- 1South China Sea Ecological Center, Ministry of Natural Resources of the People’s Republic of China, Guangzhou, China
- 2Nansha lslands Coral Reef Ecosystem National Observation and Research Station, Guangzhou, China
- 3Key Laboratory of Marine Environmental Survey Technology and Application, Ministry of Natural Resources of the People’s Republic of China, Guangzhou, China
- 4Research Center for Eco-Environmental Sciences, Chinese Academy of Sciences, Beijing, China
- 5College of Urban and Environmental Sciences, Northwest University, Xi’an, China
Introduction: Accurate species identification and biodiversity assessment of larval fish are essential for effective management and conservation of fisheries resources, as it allows for the estimation of parent stocks, assessment of future recruitment, and prediction of spawning and nursing grounds. However, traditional morphology-based identification methods have inherent limitations, highlighting the need for accurate and efficient techniques to address these challenges effectively. The Luzon Strait, a crucial channel connecting the South China Sea to Western Pacific Ocean, is renowned for its fish biodiversity. However, our knowledge of the biodiversity status of larval fish species in this region remains insufficient.
Methods: Here we employed DNA barcoding to assess larval fish species diversity in the Luzon Strait and adjacent waters. We investigated the species composition, diversity, and geographical distribution of larval fish communities in the region. Moreover, we assessed habitat types, human uses, and IUCN conservation status of each larval fish species.
Results: A total of 385 larval fish individuals were collected from 15 stations, and 354 individuals were successfully barcoded and identified, representing 147 species from 93 genera, 44 families, and 22 orders. The interspecific Kimura 2-parameter (K2P) divergence exhibited a significant increase of approximately 55-fold higher than intraspecific divergence. The phylogenetic neighbor-joining tree confirmed the distinct lineages for each taxonomic level, demonstrating the feasibility of DNA barcoding. We observed notable variations in fish species diversity and community composition among sampling stations. Non-metric multidimensional scaling analysis revealed greater diversity and dissimilarity of larval fish community compositions in the western regions compared to the eastern regions. This pattern corresponded to the grouping based on the path of the Kuroshio current, suggesting its influence on the fish community structure. Additionally, economically valuable species were identified at these stations, highlighting their ecological significance as potential spawning or nursery grounds for larval fish. We also examined the habitat type, human use, and conservation status of each larval fish species, providing comprehensive insights into their ecological significance and conservation needs.
Discussion: The establishment of a local DNA sequences database through DNA barcoding will greatly enhance the accuracy of species identification in environmental DNA (eDNA) metabarcoding applications. Altogether, this study offers valuable information for identifying important spawning and nursing grounds of fish populations, thereby supporting sustainable management and conservation of fisheries resources in this region.
Introduction
Fishes account for approximately 50% of marine vertebrate species, serving as a key component of biodiversity in global marine ecosystems. Larval fish can provide the fundamental source for the replenishment and sustainable utilization of fish stocks (Panprommin et al., 2020; Panprommin et al., 2021; Huang et al., 2022). Accurate identification and classification of larval fish species are crucial for effective fisheries management, as it enables the estimation of parent stocks and future recruitment, as well as the prediction of important spawning and nursing grounds (Baumgartner et al., 2004; Xing et al., 2022). Traditional morphological identification methods based on morphological features such as spination, body shape, fin placement, and photophores often yield misidentification due to distinct characteristics between larval and adult fish and developmental variations (Azmir et al., 2017; Sheraliev and Peng, 2021). Furthermore, limited diagnostic characteristics hinder precise identification, especially for related taxa (Valdez-Moreno et al., 2010; Kwun, 2018). Moreover, the lack of larval fish taxonomists profoundly influences accurate identifications based on morphology (Azmir et al., 2017). Therefore, the inherent limitations of morphology-based identification systems underscore the necessity for accurate and efficient approaches to identify larval fish species (Zhang and Hanner, 2012).
Molecular methods, such as DNA barcoding, have become effective tools for accurate and rapid species determination, including fish (Zhan et al., 2008; Hubert et al., 2010; Hubert et al., 2015; Panprommin et al., 2021; Huang et al., 2022). DNA barcoding utilizes a standardized DNA region, such as the mitochondrial cytochrome c oxidase subunit I (COI) gene, to identify species based on their unique genetic sequences. It has been successfully applied to identify incomplete or immature fish samples, such as fish eggs (Hou et al., 2022), larval fish (Azmir et al., 2017), and seafood products (Shehata et al., 2018). Compared to traditional morphological identification methods for larval fish species, DNA barcoding provides advantages in terms of efficiency, repeatability, and cost-effectiveness (Azmir et al., 2017). Additionally, online DNA barcode databases, such as the Barcode of Life Data System (BOLD) and the National Center for Biotechnology Information (NCBI), offer valuable resources for species annotation. These databases provide extensive reference sequences from diverse fish species, encompassing different taxonomic groups and geographic regions, facilitating reliable comparison and identification of unknown samples. These databases also provide additional information on geographical distribution, ecological traits, and morphological characteristics, which would enhance our understanding of the biology and ecology of the identified species. Environmental DNA (eDNA) metabarcoding has recently emerged as a revolutionary tool for monitoring fish biodiversity (Yao et al., 2022; Zhang et al., 2022). Nonetheless, a critical challenge arises when utilizing public databases in fish eDNA metabarcoding, leading to increased potential for ambiguous species identification or false positives. In contrast, the use of local databases can significantly enhance the efficiency of species identification, ensuring identification at the species level (Yao et al., 2022). Thus, conducting DNA barcoding analysis of larval fish in specific sea areas provides valuable insights into fish biodiversity, population dynamics, and ecological interactions in marine ecosystems, contributing to the exploration, management, and conservation of fisheries resources (Hubert et al., 2015; Yi et al., 2017).
The Luzon Strait, located between the Luzon Island in the Philippines and Taiwan, serves as a vital channel connecting the South China Sea to Western Pacific Ocean. Recognized as a hotspot for marine biodiversity, this strait holds significant ecological importance. It encompasses diverse habitats such as open ocean waters, coral reefs, seagrass beds, and mangrove forests (Gu et al., 2019). The Luzon Strait is home to a variety of coral reef-associated fish species, including snappers (e.g., Lutjanus erythropterus and L. madras), wrasses (e.g., Thalassoma amblycephalum and T. quinquevittatum), and groupers (e.g., Epinephelus lanceolatus and E. fasciatus) (Huang et al., 2015). It also supports commercially important fish species, such as skipjack tuna (Katsuwonus pelamis), yellowfin tuna (Thunnus albacares), bigeye tuna (Thunnus obesus), as well as various groupers and mackerels, highlighting its significance for fisheries (Zhang and Hanner, 2012; Xu et al., 2021). However, limited information is available regarding the larval fish diversity in this region. The Luzon Strait experiences high oceanographic variability influenced by multiple oceanic currents, eddies, and fronts. Particularly, the Kuroshio current originating from the Western North Pacific brings warm, salty, and nutrient-rich waters into the strait, significantly affecting its hydrological dynamics and fish diversity (Gu et al., 2019; Zhang et al., 2022). However, the region is facing ecological and environmental challenges due to frequent anthropogenic disturbances such as overfishing and pollution (Chang et al., 2017; Hou et al., 2018). These activities have altered fish community structures and resulted in the decline of fish resources (Olden et al., 2007; Chang et al., 2017). The destruction of habitats, including fish spawning and nursing grounds, further threatens the sustainability of marine fish populations and ecosystems (Olden et al., 2007). Therefore, monitoring the composition, diversity, and dynamics of larval fishes in the Luzon Strait and its surrounding waters is crucial for identifying potential threats and implementing effective conservation strategies.
In this study, we utilized DNA barcoding to identify larval fish species collected from stations in the Luzon Strait and adjacent waters. The aim of this study is to assess the species composition, diversity, and geographical distribution of the larval fish community in this region. Additionally, we investigated the habitat type, human use, and IUCN conservation status of each larval fish species. Our findings are expected to complement the current fish database of the Luzon Strait and surrounding waters, and further contribute to identifying important spawning and nursing grounds of fish populations, thereby supporting sustainable fisheries resource management as well as implementing conservation programs.
Materials and methods
Ethics statement
The requirement of ethical approval was waived by the Animal Experiment Administration Committee of South China Sea Ecological Center, Ministry of Natural Resources of the People’s Republic of China. The studies were conducted in accordance with the local legislation and institutional requirements.
Sample collection
In May 2016, we collected larval fish specimens from 15 stations in the Luzon Strait and adjacent waters (Figure 1; Table S1). To capture a wide variety of fish species, we employed both vertical and horizontal trawls to collect larval fish individuals by using a zooplankton net with a length of 2.8 m, bore diameter of 80 cm, and mesh size of 0.505 mm. The nets were flushed repeatedly from top to bottom to collect the larval fish individuals. For vertical trawls, nets were lowered down to approximately the seabed and dragged with a speed of 0.5-0.8 m/s. For horizontal trawls, nets were towed for 15 min with a speed of 1.0-2.0 knots/h at each station. After sampling, all specimens were immediately preserved in 95% ethanol and transported to the laboratory for subsequent analyses.
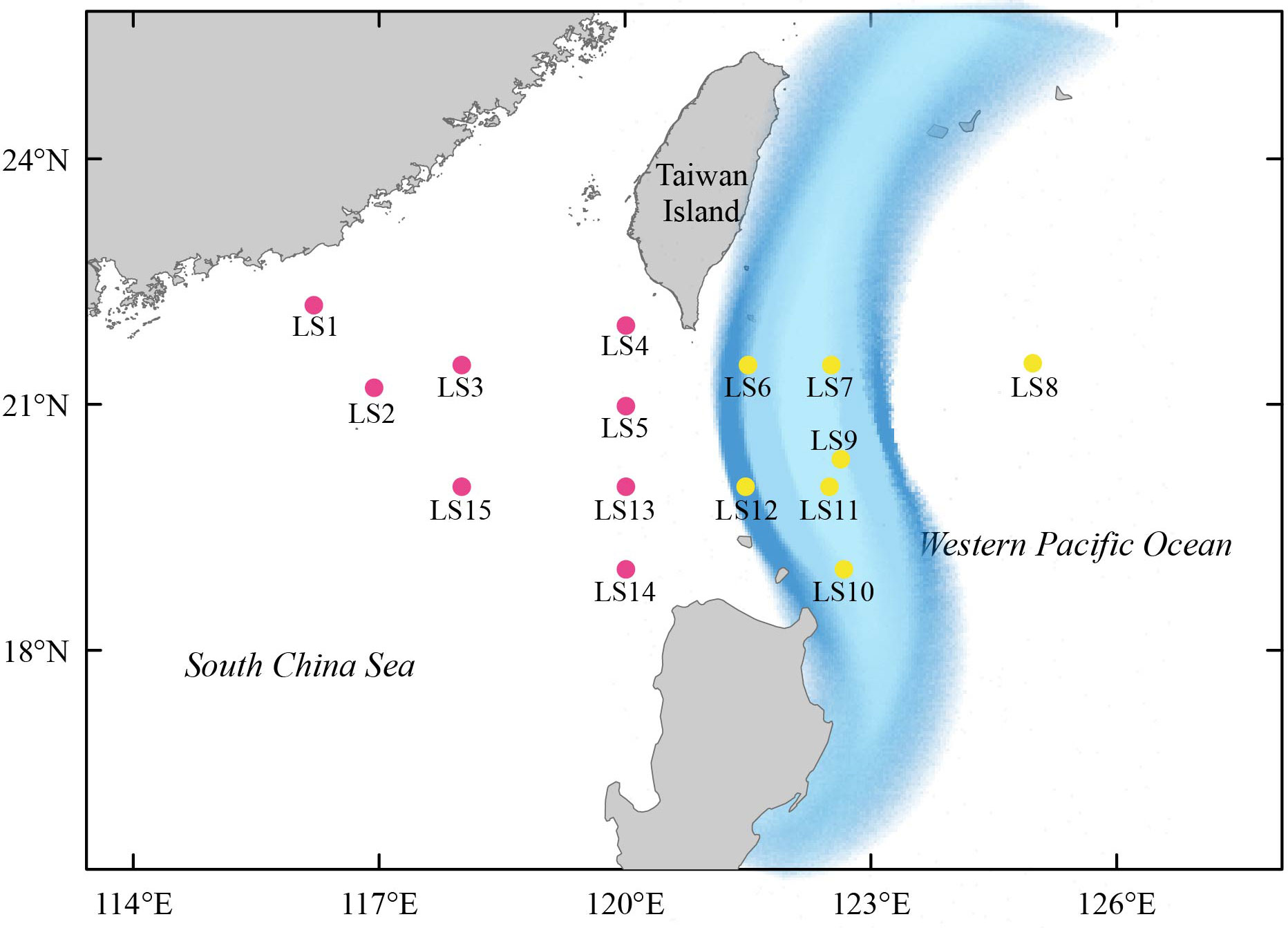
Figure 1 The map of 15 stations in the Luzon Strait and its surrounding areas where larval fish samples were collected. The path of the Kuroshio Current was approximately shown by light blue shading regions. The colored stations were corresponding to their assigned clusters as shown in Figure 6.
DNA extraction, PCR amplification and sequencing
The genomic DNA of each larval fish individual was extracted from the muscle tissues using the DNeasy Blood and Tissue Kit (QIAGEN), and the quantity and quality of extracted DNA were subsequently measured using a NanoDrop 2000 spectrophotometer (Thermo Fisher Scientific, USA). We chose the cytochrome c oxidase subunit I (COI) mitochondrial gene for fish DNA barcoding. Sequences of approximately 650 bp within the 5’ region of the COI gene were amplified using the universal primers FishF1 [5′-TCAACCAACCACAAAGACATTGGCAC-3′] and FishR1 [5′-TAGACTTCTGGGTGGCCAAAGAATCA-3′] (Ward et al., 2005). Polymerase chain reaction (PCR) amplifications were performed in a volume of 25 μL, containing 2.5 μL 10 X PCR buffer+, 2.0 μL of dNTPs (10 mM each), 1.0 μL of each primer (0.01 mM), 0.5 μL of ExTaq polymerase, 1.0 μL of template DNA (approximately 100 ng), and 18 μL ultrapure water. The PCR amplification procedures consisted of an initial denaturation step at 95 °C for 5 min, followed by 35 cycles of 95°C for 30 s, 52°C for 30 s, and 72°C for 60 s, with a final elongation at 72°C for 10 min. All PCR products were visualized on a 1.2% agarose gel, and those successfully amplified PCR products were sequenced bidirectionally with BigDye Terminator Cycle Sequencing chemistry on an Applied Biosystems 3730XL automated DNA analyzer (Applied Biosystems, USA).
Data analysis
The tracer files of sequencing samples were initially checked and edited, and the raw DNA sequences were assembled using the Lasergene package in the DNAStar software. The high-quality sequences were aligned and edited using BioEdit v.7.0.5.3 (Hall, 1999) and MEGA v.7.0 (Kumar et al., 2016). The obtained sequences were searched against two databases, Barcode of Life Data System (BOLD, http://www.boldsystems.org/) and GenBank (https://www.ncbi.nlm.nih.gov/) for species identification. We set a sequence similarity of 98% as a threshold for the species level annotation (Hou et al., 2021). In addition, the sequence with an identity from 95% to 98% matched to the database sequence was classified at the genus level, and a lower similarity than 95% was considered unidentified. The sequences were trimmed to equal length and formatted into a single fasta format using BioEdit, and subsequently arranged using ClustalW in MEGA. Intraspecific and interspecific genetic distances were calculated using the Kimura 2-parameter (K2P) distance model, and the phylogenetic tree was subsequently constructed by the neighbor-joining (NJ) method using the K2P model. The K2P distances and NJ trees were estimated with 1,000 bootstrap replicates in MEGA (Kumar et al., 2016). The NJ tree was visualized, edited, and annotated using interactive tree of life (iTOL, https://itol.embl.de/) (Letunic et al., 2021).
Diversity and geographical distribution of larval fish
We first estimated taxonomic diversity of larval fish using the species richness (i.e., the number of fish), and calculated the occurrence frequency of each larval fish species at different stations using the R package BiodiversityR (Kindt and Coe, 2005). To further measure the differences in larval fish community compositions among different sites, we conducted the non-metric multidimensional scaling (NMDS) analysis based on the Bray-Curtis similarity (Xiong et al., 2017; Xiong et al., 2019; Yang et al., 2019b). In addition, the hydrodynamic process of the Luzon Strait is greatly controlled by the Kuroshio Current (Gu et al., 2019), which would be likely to affect the diversity and community composition of larval fish. We thus divided 15 stations into two clusters based on the path of the Kuroshio current. Cluster I included eight western stations (LS1, LS2, LS3, LS4, LS5, LS13, LS14 and LS15), while cluster II included seven eastern stations (LS6, LS7, LS8, LS9, LS10, LS11 and LS12). The significance of dissimilarity in species composition between two clusters was further assessed using the analysis of similarity (ANOSIM) test (Xiong et al., 2019; Yang et al., 2019a). Both the NMDS analysis and ANOSIM test were conducted using online Omicshare CloudTools (http://www.omicshare.com/tools).
The habitat, human uses, and conservation status
Each larval fish species was searched for the habitat as well as human uses based on the FishBase webpage (http://www.fishbase.org/), which is a global biodiversity information system on fishes. We downloaded the habitat (pelagic, demersal, bathypelagic, reef-associated, etc.) and human use (fisheries, aquaculture, aquariums, etc.) data for each target species. Furthermore, the conservation status of each species was determined according to the International Union for Conservation of Nature (IUCN) webpage (https://www.iucnredlist.org/).
Results
Species identification
A total of 385 larval fish individuals were collected from 15 stations in the Luzon Strait and surrounding waters, and 361 individuals (93.77%) were successfully barcoded. The remaining 24 individuals were excluded from subsequent analyses, as they failed to produce any PCR targeted product or high-quality sequence. After conducting sequence alignment, trimming, and removing noisy sites, we obtained 623 bp DNA barcodes for the 361 fish individuals. Through blast searches against both BOLD and GenBank databases, 347 larval sequences were successfully assigned to 131 taxa to the species level, using a similarity threshold of 98%. Sixteen larval sequences were determined to the genus level with similarities ranging from 95% to 98%, while the remaining seven individuals were unidentified with similarities lower than 95%. Overall, 354 larvae with high similarities belonged to 147 fish taxa, which were categorized into 22 orders, 44 families, and 93 genera (Table S2). Among the identified larval fish, the order Myctophiformes accounted for the highest quantity (31.07%) and variety (26.53%), followed by Stomiiformes (21.19% in quantity and 11.56% in variety) and Perciformes (13.56% in quantity and 19.05% in variety) (Figure 2). Additionally, six orders including Beryciformes, Centrarchiformes, Gadiformes, Gobiiformes, Lophiiformes, and Spariformes were represented by a single species (Figure 2). The family Myctophidae (110 individuals) and the genus Vinciguerria (54 individuals) were the most abundant, accounting for approximately 31% and 15% of all fish individuals, respectively (Table S2).
Based on the Kimura 2-parameter (K2P) distance, intraspecific genetic distances ranged from 0 to 3.98%, with an average value of 0.33% (Table S3). In contrast, interspecific genetic distances varied from 5.22% to 39.18%, with an average value of 18.03% (Table S3). These findings highlighted the increased genetic divergence observed between different species compared to within species. To further validate the accuracy of larval fish species identification, we constructed a phylogenetic NJ tree based on DNA barcode sequences. The tree revealed 147 independent lineages (Figure 3), providing additional support for the reliability of species identification achieved through the utilization of the BOLD and NCBI databases.
Species diversity and geographical distribution
The species richness varied across different stations, with the highest richness observed at the station LS12 (55 species), followed by LS8 (29 species) and LS10 (24 species). In contrast, LS1 exhibited the lowest number of species, comprising only two species (Figure 4). Vinciguerria nimbaria emerged as the most frequently occurring larval fish species, which was detected at 11 out of 15 stations. Furthermore, the relative abundance of the dominant orders displayed significant variability across different stations. The order Myctophiformes dominated over half of the stations, including LS2, LS3, LS4, LS5, LS6, LS7, LS11, LS12, LS13, LS14, and LS15. In addition, LS1, LS8, LS9, and LS10 were dominated by the Labriformes (~ 85%), Syngnathiformes (~ 34%), Clupeiformes (~ 58%), and Stomiiformes (~ 33%), respectively (Figure 5).
Based on the NMDS analysis, we observed the patterns of dissimilarity in larval fish community compositions among different stations. Notably, within the second cluster (II cluster) in the eastern regions, larval fish community compositions displayed higher similarities, as shown by relatively higher Bray-Curtis similarities in the NMDS plot (Figure 6). In contrast, the fish community compositions at stations in the western regions largely varied, with lower similarities and higher dissimilarities. There was no significant difference between the community compositions of the two clusters as indicated by the results of ANOSIM (R = 0.010, P = 0.450).
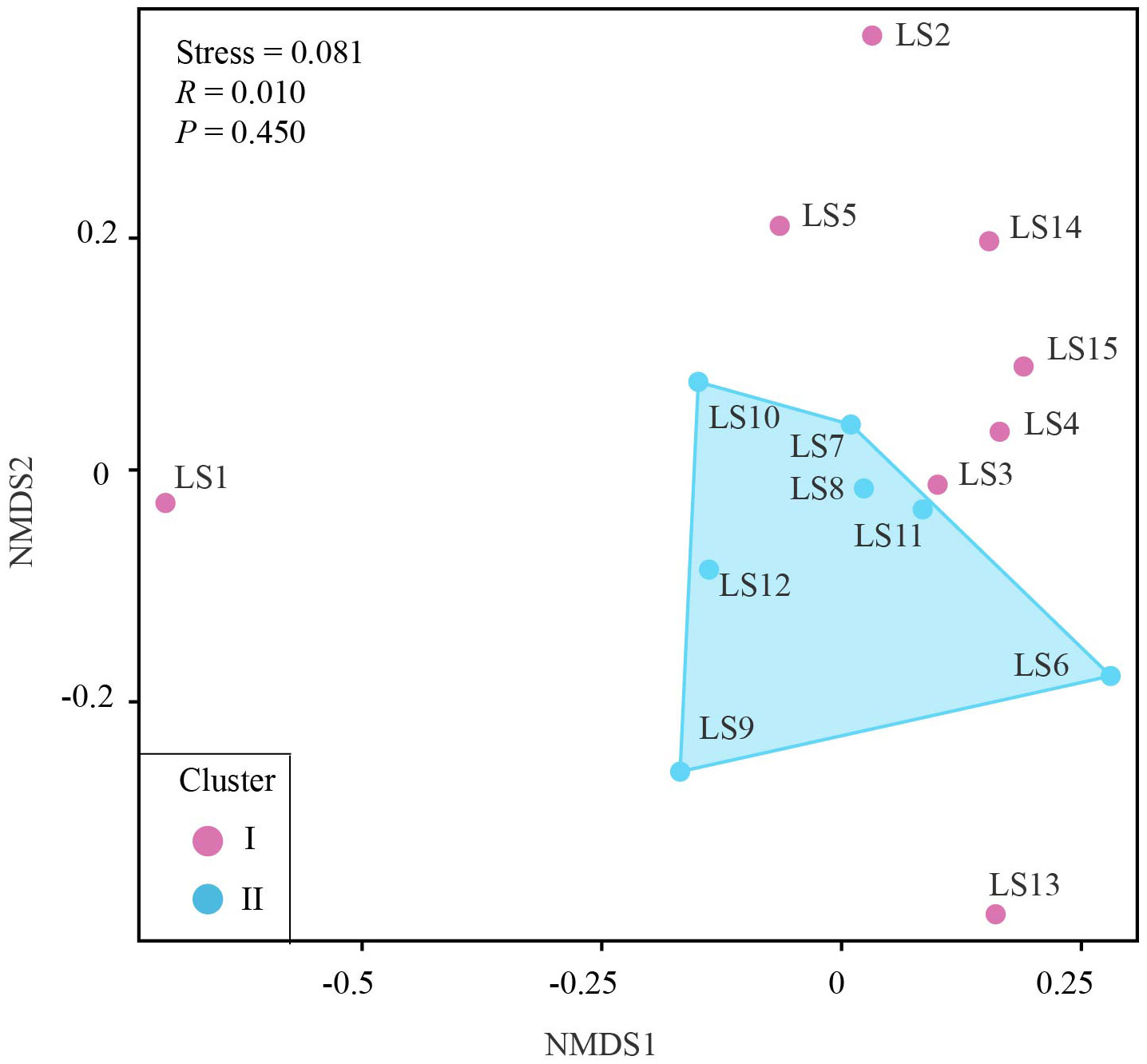
Figure 6 Nonmetric multidimensional scaling ordination (NMDS) based on the Bray-Curtis similarity of larval fish communities at each sampling station.
The habitat, human use, and IUCN status
In terms of the habitat of the identified fish species, they were categorized as follows: 36.64% were bathypelagic, 35.88% were reef-associated, 12.21% were pelagic-oceanic, 7.63% were demersal, 5.34% were benthopelagic, 1.53% were pelagic-neritic, and 0.76% were pelagic (Table S4; Figure 7). When assessing for human use, nearly half of the evaluated fish species were utilized in fisheries (49.62%), while 46.56% were not assessed (NA). Other types of human uses included aquariums (19.08%), gamefish (9.16%), bait (3.82%), and aquaculture (3.05%) (Figure 7). The remaining species (12.98%) were considered of no interest in fisheries. Among the recorded species, 35 were found to serve for multiple purposes, and Seriola dumerili was used for four purposes, including fisheries, aquaculture, gamefish, and aquariums (Table S4). The IUCN status of most species was determined to be least concern (LC, 84.73%), followed by not assessed (NA, 11.45%) and data deficient (DD, 2.29%). In addition, Istiophorus platypterus was designated as vulnerable (VU), and Xiphias gladius was categorized as near threatened (NT).
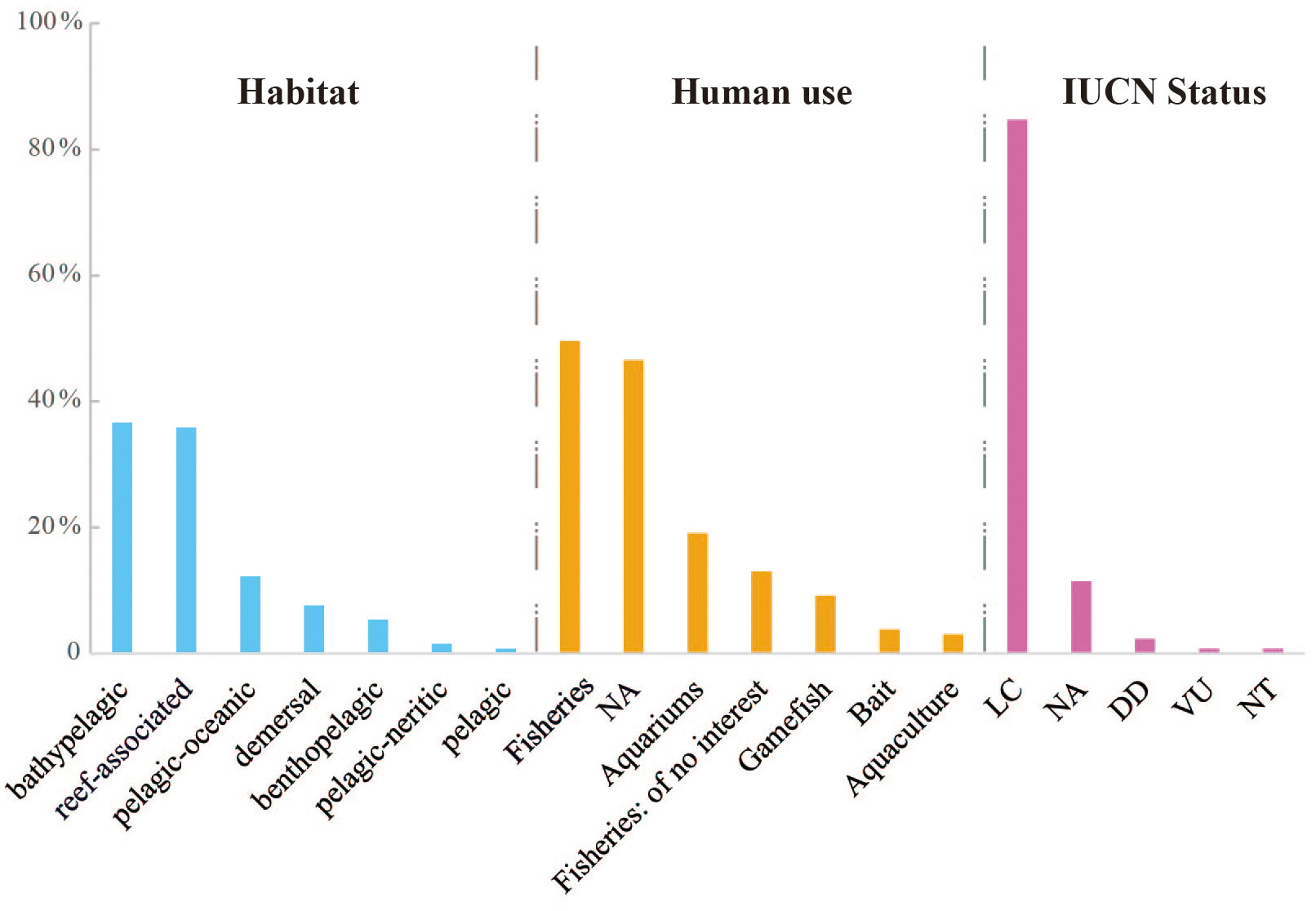
Figure 7 The percentage of fish species according to the habitat, human use and the International Union for Conservation of Nature (IUCN) status, respectively. The information of each species was obtained from the FishBase and IUCN webpages.
Discussion
In this study, we employed DNA barcoding to identify larval fish species collected from the Luzon Strait and adjacent waters. This region is home to diverse fish species, and accurate species identification at larval stages is crucial for understanding their ecology and population dynamics. The accurate determination of larval fish species solely depending on traditional morphology-based methods has proven to be challenging and time-consuming (Neira et al., 2015; Panprommin et al., 2021). However, recent advancements in molecular methods, such as DNA (meta)barcoding, have provided a powerful tool for species identification (Zhan and MacIsaac, 2015; Xiong et al., 2020). The COI gene used for DNA barcoding produced sequences with an average length of approximately 650 bp, which has been widely recognized for its efficacy in identifying larval fish species (Chang et al., 2017; Panprommin et al., 2021). Numerous studies have demonstrated the reliability and applicability of DNA barcoding for identifying larval fish species across various geographic regions, including the marine fish larval community in the South China Sea (Hou et al., 2021), larval fish in mangrove areas from Peninsular Malaysia (Azmir et al., 2017), and larval fish in tropical estuarine waters of Malacca Straits in Malaysia (Chu et al., 2019). The construction of a comprehensive local species reference database is of vital importance to ensure precise identification and complete coverage of fish diversity (Yao et al., 2022). In this study, we identified a set of larval fish in the Luzon Strait and adjacent waters, including 22 orders, 44 families, 93 genera, and 147 species. DNA barcoding allowed to delimit 96.12% of all larval fish sequences to the species level with a 98% similarity threshold. The intraspecific K2P divergence observed in this study was found to be approximately 0.33%, while the interspecific K2P divergence was approximately 18.03%. This represents an approximately 55-fold increase in genetic distance between species when compared to the average intraspecific genetic distances, which is consistent with the DNA barcoding principle that the interspecific divergence largely outscores the intraspecific divergence (Hebert et al., 2003). The constructed NJ tree based on the COI gene sequences also demonstrated that each taxonomic-level species was assigned to an independent lineage. These findings highlight the feasibility of using COI gene sequences as DNA barcodes for discriminating larval fish species in the Luzon Strait and adjacent waters.
We found that 39 of identified larval fish species (26.53%) were categorized into the order of Myctophiformes, which also emerged as the dominant order at more than half of the sampling stations. These findings indicate that this order is likely to be the dominant fish taxon in the Luzon Strait and adjacent waters. While Myctophiformes, specifically the lanternfishes, do not have high fisheries values, they are a significant component of deep-water fauna, which constitute a substantial and play a crucial role in the diet of high economic value species. Despite the ecological significance of Myctophiformes, there remains a lack of molecular studies focusing on their identification and classification (Poulsen et al., 2013; Pappalardo et al., 2015). The first attempt at lanternfish larvae identification using DNA barcoding was conducted in the Sicilian Channel of the Mediterranean Sea, where five Myctophidae species were identified from 20 lanternfish larvae fish individuals (Pappalardo et al., 2015). Similarly, a recent survey of larval fish in the South China Sea implemented DNA barcoding and revealed that the Myctophidae was one of the most abundant families (Hou et al., 2021).
Our study revealed notable variations in fish species diversity and community composition across different sampling stations. Cluster II exhibited higher species richness, with an average of 22 species, when compared with cluster I (average 11 species). Importantly, we identified several economically valuable species at these stations, including Abudefduf vaigiensis, Elagatis bipinnulata, Parupeneus barberinoides, Parupeneus heptacanthus, and Istiophorus platypterus. These findings indicate the ecological significance of these stations as potential spawning or nursing grounds. The presence of economically important species underscores the significance of protecting these areas to ensure the sustainability of fish populations and support fisheries management initiatives. The NMDS analysis in this study demonstrated that the larval fish community compositions in the western regions were more diverse and dissimilar compared to the eastern regions, as indicated by the higher dispersion of stations in the western region. Importantly, the two distinct patterns of larval fish communities observed in this study were consistent with the grouping results based on the Kuroshio current. Specifically, the cluster I, located in the western regions, was situated away from the path of the Kuroshio current. While the cluster II, located in the eastern regions, was situated within the path of the Kuroshio current. Understanding the impacts of oceanic currents can provides valuable insights into predicting and managing the fish diversity and community structures in the region, benefiting both conservation efforts and commercial fisheries (Zhan et al., 2009; Almany et al., 2017). Oceanic currents, such as the Kuroshio current, play a vital role in the transport of fish eggs and larvae, aiding their dispersal until they settle and develop into sedentary adult individuals (Chang et al., 2017; Xu et al., 2021). The Kuroshio current, originating from the western North Pacific, can bring warm, salty, and nutrient-rich waters into the strait, which is expected to cause a significant impact on the fish diversity and community structures in the Luzon Strait and surrounding waters. This inflow of nutrients sustains rich planktonic and benthic communities, providing food sources for a variety of fish species (Xu et al., 2021). The water temperature was different between northern and southern Taiwan, owing to three main ocean currents, including Kuroshio, China Coast and South China Sea (Chang et al., 2017). Consequently, the strait has become an important fishing ground for commercial fisheries. Furthermore, the Kuroshio current plays a significant role in creating different environmental conditions and habitats, which may support specific fish species with adaptive capacities to warm and high-salinity environments (Gu et al., 2019). The presence of certain species in cluster II indicates that larval fish community compositions, particularly in this cluster, are likely influenced by the Kuroshio current. For instance, Ceratoscopelus warmingii, also known as the warming’s lantern fish, is a dominant species among mesopelagic fishes and is known to thrive in warm and high-salinity habitats in tropical and subtropical waters (Kong et al., 2016; Zhang et al., 2021). Its consistent presence in approximately all stations of the cluster II (6 out of 7 stations) indicates that these stations are likely influenced by the Kuroshio current. The current provides warm, high-oxygenation, and high-salinity water conditions that are suitable for this species (Gu et al., 2019). In contrast, the most frequently occurring larval fish in stations of the cluster I was Vinciguerria nimbaria, an oceanic lightfish. This species was reported with rich biological production in low temperature environments, as they were supported by abundant zooplankton (Kim et al., 2023). The current can act as a transport mechanism for fish eggs and larvae, shape the diverse marine ecosystem by providing nutrients, and create distinct environmental conditions and habitats. Overall, these findings support that larval fish community compositions, especially in the cluster II, were likely influenced by the Kuroshio current.
The differences in fish habitat preferences observed in this study highlight the unique characteristics of the Luzon Strait and surrounding waters. In this study, the majority of fish species were found to prefer bathypelagic (36.64%) and reef-associated habitats (35.88%), while demersal-related species were less dominant, accounting for only 7.63% of all detected species. These findings contrast with a previous survey conducted in 230 sites in the South China Sea, where demersal-associated species were identified as the most dominant (37.62%), followed by reef-associated species (32.67%). The divergent habitat preferences observed could be attributed to several factors, including differences in oceanographic conditions, hydrological features, and local environmental factors specific to the Luzon Strait and surrounding waters (Xu et al., 2021; Chen et al., 2022). Overall, the fish habitat preferences observed between this study emphasize the unique ecological characteristics of the Luzon Strait and surrounding waters, as well as the significance of comprehending local factors that shape fish community compositions.
The fish species assessed in this study have significant human uses, particularly in fisheries. We identified a number of fishes with high commercial value, such as Thunnus albacares, Lutjanus madras, Lutjanus erythropterus, and Decapterus maruadsi. However, it is important to note that several of these commercially valuable fish species are facing conservation concerns. Overfishing, along with other anthropogenic impacts such as habitat degradation, biological invasion, and climate change, pose significant threats to fish populations (Chang et al., 2017; Hou et al., 2018). The decreasing population trends reported in the two pelagic-oceanic species, Swordfish (Xiphias gladius) and Sailfish (Istiophorus platypterus), are concerning and highlight the effects of overfishing on their populations. X. gladius, a highly important food and gamefish species, was found only at one station in this survey, indicating a localized distribution. The International Union for Conservation of Nature (IUCN) lists this species as near threatened (NT) due to a significant decline of at least 22% over the past 20 years (Collette et al., 2022a). This decline is consistent with the global trend of decreasing Swordfish populations, particularly in regions such as the Southwest Pacific (58% decrease), South Atlantic (45% decrease), and Indian Ocean (40% decrease), which are heavily impacted by overfishing (Collette et al., 2022b). Similarly, another pelagic-oceanic species, Sailfish (Istiophorus platypterus), was found at three stations in this study (LS1, LS10, and LS12). This species is classified as Vulnerable on the IUCN Red List. Overfishing is also the primary driver of population decline, which are commonly targeted for their value as food and gamefish (Collette et al., 2022a). Globally, Sailfish populations have declined by approximately 30% in the past 12 years (Collette et al., 2022b). The Indian Ocean stock, representing the majority (87%) of the global population, has experienced an estimated 27% to 33% decline due to overfishing. Therefore, it is crucial to implement effective conservation measures, including DNA barcoding, to ensure the survival and recovery of endangered and vulnerable fish species.
Conclusion
Our study demonstrates the efficacy of DNA barcoding as a highly effective tool for accurate species identification of larval fish in the Luzon Strait and adjacent waters. Among the 385 larval fish individuals, we successfully barcoded and identified 354 individuals, revealing 22 orders, 44 families, 93 genera, and 147 species. This comprehensive identification has provided valuable insights into the species composition, diversity, and geographical distribution of larval fish communities in the region. Furthermore, our research has shed light on the significance of the Luzon Strait and surrounding waters as crucial spawning and nursery grounds for various commercially important fish species, such as tunas, groupers, and mackerels. The presence of these species highlights the importance of protecting and managing this ecosystem to safeguard both conservation and sustainable fishing practices. While we acknowledge the challenges of traditional DNA barcoding techniques when dealing with a large sample size of fish larvae, recent advancements in biotechnology, such as next-generation sequencing and environmental DNA (eDNA) metabarcoding, have significantly improved the efficiency of assessing fish diversity. These advancements have facilitated the generation of larger genomic datasets, enabling more accurate and comprehensive species identification in larval fish. Additionally, the species information generated through our study, including habitat types, human uses, and IUCN conservation status, has the potential to support fisheries management efforts, conservation initiatives, and the implementation of effective strategies to protect and preserve the diverse marine ecosystems in the region. In conclusion, this study contributes to our understanding of larval fish diversity and their recruitment dynamics in the Luzon Strait and surrounding waters. By employing DNA barcoding techniques, we have provided valuable insights that can inform fisheries management efforts and conservation actions, ultimately ensuring the sustainable utilization and preservation of this important marine ecosystem.
Data availability statement
The original contributions presented in the study are publicly available. This data can be found here: https://figshare.com/articles/dataset/Assessing_Larval_Fish_Diversity_and_Conservation_Needs_in_the_Luzon_Strait_using_DNA_Barcoding/23949270.
Ethics statement
The animal study was approved by the Animal Experiment Administration Committee of South China Sea Ecological Center, Ministry of Natural Resources of the People’s Republic of China. The study was conducted in accordance with the local legislation and institutional requirements.
Author contributions
HL: Conceptualization, Data curation, Funding acquisition, Investigation, Methodology, Writing – original draft, Writing – review & editing. YC: Conceptualization, Data curation, Methodology, Software, Supervision, Writing – original draft, Writing – review & editing. XL: Methodology, Software, Visualization, Writing – review & editing. PZ: Data curation, Investigation, Writing – review & editing. XX: Data curation, Methodology, Writing – review & editing.
Funding
The author(s) declare financial support was received for the research, authorship, and/or publication of this article. This work was supported by the National Natural Science Foundation of China (41606191) and the National Key Research and Development Program of China (2022YFC3106302).
Conflict of interest
The authors declare that the research was conducted in the absence of any commercial or financial relationships that could be construed as a potential conflict of interest.
Publisher’s note
All claims expressed in this article are solely those of the authors and do not necessarily represent those of their affiliated organizations, or those of the publisher, the editors and the reviewers. Any product that may be evaluated in this article, or claim that may be made by its manufacturer, is not guaranteed or endorsed by the publisher.
Supplementary material
The Supplementary Material for this article can be found online at: https://www.frontiersin.org/articles/10.3389/fmars.2023.1268399/full#supplementary-material
References
Almany G. R., Planes S., Thorrold S. R., Berumen M. L., Bode M., Saenz-agudelo P., et al. (2017). Larval fish dispersal in a coral-reef seascape. Nat. Ecol. Evol. 1, 1–7. doi: 10.1038/s41559-017-0148
Azmir I. A., Esa Y., Amin S. M. N., Md Yasin I. S., MD Yusof F. Z. (2017). Identification of larval fish in mangrove areas of Peninsular Malaysia using morphology and DNA barcoding methods. J. Appl. Ichthyol 33, 998–1006. doi: 10.1111/jai.13425
Baumgartner G., Nakatani K., Gomes L. C., Bialetzki A., Sanches P. V., Makrakis M. C. (2004). Identification of spawning sites and natural nurseries of fishes in the upper Paraná River, Brazil. Environ. Biol. Fishes 71, 115–125. doi: 10.1007/s10641-004-0098-z
Chang C. H., Shao K. T., Lin H. Y., Chiu Y. C., Lee M. Y., Liu S. H., et al. (2017). DNA barcodes of the native ray-finned fishes in Taiwan. Mol. Ecol. Resour. 17, 796–805. doi: 10.1111/1755-0998.12601
Chen K. S., Chen H.-S., Chen C. Y., Su Y. L., Meng P. J., Chen M. H. (2022). Multivariate analysis of the spatial species diversity of demersal fish assemblages in relation to habitat characteristics in a subtropical national park, Taiwan. Mar. Biodivers. 52, 1–18. doi: 10.1007/s12526-021-01225-5
Chu C., Loh K. H., Ng C. C., Ooi A. L., Konishi Y., Huang S. P., et al. (2019). Using DNA barcodes to aid the identification of larval fishes in tropical estuarine waters (Malacca straits, Malaysia). Zool. Stud. 58, 1–15. doi: 10.6620/ZS.2019.58-30
Collette B. B., Di Natale A., Fox W., Graves J., Juan Jorda M., Pohlot B., et al. (2022a). Istiophorus platypterus. IUCN Red List Threatened Species. doi: 10.2305/IUCN.UK.2022-1.RLTS.T170338A46649664.en
Collette B. B., Di Natale A., Fox W., Graves J., Juan Jorda M., Pohlot B., et al. (2022b). Xiphias gladius. IUCN Red List Threatened Species. doi: 10.2305/IUCN.UK.2022-1.RLTS.T23148A46625751.en
Gu H., Zhao F., Yu W., Li R., Zhao L., Li D. (2019). Distributions of 224, 223Ra and 137Cs in the Luzon Strait and its adjacent waters and their response to Typhoon Rainbow. Acta Oceanol Sin. 38, 1–7. doi: 10.1007/s13131-019-1508-1
Hall T. A. (1999). BioEdit: a user-friendly biological sequence alignment editor and analysis program for Windows 95/98/NT. Nucleic Acids Symp. Ser. 41, 95–98. doi: 10.14601/Phytopathol_Mediterr-14998u1.29
Hebert P. D. N., Cywinska A., Ball S. L., Jeremy R., B P. R. S. L. (2003). Biological identifications through DNA barcodes. Proc. R. Soc Lond. Ser. B. 270, 313–321. doi: 10.1098/rspb.2002.2218
Hou G., Chen W. T., Lu H. S., Cheng F., Xie S. G. (2018). Developing a DNA barcode library for perciform fishes in the South China Sea: Species identification, accuracy and cryptic diversity. Mol. Ecol. Resour. 18, 137–146. doi: 10.1111/1755-0998.12718
Hou G., Chen Y., Wang J., Pan C., Lin J., Feng B., et al. (2022). Molecular identification of species diversity using pelagic fish eggs in spring and late autumn-winter in the eastern beibu gulf, China. Front. Mar. Sci. 8, 806208. doi: 10.3389/fmars.2021.806208
Hou G., Chen Y., Wang S., Wang J., Chen W., Zhang H. (2021). Formalin-fixed fish larvae could be effectively identified by DNA barcodes: A case study on thousands of specimens in south China sea. Front. Mar. Sci. 8. doi: 10.3389/fmars.2021.634575
Huang D., Chen J., Xu L., Wang X., Ning J., Li Y., et al. (2022). Larval fish assemblages and distribution patterns in the zhongsha atoll (Macclesfield bank, south China sea). Front. Mar. Sci. 8. doi: 10.3389/fmars.2021.787765
Huang D., Licuanan W. Y., Hoeksema B. W., Chen C. A., Ang P. O., Huang H., et al. (2015). Extraordinary diversity of reef corals in the South China Sea. Mar. Biodivers. 45, 157–168. doi: 10.1007/s12526-014-0236-1
Hubert N., Delrieu-Trottin E., Irisson J. O., Meyer C., Planes S. (2010). Identifying coral reef fish larvae through DNA barcoding: A test case with the families Acanthuridae and Holocentridae. Mol. Phylogenet. Evol. 55, 1195–1203. doi: 10.1016/j.ympev.2010.02.023
Hubert N., Espiau B., Meyer C., Planes S. (2015). Identifying the ichthyoplankton of a coral reef using DNA barcodes. Mol. Ecol. Resour. 15, 57–67. doi: 10.1111/1755-0998.12293
Kim S., Chin B. S., Wang S. Y. (2023). Evidence of the Intrusion of the Oceanic Lightfish (Vinciguerria nimbaria) into Korean Waters Based on High-Throughput Sequencing of Mixed Fish Eggs. J. Mar. Sci. Eng 11, 257. doi: 10.3390/jmse11020257
Kindt R., Coe R. (2005). Tree diversity analysis: a manual and software for common statistical methods for ecological and biodiversity studies. Nairobi: World Agroforestry Centre.
Kong X., Jiang Y., Gong Y., Chen Z., Zhang J., Fang J. (2016). A preliminary study on fishery biology of Ceratoscopelus warmingii in the central and northern South China Sea. South China Fisheries Sci. 12, 117–124. doi: 10.3969/j.issn.2095-0780.2016.04.015
Kumar S., Stecher G., Tamura K. (2016). MEGA7: molecular evolutionary genetics analysis version 7.0 for bigger datasets brief communication. Mol. Biol. Evol. 33, 1870–1874. doi: 10.1093/molbev/msw054
Kwun H. J. (2018). Species identification of juvenile fishes of the genus Pseudoblennius using mitochondrial DNA barcoding. Mitochondrial DNA Part B Resour. 3, 405–408. doi: 10.1080/23802359.2018.1456982
Letunic I., Bork P., Gmbh B. S. (2021). Interactive Tree Of Life (iTOL) v5: an online tool for phylogenetic tree display and annotation. Nucleic Acids Res. 49, 1–4. doi: 10.1093/nar/gkab301
Neira F. J., Perry R. A., Burridge C. P., Lyle J. M., Keane J. P. (2015). Molecular discrimination of shelf-spawned eggs of two co-occurring Trachurus spp. (Carangidae) in southeastern Australia: a key step to future egg-based biomass estimates Francisco. ICES J. Mar. Sci. 72, 614–624. doi: 10.1093/icesjms/fsu151
Olden J. D., Hogan Z. S., Zanden Vander M. J. (2007). Small size-biased extinction risk of the world ‘ s freshwater and marine fishes. Glob. Ecol. Biogeogr. 16, 694–701. doi: 10.1111/j.1466-8238.2007.00337.x
Panprommin D., Iamchuen N., Soontornprasit K., Tuncharoen S. (2020). The utility of DNA barcoding for the species identification of larval fish in the lower ing river, Thailand. Turkish J. Fish Aquat Sci. 20, 671–679. doi: 10.4194/1303-2712-v20_9_02
Panprommin D., Soontornprasit K., Tuncharoen S., Iamchuen N. (2021). Efficacy of DNA barcoding for the identification of larval fish species in the Upper and Middle Ing River, Thailand. Gene Rep. 23, 101057. doi: 10.1016/j.genrep.2021.101057
Pappalardo A. M., Cuttitta A., Sardella A., Musco M., Maggio T., Patti B., et al. (2015). DNA barcoding and COI sequence variation in Mediterranean lanternfishes larvae. Hydrobiologia 749, 155–167. doi: 10.1007/s10750-014-2161-5
Poulsen J. Y., Byrkjedal I., Willassen E., Rees D., Takeshima H., Satoh T. P. (2013). Mitogenomic sequences and evidence from unique gene rearrangements corroborate evolutionary relationships of myctophiformes (Neoteleostei). BMC Evol. Biol. 13, 1–22. doi: 10.1186/1471-2148-13-111
Shehata H. R., Naaum A. M., Gardu R. A. (2018). DNA barcoding as a regulatory tool for seafood authentication in Canada. Food Control 92, 147–153. doi: 10.1016/j.foodcont.2018.04.045
Sheraliev B., Peng Z. (2021). Molecular diversity of Uzbekistan’s fishes assessed with DNA barcoding. Sci. Rep. 11, 1–12. doi: 10.1038/s41598-021-96487-1
Valdez-Moreno M., Vásquez-Yeomans L., Elías-Gutírrez M., Ivanova N. V., Hebert P. D. N. (2010). Using DNA barcodes to connect adults and early life stages of marine fishes from the Yucatan Peninsula, Mexico: Potential in fisheries management. Mar. Freshw. Res. 61, 665–671. doi: 10.1071/MF09222
Ward R. D., Zemlak T. S., Innes B. H., Last P. R., Hebert P. D. N. (2005). DNA barcoding Australia's fish species. Phil. Trans. R. Soc. B. 360, 1847–1857. doi: 10.1098/rstb.2005.1716
Xing B., Wang C., Wu Q., Wang Y., Chen X., Sun R., et al. (2022). Species identification of larval fish in hawaiian waters using DNA barcoding. Front. Mar. Sci. 9. doi: 10.3389/fmars.2022.825395
Xiong W., Huang X., Chen Y., Fu R., Du X., Chen X., et al. (2020). Zooplankton biodiversity monitoring in polluted freshwater ecosystems: a technical review. Environ. Sci. Ecotechnol 1, 100008. doi: 10.1016/j.ese.2019.100008
Xiong W., Ni P., Chen Y., Gao Y., Li S., Zhan A. (2019). Biological consequences of environmental pollution in running water ecosystems: A case study in zooplankton. Environ. pollut. 252, 1483–1490. doi: 10.1016/j.envpol.2019.06.055
Xiong W., Ni P., Chen Y., Gao Y., Shan B., Zhan A. (2017). Zooplankton community structure along a pollution gradient at fine geographical scales in river ecosystems: The importance of species sorting over dispersal. Mol. Ecol. 26, 4351–4360. doi: 10.1111/mec.14199
Xu L., Wang X., Van Damme K., Huang D., Li Y., Wang L., et al. (2021). Assessment of fish diversity in the South China Sea using DNA taxonomy. Fish Res. 233, 105771. doi: 10.1016/j.fishres.2020.105771
Yang Y., Gao Y., Chen Y., Li S., Zhan A. (2019a). Interactome-based abiotic and biotic impacts on biodiversity of plankton communities in disturbed wetlands. Divers. Distrib. 25, 1416–1428. doi: 10.1111/ddi.12949
Yang Y., Li S., Gao Y., Chen Y., Zhan A. (2019b). Environment-driven geographical distribution of bacterial communities and identification of indicator taxa in Songhua River. Ecol. Indic 101, 62–70. doi: 10.1016/j.ecolind.2018.12.047
Yao M., Zhang S., Lu Q., Chen X., Zhang S.-Y., Kong Y., et al. (2022). Fishing for fish environmental DNA: Ecological applications, methodological considerations, surveying designs, and ways forward. Mol. Ecol. 31, 5132–5164. doi: 10.1111/mec.16659
Yi S., Zhong J., Huang S., Wang S., Wang W. (2017). Morphological comparison and DNA barcoding of four closely related species in the genera Misgurnus and Paramisgurnus (Cypriniformes: Cobitidae). Biochem. Syst. Ecol. 70, 50–59. doi: 10.1016/j.bse.2016.10.019
Zhan A., Hu J., Hu X., Lu W., Wang W., Peng W., et al. (2008). Fast identification of scallop adductor muscles using species-specific microsatellite markers. Eur. Food Res. Technol. 227, 353–359. doi: 10.1007/s00217-007-0728-3
Zhan A., Hu J., Hu X., Zhou Z., Hui M., Wang S., et al. (2009). Fine-scale population genetic structure of Zhikong scallop (Chlamys farreri): Do local marine currents drive geographical differentiation? Mar. Biotechnol. 11, 223–235. doi: 10.1007/s10126-008-9138-1
Zhan A., MacIsaac H. J. (2015). Rare biosphere exploration using high-throughput sequencing: research progress and perspectives. Conserv. Genet. 16, 513–522. doi: 10.1007/s10592-014-0678-9
Zhang J., Hanner R. (2012). Molecular approach to the identification of fish in the South China Sea. PloS One 7, 1–9. doi: 10.1371/journal.pone.0030621
Zhang J., Wang Y., Chen Z., Jiang Y., Xu S. (2021). Age and growth of Ceratoscopelus warmingii (Myctophidae) in the South China Sea based on sagittal otolith microstructure. Mar. Biol. Res. 17, 733–743. doi: 10.1080/17451000.2021.2015390
Keywords: species identification, DNA barcoding, larval fish, fish diversity, conservation needs, fisheries management, Luzon strait
Citation: Li H, Chen Y, Li X, Zhou P and Xiong X (2023) Assessing larval fish diversity and conservation needs in the Luzon strait using DNA barcoding. Front. Mar. Sci. 10:1268399. doi: 10.3389/fmars.2023.1268399
Received: 28 July 2023; Accepted: 26 September 2023;
Published: 12 October 2023.
Edited by:
Conghui Liu, Chinese Academy of Agricultural Sciences, ChinaReviewed by:
Zhiqiang Xia, University of Windsor, CanadaBin Zhou, Ocean University of China, China
Meng Yan, City University of Hong Kong, Hong Kong SAR, China
Copyright © 2023 Li, Chen, Li, Zhou and Xiong. This is an open-access article distributed under the terms of the Creative Commons Attribution License (CC BY). The use, distribution or reproduction in other forums is permitted, provided the original author(s) and the copyright owner(s) are credited and that the original publication in this journal is cited, in accordance with accepted academic practice. No use, distribution or reproduction is permitted which does not comply with these terms.
*Correspondence: Haitao Li, aGFpdGFvbGkxOTgxQDEyNi5jb20=; Yiyong Chen, eXljaGVuQHJjZWVzLmFjLmNu
†These authors have contributed equally to this work