- 1Departamento de Geociencias Marinas, Instituto de Ciencias del Mar, Consejo Superior de Investigaciones Científicas (CSIC), Barcelona, Spain
- 2Department of Earth Science, Geological Institute, Zurich, Switzerland
- 3GRC Geociències Marines, Departament de Dinàmica de la Terra i de l'Oceà, Universitat de Barcelona, Barcelona, Spain
- 4Tragsatec-Secretaria General de Pesca, Madrid, Spain
- 5Department of Geosciences, University of Malta, Msida, Malta
- 6Seafloor Processes Lab, Monterey Bay Aquarium Research Institute (MBARI), Moss Landing, CA, United States
Bottom trawling is a fishing method that involves towing of nets along the seafloor to catch demersal species. The dragging of trawling gears along the seafloor results in scraping and ploughing of the surficial sediments, leading to the formation of turbid plumes of resuspended sediments and causing measurable changes in the seabed morphology. High-resolution multibeam data, side scan sonar, sediment grain size and vessel tracking data have been used to investigate the impact of bottom trawling on the seafloor morphology and surficial sediments of the northern Catalan continental shelf (NW Mediterranean), providing new insights into the impact of this anthropogenic activity on the seafloor. Multibeam data evidenced the occurrence of large-scale erosive features as a consequence of repeated scouring by fishing gears in localized areas. They are characterized by elongated (70-300 m wide and up to 8 km long) channelized areas of high backscatter with variable incision (from 0.2 m to 1.2 m). The spatial distribution of these morphologies shows a similar pattern to that observed in the fishing intensity, with maximum values in the areas of increased trawling intensity, corresponding to the main fishing grounds. Side scan sonar data also shows higher densities of trawl marks in these areas than in the surroundings. Sediment cores collected on these features show an upward-coarsening trend in the first 4-5 cm of the core, suggesting that part of the finer fraction resuspended by trawling is winnowed, increasing the sand content of the surface sediment. The identification of such large erosive morphologies in the main fishing grounds evidences that repeated trawling over the same fishing ground during decades can result in deep excavation of the seafloor, leading to permanent large-scale morphological changes. Furthermore, the evolution of these erosive features over a 13-year interval points towards long recovery periods after the cessation of trawling activities.
1 Introduction
Bottom trawling is a fishing method that involves dragging heavy nets along the seabed to catch demersal species. It is recognized as one of the most widespread anthropogenic activities affecting the seabed, particularly on the continental shelf (Eigaard et al., 2017). Globally, about 22 million km2 of the seafloor is affected by commercial trawling each year (Halpern et al., 2008), mostly (61%) on continental shelves (Oberle et al., 2016a). Commercial trawling has direct impacts on benthic fauna and their habitats (Watling and Norse, 1998). In the Mediterranean Sea, the trawling footprint in shallow areas (<200 m) ranges between 57 and 86% (Eigaard et al., 2017). Aside from the impacts on benthic communities, bottom trawling gear has direct physical effects on the seafloor itself. The bottom trawl consists of a large conical net with a funnel-shaped mouth and a small closed end (cod end). The mouth of a trawl net has two weighted trawl doors (otter boards) that help to keep the net open horizontally and provide stability. In addition to the trawl doors, the bottom of the net consist of a thick weighted steel cable (footrope) that remains into contact with the seabed during trawling (Figure S1). The dragging of trawling gears along the seafloor results in scraping and ploughing of the surface sediments. The trawl doors erodes the seabed, creating centimeter to decimeter-scale furrows, known as trawl marks (Krost et al., 1990). In addition, the ground rope and weights of the trawl gear can cause scouring and flattening of the seabed, particularly on muddy substrates (Churchill, 1989; Jones, 1992; Martín et al., 2014). Bottom trawling also leads to sediment resuspension, contributing to the formation of turbid plumes and persistent nepheloid layers, principally when it is conducted over fine-grained sediments (Black and Parry, 1994; Pilskaln et al., 1998; Palanques et al., 2001; Durrieu de Madron et al., 2005; Palanques et al., 2014). Over larger spatial scales, high-resolution multibeam data on steep submarine canyon flanks revealed a general flattening and homogenization of the deep seafloor relief in heavily trawled areas, suggesting that recurrent trawling over the same fishing grounds would also result in a reduction in the morphological complexity (Puig et al., 2012).
The physical impact of bottom trawling on the seafloor morphology can be observed using acoustical and/or optical imaging systems such as side scan sonar, multibeam echosounder and underwater footage (see review by Oberle et al., 2018). In side scan sonar images and high frequency multibeam echosounder backscatter data, the trawl marks are visible as distinct reflectivity tracks or furrows across the seabed. These marks can be observed over large areas and in high densities, thus providing a visual representation of the extent and intensity of trawling activity in a fishing ground (Schwinghamer et al., 1998; Friedlander et al., 1999; Humborstad et al., 2004; Malik and Mayer, 2007; Smith et al., 2007). Remotely Operated Vehicle (ROV) footage provide a close-up view of the seabed, allowing for the identification and differentiation of small-scale features caused by bottom trawling gear. These features include furrow marks from trawl doors; scrape marks from sweeps and trawl wires; local accumulations of sediment displaced and piled up by the action of bottom trawling; and topographical flattening by the trawling nets (Smith et al., 2000; Humborstad et al., 2004; Smith et al., 2007).
The degree of environmental perturbation produced by bottom trawling on the seafloor largely depends on the design and rigging of the gear, its weight, the towing speed, the type of sediment and the local hydrodynamics such as tidal currents, waves or storms (Lucchetti and Sala, 2012; Buhl-Mortensen et al., 2013; O’Neill and Ivanović, 2016). Shellfish and tickler chain beam trawls can penetrate deeper into the sediment than otter trawls and seines (Buhl-Mortensen et al., 2013). The penetration of the trawling gear into the seabed is higher in muddy sediments and the persistence of trawl marks is longer (Krost et al., 1990; Smith et al., 2007). Indeed, trawl marks can persist a few days in coastal sandy areas (Depestele et al., 2016) but up to a few years in rather deeper muddy environments (Palanques et al., 2001; Gilkinson et al., 2015).
The patterns, persistence and degradation of trawl marks in coastal and shelf areas have been intensively studied during the last years because of their interest in estimating the fishing effort and assessing the physical impact of bottom trawling on the seabed (Palanques et al., 2001; Gilkinson et al., 2015; Depestele et al., 2016; Buhl-Mortensen and Buhl-Mortensen, 2018). However, evidence of the physical impact of bottom trawling over larger spatial and temporal scales on continental shelf environments remains poorly constrained (e.g., Oberle et al., 2016a; Oberle et al., 2016b). This study aims to fill this gap by investigating the impact of recurrent bottom trawling on the continental shelf seafloor morphology based on the analysis of high-resolution multibeam data (bathymetry and backscatter), side scan sonar, sediment grain size and vessel tracking data. The study focuses on the northern Catalan continental shelf in the NW Mediterranean Sea (Figure 1), where intense bottom trawling has been occurring for a long time.
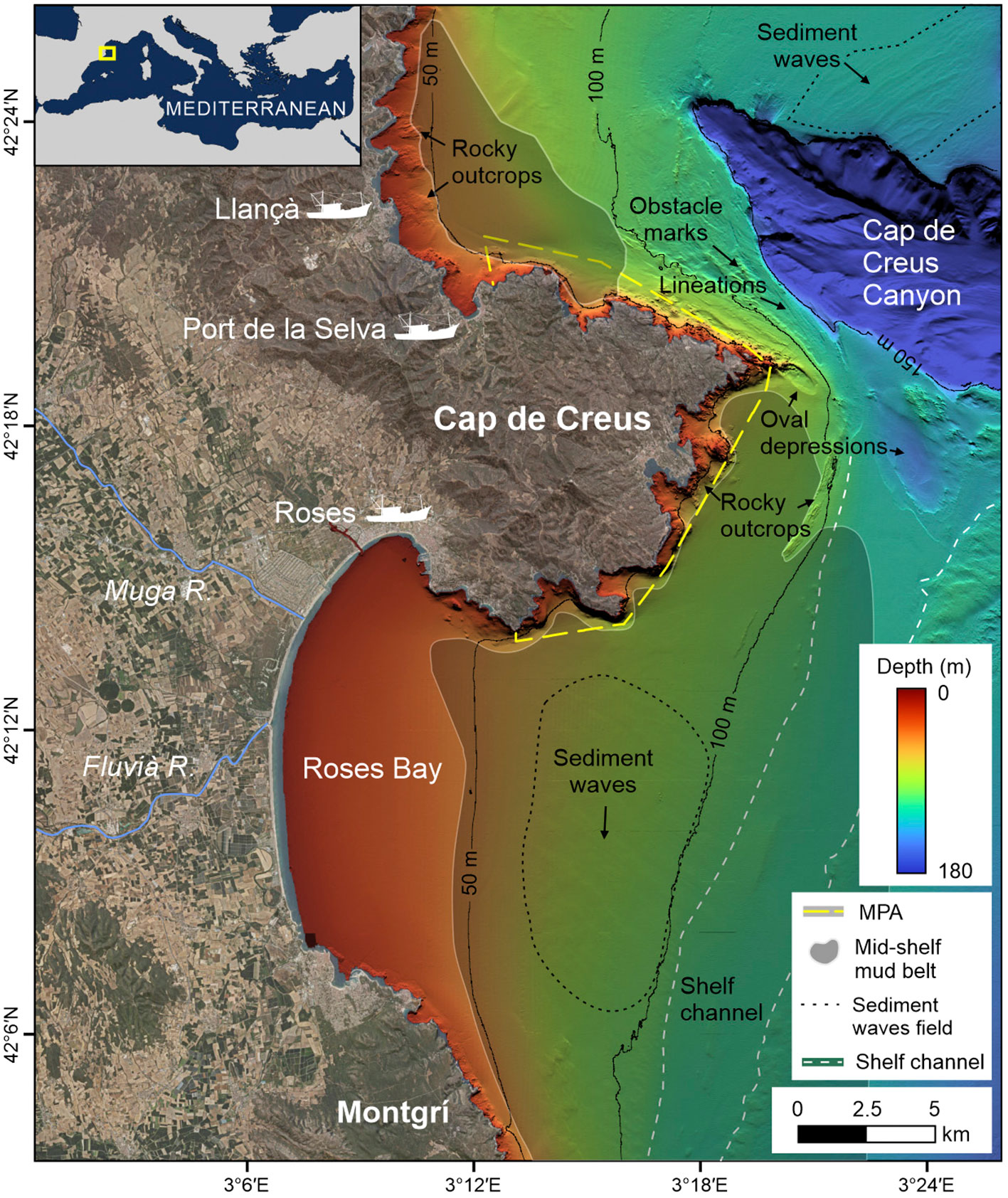
Figure 1 Shaded-relief colored multibeam bathymetry of the Catalan continental margin (4 m grid size) including the morphological interpretation by Durán et al. (2014). MPA, Marine Protected Area of the Cap de Creus Natural Park. Topographic data and orthophoto from the Institut Cartogràfic i Geológic de Catalunya (www.icgc.cat).
2 Study area
The study area extends from the north of the Cap de Creus Peninsula, to the Montgrí promontory, and is affected by fishing activities from Llançà, Port de la Selva and Roses harbors (Figure 1). The continental shelf has a complex morphology and a highly variable width, mainly related to the irregular morphology of the coastline (capes and bays) and the presence of the Cap de Creus submarine canyon (Canals et al., 2004). The shelf is narrower off the Cap de Creus headland (2.6 km) because of the proximity of the southern rim of the submarine canyon to the shore, and it is wider off Roses Bay (up to 30 km), with the shelf edge located at 110-140 m water depth (Durán et al., 2014). The seafloor relief on the inner shelf (down to 60 m depth) displays high variability; it is irregular off the rocky coast north and along the Cap de Creus Peninsula, but it is markedly homogeneous in Roses Bay, where the Muga and the Fluvia rivers discharge (Figure 1). The middle and outer shelf off the Cap de Creus Peninsula is characterized by a large area of rocky outcrops partially covered by very coarse sediment (ITGE, 1994; García-García et al., 2012; Lo Iacono et al., 2012). Erosive features, such as rocky outcrops, lineations, elongated and oval depressions, and obstacle marks dominate this area (Durán et al., 2014). Off Roses Bay, a N–S oriented, 18 km-wide area of silty sediment extends from the Cap de Creus Peninsula southwards along the mid-shelf (Figure 1) (Lo Iacono et al., 2012; Durán et al., 2014). The main seafloor feature observed in this area is a NNE–SSW oriented shallow channel that connects the eroded shelf offshore the Cap de Creus Peninsula with the Palamòs Canyon head, located southward of the study area (Lastras et al., 2011; Durán et al., 2014). At the outer shelf, the sediment is composed of medium and coarse sand or detritic bioclastic gravels interpreted as relict facies (Ercilla et al., 1994; Lo Iacono et al., 2012).
The Catalan coast is microtidal with a tidal range of less than 0.2 m. Wave climate has a seasonal pattern, with severe storms in late autumn, winter and early spring. Waves come mainly from the NNW-N and E, with the eastern storms being the most energetic ones due to the longer fetch. Northerly storms are triggered by the strong, cold and persistent northern wind (known as Tramuntana) that occurs mainly in winter. During the largest easterly storms, wave peak periods of 13 s and maximum significant wave heights of up to 7 m can be reached (Gómez et al., 2005; Sanchez-Vidal et al., 2012). These high energetic eastern storms trigger an intense alongshore current towards the south (MOPU, 1979; DGPC, 1986). The general circulation is dominated by the geostrophic Northern Current, which form a 30-km wide meandering stream that flows southward along the continental shelf break and slope (Millot, 1999), eventually entering into the continental shelf (Font et al., 1995). It moves at speeds of up to 35 cm s-1 near the surface (Durrieu de Madron et al., 1990), generating a dominant south-westward transport of suspended sediment (Arnau et al., 2004).
In the northwestern Mediterranean Sea, bottom trawling is widely distributed over the continental shelf, showing a heterogeneous distribution pattern with intensive bottom trawling in localized regions (Kroodsma et al., 2018). In the study area, up to 29 bottom trawlers from the abovementioned fishing harbors, operate over the same fishing grounds year-round on a daily basis, except for weekends, holidays, and during stormy periods, conducting multiple hauls each day. Trawlers are relatively small (20-28 m in length), with gross tonnage varying from 57 to 178 Gt (Table S1). The most commonly used fishing gear is the “otter trawl”, which involves deploying nets over the stern that are kept open horizontally using otter boards that generate big footprints on the seafloor (Eigaard et al., 2016).
Bottom trawling is allowed throughout the whole study area, albeit with some exceptions, such as the areas shallower than 50 m water depth and the coastal area around the Cap de Creus Peninsula. According to the Order of 30 July 1975 (BOE-A-1975-17128), implemented in 1988 (Spanish Royal Decree 679/1988; BOE-A-1988-16647), bottom trawling on the Spanish Mediterranean continental shelf is banned at depths shallower than 50 meters. This regulation was further implemented in Catalonia in 1999 (BOE-A-1999-4185), extending the restriction to the three nautical mile coastal boundary or within the 50 m isobath when this depth is reached at a shorter distance. In the study area, these boundaries vary throughout the year, being 50 m between September and March and 75 m between April and August. Trawling was particularly banned in the coastal area around the Cap de Creus Peninsula in 1998, after the Cap de Creus Natural Park was established by the Autonomous Government of Catalonia through Law 12/1985. The Cap de Creus Natural Park covers an area of 138 km2, 22% of which corresponds to the marine area that is included in the network of Marine Protected Areas (MPAs) under the framework of Natura 2000.
3 Data and methods
The assessment of the impact of bottom trawling on the Roses continental shelf is based on the interpretation of multibeam echosounder data (swath bathymetry and backscatter), side scan sonar, sediment cores grain size and vessel tracking data (Figure 2).
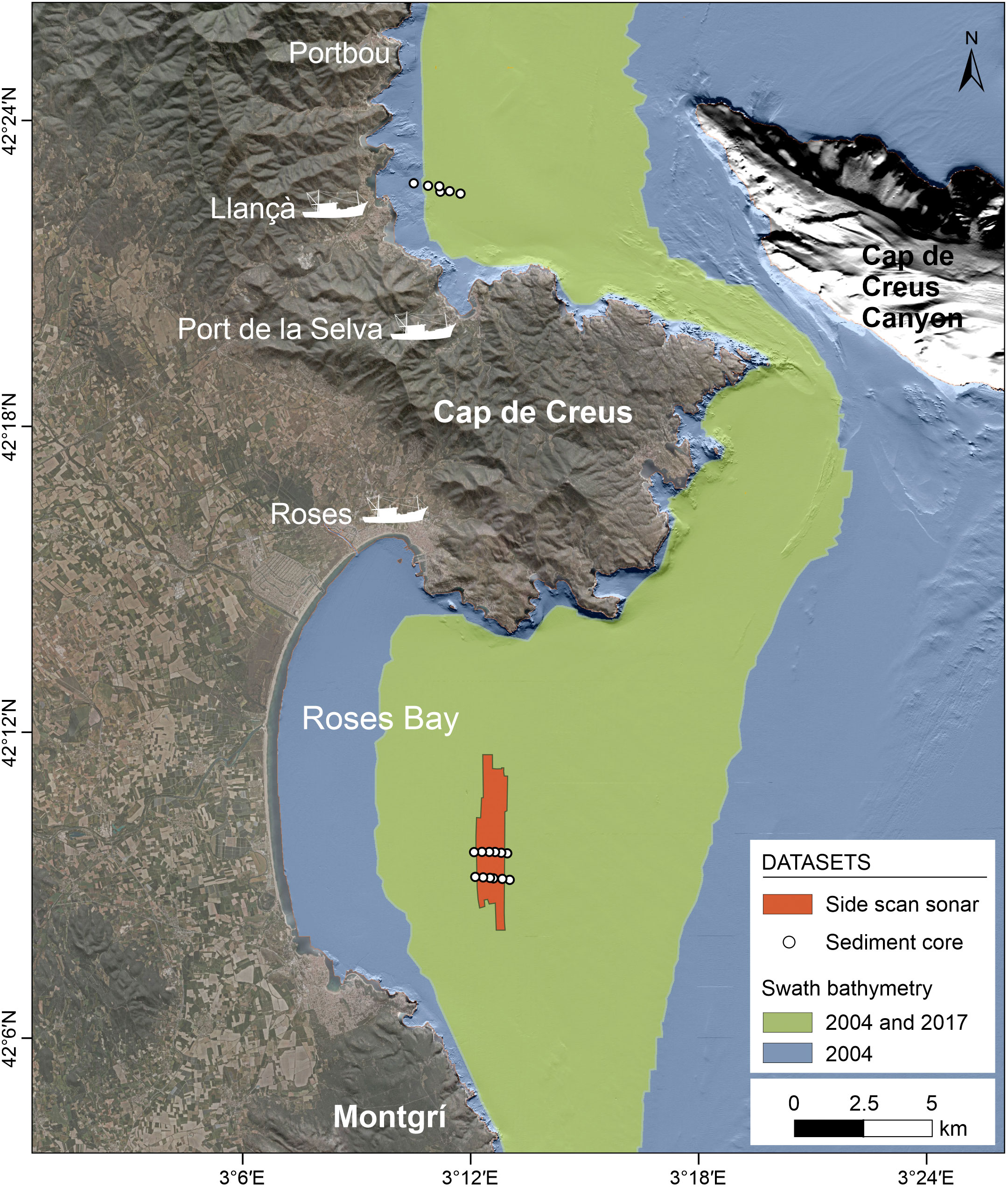
Figure 2 Shaded-relief map of the Catalan continental shelf including the multibeam bathymetry coverage acquired in two surveys (2004 and 2017) and the location of side scan sonar data and sediment cores. Topographic data and orthophoto from the Institut Cartogràfic I Geológic de Catalunya (www.icgc.cat).
3.1 Bathymetry
Swath-bathymetry covering the whole continental shelf was acquired in 2004 as part of the ESPACE Project “Characteristics of the systematic study of the Spanish continental shelf and upper slope” by the Spanish Institute of Oceanography, using a Kongsberg EM-3000D multibeam echosounder (Figure 2). The EM-3000D is a system with two sonar heads, each of them with a swath width of 130°. This system uses 254 beams and was operated at a frequency of 300 kHz to assure a narrow beam width (1.5°) and a maximum ping rate of 40 Hz, resulting in a depth accuracy of 5 cm root mean square. Sound velocity profiles (SVP) were collected to correct the acoustic data for water-column sound speed variations. An Octans Inertial Measurement Unit was used for motion corrections and a DGPS provided vessel position during the cruise. A new swath bathymetry was obtained in 2017 by the company GEOMYTSA using a RESON SeaBat 8101, as part of the project “Extension of the marine geophysical survey to a depth of 100 m between the port of Barcelona and Portbou (Girona)” commissioned by the General Subdirectorate for Coastal Protection of the Spanish Ministry for Ecological Transition and the Demographic Challenge. The SeaBat 8101 operates at a frequency of 240 kHz, using 101 beams (beam spacing 1.5°). The surveyed area in this cruise covered the middle continental shelf from 45 m to 115 m water depth (Figure 2). The 2004 survey was used to describe the morphology and backscatter characteristics of the study area whereas the 2017 survey was used to compare both datasets and assess morphological changes.
Post-processing of multibeam data (including correction for heading, depth, pitch, heave and roll) collected during the 2004 survey was conducted using the Teledyne CARIS HIPS and SIPS TM 11.7 Hydrographic Data Processing System. Tidal and sound velocity corrections were applied and the sounding data were cleaned to remove erroneous soundings. Filtered soundings were gridded into 4 m resolution bathymetric surface, providing 100% coverage of the swathed seabed. Backscatter data was processed with QPS Fledermaus software using the Geocoder algorithm for radiometric and geometric corrections, and mosaicking. The original time series registered by the multibeam sonar were corrected for beam pattern and angle varying gains (AVG) using a window size of 300, with a range of normalization between 30° and 60°. The intensity was filtered between 0 and -70 dB. No range filters were applied during processing. Backscatter strength, often measured in decibels (dB), is a measure of the amount of acoustic energy scattered from the seafloor back to the transducer. It depends not only on the environmental and system properties, but also on several seabed characteristics (Collier and Brown, 2005). Main seabed factors include seabed topography (slope), micro-topography, heterogeneity within the near-surface sediments, biotic elements, and sediment characteristics (grain size and sorting or shell content), among others. Therefore, its correlation to grain size is not straightforward (Gaida et al., 2019). In this work, backscatter imagery was used to qualitatively interpret sediment type, based on previous works in the study area (ITGE, 1994; García-García et al., 2012; Lo Iacono et al., 2012; Durán et al., 2014; Durán et al., 2017) and bottom sediment samples. According to this information, in seabed areas with homogeneous relief (no significant variations in slope), fine sediments typically result in weaker backscatter signals (dark tones in the backscatter imagery), while coarser sediments tend to produce stronger backscatter responses (light tones in the backscatter imagery).
Bathymetric and backscatter data were integrated into a geographic information system (QGIS) for the morphological analysis. Bathymetric data from the 2017 survey were processed using HYPACK HYSWEEP® software and provided as a 5 m bathymetric grid by GEOMYTSA. Final maps were projected into Universal Transverse Mercator projection zone 31 N (UTM 31N) in the European Terrestrial Reference System 1989 (ETRS89).
3.2 Side scan sonar
Side scan sonar (SSS) data were collected in the Roses continental shelf in September 2017 aboard the R/V Sarmiento de Gamboa. The side scan sonar used was the deep-towed large-scale Edge-Tech 2400-DSS/DT-1 system, emitting at a frequency of 120 kHz (Figure 2). Side scan sonar records were visualized and analyzed using the SonarWiz software from Cheasepeake Technology. Trawl marks identified and mapped in the side-scan sonar data using SonarWiz were incorporated into the geographic information system to determine density and direction.
3.3 Sediment cores
Sediment cores were also collected during the ABIDES-ROV cruise in 2017, using a HAPS bottom corer (Figure 2). Sampling locations were selected based on the spatial distribution of the areas of high backscatter observed in the 2004 bathymetric survey. A total of 18 sediment cores were retrieved in the Roses Bay (12 cores) and off Llançà (6 cores). The upper 10 cm of each sediment core were subsampled at 1 cm intervals. Grain size fractions were determined using a Horiba Partica LA-950V2 particle-size analyzer, with an accuracy of 0.6% and 0.1% precision. Prior to this analysis, 1–4 g of each sample were oxidized using 20% H2O2 for a week and then left overnight with a solution of P2O7- to disaggregate the sediment particles.
3.4 Fishing activity
The bottom trawling activity in the Llançà continental shelf during the study period (2005-2017) was obtained using data from the satellite-based tracking Vessel Monitoring Systems (VMS) provided by the Fishing Monitoring Centre of the Spanish Secretariat of Maritime Fishing (SEGEMAR). Since VMS data is broadcasted and stored in 2-hours intervals, vessel tracks were interpolated to 10-minute intervals using the R package VMSbase (Russo et al., 2014). With this higher-resolution data, fishing hauls were identified following the method explained in Paradis et al. (2021). This method first identifies trawling speeds, which for this dataset ranged between 2 and 4.3 knots. Hauls were then defined as consecutive entries that met the trawling speed criteria for at least 60 minutes, taking into account possible false-positives and false-negatives. Fishing intensity was then computed as swept area ratio (SAR) by counting the number of hauls within a 50 m radius, assuming a gear width of bottom trawlers operating in this area being ~100 m wide. SARs were then represented in 25 m x 25 m grid cells to report fishing intensity as hauls per hectare.
3.5 Geomorphometry
The quantification of the morphological impact of bottom trawling on the seafloor morphology was particularly challenging due to the low relief of the morphologies produced by trawling in relation to the general seafloor gradient. In this work, we used different terrain attributes derived from bathymetric data using QGIS. These terrain attributes include: terrain slope using an analysis sale of 3 x 3 pixels’ neighborhood; terrain aspect (3 x 3 pixels) and Bathymetric Position Index (BPI), as modified from the topographic position index defined by Weiss (2001), with an inner radius of 4, and an outer radius of 40. The BPI compares the elevation of a focal point with the mean elevation of its neighboring cells that fall within a user-defined annulus. Positive BPI values represent locations that are higher than the average of their surroundings (positive reliefs), whereas negative BPI values indicate locations that are lower than their surroundings (negative reliefs).
BPI was used to identify low-relief features developed on a slope and trace their boundaries. Since bottom trawling causes erosion of the seabed, the morphologies generated from recurrent trawling can be defined by areas of negative BPI values relative to the surroundings. The joint analysis of bathymetry, backscatter and BPI data allowed us to establish the limits of these erosional forms, defined by BPI values of 0.1-0.2. The boundaries were automatically extracted and manually verified by means of cross profiles spaced 50 m apart for each dataset (2004 and 2017). No significant changes were detected between the two boundaries extracted in each dataset, with the exception of newly identified depressions. Therefore, a single boundary resulting from the intersection of the two limits was used to calculate volume changes. For each bathymetry, the top surface of the depression was created from the elevation of the feature boundary using natural neighbor interpolation. The depth (or incision) and volume of the features were calculated by subtracting the elevation of the feature from the top surface using raster differencing. The volume change between the two surveys was derived from the difference obtained by comparing the volumes calculated independently for each bathymetry.
4 Results
4.1 Fishing activity
Satellite-based navigation tracks from bottom trawlers operating in a study area over a 13-year period (2005-2017) provided valuable insights into long-term trends in the location and fishing pressure of the most recurrently visited fishing grounds (Figures 3A, S2). Several highly-impacted fishing grounds were observed on the continental shelf over the study period, particularly off Llançà, off the Cap de Creus Peninsula and in the Roses Bay. Off Llançà, a large (13 km long) fishing ground extends primarily along the 52-62 m depth range from Portbou to Cap de Creus, following the isobaths. Total fishing intensity computed for the period 2005-2017 displays the maximum values in this area (400-750 hauls/ha), particularly to the north of Llançà (Figure 3A). Additionally, other fishing grounds can be observed in the middle and outer shelf showing different orientations (N-S, NE-SW and NW-SE) but with lower fishing intensity (40-160 hauls/ha). Off Cap de Creus Peninsula, the most important fishing ground (160-325 hauls/ha) is located off and southward of the Cap de Creus headland, at 75-100 m water depth. It displays a curved path between the coastline and an elongated rocky outcrop that extends along 6 km at 95-115 m water depth. In Roses Bay, trawlers typically follow two main N-S paths that are parallel to the isobaths at 52-56 m and 67-69 m water depth, with fishing intensities of 240-290 hauls/ha (Figure 3A).
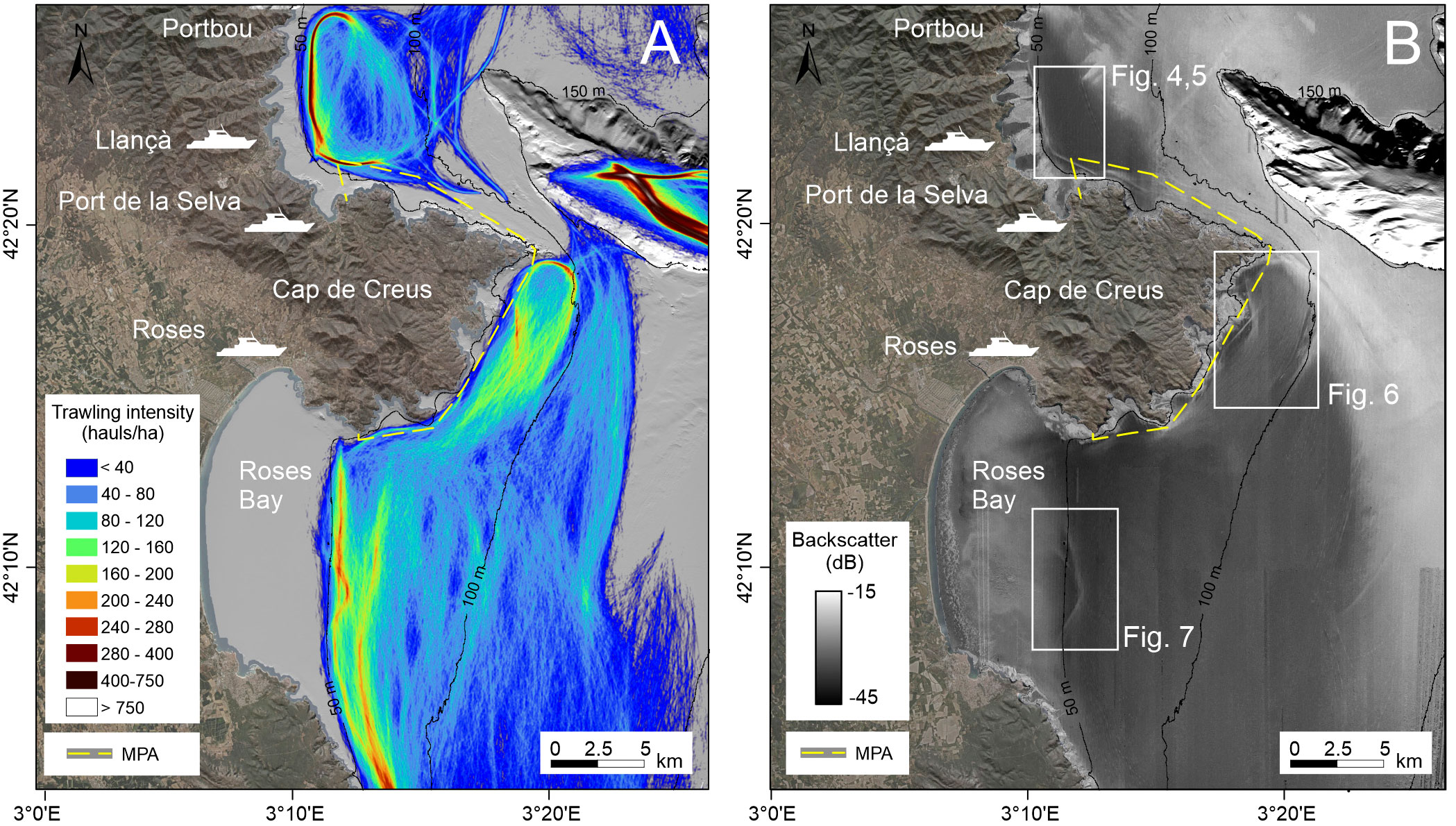
Figure 3 (A) Total fishing intensity for the period between 2005 and 2017, showing the location of the three main fishing harbors with trawlers operating in the study area (Port de La Selva, Llançà and Roses). (B) Backscatter image of 2004 (0.25 m grid size) of the continental shelf. MPA, Marine Protected Area of the Cap de Creus Natural Park. Topographic data and orthophoto from the Institut Cartogràfic i Geológic de Catalunya (www.icgc.cat). Locations of Figures 4–7 are also included.
4.2 Bathymetry and backscatter
The backscatter map reveals marked across- and along-shelf variations in reflectivity (Figure 3B). The inner shelf shows high backscatter values, particularly to the north and off the Cap de Creus Peninsula, with elongated patches of low backscatter that extend across-shelf from the shoreface down to 25-35 m off small coastal bays. Only in Roses Bay, the inner shelf is characterized by homogeneous medium backscatter. The middle shelf is dominated by low backscatter, particularly to the south of the Cap de Creus Peninsula, where a N–S oriented, 18-km wide low backscatter area extends southwards along the mid shelf, as well as to the north of the Cap de Creus Peninsula. Backscatter is high over the entire continental shelf between the Cap de Creus Peninsula and the Cap de Creus Canyon as well as along the outer shelf. The backscatter imagery also displays large, elongated areas of high backscatter off Llançà and Cap de Creus Peninsula, as well as in Roses Bay, which shows a similar pattern to that observed in the trawling activity (Figure 3).
4.2.1 Llançà shelf
In the continental shelf off Llançà, a narrow (180-220 m wide, locally up to 250 m) and discontinuous high backscatter area extends 12 km along the 43-52 m depth range in a NNW-SSE orientation and along the 52-62 depth range in a WNW-ESE orientation (Figures 3B, 4A). It covers an area of 1.6 km2 and shows very sharp boundaries from low to high backscatter intensities. This high backscatter region corresponds to a slightly depressed area in the bathymetric data (Figure 4B). BPI allows delineating this feature because of the concave profile of the depression (Figure 4C). The incision of the depression varies from 0.4 to 1.2 m (Figure 4D). The depression shows an asymmetric profile with a steep landward flank and a gentle offshore one (Figures 4E, F). The landward limit is well delineated in the gradient map by a marked increase in the mean seafloor gradient from 0.4°-0.6° to 2.2°, whereas the gradient of the seaward flank is significantly lower (>0.4). BPI values vary between -0.2 and -0.4 in the most depressed area of the trench and between 0.1 and 0.2 at the trench boundaries (Figures 4E, F).
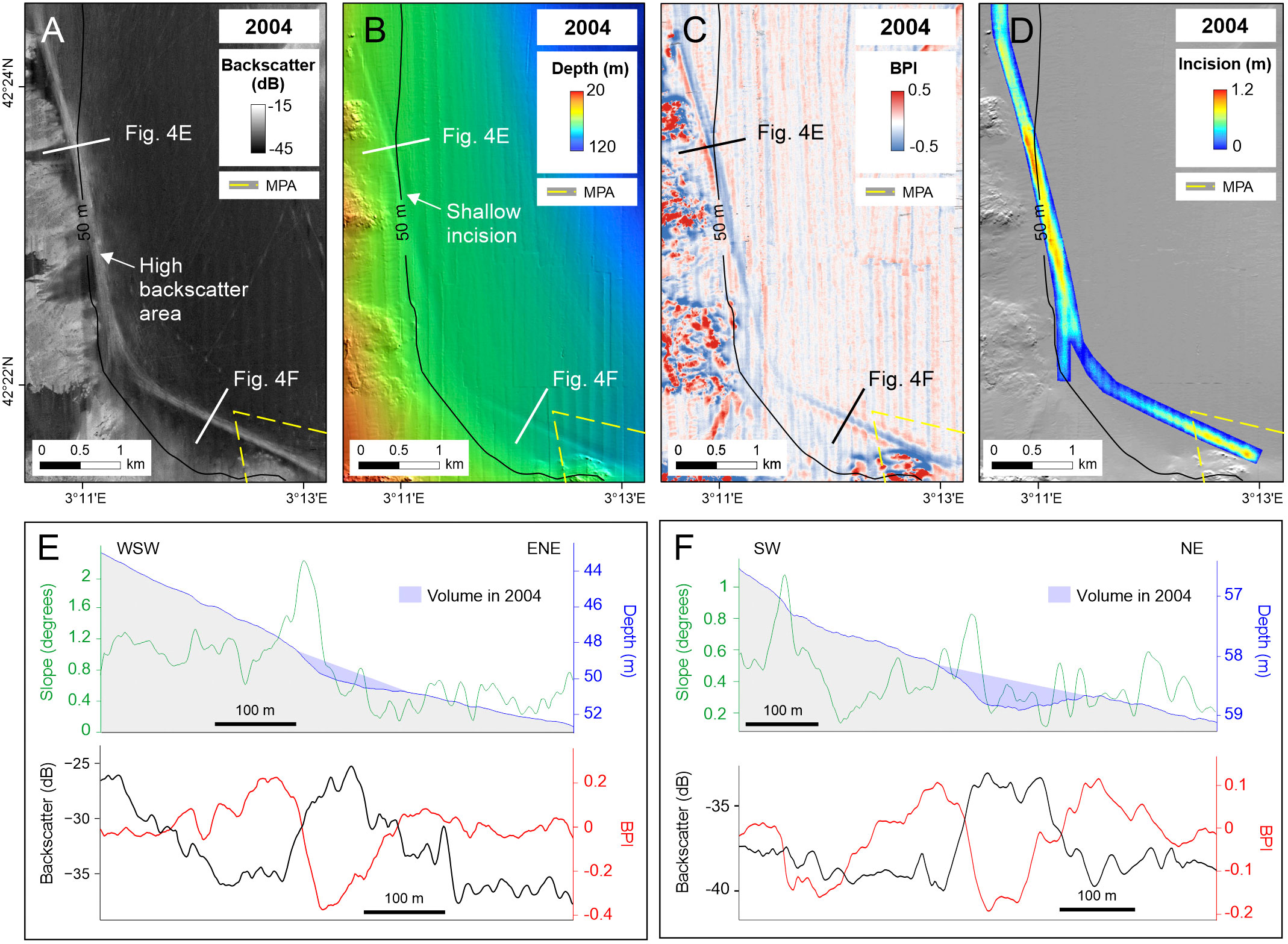
Figure 4 (A) Backscatter image, (B) multibeam bathymetry and (C) BPI maps of the Llançà inner shelf where a shallow depression was identified in the 2004 bathymetric dataset. (D) Shaded-relief map with superimposed trench incision. (E, F) across profiles at two locations along the shallow incision representing the topography, slope, BPI and backscatter values derived from the 2004 bathymetric dataset. MPA, Marine Protected Area of the Cap de Creus Natural Park.
The area occupied by the depression in 2004 was 1.4 km2, which represents an eroded volume of sediment of 582,933 m3 (Table 1). The surveyed area in 2017 does not cover the whole feature identified in 2004, but it allows the identification of a new incision, located slightly offshore of the incision mapped in 2004 (Figure 5). Although the morphological depression has a very subtle relief (0.2-0.3 m), it is well-displayed on the BPI data (Figures 5A, B). When considering the area covered in both surveys, a slight increase of about 1.18% of the volume of sediment eroded can be observed (Table 1). This loss of sediment is mainly related to the new incision mapped in 2017 and further excavation in the central and southernmost sectors of the depression (Figure 5C).
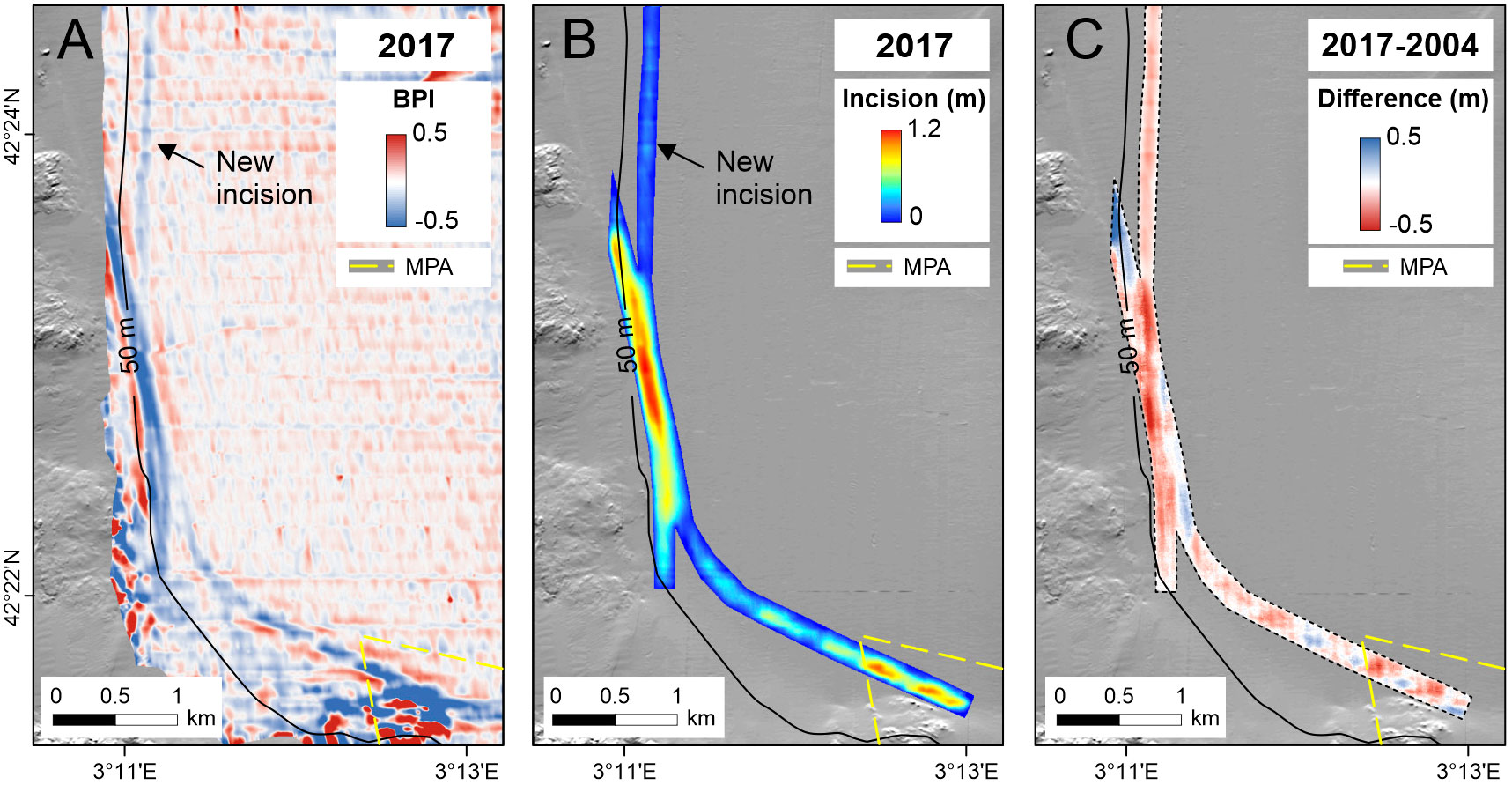
Figure 5 (A) BPI map of the Llançà inner shelf based on data collected in 2017. (B) Shaded-relief map with superimposed trench incision. (C) Difference in elevation between the 2004 and 2017 datasets. Note the formation of a new incision and local over-excavations along the depression. MPA, Marine Protected Area of the Cap de Creus Natural Park.
Offshore, on the middle shelf, other elongated areas of high backscatter and overlapped trawl marks are also observed in the backscatter imagery (Figure S3). The areas of high backscatter are 180-300 m wide and extend along 5-8 km, showing predominant N-S, NE-SW and NW-SE directions (Figure 3B). Some trawl marks show an orientation parallel to the bathymetry, converging towards the narrow continental shelf between the Cap de Creus Peninsula and the submarine canyon head, whereas other trawl marks appear crossing the continental shelf, and they can be traced over several kilometers (> 9 km). Unlike the areas of high backscatter identified on the inner shelf, these areas are more difficult to distinguish because of the smaller difference in backscatter between the morphological feature and the surrounding and because they do not show discernible relief in the bathymetric data.
4.2.2 Cap de Creus shelf
Off the Cap de Creus Peninsula, a narrow (70-160 m wide) area of high backscatter extends 5.6 km along the 70-84 m depth range displaying a N-S orientation and turning towards the SW (Figures 3B, 6A). As observed in the Llançà inner shelf, the area of high backscatter corresponds to a very subtle incision that is observed in the bathymetric data by the formation of an inflection point in the seabed slope caused by a local decrease in the gradient and negative BPI values delimiting the concave profile of the depression (Figures 6B, C). The resulting relief corresponds to an elongated (1.9 km) and narrow (60-120 m) incision that exhibits a discernible depression only in the central region (Figures 6D, E). This depression shows an asymmetric profile with the offshore flank gentler than the landward one (Figures 6F, G). Based on the 2004 dataset, the area covered by this depression is very small, less than 0.1 km, with a volume of 15,642 m3 (Figure 6D, Table 1). In 2017, the volume experienced a twofold increase owing to over-excavation, locally up to 0.8 m (Figures 6E–G, Table 1).
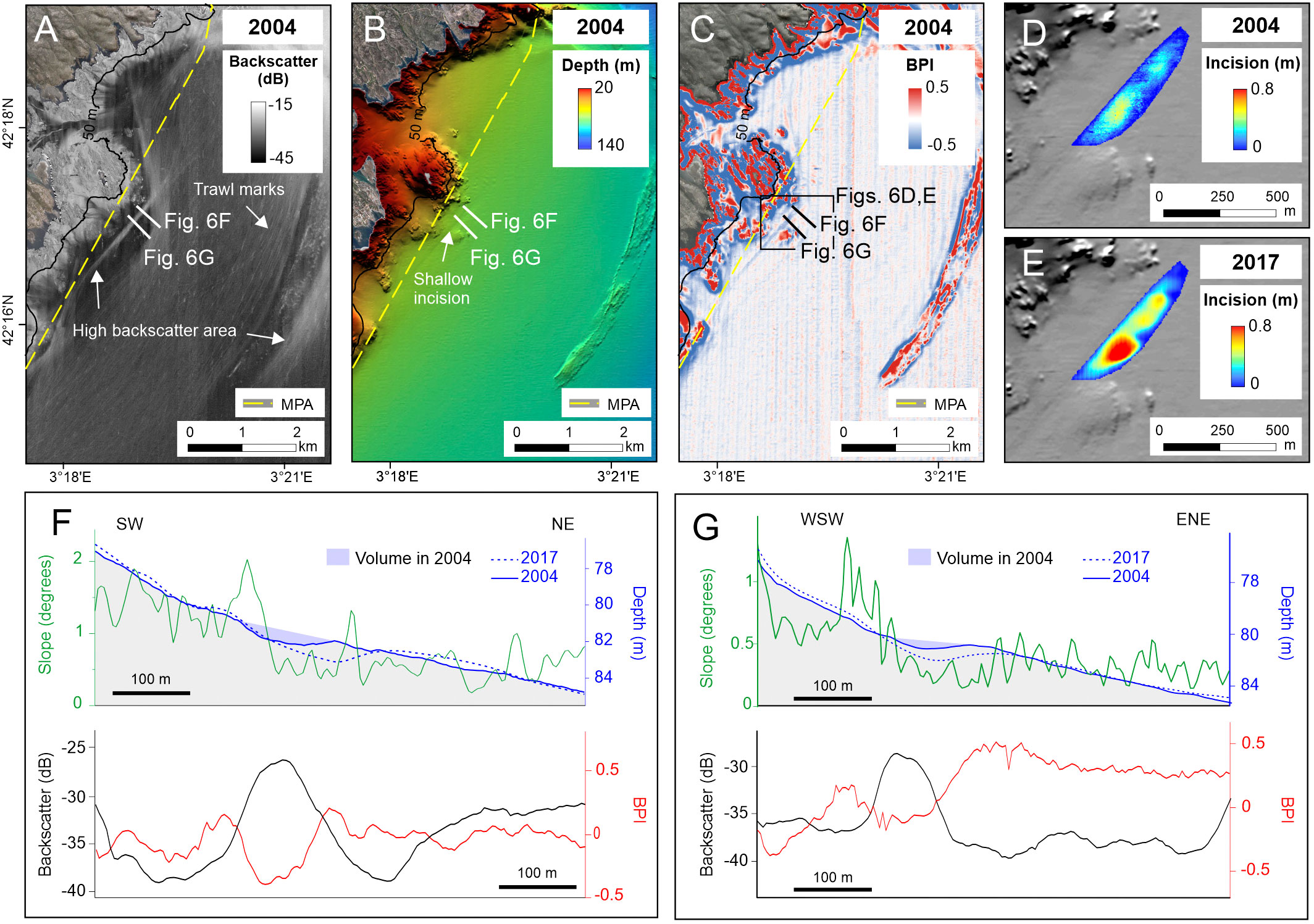
Figure 6 (A) Backscatter image, (B) multibeam bathymetry and (C) BPI maps of the Cap de Creus inner shelf where the shallow trench is located based on data collected in 2004. (D, E) Shaded-relief maps with superimposed trench incision in 2004 and 2017. (F, G) across profiles at two locations along the shallow incision representing the topography, slope, BPI and backscatter values derived from the 2004 bathymetric dataset. The topographic profiles obtained in 2017 are superimposed on those of 2004 to illustrate the main morphological changes. MPA: Marine Protected Area of the Cap de Creus Natural Park. Topographic data and orthophoto from the Institut Cartogràfic i Geológic de Catalunya (www.icgc.cat).
4.2.3 Roses shelf
Off the Roses Bay, another area of high backscatter was also observed at 50-60 m water depth showing a curve morphology with a predominant N-S orientation, parallel to the isobaths (Figures 3, 7). The area of high backscatter is 170-350 m wide and extends along 7 km. It shows a curve pattern that is less parallel to the 50-m isobath than in the previously described areas (Figure 7A). This area of high backscatter corresponds to a subtle depression in the bathymetric data, showing an alternation of positive and negative BPI values (Figures 7B, C), and an incision varying between 0.2 m and 0.6 m in the most curved area (Figure 7D). The incision is significantly shallower and wider than those observed off Llançà and Cap de Creus (Figures 7E, F). A volume of 68,780 m3 was estimated based on the 2004 dataset (Table 1). In 2017, the volume of the incision has been significantly reduced (62%) (Table 1).
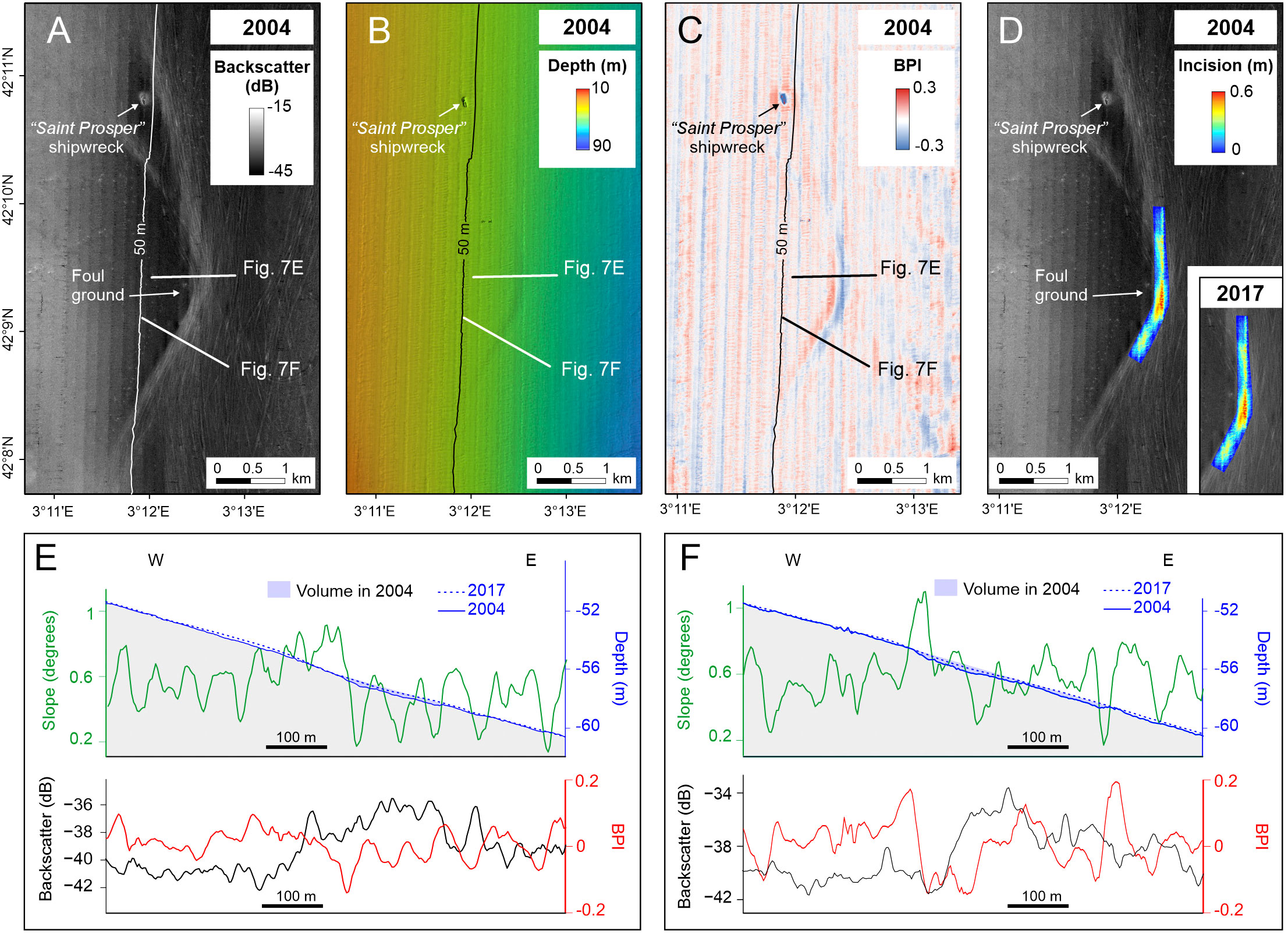
Figure 7 (A) Backscatter image, (B) multibeam bathymetry and (C) BPI maps of the Roses shelf where the shallow trench is located based on data collected in 2004. (D) Shaded-relief map with superimposed trench incision. (E, F) across profiles at two locations along the shallow incision representing the topography, slope, BPI and backscatter values derived from the 2004 bathymetric dataset. The topographic profile obtained in 2017 is superimposed on that of 2004 illustrating the infill of the depression.
4.3 Side scan sonar
Side scan sonar data acquired in an area of high backscatter of the Roses continental shelf (Figure 2) shows numerous trawl marks displayed as long, parallel to subparallel features with high reflectivity and different levels of visibility (Figure 8).
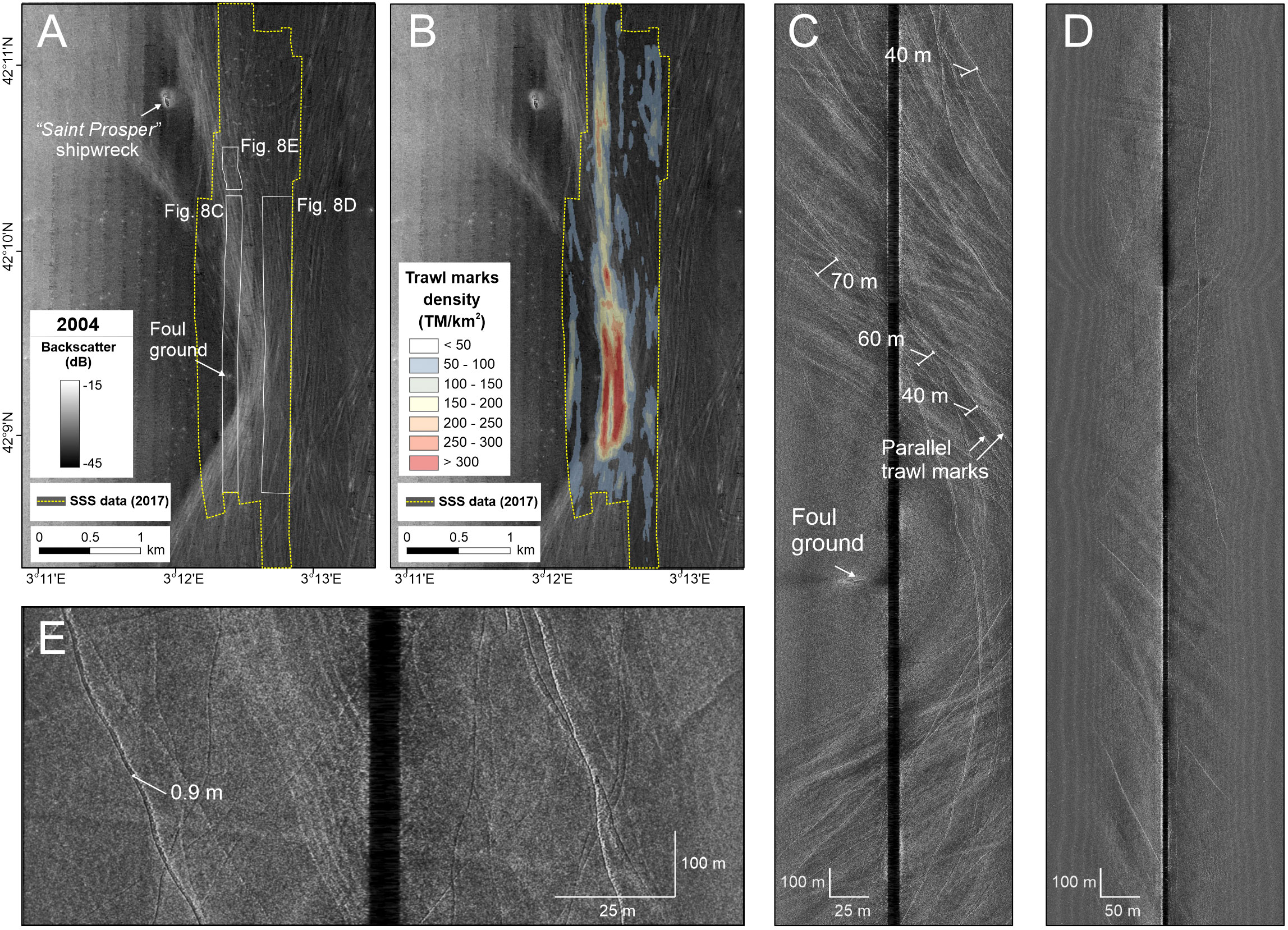
Figure 8 (A) Backscatter image (2004 dataset) showing the side scan sonar data coverage collected in 2017 and the location of (C–E). (B) Backscatter image (2004 dataset) with the density of trawl marks identified in 2017 superimposed. (C–E) side scan sonar images showing the morphology of the trawl marks.
The spatial quantification of trawl marks density, length and orientation reveals significant differences between the area of high backscatter and the adjacent shelf. The density of trawl marks (TM) in the area of high backscatter is much higher (320 TM/km2; Figures 8B, C) than in the surroundings (40-100 TM/km2; Figures 8B, D). TM in the area of high backscatter are longer (more than 8 km) and mostly show the same curvilinear morphology and N-S orientation than the high backscatter area identified in the backscatter imagery (Figure 8C). On the contrary, TM outside this area are more widely scattered and show different orientations, predominantly NE-SE (Figure 8D).
In the area of high backscatter, pairs of quasi- parallel tracks separated 40-70 m between them can be distinguished, attributed to the two doors of a given haul (Figure 8C). However, in the nearby low backscatter area, some trawl marks are hardly traceable and cannot be clearly distinguishable from the background, having a rather blurry appearance (Figure 8D). Detailed observations of the trawl scars show 1 m-wide marks corresponding to the size of the otter doors (Figure 8E). Shadows in these trawl marks indicate sediment removal at both sides of the scars produced by lateral push from the doors.
4.4 Sediments
A total of 6 sediment cores were collected off Llançà, inside and outside the area of high backscatter (Figure 9). The sediment cores retrieved in the area of high backscatter (between -29 and -24 dB) corresponding to the trawl-generated erosive feature display sediment coarsening in the upper layers (0-4 cm) (cores L3 and L4, Figure 9C). The sediment grain size of the upper layers consists mainly of silt and sand (28-62% of silt, 22-67% of sand and 5-13% of clay), coarsening upwards, whereas the lower layers are composed of silt and clay (60-79% of silt and 17-21% of clay), with a minor sand fraction (16-21% of sand). In contrast, the grain size of the sediment cores collected seaward of this area, in an area characterized by lower density of trawl marks and lower backscatter (-38 dB), is more homogeneous (i.e., cores L1 and L2, Figure 9). They are mostly composed of silt and clay (55-69% of silt and 9-13% of clay) with a low content of sand (18-36%) and with no differences along the sediment core. Sediment core L5, collected landwards of the area of high backscatter (Figure 9A), shows intermediate characteristics between the cores inside and outside the high backscatter region. It is mostly composed of silt and sand (51-70% silt and 16-40% of sand) coarsening upwards (Figure 9C). Sediment is finer (62-70% of silt and 16-26% of sand) in the lower layers (7-10 cm) of the sediment core compared to the upper layers (0-4 cm), where the sediment grain size consists of silt and sand (50-51% of silt and 37-40% of sand). Sediment core L6 was collected in the inner shelf, in an elongated area of moderate backscatter (-35 dB) that extends from the shoreline down to 40 m water depth, off the mouth of an ephemeral stream (Figure 9A). It displays a different textural pattern dominated by well-sorted fine sand (83-91% of sand and 0.23 mm mean grain size) with a very low content of finer fractions (7-15% of silt and 1.4-2.5% of clay).
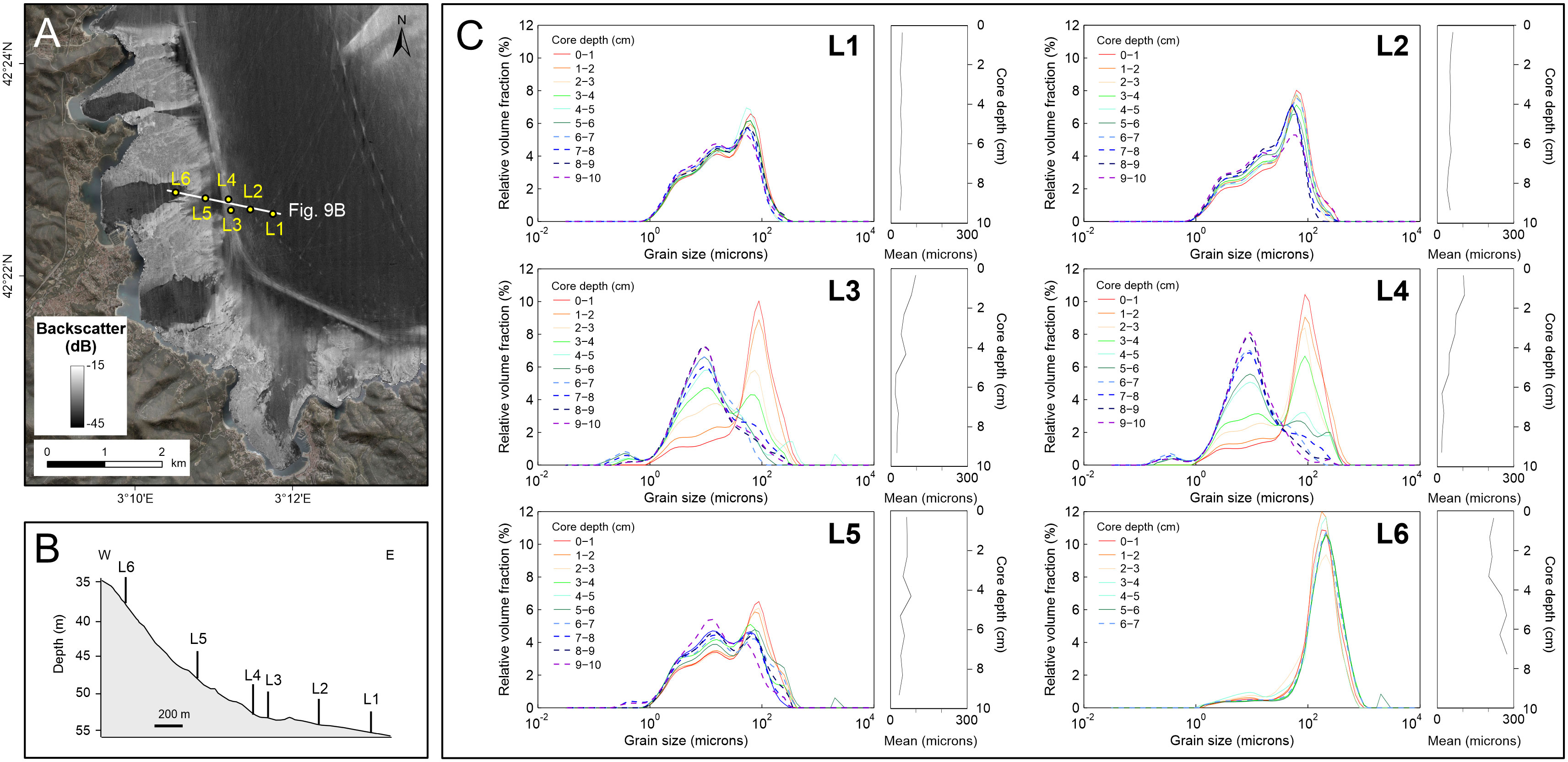
Figure 9 (A) Backscatter image (2004 dataset) showing the location of the bathymetric profile and the sediment cores collected in the continental shelf off Llançà. (B) Bathymetric profile with the location of the sediment cores retrieved on the erosive feature identified in the 2004 dataset (cores L3 and L4) and the sediment cores collected outside the trawled areas (cores L1, L2, L5 and L6). (C) Grain- size distribution of sediment samples. Note the sediment coarsening in the upper layers (0-4 cm) of the sediment cores located in the trawled areas.
A similar pattern is observed in the sediment cores collected along two sampled transects crossing the high reflectivity area in the Roses Bay (Figures 10, S4). Sediment cores R2 and R8, located in an area of moderate trawl marks density (Figure 10A) show slight coarsening trend upwards, more noticeable in the core R8 (Figure 10C). The sediment grain size of the upper layers of core R8 consists mainly of silt and sand (63-69% of silt and 18-26% of sand) with a low clay content (11-12%), whereas the lower layers are composed of silt and clay (67-73% of silt and 12-20% of clay), with a minor sand fraction (6-20% of sand). The sediment coarsening in the upper layers is more evident in the sediment cores retrieved in the area of high backscatter, corresponding to the highest density of trawl marks (i.e., cores R4 and R9, Figure 10). The sediment grain size of the upper layers (0-5 cm) consists mainly of silt and sand (61-62% of silt and 27-30% of sand) with low clay contents (9-12%), whereas the lower layers are composed of silt and clay (60-77% of silt and 11-22% of clay), with a minor sand fraction (1-28% of sand). Conversely, the sediment cores located in the area of low density (or even absence) of trawl marks (cores R5 and R11, Figure 10) are composed of homogeneous fine sediments. Sediment mostly consists of silt and sand (63-72% of silt and 17-29% of sand) with a low clay fraction (9-12%).
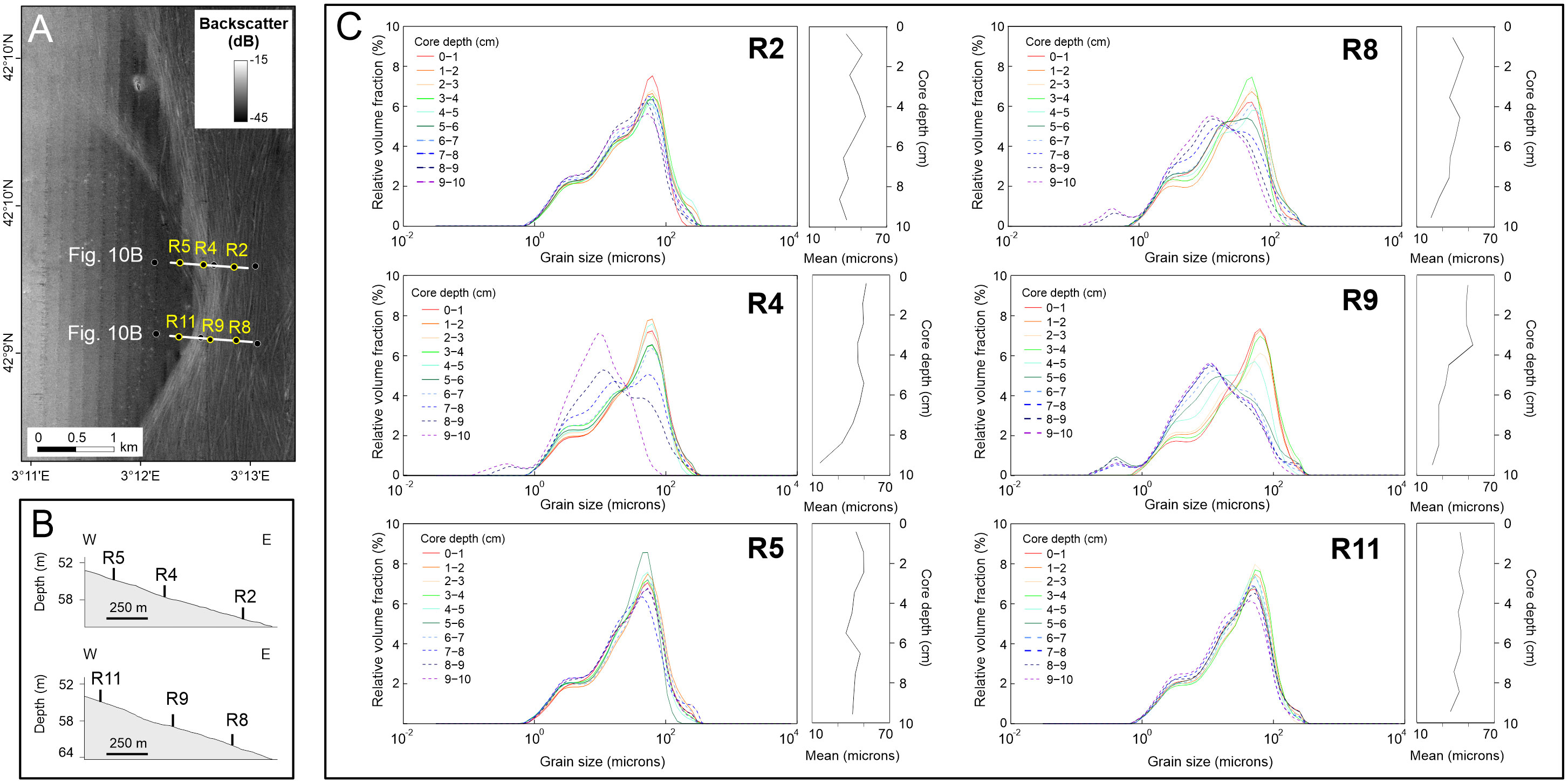
Figure 10 (A) Backscatter image (2004 dataset) showing the location of the bathymetric profile and six representative sediment cores collected in the Roses Bay. (B) Bathymetric profile showing the location of the sediment cores retrieved on areas characterized by different trawling intensity: low (cores R5 and R11), moderate (cores R2 and R8) and high (cores R4 and R9). (C) Grain-size distribution of sediment samples. Note the sediment coarsening in the upper layers (0-5 cm) of the sediment cores located in the trawled areas.
5 Discussion
5.1 Quantification of seafloor erosion by trawling activities
The physical impact of bottom trawling on the continental shelf seabed has been widely recognized by the identification and quantification of trawl marks generated by the otter boards (Friedlander et al., 1999; Smith et al., 2007; Buhl-Mortensen et al., 2016; Depestele et al., 2016; Bruns et al., 2020). In the northern Catalan margin, trawl marks appear widely distributed over the whole continental shelf, but concentrated in specific areas, as observed off Llançà and Cap de Creus, and the Roses Bay (Figures 8, S3). They are better recognized in muddy sediments, but they have also been observed in coarser sediments, even showing a decreased visibility, particularly around the Cap de Creus Peninsula.
Large-scale erosive morphological features have been also identified on the continental shelf as a result of recurrent trawling activities. They are characterized by regions with higher densities of trawl marks relative to the surrounding area (Figures 3B, S3) and exhibit a similar pattern to trawling activities, being localized in areas of increased fishing intensity (Figure 3). These morphologies are displayed on the backscatter data as narrow (less than 300 m wide) and elongated (up to 8 km long) areas of high backscatter relative to the surroundings. The increase of backscatter values in these areas can be mainly attributed to changes in the seabed topography caused by the high density of trawl marks and the reworking of the sediment due to the trawling gear. In the trawled areas, an upward-coarsening trend in the sediment is recorded in the sediment cores, produced by a reduction in the silt content of 14-50% in the first 4-5 cm of the core. Such trend is not observed in the sediment cores of the untrawled area, which are composed of fine sediments and show homogeneous vertical grain size distribution (Figures 9, 10). The coarsening of the uppermost layers suggests that part of the finer fraction resuspended by trawling gears is winnowed, increasing the sand content of the surface sediment. The fine sediment winnowed could be subsequently deposited on the bottom around the trawl marks, as observed in the Llobregat River prodelta (Palanques et al., 2001), or transported away from the trawled areas by dominant SW along-shelf currents. Slight increases in the grain size of surface sediments and upward coarsening trends caused by recurrent trawling have been observed in other fishing grounds: in the Llobregat River prodelta (Palanques et al., 2001), the Gulf of Lions (Ferré et al., 2008), the Ebro shelf (Palanques et al., 2014) or the Bay of Biscay (Mengual et al., 2016). The coarsening of the surficial sediments in the trawl-generated seafloor features in the Llançà and Roses continental shelf suggests ongoing trawling activities in the fishing grounds, also evidenced by VMS data (Figure S2).
The erosive features observed in the fishing grounds with low trawling intensity (140-160 hauls/ha over the study period), such as the fishing grounds of the middle and outer shelf off Llançà (Figure 3A), show no discernible relief in the bathymetric data but they are well displayed on backscatter data (Figures 3B, S3). However, the erosive features located in the fishing grounds with higher trawling intensity (240-750 hauls/ha during the study period) exhibits reliefs ranging from 0.2 to 1.2 m (Figures 4D, E). The shallower incision is in accordance with previous works that reported lithological and structural changes on the sediment column induced by recurrent bottom trawling down to 20 cm on the Ebro continental shelf (Palanques et al., 2014) and to roughly 35 cm on the NW Iberian shelf (Oberle et al., 2016b). The areas off Llançà with greatest incision (up to 1.2 m) correspond to areas of highest fishing intensity, up to 750 hauls/ha over the study period. These observations suggest that the repeated passage of the otter boards during successive fishing hauls of the trawling fleet operating in the same fishing ground can result in deeper excavations of the seafloor, compared to the individual tracks caused by the otter boards, inducing larger bottom trawling impacts than previously observed.
The volume of sediment eroded by recurrent trawling in the most intensively trawled areas varies from 0.11-0.18 m3/m2 in the fishing ground of the Roses Bay to 0.41 – 0.44 m3/m2 in the fishing grounds off Cap de Creus Peninsula and Llançà, respectively, based on the erosion observed in the seafloor features (Table 1). To assess the importance of fishing activities on the sediment dynamics of the continental shelf, these values were compared with the volume of sediment eroded by natural processes in other erosive features, such as lineations, small depressions and obstacle marks identified on the continental shelf between the Cap de Creus peninsula and the submarine canyon (Figures 1, S5). Lineations have lengths ranging from 0.7 to 2 km, with a negative relief between 0.2 and 1 m. Oval depressions are 120-150 m wide and up to 2 m deep. Obstacle marks are variable in length (100-400 m) and width (50-150 m) with reliefs of up to 2 m. The anthropogenically-derived trawl-generated features and the aforementioned natural features are products of distinct processes occurring in different types of sediments (muddy sediments in the fishing grounds and coarse sand and gravels in the bottom current-generated features). However, the results reveal comparable volumes of eroded sediment per unit area. It is estimated that the volume of eroded sediment ranges between 0.11 and 0.44 m3/m2 in the trawling-generated large-scale features, and between 0.10 and 1.22 m3/m2 (0.45 m3/m2 on average) in the bottom current-generated features. However, the area affected by trawling is significantly larger than the area occupied by individual bottom current-generated features (Figure S5).
Previous studies reported that suspended sediment fluxes induced by trawling erosion in muddy areas are comparable to natural-resuspension events by waves and currents (Durrieu de Madron et al., 2005; Ferré et al., 2008). These trawling-induced processes can become a dominant process controlling water turbidity during periods of high trawling intensity (Palanques et al., 2001; Palanques et al., 2014; Mengual et al., 2016). In the Bay of Biscay, in the French Atlantic continental shelf, trawling contribution to annual resuspension is in the order of 1% of the annual resuspension load, winter storms being the dominant processes. However, during the high fishing season, trawl resuspension can indeed be several times greater than natural resuspension in calm conditions (Mengual et al., 2016). In the Ebro delta, trawling introduces additional resuspended sediment that more than doubles the suspended sediment load of the bottom nepheloid layer on the middle shelf, suggesting that recurrent trawling can be able to change the modern sediment record in continental shelf environments (Palanques et al., 2014). The identification of large erosive features generated by recurrent bottom trawling on the continental shelf shows that repeated trawling over the same fishing ground can also lead to persistent changes on the seafloor morphology comparable to those caused by natural processes.
5.2 Changes in the fishing grounds evidenced by trawl-generated seafloor features
The characteristics and distribution of the erosive features generated by trawling activities can provide insights into trawling behavior and its potential cumulative impacts on the seafloor. In the northern Catalan continental shelf, the distribution of trawl marks and large-scale erosive features shows a good correspondence with the fishing grounds identified in the trawler’s tracking data during the period 2005-2017 (Figure 3). Off Llançà, most trawl marks and large-scale erosive features are concentrated along the 52-56 m isobaths, indicating that part of the trawling activities are conducted parallel to the isobaths (Figures 4A, S3). Large trawl marks also extend along the whole middle shelf converging towards the narrower shelf between the Cap de Creus Peninsula and the Cap de Creus submarine canyon head, further supporting trawlers’ preferential haul path parallel to the isobaths (Figure S3). Other trawl marks, however, appear crossing the middle shelf showing an orientation independent from water depth, probably related to the fishing practices (e.g. transits between the harbor and deeper areas).
The comparison of the 2004 and 2017 bathymetric datasets shows the bifurcation of two incisions (Figure 5C), as a result of the existence of two main fishing grounds located at different depths and presumably exploited in different periods. The NNW-SSE oriented incision off Llançà, mapped in 2004, turns continued northward along the 43-52 m depth range (Figure 4E), while the incision mapped in 2017 was located at deeper water depths (52-56 m) and shown a N-S orientation, running parallel to the 50 m isobath (Figure 5). This change in the fishing grounds could be attributed to the implementation of fishing regulations in the mid-1970s (Order of 30 July 1975, BOE-A-1975-17128), when bottom trawling in the Spanish Mediterranean continental shelves at depths less than 50 m was banned, and more precisely to the implementation of the VMS for fisheries monitoring in 2005. Prior to the fishing regulation, trawlers operated as close to shore as possible without risking the fishing gear due to the presence of shallow coastal rocky outcrops. These continued practices over decades explain the formation of a large trawl-generated erosive feature parallel to the coast along the edge of the coastal rocky outcrops, fishing at depths of less than 50 meters. The relief and visibility of this morphology in the backscatter data, coupled with the presence of trawl marks in the 2004 dataset, suggest that despite the implementation of regulations in the mid-1970s, trawlers continued operating in this coastal fishing ground.
The implementation of the VMS in 2005 resulted in the offshore displacement of the fishing activities, which led to a new fishing ground and area of erosion, as observed in the 2017 bathymetric dataset (Figure 5B). Although fishing intensity indicates activity in the new fishing ground as early as 2005, it was not until the year 2010 that fishing along this new ground intensified (Figures 11A, S2). The continuous erosion caused by fishing activities conducted over more than a decade produced an erosive feature with a sufficiently significant relief (0.2 m) to be measured with high-resolution multibeam systems (Figure 11C).
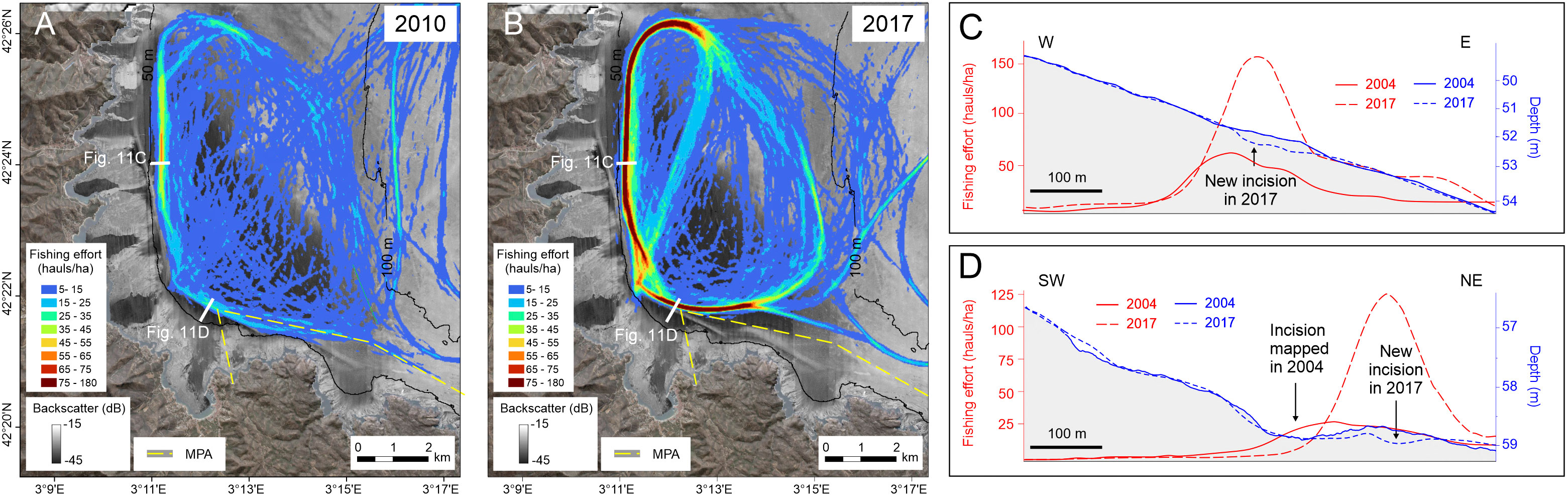
Figure 11 (A, B) Fishing intensity maps based on trawler’s tracking data in 2010 and 2017 superimposed to the backscatter image obtained in 2004. (C, D) Bathymetric profiles showing the erosion generated by repeated trawling over the same area in relation to the fishing effort. Note the formation of new depressions to the north of Llançà and to the north of Cap de Creus coinciding with the displacement of the fishing grounds, and the increase in the fishing activities in the continental shelf between 2010 (maximum fishing effort of 62 hauls/ha) and 2017 (up to 182 hauls/ha). MPA, Marine Protected Area of the Cap de Creus National Park.
To the north of Cap de Creus Peninsula, there is also a shift in location of the fishing grounds and the erosive morphologies caused by trawling. The ancient fishing ground that extended along the 52-56 isobaths following the northern limit of the MPA (Figure 11A), turned slightly northwards away from the coast in the more recent years (Figure 11B). This change in the fishing ground could also be related to the fishing regulations associated with the Cap de Creus Natural Park and its inclusion as MPA within the framework of the Natura 2000 network. Despite trawling was banned in 1998, when the MPA was established, previous works reported occasionally incursions of trawlers inside the MPA (Lloret and Riera, 2008). De Juan et al. (2013) and Demestre et al. (2015) also noted the presence of trawl marks on side scan sonar data collected in 2009, thus evidencing that periodic fishing activities were still occurring in 2009. These observations are supported by VMS data indicating fishing activity in this area up to 2010. In 2011, trawling activities shifted offshore the boundary of the MPA of the Cap de Creus Natural Park (Figure S2). As observed to the north of Llançà, this change in the trawling pattern is evidenced in the bathymetric data by the formation of another emerging incision in the new fishing ground (Figure 11D).
Off the Cap de Creus Peninsula, trawl marks show the same orientation as the trawlers track, following a direction parallel to the coast up to the Cap de Creus headland, where they then turn southwards, to continue parallel to the mid-shelf rocky outcrop (Figures 3, 6). Trawl marks observed in the multibeam echosounder data appear concentrated in the area of higher fishing activities, where the repeated passage of trawlers resulted in the formation of a slightly depressed area that became incised over time, reaching an incision of up to 0.8 m in 2007 (Figure 6E).
In the Bay of Roses, seafloor features and vessel tracking data show a similar pattern but covering a wider area than in Llançà and Cap de Creus. Overall, the fishing grounds extend along the bay, running parallel to the isobaths. Only in the central part of the bay, the trawling pattern draws a curve away from the 50 m isobath (Figure 3A). Bottom trawlers draw this curve to avoid two obstacles that can be challenging and risk potential gear loss or damage. These include a known shipwreck, the Saint Prosper shipwreck, a 106 m long oil tanker sunk in 1939, and a foul ground of unknown origin (Figure 7A). Erosion caused by recurrent trawling in this area (maximum incision of 0.6 m, Figure 7D) is not as pronounced as that observed off Llançà and Cap de Creus. This is likely associated to the fishing practices, as trawling activities in the Roses shelf are not concentrated in a single location, and instead trawlers spread out over a larger area, reducing the localized impact. Besides, natural sedimentation processes also play a crucial role in the recovery of the impacts by trawling, and the fishing grounds of the Roses Bay are likely directly affected by modern fluvial inputs from the Muga and Fluvià Rivers. Fine sediments delivered by these rivers and deposited in the middle shelf can help to fill any large-scale depressions and trawl marks caused by trawling, reducing their preservation.
5.3 Persistence of trawling impact on the seafloor morphology
The long-term morphological changes observed in the fishing grounds provide information on the persistence of the trawling impact on the seafloor morphology, and its potential recovery after cessation of activities. In the northern Catalan continental shelf, the three studied areas have shown very different morphological evolution over the study period.
The continental shelf off Llançà is the area most affected by trawling, with maximum incisions (up to 1.2 m) and volumes of sediment (> 500 000 m3) removed. In addition, the morphological evolution of this area over the study period shows increased erosion due to continued trawling in active fishing grounds. Volume variations in the shallow fishing ground (located at 43-52 m depth range) was not feasible due to the limited coverage of the 2017 dataset. However, the morphological changes observed in the small area mapped in both years suggest a moderate infilling of the depression (Figure 5C). North of Cap de Creus, however, the variations in the eroded sediment in the fishing ground that was abandoned in 2011 still do not show significant changes in 2017, seven years after its abandonment (Figures 5C, 11D), evidencing the slow recovery of the fishing ground several years after the cessation of the fishing activity.
The persistence of seafloor features caused by bottom trawling on the continental shelf has been intensively explored through morphological evolution of individual trawl marks. Their preservation is strongly influenced by multiple factors like water depth, sediment type, hydrodynamics (e.g., tidal currents, waves, storms) and trawling patterns. A minimum preservation time of 5–7 days was observed in coastal areas (Depestele et al., 2016; Bruns et al., 2020), whereas they can last for months or even a few years in rather offshore waters (Palanques et al., 2001; Gilkinson et al., 2015; Bruns et al., 2020). The penetration of the trawling gear into the seabed and the durability of the trawl marks was shown to be higher in muddy sediments compared to sandy sediments. Whereas scars caused by gears on sandy uncohesive sediments of energetic areas are covered by ripples in a few hours (BEON, 1990), trawling on muddy sediment can have longer-term effects on the seabed. Palanques et al. (2001) observed that trawl marks in muddy sediments on the Ebro Shelf (20–70 m water depth) did not show any changes after a few days but after a year they appeared with lower backscatter values. They related the relative longevity to the cohesive properties of the muddy sediment (mud content >60%). Gilkinson et al. (2015) also examined the effects of experimental clam dredging on the seabed at greater depths (65–75 m water depth) on the Scotian shelf, Canada, observing trawl marks were still visible in side scan sonar data after three years, although showing a blurry appearance. As observed in the Ebro shelf, storms were the main factor in reworking the sediments and, therefore, in flattening the trawl marks (Palanques et al., 2001; Gilkinson et al., 2015).
The long-lasting effects of trawling in the seafloor morphology in the northern Catalan continental shelf could be mainly explained by the intensity and frequency of trawling activities, sediment inputs and local hydrodynamics. The reduced impact of bottom trawling in Roses Bay compared to Llançà and Cap de Creus could be explained by the local fishing practices and natural riverine sedimentary inputs. As previously mentioned, the annual evolution of fishing activity shows a variable distribution of the vessel tracks in Roses Bay over time, minimizing the impact on a specific area (Figure S2). Conversely, the higher frequency and intensity of fishing activities in Llançà and Cap de Creus fishing grounds hinder the capacity of the seafloor to recover between trawling events. The recovery of trawl-generated features in these areas could be favored by stronger local hydrodynamics. However, despite the strong hydrodynamics of the north Catalan continental shelf, as evidenced by large-scale current-erosive features (Lo Iacono et al., 2012; Durán et al., 2014), the main fishing grounds are located in the more sheltered areas, dominated by weak currents and limited sedimentation (Durán et al., 2014), thus contributing to the persistence of trawling impacts. Only off the Cap de Creus, stronger hydrodynamics could compensate the impact of trawling, favoring its recovery. This would explain that despite being a long fishing ground, the trawling impact is concentrated only in the area of maximum fishing intensity. However, as long as fishing activities continue in these areas, the affected seafloor area will increase and become deeper, resulting in larger and long-lasting effects on the seafloor, with their subsequent impact on benthic habitats.
The penetration depth of otter trawl doors has been associated to adverse impacts on benthic habitats. Bottom trawling impacts have been shown to change benthic species composition when disturbed by gear having a significant larger impact with increasing penetration depth (Hiddink et al., 2017; Sciberras et al., 2018). In terms of recovery, benthic community abundance does not recover within 3 years when impacted by gears with penetration depth of ≥16 cm (Sciberras et al., 2018). The seabed excavation produced by recurrent bottom trawling on the north Catalan continental shelf (up to 1.2 m), deeper than the penetration depth of most benthic trawling equipment, suggests longer-lasting effects in such benthic habitats.
6 Concluding remarks
The combined analysis of high-resolution multibeam echosounder and side scan sonar data, sediment grain size and vessel tracking data in the northern Catalan continental shelf (NW Mediterranean) has provided new insights into the long-lasting impact of bottom trawling on the seafloor.
The observations demonstrate that the repeated passage of the otter boards during successive fishing hauls of the trawling fleet operating in the same fishing ground can result in deep excavation of the seafloor, generating large-scale erosive features with sufficient relief to be measured using different morphometric techniques applied to high-resolution multibeam data. In the north Catalan continental shelf, these erosive features can be up to 300 m wide and 8 km long, with a variable relief ranging from 0.2 m to 1.2 m, and maximum values in the areas of increased trawling intensity, corresponding to the main fishing grounds.
The upward-coarsening trend observed in the surficial sediments (down to 4-5 cm) of trawled areas suggests ongoing trawling activities in the fishing grounds, contributing to the maintenance of the trawl-generated large-scale erosive features.
The quantification of the volume of sediment eroded by trawling suggests that recurrent trawling over the same fishing ground during decades may cause changes in seafloor morphology comparable to those generated by natural processes. In the Catalan continental shelf, the volume of sediment eroded by trawling varies from 0.11 to 0.43 m3/m2 and is comparable to sediment volumes eroded in bottom-current-derived seafloor features identified in the study area (between 0.10 and 1.22 m3/m2, 0.45 m3/m2 on average), although the impact of trawling affects larger areas.
The distribution of trawl-generated features shows a similar pattern to that observed in the fishing intensity, and can therefore be used as indicators of fishing grounds in the absence of vessel tracking data. Furthermore, they provide information on the location of the main fishing grounds and the temporal and spatial variations of the trawling effort, such as those derived from fishing regulations. For instance, the distribution and density of trawl marks and large-scale erosional features on the Catalan continental shelf indicate that most trawling activities are conducted parallel to the isobaths, and to a lesser extent crossing the continental shelf, probably related to fishing practices such as transits between port and deeper areas. The shift between the location of fishing grounds and the erosional morphologies caused by trawling also evidences changes in fishing regulations, such as new closed areas.
The persistence of the large-scale trawl-generated erosive features on the north Catalan continental shelf is largely influenced by the trawling intensity and recurrence, limiting the ability of the seafloor to recover between trawling events. The comparison of the large-scale erosive features over a 13-year period also showed extended recovery periods following the cessation of activities, with tracks persisting longer in muddy areas.
Data availability statement
The original contributions presented in the study are included in the article/Supplementary Material. Further inquiries can be directed to the corresponding author.
Author contributions
RD: Conceptualization, Formal Analysis, Funding acquisition, Investigation, Methodology, Visualization, Writing – original draft. PP: Conceptualization, Funding acquisition, Investigation, Writing – review & editing. SP: Data curation, Investigation, Methodology, Writing – review & editing. JG: Investigation, Writing – review & editing. AP: Investigation, Writing – review & editing. CL: Investigation, Writing – review & editing. MA-C: Data curation, Writing – review & editing. AMu: Methodology, Resources, Writing – review & editing. AMi: Investigation, Methodology, Writing – review & editing.
Funding
The author(s) declare financial support was received for the research, authorship, and/or publication of this article. This research was supported by the project ABIDES (CTM2015-65142-R), the Marie Sklodowska-Curie grant agreement no. 867471 (TrawledSeas Project), and the Grant 2021 SGR 663 provided by the Generalitat de Catalunya. A.M. was supported by the David and Lucile Packard Foundation. This work is contributing to the ICM ‘Center of Excellence’ Severo Ochoa (CEX2019-000928-S).
Acknowledgments
The authors thank the Secretariat General of the Sea (SGP) of the Spanish Ministry of Agriculture, Fisheries and Food and TRAGSA for the 2004 Espace Project bathymetric dataset, the General and GEOMYTSA for the 2017 bathymetric dataset, and the Fishing Monitoring Centre of the Spanish Secretariat of Maritime Fishing (SEGEMAR) for the satellite-based tracking Vessel Monitoring Systems (VMS).
Conflict of interest
The authors declare that the research was conducted in the absence of any commercial or financial relationships that could be construed as a potential conflict of interest.
Publisher’s note
All claims expressed in this article are solely those of the authors and do not necessarily represent those of their affiliated organizations, or those of the publisher, the editors and the reviewers. Any product that may be evaluated in this article, or claim that may be made by its manufacturer, is not guaranteed or endorsed by the publisher.
Supplementary material
The Supplementary Material for this article can be found online at: https://www.frontiersin.org/articles/10.3389/fmars.2023.1270485/full#supplementary-material
References
Arnau P., Liquete C., Canals M. (2004). River mouth plume events and their dispersal in the northwestern Mediterranean Sea. Oceanography 17, 22–31. doi: 10.5670/oceanog.2004.27
BEON (1990). Effects of beamtrawl fishery on the bottom fauna of the North Sea. Beleidsgericht Ecol. Onderzoek Noordzee. BEON Report No. 8, 58pp.
Black K. P., Parry G. D. (1994). Sediment transport rates and sediment disturbance due to scallop dredging in Port Phillip Bay. Mem. Queensl. Mus. 36, 327–341.
Bruns I., Holler P., Capperucci R. M., Papenmeier S., Bartholomä A. (2020). Identifying trawl marks in North Sea sediments. Geosciences 10, 422. doi: 10.3390/geosciences10110422
Buhl-Mortensen L., Aglen A., Breen M., Buhl-Mortensen P., Ervik A., Husa V., et al. (2013). Impacts of fisheries and aquaculture on sediments and benthic fauna: suggestions for new management approaches. Fisken og Havet 2, 69.
Buhl-Mortensen P., Buhl-Mortensen L. (2018). Impacts of bottom trawling and litter on the seabed in Norwegian waters. Front. Mar. Sci. 5. doi: 10.3389/fmars.2018.00042
Buhl-Mortensen L., Ellingsen K. E., Buhl-Mortensen P., Skaar K. L., Gonzalez-Mirelis G. (2016). Trawling disturbance on megabenthos and sediment in the Barents Sea: chronic effects on density, diversity, and composition. ICES J. 73, i98–i114. doi: 10.1093/icesjms/fsv200
Canals M., Casamor J. L., Urgeles R., Farrán M., Calafat A. M., Amblás D., et al. (2004). Mapa del relleu submarí de Catalunya (Seafloor relief map of Catalonia) (Barcelona, Spain: Institut Cartogràfic de Catalunya). 1:250 000.
Churchill J. H. (1989). The effect of commercial trawling on sediment resuspension and transport over the Middle Atlantic Bight continental shelf. Cont. Shelf Res. 9, 841–864. doi: 10.1016/0278-4343(89)90016-2
Collier J. S., Brown C. J. (2005). Correlation of side scan backscatter with grain size distribution of surficial seabed sediments. Mar. Geol. 214, 431–449. doi: 10.1016/j.margeo.2004.11.011
De Juan S., Lo Iacono C., Demestre M. (2013). Benthic habitat characterisation of soft-bottom continental shelves: Integration of acoustic surveys, benthic samples and trawling disturbance intensity. Estuar. Coast. Shelf Sci. 117, 199–209. doi: 10.1016/j.ecss.2012.11.012
Demestre M., Muntadas A., de Juan S., Mitilineou C., Sartor P., Mas J., et al. (2015). The need for fine-scale assessment of trawl fishing effort to inform on an ecosystem approach to fisheries: Exploring three data sources in Mediterranean trawling grounds. Mar. Policy 62, 134–143. doi: 10.1016/j.marpol.2015.09.012
Depestele J., Ivanović A., Degrendele K., Esmaeili M., Polet H., Roche M., et al. (2016). Measuring and assessing the physical impact of beam trawling. ICES J. Mar. Sci. 73, i15–i26. doi: 10.1093/icesjms/fsv056
DGPC (Direcció General de Protecció Civil) (1986). Investigación tecnológica de las acciones a tomar para la estabilidad de las playas del Maresme (Barcelona, Spain: Generalitat de Catalunya). 318 pp.
Durán R., Canals M., Sanz J. L., Lastras G., Amblas D., Micallef A. (2014). Morphology and sediment dynamics of the northern Catalan continental shelf, northwestern Mediterranean Sea. Geomorphology 204, 1–20. doi: 10.1016/j.geomorph.2012.10.004
Durán R., Guillén J., Muñoz A. (2017). Sorted Bedforms Developed on Sandy Deposits Derived from Small Ephemeral Streams (Catalan Continental Shelf) - Atlas of Bedforms in the Western Mediterranean. Eds. Guillén J., Acosta J., Chiocci F. L., Palanques A. (Cham: Springer International Publishing), 127–133. doi: 10.1007/978-3-319-33940-5_21
Durrieu de Madron X., Ferré B., Le Corre G., Grenz C., Conan P., Pujo-Pay M., et al. (2005). Trawling-induced resuspension and dispersal of muddy sediments and dissolved elements in the Gulf of Lion (NW Mediterranean). Cont. Shelf Res. 25, 2387–2409. doi: 10.1016/j.csr.2005.08.002
Durrieu de Madron X., Nyffeler F., Godet C. H. (1990). Hydrographic structure and nepheloid spatial distribution in the Gulf of Lions continental margin. Cont. Shelf Res. 10, 915–929. doi: 10.1016/0278-4343(90)90067-V
Eigaard O. R., Bastardie F., Breen M., Dinesen G. E., Hintzen N. T., Laffargue P., et al. (2016). Estimating seabed pressure from demersal trawls, seines, and dredges based on gear design and dimensions. ICES J. Mar. Sci. 73, i27–i43. doi: 10.1093/icesjms/fsv099
Eigaard O. R., Bastardie F., Hintzen N. T., Buhl-Mortensen L., Buhl-Mortensen P., Catarino R., et al. (2017). The footprint of bottom trawling in European waters: distribution, intensity, and seabed integrity. ICES J. Mar. Sci. 74, 847–865. doi: 10.1093/icesjms/fsw194
Ercilla G., Farran M., Alonso B., Diaz J. I. (1994). Pleistocene progradational growth pattern of the northern Catalonia continental shelf (northwestern Mediterranean). Geo-Marine Lett. 14, 264–271. doi: 10.1007/BF01274062
Ferré B., Durrieu de Madron X., Estournel C., Ulses C., Le Corre G. (2008). Impact of natural (waves and currents) and anthropogenic (trawl) resuspension on the export of particulate matter to the open ocean: Application to the Gulf of Lion (NW Mediterranean). Cont. Shelf Res. 28, 2071–2091. doi: 10.1016/j.csr.2008.02.002
Font J., García-Ladona E., Gorriz E. G. (1995). The seasonality of mesoscale motion in the Northern Current of the western Mediterranean: several years of evidence. Oceanol. Acta 18, 207–219.
Friedlander A. M., Boehlert G. W., Field M. E., Mason J. E., Gardner J. V., Dartnell P. (1999). Side scan-sonar mapping of benthic trawl marks on the shelf and slope off Eureka, California. Fish. Bull. 97, 786–801.
Gaida T. C., Snellen M., van Dijk T. A. G. P., Simons D. G. (2019). Geostatistical modelling of multibeam backscatter for full-coverage seabed sediment maps. Hydrobiologia 845, 55–79. doi: 10.1007/s10750-018-3751-4
García-García A., Schoolmeester T., Orange D., Calafat A., Fabres J., Grossman E., et al. (2012). “Recent Sedimentary Processes in the Cap de Creus Canyon Head andAdjacent Continental Shelf, NE SPAIN: Evidence from Multibeam Bathymetry, Sub-Bottom Profiles and Coring,”Sediments, Morphology and Sedimentary Processes on Continental Shelves: Advances in technologies, research and applications. Eds. M.Z. Li, C.R. Sherwood and P.R. Hill. Int. Assoc. Sedimentol. Spec. Public. 44, 71-98. doi: 10.1002/9781118311172.ch4
Gilkinson K., King E. L., Li M. Z., Roddick D., Kenchington E., Han G. (2015). Processes of physical change to the seabed and bivalve recruitment over a 10-year period following experimental hydraulic clam dredging on Banquereau, Scotian Shelf. Cont. Shelf Res. 92, 72–86. doi: 10.1016/j.csr.2014.11.006
Gómez J., Espino M., Puigdefabregas J., Jerez F. (2005). Xarxa d’Instrumentació Oceanogràfica i Meteorologica de la Generalitat de Catalunya (XIOM), Boies d’onatge dades obtingudes l’any 2004 (Barcelona: Technical Report of Generalitat de Catalunya).
Halpern B. S., Walbridge S., Selkoe K. A., Kappel C. V., Micheli F., D’Agrosa C., et al. (2008). A global map of human impact on marine ecosystems. Sci. (New York N.Y.) 319 (5865), 948–952. doi: 10.1126/science.1149345
Hiddink J. G., Jennings S., Sciberras M., Szostek C. L., Hughes K. M., Ellis N., et al. (2017). Global analysis of depletion and recovery of seabed biota after bottom trawling disturbance. Proc. Natl. Acad. Sci. 114, 8301–8306. doi: 10.1073/pnas.1618858114
Humborstad O.-B., Nøttestad L., Løkkeborg S., Rapp H. T. (2004). RoxAnn bottom classification system, side scan sonar and video-sledge: spatial resolution and their use in assessing trawling impacts. ICES J. Mar. Sci. 61, 53–63. doi: 10.1016/j.icesjms.2003.10.001
ITGE (1994). Mapa Geológico de la Plataforma Continental Española y Zonas Adyacentes 1:200 000. Hoja 25/25E (Figureueres) (Madrid, Spain: Instituto Tecnológico GeoMinero de España). 82 pp.
Jones J. B. (1992). Environmental impact of trawling on the seabed: A review. New Zeal. J. Mar. Freshw. Res. 26, 59–67. doi: 10.1080/00288330.1992.9516500
Kroodsma D. A., Mayorga J., Hochberg T., Miller N. A., Boerder K., Ferretti F., et al. (2018). Tracking the global footprint of fisheries. Science 359 (6378), 904–908. doi: 10.1126/science.aao5646
Krost P., Bernhard M., Werner F., Hukriede W. (1990). Otter trawl tracks in Kiel Bay (Western Baltic) mapped by side-scan sonar. Meeresforschung 32, 10.
Lastras G., Canals M., Amblas D., Lavoie C., Church I., De Mol B., et al. (2011). Understanding sediment dynamics of two large submarine valleys from seafloor data: Blanes and La Fonera canyons, northwestern Mediterranean Sea. Mar. Geol. 280, 20–39. doi: 10.1016/j.margeo.2010.11.005
Lloret J., Riera V. (2008). Evolution of a Mediterranean coastal zone: human impacts on the marine environment of Cape Creus. Environ. Manage. 42, 977–988. doi: 10.1007/s00267-008-9196-1
Lo Iacono C., Orejas C., Gori A., Gili J. M., Requena S., Puig P., et al. (2012). “Habitats of the Cap de Creus Continental Shelf and Cap de Creus Canyon, Northwestern Mediterranean,” in GeoHAB Atlas of Seafloor Geomorphic Features and Benthic Habitats. Eds. Harris P. T., Baker E. K. (Elsevier Insigths), 457–469. doi: 10.1016/B978-0-12-385140-6.00032-3
Lucchetti A., Sala A. (2012). Impact and performance of Mediterranean fishing gear by side-scan sonar technology. Can. J. Fish. Aquat. Sci. 69, 1806–1816. doi: 10.1139/f2012-107
Malik M. A., Mayer L. A. (2007). Investigation of seabed fishing impacts on benthic structure using multi-beam sonar, side scan sonar, and video. ICES J. Mar. Sci. 64, 1053–1065. doi: 10.1093/icesjms/fsm056
Martín J., Puig P., Masqué P., Palanques A., Sánchez-Gómez A. (2014). Impact of Bottom Trawling on Deep-Sea Sediment Properties along the Flanks of a Submarine Canyon. PloS One 9 (8), e104536. doi: 10.1371/journal.pone.0104536
Mengual B., Cayocca F., Le Hir P., Draye R., Laffargue P., Vincent B., et al. (2016). Influence of bottom trawling on sediment resuspension in the ‘Grande-Vasière’ area (Bay of Biscay, France). Ocean Dyn. 66, 1181–1207. doi: 10.1016/S0924-7963(98)00078-5
Millot C. (1999). Circulation in the Western Mediterranean Sea. J. Mar. Syst. 20, 423–442. doi: 10.1016/S0924-7963(98)00078-5
MOPU (MINISTERIO DE OBRAS PÚBLICAS) (1979). Estudio de la dinámica litoral en la costa Peninsular y Onubense, Madrid, Laboratorio de Puertos Ramón Iribarren (Madrid. Spain).
Oberle F. K. J., Puig P., Martín J. (2018). “Fishing Activities,” in Submarine Geomorphology. Eds. Micallef A., Krastel S., Savini A. (Switzerland: Springer, Cham), 503–534.
Oberle F. K. J., Storlazzi C. D., Hanebuth T. J. J. (2016a). What a drag: Quantifying the global impact of chronic bottom trawling on continental shelf sediment. J. Mar. Syst. 159, 109–119. doi: 10.1016/j.jmarsys.2015.12.007
Oberle F. K. J., Swarzenski P. W., Reddy C. M., Nelson R. K., Baasch B., Hanebuth T. J. J. (2016b). Deciphering the lithological consequences of bottom trawling to sedimentary habitats on the shelf. J. Mar. Syst. 159, 120–131. doi: 10.1016/j.jmarsys.2015.12.008
O’Neill F. G., Ivanović A. (2016). The physical impact of towed demersal fishing gears on soft sediments. ICES J. Mar. Sci. 73, i5–i14. doi: 10.1093/icesjms/fsv125
Palanques A., Guillén J., Puig P. (2001). Impact of bottom trawling on water turbidity and muddy sediment of an unfished continental shelf. Limnol. Oceanogr. 46, 1100–1110. doi: 10.4319/lo.2001.46.5.1100
Palanques A., Puig P., Guillén J., Demestre M., Martín J. (2014). Effects of bottom trawling on the Ebro continental shelf sedimentary system (NW Mediterranean). Cont. Shelf Res. 72, 83–98. doi: 10.1016/j.csr.2013.10.008
Paradis S., Goñi M., Masqué P., Durán R., Arjona-Camas M., Palanques A., et al. (2021). Persistence of biogeochemical alterations of deep-sea sediments by bottom trawling. Geophys. Res. Lett. 48, e2020GL091279. doi: 10.1029/2020GL091279
Pilskaln C. H., Churchill J. H., Mayer L. M. (1998). Resuspension of sediment by bottom trawling in the gulf of maine and potential geochemical consequences. Conserv. Biol. 12, 1223–1229. doi: 10.1046/j.1523-1739.1998.0120061223.x
Puig P., Canals M., Company J. B., Martín J., Amblas D., Lastras G., et al. (2012). Ploughing the deep sea floor. Nature 489, 286–289. doi: 10.1038/nature11410
Russo T., Parisi A., Garofalo G., Gristina M., Cataudella S., Fiorentino F. (2014). SMART: A Spatially Explicit Bio-Economic Model for Assessing and Managing Demersal Fisheries, with an Application to Italian Trawlers in the Strait of Sicily, edited by: MacKenzie, B. R. PloS One 9, e86222. doi: 10.1371/journal.pone.0086222
Sanchez-Vidal A., Canals M., Calafat A. M., Lastras G., Pedrosa-Pàmies R., Menéndez M., et al. (2012). Impacts on the deep-sea ecosystem by a severe coastal storm. PloS One 7 (1), e30395. doi: 10.1371/journal.pone.0030395
Schwinghamer P., Gordon D. C., Rowell T. W., Prena J., McKeown D. L., Sonnichsen G., et al. (1998). Effects of experimental otter trawling on surficial sediment properties of a sandy-bottom ecosystem on the grand banks of newfoundland. Conserv. Biol. 12, 1215–1222. doi: 10.1046/j.1523-1739.1998.0120061215.x
Sciberras M., Hiddink J. G., Jennings S., Szostek C. L., Hughes K. M., Kneafsey B., et al. (2018). Response of benthic fauna to experimental bottom fishing: A global meta-analysis. Fish Fish. 19, 698–715. doi: 10.1111/faf.12283
Smith C. J., Banks A. C., Papadopoulou K.-N. (2007). Improving the quantitative estimation of trawling impacts from side scan-sonar and underwater-video imagery. ICES J. Mar. Sci. 64, 1692–1701. doi: 10.1093/icesjms/fsm165
Smith C. J., Papadopoulou K. N., Diliberto S. (2000). Impact of otter trawling on an eastern Mediterranean commercial trawl fishing ground. ICES J. Mar. Sci. 57, 1340–1351. doi: 10.1006/jmsc.2000.0927
Watling L., Norse E. A. (1998). Disturbance of the seabed by mobile fishing gear: A comparison to forest clearcutting. Conserv. Biol. 12, 1180–1197. doi: 10.1046/j.1523-1739.1998.0120061180.x
Keywords: submarine geomorphology, trawl marks, multibeam, backscatter, side scan sonar, sediments, fishing grounds, seafloor erosion
Citation: Durán R, Puig P, Paradis S, Guillén J, Palanques A, Lo Iacono C, Arjona-Camas M, Muñoz A and Micallef A (2023) Long-term morphological and sedimentological changes caused by bottom trawling on the northern Catalan continental shelf (NW Mediterranean). Front. Mar. Sci. 10:1270485. doi: 10.3389/fmars.2023.1270485
Received: 31 July 2023; Accepted: 30 October 2023;
Published: 16 November 2023.
Edited by:
Mariacristina Prampolini, National Research Council (CNR), ItalyReviewed by:
Sally Joan Watson, National Institute of Water and Atmospheric Research (NIWA), New ZealandZhi Huang, Geoscience Australia, Australia
Thaiënne Van Dijk, Deltares, Netherlands
Copyright © 2023 Durán, Puig, Paradis, Guillén, Palanques, Lo Iacono, Arjona-Camas, Muñoz and Micallef. This is an open-access article distributed under the terms of the Creative Commons Attribution License (CC BY). The use, distribution or reproduction in other forums is permitted, provided the original author(s) and the copyright owner(s) are credited and that the original publication in this journal is cited, in accordance with accepted academic practice. No use, distribution or reproduction is permitted which does not comply with these terms.
*Correspondence: Ruth Durán, cmR1cmFuQGljbS5jc2ljLmVz