- 1Southeast Fisheries Science Center, National Marine Fisheries Service, National Oceanic and Atmospheric Administration, Miami, FL, United States
- 2Southwest Marine Mammal Field Station, Fish and Wildlife Research Institute, Florida Fish and Wildlife Conservation Commission, Port Charlotte, FL, United States
- 3Department of Marine Biology and Ecology, Rosenstiel School of Marine, Atmospheric, and Earth Science, University of Miami, Miami, FL, United States
- 4School of Teaching and Learning, College of Education, University of Florida, Gainesville, FL, United States
- 5Marine Mammal Pathology Services, Olney, MD, United States
As the first line of defense, the integumentary system is critical in comprehensively evaluating dolphin morbidity during stranding response. Most published studies on skin lesions in bottlenose dolphins (Tursiops truncatus) lack standardized gross descriptions and methodologies for evaluating lesions. The primary objective of this study was to evaluate the effectiveness of an assessment matrix designed to consistently describe skin lesions based on a set of standardized gross description characteristics. The matrix was implemented by reviewing necropsy reports, histopathology reports, and photographs collected from bottlenose dolphins stranded in Southwest Florida from 2015 through 2019. Of the 32 dolphins that met the inclusion criteria, 19 presented with skin lesions and five reviewers described each of the 46 lesions according to a novel, standardized assessment matrix. The most common descriptor selected, in each of the respective matrix categories, were, by anatomic location, head; distribution, multifocal to coalescing; quantity, moderate (10–30); size, <2 cm; shape, punctate; margin, rounded; color modifier, hyperpigmentation; texture, smooth; and texture modifier, flat. These prevalent descriptors coincided with the frequent occurrence of histologically described hydropic degeneration (n=7, 15.2%) and were confirmed poxviral lesions in 6.52% (n=3). Identifying lesion patterns using standardized descriptors capitalizes on the unique pathogen tissue tropism and the implementation of certain disease mechanisms in the integumentary system. Therefore, it can facilitate differential disease diagnoses and guide ancillary diagnostics testing. The use of standardized descriptors will aid in etiologic identification and monitoring of skin lesions and associated diseases, advancing our understanding of dolphin morbidity and mortality.
1 Introduction
As sentinel species, marine mammals have unique qualities (e.g., long life-span, high site fidelity, predators) that allow detection of natural and anthropogenic risks to ocean and human health (Wells et al., 2004; Van Bressem et al., 2009a; Bossart, 2011; Bossart et al., 2017; Bossart and Duignan, 2018). Skin lesions on cetaceans indicate an infringement of the immune system’s first line of defense and can serve as an external sign of morbidity (Wilson et al., 1999; Zabka and Romano, 2003; Reif et al., 2009; Lauriano et al., 2014; Bossart et al., 2017; Correia et al., 2023). Infectious and noninfectious conditions have been observed in common bottlenose dolphins (Tursiops truncatus) that are either directly or indirectly associated with skin lesions.
Various types of infectious diseases (e.g., viral, bacterial, fungal) present cutaneous manifestations in many cetacean species (Geraci et al., 1979; Schulman and Lipscomb, 1999; Murdoch et al., 2008; Rehtanz et al., 2012; Townsend and Staggs, 2020). The mechanisms of these diseases and pathogen tissue tropism result in distinct lesion patterns. Identifying patterns could help differentiate between disease types and streamline diagnostics and pathogen identification. For example, dolphin poxviruses present as various forms of light to dark gray and black (hyperpigmented) skin lesions on the head, dorsum, dorsal fin, pectoral flippers, and fluke. Early stages typically present as flat to slightly raised, round to elliptical, light gray pigmented lesions with dark gray margins (i.e., ring, circle), while advanced stages transition to punctate, hyperpigmented, depressed, pitted centers (i.e., pinhole; Townsend and Staggs, 2020). Aggregation of pitted, punctate foci or the confluence of round to elliptical foci are thought to result in the formation of the tattoo lesion pattern. However, it has been theorized that the varied pattern presentation for dolphin poxvirus could reflect differences between viral strains. Irrespective of poxvirus pattern presentation, it is considered a biomarker of cetacean and habitat health, as stress is believed to influence the external manifestation of this disease (Geraci et al., 1979; Van Bressem et al., 2009b; Segura-Göthlin et al., 2021). Other infectious diseases, such as herpesviruses, papillomaviruses, Erysipelothrix rhusiopathiae, and Paracoccidioides cetii (phylogenetically distinguished from Lacazia loboi), are all documented through the manifestation of skin lesions, wherein similar lesion patterns are highlighted (Schulman and Lipscomb, 1999; Blanchard et al., 2001; Bossart et al., 2003; Reif et al., 2006; Smolarek Benson et al., 2006; Durden et al., 2009; van Elk et al., 2009; Murdoch et al., 2010; Daura-Jorge and Simões-Lopes, 2011; Hart et al., 2011; Laporta et al., 2016; Vilela et al., 2016; Ramos et al., 2018; Vilela et al., 2021).
Certain skin lesions may also be attributed to noninfectious causes, such as unfavorable environmental conditions and various kinds of external trauma that can induce stress and increase susceptibility to secondary infections (Wilson et al., 1999; Dierauf and Gulland, 2001; Hoelzel, 2002; Van Bressem et al., 2007; Maldini et al., 2010; Houser et al., 2011; Moore et al., 2013). Specifically, cetaceans exposed to fresh or lower-salinity water, may exhibit hydropic degeneration defects (ballooning or swelling) of the epidermis because of osmotic stress (Simpson and Gardner, 1972; Colbert et al., 1999; Mullin et al., 2015; Ewing et al., 2017; Duignan et al., 2020; Fazioli and Mintzer, 2020). Freshwater exposure initiates a characteristic change of the epidermis beginning with multifocal to widely disseminated pallor, circular to targetoid erosion and ulceration, and blistering with increased severity. Subsequently, secondary infection (e.g., bacterial, algal, fungal) may occur with prolonged exposure, and green to brown mats or plaques may develop as epibiota growth catches on the uneven surface of the degraded epidermis (Duignan et al., 2020). Gross skin lesion descriptions for dolphins are insufficiently reported in literature, and most papers that have described them originated from photographic identification projects. A major limitation of visual assessment studies is the lack of sample collection for lesions to obtain etiological support and corresponding histopathological evaluation. However, stranding response and carcass examination provide an opportunity for a more detailed gross evaluation, photographic documentation, histopathologic sample collection for examination, etiologic determination and the determination of lesions significance in terms of health and cause of death. First, this gap in the literature heightens the need for standardized gross descriptions, rather than interpretive examinations of skin lesions (Toms et al., 2020; Correia et al., 2023). Standardized gross descriptions are inherently valuable as a source of objectively collected data. Such descriptions can be collected regardless of collectors’ experience level. Proposed is a stepwise method based on classical medical investigative practices. Second, this research demonstrates the importance of collecting and analyzing samples histopathologically, as well as conducting additional diagnostic testing, to further support the standardized gross descriptions (Segura-Göthlin et al., 2021). A standardized assessment is used to elicit descriptive findings rather than a subjective or interpretive conclusion as to the cause for the lesion. The objective of this study was to implement a standardized methodology to generate gross descriptions of skin lesions based on a review of both gross necropsy photographs, necropsy reports, and complementary histologic findings for common bottlenose dolphins stranded between 2015 and 2019 in Southwest Florida.
2 Methods
2.1 Data collection
This study used data from Charlotte, Lee, and Collier counties, in Southwest Florida. The Florida Fish and Wildlife Conservation Commission’s (FWC) Fish and Wildlife Research Institute has a key role in cetacean stranding response and case history management for the region. Stranding data from January 1, 2015, through August 31, 2018, were examined for animal cases with appropriate decomposition levels (i.e., fresh, code 2; early advanced, code 3; Geraci and Lounsbury, 1993) to prevent identifying artifacts of advanced decomposition as skin lesions. Photographs, demographic data, necropsy reports, human interaction (HI) reports, and diagrammatic sketches of scars and lesions were reviewed for the identification and gross evaluation of skin lesions. Histology reports provided morphologic descriptions or diagnoses and identified possible etiologic agents.
Photographs were collected according to established protocols at FWC requiring images be obtained perpendicular to the carcass including dorsal, ventral and both lateral views of the head, and body including the dorsal fin, pectoral flippers and fluke surfaces. Each image collected was to be free of obstruction and glare as well as include scale and animal identification number. To standardize methods and for quality assurance, photographs of each animal were evaluated based on a two-step, scored ranking system (Supplemental Table 1) adapted from Hart et al. (2011; 2012). These photographs were scored separately for image quality for the lateral, dorsal, and ventral aspects. The photograph with the highest score per aspect was selected as representative of that aspect. This resulted in representative photographs for all aspects of the animal, which were used to determine the overall quality of photographs for each animal. Animals scored with an overall poor-quality photograph score were omitted from analyses. Each stranded dolphin was scored as present, absent, or cannot be determined (CBD) for any skin lesion, based on photographs, gross necropsy findings, and histology results, when available. This step was incorporated to control for any artifacts from decomposition or carcass recovery that might have distorted the skin to the point that lesions could not be detected.
A dolphin carcass was excluded from analyses when high-quality photos or histology results were not available, or the presence-absence for lesion detectability was designated as CBD. For each carcass, inter-rater reliability was evaluated for agreement between reviewers for photo quality and skin lesion detectability (Hallgren, 2012; McHugh, 2012); evaluations used Cohen’s kappa statistic, for which a value ≥0.80 indicated a strong level of agreement between the reviewers (McHugh, 2012). This statistic provided validation for the proposed methodology and subsequent analyses. A third reviewer served as a tiebreaker according to photo identification research protocol if the kappa statistic was <0.80 (indicating less than strong agreement, Sarasota Dolphin Research Program, 2006). If all three reviewers disagreed, another kappa value was computed to ensure that a strong level of agreement was obtained before continuing data analysis. Due to the relative nature of Cohen’s kappa, a percent agreement was compared with the kappa value if it remained <0.80 after the tiebreaker (McHugh, 2012; de Vet et al., 2013; Toms et al., 2020; Buczinski et al., 2021). The percent agreement was calculated as the number of cases reviewers agreed upon out of the number of cases reviewed (McHugh, 2012). All statistical analyses were conducted in R (v 3.6.0, R Foundation for Statistical Computing, Vienna, Austria) and RStudio (v 1.2.1335) using R packages irr, and dplyr (Gamer et al., 2019; Wickham et al., 2019).
2.2 Descriptions of skin lesions
External lesions attributed to trauma were excluded; such lesions included conspecific rake marks; evidence of interspecies interactions (e.g., shark bites); HI-related lesions, scars of unknown origin. Lesions not attributed to trauma were considered the result of an infectious, noninfectious, or other, unknown agent. Skin lesions were identified for each carcass and concisely described according to standardized evaluation criteria, including descriptions of characteristics such as anatomical location, distribution, quantity, size, shape, percent coverage, margin, color, and texture (Table 1; Supplemental Table 3) (Dorland, 2011). Anatomical location described the location and orientation of the lesion on the carcass; distribution captured the lesion’s arrangement on the compromised area. Quantity, size, and percent of carcass coverage were selected to comprehensively define the extent of a lesion using ranges (e.g., 10–30 lesions, 2–10 cm, and <25%) that were most appropriate for the lesion. The shape category included well-defined terms, selected based on the overall shapes lesions resembled. The margin descriptor was selected based on the appearance of a lesion’s edges (i.e., sharp, rounded, or indistinct). Color was selected to identify a lesion’s predominant pigment; to limit ambiguity, selections were made from a restricted list of basic colors (e.g., black, gray, white, red, pink). Another color parameter, color modifier, described the pattern of the color (e.g., solid, hyperpigmented, hypopigmented). Texture was described for each lesion by two parameters, texture itself, to describe the consistency of the lesion, and texture modifier to describe its surface contour or elevation.
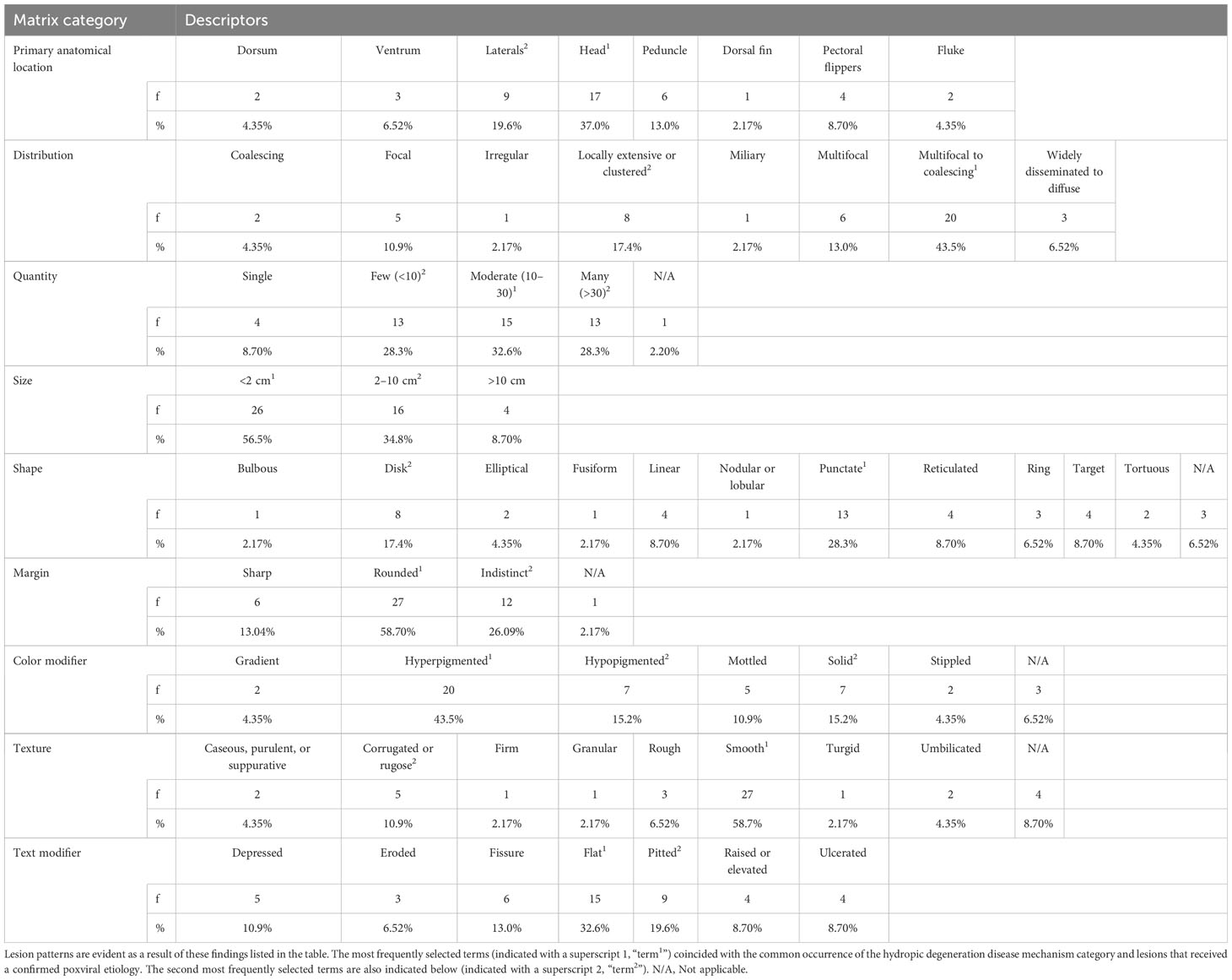
Table 1 Frequency (f) and percentage of selected terms from a standardized assessment matrix to grossly describe 46 skin lesions found on 19 stranded bottlenose dolphins.
Five separate reviewers, including biologists and a veterinary pathologist, described lesions by consensus, selecting the matrix criteria that best described changes observed. Based on histologic findings, gross lesions were assigned to classes that aligned with a predominant or unique, microscopic disease feature (the disease mechanism) that correlates with the gross manifestation. Classes of disease mechanism included disruptive defect, degenerative defect, inflammation, vascular defect, growth or cellular differentiation defect, or developmental defect. Lesions were categorized in each class of disease mechanism using the following case definitions, in order of decreasing degree of certainty: confirmed, probable, presumptive, and suspect (Koo et al., 1997).
A confirmed case had the highest level of confidence for the lesion disease mechanism categorization, relying on histologic examination for characteristic features and the possible identification of etiologic agents. A confirmed case also includes the highest level of confidence when augmented by ancillary diagnostics (e.g., molecular or microbiological tests). Unfortunately, ancillary diagnostics were not conducted in this retrospective study due to lack of sample availability or financial limitations but will be pursued in future studies.
A probable case referred to a lesion that had not been histologically examined but that was similar in gross description to a lesion on the same carcass that had been histologically examined.
A presumptive case referred to a lesion on one dolphin consistent in gross descriptions of histologically confirmed lesions on a different but associated or conspecific dolphin that are temporospatially linked (e.g., affiliated, as in a mother–calf pair or inhabitants of similar habitat) and so was given the same diagnosis.
A suspect case referred to a lesion that had not been histologically examined but whose gross descriptions were consistent with the characteristic features described only for a confirmed case and not known to be temporospatially linked.
Cases with confirmed disease mechanisms were classified as having either infectious, noninfectious, or both (by order of causation).
3 Results
Data from 37 stranded dolphins were assessed, and of those, 32 met the inclusion criteria (e.g., condition code, high-quality photograph, and skin lesion detectability). In total, 46 lesions were observed on 19 dolphins (19/32, 59.4%) and described using standardized gross description terminology derived from using the assessment matrix (Tables 1, 2; Supplemental Table 3). Case definitions were assigned to each skin lesion based on gross description, histology, etiology (via histologic examination), and ancillary diagnostics (Table 2). From eight dolphins, histology samples and histopathologic analysis were available for 14 lesions evaluated in this study (Supplemental Table 4).
Photo quality was assessed during data collection to control for false positives in skin lesion detection. After the first and second reviewers scored photos for quality with respect to obstruction from artifacts, such as glare and poor angle, the Cohen kappa statistic (kappa = 0.379, p = 0.004) revealed a low level of agreement. After the third reviewer scored the photos when the first two reviewers disagreed, the kappa score had a higher agreement (kappa = 0.898, p = 0.00). Three cases were discarded from the data set because of poor photo quality.
The review for determining whether a lesion was present, absent or its presence-absence was CBD, required a third reviewer because the initial kappa statistic between the first and second reviewers did not reflect sufficient agreement (kappa = −0.0577, p = 0.663) to meet the required minimum score (i.e., kappa ≥0.80), verifying inter-rater reliability. Even when a third reviewer served as a tiebreaker, the kappa statistic was still below the required minimum score (kappa = 0.492, p = 0.00), despite the one dispute for which all three reviewers gave a different outcome. Conversely, there was 97% agreement when the data were assessed based on correct agreement to the number of options. Recognizing the relative nature of Cohen’s kappa, the high percentage agreement, and the use of trained reviewers, we determined that the kappa statistic was unnecessary (McHugh, 2012; de Vet et al., 2013; Toms et al., 2020; Buczinski et al., 2021). Therefore, a total of two cases were omitted during vetting because external conditions prevented lesion detectability (CBD).
Terms used most often in describing skin lesions were as follows: anatomical location, head; quantity, moderate (10–30); size, <2cm; shape, punctate; distribution, multifocal to coalescing; color modifier, hyperpigmented; texture modifier, smooth; and texture, flat foci (Table 1). These terms were used in >25% of the instances in each category, developing into a lesion pattern that was associated microscopically with epidermal degenerative changes. These prevalent gross descriptors coincided with the histological description of hydropic degeneration (7/46, 15.2%) and in three cases (6.52%) confirmed poxviral lesions.
Less commonly selected descriptive terms accounted for >10% of the terms used in the respective categories: anatomical location, lateral aspects; distribution, locally extensive or clustered; quantity, few, many; size, 2–10 cm; shape, disk; margin, indistinct margins; color modifier, solid pigmentation; texture, corrugated, rugose; and texture modifier, pitted. The most prevalent disease mechanism, including those for lesions that were not examined histologically, was degenerative defects (33/46, 71.7%), followed by growth or differentiation (, 10/46, 21.7%), disruptive defects (2/46, 4.35%), and vascular defects (1/46, 2.2%). By case definition, degenerative defects were confirmed in 15.2% (n = 7/46) of cases, probable in 6.52% (3/46), presumptive in 0%, and suspect in 50.0% (23/46). Prevalence for growth or differentiation defect cases were confirmed in 8.7% (4/46), probable in 2.2% (1/46), presumptive in 0%, and suspect in 10.9%, (5/46). The disruptive defect and vascular defect cases were all confirmed cases. Confirmed cases were further evaluated based on infectious and noninfectious disease differentials, with a total of seven infectious primary differentials and seven noninfectious primary differentials, for which four had infectious as a secondary differential (Supplemental Table 4). Lesion etiologies were confirmed in three dolphins, including two cases of poxvirus (FMMSN1917, Figure 1, and FMMSN17104, Supplemental Figure 1) and one case of Paracoccidioides cetii (FMMSN1507, Figure 2).
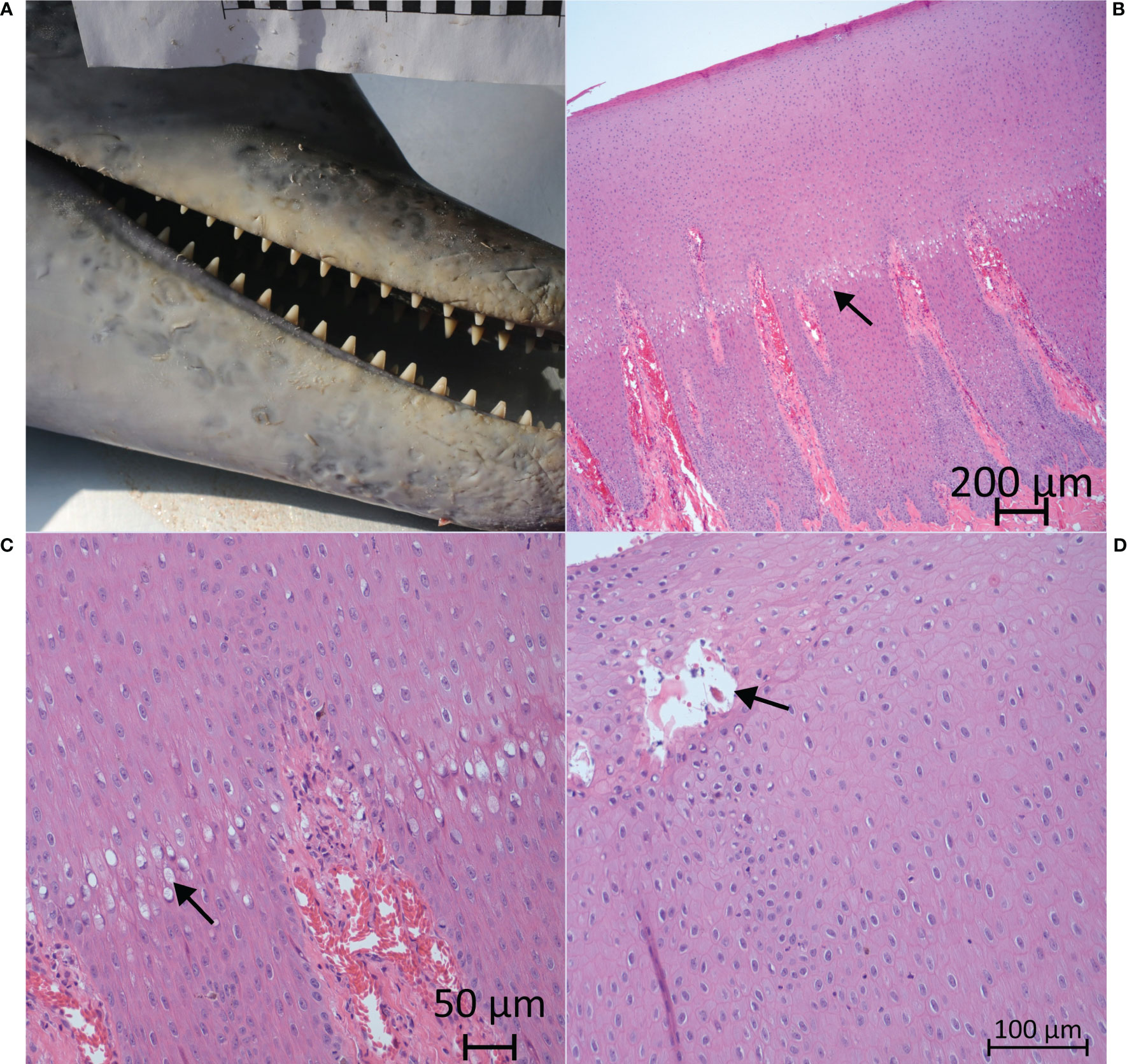
Figure 1 On the rostrum (both the mandible and the maxilla), there were many (>30), locally extensive, <2cm in diameter, ring lesions with rounded margins, gray hyperpigmentation, and a depressed, smooth texture (FMMSN1917 – L1). The gross appearance is depicted in frame A, while microscopic images from histologic examination are depicted in frames B, C, and D. Hydropic degeneration was observed through the tinctorial (color) difference as the upper layer (arrow) begins to swell (B). Higher magnification reveals a boundary of vacuolar change (arrow) that delineates the superficial hydropic degeneration defect in the sample (C), as well as a pustule (arrow) developing immediately below the stratum externum (D).
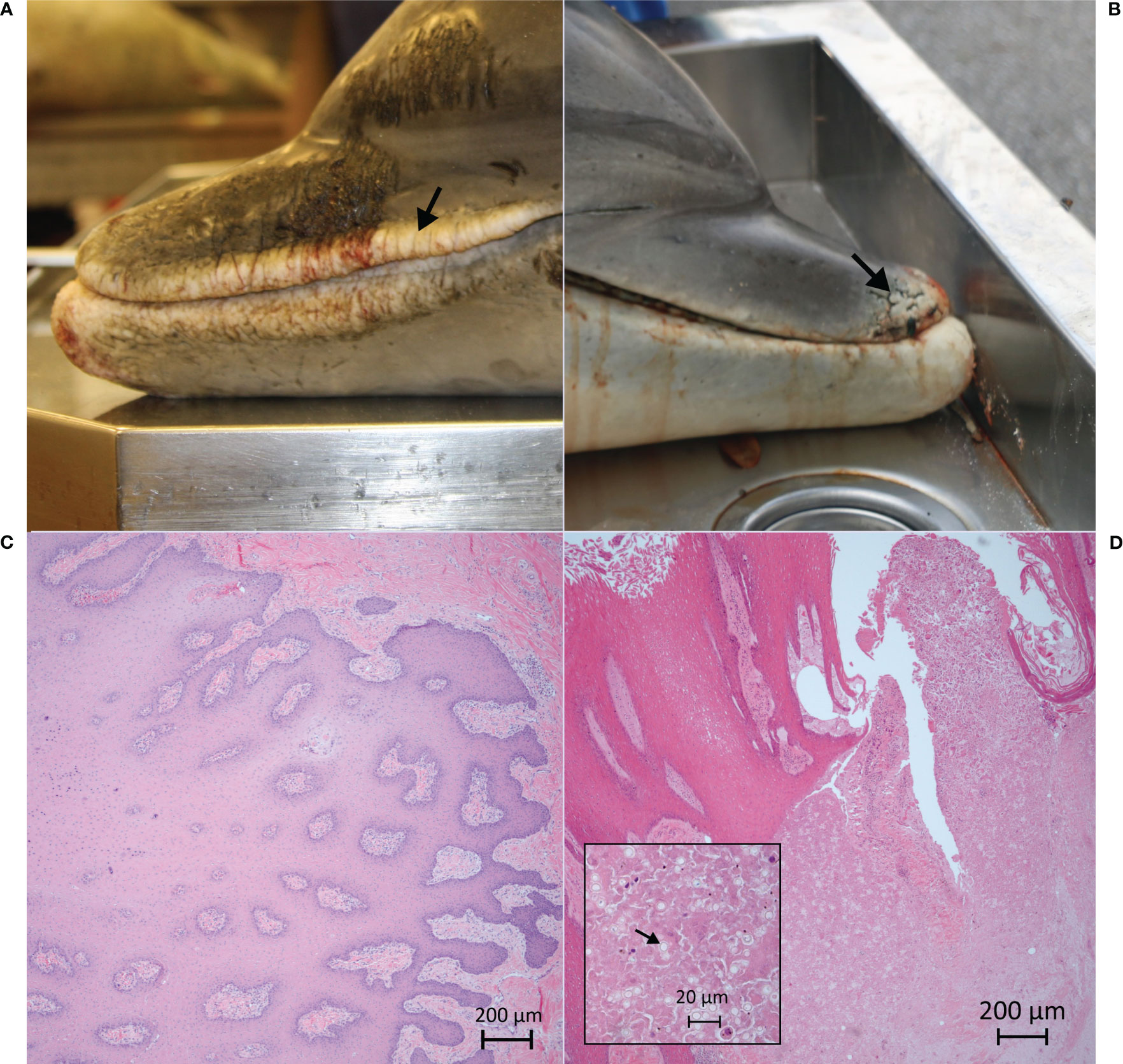
Figure 2 Visual comparison of two skin lesions. In FMMSN1502–L1 (A; see arrow), on gross examination, the rostrum and gape skin have many (10–30) locally extensive, 2- to 10-cm, reticulate, white to tan, hypopigmented lesions with sharp margins and a fissured and corrugated texture along the lip margin. In FMMSN1507–L2 (B; see arrow), on gross examination, the distal tip of the rostrum has a few (fewer than 10) focal, 2- to 10-cm, reticulate, white to tan and gray, hypopigmented lesions with sharp margins and a fissured, granular texture. Histologic examination reveals that FMMSN1507–L2’s rostrum has regionally extensive, moderate granulomatous dermatitis with hyperplasia, focal intraepidermal epithelial cyst and intralesional fungal yeast-like organisms consistent with Paracoccidioides cetii (D). FMMSN1502–L1’s lip margin has moderate, diffuse, epithelial hyperplasia with stratum externum retention, mild neutrophilic and lymphoplasmacytic dermatitis, and dermal fibroplasia (C), both grossly suggestive of P. cetii, but, the etiology was confirmed microscopically only in FMMSN1507–L2 (D; highlighted by arrow in inset).
4 Discussion
The integumentary system provides the first line of defense against the environment and pathogens. The presence of skin lesions indicates a breach and failure of this defense and regulatory system. Determining the prevalence of skin lesions can be an effective and valuable means of assessing cetacean health (Hoelzel, 2002; Zabka and Romano, 2003; Toms et al., 2020). Pathogen tissue tropism and the implementation of certain disease mechanisms can result in distinctive lesion patterns, which can suggest the pathogenesis of various diseases. Recognizing lesion patterns through standardized gross descriptions facilitates determining differential disease diagnoses and guides ancillary diagnostic testing.
Overall, use of this standardized gross descriptive assessment matrix proved to be an effective tool for characterizing lesions in a manner that does not facilitate premature assumptions of etiology. Depending on the user’s familiarity with the descriptors provided in the matrix, certain terms were recalled faster than others. For example, when more than one term could adequately describe a lesion, users may have selected the more familiar term which could result in a rater bias. Regardless, the utility of this assessment was evident when describing gross lesions found in two dolphins that stranded in 2015, which were similarly described. The lesion pattern in both cases was consistent with a P. cetii infection differential, but the one dolphin (FMMSN1502) with hypopigmented, bilateral proliferation and fissures at the lip margin did not display any fungal infiltration on histologic examination. Rather, microscopically there was diffuse, moderate epithelial hyperplasia with stratum externum retention, mild neutrophilic and lymphoplasmacytic dermatitis, and superficial dermal fibroplasia (Figure 2C). Conversely, the other dolphin (FMMSN1507) had focal, granular, and fissured lesions at the rostrum tip from which P. cetii was confirmed intralesionally on histologic examination (Figure 2D). Without the lesion description assessment, both cases would have been described as lacaziosis-like based on the widely accepted gross characteristics of the skin changes observed in each case.
For this assessment matrix to work best, reviewers must set aside any preconceived notions of the lesion pattern or etiology, and simply and consistently describe gross observations. If the user is not intentional, there is potential to select terms in favor of an expected lesion pattern or a suspected etiology. Two cases (FMMSN1917, Figure 1; FMMSN17104, Supplemental Figure 1) morphologically were described similarly, but they retained their individual variability (Supplemental Table 4). This illustrates that bias in the present study was minimal, but future reviewers should recognize that bias is always a possibility.
This work further supports that the procedure is useful and can grow further, though its application with a larger sample size should be evaluated. The four cases reviewed (FMMSN1502, FMMNS1507, FMMSN17104, and FMMSN1917) illustrates that the standardized, objective collection of gross observations makes possible the formulation of a list of specific diagnoses based on lesion patterns. This can guide histopathologic examinations and ancillary diagnostics (e.g., molecular techniques, microbial cultures, or immunohistochemistry) during disease investigations. These findings affirm the value of a standardized tool for describing lesions and the need to minimize interpretative descriptions.
The retrospective nature of this study was limited by the absence of detailed lesion descriptions, limited quality in photographs, and incomplete tissue sampling. Nevertheless, necropsy protocols and the delimitations of the study helped compensate for some of these inconsistencies over the long time period in data collection (Dierauf and Gulland, 2001). This study also emphasizes the importance of regular sampling of skin lesions for histologic examination and ancillary diagnostics (e.g., molecular techniques, microbial cultures, or immunohistochemistry), but this is challenged by logistic constraints, methods of data and sample collection, and funding. Of the 19 dolphins with skin lesions in this study, only 14 lesions from eight dolphins were sampled and examined histologically. This sampling and analysis limitation will be addressed in future studies to increase the number of confirmed cases. Additionally, adaptations to the gross description assessment matrix to encompass other descriptors for trauma, such as HI-related trauma, would provide reviewers with descriptors that improve documentation of traumatic skin defects and progress in wound healing.
Several recommendations are provided here, based on these results, to improve stranding investigations. When recovering cetacean carcasses, take a complete set of external photographs before overnight storage to prevent artifactual changes caused by decomposition, handling, and storage. Photographs should be taken, in duplicate, after the carcass has been examined for ectoparasites, thoroughly rinsed, and dried to reduce glare. The initial images should capture the external details in situ without obstruction or annotation. Within the view frame, subsequent images should include a measuring scale, animal identifier, and unique lesion identifier (e.g., Lesion-A, Lesion-B, etc.) corresponding to its descriptions in the necropsy notes. Stranding agencies prefer external lesions be notated with alphabetical identifiers (Lesion A) and internal lesions with numerical identifiers (Lesion 1). To conform with this widely accepted protocol, it is recommended for future studies to adopt this nomenclature for unique lesion identifier. The unique lesion identifier should also accompany the histologic and ancillary diagnostic samples to ensure continuity of findings between gross and histologic observations and etiology determination. In this retrospective study, the texture of lesions was difficult to determine from photographs, which may be a limitation when using this matrix for assessing skin lesions on free-ranging animals.
The use of standardized descriptions provides robust information for repeatable analysis and examination of lesions for temporospatial changes during epidemiologic studies. External examination is a critical first step in a postmortem examination. By beginning with a thorough external examination and including the methods developed in this study, stranding network partner agencies will be able to comprehensively understand the factors contributing to cetacean morbidity (Bossart et al., 2017; Correia et al., 2023).
Standardizing data collection will increase the effectiveness of monitoring, identifying, and mitigating threats to marine mammal health. Collaboration among investigators involved in post-mortem examinations and in visual and physical health assessment projects will be necessary to incorporate descriptions of skin lesions in an already multidisciplinary protocol (involving biologists, veterinary clinicians, pathologists, and epidemiologists) designed to elucidate the relationship between various skin lesions, cetacean morbidity, and ecosystem health. Combining data sets obtained from post-mortem examinations and health assessments for a given population would yield the most accurate conclusions regarding skin disorders as an indicator of population health (Hart et al., 2011; Hart et al., 2012; Hupman et al., 2017; Toms et al., 2020; Correia et al., 2023). Therefore, future use of this assessment matrix will improve the collection of baseline data and enhance research on cetacean strandings in other regions by incorporating the use of a standardized matrix that facilitates consistent data collection in disease investigation.
Data availability statement
The original contributions presented in the study are included in the article/Supplementary Material. Further inquiries can be directed to the corresponding authors.
Ethics statement
Ethical approval was not required for the study involving animals in accordance with the local legislation and institutional requirements because Research was conducted on deceased animals that were salvaged for post mortem examination.
Author contributions
RE: Conceptualization, Formal analysis, Investigation, Supervision, Visualization, Writing – original draft, Writing – review & editing. MNS: Conceptualization, Data curation, Formal analysis, Methodology, Project administration, Validation, Writing – original draft, Writing – review & editing. HH: Conceptualization, Data curation, Investigation, Methodology, Validation, Writing – review & editing. MRS: Data curation, Investigation, Methodology, Validation, Writing – review & editing. DB: Data curation, Formal analysis, Methodology, Writing – review & editing. JR: Methodology, Supervision, Writing – review & editing. DR: Data curation, Formal Analysis, Writing – review & editing.
Funding
The author(s) declare financial support was received for the research, authorship, and/or publication of this article. The stranding response and sample analysis that allowed this data collection was funded by two grant programs, NOAA’s John H. Prescott Marine Mammal Rescue Assistance Grant Program (awards NA14NMF4390180 and NA17NMF4390088) and the National Fish and Wildlife Foundation. Sample analysis (award NFWF#45897) and contributions provided by coauthor RE were provided in fulfillment of mission directives and generously contributed by the U.S. Department of Commerce, NOAA, NMFS, Southeast Fisheries Science Center, Marine Mammal and Turtle Division.
Acknowledgments
We would like to thank the Florida Fish and Wildlife Conservation Commission’s (FWC) Fish and Wildlife Research Institute for supporting this research, specifically, FWC’s Southwest Field Lab for the use of their dolphin stranding data and the staff, interns, and volunteers who contributed to the collections. The FWC is authorized under the Marine Mammal Protection Act, section 109(h), to conduct stranding response activities. Additional thanks go to Timothy Morgan, DVM, Ph.D., Dipl ACVP, and Pádraig J. Duignan, DVM, Ph.D., for their essential roles in developing the matrix implemented and further developed in this project and for their review of the manuscript. We extend our sincere appreciation to Adam M. Schaefer and the reviewers for their review, feedback, and overall improvement of this manuscript.
Conflict of interest
The authors declare that the research was conducted in the absence of any commercial or financial relationships that could be construed as a potential conflict of interest.
The author(s) declared that they were an editorial board member of Frontiers, at the time of submission. This had no impact on the peer review process and the final decision.
Publisher’s note
All claims expressed in this article are solely those of the authors and do not necessarily represent those of their affiliated organizations, or those of the publisher, the editors and the reviewers. Any product that may be evaluated in this article, or claim that may be made by its manufacturer, is not guaranteed or endorsed by the publisher.
Author disclaimer
The opinions or assertions presented are those of the authors and are not to be construed as the official position of the U.S. Department of Commerce, NOAA, NMFS.
Supplementary material
The Supplementary Material for this article can be found online at: https://www.frontiersin.org/articles/10.3389/fmars.2023.1269075/full#supplementary-material
References
Blanchard T. W., Santiago N. T., Lipscomb T. P., Garber R. L., McFee W. E., Knowles S. (2001). Two novel alphaherpesviruses associated with fatal disseminated infections in Atlantic bottlenose dolphins. J. Wildl. Dis. 37 (2), 297–305. doi: 10.7589/0090-3558-37.2.297
Bossart G. D. (2011). Marine mammals as sentinel species for oceans and human health. Vet. Pathol. 6 (3), 676–690. doi: 10.1177/0300985810388525
Bossart G. D., Duignan P. J. (2018). Emerging viruses in marine mammals. CAB Rev. 13 (052), 1–17. doi: 10.1079/PAVSNNR201813052
Bossart G. D., Fair P., Schaefer A., Reif J. (2017). Health and environmental risk assessment project for bottlenose dolphins Tursiops truncatus from the southeastern USA. I. Infectious diseases. Dis. Aquat. Org. 125, 141–153. doi: 10.3354/dao03142
Bossart G. D., Meisner R., Varela R., Mazzoli M., McCulloch S. D., Kilpatrick D., et al. (2003). Pathologic findings in stranded Atlantic bottlenose dolphins (Tursiops truncatus) from the Indian River Lagoon, Florida. Fla. Sci., 6 (3), 226–238.
Buczinski S., Boccardo A., Pravettoni D. (2021). Clinical scores in veterinary medicine: What are the pitfalls of score construction, reliability, and validation? A general methodological approach applied in cattle. Animals 11 (11), 3244. doi: 10.3390/ani11113244
Colbert A. A., Scott G. I., Fulton M. H., Wirth E. F., Daugomah J. W., Key P. B., et al. (1999). Investigation of unusual mortalities of bottlenose dolphins along the mid-Texas coastal bay ecosystem during 1992 (Charleston, NC: NOAA Technical Report NMFS 147), 25pp.
Correia A. M., Dietterle E., Dinis A., Alves F. (2023). Defining a common language to assess external deformities in free-ranging cetaceans. Mammal Rev. 53, 189–205. doi: 10.1111/mam.12318
Daura-Jorge F., Simões-Lopes P. (2011). Lobomycosis-like disease in wild bottlenose dolphins Tursiops truncatus of Laguna, southern Brazil: Monitoring of a progressive case. Dis. Aquat. Org. 93 (2), 163–170. doi: 10.3354/dao02291
de Vet H. C. W., Mokkink L. B., Terwee C. B., Hoekstra O. S., Knol D. L. (2013). Clinicians are right not to like Cohen’s. BMJ 346 (f2125), 1–7. doi: 10.1136/bmj.f2125
Dierauf L., Gulland F. M. D. (2001). CRC handbook of marine mammal medicine: Health, disease, and rehabilitation. 2nd ed (Boca Raton, Florida: CRC Press).
Dorland W. A. N. (2011). Dorland’s illustrated medical dictionary. 30th ed (Philadelphia, PA: W. B. Saunders Co).
Duignan P. J., Stephens N. S., Robb K. (2020). Fresh water skin disease in dolphins: A case definition based on pathology and environmental factors in Australia. Sci. Rep. 10 (1), 1–17. doi: 10.1038/s41598-020-78858-2
Durden W. N., St. Leger J., Stolen M., Mazza T., Londono C. (2009). Lacaziosis in bottlenose dolphins (Tursiops truncatus) in the Indian River Lagoon, Florida, USA. J. Wildl. Dis. 45 (3), 849–856. doi: 10.7589/0090-3558-45.3.849
Ewing R. Y., Mase-Guthrie B., McFee W., Townsend F., Manire C. A., Walsh M., et al. (2017). Evaluation of serum for pathophysiological effects of prolonged low salinity water exposure in displaced bottlenose dolphins (Tursiops truncatus). Front. Vet. Sci. 4. doi: 10.3389/fvets.2017.00080
Fazioli K., Mintzer V. (2020). Short-term effects of Hurricane Harvey on bottlenose dolphins (Tursiops truncatus) in Upper Galveston Bay, TX. Estuar. Coast. 43 (5), 1013–1031. doi: 10.1007/s12237-020-00751-y
Gamer M., Lemon J., Fellows I., Singh P. (2019). irr: Various coefficients of interrater reliability and agreement (R package version 0.84.1) (Vienna: R Foundation). Available at: https://CRAN.R-project.org/package=irr.
Geraci J. R., Hicks B. D., Aubin D. J. S. (1979). Dolphin pox: A skin disease of cetaceans. Can. J. Comp. Med. 43 (4), 399–404.
Geraci J. R., Lounsbury V. J. (1993). “Chapter 10, specimen and data collection,” in Marine mammals ashore: A field guide for strandings (College Station: Texas A&M University Sea Grant Publication), 175–228.
Hallgren K. A. (2012). Computing inter-rater reliability for observational data: An overview and tutorial. TQMP 8 (1), 23–34. doi: 10.20982/tqmp.08.1.p023
Hart L. B., Rotstein D. S., Wells R. S., Allen J., Barleycorn A., Balmer B. C., et al. (2012). Skin lesions on common bottlenose dolphins (Tursiops truncatus) from three sites in the northwest Atlantic, USA. PloS One 7 (3), e33081. doi: 10.1371/journal.pone.0033081
Hart L. B., Rotstein D. S., Wells R. S., Bassos-Hull K., Schwacke L. H. (2011). Lacaziosis and lacaziosis-like prevalence among wild, common bottlenose dolphins Tursiops truncatus from the west coast of Florida, USA. Dis. Aquat. Org. 95 (1), 49–56. doi: 10.3354/dao02345
Hoelzel A. R. (2002). Marine mammal biology: An evolutionary approach. 1st ed (Hoboken, NJ: John Wiley and Sons).
Houser D. S., Yeates L. C., Crocker D. E. (2011). Cold stress induces an adrenocortical response in bottlenose dolphins (Tursiops truncatus). J. Zoo Wildl. Med. 42 (4), 565–571. doi: 10.1638/2010-0121.1
Hupman K. E., Pawley M. D., Lea C., Grimes C., Voswinkel S., Roe W. D., et al. (2017). Viability of photo-identification as a tool to examine the prevalence of lesions on free-ranging common dolphins (Delphinus sp.). Aquat. Mamm. 43 (3), 264–278. doi: 10.1578/AM.43.3.2017.264
Koo D., Wharton M., Birkhead G. (1997). Case definitions for infectious conditions under public health surveillance. MMWR Recommend. Rep., 46(No. RR-10) (Atlanta, GA: Centers for Disease Control and Prevention), 1–55.
Laporta P., Fruet P. F., Siciliano S., Flores P. A. C., Loureiro J. D. (2016). Report of the Working Group on the Biology and Ecology of Tursiops truncatus in the Southwest Atlantic Ocean. LAJAM 11 (1-2), 62–70. doi: 10.5597/00216
Lauriano E. R., Silvestri G., Kuciel M., Żuwała K., Zaccone D., Palombieri D., et al. (2014). Immunohistochemical localization of toll-like receptor 2 in skin Langerhans’ cells of striped dolphin (Stenella coeruleoalba). Tissue Cell 46 (2), 113–121. doi: 10.1016/j.tice.2013.12.002
Maldini D., Riggin J., Cecchetti A., Cotter M. P. (2010). Prevalence of epidermal conditions in California coastal bottlenose dolphins (Tursiops truncatus) in Monterey Bay. AMBIO 39 (7), 455–462. doi: 10.1007/s13280-010-0066-8
McHugh M. L. (2012). Interrater reliability: The kappa statistic. Biochem. Med. (Zagreb) 27 (3), 276–282. doi: 10.11613/BM.2012.031
Moore M., van der Hoop J., Barco S., Costidis A., Gulland F., Jepson P., et al. (2013). Criteria and case definitions for serious injury and death of pinnipeds and cetaceans caused by anthropogenic trauma. Dis. Aquat. Org. 103 (3), 229–264. doi: 10.3354/dao02566
Mullin K. D., Barry K., Sinclair C., Litz J., Maze-Foley K., Fougeres E., et al. (2015). Common bottlenose dolphins (Tursiops truncatus) in Lake Pontchartrain, Louisiana 2007 to mid-2014 (Pascagoula, MS: NOAA Technical Memorandum NMFS-SEFSC-673). 43pp. doi: 10.7289/v51c1tt8
Murdoch M., Mazzoil M., McCulloch S., Bechdel S., O’Corry-Crowe G., Bossart G., et al. (2010). Lacaziosis in bottlenose dolphins Tursiops truncatus along the coastal Atlantic Ocean, Florida, USA. Dis. Aquat. Org. 92 (1), 69–73. doi: 10.3354/dao02282
Murdoch M. E., Reif J. S., Mazzoil M., McCulloch S. D., Fair P. A., Bossart G. D. (2008). Lobomycosis in bottlenose dolphins (Tursiops truncatus) from the Indian River Lagoon, Florida: Estimation of prevalence, temporal trends, and spatial distribution. EcoHealth 5 (3), 289–297. doi: 10.1007/s10393-008-0187-8
Ramos E., Castelblanco-Martínez D., Garcia J., Rojas Arias J., Foley J., Audley K., et al. (2018). Lobomycosis-like disease in common bottlenose dolphins Tursiops truncatus from Belize and Mexico: Bridging the gap between the Americas. Dis. Aquat. Org. 128 (1), 1–12. doi: 10.3354/dao03206
Rehtanz M., Bossart G. D., Fair P. A., Reif J. S., Ghim S., Jenson A. B. (2012). Papillomaviruses and herpesviruses: Who is who in genital tumor development of free-ranging Atlantic bottlenose dolphins (Tursiops truncatus)? Vet. Microbiol. 160 (3–4), 297–304. doi: 10.1016/j.vetmic.2012.05.042
Reif J. S., Mazzoil M. S., McCulloch S. D., Varela R. A., Goldstein J. D., Fair P. A., et al. (2006). Lobomycosis in Atlantic bottlenose dolphins from the Indian River Lagoon, Florida. J. Am. Vet. Med. Assoc. 228 (1), 104–108. doi: 10.2460/javma.228.1.104
Reif J. S., Peden-Adams M. M., Romano T. A., Rice C. D., Fair P. A., Bossart G. D. (2009). Immune dysfunction in Atlantic bottlenose dolphins (Tursiops truncatus) with lobomycosis. Med. Mycol. J. 47 (2), 125–135. doi: 10.1080/13693780802178493
Sarasota Dolphin Research Program. (2006). Manual for field research and laboratory activities (Sarasota, FL: Sarasota Dolphin Research Program). Available at: www.sarasotadolphin.org.
Schulman F. Y., Lipscomb T. P. (1999). Dermatitis with invasive ciliated protozoa in dolphins that died during the 1987–1988 Atlantic bottlenose dolphin morbilliviral epizootic. Vet. Pathol. 36 (2), 171–174. doi: 10.1354/vp.36-2-171
Segura-Göthlin S., Fernández A., Arbelo M., Felipe-Jiménez I., Colom-Rivero A., Almunia J., et al. (2021). The validation of a non-invasive skin sampling device for detecting cetacean poxvirus. Animals 11, 2814. doi: 10.3390/ani11102814
Simpson J. G., Gardner M. B. (1972). “Comparative microscopic anatomy of selected marine mammals,” in Mammals of the sea: Biology and medicine. Ed. Ridgway S. H. (Springfield, IL: Charles C. Thomas), 298–418.
Smolarek Benson K. A., Manire C. A., Ewing R. Y., Saliki J. T., Townsend F. I., Ehlers B., et al. (2006). Identification of novel alpha- and gammaherpesviruses from cutaneous and mucosal lesions of dolphins and whales. J. Virol. Methods 136 (1–2), 261–266. doi: 10.1016/j.jviromet.2006.03.033
Toms C. N., Stone T., Och-Adams T. (2020). ). Visual-only assessments of skin lesions on free-ranging common bottlenose dolphins (Tursiops truncatus): Reliability and utility of quantitative tools. Mar. Mamm. Sci. 36 (3), 744–773. doi: 10.1111/mms.12670
Townsend F. I., Staggs L. (2020). Atlas of skin diseases of small cetaceans. Eds. Townsend F. I. Jr., Staggs L., Forrest I. (Townsend: Fort Walton Beach, FL).
Van Bressem M., Raga A., Di Guardo G., Jepson P., Duignan P., Siebert U., et al. (2009a). Emerging infectious diseases in cetaceans worldwide and the possible role of environmental stressors. Dis. Aquat. Org. 86, 143–157. doi: 10.3354/dao02101
Van Bressem M., Van Waerebeek K., Aznar F., Raga J., Jepson P., Duignan P., et al. (2009b). Epidemiological pattern of tattoo skin disease: A potential general health indicator for cetaceans. Dis. Aquat. Org. 85, 225–237. doi: 10.3354/dao02080
Van Bressem M. F., Van Waerebeek K., Reyes J. C., Fernando F., Echegaray M., Siciliano S., et al. (2007). A preliminary overview of skin and skeletal diseases and traumata in small cetaceans from South American waters. Lat. Am. J. Aquat. Mamm. 6 (1), 7–42. doi: 10.5597/lajam00108
van Elk C. E., van de Bildt M. W., de Jong A. A., Osterhaus A. D., Kuiken T. (2009). Genital herpesvirus in bottlenose dolphins (Tursiops truncatus): cultivation, epidemiology, and associated pathology. J. Wildl. Dis. 45, 895–906. doi: 10.7589/0090-3558-45.4.895
Vilela R., Bossart G. D., St. Leger J. A., Dalton L. M., Reif J. S., Schaefer A. M., et al. (2016). Cutaneous granulomas in dolphins caused by novel uncultivated Paracoccidioides brasiliensis. Emerg. Infect. Dis. 22 (12), 2063–2069. doi: 10.3201/eid2212.160860
Vilela R., Huebner M., Vilela C., Vilela G., Pettersen B., Oliveira C., et al. (2021). The taxonomy of two uncultivated fungal mammalian pathogens is revealed through phylogeny and population genetic analyses. Sci. Rep. 11, 18119. doi: 10.1038/s41598-021-97429-7
Wells R. S., Rhinehart H. L., Hansen L. J., Sweeney J. C., Townsend F. I., Stone R., et al. (2004). Bottlenose dolphins as marine ecosystem sentinels: Developing a health monitoring system. EcoHealth 1 (3), 246–254. doi: 10.1007/s10393-004-0094-6
Wickham H., François R., Henry L., Müller K. (2019). dplyr: A grammar of data manipulation. (R package version 0.8.3) (Vienna: R Foundation). Available at: https://CRAN.R-project.org/package=dplyr.
Wilson B., Arnold H., Bearzi G., Fortuna C. M., Gaspar R., Ingram S., et al. (1999). Epidermal diseases in bottlenose dolphins: Impacts of natural and anthropogenic factors. Proc. R. Soc. London. Ser. B: Biol. Sci. 266 (1423), 1077–1083. doi: 10.1098/rspb.1999.0746
Keywords: common bottlenose dolphin, sentinel species, skin disease, strandings, morbidity, marine mammal health
Citation: Ewing RY, Sutton MN, Herring HM, Schubert MR, Boyd DM, Richardson JL and Rotstein DS (2023) Standardizing gross descriptions of skin lesions in common bottlenose dolphins (Tursiops truncatus) stranded in Southwest Florida, 2015–2019. Front. Mar. Sci. 10:1269075. doi: 10.3389/fmars.2023.1269075
Received: 29 July 2023; Accepted: 26 October 2023;
Published: 21 November 2023.
Edited by:
Ewa Krzyszczyk, Bangor University, United KingdomReviewed by:
Salvatore Siciliano, Fundação Oswaldo Cruz (Fiocruz), BrazilLonneke Liza Ijsseldijk, Utrecht University, Netherlands
Copyright © 2023 Ewing, Sutton, Herring, Schubert, Boyd, Richardson and Rotstein. This is an open-access article distributed under the terms of the Creative Commons Attribution License (CC BY). The use, distribution or reproduction in other forums is permitted, provided the original author(s) and the copyright owner(s) are credited and that the original publication in this journal is cited, in accordance with accepted academic practice. No use, distribution or reproduction is permitted which does not comply with these terms.
*Correspondence: Meghan N. Sutton, bWVnaGFuLnN1dHRvbjAyQGdtYWlsLmNvbQ==; Ruth Y. Ewing, UnV0aC5Fd2luZ0BOT0FBLmdvdg==
†These authors have contributed equally to this work and share first authorship