). Available at: https://www.fishbase.se/summary/Kyphosus-azureus (Accessed March 20, 2023).
Fishbase - Paralabrax clathratus (2023) (Fishbase). Available at: https://www.fishbase.se/summary/3335 (Accessed March 20, 2023).
Fisheries Economics of the United States, 2016 | NOAA Fisheries (2021) NOAA. Available at: https://www.fisheries.noaa.gov/content/fisheries-economics-united-states-2016 (Accessed March 4, 2023).
Free C. M., Vargas Poulsen C., Bellquist L. F., Wassermann S. N., Oken K. L. (2022). The CALFISH database: A century of California’s non-confidential fisheries landings and participation data. Ecol. Inform. 69, 101599. doi: 10.1016/j.ecoinf.2022.101599
Geldmann J., Barnes M., Coad L., Craigie I. D., Hockings M., Burgess N. D. (2013). Effectiveness of terrestrial protected areas in reducing habitat loss and population declines. Biol. Conserv. 161, 230–238. doi: 10.1016/j.biocon.2013.02.018
Gotanda K. M., Turgeon K., Kramer D. L. (2009). Body size and reserve protection affect flight initiation distance in parrotfishes. Behav. Ecol. Sociobiol. 63, 1563–1572. doi: 10.1007/s00265-009-0750-5
Hall A. E., Cameron D. S., Kingsford M. J. (2021). Partially protected areas as a management tool on inshore reefs. Rev. Fish Biol. Fish. 31, 631–651. doi: 10.1007/s11160-021-09654-y
Hamilton S., Starr R., Wendt D., Ruttenberg B., Caselle J. E., Semmens B., et al (2021) California Collaborative Fisheries Research Program (CCFRP) – Monitoring and Evaluation of California Marine Protected Areas. Available at: https://caseagrant.ucsd.edu/sites/default/files/CCFRP_Final_Report.pdf.
Handegard N. O., Michalsen K., Tjøstheim D. (2003). Avoidance behaviour in cod (Gadus morhua) to a bottom-trawling vessel. Aquat. Living Resour. 16, 265–270. doi: 10.1016/S0990-7440(03)00020-2
Heathcote R. J. P., Darden S. K., Franks D. W., Ramnarine I. W., Croft D. P. (2017). Fear of predation drives stable and differentiated social relationships in guppies. Sci. Rep. 7, 41679. doi: 10.1038/srep41679
Hixon M. A. (1981). An experimental analysis of territoriality in the california reef fish embiotoca jacksoni (Embiotocidae). Copeia 1981, 653–665. doi: 10.2307/1444571
Hoare D. J., Krause J., Peuhkuri N., Godin J.-G. J. (2000). Body size and shoaling in fish. J. Fish Biol. 57, 1351–1366. doi: 10.1111/j.1095-8649.2000.tb02217.x
Huijbers C., Nagelkerken I., Govers L., van de Kerk M., Oldenburger J., de Brouwer J. (2011). Habitat type and schooling interactively determine refuge-seeking behavior in a coral reef fish throughout ontogeny. Mar. Ecol. Prog. Ser. 437, 241–251. doi: 10.3354/meps09264
Jackson J. B. C., Kirby M. X., Berger W. H., Bjorndal K. A., Botsford L. W., Bourque B. J., et al. (2001). Historical overfishing and the recent collapse of coastal ecosystems. Science 293, 629–637. doi: 10.1126/science.1059199
Januchowski-Hartley F. A., Nash K. L., Lawton R. J. (2012). Influence of spear guns, dive gear and observers on estimating fish flight initiation distance on coral reefs. Mar. Ecol. Prog. Ser. 469, 113–119. doi: 10.3354/meps09971
Kaplan K. A., Yamane L., Botsford L. W., Baskett M. L., Hastings A., Worden S., et al. (2019). Setting expected timelines of fished population recovery for the adaptive management of a marine protected area network. Ecol. Appl. 29, e01949. doi: 10.1002/eap.1949
Kells V., Rocha L., Allen L. G. (2016). A Field Guide to Coastal Fishes From Alaska to California. (Baltimore, Maryland: Johns Hopkins University Press).
Kindinger T. L., Albins M. A. (2017). Consumptive and non-consumptive effects of an invasive marine predator on native coral-reef herbivores. Biol. Invasions 19, 131–146. doi: 10.1007/s10530-016-1268-1
Laundre J. W., Hernandez L., Ripple W. J. (2010). The landscape of fear: ecological implications of being afraid. Open Ecol. J. 3, 1–7. doi: 10.2174/1874213001003030001
Legagneux P., Ducatez S. (2013). European birds adjust their flight initiation distance to road speed limits. Biol. Lett. 9, 20130417. doi: 10.1098/rsbl.2013.0417
Lester S. E., Halpern B. S. (2008). Biological responses in marine no-take reserves versus partially protected areas. Mar. Ecol. Prog. Ser. 367, 49–56. doi: 10.3354/meps07599
Lima S. L., Dill L. M. (1990). Behavioral decisions made under the risk of predation: a review and prospectus. Can. J. Zool. 68, 619–640. doi: 10.1139/z90-092
Lowe C. G., Topping D. T., Cartamil D. P., Papastamatiou Y. P. (2003). Movement patterns, home range, and habitat utilization of adult kelp bass Paralabrax clathratus in a temperate no-take marine reserve. Mar. Ecol. Prog. Ser. 256, 205–216. doi: 10.3354/meps256205
Marine Life Protection Act (1999). Available at: https://leginfo.legislature.ca.gov/faces/codes_displayText.xhtml?lawCode=FGC&division=3.&title=&part=&chapter=10.5.&article= (Accessed March 4, 2023).
Mason T. J., Lowe C. G. (2010). Home range, habitat use, and site fidelity of barred sand bass within a southern California marine protected area. Fish. Res. 106, 93–101. doi: 10.1016/j.fishres.2010.07.008
Matassa C. M., Trussell G. C. (2011). Landscape of fear influences the relative importance of consumptive and nonconsumptive predator effects. Ecology 92, 2258–2266. doi: 10.1890/11-0424.1
Matthews K. R. (1985). Species similarity and movement of fishes on natural and artificial reefs in Monterey Bay, California. Bull. Mar. Sci. 37, 252–270.
McArthur C., Banks P. B., Boonstra R., Forbey J. S. (2014). The dilemma of foraging herbivores: dealing with food and fear. Oecologia 176, 677–689. doi: 10.1007/s00442-014-3076-6
McClanahan T. R., Mangi S. (2000). Spillover of exploitable fishes from a marine park and its effect on the adjacent fishery. Ecol. Appl. 10, 1792–1805. doi: 10.1890/1051-0761(2000)010[1792:SOEFFA]2.0.CO;2
McGowan M. M., Patel P. D., Stroh J. D., Blumstein D. T. (2014). The effect of human presence and human activity on risk assessment and flight initiation distance in skinks. Ethology 120, 1081–1089. doi: 10.1111/eth.12281
Milinski M. (1986). “Constraints Placed by Predators on Feeding Behaviour,” in The Behaviour of Teleost Fishes. Ed. Pitcher T. J. (Boston, MA: Springer US), 236–252. doi: 10.1007/978-1-4684-8261-4_9
Miller D. J., Lea R. N. (1972). Guide to the Coastal Marine Fishes of California (California: State of California, Department of Fish and Game).
Nunes J. A. C. C., Costa Y., Blumstein D. T., Leduc A. O. H. C., Dorea A. C., Benevides L. J., et al. (2018). Global trends on reef fishes’ ecology of fear: Flight initiation distance for conservation. Mar. Environ. Res. 136, 153–157. doi: 10.1016/j.marenvres.2018.02.011
Nunes J., de A. C. C., Sampaio C. L. S., Barros F. (2015). The influence of structural complexity and reef habitat types on flight initiation distance and escape behaviors in labrid fishes. Mar. Biol. 162, 493–499. doi: 10.1007/s00227-014-2578-4
Petit I. J., Gaymer C. F., Friedlander A. M., Gusmao J. B. (2022). Depth as a fish refuge from spearfishing at Rapa Nui: Flight behaviour in the Pacific rudderfish Kyphosus sandwicensis. Aquat. Conserv. Mar. Freshw. Ecosyst. 32, 727–740. doi: 10.1002/aqc.3795
Pew Charitable Trusts (2016) Older, Bigger Red Snapper Have More Offspring and Boost Species Recovery. Available at: http://pew.org/2aR0cAm (Accessed December 1, 2022).
Porzio D. (2014). Review of Selcted California Fishereies for 2013: Coastal Pelagic Finfish, Market Squid, Groundfish, Highly Migratory Species, Dungeness Crab, Basses, Surfperch, Abalone, Kelp and Edible Algae, and Marine Aquaculture (California Department of Fish and Wildlife). Available at: http://calcofi.com/publications/calcofireports/v55/Vol_55_FisheriesReview.pdf.
Qvarnström A., Price T. D. (2001). Maternal effects, paternal effects and sexual selection. Trends Ecol. Evol. 16, 95–100. doi: 10.1016/S0169-5347(00)02063-2
Saarman E. T., Carr M. H. (2013). The California Marine Life Protection Act: A balance of top down and bottom up governance in MPA planning. Mar. Policy 41, 41–49. doi: 10.1016/j.marpol.2013.01.004
Samia D. S. M., Bessa E., Blumstein D. T., Nunes J. A. C. C., Azzurro E., Morroni L., et al. (2019). A meta-analysis of fish behavioural reaction to underwater human presence. Fish Fish. 20, 817–829. doi: 10.1111/faf.12378
Sciberras M., Jenkins S. R., Kaiser M. J., Hawkins S. J., Pullin A. S. (2013). Evaluating the biological effectiveness of fully and partially protected marine areas. Environ. Evid. 2, 4. doi: 10.1186/2047-2382-2-4
Sciberras M., Jenkins S. R., Mant R., Kaiser M. J., Hawkins S. J., Pullin A. S. (2015). Evaluating the relative conservation value of fully and partially protected marine areas. Fish Fish. 16, 58–77. doi: 10.1111/faf.12044
Smith V. H. (2003). Eutrophication of freshwater and coastal marine ecosystems a global problem. Environ. Sci. pollut. Res. 10, 126–139. doi: 10.1065/espr2002.12.142
Sommer C., Fisher W., Krupp F., Schneider W., Carpenter K. E., Niem V. (1995) Guía FAO para la identificación de especies para los fines de la pesca. Available at: https://www.fao.org/3/v6250s/v6250s00.htm (Accessed March 20, 2023).
Sørdalen T. K., Halvorsen K. T., Olsen E. M. (2022). Protection from fishing improves body growth of an exploited species. Proc. R. Soc B Biol. Sci. 289, 20221718. doi: 10.1098/rspb.2022.1718
Stamoulis K. A., Harvey E. S., Friedlander A. M., Williams I. D., Weng K. C., Wiggins C., et al. (2019). Flight behavior of targeted fishes depends on variables other than fishing. Ecol. Indic. 96, 579–590. doi: 10.1016/j.ecolind.2018.09.006
Stankowich T., Blumstein D. T. (2005). Fear in animals: a meta-analysis and review of risk assessment. Proc. R. Soc B Biol. Sci. 272, 2627–2634. doi: 10.1098/rspb.2005.3251
Stenstrom J. (2018) Spearfishing California: 21 Fish Worth Hunting and How to Find Them (CAST SPEAR). Available at: https://castandspear.com/spearfishing-california/ (Accessed January 28, 2023).
Sullivan-Stack J., Aburto-Oropeza O., Brooks C. M., Cabral R. B., Caselle J. E., Chan F., et al. (2022). A scientific synthesis of marine protected areas in the United States: status and recommendations. Front. Mar. Sci. 9. doi: 10.3389/fmars.2022.849927
Tanaka Y., Minggat E., Roseli W. (2021). The impact of tropical land-use change on downstream riverine and estuarine water properties and biogeochemical cycles: a review. Ecol. Process. 10, 40. doi: 10.1186/s13717-021-00315-3
The First 10 Years: Measuring the Success of California’s Underwater Parks (2023). Available at: https://www.nrdc.org/sites/default/files/california-underwater-parks-decade-review-fs.pdf.
Tran D. S. C., Langel K. A., Thomas M. J., Blumstein D. T. (2016). Spearfishing-induced behavioral changes of an unharvested species inside and outside a marine protected area. Curr. Zool. 62, 39–44. doi: 10.1093/cz/zov006
Turnbull J. W., Johnston E. L., Clark G. F. (2021). Evaluating the social and ecological effectiveness of partially protected marine areas. Conserv. Biol. 35, 921–932. doi: 10.1111/cobi.13677
Valle C. F. (1989) The homing behavior and intertidal movements of the opaleye, Girella nigricans (Pisces: Kyphosidae) - ProQuest. Available at: https://www.proquest.com/openview/4ff807babc32330fb0b2fe33c637592c/1?pq-origsite=gscholar&cbl=18750&diss=y (Accessed September 7, 2023).
Wertz S., Shen C., Worden S., Van A., Gonzales K., Prall M., et al. (2022). Decadal management review. (California: The California Department of Fish and Wildlife). Vol. 2022. 127.
Ydenberg R. C., Dill L. M. (1986). “The Economics of Fleeing from Predators,” in Advances in the Study of Behavior. Eds. Rosenblatt J. S., Beer C., Busnel M.-C., Slater P. J. B. (Burnaby, British Columbia, Canada: Academic Press), 229–249. doi: 10.1016/S0065-3454(08)60192-8
Ziegler S. L., Brooks R. O., Hamilton S. L., Ruttenberg B. I., Chiu J. A., Fields R. T., et al. (2022). External fishing effort regulates positive effects of no-take marine protected areas. Biol. Conserv. 269, 109546. doi: 10.1016/j.biocon.2022.109546
Keywords: MPA (marine protected area), flight initiation distance (FID), California, fish, health, community, behavior, fitness
Citation: Himes L and La Valle FF (2023) Southern California marine protected areas promote bolder fish populations. Front. Mar. Sci. 10:1270603. doi: 10.3389/fmars.2023.1270603
Received: 01 August 2023; Accepted: 06 October 2023;
Published: 20 October 2023.
Edited by:
Carlo Pipitone, CNR - IAS Institute of Anthropic Impacts and Sustainability in Marine Environment, ItalyReviewed by:
Benjamin Toscano, Trinity College, United StatesJosé Lino Vieira De Oliveira Costa, University of Lisbon, Portugal
Copyright © 2023 Himes and La Valle. This is an open-access article distributed under the terms of the Creative Commons Attribution License (CC BY). The use, distribution or reproduction in other forums is permitted, provided the original author(s) and the copyright owner(s) are credited and that the original publication in this journal is cited, in accordance with accepted academic practice. No use, distribution or reproduction is permitted which does not comply with these terms.
*Correspondence: Florybeth Flores La Valle, florybeth.lavalle@pepperdine.edu