Corrigendum: Effect of live-storage period and temperature on oxygen consumption rate in the cold-water sea cucumber Parastichopus tremulus
- Møreforsking AS, Ålesund, Norway
Sea cucumbers are of great significance in marine ecosystems all over the world. The red sea cucumber Parastichopus tremulus is also considered commercially interesting but very little information is available on its ecology and physiology. This study aimed at investigating this North Atlantic species` oxygen demand in relation to live-storage period and temperature in an attempt to deliver some physiological puzzle pieces useful in a potential aquacultural context. Oxygen consumption rate (OCR) was measured in P. tremulus stored in a flow-through system for up to one year, and in recirculation systems at different temperatures (4, 8 and 12°C) for up to four weeks. Throughout the experimental period, biometric data were collected from the different treatment groups and a condition index (CI) calculated. The results showed that long-term storage affected OCR and CI, which increased and decreased with time, respectively. Storage at 4°C resulted in significantly lower OCR and higher CI compared to 8 and 12°C after four weeks, suggesting that 4°C may be best for maintaining body mass during short-term storage, whereas storage at 8 and 12°C may require supplementary feeding to avoid biomass loss. Data presented in this study may be useful for a range of applications, for example in a prospective aquaculture setting, for optimizing live seafood storage, but also for impact assessment and management of wild stocks.
1 Introduction
Several sea cucumber species are commercially exploited due to their importance as a luxury seafood and application in traditional medicine (Purcell, 2014; Yang et al., 2015). Rising Asian demand and the associated depletion of wild stocks in tropical and temperate regions (Purcell et al., 2014) has brought attention to cold-water species as well. In Europe, and Norway in particular, sea cucumbers have not been considered a potential marine resource until recently, thus research and knowledge on their biology and ecology is limited (González-Wangüemert et al., 2018; Landes et al., 2019). Sea cucumbers (holothuroids) are found in all marine water bodies in Northern Europe, apart from the lower saline Baltic Sea, and more than more than 40 species have been registered in Scandinavia (Madsen and Hansen, 1994). Feeding at the lowest levels of the food web, they are of great significance in marine ecosystems, particularly in deep-water ecosystems where they can account for more than 90% of the biomass (Pawson et al., 2011). Although echinoderms such as holothuroids have a lower oxygen consumption compared to other species (Fox, 1936), they contribute significantly to benthic nutrient cycling due to their abundance in this specific ecosystem. Because of some species’ unique ability to extract nutrients from particulate matter, they are also considered key candidate species for inclusion in sustainable aquaculture production systems such as integrated multi-trophic aquaculture (IMTA).
To better understand physiological responses to environmental changes and performance in captivity, in-depth studies investigating the functional metabolism of each species are required. Water temperature, for instance, is a crucial factor for ectothermic animals like sea cucumbers, as it impacts a wide range of physiological and intercellular processes (Schmidt-Nielsen, 1990; Burggren and Roberts, 1991). From temperate species, we know that temperature affects a range of behavioural and physiological parameters in sea cucumbers, such as feeding behaviour, growth, and feed utilization (Yang et al., 2005; Ji et al., 2008). An increase in water temperature leads to an acceleration of physiological processes inside the organism and thereby to higher oxygen demand and excretion of metabolic waste products, resulting in increased oxygen consumption and ammonia excretion rates (Zamora and Jeffs, 2012).
Breathing (respiration) in holothuroids primarily occurs through the respiratory tree which is a highly branched organ specialized for gas exchange and excretion. The paired respiratory tree is located close to the anal opening and breathing is performed by drawing and expelling water through the anus. If the respiratory tree has been eviscerated and internal organs are under regeneration, respiration may occur solely through the skin and tube feet (Hamel et al., 2022). However, presence or absence of a respiratory tree does not seem to have a significant effect on overall oxygen consumption as demonstrated by Dimock (1977) in the Pacific species Stichopus parvimensis. A third possible passage for gases and fluids is through the madreporite which in sea cucumbers is located inside the coelom (Gao and Yang, 2015, chapter 4). In addition to exhibiting an allometric relationship with body size (Astall and Jones, 1991; Kodama et al., 2015), a range of factors are known to affect the metabolic rate and thereby oxygen consumption rate (OCR) in echinoderms, e.g. salinity (Talbot and Lawrence, 2002), pH (Farmanfarmaian, 1966), partial pressure of oxygen, pO2 (Shick, 1983; Spicer, 1995; Christensen and Colacino, 2000), seasonal variation in growth (Lawrence and Lane, 1982; Fraser et al., 2004), feeding status (Vahl,1984; Brockington and Clarke, 2001; Idrisi et al., 2003; Yu et al., 2020), nutritional quality of food (Otero-Villanueva et al., 2004) and reproductive state (Giese et al., 1966; Féral and Magniez, 1988; Ru et al., 2020). Changes in locomotory activity, such as an increase during spawning season (Ru et al., 2021), or a reduction during aestivation (Ji et al., 2008), can also impact the rate of oxygen consumption. Commonly used to determine metabolic energy use in heterotrophic organisms, the rate of oxygen consumption may also serve as an indicator for the animal’s general metabolic state and is a suitable tool for uncovering potential stress responses to changing environmental conditions.
In Norway, the red sea cucumber Parastichopus tremulus has been identified as one of the species with the highest commercial potential for Asian markets (Kjerstad et al., 2015). P. tremulus has also been considered as a potential key species in Norwegian IMTA (Sunde and Christophersen, 2023). Presently only landed as by-catch, it is one of the most common sea cucumbers occurring along the Norwegian coast. Although being most abundant at depths between 100-300 m along the Norwegian west coast (Kjerstad et al., 2015), P. tremulus has also been recorded at shallow depths of ≤20 m and down to >3000 m in the deep sea in other areas (Ludwig, 1900; Grieg, 1921; MNHN, Chagnoux, 2023). This large range of bathymetric distribution implies that P. tremulus has a high natural physiological plasticity that facilitates adaptation to different environments. However, our understanding of this species’ capacity to adapt to different conditions, e.g. future elevated sea temperatures linked to climate change, remains limited. Although P. tremulus is expected to encounter relatively minor temperature fluctuations at the depths where it is most abundant, even slight variations in temperature could potentially have significant impacts on cold-water species (e.g. Emblemsvåg et al., 2022). One of the few known physiological characteristics of P. tremulus from Norwegian waters is its seasonal feeding pattern, where feeding ceases and evisceration is more prevalent during winter months (Jespersen and Lützen, 1971). This phenomenon is also observed in other Northern hemisphere species (Swan, 1961; Fankboner and Cameron, 1985). However, very few studies have been carried out on the physiological and metabolic characteristics of P. tremulus and how these compare to those of other holothuroids (Fox, 1936; Hauksson, 1977; Hauksson, 1979).
There are many reasons as to why acquiring of more in-depth knowledge is absolutely crucial. Given the increase in anthropogenic activities in coastal areas, zones of hypoxic conditions (low dissolved oxygen) could become more widespread in the future. Hence, it is essential to determine the oxygen requirements of P. tremulus under different conditions to evaluate the impact of human activities on these vital components of benthic ecosystems. Subtidal species such as the red sea cucumber may have a lower tolerance to low oxygen concentrations than intertidal species that regularly experience changing conditions (Weinrauch and Blewett, 2019), and thus be more at risk when exposed to such changes. In order to enable the preservation of our coastal marine environment and an effective management of the marine resources, we urgently need to learn more about the species` basic physiological operating principles and responses to its specific environment. Furthermore, it is requisite to determine the species` environmental requirements for development, growth, and welfare in captivity, considering its potential as an aquaculture candidate (Landes et al., 2019; Schagerström et al., 2022). However, it should be noted that the conditions conducive to growth in aquaculture may differ substantially from those in the natural habitat.
Maintaining a sea cucumber species such as P. tremulus under conditions that ensure welfare and facilitate growth, requires knowledge on physiological responses related to storage time and temperature. Therefore, this study aimed to quantify and observe the level of respiration and its development during short (weeks) and long (months) term storage in flow-through systems. The objective was to uncover potential changes in OCR associated with physiological stress under conditions in captivity and its metabolic consequences. Furthermore, we wanted to investigate the response of P. tremulus to storage at different temperatures over a period of four weeks in recirculation systems intended for storage of live seafood. We hypothesized that OCR would increase with increasing temperature, especially since 8 and 12°C diverge significantly from temperatures that are common in the species’ preferred deep-sea habitat. Although P. tremulus may encounter temperatures up to 12°C in its natural range of distribution during the summer months, temperatures in the depth where experimental animals were retrieved from rarely exceed 7°C (Nilsen and Falck, 2006). Thereby, we expected the lowest OCR to be measured at 4°C whereas rates at both 8 and 12°C would reflect a stressed state expressed by significantly higher OCR. With regard to storage period, we expected that OCR would change with duration of storage, since laboratory conditions differ from that of the natural habitat. The direction of OCR change over time is influenced by several factors and hard to predict. For instance, metabolic depression due to the lack of appropriate food could lead to a decrease in OCR while accumulated stress under laboratory conditions could lead to an increase.
2 Materials and methods
Sea cucumbers (Parastichopus tremulus) used in the experiments were collected at 130-250 m depth in the fjord system south of Molde in Møre and Romsdal county, located in the north-western part of Norway (62°N-7°E) – where they have been obtained as bycatch from pot fisheries of Norway lobster (Nephrops norvegicus). Length and total wet weight (WW) of the sea cucumbers (n=214) ranged from 60 to 220 mm (average 126.4 mm) and from 21 to 245 g (average 72.5 g), respectively. Results are presented as the average value ± standard deviation, unless stated otherwise.
At the landing site, sea cucumbers were transferred to 1 L plastic bags filled with ambient, unfiltered seawater. The bags, each containing 1-3 individuals (dependent on size), were placed in polystyrene boxes with cooling elements distributed on top. Transportation to the Møreforsking laboratory facilities in Ålesund took about one hour. Upon arrival, the animals were directly transferred into square flow-through holding tanks (100 x 100 x 50 cm, 300 L) supplied with untreated seawater (10 L min-1) pumped up from 40 m depth. During the experimental period from mid-September to mid-December 2022, sea water temperature in these tanks ranged from 10 to 12°C. Annual temperature fluctuations in incoming water peaked in October (12.5°C) and were at its minimum in March (6.4°C). Salinity varied between 31 and 32 ppt.
Three groups of sea cucumbers were collected for use in the studies: 1) obtained in November 2022 and stored for one week, 2) obtained in September 2022 and stored for 10 weeks, and 3) obtained in September-October 2021 and stored for >1 year (13-14 months) in the flow-through system prior to the experiment. Animals in group 1 and 2 were not supplied with feed other than what the incoming unfiltered seawater provided in form of organic matter. Group 3 on the other hand was fed regularly on a mixture of seaweed powder (Sargassum thunbergii) and sand at a ratio (volume:volume) of 50:50 (medium ration according to Sunde and Christophersen, 2023). All experiments were performed with standardized groups of sea cucumbers where one group consisted of three sub-groups (replicates) divided between three perforated plastic trays (L x W x H: 600 x 352 x 96 mm) with lids.
The effect of live-storage period was examined in sea cucumbers kept in a flow-through seawater system (FT). When investigating the effect of temperature, sea cucumber groups were placed in recirculation tanks (Adriatic Sea International Srl, San Clemente, Italy) each containing 300 L of filtered seawater (<1 µm). Three different temperatures, 4, 8 and 12°C (4.15 ± 0.14°C, 8.59 ± 0.37°C, 12.25 ± 0.35°C) were chosen for the trial. The water was circulated in a closed cycle, running through a charcoal filter and a refrigeration unit. No feed was added during the experiment. Water quality was monitored regularly and, if necessary, water was exchanged to ensure safe levels of ammonium, ammonia, nitrate, and nitrite (<0.36 mg NH4-N L-1, <0.006 mg NH3 L-1, <0.40 mg NH3-N L-1, <0.44 mg NH2-N L-1). Oxygen saturation remained high during the experiment (>9 mg L-1) and the pH ranged from 7.9 to 8.3. An additional set of trays containing the same number of sea cucumbers was kept in the flow-through system as a reference group (11.11 ± 0.74°C). Initial stocking density was 3.25-5.85 kg per m2 of tray area (4.7 ± 1.03 kg per m2, n=12) which was considered a low density (14 individuals per tray). The total initial biomass per tank was 3 ± 0.24 kg (n=4). After two weeks (T1) half of the biomass was removed and sampled, the remaining half was taken out two weeks later, after four weeks (T2). Water temperature was logged continuously with a 15 min interval (HOBO TidbiT v2, Onset Computer Corporation, Bourne, MA, USA) in both flow through and recirculation tanks.
Oxygen consumption rates (OCR) were measured in groups of three sea cucumbers at a time. In order to investigate the impact of storage time on oxygen demand, OCR was determined after 1, 3 and 5 weeks (group 1, FT-1), after 1 and 10 weeks (group 2, FT-2) as well as after >1 year (group 3, FT-3) of storage in the flow-through system. The effect of temperature on oxygen consumption during short-term storage was examined in a separate experiment by measuring OCR for groups of 3 animals at the start of the experiment (T0), as well as after two (T1) and four (T2) weeks of storage at the three chosen temperatures (4, 8 and 12°C) in the respective recirculation systems. All measurements were conducted during September, November and December 2022.
Sea cucumbers used for initial respiration measurements were acclimated for at least a week in running seawater (flow-through system) and the technical setup was incorporated into the holding tank of each storage group to minimize disturbance and external influences such as air exposure, temperature change and physical handling. A vertical circular respiration chamber (Ø 190 mm, depth 110 mm, Loligo© Systems AS, Viborg, Denmark) was completely submerged, and filled with in situ water from each respective holding tank. Oxygen consumption was measured via intermittent respirometry, according to the method of Vismann and Hagerman (1996), using a computer-controlled setup that recorded oxygen partial pressure and temperature every 300 seconds. Oxygen partial pressure (pO2) was measured with a temperature, salinity and pressure compensated optical oxygen meter (OXY-4 SMA (G3), PreSens–Precision Sensing GmbH, Regensburg, Germany) via a polymer optical fibre (POF), transferring excitation light to the oxygen sensor spot (SP-PSt3-NAU, detection limit 15 ppb; measurement range of 0 – 100% oxygen in dissolved or gaseous phase) and the sensor response back to the meter. Oxygen was measured contactless through the transparent chamber wall. Decline curves were recorded until pO2 decreased by at least 10% (but no further than 20%) and three replicable curves with similar regression slopes were plotted, assuming optimal acclimation had occurred. Temperature constancy was ensured throughout the measurements. Oxygen consumption (MO2) was calculated according to Equation 1:
where pO2 is the oxygen partial pressure at 100% atmospheric oxygen saturation (in mm Hg), α is the linear regression slope (in % oxygen per second), β is the oxygen solubility of the water at given temperature and salinity (in µg oxygen per L per mm Hg), v is the volume of water (in litres) in the respiration chamber including the tubing of the shunt, and 3.6 is the conversion factor to obtain oxygen consumption rates (OCR) in mg O2 h-1 (without conversion: µg O2 s-1). Furthermore, individual oxygen consumption rates (OCR) were standardized to 1 g of dry weight sea cucumber body wall tissue using Equation 2:
where OCRs is the oxygen consumption rate of a standard-weight animal, Ws is its weight, We is the measured weight of the experimental animal and OCRe is the uncorrected oxygen consumption rate of the experimental animal. OCR values are given in units of either mg oxygen per group per hour or as weight-corrected values of µg oxygen per g body wall dry weight per hour.
Individual biometric data were collected from sea cucumbers at the same sampling points as for respirometry. Body length (mouth to anus) was measured to the nearest 1 mm, using a measuring board, and animal wet weights (WW) to the nearest 0.1 g, using an SKX2202 electronic scale (Ohaus GmbH, Nänikon, Switzerland). Body wall (BW) WW was obtained after dissection from gutted (eviscerated) individuals. A standardized mid-section of the BW (ca. 30% of total WW, 8-22 g) was oven-dried at 103°C for 24-48 h to obtain dry weights (DW), and ash weight (AW) was measured after combustion at 550°C for 4 hours. The condition index (CI) was calculated based on gutted (eviscerated) sea cucumber weights according to Equation 3:
Where DWBW is the dry weight of the body wall including muscle bands and mouth parts (in grams) and WWBW is the wet weight of the BW including muscle bands and mouth parts (in grams). The CI is given in percent (%).
Statistical analyses were carried out using either Microsoft Excel 365 (Microsoft Corporation, Seattle, WA, USA) or Jamovi version 2.3 (http://www.jamovi.org). Comparisons between treatment groups for variables that were normally distributed were carried out using either Student’s t-test for comparisons between two groups or one-way analysis of variance (ANOVA) for multiple groups. Significant differences (p<0.05) were followed by a post hoc Tukey’s HSD multiple comparison test for multiple groups. For variables that failed tests for normality and homogeneity of variance, corresponding non-parametric tests were applied, either Mann-Whitney U test (two groups) or Kruskal-Wallis (multiple groups).
3 Results
3.1 Effect of live-storage period
Storage time had a significant effect on oxygen consumption rate (OCR) and condition index (CI). Weight specific OCR in animals kept for up to 5 weeks remained at similar levels (Figure 1, FT-1, one-way ANOVA, p=0.593), while OCR measurements after 10 weeks of live-storage showed a significant increase from initially ~31 µg O2 h-1g-1 body wall dry weight (BW DW) to ~55 µg O2 (Figure 1, FT-2, unequal variances t-test, p=0.005). Sea cucumbers, kept in the flow-through seawater system >1 year, displayed even higher OCR of ~81 µg O2 h-1g-1 BW DW (Figure 1, FT-3, unequal variances t-test, p=0.045). Biometric data of individuals used for respirometry are shown in Table 1.
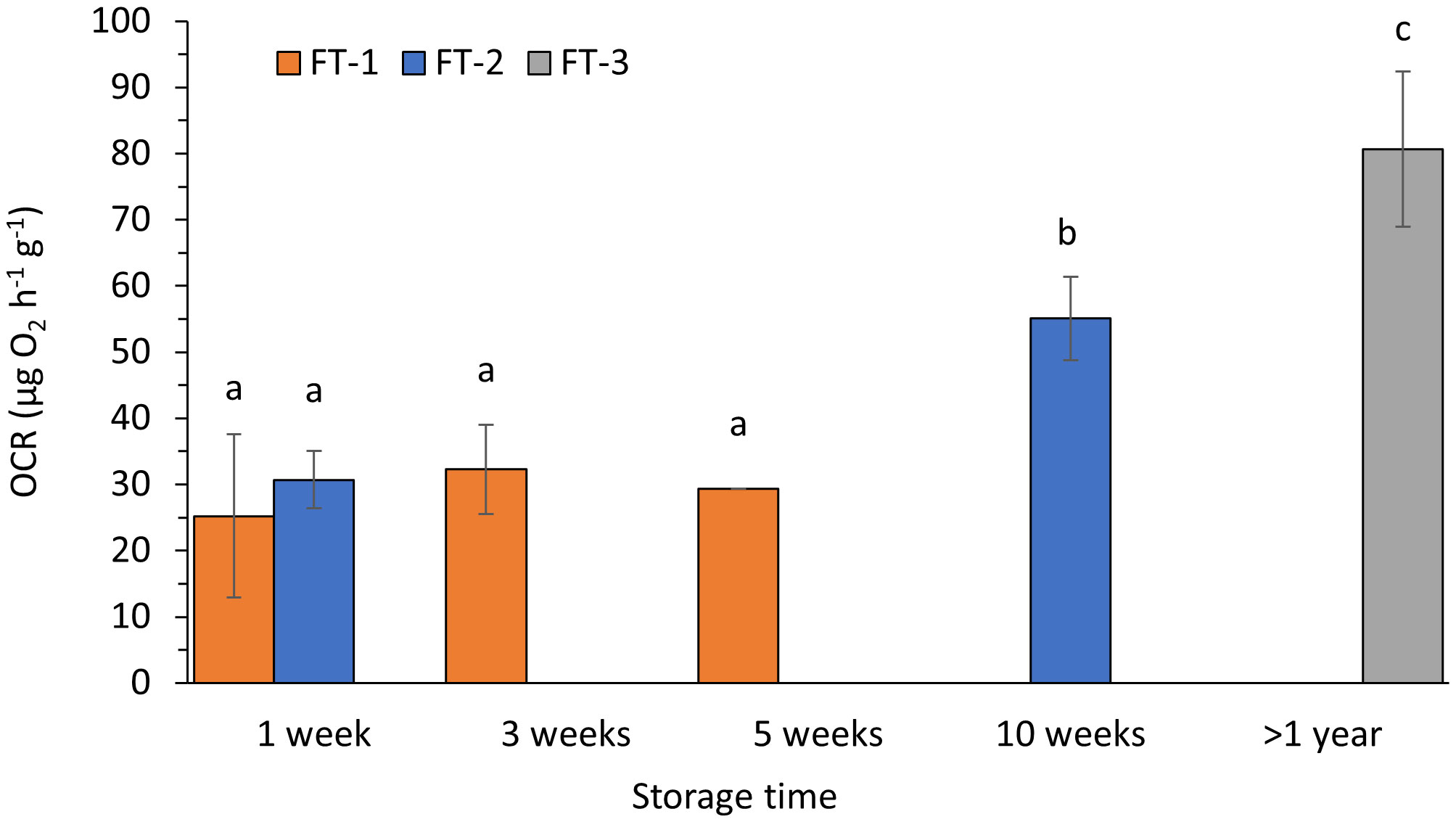
Figure 1 Oxygen consumption rates (OCR) per hour and gram body wall dry weight in P. tremulus from three sampling groups (1-3) at different live-storage time in a flow-through seawater system (FT). Data are average ± SD of three consecutive measurements per sampling point. Different letters denote significant different values.
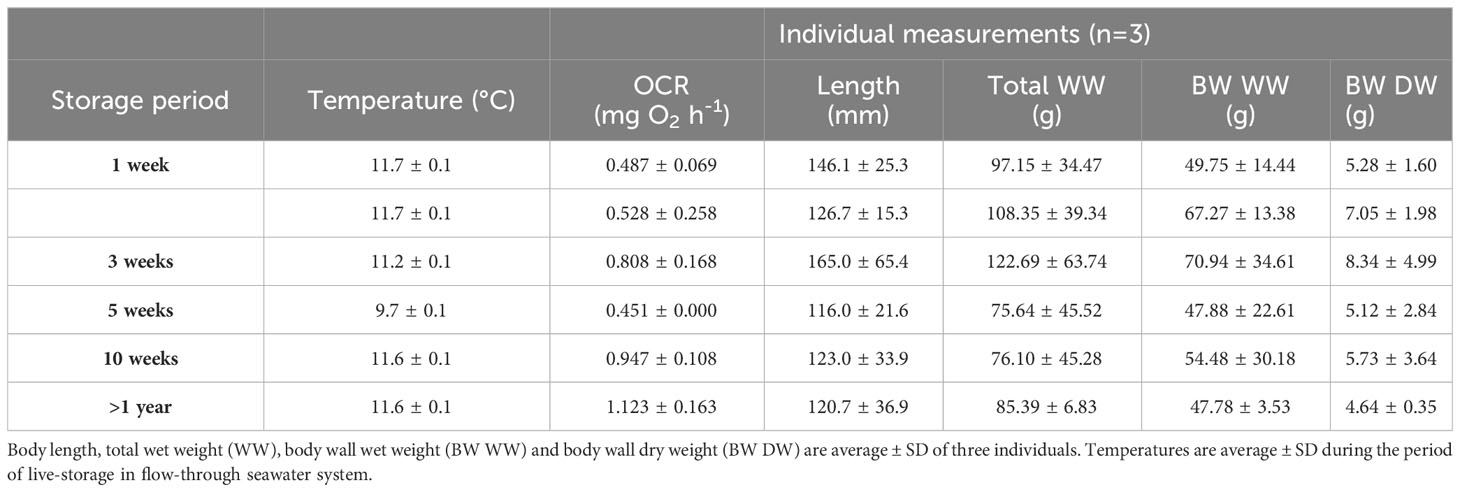
Table 1 Oxygen consumption rates (OCR) per group of three individuals are average ± SD of three consecutive measurements.
Group OCR (mg O2 h-1 for 3 individuals) showed a similar trend to that of weight specific OCR, with the highest respiration rates in sea cucumbers after medium- and long-term storage in flow-through laboratory tanks (Table 1).
The condition index (CI) showed an opposite pattern to what was found for the OCR with the highest values in FT-1 stored 1-5 weeks (in sea cucumbers (11.0-11.4 ± ~1), compared to a significant lower CI in sea cucumbers stored 10 weeks (10.23 ± 1.03), and >1 year (9.62 ± 0.48) (unequal variances t-test, p ≤0.03) (Figure 2). Although the sea cucumbers obtained in November (group 1) had a higher mean CI compared to animals caught in September (group 2) (11.13 ± 0.87 vs. 10.71 ± 1.03), the difference was not significant between groups 1 and 2 stored for 1 week (equal variances t-test, p = 0.22). No significant change in CI was seen in group 1 during 5 weeks of storage (Figure 2, FT-1, one-way ANOVA, p=0.577), or for group 2 after 10 weeks of storage (equal variances t-test, p = 0.11). The ash content (% AW) related to total wet weight (WW) did not differ significantly between the sea cucumber groups or with storage time (ANOVA, p >0.05).
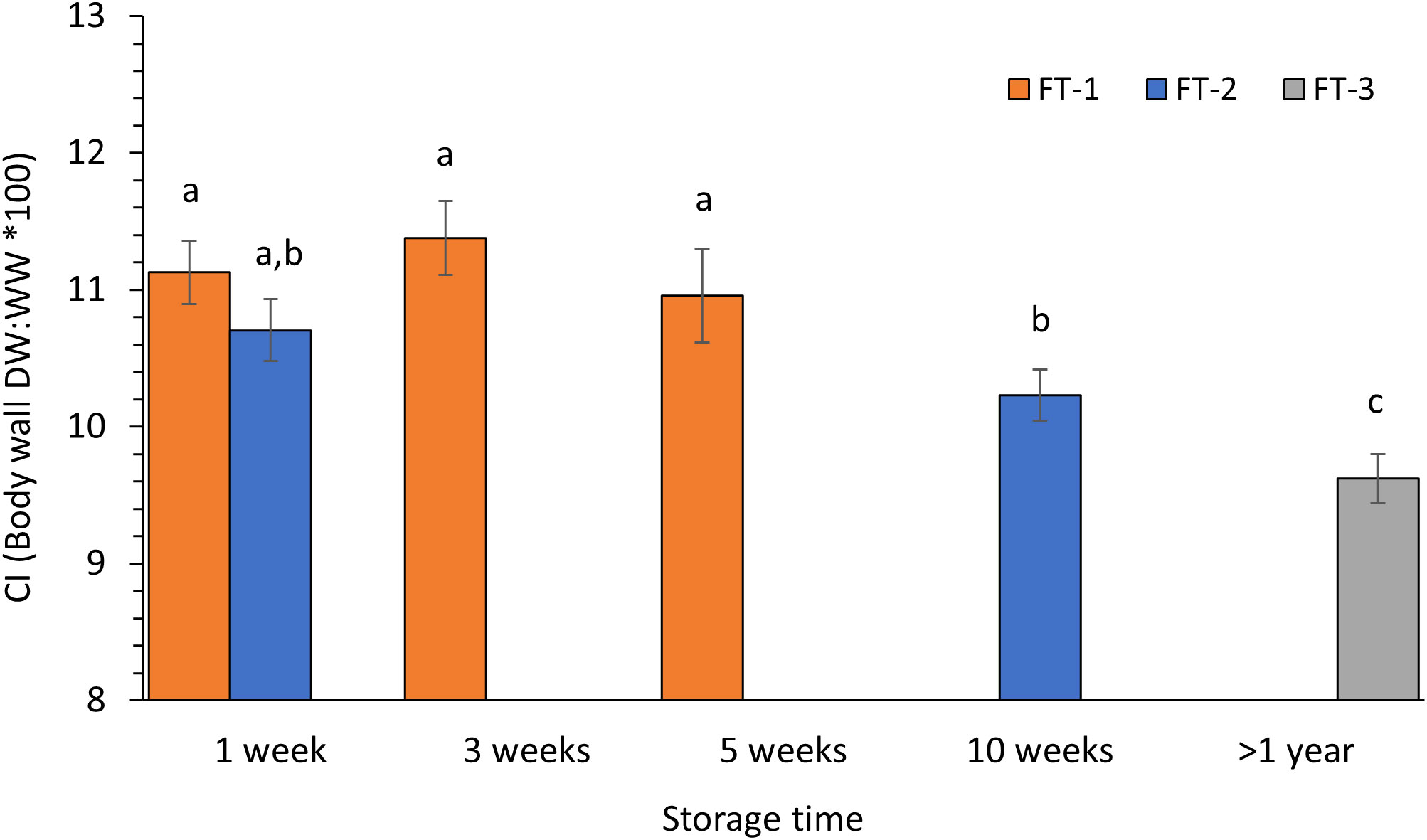
Figure 2 Condition index (CI) of P. tremulus from three sampling groups (1-3) at different live-storage time in flow-through seawater system (FT). Data are average ± SE (group 1 n=14, group 2 n= 21, group 3 n=7). Different letters denote significant different values.
3.2 Effect of temperature during short-term live storage
Of the three temperatures investigated in the short-term live storage experiment, only measurements conducted at the lowest temperature (4°C) stood out, with significantly different oxygen consumption rate (OCR) and condition index (CI). After 2 weeks of storage (T1), animals kept at 4°C had significantly lower weight specific OCR than animals kept at 12°C (Figure 3, unequal variances t-test, p=0.011). After 4 weeks (T2), this difference became even more distinctive, with significantly higher weight specific OCR measured in individuals kept at both, 8 and 12°C (one-way ANOVA, p<0.001). While oxygen demand at 4°C had almost halved compared to initial measurements at T0, consumption at 8 and 12°C remained unchanged at about 31 µg O2 h-1g-1 dry weight throughout the four-week period. Over the course of time, a general reduction in dispersion was observed for OCR measurements in all temperature treatments (Figure 3, Table 2).
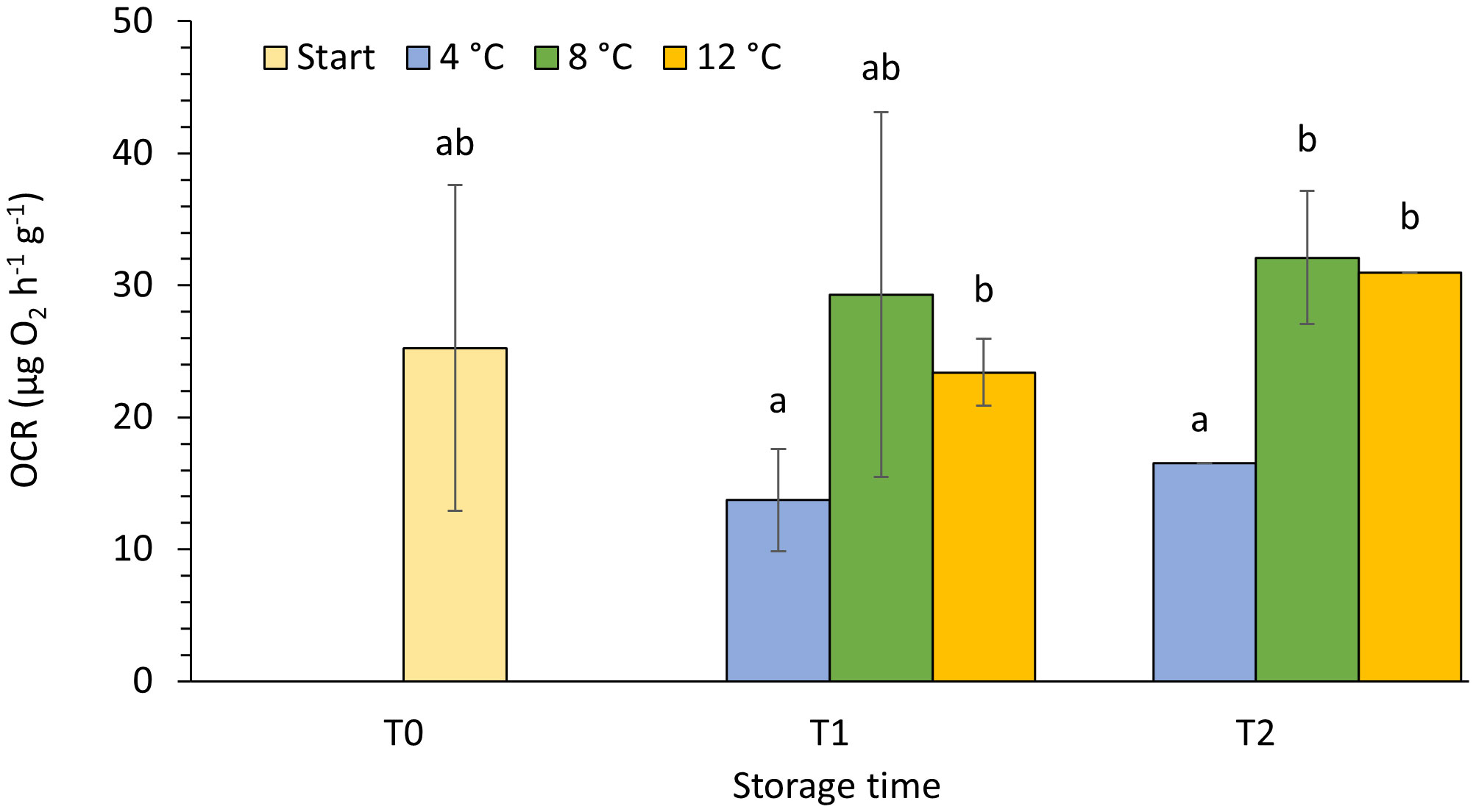
Figure 3 Oxygen consumption rates (OCR) per gram body wall dry weight and hour in P. tremulus at experimental start (T0), after 2 (T1) and 4 (T2) weeks live-storage at three different temperatures (4, 8 and 12°C). Data are average ± SD of three consecutive measurements per sampling point. Different letters denote significant different values.
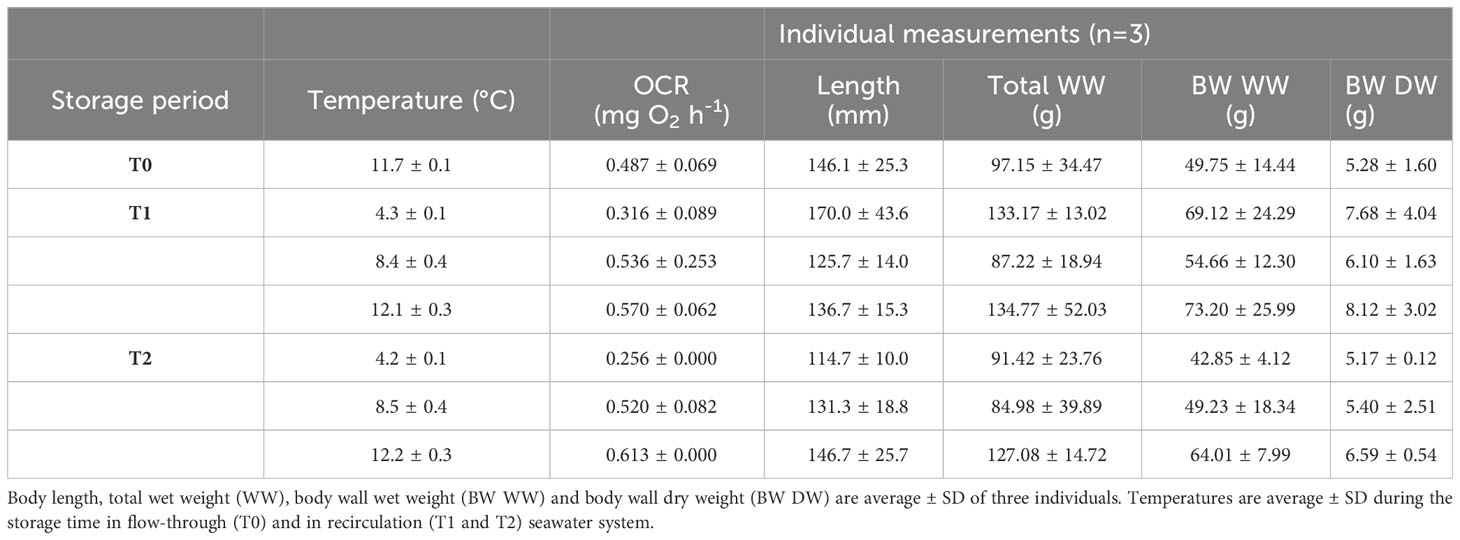
Table 2 Oxygen consumption rate (OCR) per group of three individuals are average ± SD of three consecutive measurements.
Although there was no significant change in condition index (CI) within temperature treatments (one-way ANOVA, p>0.213) over the course of the four-week experimental period, sea cucumbers kept at 4°C were in a considerable better condition at T2 than their conspecifics kept at 8 and 12°C (unequal variances t-test, p ≤ 0.015) (Figure 4). The condition indices of all three temperature groups did not test significantly different from each other at T1 (one-way ANOVA, p=0.710) and neither did the CI of the 8 and 12°C cohorts at the end of the experimental period at T2 (unequal variances t-test, p=0.46). Nevertheless, there is a trend suggesting an inverse correlation between CI and temperature that becomes more pronounced over time (Figure 4).
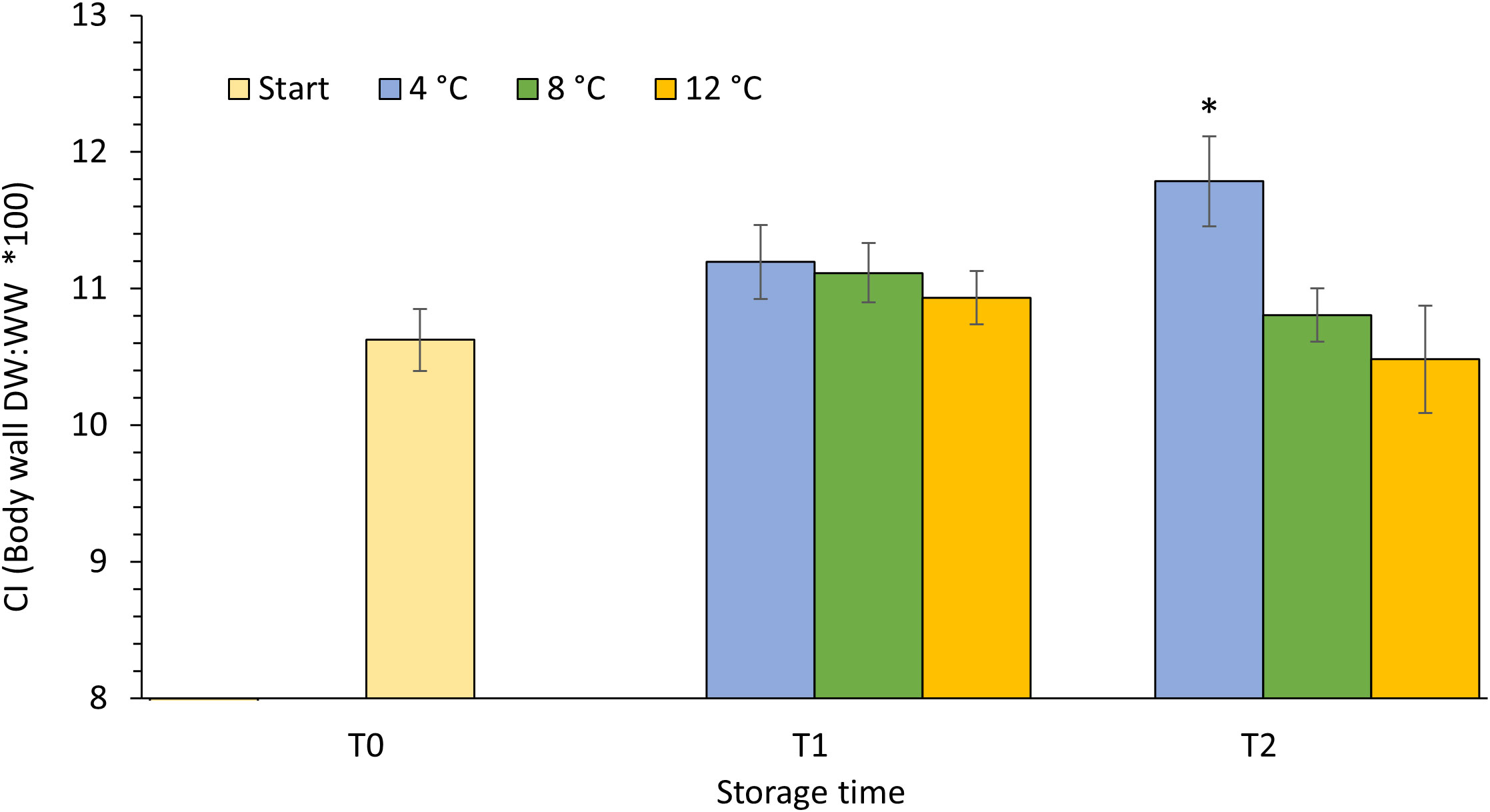
Figure 4 Condition index (CI) of P. tremulus kept at different temperatures (4, 8 and 12°C). Sampling time at experimental start (T0), and after two (T1) and four (T2) weeks in recirculation system. Data are average ± SE (n=21). Asterisk (*) denotes significant different value (4°C, T2) between temperature groups.
4 Discussion
Elevated oxygen consumption rates (OCR) were found in connection with prolonged storage period in the flow-through system but were also associated with higher water temperatures. The highest OCR values measured in this study (~81 µg O2 h-1 g-1) were associated with animals that had been fed regularly and stored for more than a year, but also animals kept without supplementary feed had a significantly higher oxygen demand (~55 µg O2 h-1 g-1 vs. ~31 µg O2 h-1 g-1) after 10 weeks of storage when compared with animals just brought in to the lab. While OCR increased with storage period, a progressive decrease in condition index (CI) was observed over time, suggesting a loss of body mass, even for the animals that had been fed. This taken into account, elevated oxygen demand under otherwise identical environmental conditions may be an indicator for cumulative stress. It is however hard to determine whether elevated OCR simply represent an artifact due to the animal’s increased sensitivity to acute disturbances, like handling in connection with respiration measurements, or if these are an expression of a more permanently raised metabolic state. There are many factors that may contribute and act as stressors. For instance, immune response to acute thermal stress comes at a higher metabolic cost (Gonzalez-Aravena et al., 2015). Furthermore, it is conceivable that the observed increased oxygen demand in connection with long-term storage, could be connected to undernourishment (sub-optimal nutrition), or starvation as found in other ectotherm species (e.g. Comoglio et al., 2005).
However, potential stressors under artificial environmental conditions in captivity often interact and are very difficult to assess, especially due to the fragmentary knowledge regarding habitat requirements and physiological mechanisms connected to internal processes and biotic and abiotic environmental factors in the wild. Previously conducted feeding experiments in our lab indicate that growth of P. tremulus is highest in spring-summer, whereas it is reduced or even negative during autumn-winter months (Christophersen and Sunde, 2022). Conditioning of P. tremulus broodstock in captivity has so far also been unsuccessful as rebuilding of the gonads after spawning has not yet been accomplished (Christophersen et al., 2023b), indicating that nutritional requirements of this species have not been met and are still largely unknown.
As sea temperatures rise due to global warming, natural cycles of nutrient availability and temperature could be disturbed and thereby have a negative effect on the fitness of wild stocks of P. tremulus. Since temperature may affect sea cucumber feeding behaviour and growth (Yang et al., 2005; Ji et al., 2008) habitat depth may influence oxygen demand between populations. Although most abundant in the deep-sea down to 800 m (Billett, 1988; Kjerstad et al., 2015), P. tremulus are also obtained from shallower parts as for example the Oslofjord (60-85 m) in Norway (Jespersen and Lützen, 1971) and the Gullmar Fjord (60 m) on the Swedish south-west coast (Holland, 1981). Since it is documented that individuals also occur at shallow depths up to 18 m (Ludwig, 1900), one could expect P. tremulus to be well adapted to a wide range of temperatures. Based on observations from the Gullmar Fjord, Schagerström et al. (2022) speculated that shallow areas (25-35 m) are visited in connection with spawning, potentially adding a seasonal component to the species` general temperature tolerance level. To identify temperature thresholds and the optimal range with respect to seasonal requirements is not just of great importance from a conservational standpoint, but also key for handling, holding, and rearing of a species with future economic potential.
This study showed that short-term storage of P. tremulus at a temperature of 4°C had a significant effect on both OCR and CI. After four weeks, sea cucumbers kept at 4°C had a significantly higher CI than individuals kept at 8 or 12°C. These observations are consistent with the fact that physiological processes run slower at lower temperatures, thereby requiring less oxygen. Body reserves are not used up as quickly, and biomass loss is reduced. Since our experiments were conducted outside the natural spawning season in June-July (Christophersen et al., 2020), we can exclude varying gonad and intestinal weight proportions as a contributing factor to differences in CI. An evaluation of the gonad status, conducted at the end of the experiments, found only small and underdeveloped gonads in most individuals. Dissection, performed at all sampling points, further showed that 77% of the experimental animals had intact intestines and respiratory trees. Gonad development, spawning or evisceration as influencing factors can therefore largely be disregarded.
As noted by Hughes et al. (2011), gaining knowledge on physiological rates, especially on a species` oxygen consumption, facilitates the modelling of its contribution to energy and carbon flow in deep-sea food webs (Rowe et al., 2008; Soetaert and van Oevelen, 2009). The measurement of OCR has long been utilized as a practical means to assess an organism’s metabolic and organic carbon consumption rate (Schmidt-Nielsen, 1990; Burggren and Roberts, 1991; Brey, 2010). However, gathering such physiological data from deep-water species presents certain challenges. It is important to acknowledge that retrieving deep-sea specimens to the surface may not be entirely without consequences, as their physiological and biochemical systems are sensitive to changes in pressure and temperature (Somero, 1998). On the other hand, echinoderms, unlike other deep-water species, do not exhibit a decrease in OCR with increasing habitat depth; instead, it remains relatively constant (Hughes et al., 2011). The measurements in this study also appear to be generally comparable to OCR measurements obtained in situ for three different deep-sea holothuroids from the northeastern Atlantic (Hughes et al., 2011), suggesting that they may also correspond to those occurring in P. tremulus in nature. Measurements conducted at 4°C after two weeks (T1) of acclimation in the lab revealed a mean consumption of 0.32 mg O2 per group of three individuals per hour (mean 133 g wet weight per individual). Hughes et al. (2011) found an in situ OCR of 0.93 mg O2 per individual (130 g wet weight) in Zygothuria lactea at 4.2°C, and 0.31 and 0.04 mg O2 per individ and hour at 2.6°C in Benthodytes gosarsi (mean 1149.8 g wet weight) and Peniagone azorica (mean 51.3 g wet weight), respectively.
Studies on oxygen consumption in P. tremulus measured in the laboratory are almost equally sparse as literature on in situ measurements. Fox (1936) might have been the first and one of the few to measure oxygen consumption in P. tremulus. He conducted measurements at 6°C and measured individual rates that ranged from 0.7 up to 2.3 µg O2 h-1 g WW-1. The mean OCR of the one individual (130 g wet weight) measured by Fox (1936) was 1.8 µg O2 h-1 g WW-1. Using total wet weight as reference, our converted values translate to average 1.65 µg O2 h-1 g WW-1 for individuals kept at 12°C and average 0.86 µg O2 h-1 g WW-1 for individuals kept at 4°C. Given the high intraspecific variance that occurs in our data set as well as in data sets gathered by Fox (1936) and Hughes et al. (2011) and which seems to be typical for aquatic and marine ectotherms (Patterson, 1992) – our measurements fit well within the range. Most publications available on physiological rates in holothuroids from the Atlantic report their results in relation to wet weight as standardization reference. To our knowledge, there is only one study where the authors chose to standardize OCR on a dry weight basis, arguing that otherwise any closer comparison of rates becomes impossible due to the high fluctuation of perivisceral fluid (Newell and Courtney, 1965). For the same reason, we decided to calculate OCR standardized on dry weight basis in our study. Newell and Courtney (1965) measured consumption rates of 23.14 to 66.43 µg O2 h-1 g DW-1 in adult Holothuria forskali at 17°C, compared to our measurements of 15 µg O2 h-1 g DW-1 in adult P. tremulus at 4°C and 27 µg O2 h-1 g DW-1 at 12°C.
While our study only contributes a tiny piece of the puzzle to the overall understanding of P. tremulus’ physiology, the results may still be useful in various contexts. Notably, knowledge regarding the species’ oxygen demand at different temperatures is crucial within any aquaculture setting. Furthermore, these data can be a useful supplement for the modelling of dynamic energy budgets (DEB), where physiological processes of individual organisms, such as ingestion, assimilation, respiration, growth, and reproduction, are linked together in a single framework to assess the species’ role in the ecosystem (van der Meer, 2006), among other things. This would also facilitate a better understanding of how climate change may impact these vulnerable yet important components of our benthic ecosystems. However, more data is needed on physiological relationships in P. tremulus in general and oxygen consumption in particular, both obtained in the field and laboratory, regarding its optimum range as well as tolerance levels.
Since P. tremulus is considered to be of commercial interest for Asian markets (Kjerstad et al., 2015), there has been increased interest in understanding the ecology and biology of the species, in order to investigate whether there is potential to develop a regulated fishery as well as an aquaculture industry in Norway (Landes et al., 2019; Christophersen and Sunde, 2022; Christophersen et al., 2023a). Worldwide, several sea cucumber species are exploited and partly overexploited with global catches exceeding 59 000 tonnes in 2019 (FAO, 2021). There are also examples for the overexploitation of wild sea cucumber species in European waters as for instance in the Mediterranean Sea and the North-East Atlantic Ocean where new target species may be at future risk (González-Wangüemert et al., 2018). The cultivation of sea cucumbers through aquaculture presents a potential solution to alleviate the strain on local wild populations. Furthermore, the inclusion of sea cucumber aquaculture in IMTA systems offers promising prospects for minimizing organic waste generated by other aquaculture practices, such as fish and shrimp farming (Dumalan et al., 2019; Neofitou et al., 2019; Jenzri et al., 2022). As per today, China dominates the global sea cucumber aquaculture industry, with Apostichopus japonicus accounting for an overwhelming majority (99.5%) of the production worldwide (FAO, 2021). Still, the demand is high, which increases the pressure on new target species from temperate regions (Purcell et al., 2014). Being able to understand the requirements of P. tremulus, will further the optimization of culture conditions and, in the future, the supply of products that are in demand on the international seafood market. The information acquired on oxygen consumption of P. tremulus under different conditions provides new knowledge on the biology of a yet understudied species, relevant for both ecosystem management and aquaculture.
Data availability statement
The raw data supporting the conclusions of this article will be made available by the authors, without undue reservation.
Ethics statement
The manuscript presents research on animals that do not require ethical approval for their study.
Author contributions
Conceptualization, resources, methodology and project administration, AL and GC. Performance of experimental work and formal analysis, AL, GC and JS. Validation, JS. Writing—review and editing, AL, GC and JS. All authors contributed to the article and approved the submitted version.
Funding
This research was funded by the research Council of Norway, project number 327148, and Møreforsking AS.
Conflict of interest
The authors declare that the research was conducted in the absence of any commercial or financial relationships that could be construed as a potential conflict of interest.
Publisher’s note
All claims expressed in this article are solely those of the authors and do not necessarily represent those of their affiliated organizations, or those of the publisher, the editors and the reviewers. Any product that may be evaluated in this article, or claim that may be made by its manufacturer, is not guaranteed or endorsed by the publisher.
References
Astall C. M., Jones M. B. (1991). Respiration and biometry in the sea cucumber Holothuria forskali. J. Mar. Biol. Assoc. UK 71 (1), 73–81. doi: 10.1017/S0025315400037401
Billett D. S. M. (1988). The ecology of deep-sea holothurians (UK: PhD thesis. The University of Southampton). Available at: https://eprints.soton.ac.uk/384501/1/88080916.pdf.
Brey T. (2010). An empirical model for estimating aquatic invertebrate respiration. Methods Ecol. Evol. 1, 92–101. doi: 10.1111/j.2041-210X.2009.00008.x
Brockington S., Clarke A. (2001). The relative influence of temperature and food on the metabolism of a marine invertebrate. J. Exp. Mar. Biol. Ecol. 258, 87–99. doi: 10.1016/S0022-0981(00)00347-6
Burggren W., Roberts J. (1991). “Respiration and metabolism,” in Environmental and Metabolic Animal Physiology. Ed. Prosser C. L. (New York, USA: Wiley-Liss, Inc.), 353–435.
Christensen A. B., Colacino J. M. (2000). Respiration in the burrowing brittlestar, Hemipholis elongata Say (Echinodermata, Ophiuroidea): a study of the effects of environmental variables on oxygen uptake. Comp. Biochem. Physiol. 127A, 201–213. doi: 10.1016/S1095-6433(00)00254-3
Christophersen G., Bjørkevoll I., Bakke S., Kjerstad M. (2020). Reproductive cycle of the red sea cucumber, Parastichopus tremulus (Gunnerus, 1767), from western Norway. Mar. Biol. Res. 16 (6-7), 423–430. doi: 10.1080/17451000.2020.1781188
Christophersen G., Jonassson J. P., Sunde J. (2023a). “Sea cucumber fisheries in Northern Europe,” in The World of sea Cucumbers. Eds. Mercier A., J.-F. Hamel A., Suhrbier C., Pearce G. E. (Academic Press, London, UK). doi: 10.1016/B978-0-323-95377-1.00051-5
Christophersen G., Meisal R., Sunde J. (2023b). Realising the reproductive potential of Parastichopus tremulus in aquaculture. SPC Beche-de-mer Inf. Bull. 43, 23–31.
Christophersen G., Sunde J. (2022). The challenge of economically viable aquaculture of the cold-water sea cucumber Parastichopus tremulus in Norway. SPC Beche-de-mer Inf. Bull. 42, 65–72.
Comoglio L., Smolko L., Amin O. (2005). Effects of starvation on oxygen consumption, ammonia excretion and biochemical composition of the hepatopancreas on adult males of the False Southern King crab Paralomis granulosa (Crustacea, Decapoda). Comp. Biochem. Physiol. B Biochem. Mol. Biol. 140 (3), 411–416. doi: 10.1016/j.cbpc.2004.11.003
Dimock R. V. (1977). Effects of evisceration on oxygen consumption by Stichopus parvimensis Clark (Echinodermata: Holothuroidea). J. Exp. Mar. Biol. Ecol. 28 (2), 125–132. doi: 10.1016/0022-0981(77)90111-3
Dumalan R. J. P., Bondoc K. G. V., Juinio-Meñez M. A. (2019). Grow-out culture trial of sandfish Holothuria scabra in pens near a mariculture-impacted area. Aquaculture 507, 481–492. doi: 10.1016/j.aquaculture.2019.04.045
Emblemsvåg M., Werner K. M., Núñez-Riboni I., Frelat R., Christensen H. T., Fock H. O., et al. (2022). Deep demersal fish communities respond rapidly to warming in a frontal region between Arctic and Atlantic waters. Global Change Biol. 28 (9), 1–12. doi: 10.1111/gcb.16113
Fankboner P. V., Cameron J. L. (1985). Seasonal atrophy of the visceral organs in a sea cucumber. Can. J. Zool. 63 (12), 2888–2892. doi: 10.1139/z85-432
Farmanfarmaian A. (1966). “The respiratory physiology of echinoderms,” in Physiology of Echinodermata. Ed. Boolootian R. A. (New York, USA: Interscience Publishers), 245–300.
Féral J.-P., Magniez P. (1988). “Relationship between rates of oxygen consumption and somatic and gonadal size in the subantarctic echinoid Abatus cordatus from Kerguelen,” in 6th International Echinoderm Congress. Eds. Burke R. D., Mladenov P. V., Lambert P., Parsley R. L. (Rotterdam, Netherlands: Balkema), 581–587.
Fox H. M. (1936). The activity and metabolism of poikilothermal animals in different latitudes. I. Proc. Zool. Soc Lond. 106 (4), 945–955. doi: 10.1111/j.1469-7998.1936.tb06295.x
Fraser K. P. P., Peck L. S., Clarke A. (2004). Protein synthesis, RNAconcentrations, nitrogen excretion, and metabolism vary seasonally in the Antarctic holothurian Heterocucumis steineni (Ludwig 1898). Physiol. Biochem. Zool. 77, 556–569. doi: 10.1086/420949
Gao F., Yang H. (2015). ““Anatomy,” in The Sea Cucumber Apostichopus japonicus. History, Biology and Aquaculture. Eds. Yang H., Hamel J.-F., Mercier A. (London, UK: Academic Press), 53–76. doi: 10.1016/B978-0-12-799953-1.00004-0
Giese A. C., Farmanfarmaian A., Hilden S., Doezema P. (1966). Respiration during the reproductive cycle in the sea urchin, Strongylocentrotus purpuratus. Biol. Bull. 130, 192–201. doi: 10.2307/1539696
Gonzalez-Aravena M., Perez-Troncoso C., Urtubia R., MaChado Cunha da Silva J. R., Mercado L., Lorgeril J., et al. (2015). Immune response of the Antarctic sea urchin Sterechinus neumayeri: cellular, molecular and physiological approach. Rev. Biol. Trop. 63 (Suppl. 2), 309–320. doi: 10.15517/rbt.v63i2.23165
González-Wangüemert M., Domínguez-Godino J. A., Cánovas F. (2018). The fast development of sea cucumber fisheries in the Mediterranean and NE Atlantic waters: from a new marine resource to its over-exploitation. Ocean Coast. Manage. 151, 165–177. doi: 10.1016/j.ocecoaman.2017.10.002
Grieg J. A. (1921). “Echinodermata,” in Report of the scientific results of the Michael Sars North Atlantic Deep Sea Expedition 1910, vol. III. (Bergen, Norway: The Trustees of the Bergen Museum).
Hamel J. F., Eeckhaut I., Conand C., Sun J., Caulier G., Mercier A. (2022). “Global knowledge on the commercial sea cucumber Holothuria scabra,” in Advances in marine biology, vol. 91. (London, UK: Academic Press), 1–286.
Hauksson E. (1977). Ernæringsøkologiske undersøkelser av Stichopus tremulus (Gunnerus), en detritus-etende holothuroid. M.Sc (Norway: University of Bergen).
Hauksson E. (1979). Feeding biology of Stichopus tremulus, a deposit-feeding holothurian. Sarsia 64 (3), 155–160. doi: 10.1080/00364827.1979.10411376
Holland N. D. (1981). Electron microscopic study of development in a sea cucumber, Stichopus tremulus (Holothuroidea), from unfertilized egg through hatched blastula. Acta Zool. 62 (2), 89–111. doi: 10.1111/j.1463-6395.1981.tb00618.x
Hughes S. J. M., Ruhl H. A., Hawkins L. E., Hauton C., Boorman B., Billett D. S. (2011). Deep-sea echinoderm oxygen consumption rates and an interclass comparison of metabolic rates in Asteroidea, Crinoidea, Echinoidea, Holothuroidea and Ophiuroidea. J. Exp.Biol. 214 (15), 2512–2521. doi: 10.1242/jeb.055954
Idrisi N., Capo T. R., Serafy J. E. (2003). Postmetamorphic growth and metabolism of long-spined black sea urchin (Diadema antillarum) reared in the laboratory. Mar. Freshw. Behav. Physiol. 36, 87–95. doi: 10.1080/1023624031000140003
Jenzri M., Gharred C., Bouraoui Z., Guerbej H., Jebali J., Gharred T. (2022). Evisceration of Holothuria poli by mechanical, chemical and hypoxia stress methods and its bioremediation potentials for the pisciculture wastewater. Aquac. Res. 53 (9), 3309–3317. doi: 10.1111/are.15838
Jespersen A., Lützen J. (1971). On the ecology of the aspidochirote sea cucumber Stichopus tremulus (Gunnerus). Norw. J. Zool. 19, 117–132.
Ji T., Dong Y., Dong S. (2008). Growth and physiological responses in the sea cucumber, Apostichopus japonicus Selenka: Aestivation and temperature. Aquaculture 283 (1–4), 180–187. doi: 10.1016/j.aquaculture.2008.07.006
Kjerstad M., Ringvold H., Søvik G., Knott E. K., Thangstad T. H. (2015). “Preliminary study on the utilisation of Norwegian red sea cucumber, Parastichopus tremulus (Gunnerus, 1767) (Holothuroidea, Echinodermata), from Norwegian waters: Resource, biology and market,” in Blue Bio-resources. Eds. Gundersen A. C., Velle L. G. (Norway: Orkana Akademisk), 109–132.
Kodama M., Sumbing J. G., Lebata-Ramos M. J. H., Watanabe S. (2015). Metabolic rate characteristics and sediment cleaning potential of the tropical sea cucumber Holothuria scabra. Jpn. Agric. Res. Q. 49 (1), 79–84. doi: 10.6090/jarq.49.79
Landes A. M., Sunde J., Christophersen G. (2019). “Atlantic sea cucumber species in the spotlight - prospects for Norwegian aquaculture,” in International perspectives on regional research and practice. Eds. Akslen-Hoel L. K., Egilsson B. (Norway: Orkana Akademisk), 1–23.
Lawrence J. M., Lane J. M. (1982). “The utilization of nutrients by post-metamorphic echinoderms,” in Echinoderm Nutrition. Eds. Jangoux M., Lawrence J. M.(Rotterdam, Netherlands: A.A. Balkema), 331–371.
Ludwig H. (1900). Arktische und subarktische Holothurien Vol. 133 (Jena, Germany: ZFauna Arctica, 1.S. Gustav Fischer Verlag), 178.
Madsen F. J., Hansen B. (1994). Echinodermata, Holothurioidea. Marine Invertebrates of Scandinavia Number 9 (Norway: Scandinavian University Press).
MNHN, Chagnoux S. (2023). The echinoderm collection (IE) of the Muséum national d’Histoire naturelle (MNHN – Paris). Version 75.297. MNHN – Museum national d’Histoire naturelle. Occurrence dataset https://doi.org/10.15468/tp2nxo accessed via GBIF.org on 2023-02-02. 2023
Neofitou N., Lolas A., Ballios I., Skordas K., Tziantziou L., Vafidis D. (2019). Contribution of sea cucumber Holothuria tubulosa on organic load reduction from fish farming operation. Aquaculture 501, 97–103. doi: 10.1016/j.aquaculture.2018.10.071
Newell R. C., Courtney W. A. M. (1965). Respiratory movements in Holothuria forskali Delle chiaje. J. Exp.Biol 42 (1), 45–57. doi: 10.1242/jeb.42.1.45
Nilsen J. E., Falck E. (2006). Variations of mixed layer properties in the Norwegian Sea for the period 1948-1999. Prog. Oceanography 70 (1), 58–90. doi: 10.1016/j.pocean.2006.03.014
Otero-Villanueva M. D. M., Kelly M. S., Burnell G. (2004). How diet influences energy partitioning in the regular echinoid Psammechinus miliaris; constructing an energy budget. J. Exp. Mar. Biol. Ecol. 304, 159–181. doi: 10.1016/j.jembe.2003.12.002
Patterson M. R. (1992). A mass transfer explanation of metabolic scaling relations in some aquatic invertebrates and algae. Science 255, 1421–1423. doi: 10.1126/science.255.5050.1421
Pawson D. L., Pawson D. J., King R. A. (2011). A taxonomic guide to the Echinodermata of the South Atlantic Bight, USA: 1. Sea cucumbers (Echinodermata: Holothuroidea). Zootaxa 2449 (1), 1–48. doi: 10.11646/zootaxa.2449.1.1
Purcell S. W. (2014). Value, market preferences and trade of beche-de-mer from Pacific Island sea cucumbers. PloS One 9, e95075. doi: 10.1371/journal.pone.0095075
Purcell S. W., Polidoro B. A., Hamel J.-F., Gamboa R. U., Mercier A. (2014). The cost of being valuable: predictors of extinction risk in marine invertebrates exploited as luxury seafood. Proc. R. Soc B 281, 20133296. doi: 10.1098/rspb.2013.3296
Rowe G. T., Wei C. L., Nunnally C., Haedrich R., Montagna P., Baguley J. G., et al. (2008). Comparative biomass structure and estimated carbon flow in food webs in the deep Gulf of Mexico. Deep Sea Res. Part 2 Top. Stud. Oceanogr. 55, 2699–2711. doi: 10.1016/j.dsr2.2008.07.020
Ru X., Zhang L., Liu S., Yang H. (2020). Plasticity of respiratory function accommodates high oxygen demand in breeding sea cucumbers. Front. Physiol. 11. doi: 10.3389/fphys.2020.00283
Ru X., Zhang L., Yang H. (2021). Plasticity of locomotor activity permits energy homeostasis during reproduction in a female sea cucumber. Front. Mar. Sci. 8, 1–9. doi: 10.3389/fmars.2021.748571
Schagerström E., Christophersen G., Sunde J., Bakke S., Matusse N. R., Dupont S., et al. (2022). Controlled spawning and rearing of the sea cucumber, Parastichopus tremulus. J. World Aquac. Soc 53 (1), 224–240. doi: 10.1111/jwas.12816
Schmidt-Nielsen K. (1990). Animal physiology: adaptation and environment (Cambridge, UK: Cambridge University Press). 4th ed.
Soetaert K., van Oevelen D. (2009). Modeling food web interactions in benthic deep-sea ecosystems. A practical guide. Oceanography 22, 128–143. doi: 10.5670/oceanog.2009.13
Somero G. N. (1998). “Adaptation to cold and depth: contrasts between polar and deep-sea animals,” in Cold Ocean Physiology. Eds. Portner H. O., Playle R. C. (Cambridge, UK: Cambridge University Press), 33–57.
Spicer J. I. (1995). Oxygen and acid-base status of the sea urchin Psammechinus miliaris during environmental hypoxia. Mar. Biol. 124, 71–76. doi: 10.1007/BF00349148
Sunde J., Christophersen G. (2023). Appetite in captivity – feeding studies of the red sea cucumber Parastichopus tremulus. Front. Mar. Sci. 9. doi: 10.3389/fmars.2022.1052968
Swan E. F. (1961). Seasonal evisceration in the sea cucumber, Parastichopus californicus (Stimpson). Nature 133 (3458), 1078–1079. doi: 10.1126/science.133.3458.107
Talbot T. D., Lawrence J. M. (2002). The effect of salinity on respiration, excretion, regeneration and production in Ophiophragmus filograneus (Echinodermata: Ophiuroidea). J. Exp. Mar. Biol. Ecol. 275, 1–14. doi: 10.1016/S0022-0981(02)00208-3
Vahl O. (1984). The relationship between specific dynamic action (SDA) and growth in the common starfish, Asterias rubens L. Oecologia 61, 122–125. doi: 10.1007/BF00379097
van der Meer J. (2006). An introduction to Dynamic Energy Budget (DEB) models with special emphasis on parameter estimation. J. Sea Res. 56 (2), 85–102. doi: 10.1016/j.seares.2006.03.001
Vismann B., Hagerman L. (1996). Recovery from hypoxia with and without sulfide in Saduria entomon: oxygen debt, reduced sulfur and anaerobic metabolites. Mar. Ecol. Prog. Ser. 143, 131–139. doi: 10.3354/meps143131
Weinrauch A. M., Blewett T. A. (2019). Anoxia tolerance in the sea cucumbers Parastichopus californicus and Cucumaria miniata reflects habitat use. J. Exp. Mar. Biol. Ecol. 520, 151203. doi: 10.1016/j.jembe.2019.151203
Yang H., Hamel J.-F., Mercier A. (2015). The Sea Cucumber Apostichopus japonicus: History, Biology and Aquaculture (Academic Press: Elsevier). doi: 10.1016/B978-0-12-799953-100007-6
Yang H., Yuan X., Zhou Y., Mao Y., Zhang T., Liu Y. (2005). Effects of body size and water temperature on food consumption and growth in the sea cucumber Apostichopus japonicus (Selenka) with special reference to aestivation. Aquac. Res. 36 (11), 1085–1092. doi: 10.1111/j.1365-2109.2005.01325.x
Yu Z., Robinson S., MacDonald B., Lander T., Smith C. (2020). Effect of diets on the feeding behavior and physiological properties of suspension-feeding sea cucumber Cucumaria frondosa. J. Oceanol. Limnol. 38 (3), 883–893. doi: 10.1007/s00343-019-9190-x
Keywords: sea cucumber, Holothuroidea, Parastichopus tremulus, oxygen consumption, live-storage, temperature, condition index
Citation: Landes AM, Sunde J and Christophersen G (2023) Effect of live-storage period and temperature on oxygen consumption rate in the cold-water sea cucumber Parastichopus tremulus. Front. Mar. Sci. 10:1248840. doi: 10.3389/fmars.2023.1248840
Received: 27 June 2023; Accepted: 25 August 2023;
Published: 12 September 2023.
Edited by:
Pedro M. Félix, Center for Marine and Environmental Sciences (MARE), PortugalReviewed by:
Fernando Diaz, Center for Scientific Research and Higher Education in Ensenada (CICESE), MexicoJosé Pedro Andrade, University of Algarve, Portugal
Copyright © 2023 Landes, Sunde and Christophersen. This is an open-access article distributed under the terms of the Creative Commons Attribution License (CC BY). The use, distribution or reproduction in other forums is permitted, provided the original author(s) and the copyright owner(s) are credited and that the original publication in this journal is cited, in accordance with accepted academic practice. No use, distribution or reproduction is permitted which does not comply with these terms.
*Correspondence: Gyda Christophersen, Z3lkYS5jaHJpc3RvcGhlcnNlbkBtb3JlZm9yc2tpbmcubm8=