- 1Department of Arctic and Marine Biology, The Arctic University of Norway (UiT), Tromsø, Norway
- 2Department of Biodiversity, Evolution and Adaptation, The British Antarctic Survey (BAS), UKRI, Cambridge, United Kingdom
- 3Department of Ecosystem Processes, Institute of Marine Research, Tromsø, Norway
Introduction: The Arctic sea ice extent in September (when it is at its lowest) has declined 13% Q10 per decade, and the Arctic Ocean is becoming a more Atlantic-influenced system. Rapid climate-forced changes are taking place in many high-latitude marine ecosystems. The Barents Sea is one such high-latitude shelf ecosystem, between approximately 70° and 80°N in the Norwegian Arctic. The purpose of the current study was to estimate zoobenthic blue carbon across multiple habitats within the Barents Sea (trough, basin, shelf, and shallows), potentially providing values to aid ecosystem-based management of these areas under future climate change scenarios.
Method: We tested this by capture and analysis of 947 high-resolution (each 405.7 × 340.6 mm, 12 MB, 5 megapixels) seabed images at 17 sites with latitudinal cline, linked to a collection of corresponding oceanographic data. Biotas within these images were identified to one of the 14 functional groups and the density was calculated. Mean stored carbon per individual was assigned by ash mass (AM) and ash-free dry mass (AFDM) of individuals caught within Agassiz trawl deployments at the same sites.
Results: Trough sites, except for one site (B16), have a low quantity of zoobenthic blue carbon compared with the shallow, shelf, and basin habitats.
Discussion: The results of a previous study focused entirely on trough habitats and are therefore difficult to scale up as the basis for a meaningful estimate of across-habitat zoobenthic blue carbon in the Barents Sea. Compared with the trough and the basin, the shelf and shallow habitats of the Barents Sea are also subjected to more trawling events through demersal fisheries and showed higher zoobenthic blue carbon stock values.
Introduction
The Arctic is a global hot spot for climate change, particularly the warming component (Masson-Delmotte et al., 2021). In response, the Arctic sea ice extent in September (when it is at its lowest) has declined by 13% per decade since 1979 (Arrigo et al., 2008; Stroeve et al., 2011). The Arctic Ocean is becoming a considerably more Atlantic-influenced system (Solan et al., 2020), with the Barents Sea carrying the largest fraction of sea ice loss in the Arctic, and could become completely ice-free in the summer by 2050 (Lind et al., 2018; Onarheim et al., 2018).
The Barents Sea Shelf ecosystem spans approximately 70° to 80°N in the northeastern Atlantic (Eriksen et al., 2017). The Barents Sea constitutes a biogeographical transition zone between a warmer boreal southern part and a cold Arctic northern part (Fossheim et al., 2015). The Barents Sea has warmed substantially over the last few decades with the expansion of relatively warm Atlantic water and the reduction in sea ice (Lundesgaard et al., 2022). The Barents Sea is said to be close to, or at, a tipping point, changing from an Arctic climate to an Atlantic climate as the water warms (Lind et al., 2018; Jørgensen, Pecuchet et al., 2022). The Barents Sea is also a highly productive Arctic-boreal ecosystem that hosts unique and one of the most biologically diverse regions of the Arctic (Denisenko et al., 2004). The Barents Sea, however, has a patchy distribution of benthic life, with considerable variability in species density and distribution (Bodil et al., 2011; Jørgensen et al., 2015; Jørgensen et al., 2016; Johannesen et al., 2016; Jørgensen et al., 2019; Bluhm et al., 2020; Csapo et al., 2021; Jørgensen, Logerwell et al., 2022; Jørgensen, Pecuchet et al., 2022). The inorganic carbonate mountain that extends approximately 100 km near Bjornøya is a good example of a patch of high benthic carbon storage (Węsławski et al., 2012).
A baseline survey of epibenthos using bottom trawls in 2022 found that the Barents Sea had at least 621 benthic taxa (ICES, 2022). In the Arctic systems, megabenthic communities comprise a significant part of benthic biomass and play an important role in carbon cycling on continental shelves (Zakharov et al., 2020). However, infauna also contribute significantly to carbon standing stocks (Zwerschke, Sands et al., 2021) and are important for further carbon sequestration through bioturbation (Solan et al., 2020). Benthic functional groups also vary there with latitude (Jørgensen et al., 2016; Jørgensen et al., 2019; Jørgensen, Pecuchet et al., 2022). The quantity of epibenthic blue carbon in the Barents Sea can be twice that of some Antarctic soft sediment continental shelves (Barnes and Sands, 2017; Souster et al., 2020).
Blue carbon is the term used for carbon captured and stored by marine ecosystems (Lovelock and Duarte, 2019). The reduction in Arctic sea ice leads to newly formed ice-free regions in the Barents Sea, allowing more light to penetrate the water column and thereby potentially to increase primary production. This can generate longer feeding periods for marine primary consumers, which, in turn, store more carbon. Ecosystem-based management could utilize nature-based solutions to mitigate climate change, including preventing reductions or destruction in the drawdown, storage, and burial of carbon by emergent natural systems (Bax et al., 2019; Barnes, Bell et al., 2021; Barnes, Sands et al., 2021; Zwerschke, Sands et al., 2021; Sands et al., 2023). Protecting benthic carbon sinks on the Arctic Ocean seafloor could help limit climate change (Barnes, Bell et al., 2021). Of all the biological carbon captured by photosynthetic activity, over half is captured by marine organisms (Nellemann et al., 2009). Research in the Sub-Antarctic found that zoobenthic blue carbon was not linked to any one particular functional group, but the accumulation and immobilization of blue carbon increased with the number of functional groups present (Barnes and Sands, 2017). The ability to sequester (long-term burial) carbon now has economic value, and the linkages between nature and the economy are often described using the concept of ecosystem services. Economists showed that, on the basis of the trajectories of two Intergovernmental Panel on Climate Change (IPCC) climate change scenarios, the Arctic Ocean could increase its storage of carbon to a value of between 27.6 billion and 1 trillion euros by 2099 (Armstrong et al., 2019). Placing monetary value estimates can help underline the importance of the ocean’s carbon storage service, informing policy and decision-making. The importance of blue carbon is evident (Hilmi et al., 2021), and the international blue carbon initiative was established to attempt to mitigate climate change through ecosystem management (https://www.thebluecarboninitiative.org). Understanding the environmental drivers that influence benthic blue carbon storage will be key to predicting how these systems will react to a changing climate in a setting of multiple, interacting stressors.
Habitat properties such as water depth, temperature, and sediment type (among others) can influence which species are present (Carroll et al., 2008; Husson et al., 2020). Research around New Zealand showed that benthic blue carbon stocks varied across habitats (Bulmer et al., 2020). This research also showed that unvegetated habitats contained the majority of carbon stocks in contrast to other more traditional blue carbon habitats (Bulmer et al., 2020). The Barents Sea is mostly comprised of unvegetated habitats, with 27 different biotopes confirmed by the MAREANO mapping project. Each of these contains elements of soft sediments and mud (www.mareano.no), which is likely to allow for greater carbon sequestration from epibenthos into sediments.
The climate is changing up to four times faster in the Norwegian Arctic than elsewhere on the globe (Rantanen et al., 2022). It is crucial to understand the implications of change (e.g., warmer seas, higher pH, retreating sea ice, freshening, etc.) for benthic ecosystems. Potentially less sea ice increases light availability and therefore primary production window and more carbon flux to the sea floor leading to more benthic storage. Benthic organisms can be used as an ecological tool for monitoring ecosystem health (Jayachandran et al., 2022). How changing sea ice dynamics will alter existing biological community composition and structure remains uncertain (März et al., 2021). The Arctic is also subjected to increased and cumulative anthropogenic stressors, such as trawling by fisheries, pollution, and noise (Jørgensen et al., 2020; Mikkelsen et al., 2023). Stressed marine ecosystems may be less resilient to climate variability challenges than unexploited marine ecosystems because of more redundancy in established communities and diversity of life history traits (Norling et al., 2007).
The Barents Sea holds one of the world’s largest fisheries of haddock and cod. In addition to this are crustaceans such as the king crab, snow crab, and deep-water shrimps and mollusks such as Islandic scallops (Johnson and Kovacs, 2009). After climate change and long-range pollutants, fishing is probably the human activity with the greatest impact on Barents Sea biodiversity. Bottom trawling has devastating effects on benthic communities (Denisenko et al., 2004). Bottom trawl fisheries are expanding into higher latitudes (Jørgensen et al., 2020; Pecuchet et al., 2020) despite a lack of in-situ studies of their impacts and therefore a limited understanding of the effects of trawling in these new areas (Kortsch et al., 2015; Jørgensen et al., 2019; Pecuchet et al., 2020). Fisheries and climate have been emphasized as major drivers of energy flows in marine ecosystems (Pedersen et al., 2021). The impact of trawling may obscure the effects of climate change on Arctic benthic communities (Souster et al., 2020). Changes in the density, diversity, and composition of mega-benthic communities associated with bottom fishing activity can affect biomass and biologically stored carbon (Jørgensen et al., 2016). Research in an Antarctic fjord for example showed that it took 10 years for benthic communities to recover from disturbance events such as an iceberg scouring, even in the productive shallows (Zwerschke, Morley et al., 2021). Iceberg scouring has a similarly destructive impact as trawling but is typically much less frequent (Smale et al., 2007; Smale et al., 2008). A perturbation study of two fjords in Svalbard showed that it took between 13 and 24 years to recover from disturbance and to return to previous community compositions (Al-Habahbeh et al., 2020).
This paper aims to i) quantify zoobenthic blue carbon (g m−2/tkm−2) and the percentage of functional group distribution within epibenthic marine communities in the trough, shelf, basin, and shallow areas of the Barents Sea; ii) estimate the future potential of Barents Sea benthic communities as part of nature-based solutions (by storing carbon) within these habitats; and iii) evaluate a multistressor approach on the quantity of benthic blue carbon and functional groups. Our null hypothesis is that there is no difference between known trawled and untrawled areas (anthropogenic perturbations) and that there is no stored carbon difference coincident with the concentration of ice and other environmental variables.
Methods
The study area
This study was conducted in the Norwegian sector of the Barents Sea (Figure 1).
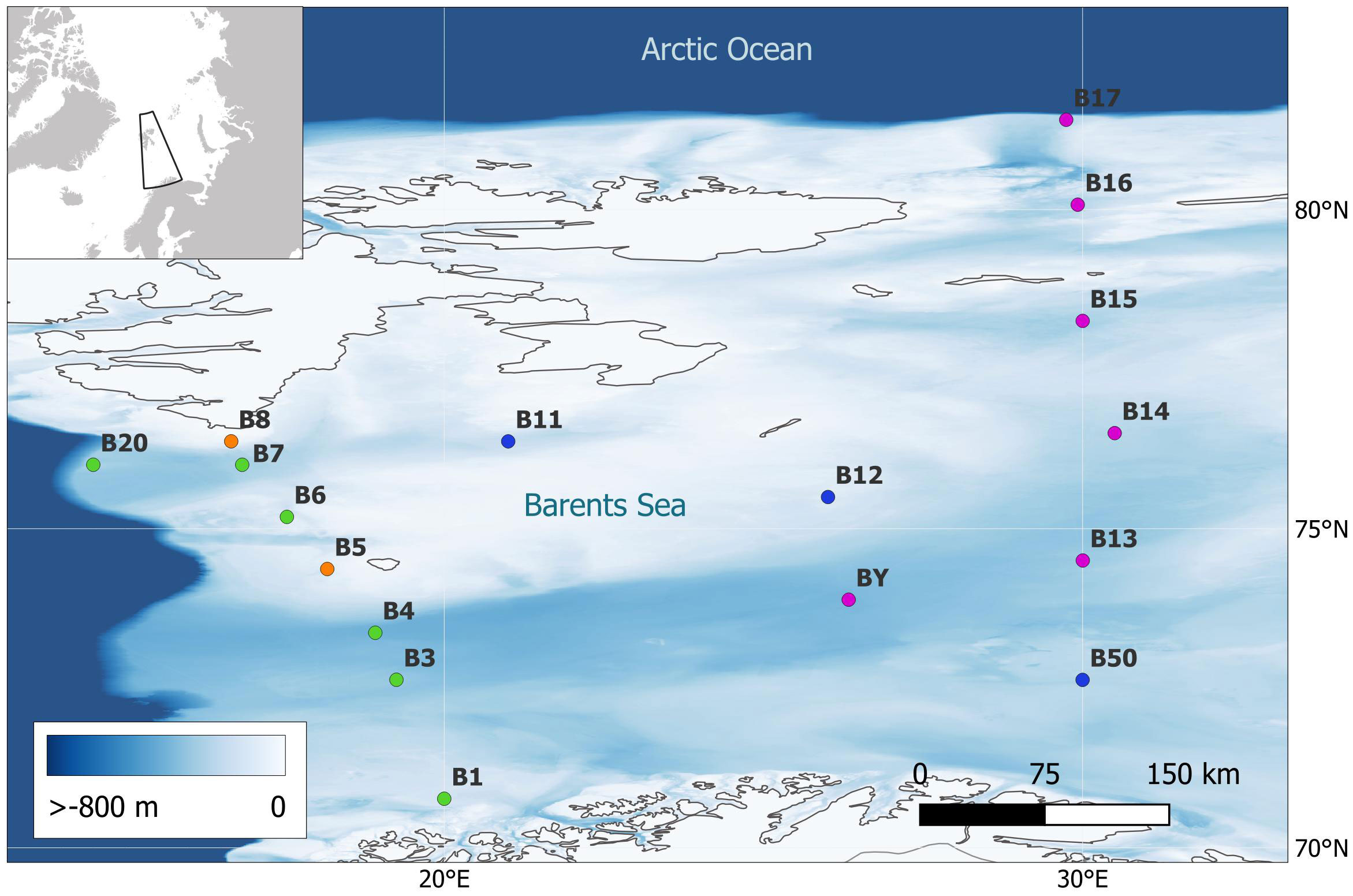
Figure 1 Locations of the study’s 17 sites, across different habitats: trough (pink), basin (purple/blue), Norwegian continental shelf (green), and shallows (orange).
The Barents Sea is a continental shelf sea north of Norway and west of Russia, covering ca. 1.6 million km2 and has an average depth of 230 m (Jakobsson et al., 2004). The Barents Sea is a biogeographical transition zone between a warmer boreal southern part and a cold Arctic northern part (Eriksen et al., 2017). Warm and saline Atlantic waters flow into the southwestern Barents Sea from the Norwegian Sea (Grøsvik et al., 2018).
Digital collection of the samples
Sampling with the Shallow Underwater Camera System (SUCS) was undertaken at 17 sites (Figure 1), across four different habitats (trough, basin, shelf, shallows) during two ChAOS (Changing Arctic Oceans, https://www.changing-arctic-ocean.ac.uk/project/chaos/) cruises in 2017 and 2019 (JR17007 and JR18006, respectively). SUCS is a non-invasive monitoring technique that captures epibenthos but does not measure infauna. SUCS was deployed with a high level of replication at each site, capturing a total of 947 high-resolution seabed images (Figure 2, each 405.7 × 340.6 mm, 12 MB, 5 megapixels).
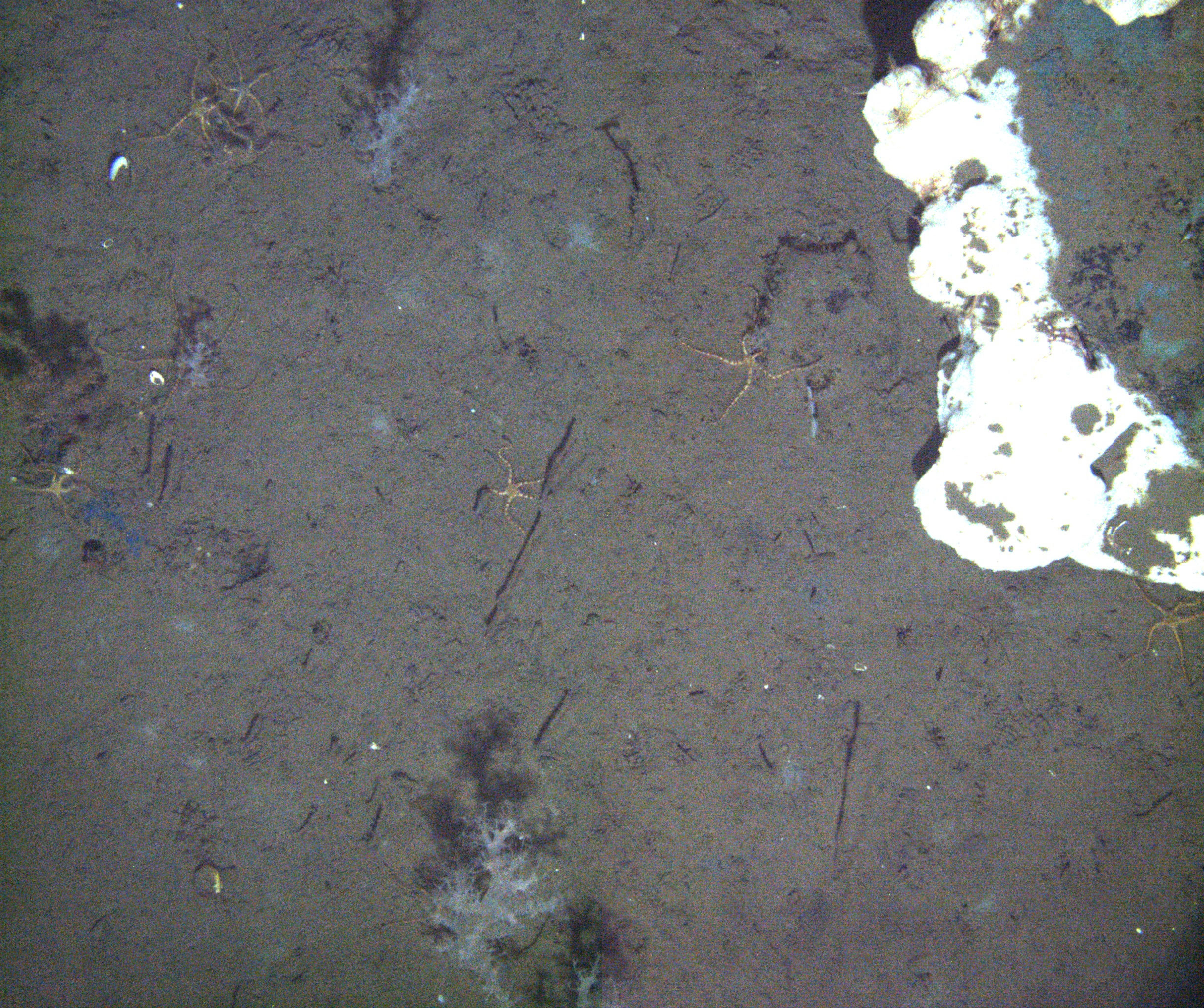
Figure 2 This image (405.7 × 340.6 mm, 12 MB, 5 megapixels) of site B16 is one of the 947 images taken in this study.
Functional groups
Images were annotated for the density of each of the 14 functional groups (Table 1) of benthos per m2. The selected functional groups were targeted around factors important for carbon pathway potential, in accordance with Barnes and Sands (2017), allowing the investigation of vulnerability to climate change and the effects on ecosystem function within a practical time frame and skills matrix, which is crucial to assess such rapidly changing regions as the Arctic.
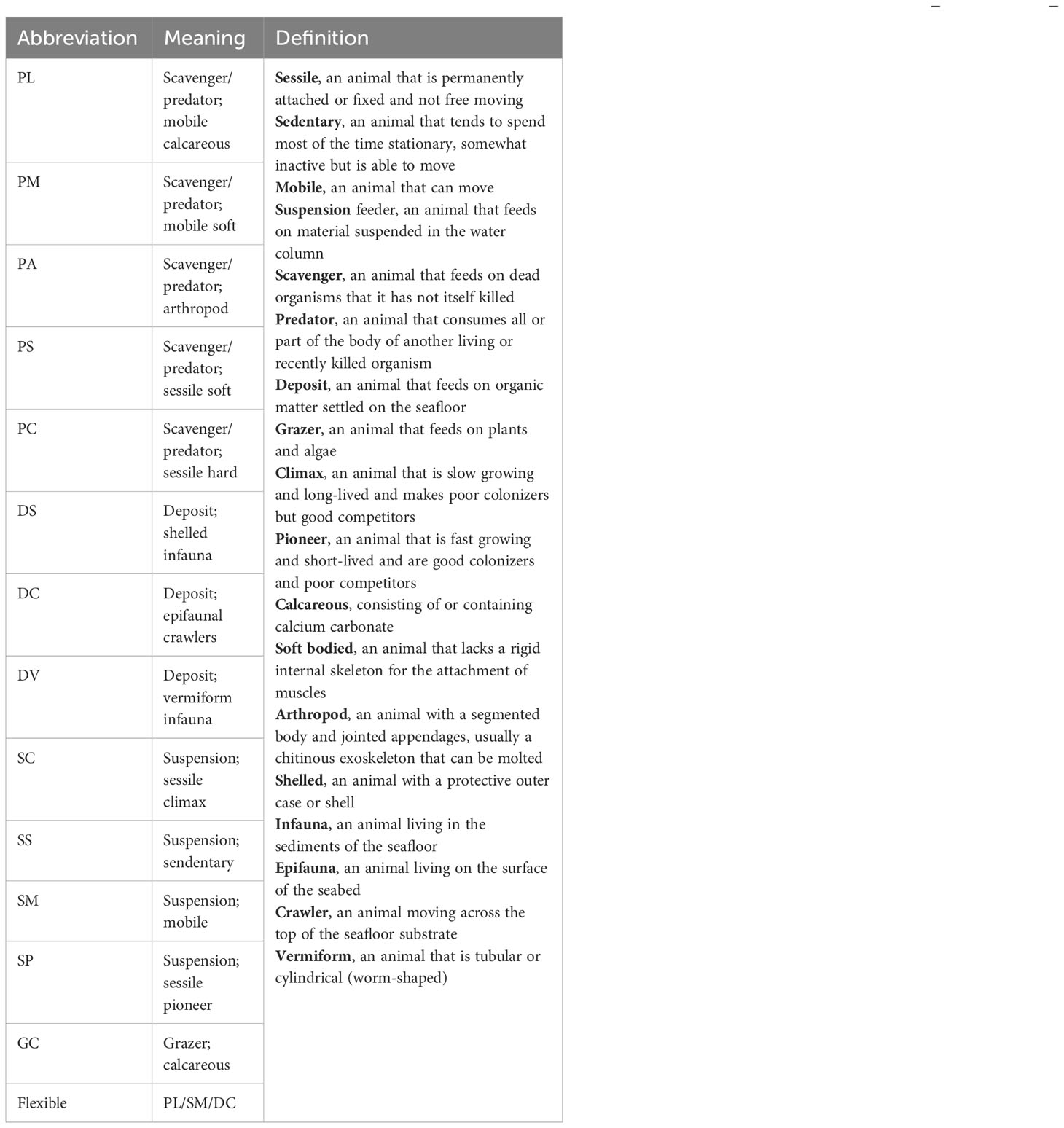
Table 1 The abbreviations and descriptions of each functional group [see Barnes and Sands (2017) for more explanation of the selection of the functional groupings].
Biological benthic sampling
Three Agassiz trawl deployments collected zoobenthos at each location after SUCS deployments by means of 5-min trawls at 0.5 knots. The Agassiz trawls were 125 cm long, 41 cm wide, and 85 cm high. The length of the cable for the trawl was 1.5 times the water depth at the site sampled. Trawled sample specimens were separated into different morphotypes and preserved in ethanol for morphometrics in the laboratory. Each morphotype was then dried at 60°C for 24 h, the dry mass (DM) was weighed and then subsequently ashed in a furnace at 475°C for 12–24 h, and the ash mass (AM) was weighed. The total carbon content was calculated as 50% of the organic mass (DM − AM) plus 12% of the inorganic skeleton mass (Souster et al., 2018). For each image, the mean blue carbon mass of each functional group was multiplied by the total number of individuals from this functional group and summed to give a standing stock of epi-zoobenthic blue carbon per image/area.
Abiotic sampling
The environmental variables for each site were measured near-seabed (typically 10 m above the seafloor) and included temperature, salinity, chlorophyll-a fluorescence, and oxygen, as obtained from the onboard CTD (conductivity–temperature–depth) casts using an SBE 911plus fitted with an auxiliary SBE43 oxygen sensor and a Chelsea MKIII Aquatracka fluorometer (ChAOS Cruise Polar Data Centre). Salinity and oxygen were both calibrated against in-situ samples. Rugosity was indicated numerically and deciphered by shadows shown on the images (0 smooth, 1 up to 10 mm, 2 up to 20 mm). The sea ice history for each site was calculated as the number of sea ice days per year across 18 years (in which a sea ice day was classified as having over 85% sea ice concentration), using sea ice remote sensing data supplied by the Polar Data Centre at the British Antarctic Survey. Habitats (trough, basin, shelf, and shallows) within the Barents Sea were characterized by previous bathymetric surveys of the region.
Anthropogenic trawling data
Fisheries can impact benthic communities by means of disturbance altering potential zoobenthic carbon values. To calculate the demersal fishing pressure for each site that was potentially impacted by trawling, data from Global Fishing Watch (https://globalfishingwatch.org/) were extrapolated on a 5-km × 5-km grid scale. Global Fishing Watch shows data for all fishing activities globally from boats with AIS (automatic identification systems), and in Norway, boats larger than 15 m and, therefore, all trawling vessels have been required by law to have AIS since 2009. The current study only considered benthic communities and therefore only focused on bottom trawling gears that have contact with the seabed. Using the R studio package, we extracted trawling activities for the Barents Sea region (70–82°N and 16–30°E) from 2013 to 2019 and then just for the grid cells where we had sampled. We extracted data for the total number of hours trawled within each grid cell containing our sample site as well as the frequency of trawling and average trawling hours for each year between 2013 and 2019.
Habitat area measurements
This study aimed to estimate blue carbon storage across four different habitats within the Barents Sea: shelf, trough, basin, and shallows. Using GIS software, existing maps for the Barents Sea (GEBCO and NGU), and scientific literature (Jørgensen, Pecuchet et al., 2022) on benthic transition zones, the estimated area coverage for each habitat (see supplementary for map and details) was calculated.
Statistical analysis
Samples
We captured 947 images across 17 sites in the Barents Sea. These were annotated for functional groups and total biologically stored carbon, as described above. Statistical analysis was carried out using the PRIMER-E software version 7 (PRIMER-e). The zoobenthic carbon content was averaged across each site, and the variability of the data of the carbon content was shown with standard deviation (SD). There were five trough sites (from Souster et al., 2020) that were sampled in both 2017 and 2019. A one-way analysis of similarities (ANOSIM) tested the samples from the five sites repeated in both years to see if the null hypothesis (no difference in zoobenthic carbon value between years) was rejected or not. If year was non-significant as a factor, then samples from both years could be pooled for analysis. A non-multidimensional scaling (nMDS) model with Bray–Curtis similarity measures on fourth root-transformed carbon data was used for the biological data. The average similarity (SIMPER) procedure was used to see which functional groups if any contributed to the difference in carbon storage across sites.
A principal component analysis (PCA) with standardized Euclidean distance was used to determine which environmental variables could best explain the observed variability in the functional groups or zoobenthic carbon data. The anthropogenic stress of trawling by fisheries was included as a factor to investigate if there was any correlation between epi-zoobenthic blue carbon and increased fishing pressure. To examine which relationships existed between the biological variables (functional groups and zoobenthic carbon) and the environmental variables and which environmental variable was most coincident with patterns in the biological data, we used the RELATE procedure (PRIMER-e). The RELATE model using Spearman’s rank coefficient was used to explore how well the biological matrix (zoobenthic carbon content) correlated with the results of the environmental matrix. The BEST BVSTEP procedure was used to identify the individual environmental variables that best explained the biological patterns.
Results
Zoobenthic Barents Sea blue carbon
One of the aims of this research was to quantify zoobenthic blue carbon across multiple habitats within the Barents Sea, which we achieved with some precision through in-situ measurements and replication. Mean zoobenthic carbon varied from the lowest value of 2.51 g m−2 at site B11 in 2017 (Table 2), followed by 2.96 g m−2 at site B15 in 2017, to the highest value of 34.42 g m−2 at site B20 in 2017. The variation in zoobenthic carbon quantity found within sites and between sites is shown by the standard deviation (Table 2). There was no significant difference between years at the five sites that were sampled in both 2017 and 2019 (Table 2, 2019 sites in bold to be clear), where replicates were pooled. Shelf and shallow habitats in the Barents Sea had the largest average quantity of benthic blue carbon per m2 compared with basin and trough sites. Except for site B16, the trough sites had low zoobenthic blue carbon values. The mean zoobenthic carbon value across all basin sites was 12.21 g m−2, trough sites 10.94 g m−2, all shelf sites 17.09 g m−2, and the shallow sites 21.43 g m−2. The estimated area for each habitat in the Norwegian sector of the Barents Sea and the estimated zoobenthic carbon value for these habitats are shown in Table 2.
Functional groups
As with zoobenthic carbon values, no relationship was found between the environmental variables and the number of functional groups present at the different sites (RELATE model little correlation when R was near zero, R = 0.13, p < 0.001 Spearman’s rank coefficient). Of the 14 functional groups, there was no particular functional group that stood out as making a larger contribution to zoobenthic carbon storage than the other functional groups. The average dissimilarity in functional groups between sites was always less than 50% when using the similarity percentage (SIMPER) analysis to see which functional groups were responsible for any differences.
Environmental variation
Topographically, the Barents Sea is characterized by basins, shelves, and shallows bisected by a central trough. Initially, we attempted to separate habitats based on the environmental variables we measured. However, distinct habitats did not emerge when all variables were taken into consideration, although the PCA dissimilarity modeling indicated a division between shelf and trough (Figure 3). None of the environmental variables were significantly correlated with quantities of zoobenthic blue carbon. Certain environmental variables such as the number of days of sea ice cover er year and trawl frequency data did not change between the 2 years investigated as these were averaged over a time frame (Table 3). The environmental variables were averaged for the site, with temperature ranging from −1.83°C at the northern Arctic site B16 to the warmer Atlantic waters of +6.64°C at B1 (Table 3). Chlorophyll-a (μg−1) was the lowest at 0.02 μg−1 at B20, B3, B16, and B17 with the highest value at B5 of 0.23 μg−1 (Table 3).
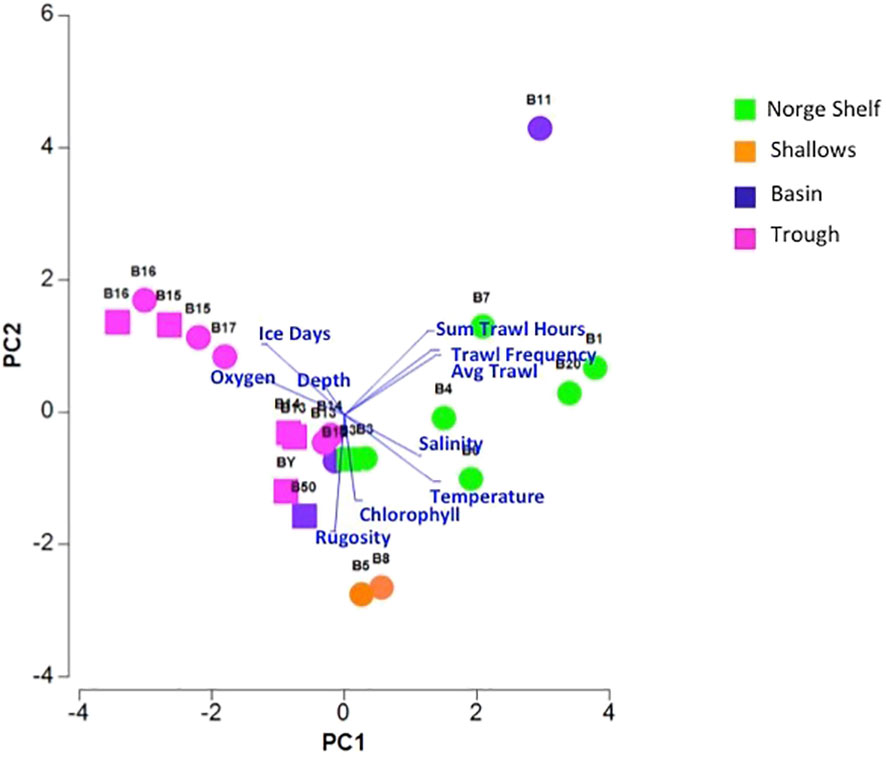
Figure 3 PCA plot of environmental variables and trawling impact. Squares are for sites in 2019, and circles are sites surveyed in 2017 (see Table 3 for further descriptions of data included in the PCA).
Although certain environmental variables such as near seabed temperature and number of sea ice days per year did not correlate with zoobenthic carbon storage value, there was a latitudinal cline in their values from warmer Atlantic waters in the south (B20) to colder Arctic waters in the north B16 (B17 is subjected to Atlantic inflow waters from the north of Svalbard) which is why it is warmer than B16 (Figure 3). The shallows showed a high value in zoobenthic blue carbon and had a greater concentration of chlorophyll-a, higher rugosity value, and greater variation in substrates. The shelf sample sites are separated by anthropogenic trawling effort and have greater frequency and duration of trawling effort compared with other habitats (Figure 3). The PCA explained 62.7% of the variation in the first two PCAs (eigenvalue PCA1 36.6, PCA2 26.1).
Discussion
Polar and subpolar seas show considerable physical responses to ongoing climate change, notably sea ice losses in time and space and the warming of near surface waters (Turner and Comiso, 2017). However, there is much geographical complexity in the magnitude, timing, and trend of such responses and less clarity about the effects of these changes on biodiversity and ecosystem functioning in marine habitats. It is not yet apparent that Arctic or east Antarctic biota follow a pattern of altered phytoplankton production (Arrigo et al., 2008; Rogers et al., 2020) and increased carbon storage in benthos (Barnes, 2015; Barnes et al., 2018) as estimated on West Antarctic continental shelves. Most apparent in the Antarctic are the emergent carbon sinks in habitats experiencing ice shelf loss (Peck et al., 2010) or rapid glacier retreat along fjords (Zwerschke, Sands et al., 2021) and would expect similar trends for the Arctic.
The initial aim of the Natural Environment Research Council (NERC)-funded project, ChAOS (https://www.changing-arctic-ocean.ac.uk/), in 2017 was to understand and quantify the impacts of climate change on Arctic ecosystems and their global consequences. Our project within ChAOS set out to investigate any effects of retreating Arctic seasonal sea ice (newly ice-free areas) on Barents Sea benthic blue carbon (Souster et al., 2020) and to identify strategies to best safeguard emergent zoobenthic blue carbon (negative feedback on climate change). Our previous work (Souster et al., 2020) explored sites along a latitudinal cline within trough habitats. This showed a significant correlation between flow rate and benthic blue carbon values, but no correlation was found with past recent sea ice duration. One possibility for why no such link was apparent was that the effect of sea ice retreat on Arctic benthic blue carbon and functional groups could have been masked by differential effects of anthropogenic trawling pressures in the Barents Sea (Jorgensen, Planque et al., 2015). Polar marine animals typically live long lives, with many of the longest known life spans of fauna being in the Arctic. This is true for polar benthic species in which it has been quantified; for example, sponges have been dated as living >300 years (Morganti et al., 2022). Arctic benthic life span varies considerably with taxon type, and those with considerable skeletal investment may persist even thousands of years after death (Barnes, Kuhn et al., 2021) However, while many Arctic benthos living below iceberg scour depths (>50 m) have the potential to live long lives, how often this occurs is not known as few species there have been accurately aged. Frequent trawling in some areas means that such potential may often not be realized.
The aim of the current study was not the quantification of benthic carbon budgets or carbon cycling but to measure and analyze one component of these, which was carbon storage and potential for sequestration. Carbon pathway losses through respiration and microbial breakdown on death are important parts of the pathways, the latter estimated to account for 50% of primary production fate and >90% of secondary production fate (Henley et al., 2020). Souster et al. (2020) and Barnes (2017) estimated that >0.5% of zoobenthic production is ultimately sequestered, which is not dissimilar to carbon sequestration rates in many systems. Respiration losses of Barents Sea benthos are much more difficult to account for because most benthos live only in deep waters (>50 m), and thus, bringing them to the surface for experimental physiology exposes them to considerable stresses, which is often fatal. What has been determined from shallow water polar SCUBA work is that basal respiration (oxygen use) levels of polar benthos tend to be very low (Souster et al., 2018).
In the current study, we show that sites with lower blue carbon values were mostly those of trough habitat (exception B16, Table 2, Figure 4). Thus, scaling up estimates from the previous study in 2020 could massively underestimate zoobenthic blue carbon in the Barents Sea. The sites with the largest carbon values (B8, B1, B20, B12, B16) also have many suspension feeders dominating those sites (SP, SC, SS, see table in the Methods section) which are likely to be vulnerable to anthropogenic impacts such as trawling. For example, Jørgensen et al. (2019) found patches of high benthic biomass in the South West of the Barents Sea (sites B8, B1, B20, and B12) created by suspension-feeding Geordia sponge fields influenced by Atlantic water. Here, we show that recent anthropogenic trawling activity is greater in duration and frequency in shelf areas (Table 3). This might be expected as it is typically an area of higher productivity, so this might support strong fish populations and thus fisheries. The shelf habitat areas could have greater carbon storage due to higher overlaying primary productivity, but there was no correlation between trawling and zoobenthic carbon storage found by this study. Jørgensen et al. (2019) identified 23 high-risk benthic species with respect to trawling and also showed a decline in benthic biomass when comparing trawled versus untrawled areas. In contrast to this study, the Jørgensen et al. (2019) study was based on wet mass rather than carbon storage values and trawled samples rather than images. Buhl-Mortensen et al. (2016) also showed that benthic density on the Barents Sea shelf was negatively correlated with trawling intensity using video transects and satellite-based vessel identification as a proxy for fishing effort.
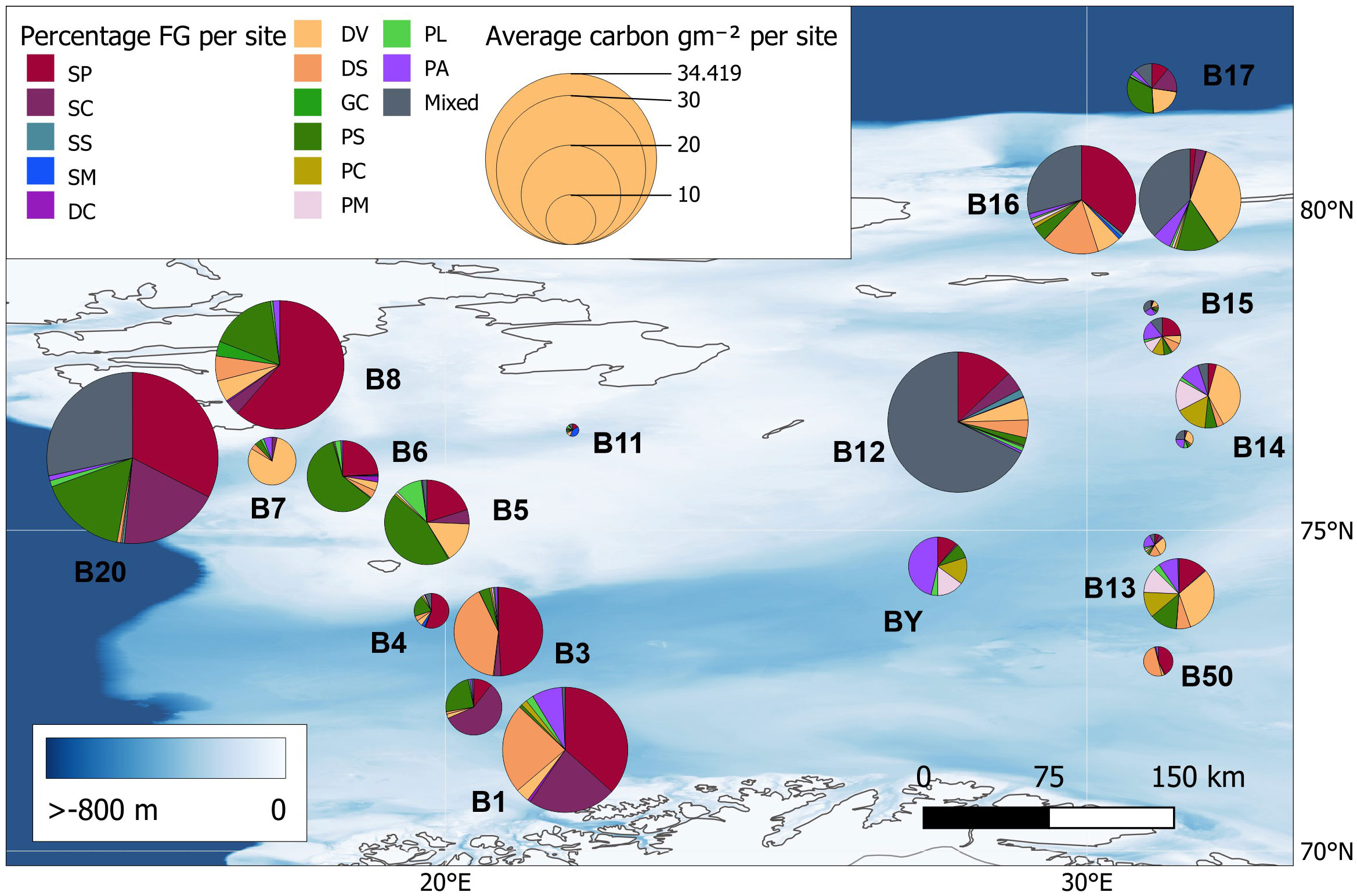
Figure 4 The percentage of each functional group (FG) at each site (see methods’ descriptions of functional groups) displayed as pie charts. The size of each chart corresponds to the average carbon value for that site (see Table 1 for trait descriptions and Table 2 for quantities of blue carbon).
Three of the sites (B1, B8, and B20) are in Atlantic-influenced (Atlantification) water masses and an area of high productivity, and it is therefore not surprising to see larger zoobenthic stored carbon values. The Barents Sea Atlantic ecosystem is fueled by an annual gross primary production of approximately 120 g m−2 compared with 60 g m−2 in seasonally ice-covered areas further north (Reigstad et al., 2011). Higher primary production input suggests that there is more potential food supply for benthos in the southern part of the Barents Sea. Phytoplankton blooms in the Arctic Ocean’s seasonal sea ice zone are expected to start earlier and occur further north with retreating and thinning sea ice cover (wherever blooms were light-limited). Previously, the marginal ice zone was considered the most productive region in the area, but Dybwad et al. (2021) revealed intense blooms and high export events in ice-covered waters of the Barents Sea, and therefore, food supply for benthos may not be as limited in ice-covered sites as we had thought. The challenge remains to understand how well represented ice versus pelagic algae are within benthic consumer diets. Cautain et al. (2022) showed that ice algae can make up to 95.8% of assimilated carbon by invertebrates; however, Søreide et al. (2013) showed that benthos use a high proportion of particulate organic matter (POM) within their diet. Research using stable isotopes to look at carbon movement from pelagic to benthic organisms in the Barents Sea has been carried out. However, they found that ice algae were not isotopically distinct enough and there was so much overlap in isotope “space” with pelagic phytoplankton or sediment organic matter to tease these issues open.
In a previous study, Souster et al. (2020) also found no obvious relationship of trough zoobenthic carbon storage with sea ice and other measured environmental variables. One possibility of why no biological and environmental correlation was found was not allowing for cumulative anthropogenic impacts such as trawling. Couce et al. (2020) showed that trawling effort can be the most important predictor of benthic community composition. The nature of physical disturbance created by trawling on the bottom can depend on many factors such as sediment composition, topography, trawling speed, construction, and weight of trawling equipment (Gray and Elliott, 2009; O’Neill and Ivanović, 2016). However, there are possibilities other than trawl disturbance differences for why no obvious environmental drivers were found to influence zoobenthic carbon storage in the current study. The Barents Sea has a very patchy distribution of benthic fauna (Bodil et al., 2011; Jørgensen, Ljubin et al., 2015; Zakharov et al., 2020) which in combination with low sample effort could hide correlations between biological and environmental variables. Barents Sea zoobenthic carbon can have high variability within and between sites, and here, this variability is shown to extend within and between different habitats (Souster et al., 2020; Table 1). The research by Cochrane et al. (2012), looking at benthic fauna and functional traits in the Barents Sea for ecosystem assessments, found that taxon richness and overall faunal biomass were the highest at the shallowest stations and the lowest in the depressions and ice-influenced areas, which supports our results found in the current research.
Loss of sea ice means some areas have the potential to experience a longer duration of phytoplankton blooms, which can translate to more time for benthos to feed, grow, and therefore give more carbon storage potential (Barnes, 2017). The current study attempted to test whether this could be detected in an Arctic sea with a more accurate representation of zoobenthic blue carbon in the Barents Sea. However, the challenge remains to find a causal relationship between this aspect of biology and potential environmental drivers. The relationship between environmental variables and the zoobenthic carbon values could be masked by the fact that environmental conditions were only measured at one time point during the year and of course conditions at our sample sites will change through the seasons; however, studies in Antarctica showed no change in zoobenthic blue carbon with seasons (Morley et al., 2022). Environmental variables did not facilitate defining specific habitats from our sample sites; however, a PCA cluster did show an obvious separation of environmental variables between the Norge shelf and trough sites and also showed an expected gradual change across sites, shown by Jørgensen, Ljubin et al. (2015) (Figure 3). Of the environmental variables, temperature, oxygen, and rugosity showed the best correlation with zoobenthic blue carbon; however, it was only a slight correlation (R = 0.224 RELATE model). The sites BY, B50, B6, B8, and B5 had high rugosity and less benthic blue carbon; however, B11 had low rugosity and low zoobenthic carbon so other variables also need to be taken into consideration. Nicastro and Bishop (2013) showed that variability in community composition can be coincident with changes in environmental variables, such as sediment grain size which we have not measured in this study. Another benthic Arctic study found that the structure of benthic communities can be distinct at each station, despite sampling areas with similar sediment types on the Barents shelf (Renaud et al., 2008). The PCA did show that anthropogenic trawling activities were greater in both frequency and duration along the shelf habitat which might be expected due to greater productivity and possibly greater quantity of fish within the southwestern section of the Barents Sea (Jorgensen, Planque et al., 2015).
The Barents Sea may be important for blue carbon natural capital, but it is also a region with multiple pressures from various anthropogenic sectors such as trawling from fisheries, pollution from oil and gas, noise disturbance, and litter, with the addition of extreme climate warming (Mikkelsen et al., 2023). Such cumulative and interacting anthropogenic impacts can mean reduced resilience across the marine ecosystem.
Conclusions and further work
We are in a climate emergency (IPCC, 2022) and new emphasis is being placed on protection for carbon-rich ecosystems to utilize their highly efficient storage to sequestration of greenhouse gas CO2 (Portner et al., 2021). Identifying blue carbon habitats and their threats and reversing decline are high on this priority list. Thus, quantifying blue carbon storage from non-conventional marine ecosystems such as epibenthos is extremely important, but this aids action on climate change and biodiversity loss only if it leads to properly protecting those marine communities so that carbon remains stored or becomes sequestered from the carbon cycle.
The Barents Sea is a very productive system and therefore has the potential to capture carbon through phytoplankton, store this when consumed, and eventually sequester it into the in-situ shelf sediments (März et al., 2021). The zoobenthos within the Barnes Sea potentially stores approximately 10 million tons of carbon per km−2 and therefore could be a good carbon sink if managed in the right way.
The Barents Sea shelf is a mud basin that is likely to be very important for longer-term sequestration. We know from a study in the Antarctic (Zwerschke, Sands et al., 2021) that infauna also contribute significantly to carbon standing stocks; therefore, measuring epibenthos in this study gives us a baseline estimate with the knowledge of the actual carbon storage contributed by benthos will be far greater. Preventing destruction and disturbance to benthic blue carbon hot spots, particularly in long-lived ecosystems, is emphasized as a priority (nature-based climate solutions) (Barnes, Bell et al., 2021) because there are very few ways of storing carbon more efficiently. Rather than having very large marine protected areas (MPAs) with little or no protection and management, we need meaningful protection in priority areas (Queiros et al., 2021). Currently, there is a drive to have 30% of our oceans protected by 2030 (Sustainable Development Goal SDG, 2030), but there is a difference between drawing lines on a map and actively managing and protecting an area. There is a critical need for integrated ecosystem-based management connecting researchers, managers, and stakeholders such as in fisheries. There is also a need for multidisciplinary research between marine geologists, biogeochemists, and ecologists when quantifying carbon sequestration into the sediments. The goal of this project was to quantify zoobenthic blue carbon storage in the Barents Sea across a range of habitats which we have achieved; however, there is still uncertainty around the drivers behind this zoobenthic blue carbon storage. The hope is that synthesis such as this will lead to informed decision-making and conservation for areas of high benthic blue carbon values. Placing a value on changes to carbon capture with climate change helps articulate the importance of the carbon storage service to society (Armstrong et al., 2019). The anthropogenic activity of demersal trawling does negatively impact benthic ecosystems (Rijnsdorp et al., 2016) and therefore zoobenthic carbon storage; however, this is challenging to quantify. It is therefore important to protect areas of high carbon storage values so that the crucial regulatory ecosystem service of maintaining our resources through climate regulation and carbon sequestration remains. We need to identify and protect areas of high zoobenthic carbon storage potential, but we also need to understand what drives the high blue carbon storage to better understand the implications of climate change on future carbon storage values.
The frequency and intensity of anthropogenic stressors and the sensitivity and resilience of the ecosystem influence the impacts of climate change on blue carbon ecosystems and their carbon stocks. Sala et al. (2021) found that a substantial increase in ocean protection should secure marine carbon stocks that are at risk from human activities. They also suggested that protecting a carbon-rich seabed should be an important part of potential nature-based solutions to limit climate change. To mitigate multiple stressors, both climatic and anthropogenic, research should focus on environmental and economic benefits to prioritize the safeguarding of blue carbon ecosystems (Barnes, Bell et al., 2021).
Data availability statement
The raw data supporting the conclusions of this article will be made available by the authors, without undue reservation.
Author contributions
TS: Conceptualization, Formal Analysis, Investigation, Methodology, Project administration, Software, Visualization, Writing – original draft, Writing – review & editing. DB: Conceptualization, Methodology, Resources, Supervision, Validation, Visualization, Writing – review & editing. RP: Supervision, Writing – review & editing. LJ: Writing – review & editing.
Funding
The author(s) declare financial support was received for the research, authorship, and/or publication of this article. Funding from two sources firstly Changing Arctic Oceans NERC grant UK NE/P006493/1 and secondly BarentsRisk Norwegian research Council 288192.
Acknowledgments
Dr. Rebecca Ross (Institute of Marine Research, IMR, Bergen) assisted with GIS and area measurements of different habitats across the Barents Sea using data from the Norwegian mapping project MAREANO Home | Mareano - The Sea in Maps and Pictures and the IMR Ecosystem survey data Page 26 BS Ecosystem 04May2021.pdf (nersc.no).
Conflict of interest
The authors declare that the research was conducted in the absence of any commercial or financial relationships that could be construed as a potential conflict of interest.
Publisher’s note
All claims expressed in this article are solely those of the authors and do not necessarily represent those of their affiliated organizations, or those of the publisher, the editors and the reviewers. Any product that may be evaluated in this article, or claim that may be made by its manufacturer, is not guaranteed or endorsed by the publisher.
References
Al-Habahbeh A. K., Kortsch S., Bluhm B. A., Beuchel F., Gulliksen B., Ballantine C., et al. (2020). Arctic coastal benthos long-term responses to perturbations under climate warming. Philos. Trans. A Math Phys. Eng. Sci. 378 (2181), 20190355. doi: 10.1098/rsta.2019.0355
Armstrong C. W., Foley N. S., Slagstad D., Chierici M., Ellingsen I., Reigstad M. (2019). Valuing blue carbon changes in the Arctic ocean. Front. Mar. Sci. 6. doi: 10.3389/fmars.2019.00331
Arrigo K. R., van Dijken G., Pabi S. (2008). Impact of a shrinking Arctic ice cover on marine primary production. Geophysical Res. Lett. 35 (19), 19603. doi: 10.1029/2008GL035028
Barnes D. K. A. (2015). Antarctic sea ice losses drive gains in benthic carbon drawdown. Curr. Biol. 25 (18), 789–790. doi: 10.1016/j.cub.2015.07.042
Barnes D. K. A. (2017). Polar zoobenthos blue carbon storage increases with sea ice losses, because across-shelf growth gains from longer algal blooms outweigh ice scour mortality in the shallows. Global Change Biol. 23, 5083–5091. doi: 10.1111/gcb.13772
Barnes D. K. A., Bell J. B., Bridges A. E., Ireland L., Howell K. L., Martin S. M., et al. (2021a). Climate Mitigation through Biological Conservation: Extensive and Valuable Blue Carbon Natural Capital in Tristan da Cunha’s Giant Marine Protected Zone. Biol. (Basel) 10 (12). doi: 10.3390/biology10121339
Barnes D. K. A., Fleming A., Sands C. J., Quartino M. L., Deregibus D. (2018). Icebergs, sea ice, blue carbon and Antarctic climate feedbacks. Philos. Trans. A Math Phys. Eng. Sci. 376 (2122). doi: 10.1098/rsta.2017.0176
Barnes D. K. A., Kuhn G., Hillenbrand C. D., Gromig R., Koglin N., Biskaborn B. K., et al. (2021b). Richness, growth, and persistence of life under an Antarctic ice shelf. Curr. Biol. 31 (24), R1566–R1567. doi: 10.1016/j.cub.2021.11.015
Barnes D. K. A., Sands C. J. (2017). Functional group diversity is key to Southern Ocean benthic carbon pathways. PloS One 12 (6), e0179735. doi: 10.1371/journal.pone.0179735
Barnes D. K. A., Sands C. J., Paulsen M. L., Moreno B., Moreau C., Held C., et al. (2021c). Societal importance of Antarctic negative feedbacks on climate change: blue carbon gains from sea ice, ice shelf and glacier losses. Naturwissenschaften 108 (5), 43. doi: 10.1007/s00114-021-01748-8
Bax N., Moreno B., Moreau C., Barnes D. K. A., Paulsen M., Held C., et al. (2019). “Carbon storage by Kerguelen zoobenthos as a negative feedback on climate change,” in The Kergeulen Plateau: Marine Ecosystem and Fisheries. Proceedings of the second symposium.
Bluhm B. A., Janout M. A., Danielson S. L., Ellingsen I., Gavrilo M., Grebmeier J. M., et al. (2020). The pan-arctic continental slope: sharp gradients of physical processes affect pelagic and benthic ecosystems. Front. Mar. Sci. 7. doi: 10.3389/fmars.2020.544386
Bodil B. A., Ambrose W. G., Bergmann M., Clough L. M., Gebruk A. V., Hasemann C., et al. (2011). Diversity of the arctic deep-sea benthos. Mar. Biodiversity 41 (1), 87–107. doi: 10.1007/s12526-010-0078-4
Buhl-Mortensen L., Ellingsen K. E., Buhl-Mortensen P., Skaar K. L., Gonzalez-Mirelis G. (2016). Trawling disturbance on megabenthos and sediment in the Barents Sea: chronic effects on density, diversity, and composition. ICES J. Mar. Sci. 73 (suppl_1), i98–i114. doi: 10.1093/icesjms/fsv200
Bulmer R. H., Stephenson F., Jones H. F. E., Townsend M., Hillman J. R., Schwendenmann L., et al. (2020). Blue carbon stocks and cross-habitat subsidies. Front. Mar. Sci. 7. doi: 10.3389/fmars.2020.00380
Carroll M. L., Denisenko S. G., Renaud P. E., Ambrose W. G. (2008). Benthic infauna of the seasonally ice-covered western Barents Sea: Patterns and relationships to environmental forcing. Deep Sea Res. Part II: Topical Stud. Oceanogr. 55 (20-21), 2340–2351. doi: 10.1016/j.dsr2.2008.05.022
Cautain I. J., Last K. S., McKee D., Bluhm B. A., Renaud P. E., Ziegler A. F., et al. (2022). Uptake of sympagic organic carbon by the Barents Sea benthos linked to sea ice seasonality. Front. Mar. Sci. 9. doi: 10.3389/fmars.2022.1009303
Cochrane S. K. J., Pearson T. H., Greenacre M., Costelloe J., Ellingsen I. H., Dahle S., et al. (2012). Benthic fauna and functional traits along a Polar Front transect in the Barents Sea – Advancing tools for ecosystem-scale assessments. J. Mar. Syst. 94, 204–217. doi: 10.1016/j.jmarsys.2011.12.001
Couce E., Engelhard G. H., Schratzberger M., Pinto R. (2020). Capturing threshold responses of marine benthos along gradients of natural and anthropogenic change. J. Appl. Ecol. 57 (6), 1137–1148. doi: 10.1111/1365-2664.13604
Csapo H. K., Grabowski M., Weslawski J. M. (2021). Coming home - Boreal ecosystem claims Atlantic sector of the Arctic. Sci. Total Environ. 771, 144817. doi: 10.1016/j.scitotenv.2020.144817
Denisenko N., Gavrilo M., Denisenko S. G., Mokievsky V. O. (2004). The Barents Sea ecoregion. Biodiversity assessment.
Dybwad C., Assmy P., Olsen L. M., Peeken I., Nikolopoulos A., Krumpen T., et al. (2021). Carbon export in the seasonal sea ice zone North of Svalbard from winter to late summer. Front. Mar. Sci. 7. doi: 10.3389/fmars.2020.525800
Eriksen E., Skjoldal H. R., Gjøsæter H., Primicerio R. (2017). Spatial and temporal changes in the Barents Sea pelagic compartment during the recent warming. Prog. Oceanogr. 151, 206–226. doi: 10.1016/j.pocean.2016.12.009
Fossheim M., Primicerio R., Johannesen E., Ingvaldsen R. B., Aschan M. M., Dolgov A. V. (2015). Recent warming leads to a rapid borealization of fish communities in the Arctic. Nat. Climate Change 5 (7), 673–677. doi: 10.1038/nclimate2647
Grøsvik B. E., Prokhorova T., Eriksen E., Krivosheya P., Horneland P. A., Prozorkevich D. (2018). Assessment of marine litter in the Barents sea, a part of the joint Norwegian–Russian ecosystem survey. Front. Mar. Sci. 5. doi: 10.3389/fmars.2018.00072
Henley S. F., Cavan E. L., Fawcett S. E., Kerr R., Monteiro T., Sherrell R. M., et al. (2020). Changing biogeochemistry of the Southern ocean and its ecosystem implications. Front. Mar. Sci. 7. doi: 10.3389/fmars.2020.00581
Hilmi N., Chami R., Sutherland M. D., Hall-Spencer J. M., Lebleu L., Benitez M. B., et al. (2021). The role of blue carbon in climate change mitigation and carbon stock conservation. Front. Climate 3. doi: 10.3389/fclim.2021.710546
Husson B., Certain G., Filin A., Planque B. (2020). Suitable habitats of fish species in the Barents Sea. Fisheries Oceanogr. 29 (6), 526–540. doi: 10.1111/fog.12493
ICES (2022). Working group on the integrated assessments of the Barents sea (WGIBAR). ICES Scientific Reports.
Jakobsson M., Grantz A., Kristoffesen Y., Mcnab R. (2004). Bathymetry and physiography of the Arctic Ocean and its constituent seas (Berlin, Heidelberg, New York: Springer).
Jayachandran P. R., Bijoy Nandan S., Jima M., Philomina J., Vishnudattan N. K. (2022). Benthic organisms as an ecological tool for monitoring coastal and marine ecosystem health. Ecol. Biodiversity Benthos, 337–362. doi: 10.1016/B978-0-12-821161-8.00004-0
Johannesen E., Jørgensen L. L., Fossheim M., Primicerio R., Greenacre M., Ljubin P. A., et al. (2016). Large-scale patterns in community structure of benthos and fish in the Barents Sea. Polar Biol. 40 (2), 237–246. doi: 10.1007/s00300-016-1946-6
Jørgensen L. L., Bakke G., Hoel A. H. (2020). Responding to global warming: New fisheries management measures in the Arctic. Prog. Oceanogr. 188. doi: 10.1016/j.pocean.2020.102423
Jørgensen L. L., Ljubin P., Skjoldal H. R., Ingvaldsen R. B., Anisimova N., Manushin I. (2015). Distribution of benthic megafauna in the Barents Sea: baseline for an ecosystem approach to management. ICES J. Mar. Sci. 72 (2), 595–613. doi: 10.1093/icesjms/fsu106
Jørgensen L. L., Logerwell E. A., Strelkova N., Zakharov D., Roy V., Nozères C., et al. (2022a). International megabenthic long-term monitoring of a changing arctic ecosystem: Baseline results. Prog. Oceanogr. 200. doi: 10.1016/j.pocean.2021.102712
Jørgensen L. L., Pecuchet L., Ingvaldsen R. B., Primicerio R. (2022b). Benthic transition zones in the Atlantic gateway to a changing Arctic ocean. Prog. Oceanogr. 204. doi: 10.1016/j.pocean.2022.102792
Jorgensen L. L., Planque B., Thangstad T. H., Certain G. (2015). Vulnerability of megabenthic species to trawling in the Barents Sea. J. Mar. Sci. 73 (1), 84–97. doi: 10.1093/icesjms/fsv107
Jørgensen L. L., Planque B., Thangstad T. H., Certain G. (2016). Vulnerability of megabenthic species to trawling in the Barents Sea. ICES J. Mar. Science: J. du Conseil 73 (suppl 1), i84–i97. doi: 10.1093/icesjms/fsv107
Jørgensen L. L., Primicerio R., Ingvaldsen R. B., Fossheim M., Strelkova N., Thangstad T. H., et al. (2019). Impact of multiple stressors on sea bed fauna in a warming Arctic. Mar. Ecol. Prog. Ser. 608, 1–12. doi: 10.3354/meps12803
Kortsch S., Primicerio R., Fossheim M., Dolgov A. V., Aschan M. (2015). Climate change alters the structure of arctic marine food webs due to poleward shifts of boreal generalists. Proc. Biol. Sci. 282 (1814). doi: 10.1098/rspb.2015.1546
Lind S., Ingvaldsen R. B., Furevik T. (2018). Arctic warming hotspot in the northern Barents Sea linked to declining sea-ice import. Nat. Climate Change 8 (7), 634–639. doi: 10.1038/s41558-018-0205-y
Lovelock C. E., Duarte C. M. (2019). Dimensions of Blue Carbon and emerging perspectives. Biol. Lett. 15 (3), 20180781. doi: 10.1098/rsbl.2018.0781
Lundesgaard Ø., Sundfjord A., Lind S., Nilsen F., Renner A. H. H. (2022). Import of Atlantic Water and sea ice controls the ocean environment in the northern Barents Sea. Ocean Sci. 18 (5), 1389–1418. doi: 10.5194/os-18-1389-2022
März C., Freitas F. S., Faust J. C., Godbold J. A., Henley S. F., Tessin A. C., et al. (2021). Biogeochemical consequences of a changing Arctic shelf seafloor ecosystem. Ambio. doi: 10.1007/s13280-021-01638-3
Masson-Delmotte V., Zhai P., Pirani A., Connors S. L., Péan C., Berger S., et al. (2021). Climate change 2021: the physical science basis. Contribution of working group I to the sixth assessment report of the intergovernmental panel on climate change (United Kingdom and New York, NY, USA: Cambridge University Press). doi: 10.1017/9781009157896
Mikkelsen N., Planque B., Arneberg P., Skern-Mauritzen M., Hansen C., Fauchald P., et al. (2023). Multiple stakeholders’ perspectives of marine social ecological systems, a case study on the Barents Sea. Ocean Coast. Manage. 242. doi: 10.1016/j.ocecoaman.2023.106724
Morganti T. M., Slaby B. M., de Kluijver A., Busch K., Hentschel U., Middelburg J. J., et al. (2022). Giant sponge grounds of Central Arctic seamounts are associated with extinct seep life. Nat. Commun. 13 (1), 638. doi: 10.1038/s41467-022-28129-7
Morley S. A., Souster T. A., Vause B. J., Gerrish L., Peck L. S., Barnes D. K. A. (2022). Benthic biodiversity, carbon storage and the potential for increasing negative feedbacks on climate change in shallow waters of the Antarctic Peninsula. Biol. (Basel) 11 (2). doi: 10.3390/biology11020320
Nellemann C., Corcoran E., Duarte C. M., Valdés L., Young C. D., Fonseca L., et al. (2009). Blue carbon. A UNEP rapid response assessment.
Nicastro A., Bishop M. J. (2013). Weak and habitat-dependent effects of nutrient pollution on macrofaunal communities of Southeast Australian estuaries. PloS One 8 (6), e65706. doi: 10.1371/journal.pone.0065706
Norling K., Rosenberg R., Hulth S., Grémare A., Bonsdorff E. (2007). Importance of functional biodiversity and species-specific traits of benthic fauna for ecosystem functions in marine sediment. Mar. Ecol. Prog. Ser. 332, 11–32. doi: 10.3354/meps332011
Onarheim I. H., Eldevik T., Smedsrud L. H., Stroeve J. C. (2018). Seasonal and regional manifestation of Arctic sea ice loss. J. Climate 31 (12), 4917–4932. doi: 10.1175/JCLI-D-17-0427.1
O’Neill F. G., Ivanović A. (2016). The physical impact of towed demersal fishing gears on soft sediments. ICES J. Mar. Sci. 73 (suppl_1), i5–i14. doi: 10.1093/icesjms/fsv125
Peck L. S., Barnes D. K. A., Cook A. J., Fleming A. H., Clarke A. (2010). Negative feedback in the cold: ice retreat produces new carbon sinks in Antarctica. Global Change Biol. 16 (9), 2614–2623. doi: 10.1111/j.1365-2486.2009.02071.x
Pecuchet L., Blanchet M. A., Frainer A., Husson B., Jorgensen L. L., Kortsch S., et al. (2020). Novel feeding interactions amplify the impact of species redistribution on an Arctic food web. Glob Chang Biol. 26 (9), 4894–4906. doi: 10.1111/gcb.15196
Pedersen T., Mikkelsen N., Lindstrøm U., Renaud P. E., Nascimento M. C., Blanchet M.-A., et al. (2021). Overexploitation, recovery, and warming of the Barents sea ecosystem during 1950–2013. Front. Mar. Sci. 8. doi: 10.3389/fmars.2021.732637
Portner B. W., Endres C. H., Bruck T., Garbe D. (2021). Life cycle greenhouse gas emissions of microalgal fuel from thin-layer cascades. Bioprocess Biosyst. Eng. 44 (11), 2399–2406. doi: 10.1007/s00449-021-02612-9
Queiros A. M., Talbot E., Beaumont N. J., Somerfield P. J., Kay S., Pascoe C., et al. (2021). Bright spots as climate-smart marine spatial planning tools for conservation and blue growth. Glob Chang Biol. 27 (21), 5514–5531. doi: 10.1111/gcb.15827
Rantanen M., Karpechko A. Y., Lipponen A., Nordling K., Hyvärinen O., Ruosteenoja K., et al. (2022). The Arctic has warmed nearly four times faster than the globe since 1979. Commun. Earth Environ. 3 (1). doi: 10.1038/s43247-022-00498-3
Reigstad M., Carroll J., Slagstad D., Ellingsen I., Wassmann P. (2011). Intra-regional comparison of productivity, carbon flux and ecosystem composition within the northern Barents Sea. Prog. Oceanogr. 90 (1-4), 33–46. doi: 10.1016/j.pocean.2011.02.005
Renaud P. E., Morata N., Carroll M. L., Denisenko S. G., Reigstad M. (2008). Pelagic–benthic coupling in the western Barents Sea: Processes and time scales. Deep Sea Res. Part II: Topical Stud. Oceanogr. 55 (20-21), 2372–2380. doi: 10.1016/j.dsr2.2008.05.017
Rijnsdorp A. D., Bastardie F., Bolam S. G., Buhl-Mortensen L., Eigaard O. R., Hamon K. G., et al. (2016). Towards a framework for the quantitative assessment of trawling impact on the seabed and benthic ecosystem. ICES J. Mar. Sci. 73 (suppl_1), i127–i138. doi: 10.1093/icesjms/fsv207
Rogers T. L., Munch S. B., Stewart S. D., Palkovacs E. P., Giron-Nava A., Matsuzaki S. S., et al. (2020). Trophic control changes with season and nutrient loading in lakes. Ecol. Lett. 23 (8), 1287–1297. doi: 10.1111/ele.13532
Sala E., Mayorga J., Bradley D., Cabral R. B., Atwood T. B., Auber A., et al. (2021). Protecting the global ocean for biodiversity, food and climate. Nature 592 (7854), 397–402. doi: 10.1038/s41586-021-03371-z
Sands C., Zwerschke N., Bax N., Barnes D., Moreau C., Downey R., et al. (2023). The growing potential of Antarctic blue carbon. Oceanography. doi: 10.5670/oceanog.2023.s1.5
Smale D. A., Barnes D. K. A., Fraser K. P. (2007). The influence of ice scour on benthic communities at three contrasting sites at Adelaide Island, Antarctica. Austral Ecol. 32 (8), 878–888. doi: 10.1111/j.1442-9993.2007.01776.x
Smale D. A., Barnes D. K. A., Fraser K. P. P., Peck L. S. (2008). Benthic community response to iceberg scouring at an intensely disturbed shallow water site at Adelaide Island, Antarctica. Mar. Ecol. Prog. Ser. 355, 85–94. doi: 10.3354/meps07311
Solan M., Wood C., Ward E., Reed A., Grange L. J., Godbold J. (2020). Climate driven benthic invertebrate activity and biogeochemical functioning across the Barents Sea Polar Front. Philos. Trans. A. doi: 10.1098/rsta.2019.0365
Søreide J. E., Carroll M. L., Hop H., Ambrose W. G., Hegseth E. N., Falk-Petersen S. (2013). Sympagic-pelagic-benthic coupling in Arctic and Atlantic waters around Svalbard revealed by stable isotopic and fatty acid tracers. Mar. Biol. Res. 9 (9), 831–850. doi: 10.1080/17451000.2013.775457
Souster T. A., Barnes D. K. A., Hopkins J. (2020). Variation in zoobenthic blue carbon in the Arctic’s Barents Sea shelf sediments. Philos. Trans. A Math Phys. Eng. Sci. 378 (2181), 20190362. doi: 10.1098/rsta.2019.0362
Souster T. A., Morley S. A., Peck L. S. (2018). Seasonality of oxygen consumption in five common Antarctic benthic marine invertebrates. Polar Biol. 41 (5), 897–908. doi: 10.1007/s00300-018-2251-3
Stroeve J. C., Serreze M. C., Holland M. M., Kay J. E., Malanik J., Barrett A. P. (2011). The Arctic’s rapidly shrinking sea ice cover: a research synthesis. Climatic Change 110 (3-4), 1005–1027. doi: 10.1007/s10584-011-0101-1
Węsławski J. M., Kędra M., Przytarska J., Kotwicki L., Ellingsen I., Skardhamar J., et al. (2012). A huge biocatalytic filter in the centre of Barents Sea shelf?**The present paper was based on the BANKMOD bilateral Norwegian – Polish projects (NFR 184719) and was completed thanks to additional financial support from the Polish Ministry of Science and Higher Education (384/W-Bankmod/2009/0 and 382/W-Akvaplan-niva/2009/0). Oceanologia 54 (2), 325–335. doi: 10.5697/oc.54-2.325
Zakharov D. V., Jørgensen L. L., Manushin I. E., Strelkova N. A. (2020). Barents Sea megabenthos: Spatial and temporal distribution and production. Mar. Biol. J. 5 (2), 19–37. doi: 10.21072/mbj.2020.05.2.03
Zwerschke N., Morley S. A., Peck L. S., Barnes D. K. A. (2021a). Can Antarctica’s shallow zoobenthos ‘bounce back’ from iceberg scouring impacts driven by climate change? Glob Chang Biol. doi: 10.1111/gcb.15617
Keywords: blue carbon, nature-based solutions, polar, Arctic, benthic, climate change
Citation: Souster TA, Barnes DKA, Primicerio R and Jørgensen LL (2024) Quantifying zoobenthic blue carbon storage across habitats within the Arctic’s Barents Sea. Front. Mar. Sci. 10:1260884. doi: 10.3389/fmars.2023.1260884
Received: 18 July 2023; Accepted: 26 December 2023;
Published: 26 January 2024.
Edited by:
Shin-ichi Ito, The University of Tokyo, JapanReviewed by:
Jan Marcin Weslawski, Polish Academy of Sciences, PolandThomas G Dahlgren, Norwegian Research Institute (NORCE), Norway
Copyright © 2024 Souster, Barnes, Primicerio and Jørgensen. This is an open-access article distributed under the terms of the Creative Commons Attribution License (CC BY). The use, distribution or reproduction in other forums is permitted, provided the original author(s) and the copyright owner(s) are credited and that the original publication in this journal is cited, in accordance with accepted academic practice. No use, distribution or reproduction is permitted which does not comply with these terms.
*Correspondence: Terri A. Souster, dGVycmkuc291c3RlckB1aXQubm8=