- 1Key Laboratory of Sustainable Development of Polar Fisheries, Ministry of Agriculture and Rural Affairs, Yellow Sea Fisheries Research Institute, Chinese Academy of Fishery Science, Qingdao, Shandong, China
- 2Joint Laboratory for Open Sea Fishery Engineering, Qingdao Marine Science and Technology Center, Qingdao, Shandong, China
The waters near the Antarctic Peninsula are characterized with unique oceanographic conditions and rich krill resources. Based on samples collected around the South Shetland Islands (SSI) in austral summer of 2019/2020, the net- phytoplankton community structure and relevant major biotic and abiotic influencing factors were investigated. Eighty-one taxa were identified by light microscope, and diatoms were the most abundant group. The most abundant species were Chaetoceros atlanticus, C. criophilus, C. dichaeta, Fragilariopsis kerguelensis and Pseudo−nitzschia lineola. The abundance and Shannon-Weaver index of net-phytoplankton ranged from 100 to 2.64×107 cells/m3 and 0.0747 to 4.0176 respectively, with significantly low values detected in the Bransfield Strait (BS) and high values in the west of the SSI. The dissimilarity was mainly caused by the differences in abundance of diatoms (including Thalassiothrix antarctica and the species in genus Rhizosolenia, Chaetoceros, Fragilariophsis). These diatoms and Dictyocha speculum were found in higher abundance in the west of the SSI, while Corethron pennatum and cryptophytes were found in higher abundance in the BS. Combined with acoustic density of krill and environmental data (Sea Surface Temperature and Sea Ice Concentration). The multivariate analysis suggested that phytoplankton community was positively affected by the SST, and the acoustic- derived krill density would be associated with the spatial distribution of pennate diatoms. This study enhances the knowledge about the selective feeding for krill and provides ecological implications for the Antarctic marine ecosystem.
1 Introduction
The Southern Ocean is recognized as a key region in the modulation and global marine carbon cycle (Gonçalves-Araujo et al., 2015). There are complex water masses around the South Shetland Islands (SSI) especially in the waters of the Bransfield Strait (BS) which connect the Bellingshausen Sea and the Weddell Sea (Gonçalves-Araujo et al., 2015). The complexity of water masses and their diverse thermohaline structures makes the regions around the SSI be a hotspot for phytoplankton assemblages and high trophic predators.
Phytoplankton plays crucial roles in the marine ecosystem and they could respond sensitively to changes in the environment (Schloss and Estrada, 1994; Vernet et al., 2008; Liu et al., 2021). High values of phytoplankton biomass have been observed in particular regions, especially at oceanic fronts, marginal ice zones and near shore straits, bays, and lees of islands (Prézelin et al., 2000; Mendes et al., 2012). During the austral summer, the distribution of phytoplankton is patchy. The blooms in the Antarctic waters were dominated by nanoflagellates (Mascioni et al., 2019) or microphytoplankton (mainly diatoms) which were both occurred and recorded. The waters along the AP exhibits high value of phytoplankton abundance (Hewes et al., 2009). In contrast, waters in the BS have been dominated by nanoflagellates and characterized by low primary production away from the melting of sea ice (Holm-Hansen and Mitchell, 1991; Lancelot et al., 1993; Kang et al., 2001). In the west of the SSI, microphytoplankton community composition has been characterized by the genus of Rhizosolenia and Chaetoceros (Luan et al., 2013).
In the context of global climate change, there was a shift from micro-diatoms to nanoflagellates (Costa et al., 2020). When water heats up, stratification of water column caused by the sea-ice melting leads to the phytoplankton bloom (Rozema et al., 2017a). As sea ice receded, diatoms bloom to higher abundance and then, are replaced by cryptophytes (Ducklow et al., 2007). Besides abiotic factors, the distribution of phytoplankton may also be affected by the consumption of Antarctic krill (Euphausia superba, hereafter krill). Krill is a key species in the Antarctic marine ecosystem linking between phytoplankton and higher trophic predators. More than 50% total krill biomass are presumed to be located in the southwest Atlantic sector, in particularly in the waters around SSI (Atkinson et al., 2004; Hewitt et al., 2004; Watters et al., 2020). Krill is an important grazer on phytoplankton and a large krill aggregation can exert great pressure on phytoplankton biomass (Froneman et al., 2000; Bernard et al., 2012). Diatoms are the major food resources for krill and krill is mainly effective at grazing particles larger than 10 μm equivalent spherical diameter (ESD) (McClatchie and Boyd, 1983; Ishii et al., 1985; Haberman et al., 2003b).
As early as in the middle of the 20th century, there were surveys about microphytoplankton in Antarctic (Froneman et al., 1997). Due to the application of molecular and pigment analysis, composition of microphytoplankton by microscope is scarce. To supplement the lack of up-to-date knowledge about the microphytoplankton community, phytoplankton community around the SSI was investigated. In addition, regions near the SSI have suffered great impacts of climate change, so phytoplankton dynamics could reinforce the understanding about the response to the regional environment change including the sea surface temperature (SST), sea ice concentration (SIC) and krill density. The objective of this study is to clarify the distribution pattern and spatial difference of net- phytoplankton, identify the possible influencing factors, and then provide some indicators for krill selective grazing.
2 Materials and methods
2.1 Samples and data collection
The net-phytoplankton samples were collected during the Antarctic krill survey conducted by the Chinese krill fishing vessel Fu Rong Hai around the SSI from 8 to 12 in March 2020. Samples were collected at 40 stations with 21 stations in the west of SSI and 19 stations in the BS (Figure 1). Net-phytoplankton samples were collected with a standard net III (net area 0.1 m2, mesh size 76 μm) by vertical hauling from 200 m depth or from the bottom to the surface when the depth was less than 200 m. The collected samples were preserved in 1 L bottles with 5% formaldehyde solution.
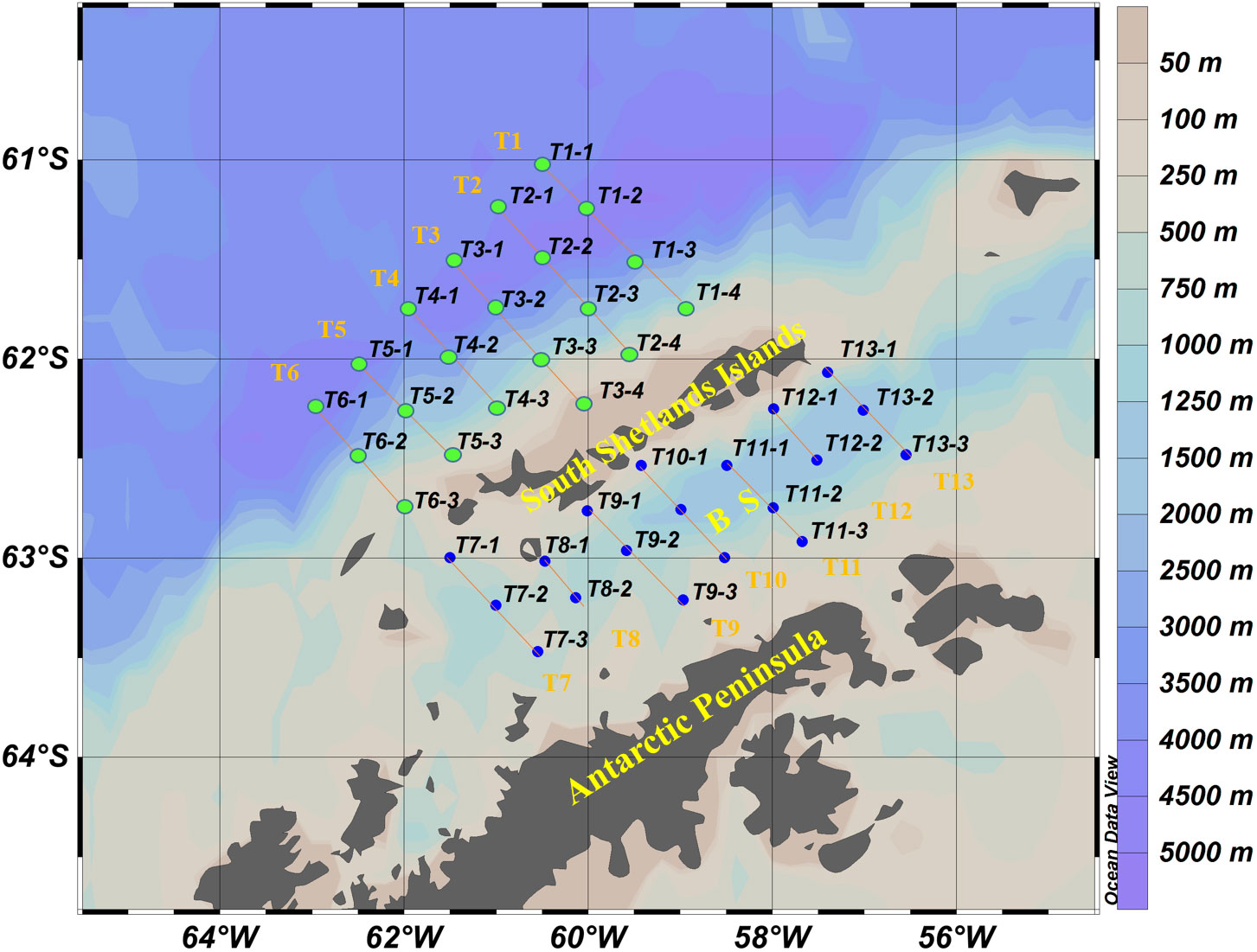
Figure 1 Map of sampling stations and transects (T1-T13) around the South Shetland Islands. BS- Bransfield Strait. The green dots were the stations in the west of SSI which defined as cluster 1, and the blue dots were the stations in the BS which defined as cluster 2.
The krill density was estimated using acoustic data collected from a hull-mounted Simrad EK60 echosounder onboard F/V Fu Rong Hai along the transects survey as shown in Figure 1. Krill backscatters were identified using the swarm-based method (Krafft et al., 2021). The acoustic backscatter at 120 kHz attributed to krill were then integrated as nautical area scattering coefficient (NASC, m2/n. mile2) from the surface exclusion layer (15 m) to the lower limit (250 m), and exported at an elementary distance sampling unit (EDSU) of 1 n.mile. In the subsequent correlation analysis, the NASC values of each station were averaged by 6 n. miles (3 n. miles before and after of each station).
Environmental data, including the SST and SIC, were obtained from the Copernicus Marine Data Store (https://resources.marine.copernicus.eu/). The spatial resolution of the original data was 0.05° × 0.05°, and temporal resolution was daily mean.
2.2 Samples and data analysis
In the land-based laboratory, the phytoplankton samples were settled for more than 48 h, and the supernatant was aspirated off. The volume of the concentrated samples was about 100 ml, 0.5 mL of which was analyzed and counted under the Nikon Eclipse Ti2-U inverted microscope with 200× to 400× magnification. The taxa identification was based on species morphology referred to the books and literatures on phytoplankton classification and the website of www.algaebase.org.
The taxa abundance (A) was calculated as:
where ni is the number of cells in species i, V1 is the analyzed volume 0.5 mL and V2 is the concentrated volume. V is the filtration volume when hauling the net which was calculated as 200 m or the depth of water minus two meters and then multiply by the net area.
Shannon-Weaver index (H’) was used to evaluate the species diversity of phytoplankton community. H’ is calculated as (Shannon and Weaver, 1949):
Dominance (Y) was calculated as:
where N is the total number of phytoplankton cells; S is the number of species and Pi is the ratio of the number of cells i to the total numbers, ni is the number of cells in species i and fi is the frequency of species i.
Phytoplankton community structure was examined by carrying out a multivariate analysis on abundance. Clustering was performed for each dataset based on the Bray- Curtis similarity matrix of log (x+1) transformed phytoplankton abundance and the average linkage group classification (Field et al., 1982; Shi et al., 2020) to distinguish the phytoplankton communities. In the process of analysis, stations in the west of SSI were defined as cluster 1, and the others were defined as cluster 2. ANOSIM (analysis of similarities) procedure was used to test the difference of phytoplankton community. To understand the circumstances of the dissimilar species that caused the difference between stratums, a similarity percentage analysis (SIMPER) was conducted. The species causing the difference between clusters were listed. The BIO-ENV analysis with Spearman rank correlation was carried out between the taxonomic and environmental data, to evaluate the best sets of environmental factors including SST, SIC and acoustic density of krill on phytoplankton communities.
3 Results
3.1 Net-phytoplankton community
Eighty-one taxa were identified in this study and the composition of phytoplankton was listed in Table 1. Diatoms were the dominant group with 67 species. Others groups including dinoflagellates, silicoflagellates and cryptophytes were less abundant. The most abundant species were all diatoms in chains including Chaetoceros atlanticus, C. criophilus, C. dichaeta, Fragilariopsis kerguelensis, and Pseudo−nitzschia lineola.
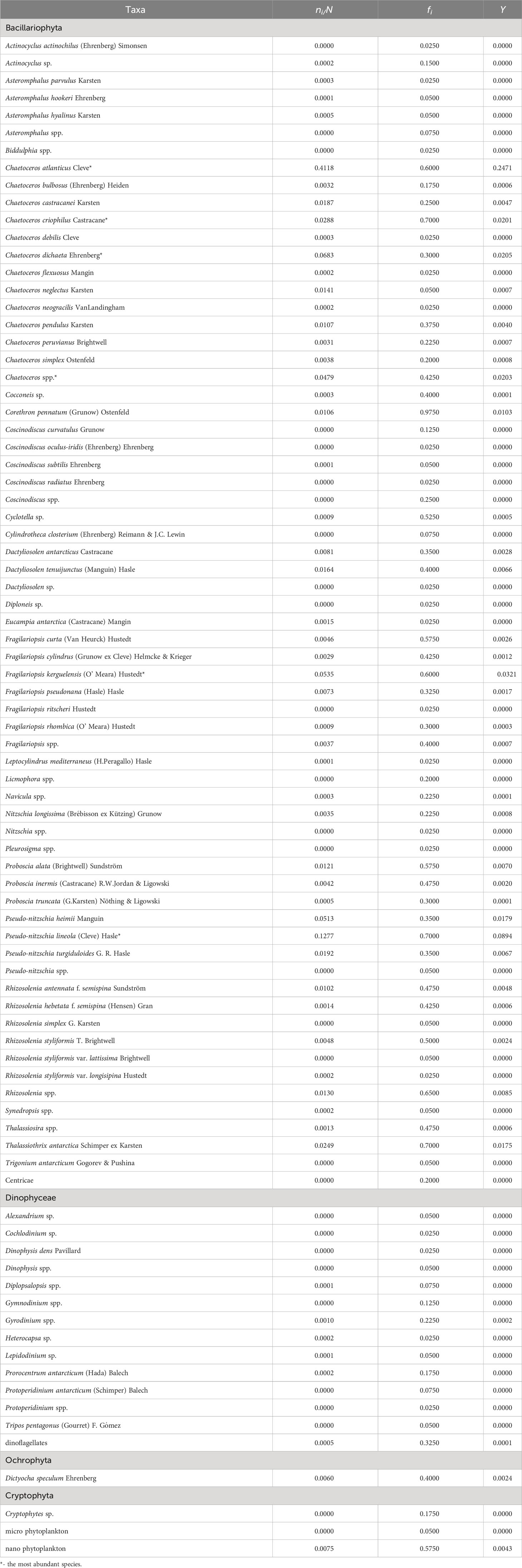
Table 1 Taxa composition of net-phytoplankton around the South Shetland Islands during 8 to 12 March 2020.
The distributions of different phytoplankton groups abundance were exhibited in Figure 2. Total phytoplankton abundance ranged from a minimum of 100 cells/m3 at T13-2 station to a maximum of 2.64×107 cells/m3 at T5-1 station. Phytoplankton abundance in the west of the SSI was apparently higher than that in the BS. The distribution patterns of different groups (diatoms, dinoflagellates and most abundant species) were similar to the total abundance. Compared with pennate diatoms, centric diatoms were more abundant in the BS.
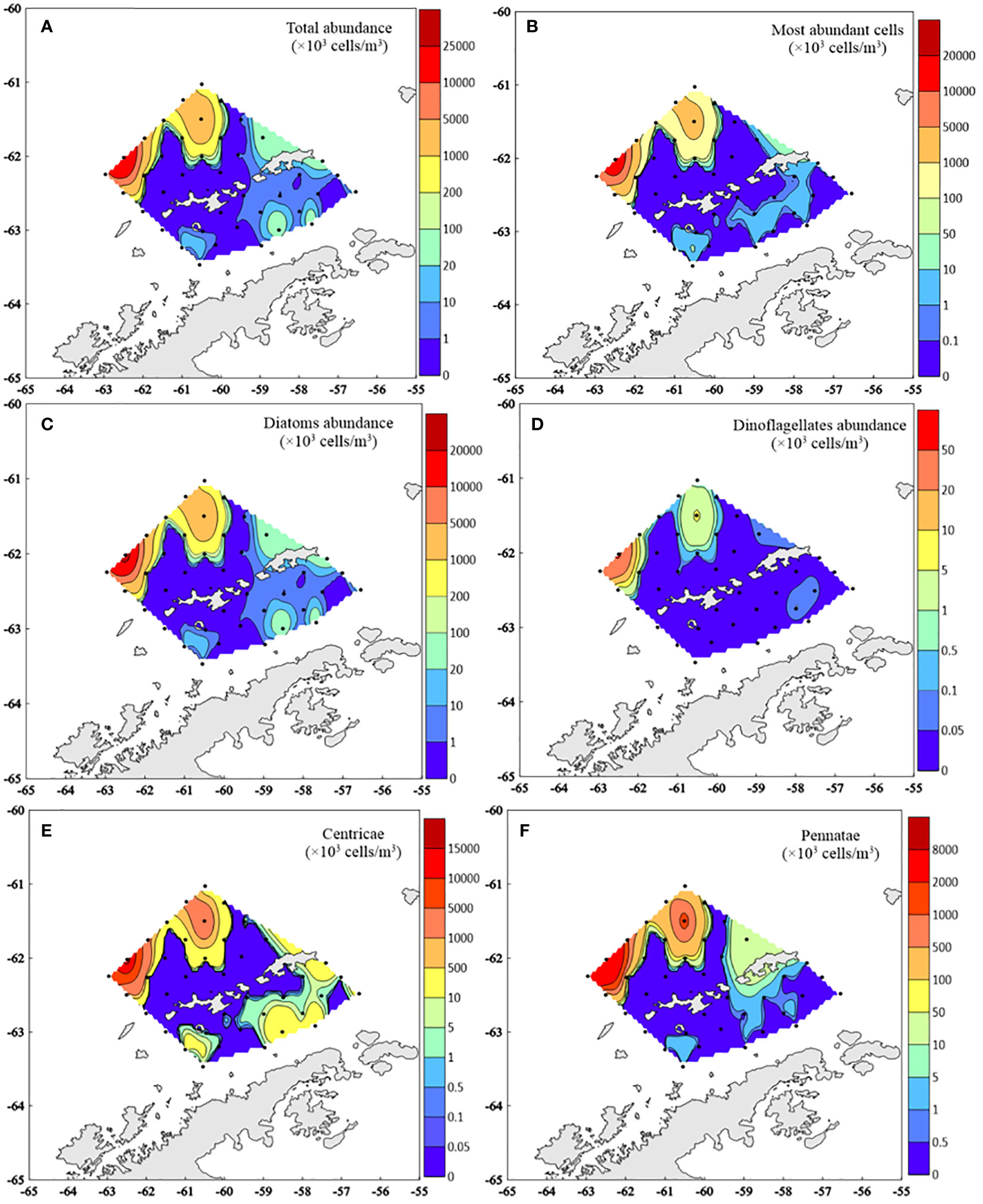
Figure 2 Distribution of different phytoplankton groups abundance (×103 cells/m3) (A- Total abundance; B- Most abundant microphytoplankton cells abundance; C- Diatoms abundance; D- Dinoflagellates abundance; E- Centricae abundance; F- Pennatae abundance).
The H’ ranged from 0.0747 to 4.0176 (Figure 3). In general, diversity indices were clearly higher in transects T1-T6 than those calculated in transects T7-T13. Interestingly, sampling stations with extremely low species diversity were almost dominated by one species namely Corethron pennatum. For example, T11-3 was the station with the lowest diversity index, where Corethron pennatum accounted for 99.33% of the total abundance. And the second lowest index was found at the station T13-1, where C. pennatum accounted for 98.90% of the total abundance.
3.2 Spatial differences of phytoplankton community
Two clusters were classified at 25% of the similarity level shown in Figure 4, which were significant different between cluster 1 and 2 (ANOSIM: R=0.513, p=0.001). In summary, cluster 1 mainly assembled the stations in the west of the SSI whereas cluster 2 in the BS.
To understand the circumstances of the dissimilar species that caused the difference between two clusters, we then conducted a similarity percentage analysis (SIMPER). The average dissimilarity was 76.65% between two clusters. In view of the significant difference, the species that contribute more than 3% were listed in Table 2 and their accumulated contribution added to 37.13%. Some diatom species contributed more for the dissimilarity between the clusters at sampling stations (Table 2), mainly referred to centric diatoms (Rhizosolenia styliformis, Chaetoceros criophilus, C. atlanticus, R. antennata f. semispina, Proboscia alata, and Corethron pennatum).
3.3 Influencing factors on the net- phytoplankton community
The SST ranged from -0.35 to 3.88 °C. The SST in the BS was apparently lower than that in the west of the SSI (Figure 5). The distribution of SIC was totally different from the SST spatial distribution. The SIC values in most stations were mostly 0, namely there was no ice cover. Only several stations including T4-3, T8-1, T9-1, T10-1 near the SSI still had a few bits of ice floes.
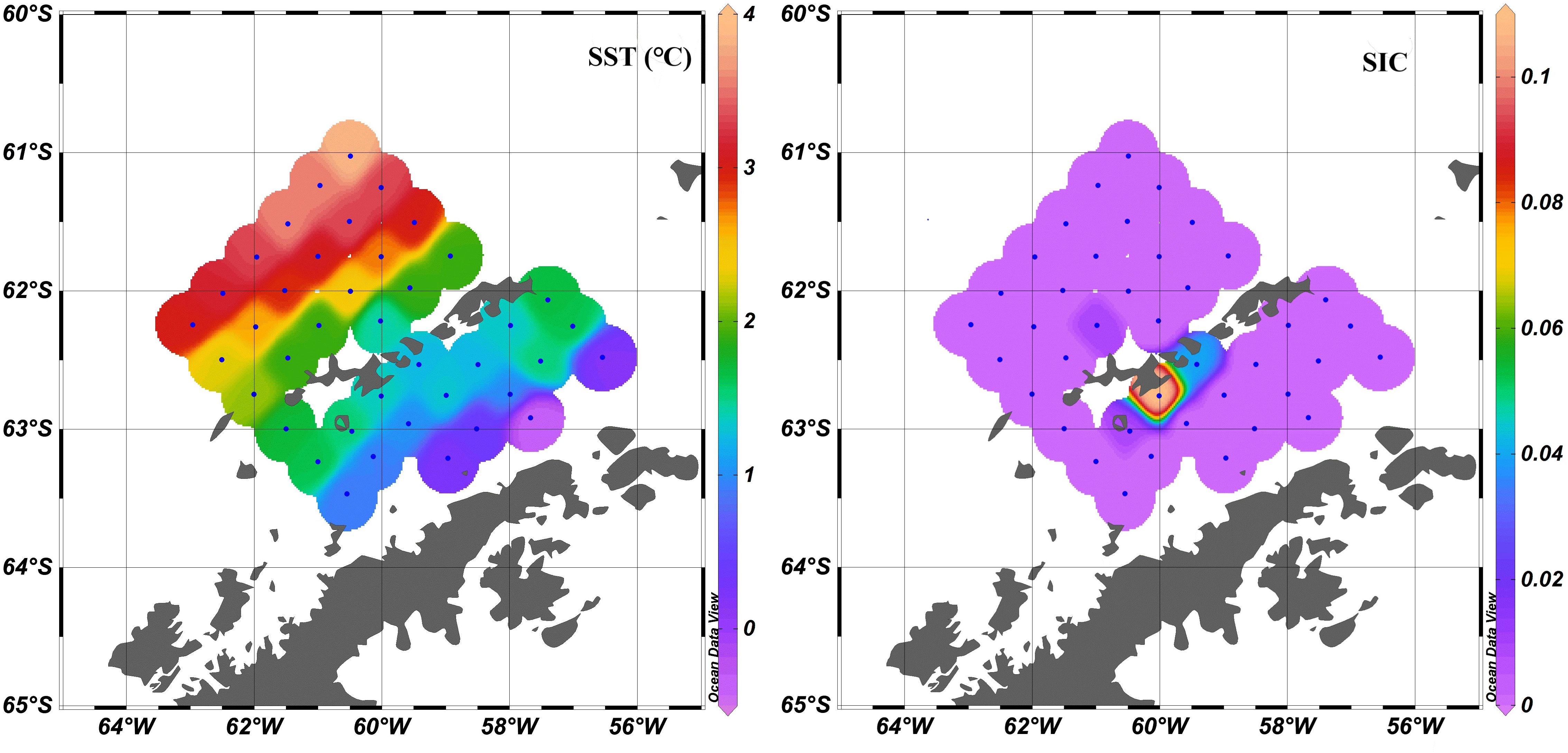
Figure 5 Distribution of sea surface temperature (left) and sea ice concentration (right). Data were obtained from the Copernicus Marine Data Store. The spatial resolution of the original data was 0.05° × 0.05°, and temporal resolution was daily mean.
Acoustic density of krill, ranged from 0 to 1194.4 m2/n. mile2, showed obvious spatial difference (Figure 6). In general, acoustic density was higher in the BS than on the west side of SSI and varied greatly between stations. More than half of stations had NASC values less than 10 m2/n. mile2, even the values in 13 stations were 0. And the high values occurred at transects T8, T9 and T10 in the BS (see Figure 1).
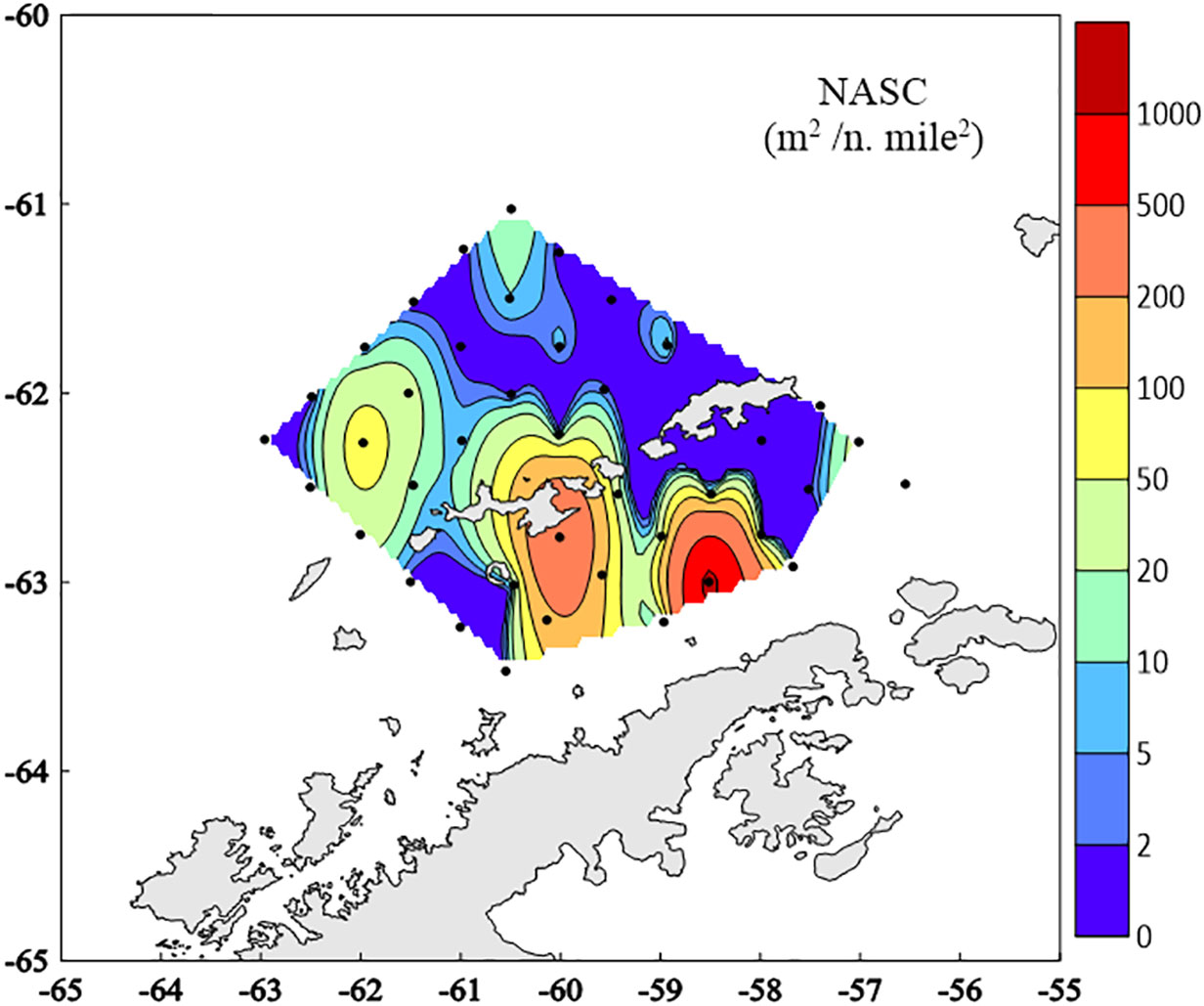
Figure 6 Krill acoustic density distribution around the South Shetland Islands during 8 to 12 March 2020. NASC- nautical area scattering coefficient.
SST was the best environmental variable to explain the variance in the study area (P=0.01). Acoustic density was analyzed with the abundance of phytoplankton communities and abundant species respectively. It was found that the acoustic density was the best factor to explain the pennate diatoms (P=0.03).
4 Discussion
4.1 Characteristics of net- phytoplankton community and its dynamics around the northern tip of the Antarctic Peninsula
The phytoplankton communities in the study area were mainly composed of the micro-diatoms in chains like Chaetoceros spp., Fragilariopsis spp., Pseudo−nitzschia spp. and Rhizosolenia spp. Diatom assemblages in Antarctic waters exist transitional characteristics. Their ecological types included eurythermic species, cold-water species and endemic species in Antarctic. The abundant species were mainly endemic species in Antarctic and cold-water species, which reflected the survival strategy of phytoplankton in the Southern Ocean. Firstly, due to larger cells are more resistant to sinking, they can stay in the euphotic layer (Sun et al., 2003). Secondly, large diatoms in chains with high ratio of superficial and volume of cells are conductive to the absorption of nutrients, especially to the absorption of limiting nutrients such as iron (Sun et al., 2003; Luan et al., 2012). Finally, some larger cells would be less susceptible to ingestion, while some cells have thick cell wall that make digestion difficult for zooplankton. In addition, we missed the pico- and some nanophytoplankton cells to some extent as we analyzed net-phytoplankton by the net sampling. These factors caused the net- phytoplankton communities to be dominated by larger cells or diatoms in chains (Sun et al., 2003).
In 1986-1987, dominant microphytoplankton were diatoms and the average cell density was 4.406×106 cells/m3 in the BS and adjacent waters of EI (Zhu, 1993). Cefarelli et al. (2011) found diatoms were dominated in the mixed layer (1.06×109–2.09×109cells/m3) and small centric diatoms were also highly abundant in the northwestern Weddell Sea between 10 March and 1 April 2009. Luan et al. (2012) used the same method we used to collect and analyze the phytoplankton community during austral summer 2010. Phytoplankton abundance varied from 387 to 1.04×107 cells/m3 which was similar to our results. Thalassiothrix antarctica, Gymnodinium sp., Chaetoceros sp., Pseudo-nitzschia lineola, Fragilariopsis kerguelensis, Chaetoceros criophilus, Corethron inerme and Fragilariopsis curta were the most abundant species. These species were also occurred in our results. Compared with previous studies above, there was little difference in composition of phytoplankton community. The dominant species or genus were similar to our study. Due to the difference in survey area, season and sampling methods, the phytoplankton abundance are varied. Water samples were collected for HPLC/CHEMTAX pigment and microscopic analysis around the tip of the AP during February/March 2008 and 2009. Phytoplankton assemblages were generally dominated by diatoms especially at coastal stations, while nanoflagellates replaced diatoms in open-ocean areas (Mendes et al., 2012). According to Mascioni et al. (2019), the highest phytoplankton abundance and biomass values were mainly represented by nanophytoflagellates, and the abundance of large bloom-forming diatoms was low in the relatively unexplored nearshore sites of the western AP during late summer of 2016 and during the spring-summer 2016-2017. As aforementioned, there are discrepancies among different results which might attribute to the conditions of sampling and methods of analyzing. So it seems to be necessary to have a long-term observation by same method at changeless location. Actually, rates of warming and sea ice loss are fastest in the southwest Atlantic sector with the impact of climate changes (Flores et al., 2012). Several studies have described a shift from large phytoplankton (diatoms) to smaller flagellated species (Moline et al., 2004; Monte-Hugo et al., 2009; Rozema et al., 2017b; Biggs et al., 2019). Therefore, long-term observation of the abundance of microphytoplankton is important to know more about size dynamics.
4.2 Relationships between environment features and net- phytoplankton community
As shown in the results, SST was the major environmental variable to explain the variance. In both laboratory (Eppley, 1972; Berges et al., 2002) and field investigations (Montagnes and Franklin, 2001; Hernando et al., 2018), temperature has been found to play an essential role in the growth of organisms, which can promote enzyme activity and metabolic processes. Higher temperature leads to accelerate phytoplankton growth and increase the matter accumulation (Winder and Sommer, 2012). With the increase of temperature, the biomass increased. In addition, Lionard et al. (2012) also found that high temperature was more favorable for the growth of large centric diatoms in phytoplankton assemblages in temperate environments. Therefore, SST might have promoted the growth of many net- phytoplankton taxa, which was highlighted by great contribution of microdiatoms.
The study area is hydrologically complex, with multiple water masses flowing from the Weddell Sea and the Bellingshausen Sea (Sangrà et al., 2011; Mendes et al., 2012). Within the surface layer of the BS, there are two major transitional waters being detected: Transitional Weddell Water (TWW) dominated by relatively cold and salty water mass flows north and west along the AP, and Transitional Bellingshausen Water (TBW) dominated by a relatively warm and fresh water mass, flows east (Gonçalves-Araujo et al., 2015). Thermohaline difference between TWW and TBW could be reflected in the phytoplankton communities. There is a well-mixed water column in the TWW where nanoplanktonic flagellates was dominant and exhibited lower chl a. On the contrary, microplanktonic diatoms were dominant and contributed higher chl a in the TBW because of the strong pycnocline and shallow upper mixed layers (Gonçalves-Araujo et al., 2015) within. In the west of the SSI, the high nutrients brought by Circumpolar Deep Water (CDW) and the deep water of subtropical Pacific Ocean accelerated the bloom of phytoplankton (Luan et al., 2012).
4.3 Grazing effect on phytoplankton by krill
Krill is a potential resource and their feeding behavior is complex, not only filtering phytoplankton and protozoa but also preying on zooplankton (Cleary et al., 2018). In addition, krill feed on algae and detritus from sea ice and seabed (Price et al., 1988; Stretch et al., 1988; Clarke and Tyler, 2008; Schmidt et al., 2014). During the period of phytoplankton bloom, krill mainly feed on diatoms. While in the scarcity phase of phytoplankton, they also choose flagellates, copepods and detritus to sustain life (Schmidt and Atkinson, 2016). Analysis of stomach contents (Meyer and El-Sayed, 1983) and studies of the comparison of krill and phytoplankton distribution (Schmidt and Atkinson, 2016) suggested that krill feeding selective.
Early studies about krill gut content established the suitable phytoplankton species for feeding (Schmidt and Atkinson, 2016). In the South Georgia, microphytoplankton was the predominant component of gut contents. Solitary and colonial cells of Nitzschia spp., Thalassiosira spp. and Fragilariopsis kerguelensis were the most abundant (Pakhomov et al., 1997). It was also found that Thalassiosira spp. are preferred by krill feeding and some small pennate diatoms such as Navicula spp. and Nitzschia spp. are barely fed (Opaliński et al., 1997). In the stomach contents study of Cleary et al. (2018), krill have a diatom-based diet, while the occasional presence of copepod suggests carnivorous supplemented diet. Compared with cryptophytes or prymnesiophytes, diatoms are recognized as high quality food for zooplankton (Ross et al., 2000). Indeed, diatom bloom and gonad development of krill occurs simultaneously in spring (Cuzin-Roudy and Labat, 1992; Schmidt et al., 2012) and the accumulation of polyunsaturated fatty acids of krill by feeding on diatoms was more effective than that by feeding on copepods (Schmidt et al., 2014). In addition, krill were more likely to feed on chain-forming diatoms than solitary phytoplankton species (Stuart, 1989; Haberman et al., 2003a). This result should be mainly concerned with the cell size. For cells with large size greater than 70 μm, krill were incapable of ingestion, while the size was favored by krill at 20-40 μm (Meyer and El-Sayed, 1983; Drits and Pasternak, 1993; Maciewska and Opalinski, 1993 and Opaliński et al., 1997).
Compared with phytoplankton distribution, there was a negative correlation between krill abundance and primary production during the survey in the South Georgia (Pakhomov et al., 1997). And the abundance of phytoplankton community dominated by diatoms rapidly decreased due to the feeding of the krill swarm only in a few hours according to the observation of the scientific cruise in the Scotia-Weddell Sea (Smetacek and Veth, 1989). However, striking differences were observed between the stomach contents of krill collected in fjords and in adjacent open waters which could not be explained by differences in the surface water phytoplankton (Cleary et al., 2018). These findings are inconclusive. The distribution of krill is concentrated along the AP, and their spatial and temporal distribution is highly variable due to the ability of krill swarms migration (Ross et al., 1996; Moline et al., 2004). Large krill swarms may contain up to 10000–30000 individuals m-3 (Hamner et al., 1983), and can rapidly clear phytoplankton up to a few litres per hour (Quetin et al., 1994). In fact, this phenomenon occurred mostly in local area. Spreading over larger areas, krill are difficult in grazing down phytoplankton (Atkinson et al., 2014). In the meanwhile, copepods were consistently part of krill diet (Schmidt et al., 2014). The distribution of zooplankton in waters also need to be considered, which may influence krill diet and increase the pressure on phytoplankton. In conclusion, it is hard to find the corresponding relationship between phytoplankton and krill density in the voyage survey even within the same region and season. This could explain why there was no correlation between phytoplankton abundance and krill density in our study.
Our research further analyzed the correlation between the distribution of pennate diatoms and the acoustic density of krill by BIO-ENV analysis. In the BS, krill density was high while the abundance of phytoplankton dominated by Corethron pennatum was low. In contrast, phytoplankton including pennate diatoms were abundant while the krill density was low in the west of SSI. Aforementioned findings were speculated that the phytoplankton assemblages were related to the krill grazing pressure. Biological processes, such as zooplankton grazing, superimposed to physical and chemical changes, can modify the abundance and dominance of different taxonomic assemblages (Cefarelli et al., 2011). Diatoms such as Thalassiosira spp., Fragilariopsis spp. and Chaetoceros spp. are feeding targets of krill, the low abundance of them and the dominance of Corethron pennatum in the BS may be the results of krill selective grazing, which seems to be the signal after krill grazing. Corethron pennatum may be the species krill refuses to eat. The structure of phytoplankton community is the result of consumption of higher trophic level including krill selective grazing. These conjectures may provide a new research direction for krill selective grazing and more evidence need to be explored.
5 Conclusion
In this study, we analyzed the structure and distribution of net-phytoplankton community near the SSI in the late austral summer 2019/2020. A total of 83 taxa (mostly at the species level) were recorded by light microscope, with diatoms being the most abundant group. There was significant difference between the BS and the west of the SSI. Combined with acoustic density of krill and environmental data including sea surface temperature and sea ice concentration, SST was the major environmental variable to explain the variance. It was also found that the acoustic density was the best factor to explain the pennate diatoms distribution. Our results clarified the composition and distribution of net-phytoplankton and provide some conjectures for selective feeding for krill. This study enhances the lack of up-to-date knowledge about the microphytoplankton community and give some conjectures about the selective feeding for krill and ecological implications for the Antarctic systems.
Data availability statement
The datasets generated for this study are available on request to the corresponding author.
Author contributions
LL conceived and led the study and writing of the manuscript. LQ, ZX and WX contributed substantially to writing the manuscript. ZJ collected the samples and provided data. ZY provided data. All authors read and approved the final manuscript.
Funding
The author(s) declare financial support was received for the research, authorship, and/or publication of this article. This work was funded by the Marine S&T Fund of Shandong Province for Qingdao Marine Science and Technology Center (No. 2022QNLM030002-1); Central Public-interest Scientific Institution Basal Research Fund, Yellow Sea Fisheries Research Institute, CAFS, China (No. 20603022022013, 20603022021017); Qingdao Postdoctoral Applied Research Project; Central Public-interest Scientific Institution Basal Research Fund, CAFS, China (No. 2023TD02); the National Natural Science Foundation of China (No.42006194).
Acknowledgments
We are grateful to the crew of the F/V Fu Rong Hai and the scientific observers onboard for helping with samples and data collection. We would also thank Professor Ruixiang Li for double checking the phytoplankton identification.
Conflict of interest
The authors declare that the research was conducted in the absence of any commercial or financial relationships that could be construed as a potential conflict of interest.
Publisher’s note
All claims expressed in this article are solely those of the authors and do not necessarily represent those of their affiliated organizations, or those of the publisher, the editors and the reviewers. Any product that may be evaluated in this article, or claim that may be made by its manufacturer, is not guaranteed or endorsed by the publisher.
References
Atkinson A., Hill S. H., Barange M., Pakhomov E. A., Raubenheimer D., Schmidt K., et al. (2014). Sardine cycles, krill declines and locust plagues: revisiting “wasp-waist” food webs. Trends. Ecol. Evol. 29, 309–316. doi: 10.1016/j.tree.2014.03.011
Atkinson A., Siegel V., Pakhomov E., Rothery P. (2004). Long-term decline in krill stock and increase in salps within the Southern Ocean. Nat. 432 (7013), 100–103. doi: 10.1038/nature02996
Berges J. A., Varela D. E., Harrison P. J. (2002). Effects of temperature on growth rate, composition and nitrogen metabolism in the marine diatom Thalassiosira pseudonana (Bacillariophyceae). Mar. Ecol. Prog. Ser. 225, 139–146. doi: 10.3354/meps225139
Bernard K. S., Steinberg D. K., Schofield O. M. E. (2012). Summertime grazing impact of the dominant macrozooplankton off the Western Antarctic Peninsula. Deep-Sea Res. Part I: Oceanogr. Res. Pap. 62, 111–122. doi: 10.1016/j.dsr.2011.12.015
Biggs T. E. G., Alvarez-Fernandez S., Evans C., Mojica K. D. A., Rozema P. D., Venables H. J., et al. (2019). Antarctic phytoplankton community composition and size structure: importance of ice type and temperature as regulatory factors. Pol. Biol. 42, 1997–2015. doi: 10.1007/s00300-019-02576-3
Cefarelli A. O., Vernet M., Ferrario M. E. (2011). Phytoplankton composition and abundance in relation to free-floating Antarctic icebergs. Deep. Res. Part II Top. Stud. Oceanogr. 58, 11–12. doi: 10.1016/j.dsr2.2010.11.023
Clarke A., Tyler P. A. (2008). Adult krill feeding at abyssal depths. Curr. Biol. 18, 282–285. doi: 10.1016/j.cub.2008.01.059
Cleary A. C., Durbin E. G., Casas M. C. (2018). Feeding by Antarctic krill Euphausia superba in the West Antarctic Peninsula: differences between fjord and open waters. Mar. Ecol. Prog. Ser. 595, 39–54. doi: 10.3354/meps12568
Costa R. P., Mendes C. R. B., Tavano V. M., Dotto T. S., Kerr R., Monteiro T., et al. (2020). Dynamics of an intense diatom bloom in the Northern Antarctic Peninsula, February 2016. Limnol. Oceanogr. 65, 2056–2075. doi: 10.1002/lno.11437
Cuzin-Roudy J., Labat J. P. (1992). Early summer distribution of Antarctic krill sexual development in the Scotia Weddell region: a multivariate approach. Polar Biol. 12, 65–74. doi: 10.1007/BF00239966
Drits A. V., Pasternak A. F. (1993). “Feeding of dominant species of the Antarctic herbivores zooplankton,” in Pelagic Ecosystems of the Southern Ocean. Ed. Voronina N. M. (Moscow: Nauka Press), 250–259.
Drits A. V., Semenova T. N. (1989). “Trophic characteristics of major planktonic phytophages from South Shetland Islands region during early spring,” in Complex Investigations of the Pelagic Zone of the Southern Ocean. Ed. Ponomareva L. A. (Moscow: Shirshov Institute Oceanology Publishers), 66–78.
Ducklow H. W., Baker K., Martinson D. G., Quetin L. B., Ross R. M., Smith R. C., et al. (2007). Marine pelagic ecosystems: the West Antarctic Peninsula. Phil. Trans. R. Soc B. 362, 67–94. doi: 10.1098/rstb.2006.1955
Eppley R. W. (1972). Temperature and phytoplankton growth in the sea. Fish Bull. Nat. Ocean Atmos. Adm. 70, 1063–1085.
Field J. G., Clarke K. R., Warwick R. M. (1982). A practical strategy for analysing multispecies distribution patterns. Mar. Ecol. Prog. Ser. 8, 37–52. doi: 10.3354/meps008037
Flores H., Atkinson A., Kawaguchi S., Krafft B. A., Milinevsky G., Nicol S., et al. (2012). Impact of climate change on Antarctic krill. Mar. Ecol. Prog. Ser. 458, 1–19. doi: 10.3354/meps09831
Froneman P. W., Pakhomov E. A., Perissinotto R., Laubscher R. K. (1997). Microphytoplankton assemblages in the waters surrounding South Georgia, Antarctica during austral summer 1994. Polar Bio. 17, 515–522. doi: 10.1007/s003000050150
Froneman P. W., Pakhomov E. A., Perissinotto R., McQuaid C. D. (2000). Zooplankton structure and grazing in the Atlantic sector of the Southern Ocean in late austral summer 1993. Part2. Biochemical zonation. Deep-Sea Res. Part I: Oceanogr. Res. Pap. 47, 1687–1702. doi: 10.1016/S0967-0637(99)00121-1
Gonçalves-Araujo R., de Souza M. S., Tavano V. M., Garcia C. A. E. (2015). Influence of oceanographic features on spatial and interannual variability of phytoplankton in the Bransfield Strait, Antarctica. J. Mar. Syst. 142, 1–15. doi: 10.1016/j.jmarsys.2014.09.007
Haberman K. L., Quetin L. B., Ross R. M. (2003a). Diet of the Antarctic krill (Euphausia superba Dana): I. Comparisons of grazing on Phaeocystis Antarctica (Karsten) and Thalassiosira Antarctica (Comber). J. Exp. Mar. Bio. Ecol. 283 (1-2), 79–95. doi: 10.1016/s0022-0981(02)00466-5
Haberman K. L., Ross R. M., Quetin L. B. (2003b). Diet of the antarctic krill (Euphausia Superba Dana): II. selective grazing in mixed phytoplankton assemblages. J. Exp. Mar. Biol. Ecol. 283 (1-2), 97–113. doi: 10.1016/S0022-0981(02)00467-7
Hamner W. M., Hamner P. P., Strand S. W., Gilmer R. W. (1983). Behavior of Antarctic krill, Euphausia superba – chemoreception, feeding, schooling and molting. Science 220, 433–435. doi: 10.1126/science.220.4595.433
Hernando M., Schloss I. R., Almandoz G. O., Malanga G., Varela D. E., De Troch M. (2018). Combined effects of temperature and salinity on fatty acid content and lipid damage in Antarctic phytoplankton. J. Exp. Mar. Biol. Ecol. 503, 120–128. doi: 10.1016/j.jembe.2018.03.004
Hewes C. D., Reiss C. S., Holm-Hansen O. (2009). A quantitative analysis of sources for summertime phytoplankton variability over 18 years in the South Shetland Islands (Antarctica) region. Deep-Sea Res. Part I: Oceanogr. Res. Pap. 56 (8), 1230–1241. doi: 10.1016/j.dsr.2009.01.010
Hewitt R. P., Watkins J., Naganobu M., Sushin V., Brierley A. S., Demer D., et al. (2004). Biomass of Antarctic krill in the Scotia Sea in January/February 2000 and its use in revising an estimate of precautionary yield. Deep. Res. Part II Top. Stud. Oceanogr. 51, 1215–1236. doi: 10.1016/j.dsr2.2004.06.011
Holm-Hansen O., Mitchell B. G. (1991). Spatial and temporal distribution of phytoplankton and primary production in the western Bransfield Strait region. Deep-Sea Res. Part I: Oceanogr. Res. Pap. 38, 961–980. doi: 10.1016/0198-0149(91)90092-T
Ishii H., Omori M., Murano M. (1985). Feeding behavior of the Antarctic krill E. superba Dana: I. Reaction to size and concentration of food particles. Trans. Tokyo Univ. Fish. 6, 117–124.
Kang S. H., Kang J. S., Lee S., Chung K. H., Kim D., Park M. G. (2001). Antarctic phytoplankton assemblages in the marginal ice zone of the northwestern Weddell Sea. J. Plankton Res. 21 (4), 150–175. doi: 10.1163/9789004276796_008
Krafft B. A., Macaulay G. J., Skaret G., Knutsen T., Bergstad O. A., Lowther A., et al. (2021). Standing stock of Antarctic krill (Euphausia superba Dana 1850) (Euphausiacea) in the Southwest Atlantic sector of the southern ocean 2018–19. J. Crustac. Biol. 41 (3), 1–17. doi: 10.1093/jcbiol/ruab046
Lancelot C., Mathot S., Veth C., de Baar H. (1993). Factors controlling phytoplankton ice-edge blooms in the marginal ice-zone of northwestern Weddell Sea during sea ice retreat 1988: field observations and mathematical modelling. Polar Biol. 13, 377–387. doi: 10.1007/BF01681979
Lionard M., Roy S., Tremblay-Létourneau M., Ferreyra G. A. (2012). Combined effects of increased UV-B and temperature on the pigment-determined marine phytoplankton community of the St. Lawrence Estuary. Mar. Ecol. Prog. Ser. 445, 219–234. doi: 10.3354/meps09484
Liu L., Fu M., Sun K., Xu Q., Xu Z., Zhang X., et al. (2021). The distribution of phytoplankton size and major influencing factors in the surface waters near the northern end of the Antarctic Peninsula. Acta Oceanol. Sin. 40 (6), 92–99. doi: 10.1007/s13131-020-1611-3
Luan Q., Sun J., Wu Q., Wang J. (2012). Phytoplankton community in adjoining water of the Antarctic Peninsula during austral summer 2010. Adv. Mar. Sci. 30 (4), 508–518.
Luan Q., Wang C., Wang X., Sun J., Niu M., Wang J. (2013). Microphytoplankton communities off the Antarctic Peninsula region in austral summer 2010/2011. Polish Polar. Res. 34 (4), 413–428. doi: 10.2478/popore–2013–0025
Maciewska K., Opalinski K. W. (1993). Spatial and temporal differentiation of food in Antarctic krill, Euphausia superba. Pol. Arch. Hydrobiol. 40, 291–311.
Mascioni M., Almandoz G. O., Cefarelli A. O., Cusick A., Ferrario M. E., Vernet M. (2019). Phytoplankton composition and bloom formation in unexplored nearshore waters of the western Antarctic Peninsula. Polar Biol. 42, 1859–1872. doi: 10.1007/s00300-019-02564-7
McClatchie S., Boyd C. M. (1983). Morphological study of sieve efficiencies and mandibular surfaces in the Antarctic krill, Euphausia superba. Can. J. Fish. Aquat. Sci. 40, 955–967. doi: 10.1139/f83-122
Mendes C. R. B., Souza M. S. D., Garcia V. M. T., Leal M. C., Brotas V., Garcia C. A. E. (2012). Dynamics of phytoplankton communities during late summer around the tip of the antarctic peninsula. Deep-Sea Res. Part I: Oceanogr. Res. Pap. 65 (Jul.), 1–14. doi: 10.1016/j.dsr.2012.03.002
Meyer M. A., El-Sayed S. Z. (1983). Grazing of Euphausia superba Dana on natural phytoplankton populations. Polar Biol. 1 (4), 193–197. doi: 10.1007/bf00443187
Moline M. A., Claustre H., Frazer T. K., Schofield O., Vernet M. (2004). Alteration of the food web along the Antarctic Peninsula in response to a regional warming trend. Global Change Biol. 10 (12), 1973–1980. doi: 10.1111/j.1365-2486.2004.00825.x
Montagnes D. J. S., Franklin D. J. (2001). Effect of temperature on diatom volume, growth rate, and carbon and nitrogen content: reconsidering some paradigms. Limnol. Oceanogr. 46, 2008–2018. doi: 10.2307/3069070
Monte-Hugo M., Doney S. C., Ducklow H. W., Fraser W., Martinson D., Stammerjohn S. E., et al. (2009). Recent changes in phytoplankton communities associated with rapid regional climate change along the western Antarctic Peninsula. Science 323 (5920), 1470–1473. doi: 10.1126/science.1164533
Opaliński K. W., Maciejewska K., Georgieva L. V. (1997). Notes of food selection in the Antarctic krill, Euphausia superba. Polar Biol. 17 (4), 350–357. doi: 10.1007/pl00013376
Pakhomov E. A., Perissinotto R., Froneman P. W., Miller D. G. M. (1997). Energetics and feeding dynamics of Euphausia Superba in the South Georgia region during the summer of 1994. J. Plankton Res. 4, 399–423. doi: 10.1093/plankt/19.4.399
Prézelin B. B., Hofmann E. E., Mengelt C., Klinck J. M. (2000). The linkage between Upper Circumpolar Deep Water (UCDW) and phytoplankton assemblages on the west Antarctic Peninsula continental shelf. J. Mar. Res. 58, 165–202. doi: 10.1357/002224000321511133
Price H. J., Boyd K. R., Boyd C. M. (1988). Omnivorous feeding behavior of the Antarctic krill Euphausia superba. Mar. Biol. 97, 67–77. doi: 10.1007/BF00391246
Quetin L. B., Ross R. M., Clarke A. (1994). “Krill energetics: seasonal and environmental aspects of the physiology of Euphausia superba,” in (ed) Southern Ocean ecology: the BIOMASS perspective. Ed. El-Sayed S. Z. (Cambridge: Cambridge University Press), 165–184.
Ross R. M., Quetin L. B., Baker K. S., Vernet M., Smoth R. C. (2000). Growth limitation in young Euphausia superba under field conditions. Limnol. Oceanogr. 45, 31–43. doi: 10.4319/lo.2000.45.1.0031
Ross R. M., Quetin L. B., Lascara C. M. (1996). “Distribution of Antarctic krill and dominant zooplankton west of the Antarctic Peninsula,” in Foundations for Ecosystem Research in the Western Antarctic Peninsula Region. Eds. Ross R., Hofmann E., Quetin L. (Washington, DC: Antarctic Research Series, American Geophysical Union), 199–217.
Rozema P. D., Biggs T., Sprong P. A. A., Buma A. G. J., Venables H. J., Evans C., et al. (2017a). Summer microbial community composition governed by upper-ocean stratification and nutrient availability in northern Marguerite Bay, Antarctica. Deep. Res. Part II Top. Stud. Oceanogr. 139, 151–166. doi: 10.1016/j.dsr2.2016.11.016
Rozema P. D., Venables H. J., van de Poll W. H., Clarke A., Meredith M. P., Buma A. G. J. (2017b). Interannual variability in phytoplankton biomass and species composition in northern Marguerite Bay (West Antarctic Peninsula) is governed by both winter sea ice cover and summer stratification. Limnol. Oceanogr. 62, 235–252. doi: 10.1002/lno.10391|
Sangrà P., Gordo C., Hernández-Arencibia M., Marrero-Díaz A., Rodríguez-Santana A., Stegner A., et al. (2011). The Bransfield current system. Deep-Sea Res. Part I: Oceanogr. Res. Pap. 58, 390–402. doi: 10.1016/j.dsr.2011.01.011
Schloss I., Estrada M. (1994). Phytoplankton composition in the Weddell-Scotia Confluence area during austral spring in relation to hydrography. Pol. Biol. 14 (2), 77–90. doi: 10.1007/bf00234969
Schmidt K., Atkinson A. (2016). “Feeding and food processing in antarctic krill (Euphausia superba dana),” in Biology and Ecology of Antarctic Krill, Advances in Polar Ecology. Ed. Siegel V., (Switzerland: Springer International Publishing) 175–224. doi: 10.1007/978-3-319-29279-3_5
Schmidt K., Atkinson A., Pond D. W., Ireland L. C. (2014). Feeding and overwintering of Antarctic krill across its major habitats: the role of sea ice cover, water depth, and phytoplankton abundance. Limnol. Oceanogr. 59, 17–36. doi: 10.4319/lo.2014.59.1.0017
Schmidt K., Atkinson A., Venables H. J., Pond D. W. (2012). Early spawning of Antarctic krill in the Scotia Sea is fuelled by “superfluous feeding” on non-ice-associated phytoplankton blooms. Deep. Res. Part II Top. Stud. Oceanogr. 59 (60), 159–172. doi: 10.1016/j.dsr2.2011.05.002
Shannon C. E., Weaver W. (1949). The mathematical theory of communication (Urbana: University of Illinois Press), 1–117.
Shi Y., Wang J., Zuo T., Shan X., Jin X., Sun J., et al. (2020). Seasonal changes in zooplankton community structure and distribution pattern in the Yellow Sea, China. Front. Mar. Sci. 7. doi: 10.3389/fmars.2020.00391
Smetacek V., Veth C. (1989). “Introduction,” in The expedition Antarktis VII/3 (EPOS Leg 2) of RV “Polarstern” in 1988/89, vol. 65 . Eds. Hempel I., Schalk P. H., Smetacek V. (Bremerhaven: Berichte zur Polar for schung, Alfred-Wegener-Institut f€ur Polar- und Meeresforschung), 1–7.
Stretch J. J., Hamner P. P., Hamner W. M., Michel W. C., Cook J., Sullivan C. W. (1988). Foraging behaviour of Antarctic krill Euphausia superba on sea ice microalgae. Mar. Ecol. Prog. Ser. 44, 131–139. doi: 10.3354/meps044131
Stuart V. (1989). Observations on the feeding of Euphausia lucens on natural phytoplankton suspensions in the southern Benguela upwelling region. Cont. Shelf Res. 9 (11), 1017–1028. doi: 10.1016/0278-4343(89)90005-8
Sun J., Liu D. Y., Ning X. R., Liu C. G. (2003). Phytoplankton in the Prydz Bay and the adjacent Indian sector of the Southern Ocean during the austral summer 2001/2002. Oceanol. Limnol. Sinica. 34 (5), 519–532.
Vernet M., Martinson D., Iannuzzi R., Stammerjohn S., Kozlowski W., Sines K., et al. (2008). Primary production within the sea-ice zone west of the Antarctic Peninsula: I. Sea ice, summer mixed layer, and irradiance. Deep. Res. Part II Top. Stud. Oceanogr. 55 (18–19), 2068–2085. doi: 10.1016/j.dsr2.2008.05.021
Watters G. M., Hinke J. T., Reiss C. S. (2020). Long-term observations from Antarctica demonstrate that mismatched scales of fisheries management and predator-prey interaction lead to erroneous conclusions about precaution. Sci. Rep. 10 (1), 2314. doi: 10.1038/s41598-020-59223-9
Winder M., Sommer U. (2012). Phytoplankton response to a changing climate. Hydrobiology> 698, 5–16. doi: 10.1007/s10750-012-1149-2
Keywords: phytoplankton, community structure, sea surface temperature, sea ice concentration, Antarctic krill
Citation: Liu L, Zhang J, Zhao Y, Luan Q, Zhao X and Wang X (2023) Net-phytoplankton communities and influencing factors in the Antarctic Peninsula region in the late austral summer 2019/2020. Front. Mar. Sci. 10:1254043. doi: 10.3389/fmars.2023.1254043
Received: 06 July 2023; Accepted: 25 October 2023;
Published: 13 November 2023.
Edited by:
Letterio Guglielmo, Anton Dohrn Zoological Station Naples, ItalyReviewed by:
Martina Mascioni, National University of La Plata, ArgentinaMárcio Silva de Souza, Federal University of Rio Grande, Brazil
Copyright © 2023 Liu, Zhang, Zhao, Luan, Zhao and Wang. This is an open-access article distributed under the terms of the Creative Commons Attribution License (CC BY). The use, distribution or reproduction in other forums is permitted, provided the original author(s) and the copyright owner(s) are credited and that the original publication in this journal is cited, in accordance with accepted academic practice. No use, distribution or reproduction is permitted which does not comply with these terms.
*Correspondence: Xinliang Wang, d2FuZ3hsQHlzZnJpLmFjLmNu