- 1Institut of Aquatic Ecology (IEA), University of Girona (UdG), Girona, Spain
- 2Université Cote d’Azur, CNRS, ECOSEAS, Nice, France
- 3Centre for Advanced Studies of Blanes (CEAB), Spanish National Research Council (CSIC), Blanes, Spain
Fucalean algae are dominant canopy-forming species that create extensive and highly productive ecosystems in the intertidal and subtidal rocky shores of temperate seas. Regrettably, these marine forests are in decline due to various human drivers, with the Mediterranean Sea one of the most threatened areas. To design appropriate restoration strategies adapted to cope with the unavoidable change in future climate conditions, the response to climate change of the candidate species must be considered. It is important to assess how the specific life history traits of the foundational species may determine environmental requirements, and thus responses to future climate change. This knowledge will allow us to predict the potential winners and losers among the species potentially inhabiting the same areas in a future context of global climate change, providing important information to fine-tune future restoration interventions. The aim of this study was to evaluate the response of two canopy-forming species inhabiting similar upper subtidal zones but with different life history traits to a combination of anomalous high temperatures and increased UV radiation. One of the species (Ericaria crinita) was perennial, slightly exposed rocky shores and dwelling in areas where extreme temperatures can be frequent; while the other (Ericaria mediterranea) a semi-perennial species that dwells in wave-exposed zones, with seawater temperatures buffered by the high hydrodynamism. Our results show that the effects of temperature and radiation are species- (mediated by the species life history traits) and life-stage specific. High temperatures strongly affected the adults of both species, especially E. mediterranea. The germlings in addition to being very susceptible to high temperatures, were also vulnerable to UV radiation, exacerbating the impacts of temperature, especially on E. crinita recruits. Interestingly, vulnerability to climate-driven impacts was determined by the specific life history traits, with i) the species dwelling in open areas the most sensitive to warming and, ii) the perennial species the most vulnerable to UV radiation. Last, we discuss how these species-specific responses to climate-driven impacts may be key in terms of species that could foster the resistance and resilience of marine ecosystems to future climate impacts.
1 Introduction
Marine macroalgal forests dominated by canopy-forming fucalean algae create extensive, dense and highly productive ecosystems in the intertidal and subtidal rocky shores of temperate and subpolar areas (Feldmann, 1937; Schiel and Foster, 2006; Ballesteros et al., 2009). These macroalgal forests are of great ecological importance in coastal marine ecosystems as they provide numerous ecosystem services such as oxygen production and carbon sequestration (Raven, 2017), habitat, nursery and refuge provisioning for other marine species, and thus enhancing the biodiversity and complexity of the systems (Steneck et al., 2002; Thiriet et al., 2016).
Some of these marine forests are declining on a global scale due to many human-induced perturbations such as pollution and habitat destruction, especially in the Mediterranean Sea (Rodriguez-Prieto and Polo, 1996; Thibaut et al., 2005; Arevalo et al., 2007; Pinedo et al., 2013; Thibaut et al., 2015; de Caralt et al., 2020; Rindi et al., 2020), but also because of overgrazing and species invasion (Chapman, 1981; Filbee-Dexter and Scheibling, 2014; Vergés et al., 2014; Ling et al., 2015; Vergés et al., 2016). Climate change has also recently become a factor leading to the loss of macroalgae forests worldwide (e.g., Smale and Wernberg, 2013; Andrews et al., 2014; Bennett et al., 2015; Xiao et al., 2015; Verdura et al., 2021). Regrettably, once lost these forests rarely recover naturally (Coleman et al., 2008; Sales et al., 2011). Therefore, significant efforts have been made to develop effective techniques to promote macroalgal forest recovery (see reviews: Wood et al., 2019; Cebrian et al., 2021; Eger et al., 2022), from mitigation of the perturbation to active restoration of populations by enhancing the recruitment of new individuals (e.g. Gianni et al., 2013; Verdura et al., 2018; Eger et al., 2022). However, all initiatives concur that to achieve the greatest chance of success restoration actions should embrace habitat variability and support adaptation to future climate change conditions (Wood et al., 2019), since restoration success could be strongly compromised by accelerated environmental modifications associated with climate change. To this effect, the environmental drivers that favour the targeted species, together with the specific traits of the species to be restored that will best adapt to future environmental conditions, rank as the main factors contributing to the successful accomplishment of habitat restoration (Bekkby et al., 2020). Specifically, canopy-forming macroalgae forests are dominated by different foundational species with a wide range of life history traits (e.g. phenology, growth rates, life cycles) and specific environmental requirements (e.g. hydrodynamics, irradiance, temperature) that may translate into different environmental requirements (Coleman and Wernberg, 2017; Orfanidis et al., 2021) and potentially contrasting vulnerability to different climate change-derived stressors.
Some recent studies have dealt with how life history traits of macroalgae canopy species, such as vegetative reproduction (Endo et al., 2021), morphological plasticity (Supratya et al., 2020) and early life-stage (Capdevila et al., 2018; Falace et al., 2021; Monserrat et al., 2022), determine future responses to warming. Most studies have focused on the impacts of rising temperatures and marine heatwaves (MHW; e.g. Arafeh-Dalmau et al., 2019; Casado-Amezúa et al., 2019; Straub et al., 2019; Smale, 2020; Verdura et al., 2021), whereas studies on the effects of other climate change-derived impacts, such as increased exposure to ultraviolet light (UV), are still very scarce (Wernberg et al., 2012).
Response against increasing and anomalous temperatures have been traduced in physiological and phenological alterations (e.g. Bevilacqua et al., 2019; Román et al., 2020; Verdura et al., 2021), abundance changes, distribution range shifts, and even local extinctions of foundational macroalgal species (e.g. see review Arafeh-Dalmau et al., 2019; Straub et al., 2019; Thomsen et al., 2019) and thus, impacting the structure and function of the whole ecosystem (Harley et al., 2012; Schiel and Foster, 2015; Smale et al., 2019).
Exposure to UV radiation causes DNA and cellular structure damage, alters the physiology and development of aquatic organisms (especially photosynthetic species) and can even cause their death, altering the diversity of communities and ecosystems (e.g. El-Sayed et al., 1996; Häder et al., 2015). In this global warming scenario, supralittoral, intertidal and shallow subtidal seaweeds can be severely affected (Häder et al., 2015; Smale, 2020; Vanhaelewyn et al., 2020; Verdura et al., 2021), which is especially worrying in areas like the Mediterranean Sea where the intensity of warming is three times higher than the global average of the oceans, and where a stronger increase in the frequency and intensity of MHWs has been observed (e.g. Diffenbaugh et al., 2007; Vargas-Yanez et al., 2008; Garrabou et al., 2022). Furthermore, in the same area, a decrease in cloudiness is predicted (Sanchez-Lorenzo et al., 2017) with the consequent increase in UV radiation reaching the surface (Bornman et al., 2011). In this regard, knowledge of the specific species traits that enhance the resistance of fucalean macroalgae to warming, UV exposure and their combination will be of paramount importance when selecting species for conservation and restoration actions.
The aim of this study was to evaluate the response of two canopy-forming algae species inhabiting same substrate (rocky) and shallow subtidal zones (high irradiance) but subjected to different environmental conditions (e.g. hydrodynamic conditions and temperature) and with different life history traits (e.g. perennial and semi-perennial; Ballesteros, 1988; Sales and Ballesteros, 2012), to a combination of anomalous high temperatures and increased UV radiation, by means of laboratory experiments. Indeed, as the different life stages can display different vulnerabilities to the same stressor, with the young stages the most affected (e.g., Nielsen and Nielsen, 2010; de Caralt et al., 2020), this study analysed the impact of the aforementioned stressors on adults and germlings of the two species. Knowledge of the specific responses to different impacts will help answer the question as to which species are vulnerable to global change and will be necessary to develop better conservation plans and restoration actions adapted to future climatic scenarios.
2 Materials and methods
2.1 Sampling of target species
Mediterranean forests dominated by macroalgae of the order Fucales (Ochrophyta) dwell at different depths between the infralittoral and the first meters of the circalittoral (Boudouresque, 1971; Boudouresque, 1972; Ballesteros, 1988; Ballesteros, 1990a; Ballesteros, 1990b; Hereu et al., 2008; Ballesteros et al., 2009). Ericaria crinita (Duby) Molinari and Guiry (Figure 1A) and Ericaria mediterranea (Sauvageau) Molinari and Guiry (Figure 1B) are two endemic Mediterranean species that can develop highly structured assemblages in the rocky upper infralittoral and sublittoral zones (between 0 and 1 m depth approximately) (Ballesteros, 1992; Ballesteros, 2002). The two species can be found a few meters apart, although they present contrasting life history traits (Figure 1). While E. crinita prefers sheltered or semi-exposed rocky shores (Sales and Ballesteros, 2009; Sales and Ballesteros, 2010; Sales and Ballesteros, 2012), E. mediterranea dwells in wave-exposed or moderately exposed shores (Boudouresque, 1969; Ballesteros, 1988; Gómez-Garreta, 2000). Accordingly, E. crinita populations can live in areas where extreme temperatures are frequent, whereas E. mediterranea develops in open areas where seawater temperatures are buffered by the high hydrodynamism (Verdura et al., 2021). Moreover, while E. crinita is perennial, keeping its branches throughout the year, E. mediterranea is semi-perennial, keeping only the axis throughout the year and losing the branches in autumn, growing back in spring (Ballesteros, 1988; and see Figures 1E, F). In fact, compared to E. crinita, E. mediterrania is a fast- growing species and has a higher annual biomass production (Ballesteros et al., 1998; Sales and Ballesteros, 2012).
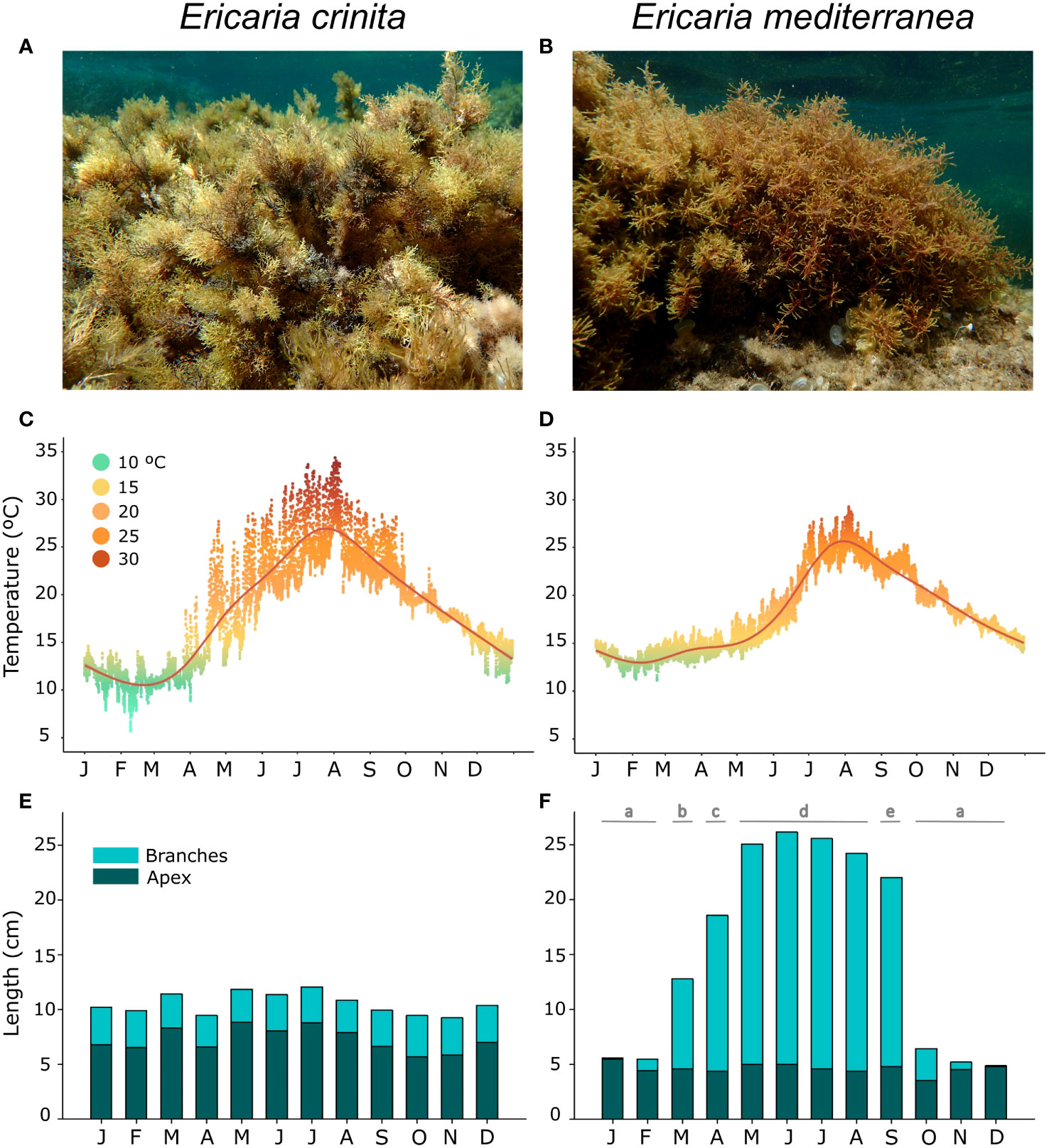
Figure 1 Differences in life history traits and environmental context of two canopy-forming species: Ericaria crinita (A), Ericaria mediterranea (B). In situ seawater temperature registered each hour for one year (2018) where a population of E. crinita (C), and E. mediterranea dwell. (D) Mean size (in cm) of the main axis over a year (2018), and total length (considering the longest branches) of the individuals of an E. crinita population (perennial species) (E) and of an E. mediterranea population (semi perennial species) (F). Significant differences among months (p-values from Tukey’s test with 95% confidence intervals) are indicated with letters.
To describe the variability on specific characteristics on thallus growth (perennial vs. semi-perennial) and the contrasting temperature conditions of the habitats where each species can inhabit, in situ temperature and thallus lengths have been monitored for both species in Costa Brava, Palamós (41°86’62.1’’N, 3°17’50.7’’E) in the North-western Mediterranean Sea. Concurrently, populations of each species were monitored monthly throughout 2018 to measure two length variables per individual, from the basal disc to the tip of the apex of the highest main axis and the length from the basal disc to the tip of the longest branch (N=80 per month, and species). Differences among total and man axis length was used as a proxy for each species’ dynamics and productivity. In situ sea temperatures were continuously recorded (hourly records) throughout 2017 and 2018 by positioning high-resolution temperature loggers (HOBO Water Temp Pro v2, ± accurate to 0.21°C) in the specific habitat dominated by each species.
Adult individuals of each species (N=36) were collected at 17th and 18th April, 2017 from subtidal rocky shores in the locality of Palamós. In Palamós, the two species (E. mediterranea and E. crinita) inhabit the same area a few meters apart but with different hydrodynamic conditions. Adult specimens were carefully removed from the rock preserving their attaching disc and transported directly to the laboratory. Once in the laboratory, epiphytes and sediment were carefully removed and the specimens were placed into tanks with natural seawater at 18°C and natural irradiance levels for three days for acclimation. Temperatures were raised at a maximum rate of two degrees per day from 18°C until the experimental temperature conditions were reached, marking the start of the experiment.
Fertile branches of E. crinita and E. mediterranea (N= 92 per each) were collected from the same two populations of the adults in late spring and immediately transported to the laboratory. Once in the laboratory, fertile branches were stored at 5°C in dark conditions for 24 hours to stimulate gametes release, before subjecting them to the different treatments.
2.2 Experimental set up
To analyse the effects of temperature and UV radiation on adults and germlings of E. crinita and E. mediterranea, six different experimental treatments were established by combining three temperature conditions (21°, 24° and 28°C) and two irradiance conditions (visible irradiance -used as a control- and visible irradiance supplemented with ultraviolet irradiance). Temperature conditions were selected to reflect normal summer water temperatures (21°C, set up as the control), maximum summer temperatures (24°C) and extremely high temperatures, experienced during MHWs in the area (28°C) (Verdura et al., 2021).
The two irradiance treatments corresponded to: i) the photosynthetic active radiation (PAR) supplying the natural intensity that these communities receive at 0 m (irradiated with 180-200 µmol m-2 s-1 of PAR, Sant, 2003); and ii) the same photosynthetic irradiance adding ultraviolet radiation (PAR+UV) since changes in stratospheric ozone, cloud cover and aerosols continue to affect surface levels of solar UVB radiation (e.g. Bais et al., 2019; Bernhard et al., 2023). The photoperiod, 14:10 (light:dark), was the natural photoperiod for the season.
For adult individuals experiments for each seaweed species were conducted in parallel for 30 days. Six replicate tanks (12L each) were used for each treatment and species, with one adult individual per tank. We therefore set up a total of 36 tanks per species. Each tank had an independent and closed-water system with air continuously pumping and with 2L of natural seawater renovation per day per tank, thus, each tank had a completely natural seawater renewal every 6 days. Each week the natural seawater was collected from the same area where the algae came from and transported and stored to the laboratory in several 60 L drums. The water temperature for each tank was regulated and kept constant (with a maximum deviation of ± 0.4 °C) at the three temperatures using temperature controllers (Teco TK 500). The supply for the absence of ultraviolet radiation treatment tanks came from PAR radiation fluorescent lamps (Master TL-D 36W/386 of Philips), while for the tanks exposed to ultraviolet radiation the same PAR fluorescent lamps were used, plus UVA and UVB radiation fluorescents (Actinic BL TL-K 40W/10-R and Ultraviolet-B 40W/12 RS SLV/25 of Philips, respectively) supplying 180-200 µmol m-2 s-1 PAR (12 W m-2) and 6-11 W m-2 UVA and 0.3-0.5 W m-2 UVB. Irradiance in each experimental condition was measured using a Li-1000 SPQA spherical sensor.
For the early life stage experiment, germlings of the two species were obtained in the laboratory facilities. For each species, three replicate culture tanks (0.5L each), each with three microscope slides on the bottom, were used for each treatment (6 treatments * 3 tanks * 3 slides). The 18 culture tanks for each species were filled with sterilised sea water and growth medium (Von Stosch modified by Guiry and Cunningham, 1984) and were maintained in culture chambers to control temperature and radiation conditions. First, to obtain the germlings, six fertile branches (of the corresponding species) were placed in each culture tank until zygote formation was detected (after three days), after which the receptacles were removed. The slides, which could be removed from the tanks for short periods of time, allowed us to observe the germlings and subsequently monitor them under a microscope throughout the experiment. The seawater with the medium was completely renewed twice a week. The temperature conditions (21°C, 24°C and 28°C) were achieved using three different growth chambers (Radiber AGP-360), each of which was subjected to PAR radiation fluorescents (Master TL-D 36W/386 of Philips) and UVA and UVB fluorescents (Actinic BL TL-K 40W/10-R and Ultraviolet-B 40W/12 RS SLV/25 of Philips, respectively) supplying 180-200 µmol m-2 s-1 PAR (12 W m-2) and 6-11 W m-2 UVA and 0.3-0.5 W m-2 UVB. Incubator chambers conditions were checked daily, to ensure the proper functioning and that temperature remained constant and correct. For the UV free treatment (PAR treatments), half the tanks in each chamber were covered with polycarbonate filter boxes (transmittance >390 nm) to cut-off UV radiation (Bischof et al., 2002). Irradiance in each experimental condition was measured using a Li-1000 SPQA spherical sensor. The photoperiod was 14:10 throughout the entire experiment, which was carried out at the facilities of the University of Girona.
2.3 Variables measured
Effects of temperature and radiation on Ericaria crinita and E. mediterranea individuals were evaluated by measuring several variables. In adult specimens, measures of biomass (wet weight in g) and optimum quantum yield (Fv/Fm) of photosystem II (PSII) were taken once a week. Changes in wet weight (expressed as a percentage) were measured for each specimen at each sampling time. The specimens were dried with absorbent paper before weighing them, following the same procedure for all the samples and sampling times. Optimum quantum yield was used as an indicator of PSII performance to assess photosynthetic efficiency. To do so, macroalgal fronds were dark incubated for 15 minutes, after which, Fv/Fm measurements were estimated by applying a saturation pulse using Pulse Amplitude Modulated Fluorometry (Diving-PAM Underwater Fluorometer, Waltz, Germany). Measuring Fv/Fm following a period of dark adaptation is a common technique for measuring stress in plants (Murchie and Lawson, 2013).
Survival and growth of viable germlings were measured under a microscope once a week. Survival of germlings was calculated counting all the live germlings attached to the microscope slides at each sampling time. Growth was assessed by measuring the maximum length of the thallus of 45 random individuals for each treatment (µm) under a microscope, and using the image analysis software ZEN 2012.
2.4 Statistical analysis
Differences among total length (square root transformation) from natural populations along a year were analysed by Linear Model (LM) with species and months as fixed factors.
The data obtained from both adult and recruit experiments were analysed by mixed-effects models (MM), which allow the inclusion of both fixed and random effects as predictor variables. Different models were fitted to analyse the effect of temperature, radiation and their interaction for each selected response variable. Specifically, wet weight variation in adults was analysed using a linear mixed model (LMM) and the Optimum Quantum Yield with a Generalised Linear Mixed Model (GLMM), with a Poisson error distribution and a logit link function. Survival and growth of germlings were analysed using a GLMM with a binomial error distribution and a logit link function, and a quasi-Poisson error distribution and a log link function, respectively. Additionally, to control for lack of independence between units of observation and to handle repeated measures over time both individual’s identity and the sampling time were considered random factors (Bolker et al., 2009; Bates et al., 2015; Harrison et al., 2018). In all the models, irradiation (2 levels), temperature (3 levels) and species (2 levels) were considered as fixed factors.
Models were fitted using the functions “lm”, “glmer”, “lmer” and “glmmPQL” from lme4 (Bates et al., 2015) and MASS packages (Ripley et al., 2013), and the Wald χ2 test was performed using the “ANOVA” function from the CAR package (Fox and Weisberg, 2019). For all the models, the assumptions of normality and equality of variance were evaluated through graphical analyses of residuals using QQ plot functions (effects, visreg, and car package). For multiple comparisons, we applied Tukey´s post-hoc tests using the “glht” function from the MULTCOMP package (Hothorn et al., 2008). All analyses were performed in the statistical environment R (R Core Team, 2019).
3 Results
3.1 Species dynamics
In situ seawater temperatures recorded over one year showed that temperatures in the habitat dominated by E. crinita, characterized by sheltered and shallow conditions, were more extreme (maximums of 31.89 °C and minimums of 8.27°C; Figure 1C). In contrast, temperatures of the habitat dominated by E. mediterranea, being a more open and exposed environment, remain within more constant ranges (maximums of 28.65 °C and minimums of 11.62 °C; Figure 1D).
The two species showed different growth dynamics (p < 0.05; Table S1), while E. crinita is characterized by constant branch lengths throughout the year (p>0.05; Table S1), with branch lengths ranging from 9.25 ± 0.18 cm in November to 12.07 ± 0.15 cm in July (Figure 1E), E. mediterranea presented significant variations among months (p<0,05) with the maximum branch lengths in June (26.17 ± 1.15 cm) and a minimum in December (4.87 ± 0.27 cm; Figure 1F).
3.2 Adult responses
UV radiation had no significant effect on the adult individuals of either of the two species (Figure 2; p > 0.005, Table S2). However, adults of both species were significantly affected by water temperatures, especially at 28°C, when biomass (especially due to the secondary and tertiary branches losses) and photosynthetic yield dropped drastically after one week for E. mediterranea and after two weeks for E. crinita (Figure 2). Vulnerability to temperature was specie dependent, while at 21°C and 24°C biomass losses (of approx. 26% and 36%, for E. crinita and E. mediterranea, respectively) were comparable among species, at 28°C biomass losses for E. mediterranea were significantly higher than for E. crinita, showing a biomass reduction of ca. 75% and 20% by day 14, respectively. At the end of the experiment (day 30) only E. crinita presented alive thallus, which displayed biomass losses of about 75%.
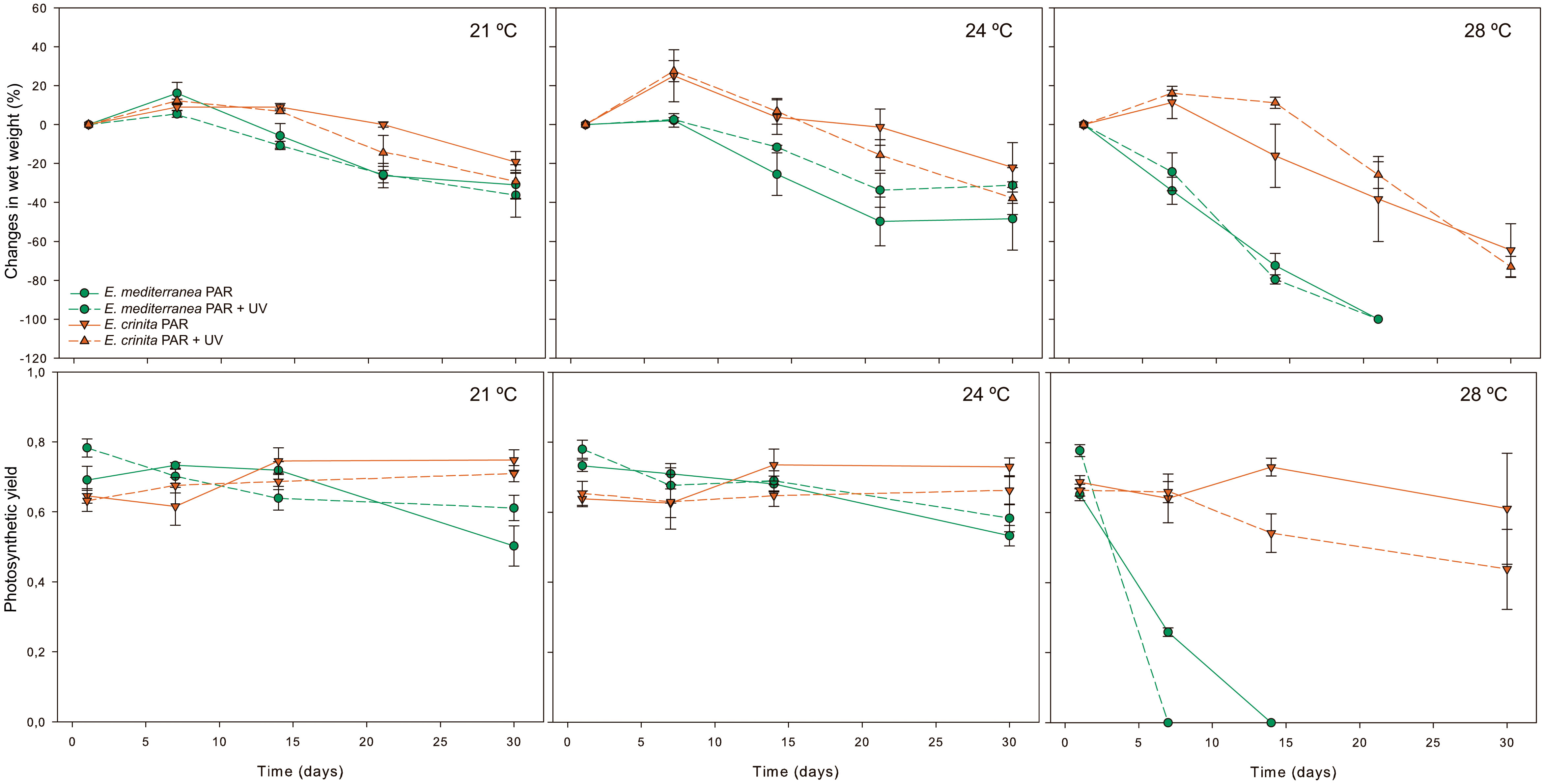
Figure 2 Biomass variation, represented as the mean percentage of wet weight variation (top plots), and photosynthetic quantum yield (bottom plots) of adult individuals of Ericaria mediterranea (green) and E. crinita (orange) over 30 days, under different temperature (21°C, 24°C and 28°C) and radiation (PAR and PAR+UV) conditions. Solid lines represent the photosynthetic active radiation (PAR), and dotted lines represent the ultraviolet radiation treatment (PAR+UV). Vertical bars are standard errors.
Similar trends were found for the photosynthetic quantum yield, whose values remained at between 0.6 and 0.8 at 21°C and 24°C for both species (p> 0.05; Table S2), in line with usual values found for healthy Cystoseira s.l. species (Abdala-Díaz et al., 2006; de Caralt et al., 2020; Verdura et al., 2021). However, at 28°C the photosynthetic quantum yield dropped by more than half (c.a. 0.258 ± 0.012) as early as day 5 in E. mediterranea (p< 0.001; Table S2), while in E. crinita it stayed high and constant under all temperature treatments throughout the experiment (c.a. >0.600; p> 0.05; Table S2).
3.3 Germling responses
Germlings of both species were significantly affected by temperature and UV radiation, and effects on both variables (survival and growth) were species- and temperature-dependent, although UV radiation always reduced germling survival (p< 0.001, Supplementary Materials Table S3; Figure 3). High temperatures (28°C) strongly reduced the survival of both species, with less than 10% survival after two weeks in all radiation conditions. UV radiation had a major impact on the E. crinita germlings, leading to zero survival after a few days of UV exposure under all temperature conditions (p < 0.05, Table S3; Figure 3). At 21 °C and 24°C, survival of the two species under PAR conditions was generally constant, with values ranging from approximately 20% to 50% for both species, while UV conditions lead to the death of almost all E. crinita germlings by days 12 and 5 for the 21° and 24 °C treatments, respectively. However, the survival of E. mediterranea under UV radiation at 21°C and 24°C was similar to the survival under PAR radiation.
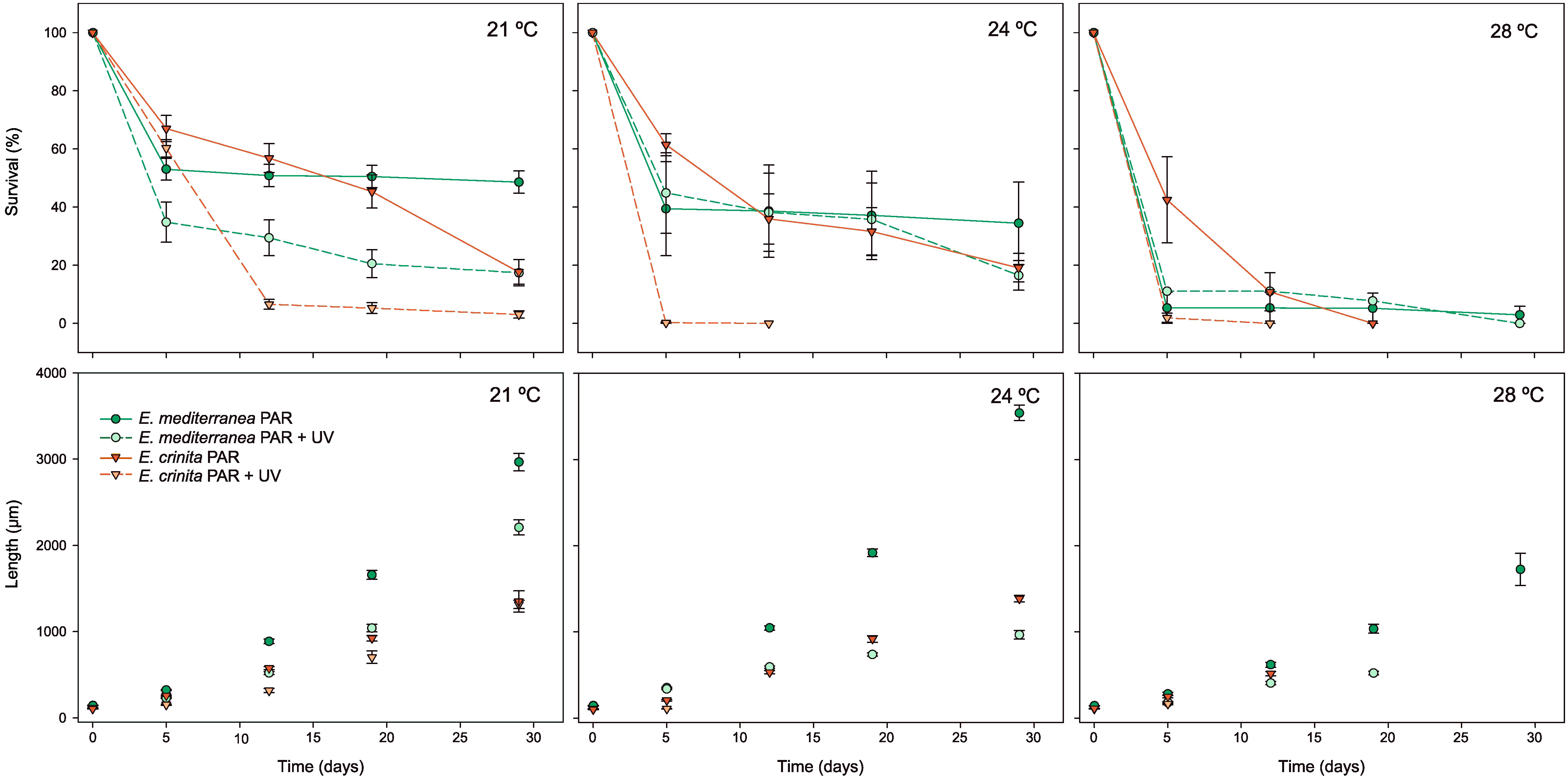
Figure 3 Survival (%) and length (µm) of germlings of Ericaria mediterranea (green) and E. crinita (orange) over 30 days, under different temperature (21°C, 24°C and 28°C) and radiation (PAR and PAR+UV) conditions. The solid line is the photosynthetic active radiation (PAR), and the dotted line is the ultraviolet radiation treatment (PAR+UV). Vertical bars represent standard errors.
E. mediterranea germlings grew faster than the E. crinita germlings. However, temperatures of 28°C negatively impacted the growth of both species (p<0.05, Table S3; Figure 3). At 28°C, growth was highly reduced in all irradiance treatments for both species, with E. mediterranea individuals under PAR conditions the only survivors at the end of the experiment (ca. 1.700 µm in length). At 21°C and 24°C, the growth of both species under PAR conditions increased steeply, reaching values of ca. 3,000 and 3,500 µm for E. mediterranea at 21°C and 24°C, respectively, and ca 1,300 µm for E. crinita at both temperatures on day 30. However, UV conditions significantly decreased E. mediterranea growth, especially at 24°C when growth was reduced by more than a third (p<0.001, Table S3; Figure 3).
4 Discussion
This study demonstrates that the responses to different climate change-derived stressors are species- and life stage-specific and might be determined by the species’ life history traits. Indeed, we show that the combined effect of different stressors may also determine overall population vulnerability, which may be a key point to be considered in future fucalean species conservation planning.
The independent impacts of temperature and UV radiation are rarely assessed in marine organisms, but in our experiment, we show that high temperatures strongly affected both species, especially in terms of development and survival in the early stages. A higher vulnerability of canopy-forming macroalgae recruits compared to adults has already been reported for several stressors such as pollution (e.g., Nielsen et al., 2014; de Caralt et al., 2020), temperature (e.g., Verdura et al., 2021) and UV radiation (e.g., Dring et al., 1996). The higher vulnerability of early life stages is a crucial but unfortunately at sometimes disregarded aspect, which should be considered in predictions of population viability. In fact, any recruitment decrease is ultimately conditioning the future viability of overall populations, even before any visible impact on adults can be reported.
Among the studied species, E. mediterranea was more vulnerable to higher temperatures than E. crinita, especially in adults, which showed a significant decrease of both biomass and photosynthetic quantum yield in just seven days at the highest temperature. Indeed, E. crinita can dwell in sheltered areas such as rock pools where the local seawater conditions can exhibit a great range of variability, reaching extremely high and extremely low temperatures in summer and winter, respectively (Verdura et al., 2021; and see Figure 1). This fact may foster biological processes that provide the species with a wider thermal tolerance range and greater resistance to high temperatures than E. mediterranea, which tends to live in highly exposed zones with more hydrodynamism and more stable temperatures (Boudouresque, 1969; Ballesteros, 1988; Gómez-Garreta et al., 2000; and see Figure 1). This trend has also been reported for other benthic marine organisms. For instance, corals living in environments with naturally high thermal variation (such as small lagoons and intertidal zones with tides) resist heat stress better than those living in areas with more a thermally stable environment due to acclimatisation or adaptation processes (Oliver and Palumbi, 2011; Castillo et al., 2012; Palumbi et al., 2014; Schoepf et al., 2015).
UV radiation effects were more subtle and mainly observable in germlings, with E. crinita more UV-susceptible. Again, different life stages may have singular requirements or responses to different environmental conditions. The contrasting sensitivity of germlings of both species to UV radiation is probably related to their contrasting life history traits. As a perennial species, E crinita has branches throughout the year, so its recruits always develop and grow under the shade of the canopy, preventing exposure to direct radiation. In contrast, E. mediterranea is a semi-perennial species and its juveniles are used to growing in the absence of the canopy protection of the adults. Therefore, in the early life stages, E. mediterranea is naturally exposed to higher irradiations and is probably more resistant (acclimatised or adapted) to these conditions. In fact, our results are in line with those obtained for other canopy-forming macroalgae, where early-stage tolerance to UV is strongly determined by the natural UV exposure context, with species dwelling in supralittoral or higher intertidal environments (more exposed) proving to be more resistant than those living at lower depths (less UV exposed) (Altamirano et al., 2003; Wiencke et al., 2006).
Although the specific mechanisms by which temperature and UV radiation physiologically stress fucalean species are still unknown, the combined effects here reported are particularly worrying given the expected warming scenarios, especially considering the expected increase in MHWs (Garrabou et al., 2022) and the elevated UV irradiance (McKenzie et al., 2010). In general terms, when multiple stressors are analysed one factor may prevail over others, such as temperature effects predominating over UV radiation in adults of the two species studied, whereas in other cases, warming can aggravate the effects of UV radiation, as observed in the germlings of the two studied Ericaria species (Altamirano et al., 2003; Steinhoff et al., 2008). For instance, after a hypothetical MHW episode, the adults of a species like E. mediterranea would be severely damaged (due to their high sensitivity to high temperatures and their relatively resistant germlings to UV radiation), while their potential recruits would have a chance to survive and grow in deforested areas thanks to their UV tolerance. However, if the foundational species behaves like E. crinita (with adults resistant to high temperatures and a high sensitivity of germlings to UV radiation), the adults will probably cope with the impact of the hypothetical MHW, likely persisting with the main axis but losing their branches, further compromising the survival of recruits since they will be affected by both the high temperatures and higher UV radiations (loss of adults´ photoprotection).
Our study highlights that any current restoration action must be able to deal with changing environmental conditions in the context of climate change to be successful in the long term (Wood et al., 2019; Abelson et al., 2020; Cebrian et al., 2021). To address this premise, and based on our results, we recommend considering how life history traits of different species can determine their vulnerability or resistance to future environmental conditions (Figure 4). For example, if recruits are highly vulnerable, cultivating them under controlled environmental conditions may become crucial. This can be especially important when species recruitment co-occurs with periods of high likelihood of MHW. By doing so, the production of a sufficient number of recruits can be enhanced to guarantee the viability of the population. Similarly, sensitivity to high UV irradiances, especially of the species recruiting under the canopy, should lead to the planned transplantation of new recruits under adult canopies or close to them. This point is highly relevant when planning the restoration of a population already affected by a MHW or other one-off impacts that have caused the natural canopy to vanish, which may force the consideration of 1- high irradiance resistant species for transplantation (according to their life history traits); 2- establishing a macroalgae habitat able to provide the recruits with shade; or 3- growing the recruits under suitable environments (laboratory or under a healthy population) and outplanting them when they have grown and are out of the most vulnerable life stage. In summary, life history traits of the species and the environmental context to which they are subjected determine the potential winners and losers in the face of change, with this information being essential for developing fine-tuned and adaptive restoration plans in the context of global climate change.
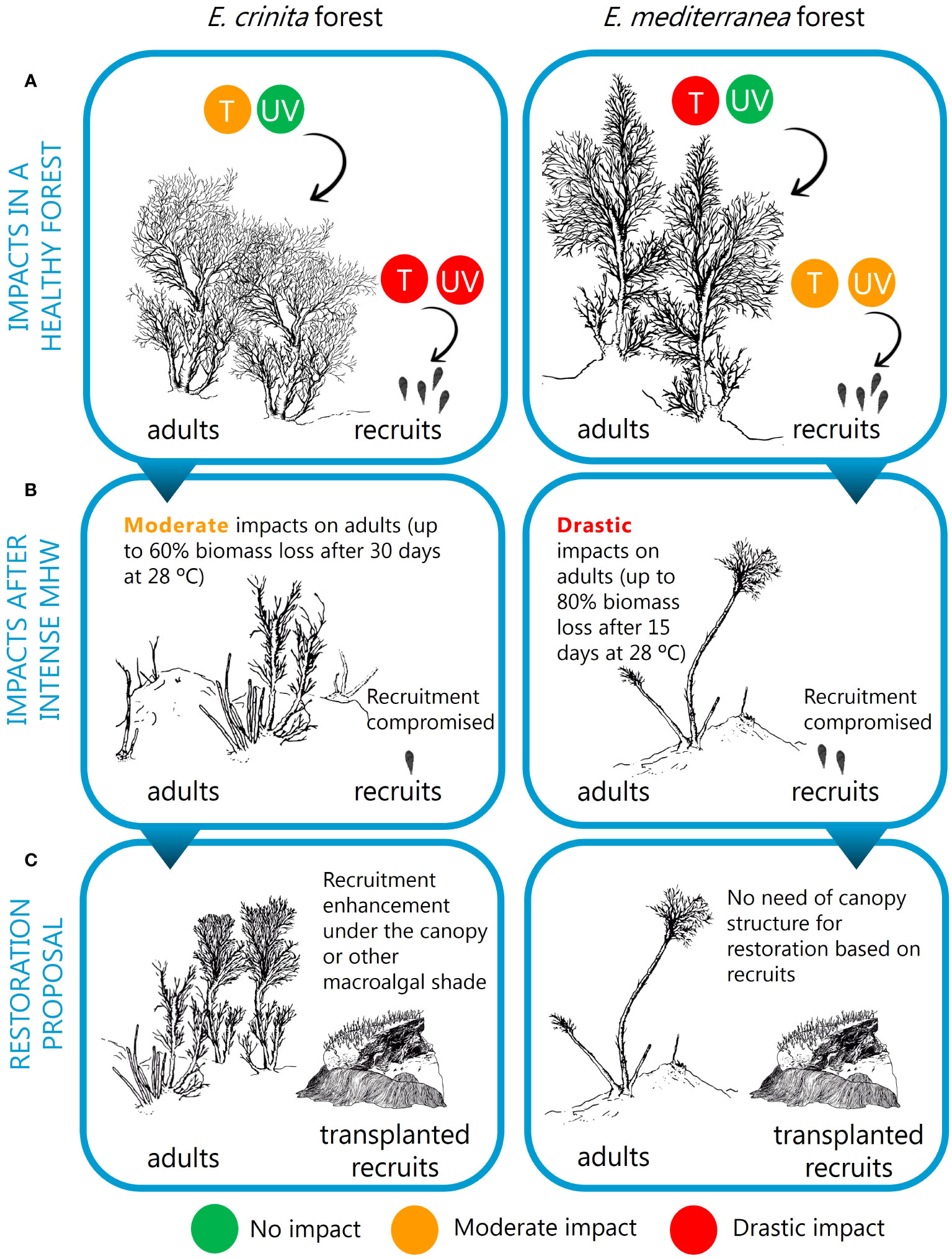
Figure 4 Predicted impacts of future MHWs on adults and recruits (A, B), and recommendations for specific restoration plans in the context of climate change, considering specific responses according to different life history traits (C). (A) Specific life history traits will determine Cystoseira s.l. populations’ vulnerability to future environmental conditions. An intense (high temperatures) and severe (long period) MHW will impact individual adults and germlings of all species, but adults of species such as E. mediterranea, adapted to exposed environments, will be more vulnerable; and E. crinita germlings, which are used to growing under the canopy, are more sensitive to UV radiation than the germlings of E. mediterranea. (B) After an MHW episode, Cystoseira s.l. populations would experience a canopy loss, and there would only be a recovery chance for the adults of the more resistant species in an MHW (like E. crinita; which experienced less than 60% biomass loss). (C) In both cases, to develop a restoration plan, we would recommend reinforcing the damaged populations by outplanting new recruits. However, species-specific sensitivity to UV will determine the need to provide or dispose of canopy shade (from adult survivors or other erect species) to protect from the specific impacts on UV, especially on the species that are more vulnerable to UV radiation in the early life stages (e.g. E. crinita).
Data availability statement
The raw data supporting the conclusions of this article will be made available by the authors, without undue reservation.
Author contributions
EC conceived the ideas. EC, JV, JS and SC set up the experiments. SC, JV, JS and AV collected the data. JV, JS and SC performed the data analyses. and SC and EC drafted the manuscript. All authors contributed to the article and approved the submitted version.
Funding
This research was supported by the Sustainable Blue Economy programme (European Union), under the grant agreement – AFRIMED - EASME/EMFF/2017/1.2.1.12/S4/01/SI2.789059; the Spanish Ministry of Science and Innovation, under the grant FORESTA - N° PID2020-112985GB-I00, funded by MCIN/AEI/10.13039/501100011033 (European Union), and under the grant FoRescue – N° PCI2022-135070-2 and PCI2022-135052-2 funded by Biodiversa+ (European Biodiversity Partnership).
Acknowledgments
SC, AV and EC are members of the MedRecover Research Group (www.medrecover.org, 2021 SGR 01073) of the Generalitat de Catalunya.
Conflict of interest
The authors declare that the research was conducted in the absence of any commercial or financial relationships that could be construed as a potential conflict of interest.
Publisher’s note
All claims expressed in this article are solely those of the authors and do not necessarily represent those of their affiliated organizations, or those of the publisher, the editors and the reviewers. Any product that may be evaluated in this article, or claim that may be made by its manufacturer, is not guaranteed or endorsed by the publisher.
Supplementary material
The Supplementary Material for this article can be found online at: https://www.frontiersin.org/articles/10.3389/fmars.2023.1248629/full#supplementary-material
References
Abdala-Díaz R. T., Cabello-Pasini A., Pérez-Rodríguez E., Álvarez R. M. C., Figueroa F. L. (2006). Daily and seasonal variations of optiman quantum yield and phenolic compounds in Cystoseira tamariscifolia (Phaeophyta). Mar. Biol. 148, 459–465. doi: 10.1007/s00227-005-0102-6
Abelson A., Reed D. C., Edgar G. J., Smith C. S., Kendrick G. A., Orth R. J., et al. (2020). Challenges for restoration of coastal marine ecosystems in the anthropocene. Front. Mar. Sci. 7. doi: 10.3389/fmars.2020.544105
Altamirano M., Flores-Moya A., Figueroa F. L. (2003). Effects of UV radiation and temperatura on growth of germlings of three species of Fucus (Phaeophycae). Aquat. Bot. 75, 9–20. doi: 10.1016/S0304-3770(02)00149-3
Andrews S., Bennett S., Wernberg T. (2014). Reproductive seasonality and early life temperatura sensitivity reflect vulnerability of a seaweed undergoing range reduction. Mar. Ecol. Prog. Ser. 495, 119–129. doi: 10.3354/meps10567
Arafeh-Dalmau N., Montaño-Moctezuma G., Martínez J. A., Beas-Luna R., Schoeman D. S., Torres-Moye G. (2019). Extreme marine heatwaves alter kelp forest community near its equatorward distribution limit. Front. Mar. Sci. 6. doi: 10.3389/fmars.2019.00499
Arévalo R., Pinedo S., Ballesteros E. (2007). Changes in the composition and structure of Mediterranean rocky-shore communities following a gradient of nutrient enrichment: descriptive study and test of proposed methods to assess water quality regarding macroalgae. Mar. pollut. Bull. 55, 104–113. doi: 10.1016/j.marpolbul.2006.08.023
Bais A. F., Bernhard G., McKenzie R. L., Aucamp P. J., Young P.J. ;., Ilyas M. (2019). Chapter 1: ozone-climate interactions and effects on solar ultraviolet radiation. Photochem. Photobiol. Sci. 18, 602–640. doi: 10.1039/c8pp90059k
Ballesteros E. (1988). Estructura y dinámica de la comunidad de Cystoseira mediterranea Sauvageau en el Mediterráneo noroccidental. Investigación Pesquera 52 (3), 313–334.
Ballesteros E. (1990a). Structure and dynamics of the community of Cystoseira zosteroides (Turner) C. Agardh (Fucales, Phaeophyceae) in the Northwestern Mediterranean. Sci. Mar. 54 (3), 217–229.
Ballesteros E. (1990b). Structure and dynamics of the Cystoseira caespitosa Sauvageau (Fucales, Phaephyceae) community in the North-Western Mediterranean. Sci. Mar. 54 (2), 155–168.
Ballesteros E. (1992). Els vegetals i la zonació litoral: espècies, comunitats i factors que influeixen en la seva distribució. Arx. Secc. Ciències IEC 101, 1–616.
Ballesteros E. (2002). “Flora Phycologica Iberica,” in Vol. 1. Fucales, vol. 66 . Ed. Gómez-Garreta A. (Spain: Universidad de Murcia), 187–188.
Ballesteros E., Garrabou J., Hereu B., Zabala M., Cebrian E., Sala E. (2009). Deep-water stands of Cystoseira zosteroides C. Agardh (Fucales, Ochrophyta) in the Northwestern Mediterranean: Insights into assemblage structure and population dynamics. Estuar. Coast. Shelf. Sci. 82, 477–484. doi: 10.1016/j.ecss.2009.02.013
Ballesteros E., Sala E., Garrabou J., Zabala M. (1998). Community structure and frond size distribution of a deep water stand of Cystoseira spinosa (Phaeophyta) in the Northwestern Mediterranean. Eur. J. Phycol. 33 (2), 121–128. doi: 10.1080/09670269810001736613
Bates D., Mächler M., Bolker B., Walker S. (2015). Fitting linear mixed-effects models using lme4. J. Stat. Software 67, 1–48. doi: 10.18637/jss.v067.i01
Bekkby T., Papadopoulou N., Fiorentino D., McOwen C. J., Rinde E., Boström C. (2020). Habitat features and their Influence on the restoration potential of marine habitats in Europe. Front. Mar. Sci. 7. doi: 10.3389/fmars.2020.00184
Bennett S., Wernberg T., Joy B. A., De Bettignies T., Campbell A. H. (2015). Central and rear-edge populations can be equally vulnerable to warming. Nat. Commun. 6, 10280. doi: 10.1038/ncomms10280
Bernhard G. H., Bais A. F., Aucamp P. J., Klekociuk A. R., Liley J. B. (2023). and mcKenzie, R Stratospheric ozone, UV radiation, and climate interactions. L Photochem. Photobiol. Sci. 21, 1–53. doi: 10.1007/s43630-023-00371-y
Bevilacqua S., Savonitto G., Lipizer M., Mancuso P., Ciriaco S., Srijemsi M., et al. (2019). Climatic anomalies may create a long-lasting ecological phase shift by altering the reproduction of a foundation species. Ecology 100, e02838. doi: 10.1002/ecy.2838
Bischof K., Peralta G., Kräbs G., van de Poll W. H., Pérez-Lloréns J. L., Breeman A. M. (2002). Effects of solar UV-B radiation on canopy structure of Ulva communities from southern Spain. J. Exp. Bot. 53 (379), 2411–2421. doi: 10.1093/jxb/erf091
Bolker B. M., Brooks M. E., Clark C. J., Geange S. W., Poulsen J. R., Stevens M. H. H., et al. (2009). Generalized linear mixed models: a practical guide for ecology and evolution. Trends Ecol. Evol. 24, 127–135. doi: 10.1016/j.tree.2008.10.008
Bornman J. F., Paul N., Tang X. (2011). Environmental effects of ozone depletion and its interactions with climate change: 2010 assessment. Photochem. Photobiol. Sci. 10, 174. doi: 10.1039/c0pp90041a
Boudouresque C. F. (1969). Étude qualitative et quantitative d’un peuplement algal à Cystoseira mediterranea dans la région de Banyuls sur Mer. Vie Milieu 20, 437–452.
Boudouresque C. F. (1971). Recherches de bionomie analytique, structurale et expérimentale sur les peuplements benthiques sciaphiles de Méditerranée occidentale (fraction algale): la sous-strate sciaphile des peuplements des grandes Cystoseira de mode battu. Bull. Mus. Natl. 31, 79–104.
Boudouresque C. F. (1972). Recherches de bionomie analytique, structurale et expérimentale sur les peuplements benthiques sciaphiles de Méditerranée Occidentale (fraction algale): le sous-strate sciaphile d’un peuplement photophile de mode calme, le peuplement à Cystoseira crinita. Bull. Mus. Natl. 32, 253–263.
Capdevila P., Hereu B., Salguero-Gómez R., Rovira G., Medrano A., Cebrian E., et al. (2018). Warming impacts on early life stages increase the vulnerability and delay the population recovery of a long-lived hábitat-forming macroalga. J. Ecol. 107, 1129–1140. doi: 10.1111/1365-2745.13090
Casado-Amezúa P., Araújo R., Bárbara I., Bermejo R., Borja Á., Díez I., et al. (2019). Distributional shifts of canopy-forming seaweeds from the Atlantic coast of Southern Europe. Biodivers. Conserv. 28 (5), 1151–1172. doi: 10.1007/s10531-019-01716-9
Castillo K. D., Ries J. B., Weiss J. M., Lima P. (2012). Decline of forereef corals in response to recent warming linked to history of thermal exposure. Nat. Clim. Change. 2, 757–760. doi: 10.1038/NCLIMATE1577
Cebrian E., Tamburello L., Verdura J., Guarnieri G., Medrano A., Linares C., et al. (2021). A roadmap for the restoration of mediterranean macroalgal forests. Front. Mar. Sci. 8. doi: 10.3389/fmars.2021.709219
Chapman A. R. O. (1981). Stability of sea urchin dominated barren grounds following destructive grazing of kelp in St. Margaret’s Bay, Eastern Canada. Mar. Biol. 62, 307–311.
Coleman M. A., Kelaher B. P., Steinberg P. D., Millar J. K. (2008). Absence of a large brown macroalga on urbanized rocky reefs around Sydney, Australia, and evidence for historical decline. J. Phycol. 44, 897–901. doi: 10.1111/j.1529-8817.2008.00541.x
Coleman M. A., Wernberg T. (2017). Forgotten underwater forests: The key role of fucoids on Australian temperate reefs. Ecol. Evol. 7, 8406–8418. doi: 10.1002/ece3.3279
Core Team. R. (2019). R: A Language and Environment for Statistical Computing (Vienna, Austria: R Foundation for Statistical Computing).
de Caralt S., Verdura J., Vergés A., Ballesteros E., Cebrian E. (2020). Differential effects of pollution on adult and recruits of a canopy-forming alga: implications for population viability under low pollutant levels. Sci. Rep. 10, 17825. doi: 10.1038/s41598-020-73990-5
Diffenbaugh N. S., Pal J. S., Giorgi F., Gao X. (2007). Heat stress intensification in the Mediterranean climate change hotspot. Geophys. Res. Lett. 34, L11706. doi: 10.1029/2007GL030000
Dring M. J., Makarov V., SchosChina E., Lorenz M., Lüning K. (1996). Influence of ultraviolet-radiation on chlorophyll fluorescence and growth in different life-history stages of three species of Laminaria (phaeophyta). Mar. Biol. 126, 183–191. doi: 10.1007/BF00347443
Eger A. M., Marzinelli E. M., Christie H., Fagerli C. W., Fujita D., Gonzalez A. P., et al. (2022). Global kelp forest restoration: past lessons, present status, and future directions. Biol. Rev. 97, 1449–1475. doi: 10.1111/brv.12850
El-Sayed S. Z., Van Dijken G. L., Gonzalez-Rodas G. (1996). Effects of ultraviolet radiation on marine ecosystems. Int. J. Environ. Stud. 51 (3), 199–216. doi: 10.1080/00207239608711081
Endo H., Sugie T., Yonemori Y., Nishikido Y., Moriyama H., Ito R., et al. (2021). Vegetative reproduction is more advantageous than sexual reproduction in a canopy-forming clonal macroalga under ocean warming accompanied by oligotrophication and intensive herbivory. Plants 10, 1522. doi: 10.3390/plants10081522
Falace A., Marletta G., Savonitto G., Candotto C. F., Srijemsi M., Bevilacqua S., et al. (2021). Is the south-mediterranean canopy-forming Ericaria giacconei (= Cystoseira hyblaea) a loser from ocean warming? Front. Mar. Sci. 8. doi: 10.3389/fmars.2021.760637
Feldmann J. (1937). Recherches sur la Végétation marine de La Méditerranée. La côte des Albères (France: Rev. Algol. Université de Paris).
Filbee-Dexter K., Scheibling R. E. (2014). Sea urchin barrens as alternative stable states of collapsed kelp ecosystems. Mar. Ecol. Prog. Ser. 495, 1–25. doi: 10.3354/meps10573
Garrabou J., Gómez-Gras D., Medrano A., Cerrano C., Ponti M., Schlegel R., et al. (2022). Marine heatwaves drive recurrent mass mortalities in the Mediterranean Sea. Glob. Change Biol. 28, 5708–5725. doi: 10.1111/gcb.16301
Gianni F., Bartolini F., Airoldi L., Ballesteros E., Francour P., Guidetti P., et al. (2013). Conservation and restoration of marine forests in the Mediterranean Sea and the potential role of Marine Protected Areas. Adv. Oceanogr. Limnol. 4, 83–101. doi: 10.1080/19475721.2013.845604
Gómez-Garreta A., Barceló Martí M., Gallardo García T., Pérez-Ruzafa I., Ribera Sigual M., Rull J. (2000). Flora Phycologica Ibérica. I. Fucales (Vol. 1) (Spain: Servicio de Publicaciones, Universidad de Murcia).
Guiry M. D., Cunningham E. M. (1984). Photoperiodic and temperature responses in the reproduction of north-eastern Atlantic Gigartina acicularis (Rhodophyta: Gigartinales). Phycol 23, 357–367. doi: 10.2216/i0031-8884-23-3-357.1
Häder D. P., Williamson C. E., Wängberg S. A., Rautio M., Rose K. C., Gao K., et al. (2015). Effects of UV radiation on aquatic ecosystems and interactions with other environmental factors. Photochem. Photobiol. Sci. 14, 108–126. doi: 10.1039/c4pp90035a
Harley C. D. G., Anderson K. M., Demes K. W., Jorve J. P., Kordas R. L., Coyle T. A. (2012). Effects of climate change on global seaweed communities. J. Phycol. 48, 1064–1078. doi: 10.1111/j.1529-8817.2012.01224.x
Harrison X. A., Donaldson L., Correa-Cano M. E., Evans J., Fisher D. N., Goodwin C. E., et al. (2018). A brief introduction to mixed effects modelling and multi-model inference in ecology. PeerJ 6 (1), e4794. doi: 10.7717/peerj.4794
Hereu B., Mangialajo L., Ballesteros E., Thibaut T. (2008). On the occurrence, structure and distribution of deep-water Cystoseira (Phaeophyceae) populations in the Port-Cros National Park (north-western Mediterranean). Eur. J. Phycol. 43 (3), 263–273. doi: 10.1080/09670260801930330
Hothorn T., Bretz F., Westfall P. (2008). Simultaneous inference in general parametric models. Biometrical J. 50 (3), 346–363. doi: 10.1002/bimj.200810425
Ling S. D., Scheibling R. E., Rassweiler A., Johnson C. R., Shears S. D., Connell S. D., et al. (2015). Global regime shift dynamics of catastrophic sea urchin overgrazing. Phil. Trans. R. Soc B 370, 20130269. doi: 10.1098/rstb.2013.0269
McKenzie R. L., Aucamp P. J., Bais A. F., Björn L. O., Ilyas M., Madronich S. (2010). Ozone depletion and climate change: impacts on UV radiation. Photochem. Photobiol. Sci. 10, 182–198. doi: 10.1039/c0pp90034f
Monserrat M., Comeau S., Verdura J., Alliouane S., Spennato G., Priouzeau F., et al. (2022). Climate change and species facilitation affect the recruitment of macroalgal marine forests. Sci. Rep. 12, 18103. doi: 10.1038/s41598-022-22845-2
Murchie E. H., Lawson T. (2013). Chlorophyll fluorescence analysis: a guide to good practice and understanding some new applications. J. Exp. Bot. 64, 3983–3998. doi: 10.1093/jxb/ert208
Nielsen H. D., Nielsen S. L. (2010). Adaptation to high light irradiances enhances the photosynthetic Cu2+ resistance in Cu2+ tolerant and non-tolerant populations of the brown macroalgae. Fucus serratus. Mar. pollut. Bull. 60, 710–717. doi: 10.1016/j.marpolbul.2009.11.025
Nielsen S. L., Nielsen H. D., Pedersen M. F. (2014). Juvenile life stages of the brown alga Fucus serratus L. @ are more sensitive to combined stress from high copper concentration and temperature than adults. Mar. Biol. 161, 1895–1904. doi: 10.1007/s00227-014-2471-1
Oliver T. A., Palumbi S. R. (2011). Do fluctuating temperature environments elevate coral thermal tolerance? Coral Reefs. 30, 429–440. doi: 10.1007/s00338-011-0721-y
Orfanidis S., Rindi F., Cebrian E., Fraschetti S., Nasto I., Taskin E., et al. (2021). Effects of natural and anthropogenic stressors on fucalean brown seaweeds across different spatial scales in the Mediterranean Sea. Front. Mar. Sci. 8. doi: 10.3389/fmars.2021.658417
Palumbi S. R., Barshis D. J., Traylor-Knowles N., Bay R. A. (2014). Mechanisms of reef coral resistance to future climate change. Science 344, 895-898. doi: 10.1126/science.1251336
Pinedo S., Zabala M., Ballesteros E. (2013). Long-term changes in sublittoral macroalgal assemblages related to water quality improvement. Bot. Mar. 56, 461–469. doi: 10.1515/bot-2013-0018
Raven J. A. (2017). The possible roles of algae in restricting the increase in atmospheric CO2 and global temperature. Eur. J. Phycol. 52 (4), 506–522. doi: 10.1080/09670262.2017.1362593
Rindi F., Gavio B., Díaz-Tapia P., Di Camillo C. G., Romagnoli T. (2020). Long-term changes in the benthic macroalgal flora of a coastal area affected by urban impacts (Conero Riviera, Mediterranean Sea). Biodivers. Conserv. 29 (7), 2275–2295. doi: 10.1007/s10531-020-01973-z
Ripley B., Venables B., Bates D. M., Hornik K., Gebhardt A., Firth D., et al. (2013). Modern applied statistivs with S. Package ‘mass’. Cran r 538, 113–120.
Rodríguez-Prieto C., Polo L. (1996). Effects of sewage pollution in the structure and dynamics of the community of Cystoseira mediterranea (Fucales, Phaeophyceae). Sci. Mar. 60, 253–263.
Román M., Román S., Vázquez E., Troncoso J., Olabarria C. (2020). Heatwaves during low tide are critical for the physiological performance of intertidal macroalgae under global warming scenarios. Sci. Rep. 10 (1), 21408. doi: 10.1038/s41598-020-78526-5
Sales M., Ballesteros E. (2009). Shallow Cystoseira (Fucales: Ochrophyta) assemblages thriving in sheltered areas from Menorca (NW Mediterranean): relationships with environmental factors and anthropogenic pressures. Estuar. Coast. Shelf Sci. 84, 476–482. doi: 10.1016/j.ecss.2009.07.013
Sales M., Ballesteros E. (2010). Long-term comparison of algal assemblages dominated by Cystoseira crinita (Fucales, Heterokontophyta) from Cap Corse (Corsica, North Western Mediterranean). Eur. J. Phycol. 45, 404–412. doi: 10.1080/09670262.2010.498585
Sales M., Ballesteros E. (2012). Seasonal dynamics and annual production of Cystoseira crinita (Fucales: Ochrophyta)-dominated assemblages from the northwestern Mediterranean. Sci. Mar. 76, 391–401. doi: 10.3989/scimar.03465.16D
Sales M., Cebrian E., Tomas F., Ballesteros E. (2011). Pollution impacts and recovery potential in three species of the genus Cystoseira (Fucales, Heterokontophyta). Estuar. Coast. Shelf Sci. 92, 347–357. doi: 10.1016/j.ecss.2011.01.008
Sanchez-Lorenzo A., Enriquez-Alonso A., Calbó J., González J. A., Wild M., Folini D., et al. (2017). Fewer clouds in the Mediterranean: Consistency of observations and climate simulations. Sci. Rep. 7, 41475. doi: 10.1038/srep41475
Sant N. (2003). Algues Bentòniques Mediterrànies: Comparació de Mètodes de Mostreig, Estructura de Comunitats i Variació En La Resposta Fotosintètica (PhD thesis) (Spain: Universitat de Barcelona).
Schiel D. R., Foster M. S. (2006). The population biology of large brown seaweeds: Ecological consequences of multiphase life histories in dynamic coastal environments. Annu. Rev. Ecol. Evol. Syst. 37, 343–372. doi: 10.1146/annurev.ecolsys.37.091305.110251
Schiel D. R., Foster M. S. (2015). The biology and ecology of giant Kelp Forests. J. Phycol. (Oakland California, USA: University of California Press) 53, 462–465. doi: 10.1111/jpy.12516
Schoepf V., Stat N., Falter J. L., McCulloch M. T. (2015). Limits to the thermal tolerance of corals adapted to a highly fluctuating, naturally extreme temperature environment. Sci. Rep. 5, 17639. doi: 10.1038/srep17639
Smale D. A., Wernberg T., Oliver E. C. J., Thomsen M., Harvey B. P., Straub S. C., et al (2019). Marine heatwaves threaten global biodiversity and the provision of ecosystem services. Nat. Clim. Chang. 9, 306–312. doi: 10.1038/s41558-019-0412-1
Smale D. A. (2020). Impacts of ocean warming on kelp forest ecosystems. New Phytol. 225 (4), 1447–1454. doi: 10.1111/nph.16107
Smale D. A., Wernberg T. (2013). Extreme climatic event drives range contraction of a habitat-forming species. Proc. R. Soc B P ROY Soc. B-BIOL Sci. 280, 20122829. doi: 10.1098/rspb.2012.2829
Steinhoff F. S., Wiencke C., Müller R., Bischof K. (2008). Effects of ultraviolet radiation and temperature on the ultrastructure of zoospores of the Brown macroalga Laminaria hyperborea. Plant Biol. 10, 388–397. doi: 10.1111/j.1438-8677.2008.00049.x
Steneck R. S., Graham M. H., Bourque B. J., Corbett D., Erlandson J. M., Estes J. A., et al. (2002). Kelp forest ecosystems: biodiversity, stability, resilience and future. Environ. Conser. 29 (4), 436–459. doi: 10.1017/S0376892902000322
Straub S. C., Wernberg T., Thomsen M. S., Moore P. J., Burrows M. T., Harvey B. P., et al. (2019). Resistance, extinction, and everything in between –The diverse responses of seaweeds to marine heatwaves. Front. Mar. Sci. 6. doi: 10.3389/fmars.2019.00763
Supratya V. P., Coleman L. J. M., Martone P. T. (2020). Elevated temperature affects phenotypic plasticity in the bull kelp (Nereocystis luetkeana, phaeophyceae). J. Phycol. 56, 1534–1541. doi: 10.1111/jpy.13049-19-267
Thibaut T., Blanfuné A., Boudouresque C. F., Verlaque M. (2015). Decline and local extinction of Fucales in the French Riviera: The harbinger of future extinctions? Mediterr. Mar. Sci. 16, 206–224. doi: 10.12681/mms.1032
Thibaut T., Pinedo S., Torras X., Ballesteros E. (2005). Long-term decline of the populations of Fucales (Cystoseira spp. and Sargassum spp.) in the Albères coast (France, North-western Mediterranean). Mar. pollut. Bull. 50, 1472–1489. doi: 10.1016/j.marpolbul.2005.06.014
Thiriet P. D., Di Franco A., Cheminée A., Guidetti P., Bianchimani O., Basthard-Bogain S., et al. (2016). Abundance and diversity of crypto- and necto-benthiccoastal fish are higher in marine forests than in structurally less complex macroalgal assemblages. PloS One 11 (10), 1–24. doi: 10.1371/journal.pone.0164121
Thomsen M. S., Mondardini L., Alestra T., Gerrity S., Tait L., South P. M., et al. (2019). Local extinction of bull kelp (Durvillaea spp.) due to a marine heatwave. Front. Mar. Sci. 6. doi: 10.3389/fmars.2019.00084
Vanhaelewyn L., van der Straten D., De Coninck B., Vandenbussche F. (2020). Ultraviolet radiation from a plant perspective: The plant-microorganism Context. Front. Plant Sci. 11. doi: 10.3389/fpls.2020.597642
Vargas-yanez M., García M. J., Salat J., García-Martínez M. C., Pascual J., Moya F. (2008). Warming trends and decadal variability in the Western Mediterranean shelf. Glob. Planet Change. 63, 177–184. doi: 10.1016/j.gloplacha
Verdura J., Sales M., Ballesteros E., Cefalì M. E., Cebrian E. (2018). Restoration of a canopy-forming alga based on recruitment enhancement: methods and long-term success assessment. Front. Plant Sci. 9, 1832. doi: 10.3389/fpls.2018.01832
Verdura J., Santamaría J., Ballesteros E., Smale D. A., Cefalí M. E., Golo R., et al. (2021). Local-scale climatic refugia offer sanctuary for hábitat-forming species during a marine heatwave. J. Ecol. 4 (109), 1758–1773. doi: 10.1111/1365-2745.13599
Vergés A., Doropoulos C., Malcolm H. A., Skye M., Gracia-Pizá M., Marzinelli E. M., et al. (2016). Long-term empirical evidence of ocean warming leading to tropicalization of fish communities, increased herbivory, and loss of kelp. Proc. Natl. Acad. Sci. U. S. A. 113 (48), 13791–13796. doi: 10.1073/pnas.1610725113
Vergés A., Steinberg P. D., Hay M. E., Poore A. G. B., Campbell A. H., Ballesteros E., et al. (2014). The tropicalization of temperate marine ecosystems: climate-mediated changes in herbivory and community phase shifts. Proc. R. Soc B Biol. Sci. 281, 20140846. doi: 10.1098/rspb.2014.0846
Wernberg T., Smale D. A., Thomsen M. S. (2012). A decade of climate change experiments on marine organisms: procedures, patterns and problems. Glob. Change Biol. 18, 1491–1498. doi: 10.1111/j.1365-2486.2012.02656.x
Wiencke C., Roleda M. Y., Gruber A., Clayton M. N., Bischof K. (2006). Susceptibility of zoospores to UV radiation determines upper depth distribution limit of Arctic kelps: evidence through field experiments. J. Ecol. 94, 455–463. doi: 10.1111/j.1365-2745.2006.01102.x
Wood G., Marzinelli E. M., Coleman M. A., Campbell A. H., Santini N. S., Kajlich L., et al. (2019). Restoring subtidal marine macrophytes in the Anthropocene: Trajectories and futureproofing. Mar. Fresh. Res. 70 (7), 936–951. doi: 10.1071/MF18226
Keywords: marine restoration, global impacts, warming, UV radiation, macroalgal forests
Citation: de Caralt S, Verdura J, Santamaría J, Vergés A and Cebrian E (2023) Importance of life history traits for vulnerability to climate change: implications for macroalgal restoration. Front. Mar. Sci. 10:1248629. doi: 10.3389/fmars.2023.1248629
Received: 27 June 2023; Accepted: 02 November 2023;
Published: 23 November 2023.
Edited by:
Ronald Osinga, Wageningen University and Research, NetherlandsCopyright © 2023 de Caralt, Verdura, Santamaría, Vergés and Cebrian. This is an open-access article distributed under the terms of the Creative Commons Attribution License (CC BY). The use, distribution or reproduction in other forums is permitted, provided the original author(s) and the copyright owner(s) are credited and that the original publication in this journal is cited, in accordance with accepted academic practice. No use, distribution or reproduction is permitted which does not comply with these terms.
*Correspondence: Sònia de Caralt, sonia.decaralt@udg.edu