- 1Marine Science Program, Biological and Environmental Science and Engineering Division, King Abdullah University of Science and Technology (KAUST), Thuwal, Saudi Arabia
- 2Red Sea Research Center (RSRC), King Abdullah University of Science and Technology (KAUST), Thuwal, Saudi Arabia
- 3Computational Bioscience Research Center (CBRC), King Abdullah University of Science and Technology, Thuwal, Saudi Arabia
- 4National Center for Wildlife, Riyadh, Saudi Arabia
- 5OceanX, New York, NY, United States
- 6NEOM Nature Reserve, NEOM, Tabuk, Saudi Arabia
- 7The Centre of Tropical Water and Aquatic Ecosystem Research (TropWATER), James Cook University, Townsville, QLD, Australia
Understanding the vertical distribution of elasmobranch species and associated ecological dynamics can be a crucial component of developing effective conservation strategies, particularly in light of their global population decline. Previous studies have primarily focused on horizontal extent and movement patterns of elasmobranchs, with limited knowledge about their vertical distribution. This knowledge gap stems from limited access to technological advancements and reliance on surface data from fisheries operations. Today, advancements in observing platforms such as electronic tracking, remotely operated vehicles and submersibles, and reductions in costs for drop cameras and BRUVs, allow for direct observation of animals at great depths, facilitating improved understanding of their ecological and trophic niches. This study reports new global depth records for three elasmobranch species observed in the Saudi Arabian Red Sea (Carcharhinus altimus, Rhinobatos punctifer, Iago omanensis), also presenting ethological evidence on Iago omanensis feeding behavior. Our findings have significant implications for conservation strategies and the development of targeted conservation measures. The provisioning of data on new depth ranges allows places like NEOM to better manage and protect deep sea habitats, due to the presence of species occurring at those depths.
Introduction
Elasmobranchs have declined worldwide due to overexploitation and unregulated fishing (Baum et al., 2003; Clarke et al., 2006; Ferretti et al., 2008; Lack and Sant, 2011). In particular, Red Sea elasmobranch populations are depleted and at high risk of local extirpation for many species (Dulvy et al., 2014; Spaet et al., 2016). The lack of information on elasmobranch size, behavior, distribution, and ecology additionally hampers conservation and management efforts. This problem is exacerbated by limited data on catches and landings, especially for some species due to misidentification (Duffy and Struthers, 2017; Ayas et al., 2020).
Accurate identification of sharks in the wild is challenging, particularly as most identification comes from landings at fish markets, where some of the diagnostic characteristics, such as bright colour and solid shape, may be altered or lost (Cliff and Thurman, 1984; Smith, 1992; Smith et al., 2004; Barragán-Méndez et al., 2018). In addition, direct observations of elasmobranchs in their natural habitats are generally scarce, particularly for those at significant depth, which limits available data below ranges easily accessible by humans (i.e., 60m). Today, the use of relatively new observational techniques (e.g., electronic tagging, baited cameras, remotely operated vehicles (ROVs), submersibles and drop cameras) provides opportunities to observe these species in their natural environment and is expanding our knowledge on their vertical distribution. These three-dimensional data provide valuable insights into elasmobranch habitat use and species interactions (Gallagher et al., 2017; Andrzejaczek et al., 2022). This information can also underpin effective management plans addressing the possible threats to which these animals are exposed (Coelho et al., 2015; Gilman et al., 2020; Murua et al., 2021). The decline of elasmobranch populations in the Eastern Red Sea has been attributed to high fishing pressure (Dulvy et al., 2014; Spaet et al., 2016). While bans on shark fishing are in place along with regulations to manage fishing activities in the region, there is no regulation on the vertical dimensions of fisheries (e.g., depth constrains on fishing). Saudi Arabia’s fisheries sector applies various fishing techniques, including artisanal methods like handline, gillnet, trolling, and traps, as well as industrial practices such as trawlers, purse seines, and shrimp fisheries. Additionally, recreational and subsistence fisheries are also significant (Tesfamichael and Pauly, 2016; Tesfamichael and Pitcher, 2006). The design of effective conservation plans requires information on critical habitats and depth extents of different species, as fisheries targeting different layers of the water column have distinct impacts on elasmobranchs (Baco et al., 2016; Goetze et al., 2011; Huvenne et al., 2016).
Here we report observations on occurrence of three elasmobranch species, the bignose shark (Carcharhinus altimus), the spotted guitarfish (Rhinobatos punctifer) and the bigeye houndshark (Iago omanensis) at previously unrecorded depths in the eastern Red Sea, extending their global depth range (Figure 1).
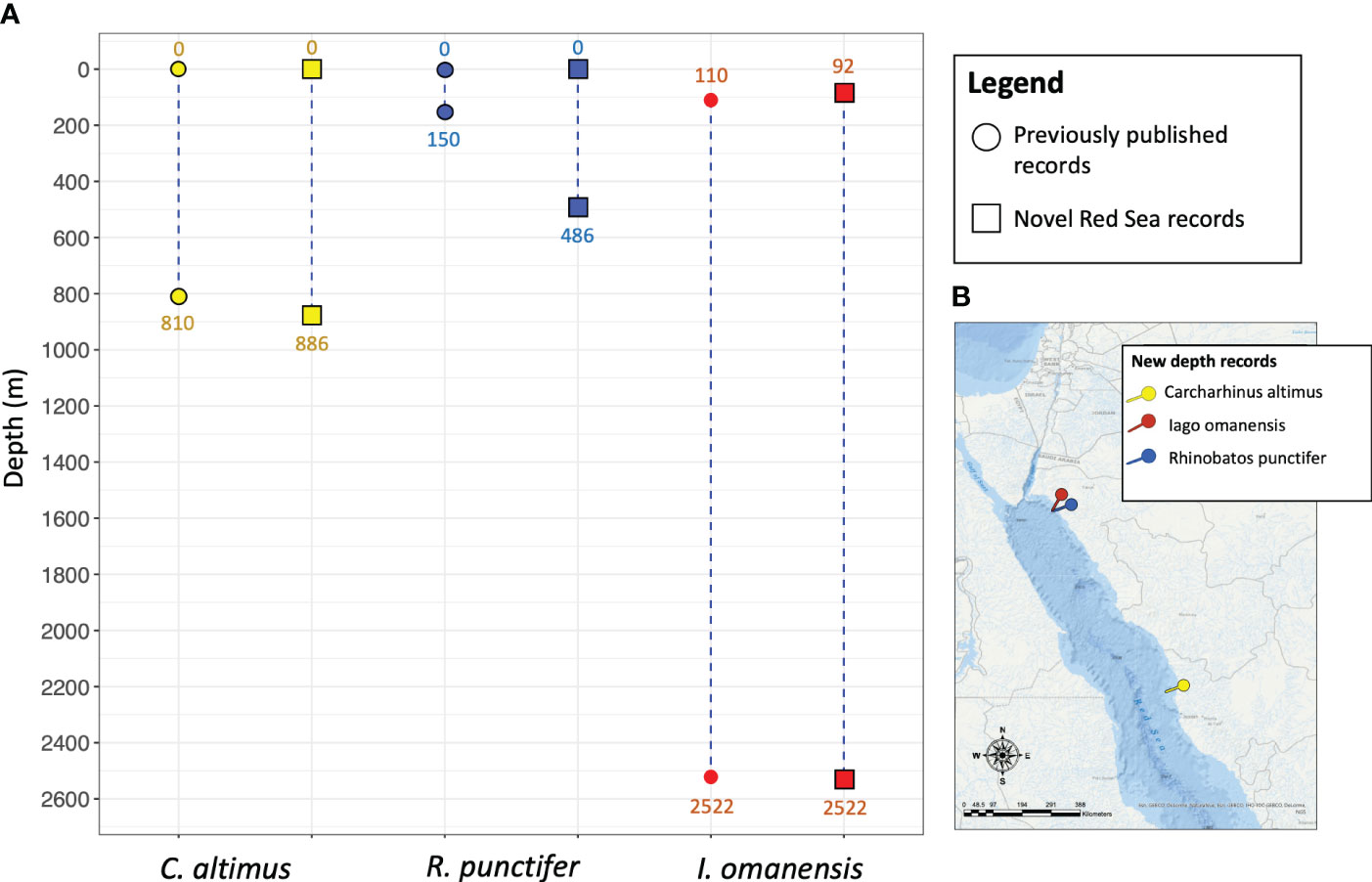
Figure 1 (A) Depth range of Carcharhinus altimus, Rhinobatos punctifer and Iago omanensis as reported in previously published literature in comparison with new depth records from the Red Sea. On the map on the lower right (B), the coordinates of the new record sightings are displayed in 3 different colors corresponding to each species (yellow: C. altimus, blue: R. punctifer, red: I. omanensis).
Materials and methods
Two separate research cruises, the Red Sea Deep Blue Expedition (RSDBE) from October-November 2020 and the Red Sea Decade Expedition (RSDE) from February-June 2022, were conducted aboard the OceanXplorer in the eastern Red Sea (Saudi Arabia). One Remotely Operated Vehicle (ROV: Argus Mariner XL model) and 2 submersibles (Triton 3300 2MKII) undertook repeated dives for various scientific purposes. During each dive, every opportunistic elasmobranch observation was recorded, noting the date and time of observations, depth using the onboard sensors, and corresponding photographs and video footage. The ROV was fitted with a HDTV 1080p F/Z Colour camera, a Imenco Night Shark Lowlight BW camera, a DSPL Super wide-angle CCD camera, a Imenco Silvertip CCD camera with LED, a Imenco Greytip CCD utility camera, four Gitup F1, 90°C lens in 6,000 m housings and a Mini Cam Arctic Rays EagleRay 4K. The submersibles were fitted with 2 benthic cameras (Red DSMC2 Monstro 8k), Wide Angle, Macro camera (Nikon 70-180mm f/4.5-5.6 AF-D), a low-light camera (Canon ME20F-SH 1080P) and a Mini Cam (Arctic Rays EagleRay 4K). Both the submersibles and the ROV were equipped with lasers that could be activated on demand, to measure objects up to 10 cm in length in front of the vehicle, and we used these measurements to extrapolate the relative total lengths (TL) of elasmobranchs individuals (i.e., distance from the tip of snout to tip of the upper caudal lobe).
Out of the total 171 ROV and Submersible dives, we performed 8 baited dives (using bonitos and sardines as bait).
Fishbase (http://www.fishbase.us), IUCN Red List assessments (Ebert et al., 2017; Rigby et al., 2020; Dulvy et al., 2021) and a recent synthesis of elasmobranch vertical habitat use (Pearce et al., 2023) were primarily referenced to establish current depth limits for C. altimus, R. punctifer, and I. omanensis (Figure 1). These resources compiled datasets from multiple additional publications for the species of interest, which warrant mention themselves. C. altimus depth records present in literature (0 – 810 m) come from the review of literature and museum collections (Ebert et al., 2013; Weigmann, 2016) and the checklist of the Hawaiian archipelago fishes (Mundy, 2005). However, the depth range of C. altimus cited in this book refers to a study carried out in Northern Australia through the use of longlines (Stevens, 1991). Depth records of R. punctifer (0 – 150 m) were largely derived from Weigmann (2016) which conducted an extensive literature review including museum collections. Relevant data was also obtained from Goldshmidt et al. (1996), which describes samples collected in the aphotic zone of the Gulf of Aqaba. I. omanensis depth data (110 - 2,522 m) are based on literature/museum collection reviews (Weigmann, 2016) and conference proceedings (Barnes, 2007). There is only a mention of the possibility of a depth extension to 2,195m, referring to a photographic survey carried out in the deep-sea area of the Eastern Red Sea (Marshall and Bourne, 1964). More recently a new depth record for I. omanensis appeared in literature (Pearce et al., 2023), however it has not yet been taken into account by Fishbase or IUCN.
The total effort from the RSDE was 43 submersible and 128 ROV dives, for a total of 913 hours of underwater survey, covering an area going from the Farasan banks to the Gulf of Aqaba. For the submersible dives we took into account only Neptune, the scientific operational submersible, as the other submersible, Nadir, was always budding with the aforementioned one, and dedicated to media operations. For the specifics on the survey effort of the RSDBE, refer to Garzon et al. (2022).
Results
Here we present the new extended depth records for the aforementioned 3 species of elasmobranchs in comparison with previous data reported in literature (Figure 1).
Carcharhinus altimus
In this study, we present a new depth record for Carcharhinus altimus in the eastern Red Sea (Figure 1). On March 31, 2022, one male C. altimus was observed at 886 meters during an ROV dive (Figure 2) (22.16512N, 38.70729E). From the analysis of photographs and videos of this animal coupled with laser measurements, its size was estimated at 1.90 m TL. In this section we also provide images gathered during RSDE ROV and submersible dives, that support the accurate identification of this species and can be used as basis for future studies (Figure 3, Supplementary Material Videos 1-3).
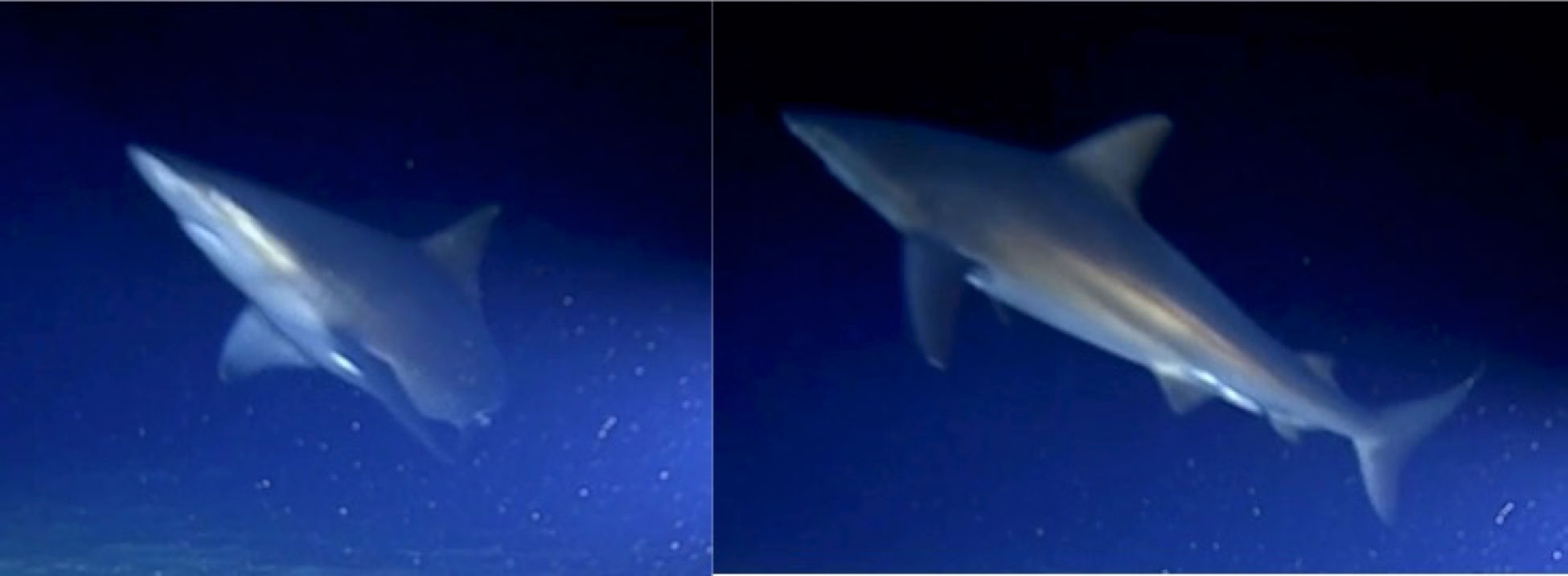
Figure 2 Adult male individual of C. altimus recorded at 886 m depth, a new depth record for this species. Footage captured during an ROV dive on 21st Feb 2022, daytime. The estimated size of this individual is 1.90 m TL.
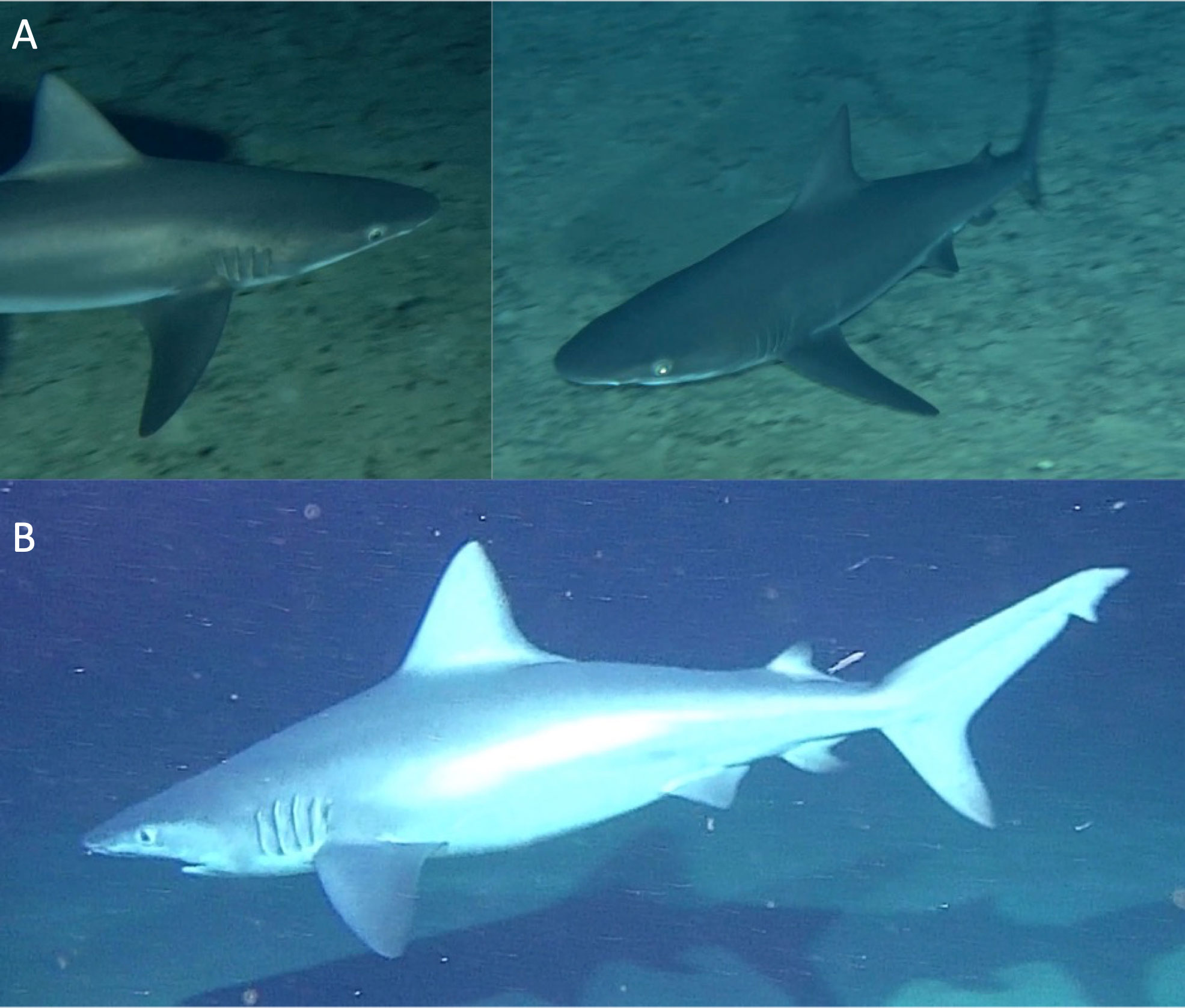
Figure 3 (A) Adult male individual of C. altimus (1.80 m TL). Footage captured during a submersible dive on 15th May 2022, daytime at a depth of 188 m. (B) Adult female of C. altimus (1.70 m TL). Footage captured during the ROV dive on 11Th March 2022, daytime at a depth of 515 m.
Rhinobatos punctifer
Through ROV and submersible dives in the eastern Red Sea, we gathered two direct observations which were both below the maximum reported depth record of R. punctifer: one observation at 318 m from the RSDE (22.48269N, 38.86660E) (Supplementary Material Video 4) and another at 486 m from the RSDBE (27.59992N, 35.33483E) (Figure 4; previously published without depth information in Garzon et al., 2022). These observations in the Red Sea support a much wider depth niche for this species, in contrast with the more neritic habitat of many other guitarfish known in other areas of the ocean (Figure 1) (e.g., Ebert and Stehmann, 2013).
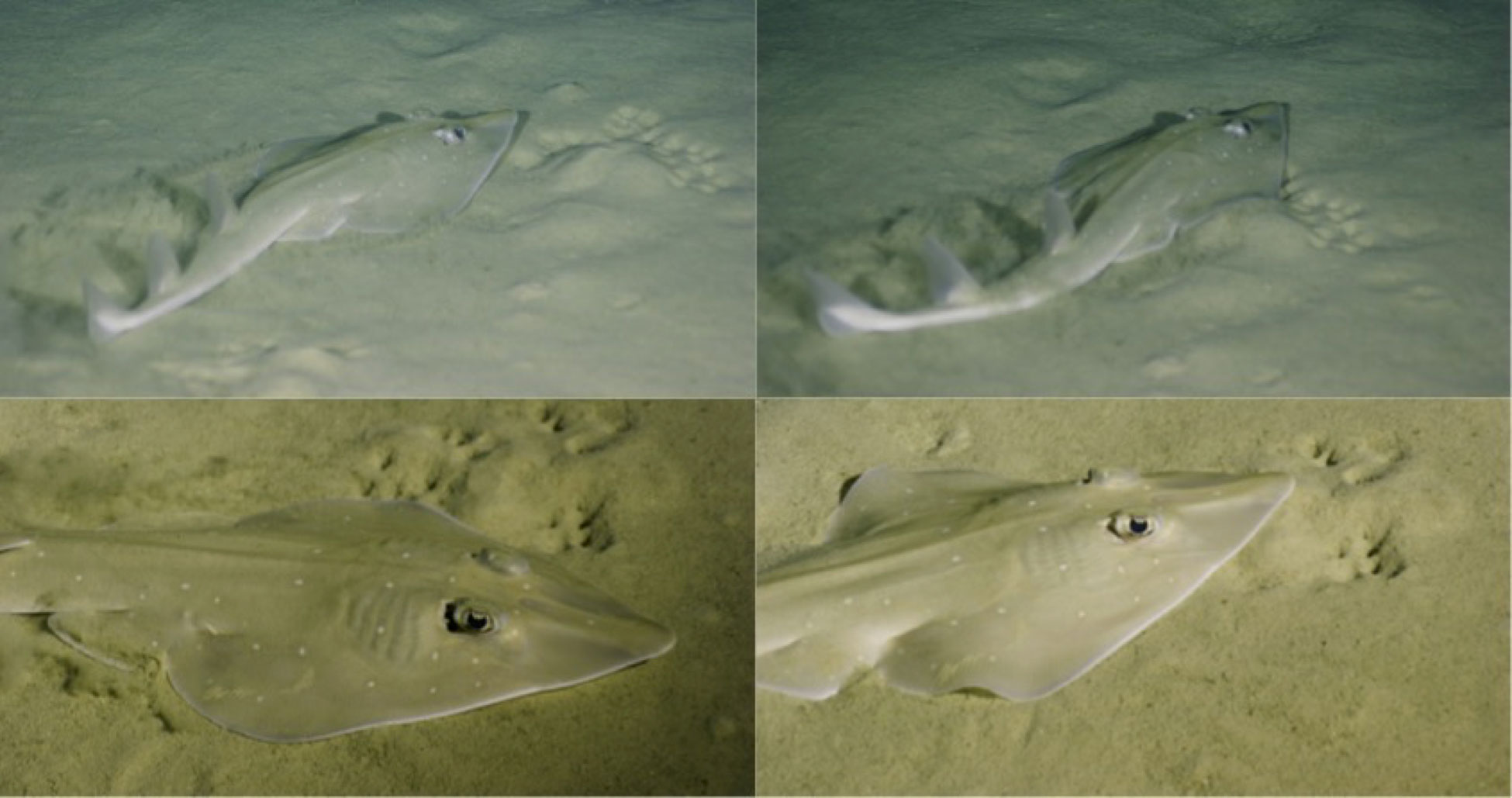
Figure 4 Imagery of an adult R. punctifer individual captured during a submersible dive on 10th October 2020, at a depth of 486 m. The characteristics white spots on the dorsal surface of the body, together with the body shape and position of the fins, are clear identification features.
Iago omanensis
We present a new range extension for Iago omanensis. Our recent findings during a 2020 cruise in the Northern Red Sea (previously published without depth records in Garzon et al., 2022) reveal a new upper limit record of 92 m for this species (Figure 1) (7.59441N, 35.30155E). Furthermore, the RSDE provided us with an opportunity to observe the behavior of I. omanensis in the wild, including feeding and potential interactions with other sharks.
The feeding behavior of I. omanensis has been inferred previously only by indirect methods, as direct observations of this species in the wild are almost inexistent. Here, we provide footage of two possibly different feeding strategies, recorded during ROV and submersible dives. The first two videos (Supplementary Material Videos 5, 6) provide evidence of a suction feeding behavior, typically used to target prey buried in the sand. Other records included lateral head shaking to cut pieces of bait that were deployed to attract sharks (baited dive, Supplementary Material Videos 7-9). During an ROV dive, we also recorded a possible species interaction in which a small I. omanensis was followed immediately after by a C. altimus individual (Supplementary Material Video 10).
In the various footage sequences from RSDE ROV and submersible dives, multiple images for different individuals of I. omanensis were obtained, some of which supported robust identification (Figure 5). We found evident differences in the colors of the pectoral and caudal fin margins, presenting clear white edges in some of the animals recorded (Figure 6). The quality of the footage was often suboptimal due to poor lighting at the depth and distance from our vehicles to the sharks. In one of the recordings the individual is small in size (~30 cm), however other individuals with average in size around 50 cm, also carried the same markings (Supplementary Material Videos 11-14).
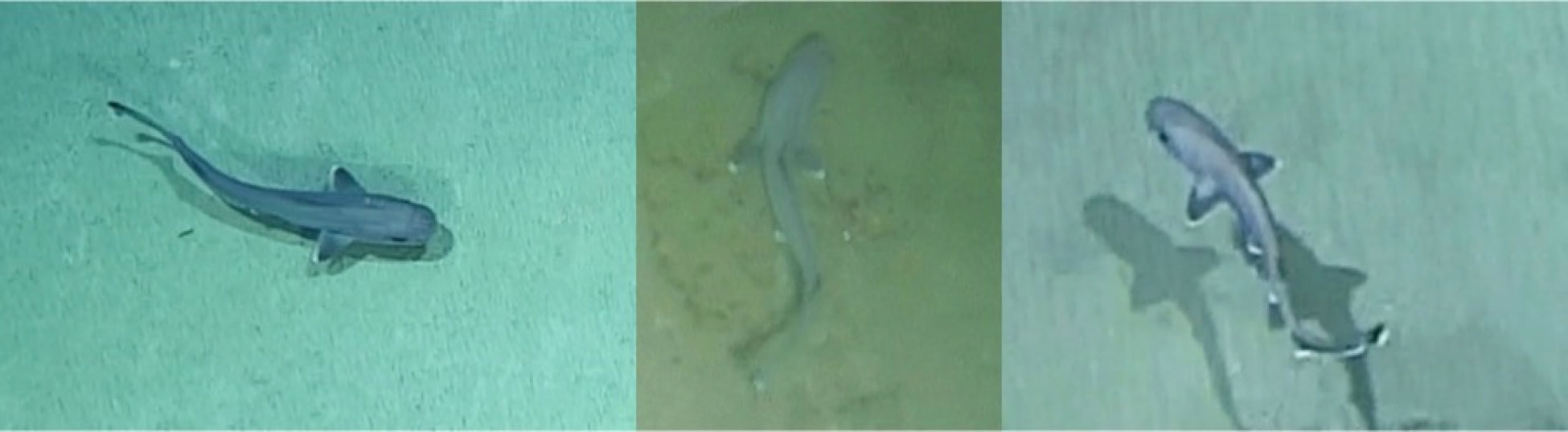
Figure 6 Three different individuals of I. omanensis which exhibit fins with white edges. The quality of the images is low due to limits imposed by the environment.
Discussion
Carcharhinus altimus
The bignose shark, C. altimus, has previously been recorded at a maximum depth of 810 m in Northern Australia (Stevens, 1991). During the RSDE we recorded this species at 886 m, extending the known depth record. C.altimus has been described as a deep-benthic shark (Compagno, 2001; Smith and Heemstra, 1986), possibly undergoing diel vertical migrations rising toward the surface at night, following prey on related biomass movements (Brierley, 2014; Behrenfeld et al., 2019). Reliable data on the specific depth range of this species or its effective distribution is scarce, often due to misidentification with other related species of the Family Carcharhinidae, like the Sandbar shark (C. plumbeus) or the Dusky shark (C. obscuros) (Duffy and Struthers, 2017; Ayas et al., 2020). This can possibly be ascribed to the almost neutral features this species possesses, preventing an easy morphological identification, particularly given many observations are fleeting. Accurate identification of C. altimus individuals remains troublesome, even though some characters can be used for identification, such as the position and size of the fins. Moreover, it is difficult to have a confident basis for their identification in the absence of accurate images of these animals in their natural environment. In our study, the correct species was identified based on distinctive characteristics observed. These include a heavy cylindrical body shape, a large and elongated snout with long nasal flaps, a prominent interdorsal ridge. The pectoral and dorsal fins are large and straight. The first dorsal fin begins in the middle of the pectoral fin and exhibits an angular or slightly rounded apex. These peculiar traits allowed for accurate species identification (Compagno et al., 1989; Grace, 2001; Serena, 2005).
Carcharinus altimus is threatened both as a target species exploited for its meat and fins and as bycatch from pelagic longline, gillnet, and bottom trawl fisheries (White et al., 2006; Ebert et al., 2013; Fields et al., 2018). As a consequence, it is assessed as Near Threatened with a decreasing population trend in the IUCN Red List. However, existing uncertainties on the correct identification of this shark species render current assessments unreliable. Increasing the amount of data available for this species is necessary to promote its protection and conservation.
Rhinobatos punctifer
Rhinobatos punctifer is a batoid inhabiting neritic waters in subtidal sandy, subtidal sandy-muddy and subtidal muddy habitats. This species was described for the first time in the Red Sea (Compagno, 1987), and subsequently reported in other areas ranging from the northern Red Sea to the Arabian Sea and Arabian Gulf (Golani, 1993; Ebert et al., 2017; Last et al., 2019; Bogorodsky et al., 2021). The species is distinguished by a brown-colored dorsal surface of the body and tail, and small sparse light spots on the dorsal side. There is limited information available for this guitarfish, further complicated by previous misidentification with similar species in the same family (Ebert et al., 2017). The deepest record of this batoid species was 150 m in the Gulf of Aqaba, dating back to 1996 (Goldshmidt et al., 1996) and our new observation extends its depth record down to 486 m. It is considered as Near Threatened on the IUCN Red List, but additional data are needed in order to better define the level of risk to which this species is exposed, due to fisheries activities in the Red Sea and habitat loss (Ebert et al., 2017). Catch data on the Spotted Guitarfish is limited, but it is captured with demersal trawls, inshore set gillnets and set net operations common in the northern Red Sea (Bonfil and Abdallah, 2004). The level of exploitation of habitats considered suitable for this organism in the Arabian Peninsula has been increasing over time, likely impacting on their populations (Valinassab et al., 2006; Bruckner et al., 2011). As above, more data on the ecology and behavior of this species are needed. The novel depth records presented in this publication provide further context into the habitats utilization of this species and offers a valuable reference for more reliable in-water future identifications.
Iago omanensis
Iago omanensis is a small Triakidae inhabiting relatively deep waters of the Red Sea, Arabian Sea and Arabian Gulf (Compagno, 2001; Randall, 1995). First described by Norman and Murray (1939), it is characterized by dark-gray dorsal and pale ventral color, with dark edges on the dorsal fins and on the upper caudal lobe of the tail. The margins of the fins appear almost transparent (Baranes and Ben-Tuvia, 2013). The recently-reviewed depth distribution of this species indicates the maximum depth at 2,522 m (Pearce et al., 2023), reported in the Suakin Trough (Red Sea). In Figure 1 we present the most recent depth distribution for this shark, including a shallower upper limit, 92 m, than the previously established 110 m (Compagno, 2001). The diet of this animal is composed of small bony fish (mainly mychtophids), remarkably abundant in the mesopelagic zone of the Red Sea (Dalpadado and Gjøsaeter, 1987; Klevjer et al., 2012), along with cephalopods and crustaceans (Waller and Baranes, 1991; Waller and Baranes, 1994; Goldshmidt et al., 1996). It has been postulated that I. omanensis is prey to larger shark species, like C. plumbeus and Galeocerdo cuvier, even though there is no evidence of this species being found in the stomach content of these predators.
We observed the presence of an individual of I. omanensis followed right after by an individual of C. altimus (Supplementary material Video 10). This may be linked to previous suggestions that bigger sharks feed on Iago omanensis in the aphotic zone of the Red Sea (Goldshmidt et al., 1996). However, our observations do not provide evidence of a predation attempt, which was not captured in the footage, and further investigations are needed in order to resolve the trophic interactions among these two species.
The Bigeye houndshark I. omanensis is assessed as Least Concern on the IUCN Red List, however the ongoing decline of local populations in the Red Sea suggest that this species is at risk, at least locally, despite limited data on its distribution and behavior (Spaet et al., 2012; Dulvy et al., 2021). The feeding behavior of I. omanensis have been postulated in previous studies only by indirect arguments, due to observations of its behavior in the wild being scarce. Our video recordings provide the first direct evidence of feeding ecology for the species, which supports previous assumptions (Nelson et al., 2001). In particular, our videos support the function of the blade-like teeth with a laterally pointed oblique cusp, similar to the characteristics of Squaloid sharks (e.g., Squalus acanthias) (Compagno, 2001; Wilga and Motta, 1998).
As reviewed in previous studies, some specimens of the genus Mustelus (closely related with the genus Iago) can present white edges on the fins in juvenile life stages (e.g., M. canis), while other Mustelus species, like M. andamanensis, M. norrisi and M. albipinnis, can have the white margins on the fins even as adults (Heemstra, 1997; Castro-Aguirre et al., 2005; White et al., 2021). Indeed, the observation of I. omanensis individuals presenting a peculiar white edge on the fins opens the door to various possibilities, like an intra-specific color variation, a possible shift in color markings with age, or the hypothesis of the presence of a not yet identified species or subspecies of Iago in the Eastern Red Sea, co-existing with the one already reported in the literature, as it happens for Mustelus canis canis and Mustelus canis insularis (Heemstra, 1997). Molecular techniques would be useful to investigate this topic further if specimens can be sampled.
Conservation and management implications
The extended global depth range of three elasmobranch species (Carcharhinus altimus, Rhinobatos punctifer, Iago omanensis) presented here can help improve conservation strategies, as different fishing techniques target different zones of the water column. With rising concern on the global decline of elasmobranch populations, an adequate understanding of their habitat use is fundamental to guide conservation strategies (Walker, 1998; Stevens et al., 2000; Baum et al., 2003; Ferretti et al., 2008).
The possible mechanisms enabling Red Sea elasmobranch to occupy deep water habitats also deserve additional attention. We suggest here that the occupation of deep Red Sea waters by these species may be related to the unique thermal regime of this region, in which temperatures remain > 21°C throughout the seafloor deeper than 2,800 m, suggesting that lower thermal thresholds of species may not be a limiting factor of vertical habitat use.
Information on the movements of the three species examined in this study is extremely limited, but future research utilizing animal-borne sensors (e.g., temperature, depth, etc.) on these species in the Red Sea will likely yield further insights into the novel depth records described here. The increasing utilization of drop cameras, submersibles and ROV to monitor marine fauna in the deep will enable additional observations of elasmobranch species at great depths, allowing an improved understanding of their ecology, habitat use and niches extent.
The newly reported depth ranges of these shark species suggest the possibility that the study area hosts depth refugia of conservation importance for Rhinobatos punctifer. This information is timely, as the northernmost section of the study area is within NEOM, a major development region of Saudi Arabia. The NEOM project has committed to protecting 95% of the 26,500 km2 region for nature, and planning is underway for a nature reserve that includes the majority of the marine environment of the region, including habitats covering the full range of depths reported in this study. These results provide important information to help ensure that the NEOM nature reserve provides conservation benefits to R. punctifer, inhabiting depths not previously recorded for this species.
Similarly, with the Kingdom’s ambitious plans to protect 30% of its marine areas by 2030 under the aegis of the National Center for Wildlife, this better understanding of depth ranges of important chondrichthyan species will allow for refined Marine Protected Area design that takes into account these three-dimensional data to protect deep water habitats alongside other coastal and marine areas of importance.
Data availability statement
Publicly available datasets were analyzed in this study. This data can be found here: https://www.fishbase.se/search.php, https://www.iucnredlist.org.
Ethics statement
Ethical approval was not required for the study involving animals in accordance with the local legislation and institutional requirements because this study uses video recordings and no handling of animals was required.
Author contributions
SF: Conceptualization, Data curation, Formal Analysis, Investigation, Methodology, Software, Visualization, Writing – original draft, Writing – review & editing. CW: Conceptualization, Data curation, Investigation, Validation, Writing – review & editing. NP: Data curation, Funding acquisition, Resources, Validation, Writing – review & editing. MR: Data curation, Writing – review & editing. PM: Conceptualization, Data curation, Funding acquisition, Resources, Validation, Writing – review & editing. PV: Data curation, Funding acquisition, Resources, Writing – review & editing. MQ: Funding acquisition, Project administration, Resources, Writing – review & editing. CD: Conceptualization, Data curation, Funding acquisition, Investigation, Methodology, Project administration, Resources, Supervision, Validation, Writing – review & editing.
Funding
The author(s) declare financial support was received for the research, authorship, and/or publication of this article. This project, along with the entire RSDE, was founded by NCW. This work was supported by King Abdullah University of Science and Technology (KAUST) baseline fundings BAS/1/1071-01-01 and The Al-Khwarizmi/KAUST Fellowship. All data collected onboard, including data collected by sensors, acoustic mapping devices, images collected by ROVs and submersibles on expedition vessels (OceanX and Al Azizi) are property of NCW. The videos, images and audio media obtained during the course of the RSDE are credited to the National Center of Wildlife, Kingdom of Saudi Arabia.
Acknowledgments
We thank the National Center of Wildlife (NCW) for the invitation to participate in the Red Sea Decade Expedition. The authors also thank the crew of the R/V OceanXplorer for the logistic support offered throughout the entire expedition. We thank MQ, VP, and CMD that designed the entire expedition.
Conflict of interest
The authors declare that the research was conducted in the absence of any commercial or financial relationships that could be construed as a potential conflict of interest.
Publisher’s note
All claims expressed in this article are solely those of the authors and do not necessarily represent those of their affiliated organizations, or those of the publisher, the editors and the reviewers. Any product that may be evaluated in this article, or claim that may be made by its manufacturer, is not guaranteed or endorsed by the publisher.
Supplementary material
The Supplementary Material for this article can be found online at: https://www.frontiersin.org/articles/10.3389/fmars.2023.1270257/full#supplementary-material
References
Andrzejaczek S., Lucas T. C.D., Goodman M. C., Hussey N. E., Armstrong A. J., Carlisle A., et al. (2022). Diving into the vertical dimension of elasmobranch movement ecology. Sci. Adv. 8 (33), 1754. doi: 10.1126/SCIADV.ABO1754/SUPPL_FILE/SCIADV.ABO1754_TABLE_S3.ZIP
Ayas D., Ciftci N., Yalcin E., Akbora H. D., Bakan M. (2020). First record of the big nose shark, Carcharhinus altimus (Springer 1950) from mersin bay. Int. J. Fish. Aquat. 8 (2), 132–6.
Baco A. R., Etter R. J., Ribeiro P. A., von der Heyden S., Beerli P., Kinlan B. P. (2016). A synthesis of genetic connectivity in deep-sea fauna and implications for marine reserve resign. Mol. Ecol. 25 (14), 3276–3298. doi: 10.1111/MEC.13689
Baranes A., Ben-Tuvia A. (2013). Two rare carcharhinids, Hemipristis elongatus and Iago omanensis, from the northern Red Sea. Israel J. Zoology 28 (1), 39–50.
Barnes A. (2007). The proceedings of the international workshop on mediterranean cartilaginous fish with emphasis on southern and eastern Mediterranean 14-16 October 2005 Ataköy Marina Istanbul-TURKEY Edited By.
Barragán-Méndez C., Sánchez-García F., Sobrino I., Mancera J. M., Ruiz-Jarabo I. (2018). Air exposure in catshark (Scyliorhinus canicula) modify muscle texture properties: A pilot study. Fishes 3 (3), 34. doi: 10.3390/FISHES3030034
Baum J. K., Myers R. A., Kehler D. G., Worm B., Harley S. J., Doherty P. A. (2003). Collapse and conservation of shark populations in the northwest Atlantic. Science 299 (5605), 389–392. doi: 10.1126/SCIENCE.1079777/SUPPL_FILE/BAUM.SOM.REV.PDF
Behrenfeld M. J., Gaube P., Della Penna A., O’Malley R. T., Burt W. J., Hu Y., et al. (2019). Global satellite-observed daily vertical migrations of ocean animals. Nature 576 (7786), 257–261. doi: 10.1038/s41586-019-1796-9
Bogorodsky S. V., Uwe Z., Saeed F. N., Weigmann S. (2021). Notes on batoid fishes of the Socotra archipelago (North-western Indian Ocean), with four new records. Zootaxa 4951 (3), 511–528. doi: 10.11646/ZOOTAXA.4951.3.5
Bonfil R., Abdallah M. (2004). Field identification guide to the sharks and rays of the red sea and gulf of aden. Field Identification Guide to Sharks Rays Red Sea Gulf Aden.
Brierley A. S. (2014). Diel vertical migration. CURBIO 24, R1074–R1076. doi: 10.1016/j.cub.2014.08.054
Bruckner A. W., Alnazry H. H., Faisal M. (2011). Sustainable fisheries: multi-level approaches to a global problem, 85-111 A paradigm shift for fisheries management to enhance recovery, resilience, and sustainability of coral reef ecosystems in the red sea.
Castro-Aguirre J. L., Antuna-Mendiola A., González-Acosta Adrián F., De la Cruz-Agüero J. (2005). Mustelus Albipinnis Sp. Nov. (Chondrichthyes: Carcharhiniformes: Triakidae) de La Costa Suroccidental de Baja California Sur, México. Hidrobiológica 15 (2), 123–130.
Clarke S. C., McAllister M. K., Milner-Gulland E. J., Kirkwood G. P., Michielsens C. G.J., Agnew D. J., et al. (2006). Global estimates of shark catches using trade records from commercial markets. Ecol. Lett. 9 (10), 1115–1126. doi: 10.1111/J.1461-0248.2006.00968.X
Cliff G., Thurman G. D. (1984). PATHOLOGICAL AND PHYSIOLOGICAL EFFECTS OF STRESS DURING CAPTURE AND TRANSPORT IN THE JUVENILE DUSKY SHARK, CARCHARHINUS OBSCURUS. Biochem. Physiol. 78 (1), 167–173. doi: 10.1016/0300-9629(84)90111-7
Coelho R., Fernandez-Carvalho J., Santos M. N. (2015). Habitat use and diel vertical migration of bigeye thresher shark: overlap with pelagic longline fishing gear. Mar. Environ. Res. 112, 91–99. doi: 10.1016/J.MARENVRES.2015.10.009
Compagno L. J. V. (2001). Sharks of the world: an annotated and illustrated catalogue of shark species known to date (Food & Agriculture Org.) Vol. 2.
Compagno L. J. V. (1987). Rhinobatos punctifer, a new species of guitarfish (Rhinobatiformes: Rhinobatidae) from the Red Sea, with notes on the Red Sea batoid fauna. Proc Calif Acad. Sci. 44, 335–342. doi: 10.3/JQUERY-UI.JS
Dalpadado P., Gjøsaeter J. (1987). Observations on mesopelagic fish from the red sea. Mar. Biol. 96 (2), 173–183. doi: 10.1007/BF00427017/METRICS
Duffy C. A. J., Struthers C. D. (2017). A major range extension for carcharhinus altimus (Springer 1950) in the south Pacific Ocean. South Pacific Ocean. Zootaxa 4323 (1), 132–138. doi: 10.11646/zootaxa.4323.1.11
Dulvy N. K., Bineesh K. K., Derrick D., Elhassan I., Haque A. B., Jabado R. W., et al. (2021). Iago Omanensis, bigeye houndshark. THE IUCN RED LIST OF THREATENED SPECIES™. doi: 10.2305/IUCN.UK.2021-2.RLTS.T161501A173438349.en
Dulvy N. K., Fowler S. L., Musick J. A., Cavanagh R. D., Kyne P. M., Harrison L. R., et al. (2014). Extinction risk and conservation of the world’s sharks and rays. Elifesciences.Org 3, 590. doi: 10.7554/eLife.00590
Ebert D. A., Khan M., Ali M., Akhilesh K. V., Jabado R. W. (2017). Spotted guitarfish rhinobatos punctifer. doi: 10.2305/IUCN.UK.2017-2.RLTS.T161447A109904426.EN
Ebert D. A., S. L. Fowler, Compagno L. J. (2013). Sharks, batoids, and chimaeras of the North Atlantic. FAO Species Catalogue for Fishery Purposes. No. 7 (Rome: Wild Nature Press), 528 p.
Ebert D. A., Stehmann M. F. W. (2013). AO Species Catalogue for Fishery Purposes No. 7. Food and Agricultural Organization of the United Nations (FAO) (Rome: FAO), 523p. doi: 10.1080/17451000.2014.950588
Ferretti F., Myers R. A., Serena F., Lotze H. K. (2008). Loss of large predatory sharks from the Mediterranean Sea. Conserv. Biol. 22 (4), 952–964. doi: 10.1111/J.1523-1739.2008.00938.X
Fields A. T., Fischer G. A., Shea S. K.H., Zhang H., Abercrombie D. L., Feldheim K. A., et al. (2018). Species composition of the international shark fin trade assessed through a retail-market survey in Hong Kong. Conserv. Biol. 32 (2), 376–389. doi: 10.1111/COBI.13043
Gallagher A. J., Shiffman D. S., Byrnes E. E., Hammerschlag-Peyer C. M., Hammerschlag N. (2017). Patterns of Resource Use and Isotopic Niche Overlap among Three Species of Sharks Occurring within a Protected Subtropical Estuary. Aquat. Ecol. 51 (3), 435–448. doi: 10.1007/S10452-017-9627-2/FIGURES/7
Garzon F., Williams C. T., Cochran J. E.M., Tanabe L. K., Abdulla A., Berumen M. L., et al. (2022). A multi-method characterization of elasmobranch & Cheloniidae communities of the north-eastern Red Sea and gulf of Aqaba. PLoS One 17 (9), e0275511. doi: 10.1371/JOURNAL.PONE.0275511
Gilman E., Chaloupka M., Bach P., Fennell H., Hall M., Musyl M., et al. (2020). Effect of pelagic longline bait type on species selectivity: A global synthesis of evidence. Rev. Fish Biol. Fisheries 30 (3), 535–551. doi: 10.1007/S11160-020-09612-0/TABLES/2
Goetze J. S., Langlois T. J., Egli D. P., Harvey E. S.. (2011). Evidence of artisanal fishing impacts and depth refuge in assemblages of Fijian reef fish. Coral Reefs 30 (2), 507–517. doi: 10.1007/S00338-011-0732-8/FIGURES/5
Golani D. (1993). An annotated list of deep-sea fishes collected in the northern Red Sea, gulf of Aqaba. Israel J. Zoology 39 (4), 299–336. doi: 10.1080/00212210.1993.10688725
Goldshmidt O., Galil B., Golani D., Lazar J. (1996) Deep-sea and extreme shallow-water habitats: affinities and adaptations.-biosystematics and ecology series 11:271-298. Food selection and habitat preferences in deep-sea fishes of the northern red sea. Available at: Www.Biologiezentrum.at.
Grace M. (2001). Field Guide to Requiem Sharks (Elasmobranchiomorphi: Carcharhinidae) of the Western North Atlantic Vol. 153 (US Department of Commerce).
Heemstra P. C. (1997). A review of the smooth-hound sharks (Genus mustelus, family triakidae) of the western atlantic ocean, with descriptions of two new species and a new subspecies. Bull. Mar. Sci. 60 (3), 894–928.
Huvenne V. A., Bett B. J., Masson D. G., Le Bas T. P., Wheeler A. J. (2016). Effectiveness of a deep-sea cold-water coral marine protected area, following eight years of fisheries closure. Biol. Conserv. 200, 60–69. doi: 10.1016/J.BIOCON.2016.05.030
Klevjer T. A., Torres D. J., Kaartvedt S. (2012). Distribution and diel vertical movements of mesopelagic scattering layers in the red sea. Mar. Biol. 159 (8), 1833–1841. doi: 10.1007/S00227-012-1973-Y/FIGURES/4
Last P. R., SÉRet B., Naylor G. J.P. (2019). Description of rhinobatos ranongensis sp. Nov. (Rhinopristiformes: Rhinobatidae) from the Andaman Sea and bay of Bengal with a review of its northern Indian ocean congeners. Zootaxa 4576 (2), 257–287. doi: 10.11646/ZOOTAXA.4576.2.3
Marshall N. B., Bourne D. W. (1964). “A PHOTOGRAPHIC SURVEY OF BENTHIC FISHES IN THE RED SEA AND GULF OF ADEN, WITH OBSERVATIONS ON THEIR POPULATION DENSITY, DIVERSITY, AND HABITS,” in Bulletin of the Museum of Comparative Zoology (Cambridge, MASS., U.S.A.: Harvard University), Vol. 132 No. 2.
Mundy C. (2005). Checklist of the fishes of the Hawaiian archipelago. Bishop Mus. Bull. Zool. 6, 1–704.
Murua H., Griffiths S. P., Hobday A. J., Clarke S. C., Cortés E., Gilman E. L., et al. (2021). Shark mortality cannot be assessed by fishery overlap alone. Nature 595 (7866), E4–E7. doi: 10.1038/s41586-021-03396-4
Nelson D. R., Tricas T. C., Gruber S. H. (2001). The behavior and sensory biology of elasmobranch fishes : an anthology in memory of Donald Richard Nelson. (Springer Science & Business Media) Vol. 20. doi: 10.1007/978-94-017-3245-1
Norman J. R., Murray J. (1939). Fishes. The John Murray expedition 1933-34. Scientific reports. Sci. Rep. 7, 1–116.
Pearce J. R., Linley T. D., Bond T., Jamieson A. J. (2023). Depth distribution of the Bigeye hound shark Iago Omanensis and other deep-sea species observed by baited-camera in the red sea. J. Mar. Biol. Assoc. United Kingdom 103, e8. doi: 10.1017/S002531542200100X
Randall J. E. (1995). Coastal Fishes of Oman (University of Hawaii Press), 439 p. doi: 10.2307/1447687
Rigby C. L., Morgan D. L., Derrick D. (2020). “Carcharhinus altimus,” in The IUCN Red List of Threatened Species 2020, e.T161564A890724. doi: 10.2305/IUCN.UK.2020
Serena F. (2005). Field identification guide to the sharks and rays of the mediterranean and black sea. Food Agric. Org. 2005 Edition (FAO - FOOD AND AGRICULTURE ORGANIZATION OF THE UNITED NATIONS).
Smith M. M., Heemstra P. C. (1986). Smiths’ Sea Fishes. (Heidelberg: Springer Berlin). doi: 10.1007/978-3-642-82858-4_14
Smith M., Warmolts D., Thoney D., Hueter R. (2004). The elasmobranch husbandry manual: captive care of sharks, rays and their relatives. Special Publication of the Ohio Biological Survey. 589 (145), 4.
Smith M. F. (1992). Capture and transportation of elasmobranchs, with emphasis on the grey nurse shark (Carcharias taurus). Mar. Freshw. Res. 43 (1), 325–343.
Spaet J. L.Y., Nanninga G. B., Berumen M. L. (2016). Ongoing decline of shark populations in the eastern Red Sea. Biol. Conserv. 201, 20–28. doi: 10.1016/J.BIOCON.2016.06.018
Spaet J. L. Y., Thorrold S. R., Berumen M. L. (2012). A review of elasmobranch research in the red sea. J. Fish Biol. 80 (5), 952–965. doi: 10.1111/J.1095-8649.2011.03178.X
Stevens J. D. (1991). Distribution, size and sex composition, reproductive biology and diet of sharks from northern Australia. Aust. J. Mar. Freshw. Res. 42, 151–199. doi: 10.1071/MF9910151
Tesfamichael D., Pauly D. (2016). The Red Sea Ecosystem and Fisheries. Tesfamichael D., Pauly D. eds. (Dordrecht: Springer Netherlands) Vol. 7
Tesfamichael D., Pitcher T. J. (2006). Multidisciplinary evaluation of the sustainability of Red Sea fisheries using Rapfish Fish. Res. 78 (2-3), 227–235. doi: 10.1016/J.FISHRES.2006.01.005
Stevens J. D., Bonfil R., Dulvy N. K., Walker P. A. (2000). The effects of fishing on sharks, rays, and chimaeras (Chondrichthyans), and the implications for marine ecosystems. ICES J. Mar. Sci. 57 (3), 476–494. doi: 10.1006/JMSC.2000.0724
Valinassab T., Daryanabard R., Dehghani R., Pierce G. J. (2006). Abundance of demersal fish resources in the persian gulf and Oman sea. J. Mar. Biol. Assoc. United Kingdom 86 (6), 1455–1462. doi: 10.1017/S0025315406014512
Walker T. I. (1998). Can shark resources be harvested sustainably? A question revisited with a review of shark fisheries. Mar. Freshw. Res. 49 (7), 553–572. doi: 10.1071/MF98017
Waller G. N. H., Baranes A. (1991). Chondrocranium morphology of northern red sea Triakid sharks and relationships to feeding habits. J. Fish Biol. 38 (5), 715–730. doi: 10.1111/J.1095-8649.1991.TB03160.X
Waller G. N. H., Baranes A. (1994). Food of iago Omanensis, a deep water shark from the northern red sea. J. Fish Biol. 45 (1), 37–45. doi: 10.1111/J.1095-8649.1994.TB01284.X
Weigmann S. (2016). Annotated checklist of the living sharks, batoids and chimaeras (Chondrichthyes) of the world, with a focus on biogeographical diversity. J. Fish Biol. 88, 837–1037. doi: 10.1111/jfb.12874
White W. T., Arunrugstichai S., Naylor G. J.P. (2021). Revision of the genus Mustelus (Carcharhiniformes: Triakidae) in the northern Indian Ocean, with description of a new species and a discussion on the validity of M. walkeri and M. ravidus. Mar. Biodivers. 51, 1–24. doi: 10.1007/s12526-021-01161-4
White W. T., Last P. R., Stevens J. D., Yearsly G. K. (2006). Economically important sharks and rays of Indonesia.
Keywords: Red Sea, biological observations, discoveries, coastal ecosystems, deep-sea observations, extension range, elasmobranchs
Citation: Frappi S, Williams C, Pilcher N, Rodrigue M, Marshall P, Pieribone V, Qurban M and Duarte CM (2023) New depth records and novel feeding observations of three elasmobranchs species in the Eastern Red Sea. Front. Mar. Sci. 10:1270257. doi: 10.3389/fmars.2023.1270257
Received: 31 July 2023; Accepted: 19 September 2023;
Published: 06 October 2023.
Edited by:
J. Marcus Drymon, Mississippi State University, United StatesReviewed by:
Samantha Andrzejaczek, School of Humanities and Sciences, Stanford University, United StatesYannis Peter Papastamatiou, Florida International University, United States
Copyright © 2023 Frappi, Williams, Pilcher, Rodrigue, Marshall, Pieribone, Qurban and Duarte. This is an open-access article distributed under the terms of the Creative Commons Attribution License (CC BY). The use, distribution or reproduction in other forums is permitted, provided the original author(s) and the copyright owner(s) are credited and that the original publication in this journal is cited, in accordance with accepted academic practice. No use, distribution or reproduction is permitted which does not comply with these terms.
*Correspondence: Sofia Frappi, U29maWEuZnJhcHBpQGthdXN0LmVkdS5zYQ==