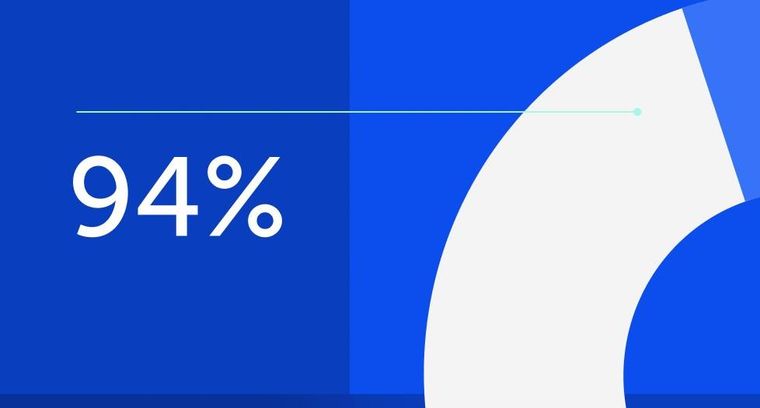
94% of researchers rate our articles as excellent or good
Learn more about the work of our research integrity team to safeguard the quality of each article we publish.
Find out more
ORIGINAL RESEARCH article
Front. Mar. Sci., 28 November 2023
Sec. Marine Megafauna
Volume 10 - 2023 | https://doi.org/10.3389/fmars.2023.1266735
Predictions of population viability can provide valid information for guiding the management and conservation of species. However, such studies are rarely conducted on cetaceans due to limited basic data. In this study, a detailed assessment of the population dynamics of Indo-Pacific humpback dolphins (Sousa chinensis) in Xiamen Bay, China was carried out using a Vortex model that was based on model parameters that were derived from monitoring data and published sources. Then, sensitivity analyses were conducted to test the relative importance of the uncertainty of the model parameters. Moreover, potential management models were evaluated to determine their effectiveness in assisting with species recovery. The baseline model projected a decline in the population abundance with a growth rate (r) of -0.031, and the probability of extinction was 58.7% over the next 100 years. The sensitivity analyses revealed that juvenile mortality and fertility were the most pivotal factors for the viability of this population. All the conservation measures, including habitat improvement, catastrophe control, and individual supplementation, contributed to population increases when compared with the baseline model. In particular, decreasing calf and juvenile mortality through habitat improvement, at high levels, was the only way to generate positive growth rates. The findings suggest that these practical management activities can reduce the risk of extinction for this species.
Identifying the factors that are responsible for the decline in long-lived and low-fecundity species can prove challenging since extensive historical data are required to evaluate and distinguish the influencing factors and trends (Chilvers, 2012). This is especially true for marine mammal species, where the monitoring of these animals at sea require a large amount of time and resources (Chilvers, 2012; Sharpe and Berggren, 2019). Additionally, they often form small and isolated populations due to aquatic habitat fragmentation (Chilvers, 2012), e.g., the Yangtze finless porpoise (Neophocaena asiaeorientalis Huang et al., 2020), Maui’s dolphin (Cephalorhynchus hectori maui Baker et al., 2002), and Indo-Pacific humpback dolphin (Sousa chinensis Jefferson and Smith, 2016). Unfortunately, small and fragmented populations are more affected by environmental factors (Chilvers, 2012).
Indo-Pacific humpback dolphins are widely distributed in the eastern Indian Ocean and throughout Southeast Asia, and their distribution is suggested to be fragmented, at least in China (Jefferson and Smith, 2016). The species regularly occur in shallow enclosed seas and show a strong preference for estuarine waters (Jefferson and Karczmarski, 2001; Parra and Ross, 2009). Previous population assessments have indicated that most individuals inhabit Chinese waters (Jefferson and Smith, 2016). However, several studies have also implied that there has been habitat loss in southern and central China along the mainland coast where S. chinensis were once frequently sighted. They are now thought to regularly occur in about eight areas (Huang et al., 2012; Wang et al., 2012a; Wang et al., 2012b; Wu et al., 2014; Jefferson and Smith, 2016). However, their population size has been gradually declining throughout their range (Jefferson and Smith, 2016; Zeng et al., 2020). In China, the Hong Kong/Pearl River Estuary population is the only one with long-term and quantitative data on the population trends, while most of the other population assessments have only started in the last 20–25 years (Jefferson and Smith, 2016; Chen et al., 2018a). The baselines information on population abundance has been investigated in Hong Kong/Pearl River Estuary (about 2600 individuals, decreasing, Chen et al., 2010; Li et al., 2019), Zhanjiang (1485 individuals, Xu et al., 2012; Xu et al., 2015), Belbu Gulf-Shatian (150–182 individuals, Chen et al., 2016), Belbu Gulf-Dafenjiang (Peng et al., 2020), eastern Taiwan Strait, Taiwan (less than 100 individuals, decreasing, Wang et al., 2012b), and Xiamen (less than 100 individuals, decreasing, Chen et al., 2018a; Zeng et al., 2020). However, information on the abundance trends of the other populations is needed for their conservation and management but is critically lacking.
Xiamen Bay is one of the traditional habitats for S. chinensis (Chen et al., 2008; Chen et al., 2011) in China, with dolphins occurring year-round (Chen et al., 2011). However, Xiamen has emerged as a pivotal area for the advancement of the economy and urbanization (Wang et al., 2017), which means that this population has been impacted by several anthropogenic influences (Chen et al., 2018a). For instance, land reclamation has potentially reduced the carrying capacity of S. chinensis in recent decades (Zeng et al., 2020). Moreover, underwater blasting from coastal construction projects can result in dolphin mortality (Wang et al., 2003). Other anthropogenic activities, including agricultural and industrial pollution, fishing, and vessel traffic, can also impact their survival (Chen et al., 2008). Furthermore, extensive mariculture causes habitat loss, and destructive fishing may contribute to habitat degradation and resource depletion (Jefferson and Hung, 2004). In addition, it has been reported that some individuals were injured as a result of collisions with vessels or fishing net entanglement (Chen et al., 2018a). These anthropogenic impacts have reduced dolphin abundance in the last few decades (Liu and Huang, 2000; Chen et al., 2018a; Zeng et al., 2020). Thus, there is an urgent need to assess the population dynamics of S. chinensis and explore potential conservation and management measures for this population. To the best of our knowledge, this is the first study that focuses on effectiveness of management actions for S. chinensis.
Population viability analysis (PVA) is a useful quantitative tool for evaluating population dynamics, testing the relative importance of model parameters, and assessing management strategies for wildlife populations (Cross and Beissinger, 2001; Reed et al., 2002; Lacy, 2019; Lacy et al., 2021). This method has been successfully applied to S. chinensis in Hong Kong and Taiwan (Huang et al., 2012; Araújo et al., 2014; Karczmarski et al., 2017). In this study, based on extensive life history information that was obtained in recent decades, we conducted a PVA using Vortex software to simulate the Xiamen population dynamics in the next 100 years. Sensitivity of population trajectories to key demographic parameters. Finally, we aimed to estimate underlying efficacy in assisting the recovery of this endangered species under different management scenarios and feasible conservation actions are proposed. Since the problems faced by other dolphins are similar to those of the Xiamen population, this study could provide a reference for the conservation of other dolphins worldwide.
From September 2017 to August 2019, boat-based surveys were conducted in Xiamen Bay and its adjacent waters of Zhangzhou, Kinmen, and Quanzhou, an area of 700 km2 (Figure 1). If the weather conditions were favorable, at least two experienced observers searched for dolphins on the deck. When dolphins were encountered, the ship slowly approached them (Chen et al., 2011). Then, the geographic coordinates were recorded via a Global Positioning System (Garmin GPSMAP 60CSx), and the photos were taken with a digital camera (Canon EOS 1Dx Mark II with 28–300 mm zoom lenses).
Based on the body color, spots, shape, and defects of the dorsal fin, and trauma-related injuries of the dolphins, the individuals were effectively identified (Jefferson and Leatherwood, 1997). Every newly identified individual was assigned a unique catalog number. Each photograph from a survey was matched against the latest catalog, and if a dolphin was different from any of the identified animals, a new individual was recorded (Chen et al., 2018a). To avoid double counting, both sides of the dorsal fin were photographed where possible. The dolphins’ photos were classified as ‘outstanding’, ‘good’, or ‘poor’ based on their clarity, contrast, angle, and the dorsal fin size in the frame. To minimize the introduction of bias and avoid misidentification, poor photographs were not used for individual identification (Chen et al., 2018a). During a total of 179 survey days, 55 individuals were successfully identified for use in mark–recapture analysis (Figure 2), and the number of sightings of individuals was between 1 and 33 (Figure 3).
Figure 2 Discovery curve of the cumulative number of Indo-Pacific humpback dolphins identified from September 2017 to August 2019.
Color pattern development is related to age in S. chinensis (Jefferson et al., 2012). They are born dark gray, almost black, and experience a gradual depigmentation process during their growth. They change from being spotted to having only a few spots, and some individuals even have a pure white body surface (Guo et al., 2020). Six age classes (unspotted calf, unspotted juvenile, mottled, speckled, spotted adult, and unspotted adult) were used in previous studies (Jefferson et al., 2012). To reduce the overlap among age classes, the life span of S. chinensis was split into four age classes in this study (calf, 0 to 1 year old; juvenile, 2 to 4 years old; subadult, female: 5 to 9 years old, male: 5 to 12 years old; and adult, female: older than 9 years old, male: older than 12 years old).
The Cormack-Jolly-Seber (CJS) model was used to estimate the apparent survival (ϕ) and recapture probability (p) in the three age classes (juvenile, subadult, and adult) using Program MARK (Alves et al., 2015). The identification data for all the marked individuals during the four consecutive months (from September 2017 to August 2019) were grouped, creating a total of six sampling occasions (Figure 2). Before fitting the CJS models, the goodness of fit (GOF) was explored using the “program RELEASE GOF” test in MARK (Chen et al., 2018a). The GOF results for three age classes (TEST 2 + TEST 3) were not significant (p>0.05), suggesting that the CJS model did not sufficiently fit the data. In addition, an estimation of the variance inflation factor ( ) was obtained using the median- estimator (Lebreton et al., 1992; Choquet et al., 2010) where: 1< < 3 indicates that the bias that is associated with data over-dispersion is imperceptible and > 3 indicates more fundamental problems (Lebreton et al., 1992; Cooch and White, 2006). In this study, fell between 1 and 3 (1.16 in the juveniles, 2.74 in the subadults, and 2.22 in the adults), and the GOF test indicated that the model structures were suitable, so we proceeded with the analysis. Two candidate models were developed to test the time effect on the estimated parameters: constant (.) and full time-variation (t). The estimated was included in the model, incorporating overdispersion in the calculation of the standard error and confidence interval (CI, 95%) of the parameter estimates ϕ and p. The selection of the candidate models was based on the corrected Quasi- Akaike Information Criterion (QAICc) given that the models were adjusted for overdispersion (Anderson et al., 1994). The best model selection was based on the lowest QAICc value when the difference between the model and the best model based on the QAICc was less than two (ΔQAICc; Burnham and Anderson, 1998). For calf mortality, Chen (2007) calculated it to be 46.15% based on stranding data that were collected from 1997 to 2004. Due to the conservation measures in recent years, we suspect that the mortality rate has decreased. Thus, calf mortality was assumed to be 40% in the baseline model.
The PVAs were carried out using Vortex 10.5.5, which can simulate stochastic demographic processes. The baseline model was run as one population projection, simulated 1000 times for each scenario, and extended to 100 years for each projection. The model can be used to predict the population dynamics 100 years into the future because of the relatively long generation interval of this species. Extinction was defined as the survival of only individuals of the same sex (Ginzburg et al., 1982). The baseline model (Table 1) was developed using demographic input parameters from this study, previously published papers, and discussions with experts on the species. The impact of inbreeding was modeled as 6.29 lethal equivalents (O’Grady et al., 2006), with 50% of inbreeding due to recessive lethals.
The breeding system of S. chinensis is polygynous (Karczmarski et al., 2017), and female and male individuals can breed at 9–10 and 12–14 years old, respectively (Jefferson, 2000; Jefferson et al., 2012). In the baseline model, the age of first calving for the females was 10, and the age of first breeding for the males was 13. Generally, females give birth to only one calf at a time, and the calving interval for them should be about 2–3 years and could potentially be up to 4–6 years (Jefferson et al., 2012). Thus, we assumed that the rate of an adult female producing a calf in any given year was 25%, and a standard deviation (SD) of 10% was assigned to the mean annual calving rate due to environmental variability. Besides, Indo-Pacific humpback dolphins seem to reproduce throughout their adult lifespan, and the maximum life span is about 38 years old (Jefferson et al., 2012). We assumed that the maximum reproductive age of females was 36 years old in this study because the calves were typically associated with their mothers for approximately two years. The calves had a sex ratio of 1:1 at birth.
Humpback dolphins in Xiamen Bay have faced serious anthropogenic impacts (Chen et al., 2018a). In particular, at least 16 dead individuals were recorded from 2002 to 2004, and underwater blasting from coastal construction projects was regarded as the definitive cause of death (Wang et al., 2003; Chen et al., 2008). Currently, there are still water projects under continuous construction affecting the survival of the dolphins in Xiamen waters. Therefore, anthropogenic activities were treated as catastrophe, with the frequency set at 5% based on historical records. The severity of factors that were related to reproduction and survival was set at 0.5 and 0.8, respectively.
Previous estimates of the population size were all less than 100 individuals (Jefferson and Hung, 2004; Chen et al., 2008; Chen et al., 2009; Chen et al., 2018a; Zeng et al., 2020). The latest reported population size was 58 (Zeng et al., 2020). Considering that 60 individuals were successfully identified, not all individuals can be identified (Zeng et al., 2020), and 58 individuals may underestimate the population size. Therefore, the population size was set at 72 individuals in this study (Chen et al., 2018a). However, there has yet to be an estimate of the carrying capacity (K) of dolphins in Xiamen Bay. Based on historical data on the population size, K was set as 150 in the baseline model.
A sensitivity analysis was conducted to assess how the ambiguity parameter values affected the model outputs. Every parameter was varied by ±5%, ± 15%, and ±25% (Table S1), while all the other parameters were maintained as baseline values. These parameters included the calf and juvenile mortality rates, reproduction rates, carrying capacity, and maximum reproductive age. A standard sensitivity index (Sx, Akçakaya and Sjögren-Gulve, 2000) was adopted for the sensitivity analysis. The baseline model outcomes were used to calculate the standard sensitivity index for each parameter, and the calculation formula is as follows:
Where X was the output value and P was the parameter of interest (Fantle-Lepczyk et al., 2018). The standard sensitivity index computed the degree of difference between the modified and baseline outcomes. The further Sx was from 0, the higher the sensitivity (Blázquez et al., 2020). It should be noted that the maximum age of reproduction in the sensitivity analysis was not based on the baseline model. The baseline value was centered at 30 years instead of 36. This was done to include ±25% of the ages that S. chinensis are known to reach within the interval (Blázquez et al., 2020).
Three management scenarios were developed, including habitat improvement, catastrophe control, and supplementation. Each scenario was simulated using three intensity levels (low, medium, and high) of the magnitude of change in the demographic variables relative to the baseline model (Table S2). The habitat improvement management models were based on reducing pollution, controlling vessel traffic, and making mariculture more sustainable. The mortality of calves and juveniles was decreased by three intensity levels (-15%, -30%, and -45% in calves; -12.5%, -25%, and -37.5% in juveniles). In the catastrophe control models, this effect was assessed by reducing the frequency of catastrophe (from 5% to 3%, catastrophe control low), by reducing its frequency and severity (1% frequency, severity on survival and reproduction reduced by 0.1, catastrophe control medium), and by completely removing its occurrence (catastrophe control high). The final model looked at the potential impacts of supplementation. The supplementation effects were simulated with three scenarios: supplementing two adults every ten years for 50 years (low supplementation), supplementing two adults every five years for 50 years (medium supplementation), and supplementing two adults every two years for 50 years (high supplementation). Considering the difference between males and females, the supplements were divided into three cases: two males, one male and one female, and two females.
When comparing management measures with no management measures, the utility of the management models was evaluated using the model outcomes to illustrate the likelihood of success (Robinson et al., 2015). Many available viability measures (e.g., population growth, probability of extinction, and minimum expected population size) were used as viability metrics, and the reported management goals were also included (Pe'er et al., 2013). A population size less than 30 was found to be highly vulnerable to extinction, but one greater than 50 could better preserve genetic diversity in the common bottlenose dolphin (Tursiops truncatus Montagu, 1821) in Sarasota Bay (Lacy et al., 2021). Moreover, 50 reproducing animals were found to maintain a low level of inbreeding depression (Franklin, 1980). Thus, we compared the baseline and management model outcomes for the expected minimum population size (EMP, above 30 and above 50) to confirm the proportions of the management models with three outputs: success, failure, or not needed (Robinson et al., 2015). Success/Success + Failure was computed to determine how often the management was expected to achieve the stated goals (Robinson et al., 2015; Fantle-Lepczyk et al., 2018).
The best-fit CJS model produced a constant apparent survival and capture probability for the three age class datasets (Table 2). The apparent survival probability values were 0.76 (95% CI: 0.41–0.93), 0.90 (95% CI: 0.59–0.98), and 0.97 (95% CI: 0.83–0.99; Table 3), for the juveniles, subadults, and adults, respectively, and the corresponding recapture rates were 0.57 (95% CI: 0.20–0.87), 0.81 (95% CI: 0.49–0.95), and 0.80 (95% CI: 0.65–0.89), respectively. In the adults, the CJS models ϕ(.)p(t) and ϕ(.)p(.) had a 1.84 difference in the QAICc, suggesting that they both fit the data well. The model ϕ(.)p(t) resulted in a constant apparent survival of 0.97 (95% CI: 0.78–0.99), and time-dependent capture probabilities for the adults (p = 1, 0.92, 0.63, 0.69, and 0.82).
Table 2 Cormack-Jolly-Seber (CJS) models fitted to three age classes for estimation of the survival rate ϕ.
Table 3 Estimate of the survival rates of Indo-Pacific humpback dolphins based on the Cormack-Jolly-Seber (CJS) models.
A declining population trend was shown (r = -0.0310, SE = 0.0005, SD = 0.1522), and the likelihood of extinction was 58.7% (SE = 0.01557) in the baseline model. Furthermore, the mean time to first extinction was 76.2 years, approximately four generations, while the median time to extinction was 93 years, approximately five generations. The mean size of the extant population (N-Extant) was 11.51 (SD = 9.74).
Although the magnitude of variation reached ±25%, a lower sensitivity was yielded for the effect of the carrying capacity (K) on both the population growth rate (r) and extant population (N-Extant; Table 4). The model was slightly more sensitive to the effect of first-year calf mortality and the maximum age of reproduction on population size. However, the growth rate was sensitive to the maximal age of reproduction. Overall, juvenile mortality and the reproductive rate were the principal parameters for the growth rate and population size (Table 4).
In general, all the management models suggested increases in the growth rate, population size, and time to extinction and lower probabilities of extinction when compared with the baseline (Table 5, Figure 4). Although all the supplementation models were an improvement over the baseline in terms of the growth rate, supplementing female individuals was more effective than supplementing male individuals. When two females were supplemented every two years, the possibility of extinction dropped from 58.7% of the baseline to 1.7% (Table 5); when the same number of males was supplemented, the extinction probability decreased only slightly (from 58.7% to 46.6%, Table 5). The mitigation of catastrophic events contributed to an increase in the population growth rate and final population size at all the intensity levels that were modeled, especially when catastrophic events were absent. Similarly, the projections implied that a lowered calf and juvenile mortality due to habitat improvement could increase population growth rates notably at all the levels of simulation. Even with low habitat improvement, the probability of extinction was markedly reduced, and habitat improvement at a high level contributed to positive growth rates. Furthermore, 76% of gene diversity was retained in the baseline model, and all the management models maintained greater initial gene diversity than that of the baseline model (from 78.45% to 90.80%; Figure 5).
Figure 4 Population size dynamics of Indo-Pacific humpback dolphins in Xiamen under different management scenarios. 10-2: supplementing two individuals every ten years; 5-2: supplementing two individuals every five years; 2-2: supplementing two individuals every two years. (A), habitat improvement; (B), catastrophe control; (C), supplementing one male and one female; (D), supplementing two males; (E), supplementing two females).
Figure 5 Genetic diversity dynamics of humpback dolphin in Xiamen Bay under different management scenarios. (A), habitat improvement; (B), catastrophe control; (C), supplementing one male and one female; (D), supplementing two males; (E), supplementing two females).
When comparing the outcomes of the management models with the baseline model, the probability that management was needed (success + failure) ranged from 4.4% to 55.5% and 0.7% to 28.0% when the EMP was greater than 30 and 50, respectively (Table 6). Supplementation of individuals with females was more effective than that with males in increasing the population size among the management actions in terms of the set target value (Table 6). Additionally, all the catastrophe control and habitat improvement models exhibited more management effectiveness than the current conditions.
Table 6 Baseline and management model outcomes with the proportion of 1000 iterations that led to one of three possible outputs (Success, Not Needed, or Failure).
The survival probability in this survey was based on a three-year mark-recapture dataset (Figure 2) that covered the majority of the distribution range of these dolphins. Only a few areas could not be investigated for political reasons, and dolphins crossing administrative boundaries is a common phenomenon (Wang et al., 2016). Therefore, the impact of the incomplete coverage of their distribution area on individual identification is thought to be small. Moreover, misidentification is one of the major problems in cetacean mark-recapture surveys (Blackmer et al., 2000). However, most S. chinensis have obvious natural markings (Jefferson et al., 2012; Wang et al., 2012b; Chan and Karczmarski, 2017; Chen et al., 2018b), making this species easy to identify via photos. Additionally, the developmental process of the color pattern during growth can be used for age differentiation (Jefferson et al., 2012). However, the body color pattern can change quickly from weaning to sexual maturity (Guo et al., 2020), and this may increase the risk of misidentification (Lin et al., 2018). Nevertheless, we feel that continued survey efforts and high capture and recapture probabilities effectively reduced the impact of this limitation.
Previous assessments of survival in the Xiamen population included individuals of different age classes. Chen et al. (2018a) and Zeng et al. (2020) estimated the apparent survival of this population to be 0.957 (95% CI: 0.918–0.978) and 0.976 (95% CI: 0.944–0.990), respectively. Chen et al. (2018) suggested that these results might be higher than the true apparent survival of the entire population, but lower than the true apparent survival of adults and subadults. Our estimate for the apparent survival probabilities for juveniles, subadults, and adults were 0.76 (95% CI: 0.44–0.93), 0.90 (95% CI: 0.75–0.96), and 0.97 (95% CI: 0.88–0.99), respectively. The estimated survival rates for adults in this study were similar to those of Chen et al. (2018) and Zeng et al. (2020). In addition, the mortality rates of S. chinensis in the Pearl River Estuary ranged from 0.061 to 0.168 (survival approximately 0.832–0.939) at ages 1 to 4 years old, 0.035 to 0.047 (survival approximately 0.953–0.965) at ages 5 to 12 years old, and 0.243 for calves (aged 0–1 year old; Huang et al., 2012; Miller, 2016). The apparent survival probabilities for juveniles (aged 1–4 years old) and subadults (aged 5–12 years old) were higher than those of the Xiamen population. However, the calf mortality that was estimated using Siler’s model in the Pearl River Estuary was lower than that of the other populations (Guissamulo and Cockcroft, 2004; Jefferson et al., 2012). The mortality rates in the other age classes may have been underestimated. Our results were similar to the mortality rates for this population based on the number of dead individuals (Chen, 2007). As the Xiamen population has been sighted all year round and the range of activities was limited (Chen et al., 2011), Chen et al. (2018) considered the apparent survival rate to represent the real survival rate. Hence, the survival analysis in this study is likely to represent the true state of this population.
Population size and risk of extinction are two of the five International Union for Conservation of Nature criteria for assessing the population and conservation status of species (IUCN, 2001). In Xiamen Bay, based on surveys that were carried out in 1994–1999, the population size was estimated to be about 60 individuals (Liu and Huang, 2000). However, this estimate has not been widely accepted. Thereafter, 80 (Jefferson and Hung, 2004) and 86 (Chen et al., 2008) individuals were reported based on distance sampling surveys that were conducted in 1997–1998 and 2004, respectively. Afterward, mark-recapture techniques identified 76 individuals in 2004–2008 (Chen et al., 2009), 72 individuals in 2007–2010 (Chen et al., 2018a) and 54–59 individuals in 2010–2015 (Zeng et al., 2020). The above survey results show that the Xiamen population is a small population. The baseline model showed a negative growth trend (-0.031), a high probability of extinction (58.7%), and about 12 individuals remaining in the next 100 years. Nevertheless, these predictions might be lower than the actual extinction risk of Xiamen population as some factors could not be quantified and were not included.
In Xiamen Bay, at least five ports and five bridges have been built over the past 20 years (Chen et al., 2018a). In addition, some additional projects are currently under construction, such as the new Xiamen airport. Project construction is a catastrophic event with a 5% simulated probability in this study, and it could drive the population into a steep decline, resulting in high probability of extinction. Conversely, when the impacts of catastrophes on survival and reproduction were removed, the extinction probability was greatly reduced. Considering that marine-related construction will continue, prevention and mitigation measures are important. Fortunately, some conservation measures have been implemented in some construction projects.
Many anthropogenic activities have consistently affected habitat quality in the Xiamen population including pollution, mariculture, and vessel traffic (Chen et al., 2008). The cumulative effect of organic pollutants, such as polychlorinated biphenyl, organochlorine pesticide, and polybrominated diphenyl ether, could decrease the population survival rates (Huang et al., 2012; Araújo et al., 2014). Mariculture may also lead to habitat loss (Chen et al., 2008). As an important port with a developed economy, a large number of vessels use Xiamen Bay. Increasing calf and juvenile survival rates through habitat improvement was predicted to result in a much larger population size than the baseline. Moreover, habitat improvement at a high level yielded a positive outcome for the humpback dolphin population, which could be more effective in boosting population growth. The major advantage of habitat improvement is that it is relatively easy to implement.
Our findings were similar to those for humpback dolphins in the eastern Taiwan Strait, where the initial population size was 74 and the probability that the population size was less than 1 after 100 years was 66.2% (Araújo et al., 2014). Generally, with a smaller population size, the population is more vulnerable to environmental and stochastic events (Lande, 1988; Fantle-Lepczyk et al., 2018). In contrast, the extinction probability was predicted to be zero in the Pearl River Estuary population, with an estimated population size of 2517 dolphins over the next 100 years (Huang et al., 2012). Although long generation times could reduce the loss of genetic diversity in closed populations (Soulé et al., 1986; Lacy et al., 2021), only 76% of genetic diversity was retained in the baseline model, which is less than the common criterion of at least 90% of initial heterozygosity (Soulé et al., 1986). Consequently, the quantitative population viability analysis suggested that the future of the Xiamen population is not optimistic.
Whether mortality or fecundity is more a significant factor in affecting the viability of cetacean populations has been debated for a long time (Manlik et al., 2016; Williams et al., 2017). Some studies have demonstrated that changes in age-specific mortality rates have a much greater influence than changes in fertility rate on the extinction risk and population growth rate (Harding et al., 2007; Araújo et al., 2014; Lacy et al., 2017; Cervin et al., 2020). However, females’ reproductive capacity was shown to be more important than the mortality of calves in bottlenose dolphins (Tursiops; Blázquez et al., 2020). The sensitivity analysis revealed that juvenile mortality and fertility had larger effects on the population growth rates and population size than calf mortality in Xiamen humpback dolphins. This has been explained by females being unlikely to reproduce again during the care of their calf. If the calf dies, the female can reproduce again to make up for the loss (Blázquez et al., 2020) but the cost of replacing older individuals, especially adult-breeding females, is higher in terms of time.
The variation in the carrying capacity had little effect on the population growth and population size and much less of an effect than the other parameters. Decreasing the carrying capacity by 25% yielded similar population growth rates to those when it was increased by 25%. In addition, the population size did not reach the carrying capacity in all the sensitivity models, which seems to indicate that the carrying capacity does not limit population growth. These results suggested that the key demographic parameters were the main threats to the humpback dolphins.
The increased population size through supplementation did not induce positive growth rates, but it reduced the probability of extinction at all the levels that were tested. The best supplementation model reduced the extinction probability from the baseline of 58.7% to 1.7%. Similar results were obtained when evaluating the effects of supplementation in vultures (Gyps fulvus Hablizl, 1783; Aresu et al., 2021). By comparing the output for the supplementation of different genders, the study confirmed that females played a greater role in populations than males. The importance of females in population dynamics has been demonstrated in the eastern Taiwan Strait population (Araújo et al., 2014). Additionally, the authors speculated that the genetic diversity that is carried by males contributed to population resilience (Araújo et al., 2014). Our study demonstrated that females were superior to males in preserving genetic diversity. Currently, there is no individual supplementation as part of the conservation measures for the Xiamen population, and it is not easy to implement this measure. However, if this measure can be implemented in the future, females would be the first choice.
In summary, the findings of this study revealed that the humpback dolphin population in Xiamen was vulnerable. Thus, conservation measures to reduce the threat to this population need to be implemented immediately. The management measures provided in this study can be used as reference for the future conservation of this population. Some of them are also easy to implement, for example, mitigation of mortality through habitat improvement is one of the best measures, which promoted positive population growth in the simulation. This measure could also apply to endangered cetacean species caused by anthropogenic activities.
The original contributions presented in the study are included in the article/Supplementary Material. Further inquiries can be directed to the corresponding authors.
Ethical review and approval was not required for the animal study because the animals were wild.
YL: Writing – review & editing, Data curation, Investigation, Methodology, Software, Writing – original draft. HZ: Data curation, Methodology, Software, Writing – review & editing. XX: Data curation, Methodology, Writing – review & editing, Investigation. BC: Methodology, Writing – review & editing. GY: Writing – review & editing, Funding acquisition.
The author(s) declare financial support was received for the research, authorship, and/or publication of this article. This work was financially supported by National Key Research and Development Program of China (Grant no. 2022YFF1301600 to GY).
Thanks to the fishermen and the members in our lab who participated in vessel-based field survey. The authors are grateful to Min Xu (Yangzhou University) for her help with data analysis.
The authors declare that the research was conducted in the absence of any commercial or financial relationships that could be construed as a potential conflict of interest.
All claims expressed in this article are solely those of the authors and do not necessarily represent those of their affiliated organizations, or those of the publisher, the editors and the reviewers. Any product that may be evaluated in this article, or claim that may be made by its manufacturer, is not guaranteed or endorsed by the publisher.
The Supplementary Material for this article can be found online at: https://www.frontiersin.org/articles/10.3389/fmars.2023.1266735/full#supplementary-material
Akçakaya H. R., Sjögren-Gulve P. (2000). Population viability analyses in conservation planning: An overview. Ecol. Bull. 48, 9–21.
Alves F., Dinis A., Nicolau C., Ribeiro C., Kaufmann M., Fortuna C., et al. (2015). Survival and abundance of short-finned pilot whales in the archipelago of Madeira, NE Atlantic. Mar. Mammal Sci. 31, 106–121. doi: 10.1111/mms.12137
Anderson D. R., Burnham K. P., White G. C. (1994). AIC model selection inoverdispersed capture–recapture data. Ecology 75, 1780–1793. doi: 10.2307/1939637
Araújo C. C., Wang J. Y., Hung S. K., White B. N., Brito D. (2014). Viability of the critically endangered eastern Taiwan Strait population of Indo-Pacific humpback dolphins Sousa chinensis. Endanger. Species Res. 24, 263–271. doi: 10.3354/esr00605
Aresu M., Rotta A., Fozzi A., Campus A., Muzzeddu M., Secci D., et al. (2021). Assessing the effects of different management scenarios on the conservation of small island vulture populations. Bird Conserv. Int. 31, 111–128. doi: 10.1017/S0959270920000040
Baker A. N., Smith A. N. H., Pichler F. B. (2002). Geographical variation in Hector's dolphin: Recognition of new subspecies of Cephalorhynchus hectori. J. R. Soc New Zeal. 32, 713–727. doi: 10.1080/03014223.2002.9517717
Blackmer A. L., Anderson S. K., Weinrich M. T. (2000). Temporal variability in features used to photo-identify humpback whales (Megaptera novaeangliae). Mar. Mammal Sci. 16, 338–354. doi: 10.1111/j.1748-7692.2000.tb00929.x
Blázquez M., Baker I., O’Brien J. M., Berrow S. D. (2020). Population viability analysis and comparison of two monitoring strategies for bottlenose dolphins (Tursiops truncatus) in the Shannon Estuary, Ireland, to inform management. Aquat. Mammals 46, 307–325. doi: 10.1578/AM.46.3.2020.307
Burnham K. P., Anderson D. R. (1998). Model selection and inference: A practical information-theoretic approach (New York: Springer-Verlag).
Cervin L., Harkonen T., Harding K. C. (2020). Multiple stressors and data deficient populations; a comparative life-history approach sheds new light on the extinction risk of the highly vulnerable Baltic harbour porpoises (Phocoena phocoena). Environ. Int. 144, 106076. doi: 10.1016/j.envint.2020.106076
Chan S. C., Karczmarski L. (2017). Indo-Pacific humpback dolphins (Sousa chinensis) in Hong Kong: Modelling demographic parameters with mark-recapture techniques. PloS One 12, e0174029. doi: 10.1371/journal.pone.0174029
Chen B. Y. (2007). “A study on the population biology and conservation of the Chinese white dolphin in Xiamen, China,” in [Doctoral Dissertation] (Nanjing: Nanjing Normal University).
Chen B. Y., Gao H. L., Jefferson T. A., Lu Y., Wang L., Li S. S., et al. (2018a). Survival rate and population size of Indo-Pacific humpback dolphins (Sousa chinensis) in Xiamen Bay, China. Mar. Mammal Sci. 34, 1018–1033. doi: 10.1111/mms.12510
Chen B. Y., Jefferson T. A., Wang L., Gao H. L., Zhang H., Zhou Y., et al. (2018b). Geographic variation in pigmentation patterns of Indo-Pacific humpback dolphins (Sousa chinensis) in Chinese waters. J. Mammal. 99, 915–922. doi: 10.1093/jmammal/gyy068
Chen B. Y., Xu X. R., Jefferson T. A., Olson P. A., Qin Q. R., Zhang H. K., et al. (2016). Conservation status of the Indo-Pacific humpback dolphin (Sousa chinensis) in the Northern Beibu Gulf, China. Adv. Mar. Biol. 73, 119–139. doi: 10.1016/bs.amb.2015.10.001
Chen B. Y., Zheng D. M., Ju J. F., Xu X. R., Zhou K. Y., Yang G. (2011). Range patterns of resident Indo-Pacific humpback dolphins (Sousa chinensis, Osbeck 1765) in Xiamen, China: Implications for conservation and management. Zool. Stud. 50, 751–762.
Chen B. Y., Zheng D. M., Yang G., Xu X. R., Zhou K. Y. (2009). Distribution and conservation of the Indo-Pacific humpback dolphin in China. Integ. Zool. 4, 240–247. doi: 10.1111/j.1749-4877.2009.00160.x
Chen B. Y., Zheng D. M., Zhai F. F., Xu X. R., Sun P., Wang Q., et al. (2008). Abundance, distribution and conservation of Chinese white dolphins (Sousa chinensis) in Xiamen, China. Mamm. Biol. 73, 156–164. doi: 10.1016/j.mambio.2006.12.002
Chen T., Hung S. K., Qiu Y., Jia X., Jefferson T. A. (2010). Distribution, abundance, and individual movements of Indo-Pacific humpback dolphins (Sousa chinensis) in the Pearl River Estuary, China. Mammalia 74, 117–125. doi: 10.1515/mamm.2010.024
Chilvers B. L. (2012). Population viability analysis of New Zealand sea lions, Auckland Islands, New Zealand’s sub-Antarctics: Assessing relative impacts and uncertainty. Polar Biol. 35, 1607–1615. doi: 10.1007/s00300-011-1143-6
Choquet R., Lebreton J. D., Gimenez O., Reboulet A. M., Pradel R. (2010). U-CARE: Utilities for performing goodness of fit tests and manipulating CApture-REcapture data. Ecography 32, 1071–1074. doi: 10.1111/j.1600-0587.2009.05968.x
Cooch E., White G. (2006) Program MARK: a gentle introduction. Available at: http://www.phidot.org/software/mark/docs/book/.
Cross P. C., Beissinger S. R. (2001). Using logistic regression to analyze the sensitivity of PVA models: A comparison of methods based on African wild dog models. Conserv. Biol. 15, 1335–1346. doi: 10.1111/j.1523-1739.2001.00031.x
Fantle-Lepczyk J., Taylor A., Duffy D. C., Crampton L. H., Conant S. (2018). Using population viability analysis to evaluate management activities for an endangered Hawaiian endemic, the Puaiohi (Myadestes palmeri). PloS One 13, e0198952. doi: 10.1371/journal.pone.0198952
Franklin I. R. (1980). “Evolutionary change in small populations,” in Conservation Biology - An evolutionary-ecological perspective, vol. 135–150 . Eds. Soule M. E., Wilcox B. A. (Sunderland, Massachusetts: Sinauer Associates).
Ginzburg L. R., Slobodkin L. B., Johnson K., Bindma A. G. (1982). Quasiextinction probabilities as a measure of impact on population growth. Risk Anal. 2, 171–181. doi: 10.1111/j.1539-6924.1982.tb01379.x
Guissamulo A., Cockcroft V. G. (2004). Ecology and population estimates of Indo-Pacific humpback dolphins (Sousa chinensis) in Maputo Bay, Mozambique. Aquat. Mamm. 30, 94–102. doi: 10.1578/AM.30.1.2004.94
Guo L., Lin W., Zeng C., Luo D., Wu Y. (2020). Investigating the age composition of Indo-Pacific humpback dolphins in the Pearl River Estuary based on their pigmentation pattern. Mar. Biol. 167, 1–12. doi: 10.1007/s00227-020-3650-x
Harding K. C., Härkönen T., Helander B., Karlsson O. (2007). Status of Baltic grey seals: Population assessment and extinction risk Vol. 6 (Tromsø, Norway: NAMMCO Scientific Publications), 33–56. doi: 10.7557/3.2720
Huang S. L., Karczmarski L., Chen J., Zhou R., Lin W., Zhang H., et al. (2012). Demography and population trends of the largest population of Indo-Pacific humpback dolphins. Biol. Conser. 147, 234–242. doi: 10.1016/j.biocon.2012.01.004
Huang J., Mei Z., Chen M., Han Y., Zhang X., Moore J. E., et al. (2020). Population survey showing hope for population recovery of the critically endangered Yangtze finless porpoise. Biol. Conserv. 241, 108315. doi: 10.1016/j.biocon.2019.108315
IUCN, S. S. C (2001). IUCN Red List categories and criteria: version 3.1 (Prepared by the IUCN Species Survival Commission) Switzerland.
Jefferson T. A. (2000). Population biology of the Indo-Pacific hump-backed dolphin in Hong Kong waters. Wildlife Monogr. 144, 1–65.
Jefferson T. A., Hung S. K. (2004). A review of the status of the Indo-Pacific humpback dolphin (Sousa chinensis) in Chinese waters. Aquat. Mamm. 30, 149–158. doi: 10.1578/am.30.1.2004.149
Jefferson T. A., Hung S. K., Robertson K. M., Archer F. I. (2012). Life history of the Indo-Pacific humpback dolphin in the Pearl River Estuary, southern China. Mar. Mammal Sci. 28, 84–104. doi: 10.1111/j.1748-7692.2010.00462.x
Jefferson T. A., Karczmarski L. (2001). Sousa chinensis. Mammal. Specise 2001, 1–9. doi: 10.1644/1545-1410(2001)655<0001:SC>2.0.CO;2
Jefferson T. A., Leatherwood S. (1997). Distribution and abundance of Indo-Pacific hump-backed dolphins (Sousa chinensis Osbeck 1765) in HongKong waters. Asian Mar. Biol. 14, 110.
Jefferson T. A., Smith B. (2016). Re-assessment of the conservation status of the Atlantic humpback dolphin, Sousa teuszii, Using the IUCN Red List Criteria. Adv. Mar. Biol. 72, 47–77. doi: 10.1016/bs.amb.2015.04.002
Karczmarski L., Huang S. L., Chan S. C. (2017). Threshold of long-term survival of a coastal delphinid in anthropogenically degraded environment: Indo-Pacific humpback dolphins in Pearl River Delta. Sci. Rep. 7, 1–10. doi: 10.1038/srep42900
Lacy R. C. (2019). Lessons from 30 years of population viability analysis of wildlife populations. Zoo Biol. 38, 67–77. doi: 10.1002/zoo.21468
Lacy R. C., Wells R. S., Scott M. D., Allen J. B., Barleycorn A. A., Urian K. W., et al. (2021). Assessing the viability of the Sarasota Bay community of bottlenose dolphins. Front. Mar. Sci. 8. doi: 10.3389/fmars.2021.788086
Lacy R. C., Williams R., Ashe E., Balcomb K. C. III, Brent L. J., Clark C. W., et al. (2017). Evaluating anthropogenic threats to endangered killer whales to inform effective recovery plans. Sci. Rep. 7, 1–12. doi: 10.1038/s41598-017-14471-0
Lande R. (1988). Genetics and demography in biological conservation. Science 241, 1455–1460. doi: 10.1126/science.3420403
Lebreton J. D., Burnham K. P., Clobert J., Anderson D. R. (1992). Modeling survival and testing biological hypotheses using marked animals: A unified approach with nase studies. Ecol. Monogr. 62, 67–118. doi: 10.2307/2937171
Li M., Wang X., Hung S. K., Xu Y., Chen T. (2019). Indo-Pacific humpback dolphins (Sousa chinensis) in the Moyang River Estuary: The western part of the world's largest population of humpback dolphins. Aquat. Conserva. 29, 798–808. doi: 10.1002/aqc.3055
Lin W. Z., Chan S. C., Chen Z., Karczmarski L., Wu Y. P. (2018). Mark-recapture technique for demographic studies of Chinese white dolphins-applications and suggestions. Acta Theriologica Sin. 38, 586–596. doi: 10.16829/j.slxb.150171
Liu W. H., Huang Z. G. (2000). Distribution and abundance of Chinese white dolphins (Sousa chinensis) in Xiamen. Acta Oceanol. Sin. 22, 95–101.
Manlik O., McDonald J. A., Mann J., Raudino H. C., Bejder L., Krützen M., et al. (2016). The relative importance of reproduction and survival for the conservation of two dolphin populations. Ecol. Evol. 6, 3496–3512. doi: 10.1002/ece3.2130
Miller P. S. (2016). A population viability analysis for the Chinese White Dolphin (Sousa chinensis) in the Pearl River Estuary (Hong Kong: IUCN/SSC Conservation Breeding Specialist Group).
O’Grady J. J., Brook B. W., Reed D. H., Ballou J. D., Tonkyn D. W., Frankham R. (2006). Realistic levels of inbreeding depression strongly affect extinction risk in wild populations. Biol. Conserv. 133, 42–51. doi: 10.1016/j.biocon.2006.05.016
Parra G. J., Ross G. J. (2009). ““Humpback dolphins: S. chinensis and S. teuszii,”,” in Encyclopedia of marine mammals, 2nd ed. Eds. Perrin W. F., W€ursig B., Thewissen J. G. M. (London: Academic Press), 576–582.
Pe'er G., Matsinos Y. G., Johst K., Franz K. W., Turlure C., Radchuk V., et al. (2013). A protocol for better design, application, and communication of population viability analyses. Conserv. Biol. 27, 644–656. doi: 10.1111/cobi.12076
Peng C. W., Wu H. P., Wang X. Y., Zhu Q., Jefferson T. A., Wang C. C., et al. (2020). Abundance and residency dynamics of the Indo-Pacific humpback dolphin, Sousa chinensis, in the Dafengjiang River Estuary, China. Mar. Mammal Sci. 36, 623–637. doi: 10.1111/mms.12663
Reed J. M., Mills L. S., Dunning J. B. Jr., Menges E. S., McKelvey K. S., Frye R., et al. (2002). Emerging issues in population viability analysis. Conserv. Biol. 16, 7–19. doi: 10.1046/j.1523-1739.2002.99419.x
Robinson O. J., Lockwood J. L., Stringham O. C., Fefferman N. H. (2015). A novel tool for making policy recommendations based on PVA: Helping theory become practice. Conserv. Lett. 8, 190–198. doi: 10.1111/conl.12146
Sharpe M., Berggren P. (2019). Indian Ocean humpback dolphin in the Menai Bay off the south coast of Zanzibar, East Africa is critically endangered. Aquat. Conserv. 29, 2133–2146. doi: 10.1002/aqc.3221
Soulé M., Gilpin M., Conway W., Foose T. (1986). The millenium ark: how long a voyage, how many staterooms, how many passengers? Zoo Biol. 5, 101–113. doi: 10.1002/zoo.1430050205
Wang D., Liu R. J., Zhao Q. Z., Yang G., Xu X. R., Huang Z. G., et al. (2003). Pathological anatomy and analysis of death causes for a Chinese white dolphin. Acta Theriol. Sin. 23, 183–184. doi: 10.3969/j.issn.1000-1050.2003.02.015
Wang X., Miao X., Wu F., Yan C., Liu W., Zhu Q. (2012a). Investigation on the distribution of Sousa chinensis in the coastal waters between Xiamen and the Pearl River Estuary. J. Oceanogr. Taiwan Str. 31, 225–230. doi: 10.1007/s11783-011-0280-z
Wang X. Y., Wu F. X., Chang W. L., Hou W., Chou L. S., Zhu Q. (2016). Two separated populations of the Indo-Pacific humpback dolphin (Sousa chinensis) on opposite sides of the Taiwan Strait: evidence from a larger-scale photo-identification comparison. Mar. Mammal Sci. 32, 390–399. doi: 10.1111/mms.12257
Wang X. Y., Wu F. X., Zhu Q., Huang S. L. (2017). Long-term changes in the distribution and core habitat use of a coastal delphinid in response to anthropogenic coastal alterations. Aquat. Conserv. 27, 643–652. doi: 10.1002/aqc.2720
Wang J. Y., Yang S. C., Fruet P. F., Daura-Jorge F. G., Secchi E. R. (2012b). Mark-recapture analysis of the critically endangered eastern Taiwan Strait population of Indo-Pacific humpback dolphins (Sousa chinensis): Implications for conservation. B. Mar. Sci. 88, 885–902. doi: 10.5343/bms.2010.1097
Williams R., Lacy R. C., Ashe E., Hall A., Lehoux C., Lesage V., et al. (2017). Predicting responses of St. Lawrence beluga to environmental change and anthropogenic threats to orient effective management actions. DFO Can. Sci. Advis. Sec. Res. Doc. 027 v, + 44p.
Wu F. X., Wang X. Y., Ding X. H., Miao X., Zhu Q. (2014). Distribution pattern of Indo-Pacific humpback dolphins (Sousa chinensis) along coastal waters of Fujian Province, China. Aquat. Mamm. 40, 341–349. doi: 10.1578/AM.40.4.2014.341
Xu X. R., Song J. Y., Zhang Z. H., Li P., Yang G., Zhou K. Y. (2015). The world's second largest population of humpback dolphins in the waters of Zhanjiang deserves the highest conservation priority. Sci. Rep. 5, 8147. doi: 10.1038/srep08147
Xu X. R., Zhang Z. H., Ma L. G., Li P., Yang G., Zhou K. Y. (2012). Site fidelity and association patterns of Indo-Pacific humpback dolphins off the east coast of Zhanjiang, China. Acta Theriologica. 57, 99–109. doi: 10.1007/s13364-011-0058-5
Keywords: population viability analysis, Sousa chinensis, conservation, habitat improvement, population dynamic
Citation: Lu Y, Zhuo H, Xu X, Chen B and Yang G (2023) Assessing the effects of different management activities on the conservation of endangered Indo-Pacific humpback dolphin. Front. Mar. Sci. 10:1266735. doi: 10.3389/fmars.2023.1266735
Received: 25 July 2023; Accepted: 13 November 2023;
Published: 28 November 2023.
Edited by:
Todd Atwood, U.S. Geological Survey, United StatesReviewed by:
Xian Sun, Sun Yat-sen University, ChinaCopyright © 2023 Lu, Zhuo, Xu, Chen and Yang. This is an open-access article distributed under the terms of the Creative Commons Attribution License (CC BY). The use, distribution or reproduction in other forums is permitted, provided the original author(s) and the copyright owner(s) are credited and that the original publication in this journal is cited, in accordance with accepted academic practice. No use, distribution or reproduction is permitted which does not comply with these terms.
*Correspondence: Bingyao Chen, YnljaGVuQG5qbnUuZWR1LmNu; Guang Yang, Z3lhbmdAbmpudS5lZHUuY24=
Disclaimer: All claims expressed in this article are solely those of the authors and do not necessarily represent those of their affiliated organizations, or those of the publisher, the editors and the reviewers. Any product that may be evaluated in this article or claim that may be made by its manufacturer is not guaranteed or endorsed by the publisher.
Research integrity at Frontiers
Learn more about the work of our research integrity team to safeguard the quality of each article we publish.