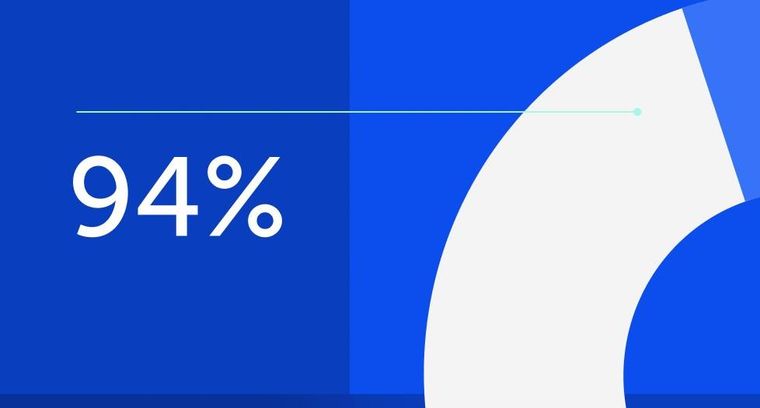
94% of researchers rate our articles as excellent or good
Learn more about the work of our research integrity team to safeguard the quality of each article we publish.
Find out more
ORIGINAL RESEARCH article
Front. Mar. Sci., 11 September 2023
Sec. Marine Conservation and Sustainability
Volume 10 - 2023 | https://doi.org/10.3389/fmars.2023.1260205
Follicle-stimulating hormone (FSH) is involved in the regulation of essential reproductive processes such as gametogenesis and follicular growth. There are presently no immunoassays for measuring FSH in turtles. Recently we produced green sea turtle (Chelonia mydas) recombinant (r) FSH as a single-chain polypeptide using the methylotrophic yeast Pichia pastoris expression system, and polyclonal antibodies for the recombinant FSH. In this work we developed a competitive enzyme-linked immunosorbent assay (ELISA) for the measurement of FSH concentrations in plasma samples from green sea turtles. We used the rFSHβα for standard, rFSHβ for coating and a cmFSHβ antibody. The sensitivity of the assay was 0.13 ng/ml and the intra-assay and inter-assay coefficients of variation were 5.54% and 13.52% respectively. Parallelism was observed between the linearized FSH standard curves and the corresponding serial dilutions of green sea turtle plasma samples. We also observed parallelism between the linearized standard and serial dilutions of plasma samples from the loggerhead sea turtle (Caretta caretta), hawksbill sea turtle (Eretmochelys imbricate), and African softshell turtle (Trionyx triunguis). The ELISA was used to study the FSH plasma concentrations during the reproductive cycles and was compared to hormonal steroid concentrations (Testosterone, Estradiol and Progesterone). This revealed a positive correlation between FSH and estradiol concentrations in females; estradiol concentrations were increased immediately after FSH elevation. In addition, nested females presented an increase in FSH concentrations prior to progesterone elevation in January to April, slightly before egg laying. This ELISA will increase our understanding of gonadotropin functions, and their effects on reproduction in the green sea turtle.
Reproductive cycles in vertebrates are regulated by the hypothalamus. The secretion of gonadotropin releasing hormone (GnRH) stimulates the pituitary gland to release the gonadotropins luteinizing hormone (LH) and follicle stimulating hormone (FSH) (Licht, 1980; Kang et al., 2020). Gonadotropins (GTH) are composed of a common α-subunit, and a unique β-subunit with a biological role specific to each hormone (Aizen et al., 2007a; Aizen et al., 2007b). Both gonadotropins regulate gonadal functions and thus are essential for reproduction. In mammals, FSH and LH have a distinct effect on endocrine regulation traits. In females, LH stimulates estrogen and progesterone production in the ovary. FSH is responsible for the development of the ovarian follicle, and stimulates secretion of estrogen from the follicle (Kluge et al., 2012).
Green turtles (Chelonia Mydas) have been subject to a long, and worldwide, history of human exploitation, resulting in endangerment of the global green turtle`s population (Seminoff et al., 2015). Since the green turtles exhibit high natal homing behavior and due to their long and complex life cycle, human intervention is needed for to increase the green turtle population of nesting females. The Israel National Sea Turtle Rescue Center established a captive breeding stock in 2002 to provide this needed help. To rehabilitate the Mediterranean green turtle population, a deeper understanding and prediction of reproductive stages are essential. Although the reproductive ecology and endocrinology of green turtles have been the subject of numerous studies, little is known regarding their reproductive biology.
There is little information on the cycling and function of FSH in green sea turtles, and the physiological significance in this species is not yet fully understood. Several studies in female reptiles have shown a stimulation of follicular development following injection of mammalian FSH (Kawazu et al., 2014; Tripathy and Rai, 2017; Kang et al., 2020). Previous studies by Licht, 1980 and Wibbels et al., 1992 regarding FSH function in green sea turtle supported these results. An increase in FSH concertation at the time of ovulation in green sea turtles from April to August has also been observed (Licht et al., 1979; Wibbels et al., 1992).
In males, FSH was shown to stimulate testicular growth, and increased production of an androgen-binding protein by Sertoli cells that are necessary for sustaining the maturing sperm cell. Furthermore, FSH also stimulates spermatogonial mitosis (Licht et al., 1979; Ng et al., 1986; Bousfield et al., 2007). In males, an increase in FSH concertation was concurrent with an increase in testosterone, reaching maximal concentration after summer testicular recrudescence (Licht et al., 1985; Mendonca and Licht, 1986).
Gonadotropins regulate second messenger pathways leading to the production and secretion of sex steroids (Aizen et al., 2007a; Aizen et al., 2007b). There are three main sex hormones that affect behavior and reproduction in vertebrates: progesterone, estradiol, and testosterone. In females, progesterone is a potential ovulation inhibitor; testosterone affects mating receptivity towards males, and estradiol, is the primary stimulus for vitellogenin (Licht et al., 1979). In males, testosterone is significantly correlated with testicular growth and spermiation, and estradiol is essential for spermatogenesis (Mahmoud and Licht, 1997).
The development of tools such ELISA or Radioimmunoassay (RIA) for the measurement of gonadotropins until now has required purified protein in large amounts and specific antibodies (Aizen et al., 2007b). Obtaining high quality and quantity of native gonadotropins requires purifying GTHs from pituitary glands, which would involve the killing of a significant number of animals. This is very problematic, especially for the green sea turtle that is an endangered species (Seminoff et al., 2015).
Recombinant FSH production has a distinct advantage since production is not dependent on pituitary availability (Kasuto and Levavi-Sivan, 2005). The yeast host system is one of the most powerful tools for expressing recombinant proteins. Methylotrophic yeast species such as Pichia pastoris have been developed for this purpose and are very popular due to their low cost (Karbalaei et al., 2020). This yeast line can produce high yields of recombinant proteins with glycosylation (Balamurugan et al., 2007). The advantages of this expression system include appropriate folding in the endoplasmic reticulum, and secretion of recombinant proteins to the medium, making the harvesting easier, and avoiding yeast cell protein contamination in the purification process (Li et al., 2007; Yang et al., 2013). Furthermore, they have high growth rate, coupled with ease of high cell-density fermentation, high levels of productivity in an almost protein-free medium, ease of genetic manipulation of well characterized yeast expression vectors as in Escherichia coli. (Li et al., 2007). The time needed to assess recombinant gene expression with the P. pastoris is approximately 3-4 weeks. This time frame includes transformation of the yeast, screening the transformants for integration, and an expression time-course analysis (Aizen et al., 2007a; Aizen, 2011). P. pastoris culture can reach 120 g/L of dry cell weight density (Aizen, 2011).
There are many immunoassay platforms available to quantify protein concentrations in biological fluids. ELISAs are preferred in many cases due to their sensitivity, specificity, accuracy, and ability to tolerate harsh buffers or pre-treatments (Molés et al., 2012). Both indirect and sandwich ELISAs allow detection of antigen or antibody at very low concentrations hence their specificity. The main advantage of competitive ELISA is that the method detects differences in antigen-antibody complex with high sensitivity, even when the specific detecting antibody is present in relatively small amounts. Competitive ELISA is less sensitive to sample dilution and sample matrix effects than the sandwich ELISA, and there is less variability between duplicate samples and between assays (Barlow et al., 1986). Taken together one should consider using a sandwich ELISA. The use of two specific antibodies, one against the beta subunit and the other against the alpha subunit allows us to specifically detect each glycoprotein hormone, depending on the anti-beta used, in its natural quaternary structure. (i.e. associated with alpha and excluding free beta detection). With regards to the alpha subunit in glycoproteins, it holds some challenges, as it is not very immunogenic and highly conserved among glycoproteins among different species and the same species (identical alpha for FSH, LH and TSH).
RIA methods were widely used for the measurement of gonadotrophin concentrations based on the principle of competitive binding. In this method, an unlabeled antigen competes with a radiolabeled antigen for binding to an antibody with the appropriate specificity (Webster et al., 1990). RIA is often based on a polyclonal antibody and may show cross-reactivity to substances other than the test analyte. ELISA, on the other hand, is based on two antibodies, which ensures high specificity for the analyte. In addition, the ELISA method can utilize antibody quantities that are higher than those allowed by RIA’s radioactive tracer (Wewer Albrechtsen et al., 2014), and ELISA exhibits lower coefficient of variation (CV) than RIA (Warnken et al., 2016).
ELISA also offers excellent performance with small sample volumes, and the procedure takes about half the amount of time as the RIA assay. In addition, RIA uses radiolabeled reagents that require a license for storage and special waste disposal. The ELISA method avoids these constraints of using radioactive chemicals (Webster et al., 1990; Wewer Albrechtsen et al., 2014).
In recent years the use of analytical methods to determine concentrations of gonadotropins such as LC-MS/MS and LC-MRM/MS were implemented due to the limited availability of high-quality antibodies, and ability to distinguish between different FSH glycovariants and showed comparable sensitivity to ELISA assays, with advantage of being faster and more selective (Melchiorre et al., 2021). The majority of assays LC-MS is currently at least complementary if not superior to immunoassay and that the benefits LC-MS offers will see its increased use over immunoassay, especially as the technology advances but we are not there yet (Cross and Hornshaw, 2016).
The reproductive biology of sea turtles has been studied both in the wild and in captivity; most of the research is based on nesting females, due to the ease of sampling during the nesting season. Only a few studies on hormonal cycles of adult males have been investigated in the wild (Wibbels et al., 1990; Tim et al., 2004; Blanvillain et al., 2008) and others have been studied in captivity (Licht et al., 1979; Licht et al., 1985; Rostal et al., 1998). To study GTH biological function we need assays for measuring GTH concentrations, and in order to achieve that we need to produce properly folded and biologically active recombinant GTHs.
In this study, we used the yeast Pichia pastoris expression system, since this system provided sufficient quantities of recombinant protein (Daly and Hearn, 2005) for the development and validation of an ELISA. We report on the production of a recombinant green sea turtle FSH (rcmFSH) and the development of a competitive ELISA for measuring green sea turtle FSH using the recombinant protein. For the first time, we were able to directly measure FSH concentrations in plasma samples, by comparing plasma FSH concentrations with steroids concentrations. We observed that FSH concentrations increased during the prior and during the nesting season from January to June (nesting season is April to August) along with progesterone concentrations. We were able to identify a pattern whereby FSH concentrations rise followed by rising estradiol concentrations. This helps us predict the reproductive stages of the turtles in the breeding facility, which can enhance successful breeding in captivity. Our main goal is to monitor annual concentrations of sex steroids and gonadotropins to characterize green turtle hormonal profiles. A better understanding of the green sea turtle reproductive biology may boost future efforts on population rehabilitation and conservation.
The Sea Turtle Rescue Center under was established by the Nature & Parks Authority of Israel is located in Alexander River National Park (Coordinates: 32.4016667, 34.8666667)
The reproduction facility features two 450 cubic meters tanks and an artificial nesting beach. Water temperature is kept at 20-22°C, and periodically altered. The turtle’s diet is composed of romaine lettuce (2% BW) and frozen fish (Herring, Flounder and Salmon, 0.2% BW). All turtles are tagged with metal plates on either their front or back limb. The research population consists of 12 females (weight: 83.58 ± 22.74 Kg; CCL: 82.81 ± 7.52 cm; CCW: 75.31 ± 7.44 cm) and 11 males (weight: 66.16 ± 17.89 Kg; CCL: 76.09 ± 8.16 cm; CCW: 69.5 ± 7.2 cm), from the captive breeding stock in Mikhmoret (Table S1). 6 of the 12 females nested in 2 nesting seasons: 474, 495, 729 and 760 in 2019; 173 and 1055 in 2020.
Blood sampling was performed approximately every two months in the Israel National Sea Turtle Rescue Center. Five ml of blood were taken from the dorsal vein of the turtle's neck using a sterile 21-gauge 8.9 cm needle. Blood was moved into a biobased blood collection tube that contains an anticoagulant agent heparin sodium. The blood samples were stored at 4°C during the collection (no more than one hour). Once collection ended, hematocrit count of each turtle was first examined to obtain information on their health. We centrifuged two capillaries for each sample at 17800 RCF for 12 min and calculated the average of the two capillaries. For blood fractionation, we centrifuged the 5 ml tubes at 3200 RCF for 15 min. Each tube contained approximately 3 ml of plasma. We divided each individual plasma sample into three different 1.5 ml Eppendorf tubes for hormone measurement, blood biochemistry and backup. The plasma was then stored at -80°C.
For steroid extraction we used a 500 µl plasma sample and added 5 ml diethyl ether to glass tubes and mixed 3 times for 15 seconds with vortex. We transferred the glass tubes to -80°C for 45 minutes, the upper phase in the glass tube was transferred to a new glass tube and the diethyl ether was evaporated by heating the tubes in a 55°C water bath. Extraction products were dissolved in 500 µl Steroid Assay Buffer (SAB). The concentrations of sex steroids (estradiol, progesterone and testosterone) were measured by commercial Cayman ELISA kits ®. (501890, 582701 and 582601 respectively). Concentration detection levels were 6.6-4,000, 7.8-1,000 and 3.9-500 pg/ml for estradiol, progesterone and testosterone respectively, with sensitivity range of 6-15 pg/ml. In order to assess the concentration range of each steroid in the research population, preliminary tests were conducted. For each steroid assay at least three dilutions were tested, with samples from both sexes and different sampling dates to assess the annual concentration variation (data not shown).
Recombinant FSH was produced using the methylotrophic yeast P. pastoris, as a biologically active, single-chain polypeptide according to Aizen et al. (2007a) protocol, green sea turtle (Chelonia mydas) FSHB (GenBank Accession No. XM_007067910.1) and green sea turtle (Chelonia mydas) CGA (GenBank Accession No. XM_007052575.1) subunits coding gene sequences were translated to amino acid sequences using the ExPASy Proteomics Server (http://ca.expasy.org/tools/dna.html). The presence of signal peptide cleavage site was predicted by SignalP 4.1 Server (Nielsen, 2017). The protein coding gene sequences were used to design two expression vectors: FSH β-subunit peptide chain for future antibody production, and FSHβα peptide chain for standard curve. FSHβα vector was constructed with a flexible linker allowing a smooth rotation of the two subunits to reach the natural structure of the entire gonadotropin. To allow flexibility to the construct tethered polypeptide, we used 2xGS-6His-2xGS between the β-subunits and α-subunits. Both vectors were transfected into chemically competent E. coli, JM109 strain via heat shock based on manufacturer’s protocol (Promega). Selection and expression were generally performed according to (Aizen et al., 2007a). Recombinant proteins were purified using nickel–nitrilotriacetic acid–agarose (Ni-NTA; Qiagen). As a negative control, P. pastoris was transformed with an expression vector that did not contain the cmFSH cDNA, and fractions were prepared in the same manner. The eluted proteins were concentrated by lyophilization, and protein concentration was measured using Bradford reagent. The eluted proteins were stored at -80°C until use. Recombinant proteins were deglycosylated using PNGaseF (Peptide N-glycosidase F) and analyzed by Western blot with anti-HIS staining.
The antiserum against green sea turtle FSH β-subunit was produced by a commercial company ADAR Biotech Ltd. (Rehovot, Israel). The antiserum was raised in two rabbits with approximately 40 ml of sera harvested from each rabbit. Sera antibodies titers against the antigen were determined by direct ELISA by ADAR Biotech Ltd. resulting in high titer of antibodies against the FSH β-subunit. Final bleeding was performed, and the antiserum was then aliquoted, and stored at -80°C.
FSH protein samples were electrophoresed on 16% SDS-polyacrylamide unstained gels with 6-8% stacking gel. We added Laemmli SDS reducing buffer (Bio-Rad, Hercules, CA) with 2-mercaptoethanol concentrated at 4X to the protein samples for SDS-PAGE under reducing conditions. Samples where heated in a Thermomixer comfort (Eppendorf AG, Hamburg, Germany) for 10 min at 100°C. The electrophoresis was carried in TGS (Tris-Glycine-SDS) running buffer (Bio-Rad, Hercules, CA) at 110V for one hour. The SDS PAGE gels were then washed once with DD H2O. Gels were blotted to nitrocellulose membranes (BioRad) using Bio-Rad Trans-Blot® Turbo TM Blotting System. Membranes were blocked with Anti-His HRP blocking (Qiagen, Limburg, Netherlands) or 5% low-fat milk powder in PBS. Bound antibodies were detected with either penta-His antibody (Qiagen, Limburg, Netherlands) or with turtle FSHβ antibody. When using anti-His antibody, the membranes were treated with penta-His-HRP antibody (1:2000 dilution) overnight at 4°C. When using anti-FSHβ, the membranes were incubated in PBS plus 1% low-fat milk powder with the antibodies (diluted 1:5000) overnight at 4°C and then with goat-antirabbit horseradish peroxidase conjugate (GAR-HRP) (diluted 1:5000) for 1h at room temperature (RT). After washing, all membranes were treated with enhanced chemiluminescence reagent, EZ-ECL kit (Biological Industries, Beit Haemek, Israel) to reveal immunoreactive bands.
Competitive ELISA for green sea turtle FSH was developed using FSHβ-subunit primary antibody, recombinant FSHβ-subunit for coating ELISA microplates, and recombinant single chain βαFSH for the standard curve. The protocol was based on (Aizen et al., 2007b).
We coated ELISA 96-well microplates (MaxiSorp™ Nunc-Immuno, Thermo Scientific™, Denmark) with 100 µl/well of recombinant green sea turtle FSHβ diluted 1.5 ng/10ml (0.015 ng/well) in a solution of carbonate buffer (50 mM sodium carbonate (NaHCO3), pH 9.6). The plates were incubated in complete darkness overnight at RT without shaking. For non-specific binding, two wells (A12 and B12) were not coated with the FSHβ solution.
The next day, coated microplates were washed (3 times X 5 min) with 200 µl/well of PBST buffer (10 mM Na2PO4, 2 mM KH2PO4 (pH 7.4), 140 mM NaCl, 3 mM KCl, and 0.05% Tween 20). The microplates were blocked with 200 µl/well of 1% bovine serum albumin (Sigma, Rehovot, Israel) in PBST buffer for 1 h in complete darkness at RT without shaking to reduce background.
Standard was diluted in 0.1% BSA in PBST with 10% of normal goat serum (NGS, Biological Industries). Unknown plasma samples were diluted (1:15 for males, 1:15 for females and 1:30 for nested females) with 0.1% BSA in PBST, each sample was diluted in a volume of 300 µl allowing duplicate analysis. The unknown samples and FSHβα standards were pre-incubated in 96-well microplates (Sarstedt AG & Co., Nümbrecht, Germany) with the primary antibody against FSHβ in final dilution of 1:5000 dissolved in 0.1% BSA in PBST in complete darkness overnight at RT without shaking.
After the pre-incubation of standard and unknown samples with the primary antibody, each sample was dispensed into the coated and blocked microplates (100 µl/well) and incubated in complete darkness overnight at RT without shaking.
The next day the plates were washed (3 X 5 min) with PBST. 100 µl/well of GAR-HRP diluted 1:5000 in PBST 0.1% BSA buffer were added and incubated for 2 h in complete darkness at RT without shaking to detect the formed antigen–antibody complexes.
Before color development the plates were washed again (3 times X 5 min) with PBST. The visualization of enzyme complexes was executed by addition of 100 ml/well of ABTS® 2-Component Microwell Peroxidase Substrate (KPL, Zotal, Israel). The reaction was carried out in complete darkness at RT for 20 min. Absorbance was read at 405 nm, using a Synergy HTX Multi-Mode Reader (BioTek, USA).
The ELISA was validated for cmFSH determinations in plasma samples of Chelonia mydas and was tested for cross-reactivity with additional turtle species (loggerhead, hawksbill and african softshell turtle). To conduct the parallelism analysis, whole blood samples were collected from sexually mature turtles held in captivity at the NPA Center. Displacement curves for plasma samples were obtained by serial dilutions of the plasma in the ELISA buffer (PBST buffer containing 10% NGS) and compared with the ELISA standard curve. The ELISA was also validated for FSH in other turtle species. Plasma samples were diluted 1:2. All samples were used in the ELISA at a total volume of 100μl solution per well. Intra assay coefficient of variation (CV) was determined by assaying 24 replicates of green sea turtle samples on the same assay plate. Inter assay variation was determined by assaying the same sample 9 times on different assay plates.
All data are presented as means ± SEM (Standard Error of the Mean). To test parallelism between displacement curves, we used linear regression in Graph-Pad Prism 9.0 software (GraphPad, San Diego, CA) and compared slopes. The significance in differences of hormone level means between groups was determined by one-way analysis of variance (ANOVA) followed by Newman–Keuls test. To test for parallelism between various regressions lines, we performed logit transformation, where logit (B/Bo) = log [r/(1-r)], and r = B/B0, B represents the binding at each point, and B0 represents the maximum binding. We used the linear regression parameter in Graph-Pad Prism 9.0 software to compare the slopes.
Western-blot analysis was performed on green sea turtle pituitary extract and the supernatant derived from yeast transformed with an expression vector containing cmFSHβα, cmFSHβ.
These proteins were immunoreacted with antibodies against Penta-His antibody (QIAGEN) (Figure 1A) or against cmFSHβ antibody (Figure 1B) for antigen specificity conformation. The anti-HIS staining FSHβ yielded bands of ~25kDa, and after deglycosylation FSHβ yielded a band of 15 kDa (Figure 1A, lane 2). FSHβα protein analysis yielded bands of ~60- 75 kDa (Figure 1A, lane 3) and a band of 27-30 kDa after deglycosylation Figure 1A, lane 4). The anti-cmFSHβ antibody staining yielded bands for cmFSHβ of ~60-120 kDa, and after deglycosylation a band of 15-25 kDa (Figure 1B, lane 2). The cmFSHβα protein analysis yielded bands of ~60-80 kDa (Figure 2B, lane 3) and a band of 25-30 kDa after deglycosylation (Figure 1B, lane 4). Green sea turtle pituitary yielded bands around 30-35 kDa (Figure 1B, lane 5-6) validating the expected size of the dimer protein in the pituitary. Transformation with vector alone served as a negative control and yielded no bands (data not shown).
Figure 1 Western blot stained with anti-His antibody (A) or anti-FSHβ antibody (B) of Ni-NTA nickel agarose beads purified FSHβ and FSHβα protein expressed by P. pastoris (GS115) and green sea turtle pituitary gland extracts, western blots were carried out under reducing conditions. M: Protein size marker, 1: FSHβ protein, 2: deglycosylated FSHβ protein, 3: FSHβα protein, 4: deglycosylated FSHβα protein, (5-6): pituitary gland protein extractions from green sea turtle (Chelonia mydas). (B) Marker (M) and lane 5,6 were spliced and grouped from Supplementary Image S3, all original westerns are in the Supplementary Images S1–S3.
Figure 2 Competitive binding curve for green sea turtle FSH standard. Each point represents the mean value of a duplicate (A). Parallelism between the standard curve of ELISA for green sea turtle FSH measurements and displacement curves obtained with serial dilutions of plasma samples from three of green sea turtle females (CM: Chelonia mydas) (B).
A competitive ELISA was developed for FSH determination in plasma samples of green sea turtle, using rcmFSHβα as standard, rcmFSHβ for coating and primary antisera against rcmFSHβ for detection. Dilution tests were carried out to determine appropriate dilutions for antigen (coating step) and antibody (competition step) to achieve optimal results. Antibody dilutions (1:5000, 1:10000, 1:20000) and four FSHβ coating concentrations (0.5 ng/ml, 1 ng/ml, 1.5 ng/ml and 5 ng/ml) were first tested to determine the combination that would result in optical density closest to 1 OD. The optimal dilution was 1:5000 for first antibody, 1.5 ng/ml for coating (rcmFSHβ), standard curve was generated using rcmFSHβα at starting concentrations of 50 ng/ml that were serially diluted (x2) down to 0.0488 ng/ml. Percentage binding showed the sigmoidal curve typical in competitive ELISAs (Figure 2A). After logit-transformation, the percentage binding data showed a linear relationship with the logarithm of the standard concentration (r2 = 0.97298, Figure 2B). Sensitivity of the assay was defined as the amount of FSH sufficient to reduce the optical density determined in the absence of FSH by two standard deviations. Sensitivity for FSH measuring was 0.13 ng/ml, with the optical density decreasing as a linear function of FSH concentration (r2 = 0.97298, Figure 2B). with percent binding B/Bo > 96%. The intra-assay CV was calculated by measuring replicates of the same sample within the assay. The intra-assay CV was estimated at 5.54% for FSH. The inter-assay CV was calculated by measuring replicates of the same sample in different assays. The inter-assay CV was estimated at 13.52%. The sensitivity of the ELISA we developed was 0.13 ng/ml for measuring FSH concentrations in plasma samples.
Assay validation was confirmed by parallelism of the standard curve and the curves of serial dilutions of green sea turtle plasma (Figure 3A). No significant difference was found between the slopes of the standard curve (-0.8168 ± 0.03318) and the curves of CM plasma serial dilutions (-0.7787 ± 0.03997). These data indicate that the recombinant FSH is immunologically like the endogenous gonadotropin found in the turtle plasma. To determine ELISA applicability in other species we diluted plasma from loggerhead sea turtle (Carettacaretta - CC, Figure 3A), hawksbill sea turtle (Eretmochelys imbricate - EI, Figure 3B) and African softshell turtle (Trionyx triunguis – TT, Figure 3B). Results showed no significant difference between the slopes of the standard curve and the slope of CC plasma samples (-0.7847 ± 0.09212), EI plasma concentrations (-0.9605 ± 0.08680) and TT plasma concentrations (-0.8301 ± 0.1789).
Figure 3 Parallelism between the standard curve of ELISA for green turtle FSH and displacement curves obtained with serial dilutions of plasma samples from three individuals loggerhead sea turtles (CC: Carreta carreta) and green sea turtle (CM: Chelonia mydas) (A) three individuals hawksbill sea turtle (EI: Eretmochelys imbricate), two African softshell turtle (TT: Trionyx triunguis) and green sea turtle (CM: Chelonia mydas) (B).
The analysis presented in this section focuses on three categories: nested females, non-nested females, and males. The data represents the seasonal variations of three sex steroids and FSH. The objective is to explore the relationship between the concentrations of the variables under examination and the reproductive activity of female green turtles. To achieve this, the turtles were classified based on their nesting behavior. In the Mediterranean region, the nesting season typically spans from May to September (Mazor et al., 2013). Considering that vitellogenesis, the process of yolk formation, typically initiates 10-12 months before nesting, the nesting females in our study were defined as those that laid eggs between May and September in our reproduction facility. Females that did not engage in nesting activities during the analyzed year were categorized as non-nesting. In terms of the nested females, the graphs display data from both a year prior to and a year following the nesting season, highlighting the shifts in the analyzed parameters.
The four females that have nested in 2019 season presented a rise in FSH concentrations from November 2018 towards June 2019 (60-237 ng/ml), then a decrease towards November 2019 (86 ng/ml) and a rise towards April 2020 that maintained same concentrations until the end of 2020 (150-204 ng/ml) (Figure 4A). The testosterone concentrations in 2019 season peaked before the nesting season (April to August) from June 2018 to April 2019 (147-1856 pg/ml) and then decreasing to 40 pg/ml in July 2019 maintaining low concentrations until September 2020 (Figure 4A). The progesterone concentrations presented a rise from November 2018 peaking in June 2019 (27-4513 pg/ml) and then decreasing to 30pg/ml maintaining low concentrations below 100pg/ml until the end of 2020. The concentrations of Estradiol remained low from January 2018 to January 2020 (6-56 pg/ml) and started to rise from April 2020 to June 2020 (50- 143 ng/ml) (Figure 4A).
Figure 4 Mean variations of FSH, progesterone, estradiol, and testosterone plasma concentration from 4 females that nested in 2019 (A) and 2 females that nested in 2020 (B). X axis represent each sampling date in the period. Right Y axis represents FSH (ng/ml) in females, Left Y axis represents progesterone (P4) estradiol (E2) and testosterone (pg/ml) concentrations.
The two females that nested in the following 2020 season also presented a rise in FSH concentrations from January 2020 to April 2020 (156-293 ng/ml) following a decrease until August 2020 and a rise in FSH concentrations until September 2021 reaching 337ng/ml (Figure 4B). The testosterone concentrations in 2020 season peaked before the nesting season (April to August) from June 2019 to September 2019 (42-948 pg/ml) and then decreasing to 273 pg/ml in April 2020 maintaining low concentrations until August 2021(30-185 pg/ml) (Figure 4B). The progesterone concentrations presented a rise from January 2020 peaking in April 2020 (46-2930 pg/ml) and then decreasing to 65pg/ml maintaining low concentrations below 111pg/ml until the end of 2021. The concentrations of Estradiol peaked prior to the nesting season in September 2019 reaching 143pg/ml and from January 2020 to November 2021 ranged from at low concentrations of 73 to 4pg/ml (Figure 4B).
The non-nesting females from January 2018 to November 2021 presented FSH concentrations ranging from 50-383ng/ml. a pattern of rise in FSH concentrations was shown every year prior to the nesting season (April to August) from September or November reaching a peak in June, different from 2018 and 2019, 2020 season FSH concentrations were higher reaching 383ng/ml compered to 135ng/ml in prior seasons (Figure 5A). The testosterone concentrations were below between 24-156pg/ml and peaked every year excluding 2018 in September reaching the highest in September 2021 159pg/ml (Figure 5A). Progesterone presented no significant pattern over 2018 to 2021and concentrations of 40-128pg/ml. The concentrations of Estradiol remained very low during 2018-2019 (8-15 pg/ml). In 2020 estradiol peaked during the nesting season reaching 74pg/ml and from November 2020 decreased to17pg/ml in November 2021 (Figure 5A).
Figure 5 Mean variations of FSH, progesterone, estradiol, and testosterone plasma concentration from 6 females that have not nested from 2018 to 2021 (A) and 12 males (B). X axis represent each sampling date in the period. Right Y axis represents FSH (ng/ml) in females (A). Left Y axis represents progesterone (P4) estradiol (E2) and testosterone (pg/ml) concentration in females (A). In males Right Y axis represents FSH and testosterone (ng/ml) concentration and Left Y axis represents progesterone (P4) and estradiol (E2) (pg/ml) concentration (B).
Throughout 2018 to 2021 males showed high concentrations of FSH in January (122-260 ng/ml), September (100-374 ng/ml), and November (150-200 ng/ml) compared to the rest of the year (50-150 ng/ml), and their estradiol concentrations corresponded to the rises in FSH concentrations. Regarding testosterone the concentrations were between 3-45 ng/ml. the progesterone concentrations were higher during the nesting season (April to August) (Figure 5B).
Herein we describe for the first time the development of an ELISA for FSH in green sea turtles, with recombinant gonadotropin we produced using the yeast P. pastoris expression system. The ELISA was also found to be applicable for other sea turtle species. We also observed plasma steroid concentration during the reproductive cycles that revealed a positive correlation between FSH and estradiol concentrations in females; estradiol concentrations were increased immediately after FSH elevation. In addition, nested females presented an increase in FSH concentrations prior to progesterone elevation in January to April, slightly before egg laying.
We must emphasize that the turtles in this research have been in the breeding facility since hatching. Our results describe patterns of FSH and steroids concentrations for turtles that were kept in captivity their entire life. With that we believe that our findings can help to better understand what is occurring during the time periods before nesting and after nesting especially for turtles that will not nest every year.
Measuring hormone concentrations in turtles is challenging on many levels. Taking blood from females arriving on the beaches to lay eggs may interfere with their normal behavior during this critical period. Finding males on the beach is rare, and so measuring their hormone concentrations may not be feasible (Goldberg et al., 2013). When injured turtles arrive at the Sea Turtle Rescue Center, they are usually young, and distinguishing between males and females is not possible, Owens et al. (1985) showed that injecting mammalian FSH in immature turtles increased the testosterone concentration in the blood and was helpful to determine if the turtles are truly males, but this method has not been tested since then (Owens and Morris, 1985). Measuring testosterone concentrations in young turtles does not correlates with their gender, especially in young age.
Therefore, studying the breeding stock at Mikhmoret was important for achieving a hormonal profile of the green sea turtles. In this research we developed and validated a competitive ELISA for measuring FSH concentrations in plasma samples from green sea turtle based on the approach of production recombinant FSH as a single chain molecule using the P. pastoris expression system. Earlier research using the P. pastoris expression system has shown that the main advantage of this system is the ability to produce a recombinant protein that is similar to the natural hormone and can elicit an In-vitro and In-vivo biological response (Kasuto and Levavi-Sivan, 2005). In addition, the protein can be efficiently purified from the culture medium (Aizen et al., 2007a; Aizen et al., 2007b). The use of a recombinant gonadotropin can increase its immunogenicity, as well as the specificity of the generated antibodies (Daly and Hearn, 2005).
Generally, immunoassays that are developed to determine GTHs concentrations have been based on native GTHs that were purified from pituitary glands (Molés et al., 2012). Purification of native FSH is a very demanding process, and to purify a sufficient amount of GTHs we would need a large number of pituitary glands, which would require killing a large number of turtles. Recombinant proteins can be produced continuously, independent of turtle pituitary gland availability. Additionally, recombinant FSH subunits can ensure there is no cross-reactivity with similar GTHs that share the same α-subunit (Aizen, 2011). The open reading frame for FSH α-subunit is 120 amino acids, and for FSH β-subunit is 138 amino acids. The subunits were cloned and validated for their predicted protein sequences from NCBI. The sequences were compared to different turtle species (The common box turtle ; T. carolina, Painted turtle; C. picta, Chinese pond turtle; C. reevesii, and Chinese softshell turtle ; P. sinensis) and were found to be practically identical. In different studies on the FSH molecular structure resemblance in FSH α-subunit between vertebrates was found (Chien et al., 2005; Shen et al., 2006). To date, an ELISA for the measurement of FSH in sea turtles or in any other reptile has not been developed.
Different studies for development of ELISA to measure FSH were done in several fish and mammalian species: tilapia, rat, mice, flatfish, yellowtail kingfish, Japanese rice fish, Japanese eel and more (Pappa et al., 1999; Shimizu et al., 2012; Chauvigné et al., 2015; Burow et al., 2019; Kazeto et al., 2019; Nocillado et al., 2019; Pappas, 2019; Ongaro et al., 2021). FSH in green sea turtles was last measured in the early 90's (Wibbels et al., 1990) using radioimmunoassay (RIA), which is based on the principle of all immunoassays: a known amount of antigen is labeled with a radioactive element and mixed with a known amount of antibody directed against that antigen (Webster et al., 1990).
The RIA method is problematic because it uses radiolabeled reagents that require a special license for storage and waste disposal (Sancho et al., 2013). In addition, ELISA is more accurate and reproducible at lower concentrations than RIA (Webster et al., 1990).
LC-MS/MS and LC-MRM/MS techniques present the potential for surpassing the accuracy of RIA and ELISA, exhibiting heightened sensitivity towards extremely low concentrations. It's crucial to consider the requirements for regular biomolecule quantitation from a broad range of fields. While immunoassays have been instrumental, they might need to evolve to match the escalating throughput demands. As long as the goals involve reduced per-sample expenses and enhanced precision, the prospects of LC and LC-MS appear promising as a prospective solution.
The FSH concentrations measured in our ELISA were 17-650 ng/ml for females and 24-710 ng/ml for males. The results for FSH level ranges in females do not concur with studies by P. Licht et al., 1979 (0.3-10.6 ng/ml) and Wibbels et al., 1992 (0.1-2 ng/ml). In these studies green sea turtle males did not reveal measurable concentrations of FSH, but observed females FSH concentrations of 3-10 ng/ml in the snapping turtle (Chelydra serpentina) (Mahmoud and Licht, 1997), 2-16 ng/ml in the musk turtle (Sternotherus odoratus) (Mendonca and Licht, 1986) and 6-26 ng/ml in the painted turtle (Chrysemys picta) (Licht et al., 1985).
Our results are higher than those reported in these previous studies. The RIA method was used in all these studies. The antisera used in these studies were not specific enough for FSH and failed to maintain selective neutralization between the gonadotropins (Licht et al., 1977). Licht and Wibbles have conducted measurements on FSH levels in females during the nesting period (20 to 50 hours after each turtle had nested). Our measurements encompass both the months preceding nesting and the period following nesting completion (every two months). It appears that FSH, as previously observed in reptiles, plays a role in elevating the levels of estradiol and testosterone and vitellogenin (Smelker et al., 2014; Bruno et al., 2021). This can explain the high concentrations of FSH observed months prior to nesting. In contrast, non-nesting females exhibited consistently lower FSH concentrations compared to the nesting females.
The ELISA developed in this study showed sensitive characteristics compared to other GTH immunoassays. High precision represented by low intra- assay and inter-assay coefficients of variation. The CV values are similar to those from immunoassays for measuring FSH reported in other species, with a range of 5–8% and 7–15% for intra-assay and inter-assay (Aizen et al., 2007b; Molés et al., 2012; Caron et al., 2018; Nocillado et al., 2019). These findings indicate that recombinant FSH was produced as expected, and that the antiserum correctly recognized the target FSH in the plasma (Kazeto et al., 2019).
The sensitivity of the ELISA was 0.13 ng/ml for measuring FSH concentrations in plasma which was similar or more sensitive in comparison to the sensitivities reported for measuring FSH in fish ELISA’s; 0.24 pg/ml for tilapia (Aizen et al., 2007b), 78 pg/ml for yellowtail kingfish (Nocillado et al., 2019) and 10 pg/ml for flatfish (Chauvigné et al., 2015). The differences in assay sensitivity may be affected by the purification level of the recombinant antigens and antisera (Nocillado et al., 2019).
To increase the specify limitations of the ELISA presented herein, one should consider developing a sandwich ELISA that will permit us to detect each glycoprotein hormone with 2 antibodies, one for the beta subunit and the other for the alpha specific epitope even though the immunogenicity of the alpha subunit is not very high.
Validation of the assay was demonstrated by parallelism between serial dilutions of standard curve and serial dilutions of displacement curves of green sea turtle FSH in plasma. These results indicate that the structure of the rcmFSH we produced is immunologically identical to the native FSH of green sea turtle (Aizen et al., 2007b; Nocillado et al., 2019). We also tested parallelism between serial dilutions of standard curve and serial dilutions of displacement curves for potential measurements of gonadotropins in other turtle species. Parallelism in tested plasma samples of three other species was observed: loggerhead sea turtle, hawksbill sea turtle and African softshell turtle. As seen in several studies on fish species, when parallelism is observed between the standard curve and several dilutions of the same sample it indicates similar protein structure between the different species (Aizen et al., 2007a; Aizen et al., 2007b; Aizen, 2011) Therefore, the structure of green sea turtle FSH is similar to the FSH of the other three turtle species. One should keep in mind that the developed ELISA assay presented herein cannot distinguish between free beta and beta associated with alpha. The two subunits of the gonadotropins are co-translated and they non-covalently combine in the endoplasmic reticulum of gonadotrope (FSH, LH), thyrotroph (TSH), or trophoblast (CG) cells (Cahoreau et al., 2015). Probably, if free FSHβ is present in the blood it can be a result of FSH dissociation.
This discovery will allow us the use of this assay for measuring FSH in these species and possibly more turtle species. In mammals, FSH in females controls the menstrual cycle and stimulates growth of follicles in the ovaries, whereas males FSH is an important hormone regulating spermatogenesis (Licht et al., 1979; Sairam and Krishnamurthy, 2001; Kluge et al., 2012). It is estimated that the mechanism is similar in most vertebrates, but the specific functions of FSH in reptiles are not fully understood (Tripathy and Rai, 2017; Kang et al., 2020). It was suggested that FSH has a distinct function in the stimulation of estradiol during the inter-nesting period that stimulates follicular development in females, and spermatogenesis in males (Wibbels et al., 1992; Mills et al., 2008; Kawazu, 2015; Tripathy and Rai, 2017).
After the development and calibration of the ELISA, we used it to measure concentrations of FSH from turtles in the Mikhmoret breeding facility using plasma samples taken since 2018. Our results show that successful nesting females that laid eggs (2019 and 2020) had an elevation in FSH concentrations during and before nesting season (January- August) compared to non-nesting females that presented relatively low FSH concentrations. FSH concentrations in non-nesting females were highest from April 2021 to September 2021. The females did not lay eggs in that year even though mating attempts were observed. We assumed that the females that haven’t nested yet didn’t reach sexual maturity and therefore the pituitary continues to secrete FSH at high concentrations in order to enter the puberty phase as in the case of other vertebrates.
High FSH concentrations increase the secretion of progesterone due to the ruptured follicle that forms a corpus luteum and produces high concentrations of progesterone (Wibbels et al., 1992; Al-Habsi et al., 2006). In female green sea turtles the high progesterone concentrations were reported slightly before nesting (Al-Habsi et al., 2006; Ikonomopoulou et al., 2014), associated with early stages of ovulation that follow the formation of new active corpora luteum (Licht et al., 1979; Licht, 1980; Al-Habsi et al., 2006) In the loggerhead turtle on the other hand high concentrations were also recorded in April prior to nesting similar to our results in the green sea turtle, but much higher concentrations were recorded when female was sampled 3 days after egg laying for 5 consecutive egg laying sessions with concentrations between 12-15ng/ml (Kakizoe et al., 2010). Additionally, increased progesterone concentrations stimulates albumin that is needed for the egg production (Owens and Morris, 1985). As shown in our results, in nested females, and relatively low in non-nested females.
Nested females showed elevated testosterone concentrations six months prior to mating (November) until the concentrations peaked three months prior to nesting in April, and gradually decreased to lower concentrations at the beginning of the nesting season (June). It has been suggested that a rise in testosterone concentrations can act as a breeding behavior initiator, and a possible trigger to male-attractive pheromones (Licht et al., 1979; Owens and Morris, 1985). Licht et al. (1979) conducted research with captive green turtles in the Cayman farm and reported that testosterone elevation was two months prior to mating until they peaked at the beginning of mating activity (mean >700 pg/ml) followed by testosterone level decrease during nesting activity. loggerhead turtle on the other hand showed testosterone concentrations elevation from February to May (Kakizoe et al., 2010) different from the green turtle.
Our results show a consistent rise in estradiol concentrations as FSH concentrations rises in both males and females. In females, we observed that estradiol concentration start to rise in November during follicular growth, declining towards July, concurring with observations from a study by Blanvillain et al. (2011) for green sea turtles and loggerhead turtles (Kakizoe et al., 2010). High concentration of estradiol were observed in April slightly before the females nested and concentration remain at the same concentrations until they decreased in September. The secretion of estradiol may serve both behavioral and physiological functions; it might increase the drive of migration to nesting beaches, regulate ovarian follicle maturation (Al-Habsi et al., 2006) and stimulate vitellogenesis (Kawazu et al., 2016).
In males, we observed a decrease in estradiol concentrations during August and September - consistent with the study by G. D. Blanvillain et al. (2011), in which the FSH concentrations dropped after the nesting season along with the drive to seek a mating partner. The gradual increase in testosterone concentrations in males during the several months before the onset of mating activity, August to November in our case, was expected as described earlier by Licht (Licht et al., 1979),
Overall FSH role in the green sea turtle was found to be correlated with the release of ovarian and testicular steroids. Further development of GTH ELISA for LH and TSH will contribute to a better understanding of the endocrine controls of the reproductive system in green sea turtle and other related species as well, clarifying the functional differentiation between these GTHs and their effects on other sex hormones. Future work should be directed towards the development and production of recombinant LH and TSH of the green sea turtle, this will help to better test and validate the assay specificity compared to other turtles’ glycoproteins hormones. This research has the potential to encourage the development of methods for increasing the fertility and reproduction of the turtles in the Mikhmoret breeding facility.
The raw data supporting the conclusions of this article will be made available by the authors, without undue reservation.
The animal study was approved by Israel Nature and Parks Authority. The study was conducted in accordance with the local legislation and institutional requirements.
OS: Formal Analysis, Investigation, Methodology, Validation, Visualization, Writing – original draft, Writing – review & editing. OR: Investigation, Methodology, Validation, Visualization, Writing – original draft, Formal Analysis, Writing – review & editing. YL: Conceptualization, Investigation, Methodology, Project administration, Resources, Writing – original draft, Writing – review & editing. JA: Conceptualization, Data curation, Formal Analysis, Funding acquisition, Investigation, Methodology, Project administration, Resources, Supervision, Validation, Visualization, Writing – original draft, Writing – review & editing.
This research was supported by the Israel Science Foundation (ISF) (Grant No. 451/21) and the internal research funding program of Faculty of Marine Sciences, Ruppin Academic Center, Israel.
The authors declare that the research was conducted in the absence of any commercial or financial relationships that could be construed as a potential conflict of interest.
All claims expressed in this article are solely those of the authors and do not necessarily represent those of their affiliated organizations, or those of the publisher, the editors and the reviewers. Any product that may be evaluated in this article, or claim that may be made by its manufacturer, is not guaranteed or endorsed by the publisher.
The Supplementary Material for this article can be found online at: https://www.frontiersin.org/articles/10.3389/fmars.2023.1260205/full#supplementary-material
Aizen J. (2011). Production , biochemical analysis and role of gonadotropins in tilapia and carp. Ph.D (Jerusalem, Israel, Hebrew University).
Aizen J., Kasuto H., Golan M., Zakay H., Levavi-Sivan B. (2007a). Tilapia follicle-stimulating hormone (FSH): immunochemistry, stimulation by gonadotropin-releasing hormone, and effect of biologically active recombinant FSH on steroid secretion1. Biol. Reprod. 76 (4), 692–700. doi: 10.1095/biolreprod.106.055822
Aizen J., Kasuto H., Levavi-Sivan B. (2007b). Development of specific enzyme-linked immunosorbent assay for determining LH and FSH levels in tilapia, using recombinant gonadotropins. Gen. Comp. Endocrinol. 153 (1-3), 323–332. doi: 10.1016/j.ygcen.2007.04.004
Al-Habsi A. A., AlKindi A. Y. A., Mahmoud I. Y., Owens D. W., Khan T., al-Abri A. (2006). Plasma hormone levels in the green turtles Chelonia mydas during peak period of nesting at Ras Al-Hadd-Oman. J. Endocrinol. 191 (1), 9–14. doi: 10.1677/joe.1.06790
Balamurugan V., Singh R. P., Saravanan P., Sen A., Sarkar J., Sahay B., et al. (2007). Development of an indirect ELISA for the detection of antibodies against Peste-des-petits-ruminants virus in small ruminants. Vet. Res. Commun. 31 (3), 355–364. doi: 10.1007/s11259-006-3442-x
Barlow R. D., Thompson S. G., Cuckle H. S., Wald N. J., Voller A. (1986). Comparison of an ELISA with a RIA method for serum alpha-fetoprotein determination in screening for fetal neural tube defects. Ann. Clin. Biochem. 23 (3), 334–339. doi: 10.1177/000456328602300315
Blanvillain G., Owens D. W., Kuchling G. (2011). "Chapter 10 - Hormones and Reproductive Cycles in Turtles," in Hormones and Reproduction of Vertebrates, eds. Norris D. O., Lopez K. H.. (London: Academic Press), 277–303. doi: 10.13140/2.1.2558.9440
Blanvillain G., Pease A. P., Segars A. L., Rostal D. C., Richards A. J., Owens D. W. (2008). Comparing methods for the assessment of reproductive activity in adult male loggerhead sea turtles Caretta caretta at Cape Canaveral, Florida. Endangered Species Res. 6 (1), 75–85. doi: 10.3354/esr00136
Bousfield G. R., Butnev V. Y., Walton W. J., Nguyen V. T., Huneidi J., Singh V., et al. (2007). All-or-none N-glycosylation in primate follicle-stimulating hormone β-subunits. Mol. Cell. Endocrinol. 260-262, 40–48. doi: 10.1016/j.mce.2006.02.017
Bruno R. S., Restrepo MaChado J. A., Guzman G. R. B., Loria J. I. R., Valverde R. A. (2021). Biomarkers of reproduction in endangered green sea turtles (Chelonia mydas) nesting at Tortuguero, Costa Rica. Conserv. Physiol. 9 (1). doi: 10.1093/conphys/coab072
Burow S., Fontaine R., von Krogh K., Mayer I., Nourizadeh-Lillabadi R., Hollander-Cohen L., et al. (2019). Establishment of specific enzyme-linked immunosorbent assay (ELISA) for measuring Fsh and Lh levels in medaka (Oryzias latipes), using recombinant gonadotropins. MethodsX 6, 1473–1479. doi: 10.1016/j.mex.2019.06.011
Cahoreau C., Klett D., Combarnous Y. (2015). Structure–function relationships of glycoprotein hormones and their subunits’ Ancestors. Front. Endocrinol. 6. doi: 10.3389/fendo.2015.00026
Caron A. G. M., Thomas C. R., Berry K. L. E., Motti C. A., Ariel E., Brodie J. E. (2018). Ingestion of microplastic debris by green sea turtles (Chelonia mydas) in the Great Barrier Reef: Validation of a sequential extraction protocol. Mar. pollut. Bull. 127, 743–751. doi: 10.1016/j.marpolbul.2017.12.062
Chauvigné F., Verdura S., Mazón M. J., Boj M., Zanuy S., Gómez A., et al. (2015). Development of a flatfish-specific enzyme-linked immunosorbent assay for Fsh using a recombinant chimeric gonadotropin. Gen. Comp. Endocrinol. 221, 75–85. doi: 10.1016/j.ygcen.2014.10.009
Chien J.-T., Shen S.-T., Lin Y.-S., Yu J. Y.-L. (2005). Molecular cloning of the cDNA encoding follicle-stimulating hormone β subunit of the Chinese soft-shell turtle Pelodiscus sinensis, and its gene expression. Gen. Comp. Endocrinol. 141 (2), 190–200. doi: 10.1016/j.ygcen.2004.12.017
Cross T., Hornshaw M. (2016). Can LC and LC-MS ever replace immunoassays? EXPERT OPINION. J. Appl. Bioanalysis 2, 108–116. doi: 10.17145/jab.16.015
Daly R., Hearn M. T. W. (2005). Expression of heterologous proteins in Pichia pastoris: a useful experimental tool in protein engineering and production. J. Mol. Recognition 18 (2), 119–138. doi: 10.1002/jmr.687
Goldberg D. W., Leitão S. A. T., Godfrey M. H., Lopez G. G., Santos A. J. B., Neves F. A., et al. (2013). Ghrelin and leptin modulate the feeding behaviour of the hawksbill turtle Eretmochelys imbricata during nesting season. Conserv. Physiol. 1 (1). doi: 10.1093/conphys/cot016
Ikonomopoulou M. P., Bradley A. J., Ibrahim K., Limpus C. J., Fernandez-Rojo M. A., Vagenas D., et al. (2014). Hormone and metabolite profiles in nesting green and flatback turtles: turtle species with different life histories. Adv. Zoology 2014, 503209. doi: 10.1155/2014/503209
Kakizoe Y., Fujiwara M., Akune Y., Kanou Y., Saito T., Uchida I. (2010). Cyclical changes of plasma sex steroids in captive breeding loggerhead turtles (Caretta caretta). J. Zoo Wildlife Med. 41 (4), 643–648, 646. doi: 10.1638/2009-0254.1
Kang H., Kenealy T. M., Cohen R. E. (2020). The hypothalamic-pituitary-gonadal axis and thyroid hormone regulation interact to influence seasonal breeding in green anole lizards (Anolis carolinensis). Gen. Comp. Endocrinol. 292, 113446. doi: 10.1016/j.ygcen.2020.113446
Karbalaei M., Rezaee S. A., Farsiani H. (2020). Pichia pastoris: A highly successful expression system for optimal synthesis of heterologous proteins. J. Cell. Physiol. 235 (9), 5867–5881. doi: 10.1002/jcp.29583
Kasuto H., Levavi-Sivan B. (2005). Production of biologically active tethered tilapia LHβα by the methylotrophic yeast Pichia pastoris. Gen. Comp. Endocrinol. 140 (3), 222–232. doi: 10.1016/j.ygcen.2004.10.016
Kawazu I. (2015). Reproductive biology of captive hawksbill turtles and the development of a technique for artificial insemination. Ph.D (Nagasaki University, Japan, Nagasaki University).
Kawazu I., Nakada K., Maeda K., Sawamukai Y. (2016). Daily changes in the blood levels of two steroids and other biochemicals related to vitellogenesis and eggshell formation during internesting intervals in a captive female loggerhead turtle. Curr. Herpetology 35 (1), 14–21, 18. doi: 10.5358/hsj.35.14
Kawazu I., Suzuki M., Maeda K., Kino M., Koyago M., Moriyoshi M., et al. (2014). Ovulation induction with follicle-stimulating hormone administration in hawksbill turtles eretmochelys imbricata. Curr. Herpetology 33 (1), 88–93, 86. doi: 10.5358/hsj.33.88
Kazeto Y., Tanaka T., Suzuki H., Ozaki Y., Fukada H., Gen K. (2019). Development and validation of enzyme-linked immunosorbent assays specific for follicle-stimulating hormone and luteinizing hormone in Japanese eel. Fisheries Sci. 85 (5), 829–837. doi: 10.1007/s12562-019-01338-8
Kluge M., Schüssler P., Schmidt D., Uhr M., Steiger A. (2012). Ghrelin suppresses secretion of luteinizing hormone (LH) and follicle-stimulating hormone (FSH) in women. J. Clin. Endocrinol. Metab. 97 (3), E448–E451. doi: 10.1210/jc.2011-2607
Li P., Anumanthan A., Gao X.-G., Ilangovan K., Suzara V. V., Düzgüneş N., et al. (2007). Expression of recombinant proteins in pichia pastoris. Appl. Biochem. Biotechnol. 142 (2), 105–124. doi: 10.1007/s12010-007-0003-x
Licht P. (1980). Evolutionary and functional aspects of pituitary gonadotropins in the green turtle, chelonia mydas. Am. Zoologist 20 (3), 565–574. doi: 10.1093/icb/20.3.565
Licht P., Breitenbach G. L., Congdon J. D. (1985). Seasonal cycles in testicular activity, gonadotropin, and thyroxine in the painted turtle, Chrysemys picta, under natural conditions. Gen. Comp. Endocrinol. 59 (1), 130–139. doi: 10.1016/0016-6480(85)90427-7
Licht P., MacKenzie D. S., Papkoff H., Farmer S. (1977). Immunological studies with the gonadotropins and their subunits from the green sea turtle Chelonia mydas. Gen. Comp. Endocrinol. 33 (2), 231–241. doi: 10.1016/0016-6480(77)90248-9
Licht P., Wood J., Owens D. W., Wood F. (1979). Serum gonadotropins and steroids associated with breeding activities in the green sea turtle Chelonia mydas: I. Captive animals. Gen. Comp. Endocrinol. 39 (3), 274–289. doi: 10.1016/0016-6480(79)90122-9
Mahmoud I. Y., Licht P. (1997). Seasonal changes in gonadal activity and the effects of stress on reproductive hormones in the common snapping turtle,Chelydra serpentina. Gen. Comp. Endocrinol. 107 (3), 359–372. doi: 10.1006/gcen.1997.6944
Mazor T., Levin N., Possingham H. P., Levy Y., Rocchini D., Richardson A. J., et al. (2013). Can satellite-based night lights be used for conservation? The case of nesting sea turtles in the Mediterranean. Biol. Conserv. 159, 63–72. doi: 10.1016/j.biocon.2012.11.004
Melchiorre C., Chhuon C., Jung V., Lipecka J., Di Rella F., Conforti A., et al. (2021). Identification and Relative Quantification of hFSH Glycoforms in Women's Sera via MS-PRM-Based Approach. Pharmaceutics 13 (6), 1–13. doi: 10.3390/pharmaceutics13060798
Mendonca M. T., Licht P. (1986). Seasonal cycles in gonadal activity and plasma gonadotropin in the musk turtle, Sternotherus odoratus. Gen. Comp. Endocrinol. 62 (3), 459–469. doi: 10.1016/0016-6480(86)90056-0
Mills S. C., Hazard L., Lancaster L., Mappes T., Miles D., Oksanen T. A., et al. (2008). Gonadotropin Hormone Modulation of Testosterone, Immune Function, Performance, and Behavioral Trade-Offs among Male Morphs of the Lizard Uta stansburiana. Am. Nat. 171 (3), 339–357. doi: 10.1086/527520
Molés G., Gómez A., Carrillo M., Zanuy S. (2012). Development of a homologous enzyme-linked immunosorbent assay for European sea bass FSH. Reproductive cycle plasma levels in both sexes and in yearling precocious and non-precocious males. Gen. Comp. Endocrinol. 176 (1), 70–78. doi: 10.1016/j.ygcen.2011.12.029
Ng T. B., Lee Y. H., Chan S. T. H. (1986). Pituitary extract of the ricefield eel Monopterus albus (synbranchidae, teleostei) exhibits gonadotropic activity in the classes mamMalia, aves, reptilia and amphibia. Comp. Biochem. Physiol. Part A: Physiol. 84 (2), 371–381. doi: 10.1016/0300-9629(86)90632-8
Nielsen H. (2017). “Predicting secretory proteins with signalP,” in Protein function prediction: methods and protocols. Ed. Kihara D. (New York, NY: Springer New York), 59–73.
Nocillado J., Palma P., Fielder S., Zanardini M., Dennis L. P., Elizur A. (2019). Development of specific enzyme-linked immunosorbent assay for yellowtail kingfish (Seriola lalandi) follicle stimulating hormone using recombinant gonadotropins. Gen. Comp. Endocrinol. 282, 113208. doi: 10.1016/j.ygcen.2019.113208
Ongaro L., Alonso C. A. I., Zhou X., Brûlé E., Li Y., Schang G., et al. (2021). Development of a highly sensitive ELISA for measurement of FSH in serum, plasma, and whole blood in mice. Endocrinology 162 (4), 1–11. doi: 10.1210/endocr/bqab014
Owens D. W., Morris Y. A. (1985). The comparative endocrinology of sea turtles. Copeia 1985 (3), 723–735. doi: 10.2307/1444766
Pappa A., Seferiadis K., Marselos M., Tsolas O., Messinis I. E. (1999). Development and application of competitive ELISA assays for rat LH and FSH. Theriogenology 51 (5), 911–926. doi: 10.1016/S0093-691X(99)00038-2
Pappas A. (2019). “Ria: principles and application,” in Radiobioassays, 1st Edition. Ed. Ashkar F. S. (Boca Raton: CRC Press), 240.
Rostal D. C., Owens D. W., Grumbles J. S., MacKenzie D. S., Amoss M. S. (1998). Seasonal reproductive cycle of the kemp's ridley sea turtle (Lepidochelys kempi). Gen. Comp. Endocrinol. 109 (2), 232–243. doi: 10.1006/gcen.1997.7026
Sairam M. R., Krishnamurthy H. (2001). The role of follicle-stimulating hormone in spermatogenesis: lessons from knockout animal models. Arch. Med. Res. 32 (6), 601–608. doi: 10.1016/S0188-4409(01)00328-9
Sancho M., Arnal J. M., García-Fayos B. (2013). Treatment of hospital radioactive liquid wastes from RIA (radioimmunoassay) by membrane technology. Desalination 321, 110–118. doi: 10.1016/j.desal.2013.03.032
Seminoff J., Allen C., Balazs G., Dutton P., Eguchi T., Haas H., et al. (2015). Status review of the green turtle (Chelonia mydas) under the Endangered Species Act.
Shen S.-T., Cheng Y.-S., Shen T.-Y., Yu J. Y.-L. (2006). Molecular cloning of follicle-stimulating hormone (FSH)-β subunit cDNA from duck pituitary. Gen. Comp. Endocrinol. 148 (3), 388–394. doi: 10.1016/j.ygcen.2006.03.013
Shimizu A., Ohkubo M., Hamaguchi M. (2012). Development of non-competitive enzyme-linked immunosorbent assays for mummichog Fundulus heteroclitus gonadotropins – Examining seasonal variations in plasma FSH and LH levels in both sexes. Gen. Comp. Endocrinol. 178 (3), 463–469. doi: 10.1016/j.ygcen.2012.07.003
Smelker K., Smith L., Arendt M., Schwenter J., Rostal D., Selcer K., et al. (2014). Plasma vitellogenin in free-ranging loggerhead sea turtles (Caretta caretta) of the northwest atlantic ocean. J. Mar. Biol. 2014, 748267. doi: 10.1155/2014/748267
Tim S. J., Mark H., Colin J. L. (2004). Body condition and physiological changes in male green turtles during breeding. Mar. Ecol. Prog. Ser. 276, 281–288. doi: 10.3354/meps276281
Tripathy M., Rai U. (2017). Temporal expression and gonadotropic regulation of aromatase and estrogen receptors in the ovary of wall lizard, Hemidactylus flaviviridis: Correlation with plasma estradiol and ovarian follicular development. Steroids 128, 23–31. doi: 10.1016/j.steroids.2017.10.005
Warnken T., Huber K., Feige K. (2016). Comparison of three different methods for the quantification of equine insulin. BMC Veterinary Res. 12 (1), 196. doi: 10.1186/s12917-016-0828-z
Webster H. V., Bone A. J., Webster K. A., Wilkin T. J. (1990). Comparison of an enzyme-linked immunosorbent assay (ELISA) with a radioimmunoassay (RIA) for the measurement of rat insulin. J. Immunol. Methods 134 (1), 95–100. doi: 10.1016/0022-1759(90)90116-D
Wewer Albrechtsen N. J., Hartmann B., Veedfald S., Windeløv J. A., Plamboeck A., Bojsen-Møller K. N., et al. (2014). Hyperglucagonaemia analysed by glucagon sandwich ELISA: nonspecific interference or truly elevated levels? Diabetologia 57 (9), 1919–1926. doi: 10.1007/s00125-014-3283-z
Wibbels T., Owens D. W., Light P., Limpus C., Reed P. C., Amoss M. S. (1992). Serum gonadotropins and gonadal steroids associated with ovulation and egg production in sea turtles. Gen. Comp. Endocrinol. 87 (1), 71–78. doi: 10.1016/0016-6480(92)90151-9
Wibbels T., Owens D. W., Limpus C. J., Reed P. C., Amoss M. S. (1990). Seasonal changes in serum gonadal steroids associated with migration, mating, and nesting in the loggerhead sea turtle (Caretta caretta). Gen. Comp. Endocrinol. 79 (1), 154–164. doi: 10.1016/0016-6480(90)90099-8
Keywords: FSH, ELISA, sea turtle, nesting, progesterone, reproduction
Citation: Soffer O, Rubin O, Levy Y and Aizen J (2023) Development of an enzyme-linked immunosorbent assay for determining FSH plasma concentrations in green sea turtle (Chelonia mydas), using recombinant gonadotropins. Front. Mar. Sci. 10:1260205. doi: 10.3389/fmars.2023.1260205
Received: 17 July 2023; Accepted: 23 August 2023;
Published: 11 September 2023.
Edited by:
James Scott Maki, Marquette University, United StatesReviewed by:
Yves Combarnous, Centre National de la Recherche Scientifique (CNRS), FranceCopyright © 2023 Soffer, Rubin, Levy and Aizen. This is an open-access article distributed under the terms of the Creative Commons Attribution License (CC BY). The use, distribution or reproduction in other forums is permitted, provided the original author(s) and the copyright owner(s) are credited and that the original publication in this journal is cited, in accordance with accepted academic practice. No use, distribution or reproduction is permitted which does not comply with these terms.
*Correspondence: Joseph Aizen, YWl6ZW5AcnVwcGluLmFjLmls
Disclaimer: All claims expressed in this article are solely those of the authors and do not necessarily represent those of their affiliated organizations, or those of the publisher, the editors and the reviewers. Any product that may be evaluated in this article or claim that may be made by its manufacturer is not guaranteed or endorsed by the publisher.
Research integrity at Frontiers
Learn more about the work of our research integrity team to safeguard the quality of each article we publish.