- 1Scripps Institution of Oceanography, Center for Marine Biodiversity and Conservation, University of California, San Diego (UCSD), La Jolla, CA, United States
- 2National Oceanic and Atmospheric Administration (NOAA) Office of Ocean Exploration and Research, Silver Spring, MD, United States
- 3Scripps Institution of Oceanography, Coastal Observing Research and Development Center, University of California, San Diego (UCSD), La Jolla, CA, United States
- 4Antarctic Ecosystem Research Division, National Oceanic and Atmospheric Administration (NOAA) Southwest Fisheries Science Center, La Jolla, CA, United States
The North Atlantic Ocean features high seasonal productivity of the brown seaweed Sargassum, which floats on the ocean surface and accumulates in large numbers in the Sargasso Sea. Sargassum blooms can stretch from the west coast of Africa to the Gulf of Mexico, and have created the largest seaweed blooms ever observed. Sargassum blooms have increased in intensity in recent years, and can negatively impact coastal communities when they wash up onshore in large quantities and decay. While seaweed sinking from surface waters to the seafloor may be an important carbon sink by removing carbon from the atmosphere, the magnitude of carbon sequestration by Sargassum and other macroalgae remains poorly understood. Given the magnitude of Sargassum blooms in the North Atlantic, they may pose a significant mechanism for carbon sequestration in the deep sea, though direct observations are rare. In this study, we documented the presence and distribution of Sargassum seaweed on the seafloor using video from ten remotely operated vehicle dives conducted on NOAA Ship Okeanos Explorer. Locations included sites in the Gulf of Mexico, in the Caribbean Sea, and off the Southeastern United States. Sargassum was observed in numbers ranging from 0 to over 112 per dive, and a frequency of between 0-11.23 observations for every 100 meters of horizontal distance. These observations suggest that Sargassum does make its way to the deep sea in potentially significant amounts. Natural systems like Sargassum sinking could serve as natural laboratories for understanding and managing seaweed burial as a climate mitigation strategy. Long-term monitoring of the fate of sunken Sargassum on the seabed is needed in order to determine how much is ultimately sequestered rather than recycled back into the system. Such observations would inform the feasibility of Sargassum farming and/or facilitated sinking as potential carbon dioxide removal strategies.
1 Introduction
Macroalgae (commonly referred to as seaweed) provide many important ecological, environmental, and economic services. Seaweed is important food and habitat to a range of animals, including commercially-important fisheries species. Seaweed has market value for direct human consumption, and compounds derived from seaweed are commonly used in a range of commercial products like cosmetics, supplements, and fertilizers (Sugumaran et al., 2022). Through photosynthesis, algae fix carbon dioxide and can ultimately sequester organic carbon in coastal sediments and in the deep ocean for hundreds to thousands of years (Raven, 2017). Protection and restoration of macroalgae habitats can help preserve these blue carbon ecosystems. Seaweed cultivation and sinking is being proposed as a possible strategy to reduce atmospheric carbon dioxide (Duarte et al., 2017; National Academies of Sciences, Engineering, and Medicine, 2022). Seaweeds are also being studied for potential use as a biofuel (Godvin et al., 2021). However, when they occur in excess, seaweeds can outcompete, smother, or block sunlight from reaching other photosynthetic organisms and can wash ashore, accumulating in noxious heaps (Rodríguez-Martínez et al., 2019).
Sargassum is a genus of brown seaweed that is widespread in temperate, subtropical, and tropical waters. Sargassum morphology includes a holdfast, a cylindrical main axis, small leaflike blades, and gas-filled bladders, or pneumatocysts (Graham et al., 2009). A flexible body helps it withstand strong currents. There are two species of Sargassum that occur in the Atlantic Ocean off the coast of the United States and in the Gulf of Mexico: Sargassum fluitans and Sargassum natans. These forms are unique in that they are free-floating and do not have a holdfast or attach to a substrate at any stage in their life cycle. They are the only species of Sargassum that are holopelagic, meaning that they remain pelagic drifters throughout their entire life cycle, and free-floating Sargassum is only found in the Atlantic Ocean (Doyle and Franks, 2015; Stiger-Pouvreau et al., 2023). These pelagic species of Sargassum can double in size every 9-13 days (Hanisak and Samuel, 1987).
The two free-floating Sargassum species can occur in extensive, highly productive rafts on the ocean’s surface that harbor distinctive communities of organisms adapted to the buoyant Sargassum habitat, including juvenile fish species such as amberjack and triggerfish (Wells and Rooker, 2004). Sargassum natans and Sargassum fluitans only reproduce asexually through fragmentation, a type of vegetative asexual reproduction where an individual Sargassum breaks into two or more parts, each of which continues to live and grow (Lee, 2008). Sargassum’s fast growth rate contributes to its rapid spread. Hereafter in this paper both Sargassum fluitans and Sargassum natans will be referred to as “Sargassum”.
1.1 Sargassum blooms
The North Atlantic Gyre is a circular system of ocean currents in the Atlantic Ocean that, through the rotating pattern of currents and effects of wind and weather, amasses Sargassum in an area known as the Sargasso Sea. The Sargasso Sea is the only sea bounded by currents rather than by land (NOAA, 2019). The Gulf Stream acts as a conduit that transports Sargassum through the Caribbean, into the Gulf of Mexico, and off the coast of the southeastern United States, and evidence suggests that much of the Sargassum transported through the Gulf of Mexico originates from the North Equatorial Recirculation Region, a complex system of currents around western Africa and South America, in addition to the Sargasso Sea (Franks et al., 2016).
Since 2011, giant floating Sargassum mats in the Atlantic have increased in density and range to form an 8,850 kilometer-long belt, called the Great Atlantic Sargassum Belt, that can extend from West Africa to the Gulf of Mexico (Wang et al., 2019). High quantities of Sargassum have washed up on beaches and inundated coastlines in the Southeast U.S., Gulf of Mexico, and the Caribbean in recent years, presenting a logistical challenge for coastal communities to manage the tons of seaweed piling up on their shores (Lamb, 2018; Conley and Oliver, 2019). Satellite measurements suggest that Sargassum blooms start to develop in the Central Western Atlantic in February-March and are transported by winds and currents to the Caribbean as massive blooms from May-August (Wang and Hu, 2017). A changing climate may have unexpected impacts on Sargassum productivity and sinking as well as its tendency to be transported, aggregated, and scattered due to climate influences on algal productivity, weather patterns, and ocean currents (Sanchez-Rubio et al., 2018).
There have been recent efforts to quantify the amount of Sargassum on the sea surface through methods such as satellite imagery analysis (Wang and Hu, 2016), numerical models (Schamberger et al., 2022), and field measurements (Ody et al., 2019). A 2019 study that analyzed satellite remote sensing data from 2000 to 2018 found a significant increase in estimated Sargassum biomass in the Great Atlantic Sargassum Belt beginning in 2011, with the highest biomass estimated at more than 20 million metric tons in June 2018, the last year of the study (Wang et al., 2019). Given the economic impacts of these Sargassum blooms, new monitoring systems (Duffy et al., 2019; Valentini and Balouin, 2020) have been developed to better plan for and respond to Sargassum accumulation on coasts.
1.2 Sargassum ecology
Sargassum is abundant in the Atlantic Ocean and forms an essential surface habitat that supports a diversity of marine organisms, including fish, invertebrates, sea turtles, marine birds, and marine mammals. Floating Sargassum mats serve as a primary nursery area for many fish species, some of which are commercially important (dolphinfishes, jacks, and amberjacks), and they provide a source of energy in an otherwise nutrient-poor area of the Atlantic (Casazza and Ross, 2010). These Sargassum mats provide essential habitat for approximately 120 species of fish and more than 120 species of invertebrates (Doyle and Franks, 2015).
Carbon sequestration is the process of storing carbon dioxide and other forms of carbon out of the atmosphere for long periods of time. It has been suggested that marine primary producers such as phytoplankton, macroalgae, mangroves, and seagrasses are more efficient at sequestering carbon than their terrestrial counterparts due to their high productivity and efficiency in trapping sediments and associated organic carbon (Mcleod et al., 2011; Arenas and Vaz-Pinto, 2015). Through photosynthesis, Sargassum converts sunlight, carbon dioxide, and ocean nutrients into sugars and other carbon compounds. This organic material, and the carbon it contains, can then end on a number of different pathways, such as washing up on beaches, being eaten by herbivores, or sinking to the bottom of the ocean. Once it ends up in deep ocean currents or seafloor sediments hundreds of meters below the surface, the carbon is prevented from being exchanged with the atmosphere over several hundred to several thousand years (Volk and Hoffert, 2013). Traditionally, seagrasses and mangroves have been considered the dominant form of oceanic carbon sequestration (Duarte and Cebrián, 1996); however, in recent years researchers have been looking at seaweeds like Sargassum’s role as important carbon sinks (N’Yeurt et al., 2012; Raven, 2017; Kokubu et al., 2019).
The gravitational sinking of Sargassum from the surface to the seafloor, and degradation of Sargassum within the water column and at the seafloor, are poorly understood processes. The mechanisms that deliver drifting seaweed to marine sediments include wind-induced Langmuir circulation that can transport floating seaweed fragments to a depth where pressure collapses its air bladders, rendering the seaweed negatively buoyant and removing it from the surface (Krause-Jensen and Duarte, 2016). Additionally, Sargassum living in the shaded understory of floating Sargassum may be prevented from photosynthesizing and lose buoyancy and die and start to degrade. It will also lose buoyancy with age and from encrustation of epizoans (Stoner, 1983). Once it achieves negative buoyancy, the Sargassum sinks at an estimated rate of 3.5 cm per second (Johnson and Richardson, 1977). At this rate, Sargassum would reach the seafloor at 1000 meters in just 8 hours. This is relatively fast for sinking particles and does not allow much time for remineralization, consumption, or degradation (Giering et al., 2020; Omand et al., 2020).
Sargassum sinking has been studied before using Autonomous Underwater Vehicle (AUV)-collected seafloor photographs in the southern part of the North Atlantic ocean (three stations between ~10-12° N and ~36-50° W), within the North Atlantic Subtropical Gyre where the Sargasso Sea is located. The biomass density of Sargassum deposited on the seafloor was estimated at 0.07 to 3.75 g/m2, which is greater than the surface biomass as estimated through literature review at 0.024-0.84 g/m2 (Baker et al., 2017). Krause-Jensen and Duarte (2016) estimated that 11 percent of macroalgal particulate organic carbon (POC) export, or 35 TgC/yr, reaches the deep sea globally, serving as an important carbon sink. Start-up companies and financial investors have noted the potential for macroalgae aquaculture for commercial products like bioplastics and food or to sink and bury on the deep seafloor to sequester carbon from the atmosphere (López Miranda et al., 2021; Oxenford et al., 2021). Sargassum in particular has been identified as a target species for such efforts because of its naturally high abundance and reproductive rate.
The factors that contribute to organic carbon export in the open ocean and its subsequent carbon sequestration are driven by a complex combination of ecological, biogeochemical, and physical oceanographic processes. Developing a predictive understanding of carbon export pathways for seaweeds including Sargassum is critical for understanding present and future rates of ocean carbon sequestration and informing the emerging marine carbon dioxide removal industry. This study provides an initial characterization of Sargassum on the seafloor by describing the number of Sargassum observed, its relationship to benthic composition, any interactions with benthic organisms, and geological features of the dive sites explored. We revisited a series of archived deep ocean exploration visual surveys that took place on seabed areas underlying known and likely Sargassum surface blooms as one step toward understanding the fate of sinking Sargassum in the marine system.
2 Methods
2.1 NOAA Ship Okeanos Explorer
The National Oceanic and Atmospheric Administration (NOAA)’s Office of Ocean Exploration and Research is a U.S. federal program dedicated to exploring the unknown ocean for public benefit, filling in knowledge gaps about the marine environment through scientific discovery, technological advancements, and access to data. It conducts interdisciplinary ocean exploration expeditions on NOAA Ship Okeanos Explorer and other research vessels. Okeanos Explorer is a 68-meter research ship outfitted with an array of mapping sonars and a dual body Remotely Operated Vehicle (ROV) system: ROV Deep Discoverer (D2) and ROV Seirios. A unique and advantageous aspect of Okeanos Explorer operations is its ability to facilitate real-time communication and collaboration with shore-based scientists (Peters et al., 2019). Scientists on the ship and on land collaborate through a live video feed, text chatroom, shared conference phone line, and video annotation system, allowing participants not only to follow along live but to provide feedback and scientific expertise in real-time during the ROV dives (Kennedy et al., 2016; Selig et al., 2019).
2.2 Deep Discoverer and Seirios ROVs
Kennedy et al. (2019) describe the technical details on the Okeanos Explorer’s seafloor mapping and ROV systems and operations. All ROV dives examined in this study were conducted with NOAA’s dual body ROV system Deep Discover (D2) and Seirios, although only video from D2 was reviewed. The main capability of D2 is the ability to capture high-definition video, with its primary camera able to zoom in and provide close-up video of relatively small organisms (Kennedy et al., 2019). Seirios is directly tethered to the Okeanos Explorer via a fiber-optic cable and is also tethered to D2, a configuration that allows Seirios to absorb the sway of the ship while keeping D2 stable. The cable provides the ROVs with power and allows for data transfer between the ROVs and the ship. Both Seirios and D2 are equipped with a suite of sensors to measure environmental parameters like temperature, dissolved oxygen, salinity, and depth. Surveys conducted with this two-body system are exploratory in nature, providing baseline information on the broader biological, geological, and physical context of poorly explored regions of the ocean (Selig et al., 2019; Cantwell et al., 2020). Because the ROV time is maximized for a variety of objectives, true quantitative analyses of the imagery are not always possible, yet important insights may still be gleaned.
2.3 ASPIRE campaign
From 2018-2022, Okeanos Explorer conducted a series of expeditions as part of the Atlantic Seafloor Partnership for Integrated Research and Exploration (ASPIRE), a multi-national collaborative ocean exploration campaign to raise the collective knowledge and understanding of the North Atlantic Ocean. Three of the four expeditions reviewed – Océano Profundo 2018: Exploring Deep-sea Habitats off Puerto Rico and the U.S. Virgin Islands (EX1811), Windows to the Deep 2019: Southeast and Mid-Atlantic U.S Continental Margin, Port Canaveral, FL to Norfolk, VA (EX1903L2), and 2019 Southeastern U.S. Deep-sea Exploration (EX1907) – were part of this ASPIRE campaign.
2.4 Site selection
A subset of expeditions and ROV dive sites were selected for this study based on the following considerations:
● Expedition took place in region with known seasonal occurrences of Sargassum (Wang and Hu, 2017).
● “Sargassum” or “seaweed” was noted in SeaTube annotations at some point during the dive.
● High algal density on the sea surface was observed through the University of South Florida’s Satellite-based Sargassum Watch System (SaWS) (Trinanes et al., 2023) - this informed selection of EX1811 Dives 7 and 8 and EX1907 Dives 11 and 12.
● Dives were selected to represent different types of bathymetric and ecological features (for instance a canyon versus coral mounds) and depths to sample a variety of site characterizations.
Details on bathymetric and ecological features are available in Supplementary Information Table 1.
2.5 ROV video footage review, Sargassum annotation, and site characterization
Okeanos Explorer ROV video footage is streamed live through SeaTube V3, a platform developed by Ocean Networks Canada (ONC) for scientists to view and annotate ROV footage both in real time and afterwards (Selig et al., 2019). SeaTube V3 provides open access and archives of dive videos, dive imagery, dive logs, navigational data, and metadata for deep ocean exploration (Jenkyns et al., 2013). An informal science chatroom developed by the Global Foundation for Ocean Exploration is also used for sidebar conversations between scientists and captures some of the annotations as well; the messages of the chat are then archived for future access1,2.
Annotations of Sargassum were done manually, by reviewing the selected footage from the Deep Discoverer ROV. Each of the dives selected for review was viewed in SeaTube V3 from the start of the ROV’s descent from the surface to the end of its ascent back to the surface to account for the possibility of Sargassum being observed in the water column. An object was identified as Sargassum based on its shape, color, texture, and movement. Figure 1 shows screen grabs of the ROV video to show the variability of what the Sargassum looks like when it reaches the seafloor.
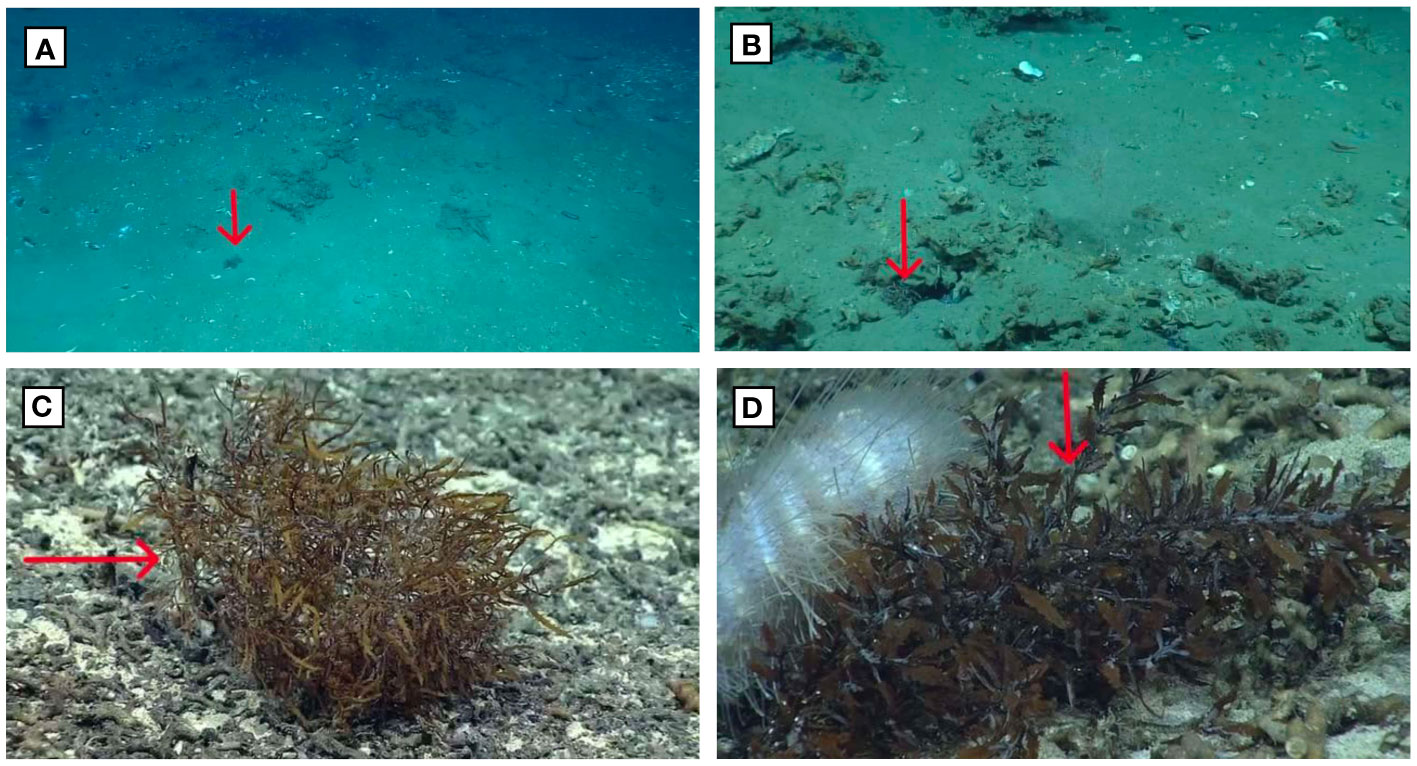
Figure 1 Images annotated as: “Likely Sargassum” (A) and “Sargassum” (B) from EX1803 Dive 6. Close-up photo of Sargassum (C) and an urchin appearing to graze on Sargassum (D) from EX1903 L2 Dive 2.
When there was an object that was potentially Sargassum but identification was questionable, higher-resolution footage of that part of the dive was accessed and viewed in NOAA’s Ocean Exploration Video Portal3. If after reviewing the high-resolution footage the object could not with absolute certainty be identified as Sargassum, the annotation included the note “likely Sargassum” to indicate a degree of uncertainty. Annotations with “likely Sargassum” designation were counted as Sargassum in this report. All other observations were identified to the Sargassum genus referencing the World Register of Marine Species (WoRMS) system of classification and annotated in SeaTube V3.
For dives with abundant Sargassum, a new Sargassum annotation was made for every new frame of view that contained Sargassum. For instance, even if there were five clumps of Sargassum in the frame, this was counted as one Sargassum annotation. As soon as the ROV had Sargassum in its frame of view that was not clearly visible in the previous annotation, this was entered as a new annotation. Each Sargassum annotation was classified as “low,” “medium,” or “high” biomass based on visual estimation of the approximate amount of Sargassum within the frame of view on a scale from 1-8 (Supplementary Information Table 2). Additionally, when mobile animals were observed on or in the near vicinity of the Sargassum detritus, this was noted, as well as whether the animal appeared to be grazing on the Sargassum. Bathymetric features (rocks, sediment, etc.) and other observations (high marine snow, presence of anthropogenic debris) were also noted in Supplementary Information Table 2.
In addition to reviewing the ROV footage and accompanying audio commentary, the archived chatroom logs that include expert perspectives, and post-dive summaries, accessed through NOAA’s Institutional Repository4, were reviewed to help characterize each dive site.
Distance transited along the seafloor was calculated by importing spatial coordinates from the ROV recorded while at depth and plotting them in ESRI’s ArcGIS Pro 2.9 software. A line was then generalized based on the points and the length in meters was calculated for each dive. Distance transited varied from approximately 250 meters to over 1,000 meters per dive.
3 Results
Video from a total of 10 NOAA Ship Okeanos Explorer ROV dive sites across four expeditions was reviewed for this study, totaling 55 hours, 54 minutes of time on bottom (Table 1). A total of approximately 7,350 linear meters were traveled horizontally. Sargassum was observed at 9 of the 10 dive sites, with a total of 237 instances of Sargassum annotated and between 0 to over 112 Sargassum observed per dive. Although we reviewed video from descent to ascent, no Sargassum was observed in the water column. Figure 2 shows location and Sargassum observations for all ROV dives included in the study.
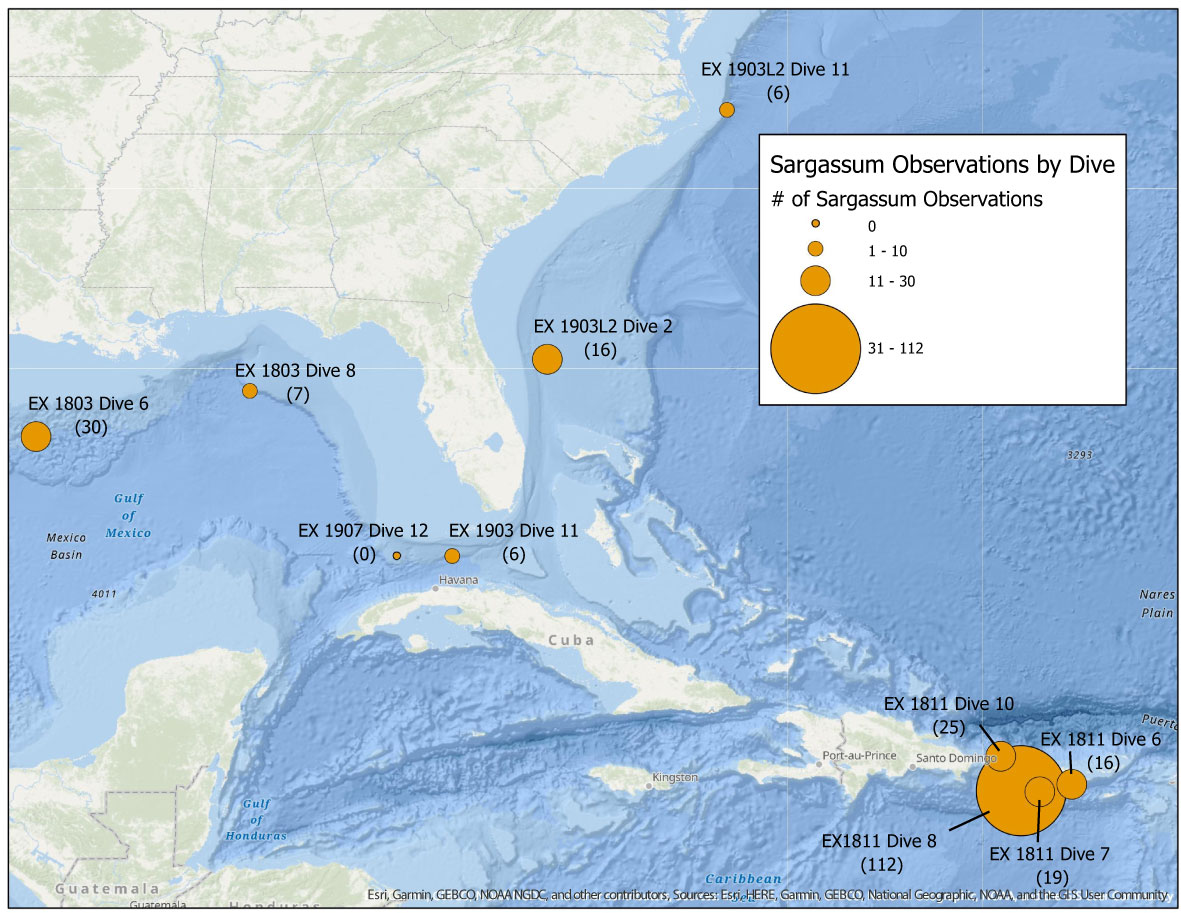
Figure 2 Location of ROV dive sites that were included in this study. The size of the circles indicates the range of the number of Sargassum observations. The number of Sargassum observations are shown in parentheses. (Gulf of Mexico 2018 (EX1803) - Dive 6: Hidalgo Basin and Dive 8: DeSoto Canyon, Océano Profundo 2018: Exploring Deep-sea Habitats off Puerto Rico and the U.S. Virgin Islands (EX1811) - Dive 6: Punta Yeguas, Puerto Rico, Dive 7: Caja de Muertos Island, Puerto Rico, Dive 8: South of La Parguera, Puerto Rico, and Dive 10: Mona Canyon, Puerto Rico, Windows to the Deep 2019: Southeast and Mid-Atlantic U.S Continental Margin, Port Canaveral, FL to Norfolk, VA (EX1903L2) - Dive 2: Stetson Mesa South Mounds, Eastern Florida and Dive 11: “Dodge” Canyon, North Carolina, 2019 Southeastern U.S. Deep-sea Exploration (EX1907) - Dive 11: Key West Deep and Dive 12: “Berg Bits”, Southwestern Florida).
3.1 Dive site characterizations
3.1.1 Gulf of Mexico 2018 (EX1803), April 11 - May 3, 2018 Pascagoula, Mississipi to Key West, Florida
Gulf of Mexico 2018 was a 23-day expedition in the Gulf of Mexico Basin to identify, map, and explore the diversity and distribution of deep-sea habitats in the region. The 15 ROV dives of this expedition, which ranged from 305 to 3,010 meters in bottom depth, focused on benthic habitats, including fish habitats, deep-sea coral and sponge communities, chemosynthetic communities (brine pools, gas seeps, mud volcanoes), and biological communities around shipwrecks (Maxon et al., 2018).
3.1.1.1 Hidalgo Basin (Dive 6)
This dive targeted Hidalgo Basin (Figure 3A), an area that was being considered for expansion of the Flower Garden Banks National Marine Sanctuary but was not ultimately included in the expansion5. Specifically, this dive explored a mound feature for hard-bottom communities, particularly deep-sea corals, sponges, and associated fauna. Approximately 250 kilometers south of central Louisiana, this is a relatively unexplored area that was first observed in 2014 by Okeanos Explorer. A high abundance of bivalve shells and carbonate rocks were seen on this dive. Anthropogenic debris from the surface – fishing line, canvas, metal container – was observed at this site. Parts of this site had signs of past seepage, including bacterial mats and a high abundance of bivalves, mostly empty shells but some living. A gastropod appeared to be grazing on Sargassum. The seafloor was heavily sedimented, with large depressions and pockmarks. There was a slight current on the bottom with high turbidity in the water column. This dive transited 932 linear meters along the seafloor. Thirty Sargassum observations were made over this distance, for an average 3.22 Sargassum observations per 100 meters (Table 1). The average depth for Sargassum observations was 1,077 meters.
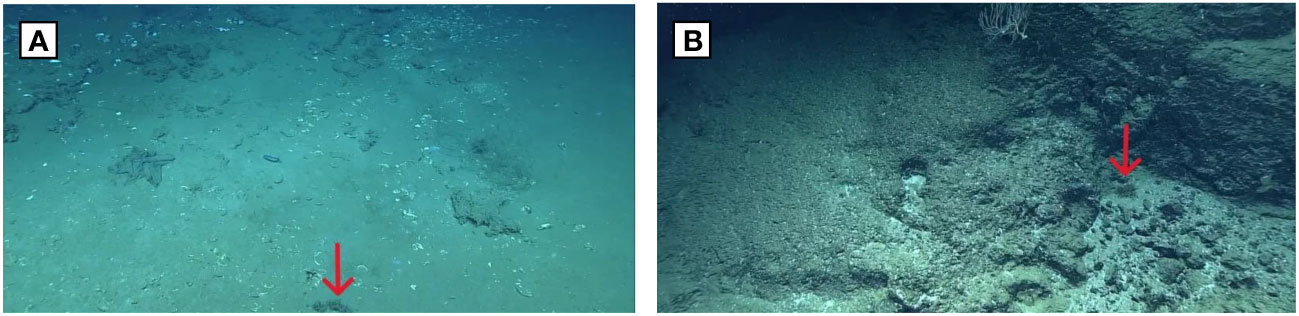
Figure 3 Dive sites reviewed from Gulf of Mexico 2018 (EX1803). (A) Hidalgo Basin (Dive6) (B) DeSoto Canyon (Dive 8).
3.1.1.2 DeSoto Canyon (Dive 8)
This dive targeted the northern end of the West Florida Escarpment in the DeSoto Canyon region (Figure 3B). At the time of the expedition this area was being considered for expansion of the Flower Garden Banks National Marine Sanctuary but was not ultimately included in the expansion. Dive 8 explored the escarpment feature at depths between 2,200-2,600 meters for hard-bottom communities, particularly deep-sea corals, sponges, and associated fauna. There have been five previous scientific ROV dives in this general area, all of which documented extensive and diverse deep-sea coral communities, which are the deepest high-density communities known in the Gulf of Mexico (McLetchie et al., 2018). Approximately 220 kilometers off Alabama, this site was characterized by a hard substrate. It also featured a steep carbonate rock wall with highly fractured detached boulders at the base of the wall. Terrace features with relatively gentle slopes had a blanket of sediment cover. This dive transited 469 linear meters along the seafloor. Seven Sargassum observations were made over this distance, for an average 1.49 Sargassum observations per 100 meters (Table 1). The average depth for Sargassum observations was 2,534 meters.
3.1.2 Océano Profundo 2018: Exploring deep-sea habitats off Puerto Rico and the U.S. Virgin Islands (EX1811), October 30 - November 20, 2018 San Juan, Puerto Rico to San Juan, Puerto Rico
Océano Profundo 2018 was a 22-day expedition to explore the unknown and poorly understood deep-water areas surrounding Puerto Rico and the U.S. Virgin Islands. The 19 ROV dives of this expedition, which ranged from 250 to 5,000 meters in depth, surveyed a diversity of habitats and geological features, including midwater habitats, deep-sea coral and sponge communities, deep-sea fish habitats, submarine canyons, and submarine landslides. In combination with deep-sea mapping operations, information and data were collected to increase understanding of deep-sea ecosystems of this poorly studied area, as well as to provide publicly-accessible data to spur further research, exploration, and management (Wagner et al., 2018).
3.1.2.1 Punta Yeguas, Puerto Rico (Dive 6)
The site was located within the Inés María Mendoza Nature Reserve, also known as Punta Yeguas (Figure 4A). This dive targeted the potential habitats of deep-water fish species, including snappers and groupers. Approximately 8 kilometers off the southeastern coast of Puerto Rico, this dive took place at approximately 860 meters depth up a steep mound, which has a prominent ridge on the crest of the mound. Flat areas were fairly heavily sedimented, and there was a slight current on the bottom. High turbidity was observed in the water column, and there was an abundance of benthic organisms swimming in the water column. This dive transited 389 linear meters along the seafloor. Sixteen Sargassum observations were made over this distance, for an average 4.11 Sargassum observations per 100 meters (Table 1). The average depth for Sargassum observations was 757 meters.
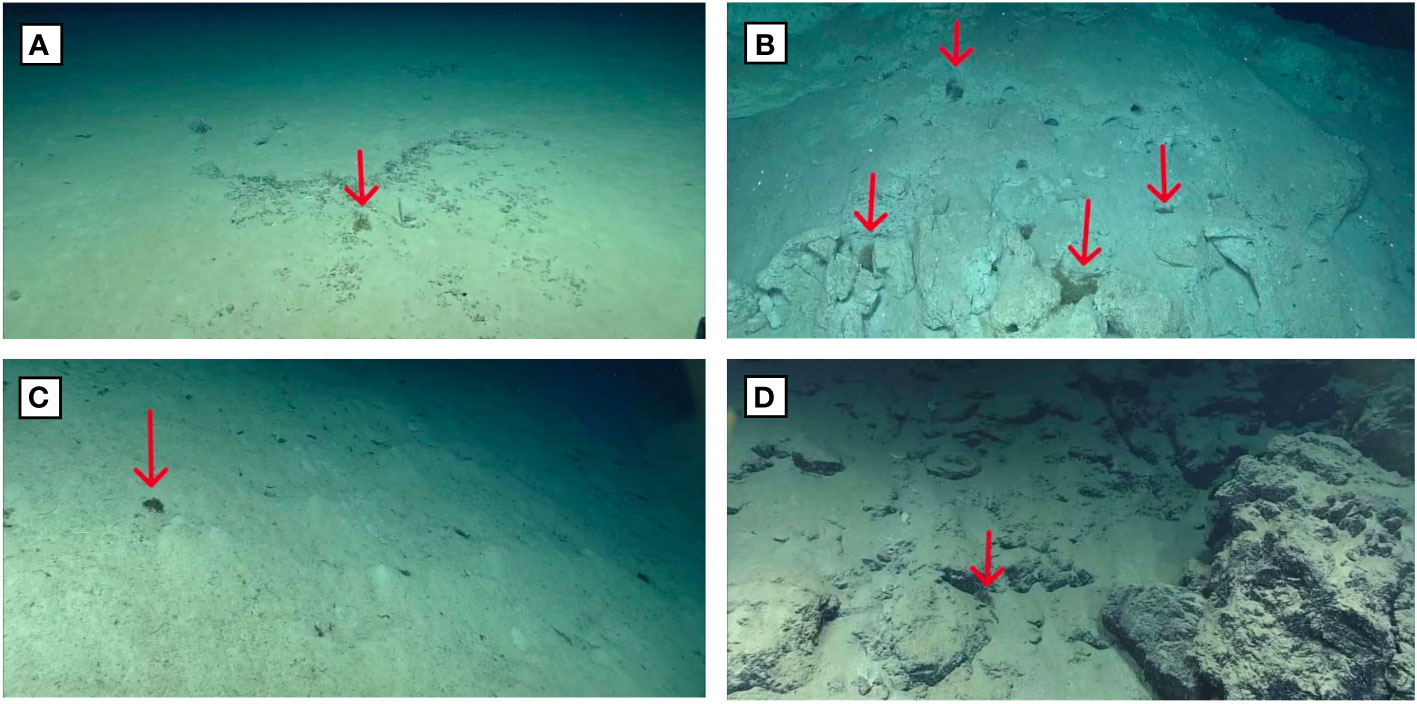
Figure 4 Dive sites reviewed from EX1811 Océano Profundo 2018: Exploring Deep-sea Habitats off Puerto Rico and the U.S. Virgin Islands. (A) Dive 6: Punta Yeguas, Puerto Rico, (B) Dive 7: Caja de Muertos Island, Puerto Rico, (C) Dive 8: South of La Parguera, Puerto Rico, (D) Dive 10: Mona Canyon, Puerto Rico.
3.1.2.2 Caja de Muertos Island, Puerto Rico (Dive 7)
This site was located to the south of the Caja de Muertos Island, south of Ponce, Puerto Rico (Figure 4B). The dive started on a steep slope (40 degrees), traversed northeast along a gentle slope area, and then moved up a mound. The habitat at the start of the dive was dominated by soft sediment with a few scattered boulders, with transitions between larger boulders to smaller boulders to soft sediment. At the beginning of the dive, small clumps of Sargassum were observed slowly tumbling along a steep, sedimented slope. This tumbling movement was not seen at any other sites included in this study. An urchin appeared to be grazing on a piece of Sargassum towards the beginning of the dive. The second half of the dive along the ridge was dominated by carbonate boulders with intermittent soft-bottom expanses. In addition to Sargassum, turtlegrass and anthropogenic debris were often observed on this dive. The ROV pilots mentioned that there had been high amounts of Sargassum observed on the surface in the area. Since Sargassum was so abundant on this dive, it is worth noting that a new Sargassum annotation was made for every new frame of view that contained Sargassum, not for every piece of Sargassum observed. Thus, while 19 Sargassum observations were recorded on this dive, the number of individual Sargassum was higher. This dive transited 572 linear meters along the seafloor. Nineteen Sargassum observations were made over this distance, for an average 3.32 Sargassum observations per 100 meters (Table 1). The average depth for Sargassum observations was 470 meters.
3.1.2.3 South of La Parguera, Puerto Rico (Dive 8)
This dive explored an unexplored ridge feature (Figure 4C) off La Parguera in southwest Puerto Rico. Beginning near the bottom of a steep slope (average 30 degree incline), this dive continued eastward toward a more moderate ridge. With a gentle slope dominated by soft sediment, the seafloor was largely homogeneous in composition, and no hard substrate was observed. Scours and burrows were common in this area, and Sargassum was often seen gathered in pits and burrows in the sediment. There were quite a few pieces of wood and other organic debris, like seagrass blades and Sargassum. Sargassum was observed in abundance at the beginning of the dive where the seafloor was relatively flat. Sargassum continued to be present on the slope, but in lesser quantities than on the flat surfaces. Many really small pieces of Sargassum were observed throughout the dive that were able to be positively identified in zoomed-in shots. During this dive, the science watch lead noted that the Sargassum on the surface had been a bit of a hazard for the ship for the past few days. Since Sargassum was so abundant on this dive, it is worth noting that a new Sargassum annotation was made for every new frame of view that contained Sargassum, not for every piece of Sargassum observed. Thus, while 112 Sargassum observations were recorded on this dive, the number of individual Sargassum was higher. This dive transited 997 linear meters along the seafloor. 112 Sargassum observations were made over this distance, for an average 11.23 Sargassum observations per 100 meters (Table 1). The average depth for Sargassum observations was 936 meters.
3.1.2.4 Mona Canyon, Puerto Rico (Dive 10)
This dive took place along the north side of the Mona Passage in the Mona Canyon (Figure 4D). Approximately 50 kilometers off the northwest side of Puerto Rico, this site is more exposed to the greater North Atlantic Ocean than the other sites from this expedition that we included in this study. This dive traversed up the western wall of a giant landslide scarp. Seafloor bathymetry and imagery in this area may provide evidence of historical landslide activity that could pose a potential geohazard to this region of the Atlantic Ocean. The dive started with high quantities of Sargassum detritus in large clumps, much larger than were observed on the other dives. At steeper features, such as large rocks on a steep canyon wall face, or on a rock avalanche geologic feature, there were many fewer Sargassum observations. Several instances of anthropogenic debris (metal cans, etc.) were also observed. Few other organisms were encountered throughout the dive. Since Sargassum was so abundant on this dive, it is worth noting that a new Sargassum annotation was made for every new frame of view that contained Sargassum, not for every piece of Sargassum observed. Thus, while 25 Sargassum observations were recorded on this dive, the number of individual Sargassum was higher. This dive transited 659 linear meters along the seafloor. Twenty-five Sargassum observations were made over this distance, for an average 3.79 Sargassum observations per 100 meters (Table 1). The average depth for Sargassum observations was 2,725 meters.
3.1.3 Windows to the Deep 2019: Southeast and Midatlantic U.S continental margin (EX1903L2), June 20 - July 12, 2019 Port Canaveral, Florida to Norfolk, Virginia
The second leg of Windows to the Deep 2019 was a 23-day expedition to explore the deepwater areas offshore Florida, Georgia, South Carolina, and North Carolina. This expedition mapped and characterized these areas, which are some of the least-explored off the U.S. East Coast, with the goal of providing baseline information to support science needs and management of sensitive habitats, maritime heritage sites, and potential resources. The 19 ROV dives of this expedition, which ranged from 298 to 3,490 meters in depth, focused on improving the knowledge of unexplored areas within the U.S. Exclusive Economic Zone (EEZ), particularly the deep-sea habitats of the U.S. continental margin and the connections between marine communities throughout the Atlantic Basin (Cantwell et al., 2019).
3.1.3.1 Stetson Mesa South Mounds, Eastern Florida (Dive 2)
This dive explored several deep-water coral mounds (Figure 5A) in the Stetson Miami Terrace Deep Water Coral Habitat Area of Particular Concern (HAPC), approximately 150 kilometers off the east coast of Florida. This dive also included several hours of water column exploration. The area explored was just inside the Gulf Stream, and it started out with a high quantity of marine snow at 500-700 meters. The general trend observed throughout the dive was a large abundance of coral rubble at the bottom of each mound with increasing abundance of live coral coverage on the east to southeastern side of each mound. A pancake urchin appeared to be grazing on a piece of Sargassum. This dive transited 1,041 linear meters along the seafloor. Sixteen Sargassum observations were made over this distance, for an average 1.54 Sargassum observations per 100 meters (Table 1). The average depth for Sargassum observations was 761 meters.
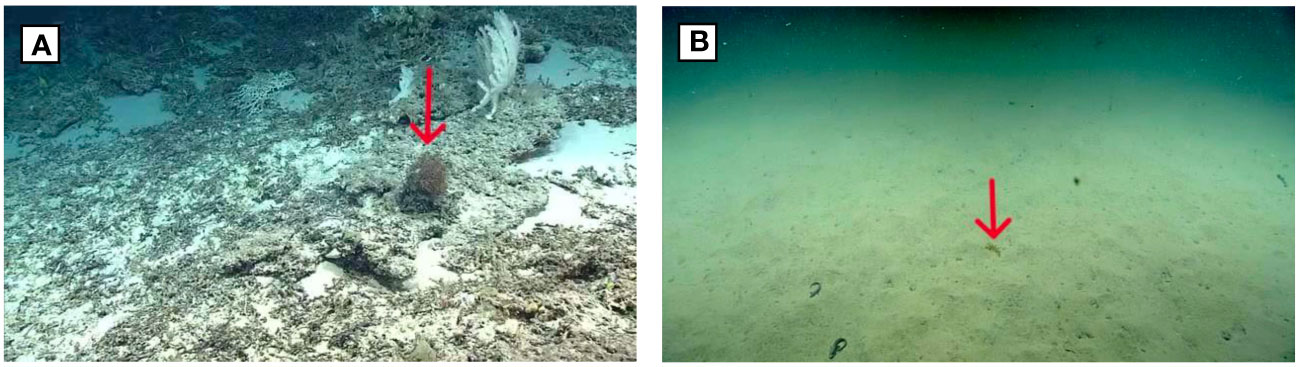
Figure 5 Dive sites reviewed from EX1903L2, Windows to the Deep 2019: Southeast and Mid-Atlantic U.S Continental Margin, Port Canaveral, FL to Norfolk, VA. (A) Dive 2: Stetson Mesa South Mounds, Eastern Florida, (B) Dive 11: “Dodge” Canyon, North Carolina.
3.1.3.2 “Dodge” Canyon, North Carolina (Dive 11)
This dive explored the Deep “Dodge” Canyon, specifically the mouth of an inner canyon/minor canyon area (Figure 5B) approximately 65 kilometers offshore of the Outer Banks, North Carolina. This site was characterized by a soft, heavily sedimented silty bottom, a lot of marine snow, and poor visibility. This is likely a result of organic material produced at the surface and in the midwater sinking and washing down the slope to accumulate on the bottom. The seafloor continued to be heavily sedimented, even while traversing up a relatively steep slope (>30 degrees), and almost no organisms were actually attached and growing on the benthos. This was a canyon dive and closer to shore than most of the other dives in this expedition, which had more elevated mounds with clearer water and exposed rocky substrate. Six Sargassum were observed on this dive, with two of the Sargassum under a light layer of silt. This dive transited 251 linear meters along the seafloor. Six Sargassum observations were made over this distance, for an average 2.39 Sargassum observations per 100 meters (Table 1). The average depth for Sargassum observations was 1,288 meters.
3.1.4 2019 Southeastern U.S. Deep-sea Exploration (EX1907), October 31-November 20, 2019 Miami, Florida to Key West, Florida
2019 Southeastern U.S. Deep-sea Exploration was a 21-day expedition to explore a diversity of poorly known deep seafloor and midwater habitats, as well as unique geological features, in areas off the U.S. Southeast. The 12 ROV dives of this expedition, which ranged from 404 to 1,218 meters in maximum depth, explored a diversity of poorly explored deepwater habitats and geological features, such as biogenic mounds, deep-sea coral and sponge habitats, and fish habitats, that are of interest to resource managers and scientists (White et al., 2019). In combination with deep-sea mapping operations, critical information and data were collected to characterize unknown and poorly known areas of the southern U.S. continental margin, with the goal of increasing our understanding of deep-sea ecosystems and supporting ecosystem-based management of marine resources (White et al., 2019).
3.1.4.1 Dive 11: Key West Deep
The primary objective of this dive was to explore and characterize a small canyon (Figure 6A) that has the potential to be suitable habitat for deep-water coral, sponges, and associated fauna. This dive explored two small mounds that rise about 5 meters from the surrounding seafloor as well as an escarpment feature and a potential seep site with authigenic carbonate and bacterial mats. The current was swift on the bottom. The soft, silty seafloor continued to be sedimented even when traversing up a relatively steep slope (>30 degrees), and there were many little mounds from bioturbation. There were large terraces on the escarpment with unconsolidated sediment cover. The vast majority of this dive was exploring near vertical inclines on the boulders and escarpment feature. Sargassum was observed on the little horizontal sandy surface that was explored. Six Sargassum were observed on this dive, including one patch of Sargassum at 19:25 that was more degraded than the other Sargassum observed in this study. This dive transited 927 linear meters along the seafloor. Six Sargassum observations were made over this distance, for an average 0.65 Sargassum observations per 100 meters (Table 1). The average depth for Sargassum observations was 1,208 meters.
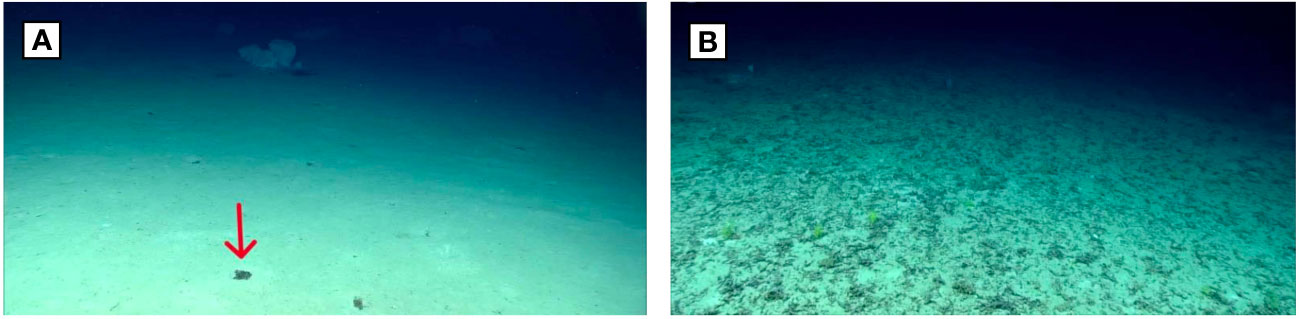
Figure 6 Dive sites reviewed from EX1907, 2019 Southeastern U.S. Deep-sea Exploration (A) Dive 11: Key West Deep, (B) Dive 12: “Berg Bits”, Southwestern Florida.
3.1.4.2 Dive 12: “Berg Bits”, Southwestern Florida
Fifty nautical miles southwest of the Dry Tortugas, this dive explored two mounds and an escarpment at the base of the “Antarctica mound” (Figure 6B) - a plateau shaped like Antarctica with “bergie bits,” or iceberg-like carved out features, surrounding the plateau. The plateau at the top of the escarpment and the seafloor in between mounds were sedimented. While approaching both mounds, small blocks from the escarpment decorated the sediment. Live coral was observed on the mounds, while standing dead coral and coral rubble was also prevalent on the mounds, along with seagrass. Most of the horizontal movement was done in transit from one feature to the next, so the ROV was moving fairly quickly and did not stop and zoom on features to allow a positive identification of Sargassum. No Sargassum were positively identified on this dive. Given the speed and distance from the ROV, it was not possible to distinguish between Sargassum and coral rubble and it is possible that there was Sargassum present. This dive transited 932 linear meters along the seafloor. The maximum depth reached was 973 meters.
4 Discussion
Because the deep sea is so under-studied, any new observations can lend valuable insight into the dynamics of deep-sea communities and how the deep sea is connected to the surface and to global environmental processes. Sargassum has been directly observed at local scales on the sea surface for centuries. The use of satellite-technologies has scaled up the spatial and temporal resolutions at which Sargassum can be observed, helping to better understand the species trajectory at the surface. However, once Sargassum dies and loses buoyancy, its fate is poorly understood after it sinks below the sea surface.
Through the use of deep submersible technologies and archived video, this study documented significant quantities of intact Sargassum on the seafloor in areas with known surface blooms. Sargassum was observed on 9 of the 10 dives reviewed for this project, in numbers ranging from 0 to more than 112 observations per dive. Given the limited spatial scale of our observations, we can surmise that Sargassum makes its way to the deep sea in likely significant amounts. Though we reviewed the descents and ascents for all the ROV dives, no Sargassum was observed within the water column, indicating that Sargassum might sink quickly once it loses its buoyancy, consistent with the relatively fast sinking rate estimated by Johnson and Richardson (1977). The relatively low volume of water imaged by the ROV may limit the ability to observe Sargassum sinking through the water column. It is also possible that some portion of the sinking Sargassum is consumed by pelagic herbivores.
Though we do not have quantitative estimates of Sargassum at the sea surface for each of the dives in this study, we do note two instances where expedition participants noted the heavy presence of Sargassum at the surface (e.g., Caja de Muertos Island and South of La Parguera). These dives correspond with some of the highest Sargassum observation numbers (more than 112 and more than 19), supporting the expectation that where Sargassum blooms are thick at the surface, large numbers sink below. Sargassum blooms have continued since this study time period, with March 2023 setting records for the most Sargassum observed in the month of March (University of South Florida (USF) and Optical Oceanography Lab, 2023). Sargassum has continued to wreak havoc in the Southeastern U.S. and Caribbean coastal communities, even interfering with ocean exploration operations6.
For this study, we considered the general environmental and ecological context of a dive site to explore whether there are areas that may be aggregators of Sargassum. There were no obvious patterns, though a few things to consider. “Dodge” Canyon off North Carolina was characterized by thick sediment and poor visibility, likely as a result of the high amount of marine snow that was observed both in the water column and on the seafloor. This marine snow indicates that there is likely high productivity on the surface to produce so much organic material. However, this large amount of marine snow may have quickly buried any Sargassum on the bottom, as two of the Sargassum that were observed there were partially covered in a fine layer of silt. Although only 6 specimens of Sargassum were observed on this dive, the second lowest out of the 10 dives surveyed, there may have been more there just buried from view. If the Sargassum is in fact being buried under silt at high surface productivity sites, this could remove the availability of the Sargassum to feeding by abyssal omnivores and scavengers such as sea urchins and gastropods, thus increasing the likelihood of sequestration. The high number of anthropogenic debris observed relatively far offshore (approximately 250 kilometers) at the Hidalgo Basin dive site indicates there might be some factor leading to accumulation here, such as currents or seafloor depressions also aggregating Sargassum. The bathymetry of the Mona Canyon dive site may have resulted in the larger piles of Sargassum observed at this dive, with the steep walls of the underwater canyon helping to funnel the Sargassum into large aggregations. The shallowest of the dives studied (Caja de Muertos, 470 meters) also included the only observation of Sargassum slowly tumbling down a slope. It is possible that, being closer to the surface, the Sargassum was more intact and thus more likely to be transported in this way.
There were three instances of invertebrates (two sea urchins and one gastropod) appearing to graze on Sargassum, observed on separate dives. This provides evidence that Sargassum is a source of food for benthic animals, with the further implication that some carbon is not being sequestered but instead is cycled back into the marine system. Bacterial degradation of the Sargassum would additionally diminish the carbon removal potential.
Part of the inherent value of exploration-driven study of the ocean is that some of the most interesting and impactful insights that may be gleaned from the research are typically unknown at the outset and unplanned for (e.g., Selig et al., 2019; Ford et al., 2020; Simon-Lledo et al., 2023). Although the Okeanos Explorer expeditions did not explicitly seek to study Sargassum on the seabed, through review of these 10 exploratory video surveys, we were able to significantly increase the number of confirmed direct observations of Sargassum on the deep seabed and contribute to broader understanding of the fate of sinking Sargassum. While observations of the Southeast U.S. and Gulf of Mexico remain sparse, they are comparatively well explored areas, with a decades-long history of submersible surveys (e.g., Harbor Branch Oceanographic Institute’s Johnson Sea-Link submersible). Further investigation could delve into available archived video footage to investigate trends in the quantity of Sargassum on the seafloor over time as blooms have increased in quantity. Additionally, we acknowledge that our study has inherent bias in that sites were intentionally selected where Sargassum were known to be observed. Further studies could use a random sampling design to provide an unbiased and quantitative assessment of Sargassum sinking at the seafloor.
Macroalgal ecosystems like those harboring Sargassum may sequester and store significant amounts of carbon from the atmosphere and ocean and hence are now recognized for their role in mitigating climate change. Developing a predictive understanding of carbon export pathways such as gravitational sinking is thus critical to diagnosing present and future rates of ocean carbon sequestration. Using ROV dive surveys to study the fate of Sargassum confirmed that relatively large amounts of Sargassum complete the first step in the process toward potential carbon sequestration on the seabed -- sinking to the seafloor. Long-term monitoring of the fate of sunken Sargassum on the seabed is needed in order to determine how much is ultimately sequestered rather than returned back into the system. Such observations would inform the feasibility of Sargassum farming and/or facilitated sinking as potential carbon dioxide removal strategies.
Further observations throughout the global ocean are required to fill in the gaps in the distribution of Sargassum in the deep sea. Further observation of the fate of Sargassum blooms on the deep seabed could serve as a natural model to inform research and development for emerging seaweed sequestration programs for climate mitigation.
Data availability statement
The original contributions presented in the study are included in the article/Supplementary Material. Further inquiries can be directed to the corresponding author.
Author contributions
AP conceived of this project, conducted the video analysis, created the plots and figures, and led the drafting of the manuscript. AN advised on deep-sea video analysis and NOAA Ship Okeanos Explorer operations and wrote sections of the manuscript. HB provided guidance on plotting the dive and Sargassum observations and CTD data analysis and conducted analysis of distance traveled. VH advised on potential machine learning applications. AN, HB, and VH all provided feedback and guidance on the project design. All authors contributed to the article and approved the submitted version.
Funding
Funding for field work was provided by NOAA Office of Ocean Exploration and Research.
Acknowledgments
This study used publicly-available data provided through NOAA Ocean Exploration and Research operations on NOAA Ship Okeanos Explorer. We thank the expedition coordinators, onboard science leads, ship crew, Global Foundation for Ocean Exploration ROV pilots, and other personnel who supported the expeditions considered in this study. We additionally thank Dr. Christopher Kelley for advising on the processing of CTD data. An early version of this study, Capstone paper “A Little Bit of Sargassum Goes A Long Way: Observations and Mapping of Sargassum fluitans and Sargassum natans from NOAA Ship Okeanos Explorer’s ROV Deep Discoverer” was published online in 2020 through escholarship.org as part of a Master of Advanced Studies Capstone project at Scripps Institution of Oceanography (Pries, 2020). This study updates and expands upon the Capstone paper by reviewing and characterizing four additional dives.
Conflict of interest
The authors declare that the research was conducted in the absence of any commercial or financial relationships that could be construed as a potential conflict of interest.
Publisher’s note
All claims expressed in this article are solely those of the authors and do not necessarily represent those of their affiliated organizations, or those of the publisher, the editors and the reviewers. Any product that may be evaluated in this article, or claim that may be made by its manufacturer, is not guaranteed or endorsed by the publisher.
Author disclaimer
The scientific results and conclusions, as well as any views or opinions expressed herein, are those of the author(s) and do not necessarily reflect the views of NOAA or the Department of Commerce.
Supplementary material
The Supplementary Material for this article can be found online at: https://www.frontiersin.org/articles/10.3389/fmars.2023.1250150/full#supplementary-material
Footnotes
- ^ https://exdata.tgfoe.org/OkeanosCruises/.
- ^ https://oceanexplorer.noaa.gov/okeanos/collaboration-tools/im-eventlog/participating-eventlog.html.
- ^ https://www.nodc.noaa.gov/oer/video/.
- ^ https://repository.library.noaa.gov/.
- ^ https://flowergarden.noaa.gov/management/sanctuaryexpansion.html.
- ^ https://oceanexplorer.noaa.gov/okeanos/explorations/22voyage-to-the-ridge/features/sargassum/sargassum.html.
References
Arenas F., Vaz-Pinto F. (2015). ““Marine algae as carbon sinks and allies to combat global warming,”,” in Marine Algae: Biodiversity, Taxonomy, Environmental Assessment, and Biotechnology. Eds. Pereira L., Neto J. M. (Boca Raton, FL: CRC Press, Taylor & Francis Group), 178–193. doi: 10.1201/b17540-6
Baker P., Minzlaff U., Schoenle A., Schwabe E., Hohlfeld M., Jeuck A., et al. (2017). Potential contribution of surface-dwelling Sargassum algae to deep-sea ecosystems in the southern North Atlantic. Deep-Sea Res. Part II: Topical Stud. Oceanography 148, 21–34. doi: 10.1016/j.dsr2.2017.10.002
Cantwell K., Kennedy B. R. C., Malik M., Suhre K. P., Medley R., Lobecker E., et al. (2020). The explorer model: lessons from 10 years of community-led ocean exploration & open data. J. Ocean Technol. 15 (3), 77–86.
Cantwell K., Wagner A., Weinnig A., Hoy S., Dunn C. J., Copeland A. (2019). EX1903L2 cruise report: windows to the deep 2019. Natl. Oceanic Atmospheric Administration Office Ocean Explor. Res, 1–53. doi: 10.25923/9ry2-fn95
Casazza T. L., Ross S. W. (2010) Sargassum: A Complex 'Island' Community at Sea. Available at: https://oceanexplorer.noaa.gov/explorations/03edge/background/sargassum/sargassum.html (Accessed February 10, 2020).
Conley L., Oliver D. (2019) Sargassum seaweed, invader of Florida and Caribbean beaches, may be the ‘new norm’. USA Today. Available at: https://www.usatoday.com/story/travel/news/2019/09/18/sargassum-seaweed-everything-you-need-to-know-florida-caribbean-beaches/2342086001/ (Accessed May 30, 2020).
Doyle E., Franks J. (2015) Sargassum Fact Sheet. Gulf and Caribbean Fisheries Institute. Available at: http://www.sargassoseacommission.org/storage/documents/GCFI_Sargassum_Fact_Sheet_Doyle:and_Franks_Sept_2015.pdf (Accessed March 12, 2020).
Duffy E.J., Benedetti-Cecchi L., Trinanes J., Muller-Karger F.E., Ambo-Rappe R., Boström C., et al (2017). Toward a Coordinated Global Observing System for seagrasses and marine macroalgae. Front. Mar. Sci. 6. doi:10.3389/fmars.2019.00317
Duarte C. M., Cebrián J. (1996). The fate of marine autotrophic production. Limnol. Oceanography 41 (8), 1758–1766. doi: 10.4319/lo.1996.41.8.1758
Duarte C. M., Wu J., Xiao X., Bruhn A., Krause-Jensen D. (2017). Can seaweed farming play a role in climate change mitigation and adaptation? Front. Mar. Sci. 4. doi:10.3389/fmars.2017.00100
Ford M., Bezio N., Collins A. (2020). Duobrachium sparksae (incertae sedis Ctenophora Tentaculata Cydippida): a new genus and species of benthopelagic ctenophore seen at 3,910 m depth off the coast of Puerto Rico. Plankton Benthos Res. 15, 296–305. doi: 10.3800/pbr.15.296
Franks J. S., Johnson D. R., Ko D. S. (2016). Pelagic sargassum in the tropical north atlantic. Gulf. Caribbean Res. 27 (1), SC6–SC11. doi: 10.18785/gcr.2701.08
Giering S. L. C., Cavan E. L., Basedow S. L., Briggs N., Burd A. B., Darroch L. J., et al. (2020). Sinking organic particles in the ocean—Flux estimates from in situ optical devices. Front. Mar. Sci. 6. doi: 10.3389/fmars.2019.00834
Godvin S. V., Dinesh K. M., Arulazhagan P., Amit K. B., Poornachander G., Rajesh B. J. (2021). Biofuel production from Macroalgae: present scenario and future scope. Bioengineered 12 (2), 9216–9238. doi: 10.1080/21655979.2021.1996019
Graham L. E., Wilcox L. W., Graham J. M. (2009). Algae. 2nd ed (San Francisco: Pearson/Benjamin Cummings), ISBN: ISBN: 0321559657.
Hanisak M. D., Samuel M. A. (1987). Growth rates in culture of several species of Sargassum from Florida, USA. Hydrobiologia 151, 399–404. doi: 10.1007/BF00046159
Jenkyns R., Gervais F., Pirenne B. (2013). “SeaScribe: An annotation software for Remotely Operated Vehicle dive operations,” in 2013 OCEANS - San Diego (San Diego, CA , USA: IEEE), 1–5. doi: 10.23919/OCEANS.2013.6741250
Johnson D. L., Richardson P. L. (1977). On the wind-induced sinking of Sargassum. J. Exp. Mar. Biol. Ecol 28, 255–267. doi: 10.1016/0022-0981(77)90095-8
Kennedy B. R., Cantwell K., Malik M., Kelley C., Potter J., Elliott, et al. (2019). The unknown and the unexplored: insights into the pacific deep-sea following NOAA CAPSTONE expeditions. Front. Mar. Sci. 6. doi: 10.3389/fmars.2019.00480
Kennedy B. R. C., Elliott K. P., Cantwell K., Mesick S., Wagner K. (2016). “Telepresence enabled exploration with NOAA Ship Okeanos Explorer,” in The E/V Nautilus and NOAA Ship Okeanos Explorer 2015 field season. Oceanography (Rockville, MD, USA: The Oceanography Society), vol. 29 . Eds. Bell K. L. C., Brennan M. L., Flanders J., Raineault N. A., 50–51. doi: 10.5670/oceanog.2016.supplement.01
Kokubu Y., Rothäusler E., Filippi J., Durieux E. D., Komatsu T. (2019). Revealing the deposition of macrophytes transported offshore: Evidence of their long-distance dispersal and seasonal aggregation to the deep sea. Sci. Rep. 9, 1. doi: 10.1038/s41598-019-39982-w
Krause-Jensen D., Duarte C. M. (2016). Substantial role of macroalgae in marine carbon sequestration. Nat. Geosci. 9 (10), 737–742. doi: 10.1038/ngeo2790
Lamb J. (2018) The great seaweed invasion. JSTOR Daily. Available at: https://daily.jstor.org/great-seaweed-invasion/ (Accessed May 20 2020).
López Miranda J. L., Celis L. B., Estévez M., Chávez V., van Tussenbroek B. I., Uribe-Martínez A., et al. (2021). Commercial potential of pelagic Sargassum spp. in Mexico. Front. Mar. Sci. 8. doi: 10.3389/fmars.2021.768470
Maxon A., Pawlenko N., White M., Skarke A., Wagner D., Cantelas F., et al. (2018). EX-18-03 expedition report: gulf of Mexico 2018 (ROV/mapping). Natl. Oceanic Atmospheric Administration Office Ocean Explor. Res, 1–77. doi: 10.25923/ksxr-vj32
Mcleod E., Chmura G. L., Bouillon S., Salm R., Björk M., Duarte C. M., et al. (2011). A blueprint for blue carbon: toward an improved understanding of the role of vegetated coastal habitats in sequestering CO2. Front. Ecol. Environ. 9, 552–560. doi: 10.1890/110004
McLetchie K., White M., Pawlenko N. (2018). Okeanos Explorer ROV Dive Summary, EX1803, Dive 8, April 25, 2018 (Silver Spring, MD, USA: National Oceanic and Atmospheric Administration, Office of Ocean Exploration and Research). Available at: https://repository.library.noaa.gov/view/noaa/21409.
National Academies of Sciences, Engineering, and Medicine (2022). A Research Strategy for Ocean-based Carbon Dioxide Removal and Sequestration (Washington, DC: The National Academies Press). doi: 10.17226/26278
NOAA (2019) What is the Sargasso Sea? National Ocean Service website. Available at: https://oceanservice.noaa.gov/facts/sargassosea.html (Accessed January 12,2020).
N’Yeurt A. D. R., Chynoweth D. P., Capron M. E., Stewart J. R., Hasan M. A. (2012). Negative carbon via ocean afforestation. Process Saf. Environ. Prot. 90, 467–474. doi: 10.1016/j.psep.2012.10.008
Ody A., Thibaut T., Berline L., Changeux T., André J. M., Chevalier C., et al. (2019). From In Situ to satellite observations of pelagic Sargassum distribution and aggregation in the Tropical North Atlantic Ocean. PloS One 14 (9), e0222584. doi: 10.1371/journal.pone.0222584
Omand M. M., Govindarajan R., He J., Mahadevan A. (2020). Sinking flux of particulate organic matter in the oceans: Sensitivity to particle characteristics. Sci. Rep. 10, 5582. doi: 10.1038/s41598-020-60424-5
Oxenford H. A., Cox S.-A., van Tussenbroek B. I., Desrochers A. (2021). Challenges of turning the sargassum crisis into gold: current constraints and implications for the caribbean. Phycology 1 (1), 27–48. doi: 10.3390/phycology1010003
Peters C., Coleman D. F., Martinez C. (2019). Expedition support from the inner space center. J. Oceanography 32 (1), 6–7. doi: 10.5670/oceanog.2019.supplement.01. Raineault, in New Frontiers in Ocean Exploration: The E/V Nautilus, NOAA Ship Okeanos Explorer, and R/V Falkor 2018 Field Season, ed Raineault, N.A., and Flanders.
Pries A. (2020). A Little Bit of Sargassum Goes A Long Way: Observations and Mapping of Sargassum fluitans and Sargassum natans from NOAA Ship Okeanos Explorer's ROV Deep Discoverer. (San Diego (CA: Scripps Institution of Oceanography). Available at: https://escholarship.org/uc/item/83q4h0j5. Masters of Advanced Studies Capstone paper.
Raven J. A. (2017). The possible roles of algae in restricting the increase in atmospheric CO2 and global temperature (506-522: European Journal of Phycology. 52:4). doi: 10.1080/09670262.2017.1362593
Rodríguez-Martínez R., Medina-Valmaseda A., Blanchon P., Monroy-Velázquez L., Almazán-Becerril A., Delgado-Pech B., et al. (2019). Faunal mortality associated with massive beaching and decomposition of pelagic Sargassum. Mar. pollut. Bull. 146, 201–205. doi: 10.1016/j.marpolbul.2019.06.015
Sanchez-Rubio H., Perry J. S., Franks D. R. J. (2018). Occurrence of pelagic Sargassum in waters of the US Gulf of Mexico in response to weather-related hydrographic regimes associated with decadal and interannual variability in global climate. Fish. Bull. 116 (1), 93–107. doi: 10.7755/FB.116.1.10
Schamberger L., Minghelli A., Chami M. (2022). Quantification of underwater Sargassum aggregations based on a semi-analytical approach applied to Sentinel-3/OLC (Copernicus) data in the tropical Atlantic Ocean. Remote Sens. 14, 5230. doi: 10.3390/rs14205230
Selig G., Netburn A., Malik M. (2019). Distributions of the pelagic Holothurian Pelagothuria in the central Pacific Ocean as observed by remotely-operated vehicle surveys. Front. Mar. Sci. 6. doi: 10.3389/fmars.2019.00684
Simon-Lledó E., Bett B. J., Benoist N. M. A., Hoving H., Aleynik D., Horton T., et al. (2023). Mass falls of crustacean carcasses link surface waters and the deep seafloor. Ecology 104 (2), e3898. doi: 10.1002/ecy.3898
Stiger-Pouvreau V., Mattio L., N’Yeurt A. D. R., Uwai S., Dominguez H., Flórez-Fernández N., et al. (2023). A concise review of the highly diverse genus Sargassum C. Agardh with wide industrial potential. J. Appl. Phycol. 35, 4 1453–4 1483. doi: 10.1007/s10811-023-02959-4
Stoner A. W. (1983). Pelagic Sargassum: evidence for a major decrease in biomass. Deep Sea Res. Part A. 30 (4), 469–474. doi: 10.1016/0198-0149(83)90079-1
Sugumaran R., Padam B. S., Yong W. T. L., Saallah S., Ahmed K., Yusof N. A. (2022). A retrospective review of global commercial seaweed production—current challenges, biosecurity and mitigation measures and prospects. International J. Environ. Res. Public Health 19 (12), 7087. doi: 10.3390/ijerph19127087
Trinanes J., Putman N. F., Goni G., Hu C., Wang M. (2023). Monitoring pelagic Sargassum inundation potential for coastal communities. J. Operational Oceanography 16 (1), 48–59. doi: 10.1080/1755876X.2021.1902682
University of South Florida (USF), Optical Oceanography Lab (2023). March Update Outlook of 2023 Sargassum blooms in the Caribbean Sea and Gulf of Mexico (St. Petersburg, FL: Optical Oceanography Lab). Accessed at https://optics.marine.usf.edu/projects/SaWS/pdf/Sargassum_outlook_2023_bulletin3_USF.pdf on May 20, 2023.
Valentini N., Balouin Y. (2020). Assessment of a smartphone-based camera system for coastal image segmentation and Sargassum monitoring. J. Mar. Sci. Eng. 8. doi: 10.3390/jmse8010023
Volk T., Hoffert M. I. (2013). “Ocean carbon pumps: analysis of relative strengths and efficiencies in ocean-driven atmospheric CO2 changes,” in The Carbon Cycle and Atmospheric CO2: Natural Variations Archean to Present Geophysical Monograph Series. Eds. Sundquist E., Broecker W., (Washington, D.C., USA: American Geophysical Union) 99–110. doi: 10.1029/GM032p0099
Wagner D., Sowers D., Williams S. M., Auscavitch S., Blaney D., Cromwell M. (2018). EX-18-11 Expedition Report: Océano Profundo 2018: Exploring Deep-Sea Habitats off Puerto Rico and the U.S. Virgin Islands. Natl. Oceanic Atmospheric Administration Office Ocean Explor. Res, 1–171. doi: 10.25923/wc2n-qg29
Wang M., Hu C. (2016). Mapping and quantifying Sargassum distribution and coverage in the Central West Atlantic using MODIS observations. Remote Sens. Environment 183, 350–367. doi: 10.1016/j.rse.2016.04.019
Wang M., Hu C. (2017). Predicting Sargassum blooms in the Caribbean Sea from MODIS observations. Geophysical Res. Lett. 44, 3265–3273. doi: 10.1002/2017GL072932
Wang M., Hu C., Barnes B. B., Mitchum G., Lapointe B., Montoya J. P. (2019). The great Atlantic Sargassum belt. Science 365 (6448), 83–87. doi: 10.1126/science.aaw7912
Wells R., Rooker J. (2004). Spatial and temporal patterns of habitat use by fishes associated with Sargassum mats in the northwestern Gulf of Mexico. Bull. Mar. Sci. 74, 81–99.
Keywords: Sargassum, macroalgae, ocean exploration, carbon sequestration, algae blooms, Okeanos Explorer, deep seafloor
Citation: Pries A, Netburn AN, Batchelor H and Hermanson VR (2023) A little bit of Sargassum goes a long way: seafloor observations of Sargassum fluitans and Sargassum natans in the Western Atlantic Ocean. Front. Mar. Sci. 10:1250150. doi: 10.3389/fmars.2023.1250150
Received: 29 June 2023; Accepted: 03 October 2023;
Published: 07 December 2023.
Edited by:
Daniel Wagner, Ocean Exploration Trust, United StatesReviewed by:
Antoine De Ramon N’Yeurt, University of the South Pacific, FijiShigeki Wada, University of Tsukuba, Japan
Copyright © 2023 Pries, Netburn, Batchelor and Hermanson. This is an open-access article distributed under the terms of the Creative Commons Attribution License (CC BY). The use, distribution or reproduction in other forums is permitted, provided the original author(s) and the copyright owner(s) are credited and that the original publication in this journal is cited, in accordance with accepted academic practice. No use, distribution or reproduction is permitted which does not comply with these terms.
*Correspondence: Ashley Pries, apries@ucsd.edu